- 1Environmental and Marine Biology, Åbo Akademi University, Turku, Finland
- 2Centre d’Etudes Biologiques de Chizé, CNRS-ULR, UMR 7372, Villiers-en-Bois, France
- 3Nature and Game Management Trust Finland, Degerby, Finland
- 4Novia University of Applied Sciences, Ekenäs, Finland
Predation risk affects the costs and benefits of prey life-history decisions. Predation threat is often higher during reproduction, especially in conspicuous colonial breeders. Therefore, predation risk may increase the survival cost of breeding, and reduce parental investment. The impact of predation risk on avian parental investment decisions may be hormonally mediated by prolactin and corticosterone, making them ideal tools for studying the trade-offs involved. Prolactin is thought to promote parental care and commitment in birds. Corticosterone is involved in allostasis and may either mediate reduced parental investment (corticosterone-fitness hypothesis), or promote parental investment through a reallocation of resources (corticosterone-adaptation hypothesis). Here, we used these hormonal proxies of incubation commitment to examine the impact of predation risk on reproduction in common eiders (Somateria mollissima) breeding in the Baltic Sea. This eider population is subject to high but spatially and temporally variable predation pressure on adults (mainly by the white-tailed eagle Haliaeetus albicilla and introduced mammalian predators) and nests (by the adult predators and exclusive egg predators such as hooded crows Corvus cornix). We investigated baseline hormonal levels and hatching success as a function of individual quality attributes (breeding experience, female and duckling body condition), reproductive investment (clutch weight), and predation risk. We expected individuals nesting in riskier environments (i.e., on islands where predation on adults or nests is higher, or in less concealed nests) to reduce their parental investment in incubation, reflected in lower baseline prolactin levels and either higher (corticosterone-fitness hypothesis) or lower (corticosterone-adaptation hypothesis) baseline corticosterone levels. Contrary to our predictions, prolactin levels showed a positive correlation with nest predation risk. The unexpected positive relationship could result from the selective disappearance of low-quality females (presumably having low prolactin levels) from risky sites. Supporting this notion, female body condition and hatching success were positively correlated with predation risk on females, and baseline prolactin concentrations were positively correlated with duckling body condition, a proxy of maternal quality. In line with the corticosterone-adaptation hypothesis, baseline corticosterone levels increased with reproductive investment, and were negatively associated with nest predation risk. Hatching success was lower on islands where nest predation risk was higher, consistent with the idea of reduced reproductive investment under increased threat. Long-term individual-based studies are now needed to distinguish selection processes occurring at the population scale from individually plastic parental investment in relation to individual quality and variable predation risk.
Introduction
Predation exerts both direct and indirect effects on prey, and these effects show considerable spatiotemporal variation (Lima, 1998; Laundré et al., 2010). Whereas predators affect prey populations by direct killing, their mere presence also induces behavioral and physiological changes in prey (Lima, 1998). Such changes include increased aggregation tendency (Lehtonen and Jaatinen, 2016), altered habitat use (Turner, 1996; Creel et al., 2005), increased sensitivity to disturbance (Gómez-Serrano, 2020), and altered activity budgets (Pöysä, 1987; Blumstein and Daniel, 2005; Öst and Tierala, 2011). During the breeding season, the presence of predators affects the costs and benefits of parental investment, often leading to an exacerbated “cost of reproduction” (Slagsvold, 1984; Magnhagen, 1991). Indeed, reproducing prey are particularly exposed to predation by the risky behaviors they adopt to find a partner, mate, and successfully raise their offspring (Thomas, 1988; Magnhagen, 1991; Arnold et al., 2012). Consequently, decreased investment in current reproduction is expected under elevated predation risk, a prediction verified in birds laying smaller clutches when exposed to high risk (Doligez and Clobert, 2003; Eggers et al., 2006; Zanette et al., 2011; Noreikiene et al., 2021).
Reproduction is a costly life-history event not only because of its effect on survival (Magnhagen, 1991; Schwarzkopf and Shine, 1992; Arnold et al., 2012), but also because of elevated energy expenditure (Krapu, 1981). Life-history theory thus predicts a trade-off between individual investment in reproduction and survival (Williams, 1966). The cost of reproduction varies depending on individual state and quality (Feifarek et al., 1983; Reznick, 1985; Hamel et al., 2009), but also prevailing environmental conditions (De Steven, 1980; Reznick, 1985). In long-lived species, current parental investment is generally expected to be higher under favorable environmental conditions and individual states, i.e., when resources are plentiful (Whittingham and Robertson, 1994; Storey et al., 2017), weather conditions are benign (Thierry et al., 2013), predation risk is low (Ghalambor and Martin, 2000), and when individuals are in good condition (Chastel et al., 1995; Tveraa et al., 1998). On top of these factors, long-lived species should invest more into current reproduction as the prospects of future reproduction diminish with advancing age (the restraint hypothesis, Curio, 1983; Heidinger et al., 2006), even if environmental conditions are far from being optimal. This prediction has been well supported empirically (Forslund and Pärt, 1995; Préault et al., 2005; Angelier et al., 2007a, b). As a consequence of this survival-reproduction trade-off, individuals are predicted to modulate their allocation to current reproduction (Reznick, 1985), and may even completely refrain from current reproduction by skipping breeding under prohibitively high costs of reproduction (Chastel et al., 1995; Erikstad et al., 1998; Bruinzeel, 2007; Öst et al., 2018).
Hormonal mechanisms are involved in the mediation of life-history decisions when coping with a range of stressors including predation risk (Ricklefs and Wikelski, 2002). In birds, prolactin and corticosterone are especially relevant to study parental investment. Prolactin is the main hormone involved in the expression of parental behavior, and prolactin levels can be a reliable proxy of parental investment and reproductive involvement (Angelier and Chastel, 2009; Angelier et al., 2016; Smiley, 2019). Consequently, circulating blood prolactin concentrations are elevated during reproduction (Criscuolo et al., 2002; Vleck and Vleck, 2011). Furthermore, individuals with higher prolactin levels may provide better parental care (Angelier and Chastel, 2009; Smiley and Adkins-Regan, 2016, 2018), such as higher brood provisioning (Miller et al., 2009; Smiley and Adkins-Regan, 2016, 2018) and incubation commitment (Angelier et al., 2015; Wang et al., 2020), or even shorter latency to return to the nest after a disturbance (Angelier et al., 2009; Hope et al., 2020). Importantly, circulating prolactin levels have been shown to decrease in response to stressor, poor body condition, or low food availability (Delehanty et al., 1997; Groscolas et al., 2008; Angelier and Chastel, 2009; Riechert et al., 2014a). This is associated with a concomitant reduction of parental care, suggesting that prolactin may mediate parental responses to environmental conditions (Angelier et al., 2016).
Corticosterone, on the other hand, is involved in the maintenance of homeostasis (McEwen and Wingfield, 2003; Romero et al., 2009) and in the response to environmental challenges (Angelier and Wingfield, 2013; Wingfield, 2013). The corticosterone-fitness hypothesis states that circulating corticosterone levels are negatively associated with performance and fitness, because elevated baseline corticosterone levels may reflect higher energetic demand induced by deteriorating environmental conditions (Wingfield et al., 1983; Kitaysky et al., 2007), and may be associated with reduced survival and breeding success (Silverin, 1986; Angelier et al., 2010; Goutte et al., 2010; Vitousek et al., 2018; Breuner and Berk, 2019). For example, high circulating corticosterone levels have been shown to be involved in a reduction of parental care (Silverin, 1986; Angelier et al., 2009; Ouyang et al., 2011; Lynn, 2016; Schoenle et al., 2017), or even induce nest abandonment (Wingfield et al., 1983; Robin et al., 1998; Groscolas and Robin, 2001; Spée et al., 2011). In contrast, circulating corticosterone levels may also be positively associated with fitness, as stated by the corticosterone-adaptation hypothesis (Bonier et al., 2009). Indeed, elevated baseline corticosterone levels may enhance energy mobilization (Romero, 2002; Breuner, 2011) and therefore promote reproductive effort (Riechert et al., 2014a). For example, elevated baseline corticosterone levels have been associated with higher incubation effort (Ouyang et al., 2013), higher brood provisioning (Miller et al., 2009; Bonier et al., 2011; Ouyang et al., 2013), or higher offspring production (Bonier et al., 2011; Love et al., 2014). Importantly, the association between fitness and corticosterone may be dose-dependent (Bonier et al., 2009). In line with hormetic mechanisms (Costantini et al., 2010; Costantini, 2014), the corticosterone-fitness hypothesis may be validated under high levels of baseline corticosterone, while the corticosterone-adaptation hypothesis may be validated under lower baseline corticosterone levels.
Here, we investigate to what extent prolactin and corticosterone—two endocrine mediators of parental investment—are affected by predation risk in a long-lived ground-nesting bird, the common eider (Somateria mollissima), hereafter eider. Specifically, baseline prolactin and corticosterone levels have been shown to be closely related to incubation commitment in several species (Ouyang et al., 2013; Schoenle et al., 2017; Bowers et al., 2019; Hope et al., 2020), including the eider (Criscuolo et al., 2002, 2005). We therefore expect female eiders, as a long-lived species, to display lower baseline prolactin levels and either higher (corticosterone-fitness hypothesis) or lower (corticosterone-adaptation hypothesis) baseline corticosterone levels at the end of incubation if they reduce their investment in incubation as a response to increased predation threat. In our study area in SW Finland, northern Baltic Sea, female eiders nest on islands showing substantial spatial and temporal variation in predation pressure on adults and their nests (Öst et al., 2018) as well as in nest concealment (Öst and Steele, 2010), allowing us to test the impact of predation risk on variation in these endocrine traits and hatching success. We analyzed baseline hormone levels (prolactin and corticosterone) as a function of direct or indirect proxies of female quality (i.e., breeding experience, female body condition, offspring body condition), energetic investment in current reproduction (clutch weight), and predation risk on incubating female eiders and nests. In addition, we examined the endocrine correlates of reproductive success (i.e., hatching success). We expect (1) higher baseline prolactin and either lower (corticosterone-fitness hypothesis) or higher (corticosterone-adaptation hypothesis) baseline corticosterone levels in high-quality breeders and/or birds producing heavier clutches, as experience and condition are known to influence hormone levels (Angelier et al., 2007b; Bókony et al., 2009; Breuner, 2011). We also expect (2) birds nesting in riskier environments (i.e., on islands where predation on adults or nests is higher, or in less concealed nests) to have lower prolactin levels and either higher (corticosterone-fitness hypothesis) or lower (corticosterone-adaptation hypothesis) corticosterone levels, reflecting lower investment in incubation under increased threat. Finally, due to the link between parental investment and both prolactin and corticosterone levels (Angelier and Chastel, 2009; Bonier et al., 2009), we expect (3) lower baseline prolactin levels and either higher (corticosterone-fitness hypothesis) or lower (corticosterone-adaptation hypothesis) baseline corticosterone levels to be associated with poorer reproductive performance (i.e., reduced hatching success).
Materials and Methods
Study Area and Species
Study Area and Female Monitoring
Fieldwork was conducted in the archipelago surrounding Tvärminne Zoological Station (59°50′N, 23°15′E), in the western Gulf of Finland, in 2013. In this area, female eiders nest on islands of various sizes (N= 17 islands, mean area ± SD = 1.78 ± 2.72 ha, min = 0.13 ha, max = 10.22 ha). Vegetation cover varies from bare rocks and sparse patches of herbs or stands of juniper (Juniperus communis) to pine (Pinus sylvestris) dominated forest. The study islands are relatively close to each other, typically only hundreds of meters apart, in a total study area of ca 15 km2. Nevertheless, islands vary considerably in predation risk on both incubating females and their nests (Öst et al., 2011), due to variable forest cover (Ekroos et al., 2012; Öst et al., 2018) and range of available nest micro-habitats in terms of concealment (Seltmann et al., 2014). Importantly, the breeding period represents a period of elevated predator-induced mortality in this ground-nesting bird, and thus the survival of adult females is lower than that of males in eider populations subject to significant predation risk (Ramula et al., 2018).
Eiders are long-lived ground-nesting birds, with a mean life expectancy of ca. 21 years (Coulson, 1984). The eider breeding population at Tvärminne has been monitored every year since 1990. Incubating female eiders were trapped on their nest with hand nets between May and early June to measure hormone levels (N = 148 out of 355 breeding females in 2013). Females incubate for about 26 days (Korschgen, 1977), and incubating birds were captured predominantly during the later phase of incubation to minimize nest abandonment (mean ± SD = 17.6 ± 4.6 days after the start of incubation, range = 6.5–25.5 days). Incubation stage was estimated using an egg floatation test (Kilpi and Lindström, 1997). Each trapped female was ringed with a standard metal ring and a unique color-ring combination, weighed to the nearest 5 g with a Pesola spring balance, and its radius-ulna length was measured to the nearest 1 mm with a wing ruler as a proxy of body size.
Breeding Experience, Body Condition, and Clutch Weight
We characterized female breeding experience using a qualitative indicator (Jaatinen and Öst, 2011). We categorized females as either experienced (already ringed when captured) or inexperienced breeders (unringed at capture; only adult breeders are ringed in the study area). As eiders are philopatric (Swennen, 1990, 1991; Coulson, 2016) and show high nest-site fidelity (Öst et al., 2011; Coulson, 2016), and as the majority of breeding females are captured annually (trapping success in 2013 = 65.1%), with a constant trapping effort since 1996 (Jaatinen and Öst, 2011), this indicator of breeding experience can be considered reliable.
For each trapped female, we calculated two indices of body condition: one measured when the bird was trapped and sampled for blood (physiological parameters are expected to vary according to the instantaneous condition at the time of sampling, Kitaysky et al., 1999; Riechert et al., 2014b), and the other estimated at hatching (to allow direct comparison between females by taking into account weight loss during incubation, see below). To avoid any weight bias due to females potentially still laying eggs, only females that had incubated for more than 5 days were kept for further analyses. Body condition at trapping was calculated as the standardized residuals of a linear regression of log-transformed weight on log-transformed radius-ulna length. As eiders fast and lose weight during incubation (Criscuolo et al., 2000), it is essential to take into account the incubation stage to compare individual differences in energy reserves, in addition to structural size. Hence, we estimated body condition at hatching. To do so, we used the standardized residuals of a linear regression of log-transformed estimated weight at hatching on log-transformed radius-ulna length (Öst et al., 2008). Weight at hatching was estimated as the weight of the female at trapping subtracted by the estimated weight that the female was expected to lose during the remaining incubation time (estimated log-transformed daily weight loss: E ± SE = −0.221 ± 0.004, t = −51.64, P < 0.001). This estimate of mean weight loss rate during incubation was derived as the slope of the linear regression of log-transformed body mass on log-transformed incubation time and projected hatching date (see Öst and Steele, 2010). In addition, for each trapped female, total clutch weight was measured to the nearest 1 g in order to control for a potential influence of clutch weight on endocrine traits. Because eggs lose mass during development, we corrected clutch mass for incubation stage, evaluated by egg floatation, by extracting the residuals obtained from the linear regression of clutch weight on incubation stage.
Endocrine Correlates of Parental Investment
In 2013, a total of 148 breeding female eiders were trapped on the nest and sampled for blood (∼1.5 mL) from the ulnar vein within 3 min following capture (mean ± SD = 2.42 ± 0.35 min, range = 1.67–3.22 min) in order to obtain baseline hormonal levels (Romero and Reed, 2005). Baseline corticosterone levels were not related to sampling time (linear regression: t = 1.23, P = 0.22, r2 = 0.004); hence the two blood samples taken after 3 min (3.12 and 3.22 min) were kept for further analyses. Blood samples were centrifuged and plasma was stored at −20°C until assay. Plasma concentrations of corticosterone and prolactin were determined by radioimmunoassay at the Centre d’Etudes Biologiques de Chizé (CEBC), as previously described (corticosterone: Lormée et al., 2003; Öst et al., 2020); prolactin: Criscuolo et al., 2002) (corticosterone, 5 assays, inter-assay CV: 14.31%, intra-assay CV: 10.97%; prolactin, 2 assays, inter-assay CV: 12.05%, intra-assay CV: 11.32%).
Hatching Success and Offspring Condition
For each nest, hatching success (0: failure, 1: success) was determined by returning to the nest at the end of incubation and monitoring the presence of ducklings. The eider is a precocial species and ducklings usually leave the nest within 24 h of hatching (Öst and Bäck, 2003). If no ducklings were found in the nest, they had either already hatched and left the nest, or the nest had been depredated. Consequently, egg shells were examined to determine if the breeding attempt had been successful or not. Hatched eggs were recognizable by their intact leathery membrane while depredated nests had either no eggs or eggs broken into pieces, usually with a bloody membrane still attached to the shells (Bolduc et al., 2005; Öst and Steele, 2010). A nest was considered as hatched if at least one duckling or one hatched egg membrane was found.
If ducklings (N = 209) were found in the nest (N = 66 out of 93 successful nests), their tarsus length was measured to the nearest 0.1 mm with a Vernier caliper and they were weighed to the nearest 1 g. Duckling body condition was estimated as a proxy of offspring quality by extracting the standardized residuals of the linear regression of log-transformed body weight against log-transformed tarsus length (Öst et al., 2020).
Assessment of Predation Risk
Nest-Site Micro-Habitat
Females breed on the ground under variable cover and mainly rely on crypsis to avoid being detected by predators. Concealment mainly offers protection from avian predators, and to a lesser extent from mammalian predators. To quantify nest concealment, we measured the amount of nest cover (including all layers of vegetation from ground level to canopy, including rocks) above each nest based on hemispherical photographs. To do so, we used a 42-mm fisheye lens mounted on an Olympus C-740 digital camera placed in each nest cup and aimed vertically (Öst and Steele, 2010; Jaatinen et al., 2014). Each picture was converted to black and white and the proportion of black pixels (corresponding to vegetation or other obstacles, e.g., rocks) was measured using Image Tool (version 3.00; University of Texas Health Science Center, San Antonio).
Island-Specific Female and Nest Predation Risk
Breeding females and their eggs are subject to predation from native and non-native species. The main predators of incubating females are the native white-tailed eagle (Haliaeetus albicilla), the population of which has increased rapidly since the 1990s (Stjernberg et al., 2005; Öst et al., 2018), the eagle owl (Bubo bubo) as well as two introduced mammals: the American mink (Neovison vison) and the raccoon dog (Nyctereutes procyonoides) (Jaatinen and Öst, 2013; Öst et al., 2018). In addition, eggs are depredated either by primary predators of female eiders, or by nest predators such as hooded crows (Corvus cornix) or gulls (Larus spp.) (Jaatinen et al., 2014). The latter consume eggs either when the female is absent from the nest or act as secondary predators after the female has been attacked on the nest.
In order to distinguish between these different types of predation, we determined for each island an index of adult female and nest predation risk, respectively. These two indices are based on confirmed cases of depredated females or nests rather than unsuccessful predation attempts, as the latter are impossible to document in the field without camera surveillance. However, one should expect a close correlation between the incidence of successful and unsuccessful predation attempts. Female predation risk was calculated as the island-specific proportion of killed nesting females (number of killed females divided by the number of breeding attempts). Island-specific nest predation risk was calculated based on hatching success information (see above). The number of successful (i.e., hatched) nests at the final nest fate census was divided by the number of nests on the island for whose fate (success or failure) was known (326 known nest fates out of 355 monitored nests; 91.8% of known nest fates; mean number of nests per island ± SD = 31 ± 17 nests). The main reason for nest failure was depredation (136 out of the 155 failed nests; 87.7%) and the low number of non-depredated but failed nests could be explained by females incubating infertile or unviable eggs. Thus, island-specific nest predation was calculated as: 1-island-specific proportion of successful nests. Both island-specific female and nest predation risk varied considerably between islands (female predation risk: mean ± SD = 0.03 ± 0.04, min = 0.00, max = 0.10; nest predation risk: mean ± SD = 0.42 ± 0.20, min = 0.00, max = 0.86, N = 17 islands).
Statistical Analyses
All statistical analyses were conducted in R 3.6.1. (R Core Team, 2018). We used linear mixed models (LMMs) (R function “lme,” nlme package; Pinheiro et al., 2019) and generalized linear mixed models (GLMMs) (R function “glmer,” lme4 package; Bates et al., 2015) to investigate variation in baseline prolactin and corticosterone levels, reproductive success and duckling body condition, in relation to the aforementioned individual and environmental explanatory variables. We checked that all models met the assumptions of normality and homoscedasticity of residuals. Consequently, baseline corticosterone levels were log-transformed as ln(x + 1) to meet model assumptions. Model selection was performed using a stepwise backward elimination method in which non-significant terms (P > 0.05) were eliminated at each step. We preferred a stepwise model selection approach due to its ease of interpretation and presentation, but acknowledge its potential limitations (Smith, 2018). To verify the robustness of our model selection procedure, we therefore also performed conditional model averaging across best-ranked models (ΔAICc < 2) for the dependent variables, using the function “dredge” in the R-package MuMIn (Barton, 2020). This robustness check confirmed that we had correctly identified the final models and significance of each included explanatory variable for nearly all analyses in this paper. The only exception was the analysis of female body condition at hatching; here, the association with adult predation risk was only a trend when performing model averaging (see Results below). For each model, we relied on the variance inflation factor (VIF) to look for multicollinearity among candidate variables. We did not detect any multicollinearity among the candidate variables of selected models (all VIF < 2.5; Allison, 2012).
Physiological Responses
To study the variation in female physiology in relation to proxies of individual quality, maternal investment and predation risk, we constructed two sets of LMMs, where baseline prolactin and corticosterone levels were included as the dependent variable, respectively. The explanatory individual state variables were body condition at trapping, breeding experience, incubation stage, radius-ulna length, and incubation-stage corrected clutch weight. Baseline corticosterone was also included in the models investigating baseline prolactin variation to assess the potential effect of corticosterone on prolactin levels (Angelier and Chastel, 2009; Angelier et al., 2009; Vleck and Vleck, 2011). Furthermore, the following predation risk related explanatory variables were included in the models: nest cover, nest predation risk and female predation risk. One extremely high baseline prolactin value (value = 343.82 ng/mL, mean ± SD = 149.56 ± 43.81 ng/mL, min = 57.56 ng/mL, max = 343.82 ng/mL) was removed from statistical analyses. To account for the potential non-independence of physiological responses of individuals breeding on the same island, island identity was added to all models as a random effect.
Hatching Success and Duckling Quality
As reproductive success followed a binomial distribution (0: failure, 1: success), we built GLMMs with a binomial error distribution, with reproductive success as the dependent variable and baseline prolactin and corticosterone levels, individual characteristics (body condition at hatching, breeding experience, radius-ulna length, and clutch weight) and environmental factors (nest cover, island-specific nest and female predation risk) as explanatory variables. As the probability of a nest being depredated is likely to decrease as it gets closer to hatching (due to a shorter time left for the predator to detect the nest), we also included incubation stage (i.e., the number of days since egg laying) as an explanatory variable. Island identity was included as a random effect to account for the potential pseudoreplication arising from repeated observations from each island.
We used LMMs to study the association between female baseline prolactin and corticosterone levels (explanatory variables) and duckling body condition (dependent variable), a proxy of offspring quality. As ducklings belonging to the same clutch are not independent from each other, and as we expect non-independence between the body conditions of ducklings hatching on the same islands, clutch and island identity were added as random effects in these models.
Body Condition at Hatching
Covariation between individual quality and reproductive success may influence the observed relationship between maternal hormone profiles and parental investment, e.g., if early breeding failure disproportionately affects poor-quality individuals. To assess whether such selective disappearance may occur, we analyzed variation in female body condition, a proxy of female quality, in relation to female attributes and predation risk. In these LMMs, female body condition at hatching was the dependent variable and breeding experience, nest predation risk and female predation risk were explanatory variables. Island identity was included as a random effect to account for the non-independence of body conditions of females nesting on the same island.
Results
Baseline Prolactin in Relation to Individual and Environmental Characteristics
The final model explaining variation in baseline prolactin levels only included nest predation risk (Table 1). Thus, baseline prolactin levels showed a significant positive association with increasing nest predation risk (nest predation risk effect, parameter estimate ± standard error: E ± SE = 45.41 ± 21.24; Table 1 and Figure 1A), but showed no significant association with female predation risk. Likewise, the final model explaining variation in baseline prolactin did not show a significant association between baseline prolactin levels and breeding experience, structural size, condition at trapping, clutch weight, or nest cover.
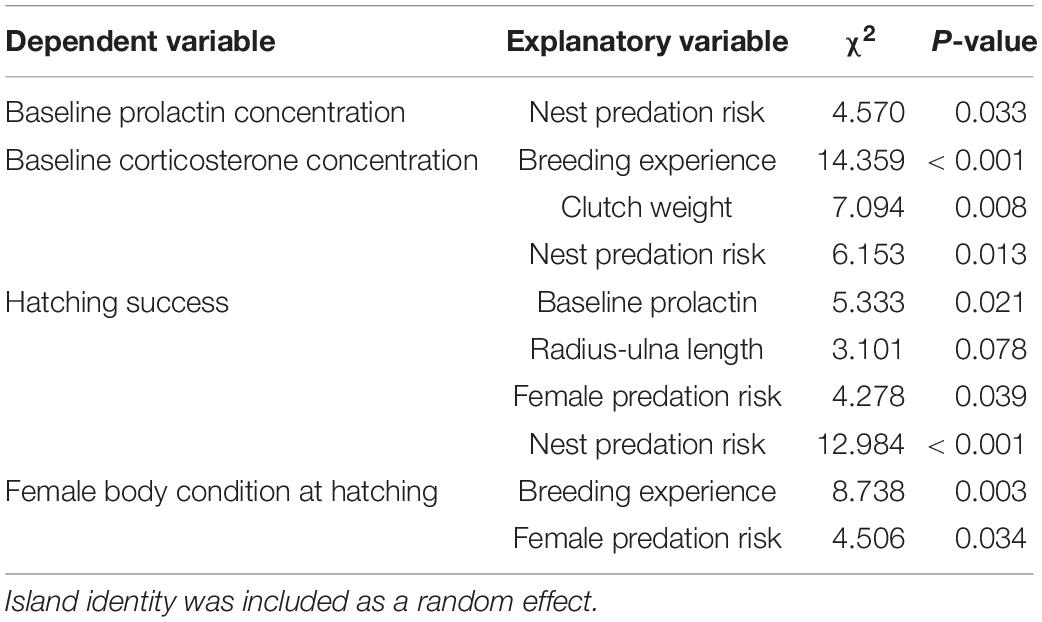
Table 1. Final LMMs and GLMM explaining baseline prolactin variation, baseline corticosterone variation, hatching success or body condition of incubating female eiders in relation to individual and environmental explanatory variables after stepwise backward elimination.
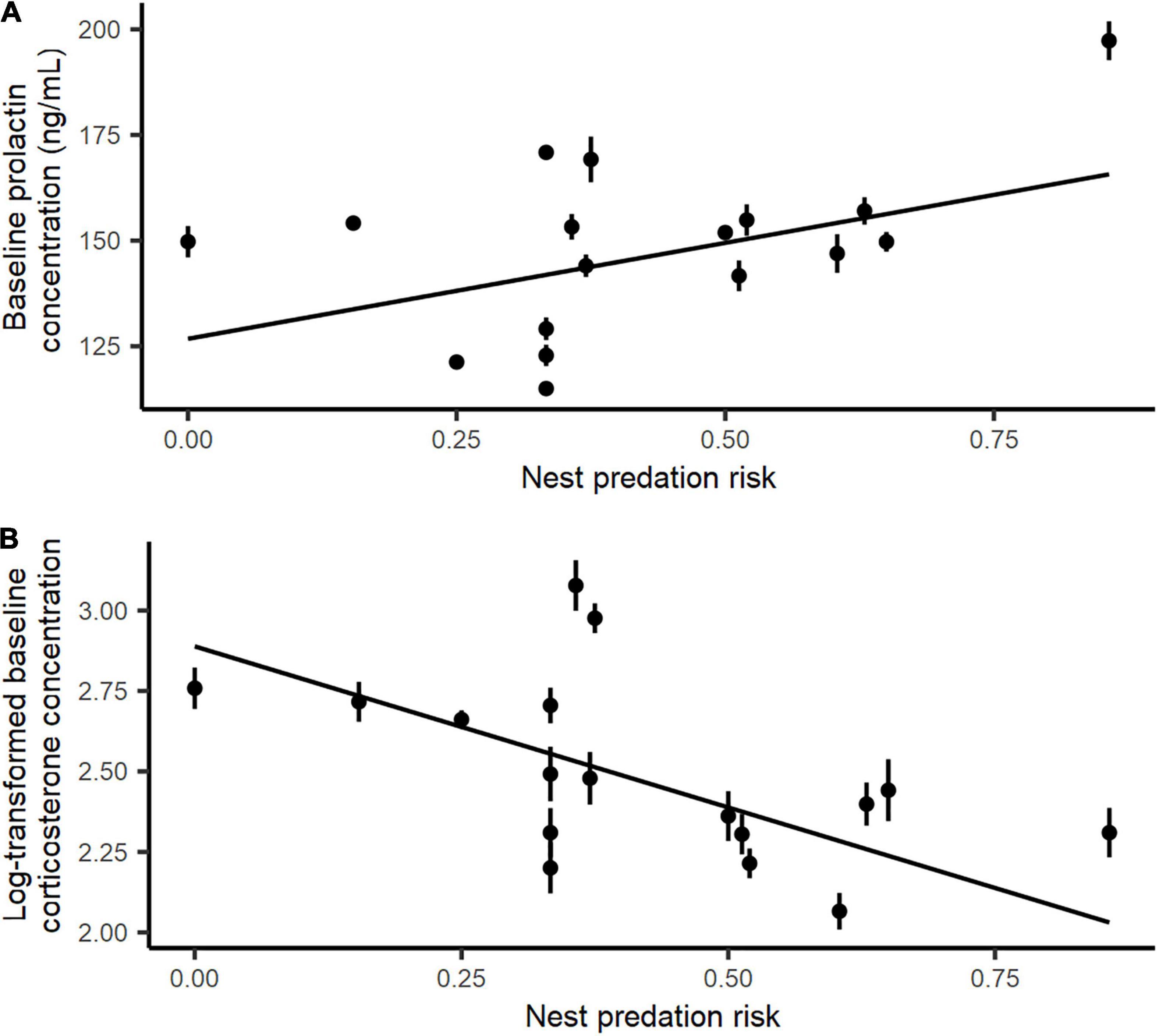
Figure 1. Relationship between baseline (A) prolactin and (B) log-transformed corticosterone levels of incubating female eiders and island-specific nest predation risk. Black dots correspond to island-specific mean hormone levels and bars account for the standard error.
Baseline Corticosterone in Relation to Individual and Environmental Characteristics
The final model explaining variation in baseline corticosterone levels included breeding experience, clutch weight and nest predation risk (Table 1). Baseline corticosterone levels were significantly higher in experienced birds compared to first-time breeders (baseline corticosterone concentration: inexperienced females: mean ± SE = 9.87 ± 0.71 ng/mL; experienced females: mean ± SE = 17.06 ± 1.25; Table 1 and Figure 2). Baseline corticosterone also showed a significantly positive relationship with clutch weight (E ± SE = 0.0013 ± 0.0005; Table 1 and Figure 2). In contrast, female structural size, body condition or nest cover were not related to baseline corticosterone concentrations. With respect to our predation indices, increasing island-specific nest predation risk had a significant negative association with corticosterone levels (E ± SE = −0.97 ± 0.39; Table 1 and Figure 1B), whereas female predation risk did not explain variation in baseline corticosterone levels.
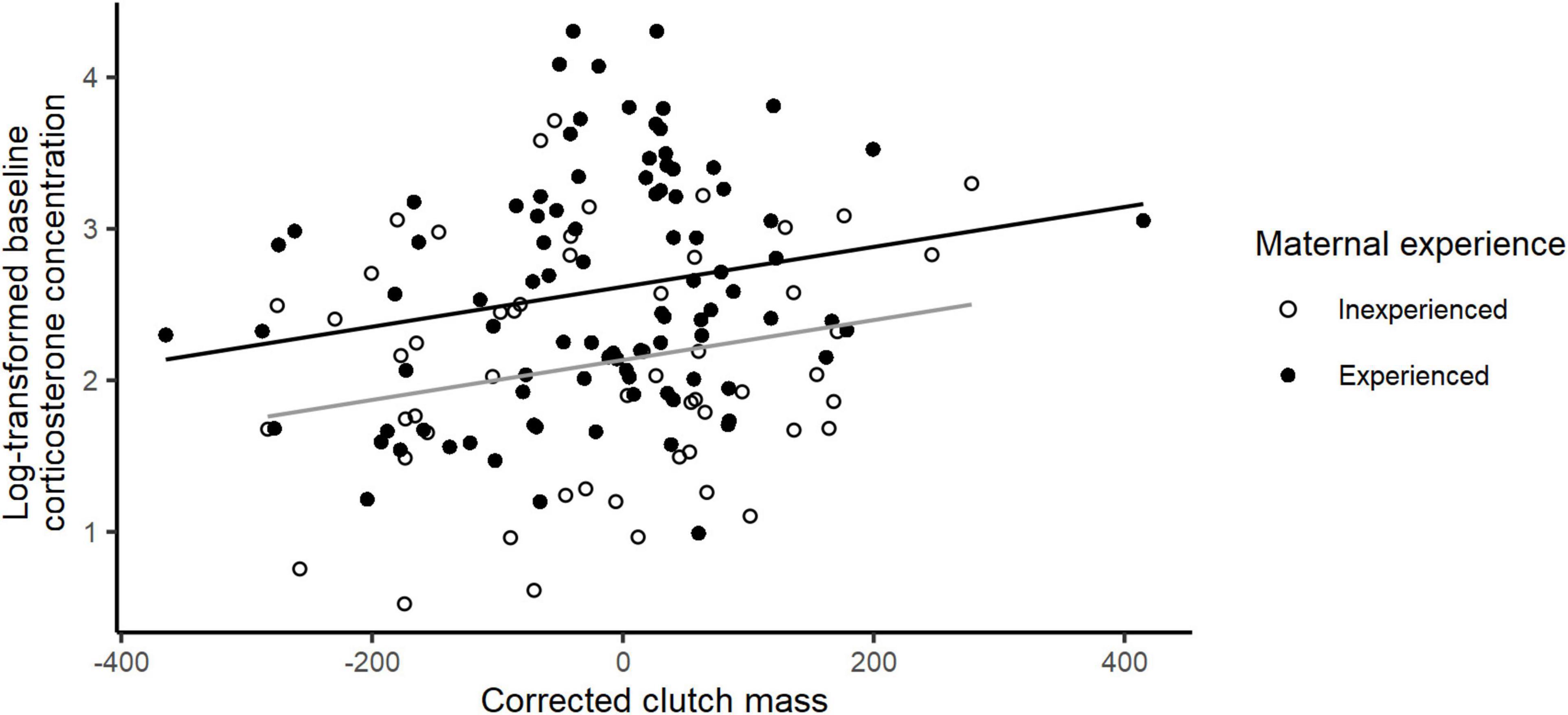
Figure 2. Relationship between baseline log-transformed baseline corticosterone levels and incubation-stage corrected clutch weight of incubating female eiders for experienced (in black) and inexperienced (in gray) breeders.
Hatching Success in Relation to Physiology, Individual and Environmental Characteristics
The final model predicting hatching success included baseline prolactin, radius-ulna length, and female and nest predation risk, as explanatory variables (Table 1). Hatching success showed a significant negative relationship with increasing baseline prolactin levels (E ± SE = −0.012 ± 0.005; Table 1 and Figure 3A), while it did not significantly vary with baseline corticosterone levels (Figure 3B). Hatching success tended to increase with female structural size, although this effect was not significant (Table 1). Other individual explanatory variables (incubation stage, body condition at hatching and breeding experience) were not selected in the final model. We also did not find any significant association between nest cover and hatching success. However, we also found that hatching success was significantly negatively related to nest predation risk (E ± SE = −8.85 ± 2.46; Table 1 and Figure 3C) and significantly positively associated with female predation risk (E ± SE = 14.18 ± 6.86; Table 1 and Figure 3D).
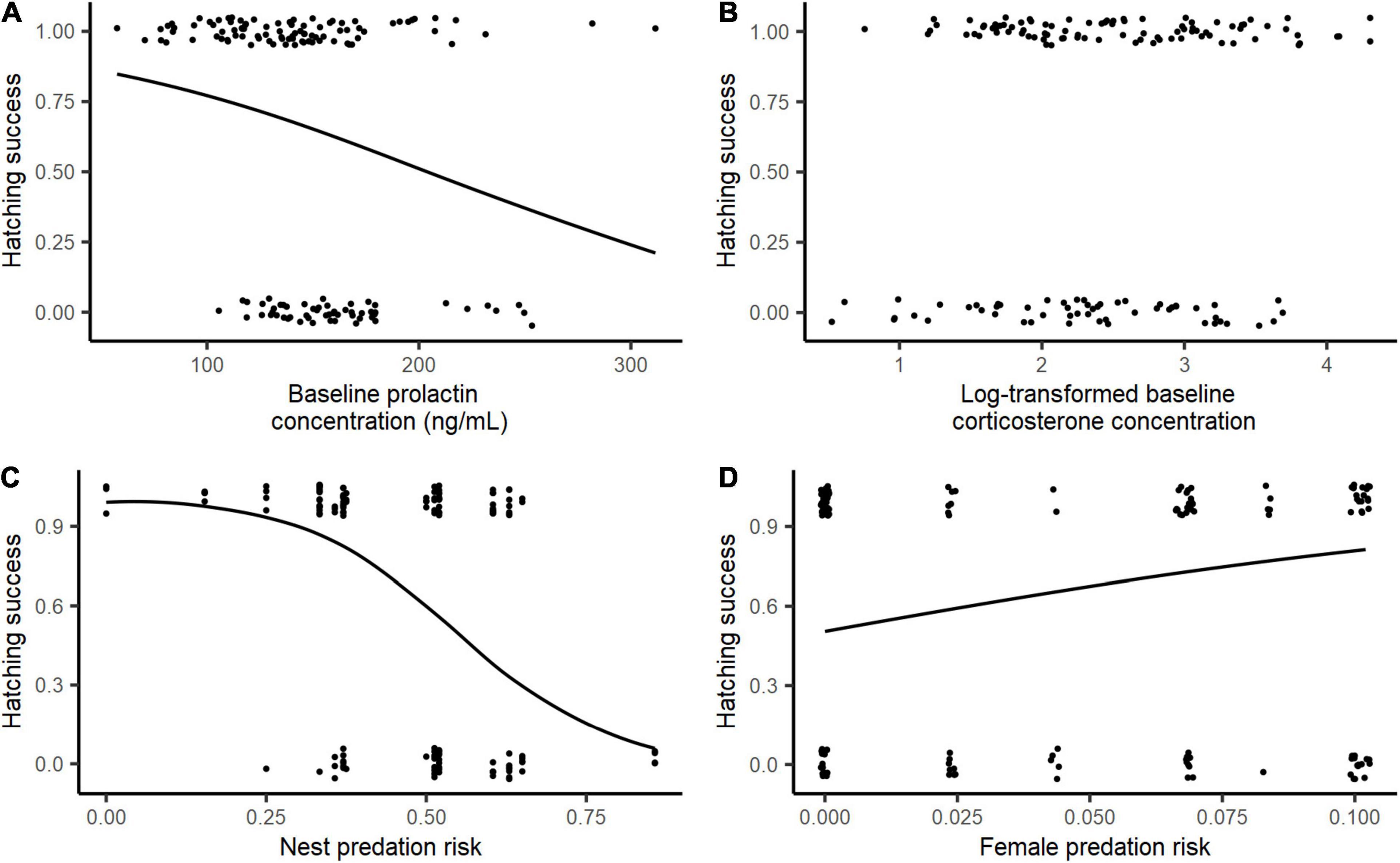
Figure 3. Relationship between hatching success of female eiders and (A) baseline prolactin levels, (B) baseline log-transformed corticosterone levels, (C) island-specific nest predation risk, and (D) island-specific female predation risk.
Female Body Condition in Relation to Individual Attributes and Predation
Female body condition at hatching was related to individual and environmental factors (Table 1). Body condition at hatching was significantly higher in more experienced birds (body condition at hatching: inexperienced females: mean ± SE = 0.16 ± 0.08; experienced females: mean ± SE = 0.62 ± 0.08; Table 1 and Figure 4). Body condition at hatching also showed a significant positive correlation with female predation risk (E ± SE = 4.03 ± 1.90; Table 1 and Figure 4), although this association was only marginally significant (E ± SE = 0.15 ± 0.08; Z = 1.756, P = 0.079) when performing conditional model averaging. In addition, female body condition at hatching was not significantly related to nest predation risk.
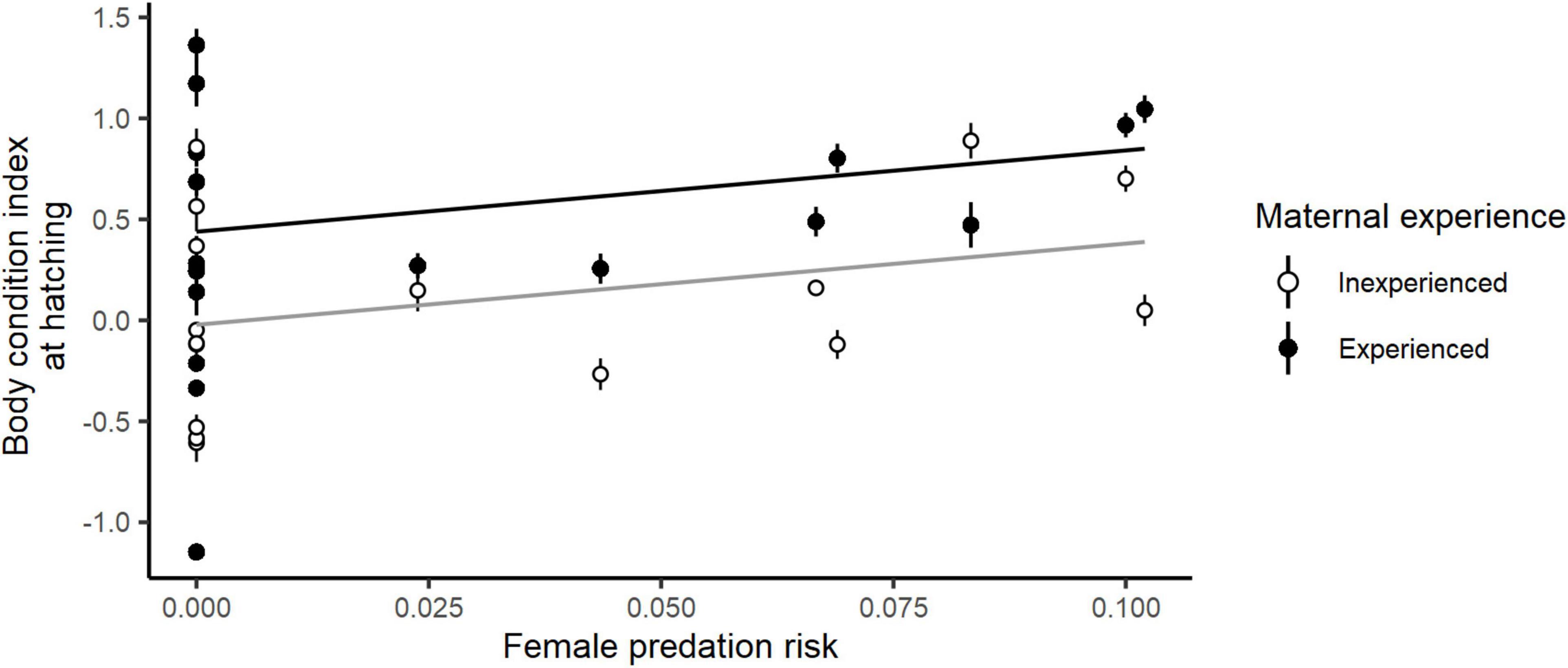
Figure 4. Relationship between the body condition index at hatching of female eiders and island-specific female predation risk for experienced (in black) and inexperienced (in gray) breeders. Dots correspond to island-specific mean body condition indices at hatching for experienced (in black) and inexperienced (in white) breeders, respectively, and bars account for the standard error.
Offspring Body Condition and Maternal Hormone Profiles
Duckling body condition was significantly positively associated with maternal baseline prolactin concentrations (E ± SE = 0.006 ± 0.003; χ2 = 4.481, P = 0.034; Figure 5A), but showed no association with maternal baseline corticosterone levels (χ2 = 4.481, P = 0.907; Figure 5B).
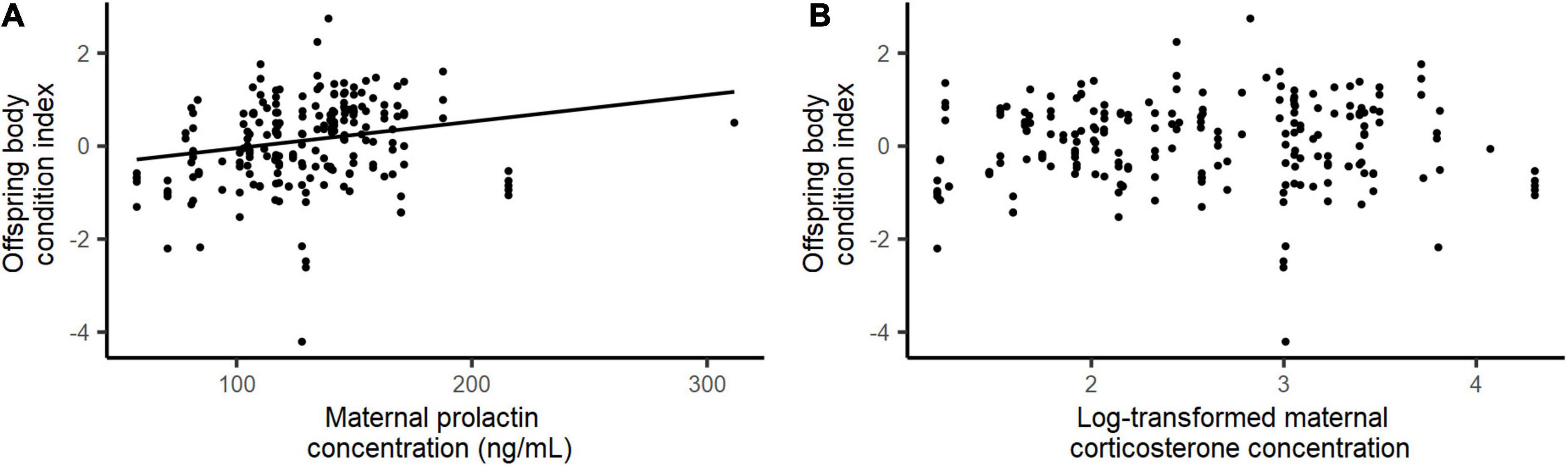
Figure 5. Relationship between the duckling body condition index and maternal baseline concentrations of (A) prolactin and (B) corticosterone (log-transformed) in eiders.
Discussion
In this study, we investigated the role of baseline prolactin and corticosterone levels, two hormonal correlates of parental investment (e.g., Criscuolo et al., 2002, 2005; Ouyang et al., 2013, in modulating incubation commitment of female eiders in response to variable predation risk on themselves and their offspring. Baseline prolactin levels were unrelated to direct proxies of female quality (body condition and breeding experience) or reproductive investment (clutch weight). However, elevated maternal prolactin levels were associated with better duckling body condition (Figure 5A), suggesting a positive association between female baseline prolactin levels and female parental commitment. Surprisingly, and contrary to our expectations, birds breeding on riskier islands (characterized by higher nest predation risk) displayed higher baseline prolactin levels. This unexpected finding may reflect an absence of low-quality individuals, characterized here by low prolactin levels, from risky nesting sites. In favor of this hypothesis, incubating females exposed to a higher threat of predation on themselves were, on average, in better body condition (i.e., presumably of higher quality) than those nesting in less dangerous sites (Figure 4), even though we did not detect any direct association between female body condition and baseline prolactin levels.
In addition, we found that baseline corticosterone levels increased along with clutch weight, our proxy of energetic investment in reproduction, and was higher in experienced females than in inexperienced breeders. These two findings are in favor of the corticosterone-adaptation hypothesis and support the idea that elevated baseline corticosterone levels may promote parental investment (Bonier et al., 2009). Accordingly, we found that birds breeding on islands with a high risk of nest predation displayed lower circulating corticosterone levels. Consequently, high nest predation risk and low prospects of successful hatching may lead females nesting in these risky areas to reduce their incubation commitment in the current clutch, through a lower allocation of resources to reproduction, mediated by lower baseline corticosterone levels.
Prolactin, Individual Quality, and Predation Risk
Contrary to our expectations, we found no influence of body condition at trapping, a proxy of individual quality, on baseline prolactin levels. This result suggests that, in incubating eiders, baseline prolactin levels are not directly affected by individual body condition. Although this result is not in line with the results from other studies (Criscuolo et al., 2002, 2003, 2006; Angelier and Chastel, 2009; Schmid et al., 2011), it could be explained by the non-linear link between prolactin and body condition in capital breeders (Drent and Daan, 1980). In such species, prolactin levels only decrease when individuals reach a very low threshold of body mass (Cherel et al., 1988). In our study, all individuals were above this threshold, which is around 1.10 kg for eiders (Korschgen, 1977). Accordingly, this lack of relationship between baseline prolactin levels and body condition has been highlighted in other capital-breeding birds with large amount of body reserves (Angelier et al., 2006; Verreault et al., 2008; Angelier and Chastel, 2009).
Likewise, and contrary to previous studies (Angelier et al., 2006, 2007b; Christensen and Vleck, 2008; Angelier and Chastel, 2009; Riechert et al., 2012; Smiley and Adkins-Regan, 2016), we did not find any significant increase in baseline prolactin levels with breeding experience, a proxy of individual quality in female eiders (Jaatinen and Öst, 2011). This discrepancy may result from the time of sampling: we caught all eiders toward the end of the incubation period, when a substantial fraction of the inexperienced breeders with low prolactin levels may already have failed. Overall, we did not find any direct evidence of a significant positive association between baseline prolactin levels and proxies of female quality (body condition and experience). Higher prolactin levels were also not related to clutch weight (a proxy of energetic investment in reproduction), but they were positively associated with duckling body condition at hatching. This finding is noteworthy because the survival and recruitment of female eiders is related to their relative body condition as ducklings (Christensen, 1999), and thus the production of heavy offspring may have positive effects on maternal fitness. Altogether, these results suggest that maternal baseline prolactin levels may be positively associated with some but not all aspects of parental quality.
Baseline prolactin levels were positively associated with nest predation risk, but unrelated to adult predation risk and nest cover. This result is not in line with most studies evidencing lower prolactin levels in individuals subject to nest predation risk (Angelier et al., 2016). However, we showed that baseline prolactin levels were positively associated with duckling body condition, an indirect proxy of female quality, and this finding could indicate that only high-quality females were found on islands where nest predation risk was high. In line with this idea, we found that female body condition, a proxy of individual quality (Lehikoinen et al., 2010), increased along with adult predation risk. Two mutually non-exclusive explanations are possible. First, only high-quality females with high prolactin levels may opt to breed at risky sites in this species frequently exhibiting intermittent breeding (the reproductive suppression model; Wasser and Barash, 1983). Supporting this idea, a long-term analysis of female body condition at Tvärminne shows that body condition has been increasing over the past 15 years, concomitantly with a steeply increasing trend in white-tailed eagle numbers and in nest predation risk (Öst et al., 2018).
Second, higher baseline prolactin levels on risky islands could be explained by the early breeding failure of low-quality females on these sites. In our study, females were trapped toward the end of the incubation period and high prolactin levels and incubation commitment may be required to reach this stage of breeding on risky sites, whereas less committed females may reach this stage on safer islands. Indeed, prolactin is known to play a role in nest attendance and high prolactin levels are associated with lower tendency to abandon the nest (Spée et al., 2010; Angelier et al., 2016; Smiley and Adkins-Regan, 2018) and a shorter latency to return to the nest after a disturbance (Angelier et al., 2009; Hope et al., 2020).
In addition, and contrary to both our a priori expectations and the previous literature (Angelier et al., 2007b, 2009, 2016; Ouyang et al., 2011; Riechert et al., 2014a), females displaying higher levels of baseline prolactin were less rather than more successful than those with lower levels. However, this result is consistent with the observed positive relationship between female prolactin levels and nest predation risk, likely arising from the selective disappearance of low-quality females with low baseline prolactin levels from the breeding pool on high-risk islands. Consequently, a putative link between high prolactin levels and high nest predation risk may lead to an indirect negative relationship between prolactin levels and reproductive success. In further support of this conclusion, there was a positive correlation between reproductive success and predation risk on adult females. This finding is consistent with the view that only high-quality females with high baseline prolactin levels opt to breed, or, alternatively, have nests that survive until the late incubation stage under high threat of predation. A positive correlation between female body condition at hatching and female predation risk (Figure 3D) is also consistent with this explanation. Summing up this evidence, we conclude that high-quality breeders characterized by high prolactin levels are likely to be overrepresented on risky sites at the end of the incubation period.
Corticosterone, Reproductive Investment, and Predation Risk
As for prolactin, we did not find any significant relationship between baseline corticosterone levels and female body condition. Such a lack of association between baseline corticosterone and body condition or body mass has also been shown in other eider populations (Bourgeon and Raclot, 2006; Criscuolo et al., 2006; D’Alba et al., 2011), and, more generally, in other capital breeders (Angelier et al., 2006; Groscolas et al., 2008). As for prolactin, this lack of a relationship could result from the fact that incubating female eiders have not reached a low threshold in body mass (Cherel et al., 1988) at the time of capture. However, we found that experienced breeders displayed higher baseline corticosterone levels than inexperienced breeders, a result in favor of the corticosterone-adaptation hypothesis (Bonier et al., 2009). Accordingly, some studies have found that circulating corticosterone levels increase with age and breeding experience in capital breeders with extended fasting periods (Angelier et al., 2006). In such species, elevated baseline corticosterone levels may be associated with an increased investment in reproduction, especially when individuals have not reached a lower threshold in body condition (Cherel et al., 1988), as is the case in our study.
In further support of the corticosterone-adaptation hypothesis, we found that baseline corticosterone levels were positively associated with clutch weight. Despite the possible occurrence of partial clutch predation (Erikstad et al., 1993; Öst et al., 2008) and conspecific nest parasitism, the frequency of which is low in our population (ca 6% of eggs; Waldeck et al., 2004), clutch weight or size can be used as a reasonable proxy for female initial energetic investment in reproduction in eiders (Hanssen et al., 2003; Jaatinen et al., 2013). Overall, corticosterone may promote energy mobilization (Romero, 2002; Breuner, 2011) and incubation effort (Breuner, 2011; DuRant et al., 2013), and moderately elevated baseline corticosterone levels could be a proxy for increased incubation commitment in our study system. Accordingly, other studies have shown that increased baseline corticosterone levels do not trigger nest abandonment in eiders (Criscuolo et al., 2005), nor do they induce nest failure unless corticosterone levels reach very high values (D’Alba et al., 2011), a result consistent with the hormetic dose response framework (Costantini et al., 2010; Costantini, 2014). The positive association between baseline corticosterone levels and both clutch weight and maternal experience may thus indicate that incubating females increase their investment in a current reproductive event when its value is elevated (i.e., heavy clutch), and when the prospect of future reproduction diminish (the restraint hypothesis, Curio, 1983; Heidinger et al., 2006).
In addition, we showed that baseline corticosterone levels were negatively related to nest predation risk, but unrelated to adult predation risk and nest cover. These results are consistent with the corticosterone-adaptation hypothesis, and lower baseline corticosterone levels in risky nesting sites may therefore suggest that female eiders reduce their investment in incubation in the face of increased nest predation risk. This interpretation is supported not only by the fact that baseline corticosterone levels in this study were unrelated to hatching success and offspring quality, but also by the positive effect of breeding experience on both parental investment (Öst and Steele, 2010) and corticosterone levels in eiders (this study). Interestingly, similar effects of increasing nest predation risk on parental investment have been demonstrated in other species (Doligez and Clobert, 2003; Eggers et al., 2006; Zanette et al., 2011).
While baseline corticosterone levels can be seen as a proxy of parental investment, they can also mirror the response to prolonged exposure to chronic environmental perturbations, such as predation risk (Scheuerlein et al., 2001; Clinchy et al., 2004; Travers et al., 2010; Angelier and Wingfield, 2013). In that context, high baseline corticosterone levels could also be related to high stress sensitivity. Under that scenario, lower baseline corticosterone levels on risky sites could result not only from individuals reducing their parental investment under high predation threat, but also from the selective disappearance of highly sensitive individuals from these risky sites. Highly sensitive female eiders could either have already abandoned their breeding attempts prior to our trapping efforts on these sites, or they could have failed their breeding attempt at the time of trapping, toward the end of incubation. Females exhibiting higher baseline corticosterone levels would thus be expected to skip breeding more often and/or to be prone to abandon their nest when nesting on sites with a high risk of nest predation (Vitousek et al., 2014). Supporting this possibility, it has been found in other species that incubating individuals displaying lower baseline corticosterone levels returned more quickly to the nest following a disturbance (Angelier et al., 2009; Edwards et al., 2013), or tended to abandon their nest less often than more sensitive individuals (Spée et al., 2010; Ouyang et al., 2012).
Perspectives
In this study, we investigated the role of baseline prolactin and corticosterone levels in modulating parental investment decisions under variable predation threat in incubating female eiders. We showed that birds breeding on islands subject to higher nest predation threat displayed higher baseline prolactin levels, and that this could reflect an absence of low-quality individuals with low prolactin levels at risky nesting sites. We also found that birds breeding on riskier islands displayed lower baseline corticosterone levels, and this may suggest that females reduce their incubation commitment on islands where predation risk on offspring is high (the corticosterone-adaptation hypothesis). Although our findings allow a better understanding of how predation risk may interact with hormonal regulation of parental investment, the correlative nature of the study does not allow us to draw causal relationships between hormone levels, parental investment and predation risk, highlighting the need for experimental studies to further clarify these relationships. In addition, our results do not allow us to distinguish effects of predation threat at the population level from effects of predation risk at the individual level. Longitudinal individual-based studies are now needed to disentangle within- and between-individual variation in hormonal levels in relation to individual quality and variable predation risk. This would allow us to distinguish natural selection processes from individual plasticity in responding to a changing environment. Such studies will eventually pave the way for a better understanding of the ability of prey to physiologically and behaviorally cope with environmental perturbations and how this impacts their reproductive life-history strategies.
Data Availability Statement
The raw data supporting the conclusions of this article will be made available by the authors, without undue reservation.
Ethics Statement
The animal study was reviewed and approved by the Handling of birds was approved by the National Animal Experiment Board (permit number ESAVI/1697/04.10.03/2012) and complied with the specific regulations of Tvärminne Zoological Station.
Author Contributions
MÖ and KJ conceived the study and conducted fieldwork. CP and FA conducted laboratory analyses. BM analyzed the data. BM, MÖ, FA, and KJ interpreted the results. All authors contributed to writing the article and approved the submitted version.
Funding
This work was supported by the Swedish Cultural Foundation in Finland (Grant Nos. 17/3317, 16/1476, 15/3296, 14/2657, 13/2654, 138139, 149014, 158026, and 168333 to MÖ) and the Academy of Finland (Grant No. 266208 to KJ and Grant No. 128039 to MÖ), as well as French CNRS fund (to FA). Open access funding was provided by Åbo Akademi University (ABO).
Conflict of Interest
The authors declare that the research was conducted in the absence of any commercial or financial relationships that could be construed as a potential conflict of interest.
Acknowledgments
We thank Ben Steele and the fieldwork team of 2013 for assistance in data collection. Facilities were provided by Tvärminne Zoological Station. We thank the two reviewers for their helpful comments in improving the manuscript.
References
Angelier, F., and Chastel, O. (2009). Stress, prolactin and parental investment in birds: a review. Gen. Comp. Endocrinol. 163, 142–148. doi: 10.1016/j.ygcen.2009.03.028
Angelier, F., Clément-Chastel, C., Welcker, J., Gabrielsen, G. W., and Chastel, O. (2009). How does corticosterone affect parental behaviour and reproductive success? A study of prolactin in black-legged kittiwakes: corticosterone, prolactin and parental behaviour. Funct. Ecol. 23, 784–793. doi: 10.1111/j.1365-2435.2009.01545.x
Angelier, F., Moe, B., Weimerskirch, H., and Chastel, O. (2007a). Age-specific reproductive success in a long-lived bird: do older parents resist stress better? J. Anim. Ecol. 76, 1181–1191. doi: 10.1111/j.1365-2656.2007.01295.x
Angelier, F., Shaffer, S. A., Weimerskirch, H., and Chastel, O. (2006). Effect of age, breeding experience and senescence on corticosterone and prolactin levels in a long-lived seabird: the wandering albatross. Gen. Comp. Endocrinol. 149, 1–9. doi: 10.1016/j.ygcen.2006.04.006
Angelier, F., and Wingfield, J. C. (2013). Importance of the glucocorticoid stress response in a changing world: theory, hypotheses and perspectives. Gen. Comp. Endocrinol. 190, 118–128. doi: 10.1016/j.ygcen.2013.05.022
Angelier, F., Weimerskirch, H., Dano, S., and Chastel, O. (2007b). Age, experience and reproductive performance in a long-lived bird: a hormonal perspective. Behav. Ecol. Sociobiol. 61, 611–621. doi: 10.1007/s00265-006-0290-1
Angelier, F., Wingfield, J. C., Parenteau, C., Pellé, M., and Chastel, O. (2015). Does short-term fasting lead to stressed-out parents? A study of incubation commitment and the hormonal stress responses and recoveries in snow petrels. Horm. Behav. 67, 28–37. doi: 10.1016/j.yhbeh.2014.11.009
Angelier, F., Wingfield, J. C., Tartu, S., and Chastel, O. (2016). Does prolactin mediate parental and life-history decisions in response to environmental conditions in birds? A review. Horm. Behav. 77, 18–29. doi: 10.1016/j.yhbeh.2015.07.014
Angelier, F., Wingfield, J. C., Weimerskirch, H., and Chastel, O. (2010). Hormonal correlates of individual quality in a long-lived bird: a test of the ‘corticosterone–fitness hypothesis.’. Biol. Lett. 6, 846–849. doi: 10.1098/rsbl.2010.0376
Arnold, T. W., Roche, E. A., Devries, J. H., and Howerter, D. W. (2012). Costs of reproduction in breeding female mallards: predation risk during incubation drives annual mortality. Avian Conserv. Ecol. 7:1. doi: 10.5751/ACE-00504-070101
Bates, D., Mächler, M., Bolker, B., and Walker, S. (2015). Fitting linear mixed-effects models using lme4. J. Stat. Softw. 67:1406. doi: 10.18637/jss.v067.i01
Blumstein, D. T., and Daniel, J. C. (2005). The loss of anti-predator behaviour following isolation on islands. Proc. R. Soc. B Biol. Sci. 272, 1663–1668. doi: 10.1098/rspb.2005.3147
Bókony, V., Lendvai, ÁZ., Liker, A., Angelier, F., Wingfield, J. C., and Chastel, O. (2009). Stress response and the value of reproduction: are birds prudent parents? Am. Nat. 173, 589–598. doi: 10.1086/597610
Bolduc, F., Guillemette, M., and Titman, R. D. (2005). Nesting success of common eiders Somateria mollissima as influenced by nest-site and female characteristics in the Gulf of the St. Lawrence. Wildlife Biol. 11, 273–279.
Bonier, F., Martin, P. R., Moore, I. T., and Wingfield, J. C. (2009). Do baseline glucocorticoids predict fitness? Trends Ecol. Evol. 24, 634–642. doi: 10.1016/j.tree.2009.04.013
Bonier, F., Moore, I. T., and Robertson, R. J. (2011). The stress of parenthood? Increased glucocorticoids in birds with experimentally enlarged broods. Biol. Lett. 7, 944–946. doi: 10.1098/rsbl.2011.0391
Bourgeon, S., and Raclot, T. (2006). Corticosterone selectively decreases humoral immunity in female eiders during incubation. J. Exp. Biol. 209, 4957–4965. doi: 10.1242/jeb.02610
Bowers, E. K., Thompson, C. F., Bowden, R. M., and Sakaluk, S. K. (2019). Posthatching parental care and offspring growth vary with maternal corticosterone level in a wild bird population. Physiol. Biochem. Zool. 92, 496–504. doi: 10.1086/705123
Breuner, C. W. (2011). “Stress and reproduction in birds,” in Hormones and Reproduction of Vertebrates, eds D. O. Norris and K. H. Lopez (Amsterdam: Elsevier), 129–151.
Breuner, C. W., and Berk, S. A. (2019). Using the van Noordwijk and de Jong resource framework to evaluate glucocorticoid-fitness hypotheses. Integr. Comp. Biol. 59, 243–250. doi: 10.1093/icb/icz088
Bruinzeel, L. W. (2007). Intermittent breeding as a cost of site fidelity. Behav. Ecol. Sociobiol. 61, 551–556. doi: 10.1007/s00265-006-0283-0
Chastel, O., Weimerskirch, H., and Jouventin, P. (1995). Influence of body condition on reproductive decision and reproductive success in the blue petrel. Auk 112, 964–972. doi: 10.2307/4089027
Cherel, Y., Robin, J.-P., and Maho, Y. L. (1988). Physiology and biochemistry of long-term fasting in birds. Can. J. Zool. 66, 159–166. doi: 10.1139/z88-022
Christensen, D., and Vleck, C. M. (2008). Prolactin release and response to vasoactive intestinal peptide in an opportunistic breeder, the zebra finch (Taeniopygia guttata). Gen. Comp. Endocrinol. 157, 91–98. doi: 10.1016/j.ygcen.2008.04.013
Christensen, T. K. (1999). Effects of cohort and individual variation in duckling body condition on survival and recruitment in the common eider Somateria mollissima. J. Avian Biol. 30:302. doi: 10.2307/3677356
Clinchy, M., Zanette, L., Boonstra, R., Wingfield, J. C., and Smith, J. N. M. (2004). Balancing food and predator pressure induces chronic stress in songbirds. Proc. R. Soc. Lond. Ser. B Biol. Sci. 271, 2473–2479. doi: 10.1098/rspb.2004.2913
Costantini, D. (2014). Does hormesis foster organism resistance to extreme events? Front. Ecol. Environ. 12, 209–210. doi: 10.1890/14.WB.005
Costantini, D., Metcalfe, N. B., and Monaghan, P. (2010). Ecological processes in a hormetic framework: hormesis in ecology. Ecol. Lett. 13, 1435–1447. doi: 10.1111/j.1461-0248.2010.01531.x
Coulson, J. (1984). The population dynamics of the eider duck Somateria mollissima and evidence of extensive non-breeding by adult ducks. IBIS 126, 525–543.
Coulson, J. C. (2016). A review of philopatry in seabirds and comparisons with other waterbird species. Waterbirds 39, 229–240. doi: 10.1675/063.039.0302
Creel, S., Winnie, J., Maxwell, B., Hamlin, K., and Creel, M. (2005). Elk alter habitat selection as an antipredator response to wolves. Ecology 86, 3387–3397. doi: 10.1890/05-0032
Criscuolo, F., Bertile, F., Durant, J. M., Raclot, T., Gabrielsen, G. W., Massemin, S., et al. (2006). Body mass and clutch size may modulate prolactin and corticosterone levels in eiders. Physiol. Biochem. Zool. 79, 514–521. doi: 10.1086/501065
Criscuolo, F., Chastel, O., Bertile, F., Gabrielsen, G. W., Maho, Y. L., and Raclot, T. (2005). Corticosterone alone does not trigger a short term behavioural shift in incubating female common eiders Somateria mollissima, but does modify long term reproductive success. J. Avian Biol. 36, 306–312. doi: 10.1111/j.0908-8857.2005.03371.x
Criscuolo, F., Chastel, O., Gabrielsen, G. W., Lacroix, A., and Le Maho, Y. (2002). Factors affecting plasma concentrations of prolactin in the common eider Somateria mollissima. Gen. Comp. Endocrinol. 125, 399–409. doi: 10.1006/gcen.2001.7767
Criscuolo, F., Chastel, O., Gabrielsen, G. W., Lacroix, A., and Le Maho, Y. (2003). “Relationship between nutritional status and prolactin levels in the common eider, a capital incubator. Backhuys, Leyden,” in Antarctic Biology in a Global Context, eds A. H. L. Huiskes, W. W. C. Gieskes, J. Rozema, R. M. L. Schorno, S. M. van der Vies, and W. J. Wolff (Leiden: Backhuys Publishers), 193–197.
Criscuolo, F., Gauthier-Clerc, M., Gabrielsen, G. W., and Le Maho, Y. (2000). Recess behaviour of the incubating common eider Somateria mollissima. Polar Biol. 23, 571–574.
D’Alba, L., Spencer, K. A., Nager, R. G., and Monaghan, P. (2011). State dependent effects of elevated hormone: nest site quality, corticosterone levels and reproductive performance in the common eider. Gen. Comp. Endocrinol. 172, 218–224. doi: 10.1016/j.ygcen.2011.03.006
De Steven, D. (1980). Clutch size, breeding success, and parental survival in the tree swallow (Iridoprocne bicolor). Evolution 34:278. doi: 10.2307/2407392
Delehanty, D. J., Oring, L. W., Fivizzani, A. J., and El Halawani, M. E. (1997). Circulating prolactin of incubating male wilson’s phalaropes corresponds to clutch size and environmental stress. Condor 99, 397–405. doi: 10.2307/1369946
Doligez, B., and Clobert, J. (2003). Clutch size reduction as a response to increased nest predation rate in the collared flycatcher. Ecology 84, 2582–2588. doi: 10.1890/02-3116
Drent, R., and Daan, S. (1980). The prudent parent: energetic adjustments in avian breeding. Ardea 68, 225–252.
DuRant, S. E., Hopkins, W. A., Hepp, G. R., and Romero, L. M. (2013). Energetic constraints and parental care: is corticosterone indicative of energetic costs of incubation in a precocial bird? Horm. Behav. 63, 385–391. doi: 10.1016/j.yhbeh.2012.12.001
Edwards, D. B., Chin, E. H., Burness, G., Gilchrist, H. G., and Schulte-Hostedde, A. I. (2013). Linking sex differences in corticosterone with individual reproductive behaviour and hatch success in two species of uniparental shorebirds. Comp. Biochem. Physiol. A Mol. Integr. Physiol. 166, 169–176. doi: 10.1016/j.cbpa.2013.05.024
Eggers, S., Griesser, M., Nystrand, M., and Ekman, J. (2006). Predation risk induces changes in nest-site selection and clutch size in the Siberian jay. Proc. R. Soc. B Biol. Sci. 273, 701–706. doi: 10.1098/rspb.2005.3373
Ekroos, J., Öst, M., Karell, P., Jaatinen, K., and Kilpi, M. (2012). Philopatric predisposition to predation-induced ecological traps: habitat-dependent mortality of breeding eiders. Oecologia 170, 979–986. doi: 10.1007/s00442-012-2378-9
Erikstad, K. E., Bustnes, J. O., and Moum, T. (1993). Clutch-size determination in precocial birds: a study of the common eider. Auk 110, 623–628.
Erikstad, K. E., Fauchald, P., Tveraa, T., and Steen, H. (1998). On the cost of reproduction in long-lived birds: the influence of environmental variability. Ecology 79, 1781–1788.
Feifarek, B. P., Wyngaard, G. A., and Allan, J. D. (1983). The cost of reproduction in a freshwater copepod. Oecologia 56, 166–168.
Forslund, P., and Pärt, T. (1995). Age and reproduction in birds — hypotheses and tests. Trends Ecol. Evol. 10, 374–378. doi: 10.1016/S0169-5347(00)89141-7
Ghalambor, C. K., and Martin, T. E. (2000). Parental investment strategies in two species of nuthatch vary with stage-specific predation risk and reproductive effort. Anim. Behav. 60, 263–267. doi: 10.1006/anbe.2000.1472
Gómez-Serrano, M. Á (2020). Four-legged foes: dogs disturb nesting plovers more than people do on tourist beaches. IBIS 2020:12879. doi: 10.1111/ibi.12879
Goutte, A., Angelier, F., Welcker, J., Moe, B., Clément-Chastel, C., Gabrielsen, G. W., et al. (2010). Long-term survival effect of corticosterone manipulation in Black-legged kittiwakes. Gen. Comp. Endocrinol. 167, 246–251. doi: 10.1016/j.ygcen.2010.03.018
Groscolas, R., Lacroix, A., and Robin, J.-P. (2008). Spontaneous egg or chick abandonment in energy-depleted king penguins: a role for corticosterone and prolactin? Hormones Behav. 53, 51–60. doi: 10.1016/j.yhbeh.2007.08.010
Groscolas, R., and Robin, J.-P. (2001). Long-term fasting and re-feeding in penguins. Comp. Biochem. Physiol. A Mol. Integr. Physiol. 128, 643–653. doi: 10.1016/S1095-6433(00)00341-X
Hamel, S., Côté, S. D., Gaillard, J.-M., and Festa-Bianchet, M. (2009). Individual variation in reproductive costs of reproduction: high-quality females always do better. J. Anim. Ecol. 78, 143–151. doi: 10.1111/j.1365-2656.2008.01459.x
Hanssen, S. A., Erikstad, K. E., Johnsen, V., and Jan, O. B. (2003). Differential investment and costs during avian incubation determined by individual quality: an experimental study of the common eider (Somateria mollissima). Proc. R. Soc. Lond. B 270, 531–537. doi: 10.1098/rspb.2002.2262
Heidinger, B. J., Nisbet, I. C. T., and Ketterson, E. D. (2006). Older parents are less responsive to a stressor in a long-lived seabird: a mechanism for increased reproductive performance with age? Proc. R. Soc. B. 273, 2227–2231. doi: 10.1098/rspb.2006.3557
Hope, S. F., DuRant, S. E., Angelier, F., Hallagan, J. J., Moore, I. T., Parenteau, C., et al. (2020). Prolactin is related to incubation constancy and egg temperature following a disturbance in a precocial bird. Gen. Comp. Endocrinol. 295:113489. doi: 10.1016/j.ygcen.2020.113489
Jaatinen, K., and Öst, M. (2011). Experience attracts: the role of age in the formation of cooperative brood-rearing coalitions in eiders. Anim. Behav. 81, 1289–1294. doi: 10.1016/j.anbehav.2011.03.020
Jaatinen, K., and Öst, M. (2013). Brood size matching: a novel perspective on predator dilution. Am. Nat. 181, 171–181. doi: 10.1086/668824
Jaatinen, K., Seltmann, M. W., Hollmén, T., Atkinson, S., Mashburn, K., and Öst, M. (2013). Context dependency of baseline glucocorticoids as indicators of individual quality in a capital breeder. Gen. Comp. Endocrinol. 191, 231–238. doi: 10.1016/j.ygcen.2013.06.022
Jaatinen, K., Seltmann, M. W., and Öst, M. (2014). Context-dependent stress responses and their connections to fitness in a landscape of fear: stress responses and their connections to fitness. J. Zool. 294, 147–153. doi: 10.1111/jzo.12169
Kilpi, M., and Lindström, K. (1997). Habitat-specific clutch size and cost of incubation in common eiders, Somateria mollissima. Oecologia 111, 297–301.
Kitaysky, A., Piatt, J., and Wingfield, J. (2007). Stress hormones link food availability and population processes in seabirds. Mar. Ecol. Prog. Ser. 352, 245–258. doi: 10.3354/meps07074
Kitaysky, A. S., Wingfield, J. C., and Piatt, J. F. (1999). Dynamics of food availability, body condition and physiological stress response in breeding Black-legged Kittiwakes: food availability and stress response in Kittiwakes. Funct. Ecol. 13, 577–584. doi: 10.1046/j.1365-2435.1999.00352.x
Laundré, J. W., Hernandez, L., and Ripple, W. J. (2010). The Landscape of fear: ecological implications of being Afraid. TOECOLJ 3, 1–7. doi: 10.2174/1874213001003030001
Lehikoinen, A., Jaatinen, K., and Öst, M. (2010). Do female ornaments indicate quality in eider ducks? Biol. Lett. 6, 225–228. doi: 10.1098/rsbl.2009.0744
Lehtonen, J., and Jaatinen, K. (2016). Safety in numbers: the dilution effect and other drivers of group life in the face of danger. Behav. Ecol. Sociobiol. 70, 449–458. doi: 10.1007/s00265-016-2075-5
Lima, S. L. (1998). Nonlethal effects in the ecology of predator-prey interactions. BioScience 48, 25–34. doi: 10.2307/1313225
Lormée, H., Jouventin, P., Trouve, C., and Chastel, O. (2003). Sex-specific patterns in baseline corticosterone and body condition changes in breeding red-footed boobies Sula sula: corticosterone and body condition in boobies. IBIS 145, 212–219. doi: 10.1046/j.1474-919X.2003.00106.x
Love, O. P., Madliger, C. L., Bourgeon, S., Semeniuk, C. A. D., and Williams, T. D. (2014). Evidence for baseline glucocorticoids as mediators of reproductive investment in a wild bird. Gen. Comp. Endocrinol. 199, 65–69. doi: 10.1016/j.ygcen.2014.01.001
Lynn, S. E. (2016). Endocrine and neuroendocrine regulation of fathering behavior in birds. Horm. Behav. 77, 237–248. doi: 10.1016/j.yhbeh.2015.04.005
Magnhagen, C. (1991). Predation risk as a cost of reproduction. Trends Ecol. Evol. 6, 183–186. doi: 10.1016/0169-5347(91)90210-O
McEwen, B. S., and Wingfield, J. C. (2003). The concept of allostasis in biology and biomedicine. Horm. Behav. 43, 2–15. doi: 10.1016/S0018-506X(02)00024-7
Miller, D. A., Vleck, C. M., and Otis, D. L. (2009). Individual variation in baseline and stress-induced corticosterone and prolactin levels predicts parental effort by nesting mourning doves. Horm. Behav. 56, 457–464. doi: 10.1016/j.yhbeh.2009.08.001
Noreikiene, K., Jaatinen, K., Steele, B. B., and Öst, M. (2021). Glucocorticoids, state-dependent reproductive investment and success in the face of danger in a long-lived bird. J. Ornithol. 162, 497–509.
Öst, M., and Bäck, A. (2003). Spatial structure and parental aggression in eider broods. Anim.Behav. 66, 1069–1075. doi: 10.1006/anbe.2003.2300
Öst, M., Lehikoinen, A., Jaatinen, K., and Kilpi, M. (2011). Causes and consequences of fine-scale breeding dispersal in a female-philopatric species. Oecologia 166, 327–336. doi: 10.1007/s00442-010-1855-2
Öst, M., Lindén, A., Karell, P., Ramula, S., and Kilpi, M. (2018). To breed or not to breed: drivers of intermittent breeding in a seabird under increasing predation risk and male bias. Oecologia 188, 129–138. doi: 10.1007/s00442-018-4176-5
Öst, M., Noreikiene, K., Angelier, F., and Jaatinen, K. (2020). Sex-specific effects of the in ovo environment on early-life phenotypes in eiders. Oecologia 192, 43–54. doi: 10.1007/s00442-019-04569-9
Öst, M., and Steele, B. B. (2010). Age-specific nest-site preference and success in eiders. Oecologia 162, 59–69. doi: 10.1007/s00442-009-1444-4
Öst, M., and Tierala, T. (2011). Synchronized vigilance while feeding in common eider brood-rearing coalitions. Behav. Ecol. 22, 378–384. doi: 10.1093/beheco/arq223
Öst, M., Wickman, M., Matulionis, E., and Steele, B. (2008). Habitat-specific clutch size and cost of incubation in eiders reconsidered. Oecologia 158, 205–216. doi: 10.1007/s00442-008-1139-2
Ouyang, J. Q., Muturi, M., Quetting, M., and Hau, M. (2013). Small increases in corticosterone before the breeding season increase parental investment but not fitness in a wild passerine bird. Horm. Behav. 63, 776–781. doi: 10.1016/j.yhbeh.2013.03.002
Ouyang, J. Q., Quetting, M., and Hau, M. (2012). Corticosterone and brood abandonment in a passerine bird. Anim. Behav. 84, 261–268. doi: 10.1016/j.anbehav.2012.05.006
Ouyang, J. Q., Sharp, P. J., Dawson, A., Quetting, M., and Hau, M. (2011). Hormone levels predict individual differences in reproductive success in a passerine bird. Proc. R. Soc. B Biol. Sci. 278, 2537–2545. doi: 10.1098/rspb.2010.2490
Pinheiro, J., Bates, D., DebRoy, S., Sarkar, D., and Team, R. C. (2019). nlme: Linear and Nonlinear Mixed Effects Models. R Package Version 3.1-140.
Pöysä, H. (1987). Feeding-vigilance trade-off in the teal (Anas crecca): effects of feeding method and predation risk. Behaviour 103, 108–122. doi: 10.1163/156853987X00297
Préault, M., Chastel, O., Cézilly, F., and Faivre, B. (2005). Male bill colour and age are associated with parental abilities and breeding performance in blackbirds. Behav. Ecol. Sociobiol. 58, 497–505. doi: 10.1007/s00265-005-0937-3
R Core Team (2018). R: A Language and Environment for Statistical Computing. Vienna: R Foundation for Statistical Computing.
Ramula, S., Öst, M., Linden, A., Karell, P., and Kilpi, M. (2018). Increased male bias in eider ducks can be explained by sex-specific survival of prime-age breeders. PLoS One 13:e0195415. doi: 10.1371/journal.pone.0195415
Reznick, D. (1985). Costs of reproduction: an evaluation of the empirical evidence. Oikos 44, 257–267.
Ricklefs, R. E., and Wikelski, M. (2002). The physiology/life-history nexus. Trends Ecol. Evol. 17, 462–468. doi: 10.1016/S0169-5347(02)02578-8
Riechert, J., Becker, P. H., and Chastel, O. (2014a). Predicting reproductive success from hormone concentrations in the common tern (Sterna hirundo) while considering food abundance. Oecologia 176, 715–727. doi: 10.1007/s00442-014-3040-5
Riechert, J., Chastel, O., and Becker, P. H. (2014b). Regulation of breeding behavior: do energy-demanding periods induce a change in prolactin or corticosterone baseline levels in the common tern (Sterna hirundo)? Physiol. Biochem. Zool. 87, 420–431. doi: 10.1086/675682
Riechert, J., Chastel, O., and Becker, P. H. (2012). Why do experienced birds reproduce better? Possible endocrine mechanisms in a long-lived seabird, the common tern. Gen. Comp. Endocrinol. 178, 391–399. doi: 10.1016/j.ygcen.2012.06.022
Robin, J.-P., Boucontet, L., Chillet, P., and Groscolas, R. (1998). Behavioral changes in fasting emperor penguins: evidence for a “refeeding signal” linked to a metabolic shift. Am. J. Physiol. Regul. Integr. Comp. Physiol. 274, R746–R753. doi: 10.1152/ajpregu.1998.274.3.R746
Romero, L. M. (2002). Seasonal changes in plasma glucocorticoid concentrations in free-living vertebrates. Gen. Comp. Endocrinol. 128, 1–24. doi: 10.1016/S0016-6480(02)00064-3
Romero, L. M., Dickens, M. J., and Cyr, N. E. (2009). The reactive scope model — A new model integrating homeostasis, allostasis, and stress. Horm. Behav. 55, 375–389. doi: 10.1016/j.yhbeh.2008.12.009
Romero, L. M., and Reed, J. M. (2005). Collecting baseline corticosterone samples in the field: is under 3 min good enough? Comp. Biochem. Physiol. A Mol. Integr. Physiol. 140, 73–79. doi: 10.1016/j.cbpb.2004.11.004
Scheuerlein, A., Van’t Hof, T., and Gwinner, E. (2001). Predators as stressors? Physiological and reproductive consequences of predation risk in tropical stonechats (Saxicola torquata axillaris). Proc. R. Soc. Lond. Ser B Biol. Sci. 268, 1575–1582. doi: 10.1098/rspb.2001.1691
Schmid, B., Chastel, O., and Jenni, L. (2011). The prolactin response to an acute stressor in relation to parental care and corticosterone in a short-lived bird, the Eurasian hoopoe. Gen. Comp. Endocrinol. 174, 22–29. doi: 10.1016/j.ygcen.2011.07.012
Schoenle, L. A., Dudek, A. M., Moore, I. T., and Bonier, F. (2017). Red-winged blackbirds (Agelaius phoeniceus) with higher baseline glucocorticoids also invest less in incubation and clutch mass. Horm. Behav. 90, 1–7. doi: 10.1016/j.yhbeh.2017.02.002
Schwarzkopf, L., and Shine, R. (1992). Costs of reproduction in lizards: escape tactics and susceptibility to predation. Behav. Ecol. Sociobiol. 31, 17–25. doi: 10.1007/BF00167812
Seltmann, M. W., Jaatinen, K., Steele, B. B., and Öst, M. (2014). Boldness and stress responsiveness as drivers of nest-site selection in a ground-nesting bird. Ethology 120, 77–89. doi: 10.1111/eth.12181
Silverin, B. (1986). Corticosterone-binding proteins and behavioral effects of high plasma levels of corticosterone during the breeding period in the pied flycatcher. Gen. Comp. Endocrinol. 64, 67–74. doi: 10.1016/0016-6480(86)90029-8
Slagsvold, T. (1984). Clutch size variation of birds in relation to nest predation: on the cost of reproduction. J. Anim. Ecol. 53:945. doi: 10.2307/4669
Smiley, K. O. (2019). Prolactin and avian parental care: new insights and unanswered questions. Horm. Behav. 111, 114–130. doi: 10.1016/j.yhbeh.2019.02.012
Smiley, K. O., and Adkins-Regan, E. (2016). Prolactin is related to individual differences in parental behavior and reproductive success in a biparental passerine, the zebra finch (Taeniopygia guttata). Gen. Comp. Endocrinol. 234, 88–94. doi: 10.1016/j.ygcen.2016.03.006
Smiley, K. O., and Adkins-Regan, E. (2018). Lowering prolactin reduces post-hatch parental care in male and female zebra finches (Taeniopygia guttata). Horm. Behav. 98, 103–114. doi: 10.1016/j.yhbeh.2017.12.011
Spée, M., Beaulieu, M., Dervaux, A., Chastel, O., Le Maho, Y., and Raclot, T. (2010). Should I stay or should I go? Hormonal control of nest abandonment in a long-lived bird, the Adélie penguin. Horm. Behav. 58, 762–768.
Spée, M., Marchal, L., Lazin, D., Le Maho, Y., Chastel, O., Beaulieu, M., et al. (2011). Exogenous corticosterone and nest abandonment: a study in a long-lived bird, the Adélie penguin. Horm. Behav. 60, 362–370. doi: 10.1016/j.yhbeh.2011.07.003
Stjernberg, T., Koivusaari, J., Högmander, J., Ollila, T., and Ekblom, H. (2005). Population size and nesting success of the white-tailed sea eagle (Haliaeetus albicilla) in Finland, 2003–2004. Yearbook Linnut Magazine 2004, 14–19.
Storey, A. E., Ryan, M. G., Fitzsimmons, M. G., Kouwenberg, A.-L., Takahashi, L. S., Robertson, G. J., et al. (2017). Balancing personal maintenance with parental investment in a chick-rearing seabird: physiological indicators change with foraging conditions. Conserv. Physiol. 5:cox055. doi: 10.1093/conphys/cox055
Swennen, C. (1990). Dispersal and migratory movements of eiders Somateria mollissima breeding in The Netherlands. Ornis Scandinavica 21:17. doi: 10.2307/3676374
Swennen, C. (1991). Ecology and Population Dynamics of the Common Eider in the Dutch Wadden Sea. Groningen: University of Groningen.
Thierry, A.-M., Massemin, S., Handrich, Y., and Raclot, T. (2013). Elevated corticosterone levels and severe weather conditions decrease parental investment of incubating Adélie penguins. Horm. Behav. 63, 475–483. doi: 10.1016/j.yhbeh.2012.12.011
Thomas, V. (1988). Body condition, ovarian hierarchies, and their relation to egg formation in Anseriform and Galliform species. Acta XIX Congressus Int. Ornithol. 19, 353–363.
Travers, M., Clinchy, M., Zanette, L., Boonstra, R., and Williams, T. D. (2010). Indirect predator effects on clutch size and the cost of egg production: Indirect predator effects on clutch size. Ecol. Lett. 13, 980–988. doi: 10.1111/j.1461-0248.2010.01488.x
Turner, A. M. (1996). Freshwater snails alter habitat use in response to predation. Anim. Behav. 51, 747–756. doi: 10.1006/anbe.1996.0079
Tveraa, T., Sether, B., Aanes, R., and Erikstad, K. E. (1998). Regulation of food provisioning in the Antarctic petrel; the importance of parental body condition and chick body mass. J. Anim. Ecol. 67, 699–704. doi: 10.1046/j.1365-2656.1998.00234.x
Verreault, J., Verboven, N., Gabrielsen, G. W., Letcher, R. J., and Chastel, O. (2008). Changes in prolactin in a highly organohalogen contaminated Arctic top predator seabird, the glaucous gull. Gen. Comp. Endocrinol. 156, 569–576. doi: 10.1016/j.ygcen.2008.02.013
Vitousek, M. N., Jenkins, B. R., and Safran, R. J. (2014). Stress and success: individual differences in the glucocorticoid stress response predict behavior and reproductive success under high predation risk. Horm. Behav. 66, 812–819. doi: 10.1016/j.yhbeh.2014.11.004
Vitousek, M. N., Taff, C. C., Hallinger, K. K., Zimmer, C., and Winkler, D. W. (2018). Hormones and fitness: evidence for trade-offs in glucocorticoid regulation across contexts. Front. Ecol. Evol. 6:42. doi: 10.3389/fevo.2018.00042
Vleck, C. M., and Vleck, D. (2011). “Hormones and regulation of parental behavior in birds,” in Hormones and Reproduction of Vertebrates, eds D. O. Norris and K. H. Lopez (Amsterdam: Elsevier), 181–203.
Waldeck, P., Kilpi, M., Andersson, M., and Öst, M. (2004). Brood parasitism in a population of common eider (somateria Mollissima). Behaviour 141, 725–739. doi: 10.1163/1568539042245132
Wang, Y., Székely, T., Zhang, Z., and Que, P. (2020). Prolactin concentrations predict parental investment and nest survival in a free-living shorebird. Horm. Behav. 119:104633. doi: 10.1016/j.yhbeh.2019.104633
Wasser, S. K., and Barash, D. P. (1983). Reproductive suppression among female mammals: implications for biomedicine and sexual selection theory. Q. Rev. Biol. 58, 513–538.
Whittingham, L. A., and Robertson, R. J. (1994). Food availability, parental care and male mating success in red-winged blackbirds (Agelaius phoeniceus). J. Anim. Ecol. 63:139. doi: 10.2307/5590
Williams, G. C. (1966). Natural selection, the costs of reproduction, and a refinement of Lack’s principle. Am. Nat. 100, 687–690. doi: 10.1086/282461
Wingfield, J. C. (2013). Ecological processes and the ecology of stress: the impacts of abiotic environmental factors. Funct. Ecol. 27, 37–44. doi: 10.1111/1365-2435.12039
Wingfield, J. C., Moore, M. C., and Farner, D. S. (1983). Endocrine responses to inclement weather in naturally breeding populations of white-crowned sparrows (Zonotrichia leucophrys pugetensis). Auk 100, 56–62. doi: 10.1093/auk/100.1.56
Keywords: corticosterone, prolactin, individual quality, parental care, predation risk, reproductive success, common eider (Somateria mollissima)
Citation: Mohring B, Angelier F, Jaatinen K, Parenteau C and Öst M (2021) Parental Investment Under Predation Threat in Incubating Common Eiders (Somateria mollissima): A Hormonal Perspective. Front. Ecol. Evol. 9:637561. doi: 10.3389/fevo.2021.637561
Received: 03 December 2020; Accepted: 28 June 2021;
Published: 21 July 2021.
Edited by:
Julien Terraube, University of the Sunshine Coast, AustraliaReviewed by:
Beate Anna Apfelbeck, Technical University of Munich, GermanyWendy Hood, Auburn University, United States
Copyright © 2021 Mohring, Angelier, Jaatinen, Parenteau and Öst. This is an open-access article distributed under the terms of the Creative Commons Attribution License (CC BY). The use, distribution or reproduction in other forums is permitted, provided the original author(s) and the copyright owner(s) are credited and that the original publication in this journal is cited, in accordance with accepted academic practice. No use, distribution or reproduction is permitted which does not comply with these terms.
*Correspondence: Bertille Mohring, YmVydGlsbGUubW9ocmluZ0BhYm8uZmk=