- 1Department of Biology, University of California, Riverside, Riverside, CA, United States
- 2Department of Biological Science, Florida State University, Tallahassee, FL, United States
- 3Experimental Zoology Group, Wageningen University & Research, Wageningen, Netherlands
- 4Department of Biological and Marine Sciences, University of Hull, Hull, United Kingdom
Sexual conflict is caused by differences between the sexes in how fitness is maximized. These differences are shaped by the discrepancy in the investment in gametes, how mates are chosen and how embryos and young are provided for. Fish in the family Poeciliidae vary from completely provisioning eggs before they are fertilized to providing virtually all resources after fertilization via the functional equivalent of a mammalian placenta. This shift in when females provision their young relative to when an egg is fertilized is predicted to cause a fundamental change in when and how sexual conflict is manifested. If eggs are provisioned before fertilization, there should be strong selection for females to choose with whom they mate. Maternal provisioning after fertilization should promote a shift to post-copulatory mate choice. The evolution of maternal provisioning may in turn have cascading effects on the evolution of diverse features of the biology of these fish because of this shift in when mates are chosen. Here we summarize what these consequences are and show that the evolution of maternal provisioning is indeed associated with and appears to govern the evolution of male traits associated with sexual selection. The evolution of placentas and associated conflict does not cause accelerated speciation, contrary to predictions. Accelerated speciation rate is instead correlated with the evolution of male traits associated with sexual selection, which implies a more prominent role of pre-copulatory reproductive isolation in causing speciation in this family.
Introduction
Intergenomic Conflict
Trivers (1974) presented the first formal exposition of intergenomic conflict. He inferred the existence of parent-offspring conflict from the genetic relatedness of mothers and offspring and among siblings. Conflict arises because the quantity of resources that is in the best interest of offspring to get from their mothers is greater than is in the best interest of mothers to give to their offspring. Conflict creates a tug of war in which selection favors maternal control over the allocation of resources to offspring at the same time that it favors offspring who acquire more from their mothers (Haig, 1993).
Trivers (1974) argued that natural selection will favor mothers that invest equally in all offspring because all offspring carry an equal complement of her genes into the next generation. Each offspring shares 100% of its genes with itself, 50% with its mother and 50% with its full siblings. A consequence of these proportions of genes in common with self-versus siblings is that selection favors offspring who are able to glean more resources from their mothers, even at the expense of their mothers and siblings, because the benefit of such selfishness is paired with a cost that is discounted by 50% for the mother and siblings. A corollary of this argument is that polygamy will increase the intensity of the conflict because an individual offspring shares only 25% of its genes with siblings sired by a different father, so the cost associated with selfishness is discounted by 75% rather than 50%.
Parent-offspring conflict can be viewed as a special case of sexual conflict because offspring are surrogates for the paternal genome. The reason each offspring shares only 50% of its genes with its mother and full siblings is that the other 50% is derived from their father. The root of the conflict between the mother and offspring is thus the presence of the paternal genome and what the father's genes gain at the expense of the mother. Trivers (1974) argument has since been expanded in diverse ways. For example, Haig (1990) shows that if offspring differ in quality and the mother is able to assess their quality, then she will maximize her fitness by allocating resources preferentially to those offspring likely to have higher fitness, rather than treat all offspring equally.
How sexual conflict is manifested is influenced by the mode of reproduction (Furness et al., 2015). If reproduction is via broadcast spawning, as in sea urchins, in which multiple individuals release eggs and sperm into the water column, then the main venue for conflict is at the sperm-egg interface. Sperm are under strong selection to be effective at penetrating eggs at the same time that eggs are under strong selection to admit one and only one sperm because polyspermy is lethal to the egg. The rapid evolution of the bindin gene on sperm is a signature of such conflict (Levitan and Ferrell, 2006).
When organisms are mobile, enabling the creation of nesting sites, as in salmon, or participating in amplexus, as in frogs, then the avenues of conflict multiply. Either form of reproduction adds some measure of paternity assurance, enhances the potential for female choice of mates, but also opens the door to male-male competition for access to females and alternative male mating strategies (Gross, 1985; Taborsky et al., 2008). The advent of pre-copulatory sexual selection, in the form of female mate choice and male-male competition, brings with it the evolution of enhanced sexual dimorphism in morphology and behavior (Anderson, 1994; Zeh and Zeh, 2003).
The evolution of internal fertilization adds new dimensions to sexual conflict; males in some lineages evolve ever more elaborate courtship to attract mates or may evolve more elaborate morphology for grasping and inseminating them, countered by female morphology to maintain control (Arnqvist and Rowe, 1995, 2002). We see the advent of an internal struggle between sperm and semen among competing males to gain access to eggs or between the male and female to fertilize eggs and influence the tendency of females to re-mate(Heifetz et al., 2000; Wolfner, 2002; Ram and Wolfner, 2007). Internal fertilization also enhances the potential for cryptic female choice, or the ability of females to influence which sperm fertilize the eggs (Eberhard, 1996). These same conditions apply to species that retain fertilized eggs, then give birth to live born young.
The evolution of matrotrophy, or the continued provisioning of young after the egg is fertilized, expands the arenas in which conflict occurs because the timing of maternal provisioning overlaps with the expression of the paternal genome in the developing embryo (Furness et al., 2015). It is either at this stage, or during post-natal provisioning of young, that Trivers (1974) concept of parent-offspring conflict comes into play. In the case of matrotrophy, prenatal conflict is predicted to be mediated by “chemical means,” such as the secretion of hormones produced by the embryo into the mother's bloodstream that elevate the delivery of nutrients to the baby (Haig, 1993). Manifestation of conflict during post-natal care instead often takes the form of begging behavior or aggression among siblings (Mock and Parker, 1997).
The Viviparity-Driven Conflict Hypothesis
Zeh and Zeh (2000) proposed the viviparity-driven conflict hypothesis (VDCH) to address the evolutionary consequences of the shift from egg laying to live bearing, but their logic applies well to the shift from lecithotrophy to matrotrophy. Their hypothesis addresses how the evolution of maternal provisioning changes how sexual conflict is manifested. Lecithotrophic females make their full investment in offspring before the egg is fertilized. If such a female is to influence who sires her offspring, then she must be choosy about who she mates with. If lecithotrophic females mate with inferior males, they risk squandering their large pre-copulatory investment. Since female appraisal of male quality is based on male behavior and/or phenotype, female choice can lead to the evolution of elaborate male morphology and courtship behavior associated with sexual selection (Arnqvist and Rowe, 2005). In contrast, placental females produce tiny, inexpensive eggs (Pires et al., 2010; Pires and Reznick, 2018). They can reduce the risk of poor mate choice by mating with multiple males, enabling them to influence which sperm fertilize eggs or through post-zygotic mechanisms of sexual selection, such as selective embryo abortion or the differential allocation of maternal resources to embryos based on genotype (Haig, 1990; Zeh and Zeh, 2000, 2001; Crespi and Semeniuk, 2004). This shift in when mates are selected shifts the emphasis from the importance of choosing with whom to mate with to instead mating with multiple males to access genetic diversity in the sperm gene pool. A consequence is that we should see less evidence of sexual selection on male morphology and courtship behavior in species with matrotrophy. Furthermore, Zeh and Zeh (2008) predicted that the evolution of matrotrophy will increase the rate of evolution of post-zygotic reproductive isolation and the rate of speciation. The basis for this prediction is that conflict may be resolved in different ways by different populations. Conflict resolution differs from adaptation because it involves each sex evolving in response to the other in a tit for tat fashion. The paths followed by different populations may thus diverge from one another such that, when the two are brought back into contact, there may be a mismatch in how conflict is resolved. The evidence in support of Zeh and Zeh's hypothesis at the time of writing was based on broad comparisons, such as between vertebrate classes. For example, mammals evolve post-zygotic RI 5–10 times faster than birds or amphibians (Prager and Wilson, 1975; Fitzpatrick, 2004). The rate at which mammals evolve post-zygotic RI depends on the structure of the placenta, possibly as a function of the scope of immunological interaction between mother and fetus (Elliot and Crespi, 2006).
The Fish Family Poeciliidae
The Poeciliidae present special opportunities for learning about how conflict shapes evolution because of the diversity within and among species in male attributes associated with sexual selection, male mating strategies, and the diversity among species in the mode of maternal provisioning (Bisazza, 1993; Pollux et al., 2009, 2014). The males of some species have bright coloration, ornamentation and elaborate courtship behavior (Bisazza, 1993; Bisazza and Pilastro, 1997). The females of some species have matrotrophy in the form of the functional equivalent of a mammalian placenta (Turner, 1937, 1940; Wourms, 1981; Pollux et al., 2014; Olivera-Tlahuel et al., 2019). Furthermore, the Poeciliidae also vary in the presence or absence of superfetation, or the ability to carry multiple broods of young in different stages of development. Superfetation is of interest because its presence is highly correlated with the presence of placentation (Pollux et al., 2014). The VDCH predicts that the evolution of the mode of maternal provisioning will shape the evolution of male attributes associated with sexual selection. We have tested these predictions by characterizing male attributes and female mode of reproduction throughout the family, constructing a DNA-based family tree, then integrating these data to make inferences about the interdependencies between male and female evolution (Pollux et al., 2014; Furness et al., 2019). Specifically, we have asked:
• How often have male attributes associated with pre-copulatory sexual selection evolved?
• How often has the equivalent of a mammalian placenta evolved?
• How often has superfetation evolved?
• How often are these traits lost? Specifically, are there differences in the evolutionary lability of these male and female attributes? If the male attributes are the product of sexual conflict, then this means that there may be tension between natural and sexual selection, making the male attributes as likely to be lost as gained.
• Are there associations between the presence of male attributes associated with pre-copulatory sexual selection and the mode of maternal provisioning? The VDCH predicts that male attributes associated with sexual selection should be concentrated in lineages that lack placentas.
• Are there patterns in the order of evolution of male traits and the mode of maternal provisioning that suggest a cause-and-effect relationship? If maternal provisioning governs the evolution of male attributes, then male attributes associated with pre-copulatory sexual selection are expected to evolve in lineages that lack placentas and, furthermore, the evolution of the placenta should be associated with the subsequent loss of such male attributes if they had been present.
• Finally, is the evolution of a placenta associated with the accelerated evolution of post-copulatory reproductive isolation and speciation, as predicted by the VDCH? Addressing this question invokes deeper issues with regard to what form of reproductive isolation dominates in governing the rate of speciation. It has been argued that pre-copulatory reproductive isolation plays a more important role in speciation than does post-copulatory reproductive isolation (Coyne and Orr, 2004; Grant and Grant, 2008). It has also been argued that sexual selection can accelerate the rate of evolution of pre-copulatory reproductive isolation (Coyne and Orr, 2004). If this is true, then we might instead expect to see higher rates of speciation in lineages with enhanced male traits associated with sexual selection, which may also mean higher rates of speciation in lineages that lack placentas. The situation in this family thus provides the opportunity to discriminate among alternative hypotheses for what form of reproductive isolation governs the rate of speciation.
The summary of results that follows is derived from our prior publications. The female reproductive data on which these analyses are based are entirely our own, generated under a uniform protocol, rather than being derived from the literature. Male data were derived from measurements on preserved specimens, aquarium observations and the literature. Details can be found in the methods and supplementary materials associated with Pollux et al. (2014). In brief, we show that male attributes associated with sexual selection and the equivalent of the mammalian placenta have evolved multiple times within the family, that there is a strong association between the presence of these male attributes and the absence of placentation, and that the evolution of maternal provisioning appears to govern the evolution of male traits, as opposed to the other way around (Pollux et al., 2014; Furness et al., 2019). All of these results are consistent with predictions derived from the VDCH (Zeh and Zeh, 2000). Finally, we show, contrary to Zeh and Zeh's prediction, that it is the presence of enhanced sexual selection, rather than placentation, that is associated with accelerated rates of speciation (Furness et al., 2019).
Sexual Dimorphism
All species in this family are sexually dimorphic in terms of size and morphology, but there is immense variation in the scope and nature of sexual dimorphism (Figure 1) (Bisazza, 1993). In most species, males are smaller than females because male growth rate decelerates dramatically or even ceases at maturity while female growth trajectories continue to follow a normal, decelerating growth trajectory. In a small number of species the largest mature males can be larger than females (Pollux et al., 2014). These are also species in which the males are highly ornamented and have elaborate courtship.
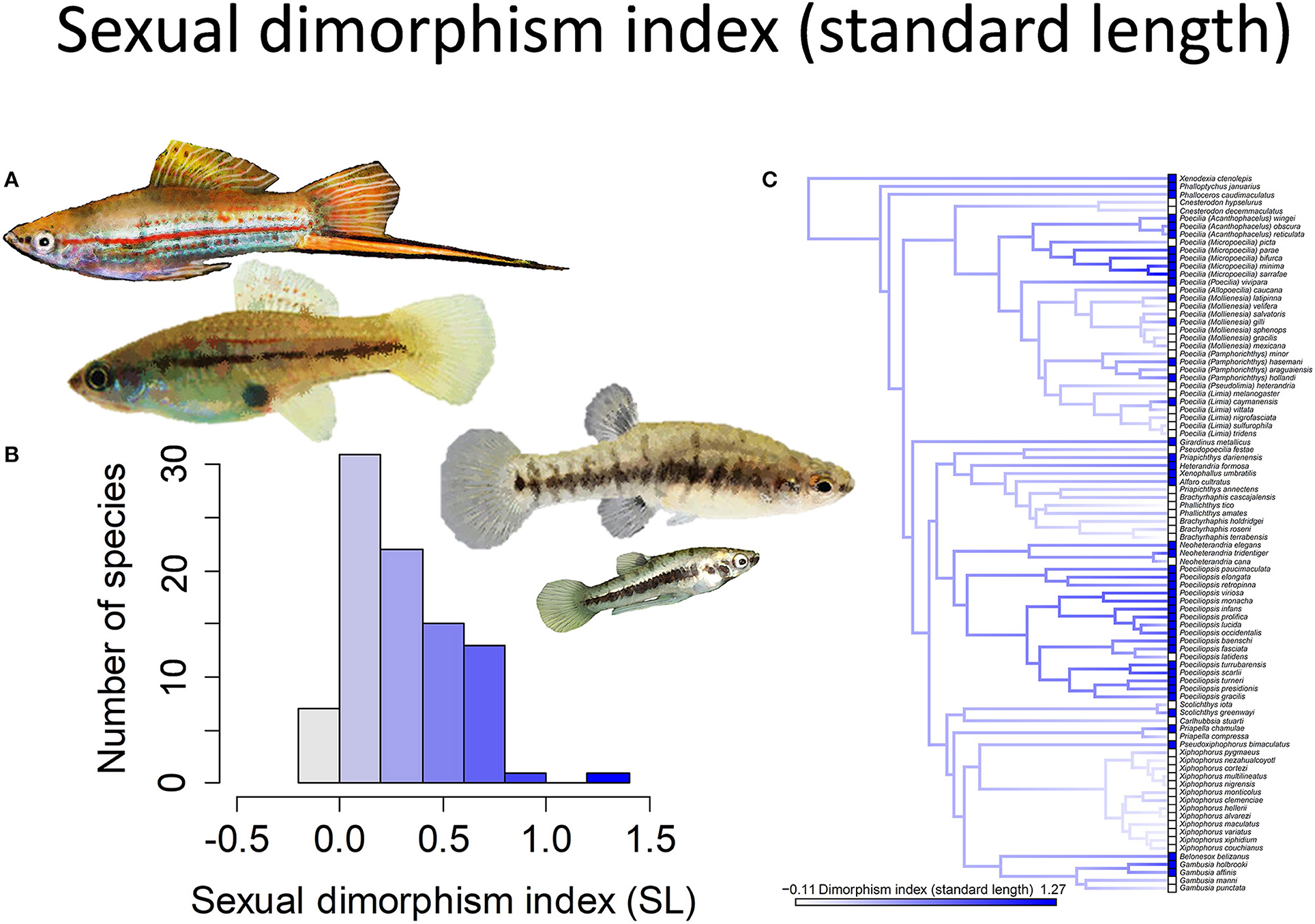
Figure 1. The magnitude of sexual size dimorphism (standard length) in the family Poeciliidae ranges from −0.11 to 1.27. Zero indicates males and females are of equal size, negative values males larger than females, and positive values females larger than males. (A) Xiphophorus hellerii males and females are of approximately equal size (pictured left) while males are considerably smaller than females in Heterandria formosa (pictured right). (B) Frequency histogram of the sexual dimorphism index (n = 90). (C) Ancestral state reconstruction of the sexual dimorphism index as a continuous character using the contMap function in the R package phytools, which estimates the Maximum likelihood ancestral states at all internal nodes and interpolates the states along each edge. The phylogeny tips depict a binary categorization of the size dimorphism index (i.e., low vs. high). The most likely ancestral state of the family, when treated as a binary character, is that of a high degree of size dimorphism (Furness et al., 2019).
The sexes differ in shape because the male anal fin metamorphoses into the gonopodium, or intromittent organ. The anal fin is fan-shaped in females and immature males. As males mature, the third through fifth rays of the anal fin become elongated, the fin stiffens and hooks, barbs and other species-specific morphological features develop at the tip of the gonopodium (Figure 2). At the same time, males develop the gonapodial suspensorium, which is a complex of bones and muscles in the abdomen associated with the mobility of the gonopodium. Males are able to rotate the gonopodium by up to 180 degrees so that it points toward their heads, rather than pointing toward the rear of the body. This change in position enables males to approach and inseminate the female from behind. The body shapes of the males of some species with the longest gonopodia appear modified in a fashion that shifts the insertion of the gonopodium toward the head. It appears that this shift plus the elongation of the gonopodium enables males to see the tip when the gonopodium is rotated 180 degrees to point anteriorly, which could in turn enable them to aim for the females gonopore as they approach them from behind (Figure 3) (Greven, 2005). Females develop an enlarged, unpaired ovary that becomes the site in which offspring develop. The differences between the confinement of the profile of the abdomen by the gonapodial suspensorium vs. the expansion of the abdomen in association with pregnancy creates a difference in the overall shape of males and females (Rosen and Bailey, 1963; Greven, 2005).
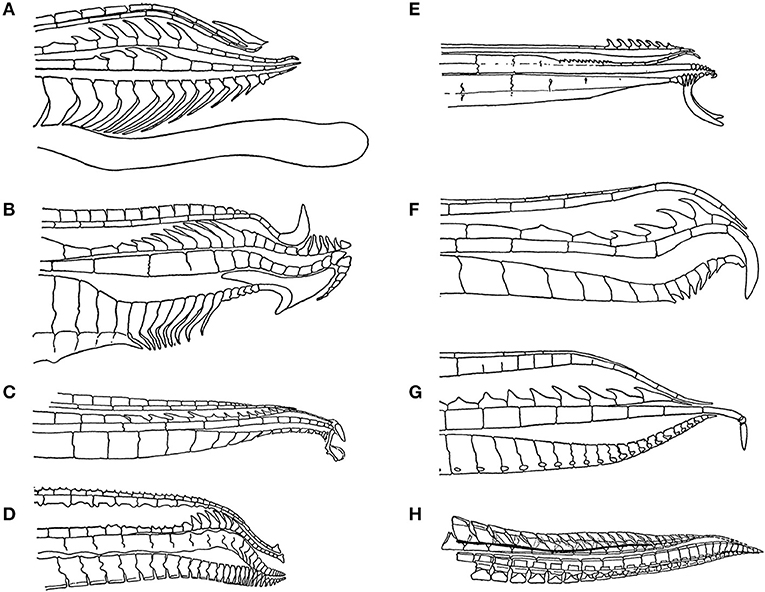
Figure 2. The tip of the male gonopodium, the intromittent organ, exhibits a great deal of variation across the family Poecillidae: (A) Poecilia reticulata, (B) Xiphophorus helleri, (C) Phalloceros caudimaculatus, (D) Belonesox belizanus, (E) Girardinus cubensis, (F) Neoheterandria elegans, (G) Heterandria formosa, and (H) Poeciliopsis elongata. Figures redrawn from Rosen and Bailey (1963).
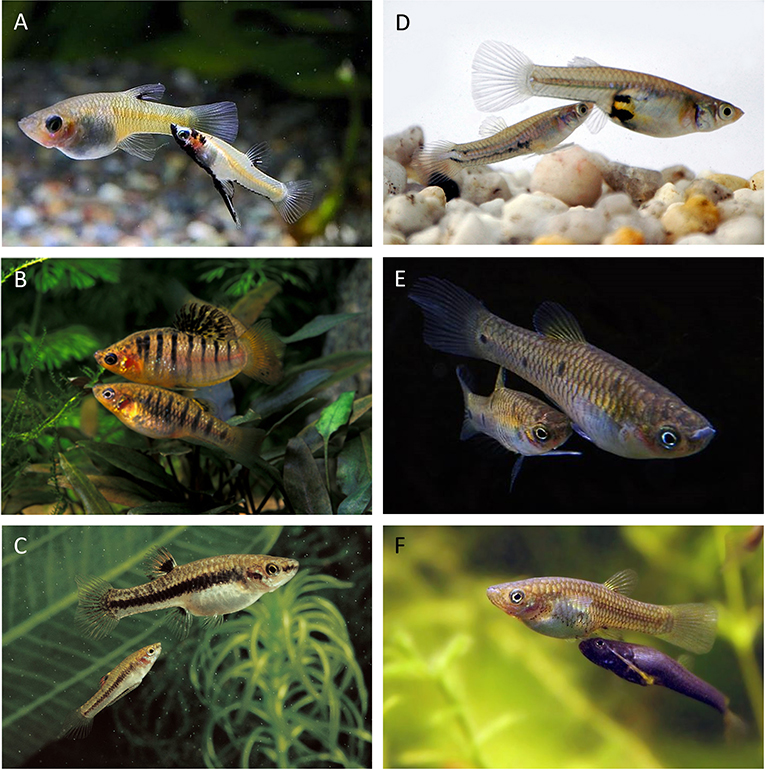
Figure 3. Examples of male mating behavior in different poeciliid species. Courtship in (A) Girardinus metallicus and (B) Limia nigrofasciata. (C) A male Heterandria formosa approaches a female from behind, in this non-courting species. Mating attempts in the form of gonopodial thrusts in (D) Poecilia parae, (E) Phallichthys quadripuctatus, and (F) Poeciliopsis occidentalis. Photo credits: (A) https://acquariofiliaconsapevole.info/pesci_girardinus_metallicus, (B) Katie Holz, (C) TFH Archives, (D) Godfrey Bourne, www.discoverlife.org, (E) http://aquaria.palo-alto.ca.us/, (F) Dennis Caldwell.
Variation Among Males and Associated Female Mating Preferences
In many species, males lack attributes associated with sexual selection. They have the same color pattern as females, lack any form of ornamentation and lack courtship. They tend to have moderately long gonopodia (30+% of the standard body length) and are much smaller than females. In a relatively small subset of species, males develop ornamentation in the form of enlarged dorsal fins, laterally compressed bodies and/or the lengthening of a subset of the caudal fin rays to form a “sword” (Figure 4). Some species also have sexual dichromatism, with males being much more brightly colored than females (Figure 5). Amongst species, the length of the gonopodium ranges from 15 to nearly 50% of the standard length of the body (Figure 6). Langerhans (2011) found, on the basis of a phylogenetic analysis, that genera in which males typically had short gonopodia were more likely to exhibit courtship displays.
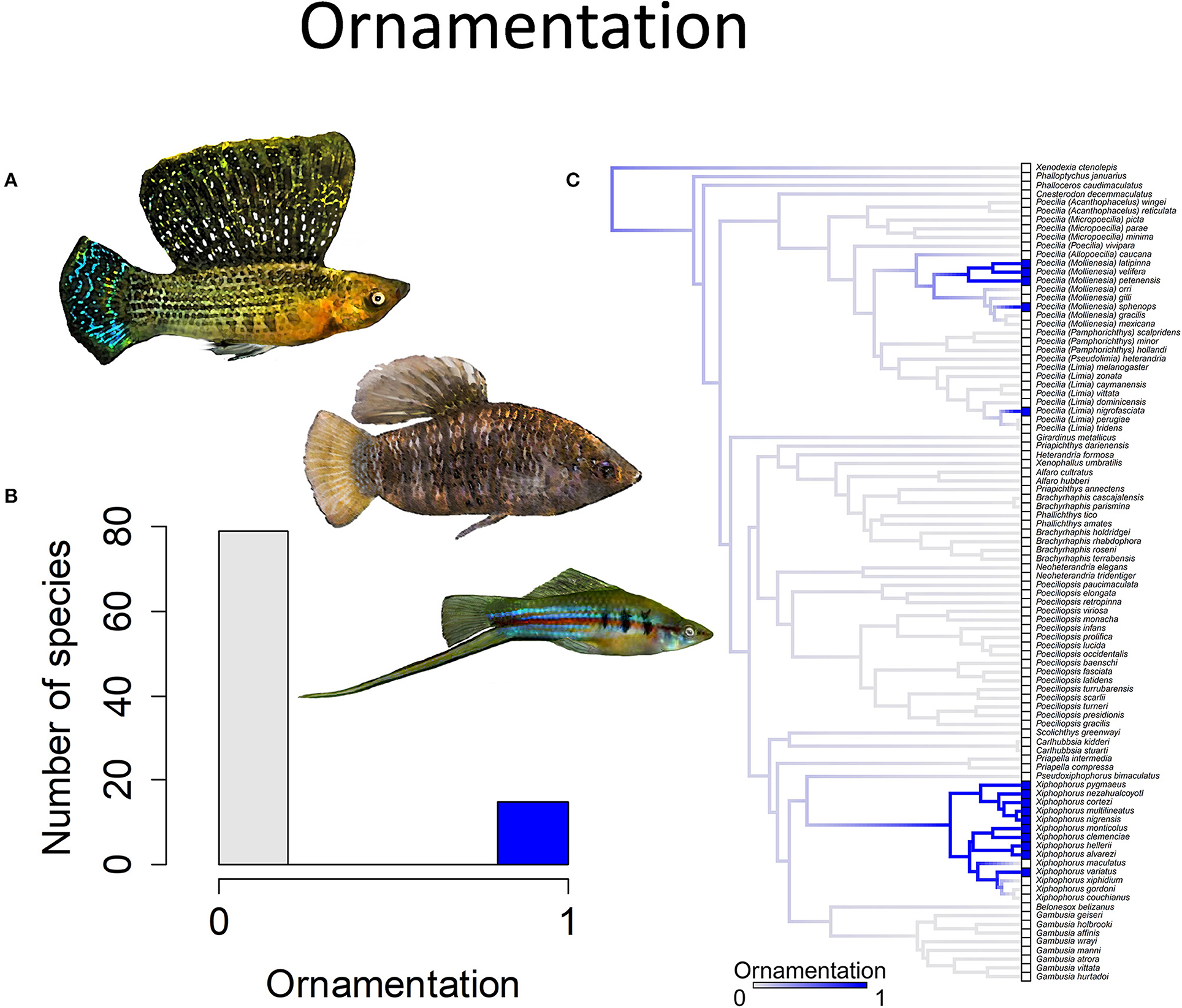
Figure 4. Ornamentation in the family Poeciliidae is when males exhibit ornamental display traits such as (A) a sail-like dorsal fin, as depicted in Poecilia velifera (top) and Limia nigrofasciata (middle), or a sword-like extension of the caudal fin, as depicted in Xiphophorus hellerii (bottom). (B) Frequency histogram of presence or absence of male ornamentation (n = 94). (C) Ancestral state reconstruction of ornamentation as a binary character was performed using stochastic character mapping in the R package phytools. Branch colors represent posterior densities of edge states based on 1000 stochastic character maps of each reconstruction. The phylogeny tips depict a binary categorization of ornamentation (i.e., absence vs. presence). Figure redrawn after Furness et al. (2019). The most likely ancestral state of the family is the absence of ornamentation (Furness et al., 2019).
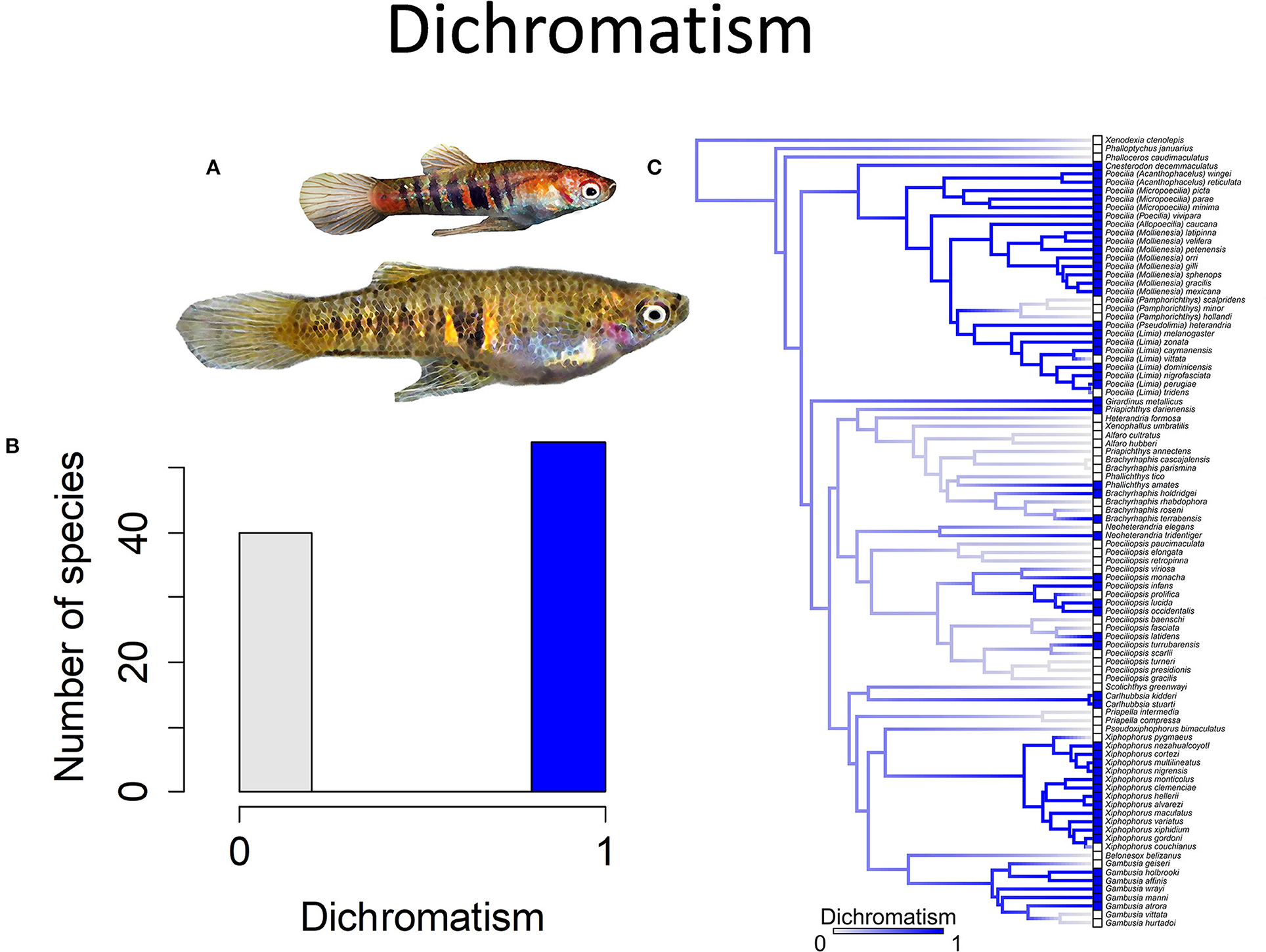
Figure 5. Sexually dichromatic coloration in the family Poeciliidae is when males exhibit brighter and more colorful patterns than females; the alternative is sexually monochromatic coloration in which males and females exhibit similar coloration patterns and intensity. (A) Neoheterandria elegans, exhibits dichromatism with males more brightly colored than females (pictured). (B) Frequency histogram of presence or absence of dichromatism (n = 94). (C) Ancestral state reconstruction of dichromatism as a binary character was performed using stochastic character mapping in the R package phytools. Branch colors represent posterior densities of edge states based on 1000 stochastic character maps of each reconstruction. The phylogeny tips depict a binary categorization of dichromatism (i.e., absence vs. presence). Figure redrawn after Furness et al. (2019). The most likely ancestral state of the family is the absence of dichromatism (Furness et al., 2019).
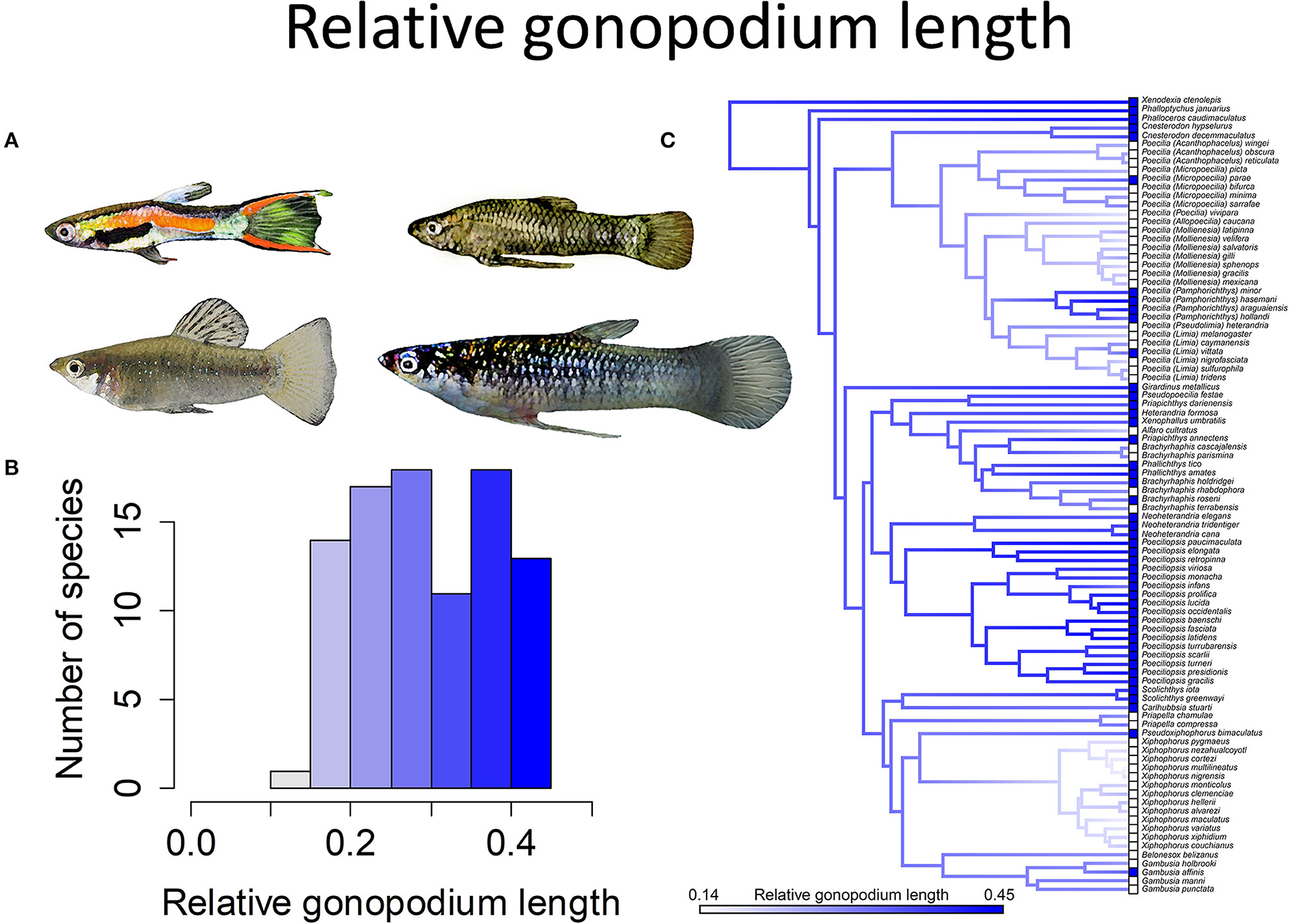
Figure 6. Relative gonopodium length in the family Poeciliidae ranges from 14 to 45% of male standard length. (A) Males of Poecilia wingei and Limia perugiae (pictured left) have relatively short gonopodia while males of Phalloptychus Januarius and Poeciliopsis elongata (pictured right) have relatively long gonopodia. (B) Frequency histogram of male gonopodium length (n = 92). (C) Ancestral state reconstruction of relative gonopodium length as a continuous character using the contMap function in the R package phytools, which estimates the Maximum likelihood ancestral states at all internal nodes and interpolates the states along each edge. The phylogeny tips depict a binary categorization of relative gonopodium length (i.e., short vs. long). The most likely ancestral state of the family, when treated as a binary character, is that of a long gonopodium (Furness et al., 2019).
Species also vary in mating strategies. Most species lack courtship. Males instead approach females from behind and attempt copulation (Figure 7). Some species have elaborate courtship displays, but males of these species often employ alternative reproductive tactics, meaning they may either court or attempt forced copulation. When mature males vary in size, larger males tend to deploy courtship displays while smaller males instead attempt forced copulations (Erbelding-Denk et al., 1994; Rios-Cardenas and Morris, 2011).
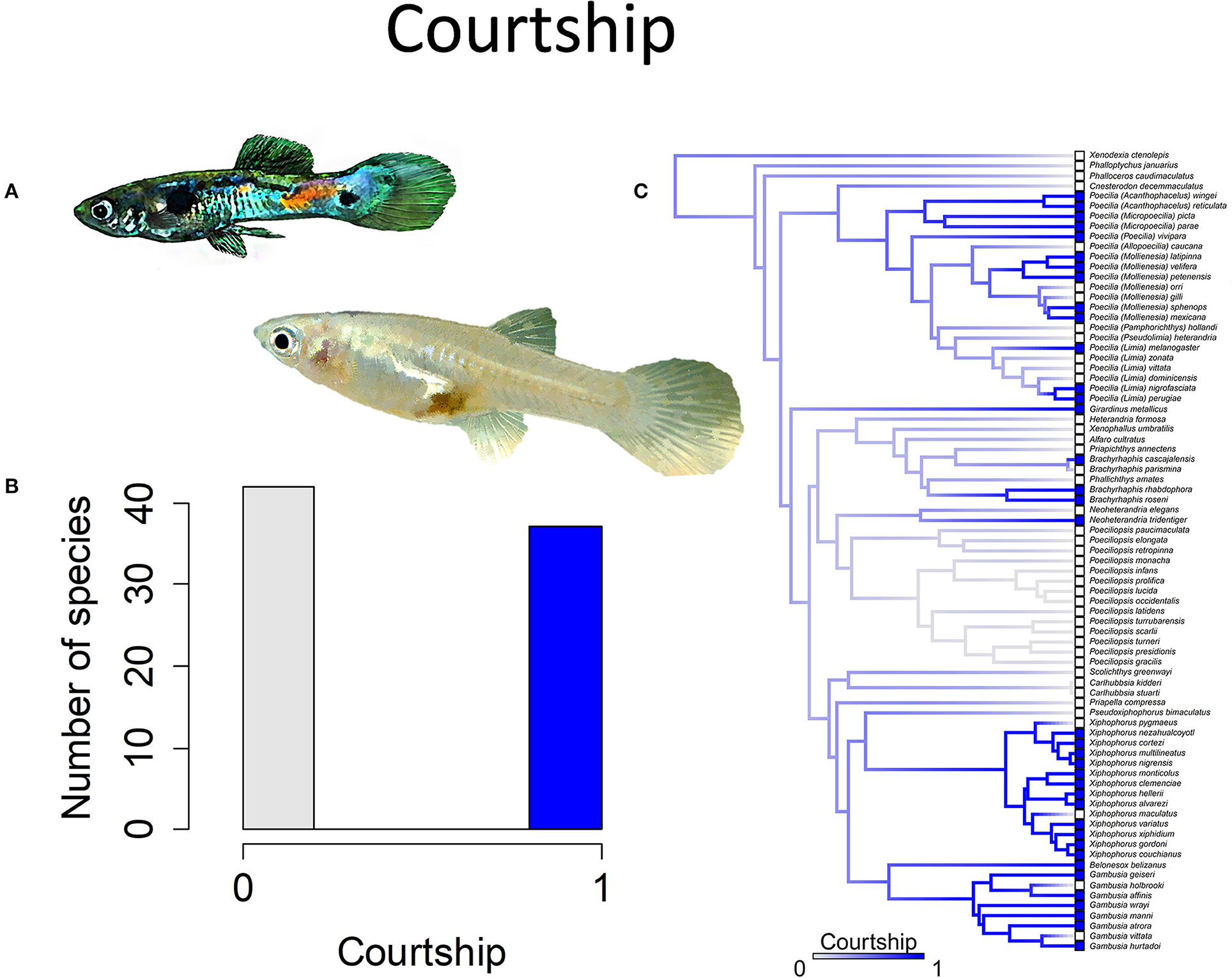
Figure 7. Courtship in the family Poeciliidae involves males displaying for females by spreading their unpaired fins and is often accompanied by stereotypical movements such as a sigmoid posture; the alternative to courtship is coercive or sneak-chase mating. (A) A male guppy, Poecilia reticulata, displays for a female (pictured) to elicit cooperation during mating. (B) Frequency histogram of presence or absence of male courtship (n = 79). (C) Ancestral state reconstruction of courtship as a binary character was performed using stochastic character mapping in the R package phytools. Branch colors represent posterior densities of edge states based on 1000 stochastic character maps of each reconstruction. The phylogeny tips depict a binary categorization of courtship (i.e., absence vs. presence). Figure redrawn after Furness et al. (2019). The most likely ancestral state of the family is the absence of courtship (Furness et al., 2019).
Female mating preferences have been studied in many of these species. In some cases, absolute preference is measured by quantifying the attention given by a female to an individual male (Basolo, 1998), while relative preference is measured by offering females dichotomous choices between live males (Ryan and Wagner, 1987; Erbelding-Denk et al., 1994; Basolo, 1995; Ptacek and Travis, 1997; Morris, 1998), video projections of different male phenotypes (Trainor and Basolo, 2000), or dummies representing different male phenotypes (MacLaren et al., 2004). Relative preference can also be measured by quantifying association patterns among individuals in free-swimming groups (Houde, 1987, 1988b). Despite differences in methods, these studies have demonstrated consistently that females prefer larger, more elaborately ornamented, and more colorful males. Female preferences are strongest when different males are presented simultaneously to a female; when the choices are presented sequentially, the preference for the larger, more ornamental male remains but is much weaker (MacLaren and Rowland, 2006).
Do these preferred males sire more offspring under realistic situations when males can interact with each other as well as with females? Several studies have answered “yes” (Houde, 1988a; Ryan et al., 1990; Kodric-Brown, 1993; Morris et al., 2010). In Limia perguiae, large, preferred males sired all of the offspring in trials in which they were paired with a smaller male but sired no offspring when placed in a group with three smaller males and six females (Erbelding-Denk et al., 1994). It is difficult to interpret these contradictory results because there were few details of the experiment provided in Erbelding-Denk et al. (1994). In particular, it is unclear whether any or all of those females were receptive; male behaviors and interactions among males change dramatically when females are receptive (Farr and Travis, 1986) and only receptive females make clear, decisive choices among males (Ptacek and Travis, 1997). Two studies suggest that preferred males do not always have more reproductive success than other males (Bisazza and Pilastro, 1997; Aspbury and Basolo, 2002). However, these studies quantified only copulations, not the siring of offspring, and both studies focused on species without courtship or male ornamentation.
A number of additional studies have examined female preferences in species without courtship (McPeek, 1992; Basolo, 1998; Bisazza and Pilastro, 2000; MacLaren and Rowland, 2006; Casner et al., 2016). In nearly every case, females prefer a larger, more ornamented male, even when that male is not the same species as the female (Basolo, 1998). Several of these authors have interpreted these results to indicate a pre-existing preference for larger size and ornamentation that males in species with courtship have evolved to exploit.
The size distribution of mature males of many species is often polymodal, highly skewed, or widely variable (Farr et al., 1986; Kolluru and Reznick, 1996; Ptacek and Travis, 1996; Arias and Reznick, 2000; Reznick et al., 2007a; Regus et al., 2013; Cohen et al., 2015; Pires and Reznick, 2018). While this size variation has been associated with species in which males are highly ornamented and different sized males deploy different mating strategies (Zimmerer and Kallman, 1989; Rios-Cardenas and Morris, 2011), our comparative analyses reveal that such variation in male size at maturity is ancestral in the family Poeciliidae (unpublished observation). It is commonly seen in species that lack courtship behavior. In a few species, investigators have found that there is a genetic basis for differences in male size at maturity. The strongest research on the genetics of male size at maturity has been applied to species in the genus Xiphophorus which have a well-described P-locus with alternative alleles that determine age and size at maturity (Kallman, 1989; Lampert et al., 2010). An analogous system of Y-linked inheritance has been reported in sailfin mollies (Travis, 1994).
Variation Among Females
The diversity we see among species in male attributes associated with mating is matched by diversity in female reproductive attributes. The ancestral mode of reproduction is most likely egg retention, meaning that eggs are fully provisioned before fertilization then retained by the female throughout development (Furness et al., 2019). This is the mode of maternl provisioning called “lecithotrophy,” or yolk feeding. From this starting point, some species have acquired two novelties—matrotrophy (placentation) and superfetation.
Morrison et al. (2017) characterized the properties of the eggs of egg-laying Cyprinodont killifish (Nothobranchius fuerzi, Rivulus hartii, Cyprinodon variegatus, plus the Atherinomorpha Oryzias latipes) in the same order as the livebearing poeciliids, in an effort to illuminate the transition from egg laying to egg retention. They found that the egg that was internalized at the inception of livebearing probably already had limited capacity to acquire resources from its mother. They showed that eggs can concentrate radiolabeled amino acids against a concentration gradient, but also that they can absorb fluorescent microspheres. The totality of their results suggest that these substances were acquired from the surrounding medium via a non-specific form of active transport like pinocytosis. If the egg internalized by the ancestral poeciliid shared these properties it means that, from the inception of livebearing, developing embryos had the capacity to acquire resources from their mother. This capacity sets the stage for conflict between embryo acquisition and maternal allocation. As such, it may represent a “preadaptation” for the evolution of matrotrophy as a product of the escalation of this conflict over resource allocation. Since the eggs of other distantly related fish species have been found to have the same ability to acquire small organic molecules from their surroundings (Terner, 1968; Siebers and Rosenthal, 1977) this property may explain why matrotrophy has evolved much more readily in fish than in amniotes; 12 of the 14 lineages of bony fishes that have evolved livebearing also evolved matrotrophy, as compared to only 2–4 of the 115 lineages of squamates that evolved livebearing (Blackburn, 2015).
These results may also explain those of earlier investigators who injected radiolabeled amino acids into pregnant females of lecithotrophic species of Poeciliidae (Marsh-Matthews et al., 2001, 2005; Marsh-Matthews and Deaton, 2006; Riesch et al., 2010). These authors found that the label appeared in developing embryos within hours of the injection. They interpreted the result as evidence for the presence of matrotrophy. Morrison et al. (2017) found that the killifish eggs acquired nutrients from the surrounding environment at a comparable rate to lecithotrophic poeciliid embryos developing inside their mother. By our interpretation, rather than demonstrating matrotrophy, these investigators were instead characterizing a form of active transport retained from the egg-laying ancestors of the Poeciliidae.
Inferences about how mothers provision their young are derived from the patterns of change in the dry mass of the young during development. This pattern of weight change is characterized with the “matrotrophy index” (MI), or the ratio of the estimated mass of offspring at birth divided by the estimated mass of the egg at fertilization. Lecithotrophic species have embryos that lose dry mass over the course of development. The embryos of matrotrophic species instead gain dry mass during development. The modal value for MI in the family is ~0.7 (Pollux et al., 2009), implying that the embryos lose 30% of their dry mass during the course of development. This is comparable to the mass difference between a newly laid bird egg and the mass of the hatching that emerges (Sotherland and Rahn, 1987), hence the inference that it represents eggs that were fully provisioned prior to fertilization. There is then a continuous distribution of greater MI values, with the tail of the distribution extending to values of >100, which means that the mass of the offspring at birth is more than 100 times the mass of the egg at fertilization (Figure 8).
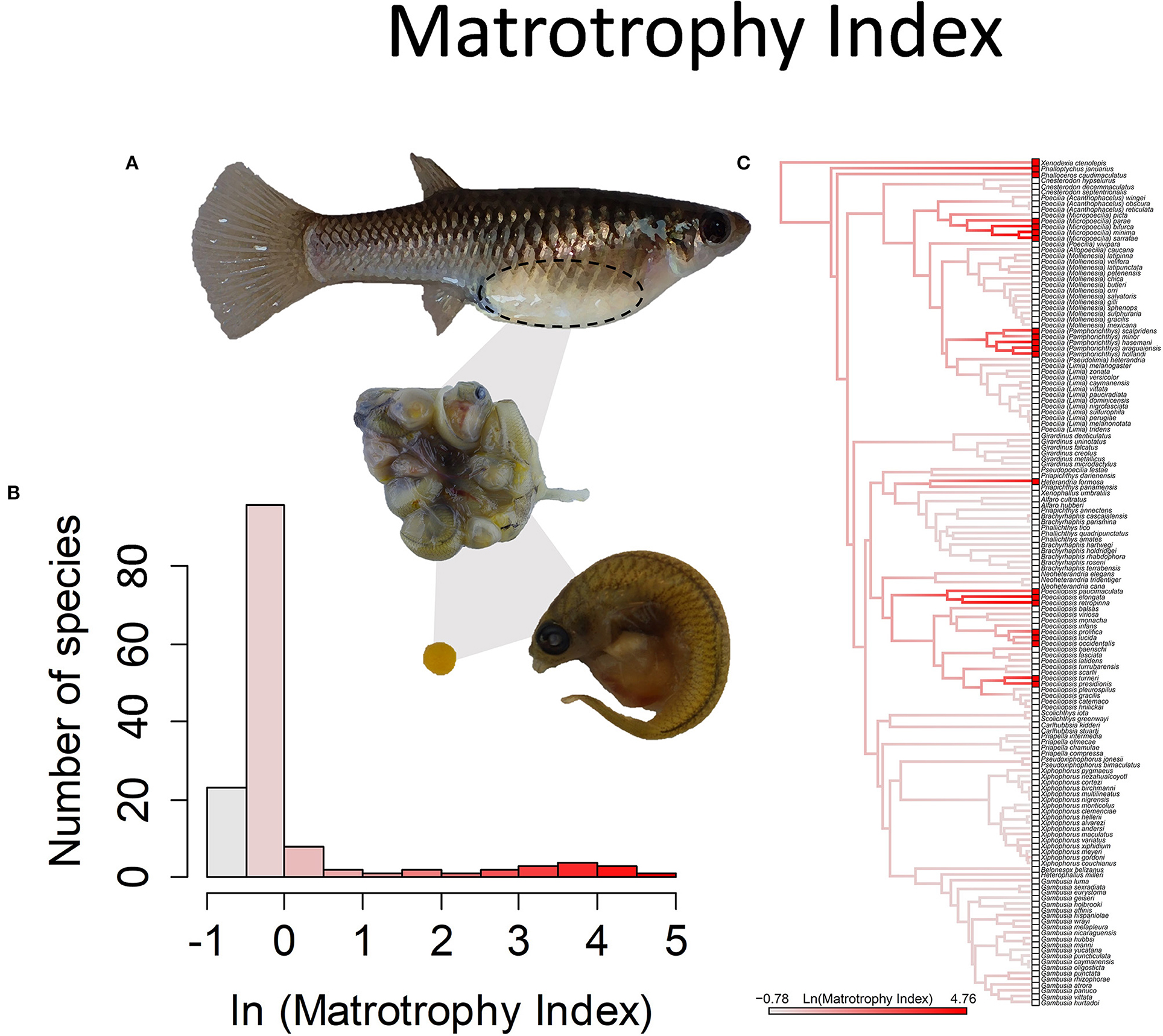
Figure 8. The Matrotrophy Index, measured as the ratio of offspring dry mass at birth to that of a just fertilized embryo, is a unitless measure of the degree of post-fertilization maternal embryo provisioning. In the family Poeciliiidae, this value ranges from 0.46 to 117. Values <1 indicate embryos lose mass over the course of gestation and are considered lecithotrophic or “yolk feeding” (a value of 0.7 represents the loss of mass in egg-laying species), and larger values indicate matrotrophy or “mother feeding.” (A) Poeciliopsis retropinna is a highly matrotrophic species (Reznick et al., 2002, Hagmayer et al., 2018, Hagmayer et al., 2020). Over the course of gestation in the ovary (pictured), embryos undergo an up to 117-fold increase in dry weight, due to nutrient provisioning by means of a follicular placenta. (B) Frequency histogram of the natural log transformed Matrotrophy Index (n = 146). (C) Ancestral state reconstruction of the Matrotrophy Index as a continuous character using the contMap function in the R package phytools, which estimates the Maximum likelihood ancestral states at all internal nodes and interpolates the states along each edge. The phylogeny tips depict a binary categorization of the placenta (i.e., absent vs. present). Please note, the reconstruction depicted here omits a basal lineage, Tomeurus gracilis, the sole non-livebearing member of the family, as well as non-livebearing outgroup taxa. A reconstruction of placentation as a binary presence or absence character, with the inclusion of these taxa, suggests the most likely ancestral state of the family is to lack placentation (Furness et al., 2019).
A second type of variation is in the presence or absence of superfetation. Most species lack superfetation, which means that they produce a single batch of eggs that is fertilized, then embryos develop and are born together (Figure 9). The process then begins again, with the yolking and fertilization of a new batch of eggs. Females of such species usually give birth every 3–4 weeks. Species with superfetation have the capacity to carry multiple broods of developing young in different stages of development (Pollux et al., 2009). Such species initiate a litter of developing young, then yolk a new batch of eggs and initiate the next litter before the previous litter is born (Turner, 1937). Species and populations within species vary in how many litters they carry at one time, ranging from as few as two to up to 8 litters at a time (Turner, 1937). A consequence of superfetation is that females give birth more frequently but to fewer young at a time. Species that typically have two developing litters at a time will give birth every 12–14 days. Those that typically have seven or eight developing litters at a time will give birth every 2 or 3 days (Turner, 1937; Pollux and Reznick, 2011).
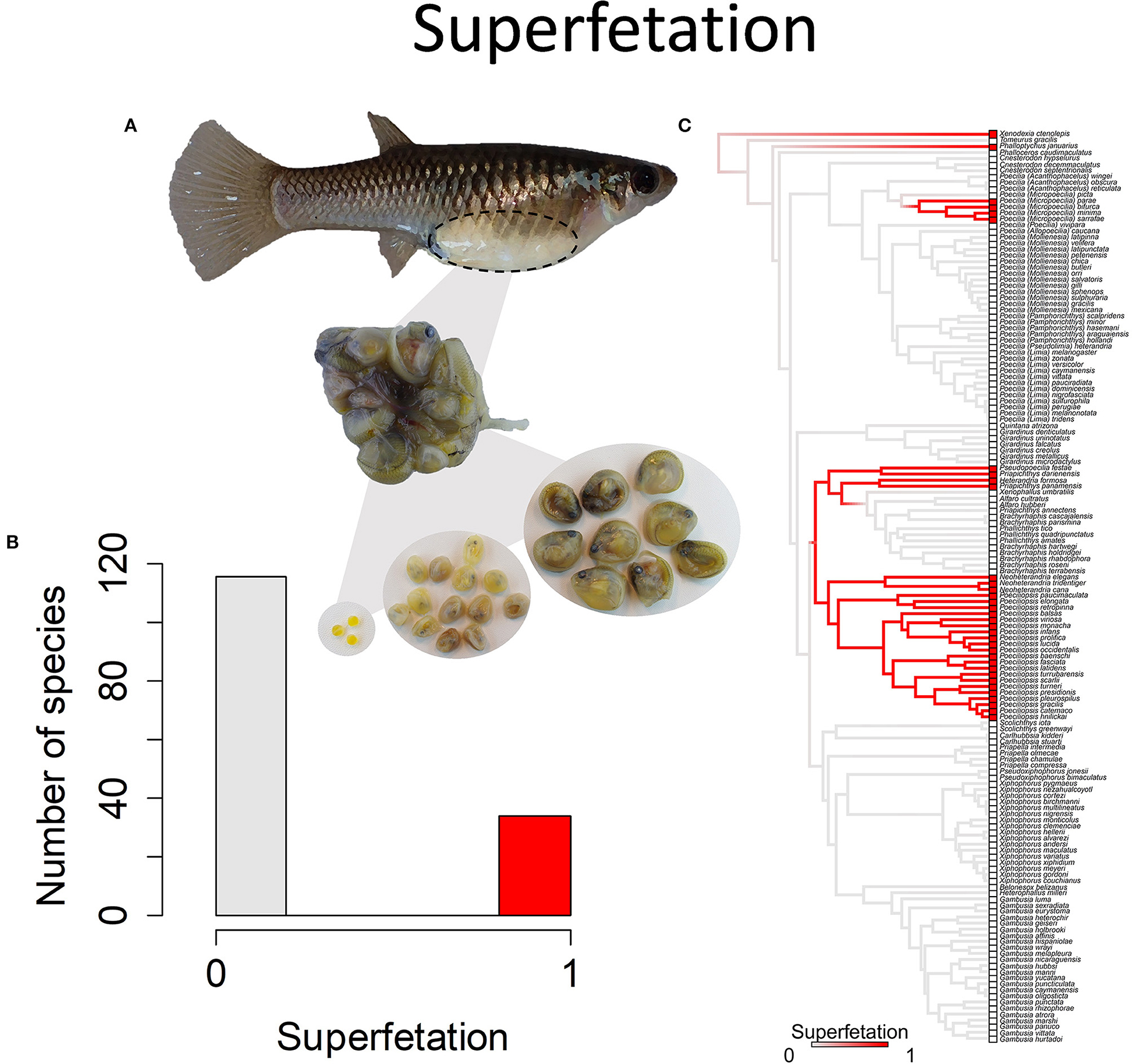
Figure 9. Superfetation refers to the capacity of females to gestate more than one brood of offspring at different stages of development. (A) Poeciliopsis retropinna exhibits superfetation (Hagmayer et al., 2018, Hagmayer et al., 2020). Broods at different stages of development (pictured) are simultaneously gestated in the ovary. (B) Frequency histogram of presence or absence of superfetation (n = 150). (C) Ancestral state reconstruction of superfetation as a binary character was performed using stochastic character mapping in the R package phytools. Branch colors represent posterior densities of edge states based on 1000 stochastic character maps of each reconstruction. The phylogeny tips depict a binary categorization of courtship (i.e., absence vs. presence). Figure redrawn after Furness et al. (2019). The most likely ancestral state of the family is the absence of superfetation, a pattern which becomes clearer with the inclusion of additional outgroup taxa (Furness et al., 2019).
Chronology of Evolution and the Signature of Conflict
Trends in Male and Female Evolution
Our next step was to integrate our comparative data on males and females with a well-resolved DNA-based family tree (Pollux et al., 2014; Reznick et al., 2017), enabling us to make inferences about the evolution of male and female traits (Furness et al., 2019). The most likely condition of males in the common ancestor of the family is to lack sexual dichromatism, ornamentation and courtship (Figures 4, 5, 7). The males were likely to have been much smaller than females (Figure 1) and the gonopodium was likely to have been relatively long (Figure 6). Courtship, dichromatism and male ornamentation proved to be evolutionarily labile; they were as likely to be lost as gained throughout the family tree (Furness et al., 2019). For example, phylogenetic analyses reveal that Poecilia latipunctata lost the enlarged dorsal fin that characterizes the rest of its clade, but retained courtship behavior (Ptacek and Breden, 1998; Ptacek et al., 2005). Xiphophorus continens has lost the sword, coloration, and courtship behavior typical of its clade (Morris et al., 2005). More generally, phylogenetic analyses reveal that the sword, typical of the genus Xiphophorus, was ancestral to the genus as a whole then repeatedly gained and lost throughout the diversification of the genus (Meyer et al., 1994).
The females of the common ancestor of the family likely had internal fertilization, bore live young, were lecithotrophic and lacked superfetation (Furness et al., 2019). Superfetation evolved four times and was lost once. The loss was in the common ancestor of a clade of Central American species, including the genera Brachyraphis, Phallichthys, and Alfaro (Figure 9). Placentotrophy evolved nine times and, once gained, was never lost (Figure 8). The independent origins of the placenta are Xenodexia, Phalloptychus, Phalloceros, Heterandria formosa, the Micropoecilia and Pamphorichthys subclades of Poecilia, and three times within the genus Poeciliopsis. There are also hints of evolving matrotrophy elsewhere in the family. For example, we have observed matrotrophy indices of between 1 and 2 in Gambusia rhizophorae (unpublished obsvn.). Zuniga-Vega et al. (2011) similarly observed matrophy indices that ranged from 1 to 5.8 in different populations of Poecilia butleri. Both species are from clades in which all other species are lecithotrophic.
Superfetation is almost always associated with matrotrophy, so much so that it was once suggested that they were different facets of the same adaptation (Constanz, 1989). The phylogenetic reconstructions reveal all possible orders of origin of superfetation and placentotrophy (Furness et al., 2019). Superfetation evolved before placentotrophy four times, placentotrophy and superfetation appear together three times, and placentotrophy evolved twice before superfetation. Superfetation appears before matrotrophy in the common ancestor of Neoheterandria and Poeciliopsis. Matrotrophy evolves later, at least three different times, within Poeciliopsis, but all other species within these two genera have superfetation without matrotrophy. The common ancestors of Phalloceros and Pamphorichthys had placentotrophy without superfetation, but superfetation has recently been seen in one population each of Phalloceros harpagos (Gorini-Pacheco et al., 2018) and Pamphorichtys hasemani (Pires and Reznick, 2018). The inclusion of these species and new information would bring the number of origins of superfetation in the family to six, and likely strengthen the already significant association between these two traits.
Why do we see this strong association between superfetation and matrotrophy? It is possible that superfetation promotes polyandry. Superfetation is known to promote polyandry in some mammals because females can re-mate each time they ovulate, so sequential litters can be sired by different males (Shackelford, 1952; Yamaguchi et al., 2004). If the same is true of the poeciliids, meaning that if females were inclined to mate after each time they gave birth, then it follows that superfetation may become associated with plancentotrophy because it would contribute to Zeh and Zeh (2000) prediction that matrotrophy favors polyandry (Pollux et al., 2014).
In conclusion, the diversity of male attributes in the family represents multiple independent gains and losses of the traits associated with sexual selection. Likewise, the placenta and superfetation have evolved repeatedly throughout the family. The multiple, independent origins of male and female traits gives us the statistical power to test whether the evolution of male and female attributes are associated with each other. The availability of a well-resolved fossil-calibrated phylogenetic tree (Pollux et al., 2014) also enables us to overlay the evolution of male and female traits, then address the order of evolution and hence make inferences about the causal relationships between the evolution of male traits and female mode of reproduction.
Associations Between Male and Female Attributes
The VDCH predicts that females of species that lack placentas will be choosier about who they mate with, making it more likely that the males of these species will have bright coloration, ornamentation, and courtship (Pollux et al., 2014). We also expected that the evolution of elaborate male displays will be accompanied by an increase in male body size since size so often plays a role in female choice or in male-male combat for access to females (Rios-Cardenas and Morris, 2011). If mating is cooperative, then selection may also favor the evolution of shorter gonopodia. Conversely, if females are placental, then the VDCH predicts that females will tend to mate with multiple males then make a post-copulatory choice of who sires her offspring. The prediction is thus that males will not experience the sort of pre-copulatory mate choice that favors the evolution of traits like bright coloration, ornamentation or courtship. Selection should instead favor the enhancement of traits associated with sneaky, forced copulation, such as the evolution of longer gonopodia and smaller male body size.
Selection could also favor the evolution of sperm attributes, such as greater sperm longevity, higher sperm velocity, and perhaps attributes of semen that promote fertility, as has been described in Drosophila (e.g., Swanson et al., 2001). However, some of these characteristics could also be favored by selection in non-placental species for males to subvert the effects of female choice. There is evidence that sperm characteristics can be associated with other sexually selected features in non-placental species (Pilastro et al., 2004; Locatello et al., 2006). This does not falsify the prediction from the VDCH; the prediction is that the major focus of selection in non-placental species is pre-copulatory while the major focus in placental species is postcopulatory. There is, to date, only a single study in a single species (guppies–Poecilia reticulata) that has attempted to parse the opportunity for sexual selection into pre- and post-copulatory phases (Devigili et al., 2015). Thus, whether sperm or components of semen evolve to circumvent female choice or simply via male-male competition is an interesting question, as is the question of whether the answer differs between placental and non-placental species.
Our comparative phylogenetic analyses support all of the predictions of the VDCH. Species with placentas have males that are smaller relative to females, have longer gonopodia and are less likely to have bright coloration, ornamentation, and courtship behavior than species that lack placentas (Pollux et al., 2014) (Figure 10).
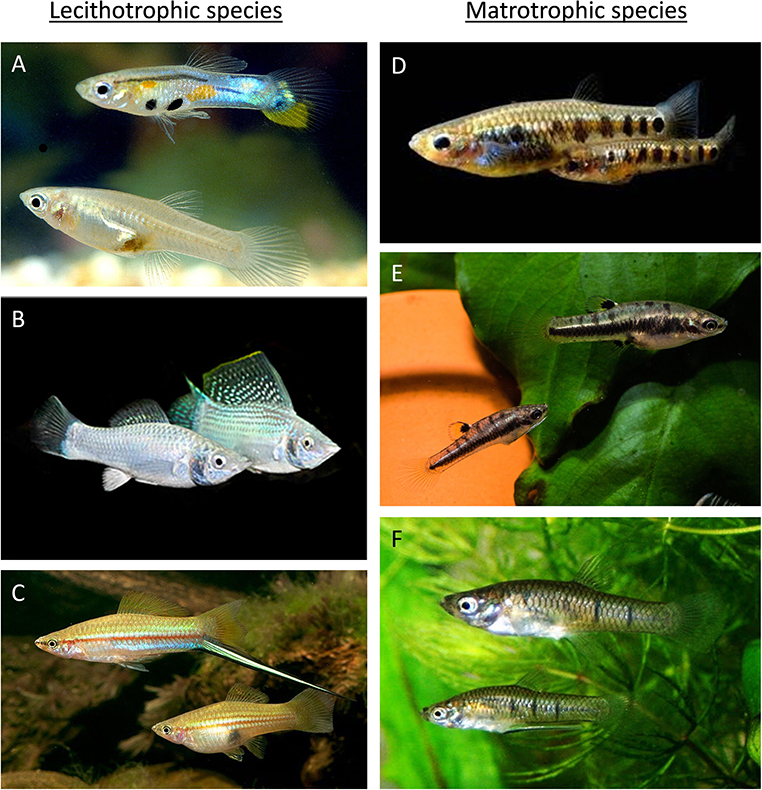
Figure 10. Lecithotrophic species include many popular in aquariums such as the sexually dichromatic guppy, Poecilia reticulata (A), the sailfin molly, Poecilia latipinna (B), and the swordtail, Xiphophorus helleri (C). Males of the latter two species also exhibit ornamental display traits in the form of a sail-like dorsal fin, and sword-like caudal fin. Males of all three species exhibit courtship and tend to have short gonopodia. In contrast, males of matrotrophic species such as (D) Poeciliopsis turneri, (E) Heterandria formosa, and (F) Phalloptychus juanarius tend to have sexually monochromatic coloration, lack ornamental display traits, and exhibit sneak-chase mating behavior rather than courtship. Furthermore, males in these species have long gonopodia and are considerably smaller than females. Photo credits: (A) Wikimedia Commons, (B) Johnny Jensen, JJPhoto.dk, (C) http://scontent-lhr3-1.xx.fbcdn.net, (D) aquafisher.org.ua, (E) Chiara Sciarone, (F) http://leo-aquarium.blogspot.com/.
The Evolution of Maternal Provisioning Governs the Evolution of Male Attributes
We next considered the joint evolution and possible inter-dependence of the evolution of male and female attributes (Furness et al., 2019). If the absence of placentation favors the evolution of male traits associated with pre-copulatory mate choice, then these traits should be significantly more likely to evolve in lineages that lack placentas than in lineages that have them. We found significant interdependence in male and female evolution for three of the five male traits associated with sexual selection—the presence or absence of courtship, sexual dichromatism and sexual size dimorphism. In all three cases, these traits were more likely to evolve when placentas were absent than when they were present, as predicted. In contrast, we did not find support for the evolution of maternal provisioning conditioned on male traits (Furness et al., 2019).
Furthermore, male traits associated with sexual selection were also lost at higher rates in lineages that lack placentas (Furness et al., 2019). An inherent feature of conflict is that the fitness of one sex is gained at a cost to the opposite sex (Rice, 1996), so sustaining the trait requires a balance between the two. Male traits that evolve under such circumstances should be less stable than traits that evolve under an alternative form of sexual selection like the good genes hypothesis, under which both sexes benefit. This elevated rate of gain and loss of male traits associated with sexual selection in lineages that lack placentas is illustrated in Figure 4 of Furness et al. (2019).
Conflict between sexual and natural selection is a second source of the evolutionary lability (accelerated gains and losses) of male traits associated with sexual selection. Traits associated with sexual selection are often costly; e.g., the bright coloration of male guppies attracts females, but also attracts the unwanted attention of predators (Endler, 1984). The contribution of these traits to fitness thus represents a balance between the costs and benefits associated with them. A tilt in that balance in favor of natural selection could result in net selection against such traits.
To summarize, species with placental reproduction tend to retain the ancestral state of males for the family, meaning that the males tend to lack sexual dichromatism, ornamentation, and courtship, be small relative to females and to have long gonopodia (Figure 10). Male traits associated with sexual selection evolved within lineages that lacked placentas. Figure 11 illustrates the complementary nature of male and female evolution in the form of mirrored phylogenetic trees of the evolution of male attributes with the evolution of placentation in females.
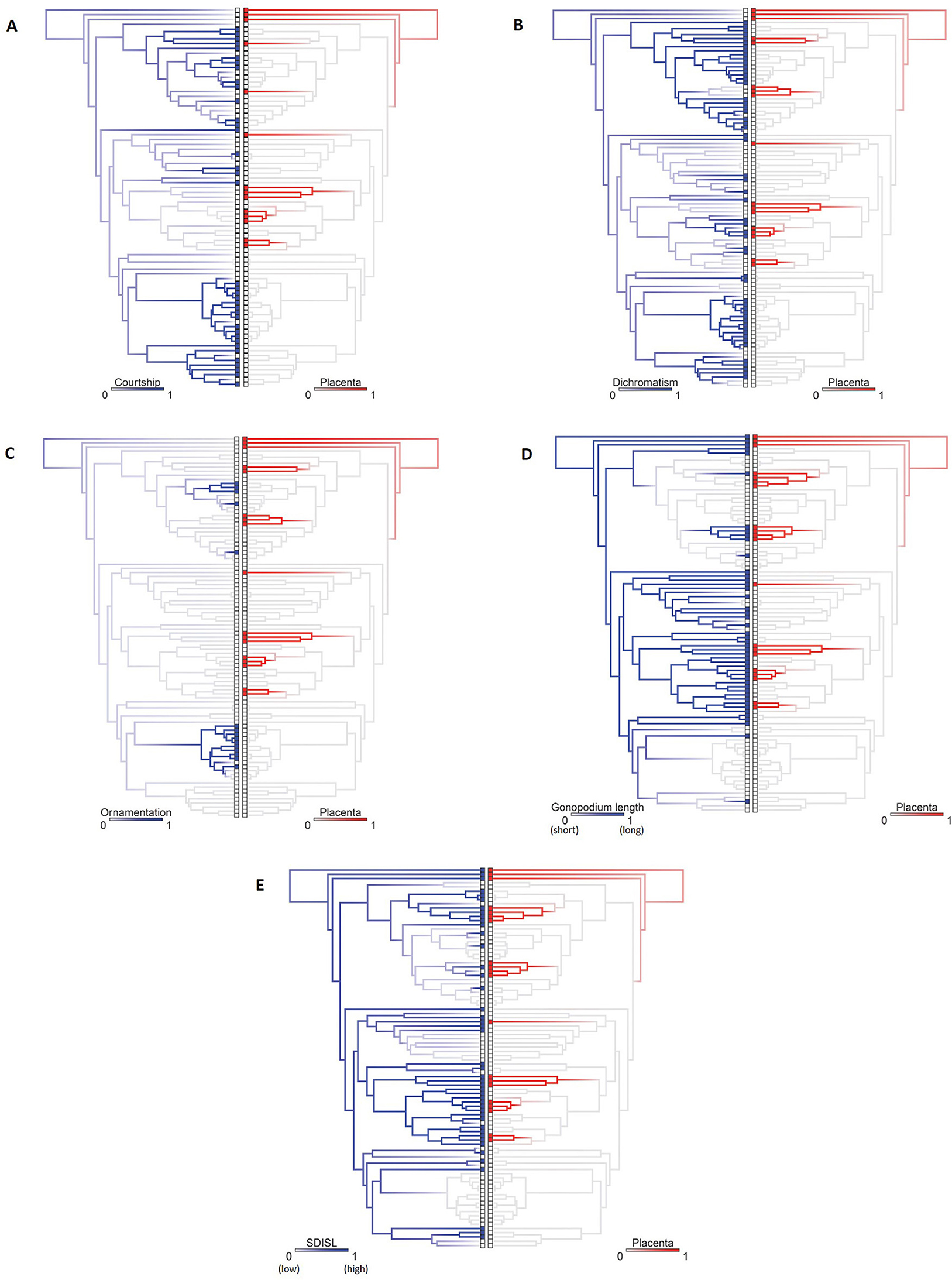
Figure 11. Mirrored trees illustrating potential correlated evolution between (A) courtship, (B) dichromatism, (C) ornamentation, (D) relative gonopodium length, and (E) sexual dimorphism index (standard length) and the placenta. Ancestral state reconstructions of both binary traits by stochastic character mapping. Phylogeny restricted to species with both traits. Branch colors represent posterior probability densities of edge states based on 1000 stochastic character maps of each econstruction. BayesTraits Discrete analyses support dependent (i.e., correlated) evolution between placentation and the absence of courtship, the absence of sexual dichromatism and increased sexual size dimorphism (males much smaller than females) but not with absence of ornamentation or long relative gonopodium length (Furness et al., 2019). The lack of association between placentation and absence of ornamentation is almost certainly due to the limited sample size of species with ornamentation. However, even though not statistically supported, all origins of ornamentation are in non-placental lineages (Xiphophorus and Poecilia). Likewise, the placenta tends to evolve in lineages with long gonopodia. In sum, species with placentas tend to lack male courtship, dichromatism, and ornamentation, have long gonopdia, and have a high degree of sexual size dimorphism (males much smaller than females). Figures redrawn from Furness et al. (2019).
Macroevolutionary Consequences of Conflict—Rate of Speciation
Zeh and Zeh (2000) predict that post-zygotic reproduction isolation will evolve more rapidly in viviparous than oviparous species because development of the embryo within the mother creates a physiological arena for genomic conflict absent in species that lay eggs (Zeh and Zeh, 2000). Reproductive isolation may evolve among separate populations as a byproduct of differences between them in how conflict is resolved. Evidence for this hypothesis includes the demonstration that oviparous (spawning or egg laying) species pairs retain the capacity to produce viable hybrids tens of millions of years following divergence, an order of magnitude longer than for many viviparous (live-bearing) species (Zeh and Zeh, 2000). Vrana (2007) details a specific mechanism in crosses among closely related species of Peromyscus mice that differ in the intensity of conflict because one is monogamous and the other polygamous. Hybrids experience offspring growth dysregulation caused by disrupted placental function and genomic imprinting. This results in severe offspring under- or over growth depending on the direction of the reciprocal cross. There is thus a direct link between placental reproduction and post-zygotic reproductive isolation. Since reproductive isolation is the hallmark of the biological species concept, a natural extension is that reproductive mode might affect the rate of speciation. If true, then this hypothesis yields the prediction that placental species will have a higher rate of speciation than lecithotrophic species.
An alternative hypothesis is that lineages in which males are enhanced by sexual selection will have higher rates of speciation because sexual selection can cause the accelerated evolution of pre-copulatory reproductive isolation (Rice and Holland, 1997; Coyne and Orr, 2004). There is, in fact, a strong association between enhanced sexual selection and the rate of speciation in cichlid and centrarchid fishes, insects and organisms that use bioluminescence as part of sexual displays (Arnqvist et al., 2000; Wagner et al., 2012; Seehausen, 2015; Smith et al., 2015; Ellis and Oakley, 2016). More generally, it is of interest to illuminate the relative importance of pre- vs. post-copulatory reproductive isolation in causing speciation to occur (Coyne and Orr, 1989, 2004). The Poeciliidae provide a rare opportunity to compare the two side by side because of the tendency for a hypothesized driver of post-copulatory reproductive isolation (placentation) to be disassociated from a hypothesized driver of pre-copulatory reproductive isolation (sexual selection).
Contrary to the prediction of Zeh and Zeh, we found that non-placental species exhibited a higher rate of speciation than placental species. Furthermore, we found that the speciation rate is higher in lineages that exhibit courtship, dichromatism, and ornamentation, and especially the combination of all three traits (Furness et al., 2019). We can separate the role of male traits from mode of maternal provisioning because males that lack these traits represent the ancestral state of the family and there remain many non-placental lineages that retain this ancestral state. It thus appears that sexual selection and the associated elaboration of male traits may be a stronger driver of speciation rate than female reproductive mode (i.e., placentation) in this family of fishes. In this view the low rate of speciation in placental lineages could be a byproduct of the shift away from pre-copulatory and toward post-copulatory mate choice, causing placental lineages to lack courtship, dichromatism, and ornamentation. These patterns of association suggest that pre-copulatory reproductive isolation dominates post-copulatory reproductive isolation in governing the rate of speciation in the poeciliid fishes.
Discussion
What Do We Know About Multiple Paternity in the Poeciliidae?
High levels of concurrent multiple paternity have been reported in 10 species of Poeciliidae (Coleman and Jones, 2011; Evans and Pilastro, 2011; Girndt et al., 2012; Dekker et al., 2020)–four species of Xiphophorus, two of Gambusia, three of Poecilia, Poeciliopsis monacha, and Heterandria formosa). Only one of these species (H. formosa) is placental.
The existing analyses of concurrent multiple paternity harbor diverse sources of bias that preclude any attempt at generalization. First, while most of these studies have considerable statistical power to test the hypothesis that more than one sire is necessary to account for the genetic diversity of offspring, the actual estimates of the numbers of sires represented in a litter are based on different underlying algorithms and statistical approaches (Jones et al., 2010) and have large sampling errors. Second, not all of these studies were able to delimit, accurately, the distribution of reproductive success among males siring offspring. It is important to do so because different distributions of paternity among embryos will produce differences in the effective level of polyandry within a brood and the nature and strength of prenatal conflicts (Zeh and Zeh, 2000; Schrader et al., 2011). A brood of 15 embryos where one male sired 13 and two males sired one embryo each presents a different scenario for conflict than a brood of 15 in which three males sire five embryos apiece. Third, there is considerable variation among studies in the number of genetic loci assayed and the number of alleles per locus. The probability of detecting concurrent multiple paternity is directly proportional to the genetic variation present in the loci used to detect it (Neff et al., 2002; Jones et al., 2010; Schrader et al., 2011) and not all of these studies have corrected their estimates for the levels of segregating genetic variation. Fourth, some studies show substantial variation among populations in the rate of concurrent multiple paternity (Trexler et al., 1997; Gao et al., 2019). This suggests that studies of single populations may not represent patterns in the species as a whole. In addition, if populations differ in levels of neutral genetic variation, conclusions about population variation in the rates of multiple paternity depend crucially on whether the estimates have been corrected for those different levels of segregating genetic variation (Schrader et al., 2011). Finally, the existing data represent an ad hoc collection of distantly related species rather than a pairing of closely related species that differ in mode of maternal provisioning.
We are currently trying to improve on the situation by comparing levels of concurrent multiple paternity in three pairs of closely related species that represent three independent origins of placentation: Poeciliopsis prolifica (matrotrophic, denoted M) and P. infans (lecithotrophic, denoted L); Poeciliopsis presidionis (M) and P. gracillis (L); and Poecilia bifurca (M) and Poecilia reticulata (L) (Meredith et al., 2010). We are doing so in a way that addresses what we feel were weak points in prior analyses of multiple paternity. We hope, provide a better assessment of whether Zeh and Zeh (2000) prediction of higher rates of multiple paternity in placental species is upheld.
Alternative Explanations for the Associations Between Male and Female Attributes
Our presentation implicitly assumes that the associations among male and female traits are solely attributable to conflict. There are adaptive hypotheses for the evolution of placentas that may offer alternatives. One such hypothesis is derived from the observation that species with placentas tend to have a lower reproductive burden (Reznick et al., 2007b; Bassar et al., 2014) and to be more streamlined (Fleuren et al., 2018, 2019), which suggests that the evolution of placentation may be favored in performance demanding habitats, such as those with high risk of predation (Hagmayer et al., 2020). High risk of predation also favors the evolution of less colorful guppies (Endler, 1978) so it is possible to imagine predation independently shaping male and female attributes. Alternatively, Trexler and DeAngelis (2003) propose that placentation is most likely to evolve in stable, high resource availability environments because it can yield a fecundity advantage. If such high resource environments are associated with high population densities and a reduced opportunity for males to monopolize females, then it could simultaneously favor a loss of male traits associated with sexual selection. Adaptive and conflict hypotheses are not necessarily exclusive of one another. For example, superfetation and placentation may have initially conveyed an adaptive advantage, but once adopted they would also have shifted the venue of sexual conflict (Furness et al., 2015). We have recently performed comparative analyses on the same data set used here and failed to find consistent family-wide associations between placentation, superfetation and habitat, and failed to find consistent support for either of these adaptive hypotheses (Furness et al., 2021), so these alternatives do not seem viable at this time.
Conclusions
We can now answer the seven questions posed at the opening of this paper.
• How often have male attributes associated with sexual selection evolved? The reversibility of the evolution of these traits makes it difficult to arrive at a precise number of events, but we can at least say that these attributes have been gained, and lost, repeatedly in the family. We can also identify “hot spots” of sexual selection in the genera Poecilia and Xiphophorus, where we see species with a concentration of those attributes associated with sexual selection.
• How often has the equivalent of a mammalian placenta evolved? Placentation evolved at least nine times. There is evidence of other origins in individual species nested within clades that are otherwise non-placental.
• How often has superfetation, a different variation on the theme of maternal provisioning, evolved? Superfetation was gained at least four times and lost at least once in the family. We also have discovered superfetation emerging two additional times in lineages that had previously evolved placentation (Pamphorichthys and Phalloceros).
• How often are these traits lost? Specifically, are there differences in the evolutionary lability of these male and female attributes? There are indeed strong differences in the reversibility of male traits associated with sexual selection and mode of reproduction in females. Male traits are much more labile. One obvious explanation is they reflect a continuous conflict of interest between sexual and natural selection to a greater degree than female mode of reproduction, which perhaps evolves as an adaptive trait, but one that shifts the venue of sexual conflict. There may be deeper differences between male and female traits in terms of the underlying genetic mechanisms and how reversible they are. For example, the genetic basis of the evolution of male traits may be relatively simple while those underlying the evolution of mode of reproduction may be the consequence of multiple, sequential steps that are more difficult to reverse (Lee and Shine, 1998; Furness et al., 2015; Furness and Capellini, 2019).
• Are there associations between the presence of male attributes associated with sexual selection and the mode of maternal provisioning? Yes. As predicted by the VDCH hypothesis, male traits associated with sexual selection are much more likely to be seen in species that lack placentas.
• Are there patterns in the order of evolution of male traits associated with sexual selection and the mode of maternal provisioning that suggest a cause-and-effect relationship? Yes. The evolution of male traits associated with sexual selection is conditioned on the mode of maternal provisioning. Species that lack placentas are much more likely to gain, and lose, male traits associated with sexual selection.
• Finally, is the evolution of a placenta associated with the accelerated evolution of post-copulatory reproductive isolation and speciation, as predicted by the VDCH? No. Lineages with placentas have slower rates of speciation than those without placentas. The reason those without placentas have higher average rates of speciation is because a subset of the non-placental lineages are the ones in which we see the elaboration of male traits associated with sexual selection. The accelerated rates of speciation are concentrated in these lineages.
Data Availability Statement
Publicly available datasets were analyzed in this study. This data can be found at: https://doi.org/10.5061/dryad.7g5b162.
Author Contributions
DR conceived of the paper, wrote the first draft, and edited the final product. AF revised the first draft and made all of the figures. JT revised the first draft and contributed some new material to the manuscript. BP contributed the male data and revised the final draft of the manuscript. All authors approved the submitted version.
Funding
This work was supported by the National Science Foundation (DEB-0416085, DEB-1754669, and PRFB Award 1523666).
Conflict of Interest
The authors declare that the research was conducted in the absence of any commercial or financial relationships that could be construed as a potential conflict of interest.
The handling editor was organizing a Research Topic with one of the authors, BP, but had no other collaboration with any of the authors.
Acknowledgments
We thank Robert Meredith for providing most of the fish illustrations used in Figures 1, 4–7, and Andres Hagmayer for the photo of the female Poeciliopsis retropinna in Figures 8, 9. We thank the associated editor and reviewers for numerous suggestions that improved the manuscript.
References
Arias, A.-L., and Reznick, D. N. (2000). Life history of Phalloceros caudimaculatus: a novel variation on the theme of livebearing in the family Poeciliidae. Copeia 2000, 792–798. doi: 10.1643/0045-8511(2000)000[0792:LHOPCA]2.0.CO;2
Arnqvist, G., Edvardsson, M., Friberg, U., and Nilsson, T. (2000). Sexual conflict promotes speciation in insects. Proc. Natl. Acad. Sci. U.S.A. 97, 10460–10464. doi: 10.1073/pnas.97.19.10460
Arnqvist, G., and Rowe, L. (1995). Sexual conflict and arms races between the sexes–a morphological adaptation for control of mating in a female insect. Proc. R. Soc. Lond. Ser. B Biol. Sci. 261, 123–127. doi: 10.1098/rspb.1995.0126
Arnqvist, G., and Rowe, L. (2002). Antagonistic coevolution between the sexes in a group of insects. Nature 415, 787–789. doi: 10.1038/415787a
Aspbury, A. S., and Basolo, A. L. (2002). Repeatable female preferences, mating order, and mating success in the poeciliid fish, Heterandria formosa. Behav. Ecol. Sociobiol. 51, 238–244. doi: 10.1007/s00265-001-0443-1
Basolo, A. L. (1995). A further examination of a pre-existing bias favoring a sword in the genus Xiphophorus. Animal Behavior 50:365–375. doi: 10.1006/anbe.1995.0252
Basolo, A. L. (1998). Evolutionary change in a receiver bias: a comparison of female preference functions. Proc. R. Soc. B Biol. Sci. 265, 2223–2228. doi: 10.1098/rspb.1998.0563
Bassar, R. D., Auer, S. K., and Reznick, D. N. (2014). Why do placentas evolve? a test of the life-history facilitation hypothesis in two clades in the genus Poeciliopsis representing two independent origins of placentas. Funct. Ecol. 28, 999–1010. doi: 10.1111/1365-2435.12233
Bisazza, A. (1993). Male competition, female mate choice, and sexual size dimorphism in Poeciliid fishes. Mar. Behav. Physiol. 23, 257–286. doi: 10.1080/10236249309378869
Bisazza, A., and Pilastro, A. (1997). Small male mating advantage and reversed size dimorphism in poeciliid fishes. J. Fish Biol. 50, 397–406. doi: 10.1111/j.1095-8649.1997.tb01367.x
Bisazza, A., and Pilastro, A. (2000). Variation of female preference for male coloration in the eastern mosquitofish Gambusia holbrooki. Behav. Genet. 30, 207–212. doi: 10.1023/A:1001914208075
Blackburn, D. G. (2015). Evolution of vertebrate viviparity and specializations for fetal nutrition: a quantitative and qualitative analysis. J. Morphol. 276, 961–990. doi: 10.1002/jmor.20272
Casner, A. M., Fackelman, H. C., Degtyareva, O., and Kight, S. L. (2016). Do female western mosquitofish, gambusia affinis, prefer ornaments that males lack? Ethology 122, 561–570. doi: 10.1111/eth.12507
Cohen, S. N., Regus, J. U., Reynoso, Y., Mastro, T., and Reznick, D. N. (2015). Comparative life histories of fishes in the subgenus Limia (Pisces: Poeciliidae). J. Fish Biol. 87:100–114.
Coleman, S. W., and Jones, A. G. (2011). Patterns of multiple paternity and maternity in fishes. Biol. J. Linn. Soc. 103, 735–760. doi: 10.1111/j.1095-8312.2011.01673.x
Constanz, G. D. (1989). Reproductive biology of poeciliid fishes, in Ecology and Evolution of Livebearing Fishes, eds Meffe, G. K., and Snelson, F. F. J., (Englewood Cliffs, NJ: Prentice Hall), 33–50.
Coyne, J. A., and Orr, H. A. (1989). Patterns of speciation in Drosophila. Evolution 43, 362–381. doi: 10.1111/j.1558-5646.1989.tb04233.x
Crespi, B., and Semeniuk, C. (2004). Parent-offspring conflict in the evolution of vertebrate reproductive mode. Am. Nat. 163, 635–653. doi: 10.1086/382734
Dekker, M. L., Hagmayer, A., Leon-Kloosterziel, K. M., Furness, A. I., and Pollux, B. J. A. (2020). High degree of multiple paternity and reproductive skew in the highly fecund live-bearing fish Poecilia gillii (Family Poeciliidae). Front. Ecol. Evol. 8:579105. doi: 10.3389/fevo.2020.579105
Devigili, A., Evans, J. P., Di Nisio, A., and Pilastro, A. (2015). Multivariate selection drives concordant patterns of pre- and postcopulatory sexual selection in a livebearing fish. Nat. Commun. 6:8291. doi: 10.1038/ncomms9291
Eberhard, W. G. (1996). Female Control: Sexual Selection by Cryptic Female Choice. Princeton, NJ: Princeton University Press.
Elliot, M. G., and Crespi, B. J. (2006). Placental invasiveness edmiates the evolution of hybrid inviability in mammals. Am. Nat. 168, 114–120. doi: 10.1086/505162
Ellis, E. A., and Oakley, T. H. (2016). High rates of species accumulation in animals with bioluminescent courtship displays. Curr. Biol. 26, 1916–1921. doi: 10.1016/j.cub.2016.05.043
Endler, J. A. (1978). A predator's view of animal color patterns. Evol. Biol. 11, 319–364. doi: 10.1007/978-1-4615-6956-5_5
Endler, J. A. (1984). Natural and sexual selection on color patterns in poeciliid fishes, in Evolutionary Ecology of Neotropical Freshwater Fishes, ed Zaret, T. M., (Dordrecht: Springer), 95–111.
Erbelding-Denk, C., Schroder, J. H., Schartl, M., Nanda, I., Schmid, M., and Epplen, J. T. (1994). Male polymorphism in Limia perugiae (Pisces, Poeciliidae). Behav. Genet. 24, 95–101. doi: 10.1007/BF01067933
Evans, J. P., and Pilastro, A. (2011). Postcopulatory sexual selection, in Ecology and Evolution of Poeciliid Fishes, Evans, J. P., Pilastro, A., and Schlupp, I., (Chicago, IL: University of Chicago Press), 197–208.
Farr, J. A., and Travis, J. (1986). Fertility advertisement by female sailfin mollies, Poecilia latipinna (Pisces, Poeciliidae). Copeia 1986, 467–472. doi: 10.2307/1445004
Farr, J. A., Travis, J., and Trexler, J. C. (1986). Behavioral allometry and interdemic variation in sexual behavior of the sailfin molly, Poecilia latipinna (Pisces, Poeciliidae). Anim. Behav. 34, 497–509. doi: 10.1016/S0003-3472(86)80118-X
Fitzpatrick, B. M. (2004). Rates of evolution of hybrid inviability in birds and mammals. Evolution 58, 1865–1870. doi: 10.1111/j.0014-3820.2004.tb00471.x
Fleuren, M., Quicazan-Rubio, E. M., van Leeuwen, J. L., and Pollux, B. J. A. (2018). Why do placentas evolve? evidence for a morphological advantage during pregnancy in live-bearing fish. PLoS ONE 13:e0195976. doi: 10.1371/journal.pone.0195976
Fleuren, M., van Leeuwen, J. L., and Pollux, B. J. A. (2019). Superfetation reduces the negative effects of pregnancy on the fast-start escape performance in live-bearing fish. Proc. R. Soc. B Biol. Sci. 286:20192245. doi: 10.1098/rspb.2019.2245
Furness, A. I., Avise, J. C., Pollux, B. J. A., Reynoso, Y., and Reznick, D. N. (2021). The evolution of the placenta in poeciliid fishes. Curr. Biol. 31, 1–8. doi: 10.1016/j.cub.2021.02.008
Furness, A. I., and Capellini, I. (2019). The evolution of parental care diversity in amphibians. Nat. Commun. 10:4709. doi: 10.1038/s41467-019-12608-5
Furness, A. I., Morrison, K. R., Orr, T. J., Arendt, J. D., and Reznick, D. N. (2015). Reproductive mode and the shifting arenas of evolutionary conflict. Ann N Y Acad Sci. 1360, 75–100. doi: 10.1111/nyas.12835
Furness, A. I., Pollux, B. J. A., Meredith, R. W., Springer, M. S., and Reznick, D. N. (2019). How conflict shapes evolution in poeciliid fishes. Nat. Commun. 10:3335. doi: 10.1038/s41467-019-11307-5
Gao, J. C., Santi, F., Zhou, L. J., Wang, X. Q., Riesch, R., and Plath, M. (2019). Geographical and temporal variation of multiple paternity in invasive mosquitofish (Gambusia holbrooki, Gambusia affinis). Mol. Ecol. 28, 5315–5329. doi: 10.1111/mec.15294
Girndt, A., Riesch, R., Schroder, C., Sehlupp, I., Plath, M., and Tiedemann, R. (2012). Multiple paternity in different populations of the sailfin molly, Poecilia latipinna. J. Evol. Biol. 19, 1595–1602. doi: 10.1163/157075611X618192
Gorini-Pacheco, B., Zandona, E., and Mazzoni, R. (2018). Predation effects on matrotrophy, superfetation and other life history traits in Phalloceros harpagos. Ecol. Freshw. Fish 27, 442–452. doi: 10.1111/eff.12359
Grant, P. R., and Grant, B. R. (2008). How and Why Species Multiply. Princeton, NJ: Princeton University Press.
Greven, H. (2005). Structural and behavioral traits associated with sperm transfer in the Poeciliidae, in Viviparous Fishs, eds Uribe, M. C., and Grier, H. J., (Homestead, FL: New Life Publications), 145–163.
Gross, M. R. (1985). Disruptive selection for alternative life histories in salmon. Nature 313, 47–48. doi: 10.1038/313047a0
Hagmayer, A., Furness, A. I., Reznick, D. N., Dekker, M. L., and Pollux, B. J. A. (2020). Predation risk shapes the degree of placentation in natural populations of live-bearing fish. Ecol. Lett. 23, 831–840. doi: 10.1111/ele.13487
Hagmayer, A., Furness, A. I., Reznick, D. N., and Pollux, B. J. A. (2018). Maternal size and body condition predict the amount of post-fertilization maternal provisioning in matrotrophic fish. Ecol. Evol. 8, 12386–12396. doi: 10.1002/ece3.4542
Haig, D. (1990). Brood reduction and optimal parental investment when offspring differ in quality. Am. Nat. 136, 550–556. doi: 10.1086/285113
Haig, D. (1993). Genetic conflicts in human pregnancy. Q. Rev. Biol. 68, 495–532. doi: 10.1086/418300
Heifetz, Y., Lung, O., Frongillo, E. A., and Wolfner, M. F. (2000). The Drosophila seminal fluid protein Acp26Aa stimulates release of oocytes by the ovary. Curr. Biol. 10, 99–102. doi: 10.1016/S0960-9822(00)00288-8
Houde, A. E. (1987). Mate choide based upon naturally occurring color pattern variation in a guppy population. Evolution 41, 1–10. doi: 10.1111/j.1558-5646.1987.tb05766.x
Houde, A. E. (1988a). The effects of female choice and male male competition on the mating success of male guppies. Anim. Behav. 36, 888–896. doi: 10.1016/S0003-3472(88)80171-4
Houde, A. E. (1988b). Genetic difference in female choice between two guppy populations. Anim. Behav. 36, 510–516. doi: 10.1016/S0003-3472(88)80022-8
Jones, A. G., Small, C. M., Paczolt, K. A., and Ratterman, N. L. (2010). A practical guide to methods of parentage analysis. Mol. Ecol. Resour. 10, 6–30. doi: 10.1111/j.1755-0998.2009.02778.x
Kallman, K. D. (1989). Genetic control of size at maturity in Xiphophorus, in Ecology and Evolution of Livebearing Fishes (Poeciliidae), eds Meffe, G. K., and Snelson, F. F. J., . (Englewood Cliffs, NJ: Prentice Hall), 453.
Kodric-Brown, A. (1993). Female choice of multiple male criteria in guppies - interacting effects of dominance, coloration, and courtship. Behav. Ecol. Sociobiol. 32, 415–420. doi: 10.1007/BF00168825
Kolluru, G. R., and Reznick, D. N. (1996). Genetic and social control of male maturation in Phallichthys quadripunctatus (Pisces: Poeciliidae). J. Evol. Biol. 9, 695–715. doi: 10.1046/j.1420-9101.1996.9060695.x
Lampert, K. P., Schmidt, C., Fischer, P., Volff, J. N., Hoffmann, C., Muck, J., et al. (2010). Determination of onset of sexual maturation and mating behavior by melanocortin receptor 4 polymorphisms. Curr. Biol. 20, 1729–1734. doi: 10.1016/j.cub.2010.08.029
Langerhans, R. B. (2011). Genital evolution, in Ecology and Evolution of Poeciliid Fishes, eds Evans, J., Pilastro, A., and Schlupp, I., (Chicago, IL: University of Chicago Press), 228–240
Lee, M. S. Y., and Shine, R. (1998). Reptilian viviparity and Dollo's law. Evolution 52, 1441–1450. doi: 10.1111/j.1558-5646.1998.tb02025.x
Levitan, D. R., and Ferrell, D. L. (2006). Selection on gamete recognition proteins depends on sex, density, genotype frequency. Science 312, 267–269. doi: 10.1126/science.1122183
Locatello, L., Rasotto, M. B., Evans, J. P., and Pilastro, A. (2006). Colourful male guppies produce faster and more viable sperm. J. Evol. Biol. 19, 1595–1602. doi: 10.1111/j.1420-9101.2006.01117.x
MacLaren, R. D., and Rowland, W. J. (2006). Differences in female preference for male body size in Poecilia latipinna using simultaneous versus sequential stimulus presentation designs. Behaviour 143, 273–292. doi: 10.1163/156853906775897897
MacLaren, R. D., Rowland, W. J., and Morgan, N. (2004). Female preferences for sailfin and body size in the sailfin molly, Poecilia latipinna. Ethology 110, 363–379. doi: 10.1111/j.1439-0310.2004.00974.x
Marsh-Matthews, E., Brooks, M., Deaton, R., and Tan, H. (2005). Effects of maternal and embryo characteristics on post-fertilization provisioning in fishes of the genus Gambusia. Oecologia 144, 12–24, doi: 10.1007/s00442-005-0030-7
Marsh-Matthews, E., and Deaton, R. (2006). Resources and offspring provisioning: a test of the Trexler-DeAngelis model for matrotrophy evolution. Ecology 87, 3014–3020. doi: 10.1890/0012-9658(2006)87[3014:RAOPAT]2.0.CO;2
Marsh-Matthews, E., Skierkowski, P., and DeMarais, A. (2001). Direct evidence for mother-to-embryo transfer of nutrients in the livebearing fish Gambusia geiseri. Copeia 2001, 1–6. doi: 10.1643/0045-8511(2001)001[0001:DEFMTE]2.0.CO;2
McPeek, M. A. (1992). Mechanisms of sexual selection operating on body size in the mosquitofish (Gambusia holbrooki). Behav. Ecol. 3, 1–12. doi: 10.1093/beheco/3.1.1
Meredith, R. W., Pires, M. N., Reznick, D. N., and Springer, M. S. (2010). Molecular phylogenetic relationships and the evolution of the placenta in Poecilia (Micropoecilia) (Poeciliidae: Cyprinodontiformes). Mol. Phylogenet. Evol. 55, 631–639. doi: 10.1016/j.ympev.2009.11.006
Meyer, A., Morrissey, J. M., and Schartl, M. (1994). Recurrent origin of a sexually selected trait in Xiphophorus fishes inferred from a molecular phylogeny. Nature 368, 539–542. doi: 10.1038/368539a0
Mock, D. W., and Parker, G. A. (1997). The Evolution of Sibling Rivalry. Oxford: Oxford University Press.
Morris, M. R. (1998). Female preference for trait symmetry in addition to trait size in swordtail fish. Proc. R. Soc. B Biol. Sci. 265, 907–911. doi: 10.1098/rspb.1998.0377
Morris, M. R., Moretz, J. A., Farley, K., and Nicoletto, P. (2005). The role of sexual selection in the loss of sexually selected traits in the swordtail fish Xiphophorus continens. Anim. Behav. 69, 1415–1424. doi: 10.1016/j.anbehav.2004.08.013
Morris, M. R., Rios-Cardenas, O., and Brewer, J. (2010). Variation in mating preference within a wild population influences the mating success of alternative mating strategies. Anim. Behav. 79, 673–678. doi: 10.1016/j.anbehav.2009.12.018
Morrison, K. R., Ngo, V., Cardullo, R. A., and Reznick, D. N. (2017). How fish eggs are preadapted for the evolution of matrotrophy. Proc. R. Soc. B Biol. Sci. 284:20171342. doi: 10.1098/rspb.2017.1342
Neff, B. D., Pitcher, T. E., and Repka, J. (2002). A Bayesian model for assessing the frequency of multiple mating in nature. J. Hered. 93, 406–414. doi: 10.1093/jhered/93.6.406
Olivera-Tlahuel, C., Moreno-Mendoza, N. A., Villagran-Santa Cruz, M., and Zuniga-Vega, J. J. (2019). Placental structures and their association with matrotrophy and superfetation in poeciliid fishes. Acta Zool. 100, 167–181. doi: 10.1111/azo.12244
Pilastro, A., Simonato, M., Bisazza, A., and Evans, J. P. (2004). Cryptic female preference for colorful males in guppies. Evolution 58, 665–669. doi: 10.1111/j.0014-3820.2004.tb01690.x
Pires, M. N., Arendt, J., and Reznick, D. N. (2010). The evolution of placentas and superfetation in the fish genus Poecilia (Cyprinodontiformes: Poeciliidae: subgenera Micropoecilia and Acanthophacelus). Biol. J. Linn. Soc. 99, 784–796. doi: 10.1111/j.1095-8312.2010.01391.x
Pires, M. N., and Reznick, D. N. (2018). Life-history evolution in the fish genus Poecilia (Poeciliidae: Cyprinodontiformes: subgenus Pamphorichthys): an evolutionary origin of extensive matrotrophy decoupled from superfetation. Biol. J. Linn. Soc. 125, 547–560. doi: 10.1093/biolinnean/bly128
Pollux, B. J. A., Meredith, R. W., Springer, M. S., Garland, T., and Reznick, D. N. (2014). The evolution of the placenta drives a shift in sexual selection in livebearing fish. Nature 513, 233–236. doi: 10.1038/nature13451
Pollux, B. J. A., Pires, M. N., Banet, A. I., and Reznick, D. N. (2009). Evolution of placentas in the fish family poeciliidae: an empirical study of macroevolution. Ann. Rev. Ecol. Evol. Syst. 40, 271–289. doi: 10.1146/annurev.ecolsys.110308.120209
Pollux, B. J. A., and Reznick, D. N. (2011). Matrotrophy limits a female's ability to adaptively adjust offspring size and fecundity in fluctuating environments. Funct. Ecol. 25, 747–756. doi: 10.1111/j.1365-2435.2011.01831.x
Prager, E. M., and Wilson, A. C. (1975). Slow evolutionary loss of potential for interspecific hybridization in birds–manifestation of slow regulatory evolution. Proc. Natl. Acad. Sci. U.S.A. 72, 200–204. doi: 10.1073/pnas.72.1.200
Ptacek, M. B., and Breden, F. (1998). Phylogenetic relationships among the mollies (Poeciliidae: Poecilia: Mollienesia group) based on mitochondrial DNA sequences. J. Fish Biol. 53, 64–81. doi: 10.1111/j.1095-8649.1998.tb01018.x
Ptacek, M. B., Childress, M. J., and Kittell, M. M. (2005). Characterizing the mating behaviours of the Tamesi molly, Poecilia latipunctata, a sailfin with shortfin morphology. Anim. Behav. 70, 1339–1348. doi: 10.1016/j.anbehav.2005.03.019
Ptacek, M. B., and Travis, J. (1996). Inter-population variation in male mating behaviours in the sailfin mollie, Poecilia latipinna. Anim. Behav. 52, 59–71. doi: 10.1006/anbe.1996.0152
Ptacek, M. B., and Travis, J. (1997). Mate choice in the sailfin molly, Poecilia latipinna. Evolution 51, 1217–1231. doi: 10.1111/j.1558-5646.1997.tb03969.x
Ram, K. R., and Wolfner, M. F. (2007). Seminal influences: Drosophila Acps and the molecular interplay between males and females during reproduction. Integr. Comp. Biol. 47, 427–445. doi: 10.1093/icb/icm046
Regus, J. U., Johnson, J. B., Webb, S. A., and Reznick, D. N. (2013). Comparative life histories of fishes in the genus Phallichthys (Pisces: Poeciliidae). J. Fish Biol. 83, 144–155. doi: 10.1111/jfb.12158
Reznick, D., Hrbek, T., Caura, S., De Greef, J., and Roff, D. (2007a). Life history of Xenodexia ctenolepis: implications for life history evolution in the family Poeciliidae. Biol. J. Linn. Soc. 92, 77–85. doi: 10.1111/j.1095-8312.2007.00869.x
Reznick, D., Meredith, R., and Collette, B. B. (2007b). Independent evolution of complex life history adaptations in two families of fishes, live-bearing halfbeaks (Zenarchopteridae, Beloniformes) and Poeciliidae (Cyprinodontiformes). Evolution 61, 2570–2583. doi: 10.1111/j.1558-5646.2007.00207.x
Reznick, D. N., Furness, A. I., Meredith, R. W., and Springer, M. S. (2017). The origin and biogeographic diversification of fishes in the family Poeciliidae. PLoS ONE 12:e0172546. doi: 10.1371/journal.pone.0172546
Reznick, D. N., Mateos, M., and Springer, M. S. (2002). Independent origins and rapid evolution of the placenta in the fish genus Poeciliopsis. Science 298, 1018–1020. doi: 10.1126/science.1076018
Rice, W. R. (1996). Sexually antagonistic male adaptation triggered by experimental arrest of female evolution. Nature 381, 232–234. doi: 10.1038/381232a0
Rice, W. R., and Holland, B. (1997). The enemies within: Intergenomic conflict, interlocus contest evolution (ICE), and the intraspecific Red Queen. Behav. Ecol. Sociobiol. 41, 1–10. doi: 10.1007/s002650050357
Riesch, R., Plath, M., Schlupp, I., and Marsh-Matthews, E. (2010). Matrotrophy in the cave molly: an unexpected provisioning strategy in an extreme environment. Evol. Ecol. 24, 789–801. doi: 10.1007/s10682-009-9335-z
Rios-Cardenas, O., and Morris, M. R. (2011). Precopulatory sexual selection, in Ecology and Evolution of Poeciliid Fishes, eds Evans, J. P., Pilastro, A., and Schlupp, I., (Chicago, IL: University of Chicago Press), 187–196.
Rosen, D. E., and Bailey, R. M. (1963). The poeciliid fishes (Cyprindontiformes), their structure, zoogeography, and systematics. Bull. Am. Mus. Nat. Hist. 126, 1–176.
Ryan, M. J., Hews, D. K., and Wagner, W. E. (1990). Sexual selection on alleles that determine body size in the swordtail Xiphophorus nigrensis. Behav. Ecol. Sociobiol. 26:231–237. doi: 10.1007/BF00178316
Ryan, M. J., and Wagner, W. E. (1987). Asymmetries in mating preferences between species–female swordtails prefer heterospecific males. Science 236, 595–597. doi: 10.1126/science.236.4801.595
Schrader, M., Travis, J., and Fuller, R. C. (2011). Do density-driven mating system differences explain reproductive incompatibilities between populations of a placental fish? Mol. Ecol. 20, 4140–4151. doi: 10.1111/j.1365-294X.2011.05264.x
Seehausen, O. (2015). Process and pattern in cichlid radiations–inferences for understanding unusually high rates of evolutionary diversification. New Phytol. 207, 304–312. doi: 10.1111/nph.13450
Shackelford, R. M. (1952). Superfetation in the ranch mink. Am. Nat. 86, 311–319. doi: 10.1086/281738
Siebers, D., and Rosenthal, H. (1977). Amino-acid absorption by developing herring eggs. Helgol. Wiss. Meeresunters. 29, 464–472. doi: 10.1007/BF01609984
Smith, A. J., Nelson-Maney, N., Parsons, K. J., Cooper, W. J., and Albertson, R. C. (2015). Body shape evolution in sunfishes: divergent paths to accelerated rates of speciation in the centrarchidae. Evol. Biol. 42, 283–295. doi: 10.1007/s11692-015-9322-y
Sotherland, P. R., and Rahn, H. (1987). On the composition of bird eggs. Condor 89, 48–65. doi: 10.2307/1368759
Swanson, W. J., Clark, A. G., Waldrip-Dail, H. M., Wolfner, M. F., and Aquadro, C. F. (2001). Evolutionary EST analysis identifies rapidly evolving male reproductive proteins in Drosophila. Proc. Natl. Acad. Sci. U.S.A. 98, 7375–7379. doi: 10.1073/pnas.131568198
Taborsky, M., Oliveira, R. F., and Brockmann, H. J. (2008). The evolution of alternative reproductive tactics: concepts and questions, in Alternative Reproductive Tactics: An Integrative Approach, eds Oliveira, R. F., Taborsky, M., and Brockmann, H. J., (Cambridge: Cambridge University Press), 1–21.
Terner, C. (1968) Studies of metabolism in embryonic development. 1. Oxidative metabolism of unfertilized and embryonated eggs of rainbow trout. Comp. Biochem. Physiol. 24, 933–940. doi: 10.1016/0010-406X(68)90805-0
Trainor, B. C., and Basolo, A. L. (2000). An evaluation of video playback using Xiphophorus helleri. Anim. Behav. 59, 83–89. doi: 10.1006/anbe.1999.1289
Travis, J. (1994). Size-dependent behavioral variation and its genetic control within and among populations, in Quantitative Genetic Approaches to Animal Behavior, ed Boake, C. B., (Chicago, IL: University of Chicago Press), 165–187.
Trexler, J. C., and DeAngelis, D. L. (2003). Resource allocation in offspring provisioning: an evaluation of the conditions favoring the evolution of matrotrophy. Am. Nat. 162, 574–585. doi: 10.1086/378822
Trexler, J. C., Travis, J., and Dinep, A. (1997). Variation among populations of the sailfin molly in the rate of concurrent multiple paternity and its implications for mating-system evolution. Behav. Ecol. Sociobiol. 40, 297–305. doi: 10.1007/s002650050345
Turner, C. L. (1937). Reproductive cycles and superfetation in Poeciliid fishes. Biol. Bull. 72, 145–164. doi: 10.2307/1537249
Turner, C. L. (1940). Pseudoamnion, pseudochorion, and follicular pseudoplacenta in poeciliid fishes. J. Morphol. 67, 59–87. doi: 10.1002/jmor.1050670103
Vrana, P. B. (2007). Genomic imprinting as a mechanism of reproductive isolation in mammals. J. Mammol. 88, 5–23. doi: 10.1644/06-MAMM-S-013R1.1
Wagner, C. E., Harmon, L. J., and Seehausen, O. (2012). Ecological opportunity and sexual selection together predict adaptive radiation. Nature 487, 366–370. doi: 10.1038/nature11144
Wolfner, M. F. (2002). The gifts that keep on giving: physiological functions and evolutionary dynamics of male seminal proteins in Drosophila. Heredity 88, 85–93. doi: 10.1038/sj.hdy.6800017
Wourms, J. P. (1981). Viviparity: the maternal-fetal relationship in fishes. Am. Zool. 21, 473–515. doi: 10.1093/icb/21.2.473
Yamaguchi, N., Sarno, R. J., Johnson, W. E., O'Brien, S. J., and MacDonald, D. W. (2004). Multiple paternity and reproductive tactics of free-ranging American minks, Mustela vison. J. Mammal. 85, 432–439. doi: 10.1644/1383939
Zeh, D. W., and Zeh, J. A. (2000). Reproductive mode and speciation: the viviparity-driven conflict hypothesis. BioEssays 22, 938–946. doi: 10.1002/1521-1878(200010)22:10<938::AID-BIES9>3.0.CO;2-9
Zeh, J. A., and Zeh, D. W. (2001). Reproductive mode and the genetic benefits of polyandry. Anim. Behav. 61, 1051–1063. doi: 10.1006/anbe.2000.1705
Zeh, J. A., and Zeh, D. W. (2003). Toward a new sexual selection paradigm: Polyandry, conflict and incompatibility (Invited article). Ethology 109, 929–950. doi: 10.1046/j.1439-0310.2003.00945.x
Zeh, J. A., and Zeh, D. W. (2008). Viviparity-driven conflict: more to speciation than meets the fly. Ann N Y Acad Sci. 1133, 126–148. doi: 10.1196/annals.1438.006
Zimmerer, E. J., and Kallman, K. D. (1989). Genetic basis for altnernative reproductive tactics in the pygmy swordtail, Xiphophrus nigrensis. Evolution 43, 1298–1307. doi: 10.1111/j.1558-5646.1989.tb02576.x
Keywords: viviparity, sexual selection, intergenomic conflict, placenta, Poeciliidae
Citation: Reznick DN, Travis J, Pollux BJA and Furness AI (2021) Reproductive Mode and Conflict Shape the Evolution of Male Attributes and Rate of Speciation in the Fish Family Poeciliidae. Front. Ecol. Evol. 9:639751. doi: 10.3389/fevo.2021.639751
Received: 09 December 2020; Accepted: 22 April 2021;
Published: 21 May 2021.
Edited by:
J. Jaime Zúñiga-Vega, National Autonomous University of Mexico, MexicoReviewed by:
Rudiger Riesch, Royal Holloway, University of London, United KingdomAndrea Pilastro, University of Padua, Italy
Copyright © 2021 Reznick, Travis, Pollux and Furness. This is an open-access article distributed under the terms of the Creative Commons Attribution License (CC BY). The use, distribution or reproduction in other forums is permitted, provided the original author(s) and the copyright owner(s) are credited and that the original publication in this journal is cited, in accordance with accepted academic practice. No use, distribution or reproduction is permitted which does not comply with these terms.
*Correspondence: David N. Reznick, ZGF2aWQucmV6bmljayYjeDAwMDQwO3Vjci5lZHU=