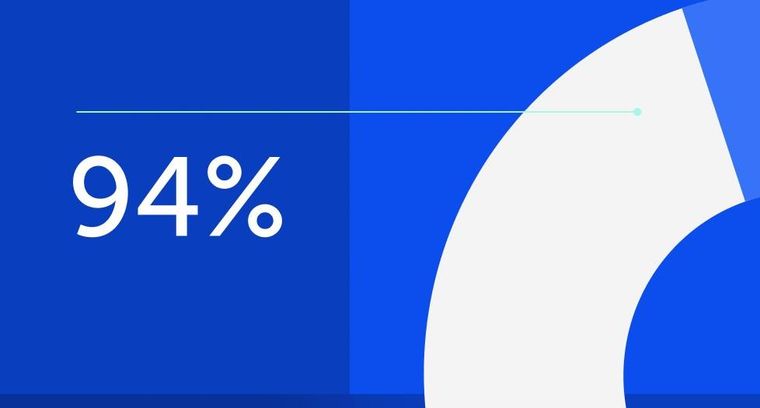
94% of researchers rate our articles as excellent or good
Learn more about the work of our research integrity team to safeguard the quality of each article we publish.
Find out more
ORIGINAL RESEARCH article
Front. Ecol. Evol., 15 June 2021
Sec. Paleontology
Volume 9 - 2021 | https://doi.org/10.3389/fevo.2021.654156
This article is part of the Research TopicEarly Avian EvolutionView all 14 articles
Recently, ∼100 Ma amber from Myanmar has become an important source of information regarding the morphology of Late Cretaceous enantiornithines. Two specimens consisting of partial hindlimbs exhibit unusual morphologies when compared to both extant avian taxa and other Cretaceous enantiornithines. Pedal morphology is extremely ecologically informative in Aves as it represents the interface between body and substrate. These seemingly bizarre pedal morphologies represent adaptations that allowed these birds to utilize certain niches present in their paleoenvironment. Specific ecological niches apply the same general pressures to different species over time, and in doing so, through natural selection, produce morphologies that function much the same, although they may be anatomically dissimilar. As such, extant animals can provide useful information pertaining to the functional morphology of extinct animals, even in the absence of direct analogs, as in the case of these two Hukawng enantiornithines. Comparisons to extant taxa in the same predicted niches of these enantiornithines can be used to either support or contradict previous hypotheses regarding the in vivo function of these unique pedal morphologies. Elektorornis chenguangi exhibits a hypertrophied third pedal digit, originally interpreted as an appendage used for probing. We support this interpretation, which allows informed speculation as to the cranial anatomy of this taxon since extant animals that probe in woody substrates consistently pair elongate probing structures with a second robust structure that functions as a means to penetrate into this hard substrate. This suggests that the rostrum of Elektorornis would have been robust and most likely edentulous. The second specimen YLSNHM01001 exhibits an unusually mediolaterally robust fourth pedal digit, nearly double the width of digit II. Given that no such morphology is present in any other bird in the Mesozoic or Cenozoic we feel the unusual morphology justifies erection of a new taxon, Fortipesavis prehendens gen. et sp. nov. Although distinct, the morphology in F. prehendens resembles the syndactyl condition in some extant avian groups, and we hypothesize the robust digit similarly functioned to increase the surface area of the foot, facilitating grip on perches through increased friction. The necessity for increased grip and the lateral placement of this digit may suggest F. prehendens utilized mobile perches similar to extant kingfishers.
The Enantiornithes (Walker, 1981) is the dominant clade of Cretaceous land birds and the most diverse recognized clade of non-neornithine birds (Chiappe and Witmer, 2002; O’Connor and Chiappe, 2011). With each new discovery, our understanding of this extinct group’s ethology and ecology grows. The vast majority of information regarding this clade comes from the Lower Cretaceous deposits in northeastern China that have produced the Jehol avifauna (Zhou and Zhang, 2007; Zhou and Wang, 2010; O’Connor and Chiappe, 2011). However, during the past 5 years, mid-Cretaceous aged Burmese amber from northern Myanmar has proved to be an unlikely but important source of information regarding this diverse clade (Xing et al., 2016, 2017, 2018a,b, 2019b,c, 2020a,b,c). Together, the record of lithic and amber fossils reveals that enantiornithines had both unique plumages and skeletal morphologies not present among extant avians (Zhang and Zhou, 2000; O’Connor and Chiappe, 2011; O’Connor et al., 2013, 2016a,b). In particular, preservation in amber commonly records particularly fine soft tissue details such as the presence of unusual scutellae scale filaments (SSFs) (Xing et al., 2019c, 2020c), skin texture (Xing et al., 2017, 2018b), and the delicate morphology of ornamental feathers (Xing et al., 2016, 2018a, 2019a, 2020a,b,c,d) that are rarely preserved in lithic fossils, and when preserved, do not record these structures in high fidelity (e.g., ornamental rachis dominated tail feathers).
Two Burmese amber enantiornithine specimens show exceptionally odd pedal morphologies that were described as unique among Aves (Xing et al., 2019b,c). Both specimens were discovered in the Late Albian-Cenomanian deposits (98.8 ± 0.6 Ma) (Shi et al., 2012) of the Angbamo locality, Hukawng Valley, Kachin Province (Tanai Township, Myitkyina District), in northern Myanmar (Figure 1A). The holotype of Elektorornis chenguangi (HPG-15-2) consists of an isolated right hindlimb and partial wing integument. The foot exhibits an unusual form of pedal digit elongation in which digit III is substantially longer than the other digits (Xing et al., 2019c). This digit also supports the proportionally longest ungual. This specific morphology is not exhibited among extant birds, nor does an exact analog appear in other living taxa. The second specimen, YLSNHM01001, an indeterminant probable enantiornithine, is even more incomplete with only remnants of digits II–IV preserved and only the skin demarcating the exterior surface of these digits together with portions of feathers belonging to the tail (Xing et al., 2019b). Digit IV is the most robust being mediolaterally expanded relative to the other digits such that digit II is approximately half the mediolateral width of digit IV. Additionally, digit IV exhibits exaggerated plantar pads as compared to most extant perching birds in that the pads are not only robust mediolaterally, but also dorsoventrally thickened such that they are separated by deep clefts.
Figure 1. (A) A map of the approximate location of the amber mines in Northern Myanmar where both specimens HPG-15-2 and YLSNHM01001 were discovered. Dark blue dotted outline = Kachin Province, gray shaded area = Hukawng Valley, red star = amber mines, and Tenai (Angbamo locality), green structures = Cretaceous-aged deposits. (B) The partial right hindlimb of Elektorornis chenguangi encased in amber (specimen HPG-15-2). (C) An interpretive osteological line-drawing of the partial right hindlimb of the Elektorornis chenguangi specimen, giving a clearer depiction of the proportionally elongated length of digit III. Scale bars = 5 mm. Photographs of specimens HPG-15-2 credited to Lida Xing, used with permission from Jingmai O’Connor.
Although both of these specimens record only partial features of the whole organism, unique morphology is clearly present. Unique morphologies invite speculation as to their in vivo function. However, the absence of direct analogs among extant avian taxa hinders attempts to infer the ecomorphological function of unique features in extinct taxa. Ecological niches have the ability to shape organismal morphology as evolutionary processes give way to physiological alterations (Wainwright, 1991; Bock, 1994; Eduardo et al., 2010; Felice et al., 2019). The morphological solutions to a specific niche’s “problems,” though not exact across taxa, are typically comparable since they represent a response to a similar set of limitations and pressures. The resultant morphologies can be anatomically disparate but should be functionally similar (Arendt and Reznick, 2008; Stayton, 2008; Pearce, 2012). Therefore, rather than looking for superficially similar morphologies with which to make functional inferences, we can explore a feature through its potential structural benefits.
Both the skeletal structure and soft tissue morphology of the avian foot are functionally important and ecologically informative because these morphologies reveal how the bird interacts with its substrate (Höfling and Abourachid, 2020), and in some groups are highly involved in the acquisition and manipulation of food (Tsang and McDonald, 2018). The placement, shape, length, and robustness of extant bird pedal digits vary greatly, and this variation correlates with ecological habit (Tsang and McDonald, 2018; Gill et al., 2019). Among Neornithes, there are several foot types based on the arrangement of the pedal digits. The most common is the anisodactyl morphology found in perching birds, in which digits II, III, and IV face cranially, and digit I (the hallux) faces caudally, facilitating grip (Lovette and Fitzpatrick, 2016).
Both unusual Burmese enantiornithines were described as arboreal, an ecological niche apparently utilized by the vast majority of enantiornithines, all of which reveal an anisodactyl morphology where pedal remains are recovered (Chiappe and Witmer, 2002). Elektorornis chenguangi was further hypothesized to have utilized its elongate third pedal digit to probe for food whereas YLSNHM01001 was hypothesized to be an aerial insectivore using its robust fourth pedal digit to grip its prey (Xing et al., 2019b,c). However, neither initial hypothesis was supported by robust comparisons with a range of taxa. Here we assess these interpretations using additional data and further explore the ecology of each of these birds using inferences drawn from avian morphologies in similar predicted ecological niches. We also explore other extant non-avian taxa with specialized features in our search for potential extant analogs. From this data, we provide more rigorous support for the initially superficial prediction that the unique pedal digit of E. chenguangi was being used as a probing device. In the case of YLSNHM01001 we also concur that this taxon was an aerial insectivore, but not for the reasons put forth by Xing et al. (2019b). These observations help us to understand how particular niches have shaped and are shaping organisms morphologically. Such studies will lead to a better understanding of the possible range of morphological adaptations to a particular ecological role and improve inferences regarding how these unusual birds and other extinct animals may have lived. We hypothesize that by observing ecomorphological features found in extant taxa from a functional perspective, we can provide better insights as to the in vivo ecology, function, and ethology of unusual morphologies exhibited in enantiornithines.
Elektorornis chenguangi (Figures 1B,C) was interpreted as a tactile prober foraging in a wooded environment (Xing et al., 2019c). Among living avian taxa, the ecological niche of wood-probing is mainly attributed to the avian family Picidae (Winkler et al., 2020a). Within this group, the bill is used for chipping or gouging woody substrate. Once a hole has been created, picids utilize their elongated tongues to then search for and acquire prey items (Winkler et al., 2020a; Figure 2C). In many species, the tongue is additionally equipped with a rostrally sheathed barbed tip to facilitate prey acquisition. Outside the Picidae, there exists another group of birds that may provide a close analog for the proposed probing function of the elongated pedal digit in E. chenguangi. Hemignathus munroi (“Akiapola”au) (Pratt, 2005) is a Hawaiian honeycreeper that has an unusual beak morphology is which the upper bill is elongated and laterally compressed whereas the lower bill is stout and comparatively shorter (Figure 2D). It uses the lower bill to hammer and gouge into woody substrate. Once an opening has been made near a presumed arthropod cavity, the bird then utilizes its elongated upper bill to probe in search of arthropods. It then manipulates the prey item either by skewering it or by dragging it out into the open where it can more easily direct it back into the mouth (Pratt, 2005).
Figure 2. In all known extant taxa that bore into and probe within hard substrate (e.g., wood), the “tool” used to extract prey items are accompanied by a structure that first allows entry into the hard substrate. The left column exhibits the morphologies used to probe and extract prey items (shaded blue), while the right column shows the morphologies used to break into the hard substrate (shaded gray). (A) The Aye-aye (Daubentonia madagascariensis) and the (B) Striped Possum (Dactylopsila trivirgata) both exhibit elongate manual digits used to probe in holes made in wood created by specialized rostromedially angled incisors which gnaw away woody material. The arrows indicate the specific digit used to probe. (C) Insectivorous woodpecker species exhibit distally barbed tips of the tongue covered in thick sheaths in order to skewer prey items within cavities which are accessed by gouging made possible by a robust conical bill and cushioned cranium. (D) The “Akiapola”au (Hemignathus munroi) probes with its distally tapered flexible upper bill, while the bottom robust bill, reminiscent to that of most woodpeckers, facilitates wood gouging with its straight, wedge-like shape. Line illustrations not drawn to scale.
The ecological niche of probing inside a hard substrate is not limited to Aves. Xing et al. (2019c) compared the predicted ecological niche of E. chenguangi to the prosimian mammal Daubentonia madagascariensis (Aye-aye), which gnaws into woody substrate and uses its elongate manus and unique, thin third manual digit to probe in woody matter for arthropods (Soligo, 2005; Figure 2A). Similarly, the marsupial Dactylopsila trivirgata (Striped possum) gnaws into woody substrate and removes arthropods with both its elongated tongue and an elongated fourth manual digit (Van Dyck, 1983; Handasyde and Martin, 1996; Figure 2B).
The specimen preserves an outline of digits II, III, and IV (Figures 3A–C). Digit I is not preserved but it most-likely faced caudally, opposing digits II–IV in an anisodactyl arrangement (Xing et al., 2019b). The fourth digit is the most robust, measuring nearly twice the mediolateral width of digit II. The plantar pads of digit IV appear exaggerated, exhibiting ventral extensions from the plantar surface of the pes similar to some members of the orders Accipitriformes, Piciformes, and Psittaciformes (Figure 4D). Only the outline of digit II’s ungual is preserved, therefore the shape and size of the digits III and IV unguals are unknown. The single preserved ungual is large, elongate, and recurved, supporting both an arboreal ecology and probable referral to the enantiornithines (Xing et al., 2019b).
Figure 3. The external surface of the partial left pes of YLSNHM01001 in (A) medial, (B) ventral, and (C) dorsal views. Note digit I (hallux) is missing from the specimen but is assumed to be a feature based on known enantiornithine pedal morphology. Scale bars = 5 mm, (A–C) Photographs of specimen YLSNHM01001 credited to Lida Xing, used with permission from Jingmai O’Connor.
Figure 4. (A) An in-life reconstruction of Fortipesavis prehendens’ pedes. The most robust digit is laterally placed on each pes and the plantar pads exhibit a slight pebbly texture. (B) The amber mold of Fortipesavis prehendens in medial view, with focus on the reticulae textured plantar pads of digit II. Inset shows larger image of plantar pad texture as signaled by the white arrow. Scale bar = 5 mm. Photo of specimen YLSNHM01001 credited to Lida Xing, used with permission from Jingmai O’Connor. (C) In-life reconstruction exhibiting the same posture as the amber specimen, exhibiting the textured plantar pads similar to the texture seen in other extant avian pedes (D) such as members of the Psittacidae (Ara chloropterus) (top) and Ramphastidae (Rhampastos toco) (bottom).
The graduated arrangement of the pedal digits with the narrowest digit located medially and the widest digit laterally placed most closely resembles the syndactyl (e.g., Alcedinidae, Meropidae) morphology. In most anisodactyl species that exhibit the syndactyly condition, it is primarily the soft tissue encasing digits III and IV that are fused such that these two digits share mediolaterally wide plantar pads whereas in zygodactyl syndactyl birds (e.g., Rhamphastidae) it is only digits II and III that are fused. In syndactyl birds, fusion of the pedal digits may be limited to the proximal portions or complete, such that the two digits are fused along their entire length forming a singular enlarged plantar surface (Winkler et al., 2020b). YLSNHM01001 does not exhibit a syndactyl condition, but the extreme width of the fourth digit in this specimen is strongly reminiscent of the fused digits III and IV in extant anisodactyl-syndactyl birds and this may suggest similarities in vivo function.
Syndactyly primarily occurs in arboreal birds and has evolved multiple times, also being exhibited in two unique families of Passeriformes, the Calyptomenidae and Eurylaimidae (colloquially known as broadbills) (Winkler et al., 2020b,d,e,f,g), as well as in the Phoeniculidae (wood hoopoes), and even some members of the Trochilidae (hummingbirds) (Botelho et al., 2015). However, in these groups fusion is minimal, typically with only the proximal portion of digits III and IV conjoined. Increasing the plantar surface of the foot increases friction with the substrate and thus improves grip during perching (Höfling and Abourachid, 2020). Predominant among anisodactyl-syndactyl birds in which digits III and IV are joined through soft tissue, thus increasing the surface area of the lateral portion of the foot similar to the condition in YLSNHM01001, are the Alcedinidae (kingfishers) and Meropidae (bee-eaters), both groups that forage from frequently mobile perches that require increased grip (Backus et al., 2015). The deep interpad sulci like those observed in YLSNHM01001 are also found in some arboreal taxa including some syndactyl species (Höfling and Abourachid, 2020).
The avian foot represents the interface between the body and substrate and in some groups is highly involved in prey acquisition and object manipulation (Tsang and McDonald, 2018; Höfling and Abourachid, 2020). As such, the foot can be highly informative with regards to ecology. Recently, two enantiornithines from the Late Cretaceous ∼100 Ma Burmese Hukawng avifauna were described with unique pedal morphologies not observed in any other bird, living or extinct (Xing et al., 2019b,c). Given the ecological diversity of modern birds and the shape of Cretaceous ecosystems, it is very likely that these enantiornithine taxa exploited ecological niches utilized by neornithines today. However, the unique morphologies observed in these two specimens suggest that these Cretaceous enantiornithines evolved their own adaptations different than those evolved by neornithines through which they exploited similar niches. Although each specimen preserves a pedal morphology not found among the approximately 10,000 species of extant birds (Neornithes), the ecological functions of these unique foot morphologies can be discussed through ecomorphological predictions and comparisons with potentially functionally analogous structures in extant taxa, not limited to other birds. Organisms have clearly changed over time, but the various “problems” or environmental pressures they experience as a result of exploiting resources in similar niches acquired through comparable ecological roles leads to the evolution of similar “solutions” or structures with comparable functions (Arendt and Reznick, 2008; Stayton, 2008; Pearce, 2012). Thus, behavioral inferences of extinct species based on unique morphologies can be explored through detailed comparisons of functional morphology.
Elektorornis chenguangi (Xing et al., 2019c) exhibits a novel appendicular appendage in which the third pedal digit has been greatly elongated such that it is approximately double the length of digit II. Among birds, digit III is most commonly the longest digit in the foot, but the proportions observed in E. chenguangi and the specific morphological trait of a singularly elongated pedal digit is not found in any extant bird, nor does a directly similar structure appear elsewhere in any extant non-avian taxa (Figures 5B–D). Xing et al. (2019c) made two predictions regarding the function of the elongate third digit: that it increased the arboreal function of the foot and that it may be a trophic adaptation, or served both functions. Here we examine these two predictions and provide additional support for interpretations that this digit was involved in a probing foraging behavior.
Figure 5. (A) Comparison of the left leg skeletal anatomy between the picid Melanerpes aurifrons (Golden-fronted Woodpecker) (left), and the passerine Turdus migratorius (American Robin) (right). Both species average nearly the same wing chord length (130 mm), and average mass (M. aurifrons: 73–99 g, T. migratorius: 60–94 g), yet the length of the tarsometatarsus (M. aurifrons: 22 mm, T. migratorius: 34 mm) and tibiotarsus is greatly reduced compared to other taxa with similar body size in the scansorial M. auriforns and other picids, sittids, and furnarids. Scale bar = 10 mm. fe, femur; tbt, tibiotarsus; tmt, tarsometatarsus. (B–D) The various hypertrophied appendages of wood-boring and probing organisms compared with similar relatives that occupy other niches. All organisms on the right-hand side column demonstrate a deviation from the average proportional morphology of many closely-related taxa, whether the appendage be either the manus (top), bill (middle), or pedes (lower). (B) Two lemurs, the Indri (Indri indri) (left) and the Aye-aye (Daubentonia madagascariensis) (right). (C) Two Hawaiian honeycreepers, the Apapane (Himatione sanguinea) (left) and “Akiapola”au (Hemignathus munroi) (right). (D) Two Cretaceous enantiornithines, Rapaxavis pani (left) and Elektorornis chenguangi (right). Figures not drawn to scale.
Many extant birds have proportionally elongated pedal digits such as members of the Charadriiformes and Passeriformes. However, in these groups all digits are proportionally elongated. In passeriforms, digital elongation serves to improve grip, facilitating perching behavior (Lovette and Fitzpatrick, 2016). Charadriiform pedal digits have become proportionally elongate in order to increase surface area to facilitate walking over soft substrates. Notably, many charadriiforms probe for food. However, members of this group utilize unique bill morphologies to probe within the soft substrate upon which they walk (Lovette and Fitzpatrick, 2016). Elektorornis chenguangi exhibits only a single cranially facing elongated pedal digit paired with an elongate hallux and large, recurved claws, morphologies all absent in extant charadriiforms, dispelling the possibility that this specialized pes morphology was for increased surface area when walking over soft surfaces. This pedal morphology also makes it highly unlikely that E. chenguangi could both probe with its elongated third pedal digit and maintain balance on soft terrain without also sinking. Therefore, the possibility that E. chenguangi probed in a soft substrate can also be ruled out.
Like other enantiornithines, the anisodactyl foot of E. chenguangi appears to be adapted for arboreality as the proportionally long hallux and long, pointed, and recurved ungual sheaths are features consistent with an arboreal lifestyle in birds and are present in all known enantiornithines (O’Connor, 2012; Lovette and Fitzpatrick, 2016; Xing et al., 2019c). Furthermore, in E. chenguangi and other enantiornithines, the penultimate phalanges are the longest non-ungual phalanges within each digit, a morphology statistically linked to an arboreal lifestyle, whereas a terrestrial ecology as in charadriiforms is indicated by phalanges that become progressively shorter distally in each digit (Hopson, 2001). Xing et al. (2019c) hypothesized that the elongated third digit aided in gripping perches since the reversed hallux in this taxon is also proportionately elongate relative to other enantiornithines and considered these two features together to indicate increased arboreal function relative to other enantiornithines.
Xing et al. (2019c) noted that arboreal skinks also display elongated digits, which similarly provide for increased grip. Skinks with longer digits were shown to move with greater agility on the ground and were also able to climb trees faster than similar species with comparatively shorter digits (Huang, 2006). The comparative increase in speed is most likely attributable to increased surface area and subsequently, greater traction. However, in the studied skinks all digits were elongated, not a singular elongated digit like that in E. chenguangi. Lizards that are primarily arboreal tend to have proportionally longer digits, while those with more terrestrial lifestyles have shorter digits (Losos et al., 2006). However, for lizards that live in arboreal settings, digit length is less important than total appendicular length with shorter limbs being a better indicator of an arboreal lifestyle (Losos et al., 2006).
A similar pattern is also observed in birds. Evolution in crown birds facing the “problem” of stability in an arboreal environment overcome this physiological issue in a similar way. When grip strength becomes a priority in arboreal and scansorial species, the metatarsus and tibiotarsus either evolve to decrease in length, shortening the hindlimb, or the hindlimbs become overall stouter in proportion to the body as seen in most members of the families Picidae, Sittidae, and Furnariidae, and even rare cases in the Parulidae (Hoyo et al., 1992; Figure 5A). Any morphological change that brings the body closer to the branch or trunk, and thus lowers the center of gravity, is advantageous for balance and stability (Grant, 1966; Schulenberg, 1983; Zeffer and Norberg, 2002; Zeffer et al., 2002). Elektorornis chenguangi, like other enantiornithines, has a proportionally long tibiotarsus and tarsometatarsus (Xing et al., 2019c; Falk et al., 2020). Proportionally long appendicular bones are not well-suited for lowering the center of gravity closer to the perching surface for birds, so the need for greater stability in an arboreal or scansorial context is most likely not the evolutionary cause of E. chenguangi’s elongated pedal digit.
The second prediction by Xing et al. (2019c) was that the elongate third pedal digit of E. chenguangi represents a tactile probing structure used in foraging behaviors. The authors hypothesized that the foot would aid in, or be the primary means of, food acquisition. Xing et al. (2019c) compared the odd digit morphology of E. chenguangi to another organism that has a somewhat similar structure, the extant Madagascan prosimian Dau. madagascariensis, a species of lemur colloquially known as the Aye-aye. Although there exists no direct analog, since no extant taxon probes with its feet, the manual digits of Dau. madagascariensis and elongated appendages in other mammals and some crown birds may still aid in understanding the ecological function of the unique appendage in E. chenguangi.
The manual digits in Dau. madagascariensis are elongated relative to most other prosimians and the third digit has been drastically diminished in robustness relative to the rest of the manual digits (Figures 3B, 4A). This thin third digit is used to probe for grubs and other arthropods within openings in woody matter created using its powerful jaw muscles and specialized dentition (Jouffroy, 1975; Milliken et al., 1991; Feistner et al., 1994; Erickson et al., 1998). The fourth digit is the longest, followed by the third digit, neither of which is twice the length of digit II (Soligo, 2005). Another potential mammalian analog, the marsupial Dac. trivirgata (Striped possum), gnaws into hard woody substrates with its rostromedially placed incisors and uses both its elongated tongue and elongated fourth manual digit to probe for prey (Van Dyck, 1983; Handasyde and Martin, 1996). Upon closer inspection, the proportions of the hand in Dau. madagascariensis and Dac. trivirgata are actually quite disparate from those in the foot of E. chenguangi (Figures 5B,D). However, these three taxa share the presence of some form of digital elongation, which in the two extant mammals, direct observation demonstrates are wood-probing adaptations.
Birds are unlikely to evolve manual probing structures like those seen in mammals since their manual digits are reduced and consolidated to form a sturdy surface for the attachment of the primary flight feathers. However, within crown birds there are still examples of taxa that probe for food that may also be relevant to ecological interpretations regarding E. chenguangi. In extant birds, the wood-probing niche is primarily utilized by the Picidae family which, like Dau. madagascariensis, are percussive foragers (Lovette and Fitzpatrick, 2016). Picids bore holes in woody matter with their bills and probe with their elongated, barbed tongues, which in some species are tipped with a keratinous sheath with recurved spines (Lovette and Fitzpatrick, 2016). Picid tongues resemble harpoons and work in a similar fashion (Figure 2C).
The wood-probing niche is also present in the Hawaiian archipelago avifauna, in which a specific group of Hawaiian honeycreepers have elongated a part of their body in order to probe, presumably similar to E. chenguangi. The Hawaiian honeycreeper genus Hemignathus is divided into two subgenera: Akialoa and Hemignathus. All members but one are now either critically endangered, thought to be extinct, or are known to be extinct (Pratt, 2005). Although a majority of species belonging to these two subgenera primarily picked and gleaned in and under flakes of bark, and occasionally, at the base of native plant leaves (Pratt, 2005), one species probes in wood—the sole remaining member of this group H. munroi (“Akiapola”au), found only on the Big Island of Hawai’i in the Hawaiian archipelago. The lower bill of H. munroi is very short, thick, and stout when compared to the upper bill which is long, laterally compressed, and tapers as it extends distally to a short point (Figure 5C). Hemignathus munroi uses its stout lower bill to hammer and gouge holes into woody matter and then probes these openings using its elongate upper bill (Perkins, 1903; Munro, 1960; Hoyo et al., 1992, 2002; Ralph and Fancy, 1996).
The behavior and tools organisms have evolved in order to exploit the wood-probing niche, while sometimes appearing physically dissimilar, are similar in their function, being elongate and with some degree of flexibility. In extant wood-probers we see several structures that have become adapted for this role: the upper bill in H. munroi, the tongue in many picids, and manual digits in Dau. madagascariensis and Dac. trivirgata. If correctly interpreted, E. chenguangi, adds pedal digits to the diversity of known probing structures. Unique to the enantiornithines, E. chenguangi is the only member of the clade to exhibit a digit III that is 120% the length of metatarsal III, and 118% the length digit II (Table 1). As in other elongated appendages evolved for the purpose of probing after a surface has been bored into, proportional differences are evident when compared to closely relate non-probing taxa (Table 1).
Table 1. (Top) Comparisons of pedal digit proportions between Cretaceous aves and (Bottom) upper and lower bill lengths in eight species of Hawaiian honeycreeper.
These structures vary in their degree of flexibility and versatility. Even though the tongue contains small bones (the hyoids), with the exception of the keratinized tip in some taxa, the extended portion of the tongue is not a rigid structure, and is much more flexible and pliable then an elongated pedal digit in which bending is limited to the location of the interphalangeal joints and their range of motion. This may also explain the relative greater diversity (as a measure of success) in the Picidae compared to these other wood-probing groups that rely on less versatile structures. Among extant probers, elongated digits (as opposed to hypertrophied cranial features like bills and tongues), are only observed in wood-probing mammals (Milliken et al., 1991; Handasyde and Martin, 1996). Thus, is appears that E. chenguangi evolved a more mammalian-style, rather than avian, means of probing. Although mammals utilize their forelimb digits, these are reduced and modified for flight in birds and thus cannot be adapted into specialized feeding structures in taxa that retain their volancy. Notably, in ornithothoracines, the hand is reduced and consolidated in a way that appears irreversible since no flightless bird has evolved elongated manual digits (McCall et al., 1998; Nudds and Davidson, 2010).
A common pattern emerges among extant taxa that have evolved to acquire prey within a wood substrate by means of an elongated and flexible appendage. In addition to the elongated structure used for extraction, all extant wood-probers have also evolved a means to enter this hard substrate. This pattern is observed in both birds (Munro, 1960; Hoyo et al., 1992, 2002; Ralph and Fancy, 1996; Pratt, 2005; Winkler et al., 2020a) and mammals (Jouffroy, 1975; Van Dyck, 1983; Milliken et al., 1991; Feistner et al., 1994; Handasyde and Martin, 1996; Erickson et al., 1998). Entering the hard substrate entails creating an opening near where the prey resides, which requires a robust structure (e.g., teeth and jaw musculature, thick bill). Among mammals, in both Dau. madagascariensis and Dac. trivirgata, access into the hard wood substrate is achieved through a pair of specialized rostromedially placed incisors, similar to rodents, paired with robust jaw muscles (Morris et al., 2018). Similarly, although not feeding within a wood-substrate, members of the families Myrmecophagidae (ant-eaters) and Orycteropodidae (aardvarks) remove hard substrates (arthropod mounds) with their robust forelimbs in order to access their prey with specialized elongated tongues (Sesoko et al., 2015; Casali et al., 2017; Legendre and Botha-Brink, 2018). In extant avian wood-probers, picids have evolved dense skulls and a suite of other features including reinforced cervical vertebrae that aid in the hammering and subsequent removal of woody matter (Lovette and Fitzpatrick, 2016), whereas the honeycreeper H. munroi has evolved a stocky, dense, lower bill that allows it to hammer into wood.
Elektorornis chenguangi would likely be physiologically capable of picking under flakes of bark or into branch junctions with its hypertrophied digit in search of prey items, yet we find this ecology less likely. Extant bird groups that pick underneath and in-between hard surfaces such as bark or glean from or within soft surfaces such as leaf clumps or mud, typically do so with an elongated bill in which the upper and lower portions are subequal (Lovette and Fitzpatrick, 2016) (e.g., the families Furnariidae, Certhiidae, Sittidae, Neosittidae, and Climacteridae). Rostral elongation is possible in enantiornithines, as evidenced from the Early Cretaceous Longipteryidae (Zhang et al., 2001; O’Connor et al., 2009). Therefore, unless the mouth was modified for a different specific behavior, it would be inefficient to forage with the foot only to then move the food to the mouth after acquisition. Among extant wood probers, the evolution of an additional specialized probing appendage is found only in taxa in which the mouth apparatus is already serving another function, such as gnawing as exhibited in Dau. madagascariensis and Dac. trivirgata, and gouging as demonstrated in picids (Figures 2A–C). Hemignathus munroi represents an odd intermediate with half of the beak serving to gouge while the other serves as a probing device (Figure 2D).
This pairing of structures observed in extant wood-probers allows us to make predictions regarding the anatomy of E. chenguangi, of which only the hindlimb is known (Figures 1B,C). If the hypothesis that E. chenguangi was a tactile wood-prober is correct, then this species most likely also required a means of removing woody substrate prior to prey acquisition. Therefore, we hypothesize E. chenguangi may have possessed a unique rostral morphology that aided in either prying or gouging hard substrates, the two primary methods utilized by extant birds to enter bark or woody substrate in order to access arthropod cavities (Mountainspring, 1987; Zusi, 1989; Simon et al., 2020; Winkler et al., 2020a). Enantiornithines varied in their cranial morphology with respect to rostral length, dentition, and presence of a complete infratemporal bar, which has led to inferences that members of this clade occupied a variety of ecological niches (O’Connor, 2019; O’Connor et al., 2020). At least one Late Cretaceous documented taxon was edentulous (Chiappe et al., 2001). This indicates that enantiornithines had the genetic potential to utilize a variety of rostral morphologies.
In addition to their specialized bill morphology, picids possess a complex suite of other specialized cranial and cervical features to cope with the impact generated by their boring behavior (Winkler et al., 2020a). Since this level of anatomical specialization is not recognized in any Cretaceous enantiornithine or other non-neornithine avian group, this suggests that it was very unlikely that E. chenguangi would be able to bore in a manner similar to picids. If E. chenguangi utilized a prying behavior as a means to enter woody substrates, we would predict an overall robust bill with a dorsoventrally and mediolaterally widened base and robust dentaries (Zusi, 1989; Simon et al., 2020). Alternatively, if a gouging action was utilized we might expect to find a rostral morphology characterized by a sub-conical, distally tapering bill with minimal curvature (e.g., picids).
We predict that with either rostral morphology (prying or gouging) E. chenguangi was likely edentulous or partial edentulous, with teeth—if present—caudally restricted. Both behaviors involve considerable force and the small, delicate teeth of most Cretaceous enantiornithines would most likely interfere with this behavior and or become damaged. Furthermore, if the elongated pedal digit was the means of prey acquisition, teeth may not have been necessary. It has been demonstrated that in birds under a relax of evolutionary pressure teeth are rapidly lost (Louchart and Viriot, 2011; Li et al., 2020). Altogether, we suggest E. chenguangi was most likely edentulous. These predictions, based on niche associated morphologies as seen in extant taxa, allow for not only for more scientifically accurate reconstructions, but also for possible identification of fragmentary material from the Hukawng recovered in the future. Should cranial material be recovered for E. chenguangi and a robust rostral morphology is not present, functional interpretations of the elongate third pedal digit as a wood-probing structure would be unsupported and the role of the elongate third pedal digit would need to be reassessed.
Xing et al. (2019c) did not specify a particular mode of probing although the comparison with Dau. madagascariensis indirectly suggests probing in wood and the suggestion that the SSFs had a tactile function may further suggest probing in an enclosed, hidden environment in which visually guided probing is not possible. Elektorornis chenguangi exhibits SSFs on the tarsometatarus and pedal digits. These unusual integumentary structures are also present in other Burmese amber enantiornithines (Xing et al., 2017, 2019a, 2020b,c,d). Although their primary function is still unclear, it has been suggested that they served a mechanosensory function (Xing et al., 2019c). These structures are present dorsally, laterally, and ventrally on other phalanges, but concentrated clusters of them can be found near the apex of digit III, just before the ungual. Similar structures are present, though less extensively, on the distal end of digit IV. Clustered groups of SSFs could represent areas of increased sensory sensitivity, which could aid in probing for prey. Tactile signals indicating an arthropod occupant within a cavity might have been the primary means of prey detection for E. chenguangi once the digit was inserted into a cavity. Herbst corpuscles, which would presumably be associated with the SSFs if they indeed had a sensory function, occur in greater abundance in the bill tip organ of taxa that forage using their rostra to probe soft substrate (Cunningham et al., 2010, 2013). Some animals that forage in similar environments in which visual cues are not the primary means of food acquisition display similar appendages that are used for tactile signals. In addition to the avian bill tip organ (Cunningham et al., 2010, 2013), biological structures like catfish barbules, and some species of birds’ rictal bristles are used for sensory purposes to locate prey (Kapoor and Bhargava, 1967; Lovette and Fitzpatrick, 2016). However, with many Burmese amber enantiornithines exhibiting pedal SSFs, with some even present on the tarsus (Xing et al., 2020b), these structures may also be a widespread feature that assisted in stability or balance when perched. Potentially, their sensory function involved in stability and balance made the evolution of the elongate third pedal as a probing mechanism possible. Histology of the SSFs may shed light on their function in the future.
Inferences regarding YLSNHM01001 are limited due to the only preserved remains being a mold of a single pes in amber. However, this mold is preserved in high-fidelity, and the observed features are quite unique. This specimen is characterized by digits that progressively become mediolaterally wider laterally on the foot such that the fourth pedal digit is twice the mediolateral width of the second (Xing et al., 2019b). Similar to E. chenguangi, there is no extant bird that exhibits the same pedal morphology that can serve as a direct analog for the morphology observed in YLSNHM01001 (Xing et al., 2019b). Although the external morphology of digits II, III, and IV are preserved, digit I, which most likely formed a caudally-facing hallux, is not preserved, hindering interpretations. The robust fourth pedal digit is atypical for enantiornithines (Chiappe and Walker, 2002; Figure 4A). In other enantiornithines, digits II and III are usually equally robust (digit IV being slightly more delicate), or the second digit is slightly more robust, as in bohaiornithids (Wang et al., 2014). In YLSNHM01001, the plantar pads of digit IV appear hypertrophied, exhibiting greater size and depth than in the adjacent digits. The digit II ungual is the only one preserved, so the shape and size of the unguals on the remaining preserved digits are unknown (Figures 3A–C). As in most other Hukawng enantiornithines, this specimen also exhibits SSFs (Xing et al., 2019b, 2020b). Although preservation is limited, the proportions of digit IV relative to digits II and III distinguish this specimen from all other known avians, therefore we feel justified in erecting a new taxon based on this specimen (see section “Systematic Paleontology”) Fortipesavis prehendens gen. et sp. nov.
Xing et al. (2019b) suggested a possible raptorial ecological niche for F. prehendens due to the size and slight “pebbly” reticulae texture on the plantar pad of digit II which is moderately similar to some extant birds of prey (Figure 4B; Xing et al., 2019b). However, the plantar pad texture is more reminiscent of non-raptorial birds such as those in the families Phasianidae, Ramphastidae, and Psittacidae, exhibiting flattened sub-circular reticule rather than the often-observed ventrally exaggerated and finely textured plantar pads of birds of prey (Figures 4B–D). Additionally, the lateral placement of the most robust digit is in fact dissimilar to the pedal morphology of most extant raptorial birds. In almost all birds of prey the most robust and often shortest digits are the medial digits I or II (Lovette and Fitzpatrick, 2016). This morphology positions the body mass on top of the prey, increasing stability and facilitating grip, and thus decreasing the likelihood of the prey escaping (Fowler et al., 2009; Tsang et al., 2019). This behavior has also been predicted for dromaeosaurids which share with extant raptorial birds a similar pes morphology in which the medial digit II is the most robust (Fowler et al., 2011). In contrast, having the most robust digit, intended for hunting or pinning prey, on the lateral side of the foot relative to the body, would most likely result in instability and decrease the pressure exerted by the body mass applied to the prey item.
In a rare example and exception among extant raptors, in Pandion haliaetus (Osprey) the fourth digit is typically the most robust. However, P. haliaetus has a unique foot structure in which the typical raptor anisodactyl morphology used by the bird when engaged in perching behavior, transforms into a zygodactyl morphology during prey capture through caudal rotation of the fourth digit. This rotation of the fourth digit provides added grip, necessary for maintaining hold of the slippery laterally compressed bodies of fish, P. haliaetus’ prey of choice (Bierregaard et al., 2020). The plantar surface of the pedal digits of this piscivorous raptor are covered in spicules which provide additional grip similar to the lingual papillae on the tongues of penguins which also feed on fish (Kobayashi et al., 1998; Allen et al., 2018). The soft tissue morphology F. prehendens is also inconsistent with interpretations regarding raptorial function. In general, the plantar pad papillae are far more exaggerated, an adaption for dispersing pressure, with a sharp texture that increases grip (Lovette and Fitzpatrick, 2016; Höfling and Abourachid, 2020). Furthermore, the interpad sulci are more weakly defined compared to F. prehendens (Höfling and Abourachid, 2020).
Although inconsistent with gripping prey, the medial to lateral graduated order of the digital diameters in F. prehendens still provides important clues as to the in vivo function of this unusual pedal morphology. Members of the avian order Podicipediformes exhibit enlarged lateral pedal digits, which are additionally lobate, a morphology that facilitates diving and swimming (Winkler et al., 2020c). However, F. prehendens clearly does not exhibit an aquatic pes morphology, lacking lobate digits, webbing, or short pedal unguals. In some extant birds with the zygodactyl foot morphology (e.g., family Ramphastidae and order Psittaciformes), the most robust or longest digit is located laterally on the foot, but because the fourth digit is reversed, it is digit II or III that is the most robust. This pes morphology has multiple functional benefits, including facilitating grip, object manipulation, and stability on moving perches (Lovette and Fitzpatrick, 2016). In F. prehendens, digits II–IV appear to be facing cranially, preserved flexed in a gripping morphology. The absence of indications of deformation strongly suggests a zygodactyl morphology was not present in F. prehendens.
As hypothesized by Xing et al. (2019b), it seems most likely that F. prehendens was anisodactyl, as in all other known enantiornithines. Various groups of extant birds have a modified anisodactyl morphology known as syndactyly in which pedal digits, typically III and IV, are either partially or completely fused such that the digits in some groups are nearly completely encased in tissue forming a single structure (e.g., Coraciiformes, Bucerotiformes) (Bock and Miller, 1959). The condition of syndactyly is not restricted to anisodactyl pedes, but also appears minimally in some zygodactyl (e.g., picids, ramphastids, capitonids) and heterodactyl (e.g., trogonids) species (Höfling and Abourachid, 2020); however, the syndactyl condition is most commonly used to described anisodactyl morphologies with some degree of fusion between the three cranially facing pedal digits (Table 2).
In such taxa, the two cranially-facing lateral digits function as one enlarged laterally-placed digit, which may share mechanical similarities with the morphology observed in F. prehendens (Figure 4A). The evolutionary origin behind syndactyly in birds is as yet undetermined, although it is clear it has evolved multiple times, possibly for different reasons in at least some occurrences. In general, this morphology increases the surface area of the foot which in turn increases friction and thus increases grip (Höfling and Abourachid, 2020). The anisodactyl, heterodactyl, and zygodactyl foot morphology and syndactyl variants of these pedal arrangements are considered to be adaptations for perching, each with their own particular benefit (Bock and Miller, 1959).
Two families belonging to the order Coraciiformes are characterized by an anisodactyl-syndactyl foot morphology and hunt from frequently mobile perches (e.g., swaying branches): the Meropidae (bee-eaters) and the Alcedinidae (kingfishers). In both groups the extent of the syndactyl fusion varies but most commonly digit II is minimally joined to digit III along the length of the proximal phalanx and digits III and IV are extensively joined such that only the penultimate phalanges are free. Meropids are generally small birds and hunt insects, whereas alcedinids are a predominately piscivorous group and occupy a larger size range compared to meropids.
Alcedinids employ a hunting strategy that includes waiting on perches near water or over streams, which often move and sway, either being blown by the wind, or erratically moved by the water current. Some meropids also hunt near rivers but in general this group utilizes open environments prone to wind (Hoyo et al., 2001). Hunting from mobile surfaces requires adaptations that provide greater stability such as head stabilization, so that a successful trajectory to a prey item can be achieved (Wallman and Letelier, 1993; Katzir et al., 2001, 2018; Ochs et al., 2017). In the coraciiforms with the anisodactyl-syndactyl morphology, greater stability is achieved through the fusion of pedal digits, which allows for less pedal movement [also accompanied by a reduction in musculature for the outer digits (Backus et al., 2015)] and a laterally placed expanded plantar surface with which to grasp surfaces which produces a firmer grip on mobile, and occasionally thin, perches through increased surface friction (Bock and Miller, 1959; Höfling and Abourachid, 2020). Similarly, the pedal morphology exhibited by F. prehendens increases the lateral surface area of the foot in contact with the perch which may suggest this taxon also exploited an ecological niche that required a firmer grasp than non-specialized arboreal birds with a typically proportioned anisodactyl pedes could provide, such as mobile perches (Höfling and Abourachid, 2020).
Both meropids and alcedinids have proportionally small pedes. Notably, the preserved traces in F. prehendens holotype also suggest a proportionally small pedes. The distal portions of the metatarsals appear quite thick, possibly indicating relatively robust, stout legs, which are not observed in other enantiornithines preserved in amber also associated with soft tissue (Xing et al., 2017, 2020c,d; Winkler et al., 2020b,d,e). This functional speculation supports interpretations that a reversed hallux was present, as in meropids and alcedinids. Syndactyl birds also commonly have exaggerated interpad sulci (Höfling and Abourachid, 2020) like that is F. prehendens, a morphology that is clearly absent in other Burmese amber enantiornithines in which the soft tissue can be observed (Xing et al., 2020c,d). These exaggerated pads likely aided in increasing surface area, which increased surface contact, and therefore increased friction.
In light of existing interpretations regarding diet in other enantiornithines (O’Connor, 2019), it is possible that F. prehendens was an aerial insectivore as originally hypothesized similar to extant meropids and eurylaimids. However, in contrast to the original interpretation which inferred the foot was adapted for grasping prey (Xing et al., 2019b), we hypothesize the foot is adapted for increased stability while possibly utilizing mobile perches and that the rostrum would most likely have been the means of prey acquisition. A piscivorous diet similar to alcedinids is also consistent with the paleoenvironment of the Burmese amber, which is interpreted as a mangrove forest. However, F. prehendens is much smaller than most alcedinids which may suggest an insectivorous diet is more likely. The accrual of additional skeletal remains, in particular pertaining to the skull, may shed additional information on the specific niche utilized by this enantiornithine lineage (Zhang et al., 2001; Wang and Zhou, 2017). A diversity of bill morphologies are observed among extant syndactyl birds with piscivorous and insectivorous diets ranging from elongate and slender (e.g., Meropidae) to stout and wide (e.g., Calyptomenidae and Eurylaimidae) preventing us from making predictions regarding the rostral morphology of F. prehendens.
It is notable that among neornithines, specialized foot morphologies such as heterodactyly, zygodactyly and syndactyl-anisodactyly are limited to altricial birds and all syndactyl neornithines are all regarded as super-altricial (Botelho et al., 2015). In contrast, enantiornithines are considered highly precocial (Zhou and Zhang, 2004; Xing et al., 2017). Although often described as super-precocial, in fact enantiornithines do not really fit into the neornithine altricial-precocial spectrum, being small arboreal birds that were likely independent and volant upon hatching and thus unlike the extant super-precocial megapodes which are primarily cursorial birds (Xing et al., 2017). A highly precocial developmental strategy is inferred wherever there is evidence of early developmental stages and appears to have been widespread in the Enantiornithes. Such a developmental strategy may have excluded enantiornithines from evolving specialized foot morphologies like those observed in altricial neornithines, thus forcing them to evolve alternative strategies to cope with certain environments, such as the proportionately mediolaterally wide digit observed in F. prehendens.
Although syndactyly has evolved multiple times during avian evolution, it appears to be a synapomorphy of Eucavitaves, so named for their tendency to nest in cavities or burrows, and it occurs most extensively in members of this clade (Yuri et al., 2013). Although this morphology and this behavior have both been secondarily lost in some lineages (e.g., members of Bucerotidae utilizing rotted holes or damaged trunks from large broken branches), it is commonly inferred that syndactyly facilitates active excavation of burrows in soft substrate (Winkler et al., 2020c). The increased plantar surface of the pedes makes for a useful spade during the process of shoveling out dirt, mud, or sand, along with the bill in some members of this group (Winkler et al., 2020b). Notably, some members within the families Gabulidae (Jacamars), and Capitonidae (Barbets) exhibit a modified syndactyl-zygodactyl foot morphology, which aids in the shoveling of substrate during burrow excavation (Hoyo et al., 2002), thus revealing that there may be a positive correlation between pedal syndactyl morphology and burrow excavation across not just eucavitaves, but throughout the neornithines. In most extant examples, when creating a burrow, the foot is positioned either on the parasagittal plane pushing substrate out, or ventrally in relation to the animal, allowing the material to pass underneath the body when excavating. Having the widest portion of the foot located laterally, rather than medially, facilitates this movement of the substrate out of the burrow or cavity in relation to the bird. Although F. prehendens similarly exhibits an enlarged laterally placed plantar surface relative to the morphology found in other enantiornithines, it is unlikely that it utilized this morphology in moving substrate during burrow or cavity construction. Members of the Eucavitates are altricial, their cavities providing added protection for their highly dependent offspring (Hoyo et al., 2002), whereas evidence indicates enantiornithines were precocial and laid their eggs partially exposed in soft sediments near water (Varricchio and Jackson, 2016; Bailleul et al., 2019).
The Hukawng is thought to have been part of the Trans-Tethyan island arc—an isolated island setting, separated from the mainland of what is now the Asian continent (Westerweel et al., 2019). These two species of morphologically specialized enantiornithines further support the prediction that geographically isolated settings (i.e., islands) have the capability to produce an array of unique and specialized fauna, particularly in birds, that exhibit morphologies disparate from other related mainland species (e.g., Hawaiian archipelago, New Guinea, Galapagos Islands) (Pratt, 2005). Like other geographically isolated settings, various niches have been adopted with unique evolutionary responses to environmental pressures. On most continents, picids dominate the wood boring and probing niche, while on isolated islands like the Hawaiian archipelago and the Galapagos islands, this niche has been filled by other bird species either by a hypertrophied hetero-bill (Hemignathus munroi) and even with tool use (Camarhynchus pallidus). Similarly, these isolated Cretaceous Trans-tethyan islands may have been free of boring/probing taxa found on the continent, allowing this niche to become utilized by enantiornithine birds. The unique way in which this niche was utilized by enantiornithines, through an elongated pedal digit, contributes to the diversity of vertebrate probing structures evolved through time.
Although the vast majority of known enantiornithine taxa appear to be arboreal on the basis of pedal morphology, this clade does exhibit ecological diversity. In the Early Cretaceous this diversity can be observed in the variation of cranial and dental morphologies found in the Jehol avifauna (O’Connor and Chiappe, 2011) whereas in the Late Cretaceous diversity is expressed through variation in the tarsometatarsus (Chiappe, 1993). Our revised ethological inferences regarding these two unusual Hukawng birds strengthen the prediction that the enantiornithines were a clade capable of high morphological diversification.
Despite the fact the remains of these two taxa represent only a small portion of each bird, they still exhibit morphologies unique among all previously known avian and non-avian taxa (i.e., elongate pedal digit for probing and mediolaterally enlarged lateral pedal digit presumed for greater stability while perched). Re-evaluation of these taxa helps to create a better understanding of both the biodiversity of the Burmese avifauna and the ways in which enantiornithines adapted to fill ecological roles.
The recognition of these specialized forms also reveals a pattern of avian faunal structure evolution similar to that observed in isolated settings today. Changes in morphology and behavior from mainland counterparts, often times with alterations in the latter affecting the former, further facilitate unique morphological responses allowing for greater ecological niche expansion (Scott et al., 2003; Pratt, 2005). Isolation-caused evolutionary changes, according to Bock (1970), begin with allopatric speciation due to an array of open niches, which gives way to secondary sympatry, and finally results in further diverged speciation events of differing ethologies and ecologies (Bock, 1970; Pratt, 2005). This clear demonstration of unique morphologies and subsequent predicted accompanying behavior in these two enantiornithines, supports the pattern that geographically isolated evolutionary pressures can produce unique physiological adaptations in birds, and that these patterns extended back into the Cretaceous.
Aves Linnaeus 1758
Ornithothoraces Chiappe, 1995
Enantiornithines Walker, 1981
Fortipesavis prehendens gen. et sp. nov. (Figures 3A–C).
YLSNHM01001, external mold of digits II–IV and distalmost portion of left tarsometatarsus preserved in amber (Xing et al., 2019b). Middle-upper Albian, 98.8 ± 0.06 Ma (Wright et al., 1996; Shi et al., 2012; Xing et al., 2019b).
The generic name Fortipesavis, derives from “Fortipes,” Latin compound for strong-footed, “avis,” Latin meaning bird. The specific name “prehendens” comes from the Latin verb to grip or grasp. “Fortipesavis prehendens,” Latin for gripping strong-footed bird.
Small bird with the unique combination of the following features: mediolateral width of digit IV greater than digit III, and that of digit III greater than that of digit II, such that the mediolateral width of digit IV is twice that of digit II (autapomorphy); plantar pads of digit IV mediolaterally wider than they are craniocaudally long and located between phalangeal joints; exaggerated plantar pads of digit IV separated by deep clefts; and digit II ungual long, recurved and sharply tapered.
Taxa utilizing the same ecological niche tend to evolve morphological structures with similar functions that are often superficially reminiscent (e.g., elongated structures used for probing). Morphological differences in functionally homologous structures between groups are likely due to natural selection modifying the morphology of a particular organism, limited by the existing structures of each particular group (e.g., the wings of volant birds cannot be modified into elaborate probing structures). Two enantiornithines known entirely from hindlimb material preserved in mid-Cretaceous amber exhibit unusual morphologies that have no obvious analogs among extant taxa. Here we explore previous predictions regarding the ecological niches of these two taxa, through additional comparative information from a wider range of extant taxa. We provide further support for the inferences regarding the probing ecology of E. chenguangi, for which we consider a wood-probing ecology most likely, and further predict the bill was robust and edentulous allowing this taxon to create openings in hard wood substrate. Crown birds that probe in hard substrates do so with the bill and or tongue, whereas mammals primarily probe with manual digits. Thus, if predictions regarding E. chenguangi are correct, the ecological adaptions for probing utilized by this taxon are unique among all known animals but are more similar to mammalian wood-probers than to extant avians that utilize this niche. Further comparison between raptorial birds and F. prehendens does not support the original interpretation that this species was a predator that relied on its pedes for prey capture. The laterally robust foot morphology is more reminiscent of the syndactyl condition and suggests this species utilized thin, mobile perches that required increased grip and stability. These results help us elucidate habitat structure in the mid-Cretaceous tropical forests of Myanmar. This study supports interpretations that the enantiornithines were ecologically diverse and employed unique morphologies to thrive in roles utilized by birds today. This study demonstrates the utility of comparative data derived from extant taxa in making ecomorphological inferences regarding extinct taxa. Additional discoveries will reveal the accuracy of these interpretations, which will in turn improve the accuracy of future predictions regarding Cretaceous enantiornithine ecology and behavior, specifically in the unusual Burmese avifauna. Continued study and understanding of how ecology shapes morphology in extant taxa will continue to aid paleontologists in understanding the incredible diversity and behavior of extinct organisms.
The original contributions presented in the study are included in the article/Supplementary Material, further inquiries can be directed to the corresponding author/s.
AC was responsible for primary investigation, conceptualization, data curation, and original draft authorship. JO’C was responsible for investigation, methodology, supervision, and draft editor. Both authors contributed to the article and approved the submitted version.
The authors declare that the research was conducted in the absence of any commercial or financial relationships that could be construed as a potential conflict of interest.
We would like to thank Ville Sinkkonen for his work as a paleoartist, and for working with us in order to bring Fortipesavis prehendens to life. We would also like to thank Steve Rogers at the Carnegie Museum in Pittsburgh and the staff within the ornithology collections at the Field Museum at Chicago for access to specimens. We would further like to thank USGS biologist Paul Banko for aid in locating historical Hawaiian honeycreeper measurements. The new taxon’s genus and species catalog number from Zoobank.org is urn:lsid:zoobank.org:pub:C7C3F5C0-CBD8-42C0-A6F2-231CA2D1C0D1.
The Supplementary Material for this article can be found online at: https://www.frontiersin.org/articles/10.3389/fevo.2021.654156/full#supplementary-material
Supplementary Figure 1 | A ternary diagram plotting seven species of similarly-sized Hawaiian honeycreepers. Bt.Diff, bill tip differences; ExC.M, exposed culmen length to mandible length ratio. The large disparity in upper and lower bill morphologies of the H. wilsoni is present even though similar tarsus lengths are fairly consistent throughout the measured species.
Allen, L. L., Morrison, K. L., Scott, W. A. E., Shinn, S., Haltiner, A. M., and Doherty, M. J. (2018). Differences between stance and foot preference evident in Osprey (Pandion haliaetus) fish holding during movement. Brain Behav. 8:e01126. doi: 10.1002/brb3.1126
Arendt, J., and Reznick, D. (2008). Convergence and parallelism reconsidered: what have we learned about the genetics of adaptation? Trends Ecol. Evol. 23, 26–32. doi: 10.1016/j.tree.2007.09.011
Backus, S. B., Sustaita, D., Odhner, L. U., and Dollar, A. M. (2015). Mechanical analysis of avian feet: multiarticular muscles in grasping and perching. R. Soc. Open Sci. 2:140350. doi: 10.1098/rsos.140350
Bailleul, A. M., O’Connor, J., Zhang, S., Li, Z., Wang, Q., Lamanna, M. C., et al. (2019). An Early Cretaceous enantiornithine (Aves) preserving an unlaid egg and probable medullary bone. Nat. Commun 10:1275. doi: 10.1038/s41467-019-09259-x
Bierregaard, R. O., Poole, A. F., Martell, M. S., Pyle, P., and Patten, M. A. (2020). “Osprey (Pandion haliaetus), version 1.0,” in Birds of the World, ed. P. G. Rodewald (Ithaca, NY: Cornell Lab of Ornithology).
Bock, W., and Miller, W. D. (1959). The scansorial foot of the woodpeckers, with comments on the evolution of perching and climbing feet in birds. Am. Mus. Novit. 1931, 1–45.
Bock, W. J. (1970). Microevolutionary sequences as a fundamental concept in macroevolutionary models. Evolution 24, 704–722. doi: 10.2307/2406551
Bock, W. J. (1994). Concepts and methods in ecomorphology. J. Biosci. 19, 403–413. doi: 10.1007/bf02703177
Botelho, J. F., Smith-Paredes, D., and Vargas, A. O. (2015). Altriciality and the evolution of Toe orientation in birds. Evol. Biol. 42, 502–510. doi: 10.1007/s11692-015-9334-7
Casali, D. M., Martins-Santos, E., Santos, A. L. Q., Miranda, F. R., Mahecha, G. A. B., and Perini, F. A. (2017). Morphology of the tongue of Vermilingua (Xenarthra: Pilosa) and evolutionary considerations: casali et al. J. Morphol. 278, 1380–1399. doi: 10.1002/jmor.20718
Chiappe, L., Norell, M., and Clark, J. (2001). A New Skull of Gobipteryx minuta (Aves:Enantiornithines) from the cretaceous of the gobi desert. Am. Mus. Novit. 2001, 1–5. doi: 10.1206/0003-0082(2001)346<0001:ansogm>2.0.co;2
Chiappe, L. M. (1993). Enantiornithine (Aves) tarsometatarsi from the cretaceous lecho formation of Northwest Argentina. Am. Mus. Novit. 3083, 1–27.
Chiappe, L. M., and Walker, C. A. (2002). “Skeletal morphology and systematics of the Cretaceous Euenantiornithines (Ornithothoraces: Enantiornithes),” in Mesozoic birds: Above the Heads of Dinosaurs, eds L. M. Chiappe and L. M. Witmer (Berkeley, CA: University of California Press), 240–267.
Chiappe, L. M., and Witmer, L. M. eds (2002). Mesozoic birds: Above the Heads of Dinosaurs. Berkeley, CA: University of California Press.
Cunningham, S. J., Alley, M. R., Castro, I., Potter, M. A., Cunningham, M., and Pyne, M. J. (2010). bill morphology of ibises suggests a remote-tactile sensory system for prey detection. Auk 127, 308–316. doi: 10.1525/auk.2009.09117
Cunningham, S. J., Corfield, J. R., Iwaniuk, A. N., Castro, I., Alley, M. R., Birkhead, T. R., et al. (2013). the anatomy of the bill tip of kiwi and associated somatosensory regions of the brain: comparisons with shorebirds. PLoS One 8:e80036. doi: 10.1371/journal.pone.0080036
Eduardo, J., Bicudo, P. W., Buttemer, W. A., Chappell, M. A., Pearson, J. T., and Bech, C. (2010). Ecological and Environmental Physiology of Birds. Oxford: Oxford University Press.
Erickson, C. J., Nowicki, S., Dollar, L., and Goehring, N. (1998). Percussive Foraging: stimuli for prey location by aye-ayes (Daubentonia madagascariensis). Int.l J. Primatol. 19, 111–122.
Falk, A. R., Lamsdell, J. C., and Gong, E. (2020). Principal component analysis of avian hind limb and foot morphometrics and the relationship between ecology and phylogeny. Paleobiology 2020, 1–23. doi: 10.1017/pab.2020.39
Feistner, A. T. C., Price, E. C., and Milliken, G. W. (1994). Preliminary observations on hand preference for tapping, digit-feeding and food-holding in captive aye-ayes (Daubentonia madagascariensis). Folia Primatol. 62, 136–141. doi: 10.1159/000156770
Felice, R. N., Tobias, J. A., Pigot, A. L., and Goswami, A. (2019). Dietary niche and the evolution of cranial morphology in birds. Proc. R. Soc. B 286:20182677. doi: 10.1098/rspb.2018.2677
Fowler, D. W., Freedman, E. A., and Scannella, J. B. (2009). Predatory Functional morphology in raptors: interdigital variation in talon size is related to prey restraint and immobilization technique. PLoS One 4:e7999. doi: 10.1371/journal.pone.0007999
Fowler, D. W., Freedman, E. A., Scannella, J. B., and Kambic, R. E. (2011). The predatory ecology of deinonychus and the origin of flapping in birds. PLoS One 6:e28964. doi: 10.1371/journal.pone.0028964
Gill, F. B., Prum, R. O., and Robinson, S. K. (2019). in Ornithology, 4 Edn, ed. W. H. Freeman (New York NY: Macmillan Learning).
Grant, P. (1966). Further Information on the relative length of the tarsus in land birds. post. Peabody Mus. Nat. Hist. 98, 1–13. doi: 10.3838/jjo.47.1
Handasyde, K., and Martin, R. (1996). Field observations of the common striped possum (Dactylopsila trivirgata) in North Queensland. Wildlife Res. 23:755. doi: 10.1071/wr9960755
Höfling, E., and Abourachid, A. (2020). The skin of birds’ feet: morphological adaptations of the plantar surface. J. Morphol. 282, 88–97. doi: 10.1002/jmor.21284
Hopson, J. (2001). “Ecomorphology of avian and nonavian theropod phalangeal proportions: implications for the arboreal versus terrestrial origin of bird flight. New perspectives on the origin and early evolution of birds,” in Proceedings of the International Symposium in Honor of John H, (Paris: Ostrum).
Hoyo, J., del Burn, H., Collar, N., and Fuller, E. (Eds.) (2002). Jacamars to Woodpeckers, Handbook of the Birds of the World. Barcelona: Lynx Edicons.
Hoyo, J., Elliot, A., and Sargatal, J., (Eds.) (2001). Mousebirds to Hornbills, Handbook of the Birds of the World. Barcelona: Lynx Edicons.
Hoyo, J., Elliot, A., Sargatal, J., and Cabot, J. (Eds.) (1992). Handbook of the Birds of the World. Barcelona: Lynx Edicons.
Huang, W.-S. (2006). Ecological characteristics of the skink, Mabuya longicaudata, on a tropical east Asian island. Copeia 2006, 293–300. doi: 10.1643/0045-8511(2006)6[293:ecotsm]2.0.co;2
Jouffroy, F. K. (1975). “Osteology and myology of the lemuriform postcranial skeleton,” in Lemur Biology, eds I. Tattersall and R. W. Sussaman (New York NY: Plenum Press), 149–191. doi: 10.1007/978-1-4684-2121-7_9
Kapoor, B. G., and Bhargava, S. C. (1967). A study on the barbels of a marine catfish, arius thalassinus. Jap. J. Lchthyol. 14, 201–298.
Katzir, G., Berman, D., Nathan, M., and Weihs, D. (2018). Sustained hovering, head stabilization and vision through the water surface in the Pied kingfisher (Cerylerudis). bioRxiv[Preprint] doi: 10.1101/409201
Katzir, G., Schechtman, E., Carmi, N., and Weihs, D. (2001). Head Stabilization in Herons. J. Comparat. Physiol. A 187, 423–432. doi: 10.1007/s003590100210
Kobayashi, K., Kumakura, M., Yoshimura, K., Inatomi, M., and Asami, T. (1998). Fine structure of the tongue and lingual papillae of the penguin. Arch. Histol. Cystol. 61, 37–46. doi: 10.1679/aohc.61.37
Legendre, L. J., and Botha-Brink, J. (2018). Digging the compromise: investigating the link between limb bone histology and fossoriality in the aardvark (Orycteropus afer). PeerJ 6:e5216. doi: 10.7717/peerj.5216
Li, Z., Wang, C.-C., Wang, M., Chiang, C.-C., Wang, Y., Zheng, X., et al. (2020). Ultramicrostructural reductions in teeth: implications for dietary transition from non-avian dinosaurs to birds. BMC Evol. Biol. 20:46.
Losos, J. B., Schoener, T. W., Langerhans, R. B., and Spiller, D. A. (2006). Rapid temporal reversal in predator-driven natural selection. Science (New York, N.Y.) 314:1111. doi: 10.1126/science.1133584
Louchart, A., and Viriot, L. (2011). From snout to beak: the loss of teeth in birds. Trends Ecol Evol 26:663–673. doi: 10.1016/j.tree.2011.09.004
Lovette, I. J., and Fitzpatrick, J. W. eds (2016). Handbook of Bird Biology, 3 Edn. Nashville, TN: John Wiley & Sons.
McCall, R. A., Nee, S., and Harvey, P. H. (1998). The role of wing length in the evolution of avian flightlessness. Evol. Ecol. 12, 569–580. doi: 10.1023/a:1006508826501
Milliken, G. W., Ward, J. P., and Erickson, C. J. (1991). Independent digit control in foraging by the aye-aye (Daubentonia madagascariensis). Folia Primatologica 56, 219–224. doi: 10.1159/000156551
Morris, P. J. R., Cobb, S. N. F., and Cox, P. G. (2018). Convergent evolution in the Euarchontoglires. Biol. Lett. 14:20180366. doi: 10.1098/rsbl.2018.0366
Nudds, R. L., and Davidson, J. S. (2010). A shortening of the manus precedes the attenuation of other wing-bone elements in the evolution of flightlessness in birds. Acta Zool. 91, 115–122. doi: 10.1111/j.1463-6395.2009.00391.x
Ochs, M. F., Zamani, M., Gomes, G. M. R., de Oliveira Neto, R. C., and Kane, S. A. (2017). Sneak peek: raptors search for prey using stochastic head turns. Auk 134, 104–115. doi: 10.1642/auk-15-230.1
O’Connor, J. K. (2019). The trophic habits of early birds. palaeogeography, palaeoclimatology. Palaeoecology 513, 178–195. doi: 10.1016/j.palaeo.2018.03.006
O’Connor, J. K., and Chiappe, L. M. (2011). A revision of enantiornithine (Aves: Ornithothoraces) skull morphology. J. Syst. Palaeontol. 9, 135–157. doi: 10.1080/14772019.2010.526639
O’Connor, J. K., Li, D.-Q., Lamanna, M. C., Wang, M., Harris, J. D., Atterholt, J., et al. (2016a). A new early cretaceous enantiornithine (Aves, Ornithothoraces) from northwestern China with elaborate tail ornamentation. J. Vertebrate Paleontol. 36:e1054035. doi: 10.1080/02724634.2015.1054035
O’Connor, J. K., Wang, X., Chiappe, L. M., Gao, C., Meng, Q., Cheng, X., et al. (2009). Phylogenetic support for a specialized clade of cretaceous enantiornithine birds with information from a new species. J. Vertebrate Paleontol. 29, 188–204. doi: 10.1080/02724634.2009.10010371
O’Connor, J. K., Wang, X., Zheng, X., Hu, H., Zhang, X., and Zhou, Z. (2016b). An Enantiornithine with a fan-shaped tail, and the evolution of the rectricial complex in early birds. Curr. Biol. 26, 114–119. doi: 10.1016/j.cub.2015.11.036
O’Connor, J. K., Zhang, Y., Chiappe, L. M., Meng, Q., Quanguo, L., and Di, L. (2013). A new enantiornithine from the yixian formation with the first recognized avian enamel specialization. J. Vertebrate Paleontol. 33, 1–12. doi: 10.1080/02724634.2012.719176
O’Connor, P. M., Turner, A. H., Groenke, J. R., Felice, R. N., Rogers, R. R., Krause, D. W., et al. (2020). Late cretaceous bird from Madagascar reveals unique development of beaks. Nature 588, 272–276.
Pearce, T. (2012). Convergence and parallelism in evolution: a neo-gouldian account. Br. J. Phil. Sci. 63, 429–448. doi: 10.1093/bjps/axr046
Perkins, R. C. L. (1903). in Fauna Hawaiiensis, Vertebrata, Vol. I, ed. D. Sharp (Cambridge: Cambridge University Press).
Ralph, C. J., and Fancy, S. G. (1996). Aspects of the life history and foraging ecology of the endangered akiapolaau. Condor 98, 312–321. doi: 10.2307/1369149
Schulenberg, T. (1983). Foraging behavior, eco-morphology, and systematics of some antshrikes (Formicariidae: Thamnomanes). Wilson Bull. 95, 505–521.
Scott, S. N., Clegg, S. M., Simon, P. B., Kikkawa, J., and Owens, P. F. (2003). Morphological shifts in island-dwelling birds: the roles of generalist foraging and niche expansion. Evolution 57, 2147–2156. doi: 10.1554/03-021
Sesoko, N. F., Rahal, S. C., Bortolini, Z., de Souza, L. P., Vulcano, L. C., Monteiro, F. O. B., et al. (2015). SKELETAL MORPHOLOGY OF THE FORELIMB OF MYRMECOPHAGA TRIDACTYLA. J. Zoo Wildlife Med. 46, 713–722.
Shi, G. G., Harlow, G. E., Wang, J., Wang, J., Yang, M., Yang, M., et al. (2012). Age constraint on Burmese amber based on U-Pb dating of zircons. Cretac. Res. 37, 155–163. doi: 10.1016/j.cretres.2012.03.014
Simon, J. C., Baker, P. E., and Baker, H. (2020). “Maui Parrotbill (Pseudonestor xanthophrys), version 1.0,” in Birds of the World, eds A. F. Poole and F. B. Gill (Ithaca, NY: Cornell Lab of Ornithology).
Soligo, C. (2005). Anatomy of the hand and arm in daubentonia madagascariensis:a functional and phylogenetic outlook. Folia Primatol. 76, 262–300. doi: 10.1159/000088034
Stayton, C. T. (2008). Is convergence surprising? An examination of the frequency of convergence in simulated datasets. J. Theor. Biol. 252, 1–14. doi: 10.1016/j.jtbi.2008.01.008
Tsang, L. R., and McDonald, P. G. (2018). A comparative study of avian pes morphotypes, and the functional implications of Australian raptor pedal flexibility. Emu Austral. Ornithol. 119, 14–23. doi: 10.1080/01584197.2018.1483203
Tsang, L. R., Wilson, L. A. B., Ledogar, J., Wroe, S., Attard, M., and Sansalone, G. (2019). Raptor talon shape and biomechanical performance are controlled by relative prey size but not by allometry. Sci. Rep. 9:7076.
Van Dyck, S. (1983). in Striped Possum. Complete Book of Australian Mammals, ed. R. Strahan (Sydney: The Australian Museum of Angus and Robertson), 144.
Varricchio, D. J., and Jackson, F. D. (2016). Reproduction in Mesozoic birds and evolution of the modern avian reproductive mode. Auk 133, 654–684. doi: 10.1642/AUK-15-216
Wainwright, P. C. (1991). Ecomorphology: experimental functional anatomy for ecological problems. Am. Zool. 31, 680–693. doi: 10.1093/icb/31.4.680
Walker, C. A. (1981). New subclass of birds from the Cretaceous of South America. Nature 292, 51–53. doi: 10.1038/292051a0
Wallman, J., and Letelier, J.-C. (1993). “Eye movements, head movements, and gaze stabilization in birds,” in Vision, Brain, and Behavior in Birds, eds H. P. Zeigler and H.-J. Bischof (Cambridge MA: The MIT Press), 245–263.
Wang, M., and Zhou, Z. (2017). A morphological study of the first known piscivorous enantiornithine bird from the Early Cretaceous of China. J. Vertebrate Paleontol. 37:e1278702. doi: 10.1080/02724634.2017.1278702
Wang, M., Zhou, Z.-H., O’Connor, J. K., and Zelenkov, N. V. (2014). A new diverse enantiornithine family (Bohaiornithidae fam. nov.) from the Lower Cretaceous of China with information from two new species. Vertebrata PalAsiatica. 2014, 31–76.
Westerweel, J., Roperch, P., Licht, A., Dupont-Nivet, G., Win, Z., Poblete, F., et al. (2019). Burma Terrane part of the trans-tethyan arc during collision with India according to palaeomagnetic data. Nat. Geosci. 12, 863–868. doi: 10.1038/s41561-019-0443-2
Winkler, D. W., Billerman, S. M., and Lovette, I. J. (2020a). “Woodpeckers (Picidae), version 1.0,” in Birds of the World, eds S. M. Billerman, B. K. Keeney, P. G. Rodewald, and T. S. Schulenberg (Ithaca, NY: Cornell Lab of Ornithology).
Winkler, D. W., Billerman, S. M., and Lovette, I. J. (2020b). “Kingfishers (Alcedinidae), version 1.0,” in Birds of the World, eds S. M. Billerman, B. K. Keeney, P. G. Rodewald, and T. S. Schulenberg (Ithaca, NY: Cornell Lab of Ornithology).
Winkler, D. W., Billerman, S. M., and Lovette, I. J. (2020c). “Grebes (Podicipedidae), version 1.0,” in Birds of the World, eds S. M. Billerman, B. K. Keeney, P. G. Rodewald, and T. S. Schulenberg (Ithaca, NY: Cornell Lab of Ornithology).
Winkler, D. W., Billerman, S. M., and Lovette, I. J. (2020d). “Bee-eaters (Meropidae), version 1.0,” in Birds of the World, eds S. M. Billerman, B. K. Keeney, P. G. Rodewald, and T. S. Schulenberg (Ithaca, NY: Cornell Lab of Ornithology).
Winkler, D. W., Billerman, S. M., and Lovette, I. J. (2020e). “Hornbills (Bucerotidae), version 1.0,” in Birds of the World, eds S. M. Billerman, B. K. Keeney, P. G. Rodewald, and T. S. Schulenberg (Ithaca, NY: Cornell Lab of Ornithology).
Winkler, D. W., Billerman, S. M., and Lovette, I. J. (2020f). “Asian and grauer’s Broadbills (Eurylaimidae), version 1.0,” in Birds of the World, eds S. M. Billerman, B. K. Keeney, P. G. Rodewald, and T. S. Schulenberg (Ithaca, NY: Cornell Lab of Ornithology).
Winkler, D. W., Billerman, S. M., and Lovette, I. J. (2020g). “African and green broadbills (Calyptomenidae), version 1.0,” in Birds of the World, eds S. M. Billerman, B. K. Keeney, P. G. Rodewald, and T. S. Schulenberg (Ithaca, NY: Cornell Lab of Ornithology).
Wright, C. W., Callomon, J. H., and Howarth, M. K. (1996). “Cretaceous ammonoidea,” in Treatise on Invertebrate Paleontology. Part I. Mollusca 4 (Revised), ed. R. L. Kaesler (Boulder: Geological Society of America), 362.
Xing, L., Cockx, P., and McKellar, R. C. (2020a). Disassociated feathers in Burmese amber shed new light on mid-Cretaceous dinosaurs and avifauna. Gondwana Res. 82, 241–253. doi: 10.1016/j.gr.2019.12.017
Xing, L., Cockx, P., McKellar, R. C., and O’Connor, J. (2018a). Ornamental feathers in Cretaceous Burmese amber: resolving the enigma of rachis-dominated feather structure. J. Palaeogeogr. 7:13.
Xing, L., Cockx, P., O’Connor, J. K., and Mckellar, R. C. (2020b). A newly discovered enantiornithine foot preserved in mid-Cretaceous Burmese amber. Palaeoentomology 3, 212–219. doi: 10.11646/palaeoentomology.3.2.11
Xing, L., McKellar, R. C., and O’Connor, J. K. (2020c). An unusually large bird wing in mid-Cretaceous Burmese amber. Cretac. Res. 110:104412. doi: 10.1016/j.cretres.2020.104412
Xing, L., McKellar, R. C., O’Connor, J. K., Bai, M., Tseng, K., and Chiappe, L. M. (2019a). A fully feathered enantiornithine foot and wing fragment preserved in mid-Cretaceous Burmese amber. Sci. Rep. 9:927.
Xing, L., McKellar, R. C., O’Connor, J. K., Niu, K., and Mai, H. (2019b). A mid-Cretaceous enantiornithine foot and tail feather preserved in Burmese amber. Sci. Rep. 9:15513.
Xing, L., McKellar, R. C., Wang, M., Bai, M., O’Connor, J. K., Benton, M. J., et al. (2016). Mummified precocial bird wings in mid-Cretaceous Burmese amber. Nat. Commun. 7:12089.
Xing, L., O’Connor, J. K., Chiappe, L. M., McKellar, R. C., Carroll, N., Hu, H., et al. (2019c). A new enantiornithine bird with unusual pedal proportions found in Amber. Curr. Biol. 29:2594. doi: 10.1016/j.cub.2019.07.042
Xing, L., O’Connor, J. K., McKellar, R. C., Chiappe, L. M., Bai, M., Tseng, K., et al. (2018b). A flattened enantiornithine in mid-Cretaceous Burmese amber: morphology and preservation. Sci. Bull. 63, 235–243. doi: 10.1016/j.scib.2018.01.019
Xing, L., O’Connor, J. K., McKellar, R. C., Chiappe, L. M., Tseng, K., Li, G., et al. (2017). A mid-Cretaceous enantiornithine (Aves) hatchling preserved in Burmese amber with unusual plumage. Gondwana Res. 49, 264–277. doi: 10.1016/j.gr.2017.06.001
Xing, L., O’Connor, J. K., Niu, K., Cockx, P., Mai, H., and McKellar, R. C. (2020d). A New Enantiornithine (Aves) Preserved in Mid Cretaceous Burmese amber contributes to growing diversity of cretaceous plumage patterns. Front. Earth Sci. 8:264.
Yuri, T., Kimball, R., Harshman, J., Bowie, R., Braun, M., Chojnowski, J., et al. (2013). Parsimony and Model-Based Analyses of Indels in avian nuclear genes reveal congruent and incongruent phylogenetic signals. Biology 2, 419–444. doi: 10.3390/biology2010419
Zeffer, A., Johansson, C., and Marmebro, A. (2002). Functional correlation between habitat use and leg morphology in birds (Aves). Biol. J. Linn. Soc. 79, 461–484. doi: 10.1046/j.1095-8312.2003.00200.x
Zeffer, A., and Norberg, M. (2002). Leg morphology and locomotion in birds: requirements for force and speed during ankle flexion. J. Exp. Biol. 206, 1085–1097. doi: 10.1242/jeb.00208
Zhang, F., and Zhou, Z. (2000). A primitive enantiornithine bird and the origin of feathers. Science 290, 1955–1959. doi: 10.1126/science.290.5498.1955
Zhang, F., Zhou, Z., Hou, L., and Gu, G. (2001). Early diversification of birds: Evidence from a new opposite bird. Kexue tongbao [Chin. Sci. Bull.] 46, 945–949. doi: 10.1007/bf02900473
Zhou, Z., and Wang, Y. (2010). Vertebrate diversity of the Jehol Biota as compared with other lagerstätten. Sci. China Earth Sci. 53, 1894–1907. doi: 10.1007/s11430-010-4094-9
Zhou, Z., and Zhang, F. (2004). A Precocial avian embryo from the lower cretaceous of china. Science 306, 653–653. doi: 10.1126/science.1100000
Zhou, Z., and Zhang, F. (2007). Mesozoic birds of China—a synoptic review. Front. Biol. China 2, 1–14. doi: 10.1007/s11515-007-0001-y
Keywords: enantiornithines, Elektorornis, ecomorphology, Burmese amber, morphology, pedal morphology
Citation: Clark AD and O’Connor JK (2021) Exploring the Ecomorphology of Two Cretaceous Enantiornithines With Unique Pedal Morphology. Front. Ecol. Evol. 9:654156. doi: 10.3389/fevo.2021.654156
Received: 15 January 2021; Accepted: 19 April 2021;
Published: 15 June 2021.
Edited by:
Nathalie Bardet, UMR 7207 Centre de Recherche sur la Paléobiodiversité et les Paléoenvironnements (CR2P), FranceReviewed by:
Delphine Angst, University of Bristol, United KingdomCopyright © 2021 Clark and O’Connor. This is an open-access article distributed under the terms of the Creative Commons Attribution License (CC BY). The use, distribution or reproduction in other forums is permitted, provided the original author(s) and the copyright owner(s) are credited and that the original publication in this journal is cited, in accordance with accepted academic practice. No use, distribution or reproduction is permitted which does not comply with these terms.
*Correspondence: Alexander D. Clark, YWMwMDA0MEBtaXgud3Z1LmVkdQ==
Disclaimer: All claims expressed in this article are solely those of the authors and do not necessarily represent those of their affiliated organizations, or those of the publisher, the editors and the reviewers. Any product that may be evaluated in this article or claim that may be made by its manufacturer is not guaranteed or endorsed by the publisher.
Research integrity at Frontiers
Learn more about the work of our research integrity team to safeguard the quality of each article we publish.