- 1Forestry Department, Federal University of Lavras, Câmpus Universitário, Lavras, Brazil
- 2School of Earth and Environmental Sciences, The University of Queensland, Brisbane, QLD, Australia
- 3Centre for Integrative Ecology, School of Life and Environmental Sciences, Deakin University, Melbourne, VIC, Australia
- 4Department of Ecology and Conservation, Federal University of Lavras, Câmpus Universitário, Lavras, Brazil
The Atlantic Forest in Brazil is a biodiversity hotspot, yet its diverse ecosystems and species are becoming increasingly threatened by habitat loss and extreme habitat fragmentation. Most habitat patches of Atlantic Forest are dispersed across agricultural landscapes (e.g., grazing and cropping) in relatively small and isolated fragments (80% < 50 ha). Forest fragments < 1 ha, scattered trees in pastures, tree lines on trenches and fences, and remnant riparian forest, collectively called here Small Landscape Elements (SLEs), are very common in this context. While these SLEs make up much of the Atlantic Forests footprint, very little is known about their role or impact on the persistence and conservation of species. In this study, we investigate the role of SLEs on landscape configuration, particularly their contribution toward landscape connectivity of individual species and the genetic flow of species between larger forest fragments. We randomly selected 20 buffers of 707 hectares within a 411,670 hectare area of the Atlantic Forest that was completely covered by forest in the past located in the south of Minas Gerais State, Brazil. The forest cover randomly varied between these buffers. We used graph theory to measure landscape connectivity as the probability of connectivity for different disperser movement types between landscape knots (habitat patches). We used three estimated dispersal distances in the models: pollen disperser insect (50 m), low-mobility seed disperser bird (100 m) and high-mobility seed disperser bird (760 m). The SLEs together increased the probability of connection by roughly 50%, for all model dispersers, if compared to a theoretical baseline landscape containing no SLEs. Of all SLEs, riparian forests contribute the most toward enhancing landscape connectivity. In these highly fragmented landscapes, such as the Atlantic Forest (>70%), the position of SLEs within the landscapes was more important than their respective areas for connectivity. Although the landscapes were deeply fragmented, we showed that the presence of SLEs can increase connectivity and reduce further biodiversity loss in the Atlantic Forest.
Introduction
Environmental degradation is strongly linked to natural habitat loss. Deforestation transforms landscape configuration, increases forest fragmentation, increases the number of small forest patches, increases isolation among forest patches, ultimately resulting in a reduction of biological diversity (henceforth, biodiversity) (Pardini et al., 2010; Banks-Leite et al., 2014; Ribeiro et al., 2019). Tropical deforestation is most commonly related to the conversion of tropical forests to agricultural land use types such as cropland or pastures (Ribeiro et al., 2019; Levis et al., 2020). Although most of this land use conversion is arguably driven by increasing the land availability for food production (FAO, 2020), losing natural habitat can also lead to perverse outcomes for food production. For example, a decline in insect pollinator abundance can reduce crop yields and changing land use can decrease the availability of water for crops (Garibaldi et al., 2011; Saturni et al., 2016; Fitton et al., 2019). This lack of connectivity caused by high instances of poorly planned land-use change is one of the greatest challenges in modern conservation (Dobson et al., 1997) and thus, maintaining and improving landscape connectivity is one of the challenges for biodiversity conservation in highly fragmented landscapes (Taylor et al., 2006). Understanding how an increasing agricultural footprint impacts the extent and landscape connectivity of tropical forests is essential to minimize further biodiversity loss and maximize agricultural yield in a sustainable way (Melo et al., 2013).
Gene flow is affected both by landscape connectivity and by the dispersal capabilities of different organisms. Landscape connectivity refers to the ease in which organisms can move in a landscape (Taylor et al., 1993), through small patches within (e.g., scattered trees and forest fragments < 1 h) and between existing protected areas (e.g., remnant riparian forest) (Ward et al., 2020). Functional connectivity considers the behavioral responses of an organism to the various landscape elements, while structural connectivity is equated with habitat contiguity and can also be inferred by landscape metrics (Tischendorf and Fahrig, 2000). For example, the area and shape of habitat patches, as well as their distribution in the landscape, using maps produced from satellite images. Landscape connectivity can be measured directly by measuring the movement of organisms within a landscape, including how organisms are affected by landscape elements (Tischendorf and Fahrig, 2000). This can be done using direct observation of an organism’s movements (e.g., radio telemetry), or experimentally using computer simulations of species with specific functional traits (Keller et al., 2013). Organisms highly specialized in forest habitats and/or with lower dispersal distances tend to be most affected by the size reduction of habitat patches and by the isolation of those patches caused by non-habitat matrices (Banks-Leite et al., 2014; Barlow et al., 2016).
Maintaining continuous and wide forest corridors is one of the most accepted means to provide functional and structural connectivity between protected areas. Global protected area targets like the Sustainable Development Goal 15 and the Aichi Target 11 both emphasize the importance of maintaining a well connected reserve network. There are also efforts by countries to nationally promote habitat connectivity, for example the Brazilian Pact for Restoration of the Atlantic Forest and the Mantiqueira Corridor is an example of a commitment to protect and maintain continuous and wide forest corridors (Pact for the Restoration of the Atlantic Forest, 20116). Research finds that large protected areas and wide corridors are best to maintain the movement of individuals across populations, avoiding population isolation, and consequently, decreasing the risk of inbreeding depression and the occurrence of genetic bottlenecks (Charlesworth and Willis, 2009; Hedrick and Garcia-Dorado, 2016; Trewick et al., 2017). However, large protected areas that are connected by wide corridors can conflict with more economic use of the land like agriculture, competing for space with crops and cattle grazing. On the other hand, small landscape elements (SLEs) like scattered trees, hedges and hedgerows, and small forest fragments can also provide landscape connectivity in areas under economic use adding significantly to biodiversity conservation (Kremen and Merenlender, 2018), without competing intensely with land economic use.
Small landscape elements (SLEs) in agricultural lands are extremely important for biodiversity. Scattered trees are considered key structures for vertebrates, arthropods, and plants, as they provide shelter, food, and landing places for volante fauna (Prevedello et al., 2018). Scattered trees have also been shown to alter the microclimate under their crowns which can facilitate tree species establishment (Siqueira et al., 2017). Additionally, scattered paddock trees provide environmental services as shade for the cattle, which has been found to increase milk and beef yield in tropical areas (Paciullo et al., 2011; Mello et al., 2017). Hedges, hedgerows and tree lines are common in agricultural lands. These types of SLEs can be very old and are normally used for landscaping or as divisions between land patches under different ownership or management (Baudry et al., 2000). Trees lines can be found along property perimeter fences or in the case of Brazil, can be a consequence of the natural colonization of trenches. These hedgerow-like structures in Brazil have a high historical value, as a heritage from the slavery period, as well as a high ecological relevance working as habitat and corridors for plants and fauna (Castro and van den Berg, 2013; Rocha et al., 2014). Although there is now strong evidence showing the ecological importance of the hedges and hedgerows associated with trenches (Castro and van den Berg, 2013; Rocha et al., 2014), less is known about the ecological importance of tree lines associated with the fences.
In this study, we investigate the role of SLEs for landscape connectivity for insect pollinators, small avian seed dispersers (e.g., genus Turdus, locally called “sabiá”) and large avian seed dispersers (e.g., genus Ramphastos, locally called “Tucano”), in Minas Gerais State, Brazil which is a region containing areas of Atlantic Forest and agricultural land (Kremen and Merenlender, 2018). We aim to identify how fragmented, and in which configuration, is the Atlantic Forest landscape. How landscape metrics change the landscape connectivity in a gradient of fragmentation. The importance of the SLEs for landscape connectivity, and which metrics of the SLEs are important for the landscape connectivity. Answering these questions is fundamental to establish strategies for conservation in agricultural lands, specifically in how we should deal with the SLEs in an agricultural landscape. Although this study was conducted in the Brazilian Atlantic Forest, the results are also relevant for other tropical forest biomes where similar processes of habitat loss and fragmentation have occurred, are still occuring, or are predicted to occur in the future. According to (Joly et al., 2014), the Brazilian Atlantic Forest can be considered as a large natural laboratory where we can test the effects of past human impact and project them to other tropical biomes where those changes will happen in the future.
Materials and Methods
Study Area
Areas in Brazil that historically were covered by vast tropical forests, like the Atlantic Forest, have since been converted to agricultural uses, slicing once intact areas of tropical forests into many small forest patches (Laurance et al., 2011; Lewis et al., 2015; Taubert et al., 2018). The area of focus for this study is an area of the Brazilian Atlantic Forest, in Minas Gerais State, south-eastern Brazil (Figure 1). The climate Köppen type is CWA and CWB, with warm and wet summer and dry winter (Dantas et al., 2007). The soils are latosols and argisols (dos Santos et al., 2011). Most of the Atlantic Forest in the south of Minas Gerais State was converted to agricultural pasture composed of exotic grasses and, in a minor proportion, to cropland (mainly annual crops and coffee). In this region, milk production is more important than beef. This land-use change occurred in the last 150 years and provoked intense forest fragmentation. Forest conversion to other land uses has been rare in the last 30 years. Other elements are present in the landscape as a large artificial reservoir for hydroelectricity, small urban areas, and eucalyptus plantations.
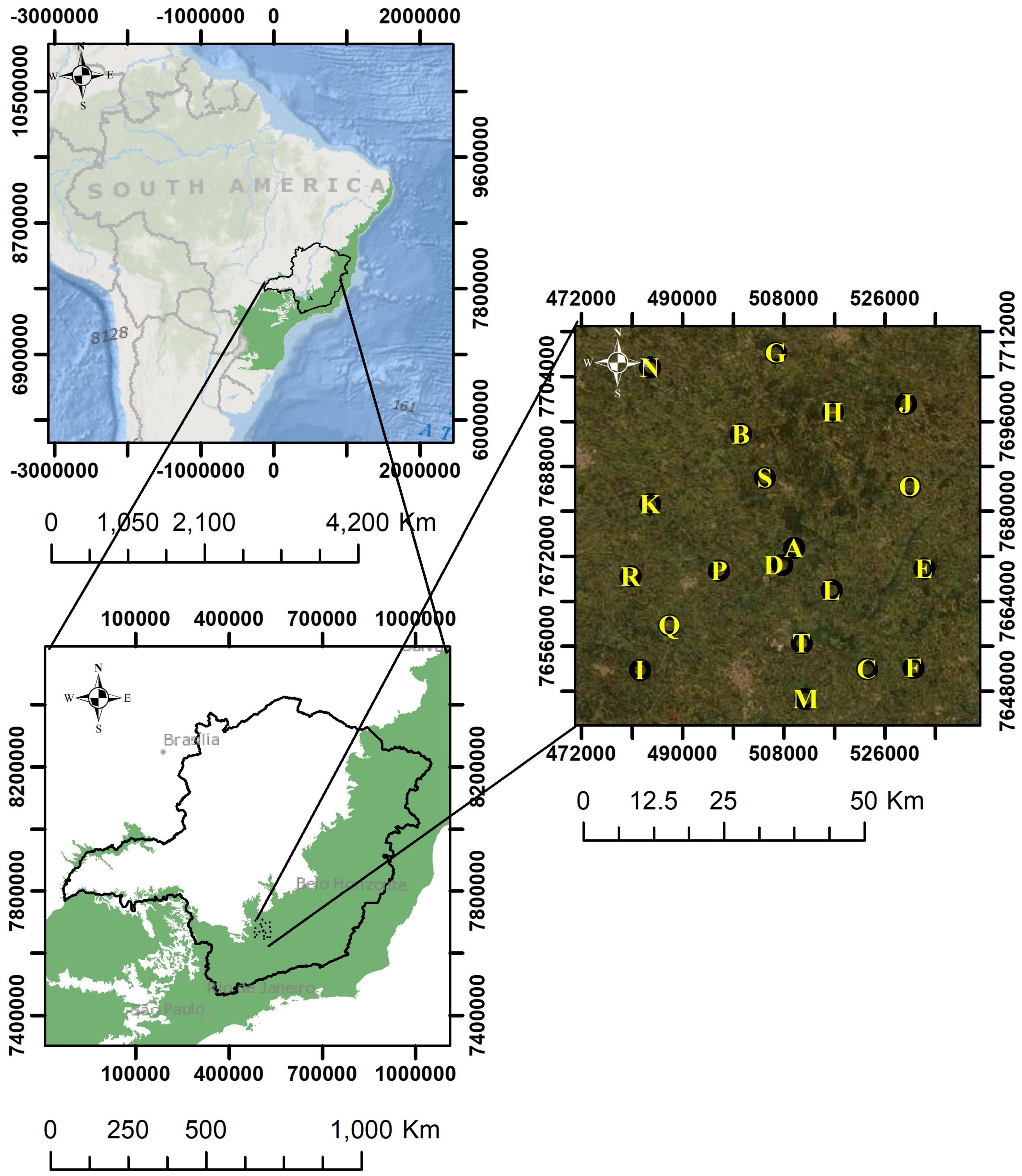
Figure 1. Study area. The characters in the image correspond to the sampled buffers (landscapes), sorted from A to T in successively lower forest cover. Atlantic Forest original extent in green (adapted from IBGE).
In Brazil, the SNUC (Sistema Nacional de Unidades de Conservação—National System of Conservation Units, Law No. 9.985/2000) states that integral protected areas must be large and be isolated from farming or other economic activities (Brasil, 2000). Within the Brazilian Atlantic Forest, small forest fragments have a variable shape, often presenting themselves as long and narrow forests (Crouzeilles et al., 2013). These small forest fragments, in general, remain standing on the property due to legal restrictions (e.g., “The General Law for Native Vegetation Protection,” Brasil, 2012). This is the case of riparian forests along watercourses partially protected in Brazil by the Law No. 12.651 of 2012 (Brasil, 2012). Small forest fragments are also left standing in gullies, rocky areas, or steep slopes where other economic activities are not viable. Some landowners believe that small forest fragments are important for water conservation and biodiversity conservation (Siqueira et al., 2017). Frequently, landowners preserve small forest fragments because of the environmental services they provide. Tisovec et al. (2019) showed that the most important services realized by landowners in an area of Atlantic Forest, and that lead them to keep the forest in their properties, are rather indirect like legacy gratification, existential gratification, feeling joy and peaceful, air purification, among others. Direct services like food, firewood, timber were less important. By a conservationist perspective, these small fragments are important to maintain the movements of organisms between habitat remnants, which can affect metapopulation structure, community assembly dynamics, gene flow and conservation strategy (Moore et al., 2008). However, the minimum necessary forest patch size will vary with taxonomic group (e.g., invertebrates, amphibians, reptiles, mammals), body size (which is related to home range size in many animals), demography and habitat characteristics (e.g., perch height, predator intensity and resource availability).
Sampling Design
We delineated a rectangular polygon of 411,670 ha comprising an area historically covered by forest (IBGE, 2004). Within this rectangular polygon, we randomly allocated 20 points to be the centers of circular buffers with 1,500 m radius and 707 ha each. To do that, we first manually found within the rectangular polygon the largest area with continuous forest cover and we set a buffer around these points. Based on this first buffer, we then randomly chose the other 19 buffers using the “Random” tool of ArcMap (Figure 1). No restriction was used for the randomization process except that the buffers must be at least 700 m from each other. Moving forward will refer to these buffers as “landscapes.”
Land Use Mapping
We used “RapidEye” satellite images to map the land use classes for the 20 landscapes. Those images have 5 m resolution which allowed us to identify the following land use categories: pastures, cropland, eucalyptus plantations, water bodies, and native tree cover. The native tree cover was separated into six categories, where five of them we called SLEs (Figure 2): scattered trees, trenches (hedgerow-like tree lines colonizing trenches), fences (hedgerow-like tree lines colonizing barbed wire fences), riparian forests, and forest fragments < 1 ha. The last category was forest fragments > 1 ha. The images were provided by the Federal University of Lavras in a partnership with the Brazilian Environmental Ministry.
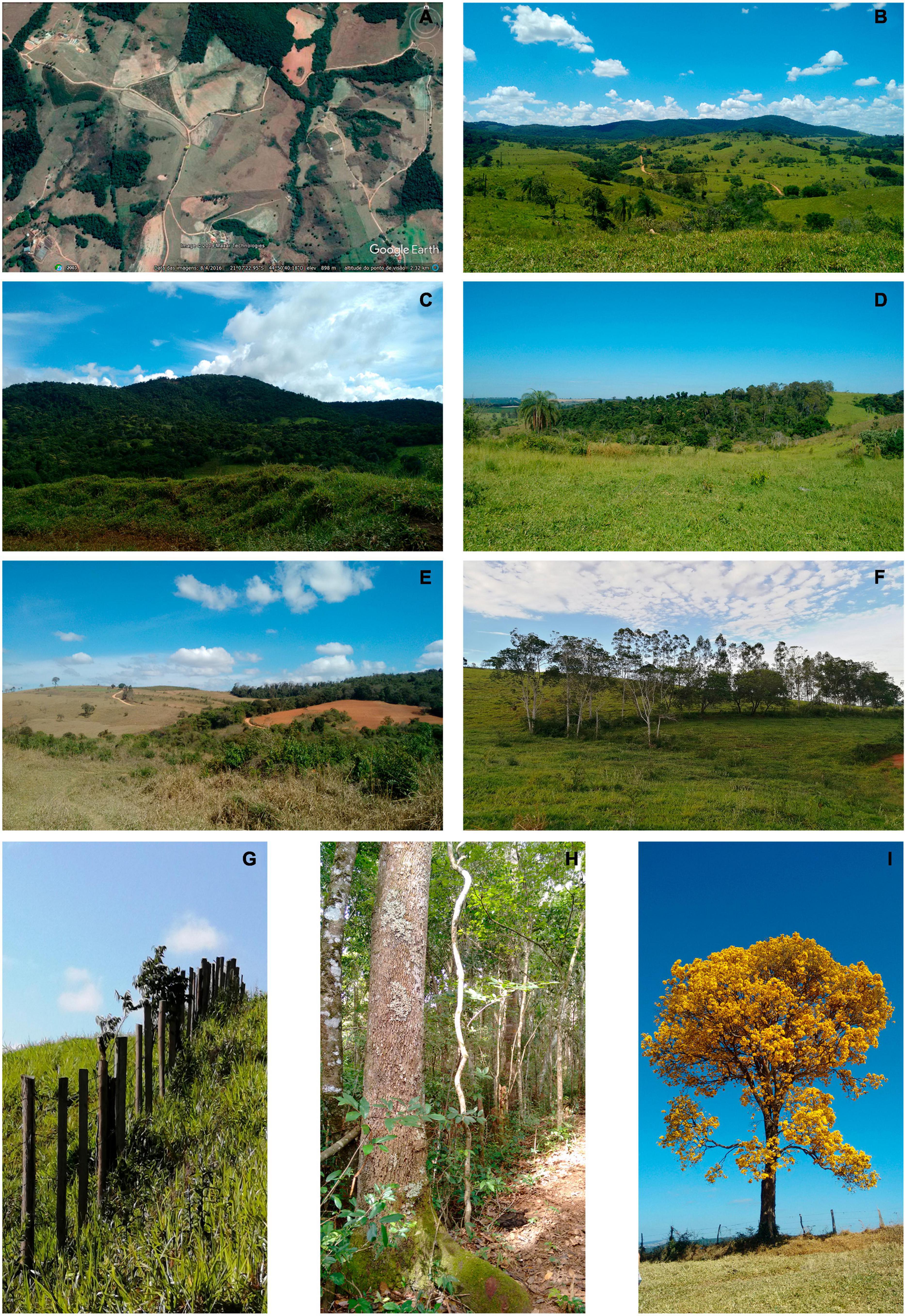
Figure 2. Small landscape elements (SLEs): (A) Landscape Google Earth with alls SLEs in Minas Gerais—Brazil, (B) local Atlantic Forest Domain, (C) Forest fragments < 1 ha, (D) Forest fragments < 1 ha, (E) Riparian forests, (F) Hedgerow-like tree lines colonizing barbed wire fences, (G) Hedgerow-like tree lines staring colonization for plants, (H) Trenches, (I) Scattered trees (Handroanthus sp.).
We identified every scattered tree in the landscapes using the images and checking them for true in the Google Earth images. Considering the RapidEye pixel size, we worked with scattered trees with crowns equal to or larger than 25 m2. To calculate the size of the crowns we randomly chose 800 scattered trees and measured their crown projections in the field. We found a mean area of 50 m2 (the smallest crown = 25 m2, the largest crown = 779.11 m2, SD = ± 63 m2) for scattered trees. Based on this information, the cover provided by each scatter tree was arbitrarily set to a fixed value of 50 m2 in all landscapes.
The trenches where hedgerow-like tree lines thrive in the region are on average 4 m wide and 1.5 m deep. Those trenches were naturally colonized by tree species, resulting in these hedgerow-like structures (Castro and van den Berg, 2013). Those linear structures are easily recognized in the images (10–20 m wide). We also mapped the tree lines along fences, separating them from the ones with trenches based on their smaller width (5–10 wide) and also using the RGB-532 band composition where older and denser forest cover (trenches) show darker color shade than tree lines following the fences.
We classified forest fragments as riparian forest when they followed the hydric net produced by the hydric flow model (ArcMap) added to the images.
In the case of clouds in the RapidEye images or doubts about the true classification during the mapping process, we first checked them in Google Earth images, Imagery—ArcGis and if the doubts persisted, we visited the areas.
Landscape Metrics
We calculated 14 metrics (Supplementary Table 1) for the landscapes in the ArcGis 10.5, extension “V-LATE 2.0” (Vector-based Landscape Analysis Tools Extension). Using those metrics (variables) we ran a Principal Component Analysis (PCA) and Spearman’s correlation using the 20 landscapes as replicas (Supplementary Figures 1, 2). Based on this, we excluded the high correlated variables resulting in a final set of seven variables for which we ran another PCA. The seven variables were (Lang and Blaschke, 2009):
1) NP (Number of SLEs): number of SLEs in the landscape.
2) TE (Total Edge): the sum of the patches’ perimeters.
3) MPE (Mean Patch Edge): the average of patches’ perimeters.
4) MSI (Mean Shape Index): it compares the patch’s shape with a circle of the same area. Closer is the value to 1, more circular is the shape.
5) MPAR (Mean Perimeter Area Ratio): the average for the landscape of the ratio of each patch perimeter and its area.
6) DIVISION (Division Index): it measures landscape fragmentation intensity, in other words, the probability that two randomly setpoints do not belong to the same undissected area. Further details in Jaeger (2000).
7) PLAND: measure the habitat amount.
A powerful tool for the indirect estimation of landscape connectivity is the use of graph theory together with the species population attributes (Pascual-Hortal and Saura, 2006; Ribeiro et al., 2019). Therefore, also calculated the Probability of Connectivity (Saura and Pascual-Hortal, 2007) to evaluate if the SLEs provide connectivity for fragments larger than 1 ha in the 20 landscapes. The PC is calculated based on the graph theory (further details in Saura and Pascual-Hortal, 2007) and reflects the probability of a successful dispersal event between knots in the landscape as a function of the Euclidean distance between one knot and the other. The PC varies from 0 to 1, where 0 is the absence of connectivity in the landscape, and 1 refers to a landscape where the knots are completely connected among themselves.
Where, n is the number of knots (SLEs and forest patches > 1 ha) in the landscape, ai and aj are the area of the knots i and j, Pij is the maximum probability of connection between the knots i and j (that depends to the functional distance a disperser can cross between knots), and Al is the landscape total area.
To evaluate the importance of the metrics of SLEs and patches > 1 ha for the connectivity, we broke the PC in 3 components: dPCintra, dPCmovement and dPCconnector (mathematical details in Saura and Rubio, 2010). The dPCintra (called here simply “area”) is the intrapatch connectivity, in other words, it is the contribution of the knot internal area to the connectivity. The dPCmovement (“flux”) is related to the knot area and its position in the landscape, representing how well is the knot connected to the other knots in the landscape. The dPCconnector (“position”) shows the importance of the knot position for the connectivity within the landscape (Saura and Rubio, 2010; Table 1).
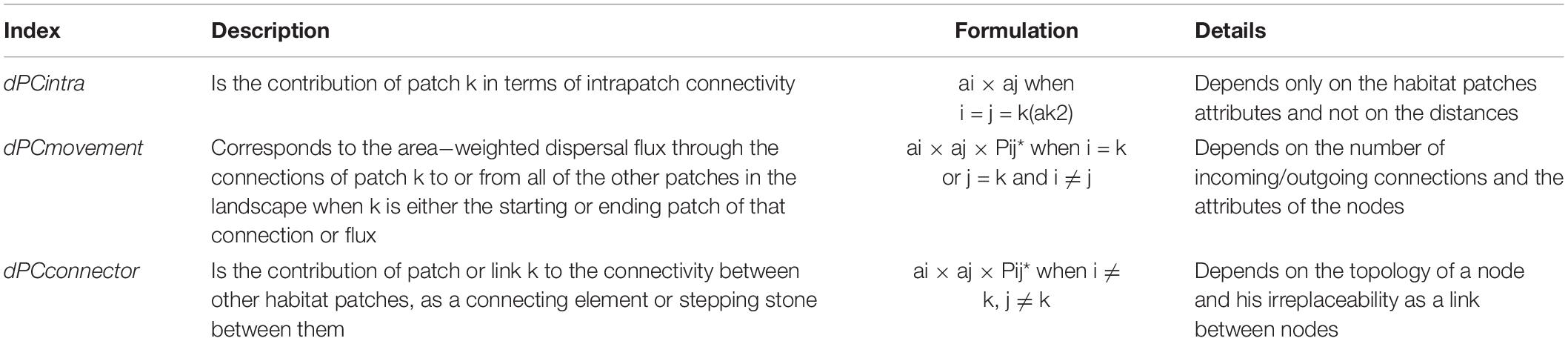
Table 1. Decomposition of PC index partitioned into three distinct fractions (intra, movement, and connector) considering the different ways in which a certain landscape (k) can contribute to habitat connectivity and availability in the landscape (Saura and Rubio, 2010).
Dispersal Distance for Probability of Connectivity Value
To calculate the Probability of Connectivity (Saura and Pascual-Hortal, 2007) we chose three arbitrary distances (50, 100, and 760 m) to represent dispersers with contrasting mobility and capacity of crossing open non-forested areas. At 50 m range are de pollen dispersers (e.g., insects), which can successfully transport pollen of Copaifera landsdorffii, a very abundant species in the whole region, at a distance of 50 m (Tarazi et al., 2010; Manoel, 2011); at 100 m range are the birds with a limited range of dispersal ability, such as Turdus (T. flavipes, T. albicollis, T. rufiventris, T. amaurochalinus, T. leucomela) and others forest understory species (Cadavid-Florez et al., 2020), called here as low-mobility seed disperser and at 760 m range are the more mobile species such as Ramphastos (R. tucanus, R. vitellinus) and others large forest canopy birds (Holbrook, 2011; Cadavid-Florez et al., 2020), called here high-mobility seed disperser.
Probability of Connectivity Models
To understand the role of the SLEs for connectivity among fragments > 1 ha, we ran models for the PC including and excluding the different SLEs. After including and excluding each SLEs, all landscape metrics have been recalculated. In the first model, all SLEs were removed from the landscapes. After that, for each model, we included only one of the SLEs. In the last model, we included all SLEs. We did all this process for the three different dispersal distances, resulting in 420 variations of the seven base models (7 models × 20 landscapes × 3 dispersal distances) (see Figure 3 for an example of the seven base models applied to the landscape L).
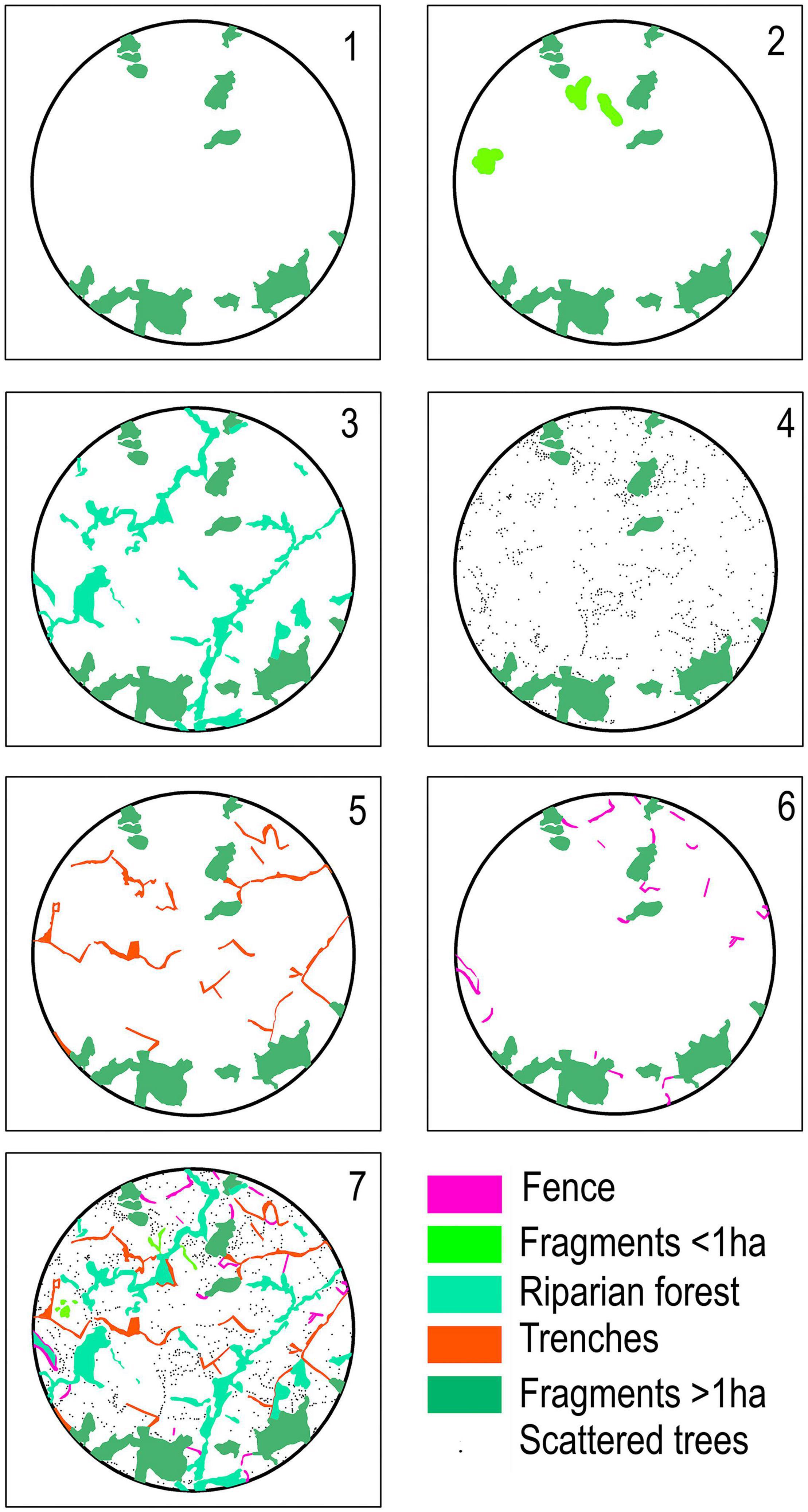
Figure 3. Example of the adopted seven base models for the PC (Probability of Connectivity) in the landscape. (1) Landscape without SLEs (only fragments > 1 ha); (2) Fragments < 1 ha (Fragments > 1 ha + fragments < 1 ha); (3) Riparian forests (Fragments > 1 ha + riparian forest); (4) Scattered trees (Fragments > 1 ha + scattered trees); (5) Trenches (Fragments > 1 ha + trenches); (6) Fences (Fragments > 1 ha + fences) and (7) Complete landscape (Fragments > 1 ha + fragments < 1 ha + riparian forest + scattered trees + trenches + fences).
We calculate the graph connections in the landscapes using the software Conefor 2.6 (Saura and Torne, 2009).
For each one of the 420 variations of the models, we produced a map with knots, the distance between the knots, and created a shapefile with the links between the knots. The results produced by the ArcGis using extension Conefor were: (1) the knot identities, (2) a matrix with Euclidean distances between knots, (3) a shapefile with the graphs. With these data, we calculated PC, dPCintra, dPCmovement, and dPCconector, in the software Conefor.
Statistical Analyses
We used Generalized Linear Models (GLM) to investigate how the landscape metrics affect the Probability of Connectivity. We ran models first relating PC to (i) the landscape metrics and SLEs metrics together and, after, relating PC to (ii) exclusively the SLEs metrics. For both models, the seed and pollen dispersers were included.
(i) PC - TE (total edge) + NP (number of patches) + MSI (Mean Shape Index) of SLEs + MPE (Mean Patch Edge) + MPAR (Mean Perimeter Area Ratio) + DIVISION (Division Index) + PLAND (amount habitat) + dispersers.
(ii) PC - SLEs area + flux between SLEs + position of SLEs + dispersers.
All models had normally distributed residuals (Shapiro–Wilk normality test, P < 0.05). We calculated for each candidate model the Akaike Information Criterion corrected for small samples (AICc), the Δi (= AICci - minimum AICc) and the Akaike weight (wi), which indicates the probability that the model i is the best model within the set.
We carried out all the statistical analysis in the software R (R Core Team, 2013). For the PCA, we used the package Vegan (Oksanen et al., 2010); for the GLM, and we used the package lme4 (Bates et al., 2014).
Results
Landscape Description
The landscapes were dominated by pastures (62.68%) followed by native tree cover (33.38%) and crops (3.02%). The other two classes water and eucalyptus plantations combined contributed to 0.92% of the land cover (Table 2 and Supplementary Figure 3). Considering exclusively native tree cover, fragments > 1 ha responded by 25.93% of the land cover and riparian forest for 4.46%. The other land cover of native tree species together added to 2.99%. Trenches (1.35%) had a greater contribution to land cover compared to fragments < 1 ha (0.87%). Hedgerow-like structures (trenches and fences) (1.67% together) responded for 55% of native tree cover, excluding fragments > 1 ha and riparian forest (Table 3 and Supplementary Figure 3).
We found that the forest cover in the landscapes is highly fragmented. The metric DIVISION (that measures the habitat splitting) gave values higher than 70% (Figure 4). Two landscapes had values under 70%: the landscape A (20%), that was chosen specifically because its amount of forest cover, and the landscape D (62.77%) (Figures 4, 5; with five landscape for example and Supplementary Figures 4A,B, all 20 landscapes in Supplementary Material).
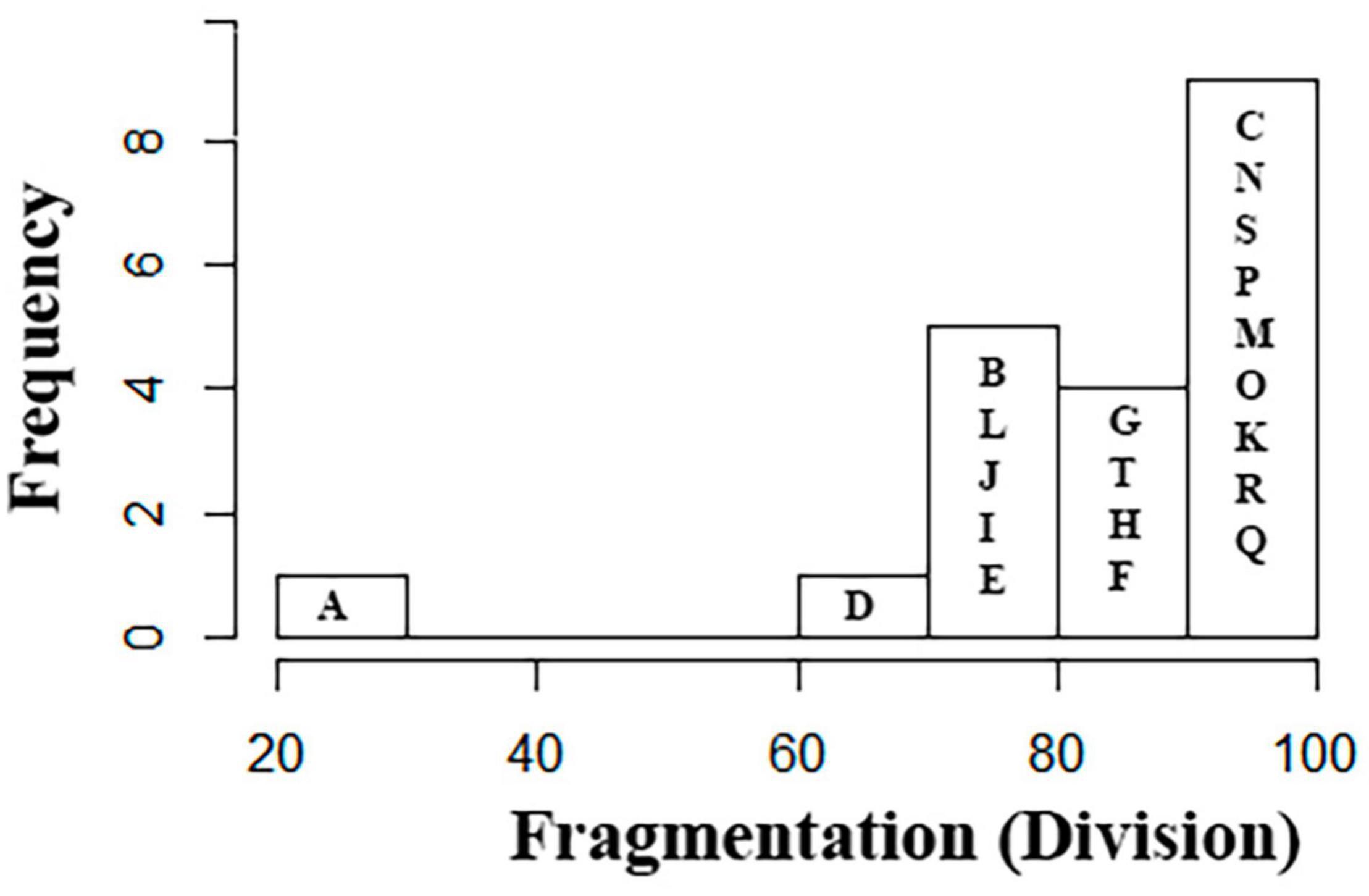
Figure 4. Histogram showing the distribution of the 20 landscapes in the fragmentation classes (metric DIVISION). The letters correspond to the landscapes on the map in Figure 1.
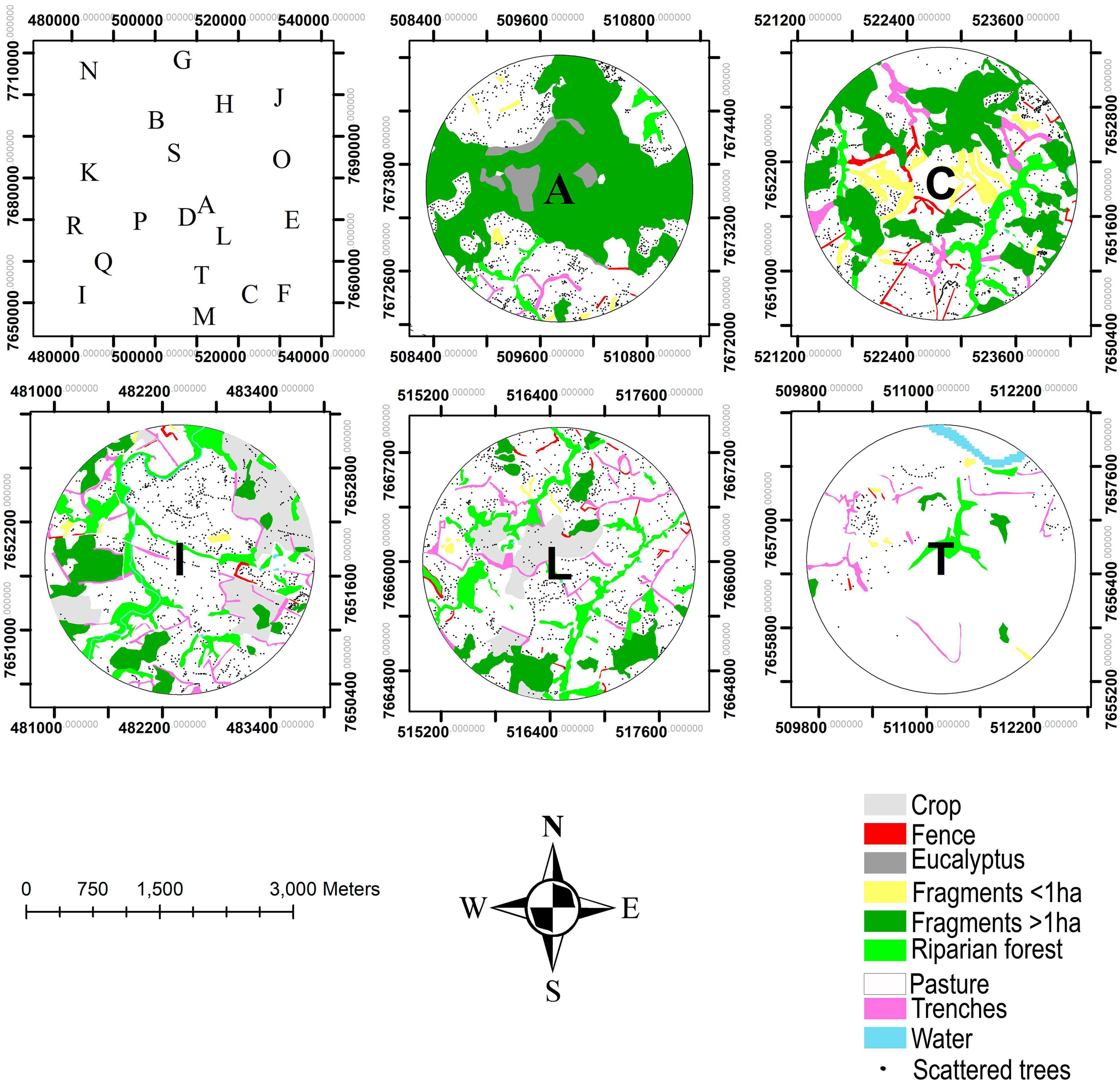
Figure 5. Example of land use maps for five landscapes identified by the characters in the center. The first frame represents the study area with the characters corresponding to the location of the 20 landscapes.
Probability of Connectivity
Connectivity responded positively to habitat amount (PLAND) and shape closer to a circle (MSI) and negatively to DIVISION, total edge length (TE), and mean patch edge length (MPE) (AICc = -294.14 e ΔAICc = 3.92; Figure 6A).
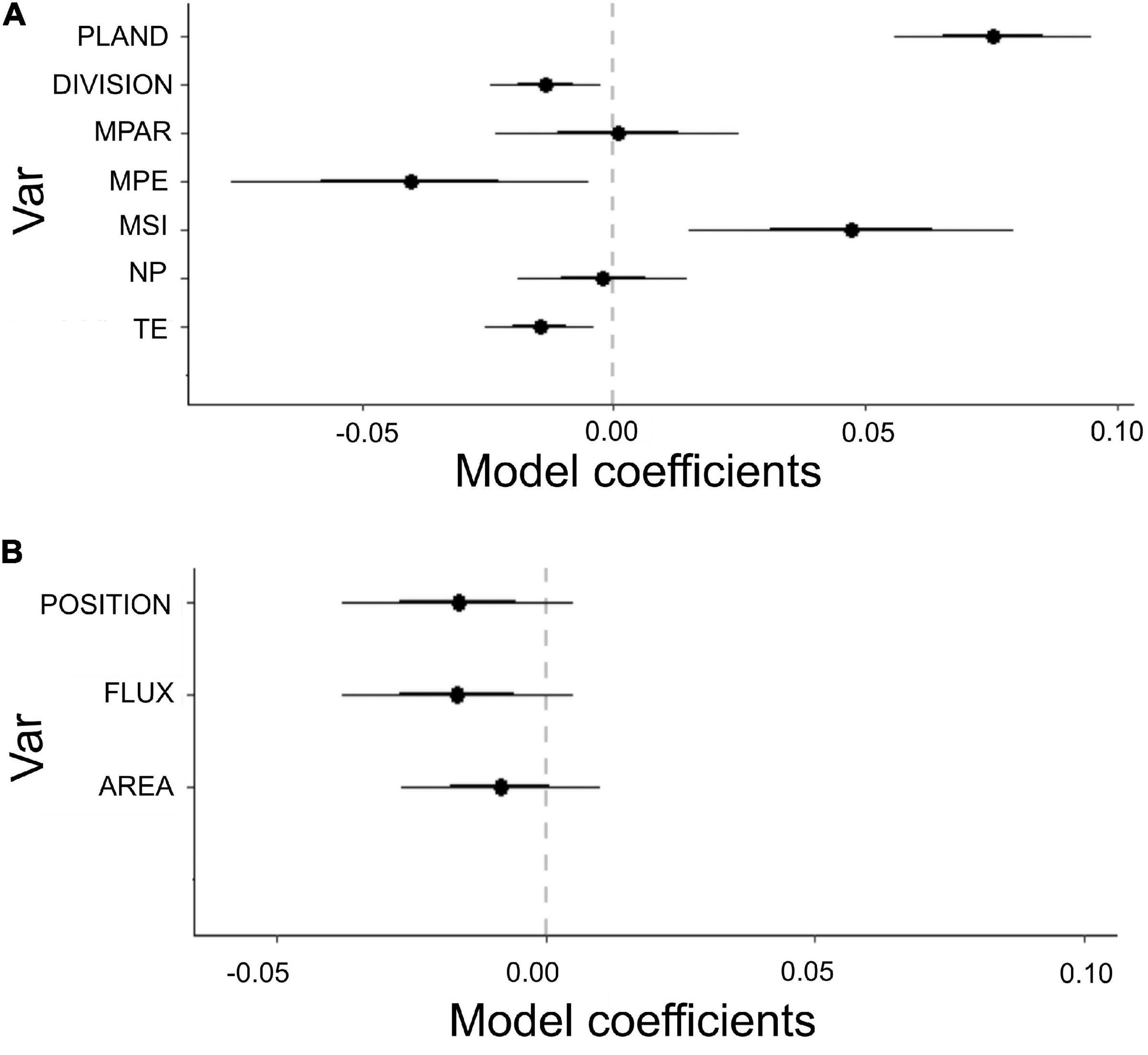
Figure 6. Estimated coefficients of the GLM models fitted to probability of connectivity (PC) when considering the mobility of different animals, (A) GLM as a function of landscape metrics: TE, total edge perimeter; NP, number of patches; MSI, patch shape; MPE, mean edge length for the patches; MPAR, patch shape complexity; DIVISION, fragmentation; PLAND, habitat amount. (B) GLM as a function of patch level metrics: FLUX, area and its position of the SLEs; POSITION, position of the SLEs for the connectivity within the landscape; AREA, SLEs area.
The geographical arrangement of the natural elements in the landscape (position) was more important for the connectivity than the elements’ area and flux (R = 0.12, p = 0.005, AICc = -142.7 and ΔAICc = 0.35; Figure 6B).
Probability of Connectivity and the SLEs
The presence of SLEs affected positively the connectivity in the studied landscapes (p = 0.00002). Riparian Forests were the most important ones for connectivity, followed by Trenches, Fences, Scattered trees, and Fragments < 1 ha (Figure 7).
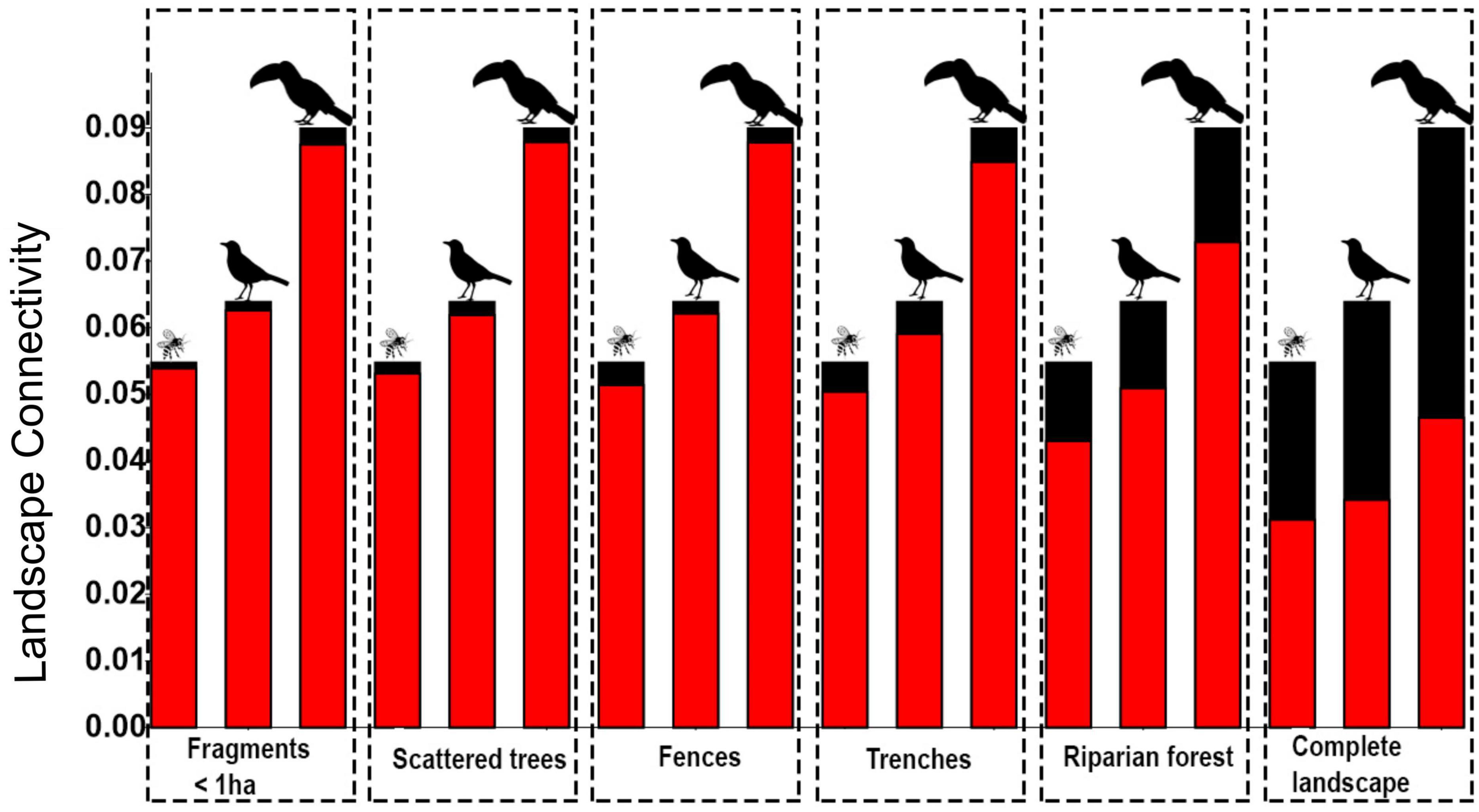
Figure 7. Connectivity (PC: the probability of connectivity) as a function of each SLE and as a function of all SLEs together (last frame). The red bars correspond to the PC without each particular SLE; the black bars indicate the effect of adding the focal SLE to the model. On top of the bars are the dispersers: insects (pollinators), low-mobility seed dispersers (smaller birds), and high-mobility seed dispersers (large body birds). Connectivity was measured in meters.
The greater was the fragmentation, the larger was the SLEs contribution to the connectivity (p < 0.05 and R2 > 0.54; Supplementary Figures 5A–C). The connectivity was less affected by fragmentation for the models with high-mobility seed disperser than for the models with low-mobility seed disperser and pollinators (Figure 7).
The presence of SLEs improved the number of links between knots (R = 0.955 and p < 0.0001; Figure 8 and Supplementary Figure 6 with a zoom of the links in Supplementary material). The number of links decreased with the decrease of disperser distance capability.
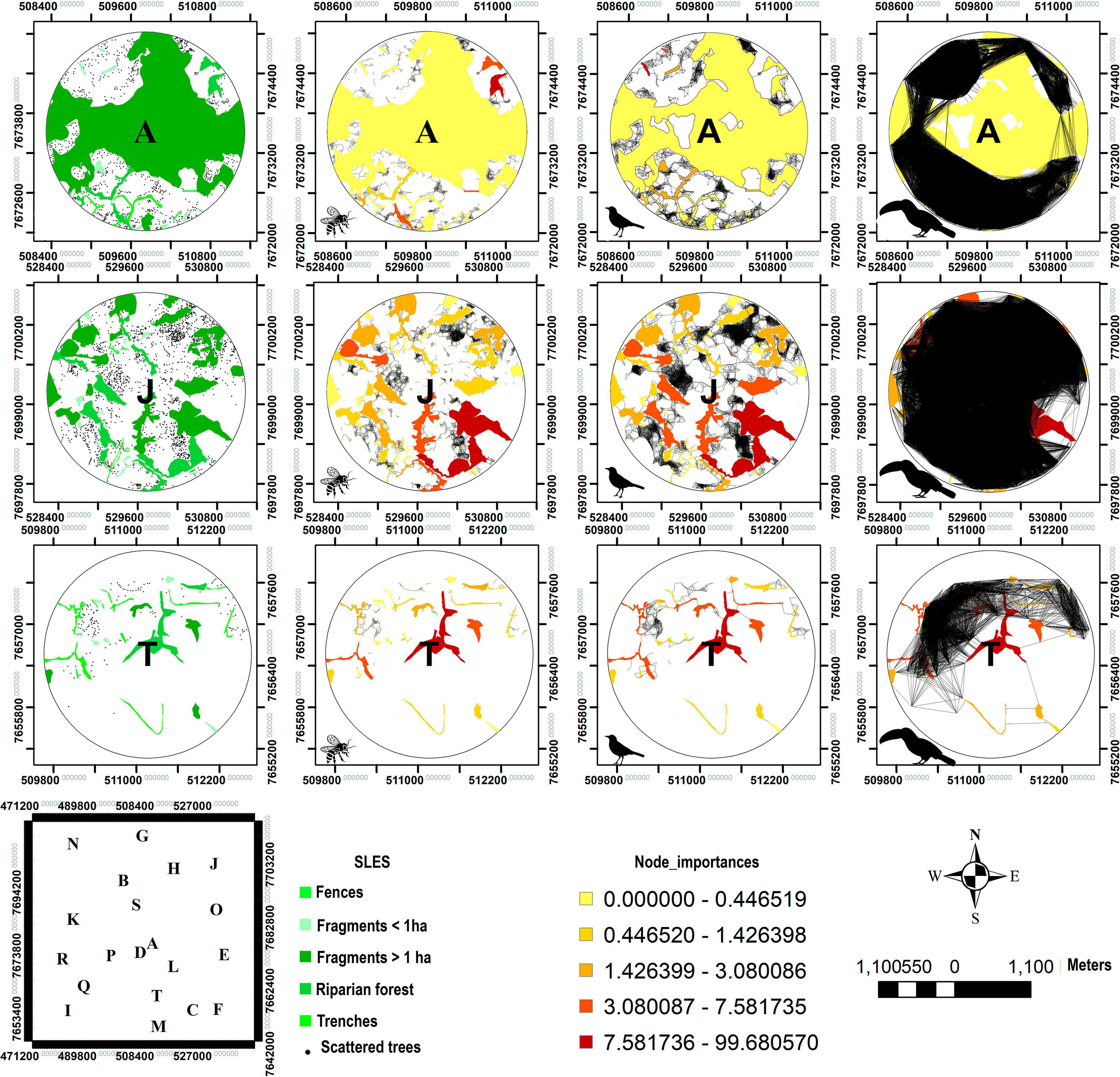
Figure 8. Graph models for connectivity. Graph representation of connectivity for three dispersers in three landscapes (A, J, and T), where A has the largest, J has an intermediary and T has the lowest amount of forest cover among the 20 analyzed landscapes. The first column shows the real tree cover of the landscapes; the second one shows the l connectivity for pollinators; the third one for the seed disperser Turdus; and the last one for the seed disperser Ramphastos. The black lines represent the graph links between knots and the orange color palette shows the importance of each knot (SLE). The polygon with the letters A-T shows the geographic position of each landscape.
Discussion
Small landscape elements (SLEs) are a common feature of the Atlantic Forest and agricultural landscape of South of Minas Gerais State, south-eastern Brazil. These SLEs also greatly increased connectivity in the landscape. Land sparing strategies are the cornerstone for conservation, however, most of the original area of distribution of the Brazilian Atlantic Forest is distributed across private lands and extremely fragmented (Rezende et al., 2018) and few opportunities exist for creating new full protected areas. Without denying the importance of this strategy, conservation in agricultural lands (land sharing) is a necessity for Atlantic Forest and other highly fragmented tropical forests around the world. Turning the economic activities in private lands more social, economic and environmentally sustainable, is a powerful complement to land sparing strategies. In this context, protection and restoration of SLEs is essential considering their value for biodiversity conservation, for example by considering how they contribute toward enhancing landscape connectivity (Castro and van den Berg, 2013; Siqueira et al., 2017). The SLEs, as we showed here, occupy a relatively small space in the agricultural lands (∼7.5% including trenches, fences, scattered trees, and riparian forests), however, they can strongly promote connectivity, doubling it when including all them together.
We found that the Atlantic Forest is highly fragmented within the studied region. The metric DIVISION showed that on average, the fragmentation was 82% (maximum possible value would be 100%), with landscape R with 96% and the one with the lowest fragmentation (and largest forest cover), the landscape A, with 20%. The forest cover was mostly replaced by pasture, but also by water (a reservoir), agriculture, and eucalyptus forest plantation. In the last 30 years, very little forest clearing occurred, however, prior to the end of the 1980s, legal protection for the forests was much weaker. During this period, the Brazilian environmental legislation was still being formulated [Article 225, Constitution of 1988 (Brasil, 1988)] and the existing legislation was poorly enforced. Also, during this period, many properties had more forest cover than the legislation demanded (Legal Reserve: 20% of the property, and Permanently Protected Area: a variable amount related to areas following watercourses and hills tops), resulting in legal forest clearing for economic activities. Nowadays, besides the fact most forest in the properties is legally protected (Law No. 12.651 of 2012, Brasil, 2012), also the forest in the region is considered officially part of the Atlantic Forest, a protected biome (Law No. 11.428 of 2006, Brasil, 2006), therefore, the forest in the region is doubled protected. Environmental law enforcement is also much more efficient now, using remote images and, often, being supported by the population, that observe and denounce illegal forest clearing.
However, although the previous Brazilian environmental legislation (Law No. 4.771 of 1965, Brasil, 1965) did not cover all environmental issues and was rather poorly imposed, it did have a positive effect on preserving riparian forests. This law conditioned the width of the forest protected to the width of the watercourse. This impact is clear on the amount of riparian forest compared to the other SLEs. Not only that, although the law protected those areas exclusively because of the hydric resources, as an indirect consequence, the connectivity between larger fragments was also favored, being the Riparian Forest the most important SLE for connectivity in the studied landscapes. This legislation was substituted by Law No. 12.651 of 2012 (Brasil, 2012) which establishes that the width of forest along the watercourse depends on the size of the property (Soares-Filho et al., 2014), allowing smaller properties to have narrower protected riparian areas. Because of that, the riparian forests are threatened to be reduced in the next years.
Like other studies (Fahrig, 2003; Banks−Leite et al., 2011; Costanza et al., 2019), we showed that connectivity was positively related to less fragmented landscapes, with more habitat and proportionally less edge, and patches with a circular shape. However, we pointed out that not only the presence of SLEs can promote connectivity but also their arrangement in the landscape, as example we found that the position of SLEs within the landscapes is more important than their respective areas for connectivity.
Considering all the SLEs together, the linear elements in the landscape—the riparian forest and the hedgerow-like elements (Trenches and Fences)—were the most important ones for promoting connectivity. However, although the SLEs vary in their effectiveness, they seem to have complementary roles, since although none of them alone contribute to much more than 20% of the connectivity, they all together increased the connectivity in 50% for all analyzed model dispersers.
One of the most important negative consequences of fragmentation is the isolation of populations. Fragmentation decreases pollen and seed dispersal affecting genetic flow (Hamilton, 1999). This dispersal loss is clearly related to the negative effect of fragmentation on the bird community and populations (Bovo et al., 2018). Birds are responsible for long-distance seed dispersal (Clark et al., 2004) promoting plants’ genetic exchange and diversity (Tarazi et al., 2010, 2013; Carvalho et al., 2015). The SLEs contribute to connect the fragments and increase genetic flow. Also, the connectivity provided by the SLEs can be very important to the landscape resilience, since birds can change they movement according to the level of landscape isolation (Giubbina et al., 2018), by using stepping-stones, using more pasture or moving farther (Ramos et al., 2020). Consider the example of the scattered trees in pastures, where Siqueira et al. (2017) observed a much larger deposition of animal dispersed seeds under scattered trees than in corresponding areas without the trees. In the present study the landscape J (26.88% of habitat), with 1,526 scattered trees, had 6,927 links for insects: 18,801 links for birds with a limited range of dispersal ability and 302,389 links for large forest canopy birds. However, the landscape K (26.25% of habitat), with 723 scattered trees, had only 1,341 links for insects, 3,413 links for birds with a limited range of dispersal ability and 54,546 links for large forest canopy birds.
In most landscapes, the open non-forested areas did not present itself as a barrier for the high-mobility seed dispersers movement, which are large body birds, however, it was a limitation for low-mobility seed dispersers (smaller birds) and particularly for pollen dispersers insects). This result was expected since large-bodied species, may exhibit higher movement capacity (Spiegel and Nathan, 2007; Neuschulz et al., 2013; Ramos et al., 2020) and, to ensure sufficient resources, should be more likely to change their behavior in response to variation in resource distribution than small-bodied species (Buchmann et al., 2012; Ramos et al., 2020).
For pollen dispersers with relatively short dispersal distance (in our model was 50 m), the open non-forested areas can be a strong barrier. In this case, the SLEs can work as corridors or stepping stones, connecting larger forest fragments. For pollinators of Copaifera langsdorffii, the species for which we shaped pollen dispersal distance for the model, areas without forest can be an insurmountable barrier (Tarazi et al., 2013). On the other hand, the large forest canopy birds can cross wide-open areas holding seeds, even large ones (30 mm of diameter) (Galetti et al., 2013; Emer et al., 2019). Therefore, specifically for pollinators and for birds with a limited range of dispersal ability, the SLEs can allow some seed and pollen exchanges between areas otherwise isolated. Nevertheless, for any disperser evaluated, the SLEs increased the connectivity more or less in the same proportion. In addition, we recommend that future research that considers species-specific dispersion distances be carried out.
In the case of pollinators, besides riparian forest, fences and trenches were especially relevant. For animals with little mobility in the non-forest habitats, linear tree structures connecting larger fragments are essential. Those linear structures, besides providing a friendly habitat for locomotion, also provide resources, particularly for insects since they have high sunlight exposition, and consequently larger flowering (Emer et al., 2019).
Although large and protected areas are fundamental for conservation (Crouzeilles et al., 2013), many times those areas are far apart and unconnected to each other. In Brazil, principally in the areas with higher and older European occupation, like the Atlantic Forest, this isolation is even more severe (Ward et al., 2020). We showed here that the SLEs present in private agricultural lands can double the connectivity among larger forest fragments, and, in the same way, between protected areas. In the region of the original Atlantic Forest, most of the large forest patches disappeared or are already in protected areas. This region concentrates 70% of the human population (IBGE, 2020) and has high land prices. In such conditions, conservation in private agricultural lands is essential as a complement to other strategies like forest restoration and protection of the last large remnants. However, very little attention has been given to the SLEs and, most times, they are even not included in the landscape maps (Haddad et al., 2015). Also, there is no specific legislation to protect them. We showed here how we urgently need to change or approach concerning the SLEs, even if we are only thinking about ecological connectivity.
Besides connectivity, the SLEs provide other important ecological services. For example, riparian forests protect watercourses increasing water quality and reducing sediment input (Dosskey et al., 2010). The trenches associated with the hedgerows provide conditions of temperature and moisture that allow the establishment of trees typical of forest interior (Castro and van den Berg, 2013). The barbed-wire fences, the scattered trees, and fragments < 1 ha provide habitat and food for the fauna and also work as nucleation spots for forest re-establishment (Sandor and Chazdon, 2014).
Considering all the above, we advocate for the SLEs are important components for conservation, providing large and irreplaceable connectivity in private agricultural lands and even between protected areas. Most SLEs do not conflict or conflict little with other economic activities in the properties, even providing some services perceived by the owners (like shade provided by the scattered trees to the cattle (Siqueira et al., 2017), or water quality linked to the riparian forests). In Brazil, there is no legislation directly protecting SLEs besides riparian forests, which are only partially protected. Legal protection for SLEs is urgent as well as including them in the studies evaluating connectivity and human-disturbed landscapes.
Data Availability Statement
The original contributions presented in the study are included in the article/Supplementary Material, further inquiries can be directed to the corresponding author/s.
Author Contributions
FS, DC, and EB: study conception and design. FS: data collection and computer simulations. FS and CA: data analysis and interpretation. FS, DC, JR, CA, VR, and EB: manuscript writing. DC, JR, CA, VR, and EB: review and editing. DC and EB: funding acquisition. All authors contributed to the article and approved the submitted version.
Conflict of Interest
The authors declare that the research was conducted in the absence of any commercial or financial relationships that could be construed as a potential conflict of interest.
Acknowledgments
We thank Manoel Siqueira, Angelim Tucunduva, Gabriel Caramaschi, Paula Coelho, Gabriela Carlos, Sara Bastos, Mateus Cardoso, and Daniela Domingos, for the help with the fieldwork. This work was supported by CAPES (Coordination for the Improvement of Higher Education Personnel); CNPq (National Council for Scientific and Technological Development); and FAPEMIG (Minas Gerais Research Funding Foundation).
Supplementary Material
The Supplementary Material for this article can be found online at: https://www.frontiersin.org/articles/10.3389/fevo.2021.614362/full#supplementary-material
References
Banks−Leite, C., Ewers, R. M., Kapos, V., Martensen, A. C., and Metzger, J. P. (2011). Comparing species and measures of landscape structure as indicators of conservation importance. J. Appl. Ecol. 48, 706–714. doi: 10.1111/j.1365-2664.2011.01966.x
Banks-Leite, C., Pardini, R., Tambosi, L. R., Pearse, W. D., Bueno, A. A., Bruscagin, R. T., et al. (2014). Using ecological thresholds to evaluate the costs and benefits of set-asides in a biodiversity hotspot. Science 345, 1041–1045. doi: 10.1126/science.1255768
Barlow, J., Lennox, G. D., Ferreira, J., Berenguer, E., Lees, A. C., Nally, R., et al. (2016). Anthropogenic disturbance in tropical forests can double biodiversity loss from deforestation. Nat. Adv. Online Publicat. 2016:nature18326. doi: 10.1038/nature18326
Bates, D., Mächler, M., Bolker, B., and Walker, S. (2014). Fitting linear mixed-effects models using lme4. arXiv 1406.5823. [preprint]. doi: 10.18637/jss.v067.i01
Baudry, J., Bunce, R., and Burel, F. (2000). Hedgerows: an international perspective on their origin, function and management. J. Environ. Manage. 60, 7–22. doi: 10.1006/jema.2000.0358
Bovo, A. A. A., Ferraz, K. M. P. M. B., Magioli, M., Alexandrinoa, E. R., Hasui, E., Ribeiro, M. C., et al. (2018). Habitat fragmentation narrows the distribution of avian functional traits associated with seed dispersal in tropical forest. Perspect. Ecol. Conserv. 16, 90–96. doi: 10.1016/j.pecon.2018.03.004
Brasil (2000). Lei Federal n° 9.985, de 18 de julho de 2000. Brasília: Diário Oficial [da] República Federativa do Brasil.
Brasil (2006). Lei n° 11.428, de 22 de dezembro de 2006. Brasília: Diário Oficial [da] República Federativa do Brasil.
Brasil (2012). Lei N° 12.651, de 25 de maio de 2012. Brasília: Diário Oficial [da] República Federativa do Brasil.
Buchmann, C. M., Jeltsch, F., and Schurr, F. (2012). Movement upscaled – the importance of individual foraging movement for community response to habitat loss. Ecography 35, 436–445. doi: 10.1111/j.1600-0587.2011.06924.x
Cadavid-Florez, L., Laborde, J., and Mclean, D. J. (2020). Isolated trees and small woody patches greatly contribute to connectivity in highly fragmented tropical landscapes. Landscape Urban Plann. 196:103745. doi: 10.1016/j.landurbplan.2020.103745
Carvalho, C., Ribeiro, M., Côrtes, M., Galetti, M., and Collevatti, R. (2015). Contemporary and historic factors influence differently genetic differentiation and diversity in a tropical palm. Heredity 115:216. doi: 10.1038/hdy.2015.30
Castro, G. C., and van den Berg, E. (2013). Structure and conservation value of high-diversity hedgerows in southeastern Brazil. Biodivers. Conservat. 22, 2041–2056. doi: 10.1007/s10531-013-0524-2
Charlesworth, D., and Willis, J. (2009). The genetics of inbreeding depression. Nat. Rev. Genet. 10, 783–796. doi: 10.1038/nrg2664
Clark, C., Poulsen, J., Connor, E., and Parker, V. (2004). Fruiting trees as dispersal foci in a semi-deciduous tropical forest. Oecologia 139, 66–75. doi: 10.1007/s00442-003-1483-1
Costanza, J. K., Riitters, K., Vogt, P., and Wickham, J. (2019). Describing and analyzing landscape patterns: where are we now, and where are we going?. Berlin: Springer.
Crouzeilles, R., Lorini, M. L., and Grelle, C. E. V. (2013). The importance of using sustainable use protected areas for functional connectivity. Biol. Conservat. 159, 450–457. doi: 10.1016/j.biocon.2012.10.023
Dantas, A. A. A., Carvalho, L. G. D., and Ferreira, E. (2007). Classificação e tendências climáticas em Lavras, MG. Ciência Agrotecnol. 31, 1862–1866.
Dobson, A. P., Bradshaw, A. D., and Baker, A. J. M. (1997). Hopes for the future: restoration ecology and conservation biology. Science 277, 515–522. doi: 10.1126/science.277.5325.515
dos Santos, H., Carvalho Junior, W. D., Dart, R. D. O., Áglio, M., de Sousa, J., Pares, J., et al. (2011). O novo mapa de solos do Brasil: legenda atualizada. Rio de janeiro: Embrapa Solos.
Dosskey, M. G., Vidon, P., Gurwick, N. P., Allan, C. J., Duval, T. P., and Lowrance, R. (2010). The role of riparian vegetation in protecting and improving chemical water quality in streams 1. JAWRA J. Am. Water Resour. Associat. 46, 261–277. doi: 10.1111/j.1752-1688.2010.00419.x
Emer, C., Galetti, M., Pizo, M. A., Jordano, P., and Verdú, M. (2019). Defaunation precipitates the extinction of evolutionarily distinct interactions in the Anthropocene. Sci. Adv. 5:eaav6699. doi: 10.1126/sciadv.aav6699
Fahrig, L. (2003). Effects of habitat fragmentation on biodiversity. Annu. Rev. Ecol. Evolut. Systemat. 34, 487–515. doi: 10.1146/annurev.ecolsys.34.011802.132419
Fitton, N., Alexander, P., Arnell, N., Bajzelj, B., Calvin, K., Doelman, J., et al. (2019). The vulnerabilities of agricultural land and food production to future water scarcity. Glob. Environ. Change 58:101944. doi: 10.1016/j.gloenvcha.2019.101944
Galetti, M., Guevara, R., Côrtes, M. C., Fadini, R., Von Matter, S., Leite, A. B., et al. (2013). Functional extinction of birds drives rapid evolutionary changes in seed size. Science 340, 1086–1090. doi: 10.1126/science.1233774
Garibaldi, L. A., Aizen, M. A., Klein, A. M., Cunningham, S. A., and Harder, L. D. (2011). Global growth and stability of agricultural yield decrease with pollinator dependence. Proc. Natl. Acad. Sci. 108, 5909–5914. doi: 10.1073/pnas.1012431108
Giubbina, M. F., Martensen, A. C., and Ribeiro, M. C. (2018). Sugarcane and Eucalyptus plantation equally limit the movement of two forest-dependent understory bird species. Austral. Ecol. 43, 527–533. doi: 10.1111/aec.12589
Haddad, N. M., Brudvig, L. A., Clobert, J., Davies, K. F., Gonzalez, A., Holt, R. D., et al. (2015). Habitat fragmentation and its lasting impact on Earth’s ecosystems. Sci. Adv. 1:e1500052. doi: 10.1126/sciadv.1500052
Hamilton, M. B. (1999). Tropical tree gene flow and seed dispersal. Nature 401:129. doi: 10.1038/45142
Hedrick, P. W., and Garcia-Dorado, A. (2016). Understanding Inbreeding Depression, Purging, and Genetic Rescue. Trends Ecol. Evolut. 31, 940–952. doi: 10.1016/j.tree.2016.09.005
Holbrook, K. M. (2011). Home range and movement patterns of toucans: implications for seed dispersal. Biotropica 43, 357–364. doi: 10.1111/j.1744-7429.2010.00710.x
Jaeger, J. A. (2000). Landscape division, splitting index, and effective mesh size: new measures of landscape fragmentation. Landscape ecology 15, 115–130. doi: 10.1023/A:1008129329289
Joly, C. A., Metzger, J. P., and Tabarelli, M. (2014). Experiences from the Brazilian A tlantic F orest: ecological findings and conservation initiatives. N. Phytol. 204, 459–473. doi: 10.1111/nph.12989
Keller, D., van Strien, M. J., Herrmann, M., Bolliger, J., Edwards, P. J., Ghazoul, J., et al. (2013). Is functional connectivity in common grasshopper species affected by fragmentation in an agricultural landscape? Agricult. Ecosyst. Environ. 175, 39–46. doi: 10.1016/j.agee.2013.05.006
Kremen, C., and Merenlender, A. (2018). Landscapes that work for biodiversity and people. Science 362:eaau6020. doi: 10.1126/science.aau6020
Laurance, W. F., Camargo, J. L., Luizão, R. C., Laurance, S. G., Pimm, S. L., Bruna, E. M., et al. (2011). The fate of Amazonian forest fragments: a 32-year investigation. Biol. Conserv. 144, 56–67. doi: 10.1016/j.biocon.2010.09.021
Levis, C., Flores, B. M., Mazzochini, G. G., Manhães, A. P., Campos-Silva, J. V., de Amorim, P. B., et al. (2020). Help restore Brazil’s governance of globally important ecosystem services. Nat. Ecol. Evolut. 4, 172–173. doi: 10.1038/s41559-019-1093-x
Lewis, S. L., Edwards, D. P., and Galbraith, D. (2015). Increasing human dominance of tropical forests. Science 349, 827–832. doi: 10.1126/science.aaa9932
Manoel, R. D. O. (2011). Distância e padrões de dispersão contemporânea de pólen e sistema de reprodução em pequeno fragmento isolado de Copaifera Langsdorfii Desf.(Leguminosae–Caesalpinoideae). São Paulo: Ilha Solteira.
Mello, A. C. T. D., Carnevalli, R. A., Shiratsuchi, L. S., Lopes, L. B., and Xavier, D. B. (2017). Improved grazing activity of dairy heifers in shaded tropical grasslands. Ciência Rural 47:20160316. doi: 10.1590/0103-8478cr20160316
Melo, F. P., Arroyo-Rodríguez, V., Fahrig, L., Martínez-Ramos, M., and Tabarelli, M. (2013). On the hope for biodiversity-friendly tropical landscapes. Trends Ecol. Evolut. 28, 462–468. doi: 10.1016/j.tree.2013.01.001
Moore, R. P., Robinson, W. D., Lovette, I. J., and Robinson, T. R. (2008). Experimental evidence for extreme dispersal limitation in tropical forest birds. Ecol. Lett. 11, 960–968. doi: 10.1111/j.1461-0248.2008.01196.x
Neuschulz, L., Brown, M., and Farwig, N. (2013). Frequent bird movements across a highly fragmented landscape: the role of species traits and forest matrix. Anim. Conservat. 2013, 170–179. doi: 10.1111/j.1469-1795.2012.00582.x
Oksanen, J., Blanchet, F. G., Kindt, R., Legendre, P., O’hara, R., Simpson, G. L., et al. (2010). Vegan: community ecology package. R package version 1.17-4. Vienna: R Core Team.
Paciullo, D. S. C., de Castro, C. R. T., de Miranda Gomide, C. A., Maurício, R. M., and Pires, M. D. F. Á, Müller, M. D. et al. (2011). Performance of dairy heifers in a silvopastoral system. Livestock Sci. 141, 166–172. doi: 10.1016/j.livsci.2011.05.012
Pact for the Restoration of the Atlantic Forest (2016). Theoretical Referential. Available online at: https://www.pactomataatlantica.org.br/referential-documents (accessed December, 2019).
Pardini, R., de Arruda Bueno, A., Gardner, T. A., Prado, P. I., and Metzger, J. P. (2010). Beyond the fragmentation threshold hypothesis: regime shifts in biodiversity across fragmented landscapes. PLoS One 5:e13666. doi: 10.1371/journal.pone.0013666
Pascual-Hortal, L., and Saura, S. (2006). Comparison and development of new graph-based landscape connectivity indices: towards the priorization of habitat patches and corridors for conservation. Landscape ecology 21, 959–967. doi: 10.1007/s10980-006-0013-z
Prevedello, J. A., Almeida−Gomes, M., and Lindenmayer, D. B. (2018). The importance of scattered trees for biodiversity conservation: A global meta−analysis. J. Appl. Ecol. 55, 205–214. doi: 10.1111/1365-2664.12943
R Core Team (2013). R: A language and environment for statistical computing. Vienna: R Foundation for Statistical Computing.
Ramos, D. L., Pizo, M. A., Ribeiro, M. C., Cruz, R. S., Morales, J. M., and Ovaskainen, O. (2020). Forest and connectivity loss drive changes in movement behavior of bird species. Ecography 43:4888. doi: 10.1111/ecog.04888
Rezende, C., Scarano, F., Assad, E., Joly, C., Metzger, J., Strassburg, B., et al. (2018). From hotspot to hopespot: An opportunity for the Brazilian Atlantic Forest. Perspect. Ecol. Conservat. 16, 208–214. doi: 10.1016/j.pecon.2018.10.002
Ribeiro, J., Colli, G. R., and Soares, A. (2019). Landscape correlates of anuran functional connectivity in rice crops: a graph-theoretic approach. J. Trop. Ecol. 35, 118–131. doi: 10.1017/S026646741900004X
Rocha, M. F., Passamani, M., and Yankous Gonçalves, and Fialho, M. (2014). Can vegetation corridors provide habitat in fragmented landscapes? A case-study in the Brazilian Atlantic Domain. Stud. Neotrop. Fauna Environ. 49, 180–184. doi: 10.1080/01650521.2014.952533
Sandor, M. E., and Chazdon, R. L. (2014). Remnant trees affect species composition but not structure of tropical second-growth forest. PLoS One 9:0083284. doi: 10.1371/journal.pone.0083284
Saturni, F. T., Jaffé, R., and Metzger, J. P. (2016). Landscape structure influences bee community and coffee pollination at different spatial scales. Agricult. Ecosyst. Environ. 235, 1–12. doi: 10.1016/j.agee.2016.10.008
Saura, S., and Pascual-Hortal, L. (2007). A new habitat availability index to integrate connectivity in landscape conservation planning: comparison with existing indices and application to a case study. Landscape Urban Plann. 83, 91–103. doi: 10.1016/j.landurbplan.2007.03.005
Saura, S., and Rubio, L. (2010). A common currency for the different ways in which patches and links can contribute to habitat availability and connectivity in the landscape. Ecography 33, 523–537. doi: 10.1111/j.1600-0587.2009.05760.x
Saura, S., and Torne, J. (2009). Conefor Sensinode 2.2: a software package for quantifying the importance of habitat patches for landscape connectivity. Environ. Model. Softw. 24, 135–139. doi: 10.1016/j.envsoft.2008.05.005
Siqueira, F. F., Calasans, L. V., Furtado, R. Q., Carneiro, V. M. C., and van den Berg, E. (2017). How scattered trees matter for biodiversity conservation in active pastures. Agricult. Ecosyst. Environ. 250, 12–19. doi: 10.1016/j.agee.2017.08.002
Soares-Filho, B., Rajão, R., Macedo, M., Carneiro, A., Costa, W., Coe, M., et al. (2014). Cracking Brazil’s forest code. Science 344, 363–364. doi: 10.1126/science.1246663
Spiegel, O., and Nathan, R. (2007). Incorporating dispersal distance into the disperser effectiveness framework: frugivorous birds provide complementary dispersal to plants in a patchy environment. Ecol. Lett. 10, 718–728. doi: 10.1111/j.1461-0248.2007.01062.x
Tarazi, R., Sebbenn, A. M., Mollinari, M., and Vencovsky, R. (2010). Mendelian inheritance, linkage and linkage disequilibrium in microsatellite loci of Copaifera langsdorffii Desf. Conserv. Genet. Resour. 2, 201–204. doi: 10.1007/s12686-010-9230-5
Tarazi, R., Sebbenn, A., Kageyama, P., and Vencovsky, R. (2013). Edge effects enhance selfing and seed harvesting efforts in the insect-pollinated Neotropical tree Copaifera langsdorffii (Fabaceae). Heredity 110:578. doi: 10.1038/hdy.2013.8
Taubert, F., Fischer, R., Groeneveld, J., Lehmann, S., Müller, M. S., Rödig, E., et al. (2018). Global patterns of tropical forest fragmentation. Nature 554:519. doi: 10.1038/nature25508
Taylor, P. D., Fahrig, L., Henein, K., and Merriam, G. (1993). Connectivity is a vital element of landscape structure. Oikos 1993, 571–573. doi: 10.2307/3544927
Taylor, P. D., Fahrig, L., and With, K. A. (2006). “Landscape connectivity: a return to the basics,” in The Connectivity Conservation, ed. K. R. Crooks (Cambridge: Cambridge University Press), 29–43.
Tischendorf, L., and Fahrig, L. (2000). On the usage and measurement of landscape connectivity. Oikos 90, 7–19. doi: 10.1034/j.1600-0706.2000.900102.x
Tisovec, C., Dufner, K., Teixeira, L., de Lima Marin, G., Coudel, E., Morsello, C., et al. (2019). Intention of preserving forest remnants among landowners in the Atlantic Forest: The role of the ecological context via ecosystem services. People Nat. 1:10051. doi: 10.1002/pan3.10051
Trewick, S. A., Pilkington, S., Shepherd, L. D., Gibb, G. C., and Morgan-Richards, M. (2017). Closing the gap: avian lineage splits at a young, narrow seaway imply a protracted history of mixed population response. Mol. Ecol. 26, 5752–5772. doi: 10.1111/mec.14323
Keywords: tropical forest, landscape connectivity, private land conservation, biodiversity conservation, remote sensing-GIS, fragmentation, habitat loss
Citation: Siqueira FF, de Carvalho D, Rhodes J, Archibald CL, Rezende VL and van den Berg E (2021) Small Landscape Elements Double Connectivity in Highly Fragmented Areas of the Brazilian Atlantic Forest. Front. Ecol. Evol. 9:614362. doi: 10.3389/fevo.2021.614362
Received: 05 October 2020; Accepted: 26 April 2021;
Published: 24 May 2021.
Edited by:
Manuel B. Morales, Autonomous University of Madrid, SpainReviewed by:
Javier Rodriguez-Perez, UMR 5142 Laboratoire de Mathématiques et de leurs Applications Pau (LMAP), FranceAsier Rodríguez Larrinaga, Misión Biológica de Galicia, Consejo Superior de Investigaciones Científicas (CSIC), Spain
Copyright © 2021 Siqueira, de Carvalho, Rhodes, Archibald, Rezende and van den Berg. This is an open-access article distributed under the terms of the Creative Commons Attribution License (CC BY). The use, distribution or reproduction in other forums is permitted, provided the original author(s) and the copyright owner(s) are credited and that the original publication in this journal is cited, in accordance with accepted academic practice. No use, distribution or reproduction is permitted which does not comply with these terms.
*Correspondence: Flávia Freire Siqueira, ZmxhdmlhZnJlaXJlbHZAZ21haWwuY29t