- 1Desert Laboratory on Tumamoc Hill, University of Arizona, Tucson, AZ, United States
- 2U.S. Geological Survey, Fort Collins Science Center, Ft. Collins, CO, United States
- 3School of Government and Public Policy, University of Arizona, Tucson, AZ, United States
- 4School of Natural Resources and the Environment, University of Arizona, Tucson, AZ, United States
- 5Arizona-Sonora Desert Museum, Tucson, AZ, United States
- 6Saguaro National Park, National Park Service, Tucson, AZ, United States
- 7Planet Labs, Inc., San Francisco, CA, United States
- 8Natural Resource Ecology Laboratory, Colorado State University, Ft. Collins, CO, United States
- 9U.S. Geological Survey, Science and Decisions Center, Reston, VA, United States
In the southwestern United States, non-native grass invasions have increased wildfire occurrence in deserts and the likelihood of fire spread to and from other biomes with disparate fire regimes. The elevational transition between desertscrub and montane grasslands, woodlands, and forests generally occurs at ∼1,200 masl and has experienced fast suburbanization and an expanding wildland-urban interface (WUI). In summer 2020, the Bighorn Fire in the Santa Catalina Mountains burned 486 km2 and prompted alerts and evacuations along a 40-km stretch of WUI below 1,200 masl on the outskirts of Tucson, Arizona, a metropolitan area of >1M people. To better understand the changing nature of the WUI here and elsewhere in the region, we took a multidimensional and timely approach to assess fire dynamics along the Desertscrub-Semi-desert Grassland ecotone in the Catalina foothills, which is in various stages of non-native grass invasion. The Bighorn Fire was principally a forest fire driven by a long-history of fire suppression, accumulation of fine fuels following a wet winter and spring, and two decades of hotter droughts, culminating in the hottest and second driest summer in the 125-yr Tucson weather record. Saguaro (Carnegia gigantea), a giant columnar cactus, experienced high mortality. Resprouting by several desert shrub species may confer some post-fire resiliency in desertscrub. Buffelgrass and other non-native species played a minor role in carrying the fire due to the patchiness of infestation at the upper edge of the Desertscrub biome. Coupled state-and-transition fire-spread simulation models suggest a marked increase in both burned area and fire frequency if buffelgrass patches continue to expand and coalesce at the Desertscrub/Semi-desert Grassland interface. A survey of area residents six months after the fire showed awareness of buffelgrass was significantly higher among residents that were evacuated or lost recreation access, with higher awareness of fire risk, saguaro loss and declining property values, in that order. Sustained and timely efforts to document and assess fast-evolving fire connectivity due to grass invasions, and social awareness and perceptions, are needed to understand and motivate mitigation of an increasingly fire-prone future in the region.
Introduction
The frequency and size of large fires across western North America are increasing with longer growing and fire seasons, record high temperatures, and more severe and hotter droughts, specifically since the mid-1980s (Abatzoglou and Williams, 2016; Westerling, 2016). An equally important driver are non-native grass invasions that have altered wildfire regimes at mid to low elevations in semi-arid areas (Fusco et al., 2019).
Desert shrublands, characterized mostly by long-lived shrubs (and cacti in the subtropics) and extensive areas of barren soil, dominate the lowest elevations (<1,200 masl) in the region. These shrublands expanded with warming and drying during the Holocene, with many species reaching their northernmost distributions only during the past few millennia (e.g., McAuliffe and Van Devender, 1998; Koehler et al., 2005; Holmgren et al., 2007). Although historical evidence is lacking, conventional wisdom has it that the wide spacing of plants and insufficient continuity of fuel renders these desert shrublands mostly fireproof, specifically in the hot subtropical deserts (Mojave, Sonoran, and Chihuahuan), which harbor mostly non-fire adapted taxa. A contributing factor to the prevalence of barren ground may be the recency of desert shrublands and a lengthy delay in the migration and establishment of perennial plants that can take advantage of the underutilized space and resources (Betancourt, 2012). The prevalence of barren soil, however, may be due in large part to seasonal aridity and insufficient soil moisture. After infrequent wet (El Niño) winters, much of the empty space between shrubs and cacti fills with native annual forbs that decompose rapidly and do not greatly affect fuel loads (Brooks, 1999). In recent times, desert fires were confined to these wet years when there was sufficient fine fuel to carry a wildland fire, most frequently in June when the fuels had dried out (McLaughlin and Bowers, 1982).
Evidence for the lack of frequent or extensive wildfires in Sonoran and Mojave Desert desertscrub vegetation prior to grass invasions is mostly indirect but still persuasive. Neither foothill palo verde (Parkinsonia micophylla) nor the giant columnar cactus (Carnegiea gigantea), the dominant species in the Arizona Upland of the Sonoran Desert, are fire resistant. Saguaro has long-lived but fire-sensitive epidermis and foothill palo verde has photosynthetic bark that can be damaged easily by fire; individuals of both are seldom consumed by fire but they are often killed by girdling or top-killed. Saguaros do not resprout. Foothill palo verdes can resprout after fire, but re-sprouting plants are susceptible to repeated fires. Recruitment of both foothill palo verde and saguaro is slow. Evidence for the lack of fire historically includes regional surges in saguaro regeneration between 1780 and 1860 throughout southern Arizona, long prior to 20th-century grass invasions and altered fire regimes (Pierson et al., 2013).
Red brome (Bromus rubens), a non-native annual grass that fuels desert fires, spread rapidly into the Mojave and Sonoran Deserts with frequent consecutive wet winters associated with the positive phase of the Pacific Decadal Oscillation (PDO) from 1977 to 1995 (Salo, 2005). Wildfires in the Arizona Upland of the Sonoran Desert increased in frequency beginning in the 1970s (McLaughlin and Bowers, 1982; Rogers, 1986; Schmid and Rogers, 1988) and tapered off with increasing aridity between 1999 and 2004 (Alford et al., 2005). That changed dramatically following the wet El Niño winter of 2004–2005, when an unprecedented ∼5,000 km2 burned in desertscrub across the Mojave and Sonoran Desert (Brooks et al., 2013). Invasive-fueled fires tend to die out in uninvaded desertscrub, much like wildfires in semi-desert native grassland, woodlands, and forests at higher elevations invariably die out downslope as they also encounter uninvaded desertscrub (see Brooks and Pyke, 2001; Parker, 2002). It remains an untested though likely assumption that wildfires were uncommon and of limited spatial extent prior to the introduction and spread of non-native grasses.
The notion of fireproof deserts has changed in recent decades with the exponential spread of both winter annual (e.g., cheatgrass: Bromus tectorum, Spanish and red brome: B. madritensis and B. rubens, Mediterranean grass: Schismus barbatus) and perennial C4, (buffelgrass: Cenchrus ciliaris, fountain grass: C. setaceus, Lehmann’s lovegrass: Eragrostis lehmanniana) non-native grasses capable of colonizing the copious bare areas available in desert shrublands. The result of these grass invasions has been a large increase in the frequency and size of wildfires at desert elevations (Schmid and Rogers, 1988; Alford et al., 2005). This is a self-perpetuating grass-fire cycle that progressively excludes native plants and results in grassification, the physiognomic conversion of shrublands to grassland (D’Antonio and Vitousek, 1992; Williams and Baruch, 2000; Brooks and Chambers, 2011; Betancourt, 2015).
A new fire mosaic is being established in the region whereby wildfires driven by invasive grasses can spread from the desert valleys to the forested mountains, and vice versa (Figure 1). Fire-adapted vegetation communities occur on the “Sky Islands” (mountains) of the southwestern U.S. and northwest Mexico in a classic elevational zonation gradient from Semi-desert Grasslands to oak and chaparral, up to mixed conifer forests (Merriam, 1890; Whittaker and Niering, 1965). Fire regimes in mountains of western North America have been altered over the last century or more. Livestock grazing and fire suppression have resulted in fuel buildup over the last 150 years, culminating in more frequent large forest fires, with record warming and drying in recent decades exacerbating the trend (Westerling, 2016). Meanwhile, non-native grass invasions and novel fire regimes in desert shrublands and increased urbanization at lower elevations in recent decades are transforming fire dynamics at the wildland-urban interface (WUI) of the Sky Islands, where the deserts meet the forests.
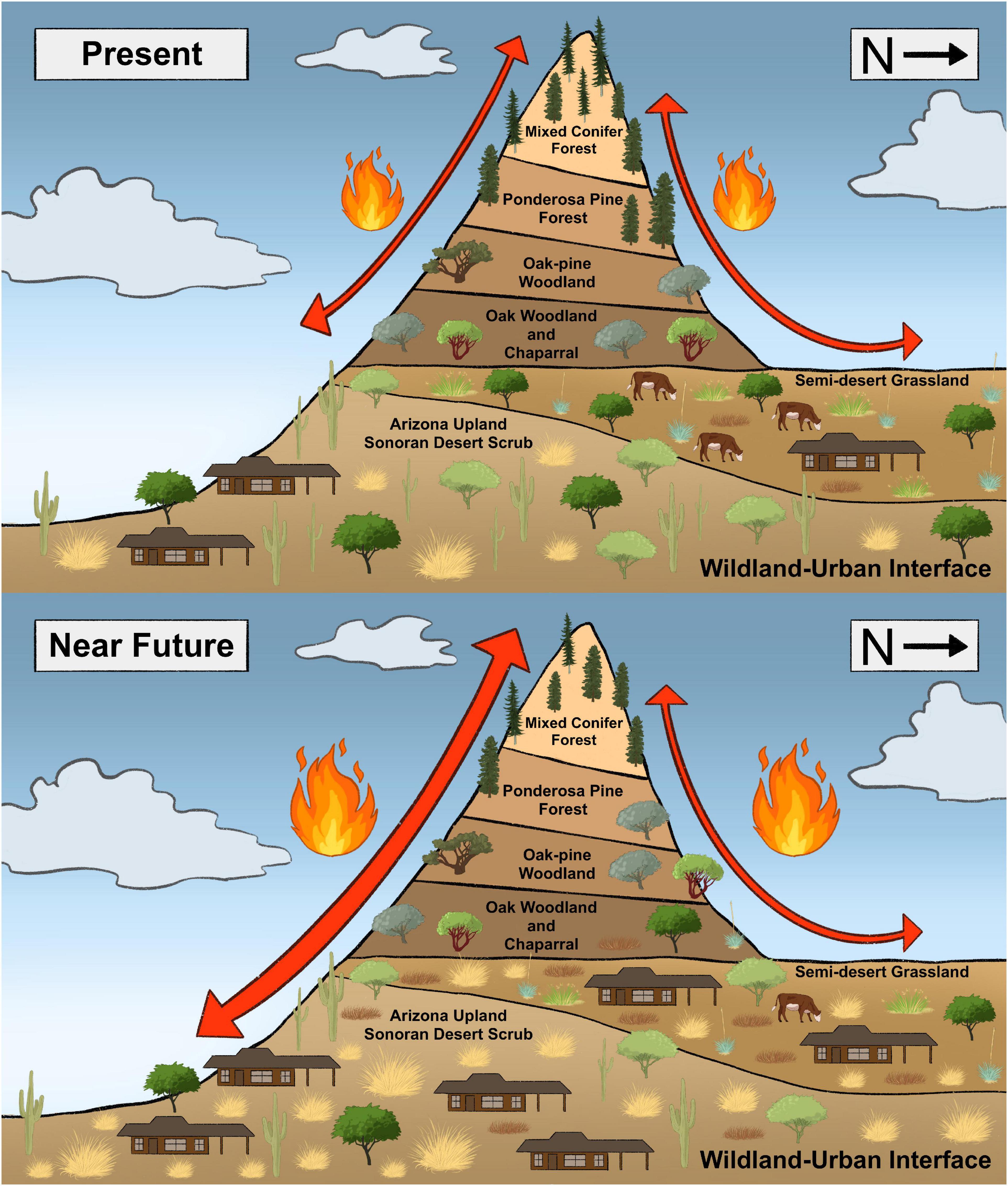
Figure 1. Conceptual representation of grassification driven fire connectivity of the Sky Islands of arid North America. Present conditions have an expanding front of non-native grasses that will promote an increase of fuels and connectivity at the wildland-urban interface and the Desertscrub/Semi-desert Grassland ecotone in the coming years and couple decades (Figure by MRS).
The socioeconomic impacts of these novel wildfire regimes are considerable and mounting. Much of the physical infrastructure and urban development in the arid parts of the western United States is concentrated at desert elevations, in precisely those areas most affected by grass invasions and associated fire risks. Population density and mobility facilitate both the spread of non-native grasses and human ignitions. Managers of public lands, transportation departments, and cities and towns now need to plan around a novel and fast-evolving fire risk, and they face escalating non-native plant (fuels) control and fire suppression costs at a time when federal, state, and local government budgets are being flatlined and are not keeping up with inflation and increasing need. Other economic costs include decreased property values and a reevaluation of fire insurance in increasingly fire-prone areas, as well as losses in tourism revenue with decaying ecological backdrops.
The impacts of grass invasions and associated wildfires are not limited to desert elevations. In the 21st century, the largest desert wildfires have burned 104–105 ha, with some spreading from the basins to the mountains, altering fire regimes in adjacent native grasslands, chaparral, woodlands, and forests. The spread of broad ignition fronts from grass-invaded desert shrublands to forested highlands, and vice versa, is probably novel. This new fire dynamic has yet to be addressed specifically in the scientific literature about grass invasions, altered wildfire regimes, and management of the WUI that characterizes mountain piedmonts in the western United States. Notable wildfires that were fueled in large part by red brome and other non-native winter annuals in summer 2005 at desert elevations, and burned across the WUI into adjacent vegetation types, include the Cave Creek Complex Fire (1,005 km2) in Central Arizona, the Southern Nevada Complex Fire (2,059 km2), and the Hackberry Fire (236 km2) in eastern California. These wildfires were preceded by an unusually wet El Niño year and a broadly synchronous bloom of red brome and other non-native winter annuals. These large fires heralded what one writer called “The Year We Lost the Desert” (Clark, 2006). After another wet winter in 2019–2020, the Bush Fire (783 km2) was fueled by red brome and Mediterranean grass on the outskirts of Phoenix in central Arizona and burned upslope into the Superstition Mountains. Further south, the Bighorn Fire (486 km2) burned over much of the Santa Catalina Mountains on the outskirts of Tucson, Arizona, a metropolitan area supporting more than a million people. The 2020 Bighorn Fire provided us the opportunity to evaluate the spread of an ignition front at the Desertscrub/Semi-desert Grassland ecotone, which is in various stages of buffelgrass and other grass invasions in the Catalina Foothills.
To better understand the changing nature of the WUI in the region, we assessed the impacts of the 2020 Bighorn Fire in desertscrub weeks to months after it happened. Our aims were to: (1) establish a baseline of expansion and infestation of buffelgrass in the Santa Catalina Foothills WUI; (2) evaluate the fire behavior and the degree to which fire spread or died out in Desertscrub invaded (or not) by buffelgrass; (3) quantify species responses to the fire, including losses of the iconic saguaro; (4) adapt a state-and-transition fire-spread model to simulate present and future fire risks associated with buffelgrass invasion; and (5) assess whether the fire affected residents’ perceptions of buffelgrass as a fire threat. We use this case study to draw broader lessons about grass invasions and altered wildfire regimes in the North American subtropics and elsewhere.
Materials and Methods
Study Area
The Tucson Basin, encompassing the Tucson Metropolitan Area in southern Arizona, spans an area of 1,200 km2 and is bounded by mountain ranges including the Tucson Mountains to the West and the Santa Catalina (Catalinas herein), Rincon, and Santa Rita Mountains to the north, east, and south, respectively. The Catalinas form part of the Coronado National Forest, established in 1908. The elevational gradient is steep, rising from ∼800 masl in the City of Tucson on the basin floor to ∼2,800 masl on Mt. Lemmon, the highest peak in the Catalinas, a distance of just 30 km. Temperature and precipitation gradients are accordingly sharp. Average annual temperature and precipitation is 20°C and 300 mm in Tucson and 10°C and 750 mm on Mt. Lemmon. These contrasts are reflected in vegetation zonation of the Catalinas, which spans seven different biomes: Arizona Upland Sonoran Desertscrub, Semi-desert Grassland, Oak Woodland, Chaparral, Oak-pine Woodland, Ponderosa Pine Forest, and Mixed Conifer Forest (e.g., Whittaker and Niering, 1965; Brusca and Moore, 2013; Figure 1). The Desertscrub biome occupies elevations below 1,200 masl and contains plant communities characterized by dense stands of foothill palo verde and saguaro, highly diverse and in various stages of invasion by non-native grasses. Directly above Desertscrub is Semi-desert Grassland, an open grassland with scattered woody species spanning 1,200 to 1,400 masl but extending to 1,700 masl on xeric slopes. Dominated by summer-flowering annual and perennial grasses, grass cover can increase by 50% after wildfires, which are frequent, mostly natural, and generally die out at Desertscrub’s edge (Whittaker and Lowe, 1984).
The most prominent and aggressive invasive grass species in the Sonoran Deserts is buffelgrass (Cenchrus ciliaris, syn. Pennisetum ciliare), a flammable grass native to Africa, the Middle East, and southern Asia and invasive in subtropical areas of Australia, North America, South America, and many islands in the Pacific Ocean (including Hawaii), Indian Ocean and the Caribbean Sea. In North America, buffelgrass was introduced to improve cattle yields (State of Sonora, Mexico) and control erosion (Arizona) beginning in the 1930s. By the 1980s, buffelgrass was spreading rapidly throughout Arizona with landscape level expansion in Sonora, Mexico (e.g., Franklin et al., 2006). In the Sonoran Desert, buffelgrass reduces species richness and diversity of native vegetation through competition (Olsson et al., 2012a) and elevates fire risk at the WUI (Olsson et al., 2012b). Buffelgrass burns hotter than most other native and non-native grasses, reaching fire temperatures of 800–900°C (McDonald and McPherson, 2013). Measured buffelgrass fuel loads range from 250 to 900 g/m2 at study sites in Southern Arizona, more than sufficient to drive grassland fire (Esque et al., 2007; McDonald and McPherson, 2013). Buffelgrass fuel loads fluctuate less than that of non-native, winter annual grasses, creating a more consistent fire hazard in the arid foresummer (May-June).
The forest fire history of the Catalinas during the past four centuries is relatively well known thanks to fire scar studies using tree rings (Iniguez et al., 2008, 2016). It is characterized by frequent and episodic surface fires that decreased dramatically at the turn of the 20th century due to prolonged livestock grazing and fire suppression. Increasing fuel loads and connectivity eventually led to major wildfires: the 2002 Bullock Fire (124 km2), the 2003 Aspen Fire (344 km2), and the 2020 Bighorn Fire (486 km2). In addition, in the summer of 2019 the Mercer Fire (0.1 km2) was ignited by lightning in a dense saguaro-palo verde stand heavily infested with buffelgrass.
The Bighorn Fire was started by a lightning strike late on June 5, 2020, in steep and rugged terrain of the Pusch Ridge Wilderness area in the southwest corner of the Catalinas, overlooking the fast-growing suburban town of Oro Valley on the northern outskirts of Tucson (Figures 2, 3). By July 23, when the fire was 100% contained, the largest recorded wildfire in the Catalina Mountains had burned 486 km2, pushed by a delayed and dry monsoon season, periodic strong winds, and the hottest summer in Tucson’s 125-yr weather record. These hot and dry conditions were preceded by a wet winter 2019 and early spring 2020, which added fine fuels in desertscrub from abundant winter annuals, including the invasive red brome.
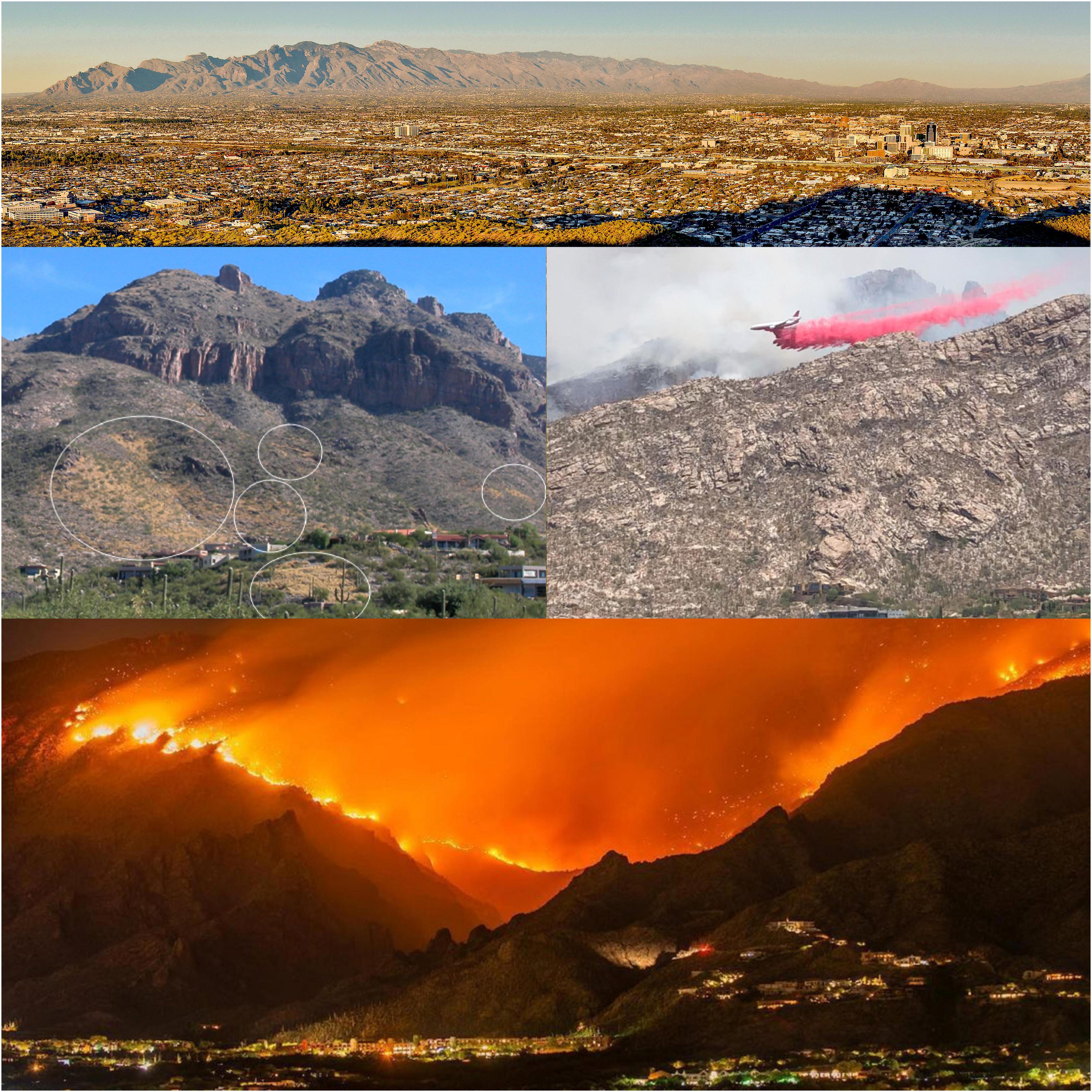
Figure 2. The Santa Catalina Mountains and 2020 Bighorn Fire, Tucson, Arizona. Counter clockwise from top: Looking north over the of City of Tucson to the Catalina Mountains from Tumamoc Hill, photo by Paul Mirocha; front range of the Catalinas with buffelgrass infested south-facing slopes (yellow patches inside white circles), photo courtesy of the Arizona-Sonora Desert Museum; The Bighorn Fire dropping down the front range of the Catalinas into Ventana Canyon and the Tucson wildland-urban interface, 20 June 2020, photo by A.T. Willett; slurry bomber dropping retardant just above the WUI on the front range of the Catalinas, 12 June 2020, photo by BTW.
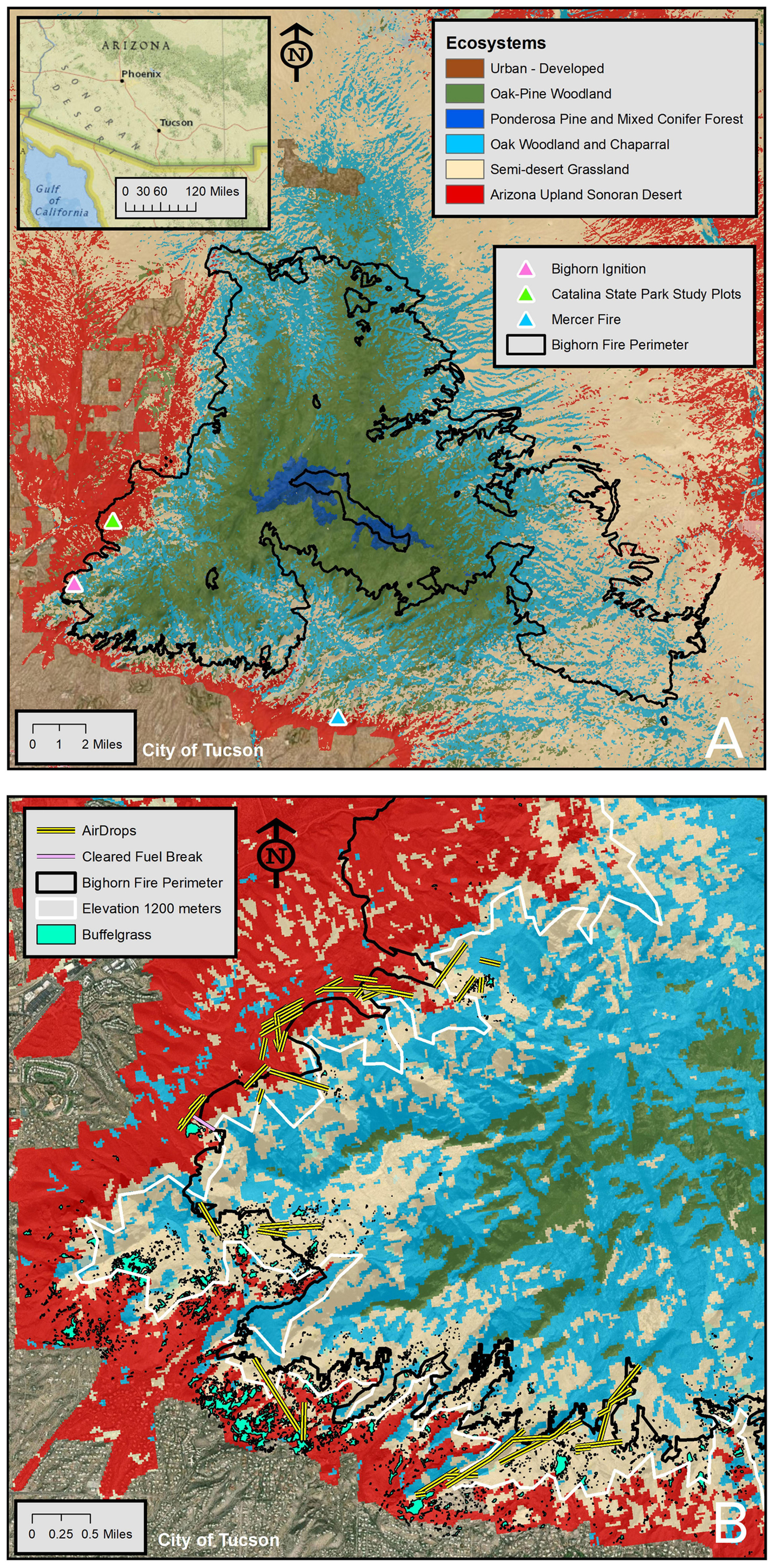
Figure 3. The Santa Catalina Mountains and the Bighorn Fire perimeter. (A) The Catalina Mountains and ecosystems. (B) Close up of the southwest portion of the Catalina Mountains and the wildland-urban interface with present-day buffelgrass infestation, Bighorn Fire suppression efforts, and the 1,200 masl Desertscrub/Semi-desert Grassland ecotone.
The fire ignited on a steep, north-facing slope on the southwestern flanks of the Catalinas at ∼ 1,200 masl. The fire moved from west to east into the Catalina front range and, on the nights of June 11, 20, and 21, dropped down into canyons at the edge of the WUI and forced alerts and evacuations of homes in the desert near the Coronado National Forest boundary (Figure 2). At the same time, the fire pushed north and by June 11th had grown to more than 25 km2, threatening nearly 1,000 homes in the municipality of Oro Valley and the Catalina Foothills community of Tucson. The fire later threatened the mountain village of Summerhaven, which was successfully defended by firefighters. The fire also threatened the town of Oracle on the north flank, and ranches to the east, before eventually dying out as humidity increased with a late onset of the monsoon.
Between August and December 2020, several of the authors undertook day hikes to locations along the lower edge of the Bighorn Fire (Supplementary Figure 1). The goals were (1) to assess the fuels that brought the fire to its lowest extent, especially the role of native and non-native species, including buffelgrass and (2) assess the landscape features that halted the fire. In some cases, it was impossible to identify the burned remains, but frequently we could identify species by re-sprouting stems. To aid identification, we also extrapolated from unburned islands of vegetation, often smaller than a meter square, spared by firebreaks created by natural features such as exposed rock formations.
Mapping Buffelgrass Infestation
Buffelgrass has been steadily expanding in the Catalina foothills, or the front (south) range of the Catalina Mountains adjacent to Tucson for several decades (Figures 2, 3B). Previous attempts to map the infestation in time and space were made in 2008 and 2016 but quality of accuracy and precision, and even positive identification, have been questioned by stakeholders. This has limited an understanding of rates of spread, treatment efforts, fire prevention or other management actions.
We used Google Earth Pro, historical aerial photography, and satellite imagery to map the buffelgrass distribution in 2018 as a close estimate of the extent of the infestation at the time of the 2020 Bighorn Fire. The entire front range of the Catalinas was scanned from 1,500 masl to the WUI. Polygons were drawn around buffelgrass patches seen on the best historical imagery. A “patch” is defined as a mostly contiguous area of buffelgrass plants or a distinct cluster of buffelgrass plants amid an area of non-buffelgrass. The assessment of whether or not a feature was identified as buffelgrass was based on expert opinion rooted in field experience and observations. Experience with remote sensing of buffelgrass via a variety of techniques and image types for effective mapping (Olsson et al., 2009, 2011; Elkind et al., 2019) led to the decision that, for this particular area, hand-drawn aerial photo interpretation was more accurate than existing surveys and automated techniques using multispectral imagery.
Identifying and mapping buffelgrass in the Catalinas is possible because of the unique growth pattern that makes it a threat in wildfire-based ecosystem transformation. The high cover of homogenous buffelgrass cover in invaded areas differs distinctly from the rich and diverse structure of nearby uninvaded areas that are dominated by bare mineral soil but boast high species and structural diversity. In a study of buffelgrass impacts on species diversity that includes portions of the Catalinas affected by the fire, Olsson et al. (2012b) found the interiors of buffelgrass patches to be characterized by >44% buffelgrass cover with very little species diversity. These characteristics make aerial photo interpretation of buffelgrass boundaries both practical and effective as demonstrated by Olsson et al. (2012b) and validated by Olsson and Morisette (2014).
Given the variable nature of the imagery, the topographical layout of the Catalinas, and the relative abundance of different imagery due to proximity to a high-value imaging target (Tucson), image quality varied across the region. As such, some areas were mapped with higher confidence than others. South and southwest-facing imagery, which have good lighting properties in satellite images, are good habitat for buffelgrass. In these areas buffelgrass patches tend to be dense and have discrete boundaries that decrease in size with distance from a central focal point (possibly the original infestation location). This pattern is easier to positively identify than patches in which buffelgrass is diffuse or scattered evenly among other vegetation. Smaller patches are harder to distinguish and confidently assess because there are fewer visual cues that convey the texture or color of a buffelgrass patch. They tend to be easier to map more confidently when larger patches are nearby because color and texture are easier to compare and spatial autocorrelation indicates that a small patch is more likely to be found next to a big patch than all by itself on an otherwise clear hillslope.
North- and north-east facing slopes tend to have higher vegetation density of native vegetation. Above 1,200 masl, Arizona Upland Sonoran Desertscrub transitions into Semi-desert Grassland and buffelgrass is found in both ecosystems, though it decreases in occurrence as elevation increases. Buffelgrass is less distinct when situated in a matrix of native and other non-native grasses of the higher-elevation desert grassland.
The accuracy of the map has been estimated by ground truthing in select areas in combination with fuel assessment hikes (see “Study area” above and Supplementary Figure 1) of the Catalina front range. Systematic statistical methods for accuracy assessment of the map have not been performed yet due to time and budget constraints. Visual comparisons of the map with previous helicopter-based surveys conducted by the USFS in 2008 and 2016 indicated dramatic improvement in accuracy and precision (Supplementary Figure 2). From a statistical perspective, in 2008, 210 polygons were mapped covering 787.25 acres (an average of 3.75 acres). In 2016, a largely disjoint set of 206 polygons were mapped covering 906.02 acres (an average of 4.4 acres per polygon). In contrast, this hand-digitized effort mapped 162 acres with 2068 polygons, an average of 0.08 acres per polygon.
Quantification of Species Responses to Fire
Saguaros
To understand the impact of fire on saguaro mortality in the Catalina Mountains, sampling plots were established in the footprint of the Mercer Fire. On August 22, 2019, a lightning strike ignited and burned 0.1 km2 of a south-facing slope of Arizona Upland Sonoran Desertscrub heavily infested with buffelgrass before running out of fuel (buffelgrass) and dying out. Ten sampling plots 20 m × 20 m in size in the fire area were established in November 2020, and five plots of the same size in the adjacent non-burned area (Figure 3). The four corners of each plot were permanently marked with rebar.
Any standing saguaros within a plot were labeled with a uniquely numbered aluminum tag. For each saguaro within a plot, we recorded whether it was dead or alive. A saguaro was considered dead if it had fallen or was completely devoid of chlorophyll (green photosynthetic skin). We measured each saguaro’s height, the range of skin (epidermis) injured by the fire, the number of established arms, the number of developing arms (nubs), and the phenology of the cactus (experiencing active growth, flowering, bearing fruits, or bearing remains of flowers or fruits). Fire-caused skin injury includes charred skin, which usually appeared at the base of a burned saguaro, as well as burn scars with a light tan color devoid of chlorophyll. Active growth of a saguaro was indicated by the presence of white, soft spines on the newest areoles of the cactus.
Data were analyzed using a linear mixed model to determine any linear relationship between the height of a saguaro and the amount of plant tissue damaged (percent of skin burned) by the Mercer Fire. Individual plots were treated as a random effect in the model and only a random intercept was evaluated. The regression was performed using the lmer function in the “lme4” package in the R environment (Bates et al., 2015). We compared the log-likelihood of this model with a null model with the same random effect to determine the significance of this relationship.
We predicted the future mortality of each saguaro based on the amount of burn damage per plant through reconstructing the relationship between mortality and burned area following Esque et al. (2004). In that study, the authors acquired a nonlinear relationship between the fire scorch range recorded one month after the 1994 Mother’s Day Fire in Saguaro National Park and the mortality of saguaros six years after the fire. We estimated the approximate values of both variables from their published figure and fitted them with a general additive model. We then used this model to predict the future mortality of saguaros surveyed at the Mercer Fire site.
Vegetation Plots
To understand the impact of the Bighorn Fire on desert vegetation and future recovery and community composition, twenty permanent 15 m × 15 m vegetation monitoring plots were established at Catalina State Park, Tucson, Arizona (Figure 3). These were divided equally between two vegetation types: Arizona Upland Sonoran Desertscrub, and Semi-desert Grassland. Within each vegetation type, five plots were established in an area burned in the Bighorn Fire, and five control plots were established outside of the burn area.
Within each plot, species richness and abundance of perennial species were recorded. Individuals were categorized as dead or alive, and in the burned plots, we counted the number of living individuals with post-fire regrowth or resprouting. For annual species, presence was recorded, but abundance and regrowth status were not. Cover estimates for each species were obtained along three parallel, 15 m long transect-intercept lines within each plot. Dead and living tissue were recorded separately, and in the burned plots, living tissue was categorized as either post-fire regrowth or unburned living tissue. Dried winter annuals from the previous season were not identifiable to species and thus were grouped. Likewise, all perennial grass species (native and non-native) in both the burned and unburned treatments could not be confidently identified to species and were labeled to “perennial grass” for most analyses. Data were taken in November, due to extremely low summer monsoon precipitation of 2020, sprouting and growth of live plants was greatly reduced.
State-and-Transition Fire-Spread Models
We used existing datasets, models, and tools, as well as recent research from the area, in a state-and-transition modeling approach to produce the most accurate set of potential outcomes from a large, complex wildfire in the short time frame that would be most useful to managers. Spatially explicit state-and-transition simulation models (STSM) can simulate landscape dynamics to evaluate complex and often uncertain future conditions (Daniel et al., 2016), including forecasting plant invasions (Frid and Wilmshurst, 2009; Frid et al., 2013; Grechi et al., 2014; Jarnevich et al., 2015). These models divide a landscape into discrete spatial units (cells) that are assigned a discrete state. While STSM can simulate some fire dynamics, these are based on parameterization from historical fires. We have dynamically linked an STSM to a fire model based on physical characteristics to overcome knowledge limitations that would otherwise prevent parameterization of the novel invasive species-driven fires (Jarnevich et al., 2019). As the simulation runs, changes to states over discrete time steps are tracked by the model and these states are passed to the fire model that then simulates fire on the landscape. The fire model provides information on which cells burned, indicating where fire transition should occur in the STSM. Transitions between states can be probabilistic or time-based and are specific to transition type (e.g., growth, treatment, disturbance, etc.). The STSM model used for this study was adapted from a STSM model developed for nearby Saguaro National Park (Supplementary Figure 3; Jarnevich et al., 2019, 2020). We merged this model with components of an earlier simulation for the Catalina Mountain study area (Supplementary Figure 4; 14,216 km2).
The model divides the landscape into seven states: uninvaded, buffelgrass seedbank, and one of five buffelgrass cover class levels [<1%, 1–10%, 11–0%, >50%, converted (>50% cover that has burned)] and allows movement between states including dispersal, establishment, patch infilling (increase in buffelgrass cover class), and fire. The uninvaded states are further split into fuel models. To implement the simulations, we used the stsim base package (version 3.1.21) with the stsim-farsite add-on package (version 3.1.21) within the SyncroSim version 2.0.41 software. The stsim-farsite package integrates the FARSITE fire area simulator software version 4.1.055 into ST-Sim (Finney, 2004).
A LANDFIRE fuel model classification of the study area (Landfire, 2020) defined fuel model classes across the landscape to simulate wildfire. Increasing numbers of fuel models added to the complexity of the STSM and thus to computational requirements. Fuel models with very small pixel counts were combined with other similar fuel model classes, and we noted the potential differences caused by the changes (Supplementary Table 1). We also modified fuel models for northern aspects (NW-N-NE) for three random timesteps to reflect the frequency of years preceded by two “wet” winters, which create an increase in annual grass fuel loads on these aspects (see Rogers and Vint, 1987). These fuel models formed the basis for scenarios that did not include buffelgrass on the landscape.
For buffelgrass scenarios, additional inputs to simulations included the initial distribution of buffelgrass in the front range of the Catalinas (from section “Mapping buffelgrass infestation”), and a previously developed habitat suitability map for buffelgrass classifying the landscape into areas susceptible or unsusceptible to buffelgrass invasion (Frid et al., 2013). For cells with buffelgrass, the fuel model was defined based on fuel levels for buffelgrass invaded areas of different cover amounts following Jarnevich et al. (2019). Previous work defined 10% buffelgrass cover as required to carry a fire (or 0.5 ton/acre of fuel, estimated based on McDonald and McPherson, 2013). Thus, all landscape states could be cross walked to a fuel model. Transition parameter values followed Saguaro National Park simulations with patch infill transitions to a higher buffelgrass cover class state based on the moderate rates of patch infill and its interaction with precipitation (Jarnevich et al., 2019, 2020).
For the FARSITE parameters (Supplementary Table 2), we incorporated most of the values used by fire modelers contemporaneously working on the Bighorn Fire in the Wildland Fire Decision Support System (Noonan-Wright et al., 2011). Because their fire duration value was based on estimates related to operational questions rather than ecological conditions, we used the mean fire duration of historic wildfires in adjacent Saguaro National Park to identify the fire duration. This value of 45 days better matched the period of active fire growth of the Bighorn Fire, and simulation results without buffelgrass matched the historic fire size frequency of the Santa Catalina Mountains. Fire history for the study area (1932–2009) shows 8.6 fires over 100 ha in size over 30 years; more recent data (2000–2019) calculates to 12 per 30 years. We also modified the fire season start and end days based on expert opinion from local fire ecologists/wildland fuels specialists. This process used established data, models and tools supplemented by current research and expert opinion to inform the most accurate potential outcomes in a rapid time frame.
Surveying Resident Perceptions
To understand the impact of the fire on area residents’ perceptions of buffelgrass as a fire risk, we surveyed a sample of Pima county residents, randomly selected but weighted to ensure representation of people living in the Catalina foothills area near the Bighorn Fire. The sample was collected via Qualtrics, a third-party marketing firm. The survey was answered by 2,147 respondents, which resulted in a final sample of 550 complete responses, pseudo response rate of 25.62%. The final sample excluded 1,113 respondents who either overfilled demographic quotas (14.11%) or did not reside in our sample area (37.73%). Other respondents were also excluded due to failed attention checks (3.03%), speeding (3.35%), or not fully completing the questionnaire (16.16%). Data collection took place in January and February of 2021. Respondents were asked whether they had heard of buffelgrass before (yes/no/maybe), and if so to rate their awareness (Likert scale from 1–10) of several buffelgrass threats (i.e., increased risk of fire, loss of saguaro cactus, and declining property values). The survey also asked whether respondents had been affected by the fire, with response options that included evacuation; evacuation of family or friends; and loss of recreational access to the Catalina Mountains. The questionnaire featured additional questions regarding a choice experiment of a hypothetical bond package for invasive species control not covered here that are the focus of ongoing research.
Results
Fire Behavior
The Bighorn Fire was ignited on a north slope below Bighorn Peak at about 1,200 masl among native Semi-desert Grassland species including sotol (Dasilyrion wheeleri), beargrass (Nolina microcarpa), bullgrass (Muhlenbergia sp.), buckwheat (Eriogonum wrightii), rosewood (Vaquelinia californica), and occasional mulberry (Morus micropylla) and scrub oak (Quercus turbinella). All were observed to be resprouting post-fire.
The fire backed down a steep north-facing slope into vegetation transitional to the Arizona Upland of the Sonoran Desert. Species in this area are mostly sotol, ocotillo (Fouquieria splendens), mesquite (Prosopis velutina), club moss (Selaginella spp.), prickly pear (Opuntia spp.), and hopbush (Dodonaea viscosa). Bunchgrasses were common, including native bush muhly (Muhlenbergia porteri), sideoats grama (Bouteloua curtipendula), purple three-awn (Aristida purpurea) and spider grass (A. ternipes) as well as non-native weeping lovegrass (Eragrostis curvula) and Lehmann’s lovegrass. The primary fuels, however, were expired winter annuals, especially native mustards (Brassicaceae), forget-me-nots (Boraginaceae), Daucus, Eriastrum, Silene, Plantago, and six-weeks fescue (Vulpia octoflora). Prominent non-native annuals included red brome.
At 975 masl near the ignition point, the fire died out where the north slope lessened below a 20% incline, and foothill palo verde, brittlebush (Encelia farinosa), and saguaro became prominent. An air drop of fire retardant nearby may have played a role as well (Figure 3B).
One kilometer west at similar elevation, the fire reached a ridge with a warmer southwest exposure and a 0.04 km2 patch of buffelgrass mixed with typical Arizona Upland vegetation, including palo verde, brittlebush, saguaro, canyon ragweed (Ambrosia ambrosioides), limber bush (Jatropha cardiophylla), and desert lavender (Hyptis emoryi). Another non-native bunchgrass, fountain grass, was also common. A fire crew had expertly cut a fuel break along the ridgeline, keeping the fire out of most of the Cenchrus (fountain and buffelgrass) and saguaros. On the burned side of the fire break, heat from the burning Cenchrus scorched and/or killed the native palo verde, brittlebush and saguaro, of which the brittle bush and saguaro do not resprout, and the palo verde resprouts weakly. These three species, emblematic of Arizona Upland Sonoran Desert, were burned more severely by the Cenchrus as compared to those places where the fire was fueled by winter annuals, due to difference in fuel loading (see McDonald and McPherson, 2013).
On the south side of the Catalinas our surveys revealed a similar suite of winter annual plants fed by rains during the winter of 2019–2020 providing continuous fine fuels on the more mesic north aspects in desert areas. The fire followed these fuels and those in the Semi-desert Grassland downhill in the southwest corner of the fire (Figure 3). At the upper elevations of desert habitats and in desert grassland areas, extensive shindaggers (Agave schottii) and Lehmann’s lovegrass provided continuous fuels bridging these ecosystems with the Oak Woodland and Chaparral.
Further downslope, and on the more xeric sides of ridges (south-facing slopes), winter annuals were less abundant among the palo verde, saguaro, brittlebush, and baby bonnets (Coursetia glandulosa) of the Arizona Upland. This ecosystem mostly escaped the flames on the south side of the Catalinas, except where the fire was carried by patches of buffelgrass and fountain grass at densities greater than 50% cover. Such patches were generally small and discontinuous along rocky hillsides, limiting the fire’s extent into Desertscrub. Large patches of buffelgrass (as mapped in 2018) were close to the Bighorn Fire perimeter (Figure 3), but the flames were backing downslope and died before reaching these patches from a lack of continuous fuels and presumably unfavorable winds at night.
Buffelgrass Infestation
Within the front range of the Catalinas we mapped 6,485 patches of buffelgrass totaling 4.43 km2, spanning an elevation range of 839 to 1,476 masl as of 2018. At the interface of desert and grassland the distribution of buffelgrass is best characterized as a mosaic of small, isolated patches. Moving downslope, these patches grow in size and coalesce into stands of buffelgrass that cover entire slopes (Figure 4). In addition, the density of buffelgrass within patches increases as elevation decreases. The distribution of buffelgrass in relation to aspect exhibits a similar pattern, with south- and southwest-facing aspects favoring the growth of larger, denser patches of buffelgrass than north- and northeast-facing aspects, which can often be entirely free of buffelgrass, at present. Most (94.6%) of the buffelgrass was found on south-facing slopes with slightly higher (50.6%) on southwest- than southeast-facing slopes (44.1%). The distribution of patch sizes was highly skewed, with just the largest seven patches comprising 25.5% of all digitized buffelgrass (Supplementary Table 3). The largest patch was 0.34 km2 (7.8% of all buffelgrass) and a total of 129 discrete patches were at least one acre in size. The distribution of buffelgrass along the elevational profile indicates that the most heavily invaded area is upslope from the WUI and downslope from the ecotone between the Arizona Upland and the higher elevation desert grassland (Figure 4).
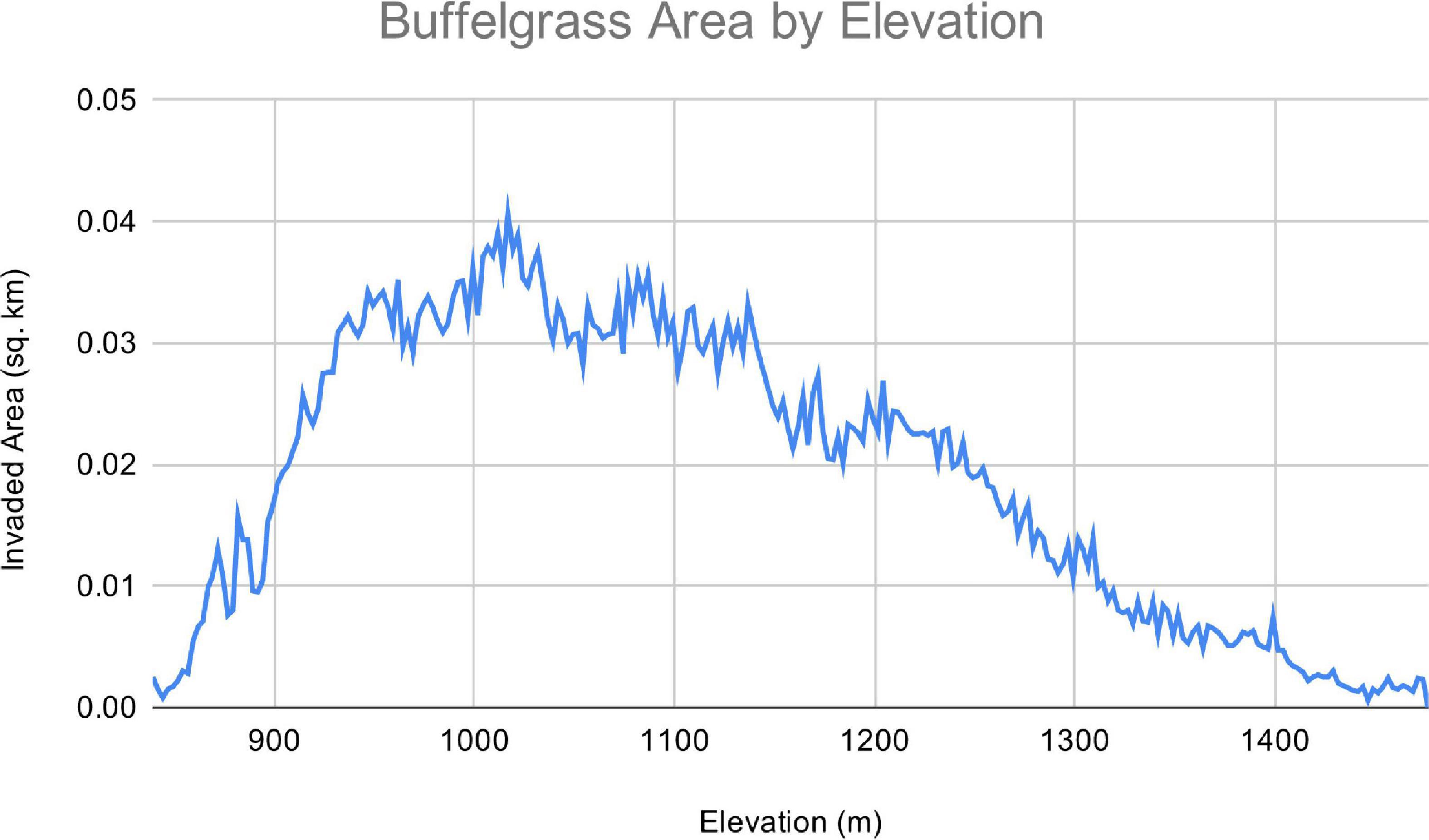
Figure 4. Buffelgrass coverage by elevation. Note the elevation with most buffelgrass coverage is above the wildland-urban interface (900–950 masl), but below the Desertscrub/Semi-desert Grassland ecotone (1,200 masl).
Species Responses to the Fire
Saguaros
We recorded 95 saguaros in the ten plots in the fire zone and 40 saguaros in the five control plots. About 94% of the saguaros in the fire zone had some fire injury; 41% had greater than 50% of the skin surface injured by the fire; and 29% had fire injury covering greater than 80% of their surface (Figure 5). The overall first-year mortality of saguaros sampled in the fire zone was 18% (17 of the 95 individuals), the majority of which are young plants. Fifteen of the seventeen dead saguaros had a height less than 2 meters. There was strong spatial variation in saguaro mortality within the fire zone. The plot mean mortality ranged from zero in four of the plots to 80% in one plot (Supplementary Figure 5). The percent of skin damaged by the fire had a significant negative relationship with the height of a saguaro ( = 35.65, p = 2.36e-09; Figure 5).
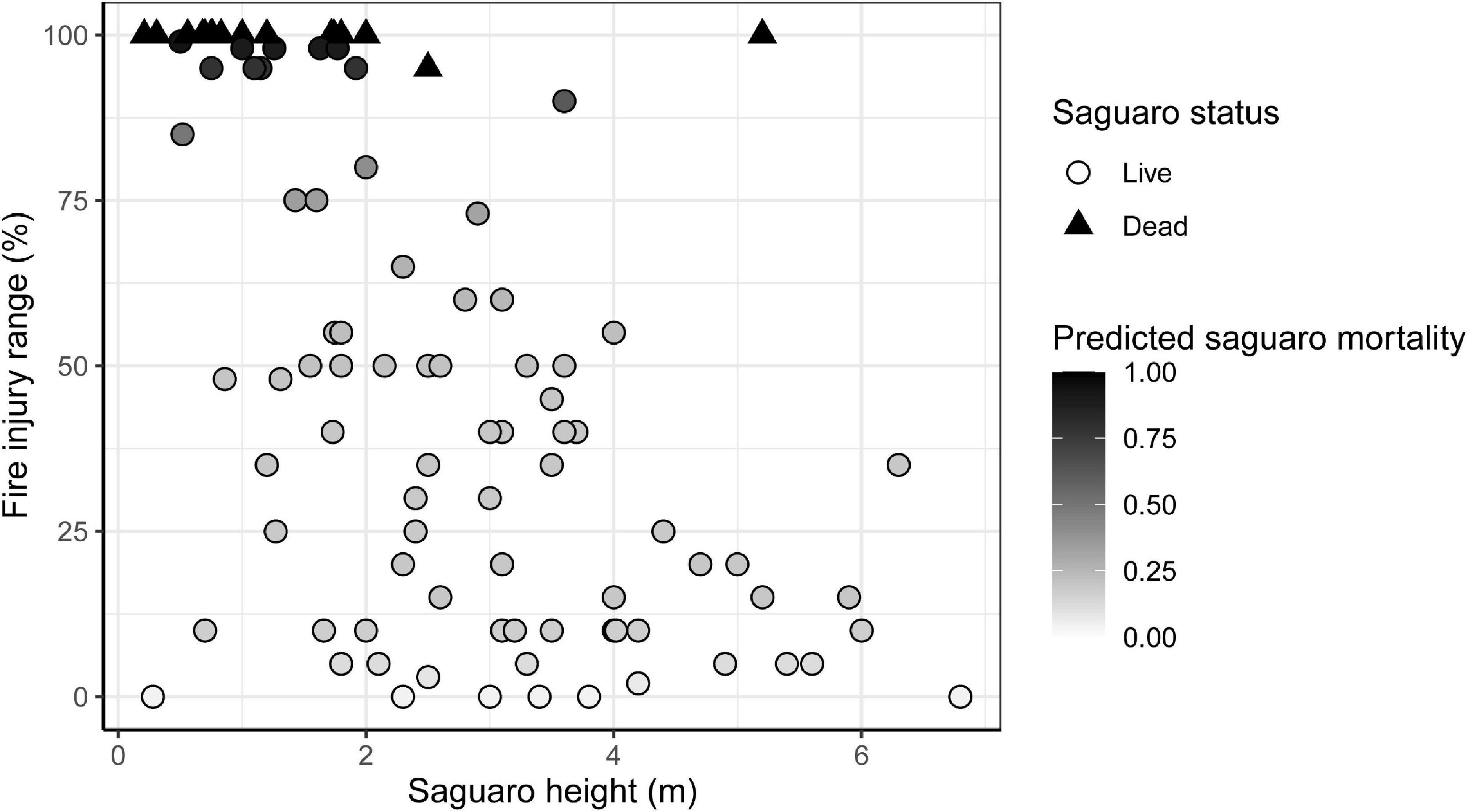
Figure 5. Impacts of fire on saguaros (Carnegiea gigantea). The relationship between individual saguaro height and the range of injury by the Mercer Fire. Dead saguaros are shown in black triangles, live saguaros are shown in circles with the grayscale gradient indicating future mortality probability of each saguaro based on the range of fire injury.
Based on the results from Esque et al. (2004), who predicted saguaro mortality six years after the fire, our one-year post-fire results show a two-step nonlinear relationship with the range of fire injury occurring on plant surface (Figure 6). Conservatively, a 50 percent chance of death is associated with approximately 85% range of fire injury. Based on this prediction, 36% of the live saguaros surveyed in the Mercer Fire zone will have a 50 percent or greater chance of dying in the future. It should be noted that high humidity during the Mercer Fire raised dead fuel moisture and dampened fire behavior, compared to fire behavior surrounding the saguaros that we examined in the Bighorn Fire.
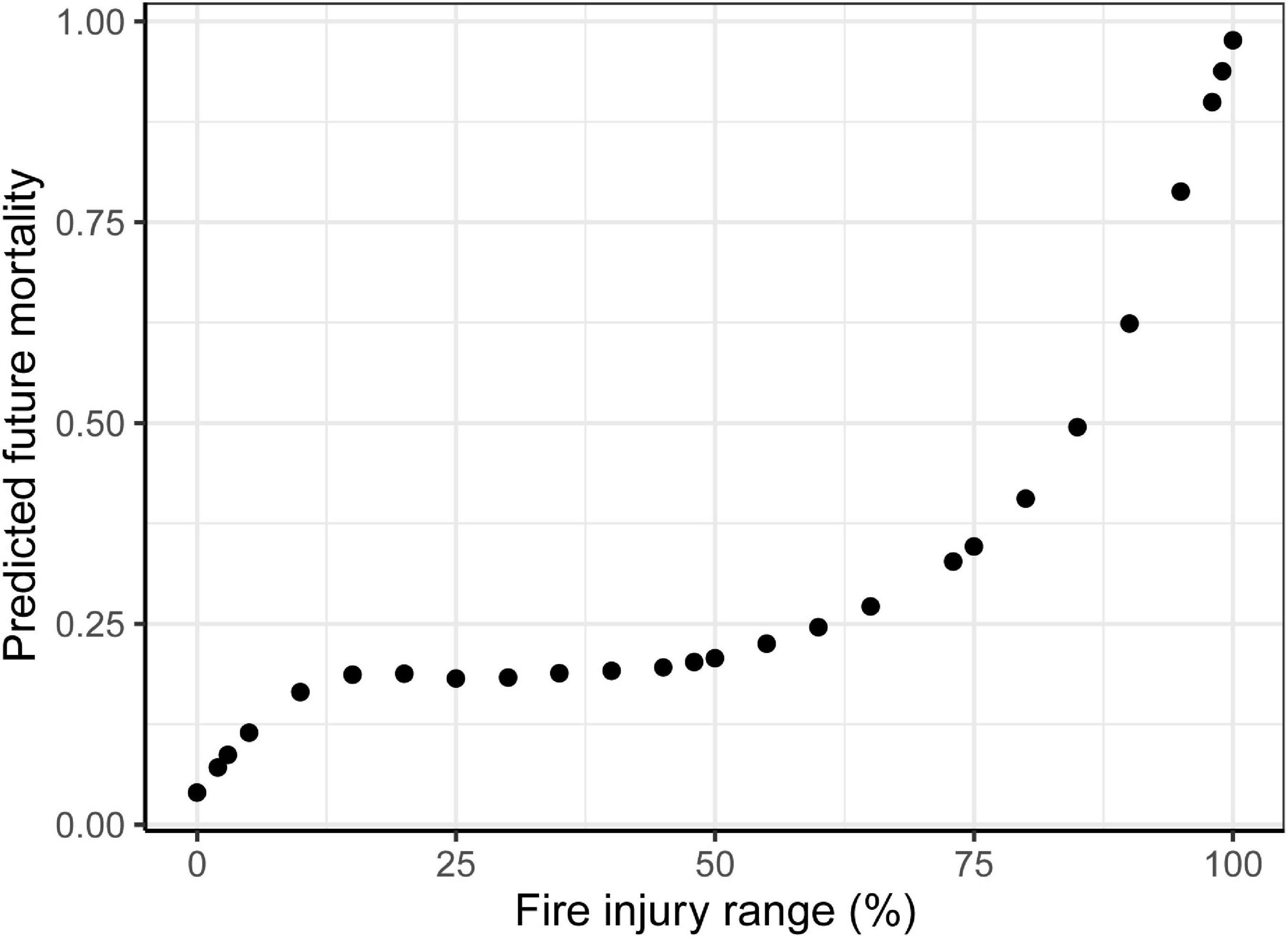
Figure 6. Predicted mortality of saguaros (Carnegiea gigantea) post-fire. The range of fire injury of saguaros surveyed in the Mercer Fire site gives the prediction of their future mortality. The prediction is based on a relationship presented Esque et al. (2004).
Vegetation Plots
At the initial post-burn time step, species richness was significantly lower in burned plots than in the control plots in both the Arizona Upland Sonoran Desertscrub (p = 0.0447) and the Semi-desert Grassland (p = 0.0063; Figure 7). Likewise, vegetation cover is much less in burned areas than unburned areas immediately after the fire (Figure 8), and the majority of the remaining cover in burned areas is from dead plants.
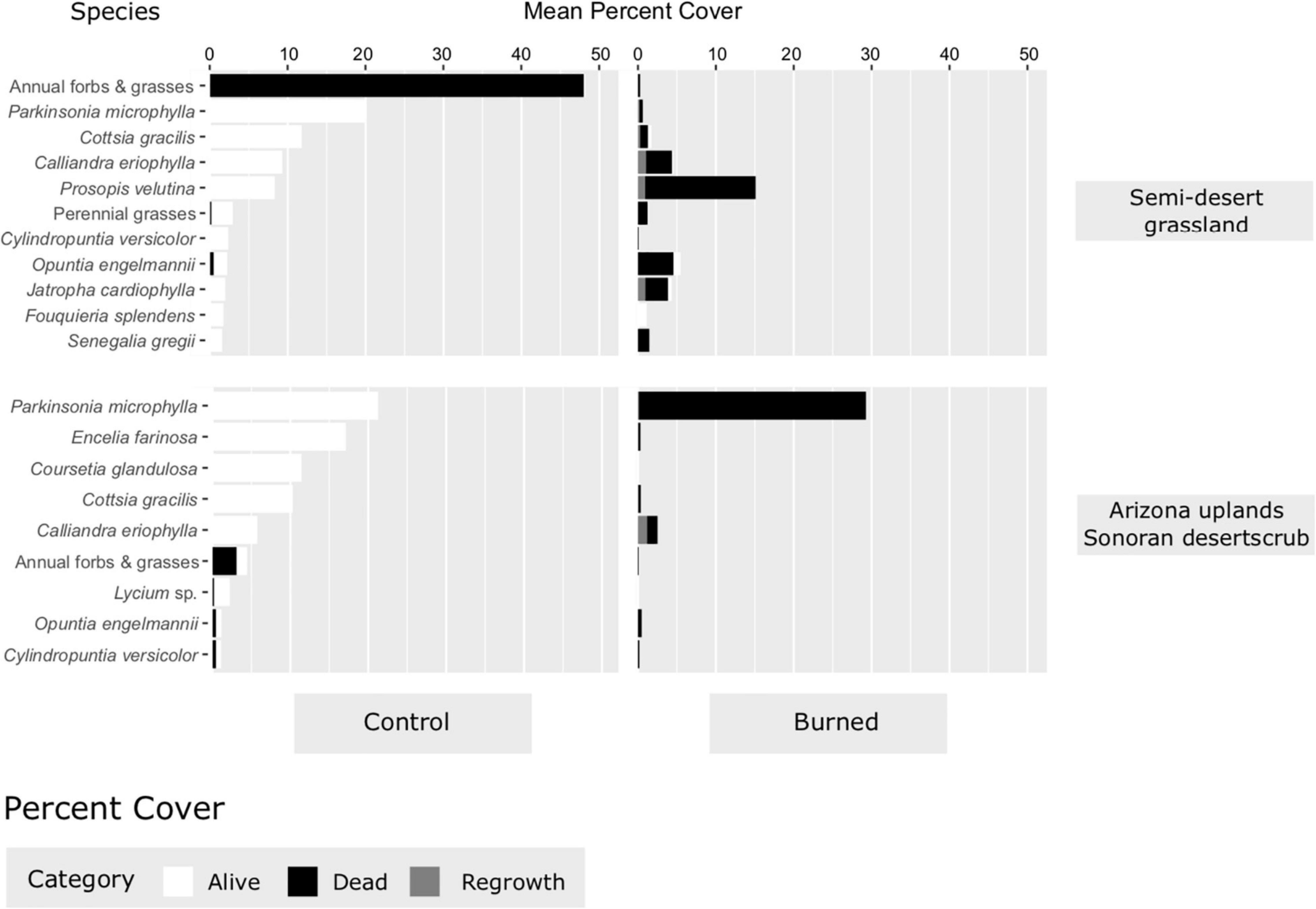
Figure 8. Percent cover of the most prominent species in burned and control plots, Catalina State Park, Tucson, Arizona.
The cover of plants observed in control plots provides some indication of the fuels present that carried the Bighorn Fire in this area. The control plots in the Semi-desert Grassland had high (approximately 50%) cover of dead annual plants, mostly from the 2019–2020 winter-spring growing season. Predominant annuals were the natives Aristida adscensionis, Cryptantha sp., Daucus pusillus, Lepidum sp., Plantago patagonica, Silene antirrhina, Stylocline micropoides, and Vulpia sp., and the non-native red brome, with all the natives roughly equaling the red brome biomass. Remnant winter annuals were much less dense in the Arizona Upland Sonoran Desertscrub, with less than 5% total cover in that vegetation type.
Most perennial species observed in the plots exhibited at least some regrowth four months post-fire (Figure 9). Many of the most common perennial desert species, including fairy-duster (Calliandra eriophylla), limberbush, slender janusia (Cottsia gracilis), and littleleaf ratany (Krameria erecta), all had high rates of survival and regrowth with over 75% of living individuals showing post-fire regrowth. Ocotillo had an initial survival rate of over 90% in both vegetation types, but few individuals had visible post-fire regrowth. However, some larger shrubs and trees appear to have survived at lower rates. Foothill palo verde, which commonly grows as a short-statured tree in Arizona Uplands Sonoran Desertscrub, suffered 68% mortality in that vegetation type, and 80% in the Semi-desert Grassland where it is also present but far less common. Resprouting from the base was observed in this species. Similarly, velvet mesquite, which is more common in the Semi-desert Grassland, suffered approximately 50% top-kill across both vegetation types, again with some individuals resprouting from the base. Hopbush, a large shrub present in both vegetation types, suffered near total mortality with only one surviving individual in the burned Semi-desert Grassland plots. Cacti generally suffered extremely high mortality, with only a few individuals of Engelmann prickly pear (Opuntia engelmannii), Graham’s fishhook cactus (Mammillaria grahamii), hedgehog cactus (Echinocereus spp.), and Christmas cholla (Cylindropuntia leptocaulis) surviving. Notably, no saguaros survived in the burned Arizona Upland Sonoran Desertscrub plots.
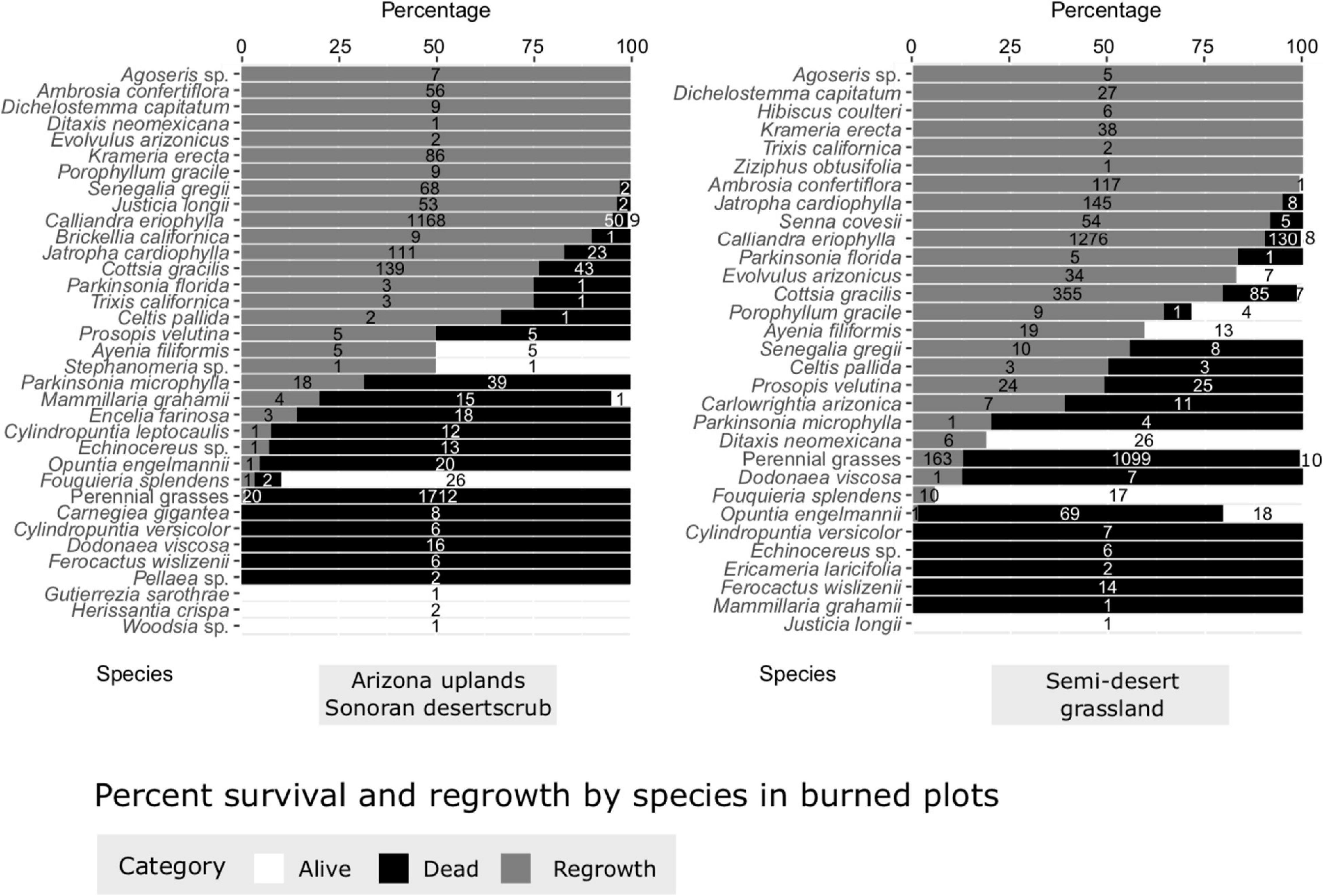
Figure 9. Percent survival and regrowth of species in burned plots, Catalina State Park, Tucson, Arizona.
Perennial grasses appeared to suffer high initial mortality due to the fire in both vegetation types, but the observed absence of regrowth is likely due to the abnormal lack of summer 2020 monsoon rains, with potential for regrowth still possible. The Semi-desert Grassland had generally low coverage of perennial grasses (Figure 8), which included the natives purple three-awn, spider grass, sideoats grama, slender grama (Bouteloua repens), tanglehead (Heteropogon contortus), and bush muhly, and less frequently Lehmann’s lovegrass and no buffelgrass. In the Arizona Uplands Sonoran Desertscrub, perennial grasses had a negligible contribution to vegetation cover, though a high number were observed in the burned plots (Figure 9). It is possible that invasive grasses were present in the burned Sonoran Desertscrub and not in the control area. Future monitoring of these long-term plots will address this uncertainty.
State-and-Transition Fire-Spread Models
The simulation began with 4.4 km2 of buffelgrass produced by the mapping effort (see Sections “Mapping buffelgrass infestation” and “Buffelgrass infestation”) and after 30 years resulted in an average of 49.2 km2 invaded by buffelgrass (range 42.9–55.2 km2; sd 3.34). Buffelgrass patches with >50% cover that burned in simulations resulted in an average of 23 km2 (range 1.1–41.8 km2; sd 11) of the landscape converted to a state where the majority of native vegetation is not expected to persist at the end of 30 years, although this was highly stochastic. With the presence of buffelgrass, the study area is likely to experience larger fires with greater area burned over the 30-year period (Figure 10). This impact is evident as more continuous buffelgrass is present on the landscape, with a noticeable increase in fires after 20 years, ca. 2040 (Figure 10). The simulations also showed a greater annual burned area below 1,200 masl in elevation in the scenario with buffelgrass (Figure 10).
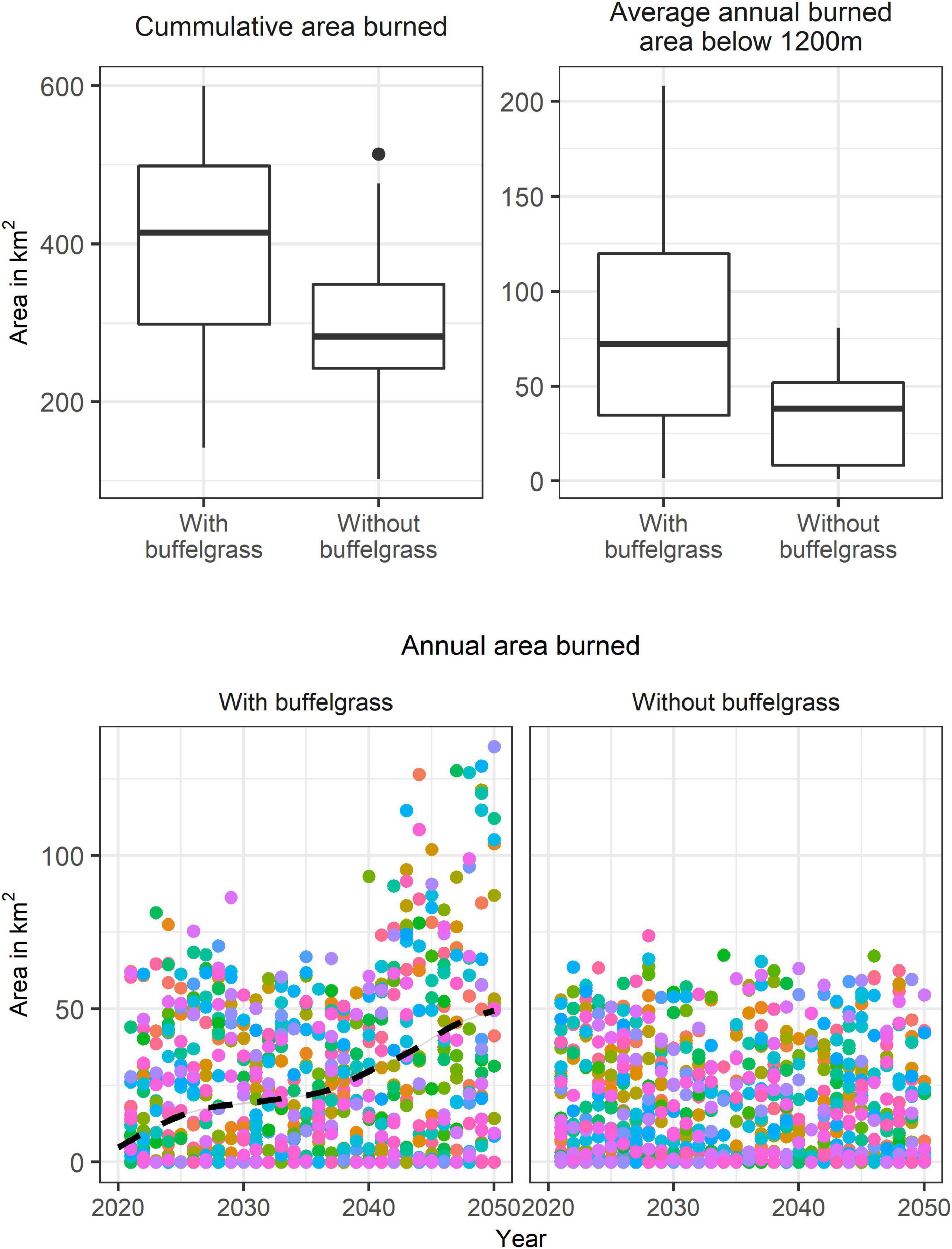
Figure 10. State-and-transition fire-spread models. Box plots and time series of 50 Monte Carlo simulations across a 30-year time horizon simulating fire for two scenarios, with and without buffelgrass spreading across the landscape. Box plots include average annual burned area below 1,200 masl and cumulative burned area over the 30-year period. Time series (bottom panels) are annual area burned colored by different Monte Carlo simulations for each simulation year for the two scenarios with the black line representing the average annual area invaded by buffelgrass over the course of the simulation.
Without mitigation, buffelgrass can be expected to continue to spread and increase in abundance. The presence and spread of buffelgrass in the Catalina Mountains will result in larger fires, more area burned resulting in the loss of native species (as a result of the converted area) and more fires burning into the desert ecosystem and toward residential housing at the WUI.
Resident Perceptions
Of the 550 survey respondents, 36 were evacuated during the Bighorn Fire, 109 had friends or family members who were evacuated, and 47 reported loss of recreation access due to the fire. In the full sample, 53% of respondents reported that they had heard of buffelgrass. Awareness of buffelgrass was significantly higher among those evacuated (p = 0.0080) and those who lost recreation access (p = 0.0023), but was not significantly different among those whose family or friends were evacuated (p = 0.5728).
When asked to rank their awareness of buffelgrass-related threats on a scale from 1 to 10, respondents reported average awareness of 4.63 for fire risk, 3.05 for saguaro loss, and 2.78 for declining property values. Evacuated respondents reported significantly higher awareness of fire risk (p = 0.0228), saguaro loss (p = 0.0001), and declining property values (p = 0.0001). Those who lost recreation access reported significantly higher awareness of fire risk (p = 0.0028) and saguaro loss (p = 0.0279) but reported no greater awareness of property loss (p = 0.1089). Awareness of threats was not significantly different among those whose family or friends were evacuated.
Discussion
The Bighorn Fire occurred at what is perhaps an inflection point in time. The fire started at an elevation just above the desert, ca. 1,200 masl, and it generally did not drop below this elevation into the desert lowlands, especially on more arid south-facing slopes (Figure 3B). The role of invasive grasses in carrying the fire, including buffelgrass, fountain grass, and Lehmann’s lovegrass was limited by their patchy distribution at the Desertscrub/Semi-desert Grassland ecotone at 1,200 masl. The Bighorn Fire moved downslope at night, with limited energy release. This allowed bare ground in desertscrub, hiking or game trails, and rock outcrops, to serve as fuel breaks, preventing further spread downslope. As shown by our state-and-transition models, rapid and continued spread and coalescence of buffelgrass patches will increasingly create a buildup of fuels and link the Desertscrub and Semi-desert Grassland communities (Figure 10). Even with the current densities, a lightning strike or human ignition further downslope, as with the 2020 Bush Fire in Central Arizona, could have ignited a fire in Sonoran Desertscrub much larger than the 2019 Mercer Fire. In many respects, the Bighorn Fire was a near miss for the Catalina Foothills WUI.
Slope exposure strongly influenced the behavior of the Bighorn Fire at lower elevations. While the south-facing slopes were largely untouched, substantial areas of north-facing slopes and north-trending piedmonts below 1,200 masl were burned due to a higher fuel load of dried winter annuals (Figure 3B, top half of figure). On these north-facing slopes, the Bighorn Fire did spread within saguaro-palo verde habitat. The Desertscrub/Semi-desert Grassland ecotone receives more winter rainfall and has experienced an increase in grass coverage (native and non-native; Turner et al., 2003) with reduced grazing pressure. These conditions are somewhat analogous to other productive desert shrubland areas that sustained large fires in 2005 and 2020 such as central Arizona (Cave Creek and Bush Fires) and the eastern California (2005 Hackberry and 2020 Dome Fires). El Niño rain events provide pulses of moisture and nutrients that lead to a profusion of annual plant growth, both native and non-native, that dry out in arid foresummer and meet the minimum fuel loads (∼100 g/m2) required to drive “grassland fire” (Scott and Burgan, 2005). Increase in the freeze-free season, increased frequency of wet winters, and an earlier onset and lengthening of the fire season are predicted to further the fire-invasive feedback loop in the western United States (Abatzoglou and Kolden, 2011).
In the Bighorn Fire, fire spread across the Desertscrub/Semi-desert Grassland ecotone was influenced by densities of both winter and perennial annual grasses interacting with landscape structure, including elevation and slope exposure, degree, roughness, and the occurrence of animal and human trails, which acted as minor fuel breaks. In some ways, this parallels the way that forest fires percolate with landscape structure higher up in the Santa Catalina Mountains (Iniguez et al., 2008). In Arizona Upland habitat, mechanical and chemical treatment options to control invasive grasses, and other management decisions (e.g., placement of new hiking trails) to prevent cross-biome fire spread could be prioritized according to landscape structure, phenology of the invasive grass (and uptake of herbicides), and predicted fire behavior (Bean, 2014; Thistle et al., 2014). This prioritization could also apply to the air drop of flame retardant by fixed wing aircraft during wildfires, as well as to the pinpoint application of herbicides from rotary wing aircraft, in the steep and inaccessible terrain of the Catalina piedmont (Bean, 2014; Thistle et al., 2014).
Despite evidence from other post-fire studies in the Sonoran Desert (Cave, 1982; McLaughlin and Bowers, 1982; Cave and Patten, 1984; Rogers, 1986; Loftin, 1987; Wilson et al., 1995; Alford et al., 2005; Shryock et al., 2015), we were surprised by the high number of desert species with resprouting capacity (Figure 9). This result begs the question of the adaptive origin of this behavior and if there is a fire history within the desert as suggested by some authors (e.g., Brown and Minnich, 1986). Much of the flora of the semi-tropical Sonoran Desert is evolved from thornscrub or dry forest environments (Axelrod, 1979). In the tropics and tropical dry forests, resprouting is a common and persistent strategy (Poorter et al., 2010), where fire is rare to absent, and is often in response to stressors such as hurricanes (Paz et al., 2018) and freezes (Bojoìrquez et al., 2019). It has also been indicated, but less clearly, that resprouting is common in areas with low site productivity such as arid environments as a possible response to drought (Vesk and Westoby, 2004). It seems that, rather than being an adaptation to fire itself in these desert species, resprouting is a response to variable causes of stress damage. The degree of resilience offered by resprouting capacity is unclear and likely not robust after repeated burns. In burned areas in the Mojave Desert, community composition remains stable in creosote (Larrea tridentata) dominated communities post-fire (Engle and Abella, 2011), though there is a marked decrease in structure and keystone species (Abella et al., 2009; Engle and Abella, 2011). It will be critical to monitor and track the long-term recovery and trajectory of community composition in multiple burned sites throughout the desert lowlands impacted by fire.
Conceptual state-and-transition models have been used to evaluate ecosystem changes applicable to a broad swath of landscape types (e.g., Cobb et al., 2017), and fire simulations have predicted vegetation changes (e.g., Conlisk et al., 2015). There are also many examples of invasive species spread models to determine their potential impact on fire risk, but without simulating fire (Wang et al., 2016). There are few examples, however, that combine simulations of the spread of invasives and associated wildfires. The types of simulations we used couple a state-and-transition model that simulates both the spread and increase in abundance of an invasive plant with a fire behavior model that allows exploration of the consequences of a non-native species that introduces non-analog conditions (Supplementary Figure 3; Jarnevich et al., 2019). These combined models allow for the co-production of simulations by scientists, land managers, policy makers and the interested public; the consideration of additional drivers (e.g., climate variability and change); and the incorporation of alternative management actions and their timing across multiple jurisdictions (e.g., Miller et al., 2017). For land managers who are facing unprecedented changes in wildland fuel complexes at an exponential rate, these products provide some basis for weighing options. Actions delayed in such a situation greatly increase future costs and decrease the odds of achieving management goals, increasing the likelihood of a cascading series of undesirable events.
Southern and central Arizona, where buffelgrass is spreading rapidly, encompass two large metropolitan areas (Tucson and Phoenix), two major universities, a vibrant multi-billion-dollar tourism industry reliant on a unique ecological backdrop, and expansive public lands – from world-renowned national parks and monuments to military facilities vital to national defense. During the past two decades, a cross-jurisdictional effort was mounted to identify, communicate, and address the ecological and socioeconomic risks posed by buffelgrass invasion, with mixed success (Brenner and Franklin, 2017; Lien et al., 2021). Despite this sustained and coordinated public messaging campaign, local and regional actions continue to fall short of controlling the expanding front of invasive grass species in the region.
The survey results presented here are in general agreement with a 2017 survey of Homeowner Association (HOA) members in Tucson (Plecki et al., 2021). Most 2017 survey respondents were aware of buffelgrass, but their knowledge of mitigation strategies were limited. Likewise, our results suggest that while a majority of residents know about buffelgrass, many have limited awareness of its impacts on fire, desert plants, and property values. Awareness of these impacts is significantly higher among those who were personally affected by the Bighorn Fire. This suggests that over time, shifting fire regimes will increase residents’ awareness of buffelgrass and demand for buffelgrass mitigation. However, the ability to respond to and manage the altered ecological state of recurring fires will likely be insufficient to mitigate the economic and biodiversity fallout without prompt action.
Conclusion
Timely case studies of extreme ecological events like the Bighorn Fire are essential, not only to understand causes and project consequences in the short and the long term, but also to inform management and policy responses and galvanize public attention. We are already witnessing a dramatic shift in the fire regimes of North American deserts, the scope of which is amplifying and increasingly impacting ecosystem processes and the WUI at the landscape-level. Real time assessment of these fire events and their aftermaths using empirical, modeling, and multidisciplinary approaches can lead to a refined understanding of the driving mechanisms of shifting states. Public attention and political willingness to act are likely to decay quickly after extreme events like the Bighorn Fire, and time is of the essence for the effective transfer of any actionable knowledge. As the pace of change accelerates, the continuous establishment of baselines and benchmarks will allow an iterative unpacking of novel states and conditions.
For example, increasing connectivity between desert valleys and the uplands is altering the relationship between hydroclimatic variability and annual area burned. In the southwestern United States, El Niño events are normally associated with more winter-spring precipitation and reduced fire occurrence in the uplands (Swetnam and Betancourt, 1990). By contrast, in central Arizona more frequent and severe fires in Sonoran Desert vegetation occur after abnormally wet winter/spring precipitation associated with both strong and weak El Niño events (1978–1979, 1987–1988, 1991–1995, 2004–2005, 2018–2019, and 2019–2020) and also during the positive phase of the Pacific Decadal Oscillation (1976–1995). In Tonto National Forest, annual area burned in Sonoran Desert vegetation was influenced by greater than normal precipitation in two and three consecutive winters (Rogers and Vint, 1987; Alford et al., 2005), which tend to happen during multi-year El Niño events. The copious winter rainfall initiated broadly synchronous blooms of red brome and other invasive winter annual grasses across the Sonoran (and Mojave) Desert, which then dried out and burned in the ensuing summer. The wide ignition fronts from wildfires driven by winter annual plants tend to travel upslope with convection and spread into forests at a time when the forests normally would not burn. By contrast, buffelgrass and other summer-flowering perennials can fuel wildfires and desert-forest connectivity in any given year, regardless of winter precipitation variability. The relationship between interannual and decadal climate variability and fire activity across all biomes could shift significantly in the near future.
A coalescing front of invasive perennial and annual grasses are creating increased connectivity between the desert and forests on mountain slopes that will likely drive heightened fire risk at the WUI in the coming decades. Future efforts aimed at better understanding the history of fire in the desert, the resiliency of desert species to fire, the association between fire and ENSO and other climatic events, social studies that address the perception of change, sustained mapping efforts, an assessment of the effectiveness of fuel breaks in limiting connectivity, and focused invasive species control will all provide an enhanced knowledge base and management actions to address this fast-evolving fire risk.
Dedication
We dedicate this paper in memory of our friend and colleague Travis M. Bean who passed away unexpectedly on May 27, 2020. Travis was instrumental in launching efforts to address and manage large scale ecosystem changes driven by invasive perennial grasses in desert environments. His commitment and joyful nature will be sorely missed.
Data Availability Statement
The raw data supporting the conclusions of this article will be made available by the authors, without undue reservation.
Author Contributions
BTW, CSJ, KAF, PG, KAH, JM, and JLB initiated the manuscript and conceptualized its components. AO undertook and led the buffelgrass mapping effort. BTW, JSB, KAF, PG, JM, YML, JAR, and MRS undertook field work and data analyses. EB, ASMGK, AML, AP, and JRS completed the survey. BTW and JLB led writing efforts, with contributions from all authors. All authors reviewed and edited a complete draft of the manuscript.
Funding
BTW and MRS were funded within the framework of the OHMi Pima County and funded by the LabEx DRIIHM, French programme “Investissements d’Avenir” (ANR-11-LABX-0010) which is managed by the ANR. EB, JLB, KAF, ASMGK, AML, AP, KAH, and JRS acknowledged the support from the National Science Foundation DEB-1924016 grant. Any use of trade, firm, or product names is for descriptive purposes only and does not imply endorsement by the U.S. Government.
Conflict of Interest
AO was employed by the company Planet Labs, Inc.
The remaining authors declare that the research was conducted in the absence of any commercial or financial relationships that could be construed as a potential conflict of interest.
The reviewer MG declared a shared affiliation, though no other collaboration, with several of the authors CSJ and JLB, to the handling Editor.
Publisher’s Note
All claims expressed in this article are solely those of the authors and do not necessarily represent those of their affiliated organizations, or those of the publisher, the editors and the reviewers. Any product that may be evaluated in this article, or claim that may be made by its manufacturer, is not guaranteed or endorsed by the publisher.
Acknowledgments
Field work was made possible via Catalina State Park permit #2020-0006 and a Coronado National Forest permit. Conversations with Alberto B rquez greatly enhanced the manuscript.
Supplementary Material
The Supplementary Material for this article can be found online at: https://www.frontiersin.org/articles/10.3389/fevo.2021.655561/full#supplementary-material
Supplementary Figure 1 | Areas visited in the Santa Catalina Mountains at the perimeter of the Bighorn Fire in the fall of 2020.
Supplementary Figure 2 | Comparison of digitization efforts based on Google Earth imagery (2018) and helicopter survey (2016) in the Santa Catalina Mountains, east of Sabino Canyon Recreation Area. Previous aerial survey boundaries shown in black have large, circular boundaries, both overestimating patch sizes and underestimating buffelgrass distribution. (A) Map showing numerous helicopter-surveyed patches in comparison to the Google Earth survey. (B) Map showing a single helicopter patch in comparison to the more numerous and complex boundaries of hand-digitized polygons from Google Earth. (C) A single patch identified from Google Earth imagery that was not mapped in the 2016 survey.
Supplementary Figure 3 | State-and-transition fire-spread model diagram. (A) State classes defined for the simulation, where uninvaded and seedbank are replicated for each fuel model class. (B) How growth transitions occur deterministically based on amount of time in the state class interacting with a “wet year” (probability of 26.7%) increase in age to decrease the overall amount of time required to transition to the next cover level.
Supplementary Figure 4 | State-and-transition fire-spread model study area including the fuel models used in the simulation and the 1,200 masl contour used to explore changes in low elevation fires caused by buffelgrass presence.
Supplementary Figure 5 | Locations of sampling plots established to assess saguaro mortality after the 2019 Mercer Fire. Ten plots were established within the zone of fire impact outlined in red. Five control plots were established outside of the fire zone. Circle size indicates the abundance of saguaros within each 20 × 20 m square plot. Grayscale indicates the degree of saguaro mortality in each plot.
Supplementary Table 1 | LANDFIRE mapped fuel loadings used in the simulation.
Supplementary Table 2 | FARSITE parameter values used in all simulations within SyncroSim.
Supplementary Table 3 | Patch sizes of the 10 largest buffelgrass patches compared to all other patches combined on the south slope of the Santa Catalina Mountains.
References
Abatzoglou, J. T., and Kolden, C. A. (2011). Climate change in western U.S. deserts: potential for increased wildfire and invasive annual grasses. Rangel. Ecol. Manag. 64, 471–478. doi: 10.2111/rem-d-09-00151.1
Abatzoglou, J. T., and Williams, A. P. (2016). Impact of anthropogenic climate change on wildfire across western US forests. Proc. Natl. Acad. Sci. U.S.A. 113, 11770–11775. doi: 10.1073/pnas.1607171113
Abella, S. R., Engel, E. C., Lund, C. L., and Spencer, J. E. (2009). Early post-fire plant establishment on a Mojave Desert burn. Madroño 57, 137–148. doi: 10.3120/0024-9637-56.3.137
Alford, E. J., Brock, J. H., and Gottfried, G. J. (2005). “Effects of fire on Sonoran Desert plant communities,” in Connecting Mountain Islands and Desert Seas: Biodiversity and Management of the Madrean Archipelago II Proc. RMRS-P-36, eds G. J. Gottfried, B. S. Gebow, L. G. Eskew, and C. B. Edminster (Fort Collins, CO: U.S. Department of Agriculture, Forest Service, Rocky Mountain Research Station), 451–454.
Bates, D., Mächler, M., Bolker, B., and Walker, S. (2015). Fitting linear mixed-effects models using lme4. J. Stat. Softw. 67, 1–48.
Bean, T. M. (2014). Tools for Improved Management of Buffelgrass in the Sonoran Desert. Ph.D. Dissertation Thesis. Tucson, ARI: University of Arizona.
Betancourt, J. L. (2012). “Reflections on the relevance of history in a nonstationary world,” in Historical Environmental Variation in Conservation and Natural Resource Management, eds J. A. Wiens, G. D. Hayward, H. D. Safford, and C. Giffen (New York, NY: Wiley-Blackwell), 307–318. doi: 10.1002/9781118329726.ch23
Betancourt, J. L. (2015). Energy flow and the ‘grassification’ of desert shrublands. Proc. Natl. Acad. Sci. U.S.A. 112, 9504–9505. doi: 10.1073/pnas.1512078112
Bojoìrquez, A., Aìlvarez-Yeìpiz, J. C., Buìrquez, A., and Martiìnez-Yriìzar, A. (2019). Understanding and predicting frost-induced tropical tree mortality patterns. Global Change Biol. 25, 3817–3828. doi: 10.1111/gcb.14775
Brenner, J. C., and Franklin, K. A. (2017). Living on the edge: emerging environmental hazards on the peri-urban fringe. Environment 59, 16–29. doi: 10.1080/00139157.2017.1374793
Brooks, M. L., and Pyke, D. A. (2001). “Invasive plants and fire in the deserts of North America,” in Proceedings of the Invasive Species Workshop: The Role of Fire in the Control and Spread of Invasive Species. Fire Conference 2000: The First National Congress on Fire Ecology, Prevention, and Management, eds K. E. M. Galley and T. P. Wilson (Tallahassee, FL: Tall Timbers Research Station), 1–14.
Brooks, M. L., and Chambers, J. C. (2011). Resistance to invasion and resilience to fire in desert shrublands of North America. Rangel. Ecol. Manag. 64, 431–438. doi: 10.2111/rem-d-09-00165.1
Brooks, M. L., Chambers, J. C., and McKinley, R. A. (2013). “Fire history, effects, and management in southern Nevada,” in The Southern Nevada Agency Partnership Science and Research Synthesis: Science to support land management in Southern Nevada - Executive Summary Gen. Tech. Rep. RMRS-GTR-304, eds J. C. Chambers, M. L. Brooks, B. K. Pendleton, and C. B. Raish (Fort Collins, CO: U.S. Department of Agriculture, Forest Service, Rocky Mountain Research Station), 25–34.
Brown, D. E., and Minnich, R. A. (1986). Fire and changes in creosote bush scrub of the western Sonoran Desert. Cal. Am. Midland Natural. 116, 411–422. doi: 10.2307/2425750
Brusca, R. C., and Moore, W. (2013). A Natural History of the Santa Catalina Mountains, Arizona with an Introduction to the Madrean Sky Islands. Tucson, ARI: Arizona-Sonora Desert Museum Press.
Cave, G. H. (1982). Ecological Effects of Fire in the Upper SONORAN Desert. Thesis. Tempe, AZ: Arizona State University.
Cave, G. H., and Patten, D. T. (1984). Short-term vegetation responses to fire in the Upper Sonoran Desert. J. Range Manag. 37, 491–496. doi: 10.2307/3898842
Clark, C. (2006). The Year We Lost the Desert. Earth Island Journal. Available online at: https://www.earthisland.org/journal/index.php/magazine/entry/the_year_we_lost_the_deserts/ (accessed January 18, 2021).
Cobb, R. C. K., Ruthrof, X., Breshears, D. D., Lloret, A. F. T., Adams, H. D., Anderegg, W. R. L., et al. (2017). Ecosystem dynamics and management after forest die-off: a global synthesis with conceptual state-and-transition models. Ecosphere 8:e02034. doi: 10.1002/ecs2.2034
Conlisk, E., Syphard, A. D., Franklin, J., and Regan, H. M. (2015). Predicting the impact of fire on a vulnerable multi-species community using a dynamic vegetation model. Ecol. Modell. 301, 27–39. doi: 10.1016/j.ecolmodel.2015.02.004
D’Antonio, C. M., and Vitousek, P. M. (1992). Biological invasions by exotic grasses, the grass/fire cycle, and global change. Annu. Rev. Ecol. Syst. 23, 63–87. doi: 10.1146/annurev.es.23.110192.000431
Daniel, C., Frid, L., Sleeter, B., and Fortin, M.-J. (2016). State-and-transition simulation models: a framework for forecasting landscape change. Methods Ecol. Evol. 7, 1413–1423. doi: 10.1111/2041-210x.12597
Elkind, K., Sankey, T. T., Munson, S. M., and Aslan, C. E. (2019). Invasive buffelgrass detection using high-resolution satellite and UAV imagery on Google Earth Engine. Remote Sens. Ecol. Conserv. 5, 318–331. doi: 10.1002/rse2.116
Engle, E. C., and Abella, S. R. (2011). Vegetation recovery in a desert landscape after wildfires: influences of community type, time since fire and contingency effects. J. Appl. Ecol. 48, 1401–1410. doi: 10.1111/j.1365-2664.2011.02057.x
Esque, T. C., Schwalbe, C. R., Haines, D. F., and Halvorson, W. L. (2004). Saguaros under siege: invasive species and fire. Desert Plants 20, 49–55.
Esque, T. C., Schwalbe, C., Lissow, J. A., Haines, D. F., Foster, D., and Garnett, M. C. (2007). Buffelgrass fuel loads in Saguaro National Park, Arizona, increase fire danger and threaten native species. Park Sci. 24, 33–56.
Finney, M. A. (2004). FARSITE: Fire Area Simulator–Model Development and Evaluation Research Paper RMRS-RP-4. Ogden, UT: U.S. Department of Agriculture, Forest Service, Rocky Mountain Research Station.
Franklin, K. A., Lyons, K., Nagler, P. L., Lampkin, D., Glenn, E. P., Molina-Freaner, F., et al. (2006). Buffelgrass (Pennisetum ciliare) land conversion and productivity in the plains of Sonora, Mexico. Biol. Conserv. 127, 62–71. doi: 10.1016/j.biocon.2005.07.018
Frid, L., and Wilmshurst, J. F. (2009). Decision analysis to evaluate control strategies for crested wheatgrass (Agropyron cristatum) in Grasslands National Park of Canada. Invasive Plant Sci. Manag. 2, 324–336. doi: 10.1614/ipsm-09-006.1
Frid, L., Holcombe, T., Morisette, J. T., Olsson, A. D., Brigham, L., Bean, T. M., et al. (2013). Using state-and-transition modeling to account for imperfect knowledge in invasive species management. Invasive Plant Sci. Manag. 6, 36–47. doi: 10.1614/ipsm-d-11-00065.1
Fusco, E., Finn, J., Balch, J., Nagy, R. C., and Bradley, B. (2019). Invasive grasses increase fire occurrence and frequency across U.S. ecoregions. Proc. Natl. Acad. Sci. U.S.A. 116, 23594–23599. doi: 10.1073/pnas.1908253116
Grechi, I., Chadès, I., Buckley, Y. M. B., Friedel, M. H., Grice, A. C., Possingham, H. P., et al. (2014). A decision framework for management of conflicting production and biodiversity goals for a commercially valuable invasive species. Agric. Syst. 125, 1–11. doi: 10.1016/j.agsy.2013.11.005
Holmgren, C. A., Norris, J., and Betancourt, J. L. (2007). Inferences about winter temperatures and summer rains from the late Quaternary record of C4 perennial grasses and C3 desert shrubs in the northern Chihuahuan Desert. J. Q. Sci. 22, 141–161. doi: 10.1002/jqs.1023
Iniguez, J. M., Swetnam, T. W., and Yool, S. R. (2008). Topography affected landscape fire history patterns in southern Arizona, USA. For. Ecol. Manag. 256, 295–303. doi: 10.1016/j.foreco.2008.04.023
Iniguez, J. M., Swetnam, T. W., and Baisan, C. H. (2016). Fire history and moisture influences on historical forest age structure in the sky islands of southern Arizona, USA. J. Biogeogr. 43, 85–95. doi: 10.1111/jbi.12626
Jarnevich, C. S., Holcombe, T. R., Thomas, C. C., Frid, L., and Olsson, A. (2015). Simulating long-term effectiveness and efficiency of management scenarios for an invasive grass. AIMS Environ. Sci. 2, 427–447. doi: 10.3934/environsci.2015.2.427
Jarnevich, C. S., Thomas, C., Young, N. E., Backer, D., Cline, S., Frid, L., et al. (2019). Developing an expert elicited simulation model to evaluate invasive species and fire management alternatives. Ecosphere 10:e02730.
Jarnevich, C. S., Young, N. E., Thomas, C. D., Grissom, P., Backer, D., and Frid, L. (2020). Assessing ecological uncertainty and simulation model sensitivity to evaluate an invasive plant species’ potential impacts to the landscape. Sci. Rep. 10:19069.
Koehler, P. A., Anderson, R. S., and Spaulding, W. G. (2005). Paleoenvironments of the mojave and colorado deserts during the late quaternary. Palaeogeogr. Palaeoclimatol. Palaeoecol. 215, 297–311.
Landfire. (2020). LANDFIRE Remap 2016 Scott and Burgan Fire Behavior Fuel Model (FBFM40) CONUS. LF Remap. Earth Resources Observation and Science Center (EROS). Sioux Falls, SD: U.S. Geological Survey.
Lien, A. M., Baldwin, E., and Franklin, K. A. (2021). Collective action and invasive species Governance in Southern Arizona. Rangel. Ecol. Manag. 74, 151–164. doi: 10.1016/j.rama.2020.10.004
Loftin, S. R. (1987). Postfire dynamics of a Sonoran Desert ecosystem. Thesis. Tempe, AZ: Arizona State University.
McAuliffe, J. R., and Van Devender, T. R. (1998). A 22,000-year record of vegetation change in the north-central Sonoran Desert. Palaeogeogr. Palaeoclimatol. Palaeoecol. 141, 253–275. doi: 10.1016/s0031-0182(98)00054-6
McDonald, C. J., and McPherson, G. R. (2013). Creating hotter fires in the Sonoran Desert: buffelgrass produces copious fuels and high fire temperatures. Fire Ecol. 9, 26–39. doi: 10.4996/fireecology.0902026
McLaughlin, S. P., and Bowers, J. E. (1982). Effects of wildfire on a Sonoran Desert plant community. Ecology 63, 246–248. doi: 10.2307/1937048
Merriam, C. H. (1890). Results of a biological survey of the San Francisco Mountain region and desert of the Little Colorado in Arizona. North Am. Fauna 3, 1–136. doi: 10.3996/nafa.3.0001
Miller, B. W., Symstad, A. J., Frid, L., Fisichelli, N. A., and Schuurman, G. W. (2017). Co-producing simulation models to inform resource management: a case study from southwest South Dakota. Ecosphere 8:e02020. doi: 10.1002/ecs2.2020
Noonan-Wright, E., Opperman, T. S., Finney, M. A., Zimmerman, T. G., Seli, R. C., Elenz, L. M., et al. (2011). Developing the U.S. Wildland Fire Decision Support System. J. Combust. 2011:14. doi: 10.1155/2011/168473
Olsson, A. D., Hannah, C., Hartfield, K., Orr, B. J., and Marsh, S. E. (2009). Using Remote Sensing to Map and Manage Buffelgrass Infestations in the Tucson and Santa Catalina mountains. Final Report for the National Park Service. Arizona Remote Sensing Center Office of Arid Lands Studies, The University of Arizona. Tucson, AZ: The University of Arizona.
Olsson, A. D., van Leeuwen, W. J., and Marsh, S. E. (2011). Feasibility of invasive grass detection in a desertscrub community using hyperspectral field measurements and Landsat TM imagery. Remote Sens. 3, 2283–2304. doi: 10.3390/rs3102283
Olsson, A. D., Betancourt, J. L., Crimmins, M. A., and Marsh, S. E. (2012a). Constancy of local spread rates for buffelgrass (Pennisetum ciliare L.) in the Arizona Upland of the Sonoran Desert. J. Arid Environ. 87, 136–143. doi: 10.1016/j.jaridenv.2012.06.005
Olsson, A. D., Betancourt, J. L., McClaran, M. P., and Marsh, S. E. (2012b). Sonoran Desert ecosystem transformation by a C4 grass without the grass/fire cycle. Divers. Distrib. 18, 10–21. doi: 10.1111/j.1472-4642.2011.00825.x
Olsson, A. D., and Morisette, J. T. (2014). Comparison of simulated HyspIRI with two multispectral sensors for invasive species mapping. Photogr. Eng. Remote Sens. 3, 217–227. doi: 10.14358/pers.80.3.217
Parker, K. C. (2002). “Fire in the Pre-European Lowlands of the American Southwest,” in Fire, Native Peoples, and the Natural Landscape, ed. T. R. Vale (Washington, DC: Island Press), 101–142.
Paz, H., Vega-Ramos, F., and Arreola-Villa, F. (2018). Understanding hurricane resistance and resilience in tropical dry forest trees: a functional traits approach. Forest Ecology and Management 426, 115–122. doi: 10.1016/j.foreco.2018.03.052
Pierson, E. A., Turner, R. M., and Betancourt, J. L. (2013). Regional demographic trends from long-term studies of saguaro (Carnegiea gigantea) across the northern Sonoran Desert. J. Arid Environ. 88, 57–69. doi: 10.1016/j.jaridenv.2012.08.008
Plecki, A. F., Akamani, K., Groninger, J. W., Brenner, J. C., and Gage, K. L. (2021). Homeowner perceptions and responses to buffelgrass invasion risk in the Tucson, Arizona Wildland-Urban Interface. Heliyon 7:e07040. doi: 10.1016/j.heliyon.2021.e07040
Poorter, L., Kitajima, K., Mercado, P., Chubiña, J., Melgar, I., and Prins, H. H. T. (2010). Resprouting as a persistence strategy of tropical forest trees: relations with carbohydrate storage and shade tolerance. Ecology 91, 2613–2627. doi: 10.1890/09-0862.1
Rogers, G. F. (1986). Comparison of fire occurrence in desert and nondesert vegetation in Tonto National Forest. Ariz. Madroño 33, 278–283.
Rogers, G. F., and Vint, M. K. (1987). Winter precipitation and fire in the Sonoran Desert. J. Arid Environ. 13, 47–52.
Salo, L. F. (2005). Red brome (Bromus rubens subsp. madritensis) in North America: possible modes for early introductions, subsequent spread. Biol. Invasions 7, 165–180. doi: 10.1007/s10530-004-8979-4
Scott, J. H., and Burgan, R. E. (2005). Standard Fire Behavior Fuel Models: A Comprehensive Set for use With Rothermel’s Surface Fire Spread Model. Gen. Tech. Rep. RMRS-GTR-153. Fort Collins, CO: U.S. Department of Agriculture, Forest Service, Rocky Mountain Research Station.
Shryock, D. F., Esque, T. C., and Chen, F. C. (2015). A 30-Year Chronosequence of Burned Areas in Arizona—Effects of Wildfires on Vegetation in Sonoran Desert Tortoise (Gopherus morafkai) Habitats. U.S. Geological Survey Open-File Report, 2015-1060. Reston, VA: U.S. Geological Survey, doi: 10.3133/ofr20151060
Schmid, M., and Rogers, G. F. (1988). Trends in fire occurrence in the Arizona upland subdivision of the Sonoran Desert, 1955 to 1983. Southwestern Nat. 33, 437–444. doi: 10.2307/3672211
Swetnam, T. R., and Betancourt, J. L. (1990). Fire-Southern Oscillation relations in the southwestern United States. Science 24, 1017–1020. doi: 10.1126/science.249.4972.1017
Thistle, H. W., White, J. A., Backer, D. M., Ghent, J. H., Throop, W. E., Bean, T. M., et al. (2014). A buffelgrass aerial spraying pilot project: spray application and deposition. Appl. Eng. Agric. 30, 17–24. doi: 10.13031/aea.30.10232
Turner, R. M., Webb, R. H., Bowers, J. E., and Hastings, J. R. (2003). The Changing Mile Revisited. Tucson, ARI: University of Arizona Press.
Vesk, P. A., and Westoby, M. (2004). Sprouting ability across diverse disturbances and vegetation types worldwide. J. Ecol. 92, 310–320. doi: 10.1111/j.0022-0477.2004.00871.x
Wang, H. H., Wonkka, C. L., Grant, W. E., and Rogers, W. E. (2016). Range expansion of invasive shrubs: implication for crown fire risk in forestlands of the southern USA. AoB Plants 8:plw012. doi: 10.1093/aobpla/plw012
Westerling, A. L. (2016). Increasing western US forest wildfire activity: sensitivity to changes in the timing of spring. Philos. Trans. R. Soc. B Biol. Sci. 371:20150178. doi: 10.1098/rstb.2015.0178
Whittaker, R. H., and Niering, W. A. (1965). Vegetation of the Santa Catalina Mountains. Ariz. A Gradient Anal. South Slope Ecol. 46, 429–452. doi: 10.2307/1934875
Whittaker, R. H., and Lowe, C. H. (1984). Vegetation of the santa catalina mountains: community types and dynamics. Vegetatio 58, 3–28. doi: 10.2307/40022817
Williams, D. G., and Baruch, Z. (2000). African grass invasion in the Americas: ecosystem consequences and the role of ecophysiology. Biol. Invasions 2, 123–140.
Wilson, R. C., Narog, M. G., Koonce, A. L., and Corcoran, B. M. (1995). “Postfire regeneration in Arizona’s giant saguaro shrub community,” in Connecting Mountain Islands and Desert Seas: Biodiversity and Management of the Madrean Archipelago II Proc. RMRS-P-36, eds G. J. Gottfried, B. S. Gebow, L. G. Eskew, and C. B. Edminster (Fort Collins, CO: U.S. Department of Agriculture, Forest Service, Rocky Mountain Research Station), 424–431.
Keywords: grass-fire cycle, wildland-urban interface, invasive species, El Niño southern oscillation (enso), invasive species management, Sonoran Desert, desert shrublands
Citation: Wilder BT, Jarnevich CS, Baldwin E, Black JS, Franklin KA, Grissom P, Hovanes KA, Olsson A, Malusa J, Kibria ASMG, Li YM, Lien AM, Ponce A, Rowe JA, Soto JR, Stahl MR, Young NE and Betancourt JL (2021) Grassification and Fast-Evolving Fire Connectivity and Risk in the Sonoran Desert, United States. Front. Ecol. Evol. 9:655561. doi: 10.3389/fevo.2021.655561
Received: 19 January 2021; Accepted: 27 September 2021;
Published: 26 October 2021.
Edited by:
Matthew Brooks, United States Geological Survey (USGS), United StatesReviewed by:
Matthew J. Germino, United States Geological Survey (USGS), United StatesEddie John Van Etten, Edith Cowan University, Australia
Copyright © 2021 Wilder, Jarnevich, Baldwin, Black, Franklin, Grissom, Hovanes, Olsson, Malusa, Kibria, Li, Lien, Ponce, Rowe, Soto, Stahl, Young and Betancourt. This is an open-access article distributed under the terms of the Creative Commons Attribution License (CC BY). The use, distribution or reproduction in other forums is permitted, provided the original author(s) and the copyright owner(s) are credited and that the original publication in this journal is cited, in accordance with accepted academic practice. No use, distribution or reproduction is permitted which does not comply with these terms.
*Correspondence: Benjamin T. Wilder, YndpbGRlckBhcml6b25hLmVkdQ==