- 1Department of Ecology, University of Innsbruck, Innsbruck, Austria
- 2Schwabeneckweg, Innsbruck, Austria
- 3Faculty of Biology, University of Innsbruck, Innsbruck, Austria
- 4Department of Botany, University of Innsbruck, Innsbruck, Austria
Climate change was already shown to increase species numbers in high elevations. In contrast, grazing might interfere with climate change effects. To disentangle both the effects remains a major challenge of alpine ecology. The present study investigated both the effects on species diversity along an elevation gradient in the Austrian Central Alps. We aimed to answer the following questions: How do species diversity and frequency of subalpine–alpine–subnival plant communities change in grazed sites with time? Do competitive plant species increase in the communities? How does grazing exclusion affect species diversity, functional groups, and strategy types? Are environmental changes (temperature, sunshine duration, precipitation) responsible for diversity changes or does grazing override climate effects? The study was carried out for 18 years along an elevation gradient from 1,958 to 2,778 m a.s.l. at Obergurgl (Tyrol, Austria), including six different plant communities. A total of 11 grazing exclusions were established. At each community, the frequency of the species was counted in 1 m2 plots yearly or at least every 3–4 years. Environmental data were obtained from the weather station Obergurgl. Changes of the community parameters and the species composition were analyzed by partial redundancy analyses and mixed-effect models. Species diversity increased with time at all grazed sites, but this increase was suppressed under grazing exclusion. Grazing exclusion effects became pronounced after 5 years. The most consistent result was the increase of bryophytes throughout. At the subalpine grassland, tall-growing species expanded in the exclosures; at the upper alpine Carex curvula grassland, snow bed species decreased with grazing exclusion. Among the environmental factors, sunshine duration of the previous year’s autumn quartal was found to be the essential variable for the changes. We concluded that diversity increases in grazed communities of the Austrian Central Alps can be attributed to climate change. An indication of slightly reduced and altered weather effects under grazing exclusion was found.
Introduction
In view of the ongoing climate warming (Gobiet et al., 2014), biodiversity changes in high elevations are one of the central topics in today’s ecological research (Winkler et al., 2019). A severe biodiversity loss is expected worldwide (Un Report, 2019) with major impacts also in high elevations, although probably with some time lags in the alpine zone (Dullinger et al., 2012; Alexander et al., 2018). Data comparing new and old relevés or data from long-term monitoring projects revealed increases in alpine plant species diversity (Grabherr et al., 1994; Holzinger et al., 2008; Pauli et al., 2012; Wipf et al., 2013) with striking accelerations during the last decades (Steinbauer et al., 2018). However, these studies focused on diversity changes of upper summit areas. Plant communities in the alpine zone were less investigated (e.g., Vittoz et al., 2009; Gritsch et al., 2016; Matteodo et al., 2016). Responses to climate change were found to be weak or even missing (Windmaißer and Reisch, 2013) or they were rather heterogeneous (Gritsch et al., 2016). Only snow bed communities seem to be an exception, showing significant changes with time (Matteodo et al., 2016; Liberati et al., 2019).
Especially in the Austrian Central Alps, a long tradition of grazing prevails in high elevations (Vorren et al., 1993; Bortenschlager, 2000). More or less all landscapes from the subalpine to the subnival zone are grazed—even today—by sheep at higher elevations and cattle/horses at the subalpine zone. Cessation of grazing may cause severe changes in community composition (Tasser and Tappeiner, 2002; Dullinger et al., 2003) as shown recently in the Tatra Mts (Czortek et al., 2018), although also accessibility of the sites has to be considered. Mayer and Erschbamer (2017) reported that alpine plant communities exhibit an individualistic reaction to grazing exclusion, depending on the kind of grazers. Abandonment of livestock grazing might decrease short-statured plants and promote competitive species (Vittoz et al., 2009) such as trees (Gehrig-Fasel et al., 2007; Vittoz et al., 2008; Wieser et al., 2019). Generally, the literature shows rather inconsistent results on the effects of grazing in high elevations (review in Pardo et al., 2015), the slow reaction of alpine species to grazing exclusion causing interpretation problems and aggravating predictions (Evju et al., 2009; Pardo et al., 2015).
All in all, alpine ecology has to deal with two major drivers governing diversity and abundance of species. On the one hand, the ongoing warming with mean temperature increases of 1.2°C during the last 55 years in the Austrian Central Alps (Fischer, 2010) might continuously enhance species numbers. On the other hand, permanent grazing activity might reduce species diversity (Song et al., 2020); however, it may also interact with climate change in the way that community changes are decelerated or avoided (Speed et al., 2012; Vowles et al., 2017; Wang et al., 2019) or enhanced during warm and dry periods (Pardo et al., 2015). In the Southern Scandes, Norway, alpine grasslands were found to shift upward by 3 m over 8 years of grazing exclusion (Speed et al., 2012). Such striking effects were never seen in the Alps. However, machine-based models predicted that alpine calcareous pastures with Carex firma will be reduced by future climate while Nardus stricta and Carex curvula pastures will gain distribution area in the Italian mountains (Dibari et al., 2020).
With our study, we aimed to assess ongoing climate change effects in grazed and ungrazed plant communities along an elevation gradient from the subalpine to the alpine and subnival zone in the Austrian Central Alps. We selected seven widespread plant communities and followed the changes in species number, frequency, and composition over time (up to 18 years of monitoring). We expected significant changes in species diversity depending on community type. In order to evaluate the impact of climate change on vegetation structure (Pardo et al., 2015), we classified the species according to functional groups in graminoids, herbs, dwarf shrubs, mosses, and lichens.
Climate change scenarios outline a migration of lowland species toward higher elevations (Pauli et al., 2007, 2012; Lamprecht et al., 2018). Alpine species are known as strictly adapted to the harsh conditions (Körner, 2003), having a limited plasticity compared to species from lower elevations (Gugger et al., 2015). Due to their higher growth rate, competitive species are particularly benefiting from warming (Farrer et al., 2015; Winkler et al., 2019) and the low-statured alpine species are threatened by competitive displacement (Alexander et al., 2015, 2018). Finally, new communities could evolve (Alexander et al., 2015). We expected that such tendencies should be observable with our long-term investigations. We grouped the species by means of the C-S-R strategy concept of Grime (1979), differentiating between competitive, stress-tolerant, and ruderal species. By this, we will be able to answer the question if competitive species tend to increase in the studied communities. However, not only may competitive species increase due to invasions from lower elevations (direct climate change effect, Farrer et al., 2015) but also predominant resident species may inhibit companion species due to their increased growth intensity and competitive ability, provoking “indirect effects” of climate change (Farrer et al., 2015). Consequently, an increase of the competitive species group might be attributed to invasion of new competitive species or by enhanced growth and competition of predominant resident species. In addition to functional attributes, we used also ecological indicator values (Landolt et al., 2010) to detect if increases in temperature values and nitrogen values occurred with time. Calculating indicator values for each studied plant community, we expected to gain information on shifts of community composition and thermophilization tendencies (Pauli et al., 2007, 2012; Gottfried et al., 2012).
Moreover, using regional weather data (temperature, sunshine duration, precipitation) for the whole study period, we aimed to analyze the environmental effects on grazed and ungrazed sites. By this, we tried to disentangle climate and grazing effects and to evaluate if grazing had the power to modify climate-induced changes in diversity (Speed et al., 2013).
Altogether, our objectives were (i) to study changes and environmental effects in species diversity, functional groups, strategy types, and ecological indicator values of all grazed and ungrazed communities; (ii) to investigate the dynamics of species composition; and (iii) to highlight grazing effects on alpine species. The overall goal of this paper was to unravel environmental vs. grazing effects. The following hypotheses were tested:
H1 Species diversity and frequency of all functional groups (graminoids, herbs, dwarf shrubs, mosses, lichens) will be enhanced with time at all grazed sites.
H2 Competitive strategists and species with high Landolt temperature values (i.e., species from lower elevations or species with wide distribution ranges) as well as species with high nitrogen values will increase at all grazed sites.
H3 Diversity will decrease in grazing exclusion plots.
H4 Changes in temperature, sunshine duration, and precipitation are responsible for diversity changes. Grazing will not override environmental effects.
Materials and Methods
Study Sites
The investigated sites (Table 1 and Supplementary Material 1) are located along an elevation gradient, from the subalpine to subnival zone at Obergurgl in the inner Ötztal (Tyrol, Austria). The study sites belong to the Long-Term Ecosystem Research Network (LTER) within the Long-Term Socio-Ecological Research (LTSER) platform Tyrolean Alps1 and LTER-Austria2. The subalpine zone (1,960–2,200 m a.s.l.) is grazed by cattle during summer; the alpine and subnival zone (2,300–2,900 m a.s.l.) by sheep; and the area of the sites S03–S05, S06, and S13 by sheep, Haflinger horses, and partly also by goats. Details on number of grazers and stocking rates are given in Mayer et al. (2009).
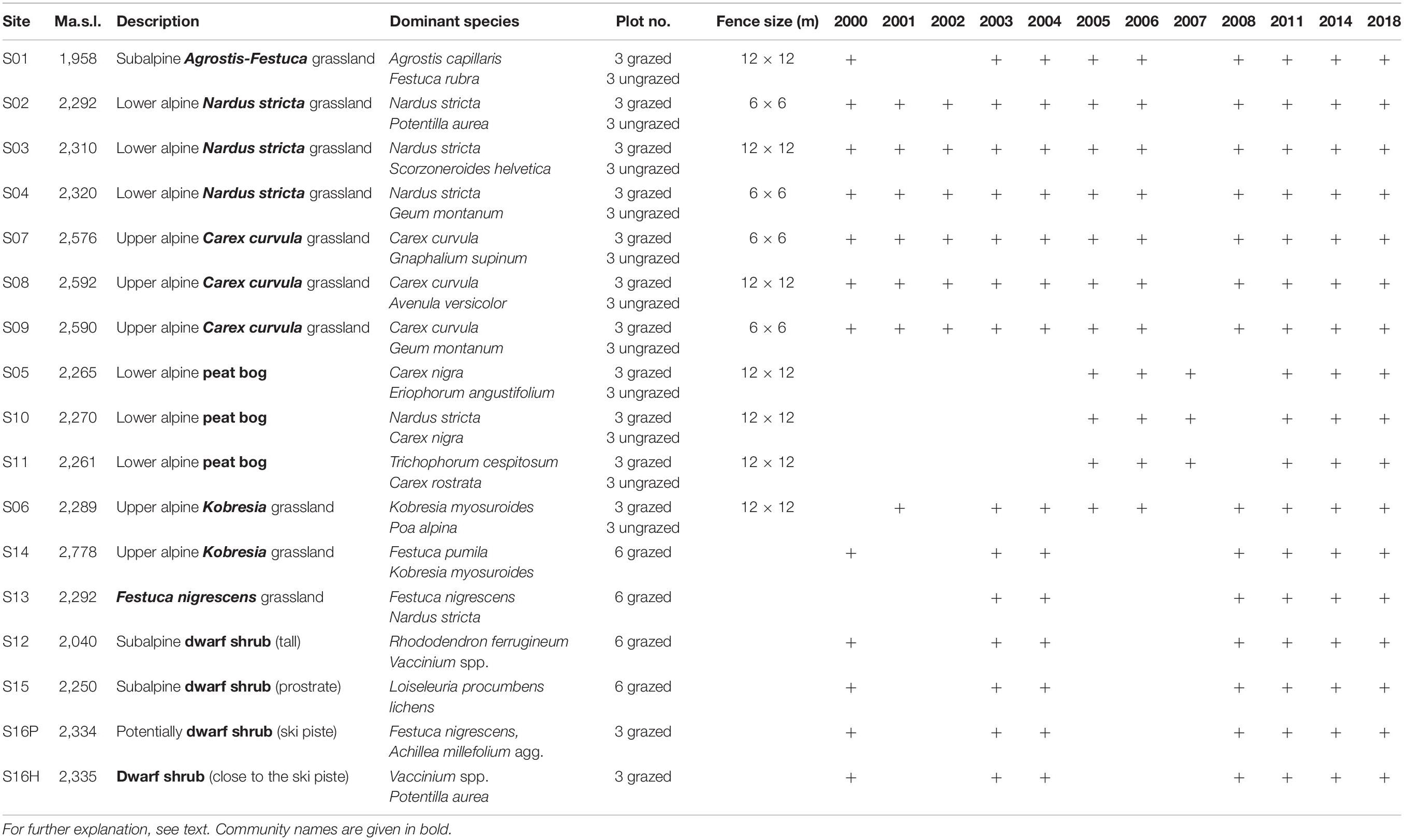
Table 1. Overview on the sites and their elevation (m above sea level, a.s.l.), description of the plant communities and the dominant species, number of grazed and ungrazed plots per community, size of the fence in m, and sampling years.
Field Work
Permanent plots of 1 m2 were marked at S01–S04, S07–S09, S12, and S14–S16 in 2000; at S06 in 2001; S13 in 2003; and at S05, S10, and S11 in 2005 (Table 1). Within fenced exclosures (Table 1), three ungrazed × 1 m2 plots were established per exclosure site together with three × 1 m2 plots as controls outside the fence. At the grazed sites without exclosures (Table 1), six × 1 m2 plots were installed. The sites were monitored regularly (except 2007 when monitoring was restricted to S05, S10, and S11) till 2018 (Table 1) by means of a frequency frame, divided into 100 subplots of 1 dm2. Within each subplot, presence/absence of the species and the functional groups (graminoids, herbs, dwarf shrubs, mosses, lichens) were recorded. The total frequency per 1 m2 was given as percentage of the 100 subplots.
The nomenclature of vascular plant species follows Fischer et al. (2008); bryophytes (with exception of Polytrichum species, which were identified at the genus level) and lichens were treated as functional groups. Some lichens were identified at the species level (Cetraria islandica, Peltigera aphthosa) and integrated in the species analyses. Vascular species not present at the first monitoring but present in 2018 were defined as newcomers. The frequency sum of each species/functional group per plot was calculated for each sampling year.
Statistical Analyses
Statistical analyses and graphs were done using PRIMER 7 with the PERMANOVA + add-on (Anderson et al., 2008; Clarke and Gorley, 2015), CANOCO 5.12 (Ter Braak and Šmilauer, 2018), and R 3.6 (R Core Team, 2019) with packages lme4, lmerTest, effects, ggplot2, and grid.
The plant communities were grouped by means of a principal coordinate ordination (PCO, PRIMER) with Bray–Curtis similarity of square-root-transformed species data (Table 1 and Supplementary Material 2). Seven communities were distinguished: siliceous subalpine Agrostis–Festuca grassland (one exclosure, one control, S01), siliceous lower alpine Nardus stricta grassland (three exclosures, three controls, S02–S04), siliceous upper alpine Carex curvula grassland (three exclosures, three controls, S07–S09), siliceous lower alpine peat bog (three exclosures, three controls, S05, S10, S11), calcareous upper alpine Kobresia grassland (one exclosure, one control, located at the Rotmoos glacier foreland, S06; one grazed subnival site, no exclosure, S14), siliceous lower alpine Festuca nigrescens grassland (one grazed site, no exclosure, S13), and siliceous subalpine dwarf shrub communities (S12, S15, S16, all of them grazed, no exclosure, Table 1). Dominant species per site are shown in Table 1 and more comprehensively in Supplementary Material 3. In total, 33 × 1 m2 plots were monitored inside the exclosures and 33 × 1 m2 outside the exclosures (controls). These plots were analyzed to detect exclosure effects. To detect climate change effects, a total of 63 × 1 m2 grazed plots were analyzed.
For the functional groups, frequency sums (graminoids, herbs, dwarf shrubs, mosses, and lichens) and frequency fractions were calculated. C-S-R strategies were extracted from Flora Indicativa (Landolt et al., 2010) and converted to numeric indicators (values 1/3, 2/3, 1 according to the number of letters per strategy type; for instance, CCS was converted to 2/3 value C). For further calculations, weighted sums and fractions of total sum were used. Landolt indicator values temperature, light, nitrogen, and soil reaction were checked in Flora Indicativa (Landolt et al., 2010) and calculated as weighted means of species frequencies (omitting species without indicator values).
The temporal changes of all grazed communities (Table 1) and their species composition were analyzed by partial redundancy analyses (pRDA on correlations, CANOCO) testing for linear trends (year as metric variable) and for additional non-linear fluctuations around this trend (year as categorical variable). To isolate these effects, and to account for repeated measures, the large differences between plots were partialed out. Species frequencies were square-root transformed and species with < 5 presences excluded. All significance tests were done with 4,999 Monte-Carlo permutations (giving minimal p-values of 0.0002).
Univariate analyses of the diversity parameters [total frequency N, species number S, Pielou evenness J, and Shannon diversity H′ (log2)] and all species (bryophytes were regarded as species, though being a functional group; the functional group containing bryophytes and Polytrichum spp. was called “mosses”) were done for a screening of the same effects (year as metric variable and year as categorical variable) by linear mixed-effect models with plot as random factor (done in R). Significances were Bonferroni-corrected for the number of species tested. The strength of time trends of all community parameters (diversity, functional group, C-S-R strategy, Landolt indicator values) was shown graphically, using the loadings of axis 1 from pRDA.
Exclosure effects on species composition were tested by PERMANOVA (also with plot as random factor, 4,999 permutations) using Bray–Curtis distances of square-root-transformed species frequencies. Effects should appear as differences in the changes over time between the fenced and grazed plots, therefore, the interaction “exclosure × years (categorical)” was the target effect for this analysis. Also for the exclosure effects, univariate analyses were performed for diversity parameters and single species, again using mixed-effect models but now with the focus on the interaction “exclosure × years.”
Data from the weather station Obergurgl (1,938 m a.s.l.) were obtained by © ZAMG (Christian Posch 18/02/2020), spanning the years 1999–2018. Quartal means of air temperature in 200 cm height, sunshine duration, and precipitation sums were calculated for months 1–3 (Q1 winter), 4–6 (Q2 spring), 7–9 (Q3 summer), and 10–12 (Q4 autumn). Two quartals of the previous year (∗_Q3p, ∗_Q4p) were included to allow analysis of delayed weather effects. The weather data were used as explanatory variables for the community parameters of all samples in a pRDA and tested for simple and conditional effects (using CANOCO’s summarize-effects option).
In all analyses, the repeated measures of plots were taken into account, as covariates in partial analyses or as random factor in mixed-effect models.
Results
Changes in Grazed Communities Over Time
All grazed communities showed more or less pronounced changes over time. Variation partitioning (Table 2) revealed that across all sites a common linear trend of change accounted for 27.3% of the variation (Yr, range for communities 17.4–35.5%) and another 13.2% were due to synchronous fluctuations between years (Yrc, range for communities 7.9–42.3%). These fractions refer to the variation remaining after accounting for the very large plot (and site) differences (86.2% of the total variation, range for communities 26.3–81.4%). The time effects were highly significant throughout (p < 0.001, except effect of Yrc in the peat bog). The diversity parameters species number S, frequency N, Pielou evenness J, and Shannon index H′ increased at all grazed communities (Figure 1). The increases of S and H′ were lowest at the peat bog and highest at the subalpine Agrostis–Festuca grassland. Also J increased with time with exception of the Festuca nigrescens grassland. Lowest changes per site occurred at S15 and S11. Details on diversity changes per site are reported in Supplementary Material 4.
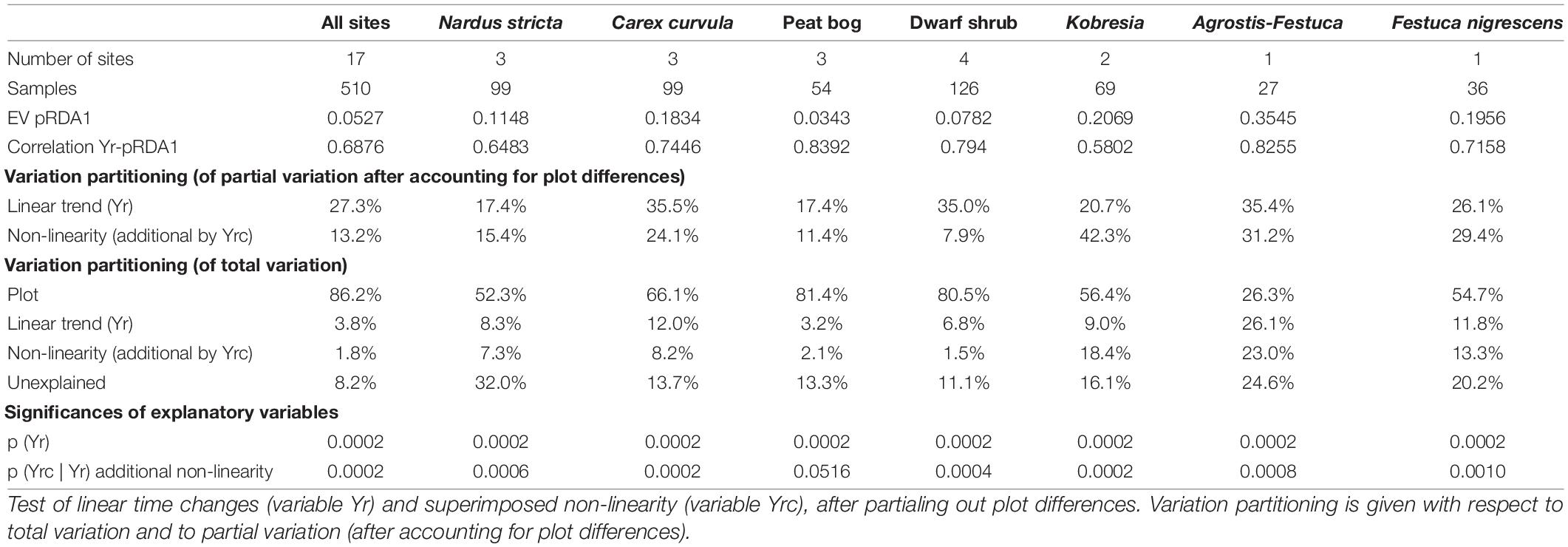
Table 2. Results of the pRDA (of all diversity parameters, total frequency, C-S-R strategies, and Landolt indicator values): changes over time for all grazed sites and grazed communities separately.
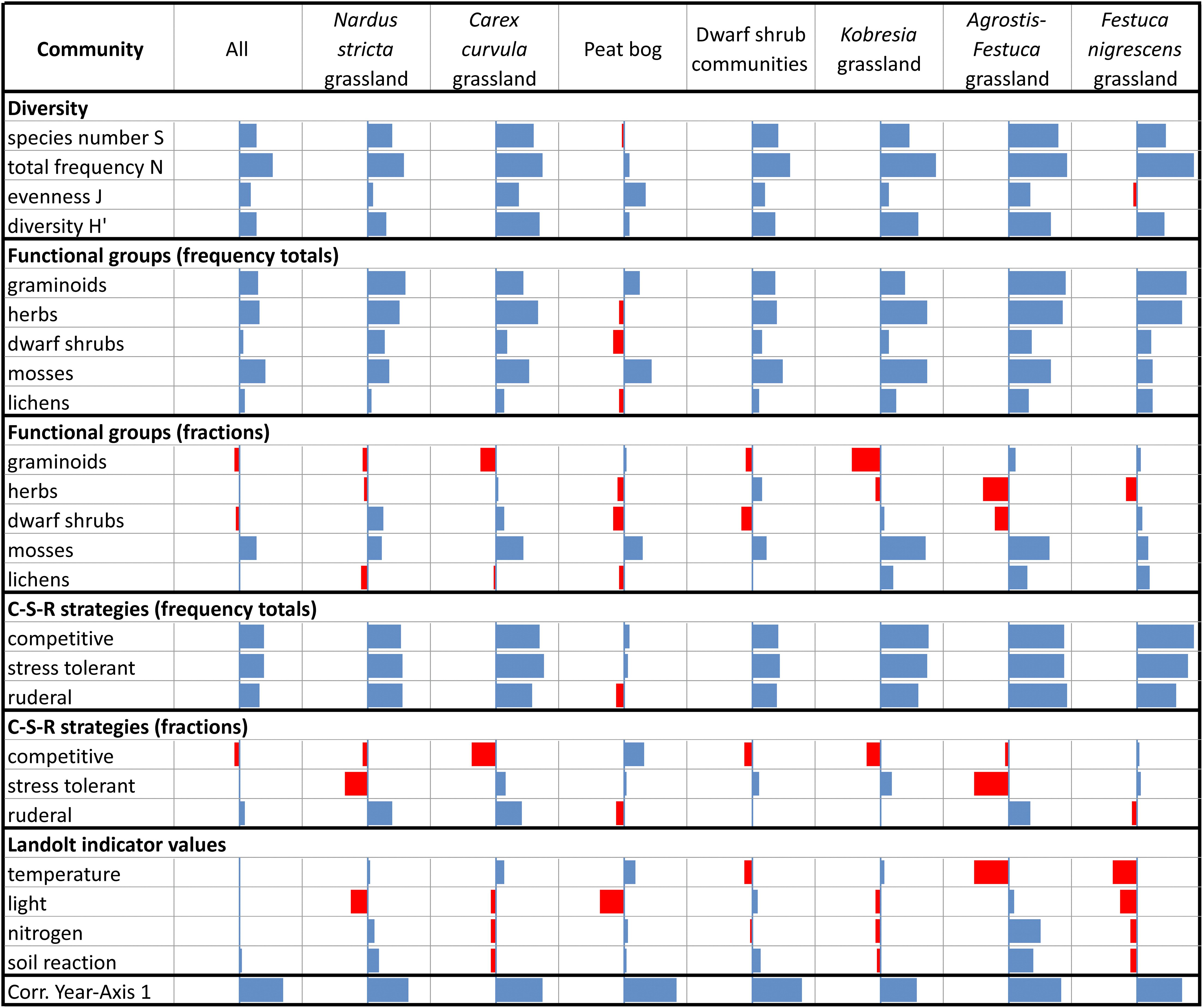
Figure 1. Strength of time trend of community parameters (grazed sites): loadings on axis 1 (correlations) from pRDA representing the linear increase or decrease over time (range of bars –1 to +1). Bottom line shows correlation of explanatory variable Yr with pRDA axis 1. Results shown for the seven communities and for all communities together.
Among the functional groups, clear linear increases resulted in all grazed communities for mosses (Figure 1). All other functional groups exhibited divergent increasing/decreasing patterns especially when considering the relative values. Small changes occurred in dwarf shrubs and in lichens (Figure 1). The competitive strategists decreased mainly at the Carex curvula and Kobresia grassland while stress tolerators decreased at the Agrostis–Festuca and Nardus stricta grassland (Figure 1). Temperature values decreased at the Agrostis–Festuca and Festuca nigrescens grassland, light values at the Nardus stricta grassland, the peat bog, and the Festuca nigrescens grassland. Nitrogen and soil reaction showed minor changes with exception of the Agrostis–Festuca grassland where these values remarkably increased (Figure 1).
Changes at the grazed sites did not follow a straight trajectory even if the main change occurred along axis 1 of the pRDA (Figure 2). There were remarkable fluctuations between the years 2000 and 2003, and also 2005 and 2008. The most relevant species/species groups for the changes were bryophytes, Polytrichum spp., Phyteuma hemisphaericum, Pinus cembra (newcomer), Luzula multiflora, Pseudorchis albida, and one species which disappeared during the second half of the study period (Diphasiastrum alpinum, Figure 2).
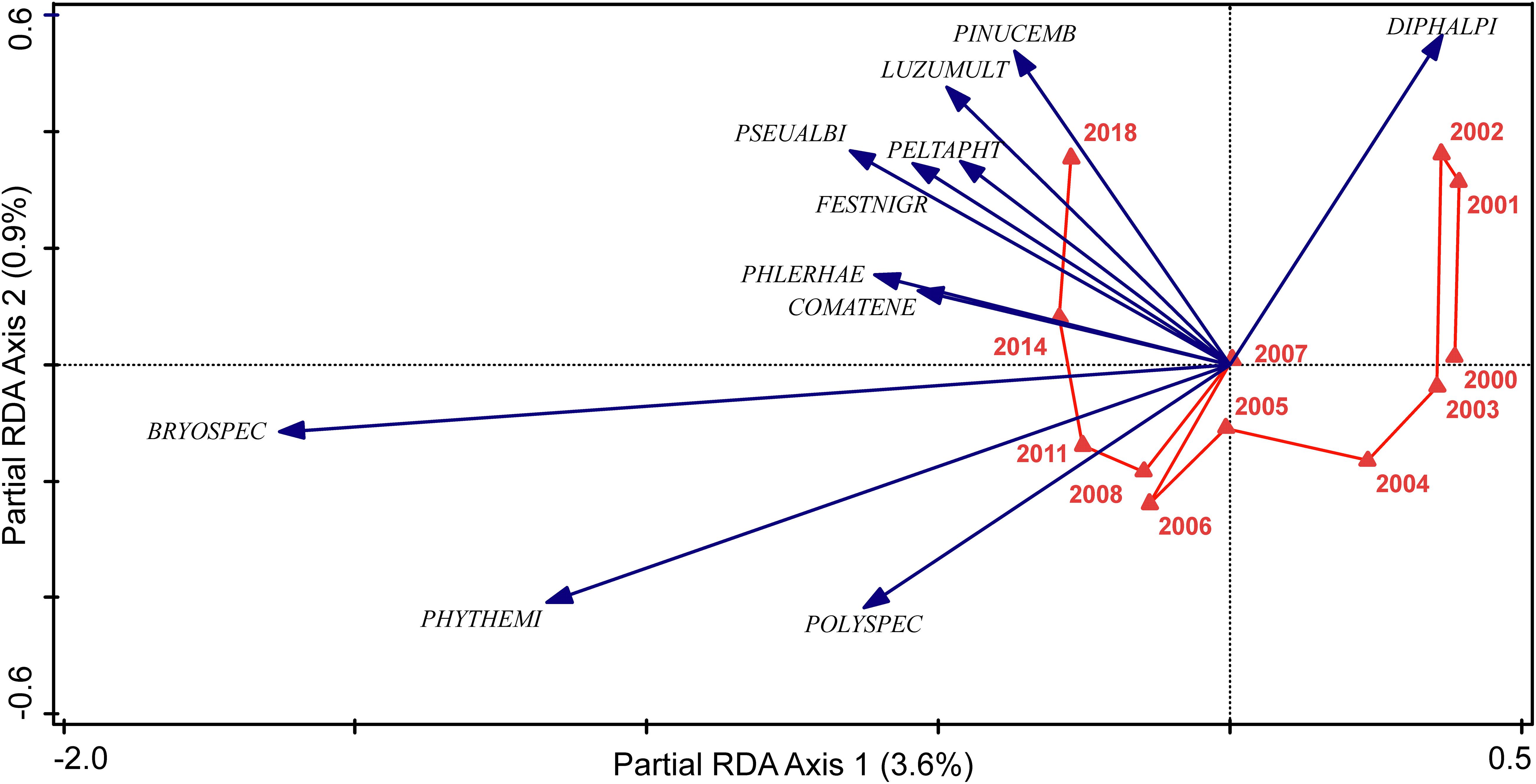
Figure 2. Changes of the grazed sites from 2000 to 2018. The most relevant species (fit > 2%) are plotted as vectors (POLYSPEC = Polytrichum species, PHYTHEMI = Phyteuma hemisphaericum, BRYOSPEC = brophyte species, COMATENE = Comastoma tenellum, PHLERHAE = Phleum rhaeticum, FESTNIGR = Festuca nigrescens, PSEUALBI = Pseudorchis albida, PELTAPHT = Peltigera aphthosa, LUZUMULT = Luzula multiflora, PINUCEMB = Pinus cembra, DIPHALPI = Diphasiastrum alpinum). Partial RDA with plot as covariate, Monte-Carlo p = 0.0002, explained variation of axes indicated.
Bryophyte frequencies linearly increased throughout the years (p < 0.001, 17 plots, Table 3) with steepest increases at S01, S10–S12, and S16P (Supplementary Material 5). A broad variety of herbs (Phyteuma hemisphaericum, Scorzoneroides helvetica, Homogyne alpina, Geum montanum, Campanula scheuchzeri, Leucanthemopsis alpina, Veronica alpina) and graminoids (Nardus stricta, Anthoxanthum odoratum agg., Carex curvula, Poa alpina, Festuca halleri, F. nigrescens) had significant linear increases together with one lichen (Cetraria islandica, Table 3). Only one species (Salix herbacea, Table 3) showed significant decreases over time.
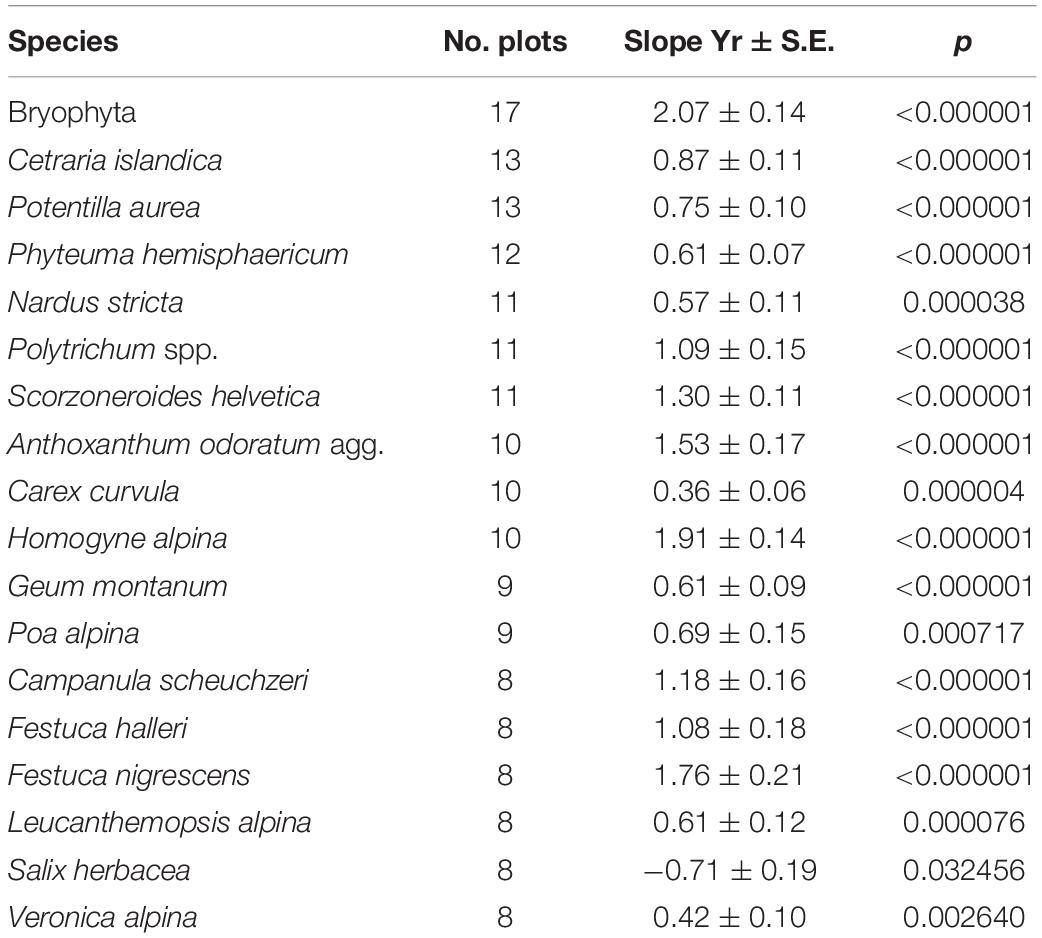
Table 3. Selection of species with significant increase/decrease (only Salix herbacea) over time (linear trend), number of grazed plots with presences, slope for Yr ± S.E., and Bonferroni-corrected p-values (p).
Grazing Exclusion Effects
Across all sites (without the peat bog because of later installation of the exclosure fences), diversity was significantly lower within the fences than in the controls outside (species number S and Shannon index H′: p < 0.001, mixed effect models), and the effect started to appear after 5 years of exclosure (Figure 3).
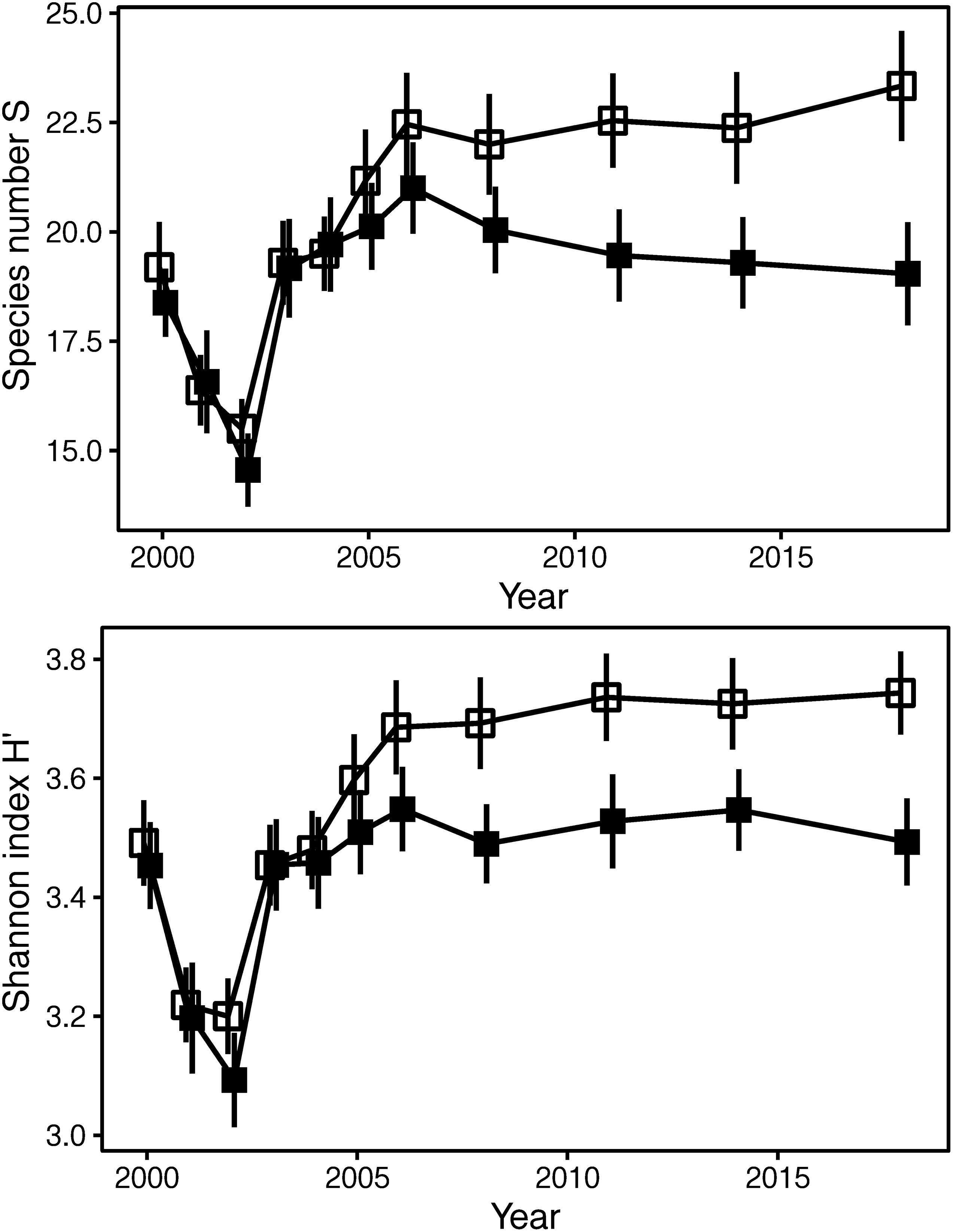
Figure 3. Grazing effects on diversity [species number S and Shannon index H′ (log2)]: time courses for exclosure and control plots diverging after 5 years. Means ± S.E. for exclosure plots (filled squares) and grazed control plots (open squares). Sites with later installation of fences excluded (peat bog: fences in 2005).
Regarding functional groups, only at the Agrostis–Festuca grassland was the exclusion effect strong with responses of both graminoids (p < 0.001) and herbs (p = 0.012). At the Nardus stricta grassland, there was a decrease in graminoids (p = 0.018) and at the Carex curvula grassland in herbs (p < 0.001), while none of the functional groups were significantly affected by grazing exclusion at the peat bog and the Kobresia grassland.
Grazing exclusion effects on species composition were not consistent across the plant communities. PERMANOVA results revealed that 8 out of 11 sites were significantly affected by grazing exclusion (Table 4). Two of the three sites at the Nardus stricta grassland (S03, S04) and the Carex curvula grassland (S07, S09), respectively, showed significant exclusion effects. Also at the peat bog only two sites had significant exclosure effects (S05, S10) while on the wettest site S11 weakest changes occurred (p = 0.07). Exclosure plots diverged also at the Agrostis–Festuca grassland (S01) and the Kobresia grassland (S06) from the control plots (p = 0.0002, Table 4). Within the exclosures, different species were significantly affected by grazing exclusion; some enhanced their frequency, and some decreased considerably with respect to the controls. At the Nardus stricta grassland, within the exclosures bryophytes increased after 10 years and Geum montanum from the beginning; Anthoxanthum odoratum agg. first increased more than in the controls but decreased in the end (Figure 4). At the Carex curvula grassland, snow bed species (Sibbaldia procumbens, Gnaphalium supinum) decreased in the exclosures while the dominating Carex curvula was not affected (the species showed parallel changes of exclosures and controls, Figure 4). At the peat bog, only Carex rostrata increased in the exclosures. At the Agrostis–Festuca grassland, increasing species were Geranium sylvaticum (in the last years) and Potentilla aurea (only temporarily over 10 years), whereas some graminoids (Nardus stricta, Poa alpina) and other herbs decreased (Trifolium pratense ssp. nivale, Galium anisophyllon, Leontodon hispidus) (not all species shown in Figure 4). At the Kobresia grassland, the dominant Kobresia myosuroides further increased, Minuartia gerardii increased temporarily during the first 10 years, and Sedum atratum reacted unclearly, because it was initially present only in the exclosure plots where it rapidly decreased toward the controls.
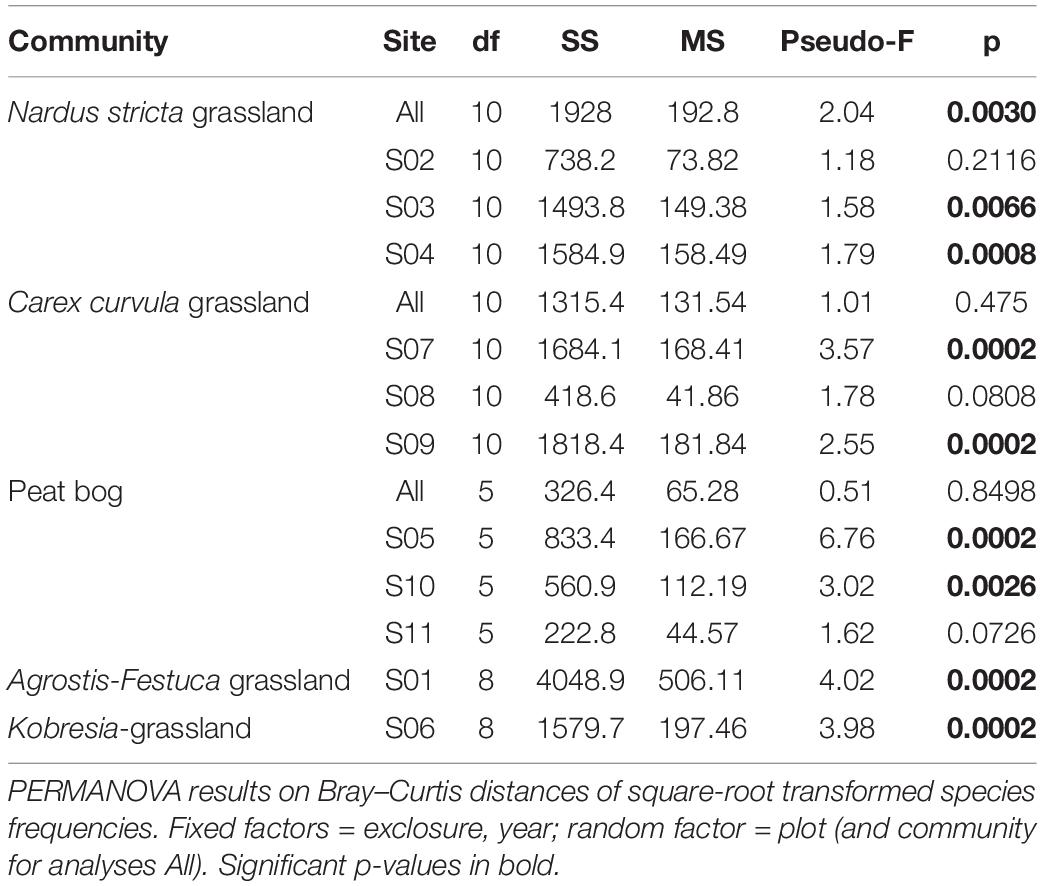
Table 4. Exclosure effects (exclosure × year interaction) on species composition of the communities and single sites.
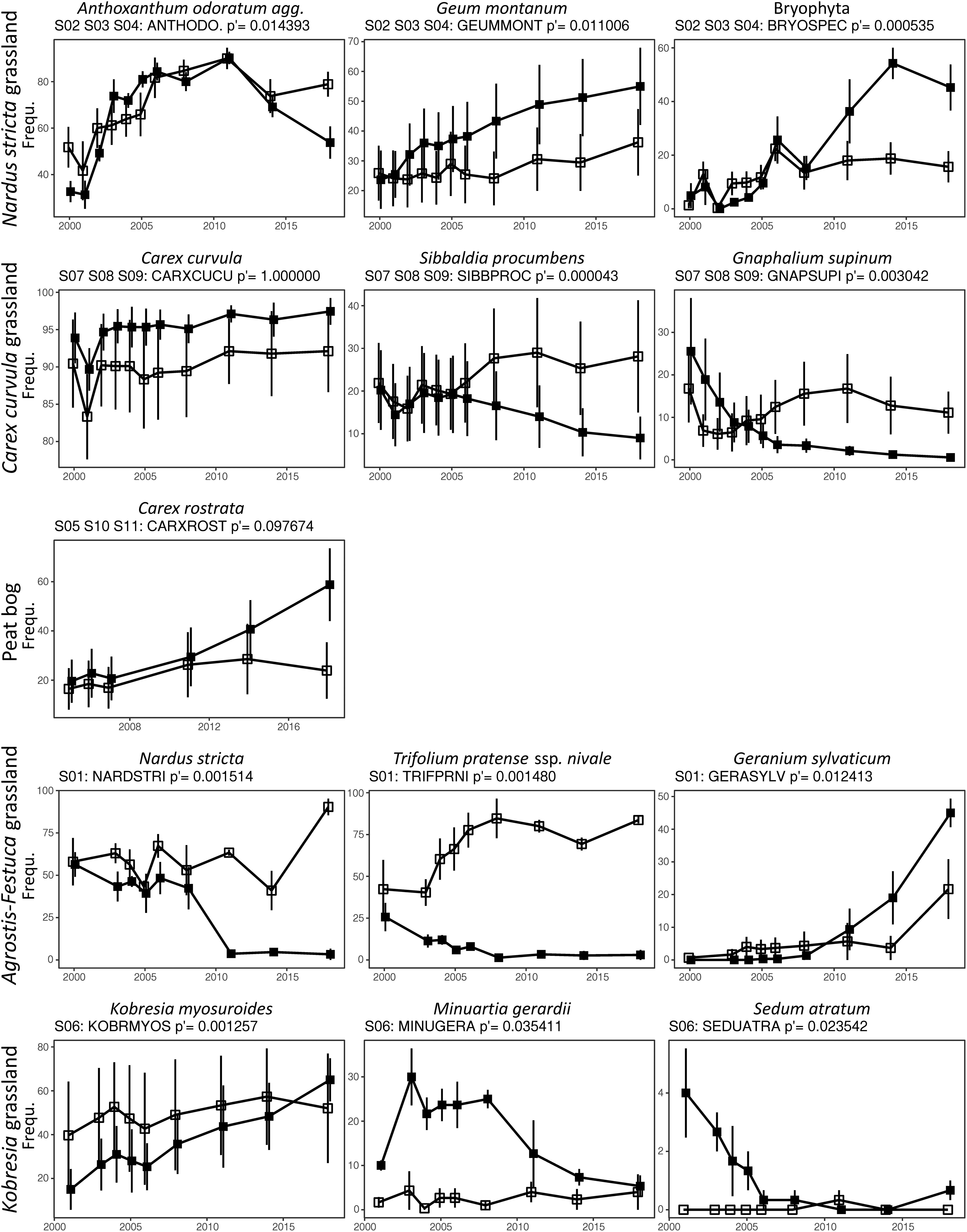
Figure 4. Grazing effects on species frequency (tested by mixed-effect models, Bonferroni-corrected p < 0.05 for the exclosure × year interaction) for the different communities. Means ± S.E. for exclosure plots (filled squares) and grazed control plots (open squares).
Environmental Effects
Increases of temperature at Obergurgl from 1999 till 2018 ranged from 0.03 to 0.08°C per year for the different quartals. The weather effects on the community parameters proved to be highly significant and mostly consistent across the communities (pRDA with site as covariate, p = 0.0002). Interestingly, the sunshine duration of the previous autumn quartal (p = 0.003) had by far the highest explanatory power (11.8% explained variation) and defined the first pRDA axis, which was highly correlated with total frequency, bryophytes, competitive and stress-tolerant species, and to a lesser degree diversity (Figure 5; see also Supplementary Material 6). Summer precipitation of the previous year and spring/summer temperatures were also positively correlated with this axis. Precipitation and temperature of the previous autumn quartal (p = 0.003) were negatively correlated. For the exclosure sites, we also tested if the weather response was affected by grazing exclusion. Indeed, there was a small but significant (p = 0.0002) effect, with a slightly attenuated (and altered) response to sunshine duration of the previous autumn quartal in the fenced plots (Supplementary Material 7).
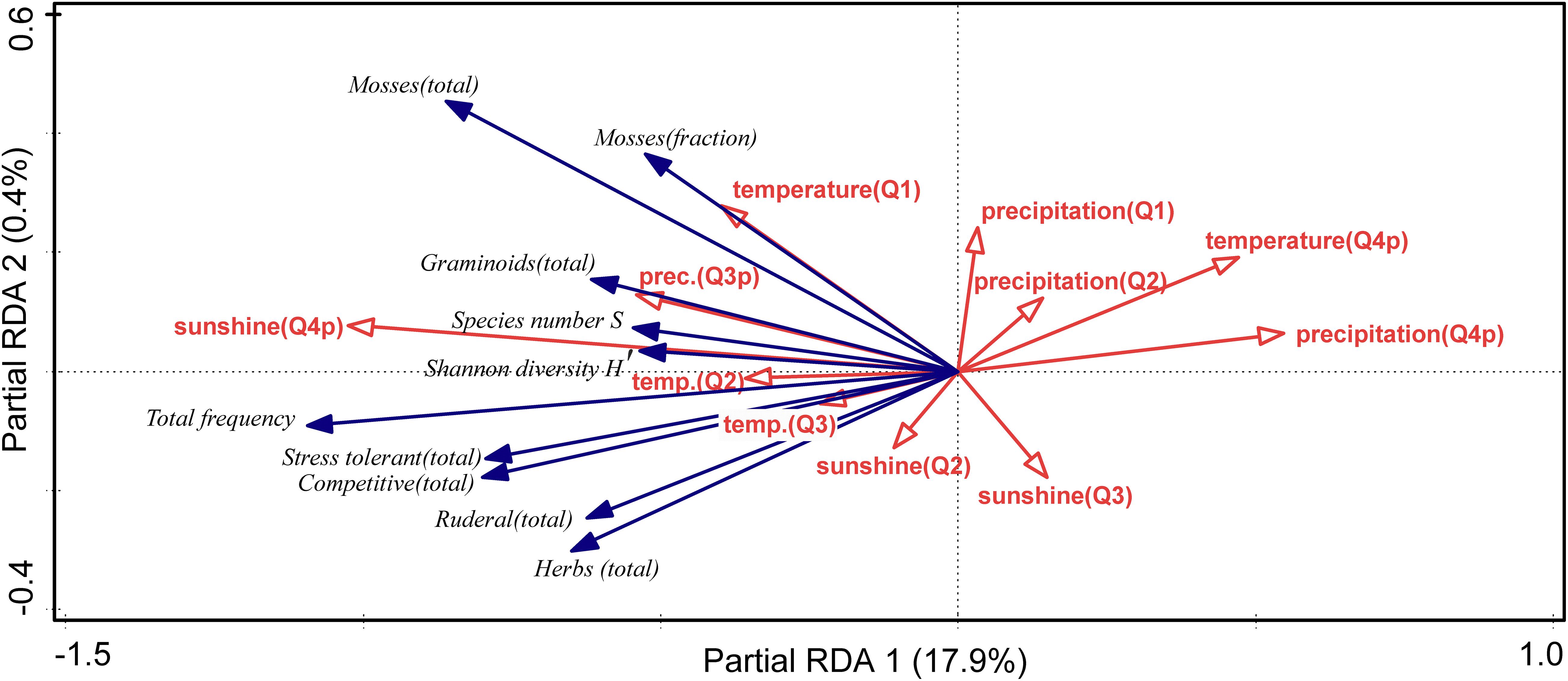
Figure 5. pRDA showing the environmental effects on diversity (S, H′), total frequency, functional groups, and strategy types of the grazed sites (total frequencies and fractions indicated for the latter). Explanatory weather variables (sunshine duration, temperature, precipitation) shown with quartals in brackets (Q1 Jan–Mar, Q2 Apr–Jun, Q3 Jul–Sep, Q3p Jul–Sep previous year, Q4p Oct–Dec previous year). Partial RDA (site as covariate) on all community parameters (variables with fit > 5% shown), explained variation of axes indicated, p = 0.0002.
Discussion
Diversity Changes in Grazed Communities
The most consistent result was that species diversity (species number, Shannon index) and species frequencies increased significantly with time at all grazed communities. Thus, hypothesis 1 can be confirmed. Primarily, bryophytes increased significantly in the course of 18 years (however, they increased also in the exclosures, see section “Discussion” below). This is in line with long-term observations in the alpine vegetation of Dovrefjell, Norway (Vanneste et al., 2017). The benefit for bryophytes from grazing was already highlighted by several other authors (Väre et al., 1996; Austrheim and Eriksson, 2001; Takala et al., 2014), showing that grazers promote certain bryophytes by creation of gaps.
Lichens—as a functional group—were not affected throughout the years in our study area, maybe due to a rather low importance in most of the investigated communities. This holds also for dwarf shrubs. At arctic sites, lichen richness was found to decline due to increased shading by dwarf shrubs and shrubs (Elmendorf et al., 2012; Vanneste et al., 2017) and litter increase (Cornelissen et al., 2001) as a consequence of climate warming.
The overall increase in species numbers in the grazed plots is in line with the accelerated species increase at high elevations in Europe (Steinbauer et al., 2018), as an effect of the ongoing climate warming. The lowest changes in diversity throughout the years occurred at S15 (prostrate dwarf shrub) and S11 (wettest site of the peat bog). At the prostrate dwarf shrub, obviously hardly any microsites for seedling recruitment (Graae et al., 2011) are present and sheep grazing also seems to have no influence on this Loiseleuria procumbens lichen heath, the dense carpet obviously preventing invasions of other species. This might be an example of “community-level resistance,” defined by Hudson and Henry (2010). Similarly, also the calcareous Kobresia grasslands showed low diversity changes. This result is comparable to the low effects in subalpine calcareous grasslands in the Apennines found by Frate et al. (2018). At the peat bog site S11, the canopy of very few species remained stable throughout time. Grazers almost avoid this site due to the high water level and unpalatable species.
No Increase of Competitive Species
Hypothesis 2 suggested that competitive strategists and species with high temperature and/or nitrogen values should increase at the grazed sites due to climate change effects. Although the absolute frequency number of all the three C-S-R strategy types increased at all grazed communities, the relative changes did not really support our hypothesis. The fraction of competitors slightly decreased at most of the grazed communities (except the peat bog, Figure 1) or did not change at all. The climate change scenarios suggest an increase of competitive, tall-growing species. This was not the case on our grazed communities. Similar to the competitors, the stress tolerators showed no uniform response in our study; only at the Agrostis–Festuca and the Nardus stricta grassland was this group reduced. In contrast, ruderals increased slightly, though not significantly. Our finding may be a clear indication of rather stable species composition and vegetation structure, probably due to long-term evolution of grazing in the area, grazing lasting already more than 6,000 years (Vorren et al., 1993; Bortenschlager, 2000).
For the Landolt temperature values, an increase was predicted due to the ongoing climate warming. However, also here rather divergent results were obtained. The findings suggest that hardly any species from lower elevations (having higher temperature values) invaded the grazed areas of the lower and upper alpine grasslands. The reaction of the light values seems to follow a decreasing trend (except at the dwarf shrub communities and Kobresia grasslands). This might be explained by the increasing cover and canopy height in the plots (Mayer and Erschbamer, 2014, 2017), promoting shade-tolerant species. Remarkable was the increasing amount of nitrogen-demanding species at the subalpine Agrostis–Festuca grassland and the changes in soil reaction values. Here, the grazed control plots were fertilized during the first years of the monitoring period, without our knowledge, by the owner of the cattle (Mayer et al., 2009). This might explain the surprising increases of nitrogen and soil reaction values. Unfortunately, we have no data on atmospheric N-deposition and amount of fertilizers spread. On the other hand, Evangelista et al. (2016) also found an increase in N-demanding species in the Apennines at 2,400–2,790 m a.s.l. after four decades.
Decreasing Diversity in Grazing Exclusions
With hypothesis 3, we predicted a decreasing diversity in grazing exclusion plots. While this can clearly be confirmed (i.e., species number S and Shannon index H′) when analyzed across the communities, the effect did not consistently occur at all sites. However, at 8 sites out of 11 there was a strong response of the entire species community (Table 4). This is by far a higher response compared to the results of the long-term study by Pardo et al. (2015) in the Pyrenees. There, two decades of grazing exclusion did not result either in diversity changes or in changes of the community structure. The authors explained the missing response mainly by (i) the absence of dwarf shrubs and trees in the surrounding, (ii) the predominance of long-lived alpine species, and (iii) possibly depletion of the species pool due to intensive grazing throughout the last centuries.
Considering the functional groups at our study sites, the communities showed a rather heterogeneous behavior: at the Nardus stricta grassland, mostly graminoids were negatively affected, at the Carex curvula grasslands mostly herbs, and at the Agrostis–Festuca grassland both. Contrasting responses of functional groups were highlighted also by Austrheim et al. (2008) in southern Norway, and the authors suggested that individual plant life histories should be known to evaluate the results. Nardus stricta is more or less avoided by grazers and may largely extend in extensively grazed areas at the treeline and in the lower alpine zone (e.g., Schaminée and Meertens, 1992). This resulted also by modeling future conditions (Dibari et al., 2020). In our sites, Nardus stricta significantly increased in most of the grazed plots, but decreased in the subalpine Agrostis–Festuca exclosure as well as at its typical grassland exclosures.
Graminoids were not affected in the Carex curvula grassland. Carex curvula is known to be highly persistent (Grabherr, 1989), lacking moderate grazing effects (Schneiter, 1977; Cernusca, 1989; Erschbamer et al., 2003). Here, the most striking indicator species for the grazing exclusion effects were snow bed species (Sibbaldia procumbens, Gnaphalium supinum). This might be an alarming signal, as snow bed species are also among the highest threatened species due to climate warming (Matteodo et al., 2016; Liberati et al., 2019). Also in the arctic tundra, herbivore removal caused increasing losses of ‘short-statured’ species (Kaarlejärvi et al., 2017).
At the subalpine Agrostis–Festuca grassland, Geranium sylvaticum became dominant throughout the years in the exclosure. The species is known as tall herb, selectively grazed by sheep (Evju et al., 2006). The developing dominance of tall-growing herbs following grazing exclusion parallels mowing cessation effects (Bohner et al., 2020), i.e., land-use abandonment in general provokes a remarkable decrease in species richness and Shannon index. This conclusion is not new: several authors highlighted the role of grazing (or mowing) in preserving grasslands and enhancing species diversity (Olff and Ritchie, 1998; Adler et al., 2001; Austrheim and Eriksson, 2001; Dupré and Diekmann, 2001; Pavlu et al., 2005; Sebastià et al., 2008; Mayer and Erschbamer, 2017 and citations therein; Wang et al., 2017; Perotti et al., 2018). With the selection of tall-growing species and their biomass accumulation, microsites for seedling recruitment may become rare on such sites (Graae et al., 2011).
The increase of bryophytes also within the exclosure plots may be attributed to enhanced shading effects by a remarkably increased canopy height due to grazing exclusion (Mayer and Erschbamer, 2017). Generally, bryophytes benefit from grazing (as mentioned above); however, canopy structure changes due to grazing exclusion led to similar frequency increases as in the grazed plots.
Environmental Factors Govern Diversity Changes
Hypothesis 4 postulated that environmental factors drive diversity changes. At our study site, a steady increase in temperature was observed (Fischer, 2010; Kuhn et al., 2013). Generally, temperature was identified as the major climatic factor, driving vegetation changes in high elevations (Körner, 2003; Gottfried et al., 2012). Indeed, temperatures of the spring and summer quartals were positively correlated with total frequency, species number, and Shannon index, while precipitation of the winter and spring quartals as well as precipitation and temperature of the autumn quartals of the previous year were negatively correlated. Additionally, water deficit in warmer summers might increase, and together with elevated N deposition, these factors might be responsible for shifts of grassland communities (Wüst-Galley et al., 2020). Sunshine during winter (quartal 4) was the weather variable with the strongest steady increase, making it the dominating predictor in our study. We had expected more influence of the variables temperature and precipitation, but this could not be confirmed.
Interaction of Climate Warming and Grazing Effects
According to Speed et al. (2011, 2012), grazing exclusion may provoke upward shifts of species, especially advances of the treeline. Such signals were hardly detected at our study area. Only one tree sapling—Pinus cembra—newly appeared at one plot (Figure 2), although not within an exclosure. The distribution range of this tree is highly depending on the bird Nucifraga caryocatactes (spotted nutcracker) and its caching activity (Neuschulz et al., 2017). The nutcrackers hardly perform seed caching above the actual treeline due to deep snow (Mattes, 1982) or the lack of landmarks at high elevations (Neuschulz et al., 2017). Our results, showing missing shrub and tree invasions within exclosures are comparable to land-use changes in the Eastern Alps: formerly mown grasslands exhibited no invasion of shrubs and trees even after 60 years of abandonment (Bohner et al., 2020). Missing effects despite climate warming and grazing such as in our study area are known also from the LTER site Niwot Ridge (Scharnagl et al., 2019). In contrast, experiments (Alexander et al., 2015) demonstrated that lowland competitors might outperform alpine plants. At our study site, no montane or colline-centered species were recorded throughout the 18 years of observation. All newcomers and species with increasing frequency belonged to the treeline and alpine species pool.
Long-term processes caused by climate warming and/or grazing exclusion may interact and are difficult to disentangle. Major changes due to climate warming can be weakened or completely overruled by high grazing intensity (Speed et al., 2012, 2013; Jäschke et al., 2018). In contrast, continuous low-intensity grazing may retard or even inhibit community changes (Speed et al., 2013; Wang et al., 2019). Pardo et al. (2015) recorded low impacts of grazing exclusion on species richness but highlighted synergistic effects with extreme weather events such as dry and warm periods. Our study was not conclusive in this respect, but we found an indication of slightly reduced and altered weather effects under grazing exclusion. Our sites generally exhibited a relatively low grazing intensity, as the grazers forage large areas with exception of the subalpine Agrostis–Festuca grassland, where six cattle per 1 ha graze from the beginning of July to the end of August each year, provoking a higher grazing pressure (Mayer et al., 2009; Mayer and Erschbamer, 2017).
The problem of disentangling climate change and grazing exclusion effects is an aspect which certainly has to be analyzed in more detail. For a more stringent interpretation, data of the local conditions per individual site would be helpful and a much higher sampling intensity would be necessary.
Shortcomings of the Study
Long-term monitoring studies are expected to give comprehensive answers to research questions such as effects of climate or land-use changes (Evangelista et al., 2016; Frate et al., 2018; Scharnagl et al., 2019). However, even in long-term studies we have to deal with directional and non-directional changes over time as well as plot variability (Spasojevic et al., 2013), exacerbating a clear interpretation. Initial differences between exclosure and control plots often aggravated the interpretation, the effects being very small in comparison to general changes over time and to site differences.
Moreover, we are aware of several limitations of the study design. First, replications of exclosures at the subalpine Agrostis–Festuca grassland and the upper alpine Kobresia grassland as well as at the grazed Festuca nigrescens grassland are missing due to local circumstances. Second, due to technical and financial problems, monitoring was not equal for all sites and years. Third, the uneven number of replicated permanent plots in the grazed and exclosure/control sites, respectively, might limit the strength of our results for species numbers.
Conclusion
Diversity increases in grazed subalpine–alpine–subnival plant communities in the Central Austrian Alps were attributed to the ongoing climate change. During the 18 years of investigation, the communities were not invaded by species from low elevations; the newcomers mainly belonged to the treeline and alpine species pool. The investigated sites were not affected by the increase of competitive species with exception of the subalpine Agrostis–Festuca exclosure. Here, grazing exclusion lead toward a tall-forb community. The missing increase of competitive species in all other sites may be attributed to the typical vegetation structure of alpine communities where already highly competitive species predominate as residents, such as Kobresia myosuroides in the subnival grasslands, Carex curvula in the upper alpine grassland, Nardus stricta in the lower alpine grassland, Vaccinium spp. in the tall dwarf shrub, or Loiseleuria procumbens in the prostrate dwarf shrub. In the Carex curvula grasslands, snow bed species decreased within the exclosures. Cessation of grazing might multiply the threat of this species group as it was found to be also endangered by climate warming.
An indication of slightly reduced and altered weather effects under grazing exclusion was found. The interaction of climate change and grazing is not easy to disentangle. However, this topic definitely should be considered for predictions of community shifts in the future.
Data Availability Statement
The original contributions presented in the study are included in the article/Supplementary Material, further inquiries can be directed to the corresponding author/s. The raw data are included in the GRASSPLOT database (https://edgg.org/databases/Grassplot).
Author Contributions
RK and BE designed the study. RM set up the exclosures and carried out the field work. NS provided equipment and set up the exclosures during the last years. RK analyzed the data. BE wrote the drafts of the manuscript. All authors contributed to the final version.
Funding
The first years of the study were financially supported by the Environment Department of the Tyrolean Federal State Government. We highly acknowledge the funding by the Austrian Academy of Science (ÖAW, MAB Programme), the Mountain Agriculture Research Unit of Innsbruck University, and the Alpine Research Centre Obergurgl (AFO). Publication costs were thankfully covered by the publication fund of the University of Innsbruck and by AFO.
Conflict of Interest
The authors declare that the research was conducted in the absence of any commercial or financial relationships that could be construed as a potential conflict of interest.
Acknowledgments
We are grateful to the Zentralanstalt für Meteorologie und Geodynamik (ZAMG, Christian Posch) for providing the weather data of Obergurgl. Thanks to the AFO for the overall support.
Supplementary Material
The Supplementary Material for this article can be found online at: https://www.frontiersin.org/articles/10.3389/fevo.2021.640103/full#supplementary-material
Footnotes
References
Adler, P. B., Raff, D. A., and Lauenroth, W. K. (2001). The effect of grazing on the spatial heterogeneity of vegetation. Oecologia 128, 465–479. doi: 10.1007/s004420100737
Alexander, J. M., Chalmandrier, L., Lenoir, J., Burgess, T. I., Essl, F., Haider, S., et al. (2018). Lags in the response of mountain plant communities to climate change. Glob. Chang. Biol. 24, 563–579. doi: 10.1111/gcb.13976
Alexander, J. M., Diez, J. M., and Levine, J. M. (2015). Novel competitors shape species’ responses to climate change. Nature 525, 515–518. doi: 10.1038/nature14952
Anderson, M. J., Gorley, R. N., and Clarke, K. R. (2008). PERMANOVA+ for PRIMER: Guide To Software And Statistical Methods. Plymouth UK: PRIMER-e Ltd.
Austrheim, G., Mysterud, A., Pedersen, B., Halvorsen, R., Hassel, K., and Evju, M. (2008). Large scale experimental effects of three levels of sheep densities on an alpine ecosystem. Oikos 117, 837–846. doi: 10.1111/j-2008.0030-1299.16543.x
Austrheim, G., and Eriksson, O. (2001). Plant species diversity and grazing in the Scandinavian mountains – patterns and processes at different spatial scales. Ecography 24, 683–695. doi: 10.1111/j.1600-0587.2001.tb00530.x
Bohner, A., Karrer, J., Walcher, R., Brandl, D., Michel, K., Arnberger, A., et al. (2020). Ecological responses of semi-natural grasslands to abandonment: case studies in three mountain regions in the Eastern Alps. Folia Geobot. 54, 1–15. doi: 10.1007/s12224-019-09355-2
Bortenschlager, S. (2000). “The Iceman’s environment,” in The Iceman And His Natural Environment: Palaeobotanical Results. Vol 4, eds S. Bortenschlager and K. Oeggl (Wien, NY: Springer), 11–24.
Cernusca, A. (1989). “Ökosystemforschung in den österreichischen Zentralalpen (Hohe Tauern),” in Struktur und Funktion von Graslandökosystemen im Nationalpark Hohe Tauern. ed. A. Cernusca (Innsbruck: Univ. Verlag Wagner, Veröff. Österr. MaB-Progr 13), 549–568.
Clarke, K. R., and Gorley, R. N. (2015). PRIMER v7: User Manual/Tutorial. Plymouth UK: PRIMER-e Ltd.
Cornelissen, J. H. C., Callaghan, T. V., Alatalo, J. M., Michelsen, A., Graglia, E., Hartley, A. E., et al. (2001). Global change and arctic ecosystems: is lichen decline a function of increases in vascular plant biomass? J. Ecol. 89, 984–994. doi: 10.1111/j.1365-2745.2001.00625.x
Czortek, P., Eycott, A. E., Grytnes, J.-A., Delimat, A., Kapfer, J., and Jaroszewicz, B. (2018). Effects of grazing abandonment and climate change on mountain summits flora: a case study in the Tatra Mts. Plant Ecol. 219, 261–276. doi: 10.1007/s11258-018-0794-6
Dibari, C., Costafreda-Aumedes, S., Argenti, G., Bindi, M., Carotenuto, F., Moriondo, M., et al. (2020). Expected changes to alpine pasture in extent and composition under future climate conditions. Agronomy 10:926. doi: 10.3390/agronomy10070926
Dullinger, S., Dirnböck, T., and Grabherr, G. (2003). Patterns of shrub invasion into high mountain grasslands of the Northern Calcareous Alps, Austria. Arct. Antarct. Alp. Res. 35, 434–441.
Dullinger, S., Gattringer, A., Thuiller, W., Moser, D., Zimmermann, N. E., Guisan, A., et al. (2012). Extinction debt of high-mountain plants under twenty-first-century climate change. Nat. Clim. Chang. 2, 619–622. doi: 10.1038/NCLIMATE1514
Dupré, C., and Diekmann, M. (2001). Differences in species richness and life-history traits between grazed and abandoned grasslands in southern Sweden. Ecogr. 24, 275–286. doi: 10.1111/j.1600-0587.2001.tb00200.x
Elmendorf, S. C., Henry, G. H. R., Hollister, R., Björk, R. G., Bjorkman, A. D., Callaghan, T. V., et al. (2012). Global assessment of experimental climate warming on tundra vegetation: heterogeneity over space and time. Ecol. Lett. 15, 164–175. doi: 10.1111/j.1461-0248.2011.01716.x
Erschbamer, B., Virtanen, R., and Nagy, L. (2003). “The impacts of vertebrate grazers on vegetation in European high mountains.” in Alpine Biodiversity in Europe. eds L. Nagy, G. Grabherr, C. H. Körner, and D. B. A. Thompson (Berlin, Heidelberg: Springer-Verlag), 377–396.
Evangelista, A., Frate, L., Carranza, M. L., Attorre, F., Pelino, G., and Stanisci, A. (2016). Changes in composition, ecology and structure of high-mountain vegetation: a re-visitation study over 42 years. AoB Plants 8:plw004. doi: 10.1093/aobpla/plw004
Evju, M., Austrheim, G., Helvorsen, R., and Mysterud, A. (2009). Grazing responses in herbs in relation to herbivore selectivity and plant traits in an alpine ecosystem. Oecologia 161:77. doi: 10.1007/s00442-009-1358-1
Evju, M., Mysterud, A., Austrheim, G., and Okland, R. H. (2006). Selecting herb species and traits as indicators of sheep grazing pressure in a Norwegian alpine habitat. Ecoscience 13, 459–468.
Farrer, E. C., Ashton, I. W., Spasojevic, M. J., Fu, S., Gonzalez, D. J. X., and Suding, K. N. (2015). Indirect effects of global change accumulate to alter plant diversity but not ecosystem function in alpine tundra. J. Ecol. 103, 351–360. doi: 10.1111/1365-2745.12363
Fischer, A. (2010). “Klima und gletscher in obergurgl.” in Glaziale und periglaziale Lebensräume im Raum Obergurgl. eds E.-M. Koch and B. Erschbamer (Alpine Forschungsstelle Obergurgl 1, Innsbruck: innsbruck university press), 53–72.
Fischer, M. A., Oswald, K., and Adler, W. (2008). Exkursionsflora für Österreich, Liechtenstein und Südtirol. Linz: Biologiezentrum der Oberösterr Landesmuseen.
Frate, L., Carranza, M. L., Evangelista, A., Stinca, A., Schaminée, J. H. J., and Stanisci, A. (2018). Climate and land use change impacts on Mediterranean high-mountains vegetation in the Apennines since the 1950s. Plant Ecol. Div. 11, 85–96. doi: 10.1080/17550874.2018.1473521
Gehrig-Fasel, J., Guisan, A., and Zimmermann, N. E. (2007). Tree line shifts in the Swiss Alps: climate change or land abandonment? J. Veg. Sci. 18, 571–582. doi: 10.1111/j.1654-1103.2007.tb02571.x
Gobiet, A., Kotlarski, S., Beniston, M., Heinrich, G., Rajczak, J., and Stoffel, M. (2014). 21st century climate change in the European Alps-A review. Sci. Tot. Env. 493, 1138–1151. doi: 10.1016/j.scitotenv.2013.07.050
Gottfried, M., Pauli, H., Futschik, A., Akhalkatsi, M., Barancok, P., Alonso, J. L. B., et al. (2012). Continent-wide response of mountain vegetation to climate change. Nat. Clim. Chang. 2, 111–115. doi: 10.1038/NCLIMATE1329
Graae, B., Ejrnaes, R., Lang, S., Meineri, E., Ibarra, P., and Bruun, H. (2011). Strong microsite control of seedling recruitment in tundra. Oecologia 166, 565–576. doi: 10.1007/s00442-010-1878-8
Grabherr, G. (1989). On community structure in high alpine grasslands. Vegetation 83, 223–227. doi: 10.1007/BF00031694
Grabherr, G., Gottfried, M., and Pauli, H. (1994). Climate effects on mountain plants. Nature 369:448. doi: 10.1038/369448a0
Gritsch, A., Dirnböck, T., and Dullinger, S. (2016). Recent changes in alpine vegetation differ among plant communities. J. Veg. Sci. 27, 1177–1186. doi: 10.1111/jvs.12447
Gugger, S., Kesselring, H., Stöcklin, J., and Hamann, E. (2015). Lower plasticity exhibited by high- versus mid-elevation species in their phenological responses to manipulated temperature and drought. Ann. Bot. 116, 953–962. doi: 10.1093/aob/mcv155
Holzinger, B., Hülber, K., Camenisch, M., and Grabherr, G. (2008). Changes in plant species richness over the last century in the eastern Swiss Alps: elevational gradient, bedrock effects and migration rates. Plant Ecol. 195, 179–196. doi: 10.1007/s11258-007-9314-9
Hudson, J. M. G., and Henry, G. H. R. (2010). High Arctic plant community resists 15 years of experimental warming. J. Ecol. 98, 1035–1041. doi: 10.1111/j.1365-2745.2010.01690.x
Jäschke, Y., Herberling, G., and Wesche, K. (2018). Environmental controls override grazing effects on plant functional traits in Tibetan rangelands. Funct. Ecol. 34, 747–760. doi: 10.1111/1365-2435.13492
Kaarlejärvi, E., Eskelinen, A., and Olofsson, J. (2017). Herbivores rescue diversity in warming tundra by modulating trait-dependent species losses and gains. Nat. Comm. 8:419. doi: 10.1038/s41467-017-00554-z
Körner, C. H. (2003). Alpine Plant Life: Functional Plant Ecology Of High Mountain Ecosystems. Berlin: Springer.
Kuhn, M., Dreiseitl, E., and Emprechtinger, M. (2013). “Temperatur und Niederschlag an der Wetterstation Obergurgl, 1953 – 2011.” in Klima, Wetter, Gletscher im Wandel. eds E.-M. Koch and B. Erschbamer (Innsbruck: innsbruck university press), 11–30.
Lamprecht, A., Semenchuk, P. R., Steinbauer, K., Winkler, M., and Pauli, H. (2018). Climate change leads to accelerated transformation of high-elevation vegetation in the central Alps. New Phytol. 220, 447–459. doi: 10.1111/nph.15290
Landolt, E., Bäumler, B., Erhardt, A., Hegg, O., Klötzli, F., Lämmler, W., et al. (2010). Flora indicativa. Ökologische Zeigerwerte und biologische Kennzeichen zur Flora der Schweiz und der Alpen. Bern: Haupt Verlag.
Liberati, L., Messerli, S., Matteodo, M., and Vittoz, P. (2019). Contrasting impacts of climate change on the vegetation of windy ridges and snowbeds in the Swiss Alps. Alp. Bot. 129, 95–105. doi: 10.1007/s00035-019-00223-5
Matteodo, M., Ammann, K., Verrecchia, E. P., and Vittoz, P. (2016). Snowbeds are more affected than other subalpine-alpine plant communities by climate change in the Swiss Alps. Ecol. Evol. 6, 6969–6982. doi: 10.1002/ece3.2354
Mattes, H. (1982). Die Lebensgemeinschaft von Tannenhäher und Arve. Birmensdorf: Ber Eidgen Anst Forstl Versuchswesen Nr. 74.
Mayer, R., and Erschbamer, B. (2014). Ongoing changes at the long-term monitoring sites of Gurgler Kamm Biosphere Reserve. Tyrol, Austria. Eco.Mont. 6, 5–14.
Mayer, R., and Erschbamer, B. (2017). Long-term effects of grazing on subalpine and alpine grasslands in the Central Alps, Austria. Basic Appl. Ecol. 24, 1–11.
Mayer, R., Kaufmann, R., Vorhauser, K., and Erschbamer, B. (2009). Effects of grazing exclusion on species composition in high-altitude grasslands of the Central Alps. Basic Appl. Ecol. 10, 447–455. doi: 10.1016/j.baae.2008.10.004
Neuschulz, E. L., Merges, D., Bollmann, K., Gugerli, F., and Böhning-Gaese, K. (2017). Biotic interactions and seed deposition rather than abiotic factors determine recruitment at elevational range limits of an alpine tree. J. Ecol. 106, 948–959. doi: 10.1111/1365-2745.12818
Olff, H., and Ritchie, M. E. (1998). Effects of herbivores on grassland plant diversity. Trends Ecol. Evol. 13, 261–265. doi: 10.1016/s0169-5347(98)01364-0
Pardo, I., Doak, D. F., García-González, R., Gómez, D., and García, M. B. (2015). Long-term response of plant communities to herbivore exclusion at high elevation grasslands. Biodivers. Conserv. 24, 3033–3047. doi: 10.1007/s10531-015-0996-3
Pauli, H., Gottfried, M., Dullinger, S., Abadaladze, O., Akhalkatsi, M., Alonso, J. L. B., et al. (2012). Recent plant diversity changes on europe’s mountain summits. Science 336, 353–355. doi: 10.1126/science.1219033
Pauli, H., Gottfried, M., Reiter, K., Klettner, C., and Grabherr, G. (2007). Signals of range expansions and contractions of vascular plants in the high Alps: observations (1994-2004) at the GLORIA master site Schrankogel. Tyrol, Austria. Glob. Chan. Biol. 13, 147–156. doi: 10.1111/j.1365-2486.2006.01282.x
Pavlu, V., Hejcman, M., Pavlu, L., Gaisler, J., Nežerková, P., and Andaluz, M. G. (2005). Vegetation changes after cessation of grazing management in the Jizerské Mountains (Czech Republic). Ann. Bot. Fen. 42, 343–349.
Perotti, E., Probo, M., Pittarello, M., Lonati, M., and Lombardi, G. (2018). A 5-year rotational grazing changes the botanical composition of sub-alpine and alpine grasslands. Appl. Veg. Sci. 21, 647–657. doi: 10.1111/avsc.12389
R Core Team. (2019). R: A language and environment for statistical computing. Vienna: R Foundation for Statistical Computing.
Schaminée, J. H. P., and Meertens, M. H. (1992). The influence of human activities on the vegetation of the subalpine zone of the Monts fu Forez (Massif Central, France). Preslia. Vol., 64. 327–342. Available online at: http://www.preslia.cz/archive/Preslia_64_1992_327-342.pdf (accessed November 18, 2020).
Scharnagl, K., Johnson, D., and Ebert-May, D. (2019). Shrub expansion and alpine plant community change: 40-year record from Niwot Ridge, Colorado. Plant Ecol. Div. 12, 407–416. doi: 10.1080/17550874.2019.1641757
Schneiter, S. (1977). Die Reaktion eines alpinen Rasens auf das Aussetzen der Beweidung. Diploma Thesis, University of Basel, Switzerland.
Sebastià, M.-T., de Bello, F., Puig, L., and Taull, M. (2008). Grazing as a factor structuring grasslands in the Pyrenees. Appl. Veg. Sci. 11, 215–222. doi: 10.3170/2008-7-18358
Song, S., Zhu, J., Zheng, T., Tang, Z., Zhang, F., Ji, C., et al. (2020). Long-term grazing exclusion reduces species diversity but increases community heterogeneity in an alpine grassland. Front. Ecol. Evol. 8:66. doi: 10.3389/fevo.2020.00066
Spasojevic, M. J., Bowman, W. D., Humphries, H. C., Seastedt, T. R., and Suding, K. N. (2013). Changes in alpine vegetation over 21 years: are patterns across a heterogeneous landscape consistent with predictions? Ecosphere 4, 1–18. doi: 10.1890/ES13-00133.1
Speed, J. D. M., Austrheim, G., and Mysterud, A. (2013). The response of plant diversity to grazing varies along an elevational gradient. J. Ecol. 101, 1225–1236. doi: 10.1111/1365-2745.12133
Speed, J. D. M., Austrheim, G., Hester, A. J., and Mysterud, A. (2012). Elevational advance of alpine plant communities is buffered by herbivory. J. Veg. Sci. 23, 617–625. doi: 10.1111/j.1654-1103.2012.01391.x
Speed, J. D. M., Austrheim, G., Hester, A. J., and Mysterud, A. (2011). Growth limitation of mountain birch caused by sheep browsing at the altitudinal treeline. Forest Ecol. Man. 261, 1344–1352. doi: 10.1016/j.foreco.2011.01.017
Steinbauer, M., Grytnes, J.-A., Jurasinski, G., Kulonen, A., Lenoir, J., Pauli, H., et al. (2018). Accelerated increase in plant species richness on mountain summits is linked to warming. Nature 556, 231–234. doi: 10.1038/s41586-018-0005-6
Takala, T., Tahvanainen, T., and Kouki, J. (2014). Grazing promotes bryophyte species richness in semi-natural grasslands. Ann. Bot. Fenn. 51, 148–160. doi: 10.5735/085.053.0102
Tasser, E., and Tappeiner, U. (2002). Impact of land use changes on mountain vegetation. Appl. Veg. Sci. 5, 173–185. doi: 10.1111/j.1654-109X.2002.tb00547.x
Ter Braak, C. J. F., and Šmilauer, P. (2018). Canoco Reference Manual And User’s Guide: Software For Ordination, Version 5.10. Ithaca: Microcomputer Power.
Un Report (2019). Available online at: https://www.un.org/sustainabledevelopment/biodiversity/ (accessed November 18, 2020).
Väre, H., Ohtonen, R., and Mikkola, K. (1996). The effect and extent of heavy grazing by reindeer in oligotrophic pine heaths in northeastern Fennoscandia. Ecography 19, 245–253. doi: 10.1111/j.1600-0587.1996.tb01251.x
Vanneste, T., Michelsen, O., Graae, B. J., Kyrkjeeide, M. O., Holien, H., and Hassel, K. (2017). Impact of climate change on alpine vegetation of mountain summits in Norway. Ecol. Res. 32, 579–593. doi: 10.1007/s11284-017-1472-1
Vittoz, P., Randin, C., Dutoit, A., Bonnet, F., and Hegg, O. (2009). Low impact of climate change on subalpine grasslands in the Swiss Northern Alps. Glob. Chan. Biol. 15, 209–220. doi: 10.1111/j.1365-2486.2008.01707.x
Vittoz, P., Rulence, B., Largey, T., and Freléchoux, F. (2008). Effects of climate and land-use change on the establishment and growth of cembran pine (Pinus cembra L.) over the altitudinal treeline ecotone in the Central Swiss Alps. Arct. Antarct. Alp. Res. 40, 225–232.. https://www.tandfonline.com/loi/uaar20,Google Scholar
Vorren, K.-D., Mörkved, B., and Bortenschlager, S. (1993). Human impact of the Holocene forest line in the Central Alps. Veg. Hist. Archaeobot. 2, 145–156. doi: 10.1007/BF00198585
Vowles, T., Gunnarsson, B., Molau, U., Hickler, T., Klemedtsson, L., and Björk, R. G. (2017). Expansion of deciduous tall shrubs but not evergreen dwarf shrubs inhibited by reindeer in Scandes mountain range. J. Ecol. 105, 1547–1561. doi: 10.1111/1365-2745.12753
Wang, D.-B., Wang, X.-Y., Wu, Y., and Lin, H.-L. (2019). Grazing buffers the effect of climate change on the species diversity of seedlings in an alpine meadow on the Tibetan Plateau. Ecol. Evol. 9, 1119–1126. doi: 10.1002/ece3.4799
Wang, Y., Heberling, G., Görzem, E., Miehe, G., Seeber, E., and Wesche, K. (2017). Combined effects of livestock grazing and abiotic environment on vegetation and soils of grasslands across Tibet. Appl. Veg. Sci 20, 327–339. doi: 10.1111/avsc.12312
Wieser, G., Oberhuber, W., and Gruber, A. (2019). Effects of climate change at treeline: lessons froms space-for time studies, manipulative experiments, and long-term observational records in the Central Austrian Alps. Forests 10:508. doi: 10.3390/f10060508
Windmaißer, T., and Reisch, C. (2013). Long-term study of an alpine grassland: local constancy in times of global change. Alp. Bot. 123, 1–6. doi: 10.1007/s00035-013-0112-9
Wipf, S., Stöckli, V., Herz, K., and Rixen, C. (2013). The oldest monitoring site of the Alps revisited: accelerated increase in plant species richness on Piz Linard summit since 1835. Plant Ecol. Div. 6, 447–455. doi: 10.1080/17550874.2013.764943
Winkler, D. E., Lubetkin, K. C., Carrell, A. A., Jabis, M. D., Yang, A., and Kueppers, L. M. (2019). “Responses of alpine plant communities to climate warming.” in Ecosystem Consequences Of Soil Warming. ed. J. E. Mohan (London, U.K: Academic Press, Elsevier), 297–346.
Keywords: diversity, environmental factors, exclosures, long term monitoring, plant community
Citation: Kaufmann R, Mayer R, Schallhart N and Erschbamer B (2021) Effects of Climate Change vs. Grazing Exclusion on Species Diversity Over 18 Years Along an Elevation Gradient in the European Alps. Front. Ecol. Evol. 9:640103. doi: 10.3389/fevo.2021.640103
Received: 10 December 2020; Accepted: 10 May 2021;
Published: 07 July 2021.
Edited by:
Veronica Fabiola Briceño Rodriguez, Australian National University, AustraliaReviewed by:
Jianshuang Wu, Institute of Environment and Sustainable Development in Agriculture, Chinese Academy of Agricultural Sciences, ChinaGrégory Loucougaray, Institut National de Recherche pour l’Agriculture, l’Alimentation et l’Environnement (INRAE), France
Copyright © 2021 Kaufmann, Mayer, Schallhart and Erschbamer. This is an open-access article distributed under the terms of the Creative Commons Attribution License (CC BY). The use, distribution or reproduction in other forums is permitted, provided the original author(s) and the copyright owner(s) are credited and that the original publication in this journal is cited, in accordance with accepted academic practice. No use, distribution or reproduction is permitted which does not comply with these terms.
*Correspondence: Brigitta Erschbamer, QnJpZ2l0dGEuRXJzY2hiYW1lckB1aWJrLmFjLmF0