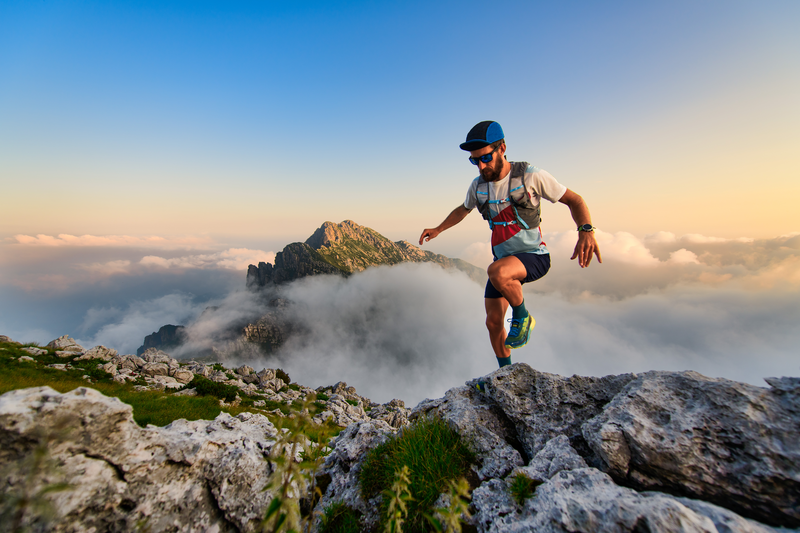
94% of researchers rate our articles as excellent or good
Learn more about the work of our research integrity team to safeguard the quality of each article we publish.
Find out more
ORIGINAL RESEARCH article
Front. Ecol. Evol. , 15 March 2021
Sec. Paleontology
Volume 9 - 2021 | https://doi.org/10.3389/fevo.2021.642814
This article is part of the Research Topic Recent Advances in the Evolution of Euarchontoglires View all 15 articles
Fossil records generally inform paleobiologists about extinct taxa and rates of evolution measured at the scale of millions of years. Good records that are densely sampled through time can reveal species level details such as longevity in local sections. Yet fossil data normally do not address details of lineage microevolution because the density through time of lineage sampling is insufficient to perceive patterns at a precision finer than 106 years in most cases. This study concerns details of a splitting event in the evolution of murine rodents, an event for which multiple fossil samples dated to a precision of 105 years fortuitously document the tempo and mode of origin of sister species, the stems of two extant tribes of mice. Evolution of early Murinae in the northern part of the biogeographically restricted Indian subcontinent between 11.6 and 10.5 Ma involved cladogenesis of two crown taxa, the extant tribes Murini and Arvicanthini. Large samples of fossil rodent teeth document their divergence from a common morphological pool. Definitive basal Murini and Arvicanthini at 10.5 Ma are similar in size and differ by subtle features of the dentition. Those features occur sporadically in the common pool of older fossil teeth at 11.2, 11.4, and 11.6 Ma as inconsistent polymorphisms. Interpreted as a single lineage in the 11.6–11.2 Ma interval, variability of this abundant murine incorporated the roots of the two crown tribes. The pattern through time suggests morphological stasis for several hundred thousand years prior to splitting. This special case informs us on one example of evolution and shows that the tempo of splitting evolution in some cases may be measured in hundreds of thousands of years, followed by stasis once daughter species have differentiated morphologically.
Gould (1985) and Reznick and Ricklefs (2009) discussed the importance of the fossil record for evolutionary biology in unveiling major events, especially those under the category of macroevolution. Generally, paleontology does not address details on how evolution proceeds at the population level because relevant data are not sampled densely enough in space and time. In special circumstances, however, the fossil record may be detailed enough to address aspects of evolution that lie between macro- and microevolution, such as species longevity, changes in abundance, and rates of morphological change. The potential for approaching microevolutionary patterns through the fossil record is great for muroid rodents in cases where stratigraphy and dating are well resolved, and multiple large samples of individuals are available.
Resolution of muroid rodent phylogenetics has had a quantum advance with the application of mitochondrial and nuclear genetic analyses. Steppan and Schenk (2017) resolved a massive amount of data to develop a well-supported hypothesis on relationships of over 900 species. This and previous works (e.g., Lecompte et al., 2008) distinguished major clades of muroids at the family, subfamily, and tribe levels, including tribes Arvicanthini and Murini, both of which have fossil members in South Asia.
Here we set out to interpret a unique fossil record for its relevance to a splitting event that marked the origin of the extant murine rodent tribes Arvicanthini and Murini. Sediments from the Siwalik Group of Pakistan yield a well dated and resolved series of fossil mouse assemblages. We have developed the alpha taxonomy of these species in a series of papers (Jacobs, 1977, 1978; Cheema et al., 2000; Kimura et al., 2015, 2017) and specified the timing of morphological divergence and the emergence of features involved in that divergence (Kimura et al., 2013a,b; Flynn et al., 2020). Early late Miocene Siwalik species assigned to the stem genera Karnimata and Progonomys, respectively, fortuitously capture the rise of distinctive morphological features that by 10.5–10 million years ago (Ma) characterize dentitions of members of the tribes Arvicanthini and Murini. These features occur in fossil samples from the same geographic area before 11 Ma (Flynn et al., 2020), but inconsistently so that species level taxonomy at that age is problematic.
We contend that the common origin of the Arvicanthini and Murini was recorded in South Asia in Siwalik fossil samples between 12 and 11 Ma. The distinct morphologies of the tribes expressed clearly in fossil species of Karnimata and Progonomys dating to 10.5 Ma and younger occur across a mix of individuals in the 11.6–11.2 Ma interval that are otherwise inseparable. These appear to be polymorphisms that were stable for hundreds of thousands of years and later characterized the extant tribes.
Our purpose is to document the simultaneous existence of arvicanthin and murinin morphologies in a series of samples from single localities of early late Miocene age, discuss the relevance of their splitting origin within a refined chronology of mouse evolution, and thereby address the tempo and mode of this special event recorded in the fossil record. We interpret these fossils to represent the common ancestral lineage for the two living mouse tribes. Possibly, they represent individuals of closely related cryptic species that did not differ in any other features preserved in the fossils. In either case the time frame for morphological separation of the two tribes is measured in hundreds of thousands of years.
The significance of understanding the tempo and mode of the specific cladogenic event we are studying is that it provides a more confident and precise date for an evolutionary divergence that is relevant for calibrating the molecular phylogeny of essentially every living species of one of the most diverse and speciose groups of mammals in existence, the true mice or Murinae. This group is native to the Old World, including Africa, Australia, and Pacific islands, and global as commensals. It contains the laboratory mouse (Mus) and laboratory rat (Rattus), long the subject of innumerable studies (da Costa et al., 2019), the former with more than forty living wild species (Suzuki, 2020) and the latter with a confusingly large number of cryptic species (Pages et al., 2010; Rowe et al., 2011). Because fossil murines are known mainly from isolated teeth, the spectrum of morphological characters is limited, which restricts the application of standard morphology-based cladistic analysis. However, the backbone provided by molecular phylogenies of the vast number of living species allows fossil mice to be related confidently.
The fossil mice from Pakistan have long been recognized as relevant to calibrating molecular evolutionary hypotheses (Jacobs and Pilbeam, 1980; Jacobs and Flynn, 2005), but earlier studies have been much improved upon by more comprehensive work (e.g., Kimura et al., 2015; Aghová et al., 2018) and the addition of newly studied fossil samples (e.g., Kimura et al., 2017). Divergence dates from Pakistan have been useful in molecular sequence studies applied to biogeography, ecology, and evolutionary patterns (e.g., Rowe et al., 2016, 2019; Bryja et al., 2017), as well as studies of pathogens and genetic mechanisms (e.g., Casola, 2018; Ma et al., 2018), and many other topics. This study contributes by providing a more explicit description of a fossil-based cladogenic event in a precise chronological framework.
The source of the fossil data is a biostratigraphy of successive rodent sites from the Miocene formations of the Siwalik Group of South Asia. Here we focus on Miocene age sites that are dated 12.5–10 Ma. Each site produces a sample of isolated rodent teeth concentrated most likely by avian predators of the time. Sites contain remains of individuals that died (and are time-averaged) over a decadal to centennial scale. The fossil rodent fauna is represented by squirrels, primitive burrowing rats, hamsters, gerbils, gundis, dormice, and dominant true mice. The series of sites that captures the cladogenic event spans at least 400,000 years from 11.6 to 11.2 Ma. Fossil sites are concentrated in a relatively small area of 200 km2 near Chinji and Nagri villages on the Potwar Plateau of northern Pakistan (area B in Barry et al., 2002: their Figure 1).
The Potwar Plateau in northern Pakistan presents an extraordinarily continuous record of Siwalik sediments shed from deformed highlands to the north, the present day mountain ranges bordering the Tibetan Plateau. Drainages carrying detritus southward continue to the present day as components of the mighty Indus River system. The highlands, principally related to the Himalayan and Hindu Kush mountain ranges, have been uplifted continuously throughout the Cenozoic Era by the tectonic forces that thrust the Indian Plate northward under the southern margin of Asia. Currently at 33° North latitude, the area has moved northward with the Indian plate by about 4° since 11 Ma (Tauxe and Opdyke, 1982). Erosional detritus carried southward and accumulating on pre-Indus floodplains (over 3000 m thick on the Potwar Plateau; Tauxe and Opdyke, 1982) comprise layers of rock of varied lithology, formations of the Siwalik Group. The layers of rock of most interest here, mainly Miocene in age, occur in a broad belt across Indo-Pakistan and as exposed on the Potwar Plateau are ∼18–6 million years old (18–6 Ma). The Siwaliks are important for evolutionary biology because the sediments entomb rich samples of the vertebrate biota that populated the region through the Miocene. Fossil bones and teeth are preserved at many levels in the sequence, with the potential that remains of populations in single lineages can be sequentially sampled through time.
The dating of terrestrial fossils through magnetic polarity stratigraphy began in the early 1970’s. At that time a consortium of universities began paleontological, stratigraphic, and paleomagnetic studies on the Potwar Plateau because of its fossil abundance known since the 19th Century, its very long fossiliferous stratigraphic section related to the uplift of the Himalaya, and as a focus to develop and validate magnetic polarity stratigraphy in developing a global polarity timescale. From those beginnings, the tradition of precise dating applied to terrestrial paleontology, evolution, and paleoecological studies in the Siwaliks has continued (Keller et al., 1977; Tauxe and Opdyke, 1982; Johnson et al., 1985).
This analysis crucially depends on dated fossil sites with resolution up to 100,000 year precision (see Barry et al., 2002). The dating method involves careful stratigraphic correlation of fossil sites to a master stratigraphic section, in which the paleomagnetic properties of successive samples of rock throughout the stratigraphic sequence build a composite magnetostratigraphy for the Siwaliks. Due to the completeness of the Potwar record, the magnetostratigraphy can be matched to the dated global geomagnetic polarity time scale, yielding age estimates for the rocks (Barry et al., 2013). Estimates are not direct dates, but the dates are relative to one another and distinguishable at a scale of 105 years. Because Siwalik strata are generally well exposed, individual fossil localities can be dated in this fashion. This method is widely applied globally (e.g., Garcés et al., 2003; Tedford et al., 2013; Van Dam et al., 2014; García-Paredes et al., 2016).
Small mammal fossils accumulated under special conditions at a number of Potwar localities. They are dominantly dental remains presumably accumulated by raptors (mostly owls) in riparian settings. Such conditions did not occur everywhere, so fossil sites are not uniformly distributed through the composite geological section (Figure 1)–but they occur densely enough in time to sample details of lineage evolution. Fossils were recovered by wet screening large quantities of fossiliferous sediment from promising localities that usually showed small bone fragments on the ground surface. After sieving, the concentrate was sorted under magnification and isolated teeth were retrieved. Samples of individual species range from a few to several tens of teeth per site. For the stratigraphic interval capturing the time of divergence of major murine groups, Table 1 shows the ages of localities, the number of rodent fossils recovered from each, and the number of those rodents that are murines. With fossils, more complete is always better than less complete, but our use of isolated teeth was necessitated because that is the nature of the samples and is mitigated by the observation that living murine rodent taxa are commonly characterized by molars (e.g., Misonne, 1969).
Figure 1. Age and stratigraphic sequence of Potwar Plateau rodent localities of the middle and late Miocene with a time scale in millions of years (Ma); key localities plotted on left. Relative abundances of each taxon and morphotype shown for each stratigraphic level. Pattern reflects evolutionary divergence and diversification of Siwalik Murinae based on species distributions through time. Stem Murinae are succeeded after 11 Ma by crown Murini represented on the one hand by Progonomys and its more derived sister clades (Aghová et al., 2018) and on the other hand by crown Arvicanthini represented by Karnimata and its more derived sister clades. Columns indicate relative abundances among all contemporary murines. The color scheme is maintained in later figures.
Table 1. Potwar Plateau rodent localities utilized in this study, their ages, and fossil sample sizes.
Siwalik fossil teeth recovered by screening represent mostly rodents, but include hedgehogs, shrews, bats, and a few other mammals. The most abundant rodents retrieved are murine mice, and these, like all crown muroids, have only molars in each quadrant behind the enlarged procumbent incisor. The largest and best represented of the molars is the first upper molar (M1). Because it is large (by mouse standards) and relatively complex, this tooth locus presents diagnostic characters and is the most informative of the molars for systematic study. In some cases, the first lower molar (m1) is also useful.
Middle Miocene fossils represent stem murines, but exhibit the apomorphic characteristics of the subfamily, namely extra cusps in M1 and a twinned anteroconid plus labial cingulum in m1 (Figure 2). These features are derived, absent in muroid outgroups. The extra cusps in M1 (anterostyle and enterostyle behind it) are lingual in position and constitute, with neighboring cusps, transverse sectorial chevrons. In middle Miocene taxa, the chevrons are poorly developed: most Potwarmus (bottom of Figure 1) lack the anterostyle, and in Antemus the enterostyle is unconnected with its chevron. Jacobs et al. (1989) described the considerable morphological variation in Antemus M1 for a large sample from locality Y491 (13.8 Ma). Toward the end of the middle Miocene (12.3 Ma) the early murine lineage, by then advanced over Antemus, exhibited full chevrons on M1, but with low ridges weakly connecting neighboring cusps. In none of these samples do we observe definitive features of crown murine tribes.
Figure 2. Diagrams of typical murine first molars, upper (above, M1) and lower (below, m1), with cusp nomenclature. Major cusps are labeled, anterior is to the left. Upper molar shows cusps arranged in transverse chevrons; lower molar shows six major cusps in opposed pairs. The axis of the upper molar metacone-hypocone complex is termed a “metaloph.”
Beginning with samples at 11.6 Ma, the debut of the late Miocene, we observe sporadic occurrence of advanced M1 features (as below) that characterize crown mouse tribes. Yet these traits occur in samples of teeth that are otherwise homogeneous. Characteristic features of Murini and Arvicanthini appear to be randomly distributed among the specimens. These mouse teeth represent either a single lineage with considerable variation, or two lineages that are cryptic as far as fossil teeth are concerned.
The key feature of the M1 that characterizes the Murini concerns the anterostyle (Jacobs, 1978). This lingual cusp is associated with the first chevron. In Murini the cusp is shifted posteriorly, its crest running anterolabially to the double anterocone. The anterior part of the M1 is therefore transversely narrow. In addition to its posterior position, the anterostyle is not a circular cusp, but relatively laterally compressed. Molar cusps are distinctly inclined posteriorly.
This combination of features appears in some teeth as a variation in fossil samples of 11.6–11.2 Ma. By 10.5–10.1 Ma, it is stable. Samples of that age and younger are readily assigned to the genus Progonomys. By the end of the Miocene, the derivative living genus Mus shows a strongly shifted anterostyle, a narrow anterior shelf surmounted by the double anterocone, and a reduced posterior cingulum (Jacobs, 1978).
This group conserved the primitive anterostyle position, not strongly repositioned posterolingual to the double anterocone. However, arvicanthins developed progressively a separately derived feature; this concerns the metacone cusp (Kimura et al., 2015). A large metacone that is posteriorly inclined, similar to the paracone, is a primitive condition for mice. The posterior inclination is most obvious in lateral view. Arvicanthins progressively fix a derived condition of the metacone, which is vertical and diminished in size. Most specimens older than 11 Ma show the primitive metacone condition, but some have a modified metacone, smaller than the paracone. By 9.2 Ma, approximately 25% of Karnimata darwini show the fully transformed metacone. Progressive development of the derived metacone in the Karnimata lineage without similar change in the posterostyle, led Kimura et al. (2015) to recognize that the genus represents a stem in the Arvicanthini-Otomyini-Millardiini clade.
Temporal change in the relative abundances of the major components of the Siwalik murine rodents in Figure 1 is based on the Harvard University-Geological Survey of Pakistan database (e.g., Barry et al., 2013), which is continuously updated. Although the master database includes fossils representing all tooth positions, we analyzed a subset (extracted December, 2020) of only upper first molars (M1) for those taxa with total number of specimens of ten or more. Complexity of M1 maximizes precision of taxonomic assignment for that tooth. The relative abundance of M1 was calculated as the number of fossils in a species (taxonomic unit) relative to the total number of murine fossils. The stratigraphic diagrams were generated with 0.1 m.y. (105 year) time bins by the strat.plot function in the package rioja (Juggins, 2020) implemented in R (R Core Team, 2020).
To visualize change through time of dental characters as they were fixed in the Mus-Arvicanthis divergence (Figure 3 and Tables 2–5), we obtained the measurements of four morphometric traits of M1 from time-ordered and sequential samples: the ratio of the major and minor axes of the anterostyle; the acute angle of the anterostyle relative to the longitudinal tooth axis; the acute angle between a line connecting the protocone-enterocone and the longitudinal tooth axis; and the acute angle of the metaloph (line through the major axis of the metacone) relative to the longitudinal tooth axis. The datasets of Kimura et al. (2013b) are combined with the latter feature, which is newly obtained for this study. Taxonomic assignments of Kimura et al. (2013b) are updated following Kimura et al. (2015, 2016, 2017) and Flynn et al. (2020). Previously, in Kimura et al. (2013b), we calculated the 95% bias-corrected and accelerated bootstrap (BCa) confidence intervals for groups with more than three entries (i.e., taxa in different time slices are treated as separate groups), and only these data are plotted in Figure 9 of Kimura et al. (2013b). In this study, we calculated both 95% bootstrap confidence intervals for groups with more than two entries. The choice of the bootstrap method did not affect our conclusions. In the bootstrap permutation, 9999 replicates were resampled. The bootstrap confidence intervals were calculated in the R package boot (Canty and Ripley, 2020) using the boot.ci function. All data are plotted in Figure 3. Due to updates in taxonomic assignments, the different bootstrap calculation method, and the selection of groups plotted in Figure 3, some details differ slightly from that of Kimura et al. (2013b, Figure 9).
Figure 3. Temporal change and variation in four morphometric characters in Siwalik murines. (A) Axis ratio of anterostyle shape (length of the minor axis relative to that of the major axis). (B) Acute angle between the anterostyle and the longitudinal axis of the tooth. (C) Acute angle formed by a line connecting the centers of protocone and enterostyle with the longitudinal axis of the tooth. (D) Acute angle between the major axis of the metacone (forms a metaloph with the hypocone) and the longitudinal axis of the tooth. Error bars indicate 95% bootstrap confidence intervals. The specimen illustrated is Progonomys debruijni (YGSP 7740) in early wear. Versions of panels (A–C) initially in Kimura et al. (2013b), updated here with revised taxonomy; panel (D) is new. Error bars represent 95% confidence intervals.
Table 2. Summary statistics for anterostyle ratio in Siwalik murine rodents, corresponding to Figure 3A.
Table 3. Summary statistics for anterostyle angle in Siwalik murine rodents, corresponding to Figure 3B.
Table 4. Summary statistics for angle formed by the protocone and enterostyle in Siwalik murine rodents, corresponding to Figure 3C.
Table 5. Summary statistics for axis angle through metacone in Siwalik murine rodents, corresponding to Figure 3D.
A time-calibrated tree (discussed below) was generated using “Stratigraphic Tree Analysis for Paleontology” (strap) in R package for proposed relationships of Siwalik murines. We constructed a tree file (.tre) with a single tree as our hypothesis by manually editing tree shape in Mesquite version 3.04 (Maddison and Maddison, 2017) based against an updated review of ranges of Siwalik fossils. For time calibration, first and last appearances were compiled as the lowermost and uppermost stratigraphic occurrences of the taxa. In the R package “strap,” the equal scaling method was used in the DatePhylo function with a root length (i.e., length of the base of the tree) of 1 million. Although the time-scaling algorithm of the “equal” method is tuned to avoid zero-length branches instead of generating best estimates of divergence times, the model ages of the fossil calibration points are consistent with our previous proposals and molecular estimates (Lecompte et al., 2008; Aghová et al., 2018), and support the proposition that the density of sampled Siwalik fossils may be sufficient to capture transitional morphological changes near divergences.
Previously, we (Flynn et al., 2020) found a good match between the stratigraphic record of clade appearances and the node age estimates from the molecular time tree of Lecompte et al. (2008). Nevertheless, the Siwalik murine record has obtained more detail in recent years from review and analysis of carefully controlled data for over 3600 specimens and therefore the evolution of murines can be traced in more detail. In samples of 9.2 Ma (locality Y182), Jacobs (1978) recognized two clearly separate species, Progonomys debruijni and K. darwini, now recognized to represent Murini in the former case and Arvicanthini in the latter (Figure 4). P. debruijni had the murine anterostyle shifted and laterally compressed. All cusps showed posterior inclination and P. debruijni was the smaller of the two. The other mouse K. darwini was clearly larger with a plesiomorphic anterostyle. Kimura et al. (2013b, 2015) recognized the significance of the morphological distinctions between Progonomys and Karnimata and distinguished a number of other dental features, including isotopic differences (Kimura et al., 2013a), that set the lineages apart especially after 9 Ma.
Figure 4. Upper first molars of the dominant murines present at locality Y182, 9.2 Ma. (A) Right M1 of Karnimata darwini, specimen YGSP 7720, and (B) smaller left M1 of Progonomys debruijni, YGSP 7736. Both at same 1 mm scale, anterior is upward.
In older samples dating to 10.5–10.1 Ma (localities Y259, 450, 311), the murines resembled the 9.2 Ma samples, but represented more primitive species, which Kimura et al. (2017) recognized as Progonomys hussaini and Karnimata fejfari (Figure 5). These earlier representatives of the Progonomys and Karnimata lineages, the stems of Murini and Arvicanthini, were less distinct, showed lower frequency of derived character states, and were much closer in size.
Figure 5. Early Karnimata and Progonomys from locality Y311, 10.1 Ma. (A) Karnimata fejfari left M1 YGSP 54147 and (B) Progonomys hussaini left M1 YGSP 541443. Progonomys is slender with more inclined cusps but is near the size of Karnimata. Both at same 1 mm scale, anterior is upward.
If the older P. hussaini and K. fejfari were more similar to each other than younger members of these genera (at least in features that could be perceived in the dentition), would still older samples (those available to us being 11.6–11.2 Ma) show more subtle differences, or appear to merge? Localities Y797 and Y791, dating to 11.2 Ma, have abundant murines, all about the same size and resembling Progonomys and Karnimata (plus a few teeth representing small “Progonomys” morganae and two unnamed mice; Flynn et al., 2020). Most individual specimens appear comparable to P. hussaini and K. fejfari, but the collections, taken as biological samples, do not sort readily into more than one species. These fossils were considered a single population also in model-based Bayesian cluster analysis (Kimura et al., 2016). Some M1’s have the murinin (Progonomys-like) morphology and some present the arvicanthin morphology (Figures 1, 3, 6). The array of morphologies observed among the M1 is not bimodal–there are intermediates. Further, other molars in the dentition (m1, second and third molars) comprise samples that show no consistent differentiation and no pattern for taxonomic association with M1. For the 11.2 Ma samples Flynn et al. (2020) could not defend two species and opted for an informal designation of “indeterminate Progonomys–Karnimata grade.” There is no record of P. hussaini and K. fejfari on the Potwar Plateau prior to 10.5 Ma. The roots of distinct Progonomys and of Karnimata lineages appear to lie in samples captured at 11.2 Ma.
Figure 6. Indeterminate Karnimata–Progonomys grade molars from locality Y797, 11.2 Ma. (A) Karnimata-like left M1 YGSP 36826 and (B) Progonomys-like left M1 YGSP 33878. Both at same 1 mm scale, anterior is upward.
The mice from Y791 and Y797, 11.2 Ma, were very close to the crown murine genera Progonomys and Karnimata but did not show one or the other derived morphologies consistently. Morphologies observed from somewhat older localities were yet more variable, but with inconsistently derived morphologies (Figure 7). Our field surveys in subjacent strata located fossil localities Y76 and Y809 at 11.4 Ma, and below these (therefore older) sites Y83 and Y504 at 11.6 Ma. The collections presented a medley of features, some specimens showing a displaced, pinched anterocone, others showing variably inclined cusps. Individual M1 were occasionally reminiscent of Progonomys in having the anterostyle displaced backward (Figure 7C), but most molars lacked derived conditions of extant mouse tribes. The M1 resembled their “post-Antemus” predecessors of 12.3 Ma, many with anterocones not elongated antero-posteriorly, and connections between adjacent cusps generally undeveloped. These “pre-Progonomys” mice were basal crown murines (stem species) containing the roots of later tribe-level radiations, but themselves undifferentiated along modern lines.
Figure 7. Four left M1 from locality Y76, 11.4 Ma, showing stem murinin-arvicanthin morphology. (A) YGSP 33586, (B) 33571, (C) 33591, and (D) 33572. Of these four, panel (C) is Progonomys-like; the others resemble Karnimata. Same 1 mm scale, anterior upward.
The documentation of multiple, sequentially progressive transitional samples, whether of mixed cryptic species or of consecutive individually variable species, provides a timescale for this cladistic event and a view of its mode. In either case, the 11 Ma divergence provided by “strap”-based stratigraphic age estimation (Figure 8) is congruent with the most recent molecular estimates of the Murini-Arvicanthini split provided by Aghová et al. (2018).
Figure 8. Time-tree for proposed phylogenetic relationships of major Siwalik murine rodents with well-defined time calibration points on the standard time scale, modeled with the “strap” package in R (Bell and Lloyd, 2015). Stem murines Potwarmus primitivus and Antemus chinjiensis are succeeded on the tree by basal crown murines, followed by a grade labeled “indeterminate Progonomys–Karnimata” (the cf. Progonomys hussaini and cf. Karnimata fejfari of Flynn et al., 2020). These are succeeded by the Progonomys and Karnimata clades, which are basal to subclades Murini-Praomyini-Apodemini and Arvicanthini-Otomyini-Millardiini, respectively. The bold lines connect range-through occurrences of the taxa in the Potwar record. The light blue node indicates a fossil calibration point evidenced by an initial split into two clades. The orange nodes indicate calibration points defined by the earliest occurrence of stem species of the clade. Horizontal arrows show the earliest occurrences of stem fossils. “Strap”-based ages of fossil calibration points are consistent with those previously proposed by Bayesian-based molecular divergence estimates in Aghová et al. (2018). Considering that the “equal” time-scaling algorithm in “strap” is tuned to avoid zero-length branches and that the molecular phylogeny is based on a framework of Bayesian statistics, the consistent age estimates between the two approaches indicate that Siwalik fossil sampling is dense enough to capture transitional morphological changes. Best practices for the use of fossils in molecular phylogenetics proposed by Parham et al. (2012) and followed by Aghová et al. (2018) yield credible divergence estimates for the Murinae tree. MRCA: most recent common ancestor.
It is well worth emphasizing the unique set of conditions that allowed this evaluation of tempo and mode in evolution using the fossil record. Siwalik Group rocks or their equivalents span a vast area along the Himalaya front from Pakistan to Myanmar. Correlative fossils from India largely complement and corroborate the Siwalik murine record in Pakistan (Patnaik, 2014, 2020). Older fossils are not relevant to this study because they are phylogenetically distant from the problem; younger fossil taxa are more derived members of Murini and Arvicanthini (e.g., Jacobs, 1978; Musser, 1987; Patnaik et al., 1993, 2018; Kotlia, 2008; Patnaik, 2020). Although fossil murines and other fossil rodents have been known in Europe (Schaub, 1938; Michaux, 1971) and globally for many years, no murine fossil known is older than the oldest murines of Pakistan. Flynn et al. (2020) have discussed this restricted biogeographic setting of the Siwaliks as the “murine cradle.”
Moreover, Siwalik murines and other small mammals were linked with the remainder of the fauna as part of a large, evolving ecosystem that responded to global conditions (Barry et al., 1985; Blois and Hadley, 2009; Figueirido et al., 2012) and to more regional abiotic and climatic changes (Flynn and Jacobs, 1982; Badgley et al., 2008; López-Antoñanzas et al., 2015). Stable isotopes have been used to track changes in Siwalik murine diets and tooth morphology has been correlated with dietary changes (Kimura et al., 2013a,b, 2016). These aspects of paleobiology, all of which have a temporal component, reiterate the importance of high-quality geologic dating and dense sampling of fossils in the Siwaliks. Finally, the group of animals being studied, with an enormous modern diversity and broad geographic range, renders the study of their deep roots both multidisciplinary and highly significant.
The middle and late Miocene age murine fossil record is rich throughout the Old World, but the oldest fossils of the subfamily are from the early Miocene of South Asia (Flynn et al., 2020). Significant events in early murine evolution appear to be centered in southern Asia, and the best fossil record that captures part of that radiation is being developed in the Siwaliks of the Indian Subcontinent (Jacobs and Downs, 1994; Jacobs and Flynn, 2005; Patnaik, 2014). This exceptional fossil record bears on the origin of two extant tribes, Murini and Arvicanthini. We contend that while the fossil record does not address population microevolution, it is relevant to fine scale evolutionary phenomena between the microevolutionary and macroevolutionary levels.
The Siwalik deposits of the Potwar Plateau contain a record of superposed fossil samples that is continuous and well dated on the scale of 100,000 years especially for the time span discussed here, 14–8 Ma. We have applied this precise biostratigraphy to a special episode in murine evolution. The relative ages of fossils are consistent with the pattern of evolution of crown clades (Lecompte et al., 2008; Aghová et al., 2018; Flynn et al., 2020).
Major morphological features are in this case an insufficient basis for tracing microevolution so we dissected molar crown morphology into distinct, definable traits and traced change through time (Figure 3). We selected four features (see section “Materials and Methods”) and quantified change from 14 to 6 Ma. These features show considerable variation until about 11 Ma, when they diverge, slowly at first, as Murini and Arvicanthini (and other minor lineages). We contend that this faithfully reflects growing divergence in morphology consistent with the scenario of a split of the two lineages from a common ancestor, with clear separation by 10.5 Ma.
Fossil samples at 9.2 Ma and younger securely represent early Murini and Arvicanthini in morphological features and dietary preferences (Kimura et al., 2015, 2016). Key species at that age are the distinct P. debruijni and K. darwini. Predecessor species, P. hussaini and K. fejfari, occur in rocks one million years older. These species were morphologically closer to each other than their successors were to their contemporaries, but still distinct, and are closer in time to the origins of the tribes. The oldest records of P. hussaini and K. fejfari are from locality Y259 at 10.5 Ma; they may well have been distinct before that. However, at 11.2 Ma we find that while the species were not yet present, samples are consistent with the hypothetical origin of Murini and Arvicanthini. Fossil samples at 11.2 Ma comprise morphological variants that resemble Progonomys and Karnimata but appear to represent a single lineage of rodents. The samples do not present a bimodal distribution of shape or size. Hypothetical cryptic species may be a possibility but cannot be distinguished morphologically.
These data speak to the mode of evolution in these murines. The split of the modern tribes was incremental. It began in a common pool of morphological variants. Extremes in variation, e.g., the murinin anterostyle, became hallmarks of mice exploiting one part of the range of variation, in contrast to the arvicanthin strategy of retained vertical cusps and broadened molars. Absolute change in M1 morphology is greater in arvicanthins, which later exploited preferentially a C4 diet. Our data suggest such a scenario but are not sufficient to claim that the tribes originated at 11.2 Ma exactly. Indeed, the lineages may have been close, but not yet morphologically distinct at that age in the features we can perceive. The fossils at 11.2 Ma may sample two cryptic species, Progonomys and Karnimata ancestors.
The Siwalik fossil record also addresses the tempo of the origin of Murini and Arvicanthini. Prior to 11.2 Ma, fossil samples show similar variation embracing early murinin and arvicanthin morphology. This suggests a very long interval of time during which basal crown murines experimented with diversification as Murini and Arvicanthini. Hundreds of thousands of years passed before the definitive tribe-equivalent morphologies emerged. Even if Progonomys and Karnimata had diverged by 11.2 Ma, the completion of their splitting process was protracted over a very long time, at least 400,000 years during this interval.
What may have gone on during these 100,000 years? While the clear separation of crown tribes may be arbitrary within tight limits, it is quite feasible that gene flow, even if limited, continued between their earliest species members for some time after their genetic divergence. The phenomenon of genetic introgression within a temporal murine metacommunity over a long interval before complete isolation of daughter species (Presgraves and Yi, 2009) has important implications for interpreting age of lineage splitting and therefore the molecular clock. The timing of cladogenesis as perceived by paleontologists from preserved morphology may post-date significantly a protracted interval of gene exchange at the base of the split. We have been fortunate to be able to view the results of one cladogenic event in detail, the split of Tribes Murini and Arvicanthini. That process may unfold quickly or take hundreds of thousands of years for significant morphological change to accumulate. Judging from our case, morphological change following sporadic genetic divergence may be a significantly protracted process.
The original contributions presented in the study are included in the article/supplementary material, further inquiries can be directed to the corresponding author/s.
All authors contributed equally to this work and each agrees to be accountable for the content of the work. YK developed much of the original comparative data and developed the temporo-stratigraphic analyses. LJ integrated the morphological and molecular patterns into a coherent whole, and organized specimen imaging. LF applied this special case for the fossil record to current molecular records and revised specimen identifications and locality dating.
JSPS KAKENHI Grant Number JP15H06884 (Grant-in-Aid for Young Scientists Start-up), JSPS KAKENHI Grant Number 18K13650, ISEM at SMU, and the American School of Prehistoric Research.
The authors declare that the research was conducted in the absence of any commercial or financial relationships that could be construed as a potential conflict of interest.
Many collaborators in the field worked to build the fossil collections on which his study is based. Scientists from the Geological Survey of Pakistan and the Pakistan Museum of Natural History, and from various institutions in the United States, especially our small mammal colleague, Will Downs, made this study possible. Roy Beavers of Southern Methodist University skillfully produced SEM images. David Pilbeam, John Barry, and Michèle Morgan supplied both feedback and data, reviewers stimulated improvements, and we thank the editors and the series editors for their patience and encouragement.
Aghová, T., Kimura, Y., Bryja, J., Dobigny, G., Granjon, L., and Kergoat, G. J. (2018). Fossils know it best: using a new set of fossil calibrations to improve the temporal phylogenetic framework of murid rodents (Rodentia: muridae). Mol. Phylogenet. Evol. 128, 98–111. doi: 10.1016/j.ympev.2018.07.017
Badgley, C., Barry, J. C., Morgan, M. E., Nelson, S. V., Behrensmeyer, A. K., Cerling, T. E., et al. (2008). Ecological changes in Miocene mammalian record show impact of prolonged climatic forcing. Proc. Natl. Acad. Sci. U.S.A. 105, 12145–12149. doi: 10.1073/pnas.0805592105
Barry, J. C., Behrensmeyer, A. K., Badgley, C. E., Flynn, L. J., Peltonen, H., Cheema, I. U., et al. (2013). “The neogene siwaliks of the potwar plateau, Pakistan,” in Fossil Mammals of Asia: Neogene Biostratigraphy and Chronology, eds X. Wang, L. J. Flynn, and M. Fortelius (New York, NY: Columbia University Press), 373–398. doi: 10.7312/columbia/9780231150125.003.0015
Barry, J. C., Johnson, N. M., Raza, S. M., and Jacobs, L. L. (1985). Neogene mammalian faunal change in Southern Asia: correlations with climatic, tectonic, and eustatic events. Geology 13, 637–640. doi: 10.1130/0091-7613(1985)13<637:nmfcis>2.0.co;2
Barry, J. C., Morgan, M. E., Flynn, L. J., Pilbeam, D., Behrensmeyer, A. K., Raza, S. M., et al. (2002). Faunal and environmental change in the late Miocene Siwaliks of northern Pakistan. Paleobiol. Memoir. 28, 1–71.
Bell, M. A., and Lloyd, G. T. (2015). STRAP: an R package for plotting phylogenies against stratigraphy and assessing their stratigraphic congruence. Palaeontology 58, 379–389. doi: 10.1111/pala.12142
Blois, J. L., and Hadley, E. A. (2009). Mammalian response to Cenozoic climatic change. Annu. Rev. Earth Planet. Sci. 37, 181–208. doi: 10.1146/annurev.earth.031208.100055
Bryja, J., Sumbera, R., Peterhans, J. C. K., Aghová, T., Bryjova, A., Mikula, O., et al. (2017). Evolutionary history of the, thicket rats (genus Grammomys) mirrors the evolution of African forests since late Miocene. J. Biogeogr. 44, 182–194. doi: 10.1111/jbi.12890
Canty, A., and Ripley, B. D. (2020). Boot: Bootstrap R (S-Plus) Functions. R Package Version 1.3-25.
Casola, C. (2018). From de novo to “de Nono”: the majority of novel protein coding genes identified with phylostratigraphy are old genes or recent duplicates. Genome Biol. Evol. 10, 2906–2918. doi: 10.1093/gbe/evy231
Cheema, I. U., Raza, S. M., Flynn, L. J., Rajpar, A. R., and Tomida, Y. (2000). Miocene small mammals from Jalalpur (District Jhelum) and their biochronologic implications. Natl. Sci. Mus. Bull. 26, 57–77. Tokyo,Google Scholar
da Costa, S. M., Rossi, M. I. D., Evangelista, A. A., and Oliveira, G. M. (2019). Origin, phylogeny and natural behavior of mice: what is their influence on welfare during their maintenance in the house facilities? Amer. J. Biomed. Sci. 5:AJBSR.MS.ID.000946. doi: 10.34297/AJBSR.2019.05.000946
Figueirido, B., Janis, C. M., Pérez-Claros, J. A., De Renzi, M., and Palmqvist, P. (2012). Cenozoic climate change influences mammalian evolutionary dynamics. Proc. Natl. Acad. Sci. U.S.A. 109, 722–727. doi: 10.1073/pnas.1110246108
Flynn, L. J., and Jacobs, L. L. (1982). Effects of changing environments on Siwalik rodent faunas of northern Pakistan. Palaeogeogr. Palaeoclimatol. Palaeoecol. 38, 129–139. doi: 10.1016/0031-0182(82)90067-0
Flynn, L. J., Kimura, Y., and Jacobs, L. L. (2020). “The murine cradle,” in Biological Consequences of Plate Tectonics: New Perspectives on Post-Gondwanaland Break-Up, eds G. V. R. Prasad and R. Patnaik (Cham: Springer), 347–362.
Garcés, M., Krijgsmann, W., Paláez-Campomanes, P., Álvarez-Sierra, M. A., and Daams, R. (2003). Hipparion dispersal in Europe: magnetostratigraphic constraints from the Daroca area (Spain). Coloq. Paleontol. 1, 171–178.
García-Paredes, I., Álvarez-Sierra, M. A., van den Hoek Ostende, L. W., Hernández-Ballarin, V., Hordijk, K., López-Guerro, P., et al. (2016). The Aragonian and Vallesian high-resolution micromammal succession from the Calatayud-Montalbán Basin (Aragón, Spain). C. R. Palevol 15, 781–789. doi: 10.1016/j.crpv.2015.09.014
Gould, S. J. (1985). The paradox of the first tier: an agenda for paleobiology. Paleoiology 11, 2–12. doi: 10.1017/s0094837300011350
Jacobs, L. L. (1977). A new genus of murid rodent from the Miocene of Pakistan and comments on the origin of the Muridae. Paleobios 25, 1–11.
Jacobs, L. L. (1978). Fossil rodents (Rhizomyidae and Muridae) from Neogene Siwalik deposits, Pakistan. Mus. North. Arizona Press Bull. 52, 1–103.
Jacobs, L. L., and Downs, W. R. (1994). “The evolution of murine rodents in Asia,” in Rodent and Lagomorph Families of Asian Origins and Diversification, Vol. 8, eds Y. Tomida, C.-k. Li, and T. Setoguchi (Tokyo: National Science Museum Monograph), 149–156.
Jacobs, L. L., and Flynn, L. J. (2005). “Of mice again: the Siwalik rodent record, murine distribution, and molecular clocks,” in Interpreting the Past: Essays on Human, Primate, and Mammal Evolution in Honor of David Pilbeam: American School of Prehistoric Research Monograph Series 5, eds D. E. Lieberman, R. J. Smith, and J. Kelley (Boston, MA: Brill Academic Publishers, Inc.), 63–80.
Jacobs, L. L., Flynn, L. J., and Downs, W. R. (1989). “Neogene rodents of southern Asia,” in Papers on Fossil Rodents in Honor of Albert Elmer Wood, Vol. 33, eds C. C. Black and M. R. Dawson (Los Angeles, CA: Natural History Museum of Los Angeles County), 157–177.
Jacobs, L. L., and Pilbeam, D. (1980). Of mice and men: fossil-based divergence dates and molecular “clocks”. J. Hum. Evol. 9, 551–555. doi: 10.1016/0047-2484(80)90062-7
Johnson, N. M., Stix, J., Tauxe, L., Cerveney, P. F., and Tahirkheli, R. A. K. (1985). Paleomagnetic chronology, fluvial processes, and tectonic implications of the Siwalik deposits near Chinji Village, Pakistan. J. Geol. 93, 27–40. doi: 10.1086/628917
Juggins, S. (2020). rioja: Analysis of Quaternary Science Data. R Package Version 0.9-26. Available online at: https://cran.r-project.org/package=rioja (accessed December 15, 2020).
Keller, H. M., Tahirkheli, R. A. K., Mirza, M. A., Johnson, G. D., Johnson, N. M., and Opdyke, N. D. (1977). Magnetic polarity stratigraphy of the Upper Siwalik Deposits, Pabbi Hills, Pakistan. Earth Planet. Sci. Lett. 36, 187–201. doi: 10.1016/0012-821x(77)90198-4
Kimura, Y., Flynn, L. J., and Jacobs, L. L. (2016). A palaeontological case study for species delimitation in diverging fossil lineages. Hist. Biol. 28, 189–198. doi: 10.1080/08912963.2015.1022175
Kimura, Y., Flynn, L. J., and Jacobs, L. L. (2017). Early late Miocene murine rodents from the upper part of the Nagri Formation, Siwalik Group, Pakistan, with a new fossil calibration point for the Tribe Apodemurini (Apodemus/Tokudaia). Fossil Imprint 73, 197–212. doi: 10.2478/if-2017-0011
Kimura, Y., Hawkins, M. T. R., McDonough, M. M., Jacobs, L. L., and Flynn, L. J. (2015). Corrected placement of Mus-Rattus fossil calibration forces precision in the molecular tree of rodents. Sci. Rep. 5:14444. doi: 10.1038/srep14444
Kimura, Y., Jacobs, L. L., Cerling, T. E., Uno, K. T., Ferguson, K. M., Flynn, L. J., et al. (2013a). Fossil mice and rats show isotopic evidence of niche partitioning and change in dental ecomorphology related to dietary shift in late Miocene of Pakistan. PLoS One 8:e69308. doi: 10.1371/journal.pone.0069308
Kimura, Y., Jacobs, L. L., and Flynn, L. J. (2013b). Lineage-specific responses of tooth shape in murine rodents (Murinae, Rodentia) to late Miocene dietary change in the Siwaliks of Pakistan. PLoS One 8:e76070. doi: 10.1371/journal.pone.0076070
Kotlia, B. S. (2008). A new species of fossil Mus (Rodentia, Muridae) from the Indian Himalaya: evolutionary and phylogenetic implications. Palaeoworld 17, 47–56. doi: 10.1016/j.palwor.2007.08.004
Lecompte, E., Aplin, K., Denys, C., Catzeflis, F., Chades, M., and Chevret, P. (2008). Phylogeny and biogeography of African Muridae based on mitochondrial and nuclear gene sequences, with a new tribal classification of the subfamily. BMC Evol. Biol. 8:199. doi: 10.1186/1471-2148-8-199
López-Antoñanzas, R., Knoll, F., Wan, S., and Flynn, L. J. (2015). Causal evidence between monsoon and evolution of rhizomyine rodents. Sci. Rep. 5:9008. doi: 10.1038/srep09008
Ma, L., Cisse, O. H., and Kovacs, J. A. (2018). A molecular window into the biology and epidemiology of Pneumocystis spp. Clin. Microbiol. Rev. 31:e00009-18. doi: 10.1128/CMR.00009-18
Maddison, W. P., and Maddison, D. R. (2017). Mesquite: A Modular System for Evolutionary Analysis. Version 3.04. Available online at: http://mesquiteproject.org (accessed December 12, 2020).
Michaux, J. (1971). Muridae (Rodentia) neogenes d’Europe Sud-Occidentale: evolution et rapports avec les formes actuelles. Paleobiol. Continentale 2, 1–67.
Misonne, X. (1969). African and Indo-Australian Muridae Evolutionary Trends: Annales. Sciences Zoologique 8. Tervuren: Musée Royal de l’Afrique Centrale, 1–219.
Musser, G. G. (1987). The occurrence of Hadromys (Rodentia: muridae) in early Pleistocene Siwalik strata in northern Pakistan and its bearing on biogeographic affinities between Indian and northeastern African murine faunas. Am. Mus. Novitates 2883, 1–36.
Pages, M., Chaval, Y., Herbreteau, V., Waengsothorn, S., Cosson, J.-F., Hugot, J.-P., et al. (2010). Revisiting the taxonomy of the Rattini tribe: a phylogeny-based delimitation of species boundaries. BMC Evol. Biol. 10:184. doi: 10.1186/1471-2148-10-184
Parham, J. F., Donoghue, P. C., Bell, C. J., Calway, T. D., Head, J. J., Holroyd, P. A., et al. (2012). Best practices for justifying fossil calibrations. Syst. Biol. 61, 346–359.
Patnaik, R. (2014). Phylogeny of Siwalik murine rodents: implications for Mus-Rattus divergence time. J. Palaeontol. Soc. India 59, 15–28.
Patnaik, R. (2020). “New data on the Siwalik murines, rhizomyines and ctenodactylines (Rodentia) from the Indian subcontinent,” in Biological Consequences of Plate Tectonics: New Perspectives on Post-Gondwanaland Break-Up, eds G. V. R. Prasad and R. Patnaik (Cham: Springer), 363–391. doi: 10.1007/978-3-030-49753-8_16
Patnaik, R., Bahadur, M., Sharma, T., and Sahni, A. (1993). A comparative analysis of the molars of Mus booduga, Mus dunni and fossil Mus of the Indian subcontinent: phylogenetic and palaeobiogeographic implications. Curr. Sci. 65, 782–786.
Patnaik, R., Kotla, S. S., Singh, N. P., Singla, A., and Kaur, J. (2018). A new murid rodent assemblage from the Upper Siwaliks, Himachal Pradesh, India: biostratigraphic, phylogenetic and paleobiogeographic implications. J. Asian Earth Sci. 162, 93–106. doi: 10.1016/j.jseaes.2017.05.030
Presgraves, D. C., and Yi, S. V. (2009). Doubts about complex speciation between humans and chimpanzees. Trends Ecol. Evol. 24, 533–540. doi: 10.1016/j.tree.2009.04.007
R Core Team (2020). R: A Language and Environment for Statistical Computing. Vienna: R. Foundation for Statistical Computing.
Reznick, D. N., and Ricklefs, R. E. (2009). Darwin’s bridge between microevolution and macroevolution. Nature 457, 837–842. doi: 10.1038/nature07894
Rowe, K. C., Achmadi, A. S., and Esselstyn, J. A. (2016). Repeated evolution of carnivory among Indo-Australian rodents. Evolution 70, 653–665. doi: 10.1111/evo.12871
Rowe, K. C., Achmadi, A. S., Fabre, P.-H., Schenk, J. J., Steppen, S. J., and Esselstyn, J. A. (2019). Oceanic islands of Wallacea as a source for dispersal and diversification of murine rodents. J. Biogeogr. 46, 2752–2768. doi: 10.1111/jbi.13720
Rowe, K. C., Aplin, K. P., Baverstock, P. R., and Moritz, C. (2011). Recent and rapid speciation with limited morphological disparity in the genus Rattus. Syst. Biol. 60, 188–203. doi: 10.1093/sysbio/syq092
Steppan, S. J., and Schenk, J. J. (2017). Muroid rodent phylogenetics: 900-species tree reveals increasing diversification rates. PLoS One 12:e0183070. doi: 10.1371/journal.pone.0183070
Suzuki, H. (2020). “Evolutionary history of the subgenus Mus in Eurasia with special emphasis on the house mouse Mus musculus,” in Papers in Honour of Ken Aplin. Records Australian Mus, Vol. 72, eds J. Louys, S. O’Connor, and K. M. Helgen (Darlinghurst NSW: Australian Museum) 317–323. doi: 10.3853/j.2201-4349.72.2020.1727.
Tauxe, L., and Opdyke, N. D. (1982). A time framework based on magnetostratigraphy for the Siwalik sediments of the Khaur area, northern Pakistan. Palaeogeogr. Palaeoclimatol. Palaeoecol. 37, 43–61. doi: 10.1016/0031-0182(82)90057-8
Tedford, R. H., Qiu, Z.-x, and Flynn, L. J. (2013). “Late Cenozoic Yushe Basin, Shanxi Province, China: geology and fossil mammals,” in History, Geology, and Magnetostratigraphy, Vol. I, (Dordrecht: Springer).
Keywords: speciation, evolution, Murinae, Miocene, Siwaliks, morphology
Citation: Kimura Y, Flynn LJ and Jacobs LL (2021) Tempo and Mode: Evidence on a Protracted Split From a Dense Fossil Record. Front. Ecol. Evol. 9:642814. doi: 10.3389/fevo.2021.642814
Received: 16 December 2020; Accepted: 19 February 2021;
Published: 15 March 2021.
Edited by:
Irina Ruf, Senckenberg Research Institute and Natural History Museum Frankfurt, GermanyReviewed by:
Paloma López-Guerrero, Universidad Complutense de Madrid, SpainCopyright © 2021 Kimura, Flynn and Jacobs. This is an open-access article distributed under the terms of the Creative Commons Attribution License (CC BY). The use, distribution or reproduction in other forums is permitted, provided the original author(s) and the copyright owner(s) are credited and that the original publication in this journal is cited, in accordance with accepted academic practice. No use, distribution or reproduction is permitted which does not comply with these terms.
*Correspondence: Lawrence J. Flynn, bGpmbHlubkBmYXMuaGFydmFyZC5lZHU=
†These authors have contributed equally to this work and share first authorship
Disclaimer: All claims expressed in this article are solely those of the authors and do not necessarily represent those of their affiliated organizations, or those of the publisher, the editors and the reviewers. Any product that may be evaluated in this article or claim that may be made by its manufacturer is not guaranteed or endorsed by the publisher.
Research integrity at Frontiers
Learn more about the work of our research integrity team to safeguard the quality of each article we publish.