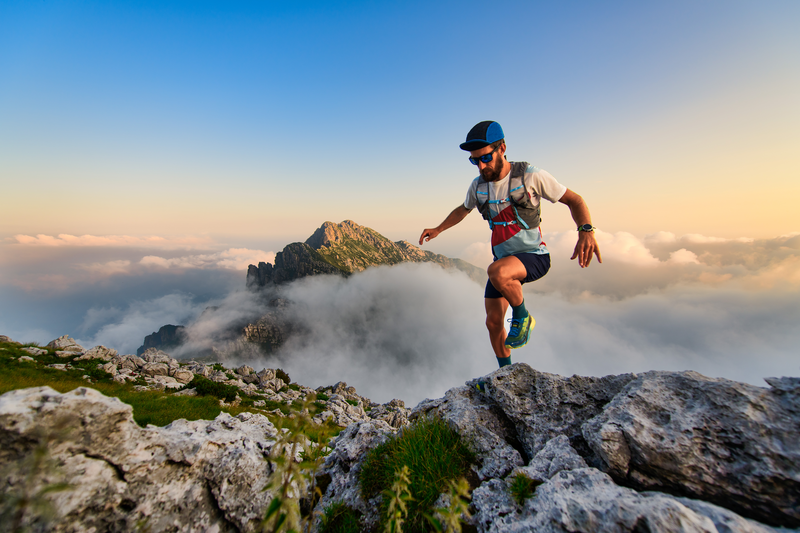
94% of researchers rate our articles as excellent or good
Learn more about the work of our research integrity team to safeguard the quality of each article we publish.
Find out more
ORIGINAL RESEARCH article
Front. Ecol. Evol. , 28 June 2021
Sec. Biogeography and Macroecology
Volume 9 - 2021 | https://doi.org/10.3389/fevo.2021.609627
This article is part of the Research Topic Habitat Modification and Landscape Fragmentation in Agricultural Ecosystems: Implications for Biodiversity and Landscape Multi-Functionality View all 14 articles
The growing replacement of native vegetation by forest plantations is considered a global threat to biodiversity. Significant variation in biotic communities among stands with similar management suggests that previous land use might have an effect on the capacity of forest plantations to harbor native species. The goal of our study was to determine the effect of land-use history on the biodiversity currently present in pine plantations in the coastal range of Central Chile. In particular, we hypothesized that plantations that directly replaced native forests should have higher diversity of plants and birds than plantations that were established in agricultural areas. We also expected that plantations of higher number of rotations should have fewer habitat-specialists and more generalists/exotics, reflecting a process of biotic homogenization. Using aerial photographs and satellite images encompassing a period of six decades, we classified 108 4-ha sampling units into native forests, and mature (17–20 year) pine plantations of first, second, and third rotation, of either forest or agricultural origin. At each site, we collected data on the abundance and richness of diurnal birds and understory plants, and analyzed their behavior in relation to the land-use history using Generalized Linear Models (GLMs). Also, we evaluated dissimilarity of communities of each pine plantation “treatment” to assess the occurrence of biotic homogenization. As predicted, pine plantations that directly replaced native forests had a higher abundance of forest specialists and less abundance of exotics and generalists than plantations of agricultural origin. In contrast, the number of rotations of pine plantations not only did not affect negatively the diversity and abundance of forest specialist species, but the models showed some signs of naturalization in the studied systems over time, such as the increase in the abundance of native herbs and a reduction in the abundance of their exotic counterparts. These results agree with the lack of evidence for a decrease in the dissimilarity of biotic communities in plantations with time, suggesting that the management of pine plantations in Central Chile is not promoting biotic homogenization, beyond the impact of the initial stages of land use change.
The transformation of natural ecosystems into artificial environments is one of the most ubiquitous expressions of the Anthropocene (Harden et al., 2014). An unfortunate consequence of the latter is that the changes produced by human land use since the 18th century, mostly for agricultural production, are considered one of the most important drivers of global biodiversity loss (Gibbs et al., 2010; Lambin and Meyfroidt, 2011; Ellis et al., 2013).
Among the different mechanisms of land use change, the replacement of natural forests by other land covers is likely one of the most significant in terms of its impact on biotic communities (Brook et al., 2003; Barlow et al., 2016; Sharp et al., 2019). In most cases, this replacement implies the direct removal of most plants from a site, and many other organisms are displaced or killed during cutting or burning operations (Shahabuddin and Kumar, 2007; Escobar et al., 2015; Owen et al., 2019). Moreover, the changes in environmental variables (i.e., luminosity, temperature, water and food availability; Li et al., 2009; Brauman et al., 2015; Andrews et al., 2017; Zhu et al., 2018), also force the evacuation of many animals, and reduce the fitness of the least mobile organisms (Brook et al., 2003; Ranius and Roberge, 2011; Stefani et al., 2017). On the other hand, the new environmental conditions offer suitable habitat for many disturbance-tolerant species that may colonize the area (Cavallero and Raffaele, 2010; Boscutti et al., 2018).
The extinction of habitat-specialist species and the colonization of disturbance tolerant and generalist species are the main mechanisms of biotic homogenization (Devictor et al., 2008; Harrison et al., 2014; Auffret et al., 2018), a process that occurs when different ecosystems subject to similar disturbances converge in terms of species composition and/or function (McKinney and Lockwood, 1999; Robertson et al., 2013; Kusuma et al., 2018). Usually, biotic homogenization takes place gradually, as neither mechanism is instantaneous. The colonization of disturbance-tolerant and generalist species increases over time, modulated by the intensity and frequency of the disturbances associated to the new land use (Acevedo and Restrepo, 2008; Belote et al., 2012; Smith et al., 2019). And even though most habitat-specialists may disappear from the site at the early stages of the land-use change, the populations of some species, especially long-lived ones, may take years or decades to become extinct (i.e., the “extinction debt,” sensu Tilman et al., 1994; Kuussaari et al., 2009).
Biotic communities are not only dependent on the physical and biological conditions of the site in which they inhabit, but also on its land-use history (Bremer and Farley, 2010; Cuddington, 2011; Meli et al., 2017). Usually the legacies of past land use can be observed in current communities through lasting alterations in the physical and biotic components that define them (Foster et al., 1998; Hermy and Veyheren, 2007; Fraterrigo, 2013), and the expression of such past effects will depend on the intensity, duration and nature of the disturbance (Foster et al., 1998; Renne and Tracy, 2013).
Forest plantations, comprised of exotic tree species, represent one of the most important land uses that have replaced native vegetation throughout the world, particularly in the Southern hemisphere (Overbeek et al., 2012). Plantations based on fast-growing species of the genera Pinus and Eucalyptus are usually managed as even-aged stands and harvested through clearcutting (INDUFOR, 2012). These plantations have been shown to generate significant changes in the physical environment in which they are established, including degradation and erosion of soils (Oyarzún and Peña, 1995; Turner and Lambert, 2000; Imaizumi et al., 2008; Soto et al., 2019) and an increase in water consumption (Licata et al., 2008; Scott and Prinsloo, 2008; Little et al., 2009), depending on the local environmental characteristics. In addition, management activities such as logging, weed control, fire or road construction, can have strong effects on biodiversity, for instance, facilitating the arrival of exotics or displacing organisms that are disturbances sensitive (Pauchard and Alaback, 2006; Tardif-Paradis et al., 2015; Cole et al., 2018; Speziale et al., 2018).
Although it is a long-known fact that the biota present in forest plantations may differ significantly from that of the original native forests (Estades and Temple, 1999; Proença et al., 2010; Braun and Koch, 2016; Castaño-Villa et al., 2019), the effect of these artificial forests as promoters of biotic homogenization has only recently received some attention (e.g., see Sweaney et al., 2015; Šibíková et al., 2019; Cifuentes-Croquevielle et al., 2020). One aspect that has been mostly absent from these analyses is the effect of time. Not only biotic homogenization is a process that might require time to develop, but the high variability observed in the biodiversity present in, otherwise similar (e.g., soil type, management) plantations (Simonetti et al., 2013; Trentini et al., 2017; Wang et al., 2019), suggests that the land-use history of the site (e.g., origin, time since the conversion, and number of rotations) may have a significant effect on current community composition.
The goal of this study is to evaluate the effect of land-use history on the biodiversity living in pine plantations, as a way to improve the scientific basis for the design of sustainable forestry management. We tested the hypothesis that land-use history significantly explains part of the variation in the composition of current plant and bird communities present in exotic pine plantations in Central Chile, modulating the expression of biotic homogenization. This history has two components: the time (number of rotations) during which the plantation has been in place, and the land use that preceded the plantation. In particular we tested three main predictions: (1) First, we predicted that the abundance and richness of forest specialists should be higher in plantations that directly replaced native forests, compared to those plantations established in sites that underwent a period of agricultural cultivation after deforestation, and that the richness and abundance of forest specialists should decline in plantations with a higher number of rotations. (2) We also expected that the abundance and richness of generalists and exotics should be higher in plantations that replaced agricultural lands and in plantations with a higher number of rotations. (3) Finally, as a consequence of the latter two, we also predicted that the dissimilarity in the community composition should decline among plantations with higher number of rotations, reflecting the process of biotic homogenization.
The study was carried out in the Coastal Range of the Maule, Ñuble, and Biobío regions (from Putú, 35.2°S, 72.2°W, to Trongol 37.6°S, 73.2°W, Figure 1), encompassing approximately 950,000 ha. These regions are considered part of a global biodiversity hotspot (Myers et al., 2000), but hold some of the oldest and most extensive commercial Pinus radiata plantations in Chile (Rogers et al., 1955). The climate is Mediterranean with oceanic influence, with warm and dry summers, and cold and rainy winters. Precipitation ranges 650–1000 mm/year (Pizarro et al., 2012; Sarricolea et al., 2016).
Figure 1. Study region and location of sample units classified as: native forest (N), first rotation pine plantation that replaced native forest (NP1), second rotation pine plantation that replaced native forest (NP2), first rotation pine plantation that replaced agricultural lands (AP1), second rotation pine plantation that replaced agricultural lands and third rotation pine plantation of unknown previous land use (UP3).
This part of the country has a long history of human use that predates the arrival of European settlers (Bengoa, 2000), but the strongest transformations occurred during the 19th and 20th centuries, first as the result of economic incentives for clearing land for crop production and, later, for the establishment of forest plantations (Bauer, 1970; Camus, 2006; Chateauneuf et al., 2011).
The conversion of the landscape into forest plantations began in the mid 20th century, as part of a government program to control soil erosion caused by unsustainable wheat production (Rogers et al., 1955; IREN-CORFO, 1965; Bauer, 1970). Subsidies and environmentally weak regulations further promoted the proliferation of P. radiata plantations that, not only were established in eroded lands, but also replaced an important amount of native forests (Echeverría et al., 2006; Nahuelhual et al., 2012; Miranda et al., 2017). Currently, a 37% of the study region is covered by pine plantations while native forests represent only a 13% (Uribe et al., 2020). Most of these remnant native forests correspond to secondary forests, mainly dominated by deciduous species, such as Nothofagus glauca and Nothofagus obliqua, and also by evergreen species such as Cryptocarya alba and Aextoxicon punctatum, belonging to the Coastal Mediterranean Sclerophyllous and coastal Mediterranean Deciduous Forests (Luebert and Pliscoff, 2017).
We determined the land-use history of pine plantations using aerial photographs (Hycon flight, 1955, OAS project, 1960), Landsat images (1975, 1985, and 1998) and Google Earth Pro images for more recent land covers. For a detailed account of the methods used in this analysis, refer to Uribe et al. (2020).
We classified current pine plantations in relation to their origin (agricultural land or native forest) and the number of rotations that had taken place since their establishment. On average, in Central Chile, pine plantations are harvested at 20 years of age (Lima, 2016), so we used that figure as a reference during the historical reconstruction. Plantations that directly replaced native forests, and were in their first and second rotation, were named NP1 and NP2, respectively. For plantations that were established in agricultural areas we used the codes AP1 for the first, and AP2, for the second rotation. We also found a proportion of plantations in their third rotation, but because the aerial photographs did not allow us to determine the land cover that preceded the plantations, we coded them UP3 (unknown origin and third rotation). However, for interpretation and analysis purposes we consider these sites most likely as pine plantations that replaced agricultural lands since during the early stages of the afforestation program all incentives were for establishing plantations in degraded lands due to intensive and unsuitable agricultural production (Camus, 2006). Finally, in this work we use N as the code to represent native forests. Native forests that were replaced by pine plantations included secondary and degraded forests (Elizalde, 1970; Luebert and Pliscoff, 2017). Agricultural lands replaced by pine plantations were mostly used for wheat production from the middle of the 19th to the middle of 20th century, but also include some vineyards, legumes and small-scale cattle production (Bauer, 1970; Saldivia and De la Jara, 2001). A summary of the evaluated conditions (treatments) stands is presented in Figure 2.
Figure 2. Representation of the conditions (treatments) evaluated. Native forest (N) and pine plantations following the sequence of changes experienced by stands. First rotation pine plantation that replaced native forest (NP1), second rotation pine plantation that replaced native forest (NP2), first rotation pine plantation that replaced agricultural lands (AP1), second rotation pine plantation that replaced agricultural lands and third rotation pine plantation of unknown previous land use (UP3). Agricultural lands (A), unknown previous cover (U). Pine plantation rotations of approximately 20 years. Red rectangle represents the time when samples were collected in each stand class.
We established a total of 108 sample units throughout the study region, trying to cover the entire range of conditions detected in the previous classification. The sample size per class was the following: NP1 (12), NP2 (21), AP1 (17), AP2 (13) and UP3 (30). In addition, we included 15 units in native forests (N) to serve as references of community composition. Forty nine units were sampled during 2008–2013, whereas the remaining 59 were assessed in 2016–2018. In all units we sampled woody plants and diurnal birds. Additionally, during the Austral spring and summer of 2017–2018 we collected data on herbaceous plants in 53 of the sample plots (8 NP1, 5 NP2, 12 AP1, 6 AP2, 12 UP3, and 10 N).
Sample units corresponded to 4-ha plots of adult (17–20 year) pine plantations or native forest (Figure 3). We selected plantations that had been subjected to a similar management scheme (i.e., pruning and thinning for knot-free wood). This allowed us to control for the effect of canopy closure on understory development (Harrington and Edwards, 1999; Trentini et al., 2017). Also, by working only with mature stands, we reduced the impact of successional changes within each plantation on community composition (Berndt et al., 2008; Uribe and Estades, 2014; Heinrichs et al., 2018). Each sample unit included four 25-m radius circular plots for the evaluation of woody plants, and four 50-m radius point count plots for birds (Figure 3). For the assessment of herbs, we used four 10 × 10-m square sub-plots (Figure 3).
To estimate the abundance of woody plants in a site, we conducted a visual assessment of the foliage volume in each sub-plot, following the general method used by Estades and Temple (1999) originally designed for the description bird habitat. For this purpose, each sub-plot was divided into four quarters (each evaluated by at least two people from different positions) and vertically sub-divided in three “layers” (0–0.3 m, >0.3–2 m, and >2–6 m), within which we estimated the foliage volume (m3) of each species. With the data for each layer and quarter for all subplots, we averaged and projected the numbers to estimate the total foliage volume (m3/ha) for each species. For herbaceous plants we conducted a simple visual estimation of the bi-dimensional cover in the 10 × 10 m plots (Gómez-García, 2008). Any unknown species was collected and/or photographed for later identification. We averaged the percentage cover for all species in the four subplots to produce a single estimate for each sample unit. Although this approach can have some limitations due to subjectivity of the observer, there is evidence that, compared to other more objective techniques, visual evaluation can detect more plant species, including rare ones (Dethier et al., 1993; Chmura and Salachna, 2016). Besides, in this work, potential individual observer effects were likely minimal, as the field team was roughly the same during the entire evaluation period, thus making data comparable among plots.
We estimated the absolute abundance (ind/ha) of diurnal birds using variable-distance point counts, to allow correction for detectability (Buckland et al., 2001). At each of the four subplots we conducted two 5-min counts (separated by a 5 min waiting period) of all birds seen or heard within the maximum observation radius (50 m). All counts were conducted during the breeding season (October–January), between sunrise and noon, during non-rainy and non-windy days (Bibby et al., 2000). We averaged the results of the eight point counts to produce an estimate of the abundance of each bird species at each sample unit.
In order to produce the variables required to test the proposed predictions, we conducted the following data classifications and calculations:
To represent forest specialists, we selected all woody plants classified as hygrophiles (following Donoso, 2006), because these species are particularly sensitive to environmental changes such as those imposed by fast growing plantations with high water consumption (e.g., Little et al., 2009; Huber et al., 2010). Due to limited ecological information to assess forest specialization or habitat requirement for many herbaceous plants, we decided to include all native species in this category (in contrast to exotic species), following Rodríguez et al. (2018). Finally, for birds we selected all species classified as forest specialists in the literature (Díaz et al., 2005; Cofré et al., 2007; Ibarra and Martin, 2015).
Generalist and disturbance-tolerant plants were represented by exotic species (both for woody and herbaceous plants) following Rodríguez et al. (2018). Because there is only one exotic bird in the area (Callipepla californica), in order to represent disturbance-tolerant birds, we used all species classified as habitat-generalists (Díaz et al., 2005; Cofré et al., 2007; Ibarra and Martin, 2015).
The abundance estimates for woody plant species were produced by adding the foliage volume of all corresponding species, expressed as percentage of total foliage volume (%). In the case of herbaceous plants, their abundance was estimated by adding the percent cover (%) of all corresponding species. Finally, the abundance of birds of each category was generated by adding the density of all corresponding bird species (ind/ha). The richness estimates were produced for each group by counting the number of corresponding species recorded within each sample unit (n). In the case of herbaceous plants, because some species could only be classified to the genus level, we did not use species richness as a response variable.
Finally, we calculated the dissimilarity in the composition of the communities within each forest class (N, NP1, NP2, AP1, AP2, and UP3) by averaging the Euclidean distance (Johnston, 1976) between the abundance of all species (all woody plants, all herbs and all bird species), for all pairs of sample units within the class. For this purpose, we worked with the relative abundance of each species of plants (woody and herbs) and the density of each bird species.
We used two approaches to study the response of species abundance and richness and community dissimilarity to plantation origin and rotation number. First, we conducted multiple comparisons using the method of Benjamini and Hochberg (1995) to test for differences between the studied forest classes (Native forests and plantations of different origin and rotation number). Second, in order to directly account for the effect of time (rotation number: 1, 2, or 3) on the relative abundance and richness of the studied species categories, we used generalized linear models with Poisson or Negative Binomial distribution (Navarro et al., 2001; Zipkin et al., 2014). In these models we excluded the data from native forest sites, in order to focus on the changes occurred during the existence of the pine plantations. For origin we assigned a value of 0 for native forest and 1 for agricultural lands.
We included the following physical covariates in the models: altitude, latitude (UTM) and terrain ruggedness. Because slope was highly correlated (r > 0.9) with terrain ruggedness, we only used this latter variable because it is usually considered more informative than slope (Riley et al., 1999). For this purpose we used an Aster Digital Elevation Model (METI and NASA products) available from the Earth Explorer server1. For birds, we also included information of the understory, using the foliage volume of woody hygrophiles, exotics, and an additional category: sclerophyllous species, a group of native plants more associated to scrub-type mediterranean ecosystems (Axelrod, 1975; Prentice et al., 1992; Luebert and Pliscoff, 2017). To account for a potential effect of the landscape attributes (Deconchat et al., 2009; Muñoz-Sáez et al., 2020), we also included the percentage of native vegetation and open areas (including forest clearcuts and agriculture-livestock land) in a 500-m radius around each sample unit. This information was obtained using Google Earth Pro 7.3.2., and selecting the images that better represented the condition of the landscape at the time of the field assessment of each unit. All geospatial processes were carried out using the free software QGIS 2.14 Essen.
All explanatory variables were standardized before the analyses. For each case we selected the best model using backward-forward stepwise, based on the Akaike Information Criterion (AIC) (Cavanaugh, 1997) and Model Selection and Multimodel Inference (Calcagno and De Mazancourt, 2010). All statistical procedures were carried out in RStudio 1.2.5033 (RStudio Team, 2019).
We recorded a total of 96 woody plant species in the sample plots (see Supplementary Table 1). Fifteen (16%) were exotic species and 81 were native from Chile, 36 (38%) of which were endemic to the Country. Out of 73 identified herbaceous species (see Supplementary Table 2), 30 (41%) were exotic, 43 were native species and of these, 19 were endemic to Chile (Rodríguez et al., 2018).
The abundance and richness of hygrophilous woody plants were significantly lower in all pine plantations compared with native forests, although we found no significant differences among plantations of different origin and number of rotations (Figures 4A,B). The best models for the abundance and richness of hygrophilous woody plants showed a negative effect of the agricultural origin (Table 1). The model for hygrophile richness also included a positive effect of rotation number (Table 1).
Figure 4. Relative abundance and richness of plants in the understory of native forests and pine plantations with different origin and number of rotations. (A) Abundance of hygrophilous woody plants. (B) Richness of hygrophilous woody plants. (C) Abundance of exotic woody plants. (D) Richness of exotic woody plants. (E) Abundance of native herbs. (F) Abundance of exotic herbs. N in x-axis is Native forest. NP, native forest transformed into pine plantation, AP, agricultural land transformed into pine plantation, UP, unknown previous land use (assumed as agricultural land) transformed into pine plantation. Lower case letters indicate the significance of differences among categories obtained by BH multiple comparisons.
Table 1. Coefficients of the best generalized linear models for the abundance and richness of plants in pine plantations with different origin and number of rotations.
The abundance of woody exotics increased significantly only in AP1 plantations (Figure 4C). The richness of exotics was significantly higher in plantations of agricultural origin, but did not differ among rotations; while in plantations that replaced native forest, the richness of exotics was only higher in NP1 sites (Figure 4D). In the case of herbs, only AP1 plantations had a significantly lower cover of native species and a higher cover of exotics (Figures 4E,F). Both the abundance and richness of exotic woody plants were positively associated to an agricultural origin (Table 1). The abundance of native herbs increased with the number of rotations, while the opposite was true for exotic herbs, which were also positively affected by the agricultural origin. Among the most consistent effects of the covariates were an increased abundance and richness of hygrophilous and exotic woody plants in more southern locations, and a positive effect of open areas in the surrounding landscape on the abundance and richness of exotic woody plants (Table 1).
We recorded a total of 32 bird species during our field sampling. Thirty one species were native, including two Chilean endemics. Eight species could be classified as forest-specialists, whereas 10 were considered as habitat-generalists (Supplementary Table 3).
The abundance and richness of forest-specialist birds declined significantly in pine plantations in relation to the reference native forests (Figures 5A,B). However, no significant differences were observed between plantations with different origin and rotation number (Figures 5A,B). Some of the significant effects of the studied covariates included a positive impact of the native forest in the surrounding landscape on the abundance and richness of forests specialists in pine plantations (Table 2).
Figure 5. Abundance and species richness of diurnal birds in native forests and pine plantations with different origin and number of rotations. (A) Abundance of forest-specialist birds. (B) Richness of Richness of forest-specialist birds. (C) Abundance of habitat-generalist birds. (D) Richness of habitat-generalist birds. N in x-axis is Native forest. NP, native forest transformed into pine plantation, AP, agricultural land transformed into pine plantation, UP, unknown previous land use (assumed as agricultural land) transformed into pine plantation. Lower case letters indicate the significance of differences among categories obtained by BH multiple comparisons.
Table 2. Coefficients of the best generalized linear models for the relative abundance and richness of birds in pine plantations with different origin and number of rotations.
In the case of generalist species, no statistical differences were found for any of the studied cases (Figures 5C,D). When including the effect of various covariates, the best models for all dependent variables showed only a positive effect of the agricultural origin on the richness of habitat-generalists, and no effect of the rotation number (Table 2). Habitat generalists showed a positive association with understory vegetation containing more xerophytic (i.e., +sclerophyllous, −hygrophilous) species (Table 2).
The dissimilarity index of woody plants showed a significant increase in AP1 plantations in relation to that of native forests (Figure 6A). Although the index declined in the subsequent rotations, UP3 plantations still had a higher dissimilarity index than N (Figure 6A). The woody plant communities in NP2 plantations were significantly more dissimilar than that of native forest sites (Figure 6A).
Figure 6. Dissimilarity index for the community of woody plants (A), herbs (B), and birds (C) between sites of the same forest class. N in x-axis is Native forest. NP, native forest transformed into pine plantation, AP, agricultural land transformed into pine plantation, UP, unknown previous land use (assumed as agricultural land) transformed into pine plantation. Lower case letters indicate the significance of differences among categories obtained by BH multiple comparisons.
In the case of herbaceous plants there was a clear increase in the dissimilarity index in first-rotation plantations, with both AP1 and NP1 being significantly more heterogeneous than any of the other analyzed forest classes (Figure 6B). The dissimilarity of bird communities declined from native forests to AP1 and NP1, and to AP2 and NP2 (Figure 6C). However, UP3 sites had bird communities as heterogeneous as native forests (Figure 6C).
This study is one of the first to directly assess the effect of previous land-use history on current biodiversity in an intensive forestry system. Our data showed that the composition of the understory of pine plantations in Central Chile was strongly influenced by the type of land use that preceded the plantation, and that these legacies may last for several decades. Similarly persistent effects of agriculture have been observed in many other systems (Fraterrigo, 2013; Munteanu et al., 2015; Neumann et al., 2017).
Our work is also among the few that have analyzed the occurrence of biotic homogenization in intensively managed forest systems (Sweaney et al., 2015; Šibíková et al., 2019; Cifuentes-Croquevielle et al., 2020). Studying a six-decade period of pine plantation forestry in Central Chile we assessed the effect of time (i.e., rotations) on the loss of heterogeneity (i.e., community dissimilarity) and the main mechanisms behind biotic homogenization: loss of habitat specialists and colonization by habitat generalists. To the best of our knowledge, this is the first study to take such a comprehensive approach.
Although data on forest-specialist woody plants and birds showed the expected decline in richness and abundance from the reference native forests to plantations (Estades and Temple, 1999; Vergara and Simonetti, 2006; Gómez et al., 2009; Braun and Koch, 2016), the trend was not observed among plantations of different rotation number (Figures 4A,B, 5A,B).
The relative abundance and species richness of hygrophiles were higher in pine plantations that directly replaced native forests than in those that were established in agricultural areas (Table 1). There are management-related explanations for the latter result. For example, the persistence of many shrub species after the conversion into pine plantations may be possible due to the resprouting abilities of many species (Ross et al., 1986; O’Hara et al., 2007). However, preparation of the land for crop cultivation usually requires the removal of stumps and main roots of woody species, which impedes vegetative reproduction of many native plants (FAO, 1977; Jorquera, 2001).
Although we predicted that the abundance and richness of habitat specialists should decline over time, due to the gradual extinction of species not adapted to the environmental conditions imposed by the new land use (Ranius and Roberge, 2011; Auffret et al., 2018), the trend was not observed among plantations of different rotation number (Figures 4A,B, 5A,B). And not only forest specialists did not decline over time, but the richness of hygrophilous woody plants and native herbs increased in plantations with more rotations (Table 1). The effect on hygrophiles is particularly interesting since the reduction of water availability is considered one of the main environmental changes produced by pine plantations (Huber et al., 2008; Álvarez-Garretón et al., 2019). Nevertheless, our results suggest that plantations may provide a relatively suitable habitat for some sensitive species, which might be able to recolonize the area after being extirpated during the initial stages of deforestation (Onaindia and Mitxelena, 2009; Jeffries et al., 2010; Becerra and Montenegro, 2013).
As expected, exotic herbaceous and woody plants were more diverse and abundant in plantations that replaced agricultural areas (Table 1). The ubiquitous presence of humans in agricultural areas and the frequent and intense disturbances to which crop fields are subjected, promote, respectively, the arrival and establishment of exotic plants, including undesirable weeds (e.g., Genista monspessulana), and naturalized useful plants (e.g., Rubus ulmifolius, Rosa moschata). The positive effect of the amount of open areas in the surrounding landscape on woody exotics (Table 1), indirectly highlights the importance of the distance to propagule source as a factor of invasibility (González-Moreno et al., 2013; Altamirano et al., 2016). The abundance of habitat generalist birds was also higher in plantations established in agricultural areas (Table 2), which agrees with our prediction.
Our second prediction stated that exotics and habitat generalists should increase their abundance and richness in plantations with more rotations, reflecting the gradual invasion of species more adapted to the disturbances associated to the management of plantations (Pawson et al., 2009; Moreira et al., 2013; Stokely et al., 2020). As occurred with specialists, the data did not support our expectations. While the richness and abundance of generalist birds did not differ between native forest and any of the studied plantation classes (Figures 5C,D), both woody and herbaceous exotics increased their richness and abundance in relation to native forests. However, the latter did not continue increasing in plantations with more rotations (Figures 4C,D,F). Moreover, the best model for the abundance of exotic herbs showed a significant decrease with rotation number (Table 1).
These results show that pine plantations in Central Chile do not seem to be promoting the invasion of exotic species beyond the effects of the initial stages of land use change. Moreover, the studied plantations might be excluding some exotic plants species over time. We can think of, at least, two potential mechanisms for the latter. First, because the transformation of agricultural land into pine plantations involves a significant reduction in the human population (Tropp, 2003; Rudel, 2009), the persistence of weeds with human-mediated dispersal (Hansen et al., 2005; Pickering and Mount, 2010; Ansong and Pickering, 2014) could have been compromised. Second, the change from a horse and oxen-based logging in the early plantations, to mostly mechanized harvesting operations (Carey and Soto, 2005), has likely reduced the abundance of many alien plants whose seeds are dispersed in ungulate dung (Chuong et al., 2016).
One of the expected outcomes of the widespread use of production systems such as forest plantations is the process of biotic homogenization (Manor et al., 2008; Sweaney et al., 2015; Kirkpatrick et al., 2018). We predicted that the community dissimilarity between sites in our study region should decline in plantations with higher number of rotations, reflecting the occurrence of biotic homogenization (Kirkpatrick et al., 2018). Having failed to obtain support for the occurrence of any of its mechanisms (i.e., a reduction of habitat specialists and an increase in habitat generalists), it is not surprising that this prediction also proved incorrect.
Interestingly, the plant communities in first-rotation plantations had a higher dissimilarity among themselves than in the reference native forests (Figures 6A,B). There are two potential explanations for this pattern. First, the high intersite heterogeneity may arise from the legacies of the different agricultural uses in different areas. Although wheat was the dominant crop before the advent of pine plantations, other uses included legumes, vineyards, and small-scale cattle ranching (Bauer, 1970; Saldivia and De la Jara, 2001), all of which may promote different sets of accompanying plant communities. Second, although we made an effort to locate native forests in good conditions to act as appropriate references, the studied stands might have already been affected by disturbances, fragmentation and decades of being surrounded by pine plantations (Estades and Temple, 1999; Sweaney et al., 2015; Miranda et al., 2017), likely reducing intersite heterogeneity. A potential sign of the latter is the high proportion of generalist vs. specialist birds recorded in our samples in native forests (Figures 5A,C). However, we are confident that the lack of pristine forest sites in the study region (Donoso and Lara, 1995; Bustamante and Simonetti, 2005; Gómez et al., 2011), and in our sample, in no way affects the main results of this study.
Although in most situations the dissimilarity of plant communities declined in the second and third rotation, in no case it reached values lower than in the native forests (Figures 6A,B). Moreover, the dissimilarity of woody plants in plantations that replaced native forests actually increased in the second rotation (Figure 6A). Although these results do not support the hypothesis of biotic homogenization among plants in the studied pine plantations, it is important to keep in mind that there might still exist an extinction debt that has not yet been paid. The ability of many woody plant species to resprout from stumps may extend their presence even in the absence of sexual reproduction (Ross et al., 1986; O’Hara et al., 2007). Interestingly, there were different trends in herbs and woody plants that might relate to the latter phenomenon (Figures 6A,B). Studies on the seed bank and vegetative reproduction abilities in the understory of plantations with different rotations would shed some light on this topic.
Up to the second rotation, the dissimilarity indexes of birds showed the predicted decline in the case of biotic homogenization (Figure 6C). However, the trend reversed in the third rotation with an increase in intersite heterogeneity. This result cannot be explained by changes in bird abundance or richness, because none of these variables changed with rotation number (Table 2). Instead, this increase in heterogeneity might be due to different relative changes in the abundance of individual bird species in different sites, likely associated to a higher diversity of forest-specialist plants in plantations with more rotations (Table 1).
Exploratory Non-metric Multidimensional Scaling (NMDS) analyses for woody plants, herbs and birds (based on Bray-Curtis dissimilarity), support the general patterns described in the previous paragraphs (Supplementary Figure 1).
We can summarize our findings with a conceptual model that may serve as a hypothesis for future studies on this topic (Figure 7). If we consider that the native forest (N) has the highest level of naturalness (e.g., the difference or ratio between specialists vs. generalist-exotic species), any land-use change (e.g., conversion into forest plantation) will reduce this level to a point that reflects the long-term level of naturalness allowed by the new land use (in this case, the plantation naturalness level, PNL, Figure 7). Depending on the severity of the disturbance involved in the transformation, the community may follow different trajectories toward the new equilibrium. For example, the direct replacement of a native forest by a plantation may be considered a “soft” conversion, in which most sensitive species will disappear gradually from the system (black line, Figure 7). Alternatively, the native forest may undergo a “hard” conversion by, as in the present study, being first transformed into agricultural use, before the establishment of the plantation (gray line, Figure 7). In the latter case, the level of naturalness will drop abruptly, driven by the rapid extinction of many habitat specialists and the invasion of many exotic and disturbance-adapted species. This decline may cause the system to reach naturalness levels lower than PNL, which will later increase as some native species recolonize the area and some of the alien species disappear, converging to the equilibrium point.
Figure 7. Model for the effect of the conversion of native forests (N) into pine plantations in Central Chile on the level of naturalness of the systems. The black and gray lines represent “soft” and “hard” conversions, respectively. See text for further explanation. PNL and the dashed line represent the plantation naturalness level.
Over the past two decades an important number of studies have explored ways of improving the compatibility of pine plantation forestry with biodiversity conservation in Central Chile. Authors have highlighted the key importance of promoting a well-developed understory (Tomasevic and Estades, 2008; Taki et al., 2010; Simonetti et al., 2013), the maintenance of woody debris (Rudolphi and Gustafsson, 2011; Uribe and Estades, 2014; Fierro and Vergara, 2019), or the design of landscapes that promote biological connectivity (Acuña and Estades, 2011; Mortelliti et al., 2015; Castillo et al., 2018). The current study provides an additional perspective over which to design the management of these industrial forests, in order to reduce their long-term negative impacts, and to enhance their potential benefits on biodiversity. From the perspective of reducing biotic homogenization, the latter implies reducing the loss of habitat specialists and preventing the invasion of exotic species.
There is strong evidence that the presence of nearby native forest patches increases the abundance of forest-specialist birds in pine plantations in Chile (Estades and Temple, 1999; this study). Although we did not find such an effect for habitat-specialist plants, we can speculate that the movement of birds between native forest fragments and the pine plantations may play a role in the recolonization of plantations by ornithocorous plants (Whittaker and Jones, 1994; Ponce et al., 2012; Spennemann, 2020). The ruggedness of the terrain in most of the study region implies that most plantations are located close to a creek with some native vegetation. Protecting creeks and native forest fragments may play a significant role in the conservation of biodiversity in pine plantation landscape (Estades and Temple, 1999; Lindenmayer et al., 2003).
One factor that might have contributed to the persistence of some forest specialist species in pine plantations in Central Chile, is the relatively low frequency of disturbances involved in their management. Any management decision leading to changes in rotation length could certainly affect the long term composition of the community, by influencing the likelihood of forest specialists to find suitable habitat (Sullivan et al., 2009; Gallé et al., 2016; Castaño-Villa et al., 2019) and by affecting the invasion rate of disturbance-adapted exotics (Zhou et al., 2019).
The main assumptions of the model that we proposed (Figure 7) are that there exists an equilibrium point in the composition of the biota that can be found in pine plantations in Central Chile, and that the trajectories of plantations with different histories will tend to converge to this equilibrium over time. It is important to consider that the level of naturalness that can be achieved in pine plantations may depend strongly on the management of these industrial forests, many aspects of which are still not clear, particularly in relation to their long-term effects (Castaño-Villa et al., 2019). One example of the latter is the impact of biomass extraction and soil amendments on the long-term fertility of tree plantations (Woods, 1990; Smaill and Garrett, 2016; Addison et al., 2019). Thus, although our study considered a period of more than six decades, more time and research are certainly needed to confirm or reject our hypotheses.
The raw data supporting the conclusions of this article will be made available by the authors, without undue reservation.
SU and CE contributed with study design and the manuscript writing. SU carried out field work and data collection. All authors performed the laboratory work and data analyses.
Funding for this study was provided by FONDECYT grants 1080463 and 1120314 to CE. SU was supported by a CONICYT Doctoral Scholarship (grant 21170437), by funds from the Vicepresidency of Academic Affairs of the University of Chile, and a grant from the School of Forest Science and Nature Conservation.
The authors declare that the research was conducted in the absence of any commercial or financial relationships that could be construed as a potential conflict of interest.
This work benefited significantly from the suggestions of three referees, the two reviewers and the editor. We are grateful to all of them. We sincerely thank Arauco, Masisa, and Mininco for kindly granting us access to their properties, and providing us with cartographical information and some logistic support. S. Uribe’s Committee members (Rosa Scherson, Audrey Grez, Cristián Echeverría, Jaime Jiménez, and Nicolás Franck) made very useful comments on an early version of this work. Valentina Echeverría, Pilar Fernández, Romina Chiappe, current and former LEVS members, and several other students and assistants that helped with the collection of field data. Finally, we thank Sergio Alvarado and Nélida Villaseñor for their help with statistical analyses.
The Supplementary Material for this article can be found online at: https://www.frontiersin.org/articles/10.3389/fevo.2021.609627/full#supplementary-material
Acevedo, M. A., and Restrepo, C. (2008). Land-cover and land-use change and its contribution to the large-scale organization of Puerto Rico’s bird assemblages. Diversity Distrib. 14, 114–122. doi: 10.1111/j.1472-4642.2007.00435.x
Acuña, M. P., and Estades, C. F. (2011). Plantation clearcut size and the persistence of early-successional wildlife populations. Biol. Conserv. 144, 1577–1584. doi: 10.1016/j.biocon.2011.02.003
Addison, S. L., Smaill, S. J., Garrett, L. G., and Wakelin, S. A. (2019). Effects of forest harvest and fertiliser amendment on soil biodiversity and function can persist for decades. Soil Biol. Biochem. 135, 194–205. doi: 10.1016/j.soilbio.2019.05.006
Altamirano, A., Cely, J. P., Etter, A., Miranda, A., Fuentes-Ramírez, A., Acevedo, P., et al. (2016). The invasive species Ulex europaeus (Fabaceae) shows high dynamism in a fragmented landscape of south-central Chile. Environ. Monit. Assess. 188:495. doi: 10.1007/s10661-016-5498-6
Álvarez-Garretón, C., Lara, A., Boisier, J. P., and Galleguillos, M. (2019). The impacts of native forests and forest plantations on water supply in Chile. Forests 10:473. doi: 10.3390/f10060473
Andrews, T., Betts, R. A., Booth, B. B. B., Jones, C. J., and Jones, G. S. (2017). Effective radiative forcing from historical land use change. Clim. Dyn. 48, 3487–3505. doi: 10.1007/s00382-016-3280-7
Ansong, M., and Pickering, C. (2014). Weed seeds on clothing: a global review. J. Environ. Manage. 144, 203–211. doi: 10.1016/j.jenvman.2014.05.026
Auffret, A. G., Kimberley, A., Plue, J., and Walden, E. (2018). Super-regional land-use change and effects on the grassland specialist flora. Nat. Commun. 9:3464. doi: 10.1038/s41467-018-05991-y
Axelrod, D. I. (1975). Evolution and biogeography of Madrean-Tethyan sclerophill vegetation. Ann. Mo. Bot. Gard. 62, 280–334.
Barlow, J., Lenox, G. D., Ferreira, J., Berenguer, E., Lees, A. C., Mac Nally, R., et al. (2016). Anthropogenic disturbance in tropical forests can double biodiversity loss from deforestation. Nature 535, 144–147. doi: 10.1038/nature18326
Bauer, A. J. (1970). Expansión Económica en una sociedad tradicional: Chile central en el siglo XIX. Santiago: Ediciones Historia.
Becerra, P. I., and Montenegro, G. (2013). The widely invasive tree Pinus radiata facilitates regeneration of native woody species in a semi-arid ecosystem. Appl. Veg. Sci. 16, 173–183. doi: 10.1111/j.1654-109X.2012.01221.x
Belote, R. T., Jones, R. H., and Wieboldt, T. F. (2012). Compositional stability and diversity of vascular plant communities following logging disturbance in Appalachian forests. Ecol. Appl. 22, 502–516. doi: 10.1890/11-0925.1
Benjamini, Y., and Hochberg, Y. (1995). Controlling the false Discovery rate: a practical and powerful approach to multiple testing. J. R. Stat. Soc. B 57, 289–300.
Berndt, L. A., Brockerhoff, E. G., and Jactel, H. (2008). Relevance of exotic pine plantations as a surrogate habitat for ground beetles (Carabidae) where native forest is rare. Biodivers. Conserv. 17, 1171–1185. doi: 10.1007/s10531-008-9379-3
Bibby, C. J., Burgess, N. D., Hill, D. A., and Mustoe, S. H. (2000). Bird Census Techniques, 2nd Edn. London: Academic Press.
Boscutti, F., Sigura, M., De Simone, S., and Marini, L. (2018). Exotic plant invasion in agricultural landscapes: a matter of dispersal mode and disturbance intensity. Appl. Veg. Sci. 21, 250–257. doi: 10.1111/avsc.12351
Brauman, K. A., Freyberg, D. L., Asce, M., and Daily, G. C. (2015). Impact of land-use change on groundwater supply: ecosystem services assessment in Kona. Hawaii. J. Water Resour. Plann. Manage. 141:A4014001.
Braun, A. C., and Koch, B. (2016). Estimating impacts of plantation forestry on plant biodiversity in southern Chile - a spatially explicit modelling approach. Environ. Monit. Assess 188:564. doi: 10.1007/s10661-016-5547-1
Bremer, L. L., and Farley, K. A. (2010). Does plantation forestry restore biodiversity or create green deserts?. a synthesis of the effects of land-use transition on plant species richness. Biodivers. Conserv. 19, 3893–3915. doi: 10.1007/s10531-010-9936-4
Brook, B. W., Sodhi, N. S., and Ng, P. K. L. (2003). Catastrophic extinctions follow deforestation in Singapore. Nature 424, 420–423. doi: 10.1038/nature01795
Buckland, S. T., Anderson, D. R., Burnham, K. P., Laake, J. L., Borchers, D. L., and Thomas, L. (2001). Introduction to Distance Sampling. Oxford: Oxford University Press.
Bustamante, R. O., and Simonetti, J. A. (2005). Is Pinus radiata invading the native vegetation in Central Chile? demographic responses in a fragmented forest. Biol. Invasions 7, 243–249. doi: 10.1007/s10530-004-0740-5
Calcagno, V., and De Mazancourt, C. (2010). glmulti: an R package for Easy Automated Model Selection with (Generalized) linear models. J. Stat. Softw. 34, 1–29. doi: 10.18637/jss.v034.i12
Carey, P., and Soto, D. (2005). Análisis de productividad de dos carros portacarga en madereo con torre a tala rasa en plantaciones de Pinus radiata. Bosque 26, 95–106. doi: 10.4067/S0717-92002005000200012
Castaño-Villa, G. J., Estevez, J. V., Guevara, G., Bohada-Murillo, M., and Fontúrbel, F. E. (2019). Differential effects of forestry plantations on bird diversity: a global assessment. Forest Ecol. Manag. 440, 202–207. doi: 10.1016/j.foreco.2019.03.025
Castillo, M. G., Hernández, H. J., and Estades, C. F. (2018). Effect of connectivity and habitat availability on the occurrence of the Chestnut-throated Huet-Huet (Pteroptochos castaneus, Rhinocryptidae) in fragmented landscapes of central Chile. Landscape Ecol. 33, 1061–1068. doi: 10.1007/s10980-018-0649-5
Cavallero, L., and Raffaele, E. (2010). Fire enhances the “competition-free” space of an invader shrub: rosa rubiginosa in northwestern Patagonia. Biol. Invasions. 12, 3395–3404. doi: 10.1007/s10530-010-9738-3
Cavanaugh, J. E. (1997). Unifying the Derivations for the Akaike and Corrected Akaike Information Criteria. Department of Statistics. Columbia, MO: University of Missouri.
Chateauneuf, A., Fuentes, A., and Garrido, F. (2011). Política y Economía del Sector Forestal Chile. Santiago: Ocho libro ediciones.
Chmura, D., and Salachna, A. (2016). The errors in visual estimation of plants cover in the context of education of phytosociology. Chem. Didact. Ecol. Metrol. 21, 75–82. doi: 10.1515/cdem-2016-0006
Chuong, J., Huxley, J., Spotswood, E. N., Nichols, L., Mariotte, P., and Suding, K. N. (2016). Cattle as dispersal vectors of invasive and introduced plants in a california annual grassland. Rangeland Ecol. Manag. 69, 52–58. doi: 10.1016/j.rama.2015.10.009
Cifuentes-Croquevielle, C., Stanton, D. E., and Armesto, J. J. (2020). Soil invertebrate diversity loss and functional changes in temperate forest soils replaced by exotic pine plantations. Sci. Rep-UK. 10:7762. doi: 10.1038/s41598-020-64453-y
Cofré, H. L., Böhning-Gaese, K., and Marquet, P. A. (2007). Rarity in Chilean forest birds: which ecological and life-history traits matter? Diversity Distrib. 13, 203–212. doi: 10.1111/j.1472-4642.2006.00312.x
Cole, E., Lindsay, A., Newton, M., and Bailey, J. D. (2018). Vegetation control and soil moisture depletion related to herbicide treatments on forest plantations in northeastern Oregon. Weed Technol. 32, 461–474. doi: 10.1017/wet.2018.24
Cuddington, K. (2011). Legacy effects: the persistent impact of ecological interactions. Biol. Theory 6, 203–210. doi: 10.1007/s13752-012-0027-5
Deconchat, M., Brockerhoff, E. G., and Barbaro, L. (2009). Effects of surrounding landscape composition on the conservation value of native and exotic habitats for native forest birds. Forest Ecol. Manag. 258S, S196–S204. doi: 10.1016/j.foreco.2009.08.003
Dethier, M. N., Graham, E. S., Cohen, S., and Tear, L. M. (1993). Visual versus random-point percent cover estimation: “objective” is not always better. Mar. Ecol. Prog. Ser. 96, 93–100.
Devictor, V., Julliard, R., and Jiguet, F. (2008). Distribution of specialist and generalist species along spatial gradients of habitat disturbances and fragmentation. Oikos 117, 507–514. doi: 10.1111/j.2008.0030-1299.16215.x
Díaz, I. A., Armesto, J. J., Reid, S., Sieving, K. E., and Wilson, M. F. (2005). Linking forest structure and composition: avian diversity in successional forests of Chiloé Island. Chile. Biol. Consev. 123, 91–101. doi: 10.1016/j.biocon.2004.10.011
Donoso, C. (2006). Las Especies Arbóreas de los Bosques Templados de Chile y Argentina: Autoecología. Valdivia: Marisa Cuneo Ediciones.
Donoso, C., and Lara, A. (1995). “Utilización de los bosques nativos en Chile: pasado, presente y futuro,” in Ecología de los bosques nativos de Chile, eds J. J. Armesto, C. Villagrán, and M. K. Arroyo (Santiago: Editorial Universitaria), 363–387.
Echeverría, C., Coomes, D., Salas, J., Rey-Benayas, J. M., Lara, A., and Newton, A. (2006). Rapid deforestation and fragmentation of chilean temperate forests. Biol. Conserv. 130, 481–494. doi: 10.1016/j.biocon.2006.01.017
Elizalde, R. (1970). La Sobrevivencia de Chile. La Conservación de sus Recursos Naturales Renovables. Santiago: Ministerio de Agricultura.
Ellis, E. C., Kaplan, J. O., Fuller, D. Q., Vavrus, S., Goldenwijk, K. K., and Verburg, P. H. (2013). Used planet: a global history. PNAS 110, 7978–7985. doi: 10.1073/pnas.1217241110
Escobar, M. A. H., Uribe, S. V., Chiappe, R., and Estades, C. F. (2015). Effect of clearcutting operations on the survival rate of small mammal. PLoS One 10:e0118883. doi: 10.1371/journal.pone.0118883
Estades, C. F., and Temple, S. (1999). Temperate-forest bird communities in a fragmented landscape dominated by exotic pine plantations. Ecol. Appl. 9, 573–585.
Fierro, A., and Vergara, P. M. (2019). A native long horned beetle promotes the saproxylic diversity in exotic plantations of Monterey pine. Ecol. Indic. 96, 532–539. doi: 10.1016/j.ecolind.2018.09.018
Foster, D. R., Motzkin, G., and Slater, B. (1998). Land-use history as long-term broad-scale disturbance: regional forest dynamics in Central New England. Ecosystems 1, 96–119.
Fraterrigo, J. M. (2013). Landscape legacies. Enc. Biodiv. 4, 524–530. doi: 10.1016/B978-0-12-384719-5.00388-9
Gallé, R., Torma, A., and Maák, I. (2016). The effect of forest age and habitat structure on the ground-dwelling ant assemblages of lowland poplar plantations. Agric. For. Entomol. 18, 151–156. doi: 10.1111/afe.12148
Gibbs, H. K., Ruesch, A. S., Achard, F., Clayton, M. K., Holmgren, P., Ramankutty, N., et al. (2010). Tropical forests were the primary sources of new agricultural land in the 1980s and 1990s. PNAS 108, 16732–16737. doi: 10.1073/pnas.0910275107
Gómez, P., Bustamante, R., San Martín, J., and Hahn, S. (2011). Estructura poblacional de Pinus radiata D.Don en fragmentos de Bosque Maulino en Chile central. Gayana bot. 68, 97–101.
Gómez, P., Hahn, S., and San Martin, J. (2009). Structure and floristic composition of a shrub under Pinus radiata D. Don plantation in Central Chile. Gayana Bot. 66, 256–268.
Gómez-García, D. (2008). “Método para el estudio de los pastos, su caracterización ecológica y valoración,” in Pastos del Pirineo, eds F. Fillat, R. García-González, D. Gómez-García, and R. Reiné (España: Consejo Superior de Investigaciones Científicas), 75–109.
González-Moreno, M., Pino, J., Careras, D., Basnou, C., Fernández-Rebollar, I., and Vilá, M. (2013). Quantifying the landscape influence on plant invasions in Mediterranean coastal hábitat. Landscape Ecol. 28, 891–903. doi: 10.1007/s10980-013-9857-1
Hansen, A. J., Knight, R. L., Marzluff, J. M., Powell, S., Brown, K., Gude, P. H., et al. (2005). Effects of exurban development on biodiversity: patterns, mechanisms, and research needs. Ecol. Appl. 15, 1893–1905.
Harden, C. P., Chin, A., English, M. R., Fu, R., Galvin, K. A., Gerlak, A. K., et al. (2014). Understanding human-landscape interactions in the “Anthropocene”. Environ. Manage. 53, 4–13. doi: 10.1007/s00267-013-0082-0
Harrington, T. B., and Edwards, M. B. (1999). Understory vegetation, resource availability, and litterfall responses to pine thinning and woody vegetation control in longleaf pine plantations. Can. J. For. Res. 29, 1055–1064.
Harrison, P. J., Buckland, S. T., Yuan, Y., Elston, D. A., Brewer, M. J., Johnston, A., et al. (2014). Assessing trends in biodiversity over space and time using the example of British breeding birds. J. Appl. Ecol. 51, 1650–1660. doi: 10.1111/1365-2664.12316
Heinrichs, S., Pauchard, A., and Schall, P. (2018). Native plant diversity and composition across a Pinus radiata D.Don plantation landscape in South-Central Chile. The impact of plantation age, logging roads and alien species. Forests 9:567. doi: 10.3390/f9090567
Hermy, M., and Veyheren, K. (2007). Legacies of the past in the present-day forest biodiversity: a review of past land-use effects on forest plant species composition and diversity. Ecol. Res. 22, 361–371. doi: 10.1007/s11284-007-0354-3
Huber, A., Iroume, A., and Bathurst, J. (2008). Effect of Pinus radiata plantations on water balance in Chile. Hydrol. Process. 22, 142–148. doi: 10.1002/hyp.6582
Huber, A., Iroumé, A., Mohr, C., and Frêne, C. (2010). Efecto de las plantaciones de Pinus radiata y Eucalyptus globulus sobre el recurso agua en la Cordillera de la Costa de la Región del Biobío. Chile. Bosque. 31, 219–230.
Ibarra, J. T., and Martin, K. (2015). Biotic homogenization: loss of avian functional richness and habitat specialists in disturbed Andean temperate forests. Biol. Conserv. 192, 418–427. doi: 10.1016/j.biocon.2015.11.008
Imaizumi, F., Sidle, R. C., and Kamei, R. (2008). Effects of forest harvesting on the occurrence of landslides and debris flows in steep terrain of central Japan. Earth Surf. Proc. Land. 33, 827–840. doi: 10.1002/esp.1574
IREN-CORFO (1965). Evaluación de la Erosión de la Cordillera de la Costa entre Valparaíso y Cautín. Informe n°3. Santiago: CORFO.
Jeffries, S. B., Wentworth, T. R., and Allen, H. L. (2010). Long-term effect of establishment practices on plant communities across successive rotations in a loblolly pine (Pinus taeda) plantation. Forest Ecol. Manag. 260, 1548–1556. doi: 10.1016/j.foreco.2010.08.003
Johnston, J. W. (1976). Similariry Índices I. What do we Measure?. Washington, DC: Pacific Northwest laboratories.
Jorquera, C. (2001). “Evolución agropecuaria de la Región de Coquimbo: análisis contextual para la conservación de la vegetación nativa,” in Libro Rojo de la Flora Nativa y de los Sitios Prioritarios Para su Conservación: Región de Coquimbo, eds F. A. Squeo, G. Arancio, and J. R. Gutiérrez (La Serena: Ediciones Universidad de La Serena), 225–237.
Kirkpatrick, L., Mitchell, S. N., and Park, J. P. (2018). The metric matters when assessing diversity: assessing lepidopteran species richness and diversity in two habitats under different disturbance regimes. Ecol. Evol. 8, 11134–11142. doi: 10.1002/ece3.4581
Kusuma, Y. W. C., Rembold, K., Tjitrosoedirdjo, S. S., and Kreft, H. (2018). Tropical rainforest conversion and land use intensification reduce understorey plant phylogenetic diversity. J. Appl. Ecol. 55, 2216–2226. doi: 10.1111/1365-2664.13201
Kuussaari, M., Bommarco, R., Heikkinen, R. K., Helm, A., Krauss, J., Lindborg, R., et al. (2009). Extinction debt: a challenge for biodiversity conservation. Trends Ecol. Evol. 21, 564–571. doi: 10.1016/j.tree.2009.04.011
Lambin, E. F., and Meyfroidt, P. (2011). Global land use change, economic globalization, and the looming land scarcity. PNAS 108, 3465–3472. doi: 10.1073/pnas.1100480108
Li, Z., Liu, W., Zhang, X., and Zheng, F. (2009). Impacts of land use change and climate variability on hydrology in an agricultural catchment on the Loess Plateau of China. J. Hydrol. 377, 35–42. doi: 10.1016/j.jhydrol.2009.08.007
Licata, J. A., Gyenge, J. E., Fernández, M. E., Schlichter, T. M., and Bond, B. J. (2008). Increased water use by ponderosa pine plantations in northwestern Patagonia, Argentina compared with native forest vegetation. Forest Ecol. Manag. 255, 753–764. doi: 10.1016/j.foreco.2007.09.061
Lima, J. L. (2016). Estudio de Caracterización de la Cadena de Producción y Comercialización de la Industria Forestal: Estructura, Agentes y Prácticas. Informe Final. Santiago: ODEPA, Ministerio de Agricultura.
Lindenmayer, D. B., Hobbs, R. J., and Salt, D. (2003). Plantation forests and biodiversity conservation. Aust. Forestry. 66, 62–66.
Little, C., Lara, A., McPhee, J., and Urrutia, R. (2009). Revealing the impact of forest exotic plantations on water yield in large scale watersheds in South-Central Chile. J. Hydrol. 374, 162–170. doi: 10.1016/j.jhydrol.2009.06.011
Luebert, F., and Pliscoff, P. (2017). Sinopsis Bioclimática y Vegetacional de Chile. Santiago: Editorial Universitaria.
Manor, R., Cohen, O., and Saltz. (2008). Community homogenization and the invasiveness of commensal species in Mediterranean afforested landscapes. Biol. Invasions 10, 507–515. doi: 10.1007/s10530-007-9147-4
McKinney, M. L., and Lockwood, J. L. (1999). Biotic homogenization: a few winners replacing many losers in the next mass extinction. Tree 14, 450–453.
Meli, P., Holl, K. D., Rey Benayas, J. M., Jones, H. P., Jones, P. C., Montoya, D., et al. (2017). A global review of past land use, climate, and active vs. passive restoration effects on forest recovery. PLos One 12:e0171368. doi: 10.1371/journal.pone.0171368
Miranda, A., Altamirano, A., Cayuela, L., Lara, A., and González, M. (2017). Native forest loss in the Chilean biodiversity hotspot: revealing the evidence. Reg. Environ. Change 17, 285–297. doi: 10.1007/s10113-016-1010-7
Moreira, F., Ferreira, A., Abrantes, N., Catry, F., Fernandes, P., Roxo, L., et al. (2013). Occurrence of native and exotic invasive trees in burned pine and eucalypt plantations: implications for post-fire forest conversion. Ecol. Eng. 58, 296–302. doi: 10.1016/j.ecoleng.2013.07.014
Mortelliti, A., Michael, D. R., and Lindenmayer, D. B. (2015). Contrasting effects of pine plantations on two skinks: results from a large-scale “natural experiment” in Australia. Anim. Conserv. 18, 433–441. doi: 10.1111/acv.12190
Muñoz-Sáez, A., Heaton, E. E., Reynolds, M., and Merelender, A. M. (2020). Agricultural adapters from the vineyard landscape impact native oak woodland birds. Agr. Ecosyst. Environ. 300:106960. doi: 10.1016/j.agee.2020.106960
Munteanu, C., Kuemmerle, T., Keuler, N. S., Müller, D., Balázs, P., Dobosz, M., et al. (2015). Legacies of 19th century land use shape contemporary forest cover. Global Environ. Chang. 34, 83–94. doi: 10.1016/j.gloenvcha.2015.06.015
Myers, N., Mittermeier, R. A., Mittermeier, C. G., da Fonseca, G. A. B., and Kent, J. (2000). Biodiversity hotspots for conservation priorities. Nature 403, 853–858.
Nahuelhual, L., Carmona, A., Lara, A., Echeverría, C., and González, M. E. (2012). Land-cover change to forest plantations: proximate causes and implications for the landscape in south-central Chile. Landscape Urban Plan. 107, 12–20. doi: 10.1016/j.landurbplan.2012.04.006
Navarro, A., Utzet, F., Puig, P., Caminal, J., and Martin, M. (2001). La distribución binomial negativa frente a la de Poisson en el análisis de fenómenos recurrentes. Gac. Sanit. 15, 447–452.
Neumann, J. L., Holloway, G. J., Hoodless, A., and Griffiths, G. H. (2017). The legacy of 20th Century landscape change on today’s woodland carabid communities. Divers. Distrib. 23, 1447–1458. doi: 10.1111/ddi.12652
O’Hara, K. L., Stancioiu, P. T., and Spencer, M. A. (2007). Understory stump sprout development under variable canopy density and leaf area in coast redwood. Forest Ecol. Manag. 244, 76–85. doi: 10.1016/j.foreco.2007.03.062
Onaindia, M., and Mitxelena, A. (2009). Potential use of pine plantations to restore native forests in a highly fragmented river basin. Ann. For. Sci. 66:305. doi: 10.1051/forest/2009002
Overbeek, W., Kröger, M., and Gerber, J. F. (2012). An overview of industrial tree plantation conflicts in the global South. Conflicts, trends and resistance struggles. EJOLT Report. 100.
Owen, S. M., Patterson, A. M., Gehring, C. A., Sieg, C. H., Scott Bagget, L., and Fulé, P. A. (2019). Large, high-severity burn patches limit fungal recovery 13 years after wildfire in a ponderosa pine forest. Soil Biol. Biochem. 139:107616. doi: 10.1016/j.soilbio.2019.107616
Oyarzún, C. E., and Peña, L. (1995). Soil erosion and overland flow in forested areas with pine plantations at coastal mountain range. Central Chile. Hydrol. Process. 9, 111–118.
Pauchard, A., and Alaback, P. (2006). Edge types defines alien plant species invasions along Pinus contorta burned, highway and clearcut forest edges. Forest Ecol. Manag. 223, 327–335. doi: 10.1016/j.foreco.2005.11.020
Pawson, S. M., Brockerhoff, E. G., and Didham, R. K. (2009). Native forest generalists dominate carabid assemblages along a stand age chronosequence in an exotic Pinus radiata plantation. Forest Ecol. Manag. 258S, S108–S116. doi: 10.1016/j.foreco.2009.08.012
Pickering, C., and Mount, A. (2010). Do tourists disperse weed seed? A global review of unintentional human-mediated terrestrial seed dispersal on clothing, vehicles and horses. J. Sustai. Tour. 18, 239–256. doi: 10.1080/09669580903406613
Pizarro, R., Valdés, R., García-Chevesich, P., Vallejos, C., Sangüesa, C., Morales, C., et al. (2012). Latitudinal analysis of rainfall intensity and mean annual precipitation in Chile. Chil. J. Agr. Res. 72, 252–261. doi: 10.4067/S0718-58392012000200014
Ponce, A. M., Grilli, G., and Galetto, L. (2012). Frugivory and ornithocorous fruits removal in the Chaco forest fragments of Córdoba (Argentina). Bosque 33, 33–41. doi: 10.4067/S0717-92002012000100004
Prentice, I. C., Cramer, W., Harrison, S. P., Leemans, R., Monserud, R. A., and Solomon, A. M. (1992). A global biome model based on plant physiology and dominance, soil properties and climate. J. Biogeogr. 19, 117–134.
Proença, V. M., Pereira, H. M., Guilherme, J., and Vicente, L. (2010). Plant and bird diversity in natural forests and in native and exotic plantations in NW Portugal. Acta Oecol. 36, 219–226. doi: 10.1016/j.actao.2010.01.002
Ranius, T., and Roberge, J. M. (2011). Effects of intensified forestry on the landscape-scale extinction risk of dead wood dependent species. Biodivers. Conserv. 20, 2867–2882. doi: 10.1007/s10531-011-0143-8
Renne, I. J., and Tracy, B. F. (2013). Disturbance intensity, timing and history interact to affect pasture weed invasion. Basic Appl. Ecol. 14, 44–53. doi: 10.1016/j.baae.2012.10.006
Riley, S. J., DeGloria, S., and Elliiot, R. (1999). A terrain ruggedness index that quantifies topographic heterogeneity. Intermountain J. Sci. 5, 23–27.
Robertson, O. J., McAlpine, C., House, A., and Maron, M. (2013). Influence of interspecific competition and landscape structure on spatial homogenization of avian assemblages. PLoS One 8:e65299. doi: 10.1371/journal.pone.0065299
Rodríguez, R., Marticorena, C., Alarcón, D., Baeza, C., Cavieres, L., Finot, V. L., et al. (2018). Catálogo de la plantas vasculares de Chile. Gayana Bot. 75, 1–430.
Rogers, E., Asenjo, P., Díaz, H., Torrealba, D., and Weinstein, J. (1955). Mensura de las Plantaciones de las Provincias de Linares a Malleco. Santiago: CORFO.
Ross, M. S., Sharik, T. L., and Smith, D. W. (1986). Oak regeneration after clear felling in Southwest Virginia. Forest Sci. 32, 157–169. doi: 10.1093/forestscience/32.1.157
Rudel, T. (2009). Tree farms: driving forces and regional patterns in the global expansion of forest plantations. Land Use Policy 26, 545–550. doi: 10.1016/j.landusepol.2008.08.003
Rudolphi, J., and Gustafsson, L. (2011). Forest regenerating after clear-cutting function as habitat for bryophyte and lichen species of conservation concern. PLoS One 6:e18639. doi: 10.1371/journal.pone.0018639
Saldivia, Z., and De la Jara, G. (2001). La Sociedad Nacional de la Agricultura en el Siglo XIX Chileno: su Rol Social y su Aporte al Desarrollo Científico-tecnológico. Revista Electrónica de Geografía y Ciencias Sociales. Gran Via de les Corts Catalanes.: Universidad de Barcelona.
Sarricolea, P., Herrera-Ossandon, M., and Meseguer-Ruiz, O. (2016). Climatic regionalisation of continental Chile. J. Maps. 13, 66–73. doi: 10.1080/17445647.2016.1259592
Scott, D. F., and Prinsloo, F. W. (2008). Longer-term effect of pine and eucalypt plantations on streamflow. Water Resour. Res. 44, 1–8. doi: 10.1029/2007WR006781
Shahabuddin, G., and Kumar, R. (2007). Effects of extractive disturbance on bird assemblages, vegetation structure and floristics in tropical scrub forest, Sariska Tiger Reserve, India. Forest Ecol. Manag. 246, 175–185. doi: 10.1016/j.foreco.2007.03.061
Sharp, A. C., Barclay, M. V. L., Chung, A. Y. C., and Ewers, R. M. (2019). Tropical logging and deforestation impacts multiple scales of weevil beta-diversity. Biol. Conserv. 234, 172–179. doi: 10.1016/j.biocon.2019.03.024
Šibíková, M., Jarolímek, I., Hegedüšová, K., Májeková, J., Mikulová, K., Slabejová, D., et al. (2019). Effect of planting alien Robinia pseudoacacia trees on homogenization of Central European forest vegetation. Sci. Total Environ. 687, 1164–1175. doi: 10.1016/j.scitotenv.2019.06.043
Simonetti, J. A., Grez, A. A., and Estades, C. F. (2013). Providing habitat for native mammals through understory enhancement in forestry plantations. Conserv. Biol. 27, 1117–1121. doi: 10.1111/cobi.12129
Smaill, S. J., and Garrett, L. G. (2016). Multi-rotation impacts of increased organic matter removal in planted forests. J. Soil Sci. Plant Nut. 16, 287–293. doi: 10.4067/S0718-95162016005000023
Smith, D., Hill, G., and Kenward, H. (2019). The development of late Holocene farmed landscapes: analysis of insect assemblages using a multi-period dataset. Holocene 29, 45–63. doi: 10.1177/0959683618804645
Soto, L., Galleguillos, M., Seguel, O., Sotomayor, B., and Lara, A. (2019). Assessment of soil physical properties’ statuses under different land covers within a landscape dominated by exotic industrial tree plantations in south-central Chile. J. Soil Water Conserv. 74, 21–32. doi: 10.2489/jswc.74.1.12
Spennemann, D. H. R. (2020). Frugivory and seed dispersal revisited: codifying the plant-centred net benefit of animal-mediated interactions. Flora 263:151534. doi: 10.1016/j.flora.2019.151534
Speziale, K. L., Di Virgilio, A., Lescano, M. N., Pirk, G., and Franzese, J. (2018). Synergy between roads and disturbance favour Bromus tectorum L. invasion. PeerJ 6:e5529. doi: 10.7717/peerj.5529
Stefani, V., Lange, D., Vilela, A. A., Ferreira, C. A., and Del-Claro, K. (2017). The influence of fire and deforestation on the floral symmetry and fitness of Adenocalymma nodosun (Bignoniaceae). Sociobiology 64, 301–309. doi: 10.13102/sociobiology.v64i3.1270
Stokely, T., Kormann, U., and Betts, M. G. (2020). Synergistic effect of wild ungulate and management intensification suppress native plants and promote exotics. Forest Ecol. Manag. 460:117772. doi: 10.1016/j.foreco.2019.117772
Sullivan, T. P., Sullivan, D. S., Lindgren, P. M. F., and Ransome, D. B. (2009). Stand structure and the abundance and diversity of plants and small mammals in natural and intensively managed forests. Forest Ecol. Manag. 258, S127–S141. doi: 10.1016/j.foreco.2009.06.001
Sweaney, N., Driscoll, D. A., Lindenmayer, D. B., and Porch, N. (2015). Plantations, not farmlands, cause biotic homogenisation of ground-active beetles in south-eastern Australia. Biol. Conserv. 186, 1–11. doi: 10.1016/j.biocon.2015.02.02
Taki, H., Inoue, T., Tanaka, H., Makihara, H., Sueyoshi, M., Isono, M., et al. (2010). Responses of community structure, diversity, and abundance of understory plants and insect assemblages to thinning in plantations. Forest Ecol. Manag. 259, 607–613. doi: 10.1016/j.foreco.2009.11.019
Tardif-Paradis, C., Simard, M. J., Leroux, G. D., Panneton, B., Nurse, R. E., and Vanasse, A. (2015). Effect of planter and tractor wheels on row and inter-row weed populations. Crop Prot. 71, 66–71. doi: 10.1016/j.cropro.2015.01.026
Tilman, D., May, R. M., Lehman, C. L., and Nowak, M. A. (1994). Habitat destruction and the extinction debt. Nature 371, 65–66.
Tomasevic, J. A., and Estades, C. F. (2008). Effects of the structure of pine plantations on their “Softness” as barriers for ground-dwelling birds in South-central Chile. Forest Ecol. Manag. 255, 810–816. doi: 10.1016/j.foreco.2007.09.073
Trentini, C. P., Campanello, P. I., Villagra, M., Ritter, L., Ares, A., and Goldstein, G. (2017). Thinning of loblolly pine plantations in subtropical Argentina: impact on microclimate and understory vegetation Forest Ecol. Manag 384, 236–247. doi: 10.1016/j.foreco.2016.10.040
Tropp, J. (2003). Displaced people, replaced narratives: forest conflicts and historical perspectives in the Tsolo district. Transkei. J. S. Afr. Stud. 29, 207–233. doi: 10.1080/0305707032000060458
Turner, J., and Lambert, M. (2000). Change in organic carbon in forest plantation soils in eastern Australia. Forest Ecol. Manag. 133, 231–247.
Uribe, S. V., and Estades, C. F. (2014). Reptiles in monterey pine plantations of the Coastal Range of Central Chile. Rev. Chi. Hist. Nat. 87, 25. doi: 10.1186/S40693-014-0025-Z
Uribe, S. V., Estades, C. F., and Radeloff, V. C. (2020). Pine plantations and five decades of land use change in central Chile. PLoS One 15:e0230193. doi: 10.1371/journal.pone.0230193
Vergara, P. M., and Simonetti, J. A. (2006). Abundance and movement of understory birds in a Maulino forest fragmented by pine plantations. Biodivers. Conserv. 15, 3937–3947. doi: 10.1007/s10531-005-2355-2
Wang, Z., Yang, H., Wang, D., and Zhao, Z. (2019). Response of height growth of regenerating trees in a Pinus tabulaeformis Carr. plantation to different thinning intensities. Forest Ecol. Manag. 444, 280–289. doi: 10.1016/j.foreco.2019.04.042
Whittaker, R. J., and Jones, S. H. (1994). The role of frugivorous bats and birds in the rebuilding of a tropical forest ecosystem. Krakatau, Indonesia. J. Biogeogr. 21, 245–258.
Woods, R. V. (1990). Second rotation decline in P. radiata plantations in South Australia has been corrected. Water Air Soil Poll. 54, 607–619. doi: 10.1007/BF00298697
Zhou, X., Zhu, H., Wen, Y., Goodale, U., Zhu, Y., Yu, S., et al. (2019). Intensive management declines in soil nutrients lead to serious exotic plant invasion in Eucalyptus plantations under successive short-rotation regimes. Land Degrad. Dev. 31, 297–310. doi: 10.1002/ldr.3449
Zhu, X., Liu, W., Jiang, X. J., Wang, P., and Li, W. (2018). Effect of land-use changes on runoff and sediment yield: implications for soil conservation and forest management in Xishuangbanna. Southwest China. Land Degrad. Dev. 29, 2962–2974. doi: 10.1002/ldr.3068
Keywords: land-use change, forest plantations, biotic homogenization, understory, birds, Pinus radiata
Citation: Uribe SV, García N and Estades CF (2021) Effect of Land Use History on Biodiversity of Pine Plantations. Front. Ecol. Evol. 9:609627. doi: 10.3389/fevo.2021.609627
Received: 23 September 2020; Accepted: 08 June 2021;
Published: 28 June 2021.
Edited by:
Elena D. Concepción, National Museum of Natural Sciences (MNCN), SpainReviewed by:
Isabel Castro, Autonomous University of Madrid, SpainCopyright © 2021 Uribe, García and Estades. This is an open-access article distributed under the terms of the Creative Commons Attribution License (CC BY). The use, distribution or reproduction in other forums is permitted, provided the original author(s) and the copyright owner(s) are credited and that the original publication in this journal is cited, in accordance with accepted academic practice. No use, distribution or reproduction is permitted which does not comply with these terms.
*Correspondence: Sandra V. Uribe, c2FuZHJ1bUB1Y2hpbGUuY2w=; Nicolás García, bmdhcmNpYUB1Y2hpbGUuY2w=
Disclaimer: All claims expressed in this article are solely those of the authors and do not necessarily represent those of their affiliated organizations, or those of the publisher, the editors and the reviewers. Any product that may be evaluated in this article or claim that may be made by its manufacturer is not guaranteed or endorsed by the publisher.
Research integrity at Frontiers
Learn more about the work of our research integrity team to safeguard the quality of each article we publish.