- 1Division of Epigenetics, DKFZ-ZMBH Alliance, German Cancer Research Center (DKFZ), Heidelberg, Germany
- 2Evonik Operations GmbH, Hanau, Germany
- 3Independent Researcher, Weidenberg, Germany
- 4Independent Researcher, Kassel, Germany
- 5Cancer Drug Development, German Cancer Research Center (DKFZ), German Cancer Consortium (DKTK), Heidelberg, Germany
The marbled crayfish (Procambarus virginalis) is a recently discovered freshwater crayfish species, which reproduces by apomictic parthenogenesis, resulting in a monoclonal, and all-female population. The animals were widely distributed through the aquarium trade and have established numerous stable wild populations through anthropogenic releases. They are highly prevalent in Madagascar, where they have become a popular source of nutritional protein. As freshwater crayfish aquaculture in open systems is a thriving, but ecologically damaging global industry, alternatives are urgently needed. Although marbled crayfish are often branded by their invasive mode of reproduction, their overall invasiveness is not higher than for other cultured crayfish species. Furthermore, their resiliency and high adaptability provide a strong rationale for evaluating them for closed, and environmentally safe aquaculture approaches. Here we describe a novel population of marbled crayfish in a former German coal mining area that is characterized by acid and polluted water. Even under these adverse conditions, animals grew to sizes, and weights that are comparable to commercially farmed freshwater crayfish. Tailored feed development and laboratory testing demonstrated highly efficient feed conversion, suggesting a considerable capacity for sustainable production in closed systems. We further show that marbled crayfish meat can be readily introduced into European meals. Finally, chemical analysis of marbled crayfish exoskeletons revealed comparably high amounts of chitin, which is a valuable source for the synthesis of chitosan and bioplastics. Our results thus suggest that production of marbled crayfish in closed systems may represent a sustainable alternative for crayfish aquaculture.
Introduction
The marbled crayfish (Procambarus virginalis) is a recently discovered freshwater crayfish species that emerged in the aquarium trade about 25 years ago (Scholtz et al., 2003; Lyko, 2017). The animals quickly established themselves as popular ornamental pets, which promoted their wide distribution (Patoka et al., 2014; Faulkes, 2015). Notably, the marbled crayfish is the only known crayfish species that reproduces by obligate apomictic parthenogenesis, a mechanism that results in the formation of an all-female, and globally monoclonal population (Martin et al., 2007; Vogt et al., 2008; Gutekunst et al., 2018; Hossain et al., 2018). Through anthropogenic releases, marbled crayfish have been introduced to various freshwater systems, where they have formed numerous stable populations (Chucholl et al., 2012). Stable wild populations of marbled crayfish have been described in a diverse range of habitats (Andriantsoa et al., 2019; Maiakovska et al., 2021). The animals have also shown the capacity to rapidly form large colonies consisting of 100 of 1,000 of animals (Andriantsoa et al., 2019; Maiakovska et al., 2021). High tolerance to various habitat parameters and high population densities are important features of invasive species.
While the introduction of marbled crayfish raises considerable ecological concerns, it also creates opportunities for human exploitation. This is exemplified by the spread of the animals on Madagascar, where their distribution area has increased 100-fold over 10 years (Jones et al., 2009; Kawai et al., 2009; Gutekunst et al., 2018). This dramatic increase was largely fueled by anthropogenic distribution, as marbled crayfish have developed into a valuable source of dietary protein (Andriantsoa et al., 2019, 2020). However, their potential benefits need to be carefully balanced against their potential negative ecological impacts (Vogt, 2021). And while marbled crayfish are subject to regulation in the European Union (Regulation No. 1143/2014 on the prevention and management of the introduction, and spread of invasive alien species), the corresponding measures have been found to be ineffective (Patoka et al., 2018). As such, it is important to develop ecologically safe frameworks for the culture of marbled crayfish.
Freshwater crayfish are increasingly popular livestock for aquaculture with a global value that now exceeds 10 billion US dollars (FAO, 2016). They are a rich source of nutritional protein, which contributes to their high global demand. Although there are more than 670 known crayfish species (Crandall and De Grave, 2017), aquaculture production is established for only a small number of them, including the noble crayfish (Astacus astacus), the narrow-clawed crayfish (Pontastacus leptodactylus), the red swamp crayfish (Procambarus clarkii), the signal crayfish, (Pacifastacus leniusculus), the yabby (Cherax destructor), the red claw (Cherax quadricarinatus), and the marron (Cherax tenuimanus). P. clarkii, which is native to Mexico and United States (Loureiro et al., 2015), now accounts for more than 95% of the global crayfish aquaculture production and its production volume has rapidly increased over the past decade (FAO, 2016). P. clarkii aquaculture is usually done in rice fields, with a target size of 20–25 grams and is based on the omnivorous feeding patterns of the animals (Wang et al., 2018). China currently produces and consumes most of the farmed P. clarkii, and with a production of more than 720,000 tons in 2015 (Wang et al., 2018).
However, P. clarkii is considered highly invasive and the farming of the animals in open culture has created significant ecological problems, including competition with other species, and the potential transmission of pathogens (Gherardi, 2006; Putra et al., 2018; Haubrock et al., 2021). Furthermore, animals that are farmed in open culture can be dispersed by birds, which further increases their invasive spread (Anastácio et al., 2014). Alternative species and approaches are therefore urgently needed. A transition to closed-system aquaculture, which is ecologically safe, would require the development of a corresponding technical solution. This includes tailored feeds that meet specific needs of the cultured animals, while targeting specific production parameters (e.g., growth, feed efficiency, and economics). Fish meal is often used as a preferred source of dietary protein in aquaculture, but its use is ecologically unfavorable. Plant-based ingredients, such as soybean and rapeseed meal, are common alternatives, but they lack methionine and lysine. Methionine is a limiting amino acid for many animals and its deficiency can directly affect animal growth (Bulbul et al., 2015). Supplementation with crystalline amino acids is therefore required to meet the amino acid demands of aquaculture livestock, including crustaceans (Nunes et al., 2014; Tan et al., 2018). As inadequate protein and feed utilization causes high ammonia production, tailored feeds also contribute to maintaining high water quality (Cowey and Walton, 1989; Council, 2011). However, marbled crayfish are currently fed with various empirically determined diets and tailored feeds are not available.
The marbled crayfish is very closely related to P. clarkii (Owen et al., 2015; Lyko, 2017), indicating that it may represent a viable alternative as an aquaculture livestock. And while it has been argued that poor growth and conservation concerns should preclude any further consideration (Vogt, 2021), marbled crayfish culture conditions have not been optimized yet, and their potential for closed-system aquaculture has not been developed. Here we present data showing that that the animals can grow to sizes that match and exceed the commercial target weight of P. clarkii. We also developed tailored feeds for closed-system aquaculture and found that feed conversion was highly efficient. Furthermore, we show that marbled crayfish exoskeletons are rich in chitin, an important raw material for the production of biodegradable plastics. Our study thus defines an integrated approach toward the sustainable utilization of marbled crayfish.
Materials and Methods
Animal Collection and Morphometric Analysis
Marbled crayfish (N = 768) were collected between October 2019 and September 2020 by hand catching at Murner See, Germany (49.348875N and 12.206505E). The crayfish plague infection status of 20 animals from the population was molecularly tested by local authorities and found to be negative. Visual inspection showed that animals were generally in good condition, without any detectable damage. Total length (rostrum to uropods) was measured using a 15 cm caliper. After standard excess water removal, animal wet weight was determined (in grams) using an SJS 60007 scale (BASETech). Data were grouped and plotted in histograms. Chemical water analysis was provided by Raiffeisen–Laborservice (Ormont, Germany).
Determination of Amino Acid Requirements Using Factorial Modeling
The amino acid profile of marbled crayfish (laboratory stock, DKFZ Heidelberg) was determined using ion-exchange chromatography (AMINOLab®, Evonik Nutrition and Care, Germany), except for tryptophan, and which was estimated using high-performance liquid chromatography (HPLC). In addition, the amino acid content of a commonly used marbled crayfish feed (NovoPleco, JBL, Neuhofen, Germany), which was used as a control feed (D1), was also analyzed. Amino acid requirements were calculated with a factorial modeling approach (Council, 2011) and are presented in Supplementary Table 1 on digestible basis and total basis, and assuming 85% digestibility for amino acids (Nunes et al., 2014). The utilization of different amino acids absorbed across the gut was assumed to be 50–60% and the maintenance requirements for different amino acids were considered to be 15–25% of the amino acids absorbed (i.e., on digestible basis).
Experimental Diets
The detailed ingredient and nutrient composition of the tailored feeds in this study are provided in Supplementary Table 1. A basal feed was formulated using soybean meal, soy protein concentrate, fish meal and krill meal as the main protein sources, and contained 29% crude protein, and 18.31 MJ/kg gross energy. The amino acid profile of the diet (Supplementary Table 1) was balanced (except for methionine) considering the requirements predicted by the factorial modeling approach. The basal tailored feed (D2) was formulated with low levels of methionine (0.45%) and methionine + cysteine (0.86%) and then supplemented with increasing levels of methionine dipeptide (AQUAVI® Met-Met): 0.07% (D3), 0.15% (D4), and 0.25% (D5). As such, D2 represents a methionine-deficient tailored feed, while D3 is a tailored feed with a methionine level that was matched to the (non-tailored) control feed. D4 was matched to the amino acid profile of the crayfish, while D5 was designed to have a methionine surplus. Feed contents were validated by amino acid analysis (Supplementary Table 1). The D2–D5 feeds were produced in pellets of 2 mm size.
Laboratory Testing of Tailored Feeds
In total, 100 adolescent animals (mean total length: 1.75 cm, SD: 0.25 cm; mean weight: 0.11 g, and SD: 0.05 g) were fed for 3 months. Per feed, 20 animals were kept in 4 tanks (25.6 × 18.1 × 13.6 cm), with five animals per tank, at 20°C under natural daylight. The animals were fed daily at 17:00 with 0.08 g of feed, as higher amounts of feed were refused by the animals. Daily mean feed intake per tank was calculated by dividing the amount of feed placed in a tank on a given day by the number of animals surviving on that day. The calculated daily mean feed intake value was totaled over the entire experimental period to calculate the total mean feed intake in each tank. Severely injured (e.g., loss of both chelae) or dead animals were removed from the trial immediately to prevent confounding effects related to cannibalistic feeding. All animals were weighed once per week (independently of their molt frequencies) to determine weight gains over time. Water parameters (Supplementary Table 2) were monitored daily for temperature and weekly for NH4, NO3, NO2, and O2 (JBL Test sets, Neuhofen, Germany). After the weekly measurement of chemical water parameters, water was exchanged with tap water of the same temperature.
Data Analysis
Survival probabilities for all animals and the groups D1–D5 were calculated in R using the CRAN packages survival (version 2.44-1.1) and survminer (version 0.4.6). Kaplan–Meier plots were generated, and survival probabilities were calculated for each feed group and for all animals (Supplementary Figure 1). To investigate differences in survival between feeds pairwise p-values were calculated using a log-rank test. Growth curves were plotted for the five different feeds (D1–D5) by the average weight of animals distributed over 14 weeks. To statistically evaluate the different feeds, we built a generalized estimating equation model (GEE) as implemented in the geeglm function of the R package geepack. The model considers weight gain per feed over the time of 14 weeks and accounts for an autoregressive first-order correlation structure with a Gaussian error distribution, and link function. The Wald statistic was used to estimate levels of significance.
The mean weight gain per tank was determined by calculating the mean final body weight per tank minus the mean initial body weight per tank. Feed conversion ratios (FCR) were calculated as:
Mean daily feed intakes (FI) were calculated as:
To account for different feeding behaviors within a group of animals, we calculated mean values for daily feed intake. These values were summed for all days to calculate the total mean feed intake per animal. Subsequently, mean FCRs were calculated for each trial group. Data was checked for normality by using the Shapiro–Wilk test (W = 0.93947, p = 0.2579). Homogeneity of variances was checked by Bartlett’s test (Bartlett’s K-squared = 1.5781, p = 0.8127) before applying parametric tests. The R internal function aov was used with the model of feed conversion ratio by feed (RES = 0.731). As the total variances between groups were statistically significant (p-value = 0.00239) a more detailed pairwise comparison between all groups was performed using the Tukey HSD test in R.
Isolation of Chitin
The following procedure is based on slightly modified literature protocols (Percot et al., 2003a,b) to remove CaCO3 and protein from shellfish shells. Peeled exoskeletons from pre-cooked marbled crayfish (wild catch from Murner See, Germany, TL 4–14 cm) and the current industry standard, the whiteleg shrimp (Litopenaeus vannamei, commercially obtained from Scheck-in Center, Heidelberg, Germany, TL 8–15 cm) were collected, and air dried. Exoskeletons were subsequently ground to powder with a Thermomix® (level 8, 30 s). From each species, 7 g of powdered exoskeletons were weighed out and added portion-wise to a magnetically stirred solution of 1 M HCl (50 mL) in a wide necked 250 mL Erlenmeyer flask at 23°C. Note: care must be taken to add the material slowly as vigorous foaming occurs, which can cause overflowing. After the addition was complete, an additional 10 mL of 1 M HCl was used to rinse the sides of the flask to ensure all material was submerged. After 75 min, the resulting slurry was vacuum filtered through a porosity #3 sintered glass Büchner funnel using a membrane pump as a source of vacuum. The solid was repeatedly rinsed with deionized H2O (6 × 50 mL) until the filtrate was neutral to pH indicator paper. After air drying with vacuum on the filter for 15 min, the powder was transferred to a 250 mL Erlenmeyer flask containing 1 M NaOH (75 mL), where it was magnetically stirred at 23°C. After 24 h, the resulting slurry was vacuum filtered through a porosity #3 sintered glass Büchner funnel as before. The solid was repeatedly rinsed with deionized H2O (6 × 50 mL) until the filtrate was neutral to pH indicator paper. The resulting solid was air dried with vacuum for ∼20 min, transferred to a 100 mL round bottom flask, dried under high vacuum (∼5 x 10–2 mbar) for 24 h, and weighed on an analytical balance. This protocol was performed in triplicate for each species.
Results
Sizes and Weights of Marbled Crayfish From an Oligotrophic Lake
Murner See in Germany is a former brown coal mining site that was flooded in 1982 to create a recreational lake with a water surface of 94 ha (Figure 1A). Water quality is characterized by substantial acidity and pollution with Aluminum and Manganese (Figure 1B). As a consequence, the lake is very oligotrophic, with limited amounts of plant detritus, mosses, leaves, and insect larvae available for the support of aquatic life. Nevertheless, the lake supports a large marbled crayfish colony (Figure 1C). To characterize this population, 768 marbled crayfish were collected by hand catching and their total lengths, and weights were determined. This revealed that the majority of animals had sizes between 4 and 12 cm (Figure 2A). Weights usually ranged from 5 to 30 g, with several animals weighing > 35 g (Figure 2B). Furthermore, wild-caught animals that were transferred to a laboratory culture system continued to grow noticeably (Figure 2C), suggesting considerable potential for commercial aquaculture. Systematic variation of key parameters, such as water temperature, stocking density, and feeding will be required to fully evaluate the production potential of marbled crayfish.
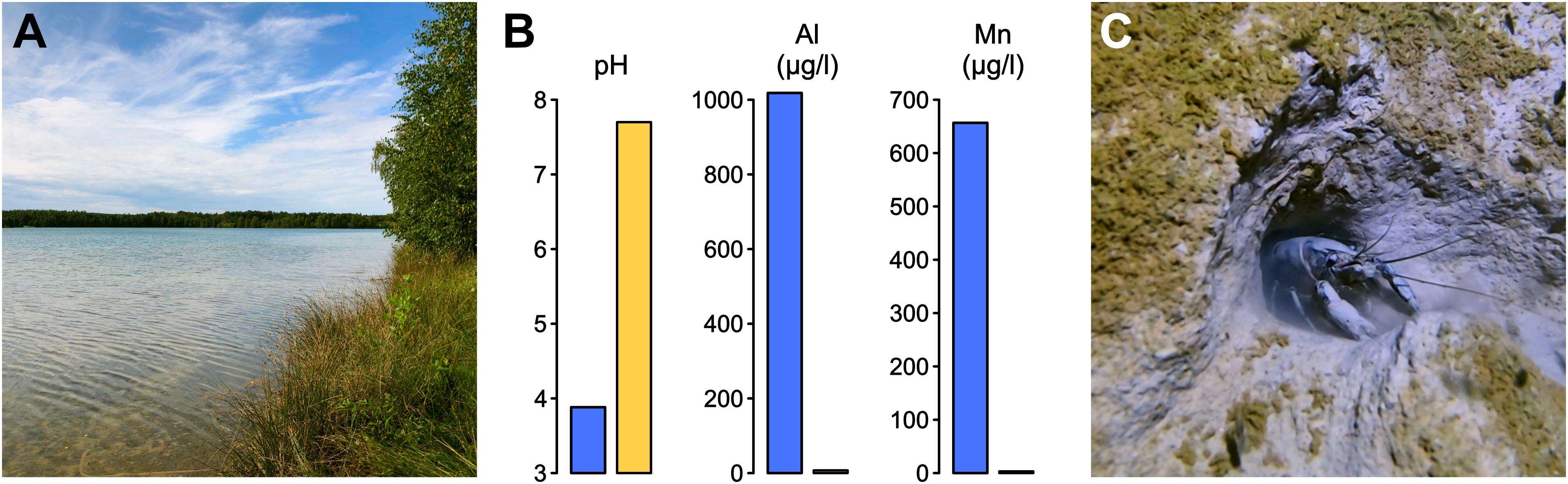
Figure 1. (A) Murner See, a German lake with a stable marbled crayfish population. The lake floods a former coal mining area. (B) Chemical water parameters of Murner See (blue bars). Comparative values are indicated for Krumme Lanke (yellow bars), another representative marbled crayfish habitat (Maiakovska et al., 2021). (C) Underwater photograph from Murner See, with a marbled crayfish specimen in front of a burrow.
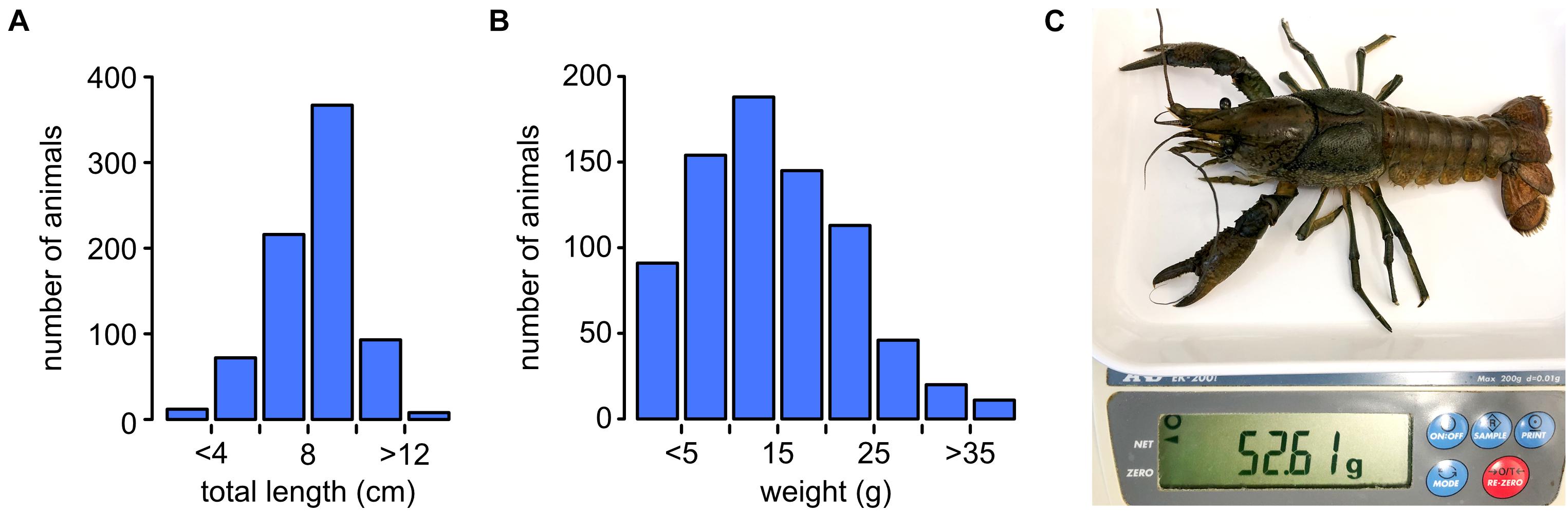
Figure 2. Size and weight of marbled crayfish. (A) Total length distribution of 768 marbled crayfish collected at Murner See. (B) Weight distribution of 768 marbled crayfish collected at Murner See. (C) A wild-caught and laboratory-reared specimen with a total length of 13.2 cm, and a weight of 52.61 g.
Formulation and Testing of Tailored Feeds
To support the development of a closed marbled crayfish culture system, we used a factorial modeling approach to formulate tailored feeds (see section “Materials and Methods” for details). These feeds were then used to determine the optimum level of methionine supplementation for marbled crayfish growth (Supplementary Table 1). We subsequently tested the tailored feeds in multiple independent groups of adolescent marbled crayfish (N = 100) over 3 months (see section “Materials and Methods” for details). Survival analysis showed no significant differences between the groups (Supplementary Figure 1), thus permitting direct comparisons. Analysis of size and weight data revealed a noticeable effect of the tailored feeds on the growth of the animals: when compared to the non-tailored control feed (D1), feed D3 (tailored, matched methionine) resulted in a significantly (p = 0.02, GEE model) increased weight gain (Figure 3). A comparison of all tailored feeds showed that animals fed with D5 (surplus methionine) and D3 (methionine matched to control) showed a faster weight gain compared to D2 (methionine deficient), and D4 (methionine matched to requirement). During the early stages of the trial (until week 8), D5 showed the best performance, and while D3 showed the best performance during the late stages of the trial (Figure 3). These findings demonstrate the importance of tailored feeds and proper methionine supplementation for the optimum growth of marbled crayfish.
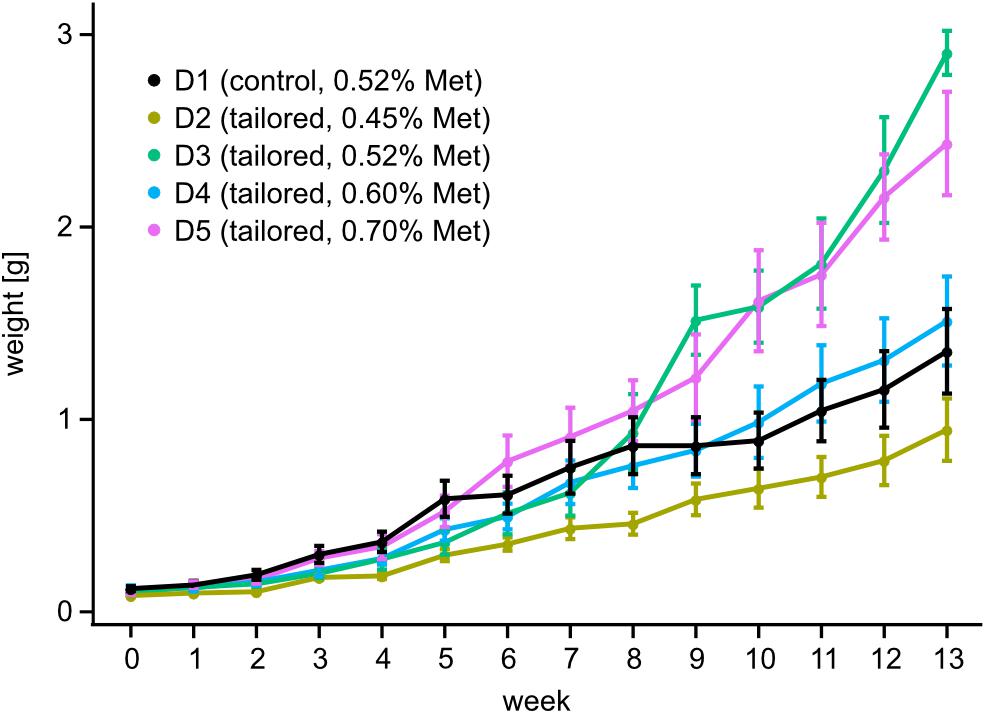
Figure 3. Growth of laboratory-cultured, adolescent marbled crayfish fed with tailored feeds. Growth curves are shown for diets D1–D5, with different methionine concentrations, as indicated. A generalized estimating equations model revealed a statistically significant weight gain for diet D3 (p = 0.02) and D5 (0.03) when compared to diet D1. Error bars indicate the standard error.
Determination of Feed Conversion Ratios
At the end of the trial, a mean weight gain of 2.81 g was observed for the tailored diet D3, while a mean gain of 1.43 g was observed for the control diet D1 (Table 1). Subsequent determination of FCR revealed that the FCR for D3 was significantly (p < 0.05, ANOVA, and RES = 0.731) better than for D1 (1.38–2.41, Table 1). These results again illustrate the superior performance of tailored feeds. Also, the methionine-deficient diet D2 showed the poorest FCR among all feeds (Table 1), which illustrates the importance of methionine supplementation for marbled crayfish feeds. The best FCR was observed for D3 (1.38, Table 1), and suggests that marbled crayfish are highly efficient feed converters.
Utilization of Marbled Crayfish for Protein and Chitin Production
We also explored the suitability of marbled crayfish as a source of nutritional protein in European meals. Indeed, we found fried marbled crayfish tails (Figure 4A) to be suitable for crayfish risotto, and for appetizers (Figure 4B). Furthermore, the shell waste that was generated in this process, could be easily collected, and preserved by air-drying (Figure 4C).
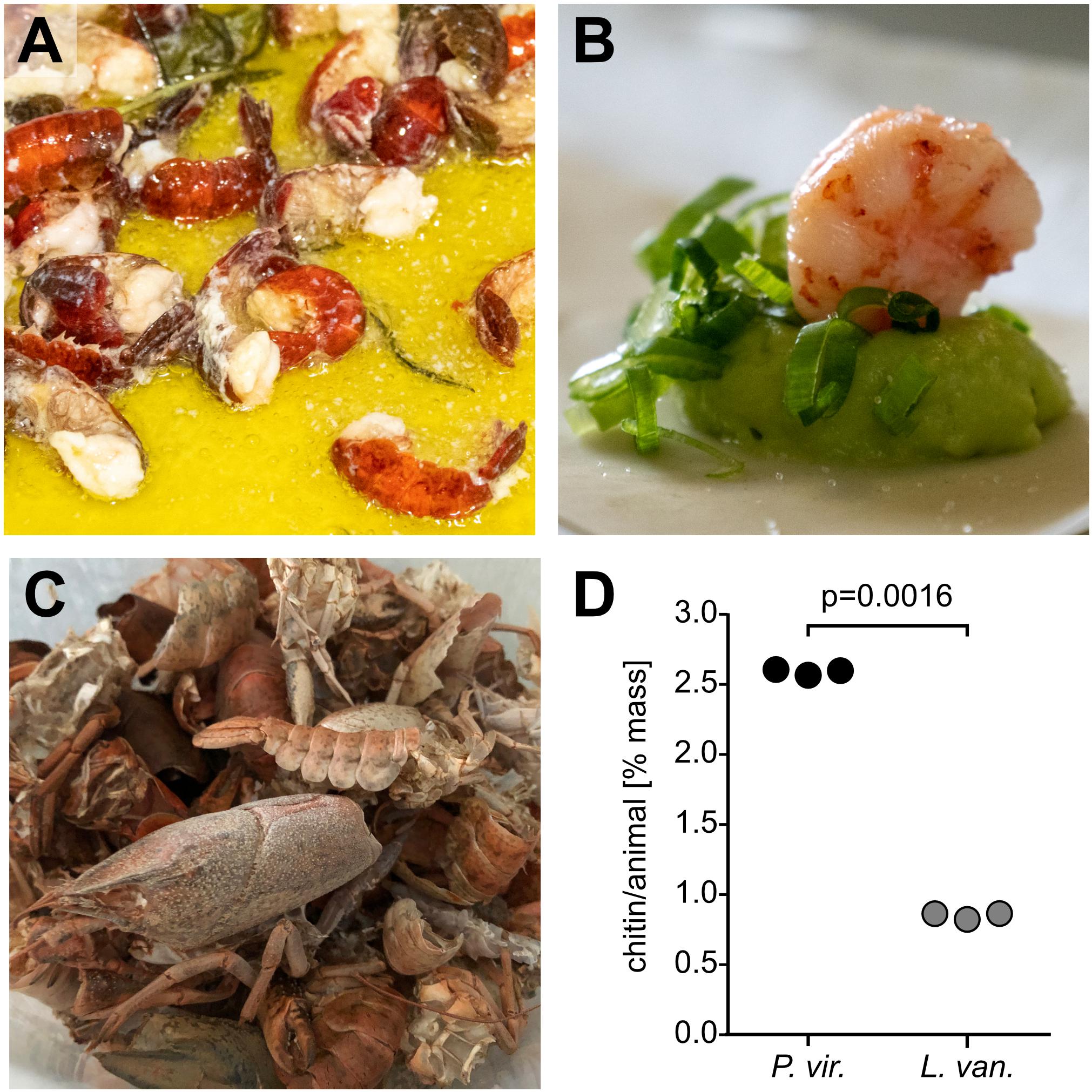
Figure 4. Utilization of marbled crayfish as a source for dietary protein and chitin. (A) Marbled crayfish tails with herbs, fried in butter. (B) Marbled crayfish tail meat on Avocado mousse. (C) Dried exoskeletons (shell waste) from marbled crayfish. (D) Comparison of chitin content per animal in marbled crayfish (P. vir) exoskeletons and whiteleg shrimp (L. van) shell waste. An unpaired two-tailed t-test showed that the difference between the two groups is highly significant (p = 0.0016).
To determine the chitin content of marbled crayfish shell waste, we used a chemical extraction protocol (see section “Materials and Methods” for details). Because they represent the major source of commercially extracted chitin and because data or material from P. clarkii were not available, we used similarly processed whiteleg shrimp (L. vannamei) shells for comparisons. This revealed a significantly higher chitin content for marbled crayfish than for L. vannamei (2.60% vs. 0.85%, p < 0.05, and Figure 4D). These findings suggest that marbled crayfish represent a valuable source of chitin.
Discussion
The increasing demand for nutritional protein and bioplastics requires the development of sustainable, and ecologically friendly production strategies. Our size and weight data of marbled crayfish revealed comparable features to commercially harvested P. clarkii, which is the dominant stock in crayfish aquaculture (Wang et al., 2018). Our findings thus contradict conclusions based on morphometric data from wild populations and laboratory colonies (Vogt, 2021). Differences in morphometric data analysis can be explained by the fact that previously reported populations were usually analyzed without any size selection and included the full spectrum of developmental stages, while our dataset is based on hand-catching, which selects bigger animals. As aquaculture production would also selectively harvest bigger animals, we consider our data more informative, and relevant. Furthermore, many of the populations that were analyzed by Vogt (2021) were located in challenging environments (cold climate, presence of predators, and lack of nutrients), while aquaculture is designed to improve and accelerate growth. Further work will be required to define optimized conditions and environments, and to determine the time that is needed to reach marketable sizes under optimized conditions.
Methionine is often the limiting amino acid to promote growth in aquatic animals, if feeds contain mainly plant proteins (Bulbul et al., 2015). Indeed, we observed the lowest growth rate for the feed with the lowest methionine level. This is consistent with the notion that methionine is a growth-limiting nutrient for marbled crayfish, similar to other crayfish, and shrimps and fish (Yan et al., 2007; Gu et al., 2013; Tan et al., 2018). Interestingly, the feed with the highest methionine level mainly promoted growth at earlier developmental stages, while later stages performed best with a feed that had an intermediate methionine level. These observations suggest different needs of methionine in different stages of crayfish development. Studies in other aquatic animals show that growth rates reach a plateau (Harding et al., 1977; Alam et al., 2001), or even decrease when the methionine requirements of the animals are overfed (Millamena et al., 1996; Sveier et al., 2001). Our results further suggest that methionine levels of 0.7% optimally support the growth of juvenile and early adolescent marbled crayfish. A balanced methionine supplementation is particularly relevant in closed aquaculture systems, where it supports rapid growth while maintaining high water quality.
Our experiments also provided first estimates for the FCR of marbled crayfish. The FCR is an important parameter for evaluating the potential of an organism for the sustainable production of biomass. In this context, it is notable that we observed FCRs as low as 1.4, which compares favorably to other species that are known as efficient feed converters, such as L. vannamei, and some insects (Hung and Quy, 2013; Oonincx et al., 2015). In comparison, the FCR of P. clarkii with similar sizes to the animals used in our study was found to be 2.8 with standard feed and could be improved to 1.9 by the addition of flavonoid feed supplements (Liu et al., 2020). Additional research is required to further optimize marbled crayfish feed conversion with less refined feeds and different culture conditions to fully realize their potential for the sustainable production of nutritional protein.
The sustainability of marbled crayfish aquaculture could be further improved by a zero-waste processing approach, which makes use of the considerable chitin content of their exoskeletons. Chitin and its derivatives, such as chitosan, are important raw materials for many industries and have found frequent use in wound bandages, filter materials, and for the production of biodegradable plastics (Park and Kim, 2010). Shrimp and crab shell waste currently provide most of the raw material for chitin extraction (Younes and Rinaudo, 2015), while the chitin content of P. clarkii shells remains unknown. Our results show that marbled crayfish exoskeletons contain 3 times more chitin per animal compared to whiteleg shrimp (L. vannamei) exoskeletons, the main source of commercially produced chitin (Younes and Rinaudo, 2015). Chitin yields strongly depend on extraction parameters but are generally consistent among crustacean species (Zainol Abidin et al., 2020), suggesting that the comparably high exoskeleton weight of marbled crayfish represents a major competitive advantage. It will be important to further determine the quality and suitability of marbled crayfish chitin for established processes.
Due to the invasiveness of marbled crayfish (Chucholl, 2015), any aquaculture approaches should be based on the use of closed (recirculating) systems to mitigate environmental risks. Recirculating aquaculture systems (RAS) are considered promising solutions for current challenges in aquaculture, as they are productive intensive farming systems that are designed to control many relevant environmental factors (Ahmed and Turchini, 2021; Naylor et al., 2021). RAS can be operated as completely closed systems with zero water exchange and with no adverse effects on biodiversity related to species escape (Ahmed and Turchini, 2021). Waste from RAS (which may potentially include crayfish eggs or small animals) is routinely processed by biosecurity systems, which include biological and chemical filtration, water sterilization, and disinfection (Ahmed and Turchini, 2021). Finally, RAS can be operated on non-arable land and even in deserts, and which further minimizes any risks of biological pollution (Ahmed and Turchini, 2021).
While RAS-based production of marbled crayfish has already been described as ecologically safe (Vogt, 2021), it has also been argued that the approach might be unprofitable. However, profitable processes for RAS-based mass production of shrimps have been established and are being further optimized (Shinji et al., 2019). As chitin extraction from shell waste could provide a significant increase in revenue, we suggest that RAS-based production of marbled crayfish might be a profitable and environmentally safe alternative to the current practices of P. clarkii farming in rice paddies. RAS-based aquaculture also allows for stringent pathogen monitoring (Ahmed and Turchini, 2021) and would thus prevent the transmission of infectious agents that threaten native crayfish populations (Keller et al., 2014; Mrugała et al., 2015). Similarly, stocking from laboratory populations, which are known to be free of crayfish plague (Vogt, 2021), would exclude a key pathogen associated with crayfish aquaculture.
Finally, it is important to notice that the role of invasive species in human livelihoods is dynamic in space and time (Shackleton et al., 2019). This is exemplified by the introduction and anthropogenic distribution of marbled crayfish in Madagascar, where the animals have had a demonstrable positive impact on household economy, and food security (Andriantsoa et al., 2020). Robustness, high adaptability and efficient feed conversion are important traits that can be found both in invasive species and in successful livestock. Given the possibilities to minimize environmental risks through the use of closed systems, it will be important to fully evaluate the potential of marbled crayfish as a livestock for sustainable aquaculture.
Data Availability Statement
The original contributions presented in the study are included in the article/Supplementary Material, further inquiries can be directed to the corresponding author.
Ethics Statement
The experiments described in this study are not subject to ethics committee approval and consent procedures. Laboratory stocks were described previously (Vogt et al., 2015) and handled according to institutional guidelines for animal care. Wild animals were collected in compliance with local fishery regulations.
Author Contributions
ST performed the experiments and analyzed the data. KM designed the feeds and the feed trial, and analyzed the data. JG performed statistical analyses. FLe collected animals and performed morphometric analyses. MT prepared marbled crayfish meals. JL and AM performed the chitin extraction. FB and FLy conceived the study. ST and FLy wrote the manuscript with input from the other authors. All authors read and approved the final manuscript.
Funding
This work was supported by a research contract from Evonik.
Conflict of Interest
KM and FB were employed by company Evonik Operations GmbH. FLy received consultation fees from Evonik Operations GmbH.
The authors declare that the research was conducted in the absence of any commercial or financial relationships that could be construed as a potential conflict of interest.
Publisher’s Note
All claims expressed in this article are solely those of the authors and do not necessarily represent those of their affiliated organizations, or those of the publisher, the editors and the reviewers. Any product that may be evaluated in this article, or claim that may be made by its manufacturer, is not guaranteed or endorsed by the publisher.
Acknowledgments
We thank Carine Legrand for help with statistics and Stephan Neumayer for stimulating discussions about crustacean aquaculture. An earlier version of this manuscript has been released as a pre-print at https://www.biorxiv.org/content/10.1101/2020.02.25.964114v2 (Tönges et al., 2020).
Supplementary Material
The Supplementary Material for this article can be found online at: https://www.frontiersin.org/articles/10.3389/fevo.2021.651981/full#supplementary-material
References
Ahmed, N., and Turchini, G. M. (2021). Recirculating aquaculture systems (RAS): environmental solution and climate change adaptation. J. Clean. Produc. 297:126604. doi: 10.1016/j.jclepro.2021.126604
Alam, M. S., Teshima, S., Ishikawa, M., Koshio, S., and Yaniharto, D. (2001). Methionine requirement of juvenile Japanese flounder Paralichthys olivaceus estimated by the oxidation of radioactive methionine. Aquacult. Nutr. 7, 201–209. doi: 10.1046/j.1365-2095.2001.00171.x
Anastácio, P. M., Ferreira, M. P., Banha, F., Capinha, C., and Rabaça, J. E. (2014). Waterbird-mediated passive dispersal is a viable process for crayfish (Procambarus clarkii). Aquatic Ecol. 48, 1–10. doi: 10.1007/s10452-013-9461-0
Andriantsoa, R., Jones, J. P. G., Achimescu, V., Randrianarison, H., Raselimanana, M., Andriatsitohaina, M., et al. (2020). Perceived socio-economic impacts of the marbled crayfish invasion in Madagascar. PLoS One 15:e0231773. doi: 10.1371/journal.pone.0231773
Andriantsoa, R., Tönges, S., Panteleit, J., Theissinger, K., Carneiro, V. C., Rasamy, J., et al. (2019). Ecological plasticity and commercial impact of invasive marbled crayfish populations in Madagascar. BMC Ecol 19:8. doi: 10.1186/s12898-019-0224-1
Bulbul, M., Kader, M. A., Ambak, M. A., Hossain, M. S., Ishikawa, M., and Koshio, S. (2015). Effects of crystalline amino acids, phytase and fish soluble supplements in improving nutritive values of high plant protein based diets for kuruma shrimp, Marsupenaeus japonicus. Aquaculture 438, 98–104. doi: 10.1016/j.aquaculture.2015.01.007
Chucholl, C. (2015). “Marbled crayfish gaining ground in Europe: the role of the pet trade as invasion pathway,” in Freshwater Crayfish: Global Overview, eds T. Kawai, Z. Faulkes, and G. Scholtz (Boca Raton, FL: CRC Press), 83–114. doi: 10.1201/b18723-8
Chucholl, C., Morawetz, K., and Groß, H. (2012). The clones are coming – strong increase in Marmorkrebs Procambarus fallax (Hagen, 1870) f. virginalis records from Europe. Aquat. Invas. 7, 511–519. doi: 10.3391/ai.2012.7.4.008
Council, N. R. (2011). Nutrient Requirements of Fish and Shrimp. Washington, DC: The National Academies Press.
Cowey, C. B., and Walton, M. J. (1989). “”Intermediary metabolism,” in Fish Nutrition, 2nd Edn, ed. J. E. Halver (New York, NY: Academic Press).
Crandall, K. A., and De Grave, S. (2017). An updated classification of the freshwater crayfishes (Decapoda: Astacidea) of the world, with a complete species list. J. Crust. Biol. 37, 1–39. doi: 10.5479/si.00810282.624
Faulkes, Z. (2015). Marmorkrebs (Procambarus fallax f. virginalis) are the most popular crayfish in the North American pet trade. Knowl. Manag. Aquat. Ecosyst. 20:2568. doi: 10.1051/kmae/2015016
Gherardi, F. (2006). Crayfish invading Europe: the case study of Procambarus clarkii. Aquat. Ecosyst. 416, 1–15. doi: 10.1080/10236240600869702
Gu, M., Zhang, W. B., Bai, N., Mai, K. S., and Xu, W. (2013). Effects of dietary crystalline methionine or oligo-methionine on growth performance and feed utilization of white shrimp (Litopenaeus vannamei) fed plant protein-enriched diets. Aquacult. Nutr. 19, 39–46. doi: 10.1111/anu.12089
Gutekunst, J., Andriantsoa, R., Falckenhayn, C., Hanna, K., Stein, W., Rasamy, J. R., et al. (2018). Clonal genome evolution and rapid invasive spread of the marbled crayfish. Nat. Ecol. Evol. 2, 567–573. doi: 10.1038/s41559-018-0467-9
Harding, D. E., Allen, O. W. Jr., and Wilson, R. P. (1977). Sulfur amino acid requirement of channel catfish: L-methionine and L-cystine. J. Nutr. 107, 2031–2035. doi: 10.1093/jn/107.11.2031
Haubrock, P. J., Oficialdegui, F. J., Zeng, Y., Patoka, J., Yeo, D. C. J., and Kouba, A. (2021). The redclaw crayfish: a prominent aquaculture species with invasive potential in tropical and subtropical biodiversity hotspots. Rev. Aquacult. 13, 1488–1530. doi: 10.1111/raq.12531
Hossain, M. S., Patoka, J., Kouba, A., and Buřič, M. (2018). Clonal crayfish as biological model: a review on marbled crayfish. Biologia 73, 841–855. doi: 10.2478/s11756-018-0098-2
Hung, L. T., and Quy, O. M. (2013). “On-farm feeding and feed management in whiteleg shrimp (Litopenaeus vannamei) farming in Viet Nam,” in On-Farm Feeding and Feed Management in Aquaculture. FAO Fisheries and Aquaculture Technical Paper No. 583, eds M. R. Hasan and M. B. New (Rome: FAO), 337–357.
Jones, J. P. G., Rasamy, J. R., Harvey, A., Toon, A., Oidtmann, B., Randrianarison, M. H., et al. (2009). The perfect invader: a parthenogenic crayfish poses a new threat to Madagascar’s freshwater biodiversity. Biol. Invas. 11, 1475–1482. doi: 10.1007/s10530-008-9334-y
Kawai, T., Scholtz, G., Morioka, S., Ramanamandimby, F., Lukhaup, C., and Hanamura, Y. (2009). Parthenogenetic alien crayfish (Decapoda: Cambaridae) spreading in Madagascar. J. Crust. Biol. 29, 562–567. doi: 10.1651/08-3125.1
Keller, N. S., Pfeiffer, M., Roessink, I., Schulz, R., and Schrimpf, A. (2014). First evidence of crayfish plague agent in populations of the marbled crayfish (Procambarus fallax forma virginalis). Knowledge Manag. Aquatic Ecosyst. 414:15. doi: 10.1051/kmae/2014032
Liu, F., Qu, Y. K., Geng, C., Wang, A. M., Zhang, J. H., Chen, K. J., et al. (2020). Effects of hesperidin on the growth performance, antioxidant capacity, immune responses and disease resistance of red swamp crayfish (Procambarus clarkii). Fish Shellfish Immunol. 99, 154–166. doi: 10.1016/j.fsi.2020.02.014
Loureiro, T. G., Anastácio, P. M., Araujo, P. B., Souty-Grosset, C., and Almerão, M. P. (2015). Red swamp crayfish: biology, ecology and invasion–an overview. Nauplius 23, 1–19. doi: 10.1590/s0104-64972014002214
Lyko, F. (2017). The marbled crayfish (Decapoda: Cambaridae) represents an independent new species. Zootaxa 4363, 544–552. doi: 10.11646/zootaxa.4363.4.6
Maiakovska, O., Andriantsoa, R., Tonges, S., Legrand, C., Gutekunst, J., Hanna, K., et al. (2021). Genome analysis of the monoclonal marbled crayfish reveals genetic separation over a short evolutionary timescale. Commun. Biol. 4:74. doi: 10.1038/s42003-020-01588-8
Martin, P., Kohlmann, K., and Scholtz, G. (2007). The parthenogenetic Marmorkrebs (marbled crayfish) produces genetically uniform offspring. Naturwissenschaften 94, 843–846. doi: 10.1007/s00114-007-0260-0
Millamena, O. M., Bautista-Teruel, M. N., and Kanazawa, A. (1996). Methionine requirement of juvenile tiger shrimp Penaeus monodon Fabricius. Aquaculture 143, 403–410. doi: 10.1016/0044-8486(96)01270-7
Mrugała, A., Kozubíková-Balcarová, E., Chucholl, C., Cabanillas Resino, S., Viljamaa-Dirks, S., Vukić, J., et al. (2015). Trade of ornamental crayfish in Europe as a possible introduction pathway for important crustacean diseases: crayfish plague and white spot syndrome. Biol. Invas. 17, 1313–1326. doi: 10.1007/s10530-014-0795-x
Naylor, R. L., Hardy, R. W., Buschmann, A. H., Bush, S. R., Cao, L., Klinger, D. H., et al. (2021). A 20-year retrospective review of global aquaculture. Nature 591, 551–563. doi: 10.1038/s41586-021-03308-6
Nunes, A. J. P., Sá, M. V. C., Browdy, C. L., and Vazquez-Anon, M. (2014). Practical supplementation of shrimp and fish feeds with crystalline amino acids. Aquaculture 431, 20–27. doi: 10.1016/j.aquaculture.2014.04.003
Oonincx, D. G., Van Broekhoven, S., Van Huis, A., and Van Loon, J. J. (2015). Feed conversion, survival and development, and composition of four insect species on diets composed of food by-products. PLoS One 10:e0144601. doi: 10.1371/journal.pone.0144601
Owen, C. L., Bracken-Grissom, H., Stern, D., and Crandall, K. A. (2015). A synthetic phylogeny of freshwater crayfish: insights for conservation. Philos. Transact. R. Soc. Lond. B Biol. Sci. 370:20140009. doi: 10.1098/rstb.2014.0009
Park, B. K., and Kim, M. M. (2010). Applications of chitin and its derivatives in biological medicine. Int. J. Mol. Sci. 11, 5152–5164. doi: 10.3390/ijms11125152
Patoka, J., Magalhães, A. L. B., Kouba, A., Faulkes, Z., Jerikho, R., and Vitule, J. R. S. (2018). Invasive aquatic pets: failed policies increase risks of harmful invasions. Biodivers. Conserv. 27, 3037–3046. doi: 10.1007/s10531-018-1581-3
Patoka, J., Petrtıl, M., and Kalous, L. (2014). Garden ponds as potential introduction pathway of ornamental crayfish. Knowl. Managt. Aquatic Ecosyst. 13:4895. doi: 10.1051/kmae/2014019
Percot, A., Viton, C., and Domard, A. (2003a). Characterization of shrimp shell deproteinization. Biomacromolecules 4, 1380–1385. doi: 10.1021/bm034115h
Percot, A., Viton, C., and Domard, A. (2003b). Optimization of chitin extraction from shrimp shells. Biomacromolecules 4, 12–18. doi: 10.1021/bm025602k
Putra, M. D., Bláha, M., Wardiatno, Y., Krisanti, M., Yonvitner, Jerikho, R., et al. (2018). Procambarus clarkii (Girard, 1852) and crayfish plague as new threats for biodiversity in Indonesia. Aquat. Conserv. Mar. Freshw. Ecosyst. 28, 1434–1440. doi: 10.1002/aqc.2970
Scholtz, G., Braband, A., Tolley, L., Reimann, A., Mittmann, B., Lukhaup, C., et al. (2003). Ecology: parthenogenesis in an outsider crayfish. Nature 421:806. doi: 10.1038/421806a
Shackleton, R. T., Shackleton, C. M., and Kull, C. A. (2019). The role of invasive alien species in shaping local livelihoods and human well-being: a review. J. Environ. Manage 229, 145–157. doi: 10.1016/j.jenvman.2018.05.007
Shinji, J., Nohara, S., Yagi, N., and Wilder, M. (2019). Bio-economic analysis of super-intensive closed shrimp farming and improvement of management plans: a case study in Japan. Fisher. Sci. 85, 1055–1065. doi: 10.1007/s12562-019-01357-5
Sveier, H., Nordås, H., Berge, G. E., and Lied, E. (2001). Dietary inclusion of crystalline D- and L-methionine: effects on growth, feed and protein utilization, and digestibility in small and large Atlantic salmon (Salmon salar L.). Aquacult. Nutr. 7, 169–181. doi: 10.1046/j.1365-2095.2001.00169.x
Tan, Q., Song, D., Chen, X., Xie, S., and Shu, X. (2018). Replacing fish meal with vegetable protein sources in feed for juvenile red swamp crayfish, Procambarus clarkii: effects of amino acids supplementation on growth and feed utilization. Aquacult. Nutr. 24, 858–864. doi: 10.1111/anu.12621
Tönges, S., Masagounder, K., Gutekunst, J., Lohbeck, J., Miller, A. K., Böhl, F., et al. (2020). Physiological properties and tailored feeds to support aquaculture of marbled crayfish in closed systems. bioRxiv [Preprint]. 2020.2002.2025.964114.
Vogt, G. (2021). Evaluation of the suitability of the parthenogenetic marbled crayfish for aquaculture: potential benefits versus conservation concerns. Hydrobiologia 848, 285–298. doi: 10.1007/s10750-020-04395-8
Vogt, G., Falckenhayn, C., Schrimpf, A., Schmid, K., Hanna, K., Panteleit, J., et al. (2015). The marbled crayfish as a paradigm for saltational speciation by autopolyploidy and parthenogenesis in animals. Biol. Open 4, 1583–1594. doi: 10.1242/bio.014241
Vogt, G., Huber, M., Thiemann, M., Van Den Boogaart, G., Schmitz, O. J., and Schubart, C. D. (2008). Production of different phenotypes from the same genotype in the same environment by developmental variation. J. Exp. Biol. 211, 510–523. doi: 10.1242/jeb.008755
Wang, Q., Ding, H., Tao, Z., and Ma, D. (2018). “Crayfish (Procambarus clarkii) cultivation in China: a decade of unprecedented development,” in Aquaculture in China: Success Stories and Modern Trends, eds J. F. Gui, Q. Tang, Z. Li, J. Liu, and S. S. De Silva (Hoboken, NJ: John Wiley & Sons).
Yan, Q., Xie, S., Zhu, X., Lei, W., and Yang, Y. (2007). Dietary methionine requirement for juvenile rockfish, Sebastes schlegeli. Aquacult. Nutr. 13, 163–169. doi: 10.1111/j.1365-2095.2007.00461.x
Younes, I., and Rinaudo, M. (2015). Chitin and chitosan preparation from marine sources. Structure, properties and applications. Mar. Drugs 13, 1133–1174. doi: 10.3390/md13031133
Keywords: livestock, invasive species, feed conversion, chitin, sustainability, aquaculture, marbled crayfish, tailored feeds
Citation: Tönges S, Masagounder K, Lenich F, Gutekunst J, Tönges M, Lohbeck J, Miller AK, Böhl F and Lyko F (2021) Evaluating Invasive Marbled Crayfish as a Potential Livestock for Sustainable Aquaculture. Front. Ecol. Evol. 9:651981. doi: 10.3389/fevo.2021.651981
Received: 11 January 2021; Accepted: 23 July 2021;
Published: 12 August 2021.
Edited by:
Bruce Arthur Osborne, University College Dublin, IrelandReviewed by:
Ivo Roessink, Wageningen University and Research, NetherlandsJiří Patoka, Czech University of Life Sciences Prague, Czechia
Copyright © 2021 Tönges, Masagounder, Lenich, Gutekunst, Tönges, Lohbeck, Miller, Böhl and Lyko. This is an open-access article distributed under the terms of the Creative Commons Attribution License (CC BY). The use, distribution or reproduction in other forums is permitted, provided the original author(s) and the copyright owner(s) are credited and that the original publication in this journal is cited, in accordance with accepted academic practice. No use, distribution or reproduction is permitted which does not comply with these terms.
*Correspondence: Frank Lyko, f.lyko@dkfz.de