- 1International Centre of Insect Physiology and Ecology (icipe), Nairobi, Kenya
- 2Department of Zoology and Entomology, University of Pretoria, Pretoria, South Africa
- 3UPR HORTSYS, Univ Montpellier, CIRAD, Montpellier, France
- 4Biopass2, Cirad-IRD-ISRA-UBG-Centre de coopération internationale en recherche agronomique pour le développement-Institut de Recherche pour le Développement-Institut Sénégalais de Recherches Agricoles-Université Gaston Berger, Dakar, Senegal
Kairomones are chemical signals that mediate interspecific interactions beneficial to organisms that detect the cues. These attractants can be individual compounds or mixtures of herbivore-induced plant volatiles (HIPVs) or herbivore chemicals such as pheromones, i.e., chemicals mediating intraspecific communication between herbivores. Natural enemies eavesdrop on kairomones during their foraging behaviour, i.e., location of oviposition sites and feeding resources in nature. Kairomone mixtures are likely to elicit stronger olfactory responses in natural enemies than single kairomones. Kairomone-based lures are used to enhance biological control strategies via the attraction and retention of natural enemies to reduce insect pest populations and crop damage in an environmentally friendly way. In this review, we focus on ways to improve the efficiency of kairomone use in crop fields. First, we highlight kairomone sources in tri-trophic systems and discuss how these attractants are used by natural enemies searching for hosts or prey. Then we summarise examples of field application of kairomones (pheromones vs. HIPVs) in recruiting natural enemies. We highlight the need for future field studies to focus on the application of kairomone blends rather than single kairomones which currently dominate the literature on field attractants for natural enemies. We further discuss ways for improving kairomone use through attract and reward technique, olfactory associative learning, and optimisation of kairomone lure formulations. Finally, we discuss why the effectiveness of kairomone use for enhancing biological control strategies should move from demonstration of increase in the number of attracted natural enemies, to reducing pest populations and crop damage below economic threshold levels and increasing crop yield.
Introduction
The foraging behaviour of a parasitoid or predator is a process by which it searches for oviposition sites and feeding resources for its survival, growth, and reproductive success [reviewed in Kramer (2001)]. Natural enemies (used hereafter in reference to both parasitoids and predators) play a key role in the biological control of chewing, sucking, and gall-feeding herbivores (Vidal and Murphy, 2018). Understanding the foraging behaviour of natural enemies is crucial for improving the biological control of herbivorous pests (e.g., Mills and Wajnberg, 2008; Gunton and Pöyry, 2016; Mills and Heimpel, 2018). Parasitoids have long served as an insect model in the study of foraging behaviour for oviposition sites, which has often been viewed as a three-sequence process: habitat location, host location, and host selection (Vinson, 1998; Fatouros et al., 2008). However, this behavioural process can also be applied to predatory insects searching for prey (Fellowes et al., 2007; Pervez and Yadav, 2018). During each of these foraging behavioural sequences, natural enemies rely on a combination of environmental stimuli to find their host and prey (hereafter jointly referred to as herbivores). Although kairomones and visual signals are exploited by natural enemies, the former is well-known to play an important role in their foraging behaviour (Colazza and Wanjberg, 2013; Lim and Ben-Yakir, 2020). Kairomones are individual semiochemical molecules or mixtures that mediate interspecific interactions between living organisms (Dicke and Sabelis, 1988; Kost, 2008), while serving as long-range, short-range, and contact cues during herbivore location by natural enemies (Afsheen et al., 2008; Heil and Ton, 2008). Kairomones exploited by natural enemies are either plant volatiles or herbivore-associated chemicals such as pheromones (Afsheen et al., 2008; Kaplan, 2012a; Kaiser et al., 2017; Peñaflor, 2019).
Herbivory-induced plant volatiles (HIPVs) and oviposition-induced plant volatiles (OIPVs) are the reliable plant volatile compounds that serve as long-range kairomones enabling natural enemies to locate herbivore-infested plants (e.g., Dicke and van Loon, 2000; Mumm and Dicke, 2010; Hilker and Fatouros, 2015; Turlings and Erb, 2018). The use of HIPVs and OIPVs to attract natural enemies has been pointed out as a novel avenue to achieve successful biological control (Kaplan, 2012a; Kelly et al., 2014; Murali-Baskaran et al., 2018; Peri et al., 2018). For example, (E)-4,8-dimethyl-1,3,7-non-atriene (DMNT), an elm plant (Ulmus minor Mill [Ulmaceae]) OIPV induced by Xanthogaleruca luteola (Müller) (Coleoptera: Chrysomelidae), attracted the parasitoid Oomyzus gallerucae (Fonscolombe) (Hymenoptera: Eulophidae) in the field (Büchel et al., 2011). In addition to HIPVs and OIPVs, kairomones include chemicals emitted from different herbivore stages (eggs, larvae/nymphs, pupae, adults), herbivore by-products (e.g., frass, honeydew, exuviae, mandibular gland secretions, defence secretions, etc.) which are exploited by natural enemies in their selection of oviposition and feeding sites [reviewed in Afsheen et al. (2008)]. For instance, application of hydrocarbons, e.g., tricosane identified in extracts of Heliothis zea (Boddie) (Lepidoptera: Noctuidae) moth scales, increased the efficiency of host location by the parasitoids Trichogramma achaeae Nagaraja and Nagarkatti (Hymenoptera: Trichogrammatidae) and Microplitis croceipes (Cresson) (Hymenoptera: Braconidae), thereby increasing the parasitisation rate in the field (Gross et al., 1975; Lewis et al., 1975).
Pheromones are highly species-specific in mediating intraspecific interactions between the emitter and its conspecifics (Dicke and Sabelis, 1988; Kost, 2008). They can be grouped into the following categories: (i) sex pheromones, (ii) aggregation pheromones, (iii) marking pheromones, and (iv) alarm pheromones (Kost, 2008; Ruther, 2013). Eavesdropping is a well-known phenomenon among natural enemies which use pheromones of herbivorous insects as kairomones to detect them (Aukema and Raffa, 2005). A typical example is reported by Kpongbe et al. (2019) who demonstrated that isopentyl-butanoate—the aggregation pheromone of Clavigralla tomentosicollis Stål (Hemiptera: Coreidae)—strongly attracts Gryon sp. (Hymenoptera: Scelionidae), the parasitoid of Clavigralla species. However, not only herbivore pheromones play a role in the attraction of herbivore enemies, i.e., pheromones released by natural enemies also attract conspecific individuals seeking for mates (Ruther, 2013), and may modulate their responses to HIPVs (Cabello et al., 2017). For example, pheromones from males of the predator Nabis pseudoferus Remane (Hemiptera: Nabidae) attracted conspecific females and enhanced their responses to HIPVs from Rhopalosiphum padi (L.) (Hemiptera: Aphididae)-infested wheat plants, whereas pheromones from males of the mirid predator Nesidiocoris tenuis (Reuter) (Hemiptera: Miridae) attracted conspecific females but were found to reduce their attraction to the HIPVs (Cabello et al., 2017).
Kairomone-based lures are deployed in crop plants of which the background odours shape the location of herbivores by natural enemies (e.g., Schröder and Hilker, 2008; Randlkofer et al., 2010). In this review, we focus on how to optimise field applications of kairomones for improving biological control of insect pests. First, we present sources of kairomones in tri-trophic systems and discuss how these kairomones are exploited by natural enemies foraging for hosts or prey. Then we provide examples of kairomone field applications, and we stress how the attraction of natural enemies into crop fields might be more efficient when using pheromones rather than HIPVs. Finally, we highlight potential ways to improve the use of kairomone-based lures for attracting enough natural enemies to reduce pest populations and crop damage below economic threshold levels while increasing crop yields in the field.
Chemical Interactions in Tri-Trophic Systems
Chemically mediated multitrophic interactions occur both below and above ground between a diversity of organisms among four trophic levels in agro-ecosystems (Aartsma et al., 2019; Stelinski et al., 2019). Research on chemical interactions aiming at controlling insect pests are, however, usually focused on tri-trophic systems typically composed of plants, herbivores and natural enemies (Price et al., 1980; Kaplan, 2012b; Mbaluto et al., 2020), and in this section we summarise these tri-trophic chemically mediated interactions at above ground level (Figure 1). Organisms at each trophic level emit chemical cues (emitters) to mediate intraspecific and/or interspecific interactions with other organisms that detect the cues (receivers). These interactions can be direct or indirect (Wootton, 1994). A direct interaction occurs between two organisms without an intermediary, whereas an indirect interaction necessitates the presence of, or occurs through, at least one intermediary which is usually a third species (Figure 1) (Wootton, 1994). Plants (emitters) emit volatile organic compounds (VOCs) which are exploited by herbivores (receivers) to locate host plants (direct interactions) (Bruce and Pickett, 2011). Plants (emitters) also release HIPVs and OIPVs to attract natural enemies (receivers) for controlling herbivores (intermediary) (indirect interactions; Figure 1) (Hilker and Fatouros, 2015; Turlings and Erb, 2018). In addition to HIPVs and OIPVs that mediate the indirect plant defences, the role of zoophytophagous-induced plant volatiles (ZIPVs) in plant-insect chemical communication has recently gained attention (Figure 1) (Pappas et al., 2015; Pérez-Hedo et al., 2015). Zoophytophagous insects are sap sucking predators which can feed on plants and consequently induce the release of volatiles that attract conspecific and heterospecific predators, as well as parasitoids (Pérez-Hedo et al., 2015; Rim et al., 2018). This effect is like that induced by herbivores feeding on plants. Compared to volatiles of unexposed tomato plant (Solanum lycopersicum L. [Solanaceae]), ZIPVs from tomato plant volatiles induced by the mirid predator Nesidiocoris tenus (Reuter) (Hemiptera: Miridae) were found to attract conspecific males and females (Rim et al., 2018) and the parasitoid Encarsia formosa Gahan (Hymenoptera: Aphelinidae) (Pérez-Hedo et al., 2015). Undamaged plants can also perceive HIPVs, OIPVs, ZIPVs, and herbivore pheromones as signals to mount defences against herbivores to the benefit of the host plant community (known as plant priming defences) (Figure 1) (Dicke and Bruin, 2001; Frost et al., 2008, Pérez-Hedo et al., 2015), but the priming defence is not observed in all plant species as reported for cotton plants exposed to pheromones (Magalhães et al., 2019) and tea plants exposed to the HIPV (E)-4,8-dimethyl-1,3,7-nonatriene (Jing et al., 2020).
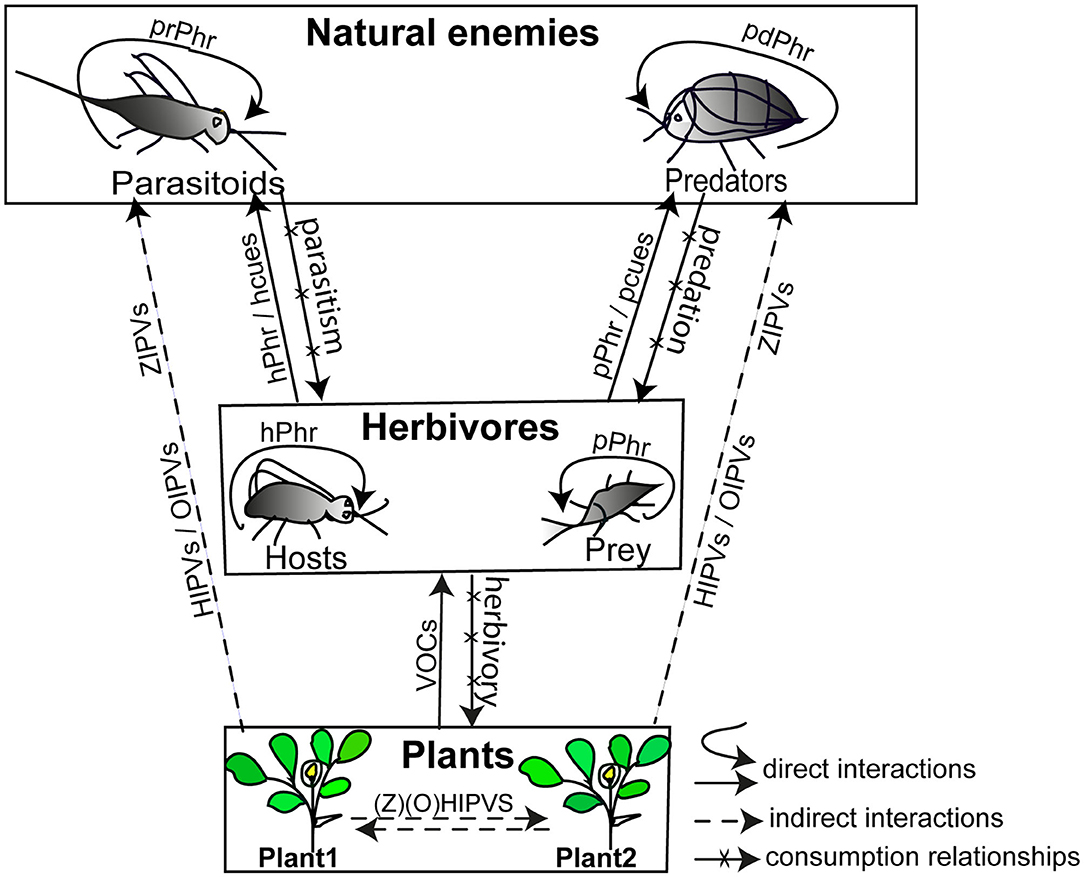
Figure 1. Typical examples of above ground chemical interactions in a tri-trophic system. Solid and dotted lines represent kairomone-based direct and indirect interactions, respectively, in which the arrow bearing the kairomone is directed from the emitter to the receiver. The solid curves indicate pheromone-based direct interactions in which male and female individuals can be an emitter and/or a receiver. HIPVs, herbivory-induced plant volatiles; OIPVs, oviposition-induced plant volatiles; ZIPVs, zoophytophagous-induced plant volatiles; VOCs, volatile organic compounds; pPhr, prey pheromone; hPhr, host pheromone; pdPhr, predator pheromone; prPhr, parasitoid pheromone; pcues, prey-associated chemical cues; hcues, host-associated chemical cues.
Natural enemies release pheromones that directly mediate interactions with their respective conspecifics (Ruther, 2013). While herbivores release pheromones to communicate with conspecific individuals to their benefit, their natural enemies eavesdrop on these communication cues to locate them to their disadvantage (Aukema and Raffa, 2005). Herbivores and their by-products such as larval frass, larval mandibular secretions, and honeydew also release chemical compounds that serve as kairomones for natural enemies in locating herbivores (Afsheen et al., 2008). Understanding chemical interactions between plants, herbivores, and natural enemies (Figure 1) is key to designing and implementing kairomone-based biological control measures in agro-ecosystems. In the following sections, we focus on above-ground chemical interactions in which natural enemies are receivers of pheromones and kairomones involved (Figure 1).
Host Plant and Herbivore Location by Natural Enemies Within the Odour Landscape
Natural enemies foraging for herbivores locate infested host plants within the heterogeneous plant community using a sequence of behaviours (Figure 2). This is a tedious task since host plants are embedded in a semiochemically heterogeneous environment composed of diverse plant species and multiherbivore communities whose signals influence the detection of reliable kairomones during host or prey location (e.g., Schröder and Hilker, 2008; Randlkofer et al., 2010). This complex dynamic sensory environment has been described as an “odour landscape” which is composed of different odour plumes (an odour plume is a blend of volatile compounds carried by the wind) (Atema, 1996). How insects track relevant or resource-indicating odour plumes within the odour landscape has recently been reviewed (Beyaert and Hilker, 2014). Foraging insects can follow a single or several relevant odour plumes during which they shift from one odour plume to another if this conveys a more reliable signal (or indicates a more suitable resource), and they continue this behaviour until they find the target host plant (Beyaert and Hilker, 2014). During foraging, natural enemies rely on a diversity of resource-indicating odours to first locate the host plant within the plant community (section Role of Herbivore-Induced Plant Volatiles in long-Range Location of Host Plants), then find the potential infesting herbivores (section Herbivore Volatiles for Host or Prey Location), and finally select and accept the target host or prey upon landing on the host plant (section Herbivore Contact Kairomones for Host/Prey Recognition and Acceptance) (Figure 2).
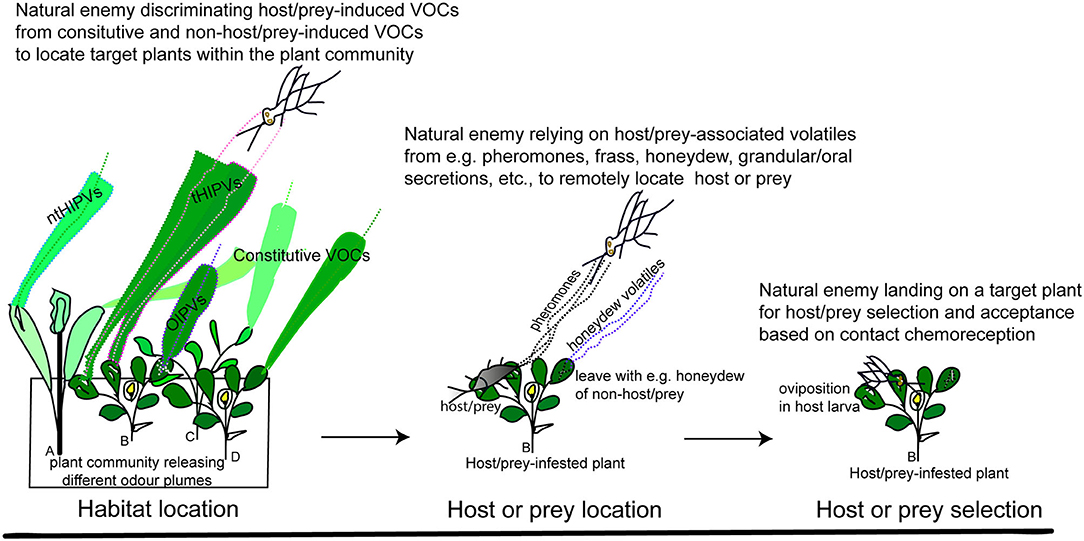
Figure 2. Schematic description of the use of kairomones during foraging behavioural sequences involved in host/prey searching by parasitoids and predators. VOCs, volatile organic compounds; tHIPVs, target herbivore-induced plant volatiles; ntHIPVs, non-target herbivore-induced plant volatiles; OIPVs = oviposition-induced plant volatiles. Plant community composed of (A) infested non-host plants; (B) infested host plants; (C) uninfested non-host plants; and (D) uninfested host plants.
Role of Herbivore-Induced Plant Volatiles in Long-Range Location of Host Plants
Plants emit VOCs while undamaged, known as constitutive VOCs, and herbivory or oviposition usually results in an increase in the constitutive VOCs and/or release of specific herbivore-induced volatiles, known as inducible VOCs (e.g., HIPVs and OIPVs). Although natural enemies may sometimes be attracted by odour plumes of constitutive VOCs, evidences have culminated in the literature that the odour plumes of HIPVs and OIPVs are those that play the key role in the foraging behaviour, serving as long-range signals that natural enemies exploit to locate plants infested by their hosts or prey within the plant community (Figure 2) (e.g., Kessler and Baldwin, 2001; Heil and Ton, 2008; Kaplan, 2012a; Beyaert and Hilker, 2014). Plants are usually infested by several herbivore species that are hosts or non-hosts for natural enemies (Vos et al., 2001). Infesting herbivore species may induce the same or different plant defence pathways (i.e., Salicylic Acid inducer [SA-inducer] and Jasmonic Acid-inducer [JA-inducer]), each affecting the composition of volatiles produced (Danner et al., 2018). The attraction of natural enemies to infested plants depends on factors such as the infesting herbivores, i.e., single or multiple species; the suitability of the herbivores, i.e., hosts or non-hosts; the infesting herbivore density; and the activated plant defence pathways, i.e., SA and/or JA. For example, the parasitoid Cotesia marginiventris (Cresson) (Hymenoptera: Braconidae) was reported to be more attracted to volatiles of maize plants infested by only Spodoptera littoralis (Boisduval) (Lepidoptera: Noctuidae), a JA-inducer, than plants infested by both S. littoralis and Euscelidius variegatus (Kirschbaum) (Hemiptera: Cicadellidae), an SA-inducer (Erb et al., 2010). In multi-herbivore systems, the location of target herbivore-infested plants by natural enemies may be disrupted by the co-infestation of non-target herbivores (Takabayashi et al., 2006). The parasitoid Cotesia plutellae (Kurdjumov) (Hymenoptera: Braconidae) were less attracted to volatile blends from cabbage plants simultaneously infested by caterpillars of both host (Plutella xylostella [L.] [Lepidoptera: Plutellidae]) and non-host (Pieris rapae [L.] [Lepidoptera: Pieridae]) species compared to volatiles released from host P. xylostella-infested plants (Shiojiri et al., 2001). However, this is not always the case, as illustrated by the fact that the larval parasitoid Cotesia glomerata (L.) (Hymenoptera: Braconidae) was more attracted to plants damaged by larvae of both host P. rapae and non-host P. xylostella compared to plants damaged by host P. rapae alone (Shiojiri et al., 2001). The herbivore-infestation level also affects the response of natural enemies to infested plants. The parasitoids C. glomerata and Cotesia vestalis (Haliday) (Hymenoptera: Braconidae) were more attracted to volatiles of cabbage plants with high levels of P. rapae and P. xylostella larval infestation than those with low infestation levels (Shiojiri et al., 2010). Whereas, relative to volatiles of healthy plants, the predator Harmonia axyridis (Pallas) (Coleoptera: Coccinellidae) was reported to prefer volatiles of cabbage plants infested by an intermediate density of Myzus persicae (Sulzer) (Hemiptera: Aphididae) adults, but it showed no preference for plants infested with low and high densities of M. persicae (Yoon et al., 2010). Therefore, natural enemy species show different attraction responses to volatiles of infested plants depending on the specificity and density of infesting herbivores (De-Moraes et al., 1998; Shiojiri et al., 2010; McCormick et al., 2012).
The infesting herbivore species and its feeding mode (e.g., leaf chewing, leaf mining, phloem sap sucking, and cell content feeding) determine the plant defence signalling pathways to be activated (either SA or JA alone, or both). Emission of volatiles is regulated by the biosynthesis pathway(s) activated, and qualitative and quantitative differences are substantial between plant volatiles from SA- vs. JA-herbivore inducers (Danner et al., 2018). Chewing herbivores are known to activate the JA-pathway (Danner et al., 2018), while leaf mining larvae likely activate both JA- and SA-pathways (Yang et al., in press). For sap sucking herbivores, however, some species like whiteflies are known to induce a single pathway, i.e., SA (Zhang et al., 2013), whereas others like stink bugs induce both JA- and SA-pathways (Giacometti et al., 2016). A comparison of volatiles from tomato plants infested by the leaf miner Tuta absoluta (Meyrick) (Lepidoptera: Gelechiidae) larva, and the phloem sap sucking feeder Bemisia tabaci (Gennadius) (Hemiptera: Aleyrodidae) adult, revealed that α- and β-copaene, as well as valencene, were released in higher amounts from plants infested by the sap sucking insect (Silva et al., 2017). Whereas (Z)-3-hexen-1-ol, (E)-β-ocimene, methyl salicylate, and β-caryophyllene were higher from plants infested by the leaf miner, in addition to some novel compounds such as (Z)-jasmone and (Z)-3-hexen-1-yl crotonate (Silva et al., 2017). Volatile compounds released by single and dual herbivore-infested plants also differ quantitatively and qualitatively. Infestation of tomato plants by both T. absoluta and B. tabaci led to increased emission of β-myrcene, limonene, γ-terpinene, and β-elemene than in plants infested only by T. absoluta or B. tabaci, whereas α-pinene emission increased in T. absoluta-infested plants (Silva et al., 2018). Conversely, cotton plants simultaneously infested by the leaf chewing larvae of Spodoptera exigua (Hübner) (Lepidoptera: Noctuidae) and adults of B. tabaci emit lower amounts of HIPVs than plants infested only by S. exigua larvae, as a result of so-called “cross-talk” between SA- and JA-signalling pathways (Rodriguez-Saona et al., 2003). Natural enemies rely on these differences to find specific herbivore-infested plants (Mumm and Dicke, 2010; Shiojiri et al., 2010). Herbivores can also suppress the plant defence mechanisms and volatile emission when feeding, which could be a strategy to deceive natural enemies from locating herbivore-infested plants. The feeding and extracts of oral secretion of the leaf chewing larvae of Spodoptera frugiperda (Smith) (Lepidoptera: Noctuidae) were found to suppress the emission of HIPVs in maize plants (De-Lange et al., 2020). Similarly, suppression of HIPV emission in host plants was reported upon herbivory by cell content feeders (e.g., spider mites and thrips) (Schausberger, 2018) and phloem sap sucking feeders (e.g., whiteflies and aphids) (Walling, 2008; Zhang et al., 2013). Suppression of HIPV emission by herbivores is known to occur through various mechanisms, but the front line mechanism is reported to operate through inoculation of the oral secretion-inhabiting bacteria during feeding, a phenomenon that deceives the plant to incorrectly perceive and identify the attacking herbivore, and to not activate the appropriate signalling defence (Felton et al., 2014; Schausberger, 2018). Unfortunately, volatile suppression, in general, is not found to prevent natural enemies from locating herbivore-infested plants (Sarmento et al., 2011; De-Lange et al., 2020), suggesting that the release rates of the specific attractive compounds or the ratios of the attractive compounds in the released HIPV blend are not necessarily affected.
Volatile compounds attractive to natural enemies include a subset of HIPV or OIPV compounds, from the same or different chemical classes (D'Alessandro and Turlings, 2006; McCormick et al., 2012). These are often mixtures of compounds in specific ratios (van Wijk et al., 2011), but sometimes these attractants are single compounds (Wei et al., 2008). For example, (R)-(+)-limonene released by the Mongolian oak, Quercus mongolicus Fisch. ex. Turcz. (Fagaceae) plants infested by the longhorned beetle, Massicus raddei (Blessig) (Coleoptera: Cerambycidae) elicited strong attraction in the predator Dastarcus helophoroides (Fairmaire) (Coleoptera: Bothrideridae) (Wei et al., 2008). The predatory mite Phytoseiulus persimilis Athias-Henriot (Acari: Phytoseiidae) was more attracted to the mixture of five compounds β-ocimene, (Z)-3-hexenyl acetate, (E)-4,8-dimethyl-1,3,7-non-atriene (DMNT), (E,E)-4,8,12-trimethyl-1,3,7,11-tridecatetraene (TMTT) and methyl salicylate than to the individual compounds or partial mixtures of these compounds identified from Tetranychus urticae Koch (Acari: Tetranychidae)-infested lima bean plants (van Wijk et al., 2011). The identification of attractant compounds from HIPVs and OIPVs is mostly specific to the tri-trophic system herbivore-plant-natural enemy and associated chemical interactions indicated in Figure 1 (Mumm and Dicke, 2010; Kaplan, 2012b; McCormick et al., 2012).
Herbivore Volatiles for Host or Prey Location
Natural enemies locate herbivores via their emitted kairomones (Figure 2) (Vet and Dicke, 1992; Afsheen et al., 2008). Volatile compounds emitted by herbivores are generally distinct from plant field background odours and constitute the most reliable sources of information for natural enemies (Vet and Dicke, 1992; Rodriguez-Saona and Stelinski, 2009). Herbivore pheromones are species-specific and usually serve as kairomones for location of suitable herbivore species by natural enemies (Aukema and Raffa, 2005). The egg parasitoid Trichogramma chilonis Ishii (Hymenoptera: Trichogrammatidae) is highly attracted to (Z)-11-hexadecenyl and (E)-12-tetradecenyl acetates which are, respectively, components of the sex pheromones of Helicoverpa assulta (Guenée) (Lepidoptera: Noctuidae) and Ostrinia furnacalis (Guenée) (Lepidoptera: Crambidae) (Boo and Yang, 2000). Other natural enemy species are instead attracted to a blend of pheromone components, as seen in the predatory bug Orius laevigatus (Fieber) (Hemiptera: Anthocoridae), which was only attracted to a mixture of 1:2.3 (R)-lavandulyl acetate and neryl (S)-2-methylbutanoate, the major components of Frankliniella occidentalis (Pergande) (Thysanoptera: Thripidae) aggregation pheromone (Vaello et al., 2017).
Apart from pheromones, natural enemies eavesdrop on volatile compounds produced by herbivore by-products such as honeydew (Leroy et al., 2012; Watanabe et al., 2016), larval frass (Reddy et al., 2002; Wei et al., 2013), and glandular defensive secretions of larvae or adults (Kopf et al., 1997; Zverava and Rank, 2004). A single component, i.e., phenyl acetaldehyde identified from honeydew excreted by the aphid, Aphis gossypii Glover (Hemiptera: Aphididae), attracted the predator Aphidoletes aphidimyza (Rondani) (Diptera: Cecidomyiidae) (Watanabe et al., 2016). Reddy et al. (2002) reported that allyl isothiocyanate, a volatile component of larval frass from P. xylostella-infested cabbage plants, was highly attractive to P. xylostella natural enemies, specifically the predator Chrysoperla carnea (Stepehens) (Neuroptera: Chrysopidae) and the parasitoids C. plutellea and T. chilonis. Moreover, the syrphid fly predator Parasyrphus nigritarsis (Zetterstedt) (Diptera: Syrphidae) was highly attracted to salicyl aldehyde, the main component of the larval secretion of the prey leaf beetle, Phratora vitellinae (L.) (Coleoptera: Chrysomelidae) (Kopf et al., 1997).
Herbivore Contact Kairomones for Host/Prey Recognition and Acceptance
Upon landing on a host plant, natural enemies have to select the target herbivore species and stages for oviposition or feeding (Figure 2) (Jaenike, 1978; Scheirs and De Bruyn, 2002; Gripenberg et al., 2010). This step of the foraging behaviour is crucial as it defines the survival of the forager and its offspring. Plants are usually infested by multiple herbivores, which makes it challenging for natural enemies to select the target species. Herbivore contact kairomones are often species-specific, and therefore natural enemies generally rely on these non-volatile signals, which are examined by antennating or probing for recognition of hosts or prey (Vinson, 1998; Bénédet et al., 2002). These cues originate from various sources such as adult/larva/nymph body surfaces, larvae oral secretions, egg coating, and wing scales (Afsheen et al., 2008; Kaiser et al., 2017). A typical example of this is O-caffeoylserine isolated from the body surface of Phenacoccus herreni Cox & Williams (Homoptera: Pseudococcidae) and which elicited strong drumming behaviour, thereby acting as a contact host-recognition kairomone in the parasitoids Acerophagus coccois Smith and Aenasius vexans Kerrich (both Hymenoptera: Encyrtidae) (Calatayud et al., 2001). Moreover, α-amylase from oral secretions of the host Chilo partellus (Swinhoe) (Lepidoptera: Crambidae) larvae mediates oviposition in the parasitoid Cotesia flavipes Cameron (Hymenoptera: Braconidae) (Bichang'a et al., 2018). Hydrocarbons extracted from herbivores are also involved in host acceptance by parasitoids, as seen in Trissolcus basalis Wollaston (Hymenoptera: Scelionidae) which uses non-adecane, a cuticular hydrocarbon from body extract of its stink bug host, Nezaria viridula (L.) (Hemiptera: Pentatomidae), to discriminate between male and female (Colazza et al., 2007) (see Kaiser et al., 2017, for a recent review on parasitoids). Gomes-Lagôa et al. (2019) further reported the crucial role of hydrocarbons in the selection of preferred stink bug host species by their parasitoids. These authors found that the parasitoid T. basalis preferred the hydrocarbons from the footprint extract of its preferred host, N. viridula, to those of Dichelops melacanthus (Dallas) and Euschistus heros (Fab.) (Hemiptera: Pentatomidae), and the parasitoid Telenomus podisi Ashmead (Hymenoptera: Scelionidae) chose hydrocarbons of the footprint extract of its preferred host, E. heros, to those of the other two stink bug species. The selective responses of the parasitoids to hydrocarbons of their preferred hosts could be explained by differences in the composition of key hydrocarbons in the blend extract (Gomes-Lagôa et al., 2019) and by the co-evolutionary host-parasitoid interactions that have enabled parasitoids to easily recognise and eavesdrop on chemical stimuli of their suitable associated hosts (Peri et al., 2013).
Unlike in parasitoids, contact kairomones involved in prey recognition and acceptance by predatory insects have been explored in few species and little attention has been paid to the identification of the chemical cues involved. However, the predator Cerceris fumipennis Say (Hymenoptera: Crabronidae) was reported to exploit methyl-branch hydrocarbons from the cuticle of Buprestidae beetles to recognise prey from non-prey Chrysomelidae beetles (Rutledge et al., 2014). The author reported that washed buprestid prey that had been treated with their crude cuticular extract were accepted by the predator, whereas those treated with crude cuticular extract of non-prey beetles were rejected, suggesting that the predator uses cuticular hydrocarbons as contact kairomone to discriminate prey from non-prey.
Use of Kairomones in Biological Control Strategies
Biological control is a pest management method where natural enemies are used to reduce populations and damages of pest organisms (Eilenberg et al., 2001). There are three main forms of biological control: classical, augmentation, and conservation (Bale et al., 2008). Classical biological control aims at introducing and releasing a co-evolved exotic natural enemy—generally a parasitoid—of an invasive herbivore into invaded areas for permanent long-term control of the invasive herbivore (Eilenberg et al., 2001; Bale et al., 2008). Augmentation biological control involves periodical releases of a natural enemy when environmental conditions tend to limit its survival, reproduction, and establishment (van Lenteren, 2000; Collier and van Steenwyk, 2004). On the other hand, conservation biological control requires no release, but habitat manipulation or improvement of control practises are implemented to provide resources to maintain and enhance survival, fitness, and reproductive success of natural enemies (Gurr et al., 2000; Zhu et al., 2014). Biological control is one of the most promising alternatives to synthetic chemical insecticides, which are widely applied for the control of herbivores but unsustainable due to the development of herbivore resistance and associated with negative impacts on the environment and human health (Gay, 2012).
Cases of successful implementation of biological control have been documented (e.g., Beddington et al., 1978; Collier and van Steenwyk, 2004). The parasitoid Aphytis melinus DeBach (Hymenoptera: Aphelinidae) was used in an augmentation biological control and successfully controlled the red scale insect Aonidiella aurantii Maskell (Homoptera: Diaspididae) in citrus orchards (Moreno and Luck, 1992). However, many cases of failures in the biological control of insect pests have been observed (Lynch and Thomas, 2000; Collier and van Steenwyk, 2004). The main issues that cause failures include an insufficient population level of natural enemies in the target crop and the emigration or dispersal of natural enemies from cropping sites, limiting the control of insect pests in the target crops (Heimpel and Asplen, 2011). In this line, recent studies have highlighted the use of kairomone-based lures as a sound solution for limiting emigration of natural enemies and increasing their populations in target crops, in turn enhancing the efficacy of natural enemies for satisfactory control of insect pests (Kelly et al., 2014; Peri et al., 2018).
The effectiveness of kairomones in attracting natural enemies to enhance biological control of insect pests has been demonstrated in crop field conditions. The use of HIPVs/OIPVs in crop fields has generally led to a lower ratio of attracted natural enemies in kairomone-treated plots relative to control plots, than when pheromones are used (Tables 1, 2). Unlike HIPVs or OIPVs, pheromones are species-specific (Aukema and Raffa, 2005; Ruther, 2013), and in most cases they are generally distinct from plant background odours and therefore reliable indicators for natural enemies searching for herbivorous insects (Vet and Dicke, 1992; Rodriguez-Saona and Stelinski, 2009). Insects are likely to detect and react to blends of odourant compounds in nature (Thomas-Danguin et al., 2014; Conchou et al., 2019), but reported studies have mainly focused on field applications of single kairomonal compounds (Kaplan, 2012a; Tables 1, 2). The studies published in the last two decades documenting the use of HIPVs, OIPVs, and pheromones, whereby natural enemies have been reported to be more attracted to kairomone-baited plots compared to control plots are summarised in the following subsections.
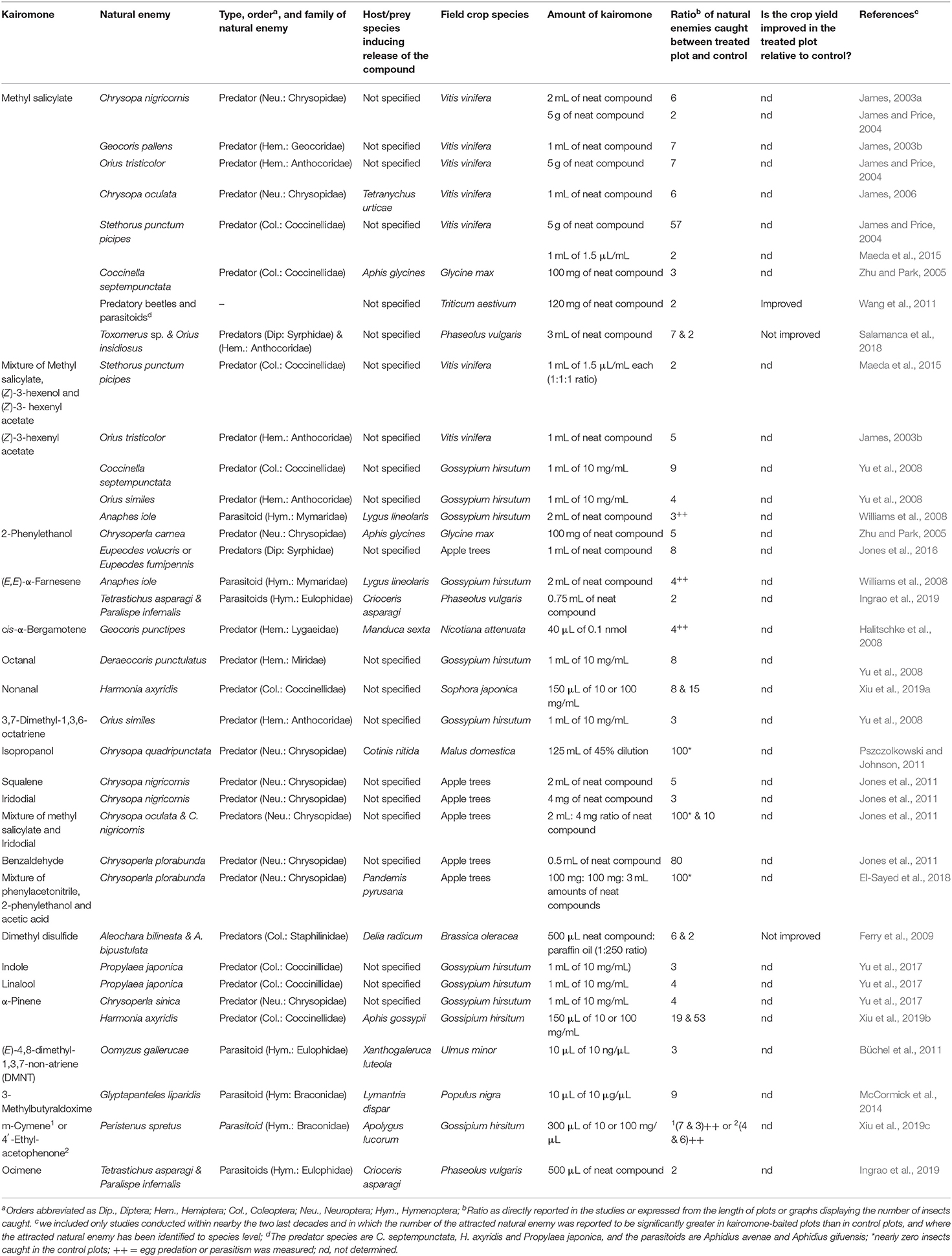
Table 1. Summary of some synthetic herbivore-induced plant volatiles applied in fields to attract natural enemies.
Field Applications of Herbivore-Induced Plant Volatiles
The use of HIPVs and OIPVs as kairomones for the recruitment of natural enemies against insect pests in crop fields has been reported (e.g., Kaplan, 2012a; Peñaflor and Bento, 2013). Applications of methyl salicylate (MeSA) through dispensers in cranberry and soybean fields attracted more natural enemies and resulted in increased predation and parasitism rates, associated with a reduced number of herbivores on plants (e.g., Mallinger et al., 2011; Rodriguez-Saona et al., 2011). A pioneer review has addressed the role of (Z)-3-hexenol in attracting natural enemies into herbivore-infested field plants (Arabidopsis thaliana, Nicotiana spp., and Phazeolus spp.) (Wei et al., 2011). A typical example was reported in a study conducted by Kessler and Baldwin (2001), where plots of natural population of Nicotiana attenuata Torrey ex S. Watson (Solanaceae) plants with (Z)-3-hexenol applied in lanolin paste resulted in an 8-fold increase in eggs and larvae mortality thanks to the attractiveness of the predator Geocoris pallens Stål (Heteroptera: Geocoridae), which preyed on a high number of eggs and larvae of Manduca quinquemaculata (Haworth) (Lepidoptera: Sphingidae), Dicyphus minimus Uhler (Heteroptera: Miridae), and Epitrix hirtipennis (Melsheimer) (Coleoptera: Chrysomelidae). Table 1 provides some additional examples of field-based applications of herbivore-induced plant volatiles that resulted in significant increase in the number of attracted natural enemies in semiochemical-field plots compared to control plots.
Use of Pheromones as Field Attractants for Natural Enemies
The application of pheromones in agricultural crop fields to enhance host location behaviour by natural enemies for the control of insect pests has been the focus of great attention (Aukema and Raffa, 2005; Pfammatter et al., 2015). Pheromones (e.g., sex, aggregation, marking, alarm-pheromones) are environmentally safe for use in crop protection. Aggregation pheromones in bark beetle species are diversified, composed of mono or pluri-chemical compounds (ipsenol, ipsdienol, cis-verbenol, trans-verbenol, frontalin, exo-brevicomin, endo-brevicomin, etc.) (Symonds and Gitau-Clarke, 2016), some of which have shown kairomonal activity in attracting predators in crop fields. For example, high numbers of the predators Thanasimus dubius (Fabricius) (Coleoptera: Cleridae) and Platysoma cylindrica (Paykull) (Coleoptera: Histeridae) were attracted to 50%-ipsdienol-baited traps in red pine, Pinus resinosa Ait. (Pinaceae), plantations (Aukema and Raffa, 2005). Although many studies have focused on the role of herbivore pheromones in field recruitment of natural enemies, sex or aggregation pheromones of natural enemies can also be used as field attractants to manipulate the behaviour of conspecifics (Wu et al., 2019), thereby increasing the natural enemy population level which could indirectly be beneficial for the control of insect pests. Table 2 presents some additional studies on the use of pheromones as field attractants for natural enemies. However, some insect pheromone components appeared to be plant volatile compounds (Tittiger and Blomquist, 2016). Such compounds were not considered in this analysis as they likely exert the same attraction effect as applied HIPVs/OIPVs under field conditions. For example, like HIPVs or OIPVs (Table 1), field application of the aphid-alarm pheromone (E)-β-farnesene, also known to be a plant compound, generally led to 2- to 3-fold more catches of aphid natural enemies in pheromone-treated plots relative to untreated plots (Vosteen et al., 2016, and reference therein).
Avenue of Research for Improving Kairomone Efficiencies and Their Assessment in Biological Control
Applications of synthetic kairomone-based lures in biological control of insect pests continue to gain attention in the field of chemical ecology (Kaplan, 2012a; Kelly et al., 2014; Murali-Baskaran et al., 2018; Peri et al., 2018; Blassioli-Moraes et al., 2019; Peñaflor, 2019). Several questions will have to be addressed in future research to improve kairomone use and thereby to efficiently benefit biological control (Kaplan, 2012b; Gish et al., 2015). Here, we present some strategies and discuss gaps for consideration when designing a kairomone-oriented control strategy for the protection of field crops. This is particularly important because many previous applications of synthetic kairomones have not considered the direct benefits for plants, especially the reduction in pest populations and crop damage, and the increase in crop yields (Hiltpold and Turlings, 2012; Kaplan, 2012a; Tables 1, 2 of this review). It is therefore opportune to examine how kairomones have been implemented in the field and assess possible options to optimise their use.
An Optimised Attract and Reward Technique
The attract and reward technique consists of the combined use of an attractant—usually a kairomone—and a reward component such as flowering companion plants (Simpson et al., 2011). The technique has gained considerable attention since it promotes early establishment of natural enemies in crop fields and reduces their subsequent emigration from these cropping sites (Simpson et al., 2011; Parolin et al., 2012). The attract component stimulates the foraging behaviour and maintenance of natural enemies in target crops, whereas the reward component offers alternative foods such as nectar and pollen which supplement the diet of natural enemies. Consumption of nectar, which is source of carbohydrates, has proved to increase the longevity and fecundity of natural enemies (Araj and Wratten, 2015, and references therein). Apart from supplying extra-floral nectars and pollen, the reward component can also offer additional benefits such as shelter and supply of alternative hosts and prey to natural enemies.
The attract and reward combination significantly increases natural enemy populations in crop fields over the application of either of these components alone (Simpson et al., 2011; Salamanca et al., 2018). The combination of MeSA as attractant and buckwheat Fagopyrum esculentum Möench (Polygonaceae) as reward plant boosted the abundance of Scelonidae wasps on broccoli plants, and that of Eulophidae wasps on sweetcorn plants (Simpson et al., 2011). Salamanca et al. (2018) reported that the combination of MeSA and coriander plants also led to an increased abundance of the predatory thrips Franklinotrips vespiformis (Crawford) (Thysanoptera: Aeolothripidae), predatory bugs Orius insidiosus (Say) (Hemiptera: Anthocoridae), and hoverflies, Toxomerus sp. (Diptera: Syrphidae), on bean plants, but no increase was found in the abundance of earwigs Doru sp. (Dermaptera: Forficulidae). However, the spatial arrangement of the attract and reward components influences the abundance of natural enemies and the control level of insect pests. For example, an increased abundance of the predator Propylea japonica (Thunberg) (Coleoptera: Coccinellidae) and a decreased population of the aphid Aphis citricola van der Goot (Hemiptera: Aphididae) were noted when MeSA was placed inside and Calendula officinalis L. (Asteraceae) reward was placed at the edge of apple tree orchards compared to the opposite arrangement (reward inside and MeSA at the edge) and the control (no MeSA, no reward) (Jaworski et al., 2019). The efficacy of the spatial arrangement supports the design of the push-pull technique which promotes intercropping of companion plants, one placed inside the crop field for repelling herbivores, i.e., “push,” and another placed at the edge of the crop field for attracting them, i.e., “pull” (Cook et al., 2007; Khan et al., 2010). Although the push-push itself increases the abundance of natural enemies compared to mono-cropping (Khan et al., 2008; Leslie et al., 2020), the combination of push-pull with the deployment of synthetic kairomone attractive to natural enemies could further increase the natural enemy population to a level required to reduce the insect pest density and crop damage to below economic threshold levels, which could be assessed in future studies.
Apart from increasing the abundance of natural enemies, the combination of attract and reward has a long-lasting effect, whereby more natural enemies can be sustained over time in a crop field with reward than those without reward. Simpson et al. (2011) used MeSA as attractant and buckwheat as reward and recorded an increase in populations of parasitoids; e.g., Dolichogenidae tasmanica (Cameron), Micropiltis demolitor Wilkinson (both Hymenoptera: Braconidae), Trichogramma pretiosum Riley (Hymenoptera: Trichogrammatidae), Diadegma semiclausum (Hellen) (Hymenoptera: Ichneumonidae), and predators of Tachinidae family in crop fields with the reward compared to those without reward, especially as time elapsed. Jaworski et al. (2019) also recorded more predator P. japonica persisting in apple tree orchards bearing MeSA and the reward, C. officinalis plant, which resulted in long-term control of the pest A. citricola compared to control orchards.
Successful implementation of the attract and reward technique has some limitations, e.g., attraction of non-target organisms. Herbivores and hyper-parasitoids/predators can also be attracted into crop fields because most field-tested HIPVs are commonly emitted volatiles with broad-scale attraction effects, and the reward plants can also serve as habitat for herbivores (Simpson et al., 2011; Orre-Gordon et al., 2013; Sarkar et al., 2018). Methyl salicylate is a common plant volatile compound used to attract natural enemies. However, it was found to be attractive to untargeted insect species such as herbivorous insect pests (e.g., P. rapae, P. xylostella, Scaptomyza flava) and hyper-parasitoids (e.g., Baryscapus galactopus (Ratzeburg) (Hymenoptera: Eulophidae), Anacharis zealandica Ashmead (Hymenoptera: Figitidae), and Diplazon laetatorius Fab. (Hymenoptera: Ichneumonidae) (Orre-Gordon et al., 2013). Buckwheat is a rewarding plant that is commonly used in an attract and reward technique. However, it was also reported to harbour herbivorous thrips such as Thrips tabaci Lindeman (Thysanoptera: Thripidae) and aphids (Orre-Gordon et al., 2013; Sarkar et al., 2018). Despite such unwanted effects, the attract and reward combination could potentially enhance conservation biological control through the recruitment of natural enemies and their retention into the vicinity of target crops (Orre-Gordon et al., 2013; Peñaflor and Bento, 2013). Ways to improve its implementation and overcome such negative impacts could include the selection of HIPVs that are attractive to a narrow range of natural enemies but not to their hosts or prey (Ferry et al., 2009), the use of natural enemy pheromone as the attractant (Ruther, 2013), reward plants which are non-hosts to the target insect pest(s) (Sarkar et al., 2018), an appropriate spatial arrangement of the attractant and reward (Jaworski et al., 2019), and the introduction of a herbivore-repellent component into the system (Cook et al., 2007; Xu et al., 2017a; Pålsson, 2019). Future studies are needed to investigate the integration of these tactics for an improved attract and reward technique to enhance biological control in agro-ecosystems.
Incorporating Olfactory Associative Learning to Improve the Efficiency of Foraging Behaviour by Natural Enemies
Olfactory associative learning is a training process that involves conditioning an insect to learn and recognise to associate a particular odour with the presence of a reward (Arthur, 1971; Turlings et al., 1993). This process tends to modify the insect's innate host preference (Weiss, 1997), which is established during larval development (Hopkins, 1917; Barron, 2001) or shortly after adult emergence (Jaenike, 1983; Barron, 2001). It is likely that insect foragers learn and use odour stimuli that increase their chances of finding suitable hosts (Jaenike, 1983; Vet et al., 1995; Scheirs and De Bruyn, 2002). In conditioned insects, olfactory associative learning develops their ability to exhibit a strong response upon perception of the learned odour (Vet and Papaj, 1992; Faber et al., 1999). Therefore, odour learning has emerged as a strategy to enhance foraging success and to consequently minimise interference of environmental background odours (Vet and Papaj, 1992; Arenas et al., 2007).
The use of olfactory associative learning in natural enemies to improve biological control has recently been a focus of great interest, especially by training or conditioning them on kairomones during the mass rearing process before their release in fields [reviewed in Giunti et al. (2015); Kruidhof et al. (2019)]. Exposure of parasitoid Hyssopus pallidus (Askew) (Hymenoptera: Eulophidae) larvae to the apple fruit extract odour led to an adult preference for apple fruit extract, whereas unexposed individuals did not show any preference (Gandolf et al., 2003). Unlike naïve individuals of the predator Anthocoris nemoralis (Fab.) (Hemiptera: Anthocoridae), individuals that had experience on MeSA-treated prey showed a pronounced preference for the volatile (Drukker et al., 2000). Interestingly, Meiners et al. (2003) showed that parasitoids that had learned to recognise a mixture of compounds could also respond to the individual blend compounds.
The efficiency of olfactory associative learning has also been reported for improving host location behaviour by natural enemies in crop fields. Females of the parasitoids C. glomerata and D. semiclausum with previous experience on HIPVs from Brassicaceae plants infested by P. brassicae and P. xylostella successfully oriented towards host-infested plants even in the presence of surrounding non-host and alternative host plant vegetation, whereas unexperienced parasitoids failed to preferentially locate the host-infested plants (Tibor et al., 2007; Kruidhof et al., 2015). Moreover, Hare et al. (1997) reported that the parasitoid A. melinus previously trained on O-caffeoyltyrosine during rearing achieved greater egg parasitisation on field populations of A. aurantii than naïve parasitoids. Similarly, the predators Cycloneda sanguinea (L.) (Coleoptera: Coccinellidae), Ceraeochrysa cubana (Hagen) (Neuroptera: Chrysopidae), and Podisus nigrispinus (Dallas) (Heteroptera: Pentatomidae) trained on mint oil, a commercial volatile blend predominantly consisting of menthol, menthone, (+)-menthyl acetate, menthofuran, isomenthone, limonene, and 1,8-cineole, consumed more eggs of Thyrinteina leucoceraea (Rindge) (Lepidoptera: Geometridae) and Diatraea saccharalis (Fab.) (Lepidoptera: Pyralidae) in field plots bearing the mint oil than in control plots (Janssen et al., 2014).
Although olfactory associative learning enhances host finding success by natural enemies, it remains unknown how long the learned odour could persist in the insect's memory. Natural enemy species differ in their intrinsic capacity to conserve information over time (short-term, mid-term, and long-term memories) (Vet and Dicke, 1992; Hoedjes et al., 2011; Mery, 2013). Moreover, it is worth considering to what extent acquired information could be conserved under natural conditions, knowing that environmental variation has been reported as one of the major factors underlying variation in learning and memory retention (Mery, 2013; Smid and Vet, 2016). Future studies should therefore be focused on investigating how to fine-tune odour learning in natural enemies for long-term memory consolidation to the benefit of their foraging success. However, periodic releases of natural enemies trained on kairomones during rearing could provide an efficient control of insect pests in crop fields (Hare et al., 1997; Giunti et al., 2015; Kruidhof et al., 2019).
Applying Volatile Kairomones Based on Field Background Odour of Target Crop
Plant field background odour is one factor that could mask the relevance of kairomone-based lures to natural enemies in agricultural fields (Schröder and Hilker, 2008; Cai et al., 2017; Xu et al., 2017b). Some HIPVs and OIPVs are common in the volatile profiles of host plants, but they are often released in different ratios between plant species. Plants therefore have different potentials for shaping the attraction of natural enemies to field applied synthetic HIPVs/OIPVs. Braasch et al. (2012) evaluated the effects of target crop species on parasitoid attraction to MeSA and found that more hymenopteran parasitoids were attracted to MeSA-baited traps in field soybean plants than in corn plants. Similarly, when (E)-β-farnesene and (E)-β-caryophyllene were deployed in beet, bean, and wheat crops, predatory hoverflies of the Syrphidae family were significantly attracted to (E)-β-caryophyllene-baited traps only in beet crops, and to (E)-β-farnesene-baited traps only in bean crops compared to unbaited controls (Heuskin et al., 2012).
Field HIPV-based lures are usually applied in crops which also release the HIPV of interest, and this may increase interference with the successful location of lures by natural enemies. Flint et al. (1979) found that the attraction of the green predatory lacewing, C. carnea, to synthetic caryophyllene applied on cotton plants reduced as the plants grew and released the compound, and detection of the lure thereby waned. Caryophyllene was, however, not attractive to C. carnea in wheat plants (Dean and Satasook, 1983) for which it is one of the most abundant volatile compounds (Jiménez-Martínez et al., 2004). In such cases, the use of concentrations beyond that of the target HIPV in the plant field background odour or in specific blends could help pinpoint the relevant concentration or blend composition that would best attract natural enemies to kairomone-based lures (Szendrei and Rodriguez-Saona, 2010; Xu et al., 2017b). Field application of a synthetic volatile blend composed of ocimene, limonene, (Z)-3-hexenol, and (Z)-3-hexenyl acetate attracted tea leafhoppers in autumn when the tea field background odour contained very low quantity of these compounds, yet the blend was unattractive to the insects in summer when the individual compounds were present in much higher concentrations in the background odour (Xu et al., 2017b).
An alternative strategy for reducing this interplay between the kairomone-based lure and volatile emission by the target field crops could be to use an attractant HIPV in crop fields where it is not released or only released in very low quantity to enhance the detection of the lure within the crop background odour. Field traps baited with phenylacetaldehyde attracted 10–100-fold more predator C. carnea than unbaited traps when deployed in cherry and peach orchards (Tóth et al., 2006). Interestingly, the volatile profiles of cherry and peach plants contain minor amounts or no phenylacetaldehyde (Najar-Rodriguez et al., 2013; Bandeira-Reidel et al., 2017; Ye et al., 2017; Maatallah et al., 2020). Therefore, unlike caryophyllene, it is likely that the predator C. carnea would be attracted to phenylacetaldehyde on cotton and wheat plants of which the headspace volatiles lack this compound (Thompson et al., 1971; Rodriguez-Saona et al., 2001; Jiménez-Martínez et al., 2004; Starr et al., 2015). Two synthetic blends which differed in their benzaldehyde and ethyl benzoate content attracted tea leafhoppers in the laboratory, but the results differed when they were deployed in tea field plantations. The blend that contained ethyl benzoate that was absent in the tea field background odour attracted more tea leafhoppers in field experiments compared to a paraffin oil control solvent (Cai et al., 2017). The blend that contained benzaldehyde, a compound which dominated the tea background odour, was no longer attractive to tea leafhoppers in crop fields (Cai et al., 2017).
Standardising Kairomone-Based Field Implementation Design to Be Able to Draw Generic Conclusions
Kairomones have been validated for the recruitment of natural enemies in crop fields across diverse agro-ecosystems (James and Price, 2004; Tóth et al., 2009; Jones et al., 2016; Cai et al., 2017; Peñaflor, 2019). However, the approaches used in these studies varied, and this may lead to some discrepancies. Although abiotic factors are hardly standardised across landscapes (Almekinders et al., 1995), a number of biotic factors and kairomone implementation practises could be made uniform in studies to strengthen the conclusions drawn from the findings of spatial and temporal studies. There is a need to improve how kairomones have been applied and assessed regarding their effectiveness with the aim of enhancing biological control strategies.
Firstly, there is a need to optimise the concentrations, compositions, and release rates of kairomones under field conditions. Since natural enemies display concentration-dependent responses in nature (James, 2006; Ferry et al., 2007; Kaplan, 2012a), conclusions drawn from studies considering only one concentration of the test kairomone are to be interpreted with caution. Field studies have demonstrated that population levels of attracted natural enemies differed according to the kairomone concentrations (Tables 1, 2). Therefore, it is crucial to test a range of concentrations to better assess the effectiveness of kairomone-based lures and their possible optimisation for field application. Using MeSA-baited yellow sticky cards in vineyards, James (2006) recorded more than 2-fold increase in green predatory lacewing Chrysopa oculata Say (Neuroptera: Chrysopidae) populations in plots baited with 99% MeSA compared to plots baited with 10 and 1% MeSA, while both of the latter did not differ from the control. Similarly, field application of different dimethyl disulfide concentrations (0.1, 1, 10, and 100% v/v dimethyl sulphide/paraffin oil) revealed that a 10% dilution caught the highest number of predators, Aleochara bipustulata (L.) and Aleochara bilineata Gyllenhal (both Coleoptera: Staphilinidae). Whereas more predatory carabid beetles were caught with a 1% concentration compared with a 100% concentration and the control (Ferry et al., 2007). It is also important that future studies focus on the use of odourant mixtures as field attractants for the recruitment of natural enemies. In a simulated model, Chan et al. (2018) showed that odourant mixtures elicit faster olfactory processing responses in insects (e.g., honeybees) and are more reliable for insect olfaction than single odourants. Insects are likely to exploit blend of odourants when foraging in nature (Thomas-Danguin et al., 2014; Conchou et al., 2019). Jones et al. (2011) reported high numbers of the predators C. nigricornis and C. oculata in apple orchard plots with traps baited with the blend of MeSA and iridodial compared to plots with traps baited with either compound alone. The release rate of kairomone-based lures should also be taken into consideration, as it can provide insight on their diffusion and the frequency of lure replacement during the study period. The solvent used to formulate the lure, the dispenser used to deliver it, and some abiotic factors (e.g., temperature and relative humidity) affect the release rate of kairomone and consequently its long-lasting effect and effectiveness in attracting natural enemies in crop fields (Jones et al., 2011; Heuskin et al., 2012; Kaplan, 2012a; Xu et al., 2017b). James (2006) used a 99% MeSA lure replaced at weekly intervals over a 5-week period and observed that the number of captured C. oculata was 5–8-fold higher in MeSA-treated plots than in the controls during the first 3 weeks, but then the captures declined to a low level in the final 2 weeks. Likewise, more predatory ladybeetle and hoverfly adults were recorded on wheat plants when the applied pheromone (E)-β-farnesene-based lure was formulated in paraffin oil compared with an alginate bead-based formulation (Xu et al., 2017b). The observed difference was explained by the effect of the experimental conditions whereby the diffusion of (E)-β-farnesene from alginate beads was limited by the high relative humidity that prevailed, as reported by Heuskin et al. (2012).
Secondly, there is need for an implementation design that enables a uniform distribution of the attracted natural enemies within the semiochemical-treated field. Studies investigating the effects of semiochemical-based lures have been implemented in diverse crop fields, but little attention has been paid to the distribution of natural enemies within the target field, which is nevertheless a key factor with regard to herbivorous insect pest control (Rodriguez-Saona et al., 2011; Braasch and Kaplan, 2012; Lim and Mainali, 2013; Vidal and Murphy, 2018). A simulated model conducted by Kaplan and Lewis (2014) revealed that the size of the kairomone field had a marked effect on the number and distribution of attracted predators, with more predators attracted to small fields compared to large fields, and with abundance of attracted predators within the target field decreasing with increasing distance from the field edge. In these scenarios, the core of the target field becomes an enemy-free space, and therefore an unprotected zone where herbivore outbreaks could occur. Apart from the field size, the density of kairomone-release points and the distance over which the formulated lure can be effective also affect also the distribution of attracted natural enemies for successful control of insect pests. By tracking the abundance of natural enemies over a gradient of distances from the HIPV lure-releasing point, 90d Predalure (AgBio Inc. Westminstewr, CO, USA), a commercially available MeSA lure, was reported to be active over a small spatial scale of 1.5 to 2.5 m (i.e., increased abundance only in a localised area surrounding the emission source) in soybean and cranberries crop fields (Mallinger et al., 2011; Rodriguez-Saona et al., 2011). A 40d Predalure was active over a broad spatial scale of 8–10 m (i.e., increased abundance both at the immediate emission source as well as in neighbouring areas) in tomato crop fields (Kelly et al., 2014). Similarly, phenylethyl alcohol and an induced maize volatile blend were also active at a broad spatial scale of 8–10 m in soybean and maize crop fields (Ockroy et al., 2001; Braasch and Kaplan, 2012). The bioactive attraction range of a formulated kairomone could generate insight on the experimental field size and the adequate lure density (i.e., number of lure sources per unit area), both of which have a profound effect on the abundance of natural enemies and the insect pest control level. For example, over a transect distance of 36 m from pheromone releasing-points, Lim and Mainali (2013) recorded high abundance of the parasitoids Ooencyrtus nezarae Ishii (Hymenoptera: Encyrtidae) and Gryon japonicum (Ashmead) (Hymenoptera: Scelionidae) associated with a high parasitism rate up to 18 m in soybean fields baited with the pheromone [blend of (E)-2-hexenyl (E)-2-hexenoate and (E)-2-hexenyl (Z)-3-hexenoate] identified from the host, Riptortus pedestris (Fab.) (Hemiptera: Alydidae). Using MeSA in hop yards and vineyards, abundance of predators increased with less lure density (James and Price, 2004), but such relationship may depend on the attracted natural enemy species and family (Gadino et al., 2012). Under field conditions, the odour concentration decreases with increasing distance from its source (Beyaert and Hilker, 2014). Therefore, when designing a kairomone-based lure field experiment, it is also important to create enough space between kairomone and kairomone-free zones to prevent interference of odours and emigration of natural enemies between both zones. Although there is no rule on how to define the space of such interzone (buffer zone), we suggest a minimum of 2-fold the distance over which the kairomone-based lure is bioactive. However, future studies are needed to be able to draw evidence-based recommendations.
Thirdly, when used to enhance conservation biological control strategies, a kairomone should not only show effectiveness in attracting natural enemies, but its application should also lead to reduction in pest populations and crop damage through increased parasitism and predation rates, and to an increase in crop yields (Kaplan, 2012a; Salamanca et al., 2018). This may require assessment of kairomone effectiveness shifting from simply looking at natural enemy catches to direct field observation when possible, or at least a combination of the two methods. The field application of synthetic HIPVs is known to prime HIPV production in neighbouring undamaged plants, hence triggering indirect defences against herbivores (Engelberth et al., 2004; von Mérey et al., 2011). James and Price (2004) reported that canopy shake samples from hop yards resulted in a 7- and 57-fold increase in Orius tristicolor (White) (Hemiptera: Anthocoridae) and Stethorus punctum picipes (Casey) (Coleoptera: Coccinellidae), respectively, in MeSA-baited compared to unbaited plots. Although it is widely acknowledged that kairomones increase the abundance of natural enemies in crop fields, it is debatable whether their increase leads to a reduction in crop damage by pests, and a subsequent increase in crop yields. In a 2-year study, Wang et al. (2011) recorded an increase in the abundance of predatory lady beetles (C. septempunctata, H. axyridis, and P. japonica) associated with a reduced density of the grain aphid, Sitobion avenae (Fab.) (Hemiptera: Aphididae), and increases in the parasitism rate and wheat yield in MeSA-baited wheat plots compared to unbaited plots. Although, Salamanca et al. (2018) reported an increased abundance of biocontrol agents (Toxomerus sp. and O. insidiosus) with a reduced spider mite damage to bean plants, the yield of beans was not improved by the application of MeSA compared to control plots. Application of MeSA also did not reduce pest populations or increase strawberry and grapevine yields (Lee, 2010; Simpson et al., 2011). We speculate that, in the latter studies, the population levels of the attracted natural enemies may not have been enough to cause a reduction of crop damage to under the economic threshold level, or the attracted natural enemies may not have been those of the primary insect pests of the target crops. The application of kairomone-based lures may sometimes lead to sex-biased recruitment of natural enemies, whereby one sex (male or female) predominantly responds to the lure, which affects the mating status and may influence natural enemy population dynamics in the target field (Jones et al., 2011; Kaplan, 2012a). One way of dealing with this would be to combine sex-specific attractants to target both sexes of natural enemies. Although the use of semiochemicals has evolved as a novel tool for environmentally friendly pest control, farmers who are the end-users continue to primarily rely on the use of chemical insecticides for crop protection. We suggest that future studies investigate the effectiveness of applying semiochemical-based lures vs. use of chemical insecticides in terms of the reduction of herbivore populations and damage, the improvement of crop yield, and cost-benefit analysis. Above all, the use of semiochemical-based lures offers great potentials for reducing crop damage by pests and improving crop productivity, and the effectiveness of their application could be further improved by taking into account the different aspects discussed earlier in this section.
Concluding Remarks
Over the last two decades, application of kairomones in crop fields has evolved as a novel approach to enhance biological control strategies through the attraction and retention of natural enemies on crops. To date, effective application measures and more effective lures are needed to increase kairomone implementation and boost its effectiveness under field conditions. The efficiency of kairomone-based lures under field conditions could be improved on the basis of the formulation, concentration, ratio, and release rate of the kairomone. Moreover, kairomone detection by natural enemies could be facilitated by taking into consideration the kairomone concentration and the crop field background odour. The control of insect pests by natural enemies could be further enhanced with the use of kairomone-based lures, the release of odour-based experienced natural enemies, and the combination of kairomone with reward plants set up in an appropriate spatial arrangement. Several field experiments have tested the use of single kairomonal compounds, and it is important that future studies assess the use of compound blends in recruiting natural enemies in crop fields. In addition to the attraction of natural enemies, future studies should be focused on evaluating the reduction of pest populations and crop damage when using kairomones compared to pesticide application, as well as the increase in crop yields, so as to be able to address and take full advantage of kairomone-based field pest control.
Author Contributions
PA and ED conceptualised the structure of the review. PA wrote the first draft of the review manuscript. CP, AY, AC, SM, and ED contributed to the development of the review content. All authors provided intellectual inputs, proofread the manuscript, and approved the final version for submission.
Acknowledgements
We thank Dr. Steve S. Baleba for comments on a previous draft of the manuscript. We gratefully acknowledge the bursary offered by the University of Pretoria, and the ICIPE core funding donors: the UK's Foreign, Commonwealth & Development Office (FCDO); the Swedish International Development Cooperation Agency (SIDA); the Swiss Agency for Development and Cooperation (SDC); the Federal Democratic Republic of Ethiopia; and the Kenyan Government. The views expressed herein do not necessarily reflect the official opinion of the donors.
Funding
We gratefully acknowledged the financial supports received from the French National Research Agency (ANR) through CIRAD (Award no. ANR-16-CE32-0010-01); the University of Pretoria and the National Research Foundation through the NRF grants of CWWP (Grant no. CPRR160502163617) and AAY (IFFR Grant no. 109380 and Y-rated Researchers Grant no. RDYR180504326262). PMA was supported by the University of Pretoria and the German Academic Exchange Service (DAAD) In-Region Postgraduate Scholarship (Personal Grant no. 91672680).
Conflict of Interest
The authors declare that the research was conducted in the absence of any commercial or financial relationships that could be construed as a potential conflict of interest.
References
Aartsma, Y., Cusumano, A., de Bobadilla, F. M., Rusman, Q., Vosteen, V., and Poelman, E. H. (2019). Understanding insect foraging in complex habitats by comparing trophic levels: insights from specialist host-parasitoid-hyperparasitoid systems. Cur. Opin. Insect Sci. 32, 54–60. doi: 10.1016/j.cois.2018.11.001
Afsheen, S., Wang, X., Li, R., Zhu, C.-S., and Lou, Y.-G. (2008). Differential attraction of parasitoids in relation to specificity of kairomones from herbivores and their by-products. Insect Sci. 15, 381–397. doi: 10.1111/j.1744-7917.2008.00225.x
Aldrich, J. R., and Cantelo, W. W. (1999). Suppression of colorado potato beetle infestation by pheromone-mediated augmentation of the predatory spined soldier bug, Podisus maculiventris (Say) (Heteroptera: Pentatomidae). Agric. For. Entomol. 1, 209–217. doi: 10.1046/j.1461-9563.1999.00026.x
Almekinders, C. J. M., Fresco, L. O., and Struik, P. C. (1995). The need to study and manage variation in agroecosystems. Neth. J. Agric. Sci. 43, 127–142. doi: 10.18174/njas.v43i2.572
Araj, S. E., and Wratten, S. D. (2015). Comparing existing weeds and commonly used insectary plants as floral resources for a parasitoid. Biol. Control 81, 15–20. doi: 10.1016/j.biocontrol.2014.11.003
Arakaki, N., Yamazawa, H., and Wakamura, S. (2011). The egg parasitoid Telenomus euproctidis (Hymenoptera: Scelionidae) uses sex pheromone released by immobile female tussock moth Orgyia postica (Lepidoptera: Lymantriidae) as kairomone. Appl. Entomol. Zool. 46, 195–200. doi: 10.1007/s13355-011-0031-4
Arenas, A., Fernández, V. M., and Farina, W. M. (2007). Floral odor learning within the hive affects honeybees' foraging decisions. Naturwissenschaften 94, 218–222. doi: 10.1007/s00114-006-0176-0
Arthur, A. P. (1971). Associative learning by Nemeritis canescens (Hymenoptera: Ichneumonidae). Can. Entomol. 103, 1137–1141. doi: 10.4039/Ent1031137-8
Atema, J. (1996). Eddy chemotaxis and odor landscapes: exploration of nature with animal sensors. Biol. Bull. 191, 129–138. doi: 10.2307/1543074
Aukema, B. H., and Raffa, K. F. (2005). Selective manipulation of predators using pheromones: responses to frontalin and ipsdienol pheromone components of bark beetles in the Great Lakes Region. Agric. For. Entomol. 7, 193–200. doi: 10.1111/j.1461-9555.2005.00250.x
Bale, J. S., van Lenteren, J. C., and Bigler, F. (2008). Biological control and sustainable food production. Philos. Trans. R. Soc. Lond. B Biol. Sci. 363, 761–776. doi: 10.1098/rstb.2007.2182
Bandeira-Reidel, R. V., Cioni, P. L., and Pistelli, L. (2017). Volatile emission of different plant parts and fruit development from Italian cherry plums (Prunus cerasifera and P. cerasifera “Pissardii”). Biochem. Syst. Ecol. 75, 10–17. doi: 10.1016/j.bse.2017.10.001
Barron, A. B. (2001). The life and death of hopkins' host-selection principle. J. Insect Behav. 14, 725–737. doi: 10.1023/A:1013033332535
Beddington, J. R., Free, C. A., and Lawton, J. H. (1978). Characteristics of successful natural enemies in models of biological control of insect pests. Nature 273, 513–519. doi: 10.1038/273513a0
Bénédet, F., Leroy, T., Gauthier, N., Thibaudeau, C., Thibout, E., and Renault, S. (2002). Gustatory sensilla sensitive to protein kairomones trigger host acceptance by an endoparasitoid. Proc. R. Soc. Lond. B Biol. Sci. 269, 1879–1886. doi: 10.1098/rspb.2002.2077
Beyaert, I., and Hilker, M. (2014). Plant odour plumes as mediators of plant-insect interactions. Biol. Rev. 89, 68–81. doi: 10.1111/brv.12043
Bichang'a, G., Da Lage, J.-L., Capdevielle-Dulac, C., Zivy, M., Balliau, T., Sambai, K., et al. (2018). α-Amylase mediates host acceptance in the braconid parasitoid Cotesia flavipes. J. Chem. Ecol. 44, 1030–1039. doi: 10.1007/s10886-018-1002-9
Blassioli-Moraes, M. C., Laumann, R. A., Michereff, M. F. F., and Borges, M. (2019). “Semiochemicals for integrated pest management,” in Sustainable Agrochemistry, ed S. Vaz Jr (Cham: Springer Nature Switzerland), 85–112. doi: 10.1007/978-3-030-17891-8_3
Boo, K. S., and Yang, J. P. (2000). Kairomones used by Trichogramma chilonis to find Helicoverpa assulta eggs. J. Chem. Ecol. 26, 359–375. doi: 10.1023/A:1005453220792
Braasch, J., and Kaplan, I. (2012). Over what distance are plant volatiles bioactive? Estimating the spatial dimensions of attraction in an arthropod assemblage. Entomol. Exp. Appl. 145, 115–123. doi: 10.1111/j.1570-7458.2012.01317.x
Braasch, J., Wimp, G. M., and Kaplan, I. (2012). Testing for phytochemical synergism: arthropod community responses to induced plant volatile blends across crops. J. Chem. Ecol. 38, 1264–1275. doi: 10.1007/s10886-012-0202-y
Branco, M., Franco, J. C., Dunkelblum, E., Assael, F., Protasov, A., Ofer, D., et al. (2006). A common mode of attraction of larvae and adults of insect predators to the sex pheromone of their prey (Hemiptera: Matsucoccidae). Bull. Entomol. Res. 96, 179–185. doi: 10.1079/BER2005415
Bruce, T. J. A., and Pickett, J. A. (2011). Perception of plant volatile blends by herbivorous insects - finding the right mix. Phytochemistry 72, 1605–1611. doi: 10.1016/j.phytochem.2011.04.011
Büchel, K., Malskies, S., Mayer, M., Fenning, T. F., Gershenzon, J., Hilker, M., et al. (2011). How plants give early herbivore alert: volatile terpenoids attract parasitoids to egg-infested elms. Basic Appl. Ecol. 12, 403–412. doi: 10.1016/j.baae.2011.06.002
Cabello, T., Rodriguez-Manzaneque, M. A., and Gallego, J. R. (2017). Can the pheromones of predators modulate responses to herbivore-induced plant volatiles? Ann. Appl. Biol. 170, 369–378. doi: 10.1111/aab.12341
Cai, X., Bian, L., Xu, X., Luo, Z., Li, Z., and Chen, Z. (2017). Field background odour should be taken into account when formulating a pest attractant based on plant volatiles. Sci. Rep. 7, 1–10. doi: 10.1038/srep41818
Calatayud, P.-A., Auger, J., Thibout, E., Rousset, S., Caicedo, A. M., Calatayud, S., et al. (2001). Identification and synthesis of a kairomone mediating host location by two parasitoids species of the casava mealybug Phenacoccus herreni. J. Chem. Ecol. 27, 2203–2217. doi: 10.1023/A:1012274703197
Chan, K. H., Hersperger, F., Marachlian, E., Smith, B. H., Locatelli, F., Szyszka, P., et al. (2018). Odorant mixtures elicit less variable and faster responses than pure odorants. PLoS Comput. Biol. 14:e1006536. doi: 10.1371/journal.pcbi.1006536
Colazza, S., Aquila, G., De Pasquale, C., Peri, E., and Millar, J. G. (2007). The egg parasitoid Trissolcus basalis uses n-nonadecane, a cuticular hydrocarbon from its stink bug host Nezara viridula, to discriminate between female and male hosts. J. Chem. Ecol. 33, 1405–1420. doi: 10.1007/s10886-007-9300-7
Colazza, S., and Wanjberg, E. (2013). “Chemical ecology of insect parasitoids: towards a new area,” in Chemical Ecology of Insect Parasitoids, eds E. Wanjberg and S. Colazza (Wiley-Blackwell: John Wiley & Sons, Ltd), 1–8. doi: 10.1002/9781118409589.ch1
Collier, T., and van Steenwyk, R. (2004). A critical evaluation of augmentative biological control. Biol. Control 32, 245–256. doi: 10.1016/j.biocontrol.2004.05.001
Conchou, L., Lucas, P., Meslin, C., Proffit, M., Staudt, M., and Renou, M. (2019). Insect odorscapes: from plant volatiles to natural olfactory scenes. Front. Physiol. 10:972. doi: 10.3389/fphys.2019.00972
Cook, S. M., Khan, Z. R., and Pickett, J. A. (2007). The use of push-pull strategies in integrated pest management. Annu. Rev. Entomol. 52, 375–400. doi: 10.1146/annurev.ento.52.110405.091407
D'Alessandro, M., and Turlings, T. C. J. (2006). Advances and challenges in the identification of volatiles that mediate interactions among plants and arthropods. Analyst 131, 24–32. doi: 10.1039/B507589K
Danner, H., Desurmont, G. A., Cristescu, S. M., and van Dam, N. M. (2018). Herbivore-induced plant volatiles accurately predict history of coexistence, diet breadth, and feeding mode of herbivores. New Phytol. 220, 726–738. doi: 10.1111/nph.14428
Dean, G. J., and Satasook, C. (1983). Response of Chrysoperla carnea (Stephens) (Neuroptera: Chrysopidae) to some potential attractants. Bull. Entomol. Res. 73, 619–624. doi: 10.1017/S0007485300009226
De-Lange, E. S. D., Laplanche, D., Guo, H., Xu, W., Vlimant, M., Erb, M., et al. (2020). Spodoptera frugiperda caterpillars suppress herbivore-induced volatile emissions in maize. J. Chem. Ecol. 46, 344–360. doi: 10.1007/s10886-020-01153-x
De-Moraes, C. M., Lewis, W. J., Paré, P. W., Alborn, H. T., and Tumlinson, J. H. (1998). Herbivore-infested plants selectively attract parasitoids. Nature 393, 570–573. doi: 10.1038/31219
Dicke, M., and Bruin, J. (2001). Chemical information transfer between plants: back to the future. Biochem. Syst. Ecol. 29, 981–994. doi: 10.1016/S0305-1978(01)00045-X
Dicke, M., and Sabelis, M. W. (1988). Infochemical terminology: based on cost-benefit analysis rather than origin of compounds? Funct. Ecol. 2, 131–139. doi: 10.2307/2389687
Dicke, M., and van Loon, J. J. A. (2000). Multitrophic effects of herbivore-induced plant volatile in an evolutionary context. Entomol. Exp. Appl. 97, 237–249. doi: 10.1046/j.1570-7458.2000.00736.x
Drukker, B., Bruin, J., and Sabelis, M. W. (2000). Anthocorid predators learn to associate herbivore-induced plant volatiles with presence or absence of prey. Physiol. Entomol. 25, 260–265. doi: 10.1046/j.1365-3032.2000.00190.x
Eilenberg, J., Hajek, A., and Lomer, C. (2001). Suggestions for unifying the terminology in biological control. BioControl 46, 387–400. doi: 10.1023/A:1014193329979
El-Sayed, A. M., Knight, A. L., Basoalto, E., and Suckling, D. M. (2018). Caterpillar-induced plant volatiles attract conspecific herbivores and a generalist predator. J. Appl. Entomol. 142, 495–503. doi: 10.1111/jen.12495
Engelberth, J., Alborn, H. T., Schmelz, E. A., and Tumlinson, J. H. (2004). Airborne signals prime plants against insect herbivore attack. PNAS 101, 1781–1785. doi: 10.1073/pnas.0308037100
Erb, M., Foresti, N., and Turlings, T. C. J. (2010). A tritrophic signal that attracts parasitoids to host-damaged plants withstands disruption by non-host herbivores. BMC Plant Biol. 10:247. doi: 10.1186/1471-2229-10-247
Faber, T., Joerges, J., and Menzel, R. (1999). Associative learning modifies neural representations of odors in the insect brain. Nat. Neurosci. 2, 74–78. doi: 10.1038/4576
Fatouros, N. E., Dicke, M., Mumm, R., Meiners, T., and Hilker, M. (2008). Foraging behavior of egg parasitoids exploiting chemical information. Behav. Ecol. 19, 677–689. doi: 10.1093/beheco/arn011
Fellowes, M. D. E., van Alphen, J. J. M., and Jervis, M. A. (2007). “Foraging behaviour,” in Insects as Natural Enemies: A Pratical Perspective, ed M. A. Jervis (Dordrecht: Springer), 1–71. doi: 10.1007/978-1-4020-2625-6_1
Felton, G. W., Chung, S. H., Hernandez, M. G. E., Louis, J., Peiffer, M., and Tian, D. (2014). “Herbivore oral secretions are the first line of protection against plant-induced defences,” in Annual Plant Reviews, Insect Plant-Interactions, eds C. Voelckel and G. Jander (Oxford: Wiley), 37–76. doi: 10.1002/9781119312994.apr0506
Ferry, A., Dugravot, S., Delattre, T., Bagnères, A.-G., Poinsot, D., and Cortesero, A. M. (2007). Identification of a widespread monomolecular odor differentially attractive to several delia radicum ground-dwelling predators in the field. J. Chem. Ecol. 33, 2064–2077. doi: 10.1007/s10886-007-9373-3
Ferry, A., Tron, L. E., Dugravot, S., and Cortesero, A. M. (2009). Field evaluation of the combined deterrent and attractive effects of dimethyl disulfide on Delia radicum and its natural enemies. Biol. Control 49, 219–226. doi: 10.1016/j.biocontrol.2009.01.013
Flint, H. M., Salter, S. S., and Walters, S. (1979). Caryophyllene: an attractant for the green lacewing. Environ. Entomol. 8, 1123–1125. doi: 10.1093/ee/8.6.1123
Frost, C. J., Mescher, M. C., Carlson, J. E., and De Moraes, C. M. (2008). Plant defense priming against herbivores: getting ready for a different battle. Plant Physiol. 146, 818–824. doi: 10.1104/pp.107.113027
Gadino, A. N., Walton, V. M., and Lee, J. C. (2012). Evaluation of methyl salicylate lures on populations of Typhlodromus pyri (Acari: Phytoseiidae) and other natural enemies in Western Oregon vineyards. Biol. Control 63, 48–55. doi: 10.1016/j.biocontrol.2012.06.006
Gandolf, M., Mattiacci, L., and Dorn, S. (2003). Preimaginal learning determines adult response to chemical stimuli in a parasitic wasp. Royal Soc. 270, 2623–2629. doi: 10.1098/rspb.2003.2541
Gay, H. (2012). Before and after silent spring : from chemical pesticides to biological control and integrated pest management — Britain, 1945–1980. Ambix 59, 88–108. doi: 10.1179/174582312X13345259995930
Giacometti, R., Barneto, J., Barriga, L. G., Pedro, M. S., Balestrasse, K., Andrade, A. M., et al. (2016). Early perception of stink bug damage in developing seeds of field-grown soybean induces chemical defences and reduces bug attack. Pest Manag. Sci. 72, 1585–1594. doi: 10.1002/ps.4192
Gish, M., De-Moraes, C. M., and Mescher, M. C. (2015). Herbivore-induced plant volatiles in natural and agricultural ecosystems: open questions and future prospects. Cur. Opin. Insect Sci. 9, 1–6. doi: 10.1016/j.cois.2015.04.001
Giunti, G., Canale, A., Messing, R. H., Donati, E., Stefanini, C., Michaud, J. P., et al. (2015). Parasitoid learning : current knowledge and implications for biological control. Biol. Control. 90, 208–219. doi: 10.1016/j.biocontrol.2015.06.007
Gomes-Lagôa, A. C., Blassioli-Moraes, M. C., Borges, M., and Laumann, R. A. (2019). Selective responses of Trissolcus basalis and Telenomus podisi to chemical footprints of preferred hosts. Physiol. Entomol. 45, 60–71. doi: 10.1111/phen.12316
Gripenberg, S., Mayhew, P. J., Parnell, M., and Roslin, T. (2010). A meta-analysis of preference-performance relationships in phytophagous insects. Ecol. Lett. 13, 383–393. doi: 10.1111/j.1461-0248.2009.01433.x
Gross, H. R., Lewis, W. J., Jones, R. L., and Nordlund, D. A. (1975). Kairomones and their use for management of entomophagous insects: III. stimulation of Trichogramma achaeae, T. pretiosum, and Microplitis croceipes with host-seeking stimuli at time of release to improve their efficiency. J. Chem. Ecol. 1, 431–438. doi: 10.1007/BF00988584
Gunton, R. M., and Pöyry, J. (2016). Scale-specific spatial density dependence in parasitoids: a multi-factor meta-analysis. Funct. Ecol. 30, 1501–1510. doi: 10.1111/1365-2435.12627
Gurr, G. M., Wratten, S. D., and Barbosa, P. (2000). “Success in conservation biological control of arthropods,” in Measures of Success in Biological Control, eds G. Gurr and S. Wratten (Dordrecht: Kluwer Academic Publishers), 105–132. doi: 10.1007/978-94-011-4014-0_4
Halitschke, R., Stenberg, J. A., Kessler, D., Kessler, A., and Baldwin, I. T. (2008). Shared signals - ‘alarm calls' from plants increase apparency to herbivores and their enemies in nature. Ecol. Lett. 11, 24–34. doi: 10.1111/j.1461-0248.2007.01123.x
Hare, J. D., Morgan, D. J. W., and Nguyun, T. (1997). Increased parasitization in the field after exposing its parasitoid, Aphytis melinus, to a synthetic kairomone. Entomol. Exp. Appl. 82, 73–81. doi: 10.1046/j.1570-7458.1997.00115.x
Heil, M., and Ton, J. (2008). Long-distance signalling in plant defence. Trends Plant Sci. 13, 264–272. doi: 10.1016/j.tplants.2008.03.005
Heimpel, G. E., and Asplen, M. K. (2011). A ‘Goldilocks' hypothesis for dispersal of biological control agents. BioControl 56, 441–450. doi: 10.1007/s10526-011-9381-7
Heuskin, S., Lorge, S., Lognay, G., Wathelet, J.-P., Bera, F., Leroy, P., et al. (2012). A semiochemical slow-release formulation in a biological control approach to attract hoverflies. J. Environ. Ecol. 3, 72–85. doi: 10.5296/jee.v3i1.1725
Hilker, M., and Fatouros, N. E. (2015). Plant responses to insect egg deposition. Annu. Rev. Entomol. 60, 493–515. doi: 10.1146/annurev-ento-010814-020620
Hiltpold, I., and Turlings, T. C. J. (2012). Manipulation of chemically mediated interactions in agricultural soils to enhance the control of crop pests and to improve crop yield. J. Chem. Ecol. 38, 641–650. doi: 10.1007/s10886-012-0131-9
Hoedjes, K. M., Kruidhof, H. M., Huigens, M. E., Dicke, M., Vet, L. E. M., and Smid, H. M. (2011). Natural variation in learning rate and memory dynamics in parasitoid wasps : opportunities for converging ecology and neuroscience. Proc. Royal Soc. B: Biol. Sci. 278, 889–897. doi: 10.1098/rspb.2010.2199
Hopkins, A. D. (1917). A discussion of H. G. Hewitt's paper on insect behaviour. J. Econ. Entomol. 10, 92–93.
Ingrao, A. J., Walters, J., and Szendrei, Z. (2019). Biological control of Asparagus pests using synthetic herbivore-induced volatiles. Environ. Entomol. 48, 202–210. doi: 10.1093/ee/nvy171
Jaenike, J. (1978). On optimal oviposition behavior in phytophagous insects. Theoretical Popul. Biol. 14, 350–356. doi: 10.1016/0040-5809(78)90012-6
Jaenike, J. (1983). Induction of host preference in Drosophila melanogaster. Oecologia 58, 320–325. doi: 10.1007/BF00385230
James, D. G. (2003a). Field evaluation of herbivore-induced plant volatiles as attractants for beneficial insects: methyl salicylate and the green lacewing, Chrysopa nigricornis. J. Chem. Ecol. 29, 1601–1609. doi: 10.1023/a:1024270713493
James, D. G. (2003b). Synthetic herbivore-induced plant volatiles as field attractants for beneficial insects. Environ. Entomol. 32, 977–982. doi: 10.1603/0046-225X-32.5.977
James, D. G. (2006). Methyl salicylate is a field attractant for the goldeneyed lacewing, Chrysopa oculata. Biocontrol Sci. Technol. 16, 107–110. doi: 10.1080/09583150500188510
James, D. G., and Price, T. S. (2004). Field-testing of methyl salicylate for recruitment and retention of beneficial insects in grapes and hops. J. Chem. Ecol. 30, 1613–1628. doi: 10.1023/B:JOEC.0000042072.18151.6f
Janssen, A., Fonseca, J. O., Colares, F., Silva, L., Pedrosa, A. R. P., Lima, E. R., et al. (2014). Time scales of associating food and odor by predator communities in the field. Behav. Ecol. 25, 1123–1130. doi: 10.1093/beheco/aru094
Jaworski, C. C., Xiao, D., Xu, Q., Ramirez-Romero, R., Guo, X., Wang, S., et al. (2019). varying the spatial arrangement of synthetic herbivore-induced plant volatiles and companion plants to improve conservation biological control. J. Appl. Ecol. 56, 1176–1788. doi: 10.1111/1365-2664.13353
Jiménez-Martínez, E. S., Bosque-Pérez, N. A., Berger, P. H., Zemetra, R. S., Ding, H., and Eigenbrode, S. D. (2004). Volatile cues influence the response of rhopalosiphum padi (Homoptera: Aphididae) to barley yellow dwarf virus–infected transgenic and untransformed wheat. J. Chem. Ecol. 33, 1207–1216. doi: 10.1603/0046-225X-33.5.1207
Jing, T., Du, W., Gao, T., Wu, Y., Zhang, N., Zhao, M. J., et al. (2020). Herbivore-induced DMNT catalyzed by CYP82D47 plays an important role in the induction of JA-dependent herbivore resistance of neighboring tea plants. Plant Cell Environ. 44, 1178–1191. doi: 10.1111/pce.13861
Jones, V. P., Horton, D. R., Mills, N. J., Unruh, T. R., Baker, C. C., Melton, T. D., et al. (2016). Evaluating plant volatiles for monitoring natural enemies in apple, pear and walnut orchards. Biol. Control. 102, 53–65. doi: 10.1016/j.biocontrol.2015.03.009
Jones, V. P., Steffan, S. A., Wiman, N. G., Horton, D. R., Miliczky, E., Zhang, Q.-H., et al. (2011). Evaluation of herbivore-induced plant volatiles for monitoring green lacewings in Washington apple orchards. Biol. Control 56, 98–105. doi: 10.1016/j.biocontrol.2010.10.001
Kaiser, L., Ode, P., van Nouhuys, S., Calatayud, P. A., Colazza, S., Cortesero, A. M., et al. (2017). “The plant as a habitat for entomophagous insects,” in Insect-Plant Interactions in a Crop Protection Perspective. Advances in Botanical Research, eds N. Sauvion, P-A. Calatayud, and D. Thiéry (Londres: Elsevier Ltd.), 179–223. doi: 10.1016/bs.abr.2016.09.006
Kaplan, I. (2012a). Attracting carnivorous arthropods with plant volatiles: the future of biocontrol or playing with fire? Biol. Control. 60, 77–89. doi: 10.1016/j.biocontrol.2011.10.017
Kaplan, I. (2012b). Trophic complexity and the adaptive value of damage-induced plant volatiles. PLoS Biol. 10:e1001437. doi: 10.1371/journal.pbio.1001437
Kaplan, I., and Lewis, D. (2014). What happens when crops are turned on? Simulating constitutive volatiles for tritrophic pest suppression across an agricultural landscape. Pest Manag. Sci. 71, 139–150. doi: 10.1002/ps.3779
Kelly, J. L., Hagler, J. R., and Kaplan, I. (2014). Semiochemical lures reduce emigration and enhance pest control services in open-field predator augmentation. Biol. Control 71, 70–77. doi: 10.1016/j.biocontrol.2014.01.010
Kessler, A., and Baldwin, I. T. (2001). Defensive function of herbivore-induced plant volatile emissions in nature. Science 291, 2141–2244. doi: 10.1126/science.291.5511.2141
Khan, Z. R., James, D. G., Midega, C. A. O., and Pickett, J. A. (2008). Chemical ecology and conservation biological control. Biol. Control 45, 210–224. doi: 10.1016/j.biocontrol.2007.11.009
Khan, Z. R., Midega, C. A. O., Bruce, T. J. A., Hooper, A. M., and Pickett, J. A. (2010). Exploiting phytochemicals for developing a ‘push-pull' crop protection strategy for cereal farmers in Africa. J. Exp. Bot. 61, 4185–4196. doi: 10.1093/jxb/erq229
Kopf, A., Rank, N. E., Roininen, H., and Tahvanainen, J. (1997). Defensive larval secretions of leaf beetles attract a specialist predator parasyrphus nigritarsis. Ecol. Entomol. 22, 176–183. doi: 10.1046/j.1365-2311.1997.t01-1-00061.x
Kpongbe, H., van den Berg, J., Khamis, F., Tam,ò, M., and Torto, B. (2019). Isopentyl butanoate : aggregation pheromone of the brown spiny bug, Clavigralla tomentosicollis (Hemiptera: Coreidae), and kairomone for the egg parasitoid Gryon sp. (Hymenoptera: Scelionidae). J. Chem. Ecol. 45, 570–578. doi: 10.1007/s10886-019-01081-5
Kramer, D. L. (2001). “Foraging behavior,” in Evolutionary Ecology, Concepts and Cases Studies, eds C. W. Fox, D. A. Roff, and D. J. Fairbairn (Oxford: Oxford University Press), 232–246. doi: 10.1093/oso/9780195131543.003.0024
Krost, C. (2008). “Chemical communication,” in Encyclopedia of Ecology, eds S. E. Jorgensen and B. D. Fath (Oxford: Elsevier), 557–575. doi: 10.1016/B978-008045405-4.00036-7
Kruidhof, H. M., Kostenko, O., Smid, H. M., and Vet, L. E. M. (2019). Integrating parasitoid olfactory conditioning in augmentative biological control: potential impact, possibilities, and challenges. Front. Ecol. Evol. 7:84. doi: 10.3389/fevo.2019.00084
Kruidhof, H. M., Roberts, A. L., Magdaraog, P., Gols, D. M. R., Vet, L. E. M., Hoffmeister, T. S., et al. (2015). Habitat complexity reduces parasitoid foraging efficiency, but does not prevent orientation towards learned host plant odours. Oecologia 179, 353–361. doi: 10.1007/s00442-015-3346-y
Krupke, C. H., and Brunner, J. F. (2003). Parasitoids of the consperse stink bug (Hemiptera: Pentatomidae) in North Central Washington and attractiveness of a host-produced pheromone component. J. Entomol. Sci. 38, 84–92. doi: 10.18474/0749-8004-38.1.84
Lee, J. C. (2010). Effect of methyl salicylate-based lures on beneficial and pest arthropods in strawberry. J. Chem. Ecol. 39, 653–660. doi: 10.1603/EN09279
Leroy, P. D., Heuskin, S., Sabri, A., Verheggen, F. J., Farmakidis, J., Lognay, G., et al. (2012). Honeydew volatile emission acts as a kairomonal message for the Asian lady beetle Harmonia axyridis (Coleoptera: Coccinellidae). Insect Sci. 19, 498–506. doi: 10.1111/j.1744-7917.2011.01467.x
Leslie, A. W., Hamby, K. A., McCluen, S. R., and Hooks, C. R. R. (2020). Evaluating a push-pull tactic for management of Epilachna varivestis mulsant and enhancement of beneficial arthropods in Phaseolus lunatus L. Ecol. Eng. 7:105660. doi: 10.1016/j.ecoleng.2019.105660
Lewis, W. J., Jones, R. L., Nordlund, D. A., and Sparks, A. N. (1975). Kairomones and their use for management of entomophagous insects: I. evaluation for increasing rates of parasitization by Trichogramma spp. in the field. J. Chem. Ecol. 1, 343–347. doi: 10.1007/BF00988836
Lim, U. T., and Ben-Yakir, D. (2020). Visual sensory systems of predatory and parasitic arthropods. Biocontrol Sci. Technol. 30, 728–739. doi: 10.1080/09583157.2020.1752362
Lim, U. T., and Mainali, B. P. (2013). Effect of aggregation pheromone trap of Riptortus pedestris (Hemiptera: Alydidae) on the distribution and composition of its egg parasitoids. Biol. Microb. Control 106, 1973–1978. doi: 10.1603/EC13154
Lynch, L. D., and Thomas, M. B. (2000). Nontarget effects in the biocontrol of insects with insects, nematodes and microbial agents: the evidence. Biocontrol News Inform. 21, 117N−130N.
Maatallah, S., Dabbou, S., Castagna, A., Guizani, M., Hajlaoui, H., Ranieri, A. M., et al. (2020). Prunus persica by-products: a source of minerals, phenols and volatile compounds. Sci. Hortic. 261:109016. doi: 10.1016/j.scienta.2019.109016
Maeda, T., Kishimoto, H., Wright, L. C., and James, D. G. (2015). Mixture of synthetic herbivore-induced plant volatiles attracts more stethorus punctum picipes (Casey) (Coleoptera: Coccinellidae) than a single volatile. J. Insect Behav. 28, 126–137. doi: 10.1007/s10905-015-9489-1
Magalhães, D. M., da Silva, I. T. F. A., Borges, M., Laumann, R. A., and Blassioli-Moraes, M. C. (2019). Anthonomus grandis aggregation pheromone induces cotton indirect defence and attracts the parasitic wasp Bracon vulgaris. J. Exp. Bot. 70, 1891–1901. doi: 10.1093/jxb/erz040
Mallinger, R. E., Hogg, D. B., and Gratton, C. (2011). Methyl salicylate attracts natural enemies and reduces populations of soybean aphids (Hemiptera: Aphididae) in soybean agroecosystems. J. Econ. Entomol. 104, 115–124. doi: 10.1603/EC10253
Mbaluto, C. M., Ayelo, P. M., Duffy, A. G., Erdei, A. L., Tallon, A. K., Xia, S., et al. (2020). Insect chemical ecology: chemically mediated interactions and novel applications in agriculture. Arthropod-Plant Interact. 14, 671–684. doi: 10.1007/s11829-020-09791-4
McCormick, A. C., Irmisch, S., Reinecke, A., Boeckler, G. A., Veit, D., Reichelt, M., et al. (2014). Herbivore-induced volatile emission in black poplar: regulation and role in attracting herbivore enemies. Plant Cell Environ. 37, 1909–1923. doi: 10.1111/pce.12287
McCormick, A. C., Unsicker, S. B., and Gershenzon, J. (2012). The specificity of herbivore-induced plant volatiles in attracting herbivore enemies. Trends Plant Sci. 17, 303–310. doi: 10.1016/j.tplants.2012.03.012
Meiners, T., Wäckers, F., and Lewis, W. J. (2003). Associative learning of complex odours in parasitoid host location. Chem. Senses 28, 231–236. doi: 10.1093/chemse/28.3.231
Mendel, Z., Assael, F., and Dunkelblum, E. (2004). Kairomonal attraction of predatory bugs (Heteroptera: Anthocoridae) and brown lacewings (Neuroptera: Hemerobiidae) to sex pheromones of Matsucoccus species (Hemiptera: Matsucoccidae). Biol. Control 30, 134–440. doi: 10.1016/j.biocontrol.2003.06.001
Mery, F. (2013). Natural variation in learning and memory. Cur. Opin. Neurobiol. 23, 52–56. doi: 10.1016/j.conb.2012.09.001
Mills, N. J., and Heimpel, G. E. (2018). Could increased understanding of foraging behavior help to predict the success of biological control? Cur. Opin. Insect Sci. 27, 26–31. doi: 10.1016/j.cois.2018.02.013
Mills, N. J., and Wajnberg, E. (2008). “Optimal foraging behavior and efficient biological control methods,” in Behavioral Ecology of Insect Parasitoids: From Theoretical Approaches to Field Applications, eds E. Wanjberg, C. Bernstein, and J. J. M. van Alphen (Blackwell: Oxford), 3–30. doi: 10.1002/9780470696200.ch1
Moreno, D. S., and Luck, R. F. (1992). Augmentative releases of Aphytis melinus (Hymenoptera: Aphelinidae) to suppress california red scale (Homoptera: Diaspididae) in Southern California lemon orchards. J. Econ. Entomol. 85, 1112–1119. doi: 10.1093/jee/85.4.1112
Mumm, R., and Dicke, M. (2010). Variation in natural plant products and the attraction of bodyguards involved in indirect plant defense. Can. J. Zool. 88, 628–667. doi: 10.1139/Z10-032
Murali-Baskaran, R. K., Chander, K., Kaushal, P., Kumar, J., Parthiban, P., Senthil-Nathan, S., et al. (2018). Role of kairomone in biological control of crop pests - a review. Physiol. Mol. Plant Pathol. 101, 3–15. doi: 10.1016/j.pmpp.2017.07.004
Najar-Rodriguez, A., Orschel, B., and Dorn, S. (2013). Season-long volatile emissions from peach and pear trees in situ, overlapping profiles, and olfactory attraction of an oligophagous fruit moth in the laboratory. J. Chem. Ecol. 39, 418–429. doi: 10.1007/s10886-013-0262-7
Ockroy, M. L. B., Turlings, T. C. J., Edwards, P. J., Fritzsche-hoballah, M. E., Ambrosetti, L., Bassetti, P., et al. (2001). Response of natural populations of predators and parasitoids to artificially induced volatile emissions in maize plants (Zea mays L.). Agric. For. Entomol. 3, 201–209. doi: 10.1046/j.1461-9555.2001.00107.x
Orre-Gordon, G. U. S., Wratten, S. D., Jonsson, J., Simpson, M., and Hale, R. (2013). ‘Attract and Reward': combining a herbivore-induced plant volatile with floral resource supplementation - multi-trophic level effects. Biol. Control 64, 106–115. doi: 10.1016/j.biocontrol.2012.10.003
Pålsson, J. (2019). Attract, Reward and Disrupt: Combining Habitat Manipulation and Semiochemicals to Enhance Pest Control in Apple Orchards. [PhD Thesis]: Swedish University of Agricultural Sciences.
Pappas, M. L., Steppuhn, A., Geuss, D., Topalidou, N., Zografou, A., Sabelis, M. W., et al. (2015). Beyond predation: the zoophytophagous predator Macrolophus pygmaeus induces tomato resistance against spider mites. PLoS ONE 10:e0127251. doi: 10.1371/journal.pone.0127251
Parolin, P., Bresch, C., Desneux, N., Brun, R., Bout, A., Boll, R., et al. (2012). Secondary plants used in biological control: a review. Int. J. Pest Manag. 58, 91–100. doi: 10.1080/09670874.2012.659229
Peñaflor, M. F. G. V. (2019). “Use of semiochemical-based strategies to enhance biological control,” in Natural Enemies of Insect Pests in Neotropical Agroecosystems: Biological Control and Functional Biodiversity, eds B. Souza, L. L. Vazquez, and R. C. Marucci (Cham: Springer Nature Switzerland), 479–488. doi: 10.1007/978-3-030-24733-1_41
Peñaflor, M. F. G. V., and Bento, J. M. S. (2013). Herbivore-induced plant volatiles to enhance biological control in agriculture. Neotrop. Entomol. 42, 331–343. doi: 10.1007/s13744-013-0147-z
Pérez-Hedo, M., Urbaneja-Bernat, P., Jaques, J. A., Flors, V., and Urbaneja, A. (2015). Defensive plant responses induced by Nesidiocoris tenuis (Hemiptera: Miridae) on tomato plants. J. Pest Sci. 88, 543–554. doi: 10.1007/s10340-014-0640-0
Peri, E., Frati, F., Salerno, G., Conti, E., and Colazza, S. (2013). Host chemical footprints induce host sex discrimination ability in egg parasitoids. PLoS ONE 8:e79054. doi: 10.1371/journal.pone.0079054
Peri, E., Moujahed, R., Wajnberg, E., and Colazza, S. (2018). “Applied chemical ecology to enhance insect parasitoid efficacy in the biological control of crop pests,” in Chemical Ecology of Insects: Applications and Associations with Plants and Microbes, ed J. Tabata (London; New York, NY: Taylor & Francis), 234–267. doi: 10.1201/9781351228398-10
Pervez, A., and Yadav, M. (2018). Foraging behaviour of predaceous ladybird beetles: a review. Eur. J. Environ. Sci. 8, 102–108. doi: 10.14712/23361964.2018.14
Pfammatter, J. A., Krause, A., and Raffa, K. F. (2015). Evaluating predators and competitors in wisconsin red pine forests for attraction to mountain pine beetle pheromones for anticipatory biological control. J. Chem. Ecol. 44, 1161–1171. doi: 10.1093/ee/nvv091
Price, P. W., Bouton, C. E., Gross, P., McPheron, B. A., Thompson, J. N., and Weis, A. E. (1980). Interactions among three trophic levels: influence of plants on interactions between insect herbivores and natural enemies. Annu. Rev. Ecol. Syst. 11, 41–65. doi: 10.1146/annurev.es.11.110180.000353
Pszczolkowski, M. A., and Johnson, D. T. (2011). Isopropanol attracts the green lacewing, Chrysopa quadripunctata (Neuroptera: Chrysopidae). Biocontrol Sci. Techn. 21, 47–50. doi: 10.1080/09583157.2010.524294
Randlkofer, B., Obermaier, E., Hilker, M., and Meiners, T. (2010). Vegetation complexity-the influence of plant species diversity and plant structures on plant chemical complexity and arthropods. Basic Appl. Ecol. 11, 383–395. doi: 10.1016/j.baae.2010.03.003
Reddy, G. V. P., Holopainen, J. K., and Guerrero, A. (2002). Olfactory responses of Plutella xylostella natural enemies to host pheromone, larval frass, and green leaf cabbage volatiles. J. Chem. Ecol. 28, 131–143. doi: 10.1023/a:1013519003944
Rim, H., Uefune, M., Ozawa, R., and Takabayashi, J. (2018). An omnivorous arthropod, Nesidiocoris tenuis, induces gender-specific plant volatiles to which conspecific males and females respond differently. Arthropod-Plant Interact. 12, 495–503. doi: 10.1007/s11829-018-9612-2
Rodriguez-Saona, C., Crafts-Brandner, S. J., and Canas, L. A. (2003). Volatile emissions triggered by multiple herbivore damage: beet armyworm and whitefly feeding on cotton plants. J. Chem. Ecol. 29, 2539–2550. doi: 10.1023/A:1026314102866
Rodriguez-Saona, C., Crafts-Brandner, S. J., Paré, P. W., and Henneberry, T. J. (2001). Exogenous methyl jasmonate induces volatile emissions in cotton plants. J. Chem. Ecol. 27, 679–695. doi: 10.1023/A:1010393700918
Rodriguez-Saona, C., Kaplan, I., Braasch, J., Chinnasamy, D., and Williams, L. (2011). Field responses of predaceous arthropods to methyl salicylate: a meta-analysis and case study in cranberries. Biol. Control 59, 294–303. doi: 10.1016/j.biocontrol.2011.06.017
Rodriguez-Saona, C., and Stelinski, L. L. (2009). “Behavior-modifying strategies in IPM: theory and practice,” in Integrated Pest Management: Innovation and Development, ed A. K. D. R. Peshin (New York, NY: Springer), 261–312. doi: 10.1007/978-1-4020-8992-3_11
Ruther, J. (2013). “Novel insights into pheromone-mediated communication in parasitic hymenopterans,” in Recent Advances in Chemical Ecology of Insect Parasitoids, eds E. Wajnberg and S. Colazza (Wiley-Blackwell: John Wiley and Sons, Ltd.), 112–144. doi: 10.1002/9781118409589.ch6
Rutledge, C. E., Silk, P. J., and Mayo, P. (2014). Use of contact chemical cues in prey discrimination by Cerceris fumipennis. Entomol. Exp. Appl. 153, 93–105. doi: 10.1111/eea.12233
Salamanca, J., Souza, B., and Rodriguez-Saona, C. (2018). Cascading effects of combining synthetic herbivore-induced plant volatiles with companion plants to manipulate natural enemies in agro-ecosystem. Pest Manag. Sci. 74, 2133–2145. doi: 10.1002/ps.4910
Sarkar, S. C., Wang, E., Wu, S., and Lei, Z. (2018). Application of trap cropping as companion plants for the management of agricultural pests: a review. Insects 9:128. doi: 10.3390/insects9040128
Sarmento, R. A., Lemos, F., Bleeker, P. M., Schuurink, R. C., Pallini, A., Olivera, A. M. G., et al. (2011). A herbivore that manipulates plant defence. Ecol. Lett. 14, 229–236. doi: 10.1111/j.1461-0248.2010.01575.x
Schausberger, P. (2018). Herbivore-associated bacteria as potential mediators and modifiers of induced plant defense against spider mites and thrips. Front. Plant Sci. 9:1107. doi: 10.3389/fpls.2018.01107
Scheirs, J., and De Bruyn, L. (2002). Integrating optimal foraging and optimal oviposition theory in plant–insect research. Oikos 96, 187–191. doi: 10.1034/j.1600-0706.2002.960121.x
Schröder, R., and Hilker, M. (2008). The relevance of background odor in resource location by insects: a behavioral approach. BioScience 58, 308–316. doi: 10.1641/B580406
Shiojiri, K., Ozawa, R., Kugimiya, S., Uefune, M., van Wijk, M., Sabelis, M. W., et al. (2010). Herbivore-specific, density-dependent induction of plant volatiles: honest or ‘cry wolf' signals? PLoS One 5:e121614. doi: 10.1371/journal.pone.0012161
Shiojiri, K., Takabayashi, J., and Yano, S. (2001). Infochemically mediated tritrophic interaction webs on cabbage plants. Popul. Ecol. 43, 23–29. doi: 10.1007/PL00012011
Silva, D. B., Bueno, V. H. P., van Loon, J. J. A., Peñaflor, M. F. G. V., Bento, J. M. S., and van Lenteren, J. C. (2018). Attraction of three mirid predators to tomato infested by both the tomato leaf mining moth tuta absoluta and the whitefly Bemisia tabaci. J. Chem. Ecol. 44, 29–39. doi: 10.1007/s10886-017-0909-x
Silva, D. B., Weldegergis, B. T., van Loon, J. J. A., and Bueno, V. H. P. (2017). Qualitative and quantitative differences in herbivore-induced plant volatile blends from tomato plants infested by either Tuta absoluta or Bemisia tabaci. J. Chem. Ecol. 43, 53–65. doi: 10.1007/s10886-016-0807-7
Simpson, M., Gurr, G. M., Simmons, A. T., Wratten, S. D., James, D. G., Leeson, G., et al. (2011). Attract and reward: combining chemical ecology and habitat manipulation to enhance biological control in field crops. J. Appl. Ecol. 48, 580–590. doi: 10.1111/j.1365-2664.2010.01946.x
Smid, H. M., and Vet, L. E. M. (2016). The complexity of learning, memory and neural processes in an evolutionary ecological context. Cur. Opin. Insect Sci. 15, 61–69. doi: 10.1016/j.cois.2016.03.008
Starr, G., Petersen, M. A., Jespersen, B. M., and Hansen, A. S. (2015). Variation of volatile compounds among wheat varieties and landraces. Food Chem. 174, 527–237. doi: 10.1016/j.foodchem.2014.11.077
Stelinski, L. L., Willett, D., Rivera, M. J., and Ali, J. G. (2019). “Tuning” communication among four trophic levels of the root biome to facilitate biological control. Biol. Control 131, 49–53. doi: 10.1016/j.biocontrol.2019.01.006
Sugawara, Y., and Ueno, T. (2020). Field evaluation of CLB as an attractant for beneficial parasitoid wasps attacking mealybug pests. J. Entomol. Zool. Stud. 8, 192–196. doi: 10.22271/j.ento
Svensson, G. P., Larsson, M. C., and Hedin, J. (2004). Attraction of the larval predator Elater ferrugineus to the sex-pheromone of its prey, Osmoderma eremita, and its implication for conservation biology. J. Chem. Ecol. 30, 353–363. doi: 10.1023/B:JOEC.0000017982.51642.8c
Symonds, M. R. E., and Gitau-Clarke, C. W. (2016). “The evolution of aggregation pheromone diversity in bark beetles,” in Advances in Insect Physiology, eds C. Tittiger and G. J. Blomquist (Amsterdam: Elsevier Ltd.), 195–234. doi: 10.1016/bs.aiip.2015.12.003
Szendrei, Z., and Rodriguez-Saona, C. (2010). A meta-analysis of insect pest behavioral manipulation with plant volatiles. Entomol. Exp. Appl. 134, 201–210. doi: 10.1111/j.1570-7458.2009.00954.x
Takabayashi, J., Sabelis, M. W., Janssen, A., Shiojiri, K., and van Wijk, M. (2006). Can plants betray the presence of multiple herbivore species to predators and parasitoids? The role of learning in phytochemical information networks. Ecol. Res. 21, 3–8. doi: 10.1007/s11284-005-0129-7
Thomas-Danguin, T., Sinding, C., Romagny, S., Mountassir, F. E., Atanasova, B., Le Berre, E., et al. (2014). The perception of odor objects in everyday life: a review on the processing of odor mixtures. Front. Physiol. 5:504. doi: 10.3389/fpsyg.2014.00504
Thompson, A. C., Baker, D. N., Gueldner, R. C., and Hedin, P. A. (1971). Identification and quantitative analysis of the volatile substances emitted by maturing cotton in the field. Plant Physiol. 48, 50–52. doi: 10.1104/pp.48.1.50
Tibor, B., Gols, R., Hemerik, L., van Lenteren, J. C., and Vet, L. E. M. (2007). Time allocation of a parasitoid foraging in heterogeneous vegetation: implications for host–parasitoid interactions. J. Anim. Ecol. 76, 845–853. doi: 10.1111/j.1365-2656.2007.01259.x
Tittiger, C., and Blomquist, G. J. (2016). Pheromone production in pine bark beetles. Adv. Insect Physiol. 50, 235–263. doi: 10.1016/bs.aiip.2016.02.002
Tóth, M., Bozsik, A., Szentkirályi, F., Letardi, A., Tabilio, M. R., Verdinelli, M., et al. (2006). Phenylacetaldehyde: a chemical attractant for common green lacewings (Chrysoperla carnea s.l., Neuroptera: Chrysopidae). Eur. J. Entomol. 103, 267–271. doi: 10.14411/eje.2006.033
Tóth, M., Szentkirályi, F., Vuts, J., Letardi, A., Tabilio, R. M., Jaastad, G., et al. (2009). Optimization of a phenylacetaldehyde-based attractant for common green optimization of a phenylacetaldehyde-based attractant for common green lacewings (Chrysoperla carnea, s.l.). J. Chem. Ecol. 35, 449–458. doi: 10.1007/s10886-009-9614-8
Turlings, T. C. J., and Erb, M. (2018). Tritrophic interactions mediated by herbivore-induced plant volatiles: mechanisms, ecological relevance, and application potential. Annu. Rev. Entomol. 63, 433–452. doi: 10.1146/annurev-ento-020117-043507
Turlings, T. C. J., Wackers, F. L., Vet, L. E. M., Lewis, W. J., and Tumlinson, J. H. (1993). “Learning of host-finding cues by hymenopterous parasitoids,” in Insect Learning, ed D. R. Papaj (Dordrecht: Springer Science), 51–78. doi: 10.1007/978-1-4615-2814-2_3
Uehara, T., Maeda, T., Shimoda, M., Fujiwara-Tsujii, N., and Yasui, H. (2019). Identification and characterization of the pheromones in the minute pirate bug Orius sauteri (Heteroptera: Anthocoridae). J. Chem. Ecol. 45, 811–817. doi: 10.1007/s10886-019-01104-1
Vaello, T., Casas, J. L., Pineda, A., De Alfonso, I., and Marcos-García, M. Á. (2017). Olfactory response of the predatory bug Orius laevigatus (Hemiptera: Anthocoridae) to the aggregation pheromone of its prey, Frankliniella occidentalis (Thysanoptera: Thripidae). Environ. Entomol. 46, 1115–1119. doi: 10.1093/ee/nvx141
van Lenteren, J. C. (2000). “Success in biological control of arthropods by augmentation of natural enemies,” in Biological Control: Measures of Success, eds G. Gurr and S. Wratten (Dordrecht: Kluwer Academic Publishers), 77–103. doi: 10.1007/978-94-011-4014-0_3
van Wijk, M., De Bruijn, P. J. A., and Sabelis, M. W. (2011). Complex odor from plants under attack : herbivore's enemies react to the whole, not its parts. PLoS ONE 6:e21742. doi: 10.1371/journal.pone.0021742
Vet, L. E. M., and Dicke, M. (1992). Ecology of infochemical use by natural enemies in a tritrophic context. Annu. Rev. Entomol. 37, 141–172. doi: 10.1146/annurev.en.37.010192.001041
Vet, L. E. M., Lewis, W. J., and Carde, R. T. (1995). “Parasitoid foraging and learning,” in Chemical Ecology of Insect 2, ed R. T. Carde (New York, NY: Springer), 65–101. doi: 10.1007/978-1-4615-1765-8_3
Vet, L. E. M., and Papaj, D. R. (1992). Effects of experience on parasitoid movement in odour plumes. Physiol. Entomol. 17, 90–96. doi: 10.1111/j.1365-3032.1992.tb00994.x
Vidal, M. C., and Murphy, S. M. (2018). Bottom-up vs. top-down effects on terrestrial insect herbivores: a meta-analysis. Ecol. Lett. 21, 138–150. doi: 10.1111/ele.12874
Vinson, S. B. (1998). The general host selection behavior of parasitoid hymenoptera and a comparison of initial strategies utilized by larvaphagous and oophagous species. Biol. Control 11, 79–96. doi: 10.1006/bcon.1997.0601
von Mérey, G., Veyrat, N., Mahuku, G., Valdez, L. R., Turlings, T. C. J., and D'Alessandro, M. (2011). Dispensing synthetic green leaf volatiles in maize fields increases the release of sesquiterpenes by the plants, but has little effect on the attraction of pest and beneficial insects. Phytochemistry 72, 1838–1847. doi: 10.1016/j.phytochem.2011.04.022
Vos, M., Berrocal, S. M., Karamaouna, F., Hemerik, L., and Vet, L. E. M. (2001). Plant-mediated indirect effects and the persistence of parasitoid-herbiore communities. Ecol. Lett. 4, 38–45. doi: 10.1046/j.1461-0248.2001.00191.x
Vosteen, I., Weisser, W. W., and Kunert, G. (2016). Is there any evidence that aphid alarm pheromones work as prey and host finding kairomones for natural enemies? Ecol. Entomol. 41, 1–12. doi: 10.1111/een.12271
Walling, L. L. (2008). Avoiding effective defenses: strategies employed by phloem-feeding insects. Plant Physiol. 146, 859–866. doi: 10.1104/pp.107.113142
Wang, G., Cui, L. L., Dong, J., Francis, F., Liu, Y., and Tooker, J. (2011). Combining intercropping with semiochemical releases: optimization of alternative control of Sitobion avenae in wheat crops in China. Entomol. Exp. Appl. 140, 189–195. doi: 10.1111/j.1570-7458.2011.01150.x
Watanabe, H., Yano, E., Higashida, K., Hasegawa, S., and Ozawa, R. (2016). An attractant of the aphidophagous gall midge Aphidoletes aphidimyza from honeydew of Aphis gossypii. J. Chem. Ecol. 42, 149–155. doi: 10.1007/s10886-016-0666-2
Wei, J., Kang, L., Wei, J., and Kang, L. (2011). Roles of (Z)-3-hexenol in plant-insect interactions. Plant Signal. Behav. 6, 369–371. doi: 10.4161/psb.6.3.14452
Wei, J.-R., Lu, X.-P., and Jiang, L. (2013). Monoterpenes from larval frass of two cerambycids as chemical cues for a parasitoid, Dastarcus helophoroides. J. Insect Sci. 13:59. doi: 10.1673/031.013.5901
Wei, J.-R., Yang, Z.-Q., Hao, H.-L., and Du, J.-W. (2008). (R)-(+)-Limonene, kairomone for Dastarcus helophoroides, a natural enemy of longhorned beetles. Agric. For. Entomol. 10, 323–330. doi: 10.1111/j.1461-9563.2008.00384.x
Weiss, M. R. (1997). Innate colour preferences and flexible colour learning in the pipevine swallowtail. Anim. Behav. 53, 1043–1052. doi: 10.1006/anbe.1996.0357
Williams, I. I. I. L, Rodriguez-Saona, C., Castle, S. C., and Zhu, S.u. (2008). EAG-active herbivore-induced plant volatiles modify behavioral responses and host attack by an egg parasitoid. J. Chem. Ecol. 34, 1190–1201. doi: 10.1007/s10886-008-9520-5
Wootton, J. T. (1994). The nature and consequences of indirect effects in ecological communities. Annu. Rev. Ecol. Syst. 25, 443–466. doi: 10.1146/annurev.es.25.110194.002303
Wu, H., Coudron, T. A., Zhang, L., Aldrich, J. R., Xu, W., Xu, J., et al. (2019). Identification and field verification of aggregation-sex pheromone from the predaceous bug, Arma chinensis. Chemoecology 29, 235–245. doi: 10.1007/s00049-019-00292-2
Xiu, C., Dai, W., Pan, H., Zhang, W., Luo, S., Wyckhuys, K. A. G., et al. (2019c). Herbivore-induced plant volatiles enhance field-level parasitism of the mirid bug Apolygus lucorum. Biol. Control 135, 41–47. doi: 10.1016/j.biocontrol.2019.05.004
Xiu, C., Xu, B., Pan, H., Zhang, W., Yan, Y., and Lu, Y. (2019b). Volatiles from Sophora japonica flowers attract Harmonia axyridis adults (Coleoptera: Coccinellidae). J. Integ. Agric. 18, 873–883. doi: 10.1016/S2095-3119(18)61927-6
Xiu, C., Zhang, W., Xu, B., Wyckhuys, K. A. G., Cai, X., and Lu, Y. (2019a). Volatiles from aphid-infested plants attract adults of the multicolored asian lady beetle Harmonia axyridis. Biol. Control 129, 1–11. doi: 10.1016/j.biocontrol.2018.11.008
Xu, Q., Hatt, S., Lopes, T., Zhang, Y., Bodson, B., Chen, J., et al. (2017a). A push – pull strategy to control aphids combines intercropping with semiochemical releases. J. Pest Sci. 91, 93–103 doi: 10.1007/s10340-017-0888-2
Xu, X., Cai, X., Bian, L., Luo, Z., Li, Z., and Chen, Z. (2017b). Does background odor in tea gardens mask attractants? Screening and application of attractants for Empoasca onukii matsuda. J. Econ. Entomol. 110, 2357–2363. doi: 10.1093/jee/tox269
Yang, J.-N., Wei, J.-N., and Kang, L. (in press). Feeding of pea leafminer larvae simultaneously activates jasmonic salicylic acid pathways in plants to release a terpenoid for indirect defense. Insect Sci. 1–43. doi: 10.1111/1744-7917.12820
Ye, L., Yang, C., Li, W., Hao, J., Sun, M., Zhang, J., et al. (2017). Evaluation of volatile compounds from chinese dwarf cherry (Cerasus humilis (Bge.) Sok.) germplasms by headspace solid-phase microextraction and gas chromatography–mass spectrometry. Food Chem. 217, 389–397. doi: 10.1016/j.foodchem.2016.08.122
Yoon, C., Seo, D.-K., Yang, J.-O., Kang, S.-H., and Kim, G.-H. (2010). Attraction of the predator, harmonia axyridis (Coleoptera: Coccinellidae), to its prey, Myzus persicae (Hemiptera: Aphididae), feeding on chinese cabbage. J. Asia-Pac. Entomol. 13, 255–260. doi: 10.1016/j.aspen.2010.06.009
Yu, H., Khashaveh, A., Li, Y., Li, X., and Zhang, Y. (2017). Field trapping of predaceous insects with synthetic herbivore-induced plant volatiles in cotton fields. Environ. Entomol. 47, 114–120. doi: 10.1093/ee/nvx201
Yu, H., Zhang, Y., Wu, K., Gao, X. W., and Guo, Y. Y. (2008). Field-testing of synthetic herbivore-induced plant volatiles as attractants for beneficial insects. Chem. Ecol. 37, 1410–1415. doi: 10.1603/0046-225X-37.6.1410
Zhang, P.-J., Li, W.-D., Huang, F., Zhang, J.-M., Xu, F.-C., and Lu, Y.-B. (2013). Feeding by whiteflies suppresses downstream jasmonic acid signaling by eliciting salicylic acid aignaling. J. Chem. Ecol. 39, 612–619. doi: 10.1007/s10886-013-0283-2
Zhu, J., and Park, K. C. (2005). Methyl salicylate, a soybean aphid-induced plant volatile attractive to the predator Coccinella septempunctata. J. Chem. Ecol. 31, 1733–1746. doi: 10.1007/s10886-005-5923-8
Zhu, P., Lu, Z., Heong, K., Chen, G., Zheng, X., Xu, H., et al. (2014). Selection of nectar plants for use in ecological engineering to promote biological control of rice pests by the predatory bug, Cyrtorhinus lividipennis (Heteroptera: Miridae). PLoS ONE 9:e108669. doi: 10.1371/journal.pone.0108669
Keywords: field application, attractant blend, HIPVs, pheromones, semiochemicals, crop yield, olfactory learning
Citation: Ayelo PM, Pirk CWW, Yusuf AA, Chailleux A, Mohamed SA and Deletre E (2021) Exploring the Kairomone-Based Foraging Behaviour of Natural Enemies to Enhance Biological Control: A Review. Front. Ecol. Evol. 9:641974. doi: 10.3389/fevo.2021.641974
Received: 15 December 2020; Accepted: 22 February 2021;
Published: 22 April 2021.
Edited by:
Anne Marie Cortesero, University of Rennes 1, FranceReviewed by:
Cesar Rodriguez-Saona, Rutgers, The State University of New Jersey, United StatesMaria Carolina Blassioli Moraes, Brazilian Agricultural Research Corporation (EMBRAPA), Brazil
Copyright © 2021 Ayelo, Pirk, Yusuf, Chailleux, Mohamed and Deletre. This is an open-access article distributed under the terms of the Creative Commons Attribution License (CC BY). The use, distribution or reproduction in other forums is permitted, provided the original author(s) and the copyright owner(s) are credited and that the original publication in this journal is cited, in accordance with accepted academic practice. No use, distribution or reproduction is permitted which does not comply with these terms.
*Correspondence: Emilie Deletre, emilie.deletre@cirad.fr
†ORCID: Pascal M. Ayelo orcid.org/0000-0002-0027-2546
Christian W. W. Pirk orcid.org/0000-0001-6821-7044
Abdullahi A. Yusuf orcid.org/0000-0002-8625-6490
Anaïs Chailleux orcid.org/0000-0001-5653-8019
Samira A. Mohamed orcid.org/0000-0001-6836-7528