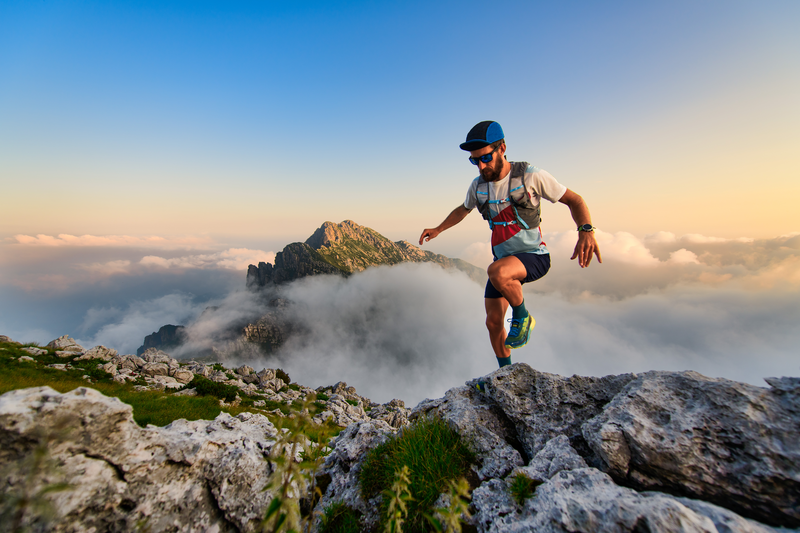
94% of researchers rate our articles as excellent or good
Learn more about the work of our research integrity team to safeguard the quality of each article we publish.
Find out more
REVIEW article
Front. Ecol. Evol. , 16 March 2021
Sec. Urban Ecology
Volume 9 - 2021 | https://doi.org/10.3389/fevo.2021.620620
This article is part of the Research Topic Historical Legacies of Land Use in Cities; Parks, Open Spaces and Potential for Green Infrastructure- Ideas of City Nature in an Urbanizing Planet View all 10 articles
Cities and towns are complex ecosystems with features that can vary dramatically in space and time. Our knowledge of the spatial structure of urban land and ecological systems is expanding. These systems have been investigated across spatial scales, urban to rural gradients, networks of urban macrosystems, and global megalopolises. However, the temporal dimensions of urban ecosystems – such as those related to ecological cycles and historical legacies – are far less understood and investigated. Here, we outline the main dimensions of time that can shape how events in urban ecosystems unfold, which we categorize as: (i) time flows and duration, (ii) synchrony, lags, and delays, (iii) trends and transitions, (iv) cycles and hysteresis, (v) legacies and priming, (vi) temporal hotspots and hot moments, and (vii) stochastic vs. deterministic processes affecting our ability to forecast the future of cities and the species that live in them. First, we demonstrate the roles of these understudied dimensions by discussing exemplary studies. We then propose key future research directions for investigating how processes over time may regulate the structure and functioning of urban land and biodiversity, as well as its effects on and implications for urban ecology. Our analysis and conceptual framework highlights that several temporal dimensions of urban ecosystems – like those related to temporal hotspots/moments and stochastic vs. deterministic processes – are understudied. This offers important research opportunities to further urban ecology and a comprehensive research agenda valuing the “Urban Chronos” – the change of urban ecosystems through time.
Cities are intrinsic spatial systems, wherein variation in ecological structure and function across small spatial scales is easily observed. As a result, it is no surprise that the spatial nature of cities is the dominant foundation for contemporary urban scholars and practitioners. Cities have been investigated extensively across diverse spatial scales – from local studies of habitat patches, observations across rural to urban developments, to surveys of global urban networks (Gilbert, 1991; McDonnell et al., 1997; Seto et al., 2012; Groffman et al., 2017). Assessments of urban morphologies have a long tradition grounded in ancient cartographic and cadastral records, now translated into modern geographic information systems, with help from big data and remote sensing. With these tools in hand, a large body of research now highlights the extent to which urban ecosystems vary across space, including complex effects of spatial variation on ecological and other facets of cities (Beninde et al., 2015; Ossola et al., 2020).
Most processes within cities – such as urbanization, regeneration, redevelopment, abandonment, densification, and transformation – are spatially structured, but these processes also happen over time. Temporality is often included in conceptual models of the dynamics of urban ecosystems, yet empirical studies rarely quantify changes over time in, e.g., the forces structuring urban biodiversity or land systems over decades to centuries (Marcus and Colding, 2014; Pickett et al., 2017). This general pattern may be due to a lack of robust, standardized data describing events and processes over the period of anthropogenic change. This lack of data has real consequences; in cities, decisions made in one decade can affect future generations of both humans and other organisms. Thus, understanding cities in a temporal context is critical for urban ecological research, practice and management.
Recent efforts toward scenario-based urban planning highlight our limited ability to anticipate the needs of future urban societies (Malekpour et al., 2015). Similarly, strategic urban planning and governance are often little informed by a solid understanding of the urban past (Howlett and Goetz, 2014; Malekpour et al., 2015). How cities are managed remains flawed by implicit assumptions that urban land is a largely static entity, unlikely to change significantly over short time scales, such as that of a single human lifespan or that of a few generations. It is no surprise that space, not time, is still considered the main driver governing cities (Marcus et al., 2019). As put by Davis (2019) in the context of regeneration studies “this reflects a broader tendency […] to emphasize the physical and spatial aspects of planned change at the expense of the temporal aspects of transformation.”
Due to these limitations, the temporal effects of urban change on the ecological facets of cities have been poorly quantified or neglected tout court. Like natural systems (Wolkovich et al., 2014; Zelnik et al., 2018), the temporal dimensions and dynamics of urban land are most likely to affect their intrinsic functioning, sustainability, and resilience (Ramalho and Hobbs, 2012). For instance, the signatures of urban and rural past can be found in the very soil where modern cities sit; silent legacies of previous land use and change (Clarke et al., 2015). Time represents an important, yet poorly understood, factor driving all urban events and their dynamics, dictating the pace and direction of change and ultimately affecting the ecology of future urban ecosystems. This is particularly relevant when considering the complex interactions between the temporal and spatial nature of cities and how their interplay can ultimately their ecology (Pickett et al., 2017).
Here, we propose several research directions to guide the investigation of time as a driver regulating structure and functioning of urban ecosystems, as well as its implications for urban ecological research. Selected studies are discussed to highlight critical knowledge gaps and opportunities related to the multiple dimensions of the “Urban Chronos,” as we define, the change of urban ecosystems through time. In doing so, we lay out a conceptual framework to move this rich and under-developed aspect of urban exploration forward.
In each section below, we first define and describe the main temporal dimensions of urban ecosystems (Figure 1), and key research that exemplifies each dimension. In identifying past research from various fields, we focus on ecological processes and functions – the connections and interactions between biotic and abiotic ecosystem elements that regulates fluxes of energy and matter and the provision of ecosystem services. In doing so, we highlight their interrelationships with other human and technical elements, such as urban planning and management. We then propose key overarching questions related to these temporal dimensions and highlight opportunities for future investigation building upon existing scholarship and practice. For the sake of clarity, we discuss each temporal dimension separately, but we recognize that they can overlap and interact with each other, as well as with the spatial dimensions of urban ecosystems. We avoid proposing a classification of these temporal dimensions, but rather we aim to delineate the main characteristics that can affect structure and functioning of urban ecosystems though the targeted analysis of key ecological literature. In addition, we recognize that some examples could fit more than one temporal dimension or other temporal processes not discussed here.
Figure 1. Exemplification of temporal dimensions, processes and questions related to urban time and its dynamics. Different letters represent different events and are conceptualized as fast (capital letters) and slow-occurring events (lower case letters). Gray arrows between events represent time flows and direction.
Like space, time is affected by significant autocorrelation, whereby a given event at a particular point in time can be affected by other events. Unlike space, however, time has a single directionality, and no change in direction is possible (Wolkovich et al., 2014). Time is a defined dimension where a series of chronological events – or processes – can only develop from past to present to future following a particular series (i.e., time flow). Each of these events develop over a specific length of time (i.e., duration).
Sequences of events might happen in the same order in different places, but the duration of these events may vary with important implications for urban systems. For example, a neighborhood at a specific location could be developed in a mere matter of months, whereas a similar real estate development elsewhere could take years to be built, if not decades. These different time flows can have important consequences on ecological systems and urban organisms, for instance when trying to adapt to long-lasting, chronic vs. short-term, acute anthropogenic disturbances (e.g., noise, pollution) (Grimm et al., 2017). Similarly, relatively small difference in the duration of storms, having similar rainfall intensity and yearly re-occurrence frequency, can determine whether these weather events can generate superficial runoff or not across urban green spaces (Ossola et al., 2015).
Urban change – the flow of homologous events in an urban system – can be ranked from slow to fast (Figure 1). For instance, little evidence exists on how past planting of tree species with different growth rates might have contributed to different levels of canopy cover across modern urban landscapes (Roman et al., 2018; Smith et al., 2019). Planting tree species with different ages or lifespans could be linked to greater urban forest turnover (Figures 2A,B). Similarly, the use of different tree species with a wide range of safe useful life expectancies [SULEs, i.e., the maximum horticultural duration of a tree stem beyond which maintenance costs exceeds replacement costs, Barrell (1993)] can determine how urban forests change over time under human management.
Figure 2. Example of tree planting interventions using trees of different size and age. (A) Tree saplings ready to be planted in a residential development in Melbourne, VIC, Australia (Author provided) and (B) planting of adult “recycled” palm trees in San Francisco, CA, United States (Courtesy: Bertrand Duperrin, Flicker). Despite streetscapes being redeveloped around the same time, these urban green spaces might have a different vegetation structure due to the different age of the trees planted. Example of an urban forest growth over seven decades. The Tiergarten in Berlin, Germany, in 1945 at the end of WWII (C) was used as a large urban farm to sustain the city’s starving population, with only a few scattered trees left standing (Courtesy: Landesarchiv Berlin). Today, Tiergarten Park (D) is a dense urban forest enjoyed by Berlin’s residents for leisure and sport activities with little evidence of its wartime past (Courtesy: Savin A., Wikimedia Commons).
The different duration of urban events and time flows may affect many aspects of urban ecosystems, raising numerous opportunities for research and practice. One might well ask “what is the effect of having similar events happening at a slower or faster pace?,” “is the rate of urban events related to their overall duration?,” and “to what extent events in urban ecosystems differ based on different time frames considered; i.e., what is the effect of temporal scales on urban ecosystems and ecology?”
Urban events can be conceptualized as happening at the same absolute time (i.e., synchronous events) or being separated in time (i.e., asynchronous events). (A)synchrony can affect all urban events regardless of their codependency (e.g., flower-pollinator systems), causation (e.g., urban heat islands and early season flowering) or independency. Perfect synchrony means that the initiation, development and termination of two or more urban events happen at the same time (Figure 1). Once historically coupled events are out of synchrony, a temporal mismatch might occur, potentially leading to the disruption of previously coupled events. For example, budburst and flowering are advanced by days to weeks in urban areas compared to surrounding rural areas due to urban areas being warmer (Neil and Wu, 2006). The cascading effects of this asynchrony on plant-animal interactions are largely uncharacterized. In a rare example along these lines, Fisogni et al. (2020) recently observed that urbanization advanced the timing of flowering in France, but not pollinator flight, potentially leading to disrupted pollination in cities. In another example, ecological theory predicts that higher trophic levels may become less synchronized due to the urban heat island effect, disrupting ecosystem services, such as biological pest control. This was observed for a scale insect pest of oak trees and its suite of parasitoid wasps in Raleigh, NC, United States, where urban warming shortened the vulnerable development period for the scale insect, and parasitoid development did not similarly advance (Meineke et al., 2014).
Generally, the potential decoupling of plant relationships with co-evolved species in urban areas (e.g., pollinators, insect herbivores, and soil microorganisms) remains underexplored. Such asynchronies have been investigated in relation to climate change (Kharouba et al., 2018), but evidence regarding urban change is scant. Similarly, while the effects of pesticide application on urban insects are relatively well-known (Botías et al., 2017), no conclusive evidence exists on how the different timing of chemical (re)application across urban ecosystems might affect insect communities over time. For example, in residential landscapes, timing of application of yard chemicals represents a complex patchwork based on individual choices of residents that, due to limited biological knowledge, might be out-of-sync with targeted phenological phases, or in sync with plant flowering, thus endangering already threatened species.
Time lag analyses on drivers structuring biological communities and their diversity – as regulated by dispersal barriers, trophic interactions, parasitism, life history, etc. – are commonplace in natural ecosystem investigations (Kampichler and van der Jeugd, 2013). In cities, however, these investigations are more limited. For instance, time lags were found when analyzing global urban biodiversity patterns and extinction debts (Hahs et al., 2009), and in South African urban grasslands, du Toit et al. (2016) found that time lags in the compositional change of communities were longer for indigenous woody grassland species than open grassland species. Moreover, evidence on urban time lags and the cascading effects on urban ecosystem function, recovery from stress and perturbations, and resilience is scant. This is an important data gap because evidence from non-urban systems demonstrates ecological time lags can determine the success of ecological management and conservation efforts (Watts et al., 2020). For instance, in road verges, multi-decadal time lags have been identified when investigating community assembly, thus posing new challenges when designing appropriate urban restoration and conservation efforts (Auffret and Lindgren, 2020). More detailed knowledge is needed on the effects of time lags and delays on urban biodiversity and ecological functioning, and how they could underpin effective urban planning and management interventions.
Temporal coupling of urban ecosystems leads to little explored research questions such as “what happens when interdependent events, that are supposed to occur at the same time, are out of sync?,” “if the events are out of sync, how far out of sync can they be and still retain the functioning of urban ecosystems?,” “can asynchrony be compounded and determine cascading effects?,” “to what extent can time lags and delays change over time affecting structure or functioning of urban ecosystems?”
In general, urban events may appear static and semi-stationary (i.e., have a null rate of change), particularly if the temporal scale of the event does not align with the temporal scale of observations. The transition between events may be slow and progressive, and thus unnoticeable if only observed during a short window of time during the transition (Figures 2, 3). However, when considering a longer temporal scale, urban properties can noticeably change, revealing clear temporal trends. In other words, urban systems that appear stationary at a particular time scale, might be non-stationary on a different time scale. For instance, neighborhoods across Detroit, MI, United States in the early 20th century appeared stable in population and housing density (Figure 3). However, because of a protracted event such as its slow economic decline, these areas saw a progressive downward trend in population and housing density, pushing the urban system into a non-stationary state that ultimately led to a much lower housing and population density, and, concomitantly a higher green space cover today. On the other hand, Joplin, MO, United States saw an abrupt drop in housing and population density because of a tornado that obliterated parts of the city in 2011 (Figure 3). While Joplin’s urban ecosystem bounced back to a state comparable to that existing prior the tornado disaster, the downward trend in Detroit’s status caused the city to reach a new dynamic equilibrium significantly different from the initial one (Figure 3). It is worth noting here that some trends can happen at spatial scales much larger than that of a city or a neighborhood and be determined by exogenous forces, such as climate, macro-economic trends, and human drivers, that reach well beyond the single urban area investigated (Figure 4A).
Figure 3. Examples of urban land system change (i.e., trends) following a catastrophic event in Joplin, MO, United States (above orange panel and line), after part of the city was obliterated by a category 5 tornado on May 22nd 2011, and following a chronic and slower change in Detroit, MI, United States caused by the decline of the local automotive industry (below in blue). While Joplin was quickly rebuilt with similar urban form, housing and population density, achieving a new equilibrium similar to that prior to the tornado, Detroit plateaued to a new urban equilibrium significantly different from the original with lower housing and population density, albeit higher green and open space cover. Imagery is courtesy of https://digital.library.wayne.edu (years 1949 and 1981) and www.google.com/earth/ (years, 2006, 2011, 2017, and 2018, accessed on 15 February 2020).
Figure 4. (A) Historical trends (1965–2017) in owner and renter residential occupancy and vacancy rate across the entire United States (in gray, blue, and orange, respectively). Ownership rapidly increased between 1995 and 2005 to then return to the original level in the decade after the global financial crisis in 2007–2008. The trend in new residential constructions in the United States (dashed line), however, had a cyclical regime (drawn with data from https://www.census.gov/econ/currentdata/, accessed on 15 February 2020). (B) Relationship between current residential ownership and tree canopy cover measured across 1,709 United States neighborhoods (i.e., census tracts) and calculated based on vegetation data measured in 1,482,206 residential properties in nine cities and 2010 United States Census data (re-drawn from data in Ossola and Hopton, 2018a).
Attempts to answer questions related to trends, rates of event change and transitions could employ historical data on urban ecosystems as well as emerging big data (Ossola et al., 2020). For instance, the use of natural history collections – herbaria and animal specimens – could help clarify the effects of urbanization on urban biodiversity, its change over time (e.g., species turnover), and the ability of organisms to survive new urban conditions within particular time periods through tolerance, plasticity, and/or adaptation (Shultz et al., 2020), and even evolution of new urban phenotypes and genotypes (Rivkin et al., 2019). Future studies could test whether some organisms may tolerate a relatively slow change to their urban habitat, but not a fast one, likewise some organisms might be able to adjust to the “press” of climate trends (i.e., acclimation) but not to the “pulse” of climate extremes (i.e., adaptation) (Harris et al., 2018).
Investigating trends in urban systems could be organized by questions like “what is the effect of having a progressive versus abrupt change to urban ecosystems?,” and further “do urban ecosystems return to prior states after a tipping point or become temporarily or permanently shifted to a different state [e.g., legacy lock-ins sensu Ziter et al. (2017)]?” Ultimately, “can transitions between events be managed or regulated?”
Urban cycles capture a series of events that repeatedly lead to comparable results. Hysteresis manifests when two events reoccur following different pathways, thus representing the simplest type of cycle (Figure 1). It is important to note that these recurrent points in time might not be the exact manifestation of a particular urban past, but rather the realization of a similar status within a particular urban facet, process or function. For instance, considering the United States real estate market, new residential constructions in the last six decades demonstrated a cyclical pattern. Each year the number of new units fluctuated between 12,000 and 25,000 new units/year. This cyclic pattern was disrupted by the 2008 financial crisis (Figure 4A). The cyclical nature of urbanization trends, land development and real estate markets is a common feature of most urban areas (Riggleman, 1933; Nyström, 1992; Gabrovski and Ortego-Marti, 2019). This reflects macrotrends in dynamics affecting urban land systems (Figure 4B) that have been captured in some urban planning theories, such as the “neighborhood life-cycle theory” in the first half of the 20th century (Metzger, 2000) and more recently the “adaptive renewal cycle” framework (Marcus and Colding, 2014) and the concept of “rotating land-use” (Davis, 2019), though their implications and impacts on urban systems are yet to be fully explored. Cyclicity is a recurrent characteristic affecting many facets of urban ecosystems like those related to climate seasonality (e.g., wet/dry seasons), sunlight availability (e.g., day–night year cycle), and recurrence of human actions and disturbance (e.g., bird feeding, mowing, pruning).
Evidence of effects of urban cycles has been documented for some organisms. For instance, altered mowing regimes can shift the composition of plant communities and insect assemblages predictably, such that reduced mowing frequency generally benefits plant and insect diversity (Sehrt et al., 2020; Watson et al., 2020). Human perceptions of outdoors organisms and the urban environment, as measured by people’s online search patterns, is strongly affected by seasons (Figure 5), as is human perceptions of colors, in mid- to high-latitude cities (Welbourne et al., 2015). This raises opportunities to deepen our knowledge on nature-human interactions and their change over time, seasons and years. Like other temporal dimensions discussed here, it is important to note that cycles in one urban ecosystem might have complex interactions with cycles of other urban systems, often connected through globalized networks of energy and materials, as well as those of natural systems.
Figure 5. (A) Example of cyclicity in urban ecosystems showing people’s online search interest for selected insect groups (solid lines) in relation to seasons (i.e., temperature dotted black line) across the conterminous United States from 2004 to 2016. People’s interest is measured through the normalized percent interest of Google searches on the insect name as reported by Google Trends (accessed November 2016). Temperature is the mean monthly temperature for the conterminous United States (accessed from https://www.ncdc.noaa.gov/cag/time-series/us/on November 2016). (B) Maps showing the United States cities with the greatest online search interest for each insect group for the 2004–2016 reference period.
Attempts to investigate urban cycles could focus on questions such as “what happens to an urban ecosystem when an existing cycle is interrupted or a new cycle is formed?,” “does the number of cyclical events affect properties and functioning of urban ecosystems and to what extent?,” and again “what is the effect of two looped events happening in a different trajectory (i.e., hysteresis)?”
Temporal legacies are the manifestation of temporal autocorrelation within and across urban ecosystems. Legacies can be thought of as carry-over effects upon subsequent events where urban ecosystems can change while retaining some manifestations of prior events (Figure 1). Due to their long-term nature, legacies can often be confounded with urban events having relatively long durations and as such can be identified by examples previously discussed (see section “Time Flow and Duration”). Here, however, we consider as legacies past, fully completed events that affect future consecutive and non-consecutive events (i.e., “time-skipping”). Urban legacies can form within and across urban systems and processes. For instance, planting of a particular species might inhibit other plant species with similar traits from existing in the same system in the future through allelopathic processes (Tabassum et al., 2020). In this example, temporal autocorrelation is self-directed within a single ecological system. On the other hand, the selection of tree species to shade urban landscapes might provide tangible benefits to the built environment only years or decades later, thus causing temporal autocorrelation between an ecological and a technical system.
Among the existing evidence on the effects of urban time, legacies are perhaps the most commonly studied though not fully understood or identified. Legacies of former urban land use have been documented by measuring contamination of contemporary soil with pollutants since banned (Nassauer and Raskin, 2014; Clarke et al., 2015), as well as changes in soil physical and chemical properties, and ecosystem services (Raciti et al., 2011; Setälä et al., 2016; Ziter and Turner, 2018). Effects of former urban planning efforts dictate the structure of contemporary urban forests (Figure 6), as well the benefits they provide to residents (Boone et al., 2010). Similarly, infrastructure and zoning plans have legacy effects on the urban fabric that can last for centuries (Ahlfeldt and Wendland, 2013; Twinam, 2018). Legacies related to former “referential housing types,” aesthetics and architectural styles (Ledent and Komossa, 2019) can further determine how contemporary urban green spaces and landscapes are structured and function (Ossola et al., 2019).
Figure 6. Legacy relationship between the historical trend in average residential parcel size (points in blue) measured across 1,503 neighborhoods (i.e., Census blocks) in nine United States cities and average residential vegetation cover (bars in orange) (re-drawn from data in Ossola and Hopton, 2018b). The historical trend in parcel size (points in blue) affects the current amount of vegetated cover that is achieved in residential parcels (i.e., the legacy in light orange). The decade of maximum housing development of a neighborhood is the decade when most buildings were built. Error bars are the respective standard errors of the mean.
Complex urban legacies that arise from both the ecological system and the coupled social and technological system and their interactions deserve closer investigation. For example, human factors can influence horticulture and landscaping resulting in preferences of the past influencing current vegetation composition and structure (Ossola et al., 2019; Avolio et al., 2020). In addition, the spatial spread of tree diseases such as Dutch Elm disease through a population of urban trees is influences by the spatial distribution of that species. Future species distributions of trees will, in turn, by influenced by the management choices made in an effort to deal with the spreading disease. Heterogeneity at one time period – distribution of the elm trees – becomes a driver of the heterogeneity in another time period – distribution of diseased trees. The distribution of diseased trees is an outcome but it, in turn, becomes the driver for the heterogeneity in management response. This process has been called dynamic heterogeneity and is a spiral of complex social-ecological interactions (Pickett et al., 2017).
An understudied temporal dimension of urban systems relates to “priming effects” – whereby the occurrence of one event, rather than an alternative one, can lead to a significantly different future status of an urban system (Figure 1). For instance, little is known about how prior colonization or planting of particular species might affect future biotic communities. Such “priority effects” (Fukami, 2015) have the potential to shape urban communities and have been found in some recent studies. For example, Aloisio et al. (2019) found that established plant communities on green roofs strongly affected which species were able to survive on these same green roofs at a later time. In addition, Johnson et al. (2015) found that after building removal, plant communities within the footprint of the original building diverged compared to those in the yard areas of parcels. If we can learn to facilitate founder species that tend to promote the establishment, survival and success of other desirable species, such as natives and other organisms that provide targeted ecosystem services, we might be able to harness more benefits from urban nature-based solutions (Dallimer et al., 2015).
Several questions arise when trying to better understand the effects of the past on contemporary cities: “how long do urban legacies last?,” “are urban events more important if they create long-lasting legacies?,” “what are the effects of interactive legacies in urban ecosystems?,” “what happens when legacies are not manifest in all consecutive events along a time series (i.e., skipping)?,” or “would the change of a foundational event determine a significantly different series of events (i.e., priming)?”
When looking at temporal dynamics in relation to the spatial dimension of urban ecosystems, we can further conceptualize places and periods with different temporality (i.e., the unfolding of events and not the absolute time) as compared to nearby locations and periods; temporal hotspots and hot moments, respectively. These concepts arise from the recognition that cities are intrinsically heterogeneous yet dynamic (Cadenasso et al., 2006) and they have been recently reframed in the context of “control points” within rural ecosystems (Bernhardt et al., 2017). A cluster of residential parcels that changed little over the last century could sit next to a group of parcels redeveloped multiple times over the same period. Thus, a temporal hotspot can be defined when urban events happen at a different rate at a location compared to similar events at nearby locations. In contrast, a hot moment refers to when urban events occur more frequently within a particular time period compared to another time period. For instance, urban tree removal is a ubiquitous event across urban landscapes (Ossola and Hopton, 2018b), but its rate could significantly increase from a baseline level after storms and hurricanes (i.e., hot moment), as well as increase when moving from unaffected areas toward those in the midst of wind gusts and tornado paths (i.e., temporal hotspot). Similarly, prolonged illumination at night under a tree can be considered a hot moment as it can locally extend the duration of daily photosynthesis in trees around artificial light sources. Considering the entirety of an urban forest, trees around light poles could also be identified as temporal hotspots whereby recurrent light pollution determines longer growth periods and seasonal phenology (Ffrench-Constant et al., 2016; Figure 7).
Figure 7. (A) Localized light pollution affecting the urban forest can be thought as a “temporal hotspot” whereby the longer duration of artificial illumination can determine longer plant growth periods and extended seasonal phenology. The image details delayed leaf drop in the most illuminated side of a canopy of Aesculus hippocastanum in a public park in Lille, France, in December 2016 (Courtesy: Lamiot, Wikimedia Commons). (B) Peak nighttime traffic can be thought as a “hot moment,” whereby the higher frequency and intensity of car illumination at determined periods can potentially affect light-sensitive organisms such as insects, plants, and wildlife. The image details car light trails on a highway in Montreal, QC, Canada (Courtesy: Pierre Vignau, Wikimedia Commons).
The recent COVID-19 pandemic can be conceptualized as a hot moment in itself, whereby sudden curfews, lockdowns, and other restrictions to people’s movements likely released anthropogenic pressures on several organisms causing many of them to quickly (re)occupy many urban areas in various regions globally (Bates et al., 2020). While global hot moments like this might be relatively rare, they might have important implications on how cities are envisioned, used and planned for the future. Thus, future studies could further investigate the effects of more localized, city-scale, hot moments, and temporal hotspots, such as those related to climate change (i.e., fires, heatwave, flood, and drought events). In particular, extreme heat events are expected to become more common in the future as a result of climate change and urban heat island effects will exaggerate these events (Li and Bou-Zeid, 2013). The urban heat island effect is more prominent in certain parts of cities due to higher concentrations of impervious surfaces and vegetation removal, such that extreme urban hot spots may arise during heatwaves, with uncertain localized implications for human health, urban environment and biodiversity (Ossola et al., 2021).
This type of thinking could help address questions like: “can temporal hotspots and hot moments be regulated to promote functions and services or mitigate unwanted effects?” and “are there specific locations and periods for events to occur so that urban functions are optimized?” By attempting to link temporal and spatial dimensions one might ask “to what extent mapping the development of urban events across landscapes and periods can help us better understand and measure urban ecosystems and their change?”
The temporal dimensions analyzed in previous sections focus on past and current urban events. These past and current events can serve as a benchmark for future urban events as well as a baseline to measure urban scenarios against, i.e., a rich suite of possible urban futures that can be envisioned and planned for Iwaniec et al. (2020). These events can be used when designing and implementing steps to reach desirable urban futures through backcasting (Bibri, 2018). Urban futures can themselves sometime “be described in terms of their duration – the time, in other words, in which they are expected to exist, last or persist before yielding to the present or to other futures” (Davis, 2019). For instance, the development of a new neighborhood in the future might well have a defined start and end date, and duration overall.
Future urban events can be further considered along a deterministic-stochastic gradient. Fully deterministic events can be predicted as they are less affected by temporal randomness, at least within the time scale considered (e.g., the recurrence of summertime in the next 50 years, Figure 5). For instance, street tree diversity in the United States changed little in the last 40 years (Ma et al., 2020); as such, without a significant and widespread change in nursery production, plant selection or an unanticipated climate disaster, it is reasonable to anticipate that street tree diversity will remain constant in the next decade or so. On the other hand, stochastic events are random by definition, and as such, it is difficult if not impossible to predict their future occurrence, development, and duration (e.g., a tornado destroying a city, Figure 3).
Stochasticity has been considered in some ecological studies looking for instance at biological control of invasive species in the wild (Evans et al., 2012) and community ecology (Ning et al., 2019; Shoemaker et al., 2020). To date, however, little effort has been placed toward evaluating how stochastic vs. deterministic processes in cities can affect biological communities and urban ecology (Caruso et al., 2017; Goddard et al., 2021). Recent research shows, however, that specialist insect herbivore populations in cities might be governed more by stochastic events rather than deterministic drivers (Herrmann et al., 2012). In contrast to urban biodiversity research, modeling approaches moved toward acknowledging the importance of stochasticity for urban technological structures (Ellam et al., 2018), stormwater quality (Obropta and Kardos, 2007), urban population growth and morphogenesis (Raimbault, 2018) as a way to improve our ability to make predictions of future urban events and properties.
More research effort could be placed toward looking back at the urban past to test and validate current urban theories and models, and how these can be projected and extrapolated into the future (Figures 2C,D). Particularly promising are studies based on “time-for-place” surrogates that could be used to better infer future properties of urban and non-urban systems. For instance, contemporary cities produce microclimatic gradients and conditions similar to those predicted under global and climate change, such as heatwaves and droughts, thus opening up unique experimental conditions to test how organisms, communities and processes might work in the future (Lahr et al., 2018). However, whether urban areas can serve as analogs for rural areas in the future, and for what biological processes [e.g., Youngsteadt et al. (2015); Wohlfahrt et al. (2019)] remains an important area for research.
When considering urban futures, numerous research questions could be framed: “To what extent can the unfolding of future events be predicted based on current and past ones?,” “how can current and past urban events inform the development of scenarios and future-casting efforts?,” or again “what is the relationship between stochastic and deterministic events and their interplay in affecting urban futures?”
In this contribution we outlined the main dimensions of urban time that can shape how events develop in urban ecosystems. Among these dimension, those related to temporal hotspots/moments and stochastic vs. deterministic processes – are understudied. We believe that – by better valuing urban time – urban ecology can achieve a richer, more nuanced and complete understanding of cities and towns through investigations that utilize the theoretical lens discussed here.
Future research is needed not only to elucidate how these dimensions affects urban ecosystems, but also how they interact with each other, the interplay with spatial dimensions of cities across scales, as well as the connections with other urban and non-urban systems. We believe that a comprehensive evaluation of how the “Urban Chronos” affects social, ecological, and technical systems is now needed to significantly advance urban ecology and ultimately its practice and societal impact.
AO initiated and led the manuscript with contributions to writing and editing by EM and MC. All authors contributed to the article and approved the submitted version.
The authors declare that the research was conducted in the absence of any commercial or financial relationships that could be construed as a potential conflict of interest.
Ahlfeldt, G. M., and Wendland, N. (2013). How polycentric is a monocentric city? Centers, spillovers and hysteresis. J. Econ. Geography 13, 53–83. doi: 10.1093/jeg/lbs013
Aloisio, J. M., Palmer, M. I., Tuininga, A. R., and Lewis, J. D. (2019). Plant colonization of green roofs is affected by composition of established native plant communities. Front. Ecol. Evol. 6:238. doi: 10.3389/fevo.2018.00238
Auffret, A. G., and Lindgren, E. (2020). Roadside diversity in relation to age and surrounding source habitat: evidence for long time lags in valuable green infrastructure. Ecol. Solut. Evidence 1:e12005. doi: 10.1002/2688-8319.12005
Avolio, M., Pataki, D. E., Jenerette, G. D., Pincetl, S., Clarke, L. W., Cavender-Bares, J., et al. (2020). Urban plant diversity in Los Angeles, California: species and functional type turnover in cultivated landscapes. Plants People Planet 2, 144–156. doi: 10.1002/ppp3.10067
Barrell, J. (1993). Pre-planning tree surveys: safe useful life expectancy (SULE) is the natural progression. Arboricultural J. 17, 33–46. doi: 10.1080/03071375.1993.9746943
Bates, A. E., Primack, R. B., Moraga, P., and Duarte, C. M. (2020). COVID-19 pandemic and associated lockdown as a “Global Human Confinement Experiment” to investigate biodiversity conservation. Biol. Conservation 248:108665. doi: 10.1016/j.biocon.2020.108665
Beninde, J., Veith, M., and Hochkirch, A. (2015). Biodiversity in cities needs space: a meta-analysis of factors determining intra-urban biodiversity variation. Ecol. Lett. 18, 581–592. doi: 10.1111/ele.12427
Bernhardt, E. S., Blaszczak, J. R., Ficken, C. D., Fork, M. L., Kaiser, K. E., and Seybold, E. C. (2017). Control points in ecosystems: moving beyond the hot spot hot moment concept. Ecosystems 20, 665–682. doi: 10.1007/s10021-016-0103-y
Bibri, S. E. (2018). Backcasting in futures studies: a synthesized scholarly and planning approach to strategic smart sustainable city development. Eur. J. Futures Res. 6:13. doi: 10.1186/s40309-018-0142-z
Boone, C. G., Cadenasso, M. L., Grove, J. M., Schwarz, K., and Buckley, G. L. (2010). Landscape, vegetation characteristics, and group identity in an urban and suburban watershed: why the 60s matter. Urb. Ecosyst. 13, 255–271. doi: 10.1007/s11252-009-0118-7
Botías, C., David, A., Hill, E. M., and Goulson, D. (2017). Quantifying exposure of wild bumblebees to mixtures of agrochemicals in agricultural and urban landscapes. Environ. Pollut. 222, 73–82. doi: 10.1016/j.envpol.2017.01.001
Cadenasso, M. L., Pickett, S. T. A., and Grove, J. M. (2006). Dimensions of ecosystem complexity: heterogeneity, connectivity, and history. Ecol. Complexity 3, 1–12. doi: 10.1016/j.ecocom.2005.07.002
Caruso, T., Migliorini, M., Rota, E., and Bargagli, R. (2017). Highly diverse urban soil communities: does stochasticity play a major role? Appl. Soil Ecol. 110, 73–78. doi: 10.1016/j.apsoil.2016.10.012
Clarke, L. W., Jenerette, G. D., and Bain, D. J. (2015). Urban legacies and soil management affect the concentration and speciation of trace metals in Los Angeles community garden soils. Environ. Pollut. 197, 1–12. doi: 10.1016/j.envpol.2014.11.015
Dallimer, M., Davies, Z. G., Diaz-Porras, D. F., Irvine, K. N., Maltby, L., Warren, P. H., et al. (2015). Historical influences on the current provision of multiple ecosystem services. Glob. Environ. Change 31, 307–317. doi: 10.1016/j.gloenvcha.2015.01.015
Davis, J. (2019). Futurescapes of urban regeneration: ten years of design for the unfolding urban legacy of London’s Olympic Games, 2008–2018. Plann. Perspect. 34, 877–901. doi: 10.1080/02665433.2018.1541757
du Toit, M. J., Kotze, D. J., and Cilliers, S. S. (2016). Landscape history, time lags and drivers of change: urban natural grassland remnants in Potchefstroom, South Africa. Landscape Ecol. 31, 2133–2150. doi: 10.1007/s10980-016-0386-6
Ellam, L., Girolami, M., Pavliotis, G. A., and Wilson, A. (2018). Stochastic modelling of urban structure. Proc. R. Soc. A Mathemat. Phys. Eng. Sci. 474:20170700. doi: 10.1098/rspa.2017.0700
Evans, J. A., Davis, A. S., Raghu, S., Ragavendran, A., Landis, D. A., and Schemske, D. W. (2012). The importance of space, time, and stochasticity to the demography and management of Alliaria petiolata. Ecol. Appl. 22, 1497–1511. doi: 10.1890/11-1291.1
Ffrench-Constant, R. H., Somers-Yeates, R., Bennie, J., Economou, T., Hodgson, D., Spalding, A., et al. (2016). Light pollution is associated with earlier tree budburst across the United Kingdom. Proc. R. Soc. B Biol. Sci. 283:20160813. doi: 10.1098/rspb.2016.0813
Fisogni, A., Hautekèete, N., Piquot, Y., Brun, M., Vanappelghem, C., Michez, D., et al. (2020). Urbanization drives an early spring for plants but not for pollinators. Oikos 129, 1681–1691. doi: 10.1111/oik.07274
Fukami, T. (2015). Historical contingency in community assembly: integrating niches, species pools, and priority effects. Annu. Rev. Ecol. Evol. Syst. 46, 1–23. doi: 10.1146/annurev-ecolsys-110411-160340
Gabrovski, M., and Ortego-Marti, V. (2019). The cyclical behavior of the beveridge curve in the housing market. J. Econ. Theory 181, 361–381. doi: 10.1016/j.jet.2019.03.003
Gilbert, O. L. (1991). The Ecology of Urban Habitats. Dordrecht: Springer Science+Business Media. doi: 10.1007/978-94-011-3068-4
Goddard, M. A., Davies, Z. G., Guenat, S., Ferguson, M. J., Fisher, J. C., Akanni, A., et al. (2021). A global horizon scan of the future impacts of robotics and autonomous systems on urban ecosystems. Nat. Ecol. Evol. 5, 219–230. doi: 10.1038/s41559-020-01358-z
Grimm, N. B., Pickett, S. T. A., Hale, R. L., and Cadenasso, M. L. (2017). Does the ecological concept of disturbance have utility in urban social–ecological–technological systems? Ecosyst. Health Sustainabil. 3:e01255. doi: 10.1002/ehs2.1255
Groffman, P. M., Avolio, M., Cavender-Bares, J., Bettez, N. D., Grove, J. M., Hall, S. J., et al. (2017). Ecological homogenization of residential macrosystems. Nat. Ecol. Evol. 1, 0191. doi: 10.1038/s41559-017-0191
Hahs, A. K., McDonnell, M. J., McCarthy, M. A., Vesk, P. A., Corlett, R. T., Norton, B. A., et al. (2009). A global synthesis of plant extinction rates in urban areas. Ecol. Lett. 12, 1165–1173. doi: 10.1111/j.1461-0248.2009.01372.x
Harris, R. M. B., Beaumont, L. J., Vance, T. R., Tozer, C. R., Remenyi, T. A., Perkins-Kirkpatrick, S. E., et al. (2018). Biological responses to the press and pulse of climate trends and extreme events. Nat. Climate Change 8, 579–587. doi: 10.1038/s41558-018-0187-9
Herrmann, D. L., Pearse, I. S., and Baty, J. H. (2012). Drivers of specialist herbivore diversity across 10 cities. Landscape Urb. Plann. 108, 123–130. doi: 10.1016/j.landurbplan.2012.08.007
Howlett, M., and Goetz, K. H. (2014). Introduction: time, temporality and timescapes in administration and policy. Int. Rev. Administrative Sci. 80, 477–492. doi: 10.1177/0020852314543210
Iwaniec, D. M., Cook, E. M., Davidson, M. J., Berbés-Blázquez, M., Georgescu, M., Krayenhoff, E. S., et al. (2020). The co-production of sustainable future scenarios. Landscape Urb. Plann. 197:103744. doi: 10.1016/j.landurbplan.2020.103744
Johnson, A. L., Tauzer, E. C., and Swan, C. M. (2015). Human legacies differentially organize functional and phylogenetic diversity of urban herbaceous plant communities at multiple spatial scales. Appl. Vegetat. Sci. 18, 513–527. doi: 10.1111/avsc.12155
Kampichler, C., and van der Jeugd, H. P. (2013). Determining patterns of variability in ecological communities: time lag analysis revisited. Environ. Ecol. Statis. 20, 271–284. doi: 10.1007/s10651-012-0219-y
Kharouba, H. M., Ehrlén, J., Gelman, A., Bolmgren, K., Allen, J. M., Travers, S. E., et al. (2018). Global shifts in the phenological synchrony of species interactions over recent decades. Proc. Natl. Acad. Sci. U.S.A. 115, 5211. doi: 10.1073/pnas.1714511115
Lahr, E. C., Dunn, R. R., and Frank, S. D. (2018). Getting ahead of the curve: cities as surrogates for global change. Proc. R. Soc. B Biol. Sci. 285:20180643. doi: 10.1098/rspb.2018.0643
Ledent, G., and Komossa, S. (2019). Referential types as clues for housing design. Urb. Morphol. 23, 125–142.
Li, D., and Bou-Zeid, E. (2013). Synergistic interactions between urban heat islands and heat waves: the impact in cities is larger than the sum of its parts. J. Appl. Meteorol. Climatol. 52, 2051–2064. doi: 10.1175/JAMC-D-13-02.1
Ma, B., Hauer, R. J., Wei, H., Koeser, A. K., Peterson, W., Simons, K., et al. (2020). An assessment of street tree diversity: findings and implications in the United States. Urb. Forestry Urb. Green. 56:126826. doi: 10.1016/j.ufug.2020.126826
Malekpour, S., Brown, R. R., and de Haan, F. J. (2015). Strategic planning of urban infrastructure for environmental sustainability: understanding the past to intervene for the future. Cities 46, 67–75. doi: 10.1016/j.cities.2015.05.003
Marcus, L., and Colding, J. (2014). Toward an integrated theory of spatial morphology and resilient urban systems. Ecol. Soc. 19:55. doi: 10.5751/ES-06939-190455
Marcus, L., Pont, M. B., and Barthel, S. (2019). Towards a socio-ecological spatial morphology: integrating elements of urban morphology and landscape ecology. Urb. Morphol. 23, 115–124.
McDonnell, M., Pickett, S., Groffman, P., Bohlen, P., Pouyat, R., Zipperer, W., et al. (1997). Ecosystem processes along an urban-to-rural gradient. Urb. Ecosyst. 1, 21–36. doi: 10.1023/A:1014359024275
Meineke, E. K., Dunn, R. R., and Frank, S. D. (2014). Early pest development and loss of biological control are associated with urban warming. Biol. Lett. 10:20140586. doi: 10.1098/rsbl.2014.0586
Metzger, J. T. (2000). Planned abandonment: the neighborhood life-cycle theory and national urban policy. Housing Policy Debate 11, 7–40. doi: 10.1080/10511482.2000.9521359
Nassauer, J. I., and Raskin, J. (2014). Urban vacancy and land use legacies: a frontier for urban ecological research, design, and planning. Landscape Urb. Plann. 125, 245–253. doi: 10.1016/j.landurbplan.2013.10.008
Neil, K., and Wu, J. (2006). Effects of urbanization on plant flowering phenology: a review. Urb. Ecosyst. 9, 243–257. doi: 10.1007/s11252-006-9354-2
Ning, D., Deng, Y., Tiedje, J. M., and Zhou, J. (2019). A general framework for quantitatively assessing ecological stochasticity. Proc. Natl. Acad. Sci. 116:16892. doi: 10.1073/pnas.1904623116
Nyström, J. (1992). The cyclical urbanization model. Geografiska Annaler Ser. B Hum. Geography 74, 133–144. doi: 10.1080/04353684.1992.11879637
Obropta, C. C., and Kardos, J. S. (2007). Review of urban stormwater quality models: deterministic, stochastic, and hybrid Approaches1. JAWRA J. Am. Water Resour. Assoc. 43, 1508–1523. doi: 10.1111/j.1752-1688.2007.00124.x
Ossola, A., Hahs, A. K., and Livesley, S. J. (2015). Habitat complexity influences fine scale hydrological processes and the incidence of stormwater runoff in managed urban ecosystems. J. Environ. Manag. 159, 1–10. doi: 10.1016/j.jenvman.2015.05.002
Ossola, A., Hoeppner, J. M., Burley, H., Gallagher, R. V., Beaumont, L. J., and Leishman, M. R. (2020). The global urban tree inventory: a database of the diverse tree flora that inhabits the world’s cities. Glob. Ecol. Biogeography. 29, 1907–1914. doi: 10.1111/geb.13169
Ossola, A., and Hopton, M. E. (2018a). Climate differentiates forest structure across a residential macrosystem. Sci. Total Environ. 639, 1164–1174. doi: 10.1016/j.scitotenv.2018.05.237
Ossola, A., and Hopton, M. E. (2018b). Measuring urban tree loss dynamics across residential landscapes. Sci. Tot. Environ. 612, 940–949. doi: 10.1016/j.scitotenv.2017.08.103
Ossola, A., Jenerette, D., McGrawth, A., Chow, W., Hughes, L., and Leishman, M. L. (2021). Urban vegetation locally mitigates daytime land surface temperature during a summer heatwave. Landscape Urb. Plann. 209, 104046. doi: 10.1016/j.landurbplan.2021.104046
Ossola, A., Locke, D. H., Lin, B. B., and Minor, E. (2019). Greening in style: urban form, architecture and the structure of front and backyard vegetation. Landscape Urb. Plann. 185, 141–157. doi: 10.1016/j.landurbplan.2019.02.014
Pickett, S. T. A., Cadenasso, M. L., Rosi-Marshall, E. J., Belt, K. T., Groffman, P. M., Grove, J. M., et al. (2017). Dynamic heterogeneity: a framework to promote ecological integration and hypothesis generation in urban systems. Urb. Ecosyst. 20, 1–14. doi: 10.1007/s11252-016-0574-9
Raciti, S. M., Groffman, P. M., Jenkins, J. C., Pouyat, R. V., Fahey, T. J., Pickett, S. T. A., et al. (2011). Accumulation of carbon and nitrogen in residential soils with different land-use histories. Ecosystems 14, 287–297. doi: 10.1007/s10021-010-9409-3
Raimbault, J. (2018). Calibration of a density-based model of urban morphogenesis. PLoS One 13:e0203516. doi: 10.1371/journal.pone.0203516
Ramalho, C. E., and Hobbs, R. J. (2012). Time for a change - Dynamic urban ecology. Trends Ecol. Evol. 27, 179–188. doi: 10.1016/j.tree.2011.10.008
Riggleman, J. R. (1933). Building cycles in the United States, 1875–1932. J. Am. Statis. Assoc. 28, 174–183. doi: 10.1080/01621459.1933.10502255
Rivkin, L. R., Santangelo, J. S., Alberti, M., Aronson, M. F. J., de Keyzer, C. W., Diamond, S. E., et al. (2019). A roadmap for urban evolutionary ecology. Evol. Appl. 12, 384–398. doi: 10.1111/eva.12734
Roman, L. A., Pearsall, H., Eisenman, T. S., Conway, T. M., Fahey, R. T., Landry, S., et al. (2018). Human and biophysical legacies shape contemporary urban forests: a literature synthesis. Urb. Forestry Urb. Green. 31, 157–168. doi: 10.1016/j.ufug.2018.03.004
Sehrt, M., Bossdorf, O., Freitag, M., and Bucharova, A. (2020). Less is more! Rapid increase in plant species richness after reduced mowing in urban grasslands. Basic Appl. Ecol. 42, 47–53. doi: 10.1016/j.baae.2019.10.008
Setälä, H. M., Francini, G., Allen, J. A., Hui, N., Jumpponen, A., and Kotze, D. J. (2016). Vegetation type and age drive changes in soil properties, nitrogen, and carbon sequestration in urban parks under cold climate. Front. Ecol. Evol. 4:93. doi: 10.3389/fevo.2016.00093
Seto, K. C., Güneralp, B., and Hutyra, L. R. (2012). Global forecasts of urban expansion to 2030 and direct impacts on biodiversity and carbon pools. Proc. Natl. Acad. Sci. U.S.A. 109, 16083–16088. doi: 10.1073/pnas.1211658109
Shoemaker, L. G., Sullivan, L. L., Donohue, I., Cabral, J. S., Williams, R. J., Mayfield, M. M., et al. (2020). Integrating the underlying structure of stochasticity into community ecology. Ecology 101:e02922. doi: 10.1002/ecy.2922
Shultz, A. J., Adams, B. J., Bell, K. C., Ludt, W. B., Pauly, G. B., and Vendetti, J. E. (2020). Natural history collections are critical resources for contemporary and future studies of urban evolution. Evol. Appl. 14, 233–247. doi: 10.1111/eva.13045
Smith, I. A., Dearborn, V. K., and Hutyra, L. R. (2019). Live fast, die young: accelerated growth, mortality, and turnover in street trees. PLoS One 14:e0215846. doi: 10.1371/journal.pone.0215846
Tabassum, S., Ossola, A., Manea, A., Cinantya, A., Fernandez Winzer, L., and Leishman, M. R. (2020). Using ecological knowledge for designing and landscaping with plants in cities. Ecol. Eng. 158:106049. doi: 10.1016/j.ecoleng.2020.106049
Twinam, T. (2018). The long-run impact of zoning: institutional hysteresis and durable capital in Seattle, 1920–2015. Regional Sci. Urb. Econ. 73, 155–169. doi: 10.1016/j.regsciurbeco.2018.08.004
Watson, C. J., Carignan-Guillemette, L., Turcotte, C., Maire, V., and Proulx, R. (2020). Ecological and economic benefits of low-intensity urban lawn management. J. Appl. Ecol. 57, 436–446. doi: 10.1111/1365-2664.13542
Watts, K., Whytock, R. C., Park, K. J., Fuentes-Montemayor, E., Macgregor, N. A., Duffield, S., et al. (2020). Ecological time lags and the journey towards conservation success. Nat. Ecol. Evol. 4, 304–311. doi: 10.1038/s41559-019-1087-8
Welbourne, L. E., Morland, A. B., and Wade, A. R. (2015). Human colour perception changes between seasons. Curr. Biol. 25, R646–R647. doi: 10.1016/j.cub.2015.06.030
Wohlfahrt, G., Tomelleri, E., and Hammerle, A. (2019). The urban imprint on plant phenology. Nat. Ecol. Evol. 3, 1668–1674. doi: 10.1038/s41559-019-1017-9
Wolkovich, E. M., Cook, B. I., McLauchlan, K. K., and Davies, T. J. (2014). Temporal ecology in the Anthropocene. Ecol. Lett. 17, 1365–1379. doi: 10.1111/ele.12353
Youngsteadt, E., Dale, A. G., Terando, A. J., Dunn, R. R., and Frank, S. D. (2015). Do cities simulate climate change? A comparison of herbivore response to urban and global warming. Glob. Change Biol. 21, 97–105. doi: 10.1111/gcb.12692
Zelnik, Y. R., Arnoldi, J.-F., and Loreau, M. (2018). The impact of spatial and temporal dimensions of disturbances on ecosystem stability. Front. Ecol. Evol. 6:224. doi: 10.3389/fevo.2018.00224
Ziter, C., Graves, R. A., and Turner, M. G. (2017). How do land-use legacies affect ecosystem services in United States cultural landscapes? Landscape Ecol. 32, 2205–2218. doi: 10.1007/s10980-017-0545-4
Keywords: urban dynamics, urban change detection, history, urban development, urban trajectories
Citation: Ossola A, Cadenasso ML and Meineke EK (2021) Valuing the Role of Time in Urban Ecology. Front. Ecol. Evol. 9:620620. doi: 10.3389/fevo.2021.620620
Received: 23 October 2020; Accepted: 23 February 2021;
Published: 16 March 2021.
Edited by:
Galina Churkina, Potsdam Institute for Climate Impact Research (PIK), GermanyReviewed by:
Elsa Anderson, Cary Institute of Ecosystem Studies, United StatesCopyright © 2021 Ossola, Cadenasso and Meineke. This is an open-access article distributed under the terms of the Creative Commons Attribution License (CC BY). The use, distribution or reproduction in other forums is permitted, provided the original author(s) and the copyright owner(s) are credited and that the original publication in this journal is cited, in accordance with accepted academic practice. No use, distribution or reproduction is permitted which does not comply with these terms.
*Correspondence: Alessandro Ossola, YW9zc29sYUB1Y2RhdmlzLmVkdS5hdQ==; YWxlc3NhbmRyby5vc3NvbGFAbXEuZWR1LmF1; YWxlc3NhbmRyby5vc3NvbGFAdW5pbWVsYi5lZHUuYXU=
Disclaimer: All claims expressed in this article are solely those of the authors and do not necessarily represent those of their affiliated organizations, or those of the publisher, the editors and the reviewers. Any product that may be evaluated in this article or claim that may be made by its manufacturer is not guaranteed or endorsed by the publisher.
Research integrity at Frontiers
Learn more about the work of our research integrity team to safeguard the quality of each article we publish.