- 1Applied Ecology and Conservation Lab, Programa de Pós-Graduação em Ecologia e Conservação da Biodiversidade, Universidade Estadual de Santa Cruz, Ilhéus, Brazil
- 2Department of Botany, Federal University of Pernambuco, Recife, Brazil
- 3Department of Biodiversity, São Paulo State University, Rio Claro, Brazil
Global biodiversity is threatened by land-use changes through human activities. This is mainly due to the conversion of continuous forests into forest fragments surrounded by anthropogenic matrices. In general, sensitive species are lost while species adapted to disturbances succeed in altered environments. However, whether the interactions performed by the persisting species are also modified, and how it scales up to the network level throughout the landscape are virtually unknown in most tropical hotspots of biodiversity. Here we evaluated how landscape predictors (forest cover, total core area, edge density, inter-patch isolation) and local characteristics (fruit availability, vegetation complexity) affected understory birds seed-dispersal networks in 19 forest fragments along the hyperdiverse but highly depauperate northeast distribution of the Brazilian Atlantic Forest. Also, our sampled sites were distributed in two regions with contrasting land cover changes. We used mist nets to obtain samples of understory bird food contents to identify the plant species consumed and dispersed by them. We estimated network complexity on the basis of the number of interactions, links per species, interaction evenness, and modularity. Our findings showed that the number of interactions increased with the amount of forest cover, and it was significantly lower in the more deforested region. None of the other evaluated parameters were affected by any other landscape or local predictors. We also observed a lack of significant network structure compared to null models, which we attribute to a pervasive impoverishment of bird and plant communities in these highly modified landscapes. Our results demonstrate the importance of forest cover not only to maintain species diversity but also their respective mutualistic relationships, which are the bases for ecosystem functionality, forest regeneration and the provision of ecological services.
Introduction
Over the last few centuries, forest ecosystems have been converted into small patches by habitat loss and fragmentation promoted by human activities (Laurance et al., 2014; Haddad et al., 2015). In this very Anthropocene scenario, land-use intensification can affect ecological processes both at the landscape and local-scale (Tscharntke et al., 2005). The decreasing amount of forest cover at the landscape scale as a result of deforestation and habitat conversion is a powerful predictor of species loss in many tropical forests (Dudley, 2005; Fahrig, 2013). Increasing fragmentation and inter-patch isolation decrease the amount of suitable habitat for wild species, affecting their dispersal abilities and movement capacity throughout the landscape (Newbold et al., 2015). Also, changes in local abiotic conditions (e.g., increasing light incidence and temperature, decreasing humidity) along a gradient from the edge to the forest interior are commonly observed at the local scale in fragmented landscapes (Murcia, 1995; Laurance et al., 2007). Therefore, the synergy between processes operating at both the landscape and the local scales may result in a marked change in species composition in those forest fragments, in which the loss of sensitive species might be compensated by the proliferation of species adapted to anthropogenic habitats (Supp and Ernest, 2014; Morante-Filho et al., 2015). However, how the interplay between landscape and local processes affects the ecological interactions between the persisting animal and plant species remains poorly understood.
Habitat loss and fragmentation strongly determine the trajectory of the local forest structure (Arroyo-Rodríguez et al., 2017), leading to a retrogressive succession process toward the homogenization of the vegetation and the predominance of pioneer plant species (Lôbo et al., 2011; Thier and Wesenberg, 2016). In the long-term, it leads to the functional erosion of plant traits related to reproduction that provide food resources for animals (Marcilio-Silva et al., 2016; Rocha-Santos et al., 2020). The loss of functional diversity of plant community might influence the availability and variability of floral, fruit, and seed resources for the associated fauna, thus increasing their vulnerability to future disturbances (Magnago et al., 2014; Pessoa et al., 2017a,b; Lim et al., 2020; Rocha-Santos et al., 2020). Specifically, in forest fragments embedded in highly deforested landscapes, the vegetation homogenization triggers a decrease in fruit biomass production and fruit quality (Pessoa et al., 2017a,b), possibly disrupting animal-plant interactions fundamental for the provision of ecological services (e.g., pollination and seed dispersal) (Mitchell et al., 2015; Valiente-Banuet et al., 2015).
Frugivorous birds are particularly sensitive to changes in local vegetation structure in forest fragments inserted in highly disturbed landscapes (Galetti et al., 2013; Morante-Filho et al., 2018). Larger frugivorous birds are the first to vanish when the small forest fragments do not support the number of fleshy fruits needed to sustain their populations (Donoso et al., 2017; Bomfim et al., 2018; Emer et al., 2018). Also, some forest-dweller frugivorous bird species may not be able to move among forest fragments (Pizo and dos Santos, 2011) and inhabit exclusively the forest interior away from the influence of edges and open areas (McCollin, 1998). Among those birds are the ones that live in the lower forest strata like the understory (Visco et al., 2015; Bradfer-Lawrence et al., 2018). On the contrary, in the remaining small forest fragments small to medium-sized frugivores/omnivores and edge-specialists bird species dominated. Typically, these birds are able to fly relatively long distances looking for food in multiple forest fragments in order to meet their daily metabolic requirements (Lees and Peres, 2009). Therefore, the structure of the understory forest strata is an important component to sustain understory bird species with direct consequences to the interactions they perform and the ecosystem functioning (García et al., 2018).
Ecological networks have been proven to be an efficient tool to unveil how natural and human-driven environmental changes affect the structure, dynamics, and the stability of ecosystems (Memmott, 2009; Hagen et al., 2012; Guimarães, 2020). For instance, in more conserved landscapes, habitat and/or diet specialist species are able to succeed and interact with each other, forming subgroups of cohesive interacting partners that can be depicted by analyzing network modularity (Schleuning et al., 2014). In contrast, species homogenization in more disturbed landscapes can concentrate interactions within some dominant species in the system, reducing interaction evenness (Rodewald et al., 2014). Similarly, the loss of species or decreasing species abundance in smaller forest fragments can affect the number of links per species by reducing the number of partners available for the interactions to occur (Muñoz et al., 2017; Emer et al., 2020).
Here, we combine network and landscape ecology to evaluate the interplay between landscape and local scale processes on understory bird seed-dispersal networks on a hyperdiverse yet highly disturbed tropical forest. We studied 19 forest sites embedded in two regions with contrasting patterns of land use in the still poorly studied northeast of the Brazilian Atlantic Forest. Thus, for each site, we evaluated: (1) the total number of interactions between frugivorous birds and plant species; (2) the mean number of links per species; (3) interaction evenness; and (4) modularity. Given that our forest fragments present simplified understory frugivorous bird assemblages (Morante-Filho et al., 2015, 2018) and low functional diversity, quality, and biomass of fruits (Pessoa et al., 2017a,b), we expect to record bird seed-dispersal networks of exceedingly small size and reduced number of interactions, especially in deforested landscapes, in which the emergent structural properties may be compromised by the loss of both interacting partners and the interactions they perform. Additionally, as our study assessed a subset of the frugivorous bird assembly, i.e., mainly the understory species, and used a specific method to quantify the interactions, we believe that these particularities could contribute to limited-sized networks. At the landscape scale, we predicted a decrease in the number of interactions and on the mean number of links per species as forest cover decreases, and edge densities and isolation increase. In this scenario, modularity is expected to decrease due to the loss of specialist bird-plant interactions and the persistence of a few generalist bird species (i.e., species adapted to use different food resources and occupy environments with distinct disturbance intensities) able to establish links with many species and hence connecting the whole network (Blake and Loiselle, 2002; Emer et al., 2018). Thus, the interactions might be concentrated within a few dominant bird species in the system, consequently decreasing network evenness in more deforested landscapes. Likewise, at the local scale we expect that the lower fruit availability associated with changes in forest structure will reduce the number of interactions, the mean number of links per species, interaction evenness and modularity due to the predominance of generalist bird species able to use the wherever resource available.
Materials and Methods
Study Area
The study region is located in the southern Bahia State, northeast region of Brazil (Figure 1), dominated by Atlantic Forest, a biome considered a biodiversity hotspot due to its high levels of endemism and deforestation (Myers et al., 2000). The region is composed of different agricultural systems and land uses, like cocoa (Theobroma cacao), rubber trees (Hevea brasiliensis) and Eucalyptus plantations, as well as cattle pasture areas (Pardini et al., 2009). Forests in southern Bahia hold a higher level of species richness and endemism, such as birds, plants and butterflies, when compared to other regions of Atlantic Forest (Cardoso da Silva et al., 2004; Faria et al., 2007). However, deforestation in the southern Bahia has reduced the diversity of birds and plants (Morante-Filho et al., 2015; Benchimol et al., 2017; Rocha-Santos et al., 2017).
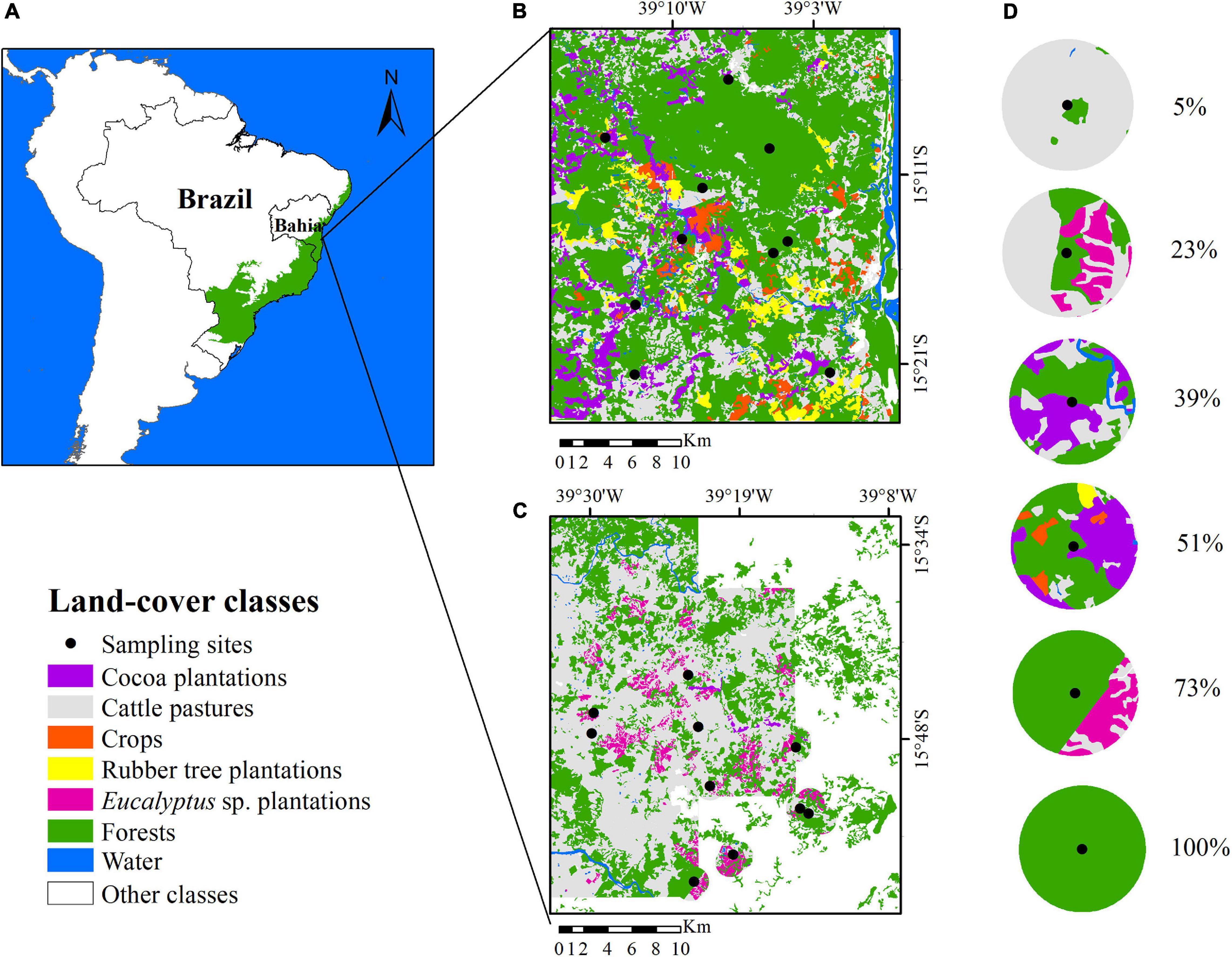
Figure 1. Study area in Atlantic Forest in Southern Bahia, northeastern Brazil (A). We show the location of the sampling sites (black dots) and land cover classes in the high forest cover (HFC) region (B), with 50% of remaining forest cover, and in the low forest cover (LFC) region (C), with 30% of remaining forest cover. In (D) are exhibited six landscapes embedded within a wide range of landscape native forest cover and different land-cover classes.
In this mosaic of remnant forest fragments immersed in human-modified landscapes, we randomly selected 20 forest sites (< 500 m a.s.l.), embedded within a gradient of forest cover (Figure 1). The sampled sites present similar soil, topography, and floristic characteristics; however, the northern region harbors a high forest cover (HFC, hereafter), 50% of which corresponds to native old-growth and secondary forest (Morante-Filho et al., 2016). The landscape matrix in the HFC region is highly heterogeneous but is dominated by shade cacao plantations (22% of the landscape matrix), and rubber trees (10%) (Figure 1B). In contrast, the southern region has a low forest cover (LFC, hereafter), represented by only 30% of forest, with a homogenous matrix predominantly of cattle pastures (86% to the landscape matrix) and Eucalyptus sp. plantations (7%) (Figure 1C). The regional climate, according to the classification of Köppen, is Af type, hot and humid without a well-defined dry season (Gouvêa, 1969). The annual averages of temperature and precipitation are 24°C and 2,000 mm/year, respectively, classified as poorly seasonal. These temperature and rainfall conditions distinguish tropical forests from seasonally dry tropical forests, as they do not show fruiting peaks in specific periods, although there is a less pronounced but significant intra-annual variation in the abundance of seed rain in our regions (Pessoa et al., 2017a; Piotto et al., 2019).
Landscape Scale—Composition and Configuration Metrics
We used high-resolution satellite images (Quick Bird and World View, 2011 and Rapid Eye, 2009–2010, with resolutions of 0.6, 0.5, and 5 m, respectively) to classified and digitized land uses through ArcGIS software (Environmental Systems Resource Institute (ESRI), 2012) at 1:10,000 scale, which is adequate for identifying patches based on the visual inspection of color, texture, shape, location, and context. We used the software FRAGSTATS 4.2 (McGarigal and Marks, 1995) to estimate landscape composition (the relative amount of different land use and cover types) and landscape configuration (the spatial arrangement of habitat and non-habitat remnants) within a specific area around each sampling site (Figure 1D). We estimated one metric of landscape composition: The percentage of remaining forest (forest cover, hereafter). We also evaluated three metrics related to landscape configuration: Cohesion index (%) that measures the physical connectedness among forest fragments, the total length of forest edge (edge density, hereafter) and total core area of forest. We considered a distance of 75 m from the edge to calculate the total core area because previous studies in the region found significant changes in forest structure (Faria et al., 2009) and in the species composition at this distance (Pardini et al., 2009). These metrics were chosen because they were previously indicated to influence richness and composition of bird and plant species in human-modified landscapes (Martensen et al., 2008; Zurita et al., 2012; Morante-Filho et al., 2018).
The effects of landscape variables on biodiversity depends on the spatial scale at which predictors are measured (Jackson and Fahrig, 2015). Thus, we calculated each landscape metric within 10 different-sized buffers from the center of each site, ranging from 100 to 1,000 m radius (Supplementary Appendix 1). We used Generalized Linear Models to identify the most appropriate landscape size (Supplementary Figure S1) to further analyze the effect of each landscape metric on several network metrics (more details below).
Local Scale—Vegetation Structure and Fruit Availability
We characterized the local vegetation given that a previous study in the exact same forest fragments have shown that vegetation structure and fruit availability are the main local drivers of the diversity of frugivorous birds (Morante-Filho et al., 2018). Accordingly, we estimated local variables by randomly establishing four plots (25 × 4 m) in each site, with a minimum inter-plots distance of 150 m. We recorded in each plot (1) all the woody plants in the understory (50–200 cm in height), and (2) all the trees above the understory layer (diameter at breast height DBH ≥ 5 cm), and (3) mean DBH per tree. We also estimated the (4) percentage of canopy openness within the plots using hemispherical photographs. The photographs were taken at 1.5 m from the soil and analyzed with Gap Light Analyzer software (Frazer et al., 1999). These four variables were summarized using a Principal Component Analysis (PCA), whose component explained 55.6% of the variation in vegetation structure and was therefore used as a proxy for vegetation complexity (Supplementary Appendix 2).
Further, we used five plots (25 × 4 m) located near the mist nets used for bird sampling (see section “Understory Seed-Dispersal Interactions”), totaling a sampling area of 0.05 ha per site, to estimate the (5) number of fruits available for birds during the sampling months. Hence the abundance of mature fruits was counted on plants between 0.5 and 2 m height, and in plants with height between 2 and 7 m, all the mature fruits of a branch were counted with the aid of an 8 × 42 binocular. Then, fruit number per individual was obtained through the multiplication of the total fruit number of focal branches by the number of branches with fruits per plant (Blake, 1990). Fruiting plants were collected using pruning shears and exsiccated for identification in the herbarium of the Executive Commission for the Planning of Cacao Cropland—CEPLAC, with the help of botanical specialists. The fruits were also collected and stored in alcohol (96%) for further comparison with fruits and seeds present in the samples of feces and food contents of birds.
Understory Seed-Dispersal Interactions
We used a zoocentric approach (sensu Jordano, 2016) to survey seed-dispersal interactions focusing on the understory birds that inhabit the interior of the forest fragments. Understory frugivorous birds are an important functional group as they contribute to the influx of pioneer seeded-plant species responsible for forest regeneration in early ecological succession (Gomes et al., 2011). Plus, recent studies in our forest sites have shown that this avian functional group is one of the most affected due to changes in landscape composition (e.g., forest loss), configuration (e.g., increase of forest edges) as well as by the local patterns of forest structure and fruit availability (Morante-Filho et al., 2018, 2020). Thus, to access the seed-dispersal interactions performed by understory birds we used mist nets—a robust sampling technique widely used to survey understory birds that enables capturing a wide range of species (including those rarely seen or heard) (Sutherland et al., 2004). Additionally, this method allows the analyses of droppings and stomach contents (Sutherland et al., 2004), allowing a more powerful characterization of seed dispersal through the in situ identification of the fruits and seeds consumed by each bird species (Costa et al., 2016).
We sampled bird assemblages from April 2017 to April 2018 over two field seasons to capture greater diversity and higher tree phenological variation. In each forest site, we opened 10 mist nets (12 m long, 2.5 m high, 31 mm mesh) to capture birds during three consecutive days per season. The mist nets were opened from 07:00 to 17:00 h and reviewed every 30 min to reduce the catching stress. We avoided sampling on rainy and windy days because such conditions reduce bird movement and may interfere in capture success. Each captured bird was temporarily marked on one of the right-wing primary feathers with non-toxic material to avoid recounting the same individual during sampling. In this study, we used a standardized effort of 600 net-hours at each site, totaling 12,000 net-hours nest by bird sampling (10 mist nets × 10 h × 3 days × 2 field seasons × 20 sites). This sampling effort is similar to other studies performed in the Brazilian tropical forest (e.g., Banks-Leite et al., 2010; Bueno and Peres, 2019). All birds were identified according to the scientific nomenclature of the South American Classification Committee (Remsen et al., 2019). We performed oral administration of tartar emetic (1.5% antimony and potassium tartrate solution); a technique widely used in ecological studies to induce birds to regurgitate the food content (Poulin et al., 1994). The solution was administered by drip through a 3 ml disposable needleless syringe using a dosage of 0.8 ml per 100 g of the corporal mass. After the solution administration, each bird was placed in a cloth bag for 15 min to regurgitate and recover from the process, thus reducing stress (Johnson et al., 2002). Recaptured birds during the same sampling period were not re-evaluated. Fecal samples were collected only when birds defecated in the mist net or inside the cloth bag. Fecal samples and regurgitated material were stored in alcohol (96%). Then, seeds and fruits present in food samples were analyzed in a laboratory through a stereoscopic magnifying glass to identify at the lower taxonomic level, using a seed bank of the Laboratory of Applied Ecology from the Universidade Estadual de Santa Cruz. In addition, we used information present in the literature and specific guides for the identification of plants, fruits, and seeds (Cestari and Pizo, 2013). The license for bird capture was granted by Comissão de Ética no Uso dos Animais (CEUA) of Universidade Estadual de Santa Cruz (UESC); Ministério do Meio Ambiente (MMA); Instituto Brasileiro do Meio Ambiente e dos Recursos Naturais Renováveis (IBAMA) and Sistema de Autorização e Informação em Biodiversidade (SISBIO); license number 53792–2, authentication code 67798558 and date of issue 06/11/2017.
Network Description
For each forest site, we built a quantitative interaction network defined by a weighted adjacency matrix Aij in which rows represent the i plant species present in the stomach and/or feces content of the j bird species, this represented in columns and including only birds captured with identifiable seeds or fruit parts in their food content. The aij elements of the matrix represent the frequency of interactions between i and j (i.e., the number of individual birds per species recorded consuming a given plant species). Because we observed relatively small networks due to the intrinsic characteristics of the study system, some widely used network metrics were not possible and/or meaningless to run such as nestedness and H’2 specialization. Therefore, we described our networks based on four complementary, non-correlated parameters as follow (Supplementary Appendix 3):
(i) total number of interactions, as the sum of all pairwise combinations of frugivorous birds and plant species in each sample site; (ii) number of links per species, as the mean number of interaction links of all species divided by the total number of species; (iii) interaction evenness, which indicates how equally distributed are the interaction links within the network. Values close to 0 indicate that the distribution of interactions is heterogeneous, here associated to the presence of super-generalist or dominant species in the system. In contrast, values close to 1 show higher homogeneity in the distribution of links, here associated to the lack of interaction-dominant species and the presence of specialist bird-plant associations (Bersier et al., 2002); (iv) modularity, which identifies cohesive sub-groups of species within the network that interact strongly among themselves, usually associated with trait matching, functional groups and/or habitat and diet requirements in ecological studies (Schleuning et al., 2014; Mello et al., 2015). We used the DIRTLPAwb algorithm (Beckett, 2016) that maximizes modularity and accounts for quantitative interaction frequencies.
Statistical Analyses
First, we assessed the sample completeness of the number of recorded interactions per fragment using Interaction Accumulation Curves (Supplementary Appendix 4) with distinct pairwise interactions as a function of the number of sampled days (Jordano, 2016). Then, we tested the correlation between all predictors using a Spearman’s test to avoid collinearity (r-values ≥ 0.70) within the same model (Supplementary Appendix 5). We used Generalized Linear Models to evaluate how network metrics are influenced by landscape and local predictors. Models including the number of interactions were constructed using Poisson distribution, while models’ using the number of links per species, interaction evenness and modularity were created using normal distribution. In addition, we included the study region as a fixed factor in the models, as previous studies have observed that contrasting land-use patterns in HFC and LFC regions affected bird and plant species (Morante-Filho et al., 2016; Rocha-Santos et al., 2017). Given that three out of four metrics of ecological networks were affected by network size (Supplementary Appendix 6), which varies greatly across forest sites, we used total bird and plant richness as an offset term in the subsequent analyses (Henriksen et al., 2019). An offset term is needed when there is a parameter that is known to have a proportional effect on the response variable. Therefore, by using an offset the coefficient of the models are maintained fixed rather than estimated, and adds a fixed value to the linear predictor, which tends to improve model fit (Zuur et al., 2009; Henriksen et al., 2019). This approach enables the assessment of how network metrics are influenced by environmental predictors, taking into account the variation in species richness.
All models were subjected to a model selection approach based on Akaike information criterion corrected for small samples (AICc), using lme4 and bbmle packages (Bolker, 2015; Bates et al., 2015). We considered the models equally plausible when the difference in AICc (ΔAICc) between models was less than 2. We also used the Akaike weight (ωAICc), which ranges from 0 to 1, and expresses the normalized relative likelihood of each model, to select the most plausible one (Anderson, 2008). Models that fitted this criterion are equally plausible to explain the observed patterns. Then, we evaluated the fit of the plausible models using a ratio between residual deviation and residual degrees of freedom, where values > 1 indicate overdispersion (McCullagh and Nelder, 1989). We corrected for overdispersion fitting a negative binomial distribution in the correspondent models. Furthermore, we evaluated the spatial autocorrelation in the residuals of the best models based on Moran’s I autocorrelation coefficient using the ape package (Paradis and Schliep, 2019).
Last, to test whether the observed network parameters differ from those expected by chance we used null models based on the Patefield algorithm (“method = 1” in the “null model” function) which randomizes the interactions among species while maintaining network size and marginal totals constant therefore constraining the frequency of rare species in the community (Dormann et al., 2009). We created 100 random networks for each studied site and used z-scores to test whether, and to which extent, the observed networks differ significantly from random expectations regarding the number of links per species, interaction evenness and modularity. Z-scores were then fitted as the response variable in the same statistical model structure described above. Network metrics and null models were estimated using the bipartite package (Dormann et al., 2009). All statistical analyses and graphs were carried out in R software version 3.5.3 (R Development Core Team, 2019).
Results
We captured 491 birds along 19 forest sites (mean = 25.84 birds ± SD = 15.91 per site), but we obtained samples of fruit or seed only from 185 birds (mean = 9.74 birds ± SD = 9.10 per site) belonging to 14 families and 28 species (Supplementary Appendices 7, 8), forming the interplay of interactions used to build the local network. Even after six days sampling and 600 net-hours we did not reach an asymptote in the number of interactions in several forest sites (see Supplementary Appendix 4), especially in those inserted in more forested landscapes. We failed to record a single interaction in the forest site with the lowest amount of forest cover (15%) because no birds were captured therein. In fact, the low number of captured birds with food content contributed to limited sampling sufficiency of interactions, given that no interaction was recorded for 62% of the birds caught in the mist nets (Supplementary Appendix 8). In contrast, sites located in LFC region showed reasonable sample completeness (Supplementary Appendix 4).
In terms of bird diversity, the families Pipridae (five species, n = 145 individuals), Dendrocolapitidae (four species, n = 6) and Thraupidae (four species, n = 4) were the most representative (Supplementary Appendix 7), showing the greater diversity of bird species reported consuming fruits. The most abundant bird species were Ceratopipra rubrocapilla (n = 52) and Dixiphia pipra (n = 51), followed by Machaeropterus regulus (n = 26), Manacus manacus (n = 15), and Schiffornis turdina (n = 6). Confirming our expectations, there was a significant difference in bird richness between regions (t = 5.0, df = 13.4, p < 0.001), in which we recorded 22 (mean = 5.2 ± SD = 1.69 per site) bird species in the HFC and 12 bird species (mean = 2.22 ± SD = 0.83 per site) in the LFC region. Among those, 16 bird species were recorded exclusively in the HFC region, six were exclusive from the LHC and the other six were common to both regions (Supplementary Appendix 7).
In turn, plant diversity was characterized by 30.837 seeds/fruits in the birds’ stomach content and feces, distributed in 68 morphospecies, of which 10 met the species level identification and another 13 the genus level. We observed a significant difference in the number of seed morphospecies between regions (t = 3.7, df = 11.2, p = 0.003), with 56 morphospecies in the HFC region (mean = 9.3 ± SD = 4.9 per site), and only 17 morphospecies in the LFC region (mean = 3.2 ± SD = 1.6 per site). Miconia hypoleuca was the only plant species found in all studied sites, being the most consumed by the majority of bird species (n = 22 species, 78.6% of the bird community) with a total of 28.734 seeds recorded, showing to be an important resource for the understory bird assemblages.
Such interplay of bird and plant diversity resulted in 268 quantitative interactions between frugivorous birds and fleshy-fruited plants, with an average of 14.11 ± 12.98 per forest site (Supplementary Appendix 8). The higher number of birds with food content, the higher number of interactions and therefore the larger-sized networks were observed in the more forested landscapes, mainly in the HFC region (Figure 2). Indeed, the number of interactions was the only network descriptor that responded to changes in both landscape and local predictors and that was mainly driven by region (Supplementary Appendix 9). Seven models were considered equally plausible to explain the relationship between local and landscape-scale predictors and the number of interactions (Table 1 and Supplementary Appendix 9). However, only two models, which consider the region and forest cover separately, were significant (Table 2 and Figure 3). Specifically, the number of interactions was only positively related to forest cover at the landscape scale (Figure 3A), with a higher number of interactions recorded in the HFC region (Figure 3B). Also, all models used to explain the variation in the number of interactions at forest sites showed a good fit (Supplementary Appendix 10) and did not show spatial autocorrelation (p > 0.05) (Supplementary Appendix 11). In contrast, the number of links per species, interaction evenness, and modularity were not affected by any landscape or local predictors evaluated in this study (Supplementary Appendix 9 and Supplementary Tables 6–8). Also, when network size was controlled for, the number of links per species, interaction evenness, and modularity did not differ from the null models (Supplementary Appendix 12), indicating the lack of significant structural properties in these simplified bird-plant networks.
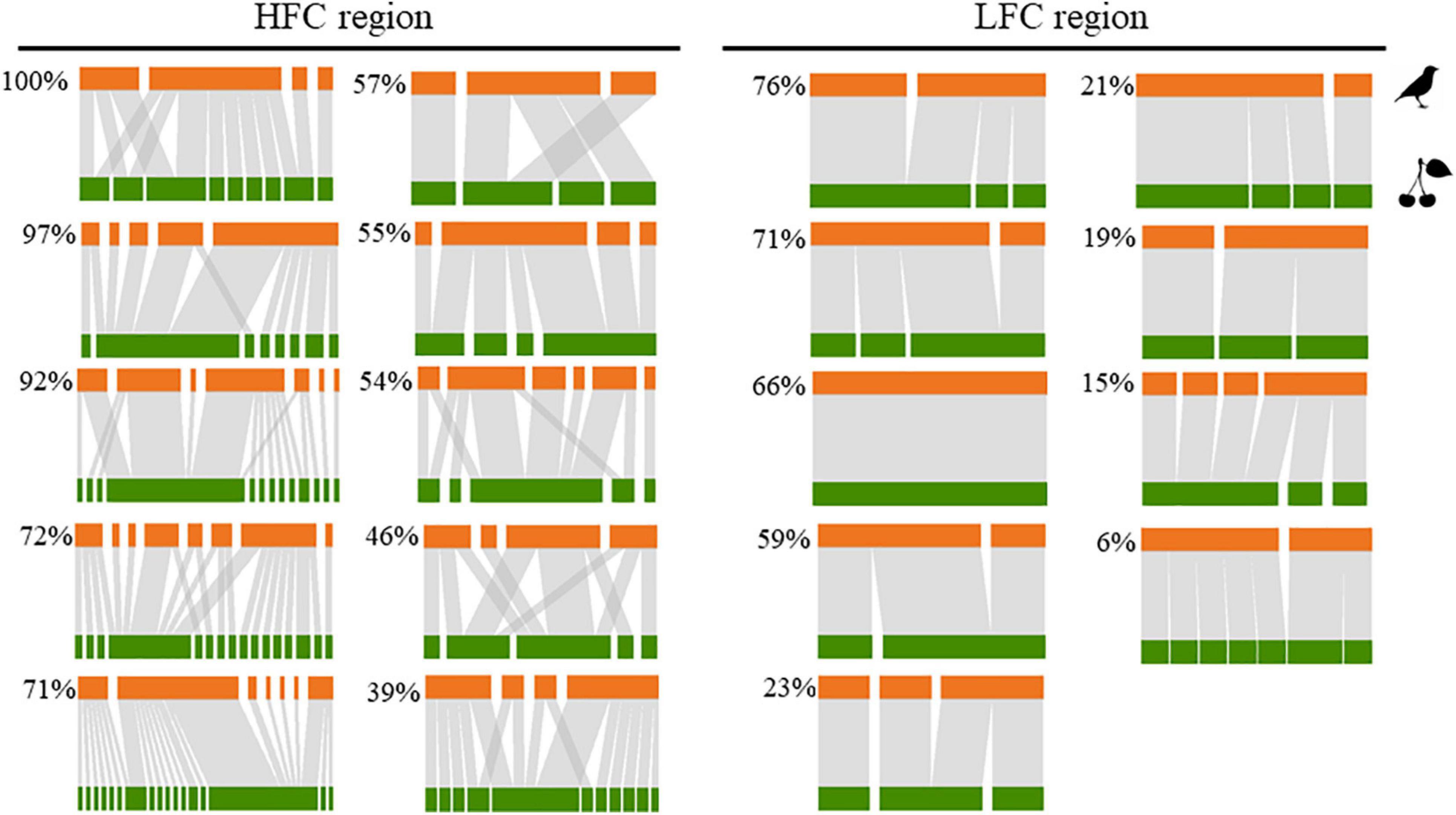
Figure 2. Bipartite seed-dispersal networks in 19 forest sites located in the Brazilian Atlantic forest. At the left, networks observed in the high forest cover (HFC) and at the right, networks observed in the low forest cover (LFC) region, and their respective values of forest cover (%). Orange bars represent bird species and green bars represent the plant species; the frequency of seed-dispersal interactions among bird and plant species are represented by the width of the vertical bars.
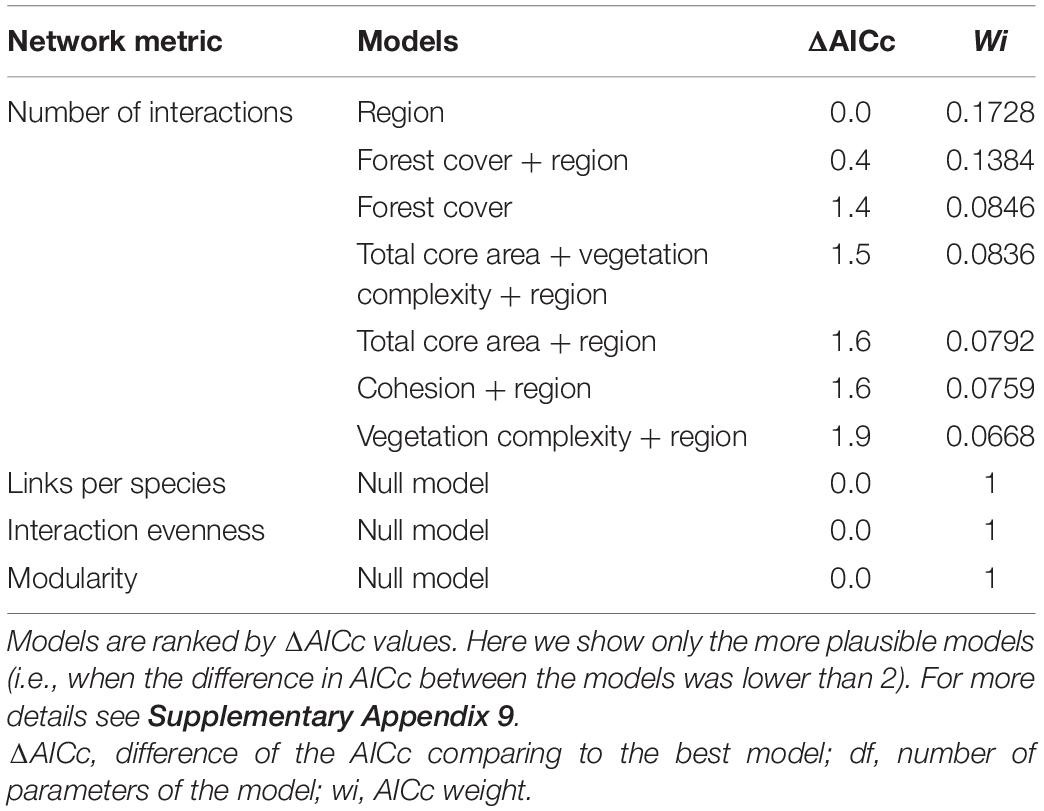
Table 1. Model selection parameters based on Akaike’s Information Criterion (AIC), used to explain the relationship between dependent variables (number of interactions, links per species, interaction evenness, and modularity) and environmental predictors (forest cover, total core area, edge density, cohesion, fruit availability, and vegetation complexity) recorded in 19 forest sites located in the Brazilian Atlantic Forest.
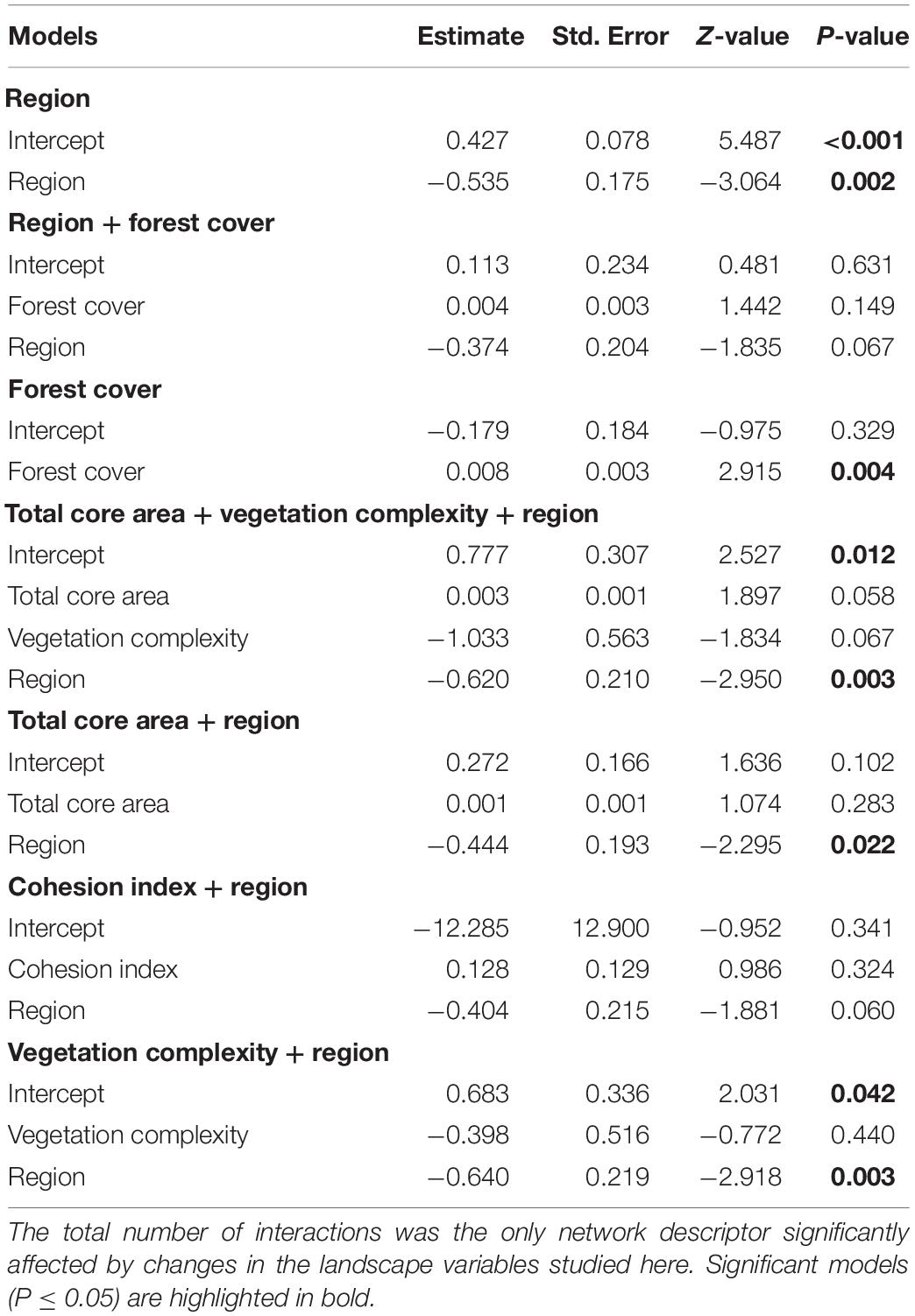
Table 2. Results from General Linear Models testing for the effects of local and landscape predictors on the number of interactions in 19 bird-plant networks.
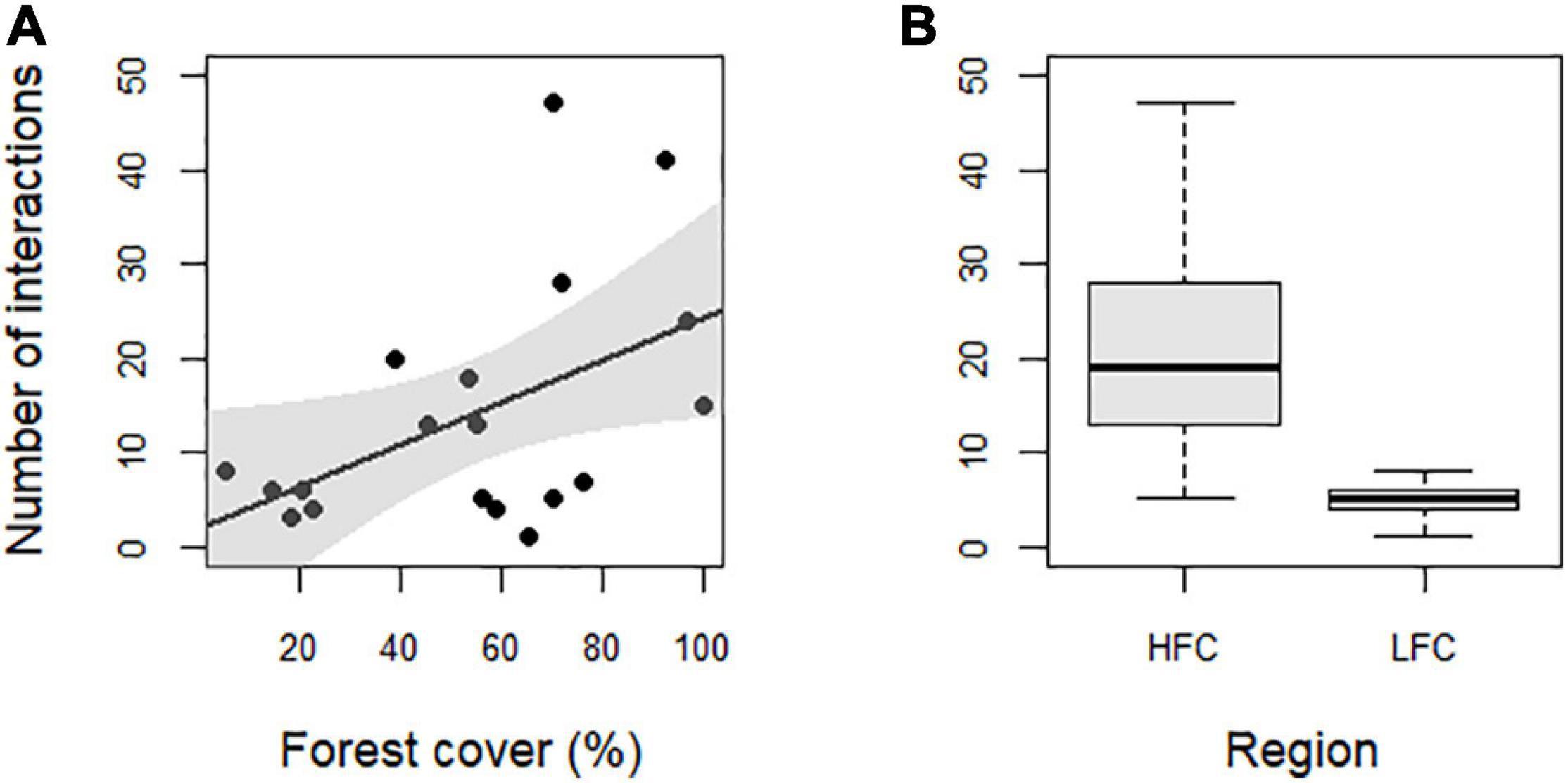
Figure 3. Relationship between the number of interactions in bird-plant networks and predictors variables. (A) The relationship between forest cover and the number of interactions. (B) The total number of interactions observed in high forest cover (HFC) and low forest cover (LFC) region.
Discussion
We showed that deforestation reduces the number of interactions between understory frugivorous birds and fleshy-fruit plant species at the landscape level but that does not change the structure of small-sized seed-dispersal networks in highly depauperate tropical forests. The observed decrease in the number of interactions reflects the decline or even the loss of bird population of specific species, as already detected in previous assessments (Morante-Filho et al., 2015, 2018). Despite such numeric decline in interactions, no significant network structural change was observed in both HFC and LFC regions, possibly because both regions encompass highly impoverished assemblages of understory bird species that have already lost part of its functionality due to defaunation (Canale et al., 2012). Importantly, our study provides further evidence about the pervasive effects of landscape-scale forest loss, not only for understory bird richness, but also for animal-plant interactions and probably the ecological services they provide.
Forest cover at the landscape scale was the best and only predictor explaining the decrease in species interactions. Previous studies have also reported the detrimental effects of forest loss on plant diversity, especially late-successional, shade-tolerant and zoochoric tree species (Benchimol et al., 2017; Rocha-Santos et al., 2017). Similarly, forest loss has been cited as the main factor driving frugivores loss in tropical forests (Coster et al., 2015; Morante-Filho et al., 2018). Indeed, forest loss can act as an important selective force, filtering frugivorous birds with specific phylogenetic and functional features, and thus interfering in the seed dispersal networks (Peña et al., 2020). In particular, recent studies reported significant changes in network structure (e.g., higher nestedness and lower modularity) after forest loss in Atlantic forest sites (Emer et al., 2020; Marjakangas et al., 2020) or changes in bird functions in deforested landscapes (Coster et al., 2015). Here, we found highly simplified seed-dispersal networks with only a few pairs of interacting species, whose structural properties have been depleted due to the loss of forest cover in these human-modified landscapes.
Our results also showed that the number of interactions depends on the regional context in which forest fragments are inserted, with a higher number of interactions recorded in the region with higher amounts of forest cover. In our study, HFC region is composed by more forested landscapes, including native remnants immersed in a biodiversity-friendly matrix such as the cocoa agroforestry systems. Altogether, these features allow a greater diversity of birds and plants in forest fragments (Faria et al., 2006; Sambuichi et al., 2012) and hence a higher number of interactions. In contrast, the LFC region is composed of small forest fragments that present declines in their regional diversity (Morante-Filho et al., 2016). Additionally, we also observed a marked change in bird and plant species composition between LFC and HFC regions (Supplementary Appendix 13). From the total interactions observed (n = 268), only 16% were recorded in the LFC region, occurring among a limited number of bird and plant species (Supplementary Table 1). In particular, many captured bird species in the LFC region are occasional frugivores, which may help to explain the extremely low number of interactions recorded. Conversely, in the HFC region we observed a more diversified bird assembly, including large frugivores such as Ramphastos vitellinius (Supplementary Appendix 7). This frugivorous bird can facilitate seed dispersal, including large-sized seed species, among forest fragments due to their high vagility. Accordingly, the absence of certain bird species can limit seed dispersal and the recruitment rates of plant species, compromising the vegetation regeneration of small and severely disturbed forest remnants (Gomes et al., 2011; Donoso et al., 2017; García et al., 2018).
Changes in composition and structure of the local vegetation, as well as fruit availability, are known to shape the diversity of frugivores (García et al., 2011; Plein et al., 2013). Previous studies in the same landscapes studied here have found that deforestation leads to both a simplification in the forest structure and a decrease in fruit biomass (Rocha-Santos et al., 2016, 2017; Pessoa et al., 2017b). Nevertheless, we found no evidence that local changes in fruit availability or vegetation complexity affected bird-plant networks, possibly because the most representative bird family recorded (Pipridae) was abundant in all sites. Despite the decrease in abundance or even the loss of bird species such as C. rubrocapilla, D. pipra, and M. regulus, the abundance of other species such as M. manacus increased in more disturbed sites. Indeed, M. manacus was the only member of this family recorded in forest sites located in landscapes with less than 20% forest cover (Supplementary Appendix 7). All the above-mentioned bird species share similar ecological features, i.e., they are forest-dweller frugivorous species that forage in the understory stratum (Supplementary Appendix 7). Thus, we suggest that functional redundancy might explain the results observed here.
Interestingly, none of the evaluated network descriptors was affected by any local or landscape variables assessed here. The lack of significant differences in the number of links per species across landscapes might be explained by the fact that the most captured birds (∼78%) belonged to the same family—Pipridae—which were recorded consuming only fruits of a few pioneer plant species in our fragments. Typically, the predominance of these species is well reported given that they are the dominant frugivorous birds in the forest understory and commonly captured in mist nets (Loiselle et al., 2007). Similarly, we also did not observe changes in interaction evenness, which were mostly high thus indicating the predominance of homogeneity in the distribution of interactions between birds and plants. This result, associated with the small size of the networks, could reflect the low fruit productivity in these forest fragments (Pessoa et al., 2017a). In this situation, the already depauperated bird assemblages (Morante-Filho et al., 2015) would heavily rely on the very few diversity of resources available, which are mainly provided by the pioneer species (e.g., Miconia hypoleuca, Miconia mirabilis, and Leandra rufescens) commonly observed in these bird diet. Indeed, these three plant species corresponded to 97% of the seeds observed in our interaction networks and were recorded in almost all forest sites, regardless of environmental differences in local or landscape-scale here evaluated. This is an alarming result because the reduced richness of frugivorous birds in the forest understory can lead to plant species homogenization via decrease in seed dispersal. In this case, seed dispersal is occurring only for a limited number of plant species, and other plant species that have lost their interaction partners can be locally extinct in the long term (Loiselle et al., 2007).
The lack of significant changes on network modularity at both the regional and local landscape scale indicates that the impoverished understory assemblage of plants and frugivorous birds do not form distinguished subgroups of interacting partners according to any specific trait, ecological niche and/or spatial segregation in these human-modified tropical forests. In our study, the seed-dispersal network is shaped by small-bodied species (e.g., the five dominant species in our study present an average body mass of 13 g) consuming and, most likely, dispersing small-seeded plant species (Silva et al., 2018). Therefore, the relatively low values of modularity found in our study can be associated with the presence of a few remaining forest birds, ecologically plastic and generalists in their diet, that feed on the available small-sized fruits. For instance, about 50% of the recorded birds present a diet consisting of different food items such as invertebrates, seeds and plants (Supplementary Appendix 7). In addition, high modularity in ecological networks is related to megadiverse communities, in which the diversity of animals that interact with particular sets of plant species could make them tightly linked within modules (Donatti et al., 2011). However, in our study we observed highly simplified networks composed by low richness of bird and plant, mainly in the more disturbed region.
Limitations
Our study has some limitations that deserve to be highlighted. First, spatio-temporal differences in fruiting in distinct forest fragments throughout the year can influence the distribution of bird species (Hasui et al., 2012) and therefore the interactions they perform. Similarly, the space-time decoupling, and the low probability of interspecific encounters between potentially mutualistic species can decrease the chances of registering interactions (Jordano, 2016). These factors can be more aggravating in studies on a landscape scale that propose to evaluate networks of interactions in different forest fragments. Second, the forests of the southern region of Bahia have low biomass of fleshy fruits available for frugivores (Pessoa et al., 2017b; Morante-Filho et al., 2018), which can reduce the register of interactions and hence hinder the identification of changes in the network structure (e.g., nestedness or modularity) (Jordano, 2016). This limitation imposed by environmental characteristics may have occurred in our study given that only 38% of the total number of captured birds had fruits or seeds in their stomach contents and feces. Finally, we observed a reduced sampling completeness of the pairwise plant-bird interactions in some forest fragments. Although our study employed a large hourly sampling effort for recording birds using mist nets, this was not enough to sample a considerable number of interactions. It is expected though that an asymptote wouldn’t be reached because a complete inventory of interactions is virtually impossible (Jordano, 2016) due to a series of constraints such as missing and forbidden links (Olesen et al., 2011). Still an ascendant curve was more evident for sites inserted in highly forested landscapes, indicating that most of the unsampled interactions are from the less disturbed region thus reinforcing the effects of forest cover on the number of interactions showed here.
Conclusion
Our results revealed a detrimental decrease in the size of bird-plant networks, triggered by landscape-scale deforestation. Depleted communities often exhibit gaps in ecosystem functionality through the loss of functional groups and decrease in the number of interaction partners (Laliberté et al., 2010; Muñoz et al., 2017). However, contrary to our expectations, the observed impoverishment of bird-plant interactions following deforestation was not mirrored by changes in network structure. Most of the interactions exclusively reported in sites immersed in more forested landscapes are shaped by a few small frugivorous birds and pioneer plants that are abundant and widely distributed in these sites.
The maintenance of network structure despite the variation in species richness and composition among sites can suggest a high functional redundancy among the remaining understory bird assemblages, thus assuring the dispersal of a few widespread pioneer species. Yet, networks are limited to a few plant and small bird species interacting among themselves, and also similar among sites regardless of the landscape or local levels of disturbance. Thus, even in our most preserved forest sites, characterized by a larger number of interacting species, network structure is functionally similar to those more disturbed forest sites.
Data Availability Statement
All relevant information, including dataset, are available in the Supplementary Material.
Ethics Statement
The animal study was reviewed and approved by Comissão de Ética no Uso dos Animais (CEUA) of Universidade Estadual de Santa Cruz.
Author Contributions
ÍM, EC, and JM-F: conceived and designed the experiments. ÍM: performed the experiments. ÍM, CE, and JM-F: data analysis and interpretation. ÍM and JM-F: writing-original draft. ÍM, CE, EC, and JM-F: writing the manuscript. All authors contributed to the article and approved the submitted version.
Funding
This work was funded by Rufford Small Grants for Nature Conservation (No. 22426–1), the Universidade Estadual de Santa Cruz (No. 00220.1100.1644/10-2018), FAPESB (No. 0525/2016), and CNPq (No. 563216/2010–7). This study was financed in part by the Coordenação de Aperfeiçoamento de Pessoal de Nível Superior—Brasil (CAPES)—Financial Code 001.
Conflict of Interest
The authors declare that the research was conducted in the absence of any commercial or financial relationships that could be construed as a potential conflict of interest.
The reviewer, MP, declared a past co-authorship with one of the authors CE, to the handling editor.
Acknowledgments
We thank the reviewers and editor for all the suggestions and improvements on the manuscript. We thank Deborah Faria for coordinating the REDE SISBIOTA. We thank Albérico Queiroz, Adrielle Leal, Fábio Soares, Thamyrys Souza and Júlia Perez for their help in the fieldwork; and local landowners for allowing us to work on their property. We thank Luiz Magnago, Jomar Gomes, and Daniel Piotto for their help in identifying some plant species. We thank Jacques Delabie for allowing access at the laboratory and Mariane Guedes for working with seed morphotyping. We also thank Gil Reuss and Diogo Caribé for helping to map the study area. This is the publication number 41 of the REDE SISBIOTA.
Supplementary Material
The Supplementary Material for this article can be found online at: https://www.frontiersin.org/articles/10.3389/fevo.2021.640210/full#supplementary-material
References
Anderson, D. R. (2008). Model Based Inference in the Life Sciences: A Primer on Evidence. New York, NY: Springer.
Arroyo-Rodríguez, V., Melo, F. P. L., Martínez-Ramos, M., Bongers, F., Chazdon, R. L., Meave, J. A., et al. (2017). Multiple successional pathways in human-modified tropical landscapes: new insights from forest succession, forest fragmentation and landscape ecology research. Biol. Rev. 92, 326–340. doi: 10.1111/brv.12231
Banks-Leite, C., Ewers, R. M., and Metzger, J. P. (2010). Edge effects as the principal cause of area effects on birds in fragmented secondary forest. Oikos 119, 918–926.
Bates, D., Mächler, M., Bolker, B., and Walker, S. (2015). Fitting linear mixed-effects models using lme4. J. Stat. Softw. 67, 1–48. doi: 10.18637/jss.v067.i01
Beckett, S. J. (2016). Improved community detection in weighted bipartite networks. R. Soc. Open Sci. 3:140536. doi: 10.1098/rsos.140536
Benchimol, M., Mariano-Neto, E., Faria, D., Rocha-Santos, L., de Souza Pessoa, M., Gomes, F. S., et al. (2017). Translating plant community responses to habitat loss into conservation practices: forest cover matters. Biol. Conserv. 209, 499–507. doi: 10.1016/j.biocon.2017.03.024
Bersier, L. F., Banašek-Richter, C., and Cattin, M. F. (2002). Quantitative descriptors of food-web matrices. Ecology 83, 2394–2407.
Blake, J. G. (1990). Quantifying abundance of fruits for birds in tropical habitats. Stud. Avian Biol. 13, 73–79.
Blake, J. G., and Loiselle, B. A. (2002). Manakins (Pipridae) in second-growth and old-growth forests: patterns of habitat use, movement, and survival. Auk 119, 132–148.
Bolker, B. (2015). Package ‘bbmle’. Tools for General Maximum Likelihood Estimation. R CRAN Repository. Available online at: http://cran.r-project.org/web/packages/bbmle/index.html (accessed September 20, 2020).
Bomfim, J. A., Guimarães, P. R. Jr., Peres, C. A., Carvalho, G., and Cazetta, E. (2018). Local extinctions of obligate frugivores and patch size reduction disrupt the structure of seed dispersal networks. Ecography 41, 1–11. doi: 10.1111/ecog.03592
Bradfer-Lawrence, T., Gardner, N., and Dent, D. H. (2018). Canopy bird assemblages are less influenced by habitat age and isolation than understory bird assemblages in Neotropical secondary forest. Ecol. Evol. 8, 5586–5597. doi: 10.1002/ece3.4086
Bueno, A. S., and Peres, C. A. (2019). Patch-scale biodiversity retention in fragmented landscapes: reconciling the habitat amount hypothesis with the island biogeography theory. J. Biogeogr. 46, 621–632.
Canale, G. R., Peres, C. A., Guidorizzi, C. E., Gatto, C. A. F., and Kierulff, M. C. M. (2012). Pervasive defaunation of forest remnants in a tropical biodiversity hotspot. PLoS One 7:e41671. doi: 10.1371/journal.pone.0041671
Cardoso da Silva, J. M., Cardoso, de Sousa, M., and Castelletti, C. H. (2004). Areas of endemism for passerine birds in the Atlantic forest. South America. Glob. Ecol. Biogeogr. 13, 85–92. doi: 10.1111/j.1466-882X.2004.00077.x
Cestari, C., and Pizo, M. A. (2013). Frugivory by the White-bearded Manakin (Manacus manacus, Pipridae) in restinga forest, an ecosystem associated to the Atlantic forest. Biota Neotropica 13, 345–350. doi: 10.1590/s1676-06032013000200038
Costa, J. M., da Silva, L. P., Ramos, J. A., and Heleno, R. H. (2016). Sampling completeness in seed dispersal networks: when enough is enough. Basic Appl. Ecol. 17, 155–164. doi: 10.1016/j.baae.2015.09.008
Coster, G. D., Banks-Leite, C., and Metzger, J. P. (2015). Atlantic forest bird communities provide different but not fewer functions after habitat loss. Proc. R. Soc. B 282, 1–8. doi: 10.1098/rspb.2014.2844
Donatti, C. I., Guimarães, P. R., Galetti, M., Pizo, M. A., Marquitti, F. M., and Dirzo, R. (2011). Analysis of a hyper-diverse seed dispersal network: modularity and underlying mechanisms. Ecol. Lett. 14, 773–781. doi: 10.1111/j.1461-0248.2011.01639.x
Donoso, I., Schleuning, M., García, D., and Fründ, J. (2017). Defaunation effects on plant recruitment depend on size matching and size trade-offs in seed-dispersal networks. Proc. R. Soc. B Biol. Sci. 284:20162664.
Dormann, C. F., Frund, J., Bluthgen, N., and Gruber, B. (2009). Indices, graphs and null models: analyzing bipartite ecological networks. Open Ecol. J. 2, 7–24. doi: 10.2174/1874213000902010007
Dudley, N. (2005). “Impact of forest loss and degradation on biodiversity,” in Forest Restoration in Landscapes, eds S. Mansourian, and D. Vallauri (New York, NY: Springer), doi: 10.1007/0-387-29112-1_3
Emer, C., Galetti, M., Pizo, M. A., Guimaraes, P. R. Jr., Moraes, S., Piratelli, A., et al. (2018). Seed-dispersal interactions in fragmented landscapes – a metanetwork approach. Ecol. Lett. 21, 484–493. doi: 10.1111/ele.12909
Emer, C., Jordano, P., Pizo, M. A., Ribeiro, M. C., da Silva, F. R., and Galetti, M. (2020). Seed dispersal networks in tropical forest fragments: area effects, remnant species, and interaction diversity. Biotropica 52, 81–89. doi: 10.1111/btp.12738
Environmental Systems Resource Institute (ESRI) (2012). ArcGIS Desktop Software, Release 10. California: Redlands.
Fahrig, L. (2013). Rethinking patch size and isolation effects: the habitat amount hypothesis. J. Biogeogr. 40, 1649–1663.
Faria, D., Laps, R. R., Baumgarten, J., and Cetra, M. (2006). Bat and bird assemblages from forests and shade cacao plantations in two contrasting landscapes in the Atlantic Forest of southern Bahia. Brazil. Biodivers. Conserv. 15, 587–612. doi: 10.1007/s10531-005-2089-1
Faria, D., Mariano-Neto, E., Martini, A. M. Z., Ortiz, J. V., Montingelli, R., Rosso, S., et al. (2009). Forest structure in a mosaic of rainforest sites: the effect of fragmentation and recovery after clear cut. For. Ecol. Manag. 257, 2226–2234. doi: 10.1016/j.foreco.2009.02.032
Faria, D., Paciencia, M. L. B., Dixo, M., Laps, R. R., and Baumgarten, J. (2007). Ferns, frogs, lizards, birds and bats in forest fragments and shade cacao plantations in two contrasting landscapes in the Atlantic forest. Brazil. Biodivers. Conserv. 16, 2335–2357.
Frazer, G. W., Canham, C. D., and Lertzman, K. P. (1999). Gap Light Analyzer (GLA), Version 2.0: Imaging Software to Extract Canopy Structure and Gap Light Transmission Indices From True-Colour Fish Eye Photographs. User’s Manual and Program Documentation. Burnaby, BC: Simon Fraser University.
Galetti, M., Guevara, R., Cortes, M. C., Fadini, R., Von Matter, S., Leite, A. B., et al. (2013). Functional extinction of birds drives rapid evolutionary changes in seed size. Science 340, 1086–1090. doi: 10.1126/science.1233774
García, D., Donoso, I., and Rodríguez-Pérez, J. (2018). Frugivore biodiversity and complementarity in interaction networks enhance landscape scale seed dispersal. Funct. Ecol. 32, 2742–2752.
García, D., Zamora, R., and Amico, G. C. (2011). The spatial scale of plant-animal interactions: Effects of resource availability and habitat structure. Ecol. Monog. 81, 103–121. doi: 10.1890/10-0470.1
Gomes, V. S. D. M., Tamashiro, J. Y., and Silva, W. R. (2011). Seed inflow to a forest patch promoted by understory frugivorous birds. Biota Neotropica 11, 95–102. doi: 10.1590/S1676-06032011000400010
Gouvêa, J. B. S. (1969). Contribuição à Geomorfologia do Sul da Bahia; Área dos Baixos Cursos dos rios Pardo e Jequitinhonha. Itabuna: Centro de Pesquisas do Cacau, 11. Comunicação Técnica n9 35.
Guimarães, P. R. Jr. (2020). The structure of ecological networks across levels of organization. Annu. Rev. Ecol. Evol. Syst. 51, 433–460. doi: 10.1146/annurev-ecolsys-012220-120819
Haddad, N. M., Brudvig, L. A., Clobert, J., Davies, K. F., Gonzalez, A., Holt, R. D., et al. (2015). Habitat fragmentation and its lasting impact on Earth’s ecosystems. Sci. Adv. 1:e1500052. doi: 10.1126/sciadv.1500052
Hagen, M., Kissling, W. D., Rasmussen, C., De Aguiar, M. A., Brown, L. E., Carstensen, D. W., et al. (2012). “Biodiversity, species interactions and ecological networks in a fragmented world,” in Advances in Ecological Research Change in Multispecies Systems Part 1. Eds U. Jacob and G. Woodward (Cambridge, MA: Academic Press), 89–120.
Hasui, E., Ramos, F. N., Tamashiro, J. Y., and Silva, W. R. (2012). Non-sequential fruit tracking by birds along an altitudinal gradient. Acta Oecol. 45, 66–78. doi: 10.1016/j.actao.2012.10.001
Henriksen, M. V., Chapple, D. G., Chown, S. L., and McGeoch, M. A. (2019). The effect of network size and sampling completeness in depauperate networks. J. Anim. Ecol. 88, 211–222. doi: 10.1111/1365-2656.12912
Jackson, H. B., and Fahrig, L. (2015). Are ecologists conducting research at the optimal scale? Glob. Ecol. Biogeogr. 24, 52–63. doi: 10.1111/geb.12233
Johnson, M. D., Ruthrauff, D. R., Jones, J. G., Tietz, J. R., and Robinson, J. K. (2002). Short-term effects of tartar emetic on re-sighting rates of migratory songbirds in the non-breeding season. J. Field Ornithol. 73, 191–196. doi: 10.1648/0273-85702002073
Jordano, P. (2016). Sampling networks of ecological interactions. Funct. Ecol. 30, 1883–1893. doi: 10.1111/1365-2435.12763
Laliberté, E., Wells, J. A., Declerck, F., Metcalfe, D. J., Catterall, C. P., Queiroz, C., et al. (2010). Land-use intensification reduces functional redundancy and response diversity in plant communities. Ecol. Lett. 13, 76–86. doi: 10.1111/j.1461-0248.2009.01403.x
Laurance, W. F., Nascimento, H. E. M., Laurance, S. G., Andrade, A., Ewers, R. M., Harms, K. E., et al. (2007). Habitat fragmentation, variable edge effects, and the landscape-divergence hypothesis. PLoS One 2:e1017. doi: 10.1371/journal.pone.0001017
Laurance, W. F., Sayer, J., and Cassman, K. G. (2014). Agricultural expansion and its impacts on tropical nature. Trends Ecol. Evol. 29, 107–116. doi: 10.1016/j.tree.2013.12.001
Lees, A. C., and Peres, C. A. (2009). Gap-crossing movements predict species occupancy in Amazonian forest fragments. Oikos 118, 280–290. doi: 10.1111/j.1600-0706.2008.16842.x
Lim, J. Y., Svenning, J., Göldel, B., Faurby, S., and Kissling, W. D. (2020). Frugivore-fruit size relationships between palms and mammals reveal past and future defaunation impacts. Nat. Commun. 11, 1–13. doi: 10.1038/s41467-020-18530-5
Lôbo, D., Leão, T., Melo, F. P. L., Santos, A. M., and Tabarelli, M. (2011). Forest fragmentation drives Atlantic forest of northeastern Brazil to biotic homogenization. Divers. Distrib. 17, 287–296. doi: 10.1111/j.1472-4642.2010.00739.x
Loiselle, B. A., Blendinger, P. G., Blake, J. G., and Ryder, T. B. (2007). “Ecological redundancy in seed dispersal systems: a comparison between manakins (Aves: Pipridae) in two tropical forests,” in Seed Dispersal: Theory and its Application in a Changing World, ed. A. J. Dennis (Wallingford: CABI), 178–195.
Magnago, L. F. S., Edwards, D. P., Edwards, F. A., Magrach, A., Martins, S. V., and Laurance, W. F. (2014). Functional attributes change but functional richness is unchanged after fragmentation of Brazilian Atlantic forests. J. Ecol. 102, 475–485. doi: 10.1111/1365-2745.12206
Marcilio-Silva, V., Pillar, V. D., and Marques, M. C. M. (2016). Functional turnover and community assemblage during tropical forest succession. Commun. Ecol. 17, 88–97. doi: 10.1556/168.2016.17.1.11
Marjakangas, E., Abrego, N., Grøtan, V., de Lima, R. A., Bello, C., Bovendorp, R. S., et al. (2020). Fragmented tropical forests lose mutualistic plant–animal interactions. Divers. Distrib. 26, 154–168. doi: 10.1111/ddi.13010
Martensen, A. C., Pimentel, R. G., and Metzger, J. P. (2008). Relative effects of fragment size and connectivity on bird community in the Atlantic Rain Forest: implications for conservation. Biol. Conserv. 141, 2184–2192. doi: 10.1016/j.biocon.2008.06.008
McCollin, D. (1998). Forest edges and habitat selection in birds: a functional approach. Ecography 21, 247–260.
McCullagh, P., and Nelder, J. A. (1989). Generalized Linear Models. Monographs on Statistics and Applied Probability, 2 Edn. London: Chapman and Hall.
McGarigal, K., and Marks, B. J. (1995). FRAGSTATS: Spatial Pattern Analysis Program for Quantifying Landscape Structure. Portland, OR: US Department of Agriculture, 122. Gen. Tech. Rep. PNW-GTR-351.
Mello, M. A. R., Rodrigues, F. A., da Costa, L. F., Kissling, W. D., Sekercioğlu, ÇH., Marquitti, F. M. D., et al. (2015). Keystone species in seed dispersal networks are mainly determined by dietary specialization. Oikos 124, 1031–1039. doi: 10.1111/oik.01613
Memmott, J. (2009). Food webs: a ladder for picking strawberries or a practical tool for practical problems? Philos. Trans. R. Soc. B Biol. Sci. 364, 1693–1699. doi: 10.1098/rstb.2008.0255
Mitchell, M. G. E., Suarez-Castro, A. F., Martinez-Harms, M., Maron, M., McAlpine, C., Gaston, K. J., et al. (2015). Reframing landscape fragmentation’s effects on ecosystem services. Trends Ecol. Evol. 30, 190–198. doi: 10.1016/j.tree.2015.01.011
Morante-Filho, J. C., Arroyo-Rodríguez, V., and Faria, D. (2016). Patterns and predictors of β-diversity in the fragmented Brazilian Atlantic forest: a multiscale analysis of forest specialist and generalist birds. J. Anim. Ecol. 85, 240–250. doi: 10.1111/1365-2656.12448
Morante-Filho, J. C., Arroyo-Rodríguez, V., Pessoa, M. S., Cazetta, E., and Faria, D. (2018). Direct and cascading effects of landscape structure on tropical forest and non-forest frugivorous birds. Ecol. Appl. 28, 2024–2032. doi: 10.1002/eap.1791
Morante-Filho, J. C., Benchimol, M., and Faria, D. (2020). Landscape composition is the strongest determinant of bird occupancy patterns in tropical forest patches. Land. Ecol. 36, 105–117. doi: 10.1007/s10980-020-01121-6
Morante-Filho, J. C., Faria, D., Mariano-Neto, E., and Rhodes, J. (2015). Birds in anthropogenic landscapes: the responses of ecological groups to forest loss in the Brazilian Atlantic forest. PLoS One 10:e0128923. doi: 10.1371/journal.pone.0128923
Muñoz, M. C., Schaefer, H. M., Böhning-Gaese, K., Neuschulz, E. L., and Schleuning, M. (2017). Phylogenetic and functional diversity of fleshy-fruited plants are positively associated with seedling diversity in a tropical montane forest. Front. Ecol. Evol. 5:93. doi: 10.3389/fevo.2017.00093
Murcia, C. (1995). Edge effects in fragmented forests: implications for conservation. Trends Ecol. Evol. 10, 58–62. doi: 10.1016/S0169-5347(00)88977-6
Myers, N., Mittermeier, R., Mittermeier, C., da Fonseca, G. A., and Kent, J. (2000). Biodiversity hotspots for conservation priorities. Nature 403, 853–858. doi: 10.1038/35002501
Newbold, T., Hudson, L. N., Hill, S. L., Contu, S., Lysenko, I., and Senior, R. A. (2015). Global effects of land use on local terrestrial biodiversity. Nature 520, 45–50. doi: 10.1038/nature14324
Olesen, J. M., Bascompte, J., Dupont, Y. L., Elberling, H., and Jordano, P. (2011). Missing and forbidden links in mutualistic networks. Proc. R. Soc. B 278, 725–732.
Paradis, E., and Schliep, K. (2019). Ape 5.0: an environment for modern phylogenetics and evolutionary analyses in R. Bioinformatics 35, 526–528. doi: 10.1093/bioinformatics/bty633
Pardini, R., Faria, D., Accacio, G. M., Laps, R. R., Mariano-Neto, E., Paciencia, M. L., et al. (2009). The challenge of maintaining Atlantic forest biodiversity: a multi-taxa conservation assessment of specialist and generalist species in an agro-forestry mosaic in southern Bahia. Biol. Conserv. 142, 1178–1190. doi: 10.1016/j.biocon.2009.02.010
Peña, R., Schleuning, M., Donoso, I., Rodríguez-Pérez, J., Dalerum, F., and García, D. (2020). Biodiversity components mediate the response to forest loss and the effect on ecological processes of plant–frugivore assemblages. Funct. Ecol. 34, 1257–1267.
Pessoa, M. S., Hambuckers, A., Benchimol, M., Rocha-Santos, L., Bomfim, J. A., Faria, D., et al. (2017a). Deforestation drives functional diversity and fruit quality changes in a tropical tree assemblage. Perspect. Plant Ecol. Evol. Syst. 28, 78–86. doi: 10.1016/j.ppees.2017.09.001
Pessoa, M. S., Rocha-Santos, L., Talora, D. C., Faria, D., Mariano-Neto, E., Hambuckers, A., et al. (2017b). Fruit biomass availability along a forest cover gradient. Biotropica 49, 45–55. doi: 10.1111/btp.12359
Piotto, D., Craven, D., Montagnini, F., Ashton, M., Oliver, C., and Thomas, W. W. (2019). Successional, spatial, and seasonal changes in seed rain in the Atlantic forest of southern Bahia. Brazil. PLoS One 14:e0226474. doi: 10.1371/journal.pone.0226474
Pizo, M. A., and dos Santos, B. T. (2011). Frugivory, post-feeding flights of frugivorous birds and the movement of seeds in a Brazilian fragmented landscape. Biotropica 43, 335–342. doi: 10.1111/j.1744-7429.2010.00695.x
Plein, M., Langsfeld, L., Neuschulz, E. L., Schultheiß, C., Ingmann, L., Töpfer, T., et al. (2013). Constant properties of plant-frugivore networks despite fluctuations in fruit and bird communities in space and time. Ecology 94, 1296–1306. doi: 10.1890/12-1213.1
Poulin, B., Lefebvre, G., and McNeil, R. (1994). Diets of land birds from northeastern venezuela. Condor 96, 354–367. doi: 10.2307/1369320
R Development Core Team (2019). R: A Language and Environment for Statistical Computing. Vienna: R Foundation for Statistical Computing.
Remsen, J. V., Areta, J. I., Cadena, C. D., Claramunt, S., Jaramillo, A., Pacheco, J. F., et al. (2019). A Classification of the Bird Species of South America. Chicago, IL: American Ornithologists’ Union.
Rocha-Santos, L., Benchimol, M., Mayfield, M. M., Faria, D., Pessoa, M. S., Talora, D. C., et al. (2017). Functional decay in tree community within tropical fragmented landscapes: effects of landscape-scale forest cover. PLoS One 12:e0175545. doi: 10.1371/journal.pone.0175545
Rocha-Santos, L., Mayfield, M. M., Lopes, A. V., Pessoa, M. S., Talora, D. C., Faria, D., et al. (2020). The loss of functional diversity: a detrimental influence of landscape-scale deforestation on tree reproductive traits. J. Ecol. 108, 212–223. doi: 10.1111/1365-2745.13232
Rocha-Santos, L., Pessoa, M. S., Cassano, C. R., Talora, D. C., Orihuela, R. L., Mariano-Neto, E., et al. (2016). The shrinkage of a forest: landscape-scale deforestation leading to overall changes in local forest structure. Biol. Conserv. 196, 1–9. doi: 10.1016/j.biocon.2016.01.028
Rodewald, A. D., Rohr, R. P., Fortuna, M. A., and Bascompte, J. (2014). Community-level demographic consequences of urbanization: an ecological network approach. J. Anim. Ecol. 83, 1409–1417. doi: 10.1111/1365-2656.12224
Sambuichi, R. H. R., Vidal, D. B., Piasentin, F. B., Jardim, J. G., Viana, T. G., Menezes, A. A., et al. (2012). Cabruca agroforests in southern Bahia, Brazil: tree component, management practices and tree species conservation. Biodivers. Conserv. 21, 1055–1077. doi: 10.1007/s10531-012-0240-3
Schleuning, M., Ingmann, L., Straub, R., Fritz, S. A., Dalsgaard, B., Dehling, M. D., et al. (2014). Ecological, historical, and evolutionary determinants of modularity in weighted seed-dispersal networks. Ecol. Lett. 17, 454–463. doi: 10.1111/ele.12245
Silva, S. M., Agne, C. E., Aleixo, A., and Bonatto, S. L. (2018). Phylogeny and systematics of Chiroxiphia and Antilophia manakins (Aves, Pipridae). Mol. Phylogenetics Evol. 127, 706–711. doi: 10.1016/j.ympev.2018.06.016
Supp, S. R., and Ernest, S. K. M. (2014). Species-level and community-level responses to disturbance: a cross-community analysis. Ecology 95, 1717–1723. doi: 10.1890/13-2250.1
Sutherland, W. J., Newton, I., and Green, E. R. (2004). Bird Ecology and Conservation: A Handbook of Techniques. Oxford: OUP Oxford.
Thier, O., and Wesenberg, J. (2016). Floristic composition and edge-induced homogenization in tree communities in the fragmented Atlantic rainforest of Rio de Janeiro. Brazil. Trop. Conserv. Sci. 9, 852–876. doi: 10.1177/194008291600900217
Tscharntke, T., Klein, A. M., Kruess, A., Steffan-Dewenter, I., and Thies, C. (2005). Landscape perspectives on agricultural intensification and biodiversity-ecosystem service management. Ecol. Lett. 8, 857–874. doi: 10.1111/j.1461-0248.2005.00782.x
Valiente-Banuet, A., Aizen, M. A., Alcántara, J. M., Arroyo, J., Cocucci, A., Galetti, M., et al. (2015). Beyond species loss: the extinction of ecological interactions in a changing world. Funct. Ecol. 29, 299–307. doi: 10.1111/1365-2435.12356
Visco, D. M., Michel, N. L., Boyle, W. A., Sigel, B. J., Woltmann, S., and Sherry, T. W. (2015). Patterns and causes of understory bird declines in human-disturbed tropical forest landscapes: a case study from Central America. Biol. Conserv. 191, 117–129.
Zurita, G., Pe’er, G., Bellocq, M. I., and Hansbauer, M. M. (2012). Edge effects and their influence on habitat suitability calculations: a continuous approach applied to birds of the Atlantic forest. J. Appl. Ecol. 49, 503–512. doi: 10.1111/j.1365-2664.2011.02104.x
Keywords: Brazilian Atlantic Forest, fragmentation, frugivory, frugivorous birds, habitat loss, habitat structure, resource availability
Citation: Menezes Pinto Í, Emer C, Cazetta E and Morante-Filho JC (2021) Deforestation Simplifies Understory Bird Seed-Dispersal Networks in Human-Modified Landscapes. Front. Ecol. Evol. 9:640210. doi: 10.3389/fevo.2021.640210
Received: 10 December 2020; Accepted: 04 June 2021;
Published: 08 July 2021.
Edited by:
Anna Traveset, Institut Mediterrani d’Estudis Avançats (CSIC-UIB), SpainReviewed by:
Daniel García, University of Oviedo, SpainMarco Aurélio Pizo, São Paulo State University, Campus Rio Claro, Brazil
Copyright © 2021 Menezes Pinto, Emer, Cazetta and Morante-Filho. This is an open-access article distributed under the terms of the Creative Commons Attribution License (CC BY). The use, distribution or reproduction in other forums is permitted, provided the original author(s) and the copyright owner(s) are credited and that the original publication in this journal is cited, in accordance with accepted academic practice. No use, distribution or reproduction is permitted which does not comply with these terms.
*Correspondence: Ícaro Menezes Pinto, aWNhcm8uYmlvLnVlc2NAZ21haWwuY29t; José Carlos Morante-Filho, amNtZmlsaG85QGhvdG1haWwuY29t