- 1Laboratory of Avian Ecology and Evolution, Department of Zoology, Center of Biosciences, Federal University of Pernambuco, Recife, Brazil
- 2Centro de Estudos Superiores de Zé Doca, Universidade Estadual do Maranhão, Zé Doca, Brazil
- 3Aquasis—Association for Research and Preservation of Aquatic Ecosystems, Caucaia, Brazil
The conversion of tropical habitats has dramatic implications on biodiversity and represents one of the greatest conservation challenges of our time. Seasonally Dry Tropical Forests (SDTF), which are disjointly distributed throughout the Neotropics, are especially susceptible to human activities. The Caatinga Dry Forest, located in the semi-arid interior of northeastern Brazil, represents not only the largest and most biologically diverse nucleus of SDTF, but also the world’s most densely populated semi-arid region, with ever-growing pressure on its natural resources. To prevent illegal logging, conservation agencies looked at forest management, where an area is divided in smaller stands which are gradually logged and allowed to regrow for a period of time, when a new cutting cycle should reinitiate. The impacts of these management schemes on biodiversity, however, remain largely untested. We conducted standardized avian surveys to evaluate the effects of forest management on the avian community at a 1,670 ha privately owned property located on the Chapada do Araripe, northeastern Brazil. This area was divided in 22 forest stands, half of which had already been logged at the time of our sampling, creating a gradient of logged and natural forests and an 11-yr chrono-sequence of forest regeneration. Our results show that logged areas present fewer individuals, fewer species, and different avian assemblages than unlogged forests. Such differences are mostly driven by forest-dependent species, which were overwhelmingly affected by forest management. Our results show that although logged forests tend to recover its height after a decade, they do not recover the originally forest cover, measured by the Normalized Difference Vegetation Index. Likewise, decade-long recovering stands continue to show lower species richness, lower bird abundance, and different avian composition than unlogged forests. We identified a set of bird species that are more affected by forest management (ecological losers) and a group of birds that apparently benefit from the referred changes in land use (ecological winners). We conclude that completely managing an entire area may cause the extirpation of several forest-dependent species. We therefore suggest keeping logged and unlogged plots intermingled, to avoid local extinctions and the complete modification of the original avifauna.
Introduction
Halting the conversion of natural habitats into anthropogenic landscapes represents one of the greatest challenges of the twenty-first century. This is particularly true for tropical regions, which concentrate most of the planet’s biodiversity (Laurance, 2007; Blackman et al., 2014). Seasonally Dry Tropical Forests (hereafter SDTF) are patchily distributed throughout the world’s tropics and are especially susceptible to human activities (Pennington et al., 2004). Dry tropical forests are subject to multiple threats, often simultaneously, including habitat fragmentation, fire, wood harvesting, conversion to croplands, and climate change (Miles et al., 2006; Antongiovanni et al., 2020). Despite those pressures, SDTF are often considered resilient habitats, because they tend to recover their original stature faster than wet forests (Vieira and Scariot, 2006). Tree species composition recovery should be straight-forward under minor disturbance regimes (Lebrija-Trejos et al., 2008). However, under heavy use, dry forests tend to recover very slowly (Vieira et al., 2006). Not only seedlings need to establish themselves during a short rainy season, but seedling survival rates are very low (Colón and Lugo, 2006; Lebrija-Trejos et al., 2011). Differently from wet forests, where soft-wooded tree species dominate the early succession period, dry forest early succession conditions can be harsh, and only tolerated by slow-growing hard-wooded species (Poorter et al., 2019).
The Caatinga Dry Forest (hereafter Caatinga), located in the semi-arid interior of northeastern Brazil, represents the largest and most biologically diverse nucleus of SDTF in the Neotropics (Miles et al., 2006). However, despite alarming rates of deforestation—nearly 60% of the Caatinga’s original vegetation cover has already been modified by human activities (Beuchle et al., 2015)—it remains the least protected ecosystem in Brazil, with ∼1% of its area fully preserved by protected areas (Leal et al., 2005; da Silva et al., 2018). Despite presenting very harsh living conditions, with nearly 30 million inhabitants, the Caatinga represents the most densely populated semi-arid region in the world (da Silva et al., 2018). Human Caatinga populations are mostly rural and present some of the lowest human development indices in Brazil (da Silva et al., 2018). This reality presents the Caatinga with multiple pressures, mainly due to livestock production, itinerant agriculture, and particularly wood harvesting to produce firewood and charcoal (Ribeiro et al., 2015; Antongiovanni et al., 2018). Some of these activities, such as subsistence firewood and overgrazing by goats, represent chronic low-intensity activities, which will affect biodiversity in the long term (Singh, 1998; Ribeiro-Neto et al., 2016; Antongiovanni et al., 2020). Other human activities, such as deforestation for charcoal production, mining, or the total conversion of woodlands for croplands and pasturelands, represent acute disturbances, which are rapid and intense, often removing all forest resources from an area, and are known to affect the biota in more dramatic and pervasive ways than chronic activities (Singh, 1998).
As a way to prevent illegal over-exploration of wood resources, governmental and environmental agencies recommend the rational management of these forests (Soares-Filho et al., 2014). Among the managing schemes, there is a practice that consists of subdividing a large area into smaller forest stands and gradually logging-off the timber of each fragment, allowing regrowth and creating a gradient of forest stands in constant regeneration. In general, they propose 25 year-cycles, after which regenerated forest stands undergo a new cutting cycle. Although modern forest management was introduced between the eighteenth and nineteenth centuries, only recently the activity stopped be seen just by an economic view (Samojlik et al., 2016). Despite its widespread use, the impacts of such forest management scheme on biodiversity remain unclear (MacDicken et al., 2015; Darrigo et al., 2016).
Monitoring biodiversity under the impact of human activities is one of the most traditional strategies for wildlife conservation (Magnusson et al., 2018). However, due to the impossibility of monitoring all species, certain groups are considered indicators of environmental quality (Lindbladh et al., 2020). Birds are often used as indicators because they have a well-defined taxonomy, have consistent information about the habitats they occupy, and are relatively easy to survey (Drever et al., 2008; Lindbladh et al., 2020). Changes in habitat structure are often reported to affect bird assemblages, however, not all species are affected equally (Shahabuddin and Kumar, 2006). Direct and indirect effects can influence different groups of birds, especially demanding species that need specific resources or habitats (Shahabuddin and Kumar, 2006; Drever et al., 2008). In fact, the impact of logging and subsequent forest recovery is predicted to vary depending on specific life history traits of the logged tree species (Vinson et al., 2015). Also, it remains to be seen if forest structure recovery results in complete or even partial recovery of animal species, which may also follow species-specific recovery patterns (Shahabuddin and Kumar, 2006).
There are few studies evaluating the effect of forest management in the Neotropics, but most of these studies were conducted in humid forests (Thiollay, 1997; Berry et al., 2008; Hamer et al., 2015; Poudyal et al., 2018; Bousfield et al., 2020). In fact, few have evaluated the effects of either selective or clear-cut logging follow by forest regeneration in SDTFs (Shahabuddin and Kumar, 2006; Maia et al., 2019). In this study, we evaluate the effect of a forest management scheme at a privately owned property located in the semi-arid interior of northeastern Brazil, where a network of trails subdivided the area in 22 different forest stands. One forest stand has been logged every year and let to regrow since 2004. At the time of our surveys, half of the stands had already been logged, creating a recovering gradient of recovering logged forests and forest in natural condition, offering a unique opportunity to evaluate the effect of forest management on avian biodiversity.
We conducted standardized avian surveys (point counts) to understand the effects of forest management on the avifauna. Specifically, we aim to (i) compare avian diversity (species richness and bird abundance) and avian composition between logged and unlogged forests; (ii) evaluate whether this effect depends on the degree of species disturbance sensitivity between forest-dependent and non-dependent species; (iii) evaluate how species composition recovered along a 11-yr regeneration chrono-sequence; and (iv) understand whether avian changes can be predicted by the structure of the vegetation. Prior to our study, we had a series of expectations, which included: (i) a reduction in avian diversity (species richness and bird abundance) and changes in species composition in logged areas; (ii) that most of this changes will be led by forest-dependent bird species; (iii) a slow recovery of avian diversity in regenerating forests; and (iv) a positive relationship between avian diversity and forest structure (forest height and tree cover). This represents one of the first studies to evaluate the sustainability of forest management in the Caatinga and aims to shed light into the effects of a common management practice.
Materials and Methods
Study Area
We conducted this study at the Fazenda Pau D’Arco (7° 20′ S, 39° 34′ W), a privately owned property located ∼26 km southwest of the city of Crato, in the Brazilian state of Ceará (Figure 1). The area, located at ∼860 meters above sea level on top of the Chapada do Araripe (Araripe Plateau), is within an Area of Environmental Protection (a category of the Brazilian protected areas system that allows human occupation and sustainable economic activities). The vegetation cover in this area is represented by a thorny dry forest, locally known as Carrasco (Prado, 2003), a type of deciduous vegetation characteristic of sedimentary plateaus (Giulietti et al., 2004). Carrascos are present on sedimentary soils and show a predominance of sub-arboreal and small tree forms (3–4 m), high density of woody plants and unstratified thin trunks (de Araújo et al., 1999). Precipitation averages ∼1,150 mm/yr, being concentrated between January and April, with a peak in March and the average annual temperature is around 25°C, ranging from 23.8 to 27.4°C (de Araújo et al., 1999; Brito and Silva, 2012).
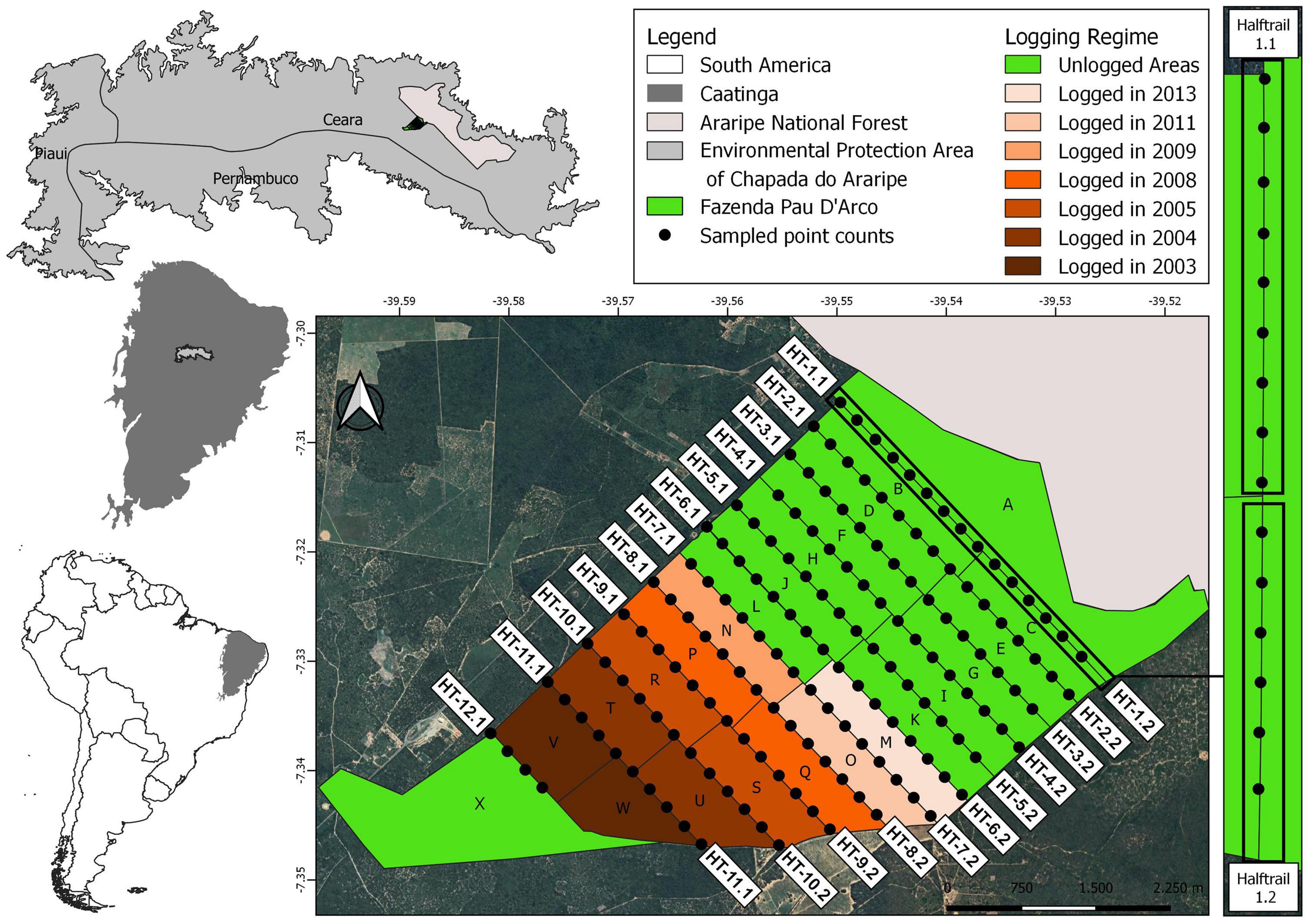
Figure 1. Location of the study area and arrangement of the 160 count points in the forest management area of Fazenda Pau D’Arco, Crato, Ceará, Brazil in 2014. Polygons represent forest stands, green areas indicate unmanaged areas and brown scale indicates different logging times (in years). Trails that divide the forest stands were split into 23 half-trails (with a forest stand on each side) and used as a sample unit.
The area under forest management covers a total of 1,670 ha, which includes 22 forest stands between 69 and 92 ha. Management at the Fazenda is conducted since 2003/2004 (Table 1), and until the end of 2014 (time of our sampling) half of the forest stands had already been logged. Until 2014, forest management had created a 11-yr chrono-sequence of forest recovery, including forest in different stages of regeneration. According to the management plan, forest stands will be clear cut every 25 years (Table 1) without machine assistance, covering the soil with branches and leaves to avoid further soil impoverishment. All forest stands will be logged by 2028, when a new forest logging cycle should be reinitiated (Table 1). According to the Brazilian Forest Code an additional area, known as Legal Reserve, needs to be set aside and preserved (Soares-Filho et al., 2014). Two such areas have been established at our study site, resulting in 24 management plots (Figure 1). One of these legal reserves (forest stand X), however, was established in what seems to be a severely modified second-growth area, and probably does not represent a proper control area, as originally envisioned by the law.
Sampling Design and Data Classification
We accessed the entire area using the 12 roads opened for logging, which divided the area in 22 forest stands (besides the legal reserves). These roads vary from 1.3 to 4.0 km (Figure 1). We established a network of point counts along these roads, systematically distributed every 250 m, a distance that we considered safe to maintain surveys independent from one another (Figure 1). We conducted avian surveys in 160 points, all of which were sampled during the dry season of 2014. Most of the point counts (138) were sampled between 15 and 25 October and the remaining points (22) (trails 2 and 6, see Figure 1) between 17 and 19 December. All point counts were conducted by the same experienced observers (JRR and FMCG) throughout the study. Given the temporal proximity of the two sample periods, we pooled all samples for analyses.
Five-minute point counts were conducted between 5:00 and 8:30 a.m., the known peak activity for birds at the site. During this period, all birds heard and observed were noted and identified. We opted for using unlimited distance for the records, but the overwhelming majority of records were likely within a 100 m radius, and given the large size of the forest stands, within the area of a given unit. The location of each observation or sound heard was mapped into a Cartesian coordinate system, which included the side of the road. Recorded individuals were visually and acoustically monitored during the censuses, to avoid double counting individuals. The species recorded at these points were classified according to their degree of forest dependence adapted from Silva and co-authors (da Silva et al., 2003). This classification includes: (1) species that rely on, and are tightly associated to, forested areas (forest-dependent species); (2) species that are often found in forests, but also occupy open areas or species that do not rely on forested areas (forest non-dependent species). We also classified species by endemism, conservation and migratory status. Species classification and nomenclature follow the Brazilian Committee of Ornithological Records (de Piacentini et al., 2015), and recent taxonomic and nomenclature changes (Bravo et al., 2021).
We obtained two types of vegetation data, including (1) a remote measurement, namely the Normalized Difference Vegetation Index (hereafter NDVI) and (2) vegetation height data. The NDVI represents a remote sensing measurement used as an indicator of the biophysical properties of the vegetation (Tucker and Sellers, 1986). We obtained these data through the Land Processes Distributed Active Archive Center (LP DAAC) provided by NASA’s Earth Science Data and Information System (ESDIS). These data were collected from a grid with 217 points equidistant for 350 m throughout our study area. Each forest stand included between 5 and 9 NDVI points and the much larger legal reserve A, included 29 points. We extracted these data matching the exact sampling period and obtained the average NDVI for each forest stand. The NDVI data was downloaded and processed using the R package MODISTools (Tuck and Phillips, 2017). We obtained tree height measurements in the field for 160 point counts. Each height estimate was calculated as the mean of three independent measurements obtained at the exact point of our avian surveys, and two measurements obtained 50 meters apart along the road. Forest height was measured using a distance meter, from the base to the highest branch in the perpendicular vegetation to the measurement site. To calculate the average height of each forest stand, we used all measurements along its perimeter, which ranged from 6 to 16 independent measurements.
Data Analysis
Except for species accumulation curves with Hill numbers, we considered the half-trails as our sample units throughout the analyses (Figure 1). These samples were classified as unlogged, transition, and logged half-trails according to the logging status of forest stands on both sides. To calculate bird abundance per sample, we merged individuals detected during point counts conducted along the half-trails. In all, 23 half-trails were analyzed (half-trail X-W was not sampled), except for the analyses involving logging time, as only 9 half-trails had already been logged on both sides at the time of our sampling. Counting species to evaluate species richness may be a biased metric due to differences in (i) the numbers of samples and (ii) the numbers of individuals detected. Even under similar standardize sampling procedures, variation in the number of individuals detected may influence the number of species observed (Gotelli and Chao, 2013). Thus, we extracted the effective number of species interpolating the species richness by the number of individuals from the Hill numbers parameterized by an order q = 0 (Chao et al., 2020). By doing this, we controlled for the bias caused by the differential sample effort, and include the estimation of unobserved species in the species richness comparisons (Gotelli and Chao, 2013; Chao et al., 2020).
Sampling effort was evaluated using a species accumulation curve, including the rarefaction and extrapolation with Hill numbers through all the samples (Gotelli et al., 2014). Additionally, we compare the observed general curve between unlogged, transition and logged curves. We compared average effective number of species (hereafter effective richness) and the number of individuals between unlogged, transition and logged areas using two-way ANOVA when data were homoscedastic, and three individual Kruskal-Wallis rank tests when data were heteroscedastic. These tests were conducted for the entire avian community, and according to species dependence on forests (dependent or non-dependent). We ordinated the avian community at each half-trail using two dimensions (k = 2) in a Non-Metric Multidimensional Scaling (NMDS), using the Bray-Curtis’s dissimilarity index to access avian species composition. To determine the degree of similarity of composition among samples and clustering of unlogged, transition and logged groups in the NMDS, we used an Analysis of Similarity (ANOSIM). Additionally, we used the NMDS scores (k = 1) to ordinate the community along the management chrono-sequence to evaluate possible composition recovery patterns.
We evaluated potential ecological winners and losers through a meta-analytic assessment for all community and for species grouped by forest dependence. We calculated the standardized mean difference (SMD) for each species and estimated the fixed effect models and confidence intervals for both all pooled species and species pooled by category of forest dependence, graphically depicted as a forest plot. We removed species with less than 5 records in the whole area and the transition records from this analysis. To evaluate the degree of habitat changes after management in the forest stands, we performed linear regressions relating vegetation height, the NDVI and the time since logging. To access the logging age for each sample, which had different “time since logging” on each side, we used the average age of these sides. Finally, we applied linear regressions to test the effects of forest management on the avifauna relating (i) vegetation height, (ii) NDVI, and (iii) time since logging with the number of individuals, the effective richness, and species composition. Prior to analyses, we tested collinearity between variables through Pearson’s correlation tests and tested all model assumptions. No data transformation was required. All analyses were conducted using R (R Core Team, 2019) implemented in RStudio.
Results
Avian Community Structure
During our point counts, we detected 3,789 individuals of 90 bird species, of 34 different avian families, which represents more than 60% of all bird species ever recorded at the Fazenda Pau D’Arco, and virtually all non-occasional residents (de Lima et al., 2021). Nearly a third of the species detected (24 species) were considered forest-dependent, whereas 2/3 (66 species) were forest non-dependent species (Supplementary Table 1). Avian assemblages recorded on our point counts were overwhelmingly composed of passerines, which accounted for 73% of the species and 93% of the individuals. Some avian families dominated these assemblages, including flycatchers (Tyrannidae, 18 spp.), antbirds (Thamnophilidae, 8 spp.), tanagers (Thraupidae, 8 spp.), and furnariids (Furnariidae, 6 spp.).
Effects of Forest Management on the Avifauna
Overall, we detected more species and more individuals in areas that had not been logged (79 spp. and 2,093 ind.) than both in transition (50 spp. and 512 ind.) and logged ones (56 spp. and 1184 ind.), an observation that held even when accounting for sampling effort, as observed by comparing species accumulation curves between areas (Supplementary Figure 1). Species accumulation curves also provide evidence that the expected species effective richness in unlogged areas contributes with most of the area’s species diversity, which is not the case of both transition and logged areas (Supplementary Figure 1).
We detected, on average, 26.9 ± 2.8 effective species and 190.3 ± 45.4 individuals per half-trail in unlogged areas, 24.7 ± 3.0 effective species and 170.7 ± 65.8 individuals in transition areas, and 24.2 ± 2.8 effective species and 131.6 ± 37.9 individuals per half-trail in logged areas. Fewer individuals were observed in logged area [Two-way ANOVA for individuals; F(1, 40) = 3.95, p < 0.05; Figure 2]. The difference between unlogged and logged areas was driven by forest dependent species, which as expected, presented more individuals [Tukey’s (HSD) post hoc test for forest dependent individuals p < 0.05] and also higher effective richness [Kruskal-Wallis for effective richness—dependent species; KW(1, 2) = 16.1, p < 0.01] in unlogged areas (Figure 2). This was not the case for forest non-dependent species, as we found no significant difference in the number of species detected in logged and unlogged areas [Kruskal-Wallis for effective richness—non-dependent species; KW(1, 2) = 0.4, p = 0.8]. Nor for the number of individuals [Tukey’s (HSD) post hoc test for forest dependent species p = 0.95; Figure 2].
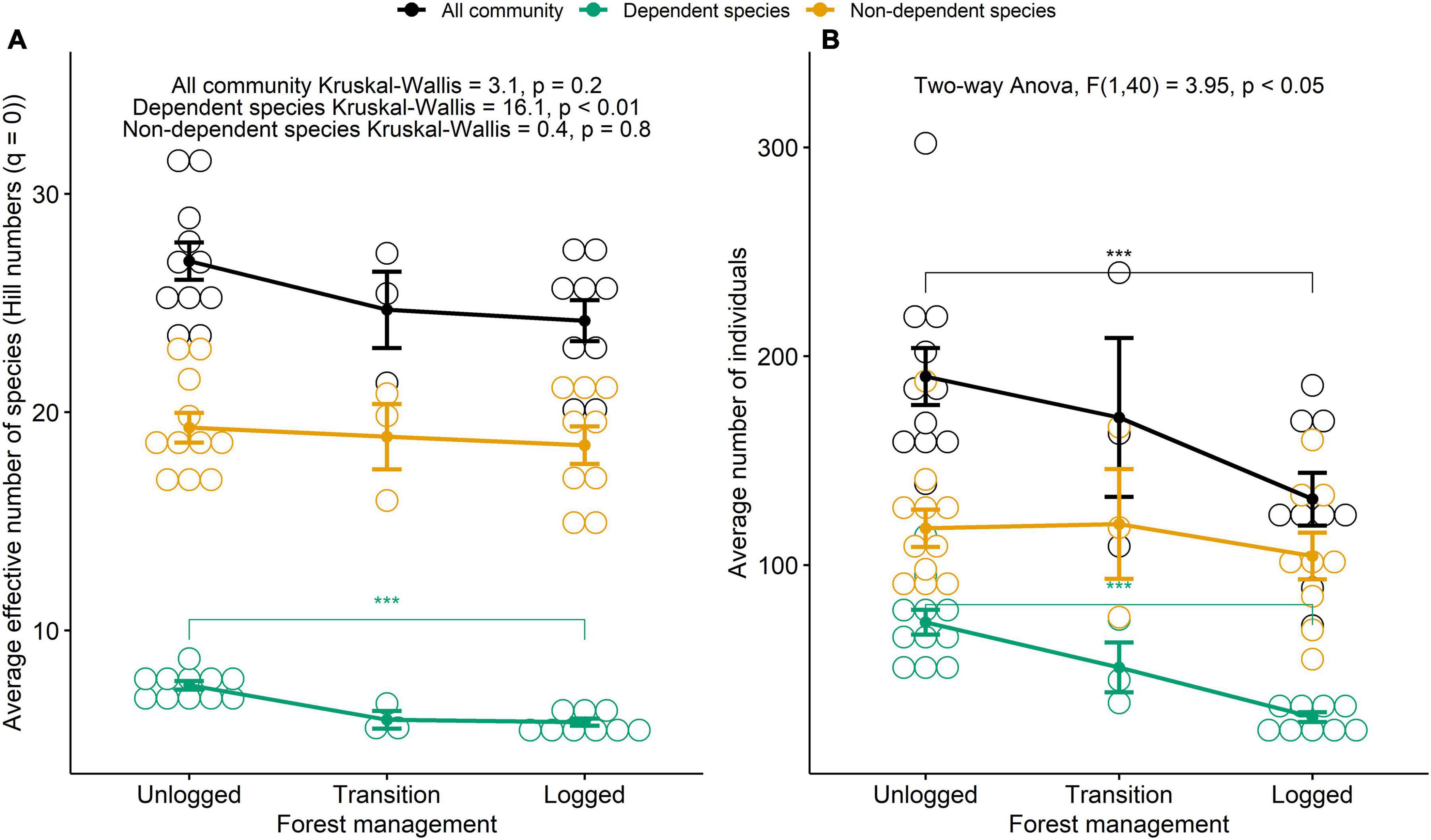
Figure 2. Scatter interval plot with average avian effective number of species (A) and average number of bird individuals (B) recorded in unlogged, transition and logged forest stands at the Fazenda Pau D’Arco, Crato, Ceará, Brazil, for all species (black), forest non-dependent species (yellow), and forest dependent species (dark green). At the top, the used statistical tests. Three Kruskal–Wallis tests for the effective number of species and a two-way ANOVA for the number of individuals. Asterisks show statistical significance according to Dunn’s post hoc test for KW and Tukey’s (HSD) post hoc test for two-way ANOVA.
Avian assemblages were distinct in unlogged and logged areas (ANOSIM; R = 0.54, p = 0.001). Species composition of unlogged and logged sites were in general more similar to areas under similar managing schemes (Figure 3). Transition areas, as expected, appeared between the two distinct groups (Figure 3), indicating a bird composition gradient. We also found that species composition (measured by NMDS scores ordinated in a single dimension) in logged regenerated areas remained distinct from unlogged areas, even after over a decade of forest recovery (Supplementary Figure 2).
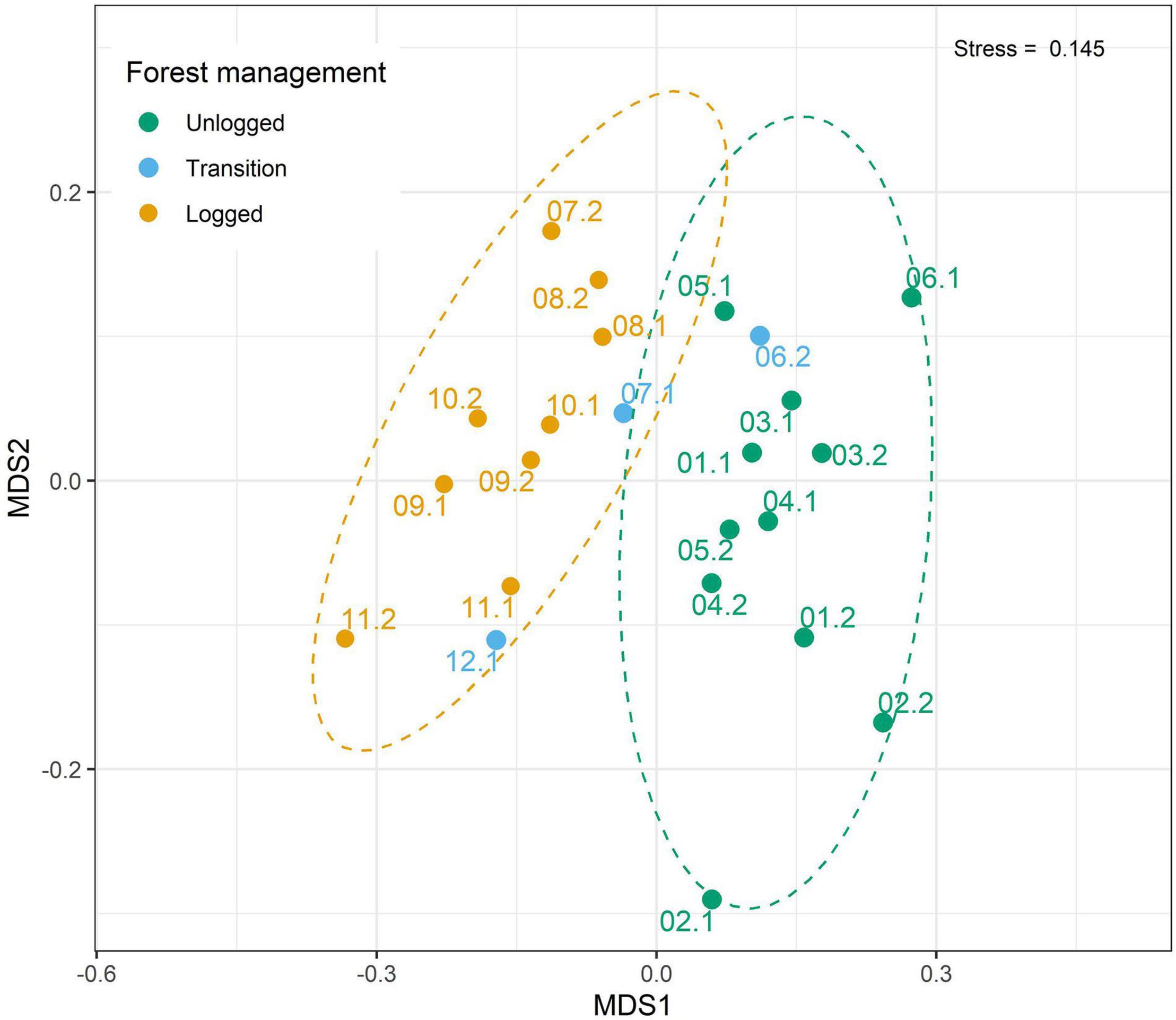
Figure 3. Non-metric multidimensional scaling (NMDS) for bird species (quantitative data) in three groups of unlogged, transition and logged areas collected in 160 point counts of 23 half-trails of the management area at Fazenda Pau D’Arco, Crato, Ceará, Brazil. Color dots indicate unlogged (dark green), transition (blue), and logged (yellow) half-trails.
Our analyses identified species-specific responses to forest management in nearly half of the species analyzed (those with adequate sampling). We identified 21 species with lower than average records in logged areas, including 11 species that presented no records in logged forest stands at all (Figure 4). We found that whereas many forest-dependent species occur exclusively in unlogged forests, only one non-dependent species was found exclusively in logged forests. However, five species were recorded more often in logged areas, suggesting that these species may indeed benefit and even thrive in managed forests (Figure 4 and Supplementary Figure 3).
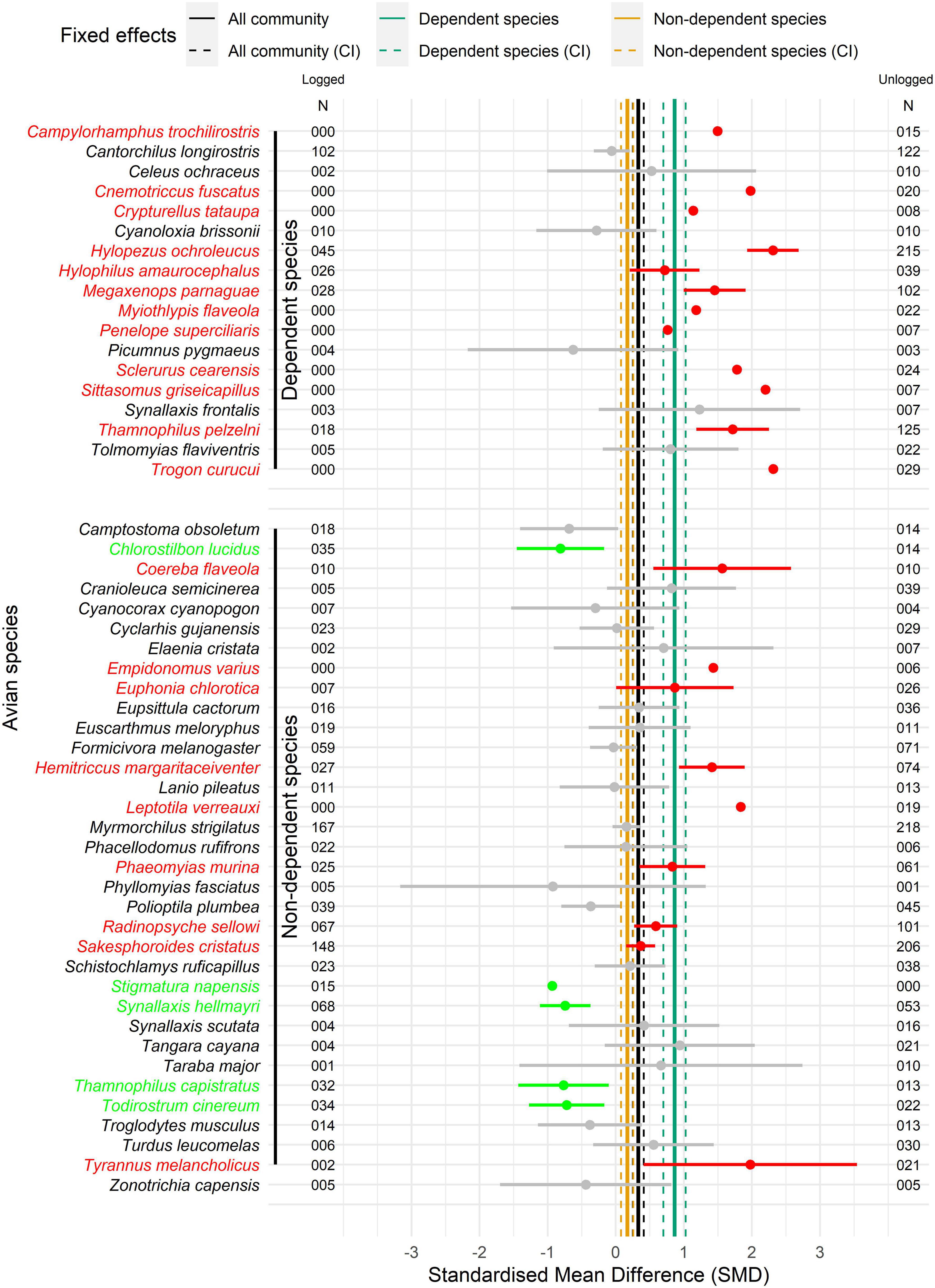
Figure 4. Forest plot of bird species for standardized mean difference (SMD) in number of individuals (N) between logged and unlogged forest stands (excluding transition stands), and respective standard deviations. Were analyzed only bird species with more than five individuals recorded in 23 half-trails of the management area at the Fazenda Pau D’Arco, Crato, Ceará, Brazil. Vertical solid lines represent the fixed effects models from all community, from dependent species and non-dependent species, and vertical dashed lines indicated respective confidence intervals (CI). Red names and point ranges indicated species statistically more abundant in unlogged forest stands and green names and point ranges represents species more abundant at logged stands. Species points without ranges are recorded only in one group of forest stands and the standard deviations are zero.
Evaluating the list of the 20 most commonly detected species in our point counts, we found that most species appeared as most abundant in areas under all managing schemes (unlogged, transition and logged forests), suggesting that abundant species may be less susceptible to changes in land use. On the other hand, whereas four species (H. ochroleucus, T. pelzelni, M. parnaguae, and H. margaritaceiventer) presented proportionally fewer individuals in transition and logged areas, three species (F. melanogaster, S. hellmayri, and P. plumbea) increased their abundance in logged areas (Table 2).
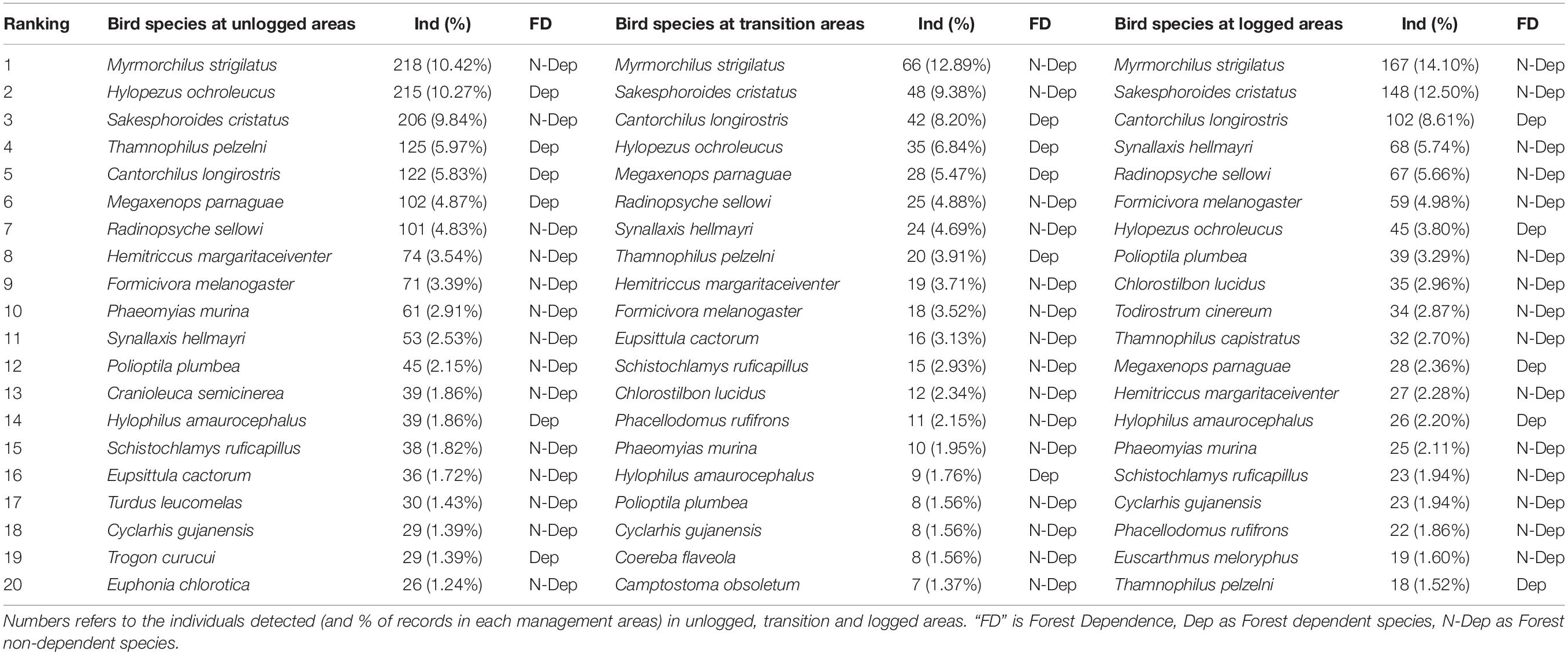
Table 2. The 20 most detected bird species in 160 point counts conducted in 23 half-trails of the management area at Fazenda Pau D’Arco, Crato, Ceará, Brazil.
We found a positive correlation between NDVI and vegetation height. In general, taller forest stands also presented higher index values (Supplementary Figure 4A). Interestingly, these two vegetation variables behaved differently along the regeneration chrono-sequence. Forest height was significantly correlated to forest age (time since logging), as older forests stands were taller than recently logged forests (Supplementary Figure 4B). On the other hand, NDVI was not correlated to forest age and held a very low explanatory power (Supplementary Figure 4C). We interpret this as a mismatch between forest height and forest cover (NDVI) in recovering forests, suggesting that whereas forest may grow quickly, their forest cover may not recover at the same pace.
Avian species composition was predicted by both forest height and NDVI, with relatively high explanatory power (Figures 5C,F). The same occurred to the average number of individuals detected, although with a lower explanatory power (Figures 5A,D). On the other hand, the average species richness was only correlated with forest height, albeit with a low explanatory power (Figure 5E), but not to NDVI (Figure 5B). This means that taller and greener forests sustain more individuals and a different avian assemblage than less green and shorter forests, whereas species richness only responds to the height of the forest and not to the forest cover. Quite surprisingly, we did not detect positive correlations between any of the avian variables measured and forest regeneration time (time since logging) (Figures 5G–I). In fact, the only significant correlation found was between the average number of individuals and regeneration time, but this was a negative correlation. In other words, we found less individuals in older regenerating forest stands (Figure 5G).
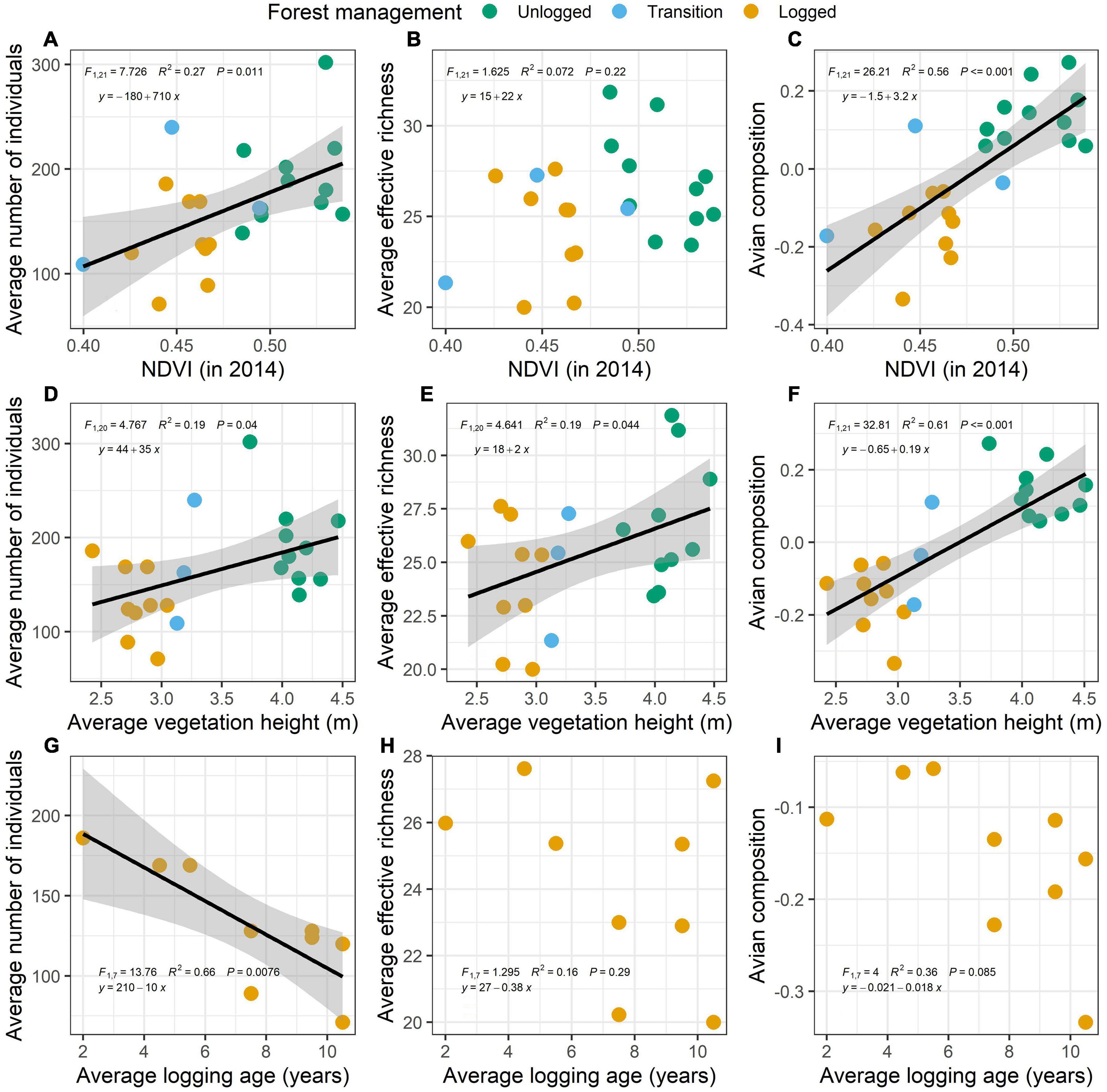
Figure 5. Linear regressions models between avian abundance (A,D,G), species richness (B,E,H), and avian composition (C,F,I) obtained from 160 point counts and averaged by 23 (or 9 as logging age) half-trails sampled and the normalized difference vegetation index (NDVI in 2014) (A–C), vegetation height (D–F), and the logging age (in years prior to 2014, the time of our sampling) (G–I), averaged from the two forest stands aside of each 23 (or 9 as logging age) half-trails of the management area at Fazenda Pau D’Arco, Crato, Ceará, Brazil. Dots indicate unlogged (dark green), transition (blue), and logged (yellow) half-trails. The regression line was derived from the data points and smooth areas are the standard deviation for significant regression models.
Discussion
As far as we are aware, this study represents the first attempt to evaluate the effect of forest management on the Caatinga avifauna, and one of the first ones in any Neotropical dry forest (Coria et al., 2015; Hilje et al., 2020; Owen et al., 2020). This kind of studies are particularly relevant today, given the widespread decline in natural habitats and the urgent need of more sustainable economic activities (Sanchez-Azofeifa et al., 2005; da Silva et al., 2018). Understanding the effects of current management schemes on biodiversity should be a primary goal of conservation biology and a top priority among governments, land managers, and policy makers. Furthermore, understanding the limitations of different managing strategies is essential to achieve a more sustainable use of ever-shrinking natural landscapes.
Although we do not have data after a complete 25-yr regeneration cycle, our study provides evidence of the potential effect of forest management on the avifauna, including the following four take-home messages. First, forest management as currently conducted at our study site (clear-cut logging followed by passive regeneration) affects the abundance, species richness and composition of the avifauna. In general, we found less individuals, less species, and a different avian composition in forest stands that have been logged. Second, we show that this effect depends on the degree of disturbance sensitivity of each species. Forest-dependent species where overwhelmingly affected by forest management, whereas forest non-dependent species were less affected, or not affected at all. Third, even after 11 years of forest recovery, bird species composition in logged areas had not yet reached the diversity level observed in unlogged areas. In fact, local species assemblages seem to have taken a different regeneration path and remained distinct from the original avifauna found in unlogged areas. We believe that this result can be explain by the fact that regenerating forests do not recover its total height or original vegetation cover after a decade, suggesting that tree species composition may be quite distinct from that found in unlogged forests.
Detecting changes in the number of species and individuals in natural environments due to human interference often represents the first findings in management studies (Chaudhary et al., 2016). Although providing evidence of changes in species composition is often more challenging, it offers important insights about species losses and replacements (Demarais et al., 2017). We detected changes in three important aspects of the avian communities, finding fewer species, fewer individuals, and different species assemblages in logged forest stands. The differences we found at the Fazenda Pau D’Arco were driven mostly by forest dependent species, which represented a third of all species detected. Forest-dependent species are often the most impacted by human activities because they require stable and complex habitats (Barrantes et al., 2016). Although most forest non-dependent species were unaffected by forest management, some of these species were negatively affected or even replaced by less demanding species after management.
This response pattern creates a scenario with ecological losers and winners (Tabarelli et al., 2012). In fact, we revealed that about one fourth of the species recorded in our point counts had fewer individuals in logged forests, whereas half of these species had zero records in forests that had been logged. According to our data, only five species presented more individuals in logged forests, suggesting that the list of “winners” is rather small. Among the ecological “losers” there are two species of global conservation concern (IUCN, 2020) the Vulnerable Ceará Leaftosser (Sclerurus cearensis) and the Near Threatened White-browed Antpitta (Hylopezus ochroleucus). Whereas the Antpitta was almost five times less abundant in logged forests, the Leaftosser was absent altogether. Similarly, we found ten species with no records whatsoever in logged forests, suggesting that they may be extirpated from the study area after all forest stands are logged by 2028. In general, disturbed environments are known to become less heterogeneous, and more specialized species tend to lose their habitats, being sometimes replaced by more generalist species (Devictor et al., 2008; Dong et al., 2020). Consequently, the identification, protection, and management of key habitats are crucial to afford the requirements of specialized species (Brambilla et al., 2020).
By applying the principle of space-for-time-substitution, where it is possible to infer a temporal trend from a study of different aged sites (Pickett, 1989), we were able to infer changes without involving a research that would take two decades to be concluded. One of the main limitations of the space-for-time approach is that areas under study may be heterogeneous or may have suffered from different histories of land use (Foster and Tilman, 2000). The Fazenda Pau D’Arco represents a rather homogeneous patch of dry forest vegetation, with virtually no differences in altitude or climatic regimes throughout its area (de Lima et al., 2021). Our results from the chrono-sequence studied, including an 11-yr regeneration period, are somewhat concerning. Avian composition, species richness, and abundance along the chrono-sequence differed from unlogged areas, even after more than a decade of forest recovery. Surprisingly, bird abundance decreased in older regenerating forest stands, compared to more recently logged forests.
These results are likely related to known patterns of tree regeneration in Neotropical dry forests. Differently from humid forests, ecological succession in dry forests is slower and pushed by high wood-density species, which are those than can cope better with low water availability and higher solar radiation (Poorter et al., 2019). It is only then that the understory becomes less hot and dry, allowing the establishment of other plant species of more rapid growth (Poorter et al., 2019). Therefore, the dynamics of recovery may vary depending on land use (Arroyo-Rodríguez et al., 2017), creating a difficulty for landscapes that need to fully recover from a clear-cut activity, as is the case at our study site. In addition to land use, the recovery of dry forests also depends on the availability of water, being more effective during the rainy season (Lebrija-Trejos et al., 2011). Besides the natural climatic conditions of the region, the limited availability of water may hamper the recovery capacity of these forests (Álvarez-Yépiz et al., 2018). In fragmented and disturbed landscapes, active methods of restoration can be more effective (Morrison and Lindell, 2011). However, passive forest restoration is more often used because is less expensive, especially when adjacent vegetation cover is present (Morrison and Lindell, 2011).
Vegetation height and forest cover are factors that can influence the richness, abundance, and composition of bird species in dry forests (Martensen et al., 2012). We did find a positive association between these three aspects of avian assemblages and both forest height and a measure of vegetation canopy greenness (NDVI), indicating the importance of assessing forest structure to understand avian responses. Human disturbance frequently reduces the complexity of the forest and affects habitat availability, followed by changes in biodiversity (Barlow et al., 2016). A third vital piece of information is related to forest composition, which we lack for our study area. Which tree species are being recovered? How tree species composition affects the structure of the vegetation? How interrelated are bird and plant species? These are questions that require immediate answers and would be key to understand habitat regeneration after clear-cut logging. Our data indicates that recovering forest tend to reach around 2.8 m within 7.5 years, but do not reach the average height of ∼4 m of unlogged forests even after a decade after logging. They also indicate that a decade may not be enough to recover the original vegetation cover.
We failed to find a positive trend between time since logging and avian regeneration. This mismatch between older regenerating stands and the avifauna may indicate that the original plant and animal composition may not be coming back in the short term, a topic that requires immediate attention. Despite the potential negative effects of habitat management on the avifauna, secondary forests still represent valuable habitats for many tropical species (Chazdon et al., 2009; Edwards et al., 2017; Sayer et al., 2017). Data on Neotropical dry forests are still incipient, and there is an ongoing debate on how birds respond to forest regeneration (Latta et al., 2018; Owen et al., 2020). Recent studies, however, suggest that secondary forests can be useful to many species, and even conserve relatively high values of avian phylogenetic diversity (Edwards et al., 2017). Furthermore, secondary forests may also be key to support the provision of ecosystem services, although these services may be less stable than in primary forests (Sayer et al., 2017). Unlike humid forests, which have been studied over the past 70 years, Neotropical dry forests have only become a focus of research in the past three decades (Stoner and Sánchez-Azofeifa, 2009).
This information is particularly important for the development of management protocols (Espírito-Santo et al., 2009). Multiple factors can influence the effectiveness and sustainability of forest management practices, including (i) local climatic conditions; (ii) the proximity and conservation of preserved areas; and (iii) natural regeneration time, which need to be compatible with ecological succession. There is evidence that forest management, like other land-sparing approaches (such as high intense timber extraction with the protection of natural reserves), is capable of sustaining higher bird abundance and species richness and maintaining higher functional diversity, than land-sharing approaches (such as moderate land use integrated with wildlife-friendly habitats across a concession) (Edwards et al., 2014; Cannon et al., 2019). Nevertheless, the environmental context and scale also seems to be an important function for biodiversity maintenance (Ekroos et al., 2016). The persistence of biodiversity is strongly linked to the proximity of surrounding contiguous natural habitats (Gilroy et al., 2014), highlighting the value of intermingling unlogged lands for sustainable forest management and to enhance forest restoration (Watson et al., 2018).
We conclude that the complete clear-cut logging and subsequent conversion of the study area into secondary forest will likely drive to the extirpation of several bird species, particularly those that depend on healthy forests to thrive. On the other hand, our results suggest that many of these forest-dependent species may manage to live in relatively smaller forest plots and could potentially recover their populations. Therefore, we strongly encourage the use of protected forest patches intermingled with logged areas, as a way to ensure the survival of all species in these logged forests. In fact, the kind of forest management applied at our study site may be sustainable for some species, but not for others. Despite the undeniable value of secondary forests for the maintenance of generalist species, we found a large number of ecological losers that seem to be less abundant in logged forests or even incapable of occupying regenerating stands, at least in the time frame studied. Having completed nearly half of the logging cycle (11 years), regenerating forests do not seem adequate to provide habitat requirements for more demanding bird species. We strongly argue for the continuous monitoring of regenerating forests, both for birds and plants, which appears to be essential in determining whether older forest stands will be able to meet the fauna needs.
Data Availability Statement
The original contributions presented in the study are included in the article/Supplementary Material, further inquiries can be directed to the corresponding author/s.
Ethics Statement
The animal study was reviewed and approved by the Instituto Chico Mendes de Conservação da Biodiversidade—ICMBio.
Author Contributions
JR, LN, and WS contributed to the research design. JR, HL, LN, and FL-C collected the data in the field. JR analyzed the data. JR and LN wrote the manuscript. All authors contributed to the article and approved the submitted version.
Funding
American Bird Conservancy funded a substantial part of this research. We are grateful to Dr. Pedro Develey of the Society for the Conservation of Birds in Brazil (SAVE Brazil) and Bennett Hennessey, Coordinator of the Brazilian Conservation Program of the American Bird Conservancy for support in this project. Additional funding for this research was granted to LN by the Fundação de Amparo a Ciência e Tecnologia de Pernambuco FACEPE (APQ- 0337-2.04/15) and the Brazilian Council for Research (CNPq, 432630/2016-3). FL-C was funded by a CNPq/FACEPE post-doctoral grant (DCR-0018-2 05/15). HL was funded by a CNPQ technical support grant (Proc. no 372272/2018-5) and is currently being funded by a CAPES/PROEX doctoral grant (PROEX Regulation—Public HEIs 88887.373219/2019-00). JR was funded by a CAPES/CNPQ MSc. grant (130684/2016-3) and by grants provided by CNPq of technical support (372602/2019-3).
Conflict of Interest
The authors declare that the research was conducted in the absence of any commercial or financial relationships that could be construed as a potential conflict of interest.
Acknowledgments
We thank the Graduate Program on Animal Biology (PPGBA) and the Federal University of Pernambuco (UFPE) for the support during this project. We are also grateful to Instituto Chico Mendes de Conservação da Biodiversidade—ICMBio and personally to Paulo Maier, Flávia Domingos, and Agnaldo for supporting and encouraging our studies at the Chapada do Araripe. We thank Stephenson Ramalho for allowing us to work at the Fazenda Pau D’Arco. We thank Inara Roberta Leal and Enrico Bernard for helping comments during early stages of this project. We thank laboratory colleagues for their support during fieldwork.
Supplementary Material
The Supplementary Material for this article can be found online at: https://www.frontiersin.org/articles/10.3389/fevo.2021.631247/full#supplementary-material
References
Álvarez-Yépiz, J. C., Martínez-Yrízar, A., and Fredericksen, T. S. (2018). Special Issue: resilience of tropical dry forests to extreme disturbance events. Forest Ecol. Manage. 426, 1–6. doi: 10.1016/j.foreco.2018.05.067
Antongiovanni, M., Venticinque, E. M., and Fonseca, C. R. (2018). Fragmentation patterns of the Caatinga drylands. Landsc. Ecol. 33, 1353–1367. doi: 10.1007/s10980-018-0672-6
Antongiovanni, M., Venticinque, E. M., Matsumoto, M., and Fonseca, C. R. (2020). Chronic anthropogenic disturbance on Caatinga dry forest fragments. J. Appl. Ecol. 57, 2064–2074. doi: 10.1111/1365-2664.13686
Arroyo-Rodríguez, V., Melo, F. P. L., Martínez-Ramos, M., Bongers, F., Chazdon, R. L., Meave, J. A., et al. (2017). Multiple successional pathways in human-modified tropical landscapes: new insights from forest succession, forest fragmentation and landscape ecology research. Biol. Rev. 92, 326–340. doi: 10.1111/brv.12231
Barlow, J., Lennox, G. D., Ferreira, J., Berenguer, E., Lees, A. C., Nally, R. M., et al. (2016). Anthropogenic disturbance in tropical forests can double biodiversity loss from deforestation. Nature 535, 144–147. doi: 10.1038/nature18326
Barrantes, G., Ocampo, D., Ramírez-Fernández, J. D., and Fuchs, E. J. (2016). Effect of fragmentation on the Costa Rican dry forest avifauna. PeerJ 4:e2422. doi: 10.7717/peerj.2422
Berry, N. J., Phillips, O. L., Ong, R. C., and Hamer, K. C. (2008). Impacts of selective logging on tree diversity across a rainforest landscape: the importance of spatial scale. Landsc. Ecol. 23, 915–929. doi: 10.1007/s10980-008-9248-1
Beuchle, R., Grecchi, R. C., Shimabukuro, Y. E., Seliger, R., Eva, H. D., Sano, E., et al. (2015). Land cover changes in the Brazilian Cerrado and Caatinga biomes from 1990 to 2010 based on a systematic remote sensing sampling approach. Appl. Geogr. 58, 116–127. doi: 10.1016/j.apgeog.2015.01.017
Blackman, A., Epanchin-Niell, R., Siikamäki, J., and Velez-Lopez, D. (2014). “Biodiversity conservation in Latin America and the Caribbean,” in Enviroment for Development, eds T. Sterner and G. Kohlin (New York, USA: RFF Press), 1–170. doi: 10.4324/9781315848433
Bousfield, C. G., Cerullo, G. R., Massam, M. R., and Edwards, D. P. (2020). Protecting Environmental And Socio-Economic Values Of Selectively Logged Tropical Forests In The Anthropocene. 1st ed. Netherlands: Elsevier Ltd, doi: 10.1016/bs.aecr.2020.01.006
Brambilla, M., Rizzolli, F., Franzoi, A., Caldonazzi, M., Zanghellini, S., and Pedrini, P. (2020). A network of small protected areas favoured generalist but not specialized wetland birds in a 30-year period. Biol. Conserv. 248:108699. doi: 10.1016/j.biocon.2020.108699
Bravo, G. A., Whitney, B. M., Belmonte-Lopes, R., Bornschein, M. R., Aristizábal, N., Beco, R., et al. (2021). Phylogenomic analyses reveal non-monophyly of the antbird genera Herpsilochmus and Sakesphorus (Thamnophilidae), with description of a new genus for Herpsilochmus sellowi. Ornithology 138, 1–16. doi: 10.1093/ornithology/ukab025
Brito, D. S., and Silva, J. M. O. (2012). Análise hidroclimática da microbacia do Rio Granjeiro/Crato-Ceará. Rev. Geo. 1, 359–369.
Cannon, P. G., Gilroy, J. J., Tobias, J. A., Anderson, A., Haugaasen, T., and Edwards, D. P. (2019). Land-sparing agriculture sustains higher levels of avian functional diversity than land sharing. Glob. Chang. Biol. 25, 1576–1590. doi: 10.1111/gcb.14601
Chao, A., Kubota, Y., Zelenı, D., Chiu, C. H., Li, C. F., Kusumoto, B., et al. (2020). Quantifying sample completeness and comparing diversities among assemblages. Ecol. Res. 35, 292–314. doi: 10.1111/1440-1703.12102
Chaudhary, A., Burivalova, Z., Koh, L. P., and Hellweg, S. (2016). Impact of forest management on species richness: global meta-analysis and economic trade-offs. Sci. Rep. 6:23954. doi: 10.1038/srep23954
Chazdon, R. L., Peres, C. A., Dent, D., Sheil, D., Lugo, A. E., Lamb, D., et al. (2009). The potential for species conservation in tropical secondary forests. Conserv. Biol. 23, 1406–1417. doi: 10.1111/j.1523-1739.2009.01338.x
Colón, S. M., and Lugo, A. E. (2006). Recovery of a subtropical dry forest after abandonment of different land uses. Biotropica 38, 354–364. doi: 10.1111/j.1744-7429.2006.00159.x
Coria, R. D., Coria, O. R., and Kunst, C. R. (2015). Influencia del rolado selectivo de baja intensidad (RBI) sobre las comunidades de aves de bosques del Chaco Occidental. Ecol. Aust. 25, 158–171. doi: 10.25260/ea.15.25.3.0.236
da Silva, J. M. C., Barbosa, L. C. F., Leal, I. R., and Tabarelli, M. (2018). “The Caatinga: Understanding the Challenges,” in Caatinga: The Largest Tropical Dry Forest Region in South America, eds J. M. C. da Silva, I. R. Leal, and M. Tabarelli (Cham, Switzerland: Springer International Publishing), 3–19.
da Silva, J. M. C., de Souza, M. A., Bieber, A. G. D., and Caio, J. C. (2003). “Aves da Caatinga: Status, uso do habitat e sensitividade,” in Ecologia e conservação da Caatinga, eds I. R. Leal, M. Tabarelli, and J. M. C. da Silva (Recife: Editora Universitária da UFPE), 237–273.
Darrigo, M. R., Venticinque, E. M., and dos Santos, F. A. M. (2016). Effects of reduced impact logging on the forest regeneration in the central Amazonia. Forest Ecol. Manage. 360, 52–59. doi: 10.1016/j.foreco.2015.10.012
de Araújo, F. S., Martins, F. R., and Shepherd, G. J. (1999). Variações estruturais e florísticas do carrasco no planalto da Ibiapaba, estado do Ceará. Rev. Bras. Biol. 59, 663–678. doi: 10.1590/S0034-71081999000400015
de Lima, H. S., Las-Casas, F. M. G., Ribeiro, J. R., Girão, W. A., Mariz, D., and Naka, L. N. (2021). Avifauna and biogeographical affinities of a carrasco -dominated landscape in north-eastern Brazil: providing baseline data for future monitoring. Bird Conserv. Int. 2021, 1–17. doi: 10.1017/S0959270921000101
de Piacentini, V. Q., Aleixo, A., Agne, C. E., Maurício, G. N., Pacheco, J. F., et al. (2015). Annotated checklist of the birds of Brazil by the Brazilian Ornithological Records Committee. Rev. Bras. Ornitol. 23, 91–298.
Demarais, S., Verschuyl, J. P., Roloff, G. J., Miller, D. A., and Wigley, T. B. (2017). Tamm review: terrestrial vertebrate biodiversity and intensive forest management in the U.S. Forest Ecol. Manage. 385, 308–330. doi: 10.1016/j.foreco.2016.10.006
Devictor, V., Julliard, R., and Jiguet, F. (2008). Distribution of specialist and generalist species along spatial gradients of habitat disturbance and fragmentation. Oikos 117, 507–514. doi: 10.1111/j.2008.0030-1299.16215.x
Dong, Z., Zhang, Q., Li, L., Lu, Z., Li, C., Ouyang, F., et al. (2020). Landscape agricultural simplification correlates positively with the spatial distribution of a specialist yet negatively with a generalist pest. Sci. Rep. 10:344. doi: 10.1038/s41598-019-57077-4
Drever, M. C., Aitken, K. E. H., Norris, A. R., and Martin, K. (2008). Woodpeckers as reliable indicators of bird richness, forest health and harvest. Biol. Conserv. 141, 624–634. doi: 10.1016/j.biocon.2007.12.004
Edwards, D. P., Gilroy, J. J., Woodcock, P., Edwards, F. A., Larsen, T. H., Andrews, D. J. R., et al. (2014). Land-sharing versus land-sparing logging: reconciling timber extraction with biodiversity conservation. Glob. Chang. Biol. 20, 183–191. doi: 10.1111/gcb.12353
Edwards, D. P., Massam, M. R., Haugaasen, T., and Gilroy, J. J. (2017). Tropical secondary forest regeneration conserves high levels of avian phylogenetic diversity. Biol. Conserv. 209, 432–439. doi: 10.1016/j.biocon.2017.03.006
Ekroos, J., ödman, A. M., Andersson, G. K. S., Birkhofer, K., Herbertsson, L., Klatt, B. K., et al. (2016). Sparing land for biodiversity at multiple spatial scales. Front. Ecol. Evol. 3:145. doi: 10.3389/fevo.2015.00145
Espírito-Santo, M. M., Sevilha, A. C., Anaya, F. C., Barbosa, R., Fernandes, G. W., Sanchez-Azofeifa, G. A., et al. (2009). Sustainability of tropical dry forests: two case studies in southeastern and central Brazil. Forest Ecol. Manag. 258, 922–930. doi: 10.1016/j.foreco.2009.01.022
Foster, B. L., and Tilman, D. (2000). Dynamic and static views of succession: testing the descriptive power of the chronosequence approach. Plant Ecol. 146, 1–10. doi: 10.1023/A:1009895103017
Gilroy, J. J., Edwards, F. A., Uribe, C. A. M., Haugaasen, T., and Edwards, D. P. (2014). Surrounding habitats mediate the trade-off between land-sharing and land-sparing agriculture in the tropics. J. Appl. Ecol. 51, 1337–1346. doi: 10.1111/1365-2664.12284
Giulietti, A. M., Neta, A. L., du, B., Castro, A. A. J. F., Gamarra-Rojas, C. F. L., Sampaio, E. V. S. B., et al. (2004). “Diagnóstico da vegetação nativa do bioma Caatinga,” in Biodiversidade da Caatinga: Áreas e ações prioritárias para a conservação, eds I. R. Leal, M. Tabarelli, M. T. Fonseca, and L. V. Lins (Brasília: Ministério do Meio Ambiente), 48–90.
Gotelli, N. J., and Chao, A. (2013). “Measuring and Estimating Species Richness, Species Diversity, and Biotic Similarity from Sampling Data,” in Encyclopedia of Biodiversity: Second Edition, eds S. A. Levin (Waltham, MA: Academic Press), 195–211. doi: 10.1016/B978-0-12-384719-5.00424-X
Gotelli, N. J., Hsieh, T. C., Sander, E. L., and Colwell, R. K. (2014). Rarefaction and Extrapolation with Hill Numbers: a Framework for Sampling and Estimation in Species Diversity Studies. Ecol. Monogr. 84, 45–67.
Hamer, K. C., Newton, R. J., Edwards, F. A., Benedick, S., Bottrell, S. H., and Edwards, D. P. (2015). Impacts of selective logging on insectivorous birds in Borneo: the importance of trophic position, body size and foraging height. Biol. Conserv. 188, 82–88. doi: 10.1016/j.biocon.2014.09.026
Hilje, B., Sánchez, N. V., Bayne, E. M., and Sánchez-Azofeifa, A. (2020). Bird assemblage recovery in a chronosequence of tropical dry forests in Costa Rica. Forests 11:629,. doi: 10.3390/F11060629
IUCN (2020). The IUCN Red List of Threatened Species. Version 2020-2. Available Online at: https://www.iucnredlist.org (accessed 25 May, 2021).
Latta, S. C., Brouwer, N. L., Mejía, D. A., and Paulino, M. M. (2018). Avian community characteristics and demographics reveal how conservation value of regenerating tropical dry forest changes with forest age. PeerJ 6:e5217. doi: 10.7717/peerj.5217
Laurance, W. F. (2007). Have we overstated the tropical biodiversity crisis? Trends Ecol. Evol. 22, 65–70. doi: 10.1016/j.tree.2006.09.014
Leal, I. R., da Silva, J. M. C., Tabarelli, M., and Lacher, T. E. (2005). Changing the course of biodiversity conservation in the Caatinga of northeastern Brazil. Conserv. Biol. 19, 701–706. doi: 10.1111/j.1523-1739.2005.00703.x
Lebrija-Trejos, E., Bongers, F., Pérez-García, E. A., and Meave, J. A. (2008). Successional change and resilience of a very dry tropical deciduous forest following shifting agriculture. Biotropica 40, 422–431. doi: 10.1111/j.1744-7429.2008.00398.x
Lebrija-Trejos, E., Pérez-García, E. A., Meave, J. A., Poorter, L., and Bongers, F. (2011). Environmental changes during secondary succession in a tropical dry forest in Mexico. J. Trop. Ecol. 27, 477–489. doi: 10.1017/S0266467411000253
Lindbladh, M., Hedwall, P. O., Holmström, E., Petersson, L., and Felton, A. (2020). How generalist are these forest specialists? What Sweden’s avian indicators indicate. Anim. Conserv. 23, 762–773. doi: 10.1111/acv.12595
MacDicken, K. G., Sola, P., Hall, J. E., Sabogal, C., Tadoum, M., and de Wasseige, C. (2015). Global progress toward sustainable forest management. Forest Ecol. Manage. 352, 47–56. doi: 10.1016/j.foreco.2015.02.005
Magnusson, W. E., Grelle, C. E. V., Marques, M. C. M., Rocha, C. F. D., Dias, B., Fontana, C. S., et al. (2018). Effects of Brazil’s political crisis on the science needed for biodiversity conservation. Front. Ecol. Evol. 6:163. doi: 10.3389/fevo.2018.00163
Maia, L. D. S., Leão, M. D. M., Barbosa, M. G., de Souza, S. A., Coutinho, C. R., and Pastori, P. L. (2019). Entomofauna diversity in areas of Caatinga under forest management in the semi-arid region of Ceará. Comun. Sci. 10, 10–20. doi: 10.14295/cs.v10i1.2422
Martensen, A. C., Ribeiro, M. C., Banks-Leite, C., Prado, P. I., and Metzger, J. P. (2012). Associations of forest cover, fragment area, and connectivity with Neotropical understory bird species richness and abundance. Conserv. Biol. 26, 1100–1111. doi: 10.1111/j.1523-1739.2012.01940.x
Miles, L., Newton, A. C., DeFries, R. S., Ravilious, C., May, I., Blyth, S., et al. (2006). A global overview of the conservation status of tropical dry forests. J. Biogeogr. 33, 491–505. doi: 10.1111/j.1365-2699.2005.01424.x
Morrison, E. B., and Lindell, C. A. (2011). Active or Passive Forest Restoration? Assessing Restoration Alternatives with Avian Foraging Behavior. Restor. Ecol. 19, 170–177. doi: 10.1111/j.1526-100X.2010.00725.x
Owen, K. C., Melin, A. D., Campos, F. A., Fedigan, L. M., Gillespie, T. W., and Mennill, D. J. (2020). Bioacoustic analyses reveal that bird communities recover with forest succession in tropical dry forests. Avian Conserv. Ecol. 15, 1–20. doi: 10.5751/ACE-01615-150125
Pennington, R. T., Lavin, M., Prado, D. E., Pendry, C. A., Pell, S. K., and Butterworth, C. A. (2004). Historical climate change and speciation: neotropical seasonally dry forest plants show patterns of both tertiary and quaternary diversification. Philos. Trans. R. Soc. Lond. B Biol. Sci. 359, 515–537. doi: 10.1098/rstb.2003.1435
Pickett, S. T. A. (1989). “Space-for-Time Substitution as an Alternative to Long-Term Studies,” in Long-Term Studies in Ecology: Approaches and Alternatives, ed. G. E. Likens (New York, NY: Springer New York), 110–135. doi: 10.1007/978-1-4615-7358-6_5
Poorter, L., Rozendaal, D. M. A., Bongers, F., de Almeida-Cortez, J. S., Almeyda Zambrano, A. M., Álvarez, F. S., et al. (2019). Wet and dry tropical forests show opposite successional pathways in wood density but converge over time. Nat. Ecol. Evol. 3, 928–934. doi: 10.1038/s41559-019-0882-6
Poudyal, B. H., Maraseni, T., and Cockfield, G. (2018). Evolutionary dynamics of selective logging in the tropics: a systematic review of impact studies and their effectiveness in sustainable forest management. Forest Ecol. Manage. 430, 166–175. doi: 10.1016/j.foreco.2018.08.006
Prado, D. E. (2003). “As caatingas da América do Sul,” in Ecologia e conservação da Caatinga, eds I. R. Leal, M. Tabarelli, and J. M. C. da Silva (Recife: Editora Universitária da UFPE), 3–73.
R Core Team (2019). R: A language and environment for statistical computing. Available Online at: https://www.r-project.org (accessed 30 September, 2019).
Ribeiro, E. M. S., Arroyo-Rodríguez, V., Santos, B. A., Tabarelli, M., and Leal, I. R. (2015). Chronic anthropogenic disturbance drives the biological impoverishment of the Brazilian Caatinga vegetation. J. Appl. Ecol. 52, 611–620. doi: 10.1111/1365-2664.12420
Ribeiro-Neto, J. D., Arnan, X., Tabarelli, M., and Leal, I. R. (2016). Chronic anthropogenic disturbance causes homogenization of plant and ant communities in the Brazilian Caatinga. Biodivers. Conserv. 25, 943–956. doi: 10.1007/s10531-016-1099-5
Samojlik, T., Fedotova, A., and Kuijper, D. P. J. (2016). Transition from traditional to modern forest management shaped the spatial extent of cattle pasturing in Białowieża Primeval Forest in the nineteenth and twentieth centuries. Ambio 45, 904–918. doi: 10.1007/s13280-016-0795-4
Sanchez-Azofeifa, G. A., Quesada, M., Rodriguez, J. P., Nassar, J. M., Stoner, K. E., Castillo, A., et al. (2005). Research priorities for Neotropical dry forests. Biotropica 37, 477–485. doi: 10.1111/j.1744-7429.2005.00066.x
Sayer, C. A., Bullock, J. M., and Martin, P. A. (2017). Dynamics of avian species and functional diversity in secondary tropical forests. Biol. Conserv. 211, 1–9. doi: 10.1016/j.biocon.2017.05.004
Shahabuddin, G., and Kumar, R. (2006). Influence of anthropogenic disturbance on bird communities in a tropical dry forest: role of vegetation structure. Anim. Conserv. 9, 404–413. doi: 10.1111/j.1469-1795.2006.00051.x
Singh, S. P. (1998). Chronic disturbance, a principal cause of environmental degradation in developing countries. Environ. Conserv. 25, 1–2. doi: 10.1017/S0376892998000010
Soares-Filho, B., Rajao, R., Macedo, M., Carneiro, A., Costa, W., Coe, M., et al. (2014). Cracking Brazil’s forest code. Science 344, 363–364. doi: 10.1126/science.1246663
Stoner, K. E., and Sánchez-Azofeifa, G. A. (2009). Ecology and regeneration of tropical dry forests in the Americas: implications for management. Forest Ecol. Manage. 258, 903–906. doi: 10.1016/j.foreco.2009.05.019
Tabarelli, M., Peres, C. A., and Melo, F. P. L. (2012). The “few winners and many losers” paradigm revisited: emerging prospects for tropical forest biodiversity. Biol. Conserv. 155, 136–140. doi: 10.1016/j.biocon.2012.06.020
Thiollay, J. M. (1997). Disturbance, selective logging and bird diversity: a Neotropical forest study. Biodivers. Conserv. 6, 1155–1173. doi: 10.1023/A:1018388202698
Tucker, C. J., and Sellers, P. J. (1986). Satellite remote sensing of primary production. Int. J. Remote Sen. 7, 1395–1416. doi: 10.1080/01431168608948944
Vieira, D. L. M., and Scariot, A. (2006). Principles of natural regeneration of tropical dry forests for restoration. Restor. Ecol. 14, 11–20. doi: 10.1111/j.1526-100X.2006.00100.x
Vieira, D. L. M., Scariot, A., Sampaio, A. B., and Holl, K. D. (2006). Tropical dry-forest regeneration from root suckers in central Brazil. J. Trop. Ecol. 22, 353–357. doi: 10.1017/S0266467405003135
Vinson, C. C., Kanashiro, M., Sebbenn, A. M., Williams, T. C. R., Harris, S. A., and Boshier, D. H. (2015). Long-term impacts of selective logging on two amazonian tree species with contrasting ecological and reproductive characteristics: inferences from eco-gene model simulations. Heredity 115, 130–139. doi: 10.1038/hdy.2013.146
Keywords: anthropogenic disturbance, ecological loser, landscape fragmentation, avian communities, Caatinga
Citation: Ribeiro JR, Las-Casas FMG, Lima HS, Silva WAG and Naka LN (2021) The Effect of Forest Management on the Avifauna of a Brazilian Dry Forest. Front. Ecol. Evol. 9:631247. doi: 10.3389/fevo.2021.631247
Received: 19 November 2020; Accepted: 25 June 2021;
Published: 16 July 2021.
Edited by:
Juliana Hipólito, National Institute of Amazonian Research (INPA), BrazilReviewed by:
Bráulio Almeida Santos, Federal University of Paraíba, BrazilEduardo Venticinque, Federal University of Rio Grande do Norte, Brazil
Copyright © 2021 Ribeiro, Las-Casas, Lima, Silva and Naka. This is an open-access article distributed under the terms of the Creative Commons Attribution License (CC BY). The use, distribution or reproduction in other forums is permitted, provided the original author(s) and the copyright owner(s) are credited and that the original publication in this journal is cited, in accordance with accepted academic practice. No use, distribution or reproduction is permitted which does not comply with these terms.
*Correspondence: Luciano Nicolás Naka, bHVjaWFuby5uYWthQHVmcGUuYnI=