- 1Ecologie Systématique Evolution, Université Paris-Sud, CNRS, AgroParisTech, Université Paris-Saclay, Orsay, France
- 2Ministry of Education Key Laboratory for Biodiversity Science and Ecological Engineering, College of Life Sciences, Beijing Normal University, Beijing, China
- 3Faculty of Exact and Natural Sciences, Institute of Biological Sciences, Siedlce, Poland
- 4House of Bird Research, Christiansfeld, Denmark
- 5Cypresvej 1, Brønderslev, Denmark
- 6Department of Bioscience, Aarhus University, Rønde, Denmark
- 7Ministry of Education Key Laboratory for Ecology of Tropical Islands, College of Life Sciences, Hainan Normal University, Haikou, China
- 8Faculty of Exact and Natural Sciences, Institute of Biological Sciences, Siedlce University of Natural Sciences and Humanities, Siedlce, Poland
The abundance and the diversity of insects in Europe have declined considerably during recent decades, while it remains unclear whether similar changes may also have occurred elsewhere. Here we used citizen science for quantifying the abundance of flying insects on windshields of cars across Europe and to a smaller extent in China. We used the abundance of insects killed against windshields of cars during 3,530 transects for a total distance of 83,019 km made by 50 observers as estimates of insect abundance. A total of 124,606 insects were recorded, or approximately 1.5 insect per km. The abundance of insects killed against windshields was highly repeatable among days for the same locality, showing consistent estimates of abundance. The main determinants of insect abundance were features of cars (driving speed and car model that can be considered noise of no biological significance), local weather (temperature, cloud cover and wind speed) and variation across the season and the day. We tested for differences in the abundance of flying insects killed on windshields of cars predicting and finding (1) a reduction in insect abundance in areas with ionizing radiation at Chernobyl compared to uncontaminated control sites in the neighborhood, (2) a reduction in the abundance of flying insects in Western compared to Eastern Europe, (3) a reduction in the abundance of flying insects killed on windshields from southern to northern Europe compared to latitudinal samples of insects from southern to northern China, and (4) a difference in abundance of insects killed on windshields of cars in Spain with a significant interaction between Spain and Denmark. Thus a number of abiotic and biotic factors accounted for temporal and spatial heterogeneity in abundance of insects, providing a useful tool for monitoring and studying determinants of spatial and temporal patterns of insect abundance. This also implies that our estimate of insect abundance may be relevant for the study of competition and for interactions at higher trophic levels.
Introduction
Surveys of insects have shown recent reductions in abundance by as much as 80%, even in nature reserves (Hallmann et al., 2017; Møller, 2020). These changes have been attributed to climate change, altered farming practice, changed land-use and underlying associated factors (Vogel, 2017). While some studies have documented large reductions in abundance of insects (Hallmann et al., 2017; Møller, 2020; Nyffeler and Bonte, 2020), others have shown smaller declines or no declines at all (Conrad et al., 2002, 2006; Shortall et al., 2009; Fox et al., 2014). This raises questions about the generality of these patterns, but also the underlying mechanisms accounting for such effects. In other words, which factors account for such effects, and which taxa differ in reductions of abundance?
Biodiversity and abundance generally show latitudinal gradients although the causes of such differences among taxa are poorly known (e.g., Rohde, 1992; Møller, 2020). A number of hypotheses has been proposed to account for such gradients, including differences in life history, in particular generation time, and differences in the relative importance of interspecific interactions. Thus, we investigated the predictors that potentially account for reductions in abundance of insects.
A decrease in the abundance of insects in recent decades makes it important to identify the factors that account for such spatial and temporal patterns (Hallmann et al., 2017; Møller, 2020). Several not mutually exclusive factors may cause reductions in insect abundance (Taylor, 1974; Pomfret et al., 2000; Poulin et al., 2010; Nocera et al., 2012). Changes in agricultural land-use and increased use of pesticides (Pomfret et al., 2000; Poulin et al., 2010; Hallmann et al., 2014) appear to have reduced the abundance of insects, and, therefore, also the abundance of insectivorous birds because the latter rely on flying insects as food (Hallmann et al., 2017; Vogel, 2017; Møller, 2019). Here we tested for effects of climate, and its spatial and temporal heterogeneity, on insect abundance using surveys of insects on cars as a method for the quantification of flying insects.
Many monitoring programs following the abundance of living beings at local, national and international scales have been conducted since the 1960s. They are currently financed by national and international census programs to provide extensive and reliable monitoring data (Blondel et al., 1970; Møller, 1983; Bibby et al., 2005; Voříšek et al., 2010). Such surveys mainly monitor birds nationally and across continents such as North America and the European Union. Similar programs exist for spiders Nyffeler and Bonte, 2020, butterflies, mammals and many other organisms (see the monitoring programs run by Museum National Histoire Naturel, Paris, France as an example). Survey sites are assumed to be randomly located and their locations geographically unbiased, although that is rarely tested explicitly. We are unaware of such explicit tests, and, therefore, we performed such tests. Surveys of birds and other living beings rely on the assumption that findings are consistent among days and hence provide repeatable findings. Such repeatability is required for tests of consistency across taxa and trophic levels. Here we provide such repeatability tests for insect counts obtained from the abundance of flying insects killed on the windshield of cars in an attempt to assess the assumption that survey sites provide repeatable findings.
We used extensive transects with cars to quantify the number of insects killed against windshields (Møller, 2013, 2019, 2020). This method has previously been verified as providing reliable information from cross-validations using three different methods [sweep-nets (Møller, 1987, 2001, 2019, 2020), sticky traps (Teglhøj, 2017) and feeding rates of nestling barn swallows Hirundo rustica (Møller, 2013)].
Citizen science has only rarely been used to quantify the abundance of flying insects, and the abundance of prey for flying insects. This method was first utilized by Møller (2013), who showed a decline in wind speed during 1997–2011 at Kraghede (Denmark) during July, but not during the months April-August. These studies showed that the abundance of insects on windscreens was highly repeatable, as was the duration of the pre-laying period of the second brood. Years with adverse weather had lower abundance of insects before laying and lower breeding success decreasing with wind speed. The number of insects sampled was repeatable among sampling events. There was also a high repeatability when relating insect abundance measured with sweep-nets and insects on windscreens. Finally feeding rates of barn swallows were highly repeatable when comparing abundance of insects with abundance on car windscreens (Møller, 2013).
The abundance of insects depended on ambient temperature, time of day and the interaction between temperature and time of day (Møller, 2019; Figure 1). A more extensive study by Møller (2019) showed a reduction by more than 80% in the abundance of insects on windscreens between 1997 and 2017. Because aerial insectivorous birds almost exclusively rely on flying insects for food, we should expect that the number of breeding pairs increased with increasing abundance of insects. That was indeed the case for barn swallows, sand martins and house martins (Møller, 2019; Figure 2).
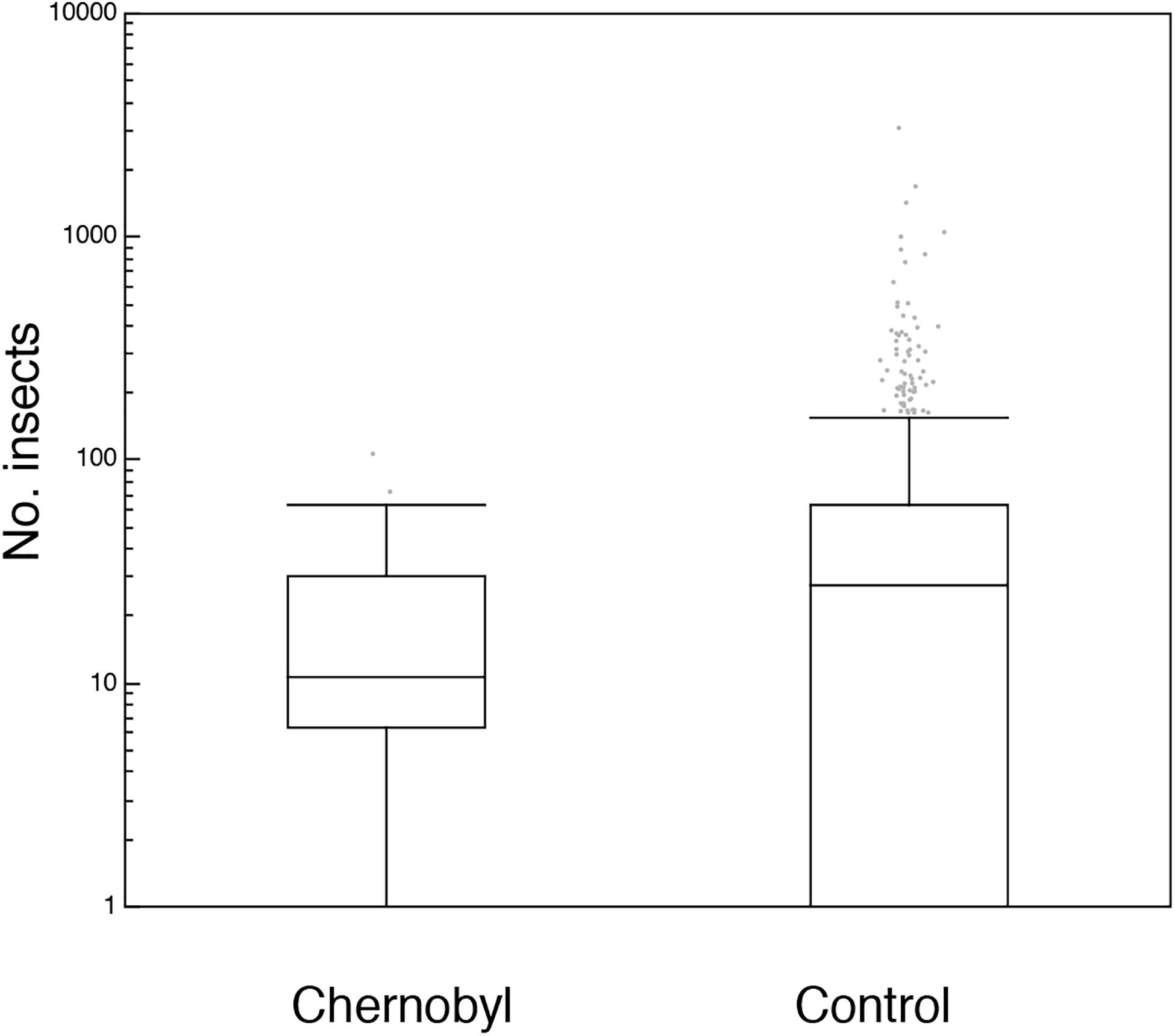
Figure 1. Box plots of the number of insect sampled with a car in Chernobyl and in uncontaminated control areas in Europe. The box plots show median, quartiles, 5- and 95-percentiles and extreme values. Observations are jittered to make all observations visible.
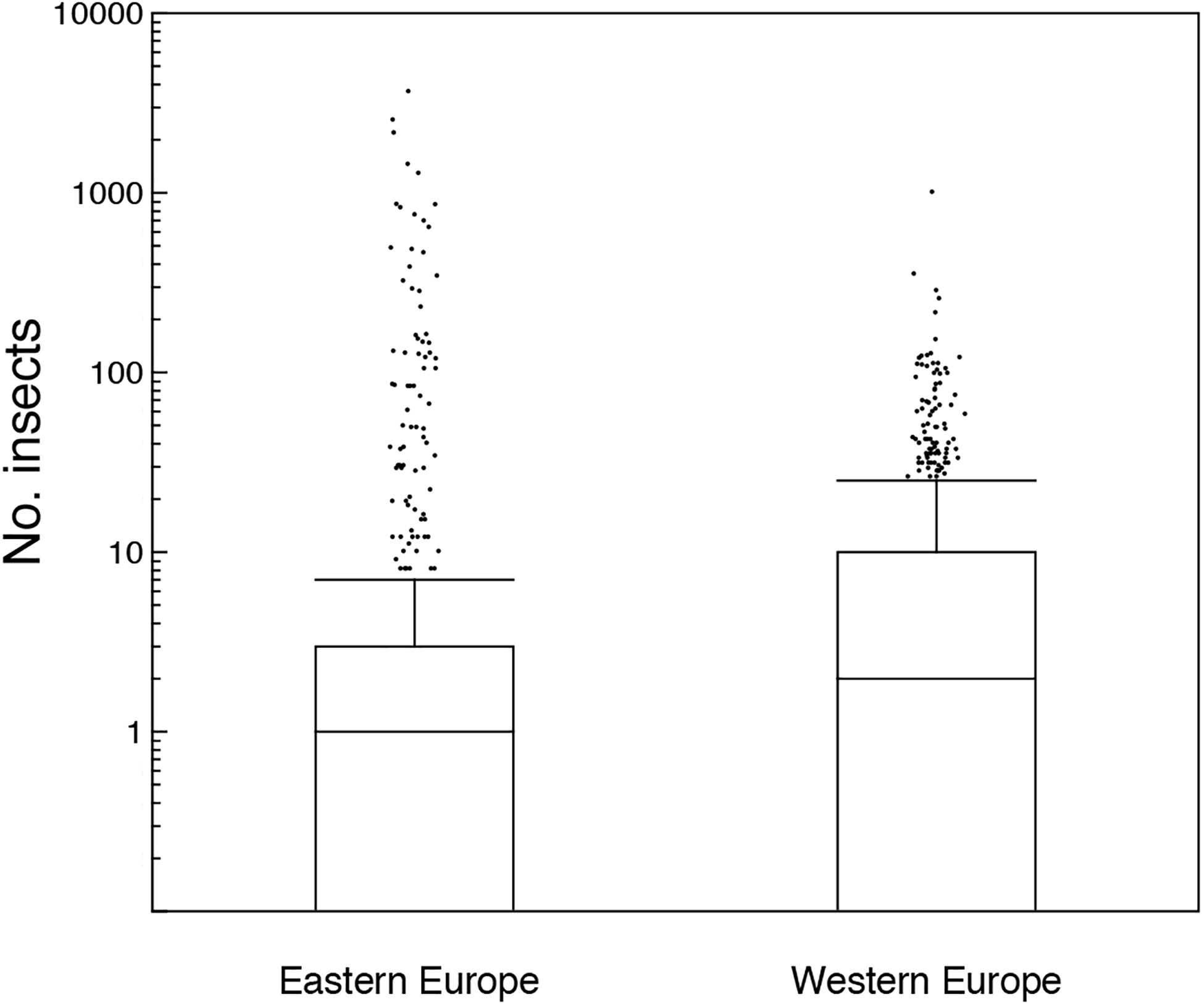
Figure 2. Box plots of the number of insect sampled with a car in Eastern and Western Europe. The box plots show median, quartiles, 5- and 95-percentiles and extreme values. Observations are jittered to make observations visible.
This simple so-called “splatometer” method described above relies on insects killed on the windscreen of cars. The Royal Society for Protection of Birds adopted this method first in 2004. A total of 324,814 insects were recorded on the windscreens of cars participating in the project, or on average 1 killed insect per 5 miles, or one insect per 8.045 km. A repeat of the project in 2019 revealed 1 insect per 10 miles or 16,090 km, or 0.125 insects per km. However, we note that this study statistically speaking only had two observations (a mean value in 2004 and another in 2019) making it difficult to draw any conclusions. Surveys of flying insects by Kent Wildlife Trust, Kent, United Kingdom under the direction of Dr. Paul Tinsley-Marshall, were performed in 2019. This innovative citizen science project using “splatometer” tests found 50% fewer insects in 2019 than in 2004. In Kent there were 50% fewer insects in 2019 with a value of 0.2 insects per mile in 2004 to 0.1 insects per mile in 2019, or 0.063 insects per km. The decline by 50% could be due to different causes including changes in pesticide use, altered morphology of windscreens of cars or changes in habitats between pairs of observation points in time. Finally, age of cars contributed to explain the abundance of killed insects with more recent car models being associated with more killed insects.
The hypothesis to be tested was that environmental conditions affected the size of insect populations. We did so by testing eight biologically meaningful predictions. First, we tested whether the abundance of insects is related to features of cars such as driving speed, car model and windscreen size and shape, which may differ in angle and area. Second, we tested whether insect abundance is related to local weather as reflected by temperature, cloud cover and wind speed that are all known to have an impact on insect abundance. Third, we tested whether insect abundance increased during spring and during the day as expected from timing of emergence of insects. Fourth, we tested whether insect abundance is reduced in Chernobyl compared to uncontaminated control sites nearby, since numerous organisms show reduced population density under radiation exposure (Møller and Mousseau, 2009, 2018; Bezrukov et al., 2015). Fifth, whether there was a higher density of insects in Eastern than in Western Europe due to less intensive agriculture in eastern Europe (Tucker and Heath, 1994; Donald et al., 2001; Schimmelfenning and Sedelmeier, 2005; Reif et al., 2011). Sixth, whether there are fewer insects in Europe than at comparable latitudinal sampling transects in China. Seventh, we tested if there was a reduction in insect abundance between 1997 and 2018 in study sites Denmark and in Spain. These two areas with multiple study sites were chosen because they provide estimates of insect abundance used for food by insectivorous birds from 1997 to 2018 (Møller, 2019). Eighth, whether there was an increase in insect abundance with increasing longitude because climatic conditions during the breeding season deteriorate toward the east.
Materials and Methods
Paired Designs
Heterogeneous field data are often difficult to analyze due to the many factors affecting the conclusions. We attempted to match paired samples whenever possible because differences between such pairs will tend to be similar in most respects.
Study Sites
This study is part of a citizen science project (Crain et al., 2014) on the determinants of the abundance of insects using the windshield of cars as a sampling device (Møller, 2013, 2019, 2020). The criteria used for inclusion of participants in this study are similar to the criteria for inclusion of participants among ornithologists in national or European, Asian or North American bird census programs. The only criterion was that participants are knowledgeable naturalists.
Study sites were chosen by participants following requests for participation from amateurs who had previously expressed interest in this project (Figure 3). Participants were not asked to drive more than they usually did for their profession and their spare time interests. Thus, study sites were located where participants lived. We asked more than 1,000 amateurs in the ornithology literature for participation, and all interested persons were asked to make contact through a web site with information in Danish and English (insect.count.dk).
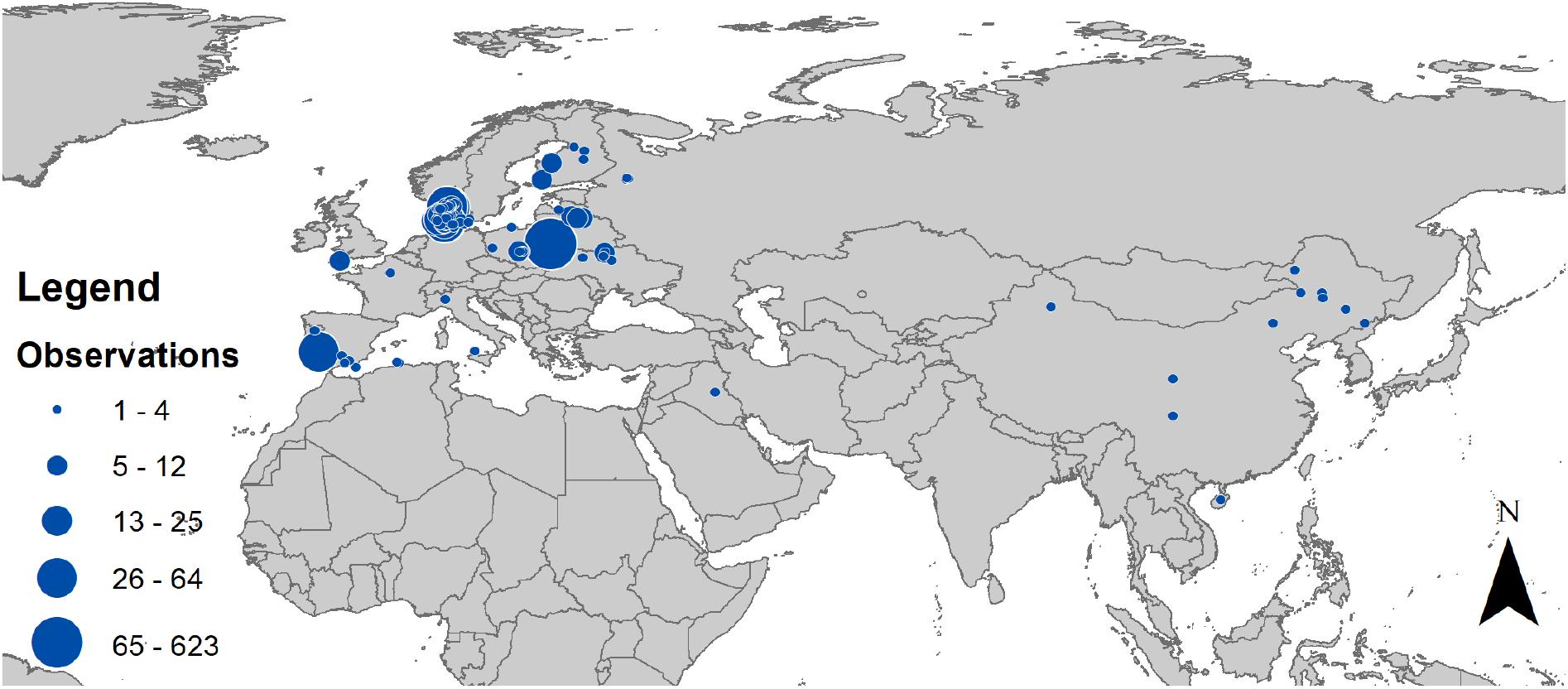
Figure 3. Map of the distribution of sampling sites for insects with cars in Europe and Asia. The size of circles is proportional to the number of samples. The map was created using ArcGIS 10.1 (ESRI, 2012).
Eastern and Western European countries were categorized with Eastern countries being those belonging to the former communist countries and Western European countries constituting the remainder. This categorization is similar to what has been used in previous studies of the reasons for differences in population trends of birds between Eastern and Western Europe (Reif et al., 2011).
Our study sites in China covered a large number of transects along a latitudinal gradient from Hainan in the south to Xinjiang and Inner Mongolia in the north. The latitudinal gradient was located along localities that were part of a latitudinal study of the brood parasitic common cuckoo Cuculus canorus in China. This gradient in China was compared with a similar gradient in Europe.
We surveyed birds in Chernobyl along roads within the Chernobyl Exclusion Zone where we have conducted research during 1991–2019. Ambient radiation at Chernobyl has a patchy distribution that ranges by more than a factor 50,000 with contaminated and clean sites often being separated by distances of just a few hundred meters. We conducted insect transects in Chernobyl using a categorization of level of ionizing radiation more or less than 0.10 μSv/h as the ambient ionizing radiation level. This criterion has been used previously in other studies that we have conducted (Møller and Mousseau, 2009, 2018; Bezrukov et al., 2015). We obtained radiation estimates from our measurements and cross-validated these with measurements by the Ministry of Emergencies.
We measured α, β, and γ radiation at ground level directly at each transect using a handheld dosimeter (Model: Inspector, SE International, Inc., Summertown, TN, United States). We measured levels several (2–3) times at each site and averaged the measurements. Our data were validated with correlation against data from the governmental measurements published by Shestopalov (1996), estimated as the midpoint of the ranges published. This analysis revealed a strong positive relationship (linear regression on log–log transformed data), suggesting that our estimates of radiation provided the same ranking of levels of radiation as did published estimates. The measurements by the Ministry of Emergencies were obtained by repeated standardized measurement of radiation at the ground level in a large number of different localities in Ukraine.
Radiation levels vary considerably at very short geographical distances due to heterogeneity in the deposition of radiation after the Chernobyl accident (Shestopalov, 1996). Our measurements at the transects ranged from 0.10 to 135.89 μSv/h.
Web Site and Web Site Contents
A web site named insect.count.dk was established on February 1 2018 with information on the project in Danish and English, its purpose, a contact email address and a dedicated excel data sheet for entering data.
We published an article in the Danish popular bird journal Fugle & Natur with a circulation of more than 16,000 on May 1 2018.
We asked national natural history or ornithology web sites for participants. These sites included web sites for citizen scientists in Finland, Belgium and Spain.
At the start of surveys the participants were asked to clean the windshield to ensure that no insects were present at the start of a transect. The data sheet requested information on locality, GPS coordinates as latitude and longitude for the start location, date, month, time of day, temperature (°C), wind (Beaufort units), precipitation (mm rain), cloud cover (in units of 0.125) (thus all weather information was recorded locally), number of insects counted on the windshield upon arrival, distance driven (km), speed of car (km/h), windshield area (height and width in cm) and car model.
Participants were only asked to survey insects when driving on roads that they would otherwise have driven for other purposes including their profession and for spare time activities. Hence participants were not asked to make detours in order to participate. There were no restrictions on the number of times that a survey of a given transect could be made nor to the landscapes and habitats along the roads. Surveys were made February–October 2018–2020.
Statistical Analyses
First, we visually inspected the distributions of the data for and deviations from normality or other frequency distributions such as binomial or poisson distributions depending on the distribution of data. Second, analyses of insect data and information on car features, climate and spatial and temporal variation were made using Generalized Linear Models (GLM). Third, data were inspected for goodness of fit to ensure that distributions did not deviate from normality. All statistical models, covariates, distributions of data link functions and fixed effects are reported in Supplementary Table 1. Fourth, we calculated repeatabilities and the associated SE using the equations in Becker (1984), Falconer and Mackay (1996), and Bell et al. (2009). The significance level was set to 0.05.
There was no evidence of collinearity between variables as revealed by variance inflation factors all being less than 5 (McClave and Sincich, 2003). All analyses were made using JMP (SAS, 2012).
Results
Summary Statistics for Insects
A total of 50 observers participated in the study with the number of transects per observer ranging from 1 to 963 transects, mean (SE) = 71.265 transects per observer (25,237). The total number of transects was 3,530 with a mean (SE) number of insects of 35.289 insects per transect (SE = 5.883), or in total 124,567 insects. Transects were 1.0–1,125 km long, with a mean (SE) = 23.518 km (0.778), or in total 83.01 km. Thus, there was between 0 and 420.333 insects per km, on average 1.329 insects per km, SE = 0.249. Transects were made between February 3 and October 6, mean June 23, SE = 0.8 days.
Nine factors explained variation in the abundance of insects accounting for 52% of the variance (Table 1). Features of cars could affect the abundance of insects killed on windshields. The number of insects should increase with faster driving speed. If cars drive faster, there should be more insects killed as we actually observed here [Test 1; Supplementary Table 1; LR = 8.049, df = 1, p = 0.0046, estimate (SE) = 0.00062 (0.00022), 95% CI = 0.00019, 0.0010]. There were also significant differences among car models in number of insects killed on windshields. If windshields differ in angle and other properties for different models of cars, we should expect such variation to be eliminated by inclusion of car brand as a random factor.
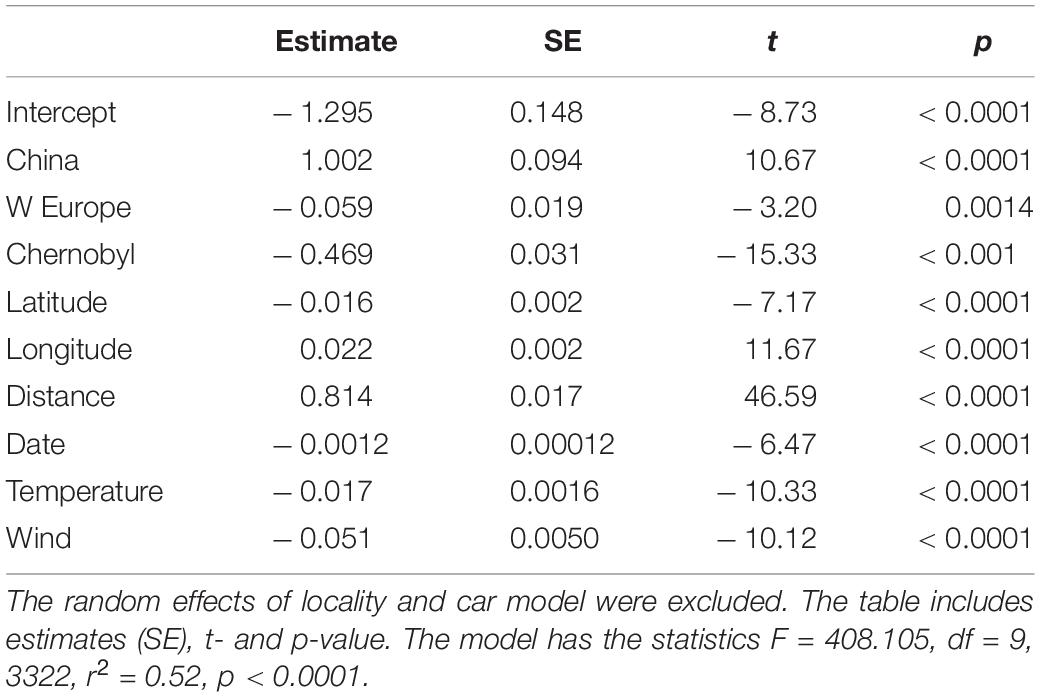
Table 1. Generalized Linear Mixed Model (GLMM) of the abundance of insects in relation to wind speed, temperature, date, distance, longitude, latitude, Chernobyl, Western or Eastern Europe and China or Europe.
Spatial Consistency in Estimates of Insect Abundance
It is an underlying assumption of surveys of all living beings that such surveys provide consistent and reliable information on abundance and diversity of organisms. We tested for consistency in abundance of insects with log10-transformed number of insects as response variable and locality as predictor variable for the 3,530 transects with two or more estimates for all 724 localities in a standard least squares regression. The model had the statistics F = 14.086, df = 722, 2806, r2 = 0.71, p < 0.0001, repeatability R (SE) = 0.727 (0.008). In a second model we predicted log10-transformed number of insects as response variable and locality, longitude, distance, date, temperature and wind as predictors. The model had the statistics F = 15.864, df = 674, 2660, r2 = 0.750, p < 0.0001. This model had as repeatability for abundance of insects of 0.739 (0.008). These repeatabilities for localities were highly consistent showing that the abundance of insects was very similar within localities among days.
Weather and Abundance of Insects
Insects are ectothermic and higher ambient temperatures should thus advance phenology [Test 2; LR = 5.759, df = 1, p < 0.0001, estimate (SE) = –0.005 (0.0002), 95% CI = –0.0083, 0.0008 mm]. Higher wind speed reduced the abundance of insects [Test 3; LR = 26.501, df = 1, p < 0.0001, estimate (SE) = –0.034 (0.007), 95% CI = –0.047, –0.021]. More extensive cloud cover should reduce temperature and hence reduce the abundance of insects as observed in the present study [test 4; LR = 26.306, df = 1, p < 0.0001, estimate (SE) = –0.177 (0.034), 95% CI = 0.245, 0.110].
Temporal Trends in the Abundance of Insects
Insect abundance should increase in spring as temperature increased. There was a significant positive relationship between date and insect abundance when controlling for windshield area, car model, speed, cloud cover, wind, temperature, time, distance and latitude (Test 5; Table 1).
We should expect more insects around noon than early in the morning or late in the evening when it is colder. There was a significant positive linear relationship between time of day when controlling for windshield area, car model, speed cloud cover, wind, temperature, time, distance and latitude [Test 6; LR = 8.130, df = 1, p = 0.0044, estimate (SE) = 0.005 (0.002), 95% CI = 0.0014 0.0075], and there was also a significant quadratic effect as predicted [Test 7; LR = 7.995, df = 1, p 0.0047, estimate (SE) = – 2.741 10–6 (9.687 10–6), 95% CI = –4.54 10–6, –8.418 10–6].
Spatial Abundance of Insects in Chernobyl and Eastern Europe
There were significantly fewer insects in contaminated areas at Chernobyl with more insects per km driven than in control areas in Eastern Europe (Figure 2; Test 8; Table 1). A number of other variables were controlled statistically in this analysis (Supplementary Table 1). There were more insects in Eastern than in Western Europe (Figure 4; Test 9; Table 1).
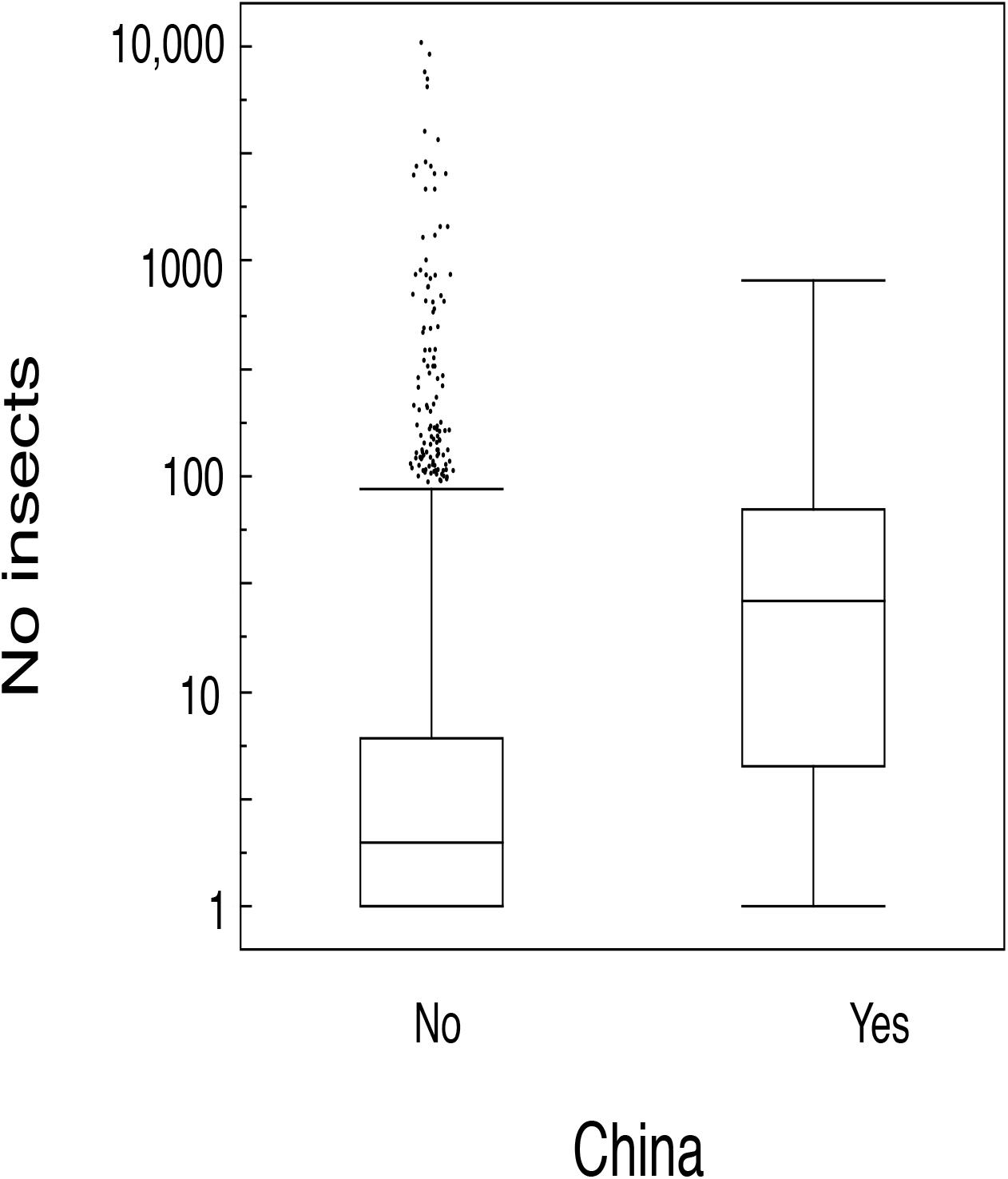
Figure 4. Box plots of the number of insects sampled with a car in China and Europe. The box plots show median, quartiles, 5- and 95-percentiles and extreme values. Observations are jittered to make observations visible.
We predicted a latitudinal trend in the abundance of insects in both countries because of latitudinal differences in productivity (Møller, 2020). There was a significant latitudinal increase in the abundance of insects (Test 10; Table 1). That was also the case separately for the abundance of insects in Europe (Test 11; Table 1). There was significantly more insects in China than in Europe independent of latitude (Figure 5; Test 12; Table 1). Finally, there was an increase in the abundance of insects with longitude (Table 1).
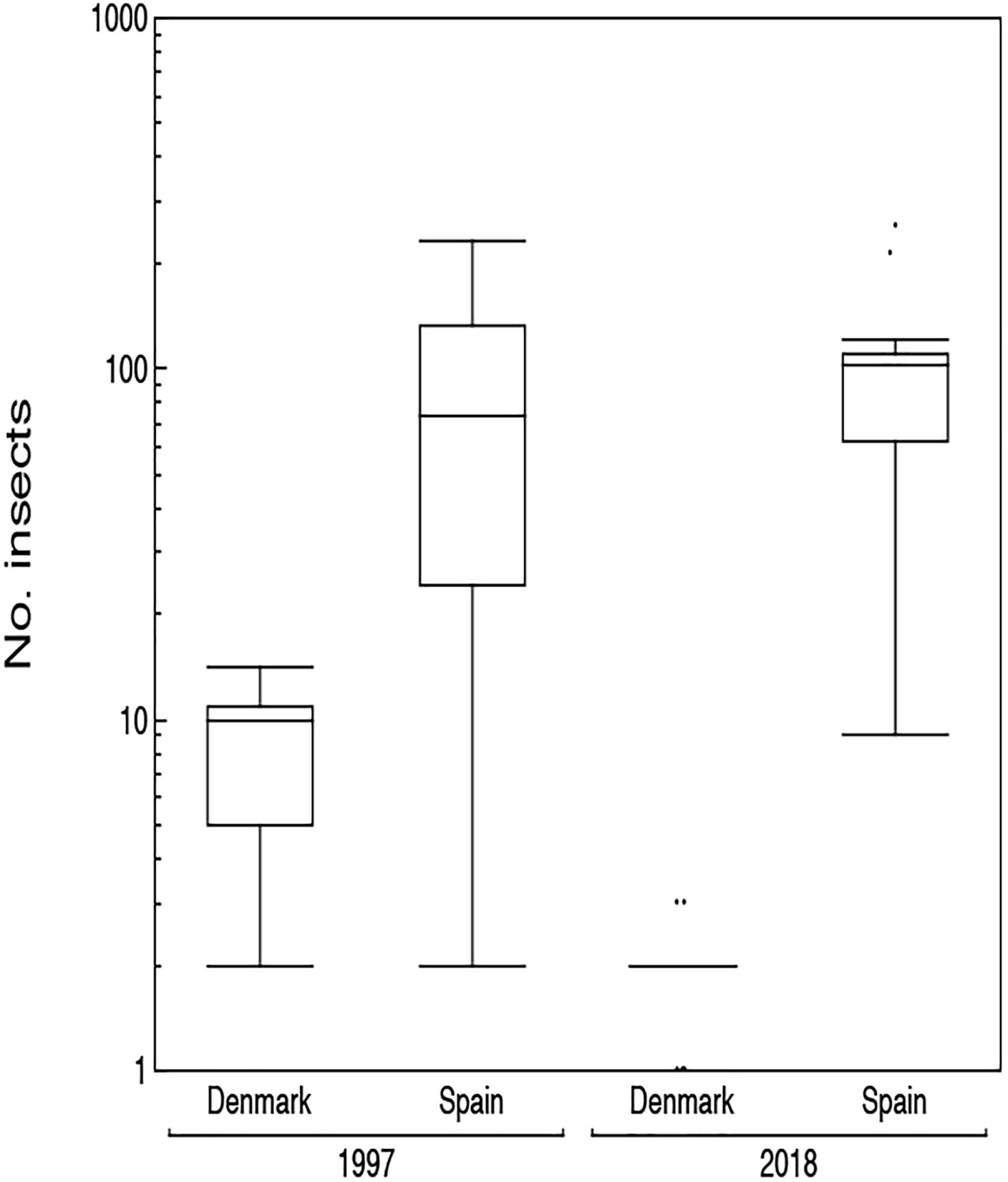
Figure 5. Box plots of the number of insects sampled with a car for Denmark and Spain in 1997 and 2018. The box plots show median, quartiles, 5- and 95-percentiles and extreme values. Observations are jittered to make them visible.
Temporal Trends in Abundance of Insects
Insect abundance was larger at Badajoz, Spain than at Kraghede, Denmark (Figure 5 and Table 2). However, there was no significant difference in insect abundance between 1997 and 2018 (Figure 5 and Table 2). In contrast, there was a significant interaction between country and year (Table 2). The variance in abundance of insects was larger in Spain than in Denmark (Table 2). The abundance of insects was 32% smaller in Denmark than in Spain, and 40% smaller in 2008 than in 1997 as shown by the interaction profiler. These results suggest that there was a in abundance of insects in Denmark, while there was no such decline in Spain.
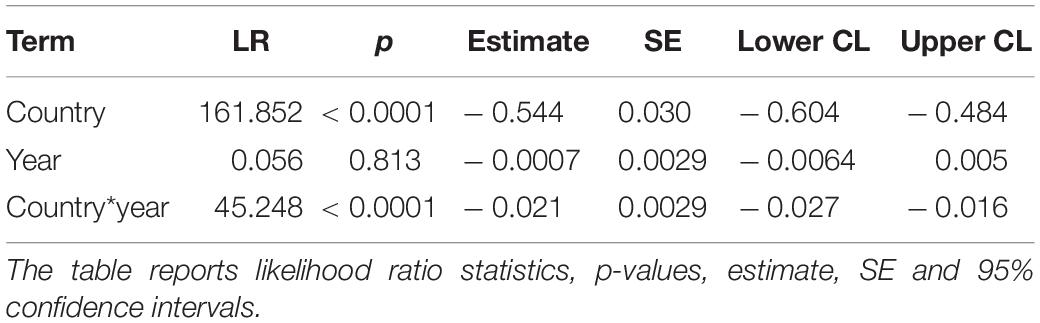
Table 2. Generalized Linear Model (GLM) of number of insects in relation to country (Spain or Denmark), year (1997 or 2018) and country by year interaction in a model with normally distributed data and an identity link function.
Discussion
The present study was based on extensive surveys of almost 124,500 km roads for a total of almost 35,500 flying insects mainly across Europe and China. Previous studies in Denmark have cross-validated abundance estimates of insects using a number of different methods to show high consistency between samples with sweep-nets, sticky plates and feeding rates of barn swallow chicks by parent birds (Møller, 2013, 2019, 2020). We documented a highly consistent repeatability among days, implying that insect surveys provided reliable information on the abundance of insects.
We identified nine factors that accounted for variation in abundance of insects. First, locality accounted for variation in abundance of insects. Second, car model accounted for variation in insect abundance. Third, temperature accounted for insect abundance. Fourth, insect abundance decreased with wind speed. Fifth, insect abundance declined with date. Sixth, insect abundance was lower at Chernobyl than in non-contaminated sites surrounding Chernobyl. Seventh, insect abundance decreased with latitude. Eight, insect abundance increased with longitude. Ninth, there were more insects in China than in Europe. Tenth, there were more insect in Eastern than in Western Europe.
The abundance of insects differed between the two study sites in Spain and Denmark, but not between 1997 and 2018 as revealed by the interaction between year and country. The repeatability of insect abundance within localities among days was high at 0.727 and 0.739, which is highly consistent (Bell et al., 2009). This indicates that the surveys were highly repeatable as required for consistent and reliable surveys of any living being.
The patterns that we have described here addresses a range of ecological questions that we have investigated. While scientific questions may suffer from publication bias (Møller and Jennions, 2002), we have reported all our findings in the present study. After the present paper was preliminarily accepted, we had an additional study accepted reporting reductions in insect abundance when pesticide use, fertilizer use and land-use were entered as predictor variables (Møller et al., 2021).
Extensive insect surveys have shown recent reductions in abundance of insects by as much as 80% during just a few decades, even in nature reserves in Germany where insects are not exterminated (Hallmann et al., 2017; Møller, 2019, 2020). These changes in abundance of insects have been attributed to altered farming practice, altered land-use and the altered underlying factors (Vogel, 2017). While some studies have documented such large reductions in abundance of insect (Hallmann et al., 2017; Tinsley-Marshall, 2019), others have shown smaller declines (Conrad et al., 2002, 2006; Shortall et al., 2009; Fox et al., 2014; Møller, 2020). This raises questions about the generality of these changes, but also the underlying mechanisms accounting for variation in insect abundance.
There is good reason to expect that insect abundance has declined since the 1960s. Among the citizen science participants in this project, 10 drivers reported that when they were driving a car in the 1960s and 1970s, they encountered so many insects that they had to make several stops during a trip of 100 km in order to clean the windshield (Hallmann et al., 2017; Tinsley-Marshall, 2019). Many participants in this project reported that they subjectively had judged that the number of insects was considerably reduced in 2018 compared to previous years due to an unusual warm and dry summer (Meteorologisk Institut, 2018). The present study recorded repeated surveys in 1997 and 2018 at the same two roads in Spain and Denmark showing a 40% decline in Denmark, but no uniform decline at the site in Spain between 1997 and 2018. The reason for the lack of decline in Spain may derive from insect abundance already having declined in 1997 due to more intense agriculture in Spain than in Denmark.
Two pieces of evidence suggest that insect abundance was negatively impacted by human perturbation of environmental conditions. The abundance of insects was reduced by two thirds in Chernobyl compared to uncontaminated control areas. This implies that the abundance of insects has been reduced across more than 50,000 km2 in Ukraine, Belarus and Russia (Møller and Mousseau, 2009, 2018; Bezrukov et al., 2015). Second, we documented differences in insect abundance between Western and Eastern Europe as revealed by similar changes during recent decades when extensive farming became predominant in Western, but subsequently also in Eastern Europe (Tucker and Heath, 1994; Donald et al., 2001; Schimmelfenning and Sedelmeier, 2005).
Factors other than agriculture and associated land-use and crops may account for the observations reported here. The present study identified clear correlations between insect abundance and temperature, cloud cover and wind speed. These effects may apply to short-term weather conditions that affect the flight activity of insects, or they may be attributed to climate change (IPCC, 2007a; Hurrell and Trenberth, 2019). It is likely that insect abundance may change in response to both factors. Møller (2013) has already reported extensive data on insect abundance based on windshield surveys during 1997–2012 as described in this paper. While temperatures during summer have increased in large parts of the temperate zone and at higher latitudes, there has been a decrease in cloud cover and wind speed (IPCC, 2007; Hurrell and Trenberth, 2019), and thus in the main study sites investigated here (Møller, 2013). Such changes in temperature, wind speed and cloud cover should increase the abundance of insects, as shown with insect abundance increasing with temperature and decreasing with wind speed and cloud cover in two study sites in Denmark and Spain in 1997 and 2018.
A number of hypotheses have been proposed to account for latitudinal gradients (e.g., Rohde, 1992; Møller, 2020). We investigated the extent to which latitude and taxa were associated with a reduction in abundance of insects. China had consistently higher abundance of insects in China than in Europe. The reason for such differences in latitudinal trends in the abundance of insects between continents remains to be identified.
In conclusion, the abundance of flying insects provides a relative estimate of the abundance of food for a range of other organisms. Here we have shown that features of vehicles, weather, climate, spatial and temporal variation and the effects of ionizing radiation may contribute independently to estimates of the abundance of flying insects and hence the abundance of food for offspring and parent birds (Møller, 2019). We hypothesize that variation in the abundance of insects will predict the abundance of insectivorous birds as already reported (Møller, 2019).
Data Availability Statement
The raw data supporting the conclusions of this article will be made available by the authors, without undue reservation.
Author Contributions
AM conceived and designed the experiments, and analyzed the data. AM, DC, EF-J, JE, KL, WL, and WW performed the experiments, and wrote and edited the manuscript. All authors contributed to the article and approved the submitted version.
Conflict of Interest
The authors declare that the research was conducted in the absence of any commercial or financial relationships that could be construed as a potential conflict of interest.
Publisher’s Note
All claims expressed in this article are solely those of the authors and do not necessarily represent those of their affiliated organizations, or those of the publisher, the editors and the reviewers. Any product that may be evaluated in this article, or claim that may be made by its manufacturer, is not guaranteed or endorsed by the publisher.
Acknowledgments
Alexandr Artemyev, Jerzy Bańbura, Karen Andersen Bennedsen, Florentino de Lope, John Dowman, Pauline Dowman, Tapio Eeva, Kari Hongisto, Indrikis Krams, Jianping Liu, Jinmei Liu, Bruno Massa, Cezary Mitrus, Arne Moksnes, Federico Morelli, Zaid al Rubaiee, Diego Rubolini, Seppo Rytkönen, Nils Kristian Sallo, Chao Shen, Jacqui Shykoff, Luis Silva, Juan J. Soler, Lone Sønnichsen, Peter Tegl høj, Tingting Yi, Haitao Wang, Yuanxin Xu, and Bo Zhou kindly helped collect the extensive data. Niels Linneberg helped with establishment and maintenance of the website.
Supplementary Material
The Supplementary Material for this article can be found online at: https://www.frontiersin.org/articles/10.3389/fevo.2021.657178/full#supplementary-material
References
Bell, A. M., Hankinson, S. J., and Laskowski, K. L. (2009). The repeatability of behaviour: a meta-analysis. Anim. Behav. 277, 71–83.
Bezrukov, V., Møller, A. P., Milinevski, G., Rushkovsky, S., Sobol, M., and Mousseau, T. A. (2015). Heterogeneous relationships between abundance of invertebrates and radiation from Chernobyl. Ecol. Indicat. 52, 128–133. doi: 10.1016/j.ecolind.2014.11.014
Bibby, C. J., Hill, D. A., Burgess, N. D., and Mustoe, S. (2005). Bird Census Techniques. London, UK: Academic Press.
Blondel, J., Ferry, C., and Frochot, B. (1970). La méthode des indices ponctuels d’abondance (I.P.A.) au des relevés d’avifaune par “stations d”ecoute’. Alauda 38, 55–71.
Conrad, K. F., Warren, M. S., Fox, R., Parsons, M. S., and Woiwod, I. P. (2006). Rapid declines of common, widespread British moths provide evidence of an insect biodiversity crisis. Biol. Cons. 132, 279–291. doi: 10.1016/j.biocon.2006.04.020
Conrad, K. F., Woiwood, I. P., and Perry, J. N. (2002). Long-term decline in abundance and distribution of the garden moth (Arctia caja) in Great Britain. Biol. Cons. 106, 329–337. doi: 10.1016/s0006-3207(01)00258-0
Crain, R., Cooper, C., and Dickinson, J. L. (2014). Citizen science: a tool for integrating studies of human and natural systems. Ann. Rev. Environ. Res. 39, 641–665. doi: 10.1146/annurev-environ-030713-154609
Donald, P. F., Green, R. E., and Haeth, M. F. (2001). Agricultural intensification and the collapse of Europe’s farmland bird populations. Proc. R. Soc. Lond. B 268, 25–29. doi: 10.1098/rspb.2000.1325
Falconer, D. S., and Mackay, T. F. C. (1996). Introduction to Quantitative Genetics, 4th Edn. New York, NY: Longman.
Fox, R., Oliver, T. H., Harrower, C., Parsons, M. S., Thomas, C. D., and Roy, D. B. (2014). Long-term changes to the frequency of occurrence of British moths are consistent with opposing and synergistic effects of climate and land-use changes. J. App. Ecol. 51, 949–957. doi: 10.1111/1365-2664.12256
Hallmann, C. A., Foppen, R. P. B., van Turnhout, C. A. M., de Kroon, H., and Jongejans, E. (2014). Declines in insectivorous birds are associated with high neonicotinoid concentrations. Nature 511:341. doi: 10.1038/nature13531
Hallmann, C. A., Sorg, M., Jongejans, E., Siepel, H., Hofland, N., Schwan, H., et al. (2017). More than 75 percent decline over 27 years in total flying insect biomass in protected areas. PLoS One 12:e0185809. doi: 10.1371/journal.pone.0185809
Hurrell, J. W., and Trenberth, K. E. (2019). “Climate change,” in Birds and Climate Change, eds P. O. Dunn and A. P. Møller (New York, NY: Oxford University Press).
IPCC (2007a). Climate Change 2007: Impacts, Adaptation and Vulnerability. Cambridge, MA: Cambridge University Press.
IPCC (2007b). Climate Change 2007: The Physical Science Basis. Cambridge, MA: Cambridge University Press.
Meteorologisk Institut (2018). Vejret i Danmark – året 2018. Available online at: http://www.dmi.dk/vejr/arkiver/maanedsaesonaar/vejret-i-danmark-aaret-2018/ (2019) (accessed December 31, 2018).
Møller, A. P. (1983). Methods for Monitoring Bird Populations in the Nordic Countries. Oslo: Nordic Council of Ministers.
Møller, A. P. (1987). Advantages and disadvantages of coloniality in the swallow Hirundo rustica. Anim. Behav 35, 819–832. doi: 10.1016/s0003-3472(87)80118-5
Møller, A. P. (2001). The effect of dairy farming on barn swallow Hirundo rustica abundance, distribution and reproduction. J. Appl. Ecol. 38, 378–389. doi: 10.1046/j.1365-2664.2001.00593.x
Møller, A. P. (2013). Long-term trends in wind speed, insect abundance and ecology of an insectivorous bird. Ecosphere 4:6.
Møller, A. P. (2019). Parallel declines in abundance of insects and insectivorous birds in Denmark over 22 years. Ecol. Evol. 9, 6581–6587. doi: 10.1002/ece3.5236
Møller, A. P. (2020). Quantifying rapidly declining abundance of insects in Europe using a paired experimental design. Ecol. Evol. 10, 2446–2451. doi: 10.1002/ece3.6070
Møller, A. P., Czeszczewik, D., Flensted-Jensen, E., Erritzøe, J., Krams, I., Laursen, K., et al. (2021). Abundance of insects and aerial insectivorous birds in relation to pesticide and fertilizer use. Avian Res. [Epub ahead of print].
Møller, A. P., and Jennions, M. D. (2002). How much variance can be explained by ecologists and evolutionary biologists? Oecologia 132, 492–500. doi: 10.1007/s00442-002-0952-2
Møller, A. P., and Mousseau, T. A. (2009). Reduced abundance of insects and spiders linked to radiation at Chernobyl 20 years after the accident. Biol. Lett. 5, 356–359. doi: 10.1098/rsbl.2008.0778
Møller, A. P., and Mousseau, T. A. (2018). Reduced colonization by soil invertebrates to irradiated decomposing wood in Chernobyl. Sci. Total Environ. 645, 773–779. doi: 10.1016/j.scitotenv.2018.07.195
Nocera, J. J., Blais, J. M., Beresford, D. V., Finity, L. K., Grooms, C., Kimpe, L. E., et al. (2012). Historical pesticide applications coincided with an altered diet of aerially foraging insectivorous chimney swifts. Proc. R. Soc. B Biol. Sci. 279, 3114–3120. doi: 10.1098/rspb.2012.0445
Nyffeler, M., and Bonte, D. (2020). Where have all the spiders gone? Observations of a dramatic population density decline in the once abundance garden spider, Araneus diadematus (Araneae: Araneidae), in the Swiss Midlands. Insects 11:248. doi: 10.3390/insects11040248
Pomfret, J. K., Nocera, J. J., Kyser, T. K., and Reudink, W. M. (2000). Linking population declines with diet quality in Vaux’s swifts. Northwest Sci. 88, 305–313. doi: 10.3955/046.088.0405
Poulin, B., Lefebvre, G., and Paz, L. (2010). Red flag for green spray: adverse trophic effects of Bti on breeding birds. J. Appl. Ecol. 47, 884–889. doi: 10.1111/j.1365-2664.2010.01821.x
Reif, J., Böhning-Gaese, K., Flade, M., Schwarz, J., and Schwager, M. (2011). Population trends of birds across the iron curtain: brain matters. Biol. Cons. 144, 2524–2533. doi: 10.1016/j.biocon.2011.07.009
Rohde, K. (1992). Latitudinal gradients in species-diversity: the search for the primary cause. Oikos 65, 514–527. doi: 10.2307/3545569
Schimmelfenning, F., and Sedelmeier, U. (2005). The Europeanization of Central and Eastern Europe. Ithaca: Cornell University Press.
Shortall, C. R., Moore, A., Smith, E., Hall, M. J., Woiwood, I. P., and Harrington, R. (2009). Long-term changes in the abundance of flying insects. Insect Conserv. Divers. 2, 251–260. doi: 10.1111/j.1752-4598.2009.00062.x
Taylor, L. R. (1974). Insect migration, flight periodicity and the boundary layer. J. Anim. Ecol. 43, 225–238. doi: 10.2307/3169
Teglhøj, P. G. (2017). A comparative study of insect abundance and reproductive success of barn swallows Hirundo rustica in two urban habitats. J. Avian Biol. 48, 846–853. doi: 10.1111/jav.01086
Tucker, G. M., and Heath, M. F. (1994). Birds in Europe: Their Conservation Status. Cambridge, MA: BirdLife International.
Vogel, G. (2017). Where have all the insects gone? Science 356, 576–579. doi: 10.1126/science.356.6338.576
Keywords: birds, changes in abundance of flying insect, Chernobyl, citizen science, windshields
Citation: Møller AP, Czeszczewik D, Erritzøe J, Flensted-Jensen E, Laursen K, Liang W and Walankiewicz W (2021) Citizen Science for Quantification of Insect Abundance on Windshields of Cars Across Two Continents. Front. Ecol. Evol. 9:657178. doi: 10.3389/fevo.2021.657178
Received: 22 January 2021; Accepted: 23 July 2021;
Published: 20 August 2021.
Edited by:
Anne Bowser, Woodrow Wilson International Center for Scholars (SI), United StatesReviewed by:
Iain James Gordon, Australian National University, AustraliaMark Chandler, Earthwatch Institute, United States
Copyright © 2021 Møller, Czeszczewik, Erritzøe, Flensted-Jensen, Laursen, Liang and Walankiewicz. This is an open-access article distributed under the terms of the Creative Commons Attribution License (CC BY). The use, distribution or reproduction in other forums is permitted, provided the original author(s) and the copyright owner(s) are credited and that the original publication in this journal is cited, in accordance with accepted academic practice. No use, distribution or reproduction is permitted which does not comply with these terms.
*Correspondence: Anders Pape Møller, YW5kZXJzLm1vbGxlckB1LXBzdWQuZnI=
†Deceased