- Department of Integrative Biology, The University of Texas at Austin, Austin, TX, United States
Sexual reproduction is nearly ubiquitous in the vertebrate world, yet its evolution and maintenance remain a conundrum due to the cost of males. Conversely, asexually reproducing species should enjoy a twofold population increase and thus replace sexual species all else being equal, but the prevalence of asexual species is rare. However, stable coexistence between asexuals and sexuals does occur and can shed light on the mechanisms asexuals may use in order to persist in this sex-dominated world. The asexual Amazon molly (Poecilia formosa) is required to live in sympatry with one of its sexual sperm hosts –sailfin molly (Poecilia latipinna) and Atlantic molly (Poecilia mexicana)—and are ecological equivalents to their host species in nearly every way except for reproductive method. Here, we compare the visual lateralization between Amazon mollies and sailfin mollies from San Marcos, Texas. Neither Amazon mollies nor sailfin mollies exhibited a significant eye bias. Additionally, Amazon mollies exhibited similar levels of variation in visual lateralization compared to the sailfin molly. Further investigation into the source of this variation –clonal lineages or plasticity—is needed to better understand the coexistence of this asexual-sexual system.
Introduction
Sexual reproduction is practiced by 99.9% of species—either exclusively or at one point in their life—despite the 2-fold cost of sex (Avise, 2008). Historical arguments suggest that this cost is more than offset by the benefit to sex that accrues from greater genetic variance (Williams, 1975; Maynard Smith, 1978; Maynard Smith and Szathmary, 1999). Recent studies further highlight the importance of breaking apart genetic associations during recombination; separating deleterious alleles from beneficial alleles, as well as spreading beneficial alleles across a wide genomic background increases the efficiency of selection and adaptation to a capricious environment (Barton and Charlesworth, 1998; Otto, 2009; Sharp and Otto, 2016; McDonald et al., 2016). Indeed, empirical studies now document the long-term, evolutionary benefit held by sexually reproducing populations compared to asexually reproducing populations (Poon and Chao, 2004; Cooper, 2007; Becks et al., 2012; Gray and Goddard, 2012; McDonald et al., 2016). Despite this, some asexual species persist (Bi and Bogart, 2010; Fradin et al., 2017; Warren et al., 2018), even to the point of being named “ancient” (Heethoff et al., 2009; Schön et al., 2009). Of particular interest are the forms of asexual reproduction—gynogenesis and hybridogenesis—that require the asexual and sexual species to coexist along much of their range (reviewed in Avise, 2008; gynogenetic: Poecilia formosa, Menidia clarkhubbsi, Phoxinus eos-neogaeus, Cobitis elongatoides-taenia, Fundulus heeroclitus; hybridogenetic: Poeciliopsis monacha-lucida, Rana esculenta). The aforementioned studies investigated asexuals and sexuals in isolation, however, further investigations are needed into how asexual species can persist alongside sexual species in light of the benefits of sex.
Coexisting asexual and sexual species often mediate direct competition through niche partitioning, in which asexuals occupy a narrow portion of the habitat or resource range of their host species (MacArthur and Pianka, 1966; Vrijenhoek, 1978; Fussey and Sutton, 1981; Schenck and Vrijenhoek, 1986; Case, 1990; Rist et al., 1997; Martins et al., 1998; Negovetic et al., 2001). However, not all coexisting species exhibit niche separations, so their coexistence proposes even more of a dilemma. One such species complex is the Amazon and sailfin molly system. The Amazon molly (Poecilia formosa) is an asexual fish originating from a single hybridization event between an Atlantic molly female (P. mexicana) and sailfin molly male (P. latipinna) over 100,000 years ago in Tampico, Mexico (Schartl et al., 1995b; Stöck et al., 2010; Warren et al., 2018 but see Alberici da Barbiano et al., 2013). Amazon mollies reproduce via gynogenesis –females usually require the sperm of a closely related species to trigger embryogenesis, but the paternal genome is usually excluded (Schlupp, 2005; occasional paternal introgression discussed in Schartl et al., 1995a). This type of reproduction requires that they occupy the range of one of their host species, thus placing them in direct competition. Indeed, the Amazon molly now covers a broad range of habitats from Rio Tuxpan, Mexico to the Nueces River, Texas USA –as well as introduced locations in central Texas, always sympatric with one of its sexual hosts (Schlupp et al., 2002). Previous investigations into this asexual-sexual system found few significant differences among morphological traits, physiological traits, and ecological traits (Table 1). Their continued coexistence with their sexual host may rely on genetic and phenotypic variation. This variation may occur at the population level, with genetic variation partitioned amongst different clonal lineages (Lampert et al., 2006; Stöck et al., 2010; Alberici da Barbiano et al., 2013; Warren et al., 2018). Phenotypic variation can also result from behavioral plasticity. Cognitive behaviors are often the most plastic phenotype (Bell et al., 2009). Plasticity in asexual species may provide them with a broad range of responses to environmental change and thus assist in persistence.
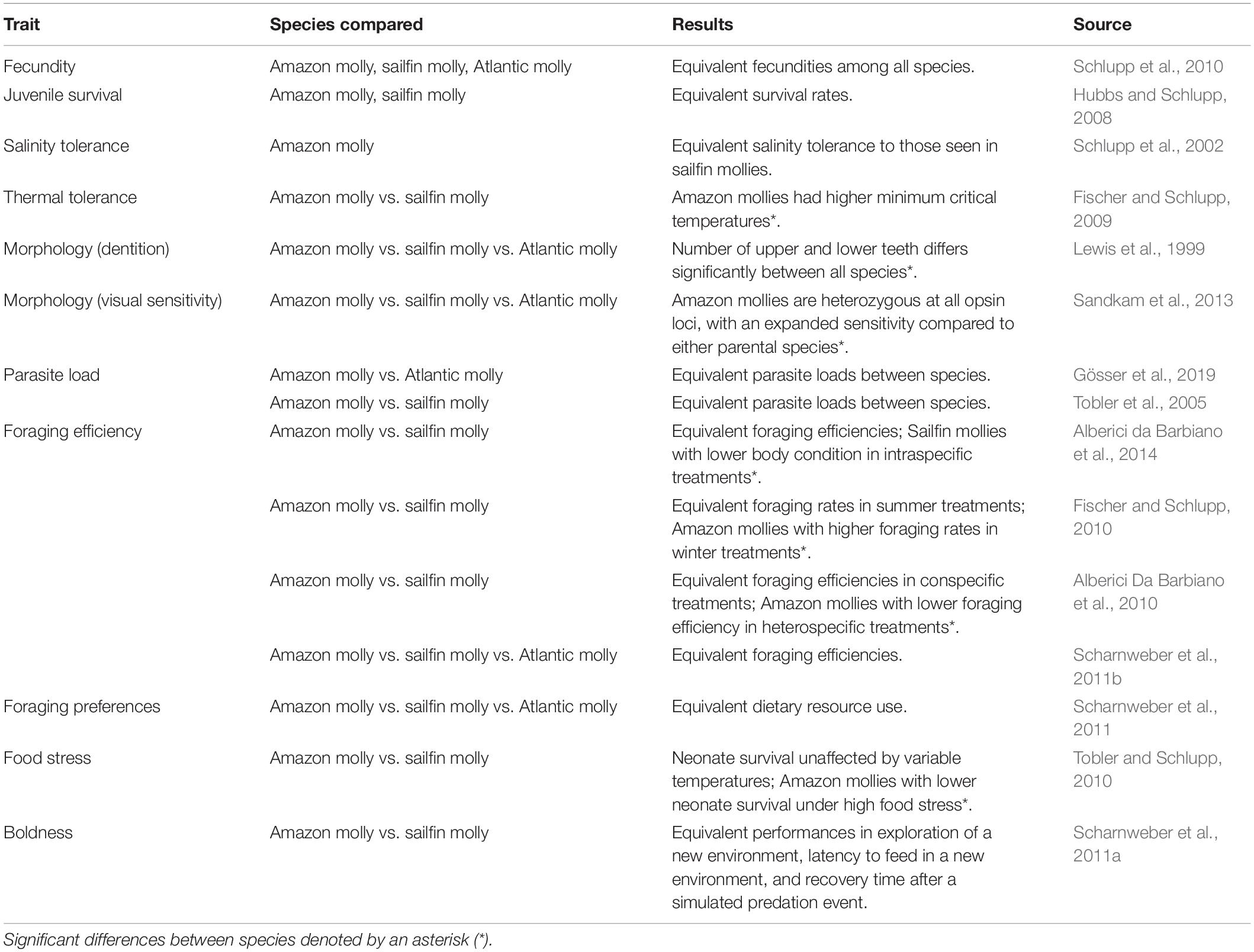
Table 1. Summary table of all traits previously compared between the Amazon molly and its sexual host.
In this study we focus on cognitive behaviors –which is lacking in previous comparisons—to determine if average performance or variation in performance could shed light on the coexistence of the asexual Amazon molly with its sexual host. We used a well-studied cognitive task—visual lateralization (Clayton, 1993; McGrew and Marchant, 1999; Pascual et al., 2004; Rogers et al., 2004; Vallortigara and Rogers, 2005; Rogers and Vallortigara, 2008). Visual lateralization is known to vary based on the stimulus (Bisazza et al., 1997a,b, 1998, 1999; De Santi et al., 2001; Sovrano et al., 2001; Fuss et al., 2019), environmental pressures such as predation (Brown et al., 2004; Ferrari et al., 2015), and density dependent selection (Ghirlanda and Vallortigara, 2004; Nakajima et al., 2004). Visual lateralization is present in many poeciliid species in response to some but not all stimuli, including the two species used in this study: Girardinus falcatus, Gambusia holbrooki, G. nicaraguensis, Brachyrhaphis roseni (Bisazza et al., 1997b), Poecilia latipinna, P. formosa, P. mexicana, P. reticulata (Fuss et al., 2019). Additionally, eye bias is heritable for one poeciliid species (Bisazza et al., 2000). We chose to examine lateralization using a mirror image scrutiny test (Sovrano et al., 1999; De Santi et al., 2001). The mirror image scrutiny test assesses the preference for left- or right-eye use when examining a reflection. Here we compare eye use between the asexual Amazon molly and sexual sailfin molly.
Materials and Methods
Sampling was approved by Texas State University (permit 04-523-691) and all animal procedures were approved by the University of Texas Institutional Animal Care and Use Committees (AUP-2018-00089).
Specimen
We collected sailfin and Amazon mollies from San Marcos, Texas (29.89, −97.93) in August 2018 and 2019 and brought them back to the lab at the University of Texas in Austin. We separated species into 37-liter tanks with approximately eight individuals to a tank; male sailfin mollies were included in this total and the ratio of males to females was balanced for both species. Each tank contained gravel and air filtration and set to a 14:10 light dark schedule. All fish were fed twice daily ad libitum with commercial fish flakes (Tetramin, Germany). We injected unique elastomer tags into fish at least 72 h prior to experimentation, to track identity throughout experimentation. We tested 25 female sailfin mollies and 27 Amazon mollies in June–August 2019 (2018 collection specimen, both species) and June 2020 (2019 collection specimen, both species). Prior to experiments, we removed fish from communal tanks and placed them individually in 3-L isolation tanks for 24 h.
Apparatus
The experiment consisted of two identical tanks (length × width × height: 41 × 21 × 26 cm) to control for any tank effect. Tanks contained 20 cm high Acrylic mirrors on the two longer walls and white corrugated plastic board completely covering the two shorter walls. Black corrugated poster board covered the exterior of the long walls to prevent visuals of the room. Blue filter (Marineland magnum bonded pad filter media) covered the bottom of the tank and each tank was filled with 15 cm of treated water, aerated prior to trial. A camera (Microsoft lifecam cinema, 720p HD) attached to the center of an aquarium light (NICREW deluxe LED aquarium light, full spectrum 18-Watt, 1,200 LM, 7,500 K) was positioned above each tank using a desk clamp mount; the clamp mount was visible from the tank so we positioned it on the right for one tank and on the left for the other tank to account for any side bias. An isolation zone created from gray PVC (diameter 10 cm) affixed with a plastic lid was used for each trial.
Procedure
This procedure follows De Santi et al. (2001). Each fish was randomly assigned an experimental tank order. We removed the fish from isolation using a small fish net and placed into a beaker with approximately 300 mL of water. We then gently poured the fish into the isolation zone positioned in the center of the tank for a 5 min acclimation period. Recording (Debut video capture, NCH software) started at the end of acclimation after removing the isolation zone and lasted for a 10 min period. A second trial immediately followed in the other tank. We measured standard length prior to returning the fish to the isolation tank. We subsequently drained, cleaned, and refilled both testing tanks with treated water in preparation for the next test subject.
Videos were scored using BORIS (version 7.6). The scoring code consisted of a left ocular use, right ocular use, or no direct ocular use. See Figure 1 for how ocular use was determined. The video was transformed into a series of frames occurring every 0.1 s. Scoring began at the 10 s mark and continued every 2 s until the 10 min mark. We saved the results for each individual tank as well as combined both tank results for total ocular use (referred to as “combined” in subsequent analyses).
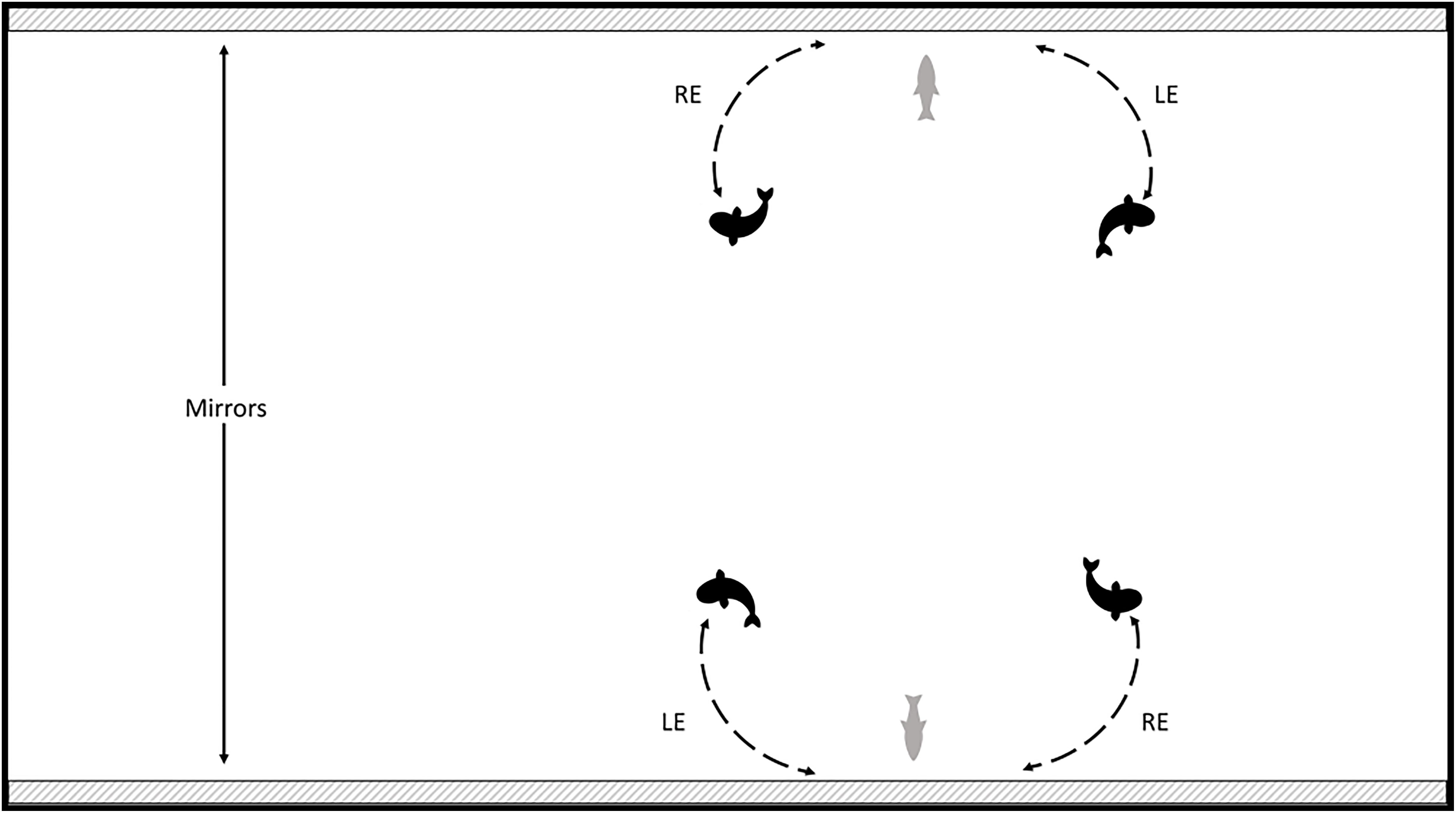
Figure 1. Qualifications for calculating laterality index based on ocular use with closest mirror. LE is left eye use, and RE is right eye use. The gray fish in the middle indicates no specific eye use.
Analyses were performed in R studio 1.1.456. We calculated each fish’s laterality index score for tank A, tank B, and combined video results following Bisazza et al. (1997b): (left-eye use—right-eye use)/(left-eye use + right-eye use) ∗ 100; positive values would indicate a preference for left-eye use and negative values would indicate preference for right-eye use. We performed a paired t-test test to determine if the laterality index score differed significantly between tanks, thus detecting any tank effects. A two-tailed one-sample t-test determined within species eye bias with the null assumption of a zero index score. Additionally, we calculated Pearson’s correlation coefficients to determine the repeatability of eye use across trials in both species. We used a t-test to determine differences in mean eye bias between the species as well as a t-test examining overall laterality; overall laterality is based on the absolute value of the index scores to indicate the degree to which each species is lateralized, regardless of direction. Lastly, we performed a two-tailed F-test on index scores to determine if variation in eye preference for sailfin mollies was greater or less than variation in Amazon mollies.
Results
All raw data can be found in the Supplementary Material. Normality was confirmed using a Kolmogorov-Smirnov test for both species (sailfin: D = 0.19, p = 0.28; Amazon: D = 0.23, p = 0.10). We found no clear difference in eye laterality between tanks A and B for each species (paired t-test: sailfin: df = 24, p = 0.61, 95% CI -11.67—7.00; Amazon: df = 26, p = 0.24, 95% CI -16.24—4.23; Figure 2). Therefore, we used the laterality index score calculated from combined A and B video results for all subsequent analyses (excluding Pearson’s correlation).
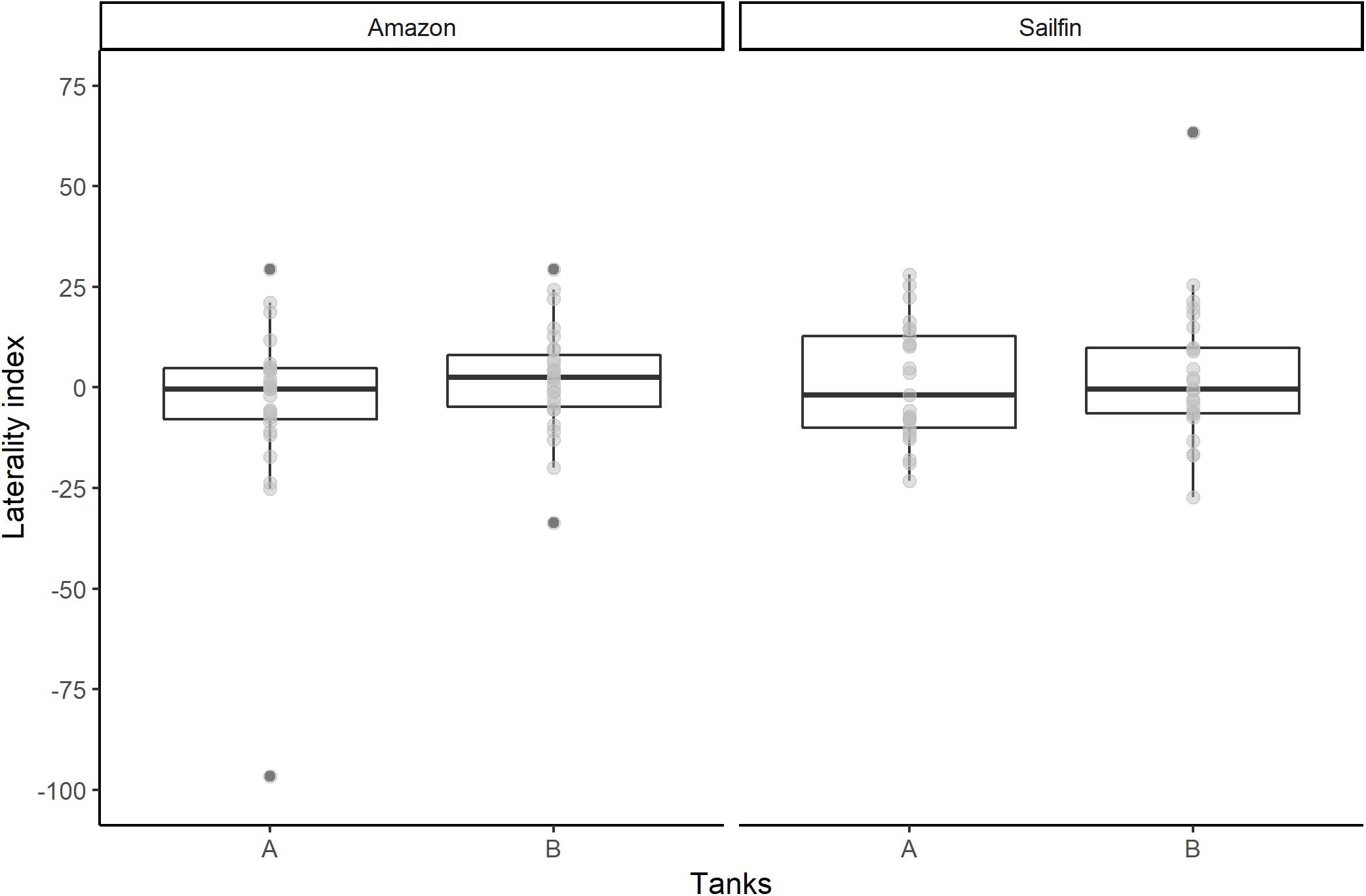
Figure 2. Boxplots (—, median; □, 25–75th percentile; outliers in dark gray; raw data in light gray) of laterality index scores across tanks for both species. Eye use did not significantly differ between tank A and B for either Amazon mollies or sailfin mollies (paired t-tests).
Ocular preference within species was determined as a significant deviation from a zero laterality index score. Neither sailfin mollies nor Amazon mollies showed a significant eye bias during mirror image scrutiny (one-sample two-tailed t-test: sailfin: p = 0.33, mean = 2.40, 95% CI −2.62—7.42; Amazon: p = 0.63, mean = −1.21, 95% CI −6.34—3.92; Figure 3). There was also no significant correlation between eye use in tank A and tank B for either species (Pearson’s correlation coefficient: sailfin: R = 0.05, p = 0.81; Amazon: R = -0.005, p = 0.98). Sailfin mollies did not clearly differ in mean eye preference when compared to Amazon mollies (two-tailed t-test: df = 49.99, p = 0.30, 95% CI −3.39—10.61). Additionally, there was no clear difference in the degree of lateralization between Amazon and sailfin mollies (two-sided t-test: df = 49.70, p = 0.81, 95% CI −4.55—5.80). Lastly, we did not find a significant difference in variation between the species (F-test: F = 0.88, p = 0.76; Figure 4).
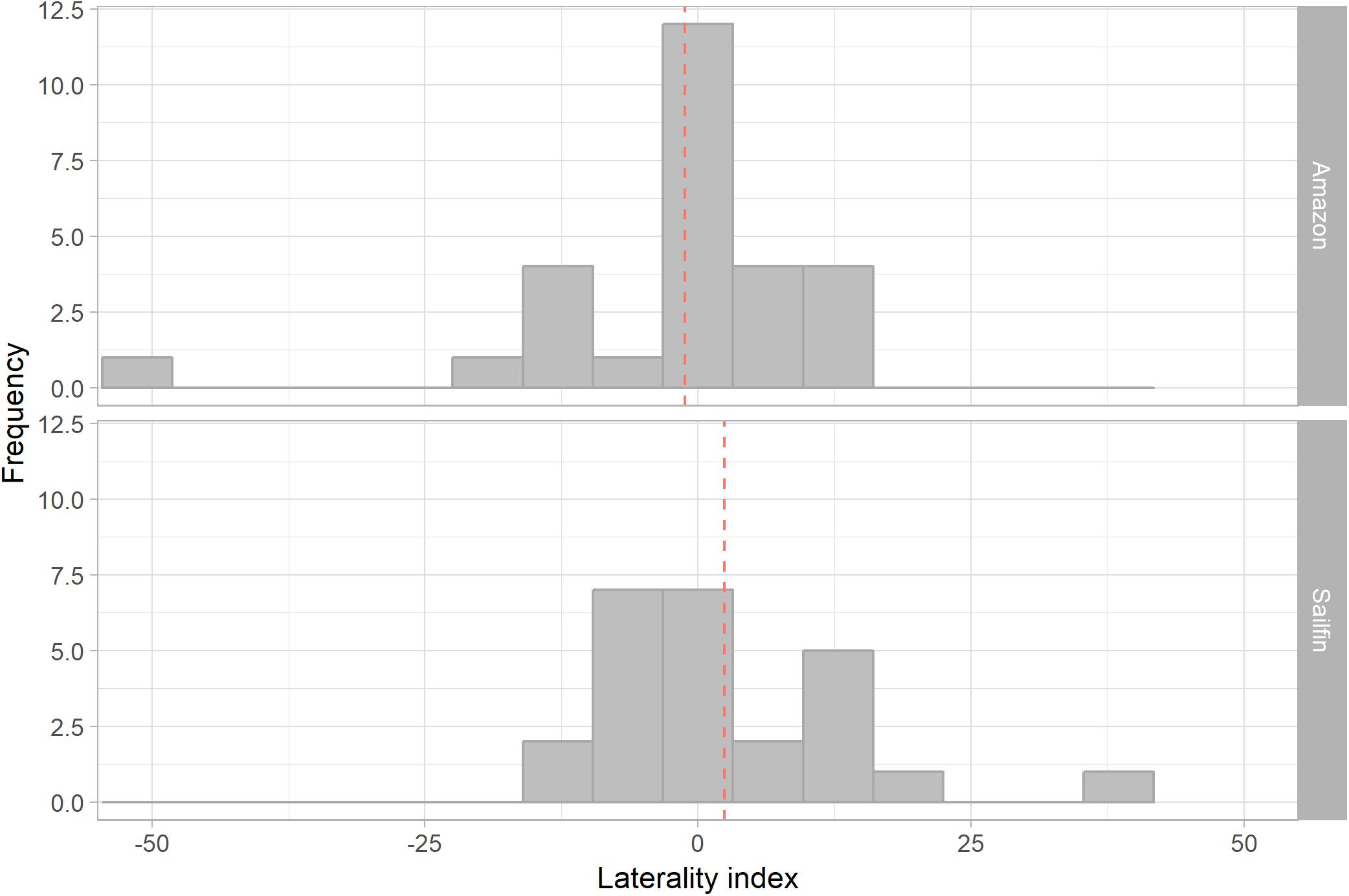
Figure 3. Frequency of laterality index scores among Amazon and sailfin mollies. The red dashed line represents the group mean. Variation in laterality index scores was not clearly different between Amazon and sailfin mollies.
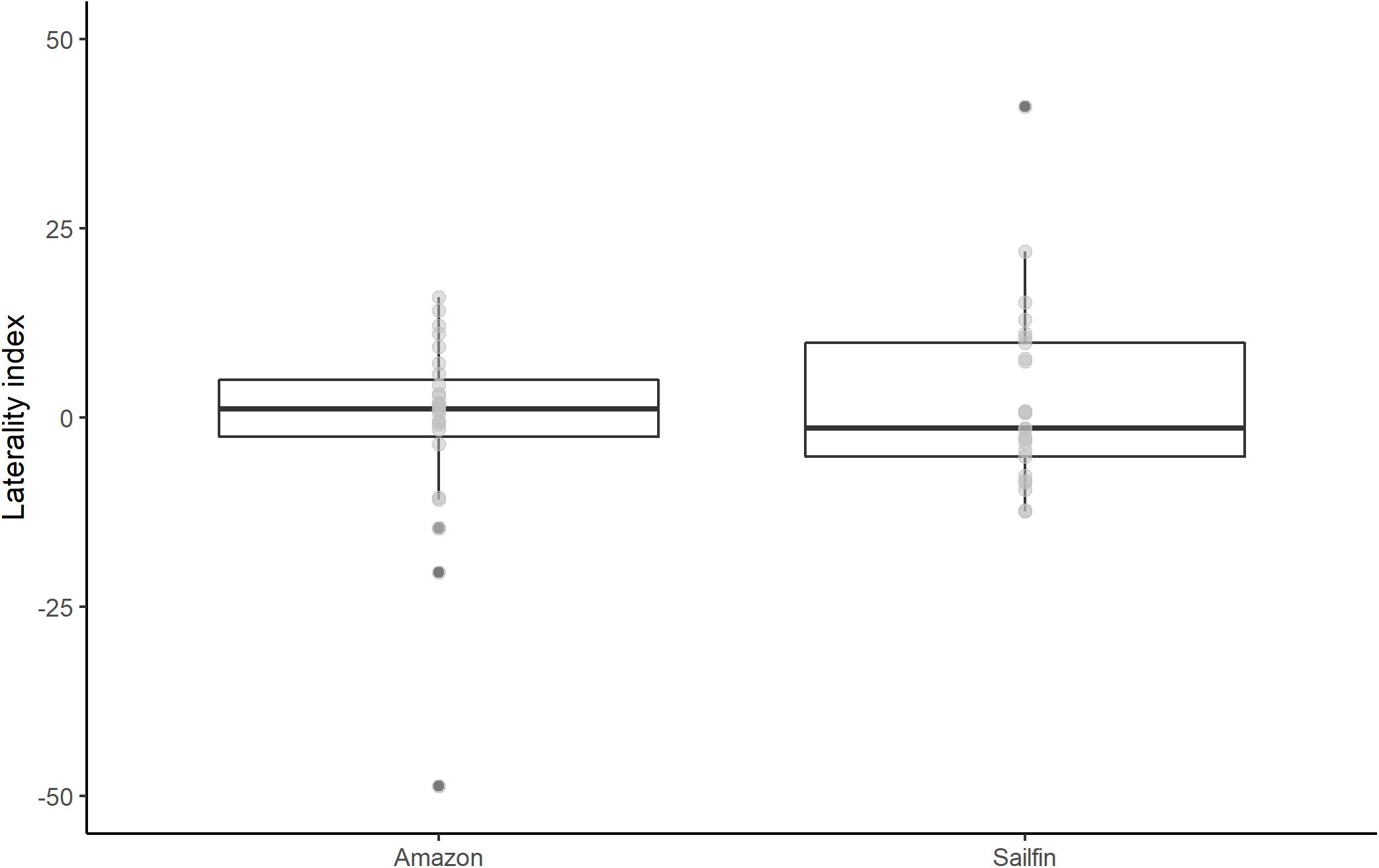
Figure 4. Boxplots (, median; □, 25–75th percentile; outliers in dark gray; raw data in light gray) of laterality index scores for Amazon and sailfin mollies. Neither species exhibited a significant eye bias (one-sample t-tests), nor did the species clearly differ in their average laterality index score (two-sample t-tests).
Discussion
Asexual species are often touted as evolutionary dead ends due to mutation accumulation and the lack of recombination (Muller, 1964; Maynard Smith, 1978; Lynch et al., 1993; Lynch and Gabriel, 2006), yet some asexual species persist despite these costs (Heethoff et al., 2009; Schön et al., 2009; Bi and Bogart, 2010; Fradin et al., 2017; Warren et al., 2018). The asexual Amazon molly lives in direct competition with its sexual counterpart, the sailfin molly and they appear to be ecological equivalents in morphology, physiology, and ecology (Table 1). Our investigation into cognitive behavior, specifically visual lateralization, shows no significant difference in average performance or variation between the Amazon molly and sailfin molly. While our results showing similar average performance are in line with previous comparative studies between these species, our variation results highlight a potential mechanism for the persistence of the asexual Amazon molly.
Neither species clearly expressed visual lateralization in this study. Organisms with laterally positioned eyes discriminate conflicting stimuli through separate but parallel processing (Rogers, 2000), increasing their ability to distinguish between two stimuli [food vs. non-food (Vallortigara et al., 1998; Güntürkün et al., 2000) and food vs. predator (Güntürkün et al., 2000; Rogers, 2000; Rogers et al., 2004)]. Furthermore, mixed visual lateralization in schooling fish determine the fishes’ position within the school and overall school cohesion (Dadda and Bisazza, 2006; Bibost and Brown, 2013). A number of studies using poeciliid species found a clear eye bias, with female poeciliids commonly exhibiting a left-eye bias for conspecific stimuli and a right-eye bias for predator or male stimuli (Bisazza et al., 1997a,b, 1998, 1999; De Santi et al., 2001; Fuss et al., 2019). Therefore, the type of stimulus used, particularly the social stimulus, can affect lateralization. Fuss et al. (2019) found a statistically significant left-eye bias for female sailfin mollies and Amazon mollies when viewing a female group stimulus, although no such bias when viewing a single female. While most fish are not thought to recognize their own reflection (Kohda et al., 2019), the lack of visual bias and similar variation in both species of our study could indicate a lack of motivation to perform lateralization for the mirror reflection meant to represent a single female. The lack of motivation to a mirror image may be specific to the species used here, as previous studies using the mirror image scrutiny setup obtained lateralized responses from other fish species (Sovrano et al., 1999; De Santi et al., 2001). Further testing with different stimuli might show the degree to which the social environment influences visual lateralization for both species. Predation can also influence the strength and direction of lateralization: high-predation environments induced a strong lateralized response to novel and predator stimuli in the poeciliid Brachyraphis episcopi but no clear lateralization appeared for B. episcopi from low-predation environments (Brown et al., 2004). While predatory species are present at our San Marcos site (personal observation), the degree of predation and its influences on visual lateralization in this population are unknown.
Previous comparative studies, including one on visual lateralization (Fuss et al., 2019), focus on the average performance between the Amazon molly and sailfin molly. Here we also focus on the variation in behavior; variation in asexual populations may indicate a potential mechanism to circumvent the classic costs of this reproductive style and thus perpetuate their coexistence with a sexual species. The Amazon and sailfin mollies of our study exhibited similar levels of variation in the visual lateralization task. One way to obtain variation in an asexual population is through clonal lineages (Schartl et al., 1995b; Stöck et al., 2010; Alberici da Barbiano et al., 2013; Warren et al., 2018). Populations of Amazon mollies may contain multiple clonal lineages (Lampert et al., 2006; Stöck et al., 2010; Gösser et al., 2019); it is currently unknown how many clonal lineages existed or developed since the introduction of Amazon mollies to our study site in the 1950s (Schlupp et al., 2002). However, it is possible that our sample contains individuals from multiple clonal lineages thus leading to higher-than-expected levels of variation. Two invertebrate studies investigating morphological variation similarly found equal or higher levels of variation in clonal populations compared to the sexual populations (Oliver and Herrin, 1976; Atchley, 1977). However, neither study addressed the effects of multiple clonal lineages on the variation seen within these wild populations. Studies that account for clonal type or lineage find that the asexual species contain less phenotypic variation than the sexual species, and variation within the asexual species is partitioned among clonal lines (Parker, 1979; Vrijenhoek, 1984; Cullum, 2000; however see Doeringsfeld et al., 2004 and discussion below). Further investigation into the clonal lineages potentially present in our sample is underway.
Asexual populations can also achieve variation via plastic responses to environmental characteristics. Doeringsfeld et al. (2004) found similar levels of morphological variation in one clonal lineage (i.e., one genotype) of asexual dace (Phoxinus eos-neogaeus) as the sexual host species. They attributed this finding to the plasticity of the asexual genome, thus allowing the asexual species to cohabit the broad range of their hosts. Indeed, further work on this asexual complex showing epigenetic variation associated with pH tolerance rather than genetic variation emphasizes the role of environmentally induced plasticity in the maintenance of asexual-sexual systems (Massicotte and Angers, 2012). The similar levels of variation and lack of repeatability between trials may be the result of plastic responses to the rearing or testing environment; a previous study with Amazon mollies highlights the potential of the rearing environment to invoke plasticity in behavior (Bierbach et al., 2017). It is clear future work investigating the coexistence of the Amazon molly with its sexual host must include the variance brought about by clonal lineages and environmentally induced plasticity.
Data Availability Statement
The original contributions presented in the study are included in the article/Supplementary Material, further inquiries can be directed to the corresponding author/s.
Ethics Statement
The animal study was reviewed and approved by the University of Texas Institutional Animal Care and Use Committees.
Author Contributions
AC and MR conceived and designed the study and drafted the manuscript. AC collected field specimen, executed experiments, and performed the data analysis. Both authors contributed to the article and approved the submitted version.
Funding
This research was funded in part by grants from the Texas Ecolab and the Integrative Biology Department at the University of Texas at Austin (AC) and the Clark Hubbs Regents Professorship (MR).
Conflict of Interest
The authors declare that the research was conducted in the absence of any commercial or financial relationships that could be construed as a potential conflict of interest.
Acknowledgments
We thank A. Wallendorf for field site correspondence and the Meadows Center for Water and the Environment for access to the sampling sites. We also thank the numerous undergraduate assistants who helped in field collection, video recording and video scoring. This work was previously presented as a poster at the Society for Integrative Biology, Austin 2020.
Supplementary Material
The Supplementary Material for this article can be found online at: https://www.frontiersin.org/articles/10.3389/fevo.2021.605943/full#supplementary-material
References
Alberici da Barbiano, L., Gompert, Z., Aspbury, A. S., Gabor, C. R., and Nice, C. C. (2013). Population genomics reveals a possible history of backcrossing and recombination in the gynogenetic fish Poecilia formosa. Proc. Natl. Acad. Sci. U.S.A. 110, 13797–13802. doi: 10.1073/pnas.1303730110
Alberici da Barbiano, L., Robinson, R. J., Tobler, M., Aspbury, A. S., and Gabor, C. R. (2014). Differences in resource assimilation between the unisexual Amazon molly, Poecilia formosa (Poeciliidae) and its sexual host (Poecilia latipinna). Environ. Biol. Fishes 97, 875–880. doi: 10.1007/s10641-013-0188-5
Alberici Da Barbiano, L., Waller, J., and Gabor, C. R. (2010). Differences in competitive efficiency between a sexual parasite and its host provide insight into the maintenance of a sperm-dependent vertebrate species. J. Freshw. Ecol. 25, 523–530. doi: 10.1080/02705060.2010.9664401
Atchley, W. R. (1977). Evolutionary consequences of parthenogenesis: evidence from the Warramaba virgo complex. Proc. Natl. Acad. Sci. U.S.A. 74, 1130–1134. doi: 10.1073/pnas.74.3.1130
Avise, J. C. (2008). Clonality: The Genetics, Ecology, and Evolution of Sexual Abstinence in Vertebrate Animals. New York, NY: Oxford University Press.
Barton, N. H., and Charlesworth, B. (1998). Why sex and recombination? Science 218, 1986–1990. doi: 10.1126/science.281.5385.1986
Becks, L., Agrawal, A. F., and Barton, N. H. (2012). The evolution of sex is favoured during adaptation to new environments. PLoS Biol. 10:1001317. doi: 10.1371/journal.pbio.1001317
Bell, A. M., Hankison, S. J., and Laskowski, K. L. (2009). The repeatability of behaviour: a meta-analysis. Anim. Behav. 77, 771–783. doi: 10.1016/j.anbehav.2008.12.022
Bi, K., and Bogart, J. P. (2010). Time and time again: unisexual salamanders (genus Ambystoma) are the oldest unisexual vertebrates. BMC Evol. Biol. 10:238. doi: 10.1186/1471-2148-10-238
Bibost, A., and Brown, C. (2013). Laterality influences schooling position in rainbowfish, Melanotaenia spp. PLoS One 8:e80907. doi: 10.1371/journal.pone.0080907
Bierbach, D., Laskowski, K. L., and Wolf, M. (2017). Behavioural individuality in clonal fish arises despite near-identical rearing conditions. Nat. Commun. 8:15361. doi: 10.1038/ncomms15361
Bisazza, A., De Santi, A., and Vallortigara, G. (1999). Laterality and cooperation: mosquitofish move closer to a predator when the companion is on their left side. Anim. Behav. 57, 1145–1149. doi: 10.1006/anbe.1998.1075
Bisazza, A., Facchin, L., Pignatti, R., and Vallortigara, G. (1998). Lateralization of detour behaviour in poeciliid fish: the effect of species, gender and sexual motivation. Behav. Brain Res. 91, 157–164. doi: 10.1016/S0166-4328(97)00114-9
Bisazza, A., Facchin, L., and Vallortigara, G. (2000). Heritability of lateralization in fish: concordance of right-left asymmetry between parents and offspring. Neurophsychologia 38, 907–912. doi: 10.1016/S0028-3932(00)00018-X
Bisazza, A., Pignatti, R., and Vallortigara, G. (1997a). Detour tests reveal task-and stimulus-specific behavioural lateralization in mosquitofish (Gambusia holbrooki). Behav. Brain Res. 89, 237–242. doi: 10.1016/S0166-4328(97)00061-2
Bisazza, A., Pignatti, R., and Vallortigara, G. (1997b). Laterality in detour behaviour: interspecific variation in poeciliid fish. Anim. Behav. 54, 1273–1281. doi: 10.1006/anbe.1997.0522
Brown, C., Gardner, C., and Braithwaite, V. A. (2004). Population variation in lateralized eye use in the poeciliid Brachyraphis episcopi. Proc. Biol. Sci. 271, S455–S457. doi: 10.1098/rsbl.2004.0222
Case, T. J. (1990). Patterns of coexistence in sexual and asexual species of Cnemidophorus lizards. Oecologia 83, 220–227. doi: 10.1007/BF00317756
Clayton, N. (1993). Lateralization and unilateral transfer of spatial memory in marsh tits. J. Comp. Physiol. 171, 799–806. doi: 10.1007/BF00213076
Cooper, T. F. (2007). Recombination speeds adaptation by reducing competition between beneficial mutations in populations of Escherichia coli. PLoS Biol. 5:e225. doi: 10.1371/journal.pbio.0050225
Cullum, A. J. (2000). Phenotypic variability of physiological traits in populations of sexual and asexual whiptail lizards (genus Cnemidophorus). Evol. Ecol. Res. 2, 841–855.
Dadda, M., and Bisazza, A. (2006). Does brain asymmetry allow efficient performance of simultaneous tasks? Anim. Behav. 72, 523–529. doi: 10.1016/j.anbehav.2005.10.019
De Santi, A., Sovrano, V. A., Bisazza, A., and Vallortigara, G. (2001). Mosquitofish display differential left- and right-eye use during mirror image scrutiny and predator inspection responses. Anim. Behav. 61, 305–310. doi: 10.1006/anbe.2000.1566
Doeringsfeld, M. R., Schlosser, I. J., Elder, J. F., and Evenson, D. P. (2004). Phenotypic consequences of genetic variation in a gynogenetic complex of Phoxinus eos-neogaeus clonal fish (Pisces: Cyprinidae) inhabiting a heterogeneous environment. Evolution 58, 1261–1273. doi: 10.1111/j.0014-3820.2004.tb01705.x
Ferrari, M. C. O., McCormick, M. I., Allan, B. J. M., Choi, R. B., Ramasamy, R. A., and Chivers, D. P. (2015). The effects of background risk on behavioural lateralization in a coral reef fish. Funct. Ecol. 29, 1553–1559. doi: 10.1111/1365-2435.12483
Fischer, C., and Schlupp, I. (2009). Differences in thermal tolerance in coexisting sexual and asexual mollies (Poecilia, Poeciliidae, Teleostei). J. Fish Biol. 74, 1662–1668. doi: 10.1111/j.1095-8649.2009.02214.x
Fischer, C., and Schlupp, I. (2010). Feeding rates in the sailfin molly Poecilia latipinna and its coexisting sexual parasite, the gynogenetic Amazon molly Poecilia formosa. J. Fish Biol. 77, 285–291. doi: 10.1111/j.1095-8649.2010.02672.x
Fradin, H., Kiontke, K., Zegar, C., Gutwein, M., Lucas, J., Kovtun, M., et al. (2017). Genome architecture and evolution of a unichromosomal asexual nematode. Curr. Biol. 27, 2928–2939.e6. doi: 10.1016/j.cub.2017.08.038
Fuss, T., Nöbel, S., and Witte, K. (2019). It’s in the eye of the beholder: visual lateralisation in response to the social environment in poeciliids. J. Fish Biol. 94, 759–771. doi: 10.1111/jfb.13953
Fussey, G. D., and Sutton, S. L. (1981). The Identification and distribution of the bisexual and parthenogenetic forms of Trichoniscus pusillus (Isopoda: Oniscoidea) in Ireland. Ir. Nat.’ J. 20, 196–199.
Ghirlanda, S., and Vallortigara, G. (2004). The evolution of brain lateralization: a game-theoretical analysis of population structure. Proc. Biol. Sci. 271, 853–857. doi: 10.1098/rspb.2003.2669
Gösser, F., Schartl, M., García-De León, F. J., Tollrian, R., and Lampert, K. P. (2019). Red queen revisited: immune gene diversity and parasite load in the asexual Poecilia formosa versus its sexual host species P. mexicana. PLoS One 14:e0219000. doi: 10.1371/journal.pone.0219000
Gray, J. C., and Goddard, M. R. (2012). Sex enhances adaptation by unlinking beneficial from detrimental mutations in experimental yeast populations. BMC Evol. Biol. 12:43. doi: 10.1186/1471-2148-12-43
Güntürkün, O., Diekamp, B., Manns, M., Nottelmann, F., Prior, H., Schwarz, A., et al. (2000). Asymmetry pays: visual lateralization improves discrimination success in pigeons. Curr. Biol. 10, 1079–1081. doi: 10.1016/S0960-9822(00)00671-0
Heethoff, M., Norton, R. A., Scheu, S., and Maraun, M. (2009). “Parthenogenesis in oribatid mites (acari, oribatida): evolution without sex,” in Lost Sex: The Evolutionary Biology of Parthenogenesis, eds I. Schön, K. Martens, and P. van Dijk (Dordrecht: Springer Netherlands), 241–257. doi: 10.1007/978-90-481-2770-2
Hubbs, C., and Schlupp, I. (2008). Juvenile survival in a unisexual/sexual complex of mollies. Environ. Biol. Fishes 83, 327–330. doi: 10.1007/s10641-008-9343-9
Kohda, M., Hotta, T., Takeyama, T., Awata, S., Tanaka, H., Asai, J., et al. (2019). If a fish can pass the mark test, what are the implications for consciousness and self-awareness testing in animals? PLoS Biol. 17:e3000021. doi: 10.1371/journal.pbio.3000112
Lampert, K. P., Lamatsch, D. K., Schories, S., Hopf, A., García De León, F. J., and Schartl, M. (2006). Microsatellites for the gynogenetic Amazon molly, Poecilia formosa: useful tools for detection of mutation rate, ploidy determination and overall genetic diversity. J. Genet. 85, 67–71. doi: 10.1007/BF02728973
Lewis, S. R., Rasch, E. M., Hossler, F. E., Kalbfleisch, J. H., and Monaco, P. J. (1999). Comparative study of dentition among species of Poecilia (Pisces). J. Morphol. 239, 271–282. doi: 10.1002/(SICI)1097-4687(199903)239:3<271::AID-JMOR4<3.0.CO;2-B
Lynch, M., Burger, R., Butcher, D., and Gabriel, W. (1993). The mutational meltdown in asexual populations. J. Hered. 84, 340–344. doi: 10.1093/oxfordjournals.jhered.a111354
Lynch, M., and Gabriel, W. (2006). Mutation load and the survival of small populations. Evolution 44:1725. doi: 10.2307/2409502
MacArthur, R. H., and Pianka, E. R. (1966). On optimal use of a patchy environment. Am. Nat. 100, 603–609. doi: 10.1086/282454
Martins, M. J., Collares-Pereira, M. J., Cowx, I. G., and Coelho, M. M. (1998). Diploids v. Triploids of Rutilus alburnoides: spatial segregation and morphological differences. J. Fish Biol. 52, 817–828. doi: 10.1006/jfbi.1997.0628
Massicotte, R., and Angers, B. (2012). General-purpose genotype or how epigenetics extend the flexibility of a genotype. Genet. Res. Int. 2012:317175. doi: 10.1155/2012/317175
Maynard Smith, J., and Szathmary, E. (1999). The Origins of Life: From the Birth of Life to the Origin of Language. New York, NY: Oxford University Press.
McDonald, M. J., Rice, D. P., and Desai, M. M. (2016). Sex speeds adaptation by altering the dynamics of molecular evolution. Nature 531, 233–236. doi: 10.1038/nature17143
McGrew, W. C., and Marchant, L. E. (1999). Laterality of hand use pays off in foraging success for wild chimpanzees. Short Commun. 40, 509–513. doi: 10.1007/bf02557586
Muller, H. J. (1964). The relation of recombination to mutational advance. Mutat. Res. 106, 2–9. doi: 10.1016/0027-5107(64)90047-8
Nakajima, M., Matsuda, H., and Hori, M. (2004). Persistence and fluctuation of lateral dimorphism in fishes. Am. Nat. 163, 692–698. doi: 10.1086/382733
Negovetic, S., Anholt, B. R., Semlitsch, R. D., and Reyer, H. U. (2001). Specific responses of sexual and hybridogenetic European waterfrog tadpoles to temperature. Ecology 82, 766–774. doi: 10.1890/0012-9658(2001)082[0766:srosah]2.0.co;2
Oliver, J. H., and Herrin, C. S. (1976). Differential variation of parthenogenetic and bisexual Haemaphysalis longicornis (Acari: Ixodidae). J. Parasitol. 62, 475–484. doi: 10.2307/3279161
Parker, E. D. Jr. (1979). Phenotypic consequences of parthenogenesis in Cnemidophorus lizards. I. variability in parthenogenetic and sexual populations. Evolution 33, 1150–1166. doi: 10.2307/2407474
Pascual, A., Huang, K.-L., Neveu, J., and Préat, T. (2004). Neuroanatomy: brain asymmetry and long-term memory. Nature 427, 605–606. doi: 10.1038/427605a
Poon, A., and Chao, L. (2004). Drift increases the advantage of sex in RNA bacteriophage Phi6. Genetics 166, 19–24. doi: 10.1534/genetics.166.1.19
Rist, L., Semlitsch, R. D., Hotz, H., and Reyer, H. U. (1997). Feeding behaviour, food consumption, and growth efficiency of hemiclonal and parental tadpoles of the Rana esculenta complex. Funct. Ecol. 11, 735–742. doi: 10.1046/j.1365-2435.1997.00147.x
Rogers, L. J. (2000). Evolution of hemispheric specialization: advantages and disadvantages. Brain Lang. 73, 236–253. doi: 10.1006/brln.2000.2305
Rogers, L. J., Zucca, P., and Vallortigara, G. (2004). Advantages of having a lateralized brain. Proc. Biol. Sci. 271, S420–S422. doi: 10.1098/rsbl.2004.0200
Rogers, L. J., and Vallortigara, G. (2008). From antenna to antenna: lateral shift of olfactory memory recall by honeybees. PLoS One 3:e2340. doi: 10.1371/journal.pone.0002340
Sandkam, B. A., Joy, J. B., Watson, C. T., Gonzalez-Bendiksen, P., Gabor, C. R., and Breden, F. (2013). Hybridization leads to sensory repertoire expansion in a gynogenetic fish, the Amazon Molly (Poecilia formosa): a test of the hybrid-sensory expansion hypothesis. Evolution 67, 120–130. doi: 10.1111/j.1558-5646.2012.01779.x
Scharnweber, K., Plath, M., and Tobler, M. (2011a). Examination of boldness traits in sexual and asexual mollies (Poecilia latipinna, P. formosa). Acta Ethol. 14, 77–83. doi: 10.1007/s10211-011-0097-6
Scharnweber, K., Plath, M., and Tobler, M. (2011b). Feeding efficiency and food competition in coexisting sexual and asexual livebearing fishes of the genus Poecilia. Environ. Biol. Fishes 90, 197–205. doi: 10.1007/s10641-010-9732-8
Scharnweber, K., Plath, M., Winemiller, K. O., and Tobler, M. (2011). Dietary niche overlap in sympatric asexual and sexual livebearing fishes Poecilia spp. J. Fish Biol. 79, 1760–1773. doi: 10.1111/j.1095-8649.2011.03114.x
Schartl, M., Nanda, I., Schlupp, I., Wilde, B., Epplen, J. T., Schmid, M., et al. (1995a). Incorporation of subgenomic amounts of DNA as compensation for mutational load in a gynogenetic fish. Nature 373, 69–71.
Schartl, M., Wilde, B., Schlupp, I., and Parzefall, J. (1995b). Evolutionary origin of a parthenoform, the Amazon molly, Poecilia formosa, on the basis of a molecullar genealogy. Evolution 49, 827–835. doi: 10.1111/j.1558-5646.1995.tb02319.x
Schenck, R. A., and Vrijenhoek, R. C. (1986). Spatial and temporal factors affecting coexistence among sexual and clonal forms of Poeciliopsis. Evolution 40, 1060–1070. doi: 10.1111/j.1558-5646.1986.tb00573.x
Schlupp, I. (2005). The evolutionary ecology of gynogenesis. Annu. Rev. Ecol. Syst. 36, 399–417. doi: 10.1146/annurev.ecolsys.36.102003.152629
Schlupp, I., Parzefall, J., and Schartl, M. (2002). Biogeography of the Amazon molly, Poecilia formosa. J. Biogeogr. 29, 1–6. doi: 10.1046/j.1365-2699.2002.00651.x
Schlupp, I., Taebel-Hellwig, A., and Tobler, M. (2010). Equal fecundity in asexual and sexual mollies (Poecilia). Environ. Biol. Fishes 88, 201–206. doi: 10.1007/s10641-010-9630-0
Schön, I., Rossetti, G., and Martens, K. (2009). “Lost sex: the evolutionary biology of parthenogenesis,” in Lost Sex: The Evolutionary Biology of Parthenogenesis, eds I. Schön, K. Martens, and P. van Dijk (Dordrecht: Springer Netherlands), 217–240. doi: 10.1007/978-90-481-2770-2
Sharp, N. P., and Otto, S. P. (2016). Evolution of sex: using experimental genomics to select among competing theories. BioEssays 38, 751–757. doi: 10.1002/bies.201600074
Sovrano, V. A., Bisazza, A., and Vallortigara, G. (2001). Lateralization of response to social stimuli in fishes: a comparison between different methods and species. Physiol. Behav. 74, 237–244. doi: 10.1016/S0031-9384(01)00552-2
Sovrano, V. A., Rainoldi, C., Bisazza, A., and Vallortigara, G. (1999). Roots of brain specializations: preferential left-eye use during mirror-image inspection in six species of teleost fish. Behav. Brain Res. 106, 175–180. doi: 10.1016/S0166-4328(99)00105-9
Stöck, M., Lampert, K. P., Möller, D., Schlupp, I., and Schartl, M. (2010). Monophyletic origin of multiple clonal lineages in an asexual fish (Poecilia formosa). Mol. Ecol. 19, 5204–5215. doi: 10.1111/j.1365-294X.2010.04869.x
Tobler, M., and Schlupp, I. (2010). Differential susceptibility to food stress in neonates of sexual and asexual mollies (Poecilia. Poeciliidae). Evol. Ecol. 24, 39–47. doi: 10.1007/s10682-008-9288-7
Tobler, M., Wahli, T., and Schlupp, I. (2005). Comparison of parasite communities in native and introduced populations of sexual and asexual mollies of the genus Poecilia. J. Fish Biol. 67, 1072–1082. doi: 10.1111/j.0022-1112.2005.00810.x
Vallortigara, G., and Rogers, L. J. (2005). Survival with an asymmetrical brain: advantages and disadvantages of cerebral lateralization. Behav. Brain Sci. 28, 575–589. doi: 10.1017/S0140525X05000105
Vallortigara, G., Rogers, L. J., Bisazza, A., Lippolis, G., and Robins, A. (1998). Complementary right and left hemifield use for predatory and agonistic behavior in toads. NeuroReport 9, 3341–3344. doi: 10.1097/00001756-199810050-00035
Vrijenhoek, R. C. (1978). Coexistence of clones in a heterogeneous environment. Science 199, 549–552. doi: 10.1126/science.199.4328.549
Vrijenhoek, R. C. (1984). “The evolution of clonal diversity in Poeciliopsis,” in Evolutionary Genetics of Fishes, ed. B. Turner (New York, NY: Plenum Press), 399–429. doi: 10.1007/978-1-4684-4652-4_8
Warren, W., García-Pérez, R., Xu, S., Lampert, K., Chalopin, D., Stöck, M., et al. (2018). Clonal polymorphism and high heterozygosity in the celibate genome of the Amazon molly. Nat. Ecol. Evol. 2, 669–679. doi: 10.1038/s41559-018-0473-y
Keywords: clones, mirror image response, Poecilia formosa, Poecilia latipinna, sexual reproduction
Citation: Connelly AD and Ryan MJ (2021) Phenotypic Variation in an Asexual-Sexual Fish System: Visual Lateralization. Front. Ecol. Evol. 9:605943. doi: 10.3389/fevo.2021.605943
Received: 13 September 2020; Accepted: 20 January 2021;
Published: 11 February 2021.
Edited by:
Andrea S. Aspbury, Texas State University, United StatesReviewed by:
Jonathan Mee, Mount Royal University, CanadaCaitlin R. Gabor, Texas State University, United States
Copyright © 2021 Connelly and Ryan. This is an open-access article distributed under the terms of the Creative Commons Attribution License (CC BY). The use, distribution or reproduction in other forums is permitted, provided the original author(s) and the copyright owner(s) are credited and that the original publication in this journal is cited, in accordance with accepted academic practice. No use, distribution or reproduction is permitted which does not comply with these terms.
*Correspondence: Allison D. Connelly, YWNkYXZpc0B1dGV4YXMuZWR1