- 1Sustainable Development Study Centre, GC University Lahore, Lahore, Pakistan
- 2Department of Botany, GC University Lahore, Lahore, Pakistan
- 3Plant Ecophysiology and Evolution Group, State Key Laboratory for Conservation and Utilization of Subtropical Agro-Bioresources, Guangxi Key Laboratory of Forest Ecology and Conservation, and College of Forestry, Guangxi University, Nanning, China
Limestone quarrying is an active mining practices generating bulk of solid remains and altering the habitat by the removal of plants; however, the utilization of such waste for the growth of plants has not been investigated much. The present study aimed to evaluate the effects of limestone quarry waste on the growth of two native plants by analyzing its physicochemical properties and utility for plantation purposes, while determining whether mitigation measures would be required for the habitat restoration of quarry site. Two species, Acacia modesta and Adhatoda vasica were selected from the quarry site habitat. These plants were grown in different proportions of quarry waste, and garden soil was used as a control. Growth was assessed by recording plant height, number of branches per plant, root and shoot length, and total biomass. We also analyzed the N, P, K, Na, Ca, and Mg contents of the root and shoot tissues of both species. We found a significant increase in plant height (1.24- and 1.19-fold greater than controls for A. modesta and A. vasica, respectively). Differences in the number branches, root, shoot length, and biomass were also found. A significant and positive relationship was found between the mineral content in roots and the total plant biomass across both species. We conclude that (1) the mining solid waste contained the necessary minerals for the studied plant species and no amelioration would be required for restoration of such sites with the selected indigenous plants; and (2) the quarry waste promoted the growth of the two selected species. The results of the present study can be used to plan habitat restoration in limestone mining areas that have lost plant cover.
Introduction
Mineral deposits are finite and will eventually be exhausted (Jowitt et al., 2020). Mining of minerals is a temporary land use change that alters the original soil strata and produces large amounts of soil remains (Johnson et al., 1994; Basommi et al., 2016). The increasing demand for minerals is causing expansions in mining areas; at present, 57,277 km2 is being used for mining operations on a global scale (Maus et al., 2020). In many mining operations, waste production, and its disposal can cause extensive and long-lasting disturbances on the natural ecological processes such as changes in species composition, primarily due to unstable substrates for plant growth (Ballesteros et al., 2014; Virah-Sawmy et al., 2014; Reta et al., 2018; Sonter et al., 2018). On a large scale, mining operations impact both the abiotic and biotic components of particular ecosystems (Hester et al., 1994; Millennium Ecosystem Assessment, 2005). The abiotic impacts include changes in soil profile, nutrients, texture, and other physicochemical properties such as pH electrical conductivity and production of particulate matter due to crushing of rocks. The assessment of the physicochemical properties of solid waste is essential to understand its fertility status. Khan et al. (2020) and Okereafor et al. (2020) found that some mining operations can release toxic elements such as arsenic, lead, and nickel, which can pollute the soil and water. Mining can impact on ecology through habitat loss and fragmentation (Gajic et al., 2018). Ntshane and Gambiza (2016) reported 20–30% habitat loss due to mining in South Africa. Sonter et al. (2015) found that a significant amount of carbon emissions ascribed to deforestation was linked with charcoal mining. Similarly, mining in Indonesia resulted in a sixfold increase in habitat loss (Paull et al., 2006).
The utilization of large amounts of mining waste for establishing vegetation in mining areas depends on its physicochemical properties. As a general rule, only a few stress-tolerant plant species are able to grow in postmining substrates (Zhang et al., 2017). This is mainly due to high concentration of minerals, scarce availability of organic matter, and moisture and disruption of soil strata (Zeleznik and Skousen, 1996; Gorman et al., 2001; Grant and Koch, 2007; Skousen and Zipper, 2014). Furthermore, the mining areas can also be inhabited by some exotic species, such as Monty et al. (2019), who recorded the significant invasion of alien plants on limestone quarry wastes, which resulted in indigenous species habitat loss. Additionally, limestone quarries can cause soil erosion and dereliction of mined sites (Langer, 2001). A rapid solution for avoiding soil erosion and plant invasion is the plantation of native species for rehabilitating postmining landscapes. Native plant species can repopulate these postmining landscapes and eventually restore native vegetation.
The social and legislative context in many parts of the modern world means that some form of postmining land use and natural ecosystem reestablishment goals will be set before the permission is granted for a new mine (Skousen et al., 1994; Skousen and Zipper, 2014). Species reestablishment and sustainability are the major factors that determine the success of ecological restoration (Rodríguez-Seijo and Andrade, 2017; Li et al., 2018; Song, 2018). Species selection for restoration can be based on the chemical analysis of plant tissues, which can be indicative of species capacities to uptake the minerals in the solid waste of mining sites. Species more apt at mineral uptake can be effective in site restoration and more easily established (Marcus et al., 2018) such plant-tissue analyses that can be helpful in predicting plant growth. Minerals in mining waste can have positive or negative impacts on plant growth, e.g., plant height and branch sprouting (Jim, 2001; Gajic et al., 2018).
Restoration actions should aim to reestablish ecosystem services through species diversity and survival (Montoya et al., 2012). Appropriate measures, such as the possible solid waste amelioration with fertile soil and the selection of suitable species for mineral uptake, should be taken for the utilization of mining solid waste to revegetate the mining site. These cannot only reduce the impacts of mining on such transformed habitats but also increase the population sizes of the selected species (Macdonald et al., 2015; Gentili et al., 2020). Such species are suitable for ground stabilization and to green a barren anew (Bengson, 1995; Yirdaw and Luukkanen, 2003). Furthermore, being adaptive to local environmental and edaphic conditions, these native species can be established as self-sustaining plant communities. Therefore, the use of native plant species for landscape restoration in mining areas or to replace exotic plants can help to reverse the trend of species loss (Richards et al., 1998; Román et al., 2015).
Pakistan has extensive deposits of limestone in the provinces of Punjab, Sindh, Balochistan, and northern areas. These deposits are located in geological formations that range in age from the pre-Cambrian to the Eocene (Hamid et al., 2012). The presence of these deposits attracts mining companies, resulting in accelerated habitat change for the indigenous plants (Drewes et al., 2007). In the limestone quarry, Acacia modesta forms a bi-species climatic climax community with Olea cuspidata, while Adhatoda vasica and Dodonea viscosa both are understory shrubs (Champion et al., 1965). All of these species are valuable sources of livelihood for the local community as they are a source of traditional medicines, fodder, and animal grazing and control soil erosion (Khan, 2013; Khan et al., 2019). Considering the ongoing acceleration of mining impact on natural vegetation, there is a need to utilize the mining solid waste. As the quarry sites with waste heaps become barren, revegetation may begin by planting more productive species such as herbs and shrubs of the area, capable of using the existing postmining solid waste (Swanson et al., 2010). The present study was designed with the following objectives:
(1) To analyze the physicochemical properties of solid waste from the limestone quarry.
(2) To assess the effects of this waste on the growth of selected plant species.
As the restoration of an abandoned site with limestone waste may follow the natural succession, we hypothesized that the shrub A. vasica being the early colonizer may accumulate more minerals in its tissues than A. modesta.
Materials and Methods
Study Site and Quarry Waste
The study was carried out in the limestone quarry of Tobar, in the Jhelum District of Pakistan, located in the salt range (Figure 1). This site is 9 km away from the chemical production facility of a multinational company, where limestone is one of the raw materials used in the manufacturing process. The quarrying operation produces a large amount of solid waste at a rate of 88.18–132 tons/day (Hayyat, 2008). Solid waste from limestone quarrying has a variety of uses, ranging from the manufacturing of masonry blocks to cement production (Felekoglu, 2007; Turgut, 2008). In Pakistan, limestone is cheap and its transport expensive; therefore, the use of limestone quarry waste is limited. The average annual production of limestone is 9.95 tons in Pakistan. Mining has a significant potential for the employment of local people, but the related policies are to be redesigned for sustainability (Government of Pakistan, 2004; Ali and Rehman, 2020).
Waste Material
Solid waste was collected from the mining quarry sites located in Tobar, Punjab, Pakistan (Figures 2a,b). Ten different 30-kg samples of waste were collected randomly from different locations of the waste heap, avoiding particle size >3.0 mm. The samples were mixed to form a composite sample, which was then used for physicochemical analyses.
Physicochemical Analysis of Soil and Plant Tissues
In the laboratory, solid waste samples were crushed in a grinding mill. The particle size of solid waste from the quarry was determined by sieve, passing 0.25–2.25-mm-diameter particle sizes (McLean and Ivimey-Cook, 1952). To ensure the availability of solid waste in the treatments, the material retained on the sieve was reground until the entire sample passed through the sieve. The textural class of the soil was determined using a hydrometer according to the method described by Bouyoucos (1962). Garden soil was used to prepare different concentrations of solid quarry waste for the various treatments. A soil:water suspension (1:1) was prepared (He et al., 2012) to determine the pH using a pH meter (inoLab, Germany). A soil saturation extract was prepared for further analyses. The electrical conductivity was measured using an EC meter (SEnsoDirect Con 200, Netherlands). The nitrogen (N) percentage in the samples was determined according to Jones (1991) using the Kjeldahl method. To estimate the phosphorus content (P), a UV-VIS spectrophotometer (SpectroScan 80 D, Cyprus) was used to measure absorbance at 410 nm, as described by Olsen and Sommers (1982). Calcium (Ca) and magnesium (Mg) were determined by titration, while sodium (Na) and potassium (K) were quantified using flame photometry (AFP 100, United Kingdom).
Experimental Setup
The experiment was carried out in ceramic pots (30 cm in diameter) filled with various garden soil/solid waste mixtures, prepared at the Botanic Garden, GC University, Lahore, Pakistan (Table 1). Five treatments for each species and five replicates of each treatment were prepared. Seeds of A. modesta and cuttings of A. vasica were collected from the study area and raised at the nursery at the Botanic Garden, GC University. Eight-week old plants of uniform height 24 cm (±0.47) for A. modesta and 15 cm (±0.79) for A. vasica were used for the experiment. One plant of each species was grown in each pot and growth data, that is, plant height (cm) and number of branches per plant were measured every 15 days and harvested after 165 days.
Plants Harvesting and Biomass Assessment
After 165 days, all the plants from both species were carefully extracted from the pots, relieved from excessive soil, placed in labeled zipper bags, and brought to the laboratory for further analyses. The parameters recorded for each plant included the following: plant height (cm), number of branches, root length (cm), and dry weight (g) of above- and belowground parts. To obtain the dry weight, the plants were oven-dried to a constant weight at 78°C. Before chemical analyses, plant materials were passed through a stainless-steel grinder with a 20-mesh sieve, and then mixed thoroughly. Mass-based chemical analysis of plant shoot and root tissues was conducted to determine N, P, Na, P, Ca, and Mg contents, as described in the “Physicochemical Analysis of Soil and Plant Tissues” section mentioned above.
Statistical Analysis
Treatment means, standard errors, and least significant differences were calculated for various parameters. One-way analysis of variance (ANOVA) was conducted using the software package COSTAT, version 3.03, to determine the significance of differences among treatment means P < 0.05. Sigma Plot v.14 was used to generate the graphics. SPSS v. 13 was used to analyze the effects of treatment and their different duration on the growth of both species. Plant growth was considered a response variable, while treatment and duration were considered factors.
Results
Physicochemical Analysis of Solid Waste and Garden Soil
The physicochemical analysis of quarry waste and garden soil showed that the particle size of solid waste can be divided into four different classes i.e., ≥2.00, ≥1.00, ≥0.25, and ≤0.25 mm. In the quarry waste used in the present study, the most abundant class was the ≥2.00-mm class; 44.75 ± 7.77% of the quarry waste had this particle size. The second most abundant was the ≤0.25-mm-size class (33.05 ± 12.79%). There were almost equal percentages of particles in the ≥1.00- and ≥0.25-mm-size classes, but greater variations in the percentage of particles in the latter size class. The texture of the solid waste was sandy-gravel, while that of the garden soil was loamy-sand. The pH of the solid waste was slightly lower than that of the soil. The electrical conductivity of the solid waste was higher than that of garden soil. The soil contained higher levels of N, P, K, Mg, and Na than the solid waste. The solid waste had a higher Ca content than garden soil (Table 2).
Effect of Quarry Waste on Plant Height and Number of Branches per Plant
After 165 days of growth, significant differences in the total plant height and number of branches per plant were measured among the different treatments for both species. The maximum height per plant was in T4 (76.66 ± 1.85, 52.31 ± 1.73) and the minimum in T0 (52.0 ± 0.91, 30.1 ± 1.06) for both A. modesta and A. vasica, respectively. Similarly, the maximum number of branches was also in T4 (8.30 ± 1.17) and the minimum in T0 (6.20 ± 0.47) for A. modesta. A. vasica did not exhibit significant differences in its branch number (Figure 3). The changes in the total plant height and number of branches for both species at intervals of 15 days are shown in Supplementary Figures 1–4. Furthermore, the mixed-model analysis showed that the solid waste treatment, number of days, and the interaction of solid waste treatment with the number of days had a significant effect on the total plant height for both species (Table 3).
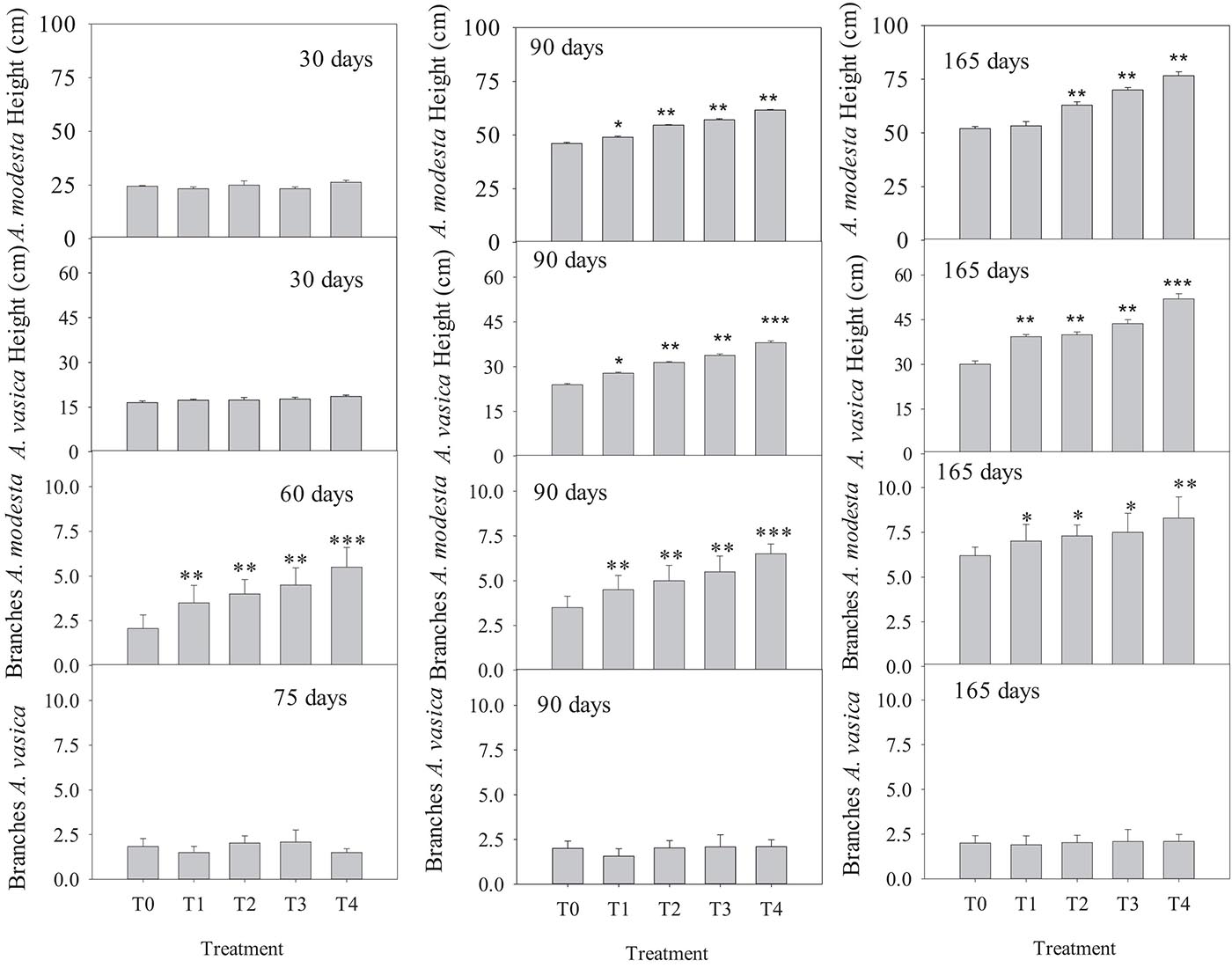
Figure 3. Effect of quarry waste on plant height (cm) and number of branches per plant in Acacia modesta and Adhatoda vasica during the initial months, middle months, and before the harvest. The asterisk indicate the significant difference of treatment means with the control (T0): ∗P < 0.05, ∗∗P < 0.01, and ∗∗∗P < 0.0001.
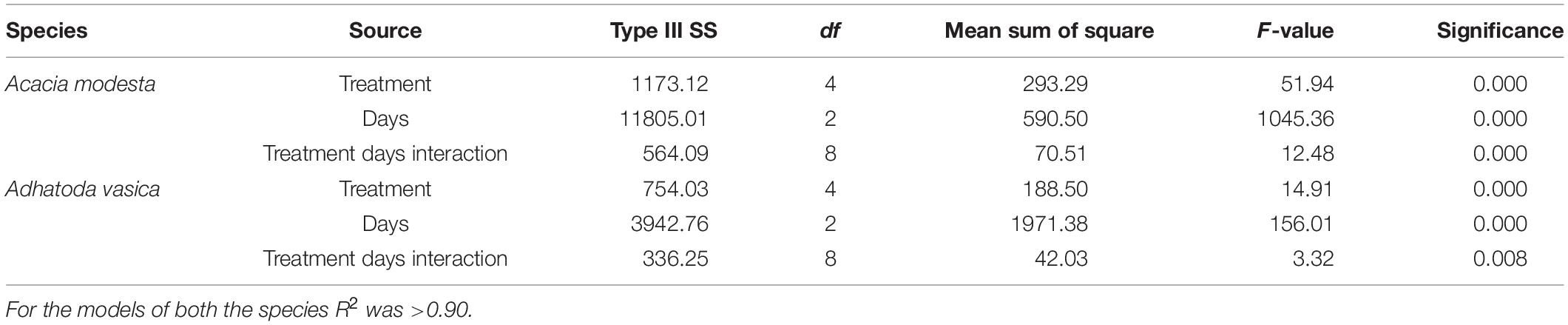
Table 3. The effect of solid waste treatment, duration of days, and their interactions on the growth of Acacia modesta and Adhatoda vasica.
Effect of Quarry Waste on Aboveground, Belowground, and Total Biomass per Plant
There were significant differences in the aboveground biomass in all treatments from T1 to T4 compared with T0. The lowest aboveground biomass was observed in T0, while the highest aboveground biomass was observed in T4. However, the belowground biomass of T1 and T2 was not significantly different from T0, but a significant difference was observed between T3 and T4 as compared with T0. The total biomass of A. modesta was significantly different from that of T1 to T4 in comparison with that of T0 (Figures 4A–C). For A. vasica, the aboveground biomass was significantly greater in T4 than in all other treatments. There were significant differences in the aboveground biomass between T0 and T2, T3, and T4. The belowground biomass was lowest in T0 and highest in T4. The root biomass tended to increase as the percentage of quarry waste in the soil matrix increased. However, significant differences were observed between T3, T4, and T0. The total biomass of A. vasica was significantly greater in the T2, T3, and T4 than T0 (Figures 4D–F).
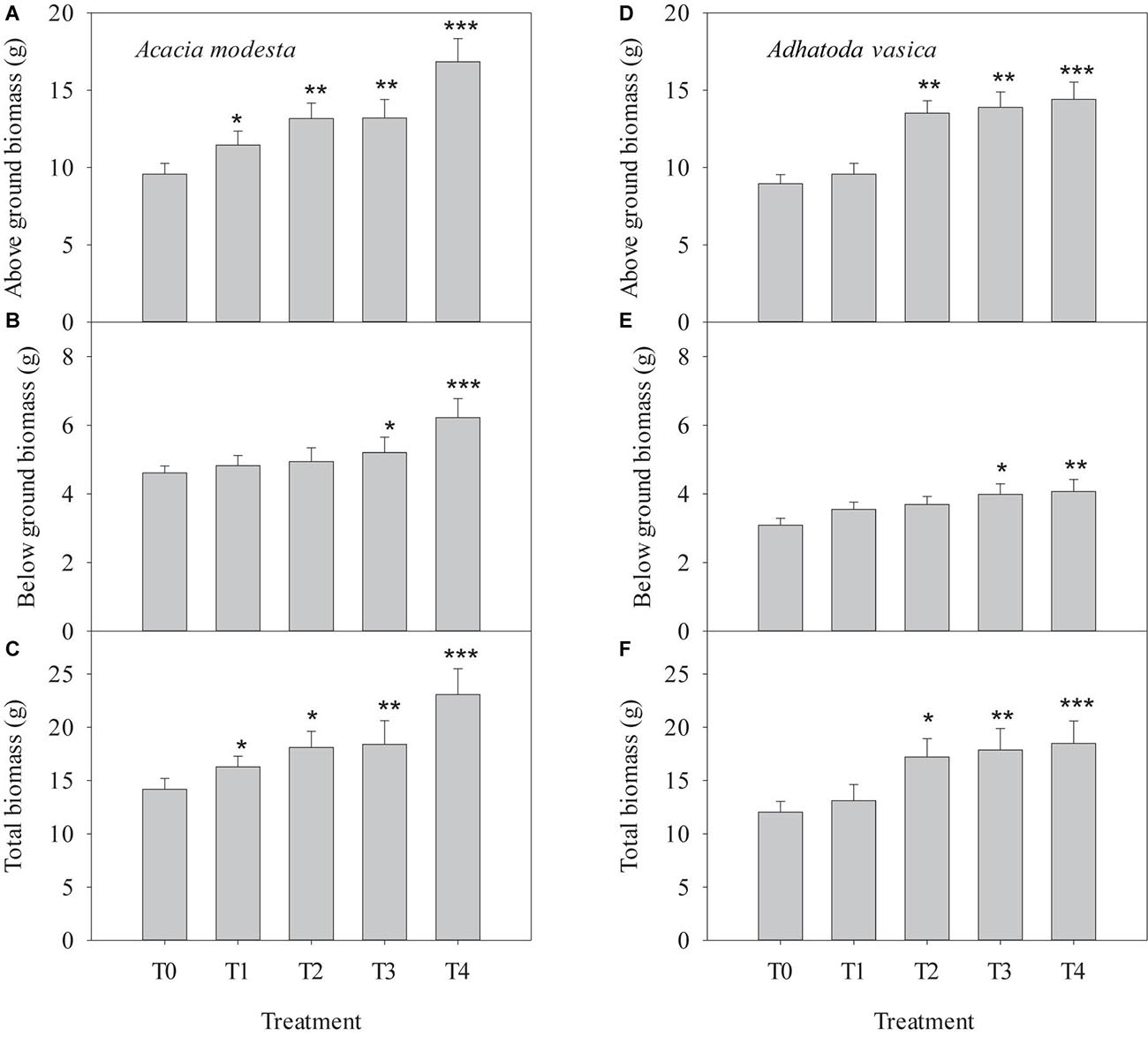
Figure 4. Effect of quarry waste on above ground biomass, below ground biomass, and total biomass in Acacia modesta (A–C) and Adhatoda vasica (D–F). The asterisk indicate the significant difference of treatment means with the control (T0): *P < 0.05, **P < 0.01, and ***P < 0.0001.
Chemical Analyses of Shoot and Root Tissues of A. modesta and A. vasica
For both species, the highest nitrogen content was observed in T4. There were significant differences among the different treatments in terms of mass-based N content percentage (%). Roots showed higher N content than shoots; this pattern was observed in all treatments. The highest P content was in T4 and the lowest in T0, with significant variations among the other treatments. The same pattern was observed for K (highest in T4, lowest in T0, with significant differences among others). Na content was highest in T0 and lowest in T4, and there were significant differences among the other treatments. In both roots and shoots, the highest Ca concentration was observed in T4 and lowest in T0, with significant differences among the others and a lower Ca content in the roots than in the shoots. The Mg concentration was highest in T4 and lowest in T0, but the difference between T4 and T3 was not significant. The magnesium concentration was lower in the roots than in the shoots in all treatments (Tables 4, 5).

Table 4. Chemical analyses of shoot and root tissues of Acacia modesta grown in different concentrations of quarry waste.

Table 5. Chemical analyses of shoot and root tissues of Adhatoda vasica grown in different concentrations of quarry waste.
Correlation of Minerals With Total Plant Biomass
In the studied treatments, a significant and positive relationship was found between the total biomass of A. modesta and A. vasica, respectively, with the N content (R2 = 0.82 and 0.90), P (R2 = 0.89 and 0.96), K (R2 = 0.89 and 0.57), Ca (R2 = 0.97 and 0.70), and Mg (R2 = 0.80 and 0.67) in roots, while the significant and inverse relationship with R2 = 0.84 and 0.70 was found between the Na concentration in roots and total biomass across both A. modesta and A. vasica, respectively. For the relationships between total biomass and P, Na, and Mn the slope was higher for A. vasica than for A. modesta while the slope of relationship between total biomass and K was higher for A. modesta than for A. vasica. However, both species had similar slopes for their relationship with Ca content and total biomass (Figure 5).
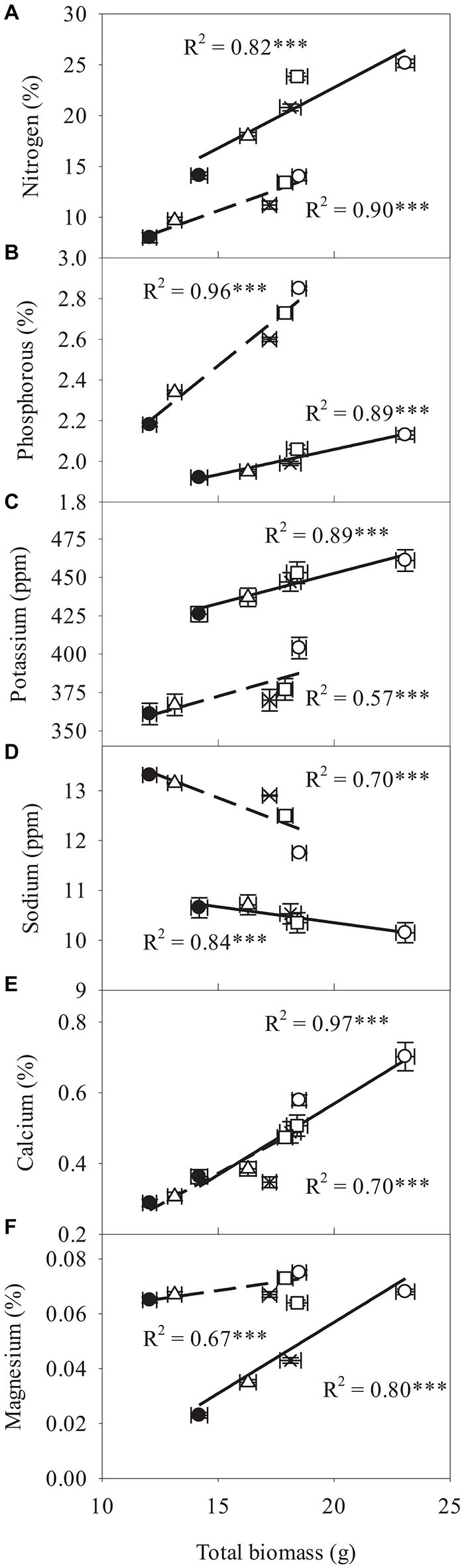
Figure 5. Relationship of total plant oven-dried biomass with nitrogen (A), phosphorus (B), potassium (C), sodium (D), calcium (E), and magnesium (F) in the roots of Acacia modesta (solid lines) and Adhatoda vasica (dashed lines) in T0 (filled circles), T1 (empty triangles), T2 (multiplication symbol), T3 (empty squares), and T4 (empty circles). Each symbol indicates the mean ± SE. ∗∗∗P < 0.0001, all the relationships were significant. Linear function was fitted for all the relationships.
Discussion
Our results showed the enhanced growth of the two selected species grown in the mining waste which provides novel information about habitat restoration by utilizing the two selected woody species A. modesta and A. vasica on mining wastelands. Furthermore, our hypothesis regarding the comparative mineral uptake between the two species was partially supported. The biomass of A. vasica accumulated more Mg, P, and Na and less N and K than A. modesta, while both species accumulated similar amounts of Ca. Such species-specific mineral uptake behavior improves our understanding of the ecological restoration of mining sites with varying mineral concentrations. The enhanced growth of both species in the quarry waste, could be due to the high Ca content (2.37%) along with other nutrients such as N, P, K, and Mg and their uptake by the plants, as shown by their significant correlation with the total biomass. Furthermore, the presence of gravel in the mining soil can lower the substrate water-holding capacity, which could prevent the invasion of Prosopis juliflora (Khan et al., 2019).
Although mining changed the original soil strata inhabited by these species, the solid waste did not have an adverse impact on plant growth, and the treatments seemed likely to have fertilization effect. The treatment efficiency in the long terms was also observed on the growth of both species, indicating the suitability of both species for restoration of solid waste with the mentioned physiochemical properties of soil. Our findings support Richardson and Evans (1986), who utilized grasses for the restoration of limestone quarrying landscapes. They also found that the waste did not contain any toxic elements and promoted the growth of the selected species. Furthermore, our study also revealed that the solid waste areas can be converted into green patches using indigenous species without ameliorating the existing soil; some other exotic species capable of growing in soils with high Ca content could be planted to establish vegetation. These findings can help improve ecosystem restoration in limestone mining activities where habitats have been altered due to excavation.
Our results are also consistent with prior ones reporting that limestone quarry waste is mainly composed of calcium carbonate, which could promote plant growth (Jim, 2001; Elsayed et al., 2017; Marcus et al., 2018). Calcium is a necessary micronutrient and is linked with various ion movements in the plasma. Its uptake can also regulate photosynthesis, carbohydrates, nitrogen assimilation, and the enzymes involved in it (Singh et al., 2018), which can enhance not only the performance of cellular mechanisms but also stress tolerance (Marschner, 1995; Konno et al., 2002; Matthew et al., 2011; Saito and Uozumi, 2020). Ecologists have classified plant species into calcifuges, which occur in soils with low Ca content, and calcicoles, which grow on calcareous soils (Lee, 1999). A. modesta and A. vasica are indigenous to the study area and have the potential to grow better in calcareous soils. However, we found that the Ca content was not uniform in both above- and belowground organs, as the roots had less Ca than shoots, which could be due to the utilization of calcium in the aerial parts for various metabolic processes (Clarkson, 1984).
Our results were consistent with those of Fu et al. (2004), in which Pteroceltis tatarinowii seedlings were grown in Hoagland nutrient solutions with three Ca concentrations (5, 10, and 15 mmol L–1) or without Ca (control). Their results showed that the Ca content in the roots, leaves, and bark of P. tatarinowii increased with increasing Ca concentrations, and higher Ca content was found in leaves than in roots (Marschner, 1995). Kuznetsova et al. (2010) reported that indigenous Pinus sylvestris grew better with high Ca concentrations, and could be a candidate species for mining sites with high calcium content. The inverse correlation of total biomass with sodium concentration supports the findings of Bethke and Drew (1992) and Karim et al. (1993). As in non-halophytic species, high concentrations of sodium ions can reduce plant growth by disturbing plant water relations, unbalancing plant nutrition, and affecting several plant physiological and biochemical processes, which lead to a reduction in plant growth (Misra et al., 2006; Taffouo et al., 2009). Furthermore, the garden soil had more sodium than the solid waste, hence, the decrease in sodium content with the decrease in garden soil in the three treatments, resulted in increased biomass.
Compared with A. modesta, A. vasica absorbed higher amounts of all minerals from the mining waste, as shown in Figures 5A–F. Kasowska et al. (2018) reported some species that could uptake comparatively more minerals from mining waste. A. vesica being the shrubby species and considered to be the initial colonizer could have the potential for higher uptake of minerals, except calcium, as both species had similar uptake of Ca. Although an inverse relationship was found between the biomass and the Na uptake, A. vasica had a larger uptake of Na than A. modesta in a given treatment, indicating its potential to uptake the growth-reducing minerals more than the later colonizers such as trees. The results of the present study show that both A. modesta and A. vasica will be helpful for establishing vegetation on limestone quarry sites with high calcium content.
Conclusion
Both native species (A. modesta and A. vasica) showed increased growth in limestone quarry waste compared with the control. Adaptive to the mining substrate, both of these species can be utilized for the restoration of such mined landscapes without any soil amelioration. Planting these species will not only provide an ecological solution for the disposal of the limestone quarry waste but will also create opportunities for other environmental goods and services, such as timber production, habitat formation, carbon sequestration, erosion control, and natural ecosystem functioning.
Data Availability Statement
The raw data supporting the conclusions of this article will be made available by the authors, without undue reservation.
Author Contributions
MH, ZS, and AK designed the experiment. MH and ZS did the field work. MH and RM conducted the laboratory analysis. MH, ZS, AK, and K-FC analyzed and interpreted the data. All authors contributed the manuscript writing.
Conflict of Interest
The authors declare that the research was conducted in the absence of any commercial or financial relationships that could be construed as a potential conflict of interest.
Acknowledgments
We thank the administration and workers at the quarry site, Tobar District Jhelum, Pakistan, for their cooperation and hospitality. We are grateful to our colleagues who accompanied us in the field and assisted with laboratory work at GC University Lahore. We are also grateful to Bagui Scholarship (C33600992001), Guangxi University, China for the support.
Supplementary Material
The Supplementary Material for this article can be found online at: https://www.frontiersin.org/articles/10.3389/fevo.2021.637833/full#supplementary-material
Supplementary Figure 1 | Effect of quarry waste on plant height (cm) of Acacia modesta over time. The asterisks indicate the significant difference of treatment means with the control (T0): ∗P < 0.05, ∗∗P < 0.01, and ∗∗∗P < 0.0001.
Supplementary Figure 2 | Effect of quarry waste on number of branches per plant in Acacia modesta over time. The asterisks indicate the significant difference of treatments means with the control (T0): ∗P < 0.05, ∗∗P < 0.01, and ∗∗∗P < 0.0001.
Supplementary Figure 3 | Effect of quarry waste on plant height of Adhatoda vasica over time. The asterisks indicate the significant difference of treatments means with the control (T0): ∗P < 0.05, ∗∗P < 0.01, and ∗∗∗P < 0.0001.
Supplementary Figure 4 | Effect of quarry waste on the number of branches per plant in Adhatoda vasica over time.
References
Ali, D., and Rehman, A. U. (2020). Adoption of autonomous mining system in Pakistan-Policy, skillset, awareness and preparedness of stakeholders. Resour. Policy 68:101796. doi: 10.1016/j.resourpol.2020.101796
Ballesteros, M., Cañadas, E. M., Foronda, A., Peñas, J., Valle, F., and Lorite, J. (2014). Central role of bedding materials for gypsum-quarry restoration: an experimental planting of gypsophile species. Ecol. Eng. 70, 470–476. doi: 10.1016/j.ecoleng.2014.06.001
Basommi, L. P., and Guan, Qf, Cheng, Dd, and Singh, S. K. (2016). Dynamics of land use change in a mining area: a case study of Nadowli District, Ghana. J. Mt. Sci. 13, 633–642. doi: 10.1007/s11629-015-3706-4
Bengson, S. A. (1995). Stabilization of copper mine tailings: two decades of management in the arid Southwest. Mining Environ. Manag. 3, 14–17.
Bethke, P. C., and Drew, M. C. (1992). Stomatal and non stomatal components to inhibition of photosynthesis in leaves of Capsicum annum during progressive to NaCl salinity. Plant Physiol. 99, 219–226. doi: 10.1104/pp.99.1.219
Bouyoucos, G. J. (1962). Hydrometer method for making particle size analysis. Agron. J. 54, 464–465. doi: 10.2134/agronj1962.00021962005400050028
Champion, H. G., Seth, S. K., and Khattak, G. M. (1965). Forest Types of Pakistan. Peshawar: Forest Institute.
Clarkson, D. T. (1984). Calcium transport between tissues and its distribution in the plant. Plant Cell Environ. 7, 449–456. doi: 10.1111/j.1365-3040.1984.tb01435
Drewes, H., Ahmad, Z., and Khan, R. (2007). Resource Evaluation of Selected Minerals and Industrial Commodities of the Potwar Plateau Area, Northern Pakistan. Quetta: Geological Survey of Pakistan.
Elsayed, F. A., Abeer, H., Abdulaziz, A. A., Stephan, W., and Dilfuza, E. (2017). Calcium application enhances growth and alleviates the damaging effects induced by Cd stress in sesame (Sesamum indicum L.). J. Plant Interact. 12, 237–243. doi: 10.1080/17429145.2017.1319500
Felekoglu, B. (2007). Utilization of high volumes of limestone quarry wastes in concrete industry (self-compacting concrete case). Resour. Conserv. Recycl. 51, 770–791. doi: 10.1016/j.resconrec.2006.12.004
Gajic, G., Lola, D., Olga, K., SneŽana, J., Miroslava, M., and Pavle, P. (2018). Ecological potential of plants for phytoremediation and ecorestoration of fly ash deposits and mine wastes. Front. Environ. Sci. 6:124. doi: 10.3389/fenvs.2018.00124
Gentili, R., Casati, E., Ferrario, A., Monti, A., Montagnani, C., Caronni, S., et al. (2020). Vegetation cover and biodiversity levels are driven by backfilling material in quarry restoration. Catena 195:104839. doi: 10.1016/j.catena.2020.104839
Gorman, J., Skousen, J. G., Sencindiver, J., and Ziemkiewicz, P. (2001). “Forest productivity and mine soil development under a white pine plantation versus natural vegetation after 30 years,” in Proceedings of the 18th Annual Meeting, American Society for Surface Mining and Reclamation,2–7 (Albuquerque, NM), 103–111. doi: 10.21000/JASMR01010103
Government of Pakistan (2004). IOSMMBI (Investment Oriented Study on Minerals and Mineral Based Industries), Vol. I. Islamabad: Ministry of industries & Production.
Grant, C., and Koch, J. (2007). Decommissioning Western Australia’s first bauxite mine: co-evolving vegetation restoration techniques and targets. Ecol. Mgmt. Restor. 8, 92–105. doi: 10.1111/j.1442-8903.2007.00346
Hamid, K., Alvi, U., Mahmood, R., and Khan, S. (2012). Geochemical evaluation of limestone deposits of Pakistan. J. Himalayan Earth Sci. Peshawar. 45:2.
Hayyat, M. U. (2008). ). A Study of Environmental Impacts of Limestone Quarrying Near Village Tobar, District Jhelum. M. Phil Thesis, GC University Lahore, Lahore.
He, Y., DeSutter, T., Prunty, L., Hopkins, D., Jia, X., and Wysocki, D. A. (2012). Evaluation of 1:5 soil to water extract electrical conductivity methods. Geoderma 18, 12–17. doi: 10.1016/j.geoderma.2012.03.022
Hester, R. E., Harrison, R. M., Johnson, M. S., Cooke, J. A., and Stevenson, J. K. W. (1994). Revegetation of Metalliferous Wastes and Land After Metal Mining. Mining and its Environmental Impact. Letchworth: Royal Society of Chemistry, doi: 10.1039/9781847551467-00031
Jim, C. Y. (2001). Ecological and landscape rehabilitation of a quarry site in Hong Kong. Restor. Ecol. 9, 85–94. doi: 10.1046/j.1526-100x.2001.009001085
Johnson, M. S., Cooke, J. A., and Stevenson, J. K. (1994). “Revegetation of metalliferous wastes and land after metal mining,” in Mining and Its Environmental Impact. Issues Environmental Science and Technology, eds R. E. Hester and R. M. Harrison (Letchworth: Royal Society of Chemistry), 31–48.
Jones, J. B. Jr. (1991). Kjeldahl Method for Nitrogen Determination. Athens, GA: Micro-Macro Publishing, 79.
Jowitt, S. M., Mudd, G. M., and Thompson, J. F. H. (2020). Future availability of non-renewable metal resources and the influence of environmental, social, and governance conflicts on metal production. Commun. Earth Environ. 1:13. doi: 10.1038/s43247-020-0011-0
Karim, M. A., Nawata, E., and Sigenaga, S. (1993). Effects of Salinity and Water stress on the growth, yield and physiological characteristics in hexaploid triticale. Jpn. J. Trop. Agric. 37, 46–52.
Kasowska, D., Gediga, K., and Spiak, Z. (2018). Heavy metal and nutrient uptake in plants colonizing post-flotation copper tailings. Environ. Sci. Pollut. Res. 25, 824–835.
Khan, A. J., Akhter, G., Gabriel, H. F., and Shahid, M. (2020). Anthropogenic effects of coal mining on ecological resources of the central indus basin. Pak. Int. J. Environ. Res. Public Health 17:1255. doi: 10.3390/ijerph17041255
Khan, A. U., Sharif, F., Siddiq, Z., Hayyat, M. U., Shahzad, L., and Gratzfeld, J. (2019). Piloting restoration initiatives in subtropical scrub forest: specifying areas asserting adaptive management. Environ. Monit. Assess. 191:675. doi: 10.1007/s10661-019-7822-4
Khan, I. (2013). Toxic and some essential metals in medicinal plants used in herbal medicines: a case study in Pakistan. Afr. J. Pharm Pharmacol. 7, 1389–1395. doi: 10.5897/AJPP2012.1894
Konno, H., Nakashima, S., Maitani, T., Nakato, T., and Katoh, K. (2002). Pectin-bound β galactosidase present in cell walls of carrot cells under the different calcium status. Physiol. Plantm 114, 213–222. doi: 10.1034/j.1399-3054.2002.1140207.x
Kuznetsova, T., Mandre, M., Kloseiko, J., and Parn, H. (2010). A comparison of the growth of Scots pine (Pinus sylvestris L.) in a reclaimed oil shale post mining area and in a Calluna site in Estonia Environ. Monit. Assess. 166, 257–265.
Langer, W. H. (2001). Potential Environmental Impacts of Quarrying Stone in Karst-A Literature Review. Open-File Report. OF–01–0484. Reston, VA: Geological Survey.
Li, R., Zhang, W., Yang, S., Zhu, M., Kan, S., Chen, J., et al. (2018). Topographic aspect affects the vegetation restoration and artificial soil quality of rock-cut slopes restored by external-soil spray seeding. Sci. Rep. 8:12109.
Macdonald, S. E., Landhäusser, S. M., Skousen, J., Franklin, J., Frouz, J., Hall, S., et al. (2015). Forest restoration following surface mining disturbance: challenges and solutions. New For. 46, 703–732. doi: 10.1007/s11056-015-9506-4
Marcus, A. R., Correia, R. A., Flores, R. M., Prado, W. N., Danilo, E. R., and Diego, W. V. (2018). Using limestone to improve soil fertility and growth of mango (Mangifera Indica L.). Commun. Soil Sci. Plant Anal. 49, 903–912. doi: 10.1080/00103624.2018.1448407
Matthew, G., Maclin, D., Bradleigh, J. H., Bo, X., Simon, J. C., Brent, N. K., et al. (2011). Calcium delivery and storage in plant leaves: exploring the link with water flow. J. Exp. Bot. 62, 2233–2250.
Maus, V., Giljum, S., Gutschlhofer, J., da Silva, D. M., Probst, M., Gass, S. L. B., et al. (2020). A global-scale data set of mining areas. Sci. Data 7:289. doi: 10.1038/s41597-020-00624-w
McLean, R. C., and Ivimey-Cook, W. R. (1952). Text Book of Practical Botany. Islamabad: National Book Foundation.
Millennium Ecosystem Assessment (2005). Ecosystems and Human Well-Being: Synthesis, World Resource Institute. Washington, DC: ISLAND PRESS.
Misra, A., Latowski, D., and Stirzalka, K. (2006). The xanthophyll cycle activity in kidney bean and cabbage leaves under salinity stress. Russ. J. Plant Physl. 53, 102–109. doi: 10.1134/S1021443706010134
Montoya, D., Rogers, L., and Memmott, J. (2012). Emerging perspectives in the restoration of biodiversity-based ecosystem services. Trends Ecol. Evol. 27, 666–672. doi: 10.1016/j.tree.2012.07.004
Monty, A., Jorion, A., Pitz, C., Géron, C., and Mahy, G. (2019). Alien invasive plants in Belgian limestone quarries. Biotechnol. Agron. Soc. Environ. 23, 160–164. doi: 10.25518/1780-4507.17984
Ntshane, B. C., and Gambiza, J. (2016). Habitat assessment for ecosystem services in South Africa. Int. J. Biodiv. Sci. Ecosyst. Serv. Manage. 12, 242–254. doi: 10.1080/21513732.2016.1217935
Okereafor, U., Makhatha, M., Mekuto, L., Uche-Okereafor, N., Sebola, T., and Mavumengwana, V. (2020). Toxic metal implications on agricultural soils, plants, animals, aquatic life and human health. Int. J. Environ. Res. Public Health 17:2204. doi: 10.3390/ijerph17072204
Olsen, S. R., and Sommers, L. E. (1982). “Phosphorus,” in Methods of Soil Analysis, Argon No. 9, Part 2: Chemical and Microbiological Properties, 2nd Edn, ed. A. L. Page (, Madison WI: America Society of Argonmers), 403–430.
Paull, D., Banks, G., Ballard, C., and Gillieson, D. (2006). Monitoring the environmental impact of mining in remote locations through remotely sensed data. Geocarto Int. 21, 33–42. doi: 10.1080/10106040608542372
Reta, G., Dong, X., Li, Z., Su, B., Hu, X., Bo, H., et al. (2018). Environmental impact of phosphate mining and beneficiation: review. Int. J. Hydro. 2, 424–431. doi: 10.15406/ijh.2018.02.00106
Richards, T. R., Jeanne, C. C., and Christopher, R. (1998). Use of native plants on federal lands: policy and practice. J. Range Manage. 51, 625–632. doi: 10.2307/4003603
Richardson, J. A., and Evans, M. E. (1986). Restoration of grassland after limestone quarrying. J. Appl. Ecol. 23, 317–332. doi: 10.2307/2403099
Rodríguez-Seijo, A., and Andrade, M. L. (2017). Characterization of soil physico-chemical parameters and limitations for revegetation in serpentine quarry soils (NW Spain). J. Soil Sediment. 17, 1321–1330. doi: 10.1007/s11368-015-1284-2
Román, F. D., Huayllani, M., Michi, A., Ibarra, F., Muro, R. L., Vazquez, T., et al. (2015). Reforestation with four native tree species after abandoned gold mining in the Peruvian Amazon. Ecol. Eng. 85, 39–46. doi: 10.3390/f10080678
Saito, S., and Uozumi, N. (2020). Calcium-regulated phosphorylation systems controlling uptake and balance of plant nutrients. Front. Plant Sci. 11:44. doi: 10.3389/fpls.2020.00044
Singh, R., Parihar, P., and Prasad, S. M. (2018). Sulfur and calcium simultaneously regulate photosynthetic performance and nitrogen metabolism status in as-challenged brassica juncea L. Seedlings. Front. Plant Sci. 9:772. doi: 10.3389/fpls.2018.00772
Skousen, J., and Zipper, C. (2014). Post-mining policies and practices in the Eastern USA coal region. Intl. J. Coal Sci. Technol. 1, 135–151.
Skousen, J. G., Johnson, C. D., and Garbutt, K. (1994). Natural revegetation of 15 abandoned mine land sites in West Virginia. J. Environ. Qual. 23, 1224–1230. doi: 10.2134/jeq1994.00472425002300060015x
Song, U. (2018). Selecting plant species for landfill revegetation: a test of 10 native species on reclaimed soils. J. Ecol. Environ. 42:30.
Sonter, L. J., Ali, S. H., and Watson, J. E. M. (2018). Mining and biodiversity: key issues and research needs in conservation science. Proc. R. Soc. B 285:20181926. doi: 10.1098/rspb.2018.1926
Sonter, L. J., Barrett, D. J., Moran, C. J., and Soares, B. S. (2015). Carbon emissions due to de-forestation for the production of charcoal used in Brazil’s steel industry. Nat. Clim. Change 5, 359–363. doi: 10.1038/nclimate
Swanson, M., Franklin, J. F., Beschta, R., Crisafulli, C. M., DellaSala, D. A., Hutto, R. L., et al. (2010). The forgotten stage of forest succes-sion: early-successional ecosystems on forest sites. Front. Ecol. Environ. 9:117–125. doi: 10.1890/090157
Taffouo, V. D., Kouamou, J. K., Ngalangue, L. M. T., Ndjeudji, B. A. N., and Akoa, A. (2009). Effects of salinity stress on growth, ions partitioning and yield of some cowpea (Vigna ungiuculata L., walp) cultivars. Int. J. Bot. 5, 135–143. doi: 10.3923/ijb.2009.135.143
Turgut, P. (2008). Properties of masonry blocks produced with limestone sawdust and glass powder. Constr. Build. Mater. 22, 1422–1427. doi: 10.1016/j.conbuildmat.2007.04.008
Virah-Sawmy, M., Ebeling, J., and Taplin, R. (2014). Mining and biodiversity offsets: a transparent and science based approach to measure ‘no-net-loss’. J. Environ. Manage. 143, 61–70. doi: 10.1016/j.jenvman.2014.03.027
Fu, X., Fang, S., Tian, Y., and Hong, S. (2004). Effect of Ca+2 concentration on the growth and bark quality of Pteroceltis tatarinowii. J. Appl. Ecol. 15, 1502–1506.
Yirdaw, E., and Luukkanen, O. (2003). Indigenous woody species diversity in Eucalyptus globulus Labill. Ssp. Globulus plantations in the Ethiopian highlands. Biodivers. Conserv. 12, 567–582.
Zeleznik, J. D., and Skousen, J. G. (1996). Survival of three tree species on old reclaimed surface mines in Ohio. J. Environ. Qual. 25, 1429–1435. doi: 10.2134/jeq1996.00472425002500060037x
Keywords: Acacia modesta, calcium, mining, habitat restoration, biomass, mineral uptake
Citation: Hayyat MU, Siddiq Z, Mahmood R, Khan AU and Cao K-F (2021) Limestone Quarry Waste Promotes the Growth of Two Native Woody Angiosperms. Front. Ecol. Evol. 9:637833. doi: 10.3389/fevo.2021.637833
Received: 04 December 2020; Accepted: 11 March 2021;
Published: 29 April 2021.
Edited by:
Yuanzhi Li, Sun Yat-sen University, ChinaReviewed by:
Rodolfo Gentili, University of Milano-Bicocca, ItalyWenqi Luo, Sun Yat-sen University, China
Copyright © 2021 Hayyat, Siddiq, Mahmood, Khan and Cao. This is an open-access article distributed under the terms of the Creative Commons Attribution License (CC BY). The use, distribution or reproduction in other forums is permitted, provided the original author(s) and the copyright owner(s) are credited and that the original publication in this journal is cited, in accordance with accepted academic practice. No use, distribution or reproduction is permitted which does not comply with these terms.
*Correspondence: Zafar Siddiq, zafareco@outlook.com; Kun-Fang Cao, kunfangcao@gxu.edu.cn