- 1Department of Animal Ecology, Netherlands Institute of Ecology (NIOO-KNAW), Wageningen, Netherlands
- 2Department of Integrative Biology, Oklahoma State University, Stillwater, OK, United States
- 3Department of Biodiversity, Ecology and Evolution, Complutense University of Madrid, Madrid, Spain
In migratory species, the timing of arrival at the breeding grounds is a life-history trait with major fitness consequences. The optimal arrival date varies from year-to-year, and animals use cues to adjust their arrival dates to match this annual variation. However, which cues they use to time their arrival and whether these cues actually predict the annual optimal arrival date is largely unknown. Here, we integrate causal and evolutionary analysis by identifying the environmental variables used by a migratory songbird to time its arrival dates and testing whether these environmental variables also predicted the optimal time to arrive. We used 11 years of male arrival data of a pied flycatcher population. Specifically, we tested whether temperature and normalized difference vegetation index (NDVI) values from their breeding grounds in the Netherlands and from their wintering grounds in Ivory Coast explained the variation in arrival date, and whether these variables correlated with the position of the annual fitness peak at the breeding grounds. We found that temperature and NDVI, both from the wintering and the breeding grounds, explained the annual variation in arrival date, but did not correlate with the optimal arrival date. We explore three alternative explanations for this lack of correlation. Firstly, the date of the fitness peak may have been incorrectly estimated because a potentially important component of fitness (i.e., migration date dependent mortality en route or directly upon arrival) could not be measured. Secondly, we focused on male timing but the fitness landscape is also likely to be shaped by female timing. Finally, the correlation has recently disappeared because climate change disrupted the predictive value of the cues that the birds use to time their migration. In the latter case, birds may adapt by altering their sensitivity to temperature and NDVI.
Introduction
In seasonal habitats, the timing of annual cycle stages such as flowering and bud burst in plants, and reproduction, molt or migration in animals, is matched with a short window in which conditions are optimal for these stages. Organisms often suffer major fitness costs if their annual cycle stages occur outside this “optimal window” and hence, in several species, the timing of these stages is often under strong stabilizing selection (Perrins, 1970; Noordwijk et al., 1995; Brown and Brown, 2000; Bêty et al., 2004; Smith and Moore, 2005). Examples range from birds (Perrins, 1970; Noordwijk et al., 1995; Brown and Brown, 2000; Bêty et al., 2004; Smith and Moore, 2005) to fish (Einum and Fleming, 2000), plants (Kelly and Levin, 1997; Donohue et al., 2005; Koenig et al., 2012), and invertebrates (Philippart et al., 2003). Optimal time windows may be very narrow and vary in date and/or duration from one year to the other. For instance, the timing of the optimal window for breeding may be related to the moment when food abundance is at its peak (Noordwijk et al., 1995; Visser et al., 2006), while the local climate would be an important determinant of optimal arrival date at the breeding grounds in migratory birds (Brown and Brown, 2000; Erni et al., 2005).
Matching the timing of annual cycle stages with the year-to-year variation in the optimal time window is not straightforward. The timing of annual cycle stages often has to be made well in advance of the time when the stages are under selection, since many of these stages require a period of preparation (Noordwijk and Müller, 1994; Visser et al., 2004, 2010). For instance, to take full advantage of an optimum in food availability during the reproductive stage, a bird must have chicks in the nest at this precise moment, but having chicks in the nest depends mainly on when eggs were laid, which happens several weeks earlier in the season (Visser, 2008, 2013). Individuals therefore respond to environmental conditions that serve as so-called cues, which affect the timing of these stages. Importantly, these cues are only useful if they are predictive, i.e., when they correlate with or causally affect the fitness landscape for that annual cycle stage (Noordwijk and Müller, 1994; Visser et al., 2004; Figure 1).
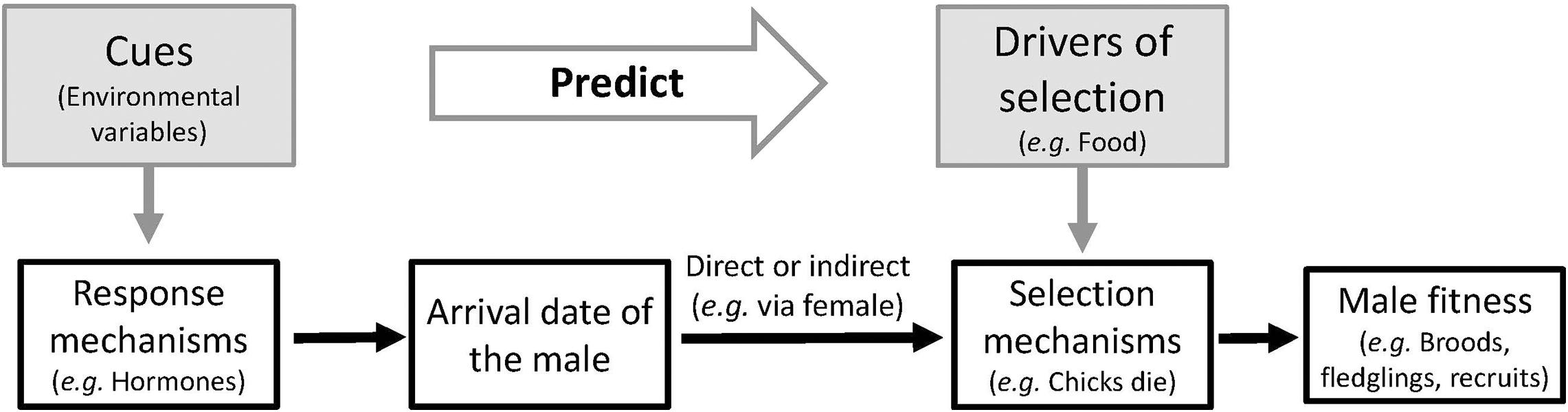
Figure 1. Link between environmental variables that are correlated with year-to-year variation in arrival date (cues) and their effect on the fitness consequences of arrival date: The year-to-year variation in fitness depends on the interaction between the environmental drivers of selection and individual decisions. Because decisions on arrival date are not made in the same (spatial and temporal) environment of selection, animals use cues to predict conditions of the environment at the time of selection (adapted from Noordwijk and Müller, 1994; Visser et al., 2004, 2010).
Migratory animals are particularly interesting to study the phenology of key annual cycle stages, as their decisions on the timing of some stages need to be made not only at an earlier time than the optimal time window, but also at a different geographical location. Long-distance migrants overwinter far away from their breeding grounds, and hence cannot directly sense the conditions they will face upon arrival and during breeding (Both and Visser, 2001; Visser and Both, 2005). Photoperiodic variation is considered to be the major cue that these animals use to time their migration (Gwinner, 1987, 1989a,b; Dawson, 2002; Coppack and Pulido, 2004; Pulido, 2007). However, the photoperiodic regime is invariable among years and cannot predict annual variation in optimal conditions, making it unreliable to fine-tune the timing of migration to the among-year variation in timing of the optimal window.
The fine-tuning of migration timing is related to environmental conditions (Pulido, 2007). For instance, some birds arrive earlier on their breeding grounds when temperatures are higher before departure from non-breeding areas during spring migration (Both et al., 2005; Marra et al., 2005). Moreover, weather conditions can not only affect departure decisions (Gordo, 2007; Bauer et al., 2008; Eikenaar and Schmaljohann, 2014; Deppe et al., 2015; Ouwehand and Both, 2017) but en route decisions as well, leading to minor adjustments in timing of spring arrival (Hüppop and Hüppop, 2003; Hüppop and Winkel, 2006; Bauer et al., 2008; Both, 2010; Haest et al., 2018). It is poorly known if the environmental conditions in the overwintering grounds that affect arrival date also correlate with the conditions at the breeding grounds. However, if they do correlate, then it is possible for environmental variables at the wintering grounds to also predict the annual variation in fitness peaks at the breeding sites. Some studies suggest that large-scale correlations in climatic conditions exist between Europe and Africa (Lehikoinen et al., 2004; Saino and Ambrosini, 2008). This means that individuals may acquire information (e.g., temperature, weather) before and during migration to predict the conditions at the breeding grounds, and thus time their arrival in relation to the optimal time window (Bauer et al., 2008; Both, 2010; Tøttrup et al., 2010).
Identifying the climatic variables that allow birds to predict the fitness peak later in the season is relevant in light of current environmental changes. It is known that many bird species have advanced their migration dates, suggesting that migrants are able to adjust their migration timing to the environmental conditions, but this pattern is not consistent across species (Both and Visser, 2001; Cotton, 2003; Marra et al., 2005; Gienapp et al., 2007; Usui et al., 2016; Mayor et al., 2017). In contrast, the relationship between advancements in timing of migration and shifts in optimal conditions to migrate is poorly known since individual fitness data are rarely available (Visser and Both, 2005). If the environmental variables the animals use for timing their migration merely correlate with the drivers of selection, rather than causally affecting the fitness landscape, climate change may decrease the reliability of these cues by disrupting these correlations, as there is both spatial and temporal variation in the rate of climate change (Easterling et al., 1997; Vose et al., 2005; Stocker et al., 2013).
Here we analyzed 11 years of data of individual male arrival date and breeding success of a long-distance migratory passerine, the European pied flycatcher (Ficedula hypoleuca) to understand which environmental variables serve as cues for the timing of arrival, and whether these cues are indeed predictors of the annual variation in the optimal arrival date (i.e., the fitness landscape). For that, we explored temperature and the normalized difference vegetation index (NDVI) in the breeding and wintering grounds as potential cues and predictors of optimal arrival date.
Materials and Methods
Study System and Study Area
Pied flycatchers [Ficedula hypoleuca ([Pallas], 1764) Muscicapidae] are small long-distance migratory birds that breed in Europe and winter in Africa. Due to their propensity to use nest boxes, it is also possible to precisely monitor their breeding. Long-term data collection was conducted in the southern forests of the Hoge Veluwe National Park (Netherlands; 52°02′07″N 5°51′32″E). Voucher material of this pied flycatcher population was deposited in the ornithology collection of the Naturalis Biodiversity Center (Leiden, Netherlands) under the inventory numbers RMNH 592347, RMNH 592348, and RMNH 592349.
Male Arrival Dates
Male individual arrival data were collected from 2005 to 2015 (11 years). Male flycatchers settle soon upon arrival in a territory from which they are rarely displaced, and advertise a cavity or nest box to the females by singing continuously at or close to the potential nest site, where they can eventually breed (Potti, 1998; Both et al., 2016). Their advertising behavior made them very conspicuous, which provides very high daily detection probabilities (>80%; Both et al., 2016). Individual arrival date of males was assessed by daily field observations of singing males in the study area from early April until the first week of May. These field observations were made by one to three (typically two) trained observers who walked independently pre-established routes covering the whole study area and visiting all nest boxes. Routes and direction of the routes were alternated daily among observers in order to prevent biases due to among-observer variation in detection probability and accuracy. Although marking all male birds with unique color ring combinations was logistically impossible (due to potential leg injury and to the relatively large population size), we used field descriptions of male plumage characteristics and the presence/absence and position of one metal and one (sometimes two) color rings to establish whether a bird initially singing in a particular nest box was indeed the same that ended up breeding in it (see Potti, 1998; Both et al., 2016 for similar approaches). In the Netherlands, male pied flycatchers display a relatively large individual variation in plumage characteristics, such as the color of dorsal feathers (from female-like brown to almost fully black) and the size and shape of their forehead patch (see Drost, 1936; Lundberg and Alatalo, 1992; Both et al., 2012 and Supplementary Material). This method allows us to assign with high certainty the arrival date to more than half of the singing males in the study area (Visser et al., 2015). Likewise, the reliability of this approach is supported by a strong correlation (r = 0.92) between the arrival dates estimated by visual observations and the arrival dates obtained from geolocator data (Both et al., 2016).
During the chick-rearing phase (see below), males were caught, described again in terms of plumage characteristics and ringed. In most years (but not in 2005 and 2012) we also collected data on “bachelors,” i.e., males that were unpaired when most females had arrived. Those males were captured and identified when they were inspecting and advertising their potential nest site using spring traps installed at the entrance of the nest boxes. Some of those presumed bachelors became breeding birds later in the season. Arrival data of these bachelor males were also used in the analyses except for number of fledglings and recruits.
Individual Breeding Success
Apart from data on timing of arrival, we also collected information on individual breeding success using a standardized nest monitoring protocol (Visser et al., 2015). For all years in which we had arrival data we collected data on whether the male obtained a social female or not (i.e., probability of obtaining a mate, as described above), number of chicks successfully raised (i.e., number of fledglings), and whether individual offspring recruited as a breeding bird (a so-called recruit) in the following year(s) or not. Female pied flycatchers only have a single brood per season, with cases of second broods being rare (Both et al., 2019), but males may attract and pair with a second female after their first female starts to incubate. In those few cases of polygyny in which males were paired with two females with similar laying dates (33 out of 676 observations), both broods were included.
Environmental Variables
Data on Dutch daily mean temperatures were obtained from the Dutch meteorological institute database (KNMI – https://www.knmi.nl/nederland-nu/klimatologie/, accessed on February 2016). For these, we used data from the Deelen weather station which is directly adjacent to the study area. For African temperatures, we obtained daily air gridded temperatures for Ivory Coast from the NCEP Daily Global Analyses data provided by the NOAA/OAR/ESRL PSD, Boulder, CO, United States, from their Web site at https://www.esrl.noaa.gov/psd/ (Accessed on January 2018). Previous geolocator data pinpoint Ivory Coast as the main wintering location of our study population (Tomotani et al., 2019), which is additionally supported by published data from other geographically close pied flycatcher populations (Ouwehand et al., 2016). We then obtained NDVI data from the MODIS/Terra Vegetation Indices 16-Day L3 Global 0.05 Deg CMG V006 (Didan, 2015) and extracted average values for the whole Ivory Coast and for the breeding location at the Hoge Veluwe National Park in the Netherlands. We opted for only using wintering and breeding grounds temperatures because en route temperatures (timing and location) would be highly dependent on individual migration routes and timing of migration, and this information was not available.
Finally, we obtained data on the photoperiodic variation of the Netherlands from the National Oceanic and Atmospheric Administration, United States (NOAA, https://www.noaa.gov/ accessed on February 2016). We considered the civil twilight as the boundary of the effective light phase relevant for the birds (see, Gwinner, 1989a). Because photoperiodic variation on the breeding and wintering grounds has a high correlation, changing in the same direction at the same time, it was not necessary to obtain and model day-length data for Ivory Coast separately (Supplementary Figure 1).
Data Analysis
All analyses were performed in R version 3.4.3 (R Core Team, 2017). To define the minimal adequate models, in all cases we used backward model selection, i.e., sequentially dropping non-significant terms starting with interactions, retaining only significant terms. All statistics are reported at the point that the term was dropped from the model.
Cues Used for Arrival Date
To assess which environmental variables affect arrival date, we followed the method described in Gienapp et al. (2005) and used proportional hazard models (Cox, 1992) with random effects implemented in the R “survival” and “coxme” packages (Therneau, 2015). Proportional hazard models calculate the daily probability of an event to occur, and how this depends on explanatory variables, which can be time-dependent (i.e., variables that change their value during the time an individual is “at risk”). Using this approach is biologically more realistic than using fixed time windows over which these environmental variables are averaged (Gienapp et al., 2005). The proportional hazard approach also allows the inclusion of day-length effects as a proxy for time of year.
First, we defined the most relevant period over which the environmental variables affected the arrival dates. For both Dutch and Ivory Coast temperatures and NDVI values, the value at day t was calculated as the average over six periods of increasing length ending at day t (window duration from 5 to 30 days with a 5-day increment for each period). For example, for the 30th of April, we produced six windows with averaged temperatures and NDVI of the Netherlands and Ivory Coast: from days 25-April to 30-April (5 days), 20-April to 30-April (10 days), 15-April to 30-April (15 days), 10-April to 30-April (20 days), 5-April to 30-April (25 days), and 1-April to 30-April (30 days). In the case of Ivory Coast NDVI and temperatures, we also used lagged shifting windows of the same length (20 days) but ending 20–80 days before day t [based on the time taken during spring migration; (Ouwehand et al., 2016)]. Thus, for the same 30th of April example, averages over 20 days were made for four additional periods: 21-January to 10-February (80-day lag), 10-February to 2-March (60-day lag), 2-March to 22-March (40-day lag), and 22-March to 11-April (20-day lag). In total, we compared 24 possible combinations of window durations (5, 10, 15, 20, 25, or 30 days) and window lags (20, 40, 60, 80 days) using the model log-likelihood (see Supplementary Table 1).
After defining the position and duration of the climate window, we tested, also with proportional hazard models, which variables significantly explained the variation in arrival date of the pied flycatchers. We fitted Ivory Coast (with and without lag) and Dutch temperatures, Ivory Coast (with and without lag) and Dutch NDVI and the two-way interactions between day-length and Dutch temperature, day-length and lagged Ivory Coast temperature and day-length and lagged Ivory Coast NDVI to investigate whether the effects of environmental variables differ throughout the season. In this analysis we also included characteristics of the birds that could potentially explain variation in arrival dates: age and the Drost score (variation in male color from 1, completely black males, to 7, brown males; Drost, 1936) which could be correlated with age, metabolic or behavioral differences (Ivankina et al., 2001, 2007; Kerimov et al., 2013). Given the difficulties of aging the breeding birds based on plumage characteristics, age was solely assigned based on ringing data records, distinguishing three categories: “2nd-calendar-year birds” (SCY), i.e., birds that hatched the year before (when they were ringed as chicks); “after second-calendar-year-birds” (ASCY), i.e., birds that were at least 2 years old; and “unknown,” i.e., for non-ringed breeders in which age cannot be reliably assigned). The interaction between age and the environmental variables was also included to test whether those variables affected younger and older birds in a different way. Individual was included as random effect to control for multiple observations of the same individual.
Fitness Consequences of Arrival Date
To test whether male fitness depended on arrival date, we regressed fitness against the arrival date in interaction with the environmental variable. To account for the expected non-linear effect of fitness versus arrival date, we also included arrival date squared. A significant interaction of arrival date and the particular environmental variable indicates that the environmental variable correlates with the date of arrival where fitness is maximized in that year (i.e., the position of the fitness peak).
We used three fitness components to assess the fitness consequences of arrival date: the probability of obtaining a mate, number of fledglings of a male brood(s) and the probability that at least one of these fledglings recruited as a breeding bird in the following years (probability of obtaining a recruit). Tests were done with linear mixed effect models with year and individual as a random effects and “nloptwrap” as optimizer (“lme4” R package, Bates et al., 2015) for each environmental variable and each fitness component separately. We used a logit-link and Binomial error distribution for the probability of obtaining a mate and obtaining a recruit (both modeled as whether or not the male obtained a mate or recruit, respectively) and Poisson distribution for the number of fledglings. Model comparison was performed with parametric bootstrap with 2000 simulations (“PBmodcomp” implementation of the R package “pbkrtest,” Halekoh and Højsgaard, 2014). Sample sizes varied in the analysis of each fitness components, because some components were affected by one of the experimental manipulations done in this population over the past decades (see, for instance, Bauchau and Seinen, 1997; Tomotani et al., 2016).
Finally, we looked at whether the mean arrival date (observed) correlated with the optimal arrival date. In order to extract the latter, we fitted a model of individual fitness (probability of obtaining a mate or number of fledglings) against year, arrival date, arrival date squared, and the interaction between arrival date and year (with individual as random effect) and regressed the estimates for the year × arrival date term (which represents the position of the annual fitness peak) against the mean arrival date of each year.
Results
Cues Used for Arrival Date
The most relevant period over which the environmental variables affected the arrival dates included Ivory Coast temperatures and NDVI with the window moved by a 60-day lag (thus starting, on average, around the 18th of February), as well as a window duration of 25 days for the non-lagged Ivory Coast and Dutch temperatures and NDVI (Supplementary Table 1).
Higher temperatures in both the Netherlands and Ivory Coast were related to earlier arrival; with a significant interaction between temperature and day-length, indicating that the temperature effect was weaker later in the season (coefficients indicate a change in the “hazard” for the event to occur: Netherlands: coefficient for the interaction = −0.01 ± 0.001, coefficient for temperature = 5.88 ± 1.1; Ivory Coast: coefficient for the interaction = −0.14 ± 0.03, coefficient for temperature = 132.09 ± 30.60; Table 1). Finally, the non-lagged Ivory Coast temperature was also related to an earlier arrival (coefficient = 0.55 ± 0.47; Table 1), but without the significant interaction with day-length (Table 1).
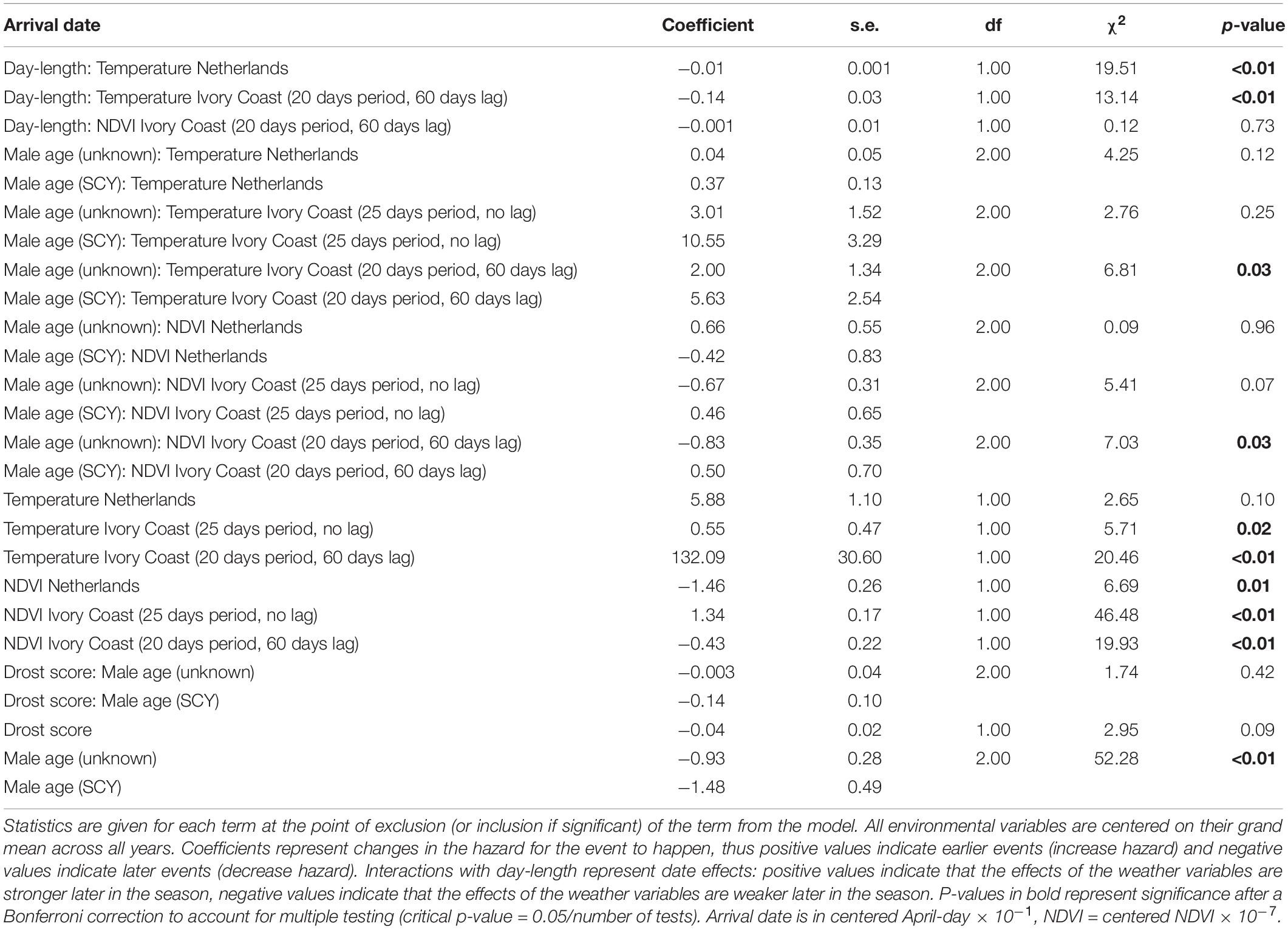
Table 1. Test of the environmental variables that affect the arrival date of male pied flycatchers, using a proportional hazard model (676 events).
Higher NDVI in the Netherlands and higher lagged NDVI in Ivory Coast were related to later arrival (Netherlands: coefficient = −1.46 ± 0.26; Ivory Coast: coefficient = −0.56 ± 0.22; Table 1). On the other hand, higher NDVI in Ivory Coast in the month prior to arrival (not lagged) was related to earlier arrival (coefficient = 1.34 ± 0.17; Table 1).
Male arrival was also explained by age. After second calendar year (ASCY) males tended to arrive earlier than second calendar year birds (SCY; coefficient for SCY = −1.48 ± 0.49; Table 1). We also found a significant interaction between age and lagged Ivory Coast temperatures, with a stronger temperature effect in younger birds (coefficient for the interaction: SCY relative to ASCY = 5.63 ± 2.54; Table 1) as well as between age and lagged Ivory Coast NDVI, again, with a stronger effect in SCY (coefficient for the interaction: SCY relative to ASCY = 0.50 ± 0.70; Table 1). Thus, higher temperatures and NDVI values have a stronger effect on the arrival of younger birds (SCY) than of older birds (ASCY). Drost scores (male color) did not explain variation in arrival dates (Table 1).
Fitness Consequences of Arrival Date
We observed a significant relationship between arrival date and both the probability of obtaining a mate (Table 2) and the number of fledglings produced (Table 3), but found no relationship between arrival date and the probability of obtaining a recruit (Table 4). Late arriving males had a much lower probability of obtaining a mate than early arriving males (Table 2 and Supplementary Figure 2A). The number of fledglings generally declined with arrival date but the decline was steeper in late-arriving males (Table 3 and Supplementary Figure 2B). Yearly recruitment was low overall with 11–49 recruits per year.
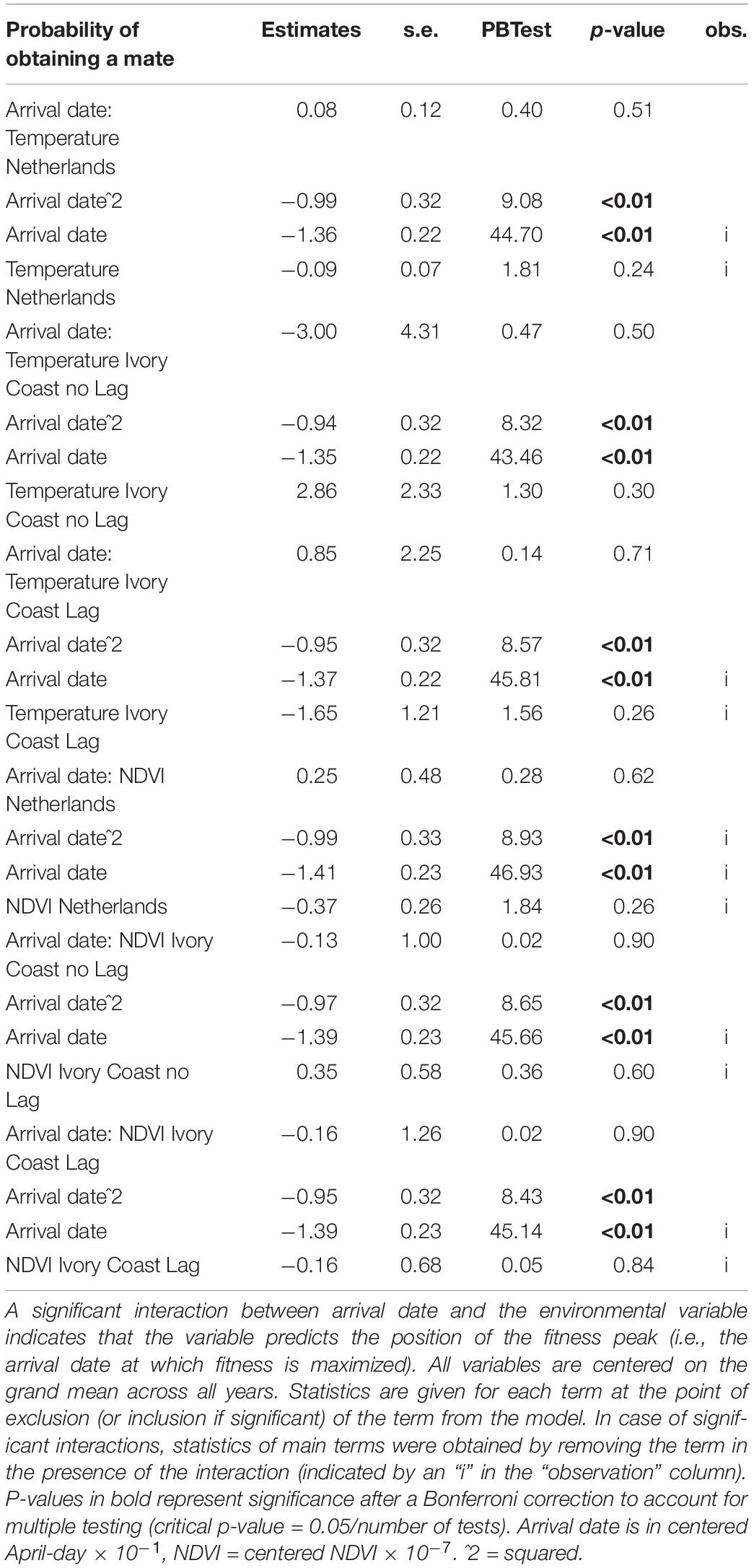
Table 2. Test of which environmental variables affect the fitness landscape of arrival dates of Pied Flycatchers, for the fitness component “probability of obtaining a mate” (529 observations).
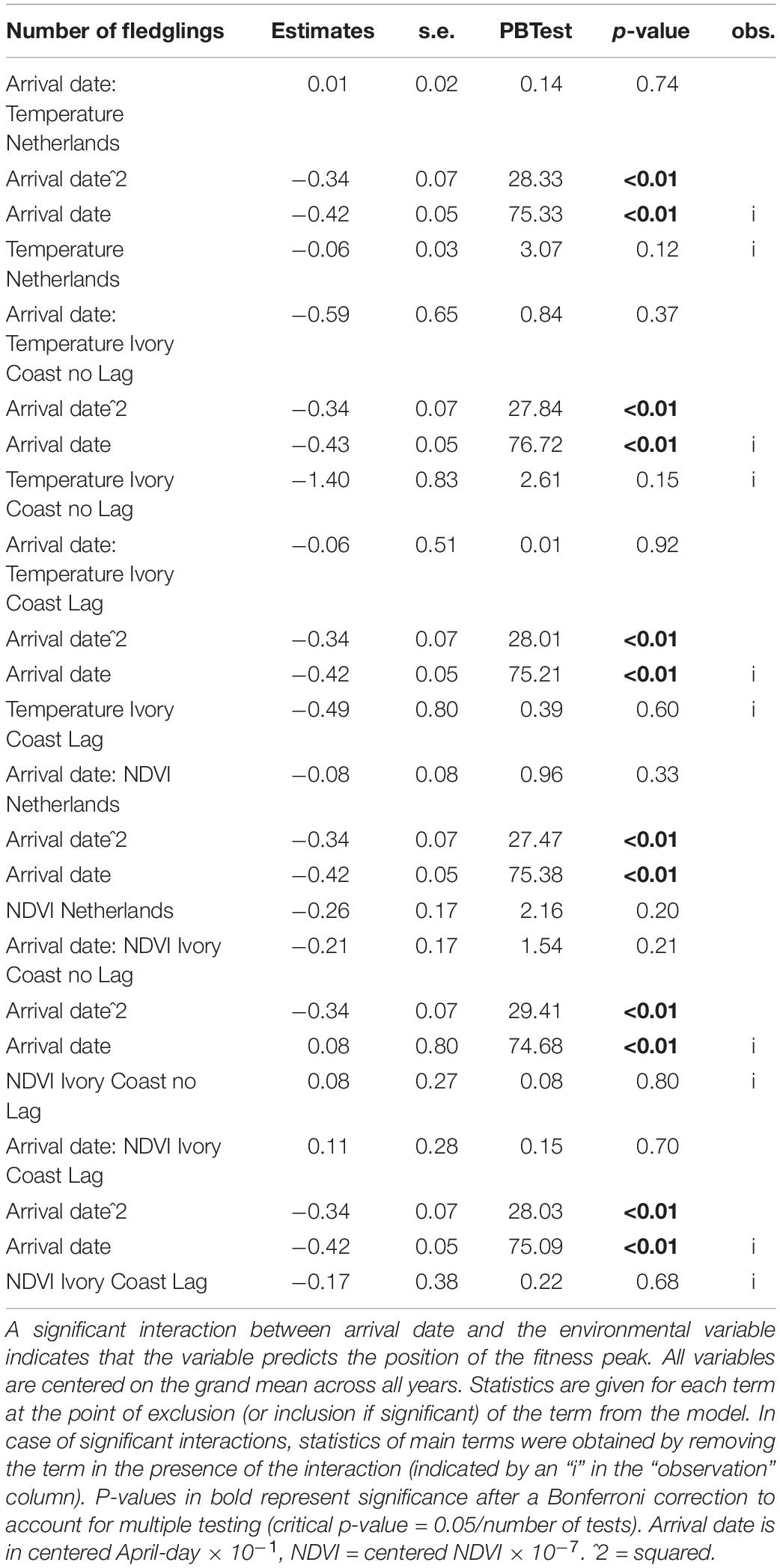
Table 3. Test of which environmental variables affect the fitness landscape of arrival dates of Pied Flycatchers, for the fitness component “number of fledglings” (676 observations).
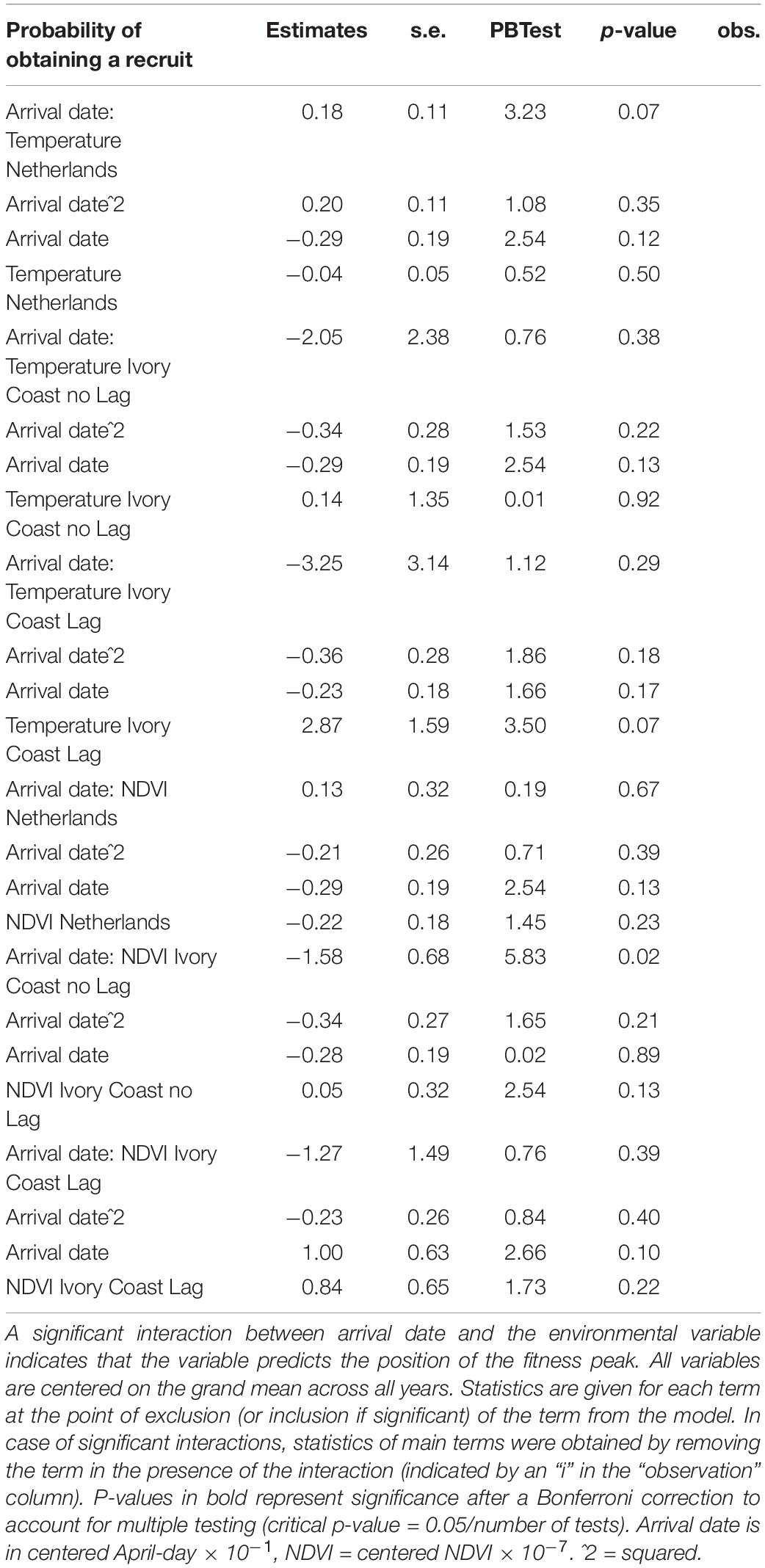
Table 4. Test of which environmental variables affect the fitness landscape of arrival dates of Pied Flycatchers, for the fitness component “probability of obtaining a recruit” (450 observations).
Although we found a relationship between arrival date and fitness, when we tested whether the position of this fitness peak was affected by environmental variables, we did not find an effect: none of the environmental variables correlated with the position of the fitness peak regardless of the fitness measure used (represented by the interaction between arrival date and the environmental variable, Tables 2, 3). In accordance with this result, when we correlated the observed mean arrival date and the calculated optimal arrival date of a given year, we did not observe a relationship. This was the case for both when the probability of obtaining a mate and when the number of fledglings – the two fitness measures that were related to arrival date – were used (for the probability of obtaining a mate: estimate = −0.38 ± 1.84, F1,6 = 0.04, p-value = 0.84; for the number of fledglings: estimate = −0.47 ± 0.46, F1,9 = 1.06, p-value = 0.33; Figure 2). Therefore, there is a mismatch between optimal and mean arrival date and this is partly related to the very low variation in the optimal arrival date between years (Figure 2).
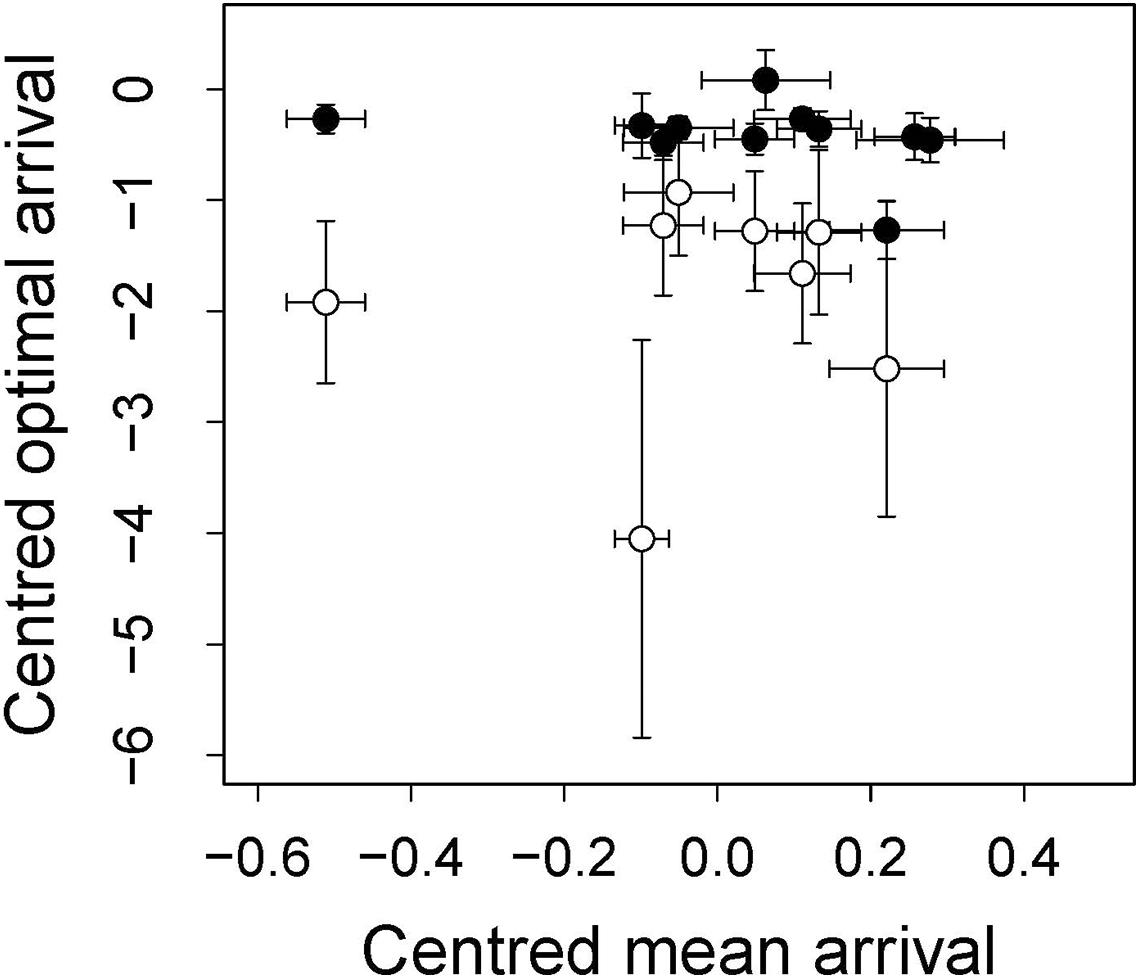
Figure 2. Relationship between optimal and mean arrival dates. Optimal arrival date was extracted from the estimates of the model testing the relationship between probability of obtaining a mate (open dots) or number of fledglings (closed dots) and year, arrival and arrival squared, also including the interaction between year and arrival. Mean arrival date was calculated averaging the arrival dates of all males per year. Both optimal and mean arrival dates are centered on the grand mean. Each point represents 1 year.
Discussion
Temperature and NDVI values in the Netherlands and Ivory Coast explained year-to-year variation in timing of arrival at the breeding grounds. These variables, however, did not correlate with the year-to-year variation in optimal arrival date, i.e., the arrival date at which fitness was maximized in that year. The lack of a relationship between the optimal and mean arrival dates suggests that this is not because we overlooked potential cues. These results are surprising: why would birds use cues for their arrival date that do not lead to an arrival date with a higher fitness return?
If there are no environmental variables that are predictive for the year-to-year variation in the conditions the birds will encounter when arriving at the breeding grounds, the best strategy for arrival date would be to arrive at the same time every year, at a date which on average over years is closest to the optimal arrival date (e.g., Gienapp et al., 2014). For example, the birds could make the arrival decision by relying solely on endogenous rhythms and/or responding directly to the photoperiodic changes in their wintering grounds (Gwinner, 1996), which show no year-to-year variation. But while repeatability of individual arrival dates is moderate in some species (e.g., pied flycatcher: 0.08–0.3 Both et al., 2016; purple martins: 0.3 Fraser et al., 2019; Eastern kingbird: 0.05–0.39 Cooper et al., 2009; also see Pulido, 2007; Both et al., 2016 for a revision), there is still variation between years (Both et al., 2016).
Our data show, however, that environmental variables from both the breeding and wintering grounds do explain year-to-year variation in arrival dates. Other studies have also reported effects of environmental conditions, both en route and at the breeding grounds, on the variation in timing of spring arrival, or, at least, the timing of passage dates, in the pied flycatcher (Ahola et al., 2004; Both et al., 2005; Saino et al., 2007). It is generally accepted that several migratory bird species migrate earlier in warmer springs (Gordo, 2007) and that timing of migration advances with climate change (Walther et al., 2002; Cotton, 2003; Gienapp et al., 2007, but see Both and Visser, 2001; Jenni and Kery, 2003; Tomotani et al., 2018). These studies and our results here suggest that there is plasticity in timing of arrival (but see Pulido, 2007), arguing against the inflexible strategy as the main (or only) mechanism. So, the question remains why the cues that are used by the pied flycatcher in their arrival date do not predict the timing of the fitness peak associated with arrival date. We discuss below three hypotheses: incomplete fitness measure, fitness benefits via females and disruption of cues due to climate change.
Incomplete Fitness Measure
A potential reason why we were unable to detect a correlation between environmental variables that are used as cues and their effect on the position of the fitness peak are our fitness measures. While we are able to precisely determine the breeding success of the birds that we were able to identify upon arrival, we have no data of birds that did not successfully complete their migration or of birds that completed the migration but bred elsewhere. While mortality during migration can be estimated from direct tracking (e.g., Klaassen et al., 2013; Rotics et al., 2016) or extensive re-sighting studies (e.g., Sillett and Holmes, 2002; Leyrer et al., 2013; Lok et al., 2015), data on mortality related to timing of migration, which is what is relevant for our research, is difficult, if not impossible, to obtain, since for obtaining an arrival date the individual must be alive (but see, e.g., Brown and Brown, 2000; Newton, 2007). If there is a departure-date-related mortality, the proper fitness measurement would be a measurement that includes all mortality events between departure from the wintering grounds and breeding (i.e., en route and arrival before breeding), and then the reproductive success, that are related to the departure decision. This could potentially shift the position of the fitness peak that we obtained only using the reproductive success of the birds that did arrive (Jonzén et al., 2007; Lof et al., 2012).
There are few data on migration-timing-dependent mortality, particularly for small migrants such as pied flycatchers. The few existing examples show that poor weather conditions during migration, or when birds arrive at the breeding grounds, can heavily impact the early arriving birds thereby shaping the optimal timing of arrival (Brown and Brown, 2000; Newton, 2007). This acute effect of weather upon arrival could shift the fitness peak to a later date when spring is cold, if the low temperatures lead to a higher mortality for early arriving birds. Such effect of the temperature at the breeding grounds was previously shown for the pattern of recruitment of pied flycatcher chicks of the same population as the present study: selection for early arrival at the moment of recruitment to the breeding population was stronger in warmer than colder years, likely due to lower survival of early recruiting birds in cold springs (Visser et al., 2015). Thus, part of our missing fitness component could be explained by temperatures in the Netherlands between arrival and breeding for both experienced and first-time breeders.
Another cue that explained the variation in arrival dates was the NDVI, which could indicate that the food availability at the wintering grounds and, in turn, the fueling and individual condition prior to departure play a role in the arrival timing. Timing of migration may indeed be dependent on the individual condition due to carry-over effects from good wintering habitats and this “condition” could potentially modify the relationship between the mortality and the departure date from the wintering grounds (Norris et al., 2004; Saino et al., 2004; Pulido, 2007; Studds and Marra, 2011). For example, higher NDVI could improve the condition of early migrants and lead to lower mortality of early birds shifting the fitness peak to an earlier date (due to a flatter relationship between mortality and departure). Indeed, our results show that higher lagged NDVI values correlate with earlier arrivals (Table 1).
Finally, high temperatures in Ivory Coast were also correlated to a later arrival, suggesting a similar role for the Ivory Coast temperatures as the NDVI. Tøttrup et al. (2012), for example, reported that droughts in the wintering grounds caused delays in arrival date of songbirds; the reason for this was a prolonged stay in stopover sites, suggesting that these birds had a slower fueling rate. Thus, temperatures at the wintering grounds have a similar potential to affect the slope of the mortality and departure relationship thereby shifting the optimal arrival date.
The absence of a correlation between the cues that explain the variation of arrival dates and the position of the fitness peak could, therefore, be caused by these various ways that the unmeasurable date-dependent mortality between departure of the wintering grounds and reproduction at the breeding grounds shift the fitness peak.
Fitness Benefits via Females
This study focused on the causes and fitness consequences of variation in male arrival time, using three fitness components: the probability of obtaining a mate, the number of fledglings and the probability to obtain recruits. The probability of a male to find a mate is related to male arrival as early arriving males will be more likely to attract a female (Canal et al., 2012; Tomotani et al., 2017). On the other hand, the number of fledglings and probability of obtaining recruits are fitness components much more related to females and thus the fitness benefits of early arriving for males must be because for instance early arriving males pair with early laying females (Saino et al., 2004; Cooper et al., 2010; Tomotani et al., 2017) or occupy higher quality territories. Thus, the fitness consequences of male arrival time may (partly) arise through correlation with female timing, especially female breeding time, which is related to reproductive success and to local environmental conditions (Dunn and Winkler, 1999; Townsend et al., 2013; Visser et al., 2015; Tomotani et al., 2018; Shave et al., 2019).
For the purposes of this study, female timing can be seen solely as a part of the causal chain by which male arrival is linked to fitness. It is likely that the male fitness landscape at the breeding grounds is, at least, partly shaped by the timing of breeding of the females that are available at male arrival.
Disruption of Cues Due to Climate Change
Another potential reason for the lack of a correlation between the cues used for arrival date and their effects on the fitness peak date could be that cues have become unreliable due to climate change. The environmental variables that the birds use to time their arrival are certainly not the ones that determine the optimal arrival date, but they only correlate to these causal variables that determine the optimal arrival date (the drivers of selection). Climate change may have altered the correlation between the cues and causal variables, especially because climate change has not affected temperatures in a uniform manner across different regions and moments of the year (Easterling et al., 1997; Vose et al., 2005; Serreze and Francis, 2006). Climate change has also disrupted the relationship between climatic and weather variables at large spatial scales, so called “teleconnections” (Diaz Henry et al., 2002). Consequently, the cues used by the birds to time their migration may actually have become unreliable over time and therefore we now no longer find a correlation between the actual and the optimal arrival dates (Figure 2). Adaptation to climate change, in this case, would require a change in the bird sensitivity (slope of plasticity of arrival date) to the cues so that a correlation is re-established.
Conclusion
The lack of adjustment in timing of breeding and/or migration in some pied flycatcher populations but not in others (Both and Visser, 2001; Ahola et al., 2004; Both et al., 2016; Tomotani et al., 2018) reflect the need of detailed studies on the predictability and use of cues across populations. In the present study we show that the timing of arrival of male pied flycatchers is a plastic trait as it is affected by environmental variables. Our data suggest that, to assess the reliability of cues as predictors for fitness, it is perhaps not sufficient to only use breeding success as fitness measurement, since information on how females shape the fitness landscape may also be important. Alternatively, cues that used to be predictive for the drivers of selection may no longer be so now due to climate change. If this is the case, we would expect selection on the sensitivity to the cues and, if this sensitivity is heritable, pied flycatchers could adapt to this disruption of cues and drivers of selection. Therefore, a better understanding of the mortality related to migration timing is essential to distinguish between these scenarios, allowing a more complete evaluation of the impacts of climate change on migratory animals.
Data Availability Statement
The raw data supporting the conclusions of this article will be made available by the authors, without undue reservation.
Ethics Statement
The animal study was reviewed and approved by the Animal Experimental Committee of the Royal Netherlands Academy of Arts and Sciences (KNAW).
Author Contributions
BT, PG, IH, and MV: conceptualization. BT, PG, IH, FP, and MV: methodology. BT, IH, FP, and MT: investigation. BT and PG: formal analyses. BT and MV: data curation, project administration, and funding acquisition. BT, PG, and MV: writing – original draft. BT, PG, IH, MT, FP, and MV: writing – review and editing. PG and MV: supervision. All authors contributed to the article and approved the submitted version.
Funding
This study was supported by a doctoral grant from CNPq (Conselho Nacional de Desenvolvimento Científico e Tecnológico, Brazil) to BT (proc. # 237790/2012-2).
Conflict of Interest
The authors declare that the research was conducted in the absence of any commercial or financial relationships that could be construed as a potential conflict of interest.
Acknowledgments
We are grateful to the board of the National Park “De Hoge Veluwe” for the permission to conduct our research on their property. Chiel Boom and Liam Bailey for the assistance with obtaining and cleaning the climatic data. Finally, we thank numerous researchers, technicians, students, and volunteers who helped to collect and manage the detailed data on arrival and breeding of pied flycatchers.
Supplementary Material
The Supplementary Material for this article can be found online at: https://www.frontiersin.org/articles/10.3389/fevo.2021.630823/full#supplementary-material
References
Ahola, M., Laaksonen, T., Sippola, K., Eeva, T., Rainio, K., and Lehikoinen, E. (2004). Variation in climate warming along the migration route uncouples arrival and breeding dates. Glob. Change Biol. 10, 1610–1617. doi: 10.1111/j.1365-2486.2004.00823.x
Bates, D., Mächler, M., Bolker, B., and Walker, S. (2015). Fitting linear mixed-effects models using lme4. J. Stat. Softw. 67, 1–48.
Bauchau, V., and Seinen, I. (1997). Clutch desertion and re-nesting in pied flycatchers: an experiment with progressive clutch removal. Anim. Behav. 54, 153–161. doi: 10.1006/anbe.1996.0460
Bauer, S., Gienapp, P., and Madsen, J. (2008). The relevance of environmental conditions for departure decision changes en route in migrating geese. Ecology 89, 1953–1960. doi: 10.1890/07-1101.1
Bêty, J., Giroux, J.-F., and Gauthier, G. (2004). Individual variation in timing of migration: causes and reproductive consequences in greater snow geese (Anser caerulescens atlanticus). Behav. Ecol. Sociobiol. 57, 1–8. doi: 10.1007/s00265-004-0840-3
Both, C. (2010). Flexibility of timing of avian migration to climate change masked by environmental constraints en route. Curr. Biol. 20, 243–248. doi: 10.1016/j.cub.2009.11.074
Both, C., Bijlsma, R. G., and Ouwehand, J. (2016). Repeatability in spring arrival dates in pied flycatchers varies among years and sexes. Ardea 104, 3–21. doi: 10.5253/arde.v104i1.a1
Both, C., Bijlsma, R. G., and Visser, M. E. (2005). Climatic effects on timing of spring migration and breeding in a long-distance migrant, the pied flycatcher Ficedula hypoleuca. J. Avian Biol. 36, 368–373.
Both, C., Robinson, R. A., van der Jeugd, H., and Bensch, S. (2012). Long-distance dispersal in migratory pied flycatchers Ficedula hypoleuca is relatively common between the UK and the Netherlands. J. Avian Biol. 43, 193–197. doi: 10.1111/j.1600-048X.2012.05721.x
Both, C., Ubels, R., and Ravussin, P.-A. (2019). Life-history innovation to climate change: can single-brooded migrant birds become multiple breeders? J. Avian Biol. 50, 01951. doi: 10.1111/jav.01951
Both, C., and Visser, M. E. (2001). Adjustment to climate change is constrained by arrival date in a long-distance migrant bird. Nature 411, 296–298. doi: 10.1038/35077063
Brown, C. R., and Brown, M. B. (2000). Weather-Mediated natural selection on arrival time in cliff swallows (Petrochelidon pyrrhonota). Behav. Ecol. Sociobiol. 47, 339–345.
Canal, D., Jovani, R., and Potti, J. (2012). Multiple mating opportunities boost protandry in a pied flycatcher population. Behav. Ecol. Sociobiol. 66, 67–76. doi: 10.1007/s00265-011-1253-8
Cooper, N. W., Murphy, M. T., and Redmond, L. J. (2009). Age- and sex-dependent spring arrival dates of Eastern Kingbirds. J. Field Ornithol. 80, 35–41. doi: 10.1111/j.1557-9263.2009.00203.x
Cooper, N. W., Murphy, M. T., Redmond, L. J., and Dolan, A. C. (2010). Reproductive correlates of spring arrival date in the Eastern Kingbird Tyrannus tyrannus. J. Ornithol. 152, 143–152. doi: 10.1007/s10336-010-0559-z
Coppack, T., and Pulido, F. (2004). “Photoperiodic response and the adaptability of avian life cycles to environmental change,” in Birds and Climate Change, eds A. P. Moller, W. Fielder, and P. Berthold (Chicago, IL: Academic Press), 131–150.
Cotton, P. A. (2003). Avian migration phenology and global climate change. Proc. Natl. Acad. Sci. U.S.A. 100, 12219–12222. doi: 10.1073/pnas.1930548100
Cox, D. R. (1992). “Regression models and life-tables,” in Breakthroughs in Statistics, eds S. Kotz and N. L. Johnson (New York, NY: Springer), 527–541.
Dawson, A. (2002). Photoperiodic control of the annual cycle in birds and comparison with mammals. Ardea 90, 355–367.
Deppe, J. L., Ward, M. P., Bolus, R. T., Diehl, R. H., Celis-Murillo, A., Zenzal, T. J., et al. (2015). Fat, weather, and date affect migratory songbirds’ departure decisions, routes, and time it takes to cross the Gulf of Mexico. Proc. Natl. Acad. Sci. 112, E6331–E6338. doi: 10.1073/pnas.1503381112
Diaz Henry, F., Hoerling Martin, P., and Eischeid Jon, K. (2002). ENSO variability, teleconnections and climate change. Int. J. Climatol. 21, 1845–1862. doi: 10.1002/joc.631
Didan, K. (2015). MOD13C1 MODIS/Terra Vegetation Indices 16-Day L3 Global 0.05Deg CMG V006. 2015, distributed by NASA EOSDIS Land Processes DAAC. doi: 10.5067/MODIS/MOD13C1.006
Donohue, K., Dorn, L., Griffith, C., Kim, E., Aguilera, A., Polisetty, C. R., et al. (2005). The evolutionary ecology of seed germination of Arabidopsis thaliana: variable natural selection on germination timing. Evolution 59, 758–770. doi: 10.1111/j.0014-3820.2005.tb01751.x
Drost, R. (1936). Ueber das brutklei männlicher trauerfliegenfänger, muscicapa hypoleuca. nach untersuchungen an helgoländer zugvögeln und an nord- und mitteleuropäischen stücken. Vogelzug 7, 179–186.
Dunn, P. O., and Winkler, D. W. (1999). Climate change has affected the breeding date of tree swallows throughout North America. Proc. R. Soc. Lond. B Biol. Sci. 266, 2487–2490. doi: 10.1098/rspb.1999.0950
Easterling, D. R., Horton, B., Jones, P. D., Peterson, T. C., Karl, T. R., Parker, D. E., et al. (1997). Maximum and minimum temperature trends for the globe. Science 277, 364–367. doi: 10.1126/science.277.5324.364
Eikenaar, C., and Schmaljohann, H. (2014). Wind conditions experienced during the day predict nocturnal restlessness in a migratory songbird. IBIS 157, 125–132. doi: 10.1111/ibi.12210
Einum, S., and Fleming, I. A. (2000). Selection against late emergence and small offspring in atlantic salmon (Salmo salar). Evolution 54, 628–639. doi: 10.1111/j.0014-3820.2000.tb00064.x
Erni, B., Liechti, F., and Bruderer, B. (2005). The role of wind in passerine autumn migration between Europe and Africa. Behav. Ecol. 16, 732–740.
Fraser, K. C., Shave, A., de Greef, E., Siegrist, J., and Garroway, C. J. (2019). Individual variability in migration timing can explain long-term, population-level advances in a songbird. Front. Ecol. Evol. 7:324. doi: 10.3389/fevo.2019.00324
Gienapp, P., Hemerik, L., and Visser, M. E. (2005). A new statistical tool to predict phenology under climate change scenarios. Glob. Change Biol. 11, 600–606. doi: 10.1111/j.1365-2486.2005.00925.x
Gienapp, P., Leimu, R., and Merila, J. (2007). Responses to climate change in avian migration time–Microevolution versus phenotypic plasticity. Clim. Res. 35, 25–35.
Gienapp, P., Reed, T. E., and Visser, M. E. (2014). Why climate change will invariably alter selection pressures on phenology. Proc. R. Soc. Lond. B Biol. Sci. 281, 20141611. doi: 10.1098/rspb.2014.1611
Gordo, O. (2007). Why are bird migration dates shifting? A review of weather and climate effects on avian migratory phenology. Clim. Res. 35, 37–58. doi: 10.3354/cr00713
Gwinner, E. (1987). “Photoperiodic synchronization of circannual rhythms in gonadal activity,migratory restlessness, body weight, and molt in the garden warbler, Sylvia borin,” in Comparative Physiology of Environmental Adaptations, ed. P. Pévet (Basel: Karger), 30–44.
Gwinner, E. (1989a). Einfluß der photoperiode auf das circannuale system des halsbandschnäppers (Ficedula albicollis) und des Trauerschnäppers (F. hypoleuca). J. Für Ornithol. 130, 1–13. doi: 10.1007/BF01647157
Gwinner, E. (1989b). Photoperiod as a modifying and limiting factor in the expression of avian circannual rhythms. J. Biol. Rhythms 4, 125–138. doi: 10.1177/074873048900400210
Gwinner, E. (1996). Circannual clocks in avian reproduction and migration. IBIS 138, 47–63. doi: 10.1111/j.1474-919X.1996.tb04312.x
Haest, B., Hüppop, O., and Bairlein, F. (2018). The influence of weather on avian spring migration phenology: what, where, and when? Glob. Change Biol. 24, 5769–5788. doi: 10.1111/gcb.14450
Halekoh, U., and Højsgaard, S. (2014). A kenward-roger approximation and parametric bootstrap methods for tests in linear mixed models - the r package pbkrtest. J. Stat. Softw. 59, 1–30.
Hüppop, O., and Hüppop, K. (2003). North Atlantic Oscillation and timing of spring migration in birds. Proc. R. Soc. Lond. B Biol. Sci. 270, 233–240. doi: 10.1098/rspb.2002.2236
Hüppop, O., and Winkel, W. (2006). Climate change and timing of spring migration in the long-distance migrant Ficedula hypoleuca in central Europe: the role of spatially different temperature changes along migration routes. J. Ornithol. 147, 344–353. doi: 10.1007/s10336-005-0049-x
Ivankina, E. V., Grinkov, V. G., and Kerimov, A. B. (2001). Male colour type and lifetime breeding success in the pied flycatcher Ficedula hypoleuca. Acta Ornithol. 36, 91–96. doi: 10.3161/068.036.0105
Ivankina, E. V., Kerimov, A. B., Grin’kov, V. G., and Bushuev, A. V. (2007). Structural and functional aspects of variation of the breeding plumage ornamentation in the male Pied Flycatcher Ficedula hypoleuca (Aves: passeriformes). Zh. Obshch. Biol. 68, 278–295.
Jenni, L., and Kery, M. (2003). Timing of autumn bird migration under climate change: advances in long-distance migrants, delays in short-distance migrants. Proc. R. Soc. B Biol. Sci. 270, 1467–1471. doi: 10.1098/rspb.2003.2394
Jonzén, N., Hedenström, A., and Lundberg, P. (2007). Climate change and the optimal arrival of migratory birds. Proc. R. Soc. Lond. B Biol. Sci. 274, 269–274. doi: 10.1098/rspb.2006.3719
Kelly, M. G., and Levin, D. A. (1997). Fitness consequences and heritability aspects of emergence date in Phlox drummondii. J. Ecol. 85, 755–766. doi: 10.2307/2960599
Kerimov, A. B., Rogovin, K. A., Ivankina, E. V., Bushuev, A. V., Sokolova, O. V., and Ilyina, T. A. (2013). Specific immunity and polymorphism of breeding plumage in pied flycatcher (Ficedula hypoleuca) males (Aves: Passeriformes). Biol. Bull. Rev. 3, 232–240. doi: 10.1134/S2079086413030067
Klaassen, R. H. G., Hake, M., Strandberg, R., Koks, B. J., Trierweiler, C., Exo, K., et al. (2013). When and where does mortality occur in migratory birds? Direct evidence from long−term satellite tracking of raptors. J. Anim. Ecol. 83, 176–184. doi: 10.1111/1365-2656.12135
Koenig, W. D., Funk, K. A., Kraft, T. S., Carmen, W. J., Barringer, B. C., and Knops, J. M. H. (2012). Stabilizing selection for within-season flowering phenology confirms pollen limitation in a wind-pollinated tree. J. Ecol. 100, 758–763. doi: 10.1111/j.1365-2745.2011.01941.x
Lehikoinen, E. S. A., Sparks, T. H., and Zalakevicius, M. (2004). Arrival and departure dates. Adv. Ecol. Res. 35, 1–31.
Leyrer, J., Lok, T., Brugge, M., Spaans, B., Sandercock, B. K., and Piersma, T. (2013). Mortality within the annual cycle: seasonal survival patterns in afro-siberian red knots Calidris canutus canutus. J. Ornithol. 154, 933–943. doi: 10.1007/s10336-013-0959-y
Lof, M. E., Reed, T. E., McNamara, J. M., and Visser, M. E. (2012). Timing in a fluctuating environment: environmental variability and asymmetric fitness curves can lead to adaptively mismatched avian reproduction. Proc. R. Soc. Lond. B Biol. Sci. 279, 3161–3169. doi: 10.1098/rspb.2012.0431
Lok, T., Overdijk, O., and Piersma, T. (2015). The cost of migration: spoonbills suffer higher mortality during trans-Saharan spring migrations only. Biol. Lett. 11:20140944. doi: 10.1098/rsbl.2014.0944
Marra, P. P., Francis, C. M., Mulvihill, R. S., and Moore, F. R. (2005). The influence of climate on the timing and rate of spring bird migration. Oecologia 142, 307–315. doi: 10.1007/s00442-004-1725-x
Mayor, S. J., Guralnick, R. P., Tingley, M. W., Otegui, J., Withey, J. C., Elmendorf, S. C., et al. (2017). Increasing phenological asynchrony between spring green-up and arrival of migratory birds. Sci. Rep. 7:1902. doi: 10.1038/s41598-017-02045-z
Newton, I. (2007). Weather−related mass−mortality events in migrants. IBIS 149, 453–467. doi: 10.1111/j.1474-919X.2007.00704.x
Noordwijk, A. J., McCleery, R. H., and Perrins, C. M. (1995). Selection for the timing of great tit breeding in relation to caterpillar growth and temperature. J. Anim. Ecol. 64, 451–458. doi: 10.2307/5648
Noordwijk, A. J., and Müller, C. B. (1994). “On adaptive plasticity in reproductive traits, illustrated with lay date in the great tit and colony inception in a bumble bee,” in Animal Societies Individuals, Interactions and Organization, eds A. Rossiter and P. J. Jarman (Kyoto: Kyoto University Press), 180–194.
Norris, D. R., Marra, P. P., Kyser, T. K., Sherry, T. W., and Ratcliffe, L. M. (2004). Tropical winter habitat limits reproductive success on the temperate breeding grounds in a migratory bird. Proc. R. Soc. Lond. B Biol. Sci. 271, 59–64. doi: 10.1098/rspb.2003.2569
Ouwehand, J., Ahola, M. P., Ausems, A. N. M. A., Bridge, E. S., Burgess, M., Hahn, S., et al. (2016). Light-level geolocators reveal migratory connectivity in European populations of pied flycatchers Ficedula hypoleuca. J. Avian Biol. 47, 69–83. doi: 10.1111/jav.00721
Ouwehand, J., and Both, C. (2017). African departure rather than migration speed determines variation in spring arrival in pied flycatchers. J. Anim. Ecol. 86, 88–97. doi: 10.1111/1365-2656.12599
Pallas, P. S. (1764). Adumbratiunculae Avium Variarum Praecedenti Elencho Insertarum, Sed Quae in Systemate Naturae Illustl: Linnaei nondum extant (The Hague: Pieter van Os), 1–7.
Perrins, C. M. (1970). The timing of birds‘ breeding seasons. IBIS 112, 242–255. doi: 10.1111/j.1474-919X.1970.tb00096.x
Philippart, C. J. M., van Aken, H. M., Beukema, J. J., Bos, O. G., Cadée, G. C., and Dekker, R. (2003). Climate-related changes in recruitment of the bivalve Macoma balthica. Limnol. Oceanogr. 48, 2171–2185. doi: 10.4319/lo.2003.48.6.2171
Potti, J. (1998). Arrival time from spring migration in male pied flycatchers: individual consistency and familial resemblance. Condor 100, 702–708. doi: 10.2307/1369752
Pulido, F. (2007). Phenotypic changes in spring arrival: evolution, phenotypic plasticity, effects of weather and condition. Clim. Res. 35, 5–23.
R Core Team (2017). R: A Language and Environment for Statistical Computing. Vienna: R Foundation for Statistical Computing.
Rotics, S., Kaatz, M., Resheff, Y. S., Turjeman, S. F., Zurell, D., Sapir, N., et al. (2016). The challenges of the first migration: movement and behaviour of juvenile vs. adult white storks with insights regarding juvenile mortality. J. Anim. Ecol. 85, 938–947. doi: 10.1111/1365-2656.12525
Saino, N., and Ambrosini, R. (2008). Climatic connectivity between Africa and Europe may serve as a basis for phenotypic adjustment of migration schedules of trans-Saharan migratory birds. Glob. Change Biol. 14, 250–263. doi: 10.1111/j.1365-2486.2007.01488.x
Saino, N., Rubolini, D., Jonzen, N., Ergon, T., Montemaggiori, A., Stenseth, N. C., et al. (2007). Temperature and rainfall anomalies in Africa predict timing of spring migration in trans-Saharan migratory birds. Clim. Res. 35, 123–134. doi: 10.3354/cr00719
Saino, N., Szép, T., Romano, M., Rubolini, D., Spina, F., and Møller, A. P. (2004). Ecological conditions during winter predict arrival date at the breeding quarters in a trans-Saharan migratory bird. Ecol. Lett. 7, 21–25. doi: 10.1046/j.1461-0248.2003.00553.x
Serreze, M. C., and Francis, J. A. (2006). The arctic amplification debate. Clim. Change 76, 241–264. doi: 10.1007/s10584-005-9017-y
Shave, A., Garroway, C. J., Siegrist, J., and Fraser, K. C. (2019). Timing to temperature: egg-laying dates respond to temperature and are under stronger selection at northern latitudes. Ecosphere 10:e02974. doi: 10.1002/ecs2.2974
Sillett, T. S., and Holmes, R. T. (2002). Variation in survivorship of a migratory songbird throughout its annual cycle. J. Anim. Ecol. 71, 296–308. doi: 10.1046/j.1365-2656.2002.00599.x
Smith, R. J., and Moore, F. R. (2005). Arrival timing and seasonal reproductive performance in a long-distance migratory landbird. Behav. Ecol. Sociobiol. 57, 231–239. doi: 10.1007/s00265-004-0855-9
Stocker, T. F., Qin, D., Plattner, G. K., Tignor, M., Allen, S. K., Boschung, J., et al. (2013). IPCC, 2013: Climate Change 2013: The Physical Science Basis. Contribution of Working Group I to The Fifth Assessment Report of the Intergovernmental Panel on Climate Change. Cambridge: Cambridge University Press.
Studds, C. E., and Marra, P. P. (2011). Rainfall-induced changes in food availability modify the spring departure programme of a migratory bird. Proc R Soc B 278, 3437–3443. doi: 10.1098/rspb.2011.0332
Therneau, T. M. (2015). A Package for Survival Analysis in S. Available Online at: https://cran.r-project.org/web/packages/survival/index.html (accessed February 25, 2016).
Tomotani, B. M., Caglar, E., de la Hera, I., Mateman, A. C., and Visser, M. E. (2017). Early arrival is not associated with more extra-pair fertilizations in a long-distance migratory bird. J. Avian Biol. 48, 854–861. doi: 10.1111/jav.01317
Tomotani, B. M., de la Hera, I., Lange, C. Y. M. J. G., van Lith, B., Meddle, S. L., Both, C., et al. (2019). Timing manipulations reveal the lack of a causal link across timing of annual-cycle stages in a long-distance migrant. J. Exp. Biol. 222:jeb201467. doi: 10.1242/jeb.201467
Tomotani, B. M., Gienapp, P., Beersma, D. G. M., and Visser, M. E. (2016). Climate change relaxes the time constraints for late-born offspring in a long-distance migrant. Proc. R. Soc. Lond. B Biol. Sci. 283:20161366. doi: 10.1098/rspb.2016.1366
Tomotani, B. M., van der Jeugd, H., Gienapp, P., de la Hera, I., Pilzecker, J., Teichmann, C., et al. (2018). Climate change leads to differential shifts in the timing of annual cycle stages in a migratory bird. Glob. Change Biol. 24, 823–835. doi: 10.1111/gcb.14006
Tøttrup, A. P., Klaassen, R. H. G., Kristensen, M. W., Strandberg, R., Vardanis, Y., and Lindström, Å, et al. (2012). Drought in Africa caused delayed arrival of european songbirds. Science 338, 1307–1307. doi: 10.1126/science.1227548
Tøttrup, A. P., Rainio, K., Coppack, T., Lehikoinen, E., Rahbek, C., and Thorup, K. (2010). Local temperature fine-tunes the timing of spring migration in birds. Integr. Comp. Biol. 50, 293–304. doi: 10.1093/icb/icq028
Townsend, A. K., Sillett, T. S., Lany, N. K., Kaiser, S. A., Rodenhouse, N. L., Webster, M. S., et al. (2013). Warm springs, early lay dates, and double brooding in a North American migratory songbird, the black-throated blue warbler. PLoS One 8:e59467. doi: 10.1371/journal.pone.0059467
Usui, T., Butchart, S. H. M., and Phillimore, A. B. (2016). Temporal shifts and temperature sensitivity of avian spring migratory phenology: a phylogenetic meta-analysis. J. Anim. Ecol. 82, 250–261. doi: 10.1111/1365-2656.12612
Visser, M. E. (2008). Keeping up with a warming world; assessing the rate of adaptation to climate change. Proc. R. Soc. Lond. B Biol. Sci. 275, 649–659. doi: 10.1098/rspb.2007.0997
Visser, M. E. (2013). “Phenological shifts in animals under contemporary climate change,” in Encyclopedia of Biodiversity, ed. S. A. Levin (Amsterdam: Elsevier), 716–727.
Visser, M. E., and Both, C. (2005). Shifts in phenology due to global climate change: the need for a yardstick. Proc. R. Soc. Lond. B Biol. Sci. 272, 2561–2569. doi: 10.1098/rspb.2005.3356
Visser, M. E., Both, C., and Lambrechts, M. M. (2004). Global climate change leads to mistimed avian reproduction. Adv. Ecol. Res. 35, 89–110.
Visser, M. E., Caro, S. P., van Oers, K., Schaper, S. V., and Helm, B. (2010). Phenology, seasonal timing and circannual rhythms: towards a unified framework. Philos. Trans. R. Soc. Lond. B Biol. Sci. 365, 3113–3127. doi: 10.1098/rstb.2010.0111
Visser, M. E., Gienapp, P., Husby, A., Morrisey, M., de la Hera, I., Pulido, F., et al. (2015). Effects of spring temperatures on the strength of selection on timing of reproduction in a long-distance migratory bird. PLoS Biol. 13:e1002120. doi: 10.1371/journal.pbio.1002120
Visser, M. E., Holleman, L. J. M., and Gienapp, P. (2006). Shifts in caterpillar biomass phenology due to climate change and its impact on the breeding biology of an insectivorous bird. Oecologia 147, 164–172. doi: 10.1007/s00442-005-0299-6
Vose, R. S., Easterling, D. R., and Gleason, B. (2005). Maximum and minimum temperature trends for the globe: an update through 2004. Geophys. Res. Lett. 32:L23822. doi: 10.1029/2005GL024379
Keywords: cues, mortality, climate change, Ficedula hypoleuca, long-distance migration, Africa, European pied flycatcher
Citation: Tomotani BM, Gienapp P, de la Hera I, Terpstra M, Pulido F and Visser ME (2021) Integrating Causal and Evolutionary Analysis of Life-History Evolution: Arrival Date in a Long-Distant Migrant. Front. Ecol. Evol. 9:630823. doi: 10.3389/fevo.2021.630823
Received: 18 November 2020; Accepted: 01 February 2021;
Published: 22 February 2021.
Edited by:
Piotr Jablonski, Seoul National University, South KoreaReviewed by:
Piotr Matyjasiak, Cardinal Stefan Wyszyński University, PolandKevin C. Fraser, University of Manitoba, Canada
Copyright © 2021 Tomotani, Gienapp, de la Hera, Terpstra, Pulido and Visser. This is an open-access article distributed under the terms of the Creative Commons Attribution License (CC BY). The use, distribution or reproduction in other forums is permitted, provided the original author(s) and the copyright owner(s) are credited and that the original publication in this journal is cited, in accordance with accepted academic practice. No use, distribution or reproduction is permitted which does not comply with these terms.
*Correspondence: Barbara M. Tomotani, Yi50b21vdGFuaUBuaW9vLmtuYXcubmw=