- 1State Key Laboratory of Grassland and Agro-Ecosystems, School of Life Sciences, Lanzhou University, Lanzhou, China
- 2Centre for Ecology and Conservation, College of Life and Environmental Sciences, University of Exeter, Cornwall, United Kingdom
Ecological restoration is widely used to mitigate the negative impacts of anthropogenic activities. There is an increasing demand to identify suitable restoration management strategies for specific habitat and disturbance types to restore interactions between organisms of degraded habitats, such as pollination. In the Tibetan Plateau, alpine meadows have suffered severe degradation due to overgrazing and climate change. Protecting vegetation by fencing during the growing season is a widely applied management regime for restoration of degraded grasslands in this region. Here, we investigated the effect of this restoration strategy on plant–pollinator communities and plant reproduction in the eastern Tibetan Plateau. We collected interaction and seed set data monthly across three grazed (grazed all year) and three ungrazed (fenced during growing season) alpine meadows in growing seasons of two consecutive years. We found ungrazed meadows produced more flowers and attracted more pollinator visits. Many common network metrics, such as nestedness, connectance, network specialization, and modularity, did not differ between grazing treatments. However, plants in ungrazed meadows were more robust to secondary species extinction than those in grazed meadows. The observed changes in the networks corresponded with higher seed set of plants that rely on pollinators for reproduction. Our results indicate that protection from grazing in growing seasons improves pollination network stability and function and thus is a viable restoration approach for degraded meadows.
Introduction
Ecosystem degradation caused by land use change contributes globally to biodiversity decline (Kleijn et al., 2009; Barnes et al., 2014). One major driver of decline in grassland biodiversity is overgrazing by herbivores (Akiyama and Kawamura, 2007). For example, alpine meadows of the Tibetan Plateau have suffered severe degradation and desertification due to overgrazing and altered precipitation (Klein et al., 2004), reducing grassland productivity, vegetation cover, and species diversity (Cao et al., 2013; Hilker et al., 2014). Ecological restoration aims to mitigate negative impacts by restoring species assemblages and ecosystem functions (Aerts and Honnay, 2011; Montoya et al., 2012). There has been an increasing awareness that the restoration of interactions between organisms is critically important as it is crucial to maintain biodiversity (McCann, 2007; Valiente-Banuet et al., 2015). For example, the mutualistic interaction between flowering plants and their animal pollinators have played an important role in the evolution of angiosperms (Ehrlich and Raven, 1964), to the extent that, approximately 87.5% of flowering plants rely on pollinators for reproduction (Ollerton et al., 2011). Changes in land use and habitats negatively affect pollinators, plants, and their interactions at individual, population, and community levels (Hallmann et al., 2017; Forister et al., 2019). While some of these negative impacts can be mitigated or reversed through restoration interventions (e.g., Kaiser-Bunbury et al., 2017), there is an increasing demand to identify suitable restoration management strategies for specific habitat and disturbance types.
To restore and conserve alpine meadows, Tibetan herders have followed government guidelines and adjusted grazing regimes since 2003. In severely degraded areas, grasslands were ungrazed during the growing season but grazed in winter (generally from October to March). Grazing exclusion during the growing season can significantly increase coverage and productivity of plant community in degraded grasslands (Li et al., 2017), which suggests that this approach is an effective restoration practice for the plant community per se. To understand the cascading effects on higher trophic levels, such as pollinators, it is important to study the response of plant–pollinator communities to this reduced grazing regime.
The interactions between multiple plant and pollinator species in a community can be studied with a network approach. In recent years, ecologists have identified several topological characteristics of pollination networks and proposed the ecological significance of the metrics describing the structure (Bascompte and Jordano, 2007; Simmons et al., 2019), which are useful tools in restoration ecology (Raimundo et al., 2018). For example, pollination networks usually show nested (interaction partners of specialist nested within those of generalist) and modular (existence of tightly linked groups of species) structures, both of them are relevant to the biodiversity and stability of ecological communities (Bastolla et al., 2009; Thébault and Fontaine, 2010). Here, we use a network approach to describe the changes of plant–pollinator communities due to vegetation restoration. Based on previous studies that showed that overgrazing reduces abundance of flowers and pollinators while protection from grazing restores the vegetation of degraded grasslands (Xie et al., 2008; Li et al., 2017), we hypothesized that grazing exclusion during the growing season will increase flower abundance and consequently pollinator species richness. We predicted that this increase in species richness will positively affect the interaction diversity of pollination networks. We further anticipated that enhanced interaction diversity will increase the overlap of mutualists and thus increase the functional redundancy of pollination networks, resulting in low values of H and d′, which measure network and species level specialization, respectively. With more species and higher functional redundancy, pollination networks in restored meadows were predicted to have a greater ability to withstand perturbations, which is reflected by higher network robustness to secondary extinction (Memmott et al., 2004; Kaiser-Bunbury et al., 2010). Because alpine plants tend to be extensively pollen limited (Bingham and Ort, 1998), we further predicted that more frequent visits by a more diverse set of pollinators in the restored meadows would increase plant reproductive success.
The goal of this study was to investigate the impact of grazing exclusion during the growing season on plant–pollinator communities and assess the efficacy of these interventions as a tool for alpine ecosystem restoration. To do so, we carried out plant–pollinator observations monthly in growing seasons over two consecutive years and measured pollen limitation and reproductive success of plants at three sites to examine how plant–pollinator interaction networks and pollination function differ between grazed (across whole year) and ungrazed (during growing season) meadows.
Materials and Methods
Study Sites and Setup
The study was conducted at three alpine meadow sites at the Research Station of Alpine Meadow and Wetland Ecosystems of Lanzhou University, Gansu Province, China. One site was located in Hezuo Branch Station (34°55′N, 102°53′E; 2,900 m a.s.l.), with peak flowering season during June to August; the mean annual temperature here is 2°C, and the mean annual precipitation is 560 mm. The other two sites were located in Azi Branch Station (33°40′N, 101°52′E; 3,530 m a.s.l.) approximately 1 km apart, with peak flowering season during July to August; the mean annual temperature here is 1.2°C, and mean annual precipitation is 670 mm. The meadows at all study sites have relatively high species richness (30–40 plant species per 0.25 m2) with dominant grass and sedge species, including Kobresia capillifolia, Agrostis hugoniana, Agrostis trinii, Poa poophagorum, Elymus nutans, and forbs, including Anemone rivularis, Saussurea nigrescens, and Potentilla anserina (Luo et al., 2006).
Each study site consisted of one grazed plot (grazing across the whole year) and one ungrazed plot (grazing exclusion during the growing season). The ungrazed plots (∼1 ha) were fenced by wires. In Hezuo and Azi, the ungrazed plots have excluded livestock (primarily yaks) from March to October each year since 2009 and 2011, respectively. In each plot, one study area (20 m × 25 m) was randomly selected (>10 m from the fence). Plant–pollinator surveys were carried out in two consecutive years. In 2016, the plots at Hezuo were surveyed three times (June, July, and August), while plots at Azi were surveyed twice (July and August) due to their later phenology. A similar survey was replicated in 2017, but only one study site at Azi was surveyed due to logistic constraints.
Pollinator Visitation
To record plant–pollinator interactions, we used timed observations (equal observation time to each plant species), which reduced the risk of under-sampling (Gibson et al., 2011). Video recordings were conducted monthly in each plot between 9:30 and 17:00 during sunny and windless weather using camcorders (Sony HDR) on tripods. In each survey, we recorded all species in the study area, five (2016) and eight (2017) individuals of each plant species in the plot were randomly selected. Camcorders recorded activity at one floral unit (i.e., single flower, umbel, spike, or capitulum depending on the species) per individual. Each individual plant was recorded three times for 15 min with 30–60 min breaks between intervals. In total, we recorded each species for 225 min per plot per month in 2016 and 360 min in 2017. The video recordings of the grazed and ungrazed plots within one study site were conducted synchronously. When video recording was off, we randomly walked in the plot to collect insect specimens using sweep nets, specimens were subsequently identified by specialist entomologists to provide a reference collection of flower visitors (66.29% specimens of flower visitors were collected). Videos were examined in the lab, we recorded pollinator visits whenever insects in the videos contacted floral reproductive organs. Given the limitation of video resolution, only 39 (43.82%) species names can be determined; small and indistinguishable taxa could only be identified to morpho-species level. The visitation rate of each plant species was estimated as the mean visitation rate of recorded plant individuals, and the visitation rate of each plant individual is the number of pollinator visits observed in 45 min (i.e., three 15 min observation). Floral abundance of each species was recorded by counting floral units of each species in three 0.5 m × 0.5 m quadrats, which were randomly selected for each plot and month. By sampling floral abundance in our communities with relatively high species abundance and evenness, we recorded all flowering plant species which were included in the pollinator observation. Floral abundance of each plot was the sum of the floral abundance of all species. We calculated visitation frequency between a pollinator species i and a plant species j as visitation rate of i multiplied by the floral abundance of j (Kaiser-Bunbury et al., 2009). Visitation frequency was used to calculate network metrics. In total, we compiled 24 plant–pollinator networks which comprised 12 grazed networks and 12 ungrazed networks (Hezuo station: 1 site × 2 treatments × 3 months × 2 years; Azi station: 2016: 2 sites × 2 treatments × 2 months, 2017: 1 site × 2 treatments × 2 months).
Plant Performance
To examine the effect of the grazing exclusion treatment on plant reproductive performance, in both years of surveys, we quantified seed production of 18 common plant species (see species list and details in Supplementary Table 2) which flowered at both grazed and ungrazed habitats (62.07% of all studied plant species which flowered at both habitats). We experimentally assessed the degree of pollinator dependence of these species for seed production based on an index that measures the capacity of plant species to produce seeds without pollinators and ranges from 0, which means no dependence on pollinators for reproduction, to 1, which means totally dependent on pollinators for reproduction (Lázaro et al., 2015). According to the degree of pollinator dependence, plant species were divided into a high-dependence group (four species; > 0.9) and low-dependence group (14 species; < 0.4). In each month and plot, we randomly collected 10 (single flower and capitulum) or 30 mature fruits (spike and umbel) from 10 individuals of each species to quantify seed set.
To assess the effect of grazing exclusion on the extent of pollen limitation of flowering plants, we performed supplement hand-pollination experiments to eight species (two high-dependence species and six low-dependence species) in four plots in 2017 (see species list and details in Supplementary Table 2). Specifically, we selected 10 pairs of each species in each plot and marked one flower bud on each individual. Paired individuals were adjacent and had similar size. One flower of each pair received supplemental hand-pollination, and the other one was open-pollinated. The supplement pollen was deposited from flowers of five individuals in each plot. Mature fruits were collected, and seeds were counted to calculate the index of pollen limitation. The index was based on the formula PL = 1 − (N/S), where N was the seed set of open-pollinated flowers and S was the seed set of hand-pollinated flowers (Larson and Barrett, 2000). This approach is suitable only for relative comparisons of within-species differences in pollen limitation between treatments as measurements on the flower level may overestimate the extent of pollen limitation due to the possible resource reallocation (Knight et al., 2006).
Pollination Network Metrics
To evaluate the impact of grazing exclusion on the architecture of plant–pollinator networks, we calculated network structure metrics and compared those between grazed and ungrazed habitats. The chosen metrics were as follows:
(1) Interaction diversity (Shannon’s diversity of interactions).
(2) Interaction evenness (a measure of uniformity of interaction frequency distribution among species).
(3) Connectance, which is calculated by dividing the number of observed links by the number of possible links.
(4) Nestedness, which shows the extent that specialist species tend to interact with generalists (Bascompte et al., 2003). We used the NODFc metric to measure nestedness, which is normalized index and controlled the impacts of connectance and network size on nestedness (Song et al., 2017).
(5) Specialization of network (H), plant species (d′pl), and pollinator species (d′pol), which range from 0 to 1, where 1 is highly specialized and can be compared directly within (d′) and between (H2′) networks (Blüthgen et al., 2006).
(6) Modularity reflects the tendency of a network to be organized in distinct clusters, where species within a cluster interact more frequently with each other than with species from other clusters (Olesen et al., 2007). We used Beckett’s algorithm to calculate modularity (Beckett, 2016), where higher values indicate a more modular structure. Modularity could mitigate the spread of disturbance through the network (Gilarranz et al., 2017).
(7) Robustness of plant species, which indicates vulnerability of plant species to face random extinction of pollinator species; a greater metric indicates a more robust network (Memmott et al., 2004).
(8) Web asymmetry, which indicates the balance between the number of pollinator species and plant species (Blüthgen et al., 2007). A higher value indicates more pollinator species than plant species. Web asymmetry is relevant to the robustness of networks by influencing the redundancy of pollinators (Santamaría et al., 2014). All network metrics were calculated in R (R Core Team, 2019) using package “maxnodf” (Hoeppke and Simmons, 2021) and “bipartite” (Dormann et al., 2008, 2009).
Statistical Analysis
All analyses were conducted in R version 3.5.1 (R Core Team, 2019) with the packages lme4, lmerTest, and MuMIn. To test the response of pollinator richness and network metrics to grazing exclusion, we used linear mixed models (LMMs). To evaluate the effect of treatment on floral abundance, we used generalized LMMs (GLMMs) with Poisson distribution. In both sets of models, we included grazing type (grazed and ungrazed) as a fixed factor and month and site as random factors. We add network size as a covariate in the models for network metrics that are known to be sensitive to network size, i.e., connectance and modularity (Blüthgen, 2010; Fründ et al., 2016). At the species level, the effect of grazing type (fixed effect) on d′pl and d′pol was analyzed using LMMs, month, site, and plant (d′pl) or pollinator (d′pol) species were fitted as random effects. All above models were checked for the normality and homoscedasticity.
Pollinator visitation rates were compared between grazing types using GLMMs with Poisson distributions. The response variable was the visitation rate of plant individual. Visitation rates of each insect order (Coleoptera, Diptera, Hymenoptera, and Lepidoptera) were also separately analyzed. In the above five GLMMs, we used grazing types as fixed effect and individuals nested within plant species, month, and site as random effects.
The effects of grazing exclusion on pollen limitation and seed production were examined by GLMMs with binominal distributions. The response variable was the seed set of each plant individual. In the pollen limitation analysis model, we fitted grazing type, pollination treatment (open pollination and supplemental pollination), pollinator dependence (high dependence and low dependence), and their two-way and three-way interaction terms as fixed effects, plant pairs nested within plant species as a nested random effect, and site as a crossed random effect. In the seed production analysis model, grazing type, pollinator dependence, and their interaction term were fitted as fixed effects and plant individuals nested within plant species, month, and site were fitted as random effects. If interaction terms of fixed effects were detected in the above models, we tested the effects of grazing types and pollination treatments on seed set for high-dependence and low-dependence species individually. In all GLMMs, observation-level random factor (as many levels as observations) was included in the models when overdispersion was detected.
Results
Pollinator Community
In total, we surveyed 47 plant species, eight of them flowered only in grazed grasslands, 10 of them flowered only in ungrazed grasslands, and 29 of them flowered in both habitats. We observed 2,512 visits by 89 pollinator morpho-species in 4,311 15 min observation sessions (1,078 h total). Pollinators included species of Diptera (32), Hymenoptera (20), Coleoptera (18), Lepidoptera (13), and other groups (6) (Supplementary Table 3). Pollinator visitation rate (grazed: 2.06 ± 0.12; ungrazed: 2.55 ± 0.18 visits/h/flower unit, z = 4.239, P < 0.001) was higher in the ungrazed communities than in the grazed communities (Figure 1), and there was a tendency toward higher pollinator richness in ungrazed habitats (grazed: 15.25 ± 1.86; ungrazed: 18.58 ± 1.67, t = 2.071, P = 0.053). Specifically, species richness of Diptera (Figure 1A) and visitation rates of Diptera, Hymenoptera, and Lepidoptera were increased by grazing exclusion treatment (Figure 1B).
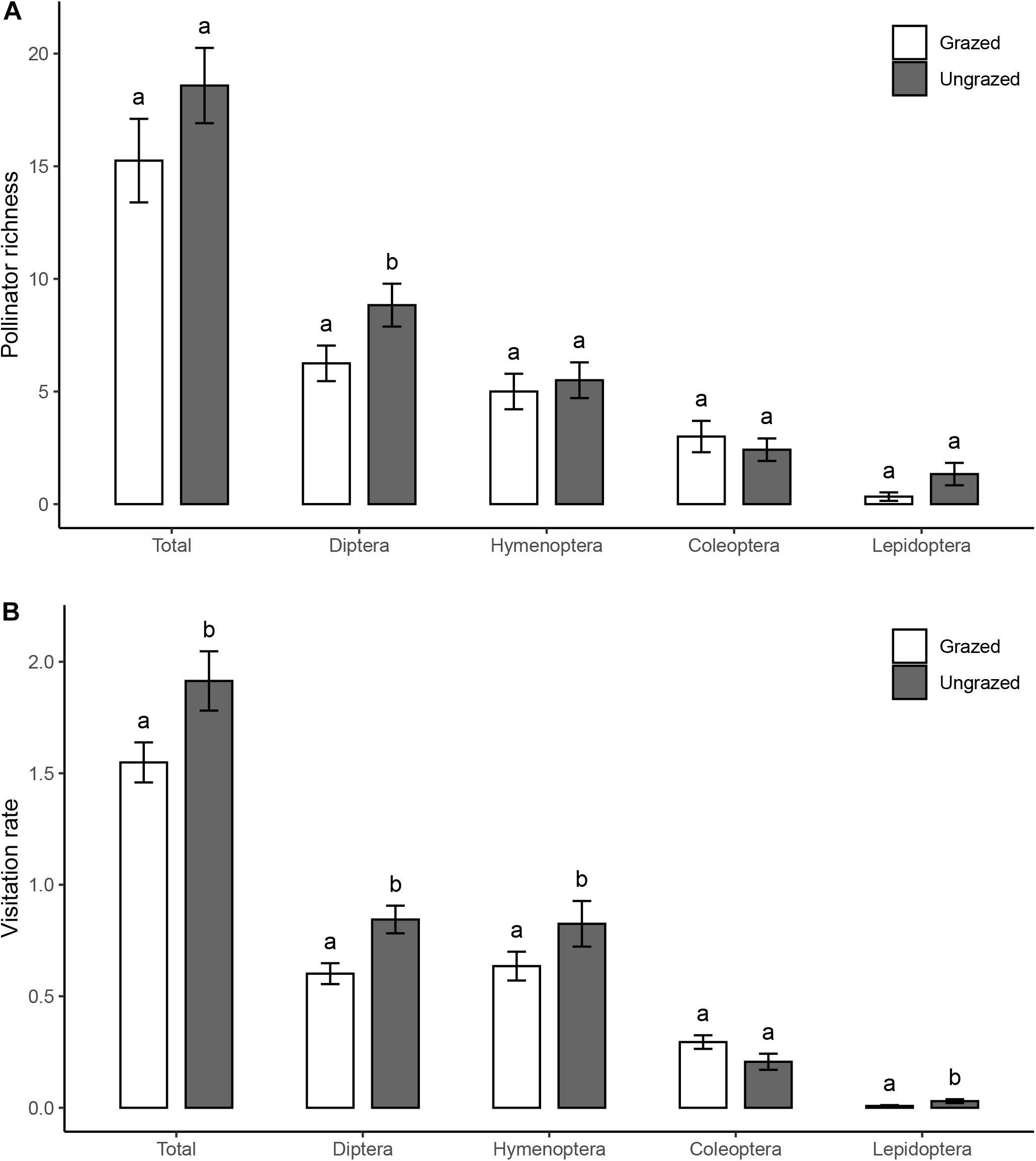
Figure 1. (A) Number of total and of each order pollinator species (n = 24 networks) in grazed and ungrazed habitats. (B) Visitation rate from total and of each order pollinators (based on data of the individual observation of each plant species in each network, n = 1,414). Bar plots show mean ± SE. White bars represent grazed habitats, and gray bars represent ungrazed habitats. Letters shown above the bar plots indicate the statistical difference between grazed and ungrazed habitats (P = 0.05). Results from statistical models are given in Table 1A and Supplementary Table 1.
Network Structure and Metrics
The grazing exclusion treatment did not alter the interaction diversity, interaction evenness, connectance, nestedness, modularity, and specialization of networks, while web asymmetry of ungrazed communities was higher than that of grazed communities (Figure 2 and Table 1A). Protection from grazing also increased plant robustness to random extinction of pollinator species (Figure 2 and Table 1A). Plants in ungrazed communities became more specialized (marginally significant, t = 1.928, P = 0.055) compared to those in grazed communities, a pattern that was not seen for pollinators (Table 1A).
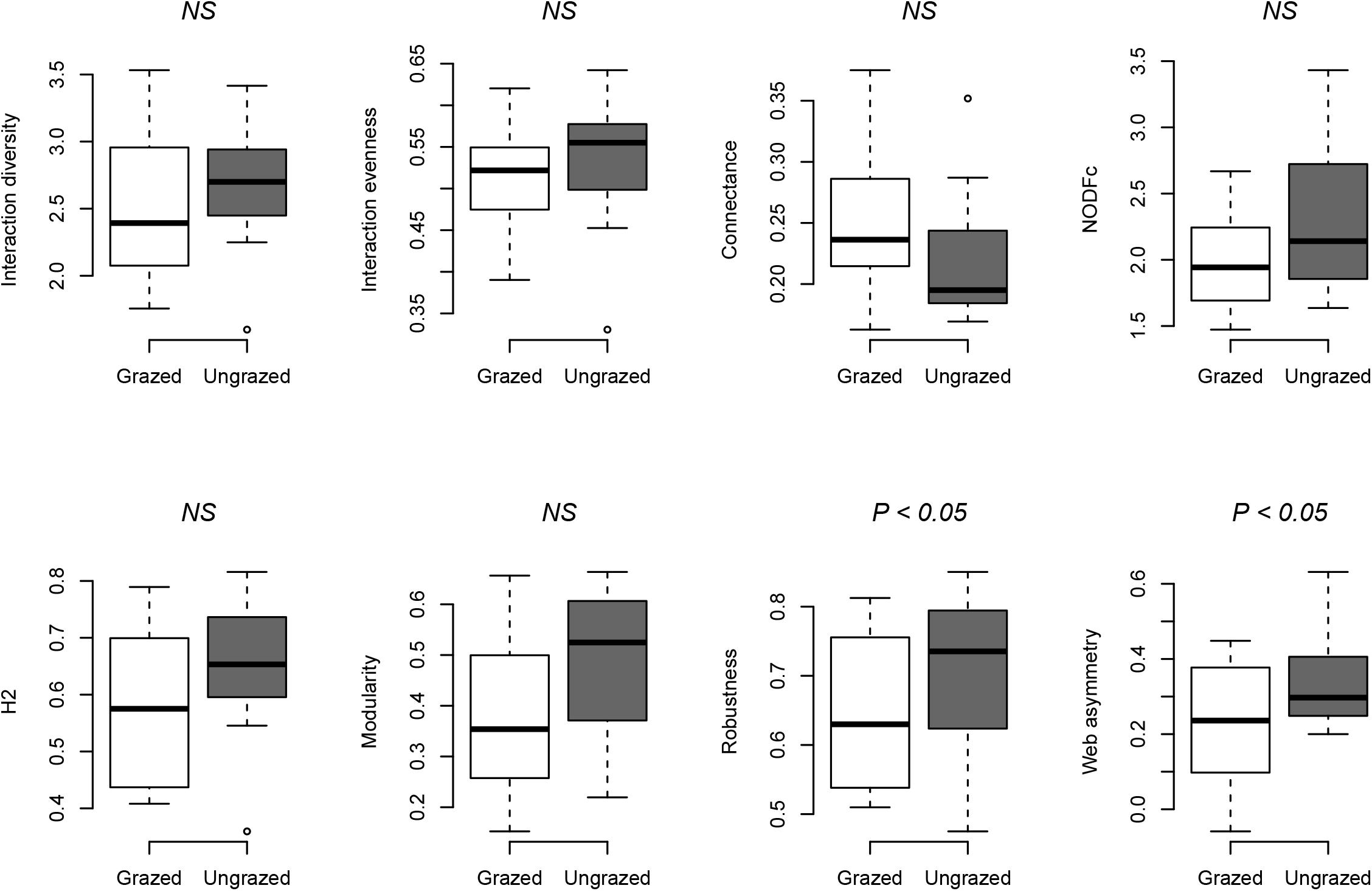
Figure 2. Box plots illustrating the network structure metrics in grazed and ungrazed networks (n = 24 networks). The horizontal lines across boxes are medians. The bottom and top limits of each box are the lower and upper quartiles (25 and 75%, respectively). White boxes represent grazed habitats, and gray boxes represent ungrazed habitats. Statistics are shown in Table 1. P-values are shown above the box plots; NS indicates no significant difference.
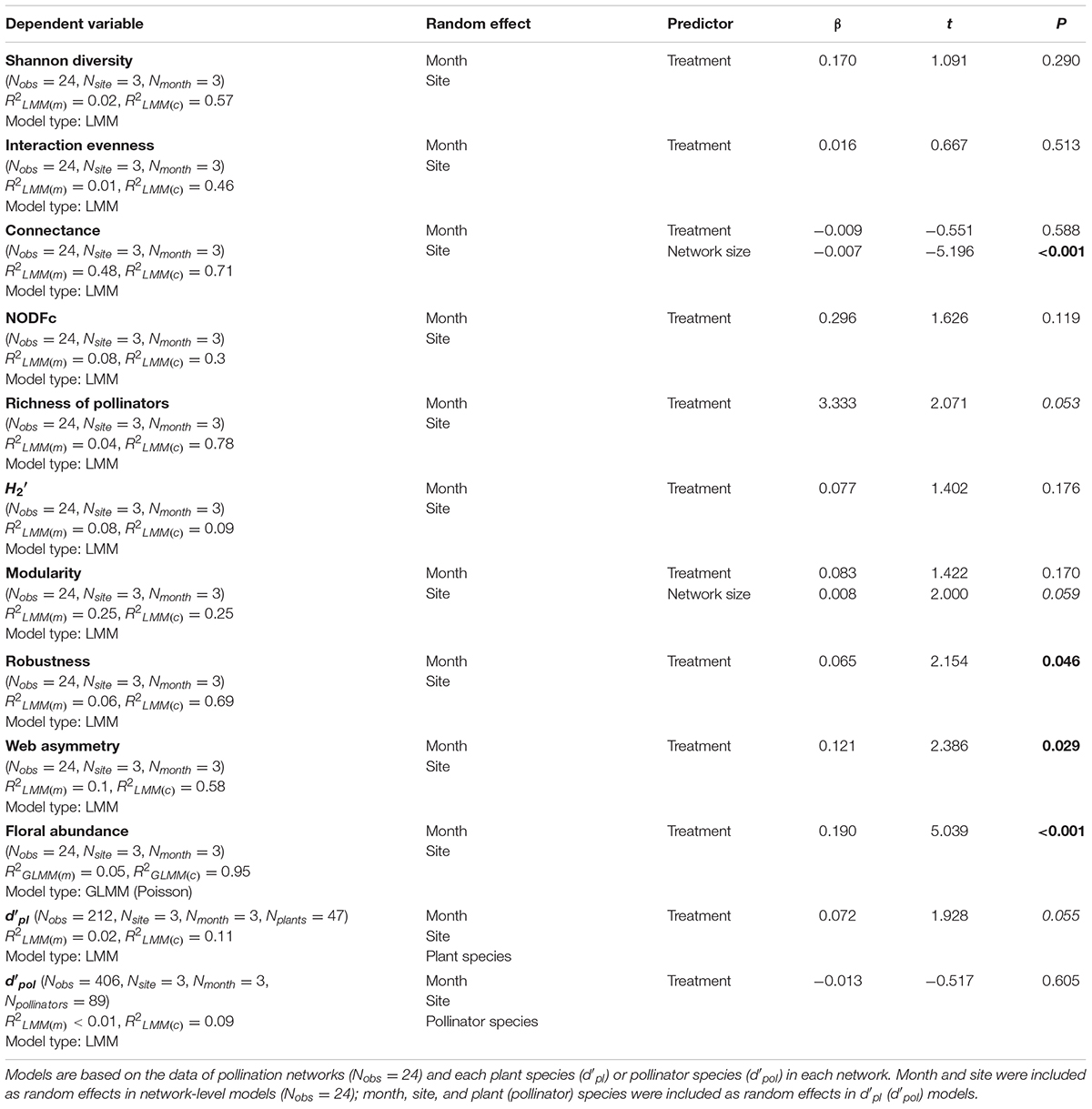
Table 1A. LMMs and GLMMs with Poisson distribution explaining the effects of treatment (grazed versus ungrazed) on plant–pollinator communities and network structure.
Plant Performance
Ungrazed plots had higher floral abundance (173.0 ± 37.3 flower units/m–2) than grazed plots (143.1 ± 19.4 flower units/m–2; Table 1A). In both grazed and ungrazed communities, hand pollination increased seed set of plants compared to open pollination, suggesting that plants in both types of communities are pollen limited (Figure 3). However, pollen limitation was stronger in grazed plots (grazed: 0.25 ± 0.02; ungrazed: 0.16 ± 0.03, t = −2.882, P < 0.01) (Table 1B). This effect was similar for plants that relied strongly and weakly on pollinators for seed set. When including more species (18 species), analysis showed that the grazing exclusion treatment increased the seed set of the high-dependence species rather than low-dependence species (Figure 4 and Table 1B).
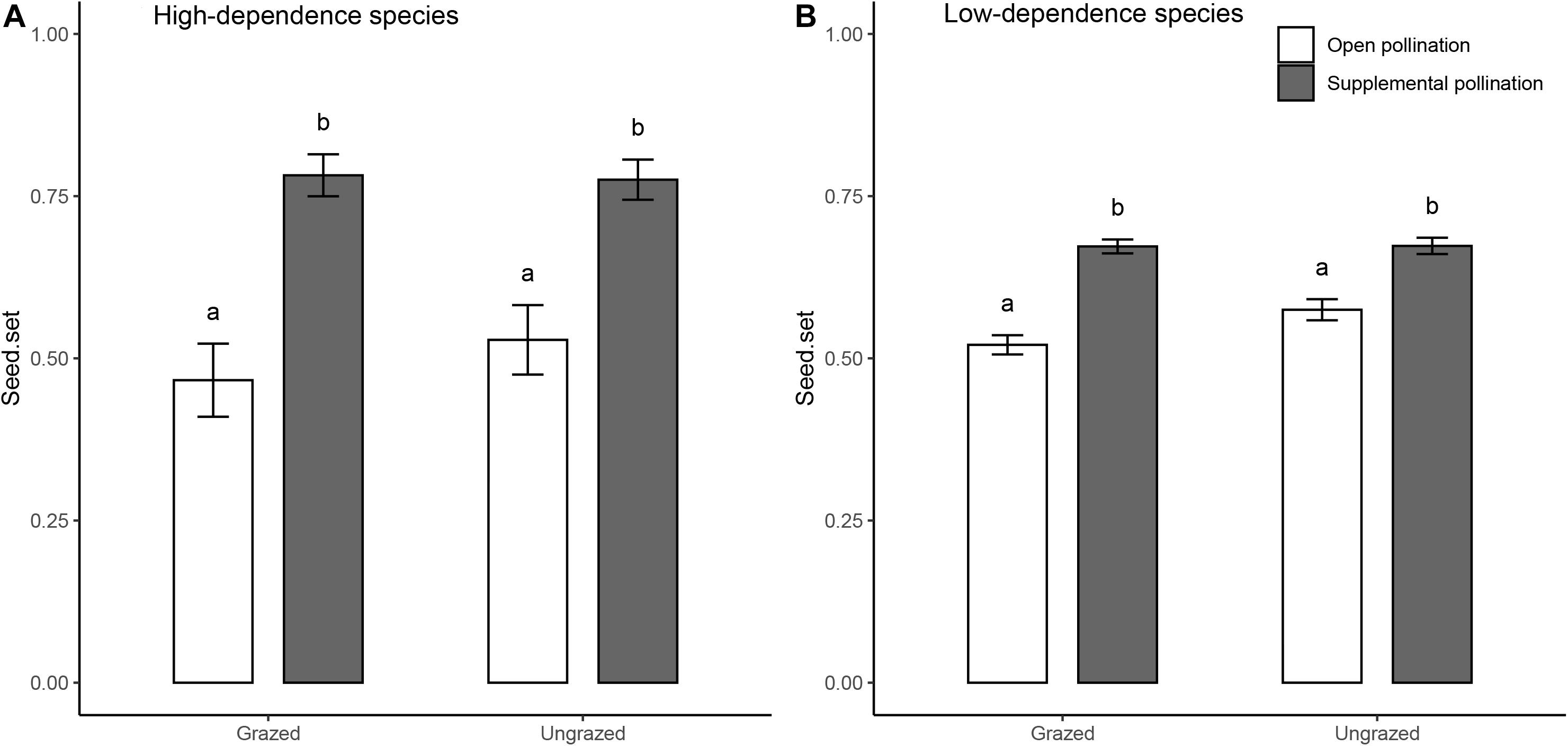
Figure 3. The effects of supplemental pollination on the seed set of two high-dependence (A) and six low-dependence (B) species in grazed and ungrazed habitats. Letters shown above the bar plots indicate the statistical difference between open pollination and supplemental pollination (P = 0.05).
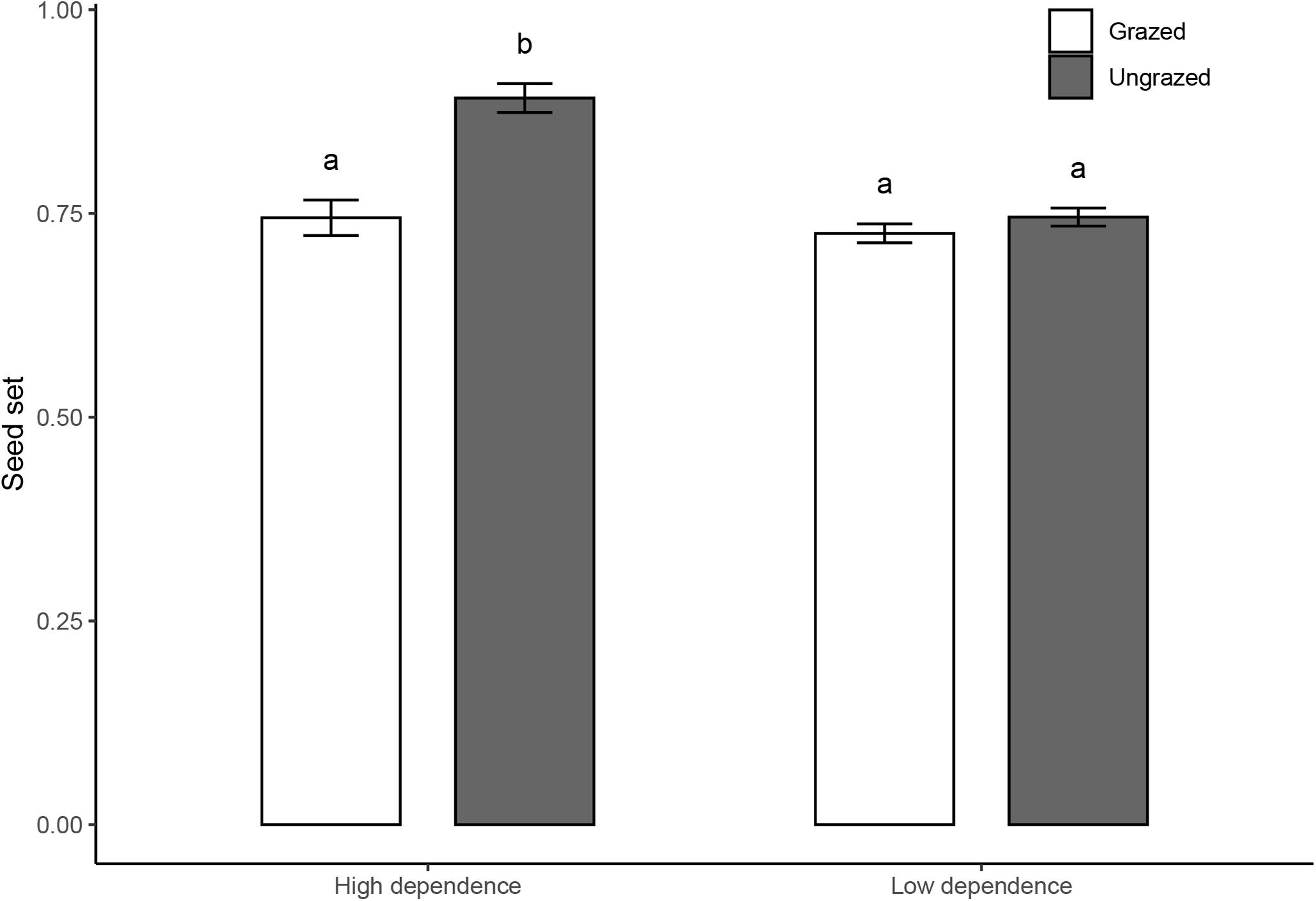
Figure 4. Seed set (mean ± SE) of four high-dependence and 14 low-dependence species in grazed and ungrazed Tibetan alpine meadows. White bars represent grazed habitats, and gray bars represent ungrazed habitats. Letters shown above the bar plots indicate the statistical difference between grazed and ungrazed habitats (P = 0.05).
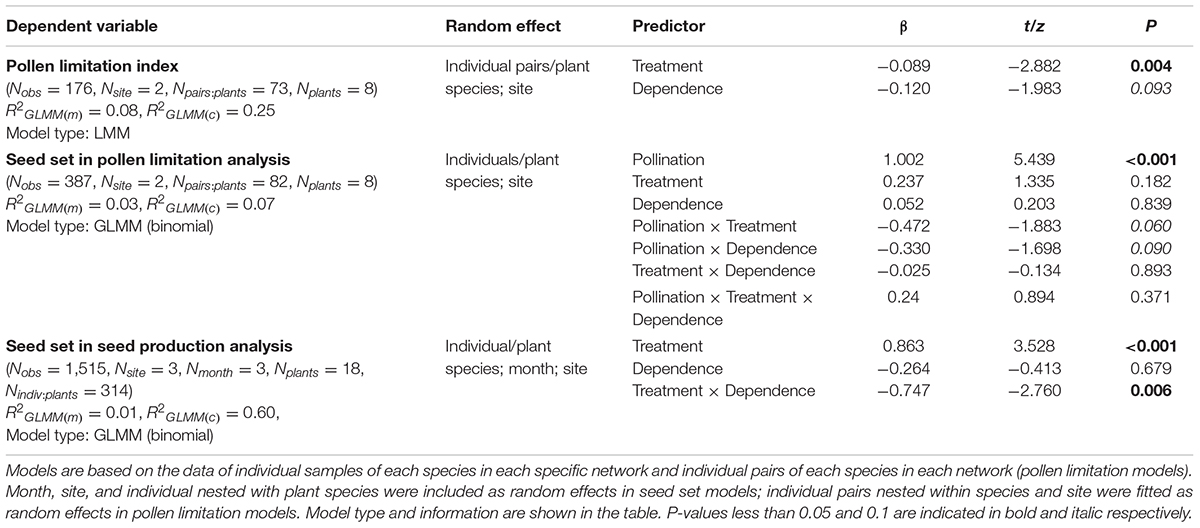
Table 1B. GLMMs with binomial distributions explaining the effects of treatment (grazed versus ungrazed), pollination (open pollination versus supplemental pollination), and pollinator dependence (high dependence versus low dependence) on seed set of plants.
Discussion
We showed that a reduction in grazing positively affected plant–pollinator interactions in Tibetan alpine meadows. Observed changes in interactions and functions, however, are not necessarily reflected by changes in the structure of plant–pollinator networks. Earlier studies have shown that grazing exclusion during growing seasons can increase vegetation cover and biomass of grasslands (Wu et al., 2008). Here, we showed that the benefits from reduced grazing were passed on to a higher trophic levels with positive feedback loops for plants through increased pollination function. Independent of treatments, pollinator communities were dominated by flies and hoverflies, which adapt well to harsh climatic conditions of alpine regions, making them important pollinators of alpine meadows (Elberling and Olesen, 1999). The observed increase in pollinator richness and visitation rate may be driven by higher floral abundance in ungrazed meadows, as more abundant and diverse floral displays attract more pollinator species and interactions (Sieber et al., 2011). Some plant species (e.g., Trollius farreri, Cirsium setosum, Picris japonica, and Aster souliei) only flowered in ungrazed meadows and attracted large numbers of Diptera species (59.38% of Diptera species in this study were observed on these four species in total). Large floral displays and flowers that are easily accessible make these species particularly attractive to flies (Inouye et al., 2015). It is therefore likely that the attractiveness of a few plants in meadows with reduced grazing benefits the entire community by increasing pollination function across the community (Mesgaran et al., 2017).
Despite the observed changes in plant–pollinator interactions, many network metrics failed to detect these ecological processes. For instance, interaction diversity, interaction evenness, connectance, nestedness, modularity, and network-level generalization were similar between treatments. Although no statistical significance was found in network-level generalization analysis, there was a trend toward higher specialization in ungrazed networks. Furthermore, species-level specialization of plants (d′pl) in ungrazed meadows was higher than that in grazed habitats. In line with this pattern, in a review about how environmental quality influence pollination network metrics, Soares et al. (2017) found that better environmental quality was usually associated with higher H2′ and d′ values (Burkle and Knight, 2012; Weiner et al., 2014). For example, Marrero et al. (2014) found that grasslands in Argentina restored by long-time fencing had more specialized networks than grazed grasslands. In all cases reviewed by Soares et al. (2017), a decrease in specialization was attributed to simplification of the network structure, with less species and interaction, which also accords with our results. However, an increase in number of species and interactions may also be associated with a loss of network specialization; as found by Kaiser-Bunbury et al. (2017), networks in habitats restored by removing exotic plants were more generalized and more diverse than those in unrestored habitats. This pattern was largely due to the shift by two super-generalist and abundant pollinators from alien to native flowers in restored communities, thus reducing the homogenization of interaction and the network specialization.
We found that the pollination networks in the ungrazed meadows are more robust to secondary extinction caused by random loss of pollinator species. This may be attributed to the increased web asymmetry of pollination networks in ungrazed habitats. In our study, robustness of plants was positively correlated with web asymmetry (Pearson’s correlation = 0.545, P = 0.006), which is consistent with predictions by simulation (Pastor et al., 2012) and empirical results (Santamaría et al., 2014). In our study, Asteraceae species (e.g., C. setosum, Saussurea hieracioides, A. souliei, and Taraxacum mongolicum) may contribute the most to asymmetry of ungrazed networks. For example, the most asymmetric network in our study (web asymmetry = 0.63) has two generalist Asteraceae plants (both degree = 12), C. setosum and S. hieracioides, which both interact with six pollinator species that only have one partner, resulting in a high-value web asymmetry. The larger web asymmetry in ungrazed habitats indicates more pollinator species per plant (Memmott et al., 2004; Ramos-Jiliberto et al., 2012), which may be linked to the decreased co-extinction risk of plant species as a result of pollinator loss. Our results suggest that a reduction in grazing times can enhance plant community robustness in the face of potential pollinator loss caused by disturbances (Xie et al., 2008) but that these changes are not persistently evident in the network structure.
Most importantly, from a conservation perspective, our data showed that increased pollinator visitation in ungrazed meadows was positively related to plant reproduction performance, which suggests that the pollination functions of degraded alpine meadows were at least partially restored. However, whether the pollination function of the ungrazed grassland resemble those of undisturbed grasslands is unknown, as no such natural grassland exist in our study area. Consistent with other studies of alpine plants (Bingham and Ort, 1998), seed production was pollen limited at our study sites. Reduced grazing markedly reduced pollen limitation and resulted in increased seed set. This conclusion was corroborated by the increased seed set of high-dependence species in ungrazed meadows. Stavert et al. (2019) experimentally showed that the complementarity in pollinator use among plant species increased seed production of plants. Therefore, the higher visitation rate to high-dependence plants and specificity of pollinators in the ungrazed meadows appeared to improve plant reproductive performance. However, this is not true for the low-dependence plant species. Pollen limitation of low-dependence species was marginally lower than that of high-dependence species, which supports previous findings that showed a positive relationship between pollen limitation and degree of pollinator dependence (Lázaro et al., 2015). Reproductive assurance provided by autonomous self-fertilization may make the seed production of low-dependence plants less limited by pollen and not sensitive to the changed pollinator visitation (Morgan and Wilson, 2005). Furthermore, most low-dependence species (12 of 14 species) have actinomorphic and open flowers, presenting a generalized pollination system (Fenster et al., 2004). More generalized pollination is known to likely increase the heterospecific pollen loads (Arceo-Gómez et al., 2016). Therefore, the stigmas of low-dependence species might be clogged more by heterospecific pollen, which may be an alternative reason that the increased visitation in ungrazed plots did not increase seed production of low-dependence species.
Conclusion
To conclude, our study provides support for the restoration regime of reducing grazing times on alpine meadows in the Tibetan Plateau. Protecting plants from grazing during the growing season can increase floral abundance and pollinator richness, which in turn may increase community robustness to perturbations. Most importantly, plants receive more pollen and produce more seeds in areas with reduced grazing pressure, which suggests that this management strategy has positive effects on the long-term viability of the Tibetan alpine meadows.
Data Availability Statement
The raw data supporting the conclusions of this article will be made available by the authors, without undue reservation.
Author Contributions
EG, ZZ, and YW designed the research. EG, YW, and CB conducted the field work. EG performed the data analyses with input from CK-B. EG, ZZ, and CK-B wrote the initial manuscript. All other authors contributed to writing and commented on the manuscript. All authors contributed to the article and approved the submitted version.
Funding
This work was funded by the National Key Research and Development Program of China (Grant No. 2017YFC0504801), the Second Tibetan Plateau Scientific Expedition and Research (Grant No. 2019QZKK0302) and the Natural Science Foundation of China (Grant Nos. 31570229 and 31870411) to ZZ.
Conflict of Interest
The authors declare that the research was conducted in the absence of any commercial or financial relationships that could be construed as a potential conflict of interest.
Acknowledgments
We thank Xinwei Li, Jieyu Yang, and Lili Yang for help in the field and lab. The help with insect identification of Hongjian Wang is greatly appreciated. We thank Lorne M. Wolfe for useful comments on previous drafts. We appreciate two reviewers for their helpful comments to this work.
Supplementary Material
The Supplementary Material for this article can be found online at: https://www.frontiersin.org/articles/10.3389/fevo.2021.632961/full#supplementary-material
References
Aerts, R., and Honnay, O. (2011). Forest restoration, biodiversity and ecosystem functioning. BMC Ecol. 11:29. doi: 10.1186/1472-6785-11-29
Akiyama, T., and Kawamura, K. (2007). Grassland degradation in China: methods of monitoring, management and restoration. Grassl. Sci. 53, 1–17. doi: 10.1111/j.1744-697X.2007.00073.x
Arceo-Gómez, G., Abdala-Roberts, L., Jankowiak, A., Kohler, C., Meindl, G. A., Navarro-Fernandez, C. M., et al. (2016). Patterns of among- and within-species variation in heterospecific pollen receipt: the importance of ecological generalization. Am. J. Bot. 103, 396–407. doi: 10.3732/ajb.1500155
Barnes, A. D., Jochum, M., Mumme, S., Haneda, N. F., Farajallah, A., Widarto, T. H., et al. (2014). Consequences of tropical land use for multitrophic biodiversity and ecosystem functioning. Nat. Commun. 5:5351. doi: 10.1038/ncomms6351
Bascompte, J., and Jordano, P. (2007). Plant-animal mutualistic networks: the architecture of biodiversity. Annu. Rev. Ecol. Evol. Syst. 38, 567–593. doi: 10.1146/annurev.ecolsys.38.091206.095818
Bascompte, J., Jordano, P., Melián, C. J., and Olesen, J. M. (2003). The nested assembly of plant–animal mutualistic networks. Proc. Natl. Acad. Sci. U.S.A. 100, 9383–9387. doi: 10.1073/pnas.1633576100
Bastolla, U., Fortuna, M. A., Pascual-Garcia, A., Ferrera, A., Luque, B., and Bascompte, J. (2009). The architecture of mutualistic networks minimizes competition and increases biodiversity. Nature 458, 1018–1020. doi: 10.1038/nature07950
Beckett, S. J. (2016). Improved community detection in weighted bipartite networks. R. Soc. Open Sci. 3:140536.
Bingham, R. A., and Ort, A. R. (1998). Efficient pollination of alpine plants. Nature 391, 238–239. doi: 10.1038/34564
Blüthgen, N. (2010). Why network analysis is often disconnected from community ecology: a critique and an ecologist’s guide. Basic Appl. Ecol. 11, 185–195. doi: 10.1016/j.baae.2010.01.001
Blüthgen, N., Menzel, F., and Blüthgen, N. (2006). Measuring specialization in species interaction networks. BMC Ecol. 6:9. doi: 10.1186/1472-6785-6-9
Blüthgen, N., Menzel, F., Hovestadt, T., Fiala, B., and Blüthgen, N. (2007). Specialization, constraints, and conflicting interests in mutualistic networks. Curr. Biol. 17, 341–346. doi: 10.1016/j.cub.2006.12.039
Burkle, L. A., and Knight, T. M. (2012). Shifts in pollinator composition and behavior cause slow interaction accumulation with area in plant-pollinator networks. Ecology 93, 2329–2335. doi: 10.1890/12-0367.1
Cao, J., Yeh, E. T., Holden, N. M., Qin, Y., and Ren, Z. (2013). The roles of overgrazing, climate change and policy as drivers of degradation of China’s grasslands. Nomad. Peoples 17, 82–101. doi: 10.3167/np.2013.170207
Dormann, C. F., Fründ, J., Blüthgen, N., and Gruber, B. (2009). Indices, graphs and null models: analyzing bipartite ecological networks. Open Ecol. J. 2, 7–24. doi: 10.2174/1874213000902010007
Dormann, C. F., Gruber, B., and Fründ, J. (2008). Introducing the bipartite package: analysing ecological networks. R News 8, 8–11.
Ehrlich, P. R., and Raven, P. H. (1964). Butterflies and plants: a study in coevolution. Evolution 18, 586–608. doi: 10.2307/2406212
Elberling, H., and Olesen, J. M. (1999). The structure of a high latitude plant-flower visitor system: the dominance of flies. Ecography 22, 314–323. doi: 10.1111/j.1600-0587.1999.tb00507.x
Fenster, C. B., Armbruster, W. S., Wilson, P., Dudash, M. R., and Thomson, J. D. (2004). Pollination syndromes and floral specialization. Annu. Rev. Ecol. Evol. Syst. 35, 375–403. doi: 10.1146/annurev.ecolsys.34.011802.132347
Forister, M. L., Pelton, E. M., and Black, S. H. (2019). Declines in insect abundance and diversity: we know enough to act now. Conserv. Sci. Pract. 1:e80. doi: 10.1111/csp2.80
Fründ, J., McCann, K. S., and Williams, N. M. (2016). Sampling bias is a challenge for quantifying specialization and network structure: lessons from a quantitative niche model. Oikos 125, 502–513. doi: 10.1111/oik.02256
Gibson, R. H., Knott, B., Eberlein, T., and Memmott, J. (2011). Sampling method influences the structure of plant-pollinator networks. Oikos 120, 822–831. doi: 10.1111/j.1600-0706.2010.18927.x
Gilarranz, L. J., Rayfield, B., Liñán-Cembrano, G., Bascompte, J., and Gonzalez, A. (2017). Effects of network modularity on the spread of perturbation impact in experimental metapopulations. Science 357, 199–201. doi: 10.1126/science.aal4122
Hallmann, C. A., Sorg, M., Jongejans, E., Siepel, H., Hofland, N., Schwan, H., et al. (2017). More than 75 percent decline over 27 years in total flying insect biomass in protected areas. PLoS One 12:e0185809. doi: 10.1371/journal.pone.0185809
Hilker, T., Natsagdorj, E., Waring, R. H., Lyapustin, A., and Wang, Y. (2014). Satellite observed widespread decline in Mongolian grasslands largely due to overgrazing. Glob. Chang. Biol. 20, 418–428. doi: 10.1111/gcb.12365
Hoeppke, C., and Simmons, B. I. (2021). Maxnodf: an R package for fair and fast comparisons of nestedness between networks. Methods Ecol. Evol. 1–6. doi: 10.1111/2041-210X.13545
Inouye, D. W., Larson, B. M., Ssymank, A., and Kevan, P. G. (2015). Flies and flowers III: ecology of foraging and pollination. J. Pollinat. Ecol. 16, 115–133.
Kaiser-Bunbury, C. N., Memmott, J., and Müller, C. B. (2009). Community structure of pollination webs of Mauritian heathland habitats. Perspect. Plant Ecol. Evol. Syst. 11, 241–254. doi: 10.1016/j.ppees.2009.04.001
Kaiser-Bunbury, C. N., Mougal, J., Whittington, A. E., Valentin, T., Gabriel, R., Olesen, J. M., et al. (2017). Ecosystem restoration strengthens pollination network resilience and function. Nature 542, 223–227. doi: 10.1038/nature21071
Kaiser-Bunbury, C. N., Muff, S., Memmott, J., Muller, C. B., and Caflisch, A. (2010). The robustness of pollination networks to the loss of species and interactions: a quantitative approach incorporating pollinator behaviour. Ecol. Lett. 13, 442–452. doi: 10.1111/j.1461-0248.2009.01437.x
Kleijn, D., Kohler, F., Báldi, A., Batáry, P., Concepción, E., Clough, Y., et al. (2009). On the relationship between farmland biodiversity and land-use intensity in Europe. Proc. R. Soc. B Biol. Sci. 276, 903–909. doi: 10.1098/rspb.2008.1509
Klein, J. A., Harte, J., and Zhao, X. Q. (2004). Experimental warming causes large and rapid species loss, dampened by simulated grazing, on the Tibetan plateau. Ecol. Lett. 7, 1170–1179. doi: 10.1111/j.1461-0248.2004.00677.x
Knight, T. M., Steets, J. A., and Ashman, T. L. (2006). A quantitative synthesis of pollen supplementation experiments highlights the contribution of resource reallocation to estimates of pollen limitation. Am. J. Bot. 93, 271–277. doi: 10.3732/ajb.93.2.271
Larson, B. M. H., and Barrett, S. C. H. (2000). A comparative analysis of pollen limitation in flowering plants. Biol. J. Linn. Soc. 69, 503–520.
Lázaro, A., Lundgren, R., and Totland, Ø (2015). Pollen limitation, species’ floral traits and pollinator visitation: different relationships in contrasting communities. Oikos 124, 174–186. doi: 10.1111/oik.01525
Li, W., Cao, W., Wang, J., Li, X., Xu, C., and Shi, S. (2017). Effects of grazing regime on vegetation structure, productivity, soil quality, carbon and nitrogen storage of alpine meadow on the Qinghai-Tibetan plateau. Ecol. Eng. 98, 123–133. doi: 10.1016/j.ecoleng.2016.10.026
Luo, Y., Qin, G., and Du, G. (2006). Importance of assemblage-level thinning: a field experiment in an alpine meadow on the Tibet plateau. J. Veg. Sci. 17, 417–424. doi: 10.1111/j.1654-1103.2006.tb02462.x
Marrero, H. J., Torretta, J. P., and Medan, D. (2014). Effect of land use intensification on specialization in plant–floral visitor interaction networks in the Pampas of Argentina. Agric. Ecosyst. Environ. 188, 63–71. doi: 10.1016/j.agee.2014.02.017
Memmott, J., Waser, N. M., and Price, M. V. (2004). Tolerance of pollination networks to species extinctions. Proc. Biol. Sci. 271, 2605–2611. doi: 10.1098/rspb.2004.2909
Mesgaran, M. B., Bouhours, J., Lewis, M. A., and Cousens, R. D. (2017). How to be a good neighbour: facilitation and competition between two co-flowering species. J. Theor. Biol. 422, 72–83. doi: 10.1016/j.jtbi.2017.04.011
Montoya, D., Rogers, L., and Memmott, J. (2012). Emerging perspectives in the restoration of biodiversity-based ecosystem services. Trends Ecol. Evol. 27, 666–672. doi: 10.1016/j.tree.2012.07.004
Morgan, M. T., and Wilson, W. G. (2005). Self-fertilization and the escape from pollen limitation in variable pollination environments. Evolution 59, 1143–1148. doi: 10.1111/j.0014-3820.2005.tb01050.x
Olesen, J. M., Bascompte, J., Dupont, Y. L., and Jordano, P. (2007). The modularity of pollination networks. Proc.Natl. Acad. Sci. U.S.A. 104, 19891–19896. doi: 10.1073/pnas.0706375104
Ollerton, J., Winfree, R., and Tarrant, S. (2011). How many flowering plants are pollinated by animals? Oikos 120, 321–326. doi: 10.1111/j.1600-0706.2010.18644.x
Pastor, J. M., Santamaría, S., Méndez, M., and Galeano, J. (2012). Effects of topology on robustness in ecological bipartite networks. Netw. Heterogen. Media 7, 429–440. doi: 10.3934/nhm.2012.7.429
Raimundo, R. L. G., Guimaraes, P. R. Jr., and Evans, D. M. (2018). Adaptive networks for restoration ecology. Trends Ecol. Evol. 33, 664–675. doi: 10.1016/j.tree.2018.06.002
Ramos-Jiliberto, R., Valdovinos, F. S., de Espanés, P. M., and Flores, J. D. (2012). Topological plasticity increases robustness of mutualistic networks. J. Anim. Ecol. 81, 896–904. doi: 10.1111/j.1365-2656.2012.01960.x
Santamaría, S., Galeano, J., Pastor, J. M., and Méndez, M. (2014). Robustness of alpine pollination networks: effects of network structure and consequences for endemic plants. Arct. Antarct. Alp. Res. 46, 568–580. doi: 10.1657/1938-4246-46.3.568
Sieber, Y., Holderegger, R., Waser, N. M., Thomas, V. F. D., Braun, S., Erhardt, A., et al. (2011). Do alpine plants facilitate each other’s pollination? Experiments at a small spatial scale. Acta Oecol. 37, 369–374. doi: 10.1016/j.actao.2011.04.005
Simmons, B. I., Cirtwill, A. R., Baker, N. J., Wauchope, H. S., Dicks, L. V., Stouffer, D. B., et al. (2019). Motifs in bipartite ecological networks: uncovering indirect interactions. Oikos 128, 154–170. doi: 10.1111/oik.05670
Soares, R. G. S., Ferreira, P. A., and Lopes, L. E. (2017). Can plant-pollinator network metrics indicate environmental quality? Ecol. Indic. 78, 361–370. doi: 10.1016/j.ecolind.2017.03.037
Song, C., Rohr, R. P., and Saavedra, S. (2017). Why are some plant–pollinator networks more nested than others? J. Anim. Ecol. 86, 1417–1424. doi: 10.1111/1365-2656.12749
Stavert, J. R., Bartomeus, I., Beggs, J. R., Gaskett, A. C., and Pattemore, D. E. (2019). Plant species dominance increases pollination complementarity and plant reproductive function. Ecology 100:e02749. doi: 10.1002/ecy.2749
Thébault, E., and Fontaine, C. (2010). Stability of ecological communities and the architecture of mutualistic and trophic networks. Science 329, 853–856. doi: 10.1126/science.1188321
Valiente-Banuet, A., Aizen, M. A., Alcántara, J. M., Arroyo, J., Cocucci, A., Galetti, M., et al. (2015). Beyond species loss: the extinction of ecological interactions in a changing world. Funct. Ecol. 29, 299–307. doi: 10.1111/1365-2435.12356
Weiner, C. N., Werner, M., Linsenmair, K. E., and Bluthgen, N. (2014). Land-use impacts on plant-pollinator networks: interaction strength and specialization predict pollinator declines. Ecology 95, 466–474. doi: 10.1890/13-0436.1
Wu, G.-L., Du, G.-Z., Liu, Z.-H., and Thirgood, S. (2008). Effect of fencing and grazing on a Kobresia-dominated meadow in the Qinghai-Tibetan plateau. Plant Soil 319, 115–126. doi: 10.1007/s11104-008-9854-3
Keywords: pollination network, ecological restoration, the Tibetan Plateau, grazing exclusion, pollination function
Citation: Gao E, Wang Y, Bi C, Kaiser-Bunbury CN and Zhao Z (2021) Restoration of Degraded Alpine Meadows Improves Pollination Network Robustness and Function in the Tibetan Plateau. Front. Ecol. Evol. 9:632961. doi: 10.3389/fevo.2021.632961
Received: 24 November 2020; Accepted: 11 March 2021;
Published: 13 April 2021.
Edited by:
Alice Classen, University of Wuerzburg, GermanyReviewed by:
Edith Villa-Galaviz, Universidad Autónoma de Yucatán, MexicoMaria Florencia Miguel, CONICET Mendoza, Argentina
Copyright © 2021 Gao, Wang, Bi, Kaiser-Bunbury and Zhao. This is an open-access article distributed under the terms of the Creative Commons Attribution License (CC BY). The use, distribution or reproduction in other forums is permitted, provided the original author(s) and the copyright owner(s) are credited and that the original publication in this journal is cited, in accordance with accepted academic practice. No use, distribution or reproduction is permitted which does not comply with these terms.
*Correspondence: Zhigang Zhao, emhhb3poZ0BsenUuZWR1LmNu