- Institute of Zoology, University of Regensburg, Regensburg, Germany
Finding and recognizing a suitable mate is a key prerequisite to reproductive success. Insects often recognize prospective mates using chemical cues and signals. Among these, cuticular lipids commonly serve for mate recognition at close range. The lipid layer on the surface of insects is comprised predominantly of cuticular hydrocarbons (CHCs), though more polar compounds may also be present. While the composition of the cuticular profile is typically species specific, many species additionally show differentiation between the sexes by sex specific compounds and/or compound ratios. It is often assumed that a clear sexual dimorphism of cuticular lipid profiles is a prerequisite for a potential function as sex pheromones. Both, sex specific single compounds or the profiles as a whole have been shown to serve as sex pheromones in parasitoid wasps. Here, we studied parasitoid wasps of the species Tachinaephagus zealandicus (Encyrtidae, Hymenoptera). Chemical analyses revealed that this species presents a case where males and females produce the same set of CHCs in similar relative amounts. To test whether these wasps nonetheless can use the cuticular lipids for close range mate recognition, we tested the reaction of males toward freeze-killed conspecifics. Males showed copulation behavior exclusively toward dead females, but not toward dead males. Dead females from which the cuticular lipids had been removed did not elicit copulation behavior by tested males. Reapplication of female whole body extracts restored bioactivity, and males reacted with copulation attempts as often as toward the freeze-killed females. Bioassays with lipid fractions revealed that only the CHC fraction was bioactive on its own. Here, again, males reacted to female, but not to male CHCs. Our results indicate that these wasps are capable of using CHCs for close range sex recognition despite the similarity of male and female profiles.
Introduction
Chemical communication with pheromones is widespread in the animal kingdom (Wyatt, 2014). The most essential pheromone function may be that of the sex pheromones, as they serve to find and acquire a mate. In insects, this function can be mediated by compounds from the cuticular lipid layer that covers their outer surface (Howard and Blomquist, 2005). Compared to highly volatile sex pheromones that can attract mates already from a distance (e.g., many lepidopteran pheromones: Cardé and Haynes, 2004), cuticular lipids are less volatile and thus usually detected at close range (Blomquist and Bagnères, 2010a). The primary function of the lipid layer is thought to be the prevention of desiccation (Ramsey, 1935; Gibbs and Rajpurohit, 2010). In many cases it is additionally used for chemical communication (Blomquist and Bagnères, 2010a; Leonhardt et al., 2016). The composition of cuticular lipids is usually complex, and may contain compounds from several compound classes. However, the most ubiquitous constituents are hydrocarbons (Blomquist and Bagnères, 2010b). Through different combinations of compounds and/or compound ratios, cuticular hydrocarbon (CHC) profiles are usually species specific (Bagnères and Wicker-Thomas, 2010; Guillem et al., 2016), and often differ additionally between the sexes (reviewed in Thomas and Simmons, 2008). In many insects, CHCs can thus serve as contact sex pheromones, e.g., in Coleoptera (Ginzel et al., 2006; Lacey et al., 2008; Geiselhardt et al., 2009), Diptera (Carlson et al., 1971; Ferveur, 2005), and Hymenoptera (Ruther et al., 2011; Kühbandner et al., 2012; Würf et al., 2020). Sexual behavior may be elicited by one or a few sex specific key compounds (Ginzel et al., 2003; Lacey et al., 2008; Silk et al., 2009; Olaniran et al., 2013). In other cases, however, sex pheromone function is not quite as straight forward. Sex specific key compounds may trigger a full response only when presented together with the remaining CHC background or other compounds (Kühbandner et al., 2012; see discussion in Hughes et al., 2015). Additionally, the CHC profiles of potential mating partners may be detected as a whole, as is indicated when their sex pheromone function can be disturbed by changes in the overall composition or in the relative amounts of single compounds (Kühbandner et al., 2013; Würf et al., 2020).
While there are reports of species in which both sexes produce the same CHC compounds, in some cases even in similar or equal relative amounts (Geiselhardt et al., 2009; Ablard et al., 2012 and references therein; Mitra et al., 2015; reviewed in Howard and Blomquist, 2005), only relatively few studies have investigated whether CHCs can nonetheless serve as sex pheromones and for mate recognition in such cases (Stoffolano et al., 1997; Hemptinne et al., 1998; Howard, 1998; Peterson et al., 2007; Geiselhardt et al., 2009; Ablard et al., 2012; Snellings et al., 2018). Rather, it is often assumed that a lack of obvious differentiation (sex-specific compounds or highly divergent relative amounts of the same compounds) between the sexes precludes CHCs from functioning as sex pheromones. Such a conclusion may, however, be premature. For example, CHCs can elicit sexual behavior without functioning to discriminate between the sexes (i.e., sexual behavior in response to CHCs is not restricted to individuals of the opposite sex, but shown toward conspecifics in general: Stoffolano et al., 1997; Hemptinne et al., 1998; Geiselhardt et al., 2009). Furthermore, with the exception of 2-methylalkanes and symmetric methyl-branched compounds, methyl-branched CHCs possess at least one chiral center, but the presence and the absolute configuration of different stereoisomers cannot be detected through coupled gas chromatography-mass spectrometry (GC/MS), which is used in the majority of studies (see Bello et al., 2015). Thus, if the sexes produce different stereoisomers of the same compounds, they can function as sex specific mate recognition signals despite otherwise similar CHC profiles (Ablard et al., 2012).
One species rich group of insects in which CHCs have been shown to be involved in mate recognition is the parasitoid wasps. These hymenopterans lay their eggs in or on an arthropod host, on which the developing larvae feed (Quicke, 1997). Sexual behavior of adult male wasps can be mediated by female CHCs in combination with other compounds (Kühbandner et al., 2012, 2013; Stökl et al., 2014; Weiss et al., 2015), or by female CHCs alone (Steiner et al., 2006; Ruther et al., 2011; Ablard et al., 2012; Weiss et al., 2015; Böttinger et al., 2020; Würf et al., 2020). In most of these cases, CHC profiles were found to differ qualitatively and quantitatively between mature males and females, while only Ablard et al. (2012) found both sexes of Ooencyrtus kuvanae (Hymenoptera, Encyrtidae) to show exactly the same CHC peaks in GC/MS analyses. Other studies on parasitoid wasps also report cases in which CHC profiles of both sexes were qualitatively identical, though their function as a sex pheromone was not assessed (Howard and Liang, 1993; Howard and Pérez-Lachaud, 2002).
Here, we investigated the parasitoid wasp Tachinaephagus zealandicus (Hymenoptera, Encyrtidae). Observations of their mating behavior indicated that mating partners are recognized at short range. Males orient toward females passing by in close proximity, and then follow them while approaching from the side in their typical courtship behavior. Once the females stop, the males will attempt to copulate (Olton and Legner, 1974; van den Assem, 1976; personal observation). Preliminary chemical analyses indicated that with this species we can study a case of both sexes producing the same CHC compounds in similar amounts. We chemically analyzed the cuticular lipids, and determined whether CHCs play a role in mate recognition despite the similarity of male and female profiles. For this, we assessed whether male sexual behavior is elicited by cuticular lipids, and whether the sexes can be distinguished. Then we tested whether the recognition of potential mating partners is indeed mediated by CHCs, or if other, more polar, compounds are involved as well.
Materials and Methods
Insects
The wasps used in this study originated from carcass traps (similar as described in Moretti et al., 2008) set out in Giessen, Germany. The laboratory stock (bred in several matrilines, i.e., originating from one female each) was reared on larvae of Lucilia caesar and Calliphora vomitoria at 25°C in a thermal cabinet under a 16:8 h light:dark cycle. As T. zealandicus is a gregarious parasitoid (several eggs are laid into each host, Olton and Legner, 1974), parasitized fly pupae were dissected after 21 days to separate the wasps. The sex ratio is usually biased toward females, thus additional male wasps were obtained by dissecting hosts parasitized by virgin female wasps (only male offspring due to haplodiploidy). Wasp pupae were removed from the hosts, placed singly in 1.5 ml reaction vials and kept at 25°C until wasp eclosion. After eclosion from the pupal exuvia, wasps were kept in the thermal cabinet for a minimum of 24 h before extraction of cuticular lipids or testing in bioassays. Usually, wasps will emerge from the host puparium about 24 to 48 h after eclosion from the pupal exuvia, and mating normally occurs shortly thereafter (Olton and Legner, 1974; personal observation). Therefore, a minimum of 24 h delay before extraction/testing would best reflect the timespan after which adults would usually encounter each other.
Chemical Analysis
For chemical analyses, ten females and ten males aged 24 to 48 h after eclosion from the pupal exuvia were killed by freezing at −21°C. Subsequently, they were each extracted singly for 5 min in 16 μl of dichloromethane (DCM) containing 6.25 ng μl–1 1-octadecene (not naturally occurring in the lipid profile) as internal standard. Thereafter, wasps were removed, and the extracts were chemically analyzed by gas chromatography and coupled mass spectrometry on a Shimadzu GC/MS QP2010 Plus fitted with a BPX-5 column (30 m length, 0.25 mm diameter, 0.25 μm film thickness). One microliter of extract was injected by autosampler in splitless mode at an injection temperature of 300°C. The oven program began at 150°C and the temperature was raised to 300°C at 8°C min–1. The final temperature was held constant for another 15 min. Helium was used as carrier gas at a flow rate of 1.73 ml min–1. The mass spectrometer recorded a mass range of m/z 35–600 after electron ionization at 70 eV. Compounds were determined based on their linear retention indices (LRIs), as well as the characteristic fragmentation patterns in the mass spectra. The positions of methyl-branches were determined based on diagnostic ion pairs and comparison of the compounds’ LRIs to those shown by Carlson et al. (1998). Double bond position was determined by dimethyl-disulfide derivatization and interpretation of the resulting mass spectra (Dunkelblum et al., 1985; Carlson et al., 1989).
Extraction and Fractionation
To obtain cuticular lipid extracts, 100 individuals of the same sex (aged between 24 and 48 h after eclosion from the pupal exuvia) were killed by freezing at −21°C. Thereafter, they were placed together in a 2 ml glass vial and extracted with 1.5 ml of DCM for 1 min. The extract was transferred to a clean glass vial, and the wasps were rinsed with another 500 μl, subsequently adding this second extract to the first. Thereafter, the solvent was carefully evaporated under a stream of nitrogen, and the cuticular lipids were re-dissolved in 100 μl of DCM, leading to a concentration of one animal equivalent of cuticular lipids per microliter of solvent. Silica gel cartridges (Chromabond 100 mg, Macherey-Nagel, Düren, Germany) were used to separate extracts into three fractions of differing polarity. The cartridges were pre-rinsed with 1 ml of hexane, DCM, methanol, then again DCM and hexane. After equilibration with 1 ml of hexane the extract was applied. Extract fractions were eluted with 1 ml of hexane (“H,” non-polar fraction containing hydrocarbons), 1 ml of DCM (“D,” fraction containing lipids with intermediate polarity) and 1 ml of methanol (“M,” polar lipids). Each fraction was evaporated under a gentle stream of nitrogen, and then re-dissolved in 100 μl DCM (1 individual equivalent per microliter).
Bioassays
Male reactions (copulation attempts) were assessed in a small mating arena (3 mm high fluorescent acrylic glass plate with a circular hole of 20 mm diameter positioned on top of a glass plate and covered with a glass slide) that was lit from below. Single males (virgin, naïve, age 24–70 h after eclosion from the pupal exuvia, maximum of two replicates per matriline for each bioassay) were placed in a mating arena containing a test stimulus (dead wasp; exact treatments described below) and observed for 5 min. The test stimulus was placed near the side of the arena to raise the chances of encounter. Males that did not approach the test stimulus closely enough to ensure detection (antennae less than 1 mm away from the stimulus) were excluded from the analyses, and the setup was repeated with a new male and freshly prepared stimulus. All males were tested once only, and for each repetition (total of n = 30 repetitions for each assay) a new test stimulus was used. Between repetitions, the arena was cleaned with ethanol. We conducted three experiments with two parts each. In the first experiment we tested whether males can distinguish females from males, and whether cuticular lipids are required to elicit sexual behavior. We confronted males with either a dead male, a dead female or a dead female from which the cuticular lipids had been removed through Soxhlet-extraction (9 h; female “dummy”). All further treatments involved the application of solvent, extracts or extract fractions on female dummies. It was anticipated that some of the applied solvent (and the compounds dissolved therein) would seep into the dummy and onto the glass plate below the dummy during the application. Thus, we compensated for this expected loss by applying two animal equivalents or the respective amount of pure solvent. In the subsequent assay, female dummies were treated either with female-derived extract (2 μl, amount equivalent to two females) or with a solvent control (2 μl DCM) to ascertain that cuticular lipids are involved in the recognition process. In the second experiment, we tested male reactions toward dummies treated with either a solvent control (2 μl DCM) or one of the three fractions (H, D, and M; always 2 μl, two female equivalents) obtained from female-derived extracts. The following assay then tested whether male reactions to the bioactive female fraction (H, see section “Results”) differ from those to the same fraction of male-derived extract. In the next experiment we assessed whether the other two female fractions contain compounds with an additive or synergistic function, by confronting males with female dummies treated with a solvent control or female-derived H combined with D, with M, or with both of the two remaining fractions. In the final assay we tested whether the addition of one extract fraction (D or M) to the H fraction of the opposite sex would influence male reactions, i.e., leading to female H fraction being less attractive or male H fraction being more attractive as in tests with pure H fractions. All tested dummies were treated with two animal equivalents as in the previous bioassays.
Statistical Analyses
To analyze the similarity between male and female lipid profiles, we calculated the relative peak areas, dividing the integrated peak area of each peak by the sum of all peak areas of that sample (omitting the internal standard). Only peaks that contributed over 0.25% to the overall profile in five or more samples of at least one of the sexes were retained in further analyses by one-way analysis of similarities (ANOSIM) and similarity percentages (SIMPER) in Primer v6 (Primer-E, Plymouth; Clarke, 1993; Clarke and Gorley, 2006). The ANOSIM value R (1 > R > −1) indicates the degree of separation between groups, from no separation (0) to maximum separation (1). Negative values (down to −1) indicate that separation is higher within groups than between them. SIMPER determines the contribution of each CHC compound to the overall Bray-Curtis dissimilarity between the groups.
Bioassay results were analyzed with χ2 tests in R v3.6.1 (R Core Team, 2019). We compared the numbers of repetitions in which copulation behavior was shown toward the respective test stimuli. Bonferroni-Holm corrections were applied when several pairwise comparisons were conducted.
Results
Chemical Analyses
Neither male nor female cuticular lipid profiles contained exclusive peaks, and the compounds were present in similar relative amounts in both sexes (Figure 1 and Table 1). Overall, females produced a higher quantity of compounds, on average a third more CHC than males (CHC amount of females 783 ± 337 ng, males 582 ± 205 ng; average ± standard deviation). In total, we determined 68 CHC compounds, 25 of which were excluded from further analyses due to their low relative contribution (<0.25%) to the total profiles of both sexes. 24 of the remaining compounds co-eluted with at least one compound. The quantitative contribution of the single peaks constituting such overlapping peaks was assessed through manual integration. One diagnostic fragment ion per compound was chosen and the fragment chromatograms were overlaid (display factors were adjusted based on representative mass spectra for each compound). Peak separation was then set at the crossing point of the diagnostic fragment ion chromatograms. The evaluation of the resulting peak areas revealed that these compounds contributed similar relative amounts to overlapping peaks in both male and female samples (Table 1). Therefore, such overlapping peaks were analyzed together as one peak, resulting in a total of 29 peaks that were analyzed for profile similarity (Table 1). Overall, the CHC profiles differed between the sexes, though with an intermediate measure of differentiation (ANOSIM: R = 0.558, p < 0.001). For only seven of the 29 peaks were the average relative amounts more than 50% (but not more than three times) higher in one of the sexes (compounds 3, 5, 6, 11, 12, 18, and 28, Table 1). SIMPER analysis revealed that four compound peaks contributed to more than 50% of the Bray-Curtis difference between males and females, two of which were more abundant in females (in order of relative contribution to dissimilarity): 7-pentacosene (7-C25:1) and heptacosane (C27).
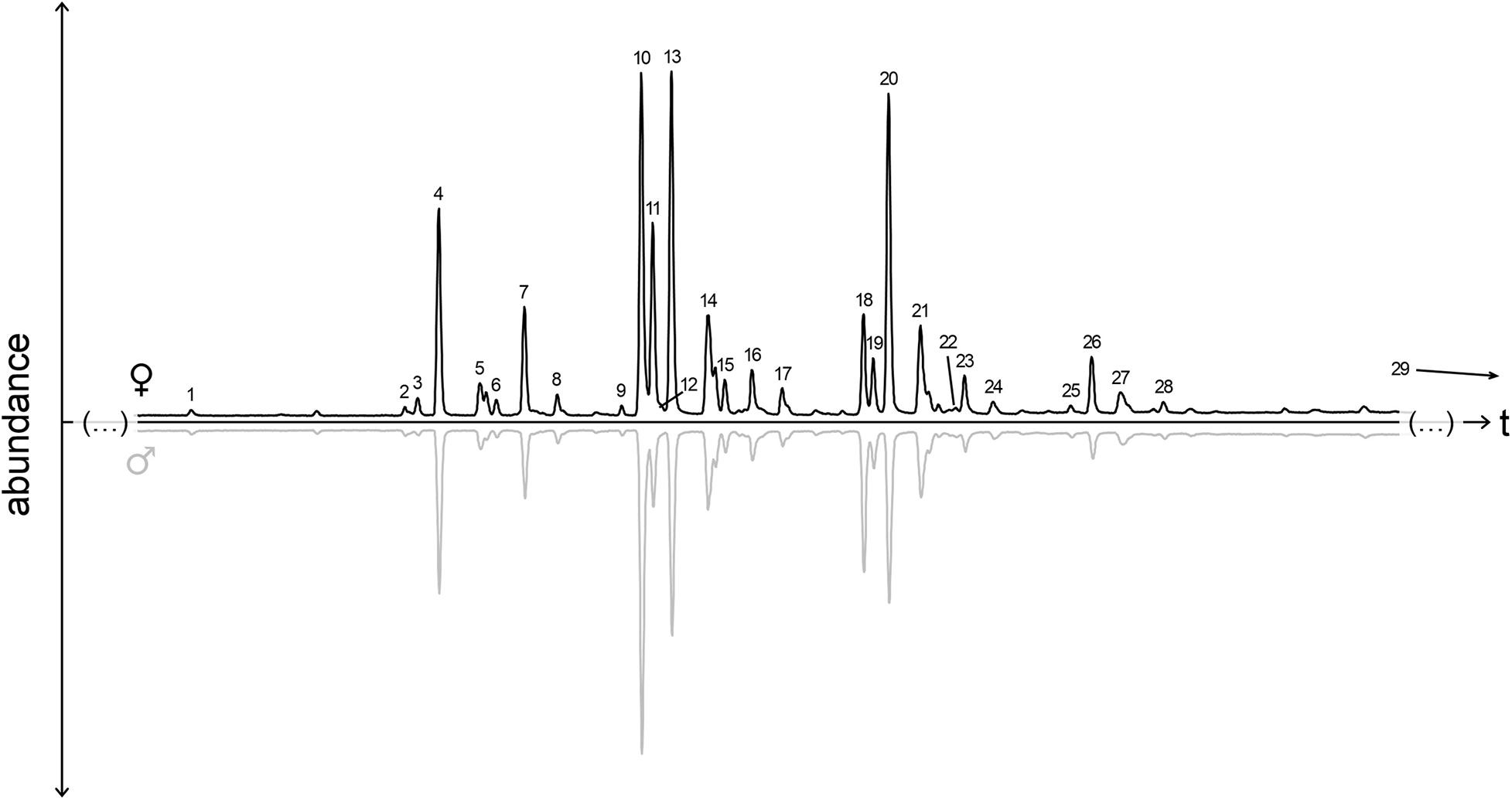
Figure 1. Composition of cuticular hydrocarbon profiles of female and male Tachinaephagus zealandicus. Representative chromatograms of extracts from a female (top) and a male (bottom), scaled relative to the amount of internal standard (1-octadecene, 6.25 ng, not shown; elutes before peak 1). Peak numbers correspond to the compounds listed in Table 1. Peak 29 (11-methylheptatriacontane) not shown, elutes much later than peak 28.
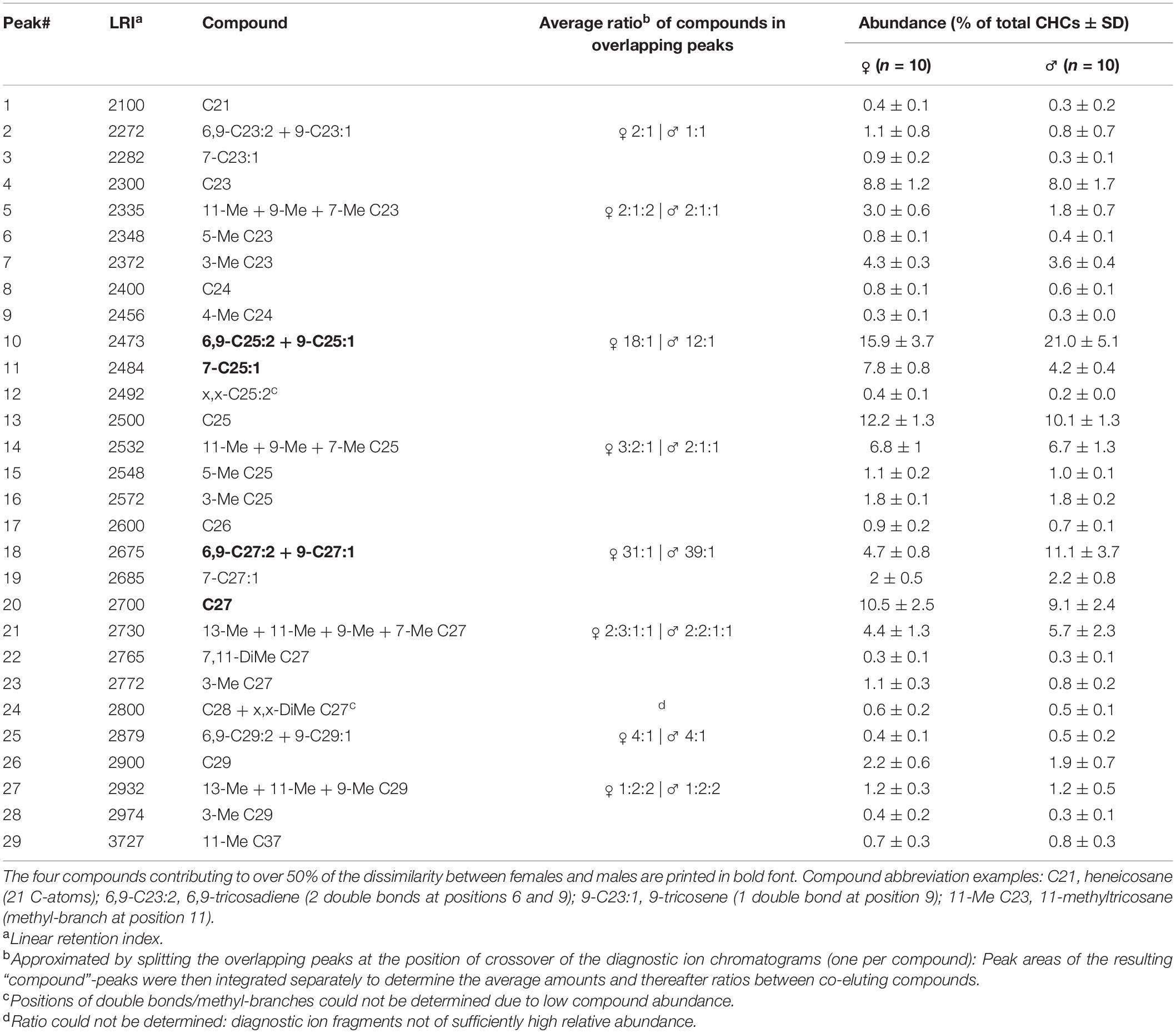
Table 1. Cuticular hydrocarbons (CHCs, >0.25% relative amount of total profile) found in crude extracts of male and female Tachinaephagus zealandicus.
Bioassays
Male wasps attempted to copulate with dead females, but never with dead males, nor with dead females from which the cuticular lipids had been removed by Soxhlet-extraction (Figure 2A; χ2 test overall: χ2 = 73.125, p < 0.001; pairwise with Bonferroni-Holm correction: male/dummy vs. female: χ2 = 45.882, p < 0.001, male vs. dummy: identical values, p = 1). Bioactivity could be restored by reapplying female-derived extracts (Figure 2B; χ2 test: χ2 = 45.882, p < 0.001). The bioassays with fractions of female-derived extracts demonstrated that only the non-polar H fraction containing the CHCs was bioactive on its own, while more polar lipid fractions (D, M) did not elicit any copulation attempts (Figure 3A; χ2 test overall: χ2 = 55.385, p < 0.001; pairwise with Bonferroni-Holm correction: solvent control/D/M vs. H: χ2 = 21.818, p < 0.001, other comparisons: identical values, p = 1). Male CHCs also never elicited copulation behavior (Figure 3B, χ2 test: male CHCs vs. female CHCs: χ2 = 32,308, p < 0.001). Males responded to female CHCs in combinations with the other female-derived extract fractions (Figure 4A, χ2 test overall: χ2 = 57.189, p < 0.001; pairwise comparisons with Bonferroni-Holm correction, solvent control vs. H + DM: χ2 = 32,308; vs. H + D: χ2 = 42,857; vs. H + M: χ2 = 40; all p < 0.001), but there was no difference between male responses, suggesting that the D and M fractions contained no compounds relevant to the pheromone (Figure 4A, pairwise χ2 comparisons with Bonferroni-Holm correction: H + DM vs. H + D: χ2 = 1.491, p = 0.350; H + DM vs. H + M: χ2 = 0.8, p = 0.552; H + D vs. H + M: χ2 = 0.111, p = 1). Furthermore, neither the female-derived nor the male-derived extract fractions D and M influenced male behavior in bioassays combining female CHCs with those male fractions and vice versa. Both mixtures containing female CHCs were significantly more attractive than the mixtures containing male CHCs, which never elicited copulation attempts (Figure 4B, χ2 test overall: χ2 = 70.622, p < 0.001; pairwise comparisons with Bonferroni-Holm correction: ♀H + ♂D vs. ♂H + ♀D/♀M: χ2 = 40, p < 0.001; ♀H + ♂M vs. ♂H + ♀D/♀M: χ2 = 30, p < 0.001; ♀H + ♂D vs. ♀H + ♂M: χ2 = 1.364, p = 0.387).
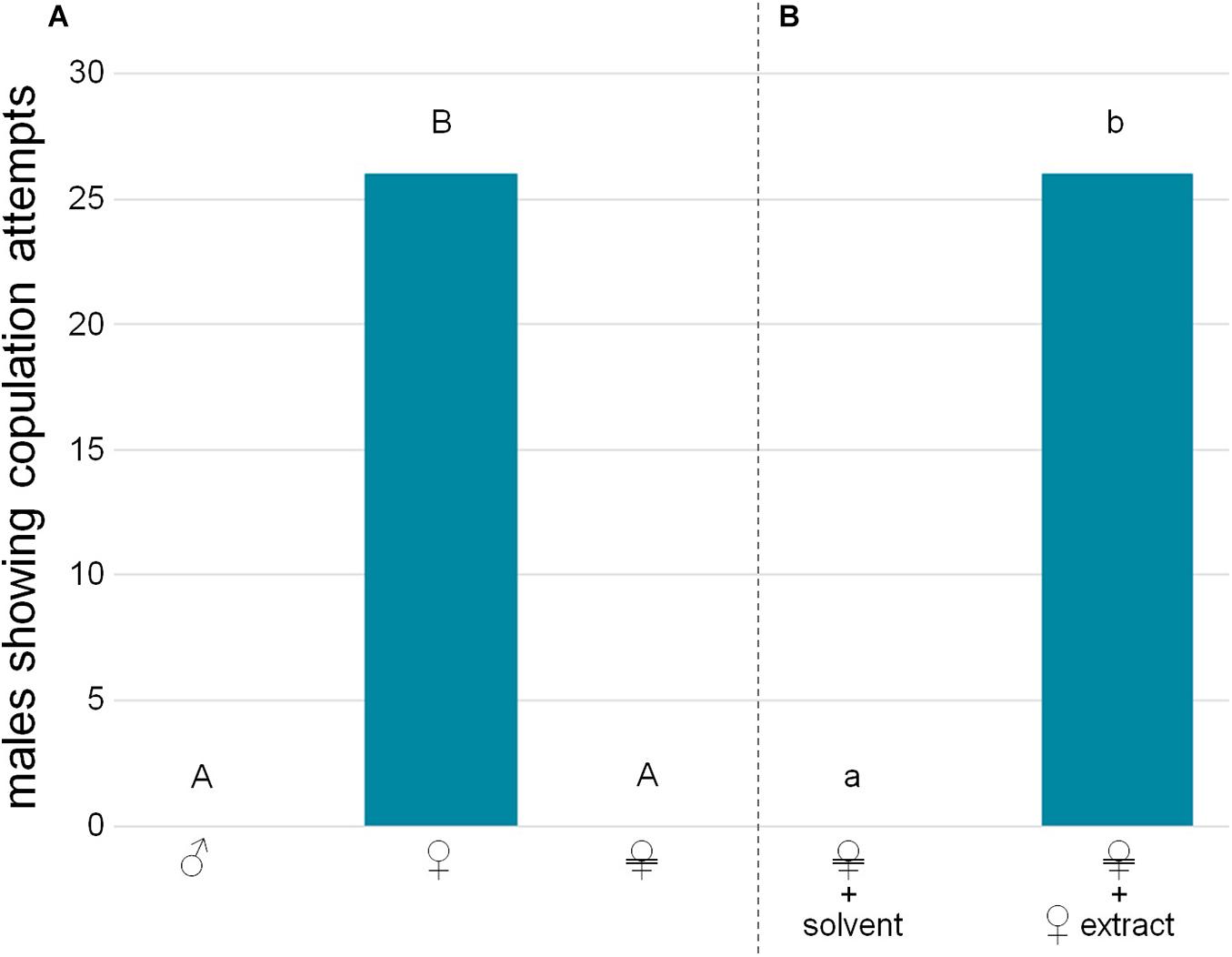
Figure 2. Copulation attempts by virgin males. (A) Males were confronted with a dead male, a dead female, or a Soxhlet-extracted female (female symbol crossed with two horizontal bars). (B) Males were confronted with a Soxhlet-extracted female treated either with solvent (dichloromethane) or female-derived extract (two female equivalents). Significant differences in male reactions are depicted by different letters [χ2 tests, (A) with Bonferroni-Holm correction, capital letters, (B) lower case letters. In all significant cases p < 0.001, n = 30 for each group].
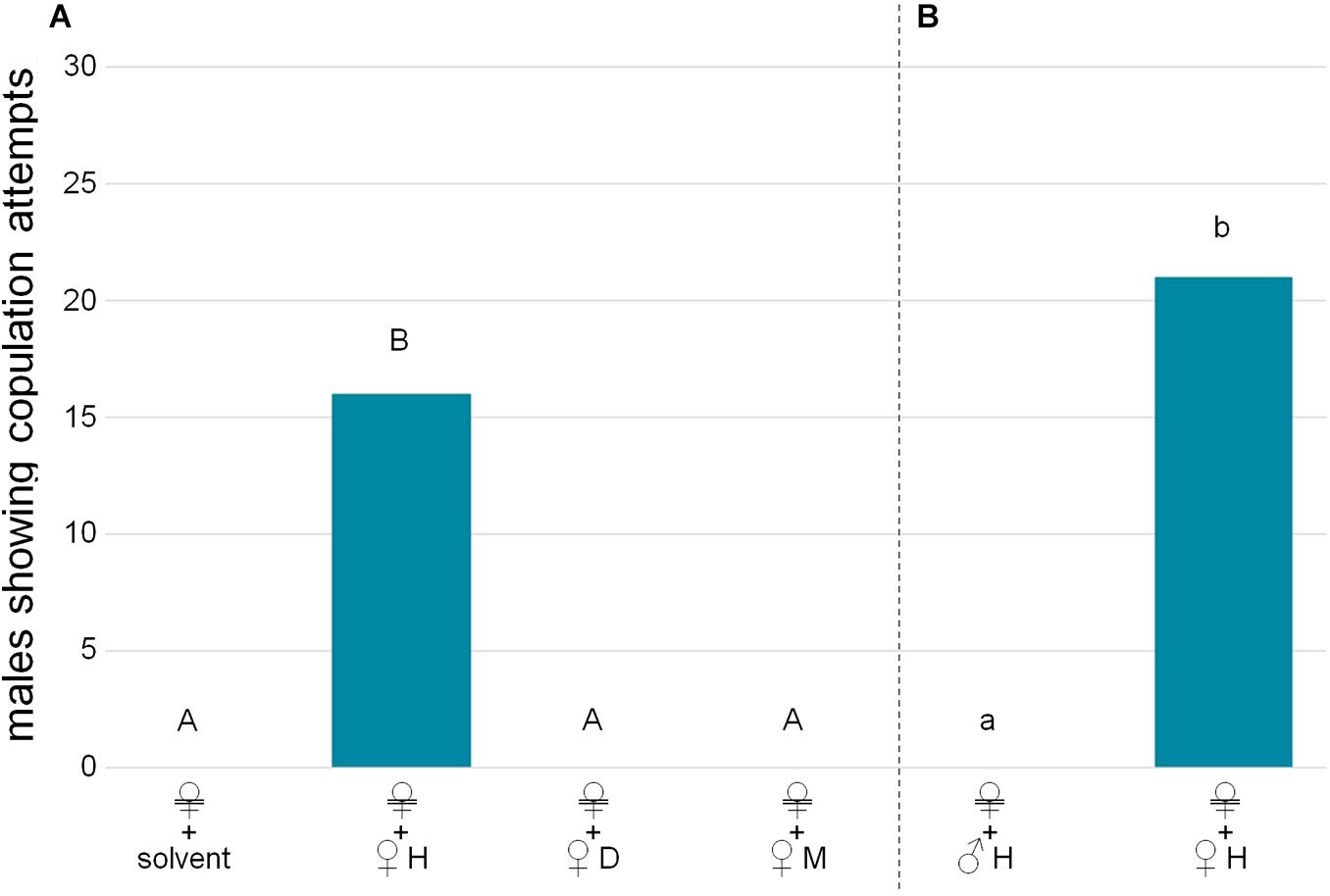
Figure 3. Bioactivity of female-derived extract fractions. Female-derived extracts were applied to a silica gel cartridge and eluted with hexane (H, non-polar fraction containing hydrocarbons), dichloromethane (D, fraction containing lipids of low to medium polarity) and methanol (M, fraction containing lipids of higher polarity). (A) Males were confronted with Soxhlet-extracted females treated either with a solvent control or a female-derived extract fraction (H, D, or M, two female equivalents). (B) As the H fraction was the only fraction bioactive on its own, males were confronted with Soxhlet-extracted females treated with the H fraction of either male-derived extracts or of female-derived extracts (amounts equivalent to two individuals). Significant differences in male reactions are depicted by different letters [χ2 tests, (A) with Bonferroni-Holm correction, capital letters, (B) lower case letters. In all significant cases p < 0.001, n = 30 each].
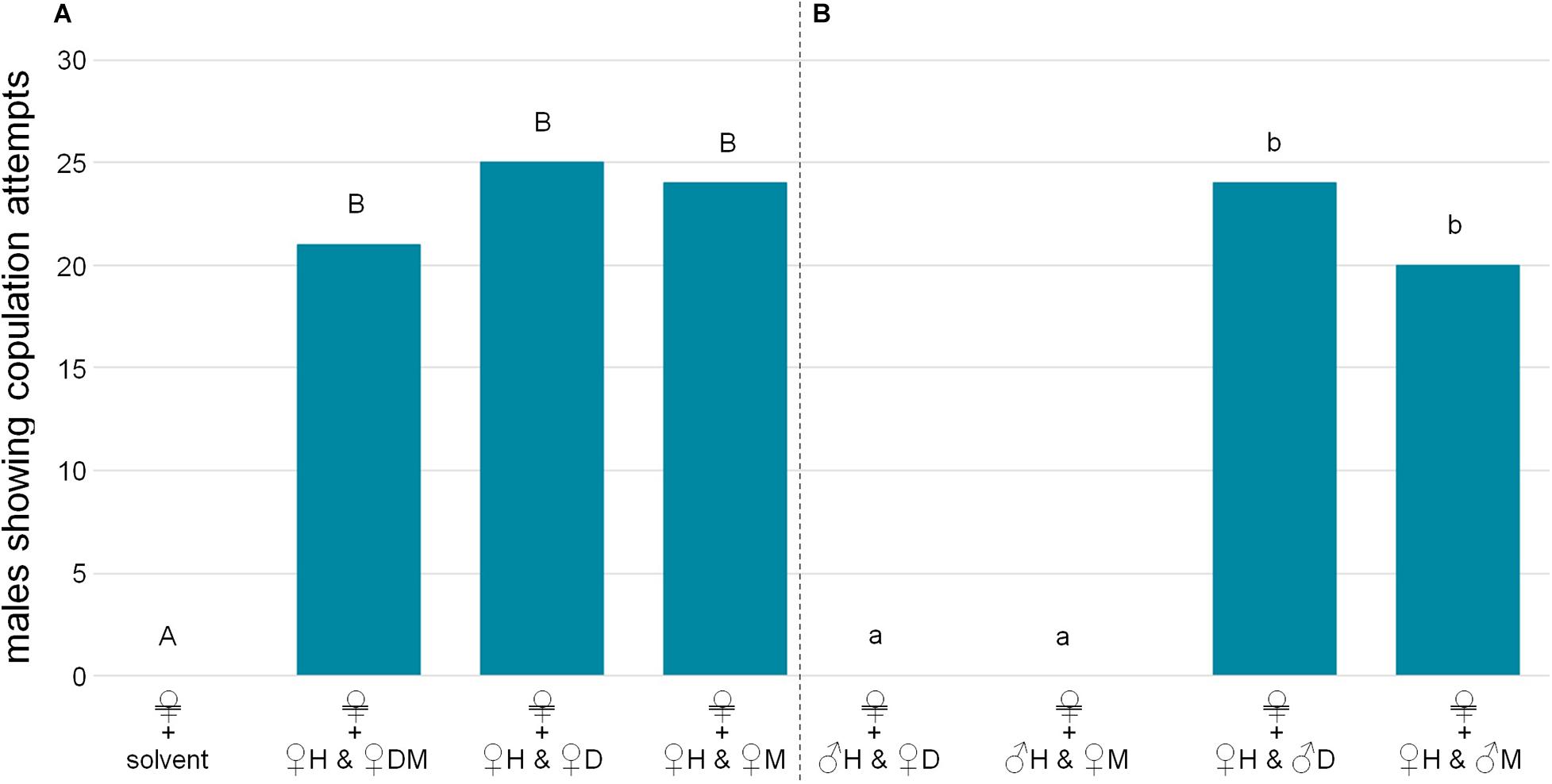
Figure 4. Bioactivity of combinations of extract fractions. Extracts were applied to a silica gel cartridge and eluted with hexane (H, non-polar fraction containing cuticular hydrocarbons), dichloromethane (D, fraction containing lipids of relatively low to medium polarity) and methanol (M, fraction containing lipids of relatively high polarity). (A) Males showed a similar number of copulation attempts toward Soxhlet-extracted females treated with the female-derived H fraction in combination with one or both of the remaining female-derived fractions. (B) The male-derived H fraction in combination with female-derived D or M fractions was not bioactive, while female-derived H fraction elicited copulation attempts despite being presented in combination with male-derived D or M fractions. Significant differences in male reactions are depicted by different letters [χ2 tests with Bonferroni-Holm correction, (A) capital letters, (B) lower case letters. In all significant cases p < 0.001, n = 30 for each group].
Discussion
Cuticular hydrocarbons mediate mate recognition in T. zealandicus. Males only showed copulation behavior toward dead females or dummies treated with female CHCs, be it in the form of crude extracts, after recombining them with more polar fractions, or alone. Male CHCs never elicited sexual behavior from tested males. The addition of more polar fractions from female-derived extracts did not modulate the effectiveness of CHCs in eliciting copulation attempts of males. Furthermore, more polar fractions of female-derived extracts could not render male CHCs attractive, nor could male-derived polar fractions make female CHCs less so. Therefore, we conclude that female-derived CHCs constitute the sex pheromone of this species. Male wasps could easily distinguish male from female CHCs, and only responded to the latter although both sexes of T. zealandicus apparently produce the same CHC compounds in relatively similar relative amounts. While the CHC profiles could be distinguished by multivariate statistical analysis, only a few peaks contributed strongly to the difference between male and female profiles. Each sex produced only one compound that had a relative abundance of more than twice (but less than three times) the amount found in the respective other sex. These are not very obvious differences, and might have been missed without the multivariate analysis. In several other parasitoid wasps, CHCs of either mature males or females comprised at least one exclusive compound, and/or differed more markedly in the relative amounts of shared compounds (Ruther et al., 2011; Kühbandner et al., 2012; Buellesbach et al., 2018; Böttinger et al., 2020). For example, despite otherwise relatively similar profiles, Urolepis rufipes (Hymenoptera, Pteromalidae) females produce 7-methyltritriacontane, which is absent in male CHC profiles, and 7-methylhentriacontane was present at an almost four-fold higher relative abundance in females than in males (Würf et al., 2020). As is the case in the present study, males and females of O. kuvanae produce the same compounds, but differ consistently regarding the relative abundances. Two methyl-branched CHCs were found to be more abundant in males, and, although no values were given, the GC profiles indicated the peaks to be several times larger in males than in females (Ablard et al., 2012). In comparison, the differences in sex specific relative abundances of CHCs in T. zealandicus seem rather small (but see studies on Choetospila elegans (Howard and Liang, 1993) and Cephalonomia hyalinipennis (Howard and Pérez-Lachaud, 2002), where no differences between male and female profiles could be found). Nonetheless, male T. zealandicus could clearly distinguish between male and female CHC profiles. It may be that the profiles are detected as a whole (see Kühbandner et al., 2013; Würf et al., 2020), and even small (but consistent) differences in the relative CHC composition, such as the slightly higher relative amounts of 7-C25:1 and C27 in female profiles, are sufficient for mate recognition in this species. However, the fact that an experimenter can distinguish CHC profiles does not automatically imply that the tested insects do so as well. In the mustard leaf beetle Phaedon cochleariae, CHCs also elicit copulation behavior. While both sexes produce the same set of CHCs, they differ in their relative amounts and were distinguishable by canonical discriminant analysis. Despite this, males showed sexual behavior toward both, dummies treated with either female-derived or male-derived CHCs. Thus, although CHCs elicited sexual behavior, they did not serve for sex discrimination (Geiselhardt et al., 2009). Similar results were found for the two-spot ladybird Adalia bipunctata (Hemptinne et al., 1998) and the black blow fly Phormia regina (Stoffolano et al., 1997). In such cases, other factors, such as behavior (Hemptinne et al., 1998) or physical cues (Geiselhardt et al., 2009) may serve to identify mating partners of the opposite sex.
One often neglected aspect in studies of CHC functions for chemical communication is the potential occurrence of different stereoisomers of methyl-branched CHCs. While the signal function does not have to be enantioselective (see Kühbandner et al., 2013), there are examples in which the stereochemistry of methyl-branched CHCs influences the behavioral reaction. Ablard et al. (2012) showed that different combinations of the stereoisomers of two methyl-branched alkanes more abundant in males of O. kuvanae elicited different reactions in bioassays. Dead females treated with a blend of (S)-5-methylheptacosane and (5R,17S)-5,17-dimethylheptacosane were more attractive to males than untreated dead females, while a treatment with the mixture of (R)-5-methylheptacosane and (5R,17R)-5,17-dimethylheptacosane was repellent in a similar way as dead conspecific males were. Apart from the work on O. kuvanae, several other studies indicate that chiral methyl-branched CHCs are not only produced, but also perceived enantioselectively (Bello et al., 2015; Hughes et al., 2015; Sharma et al., 2015; de Narbonne et al., 2016). This may also apply for T. zealandicus. Future experiments should therefore include bioassays with fractionated CHCs. Fractionation on silver nitrate treated silica gel cartridges, for instance, separates saturated hydrocarbons from mono- and dienes, and a treatment with molecular sieves can thereafter separate methyl-branched and n-alkanes. The compound class fractions can then be tested singly or in combination (Greene and Gordon, 2007; Böröczky et al., 2009; Bello et al., 2015). If methyl-branched CHCs were found to play a role, answering the final question of whether mate recognition might be encoded through specific stereoisomers would require the chemical synthesis and separate testing of the respective compounds (see Ablard et al., 2012; Kühbandner et al., 2013; Hughes et al., 2015; Würf et al., 2020).
There is a large diversity in close range sexual chemical communication, mediated either by key compounds or encoded in complex profiles. While striking differences between male and female CHC profiles often provide a good starting point for investigations, the absence of such sex specific differences does not necessarily preclude CHCs from functioning as sex pheromone during mate recognition. It should therefore not be assumed that small or no detectable differences mean no signal function, and, in the reverse, that detected differences indicate that the study species can and does discriminate on that basis as well. Rather, the mode of signal function should be assessed by systematic bioassays for each respective species.
Data Availability Statement
The raw data supporting the conclusions of this article are available upon request to the corresponding author.
Author Contributions
TP initiated the study, conceived and performed the experiments, analyzed the data, and wrote the manuscript. SJ performed the experiments and analyzed the data. JR contributed to writing the manuscript. All authors contributed to the article and approved the submitted version.
Conflict of Interest
The authors declare that the research was conducted in the absence of any commercial or financial relationships that could be construed as a potential conflict of interest.
Acknowledgments
The authors thank Lea C. Böttinger and Prayan Pokharel for their help in collecting wasps to start the laboratory culture, and the two reviewers for their comments on the manuscript.
References
Ablard, K., Gries, R., Khaskin, G., Schaefer, P. W., and Gries, G. (2012). Does the stereochemistry of methylated cuticular hydrocarbons contribute to mate recognition in the egg parasitoid wasp Ooencyrtus kuvanae? J. Chem. Ecol. 38, 1306–1317. doi: 10.1007/s10886-012-0189-4
Bagnères, A. G., and Wicker-Thomas, C. (2010). “Chemical taxonomy with hydrocarbons,” in Insect Hydrocarbons: Biology, Biochemistry and Chemical Ecology, eds G. J. Blomquist and A. G. Bagnères (Cambridge: Cambridge University Press), 121–162. doi: 10.1017/cbo9780511711909.008
Bello, J. E., McElfresh, J. S., and Millar, J. G. (2015). Isolation and determination of absolute configurations of insect-produced methyl-branched hydrocarbons. Proc. Natl. Acad. Sci. U.S.A. 112, 1077–1082. doi: 10.1073/pnas.1417605112
Blomquist, G. J., and Bagnères, A. G. (2010a). Insect Hydrocarbons: Biology, Biochemistry and Chemical Ecology. Cambridge: Cambridge University Press.
Blomquist, G. J., and Bagnères, A. G. (2010b). “Introduction: history and overview of insect hydrocarbons,” in Insect Hydrocarbons: Biology, Biochemistry and Chemical Ecology, eds G. J. Blomquist and A. G. Bagnères (Cambridge: Cambridge University Press), 3–18. doi: 10.1017/cbo9780511711909.002
Böröczky, K., Crook, D. J., Jones, T. H., Kenny, J. C., Zylstra, K. E., Mastro, V. C., et al. (2009). Monoalkenes as contact sex pheromone components of the woodwasp Sirex noctilio. J. Chem. Ecol. 35, 1202–1211. doi: 10.1007/s10886-009-9693-6
Böttinger, L. C., Hüftlein, F., and Stökl, J. (2020). Mate attraction, chemical defense, and competition avoidance in the parasitoid wasp Leptopilina pacifica. Chemoecology doi: 10.1007/s00049-020-00331-3
Buellesbach, J., Vetter, S. G., and Schmitt, T. (2018). Differences in the reliance on cuticular hydrocarbons as sexual signaling and species discrimination cues in parasitoid wasps. Front. Zool. 15:22. doi: 10.1186/s12983-018-0263-z
Cardé, R. T., and Haynes, K. F. (2004). “Structure of the pheromone communication channel in moths,” in Advances in Insect Chemical Ecology, eds R. T. Cardé and J. G. Millar (Cambridge: Cambridge University Press), 283–332. doi: 10.1017/cbo9780511542664.009
Carlson, D. A., Bernier, U. R., and Sutton, B. D. (1998). Elution patterns from capillary GC for methyl-branched alkanes. J. Chem. Ecol. 24, 1845–1865. doi: 10.1023/a:1022311701355
Carlson, D. A., Mayer, M. S., Silhacek, D. L., James, J. D., Beroza, M., and Bierl, D. A. (1971). Sex attractant pheromone of the house fly: isolation, identification and synthesis. Science 174, 76–78. doi: 10.1126/science.174.4004.76
Carlson, D. A., Roan, C.-S., Yost, R. A., and Hector, J. (1989). Dimethyl disulfide derivatives of long chain alkenes, alkadienes, and alkatrienes for gas chromatography/mass spectrometry. Anal. Chem. 61, 1564–1571. doi: 10.1021/ac00189a019
Clarke, K. R. (1993). Non-parametric multivariate analyses of changes in community structure. Aust. J. Ecol. 18, 117–143. doi: 10.1111/j.1442-9993.1993.tb00438.x
de Narbonne, M. M., Van Zweden, J. S., Bello, J. E., Wenseleers, T., Millar, J. G., and D’Ettorre, P. (2016). Biological activity of the enantiomers of 3-methylhentriacontane, a queen pheromone of the ant Lasius niger. J. Exp. Biol. 219, 1632–1638. doi: 10.1242/jeb.136069
Dunkelblum, E., Tan, S. H., and Silk, P. J. (1985). Double-bond location in monounsaturated fatty acids by dimethyl disulfide derivatization and mass spectrometry: application to analysis of fatty acids in pheromone glands of four lepidoptera. J. Chem. Ecol. 11, 265–277. doi: 10.1007/bf01411414
Ferveur, J. F. (2005). Cuticular hydrocarbons: their evolution and roles in Drosophila pheromonal communication. Behav. Genet. 35, 279–295. doi: 10.1007/s10519-005-3220-5
Geiselhardt, S., Otte, T., and Hilker, M. (2009). The role of cuticular hydrocarbons in male mating behavior of the mustard leaf beetle, Phaedon cochleariae (F.). J. Chem. Ecol. 35, 1162–1171. doi: 10.1007/s10886-009-9704-7
Gibbs, A. G., and Rajpurohit, S. (2010). “Cuticular lipids and water balance,” in Insect Hydrocarbons: Biology, Biochemistry and Chemical Ecology, eds G. J. Blomquist and A. G. Bagnères (Cambridge: Cambridge University Press), 100–120. doi: 10.1017/cbo9780511711909.007
Ginzel, M. D., Blomquist, G. J., Millar, J. G., and Hanks, L. M. (2003). Role of contact pheromones in mate recognition in Xylotrechus colonus. J. Chem. Ecol. 29, 533–545. doi: 10.1023/A:1022894419521
Ginzel, M. D., Moreira, J. A., Ray, A. M., Millar, J. G., and Hanks, L. M. (2006). (Z)-9-nonacosene – major component of the contact sex pheromone of the beetle Megacyllene caryae. J. Chem. Ecol. 32, 435–451. doi: 10.1007/s10886-005-9010-y
Greene, M. J., and Gordon, D. M. (2007). Structural complexity of chemical recognition cues affects the perception of group membership in the ants Linephithema humile and Aphaenogaster cockerelli. J. Exp. Biol. 210, 897–905. doi: 10.1242/jeb.02706
Guillem, R. M., Drijfhout, F. P., and Martin, S. J. (2016). Species-specific cuticular hydrocarbon stability within European Myrmica ants. J. Chem. Ecol. 42, 1052–1062. doi: 10.1007/s10886-016-0784-x
Hemptinne, J.-L., Lognay, G., and Dixon, A. F. G. (1998). Mate recognition in the two-spot ladybird beetle, Adalia bipunctata: role of chemical and behavioural cues. J. Insect Physiol. 44, 1163–1171. doi: 10.1016/s0022-1910(98)00081-x
Howard, R. W. (1998). Ontogenetic, reproductive, and nutritional effects of the cuticular hydrocarbons of the host-specific ectoparasitoid Cephalonomia tarsalis (Hymenoptera: Bethylidae). Ann. Entomol. Soc. Am. 91, 101–112. doi: 10.1093/aesa/91.1.101
Howard, R. W., and Blomquist, G. J. (2005). Ecological, behavioral, and biochemical aspects of insect hydrocarbons. Annu. Rev. Entomol. 50, 371–393. doi: 10.1146/annurev.ento.50.071803.130359
Howard, R. W., and Liang, Y. (1993). Cuticular hydrocarbons of winged and wingless morphs of the ectoparasitoid Choetospila elegans Westwood (Hymenoptera: Pteromalidae) and its host, larval lesser grain borer (Rhyzopertha dominica) (Coleoptera: Bostrichidae) comp. Biochem. Physiol. B 106, 407–414. doi: 10.1016/0305-0491(93)90321-U
Howard, R. W., and Pérez-Lachaud, G. (2002). Cuticular hydrocarbons of the ectoparasitic wasp Cephalonomia hyalinipennis (Hymenoptera: Bethylidae) and its alternative host, the stored product pest Caulophilus oryzae (Coleoptera: Curculionidae). Arch. Insect Biochem. Physiol. 50, 75–84. doi: 10.1002/arch.10034
Hughes, G. P., Bello, J. E., Millar, J. G., and Ginzel, M. D. (2015). Determination of the absolute configuration of female-produced contact sex pheromone components of the longhorned beetle, Neoclytus acuminatus acuminatus (F). J. Chem. Ecol. 41, 1050–1057. doi: 10.1007/s10886-015-0639-x
Kühbandner, S., Bello, J. E., Mori, K., Millar, J. G., and Ruther, J. (2013). Elucidating structure-bioactivity relationships of methyl-branched alkanes in the contact sex pheromone of the parasitic wasp Lariophagus distinguendus. Insects 4, 743–760. doi: 10.3390/insects4040743
Kühbandner, S., Sperling, S., Mori, K., and Ruther, J. (2012). Deciphering the signature of cuticular lipids with contact sex pheromone function of a parasitic wasp. J. Exp. Biol. 215, 2471–2478. doi: 10.1242/jeb.071217
Lacey, E. S., Ginzel, M. D., Millar, J. G., and Hanks, L. M. (2008). 7-methylheptacosane is a major component of the contact sex pheromone of the cerambycid beetle Neoclytus acuminatus acuminatus. Physiol. Entomol. 33, 209–216. doi: 10.1111/j.1365-3032.2008.00624.x
Leonhardt, S. D., Menzel, F., Nehring, V., and Schmitt, T. (2016). Ecology and evolution of communication in social insects. Cell 164, 1277–1287. doi: 10.1016/j.cell.2016.01.035
Mitra, A., Palavalli Nettimi, R., Ramachandran, A., Saha, P., and Gadagkar, R. (2015). Males and females of the social wasp Ropalidia marginata do not differ in their cuticular hydrocarbon profiles and do not seem to use any long-distance volatile mate attraction cues. Insect Soc. 62, 281–289. doi: 10.1007/s00040-015-0408-4
Moretti, T. D. C., Ribeiro, O. B., Thyssen, P. J., and Solis, D. R. (2008). Insects on decomposing carcasses of small rodents in a secondary forest in Southeastern Brazil. Eur. J. Entomol. 105, 691–696. doi: 10.14411/eje.2008.094
Olaniran, O. A., Sudhakar, A. V. S., Drijfhout, F. P., Dublon, I. A. N., Hall, D. R., Hamilton, J. G. C., et al. (2013). A male-predominant cuticular hydrocarbon, 7-methyltricosane, is used as a contact pheromone in the western flower thrips Frankliniella occidentalis. J. Chem. Ecol. 39, 559–568. doi: 10.1007/s10886-013-0272-5
Olton, G. S., and Legner, E. F. (1974). Biology of Tachinaephagus zealandicus (Hymenoptera: Encyrtidae), parasitoid of synanthropic diptera. Can. Entomol. 106, 785–800. doi: 10.4039/Ent106785-8
Peterson, M. A., Dobler, S., Larson, E. L., Juárez, D., Schlarbaum, T., Monsen, K. J., et al. (2007). Profiles of cuticular hydrocarbons mediate male mate choice and sexual isolation between hybridising Chrysochus (Coleoptera: Chrysomelidae). Chemoecology 17, 87–96. doi: 10.1007/s00049-007-0366-z
R Core Team. (2019). R: A Language and Environment for Statistical Computing. Vienna: R Foundation for Statistical Computing.
Ruther, J., Döring, M., and Steiner, S. (2011). Cuticular hydrocarbons as contact sex pheromone in the parasitoid Dibrachys cavus. Entomol. Exp. Appl. 140, 59–68. doi: 10.1111/j.1570-7458.2011.01129.x
Sharma, K., Enzmann, B., Schmidt, Y., Moore, D., Jones, G., Parker, J., et al. (2015). Cuticular hydrocarbon pheromones for social behavior and their coding in the ant antenna. Cell Rep. 12, 1261–1271. doi: 10.1016/j.celrep.2015.07.031
Silk, P. J., Ryall, K., Lyons, D. B., Sweeney, J., and Wu, J. P. (2009). A contact sex pheromone component of the emerald ash borer Agrilus planipennis Fairmaire (Coleoptera: Buprestidae). Naturwissenschaften 96, 601–608. doi: 10.1007/s00114-009-0513-1
Snellings, Y., Herrera, B., Wildemann, B., Beelen, M., Zwarts, L., Wenseleers, T., et al. (2018). The role of cuticular hydrocarbons in mate recognition in Drosophila suzukii. Sci. Rep. 8:4996. doi: 10.1038/s41598-018-23189-6
Steiner, S., Hermann, N., and Ruther, J. (2006). Characterization of a female-produced courtship pheromone in the parasitoid Nasonia vitripennis. J. Chem. Ecol. 32, 1687–1702. doi: 10.1007/s10886-006-9102-3
Stoffolano, J. G., Schauber, E., Yin, C.-M., Tillman, J. A., and Blomquist, G. J. (1997). Cuticular hydrocarbons and their role in copulatory behavior in Phormia regina (Meigen). J. Insect Phys. 43, 1065–1076. doi: 10.1016/S0022-1910(97)00050-4
Stökl, J., Dandekar, A.-T., and Ruther, J. (2014). High chemical diversity in a wasp pheromone: a blend of methyl 6-methylsalicylate, fatty alcohol acetates and cuticular hydrocarbons releases courtship behavior in the Drosophila parasitoid Asobara tabida J. Chem. Ecol. 40, 159–168. doi: 10.1007/s10886-014-0378-4
Thomas, M. L., and Simmons, L. W. (2008). Sexual dimorphism in cuticular hydrocarbons of the Australian field cricket Teleogryllus oceanicus (Orthoptera: Gryllidae). J. Insect Phys. 54, 1081–1089. doi: 10.1016/j.jinsphys.2008.04.012
van den Assem, J. (1976). Male courtship behaviour, female receptivity signal, and size differences between the sexes in Pteromalinae (Hym., Chalcidoidea Pteromolidae), and comparative notes on other Chalcidoids. Neth. J. Zool. 26, 535–548. doi: 10.1163/002829676x00208
Weiss, I., Hofferberth, J., Ruther, J., and Stökl, J. (2015). Varying importance of cuticular hydrocarbons and iridoids in the species-specific mate recognition pheromones of three closely related Leptopilina species. Front. Ecol. Evol. 3:19. doi: 10.3389/fevo.2015.00019
Würf, J., Pokorny, T., Wittbrodt, J., Millar, J. G., and Ruther, J. (2020). Cuticular hydrocarbons as contact sex pheromone in the parasitoid wasp Urolepis rufipes. Front. Ecol. Evol. 8:180. doi: 10.3389/fevo.202000180
Keywords: cuticular hydrocarbons, sex pheromone, chemical communication, mate recognition, Tachinaephagus zealandicus
Citation: Jungwirth S, Ruther J and Pokorny T (2021) Similar Is Not the Same – Mate Recognition in a Parasitoid Wasp. Front. Ecol. Evol. 9:646667. doi: 10.3389/fevo.2021.646667
Received: 27 December 2020; Accepted: 03 March 2021;
Published: 22 March 2021.
Edited by:
William Benjamin Walker III, Temperate Tree Fruit and Vegetable Research Unit, Agricultural Research Service, United States Department of Agriculture (USDA-ARS), United StatesReviewed by:
Federico Cappa, University of Florence, ItalyFlorian Menzel, Johannes Gutenberg University Mainz, Germany
Copyright © 2021 Jungwirth, Ruther and Pokorny. This is an open-access article distributed under the terms of the Creative Commons Attribution License (CC BY). The use, distribution or reproduction in other forums is permitted, provided the original author(s) and the copyright owner(s) are credited and that the original publication in this journal is cited, in accordance with accepted academic practice. No use, distribution or reproduction is permitted which does not comply with these terms.
*Correspondence: Tamara Pokorny, tamara.pokorny@biologie.uni-regensburg.de