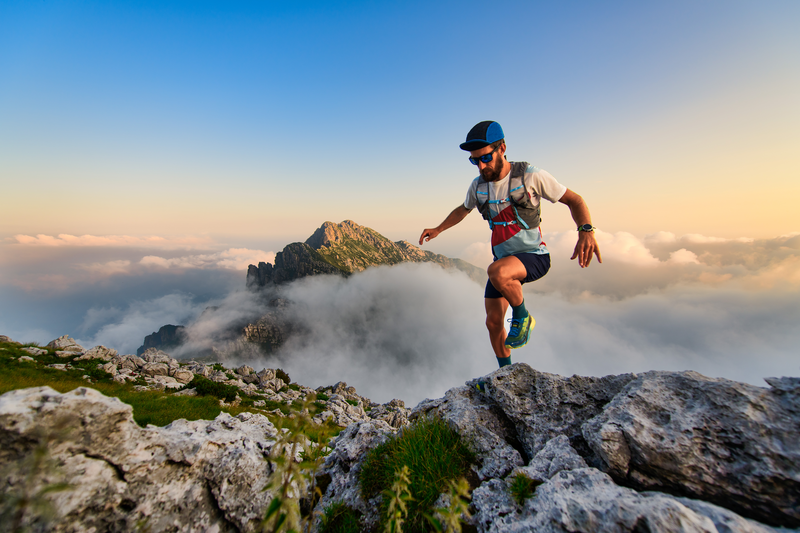
95% of researchers rate our articles as excellent or good
Learn more about the work of our research integrity team to safeguard the quality of each article we publish.
Find out more
ORIGINAL RESEARCH article
Front. Ecol. Evol. , 04 May 2021
Sec. Conservation and Restoration Ecology
Volume 9 - 2021 | https://doi.org/10.3389/fevo.2021.604509
This article is part of the Research Topic Overcoming the Global Climate Crisis: Solutions to Minimize the Loss of Mediterranean Plants View all 6 articles
The soil system has been frequently overlooked during plant reintroduction planning and practice since working with soils and plant roots can be difficult, particularly in saline environments. Coastal saline environments are major contributors to regional and global biodiversity and an important source of endemic species. However, various species are in decline or considered threatened, particularly halophytes (salt tolerant) due to negative anthropic impacts. The Lusitanian endemic halophyte Limonium daveaui formerly had a large distribution range along the west coast of Portugal but currently it shows a restricted distribution in the Tagus estuary. Field surveys revealed that this critically endangered species forms few local populations with small size invaded by exotic species. In this study, we investigated the potential utilization of Technosols, an innovative sustainable, ecological engineering method combined with brackish water irrigation for potential L. daveaui reintroduction in native habitats. Seed germination percentages were evaluated in different environmental conditions. Through a microcosm assay, a Technosol was constructed using a saline Fluvisol with a mixture of low value inorganic and organic wastes, which were chemically characterized. Plants were cultivated in the Fluvisol and Technosol and irrigated with brackish water collected in the nearby area. To assess plant growth, morphometric parameters and the plants’ physiological status were assessed and the fresh and dry biomass determined. Results showed that seed germination was higher on moist filter paper with distilled water than in Fluvisol or Technosol. Plants grown in Technosol had a greater development, with higher values of photosynthetic indexes and biomass production than in Fluvisol. Our findings provide a basis for future in situ conservation studies and support the idea that eco-friendly soil technology approaches are beneficial to conserve rare halophyte species.
Reintroduction of native species is an important issue in conservation and has been increasingly acknowledged in international treaties and legislation, particularly endemic species (Maunder, 1992; Godefroid et al., 2011; Maschinski and Albrecht, 2017). Conservation of coastal endemics from rocky cliffs, sand dunes and salt marshes is challenging due to the high degree of habitat specialization of these species and their vulnerability to disturbance by several natural factors, invasive species and human activities (Baumberger et al., 2012; Martins et al., 2013; Caperta et al., 2014; Lechuga-Lago et al., 2017; Olsson et al., 2019).
The Portuguese coast is rich in endemic flora due to a singular biogeographic position (Braun-Blanquet et al., 1972; Asensi et al., 1993; van der Maarel and van der Maarel-Versluys, 1996; Costa et al., 2012) and about 35% of all Portuguese Natura 2000 habitats consist of coastal habitats (Costa et al., 2007; Duarte et al., 2016). The critically endangered endemic species Limonium daveaui Erben had a large distribution in the marshes of the Tagus Estuary (SW Portugal, SW Europe) in the past (Erben, 1978, 1993; Franco, 1984; Espírito-Santo et al., 2012; Caperta and Carapeto, 2020) but currently it is narrowly distributed in this area (Caperta and Carapeto, 2020). Among the reasons invoked for the continuous decline of the species in this estuary are the deterioration of its habitat quality due to invasive species (e.g., Carpobrotus edulis) and anthropic pressures (Caperta and Carapeto, 2020) like industry activities (petrochemical and steelwork) with huge requirements for space and suitable terrain for industrial complexes in the Lisbon Metropolitan Area (Costa, 2013; Fernandes et al., 2020). Moreover, the Tagus estuary is also affected by other anthropic activities (chemicals, ship construction and repair and cement manufacture), agriculture-fertilizers and pesticides (Duarte et al., 2014). Due to these activities, this estuary was considered as contaminated since 1985 (Figueres et al., 1985), with high levels of anthropic Hg in Fluvisols (Cesário et al., 2016).
A detailed understanding of the biology and microenvironmental requirements including soil is needed for successful reintroduction of rare species (Holl and Hayes, 2006; Dunwiddie and Martin, 2016). Conservation practitioners are increasingly using diverse soil-based technologies to propagate and restore rare plant species (Haskins and Pence, 2012). One of the most challenging aspects of rare species reintroduction is the low success obtained when seeds rather than seedlings are used to start new populations in situ (Davy, 2002; Godefroid et al., 2011; Albrecht and Maschinski, 2012). Physically adverse environments and soil disturbance have also been shown to limit the possible success of species reintroduction using directly sown seeds (Wang et al., 2014; Menges et al., 2016; Cao et al., 2018; van Regteren et al., 2020), as observed in salt marshes where soil disturbance reduces successful seedling establishment (Balke et al., 2011). In the surface layer of sediments/soils, poor drainage may result in the build-up of potentially toxic dissolved species, high salinity, and anoxia that make seed germination difficult (Spencer et al., 2017). An alternative environmentally friendly management of Fluvisols is via elaboration of Technosols, man-made tailored soils, whose properties and pedogenesis are dominated by their technical origin (IUSS Working Group, 2015). These soils could be elaborated from wastes and employed in the subsequent recovery of degraded and/or polluted soils, improving their physicochemical and biological properties (Macía et al., 2014; Cortinhas et al., 2020), while contributing to the circular economy (Breure et al., 2018).
This study aims to assess the potential of tailored soils to improve (i) soil properties, (ii) seed germination, (iii) seedling establishment, and (iv) plant growth of an endemic, critically endangered species, through a microcosm assay using estuarine water irrigation. The study also hopes to develop awareness of eco-friendly soil technologies for conservation of rare species.
The Fluvial beach “Praia do Sobralinho,” Sobralinho, in the Tagus Estuary (38°54′16.1″N, 9°01′09.0″W), in the Municipal Council of Vila Franca de Xira1, is integrated in the Lisbon metropolitan area, on the west coast of Portugal (Instituto da Conservação da Natureza e das Florestas [ICNF]., 2012; Figure 1). The saline tide reaches about 50 km upstream from the river mouth, near Vila Franca de Xira (Guerreiro et al., 2015).
Figure 1. The study area located in Sobralinho salt marsh area, on the right bank of the river Tagus, in the Municipal Council of Vila Franca de Xira, west Portugal.
The upper saltmarsh area encompasses plant communities with diverse halophytes found in NATURA 2000: Habitat 1420 Mediterranean and thermo-Atlantic halophilous scrubs (Sarcocornetea fruticosi) and in the priority Habitat 2130 Mediterraneo-Atlantic fixed gray dunes (The Environmental Protection Agency of Portugal [APA], 2011), where the Lusitanian endemic Limonium daveaui (syn. Limonium auriculae-ursifolium (Pourr.) Druce subsp. lusitanicum (Pignatti) Pignatti; Limonium ramosissimum (Poir.) Maire subsp. confusum (Gren. et Godr.) Pignatti (Pignatti, 1971) thrives (Espírito-Santo et al., 2012). This species comprises perennial plants with a basal rosette, and oblanceolate-spatulate glabrous, three parallel veined leaves. The inflorescences (scapes) are branched in the lower third, with a few sterile branches, and present bluish violaceous flowers blooming between June and August (Erben, 1978, 1993; Franco, 1984).
A microcosm assay was set up with a Salic Fluvisol (Eutric) (FLU; IUSS Working Group, 2015) collected in Sobralinho salt marsh area, which was dried and sieved (<2 mm) and a Technosol (TEC). The TEC was produced with 85% of FLU and 15% of organic/inorganic wastes mixture that were added and mixed manually. The wastes mixture used as amendments was constituted by sludge and waste kieselguhr from breweries, medium sand (0.25–0.5 mm), gravel limestone (2 mm < Ø < 5 mm) and residual biomass obtained from pruning, in the proportion 1.5:0.5:3:2:3, respectively. The FLU and TEC were potted and incubated at 70% of the maximum water-holding capacity, in the dark, for 28 days. After the incubation, and before seedling transplantation, composite samples from each pot (0–15 cm of depth) were collected and analyzed. This process was repeated at the end of the assay. For the microcosm assay, the seedlings were obtained from seeds germinated on wet filter paper with deionized water and after 2.5 months (∼10 cm) they were transplanted to the substrata, in five replicates, irrigated with brackish water collected from a channel located in the Tagus estuary, keeping the substrata at 70% of the maximum water-holding capacity (400 ml).
The FLU, amendments and TEC were characterized following methods in Póvoas and Barral (1992) for: pH and electric conductivity (EC) in a water suspension (1:2.5 m/V); extractable K (Kextract; Egner–Riehm method) and P (Pextract; Olsen method); total N (Ntotal; Kjeldahl method); organic C (Corg) by wet combustion; micronutrients (Lakanen and Erviö, 1971). For the FLU, the cation exchange capacity (CEC) by 1 mol/dm3 ammonium acetate, particle size distribution analysis by sieving and sedimentation, and multielemental concentration by ICP-MS after acid digestion (Activation Laboratories, 2020) were determined.
The brackish water was analyzed for: pH (potentiometry), EC (conductivimetry), Cl– (Mohr, 1945), HCO3– (titration method using HCl solution methyl orange as indicator), Na, Ca, Mg, K, P, Fe, Zn, Mn, and Cu (atomic absorption spectrometry). The Na adsorption ratio (SAR) was calculated by ([Na]/(([Ca] + [Mg])1/2)) (Ayers and Westcot, 1985; Lesch and Suarez, 2009).
The L. daveaui seeds were collected in Samouco salterns complex and germinated in transparent boxes containing filter paper, TEC and FLU, respectively. Each box contained 36 seeds equally distant from each other and 170 ml of deionized water. The boxes were exposed at the temperature 20–25 °C with a 16 h light and 8 h dark photoperiod, in a controlled chamber (Rumed) as described in Róis et al. (2012).
To understand and compare the evolution of plant vegetative and reproductive growth in the substrata, morphometric and photosynthetic parameters were measured every 2.5 months, one measure in each season of the year. The measurements were taken at transplantation, in winter (T0); in spring (T1), in summer (T2) and at the end of the microcosm assay, in autumn (T3). The number of live and dead leaves, leaf length and width, scape number and length were determined. For leaf size measurements about three leaves/individual were labeled (15 leaves/treatment) at the beginning of the assay (T0). The Photochemical Reflectance Index (PRI) was measured with a PlantPen model PRI 200, which determined the photosynthetic light use efficiency in these leaves. The Normalized Difference Vegetation Index (NDVI) that is an indicator of chlorophyll content in plants and directly related to their photosynthetic capacity was also evaluated using the same leaves, with a PlantPen model NDVI 300. These measures were non-destructive, they were obtained through an optical window in the instruments. At the end of the experiment, the fresh and dried biomass of both aerial parts and roots were determined in a digital analytical balance (Sartorius ENTRIS124-1S, 120 × 0.0001 g, Sartorius Stedim Biotech North America Inc., New York, United States). The fresh biomass was oven-dried at 60°C, until a constant weight was reached.
The germination and biomass data were analyzed by one-way ANOVA (substrata) and the remaining data by two-way ANOVA (Time ( Substrata). When the data were statistically significant (p < 0.05), all pairwise-comparisons were carried out by Tukey HSD post hoc test. A Correlation Matrix Principal Component Analysis (PCA using normalised normalized data) was performed and the Pearson correlation coefficient (r > 0.7). was used to correlate the nutrient concentrations of substrata in T0 and the morphometric and photosynthetic indexes in T3. The tests were made using the statistical software R studio version 1.1.423 for Windows.
The FLU had a silty clay loam texture, was slightly alkaline, with a high EC and low values of Corg, Ntotal, extractable P and K (Table 1, Figure 2A, and Supplementary Figure 1A). These data indicate that this soil is saline-sodic with a 25% of Naexchangeable and 41.48 of SAR. The multielemental composition of the Fluvisol showed that concentrations of the potentially hazardous elements were below the maximum allowed values for agricultural use (The Environmental Protection Agency of Portugal [APA], 2019), except for As concentration (15.6 mg/kg), which was slightly higher than the allowed concentration limit (11 mg/kg; The Environmental Protection Agency of Portugal [APA], 2019). This can be attributed to a widespread industrial waste such as wastes resulting from chemical and metallurgical plants located close to the river Tagus, deposited in the estuary in the past (De Bettencourt, 1988).
Table 1. Chemical characteristics of the Fluvisol and amendments (mean ± SD, n = 3) collected in Sobralinho, in the Municipal Council of Vila Franca de Xira, west Portugal.
Figure 2. Nutrients concentration in Fluvisol and in Technosol (means ± SD; n = 5) in the beginning (T0) (A) and at the end (T3) of assay (B). Different letters for the same element indicate significant differences between time and substrata (p < 0.001, two-way ANOVA (time x substrata) followed by Tukey HSD test).
The FLU had a high Naexchangeable percentage and SAR contributing to soil aggregate degradation and colloid dispersion, promoting clogging of soil pores and making plants root penetration difficult. This leads to a lack of drainage, water puddle formation and oxygen deficiency (Brady and Weil, 2008). Further, the ratio [Ca]/[Mg] < 1.5 indicates very unfavorable conditions for soil physical properties (INIA-LQARS, 2000). For the saline-sodic soil reclamation it is necessary to reduce the Naexchangeable percentage replacing the Na+ ion to the Ca2+ ion, in the exchange complex (Brady and Weil, 2008). While some studies favor gypsum use (Ca2SO4) to form Na2SO4 that can be leached (Mozheiko, 1969; Carter et al., 1977), other works support the application of organic compounds in addition to the gypsum to improve soil nutrition, aggregation, water-holding capacity, infiltration rate and decrease in EC (Hanay et al., 2004; Diacono and Montemurro, 2015). Tailored soils can be utilized as an alternative to improve salt-affected soil properties (Macía et al., 2014; Cortinhas et al., 2020). Moreover, the cultivation of species well adapted to the saline environments (halophytes) contribute to soil recovery (Manousaki and Kalogerakis, 2011; Devi et al., 2016; Liang et al., 2017). These plants can survive in saline environments due to protection mechanisms such as the Na+ and Cl– compartmentation, Ca2+ and K+ uptake, succulence, synthesis of particular compounds and the presence of salt glands (Flowers et al., 2010; Song and Wang, 2014; Caperta et al., 2020).
In this study, the TEC soil fertility was increased due to high content of Ntotal, Corg, extractable P and K present in sludge and waste kieselguhr (Table 1). The residual biomass applied as a source of C and other nutrients in the long run by slow mineralization also contributed to improve soil texture/aggregation. The addition of sand and limestone gravel enhanced the texture and permeability of the Fluvisol used in the TEC construction (Supplementary Figures 1B,D) as also shown previously (Macía et al., 2014; Cortinhas et al., 2020). No significant differences among TEC and FLU were found in terms of pH and CE. Nonetheless, the TEC presented a significant increase in the concentration of all determined nutrients, mainly in P (p = 2.16 × 10–5), Fe (p = 2 × 10–6) and Cu (p = 2 × 10–6), except for Mn (p = 1.07 × 10–7) (Figure 2A). The soil improvement in these nutrients is principally related to the wastewater sludge that was rich in P, Fe and Cu (Table 1). The mixture of amendments was suitable to improve soil fertility, texture, and structure (Supplementary Figure 1B) as well as soil permeability and drainage avoiding waterlogging. These latter two soil properties are extremely important since the remaining populations of L. daveaui in the Tagus estuary thrive in Fluvisols and saline sands, mainly inundated with salty water by the equinoctial tides (Caperta and Carapeto, 2020).
The brackish water was strongly saline, had a neutral pH and high concentrations of Cl– (207 mmol/L), HCO3– (4.37 mmol/L), Na+ (187 mmol/L), Ca2+ (5.24 mmol/L), and Mg2+ (22.14 mmol/L) (Table 2) as was to be expected in estuarine water (Santos et al., 2017; Cortinhas et al., 2020). The calculated SAR of water was high (35.4) but tolerated by halophyte species (Haro et al., 1993; Flowers and Colmer, 2008; Table 2). The maritime fog mostly observed from November to February each year in the Lisbon area (Belo-Pereira and Santos, 2016), is deposited as occult precipitation by condensing or sublimating directly onto plant surfaces (Karavoltsos et al., 2017), probably contributing to the water balance of plants in these saline habitats.
Table 2. Chemical characteristics of irrigation water (mean ± SD, n = 3) collected in Sobralinho, in the Municipal Council of Vila Franca de Xira, west Portugal.
After plant growth (T3), the soils irrigated with brackish water show no change in pH values, however the EC values of FLU (5.6 dS/m) and TEC (5.9 dS/m) had a significant (p < 1 × 10–7) increase (34.03; 32.48 dS/m, respectively) due to the high salinity of estuarine water. In T3 (Figure 2B), the organic C, Ntotal and extractable P concentrations did not show significant variations compared to T0 (Figure 2A). At the end of the assay (Figure 2B), the Zn content of TEC increased significantly as well as the extractable K in both soils (p < 5 × 10–6). In contrast, the Fe and Cu values decreased significantly (p < 9 × 10–4) from T0 (Figure 2A) to T3 (Figure 2B).
The findings of a significantly different (p = 5.95 × 10–8) higher seed germination percentage in distilled water and in FLU compared to TEC (Figure 3) can be due to the effect of high salinity (i.e, lowest osmotic potential) on Limonium seed germination (Boorman, 1967; Luque et al., 2013; Al Hassan et al., 2017) as well as of other halophyte species (Debez et al., 2018; Lombardi et al., 2019).
Figure 3. The percentage of Limonium daveaui seeds germination (mean ± SD; n = 144). The substrata (moist filter paper, Fluvisol and Technosol) with different letters are significantly different (p < 0.05, one-way ANOVA (substrata) followed by Tukey HSD test).
Due to FLU low permeability, a water film is retained on the surface of the substrate allowing the seeds to float (Supplementary Figure 1C), whereas at the surface of the relatively porous TEC this water film does not occur (Supplementary Figure 1). Although Fluvisols and rocky deposits seem to be suitable for halophyte seed germination, for endemic Limonium species seed germination by directly sowing them in the field did not prove to be successful (Caperta and Vasco Silva, unpublished research).
Transplanting and even translocating plants to start new populations of rare and endemic species have shown better results than using seeds in situ (Davy, 2002; Godefroid et al., 2011; Albrecht and Maschinski, 2012). A comparison of plants grown in FLU and in TEC irrigated with estuarine water (Figure 4) revealed that there were no significant differences for the growth parameters measured in plants growing in both soils. However, there was a significant increase in the leaf number in T2 (p ≤ 6.51 × 10–4) followed by a small decrease in T3 (Figure 4). Plants grown in TEC presented smaller leaves than plants cultivated in FLU. Nonetheless, the former had a higher number of leaves, which can contribute to a higher photosynthetic rate (Figure 4). Between T2 and T3, the plants started to bloom, which may have influenced a reduction in leaf number. The plants cultivated in TEC had a higher vegetative and reproductive growth and produced flowering stems that emerged earlier than in FLU. Although only one individual per treatment presented a flowering stem, the plant grown in TEC produced more and larger scapes than that in FLU (Figure 4). The TEC substrate, rich in essential nutrients and with better texture and structure for plants allowed better plant development. These findings suggest that plant cultivation in Technosols contributes to the reproductive success of L. daveaui as also found previously in Limonium algarvense (Cortinhas et al., 2020).
Figure 4. Plant growth parameters assessed during the microcosm assay [mean ± SD (except scape total number); n = 15]. T0, transplantation, in winter; T1, Spring; T2, Summer; and T3, Autumn. Different letters meaning significant differences (p < 0.0 5, two-way ANOVA (time × substrata) followed by Tukey HSD test).
In terms of the physiological status there were no significant differences in NDVI and PRI between plants raised in different substrata (Figure 5). However, the plants cultivated in FLU presented lower values in all the reference periods than individuals grown in TEC, supporting that this soil was a more stressful environment for plants than TEC (Figure 5). The NDVI and PRI values of plants raised in both substrata dropped significantly (p < 2.4 × 10–6) after the spring (T1). While the PRI value only reduced significantly from T1 to T2 (p = 5 × 10–7), the NDVI value decreased significantly between T1-T2 (p < 1 × 10–7) and T2-T3 (p < 1 × 10–7). Stress factors such as leaves senescence, salinity, light excess, or high temperature or in combination can lead to PRI and NDVI reduction (Bieto and Talón, 2008; Yudina et al., 2020). In this study, reduction of these indexes at least could be associated to leaves senescence that occurred when plants start flowering.
Figure 5. The NDVI (A) and PRI (B) indexes evaluated during the microcosm assay in different reference periods (mean ± SD; n = 15). T0, transplantation, in winter; T1, Spring; T2, Summer; and T3, Autumn. Different letters meaning significant differences (p < 0.05, two-way ANOVA (time × substrata) followed by Tukey HSD test.
The dry mass of roots, aerial part and flowers represented ∼40% of their weight. The aerial (leaves and scapes) and roots biomass did not show significant differences among substrata (Figure 6B). However, plants from TEC showed high flowers production than FLU (Figure 6B). The roots of plants cultivated in FLU were concentrated in the bottom of pots (Figure 6A), while in plants grown in TEC roots spread uniformly all over the substrate (Figure 6A). The deficient FLU structure, due to colloid dispersion, is an obstacle to roots penetration and oxygen circulation. The poor structure and the compaction due to high Naexchangeable percentage and SAR can lead to plant death as found previously in Cortinhas et al. (2020).
Figure 6. Biomass production at the end of the microcosm assay using brackish water irrigation (A) Fresh biomass produced. (A1) Plant roots cultivated in Fluvisol concentrated at bottom of the pot. (A2) Plant roots (white arrows) grown in Technosol spreading uniformly over the soil. (A3) Plant grown in Fluvisol exhibiting one scape. (A4) Plant cultivated in Technosol showing two scapes. (B) Dry biomass of leaves, roots, and flowers [mean ± SD (except dry flowers total); n = 5]. The aerial part represents the leaves and scapes; *, only one individual produced scapes. No significant differences were found between substrata (p > 0.05, one-way ANOVA followed by Tukey HSD test).
The PCA correlated nutrient concentrations determined in substrata in the beginning of the assay (T0) with the morphometric parameters and the photosynthetic indexes measured in plants at the end of the assay (T3). The first two components explain 71.14% of data variation. The third principal component only corresponded to 12.78% of variation and did not show correlation with any of the evaluated variables (r < 0.60); as such, it was not represented in this analysis.
The first principal component represented 53.12% of data variation with the pH and nutrients being the variables having the highest correlation (r > 0.70) with that component (Figure 7 and Supplementary Table 1). This contributes to clearly separate the individuals cultivated in TEC from plants cultivated in FLU (Figure 7). The PCA revealed that TEC had higher nutrient concentrations (except for Mn) but lower pH value than FLU. All the nutrients showed correlation (r > 0.70) among them (Supplementary Table 2) and the pH was negatively correlated with C, Fe, and Cu (−0.77 < r < −0.70), but positively with Mn (r = 0.78).
Figure 7. The first two axes of Principal Component Analysis. This analysis was based on pH, CE, organic carbon (C), nutrients concentration (N total (N), extractable P (P), extractable K (K), Fe, Mn, Zn, Cu) determined in the soils in the beginning of the assay (T0) and the morphometric parameters (leaf number, leaf length, leaf width, scape number, scape length) and photosynthetic indexes [normalized difference vegetation index (NDVI) and photochemical reflectance index (PRI)] measured in plants at the end of the assay (T3). Percentages of total variance explained by the functions are given in parenthesis.
In the second principal component, which represented 18.02% of data variability (Figure 7), leaf and scape number were the parameters that mostly contributed to the observed variability (r ≥ 0.70; Supplementary Table 1). All the growth parameters and the values of the photosynthetic indexes were also higher in the plants cultivated in the TEC than plants grown in the FLU. The NDVI was strongly correlated (0.95 < r < 0.96) with leaf size (Supplementary Table 2).
This work contributes to the knowledge of L. daveaui microenvironmental requirements particularly soil properties. The findings demonstrated that eco-friendly soil technologies have an enormous potential to improve the reintroduction success of this species. As such, plants will be pre-grown in the Technosol and then will be planted on the sites in modified soil. The amendments that have shown suitable to improve the physical and chemical proprieties of the saline-sodic Fluvisol will be added and mixed with this soil. The plants will be subjected to irrigation with brackish water collected directly from a channel located in the Tagus estuary. From a circular economy perspective, this approach that uses cost-effective subproduct/wastes contributes to enhance soil fertility and structure, valuing underused resources such as saline soils, not used in conventional reintroduction schemes, but appropriate for halophyte species.
The original contributions presented in the study are included in the article/Supplementary Materials, further inquiries can be directed to the corresponding author/s.
MA and ACa designed and coordinated the study. ACo, TF, MA, and ACa performed the experimental assay. ACo and TF processed the raw data and realized the statistical analyses. ACo, MA, and ACa drafted the manuscript. All authors read and approved the manuscript.
The authors declare that the research was conducted in the absence of any commercial or financial relationships that could be construed as a potential conflict of interest.
This research was funded by Fundação para a Ciência e a Tecnologia (FCT, www.fct.pt/) by project PTDC/AGRPRO/4285/2014 and grant SFRH/BD/130256/2017 attributed to ACo. Publication costs were funded by FCT through project UID/AGR/04129/2020 (LEAF). The Open Access was funded by LEAF Project UID/AGR/04129/2020.
We want to thank Arqta Vitória Cochicho (Municipal Council Vila Franca de Xira, Lisbon, Portugal; https://www.cm-vfxira.pt/) for providing permission for Fluvisol and estuarine water collection, and to Dra. Sandra Borges (Sociedade Central de Cervejas e Bebidas, S.A., http://www.centralcervejas.pt/) for providing the sludge and waste kieselguhr.
The Supplementary Material for this article can be found online at: https://www.frontiersin.org/articles/10.3389/fevo.2021.604509/full#supplementary-material
Supplementary Figure 1 | Physical appearance of Fluvisol (A) and Technosol (B). The Fluvisol was collected in Sobralinho salt marsh area in the Municipal Council of Vila Franca de Xira, west Portugal. The Technosol was produced with Fluvisol and organic/inorganic wastes’ as amendments. Seed germination (white arrows) on a water film retained in the Fluvisol surface (C) and in the Technosol surface, where the water film was not present (D).
Supplementary Table 1 | Correlation matrix between the first two principal components (PC1 and PC2) on the normalized data and the original variables. This matrix includes the pH, CE, organic carbon (C) and nutrient concentration (N total (N), extractable P (P), extractable K (K), Fe, Mn, Zn, Cu) values determined in the substrata in the beginning of the assay (T0) and morphometric parameters (leaf number, leaf length, leaf width, scape number, scape length) and photosynthetic indexes (Normalised Difference Vegetation Index (NDVI) and Photochemical Reflectance Index (PRI)) obtained in plants at the end of the assay (T3).
Supplementary Table 2 | Correlation matrix between variables. This matrix includes the pH, CE, organic carbon (C) and nutrient concentration (N total (N), extractable P (P), extractable K (K), Fe, Mn, Zn, Cu) values determined in the substrata in the beginning of the assay (T0) and morphometric parameters (leaf number, leaf length, leaf width, scape number, scape length) and photosynthetic indexes (Normalised Difference Vegetation Index (NDVI) and Photochemical Reflectance Index (PRI)) obtained in plants at the end of the assay (T3).
Activation Laboratories (2020). Metals in Soil. Available at: https://actlabs.com/environmental/services/metals-in-soil/(accessed June 1, 2020).
Al Hassan, M., Estrelles, E., Soriano, P., López-Gresa, M. P., Bellés, J. M., Boscaiu, M., et al. (2017). Unraveling salt tolerance mechanisms in halophytes: a comparative study on four Mediterranean Limonium species with different geographic distribution patterns. Front. Plant Sci. 8:1438. doi: 10.3389/fpls.2017.01438
Albrecht, M. A., and Maschinski, J. (2012). “Influence of founder population size, propagule stages, and life history on the survival of reintroduced plant populations,” in Plant Reintroduction in a Changing Climate, eds J. Maschinski and K. E. Haskins (Washington, DC: Island Press), 171–188. doi: 10.5822/978-1-61091-183-2_10
Asensi, A., Diez Garretas, B., and van der Maarel, E. (1993). “Dry coastal ecosystems of Portugal,” in Ecosystems of the World, 2A: Dry Coastal Ecosystems: Polar Regions and Europe, ed. E. van der Maarel (Amsterdam: Elsevier), 342–347.
Ayers, R. S., and Westcot, D. W. (1985). Water Quality for Agriculture. Rome: Food and Agriculture Organization of the United Nations. FAO Irrígation and Draínage Paper, 29 Rev. l.
Balke, T., Bouma, T. J., Horstman, E. M., Webb, E. L., Erftemeijer, P. L., and Herman, P. M. (2011). Windows of opportunity: thresholds to mangrove seedling establishment on tidal flats. Mar. Ecol. Prog. Ser. 440, 1–9. doi: 10.3354/meps09364
Baumberger, T., Affre, L., Croze, T., and Mesléard, F. (2012). Habitat requirements and population structure of the rare endangered Limonium girardianum in Mediterranean salt marshes. Flora 207, 283–293. doi: 10.1016/j.flora.2011.11.008
Belo-Pereira, M., and Santos, J. A. (2016). A persistent wintertime fog episode at Lisbon airport (Portugal): performance of ECMWF and AROME models. Meteorol. Appl. 23, 353–370. doi: 10.1002/met.1560
Bieto, J. A., and Talón, M. (2008). Fundamentos de Fisiología Vegetal. Madrid: McGraw-Hill Interamericana.
Boorman, L. A. (1967). Limonium vulgare Miller and L. humile Miller. J. Ecol. 55, 221–232. doi: 10.2307/2257727
Brady, N. C., and Weil, R. R. (2008). The Nature and Properties of Soils. New Jersey: Pearson Prentice Hall.
Braun-Blanquet, J., Rozeira, A., and Pinto da Silva, A. R. (1972). Résultats de deux excursions géobotaniques à travers le Portugal septentrional et moyen. IV. Esquisse sur la végétation dunale. Agron. Lusit. 33, 217–234.
Breure, A. M., Lijzen, J. P. A., and Maring, L. (2018). Soil and land management in a circular economy. Sci. Total Environ. 624, 1125–1130. doi: 10.1016/j.scitotenv.2017.12.137
Cao, H., Zhu, Z., Balke, T., Zhang, L., and Bouma, T. J. (2018). Effects of sediment disturbance regimes on Spartina seedling establishment: implications for salt marsh creation and restoration. Limnol. Oceanogr. 63, 647–659. doi: 10.1002/lno.10657
Caperta, A., and Carapeto, A. (2020). “Limonium daveaui: ficha de avaliação do risco de extinção,” in Lista Vermelha da Flora Vascular de Portugal Continental, (Lisboa: Sociedade Portuguesa de Botânica Associação Portuguesa de Ciência da Vegetação).
Caperta, A. D., Espírito-Santo, M. D., Silva, V., Ferreira, A., Paes, A. P., Róis, A. S., et al. (2014). Habitat specificity of a threatened and endemic, cliff-dwelling halophyte. AoB Plants 6:lu032. doi: 10.1093/aobpla/plu032
Caperta, A. D., Róis, A. S., Teixeira, G., Garcia-Caparros, P., and Flowers, T. J. (2020). Secretory structures in plants: lessons from the Plumbaginaceae on their origin, evolution and roles in stress tolerance. Plant. Cell. Environ. 43, 2912–2931. doi: 10.1111/pce.13825
Carter, M. R., Webster, G., and Cairns, R. (1977). Amelioration of a brown solodized solonetz soil by a surface application of gypsum plus ammonium nitrate. Can. J. Soil. Sci. 57, 139–145. doi: 10.4141/cjss77-018
Cesário, R., Monteiro, C. E., Nogueira, M., O’Driscoll, N. J., Caetano, M., Hintelmann, H., et al. (2016). Mercury and methylmercury dynamics in sediments on a protected area of Tagus Estuary (Portugal). Water Air Soil Pollut. 227:475. doi: 10.1007/s11270-016-3179-2
Cortinhas, A., Caperta, A. D., Teixeira, G., Carvalho, L., and Abreu, M. M. (2020). Harnessing sediments of coastal aquaculture ponds through technosols construction for halophyte cultivation using saline water irrigation. J. Environ. Manag. 261:109907. doi: 10.1016/j.jenvman.2019.109907
Costa, J. (2013). Urbanismo e Adaptação às Alterações Climáticas: As Frentes de Água. Lisboa: Livros Horizonte.
Costa, J. C., Monteiro-Henriques, T., Neto, C., Arsénio, P., and Aguiar, C. (2007). The application of the habitats directive in Portugal. Fitosociologia 44, 23–28.
Costa, J. C., Neto, C., Aguiar, C., Capelo, J., Espírito-Santo, M. D., Honrado, J., et al. (2012). Vascular plant communities in Portugal (Continental, the Azores and Madeira). Glob. Geobot. 2, 1–180.
Davy, A. (2002). “Establishment and manipulation of plant populations and communities in terrestrial systems,” in Handbook of Ecological Restoration. Principles of Restoration, eds M. R. Perrow and A. J. Davy (Cambridge: Cambridge University Press), 223–241.
De Bettencourt, A. M. M. (1988). On arsenic speciation in the Tagus estuary. Neth. J. Sea Res. 22, 205–212. doi: 10.1016/0077-7579(88)90024-5
Debez, A., Belghith, I., Pich, A., Taamalli, W., Abdelly, C., and Braun, H. P. (2018). High salinity impacts germination of the halophyte Cakile maritima but primes seeds for rapid germination upon stress release. Physiol. Plant. 164, 134–144. doi: 10.1111/ppl.12679
Devi, S., Nandwal, A. S., Angrish, R., Arya, S. S., Kumar, N., and Sharma, S. K. (2016). Phytoremediation potential of some halophytic species for soil salinity. Int. J. Phytoremediation 18, 693–696. doi: 10.1080/15226514.2015.1131229
Diacono, M., and Montemurro, F. (2015). Effectiveness of organic wastes as fertilizers and amendments in salt-affected soils. Agriculture 5, 221–230. doi: 10.3390/agriculture5020221
Duarte, B., Silva, G., Costa, J. L., Medeiros, J. P., Azeda, C., Sá, E., et al. (2014). Heavy metal distribution and partitioning in the vicinity of the discharge areas of Lisbon drainage basins (Tagus Estuary, Portugal). J. Sea Res. 93, 101–111. doi: 10.1016/j.seares.2014.01.003
Duarte, I., Rego, F. C., Casquilho, J. P., and Arsénio, P. (2016). A relevance index for the habitat areas of Natura 2000 network based on their rarity and representativeness. Ecol. Indic. 61, 202–213. doi: 10.1016/j.ecolind.2015.09.015
Dunwiddie, P. W., and Martin, R. A. (2016). Microsites matter: improving the success of rare species reintroductions. PLoS One 11:e0150417. doi: 10.1371/journal.pone.0150417
Erben, M. (1978). Die Gattung Limonium im südwestmediterranen Raum. Mitt. Bot. Staatssamml. München. 14, 361–626.
Erben, M. (1993). “Limonium Mill,” in Flora Iberica, eds S. Castroviejo, C. Aedo, S. Cirujano, M. Lainz, P. Montserrat, R. Morales, et al. (Madrid: Real Jardín Botánico CSIC), 2–143.
Espírito-Santo, M. D., Alves, H. N., Caperta, A. D., and Moreira, I. (2012). “Plantas endémicas do litoral de Portugal Continental in Monteiro,” in Gestão e Conservação da Flora e da Vegetação de Portugal e da África Lusófona, eds A. Gomes da Silva and R. Jorge (Lisboa: ISA Press), 267–302.
Fernandes, A., Figueira de Sousa, J., Costa, J. P., and Neves, B. (2020). Mapping stakeholder perception on the challenges of brownfield sites’ redevelopment in waterfronts: the Tagus Estuary. Eur. Plan. Stud. 28, 2247–2464. doi: 10.1080/09654313.2020.1722985
Figueres, G., Martin, J. M., Meybeck, M., and Seyler, P. (1985). A comparative study of mercury contamination in the Tagus Estuary (Portugal) and major French Estuaries (Gironde, Loire, Rhône). Estuar. Coast. Shelf Sci. 20, 183–203. doi: 10.1016/0272-7714(85)90037-X
Flowers, T. J., and Colmer, T. D. (2008). Salinity tolerance in halophytes. New Phytol. 179, 945–963. doi: 10.1111/j.1469-8137.2008.02531.x
Flowers, T. J., Galal, H. K., and Bromham, L. (2010). Evolution of halophytes: multiple origins of salt tolerance in land plants. Funct. Plant Biol. 37, 604–612. doi: 10.1071/FP09269
Franco, J. D. A. (1984). Nova Flora de Portugal (Continente e Açores) Vol II Clethraceae – Compositae. Lisboa: Author’s Edition.
Godefroid, S., Piazza, C., Rossi, G., Buord, S., Stevens, A. D., Aguraiuja, R., et al. (2011). How successful are plant species reintroductions? Biol. Conserv. 144, 672–682. doi: 10.1016/j.biocon.2010.10.003
Guerreiro, M., Fortunato, A. B., Freire, P., Rilo, A., Taborda, R., Freitas, M. C., et al. (2015). Evolution of the hydrodynamics of the Tagus estuary (Portugal) in the 21st century. J. Integr. Coast. Zone Manag. 15, 65–80. doi: 10.5894/rgci515
Hanay, A., Büyüksönmez, F., Kiziloglu, F. M., and Canbolat, M. Y. (2004). Reclamation of saline-sodic soils with gypsum and MSW compost. Compost Sci. Util. 12, 175–179. doi: 10.1080/1065657X.2004.10702177
Haro, R., Bañuelos, M. A., Quintero, F. J., Rubio, F., and Rodríguez-Navarro, A. (1993). Genetic basis of sodium exclusion and sodium tolerance in yeast. A model for plants. Physiol Plant 89, 868–874. doi: 10.1111/j.1399-3054.1993.tb05298.x
Haskins, K. E., and Pence, V. (2012). Transitioning Plants to New Environments: Beneficial Applications of Soil Microbes in Plant Reintroduction in a Changing Climate. Washington, DC: Island Press.
Holl, K. D., and Hayes, G. F. (2006). Challenges to introducing and managing disturbance regimes for Holocarpha macradenia, an endangered annual grassland forb. Biol. Conserv. 20, 1121–1131. doi: 10.1111/j.1523-1739.2006.00416.x
INIA-LQARS (2000). Manual de Fertilização das Culturas. Lisboa: Laboratório Químico Agrícola Rebelo da Silva.
Instituto da Conservação da Natureza e das Florestas [ICNF]. (2012). Reserva Natural do Estuário do Tejo. Available at: http://www2.icnf.pt/portal/ap/r-nat/rnet (accessed May 25, 2020).
IUSS Working Group (2015). World Reference Base for Soil Resources 2014 (update 2015) International Soil Classification System for Naming Soils and Creating Legends for Soil Maps. Rome: FAO. World Soil Resources Report No. 106.
Karavoltsos, S., Fotiadis, F., Michopoulos, P., Sakellari, A., Plavšić, M., Bourletsikas, A., et al. (2017). Organic complexation of copper in throughfall and open field bulk deposition: influence of the tree canopy of Mediterranean forest ecosystems. Chemosphere 167, 28–35. doi: 10.1016/j.chemosphere.2016.09.131
Lakanen, E., and Erviö, R. (1971). A comparison of eight extractants for the determination of plant available micronutrients in soils. Acta Agr. Fenn. 123, 223–232.
Lechuga-Lago, Y., Novoa, A., Le Roux, J. J., and González, L. (2017). Understanding the influence of urbanization on invasibility: Carpobrotus edulis as an exemplar. Biol. Invasions. 19, 3601–3611. doi: 10.1007/s10530-017-1593-z
Lesch, S. M., and Suarez, D. L. (2009). A short note on calculating the adjusted SAR Index. Am. Soc. Agric. Biol. Eng. 52, 493–496. doi: 10.13031/2013.26842
Liang, L., Liu, W., Sun, Y., Huo, X., Li, S., and Zhou, Q. (2017). Phytoremediation of heavy metal contaminated saline soils using halophytes: current progress and future perspectives. Environ. Rev. 25, 269–281. doi: 10.1139/er-2016-0063
Lombardi, T., Bedini, S., and Bertacchi, A. (2019). Germination ecology of the aromatic halophyte Artemisia caerulescens L.: influence of abiotic factors and seed after-ripening time. Folia Geobot. 54, 115–124. doi: 10.1007/s12224-019-09345-4
Luque, E. G., Fernández, I. C. D., and Mercado, F. G. (2013). Effect of salinity and temperature on seed germination in Limonium cossonianum. Botany 91, 12–16. doi: 10.1139/cjb-2012-0157
Macía, P., Fernández-Costas, C., Rodríguez, E., Sieiro, P., Pazos, M., and Sanromán, M. A. (2014). Technosols as a novel valorisation strategy for an ecological management of dredged marine sediments. Ecol. Eng. 67, 182–189. doi: 10.1016/j.ecoleng.2014.03.020
Manousaki, E., and Kalogerakis, N. (2011). Halophytes present new opportunities in phytoremediation of heavy metals and saline soils. Ind. Eng. Chem. Res. 50, 656–660. doi: 10.1021/ie100270x
Martins, M. C., Neto, C. S., and Costa, J. C. (2013). The meaning of mainland Portugal beaches and dunes’ psammophilic plant communities: a contribution to tourism management and nature conservation. J Coast Conserv. 17, 279–299. doi: 10.1007/s11852-013-0232-9
Maschinski, J., and Albrecht, M. A. (2017). Center for plant conservation’s best practice guidelines for the reintroduction of rare plants. Plant Divers. 39, 390–395. doi: 10.1016/j.pld.2017.09.006
Maunder, M. (1992). Plant reintroductions: an overview. Biodivers. Conserv. 1, 51–61. doi: 10.1007/BF00700250
Menges, E. S., Smith, S. A., and Weekley, C. W. (2016). Adaptive introductions: how multiple experiments and comparisons to wild populations provide insights into requirements for long-term introduction success of an endangered shrub. Plant Divers. 38, 238–246. doi: 10.1016/j.pld.2016.09.004
Mozheiko, A. M. (1969). Chemical Reclamation o Sodic Solonetzes in the Southern Part of the Midddle Dnieper Region by the Application of Gypsum and Calcium Chloride. Agrokémia és talajtan 18, 310–314.
Olsson, L., Barbosa, H., Bhadwal, S., Cowie, A., Delusca, K., Flores-Renteria, D., et al. (2019). ““Land Degradation,” in Climate Change and Land: An IPCC Special Report on Climate Change, Desertification, Land Degradation, Sustainable Land Management, Food Security, and Greenhouse gas Fluxes in Terrestrial Ecosystems, eds P. R. Shukla, J. Skea, E. Calvo Buendia, V. Masson-Delmotte, H.-O. Pörtner, D. C. Roberts, et al. (Geneva: IPCC).
Pignatti, S. (1971). “Studi sui Limonium VIII,” in Notulae Systematicae ad Floram Europaeam Spectantes, Vol. 64, ed. V. H. Flora Europaea (Heywood: Botanical Journal of the Linnean Society), 353–381.
Póvoas, I., and Barral, M. F. (1992). Métodos de Análise de Solos. Comunicações do Instituto de Investigação Científica Tropical. Lisbon: Instituto de Investigação Científica Tropical. Série de Ciências Agrárias N. °10.
Róis, A. S., Teixeira, G., Sharbel, T. F., Fuchs, J., Martins, S., Espírito-Santo, D., et al. (2012). Male fertility versus sterility, cytotype, and DNA quantitative variation in seed production in diploid and tetraploid sea lavenders (Limonium sp., Plumbaginaceae) reveal diversity in reproduction modes. Plant Reprod. 25, 305–318. doi: 10.1007/s00497-012-0199-y
Santos, E. S., Abreu, M. M., Peres, S., Magalhães, M. C. F., Leitão, S., Pereira, A. S., et al. (2017). Potential of Tamarix africana and other halophyte species for phytostabilisation of contaminated salt marsh soils. J. Soils Sediments 17, 1459–1473. doi: 10.1007/s11368-015-1333-x
Song, J., and Wang, B. (2014). Using euhalophytes to understand salt tolerance and to develop saline agriculture: Suaeda salsa as a promising model. Ann. Bot. 115, 541–553. doi: 10.1093/aob/mcu194
Spencer, K. L., Carr, S. J., Diggens, L. M., Tempest, J. A., Morris, M. A., and Harvey, G. L. (2017). The impact of pre-restoration land-use and disturbance on sediment structure, hydrology and the sediment geochemical environment in restored saltmarshes. Sci. Total Environ. 587, 47–58. doi: 10.1016/j.scitotenv.2016.11.032
The Environmental Protection Agency of Portugal [APA] (2019). Solos Contaminados – Valores de Referência Para o Solo. Amadora: The Ministry of Agriculture, the Sea.
The Environmental Protection Agency of Portugal [APA] (2011). Guide for the Assessment of Imminent Threats and Environmental Damages. Lisboa: The Ministry of Agriculture, the Sea.
van der Maarel, E., and van der Maarel-Versluys, M. (1996). Distribution and conservation status of littoral vascular plant species along the European coasts. J. Coast Conserv. 2, 73–92. doi: 10.1007/bf02743039
van Regteren, M., Meesters, E. H., Baptist, M. J., De Groot, A. V., Bouma, T. J., and Elschot, K. (2020). Multiple environmental variables affect germination and mortality of an annual salt marsh pioneer: Salicornia procumbens. Estuar. Coast. 43, 1489–1501.
Wang, N., Jiao, J. Y., Lei, D., Chen, Y., and Wang, D. L. (2014). Effect of rainfall erosion: seedling damage and establishment problems. Land Degrad. Dev. 25, 565–572. doi: 10.1002/ldr.2183
Yudina, L., Sukhova, E., Gromova, E., Nerush, V., Vodeneev, V., and Sukhov, V. (2020). A light-induced decrease in the photochemical reflectance index (PRI) can be used to estimate the energy-dependent component of non-photochemical quenching under heat stress and soil drought in pea, wheat, and pumpkin. Photosynth. Res. 146, 175–187.
Keywords: estuarine water, Limonium daveaui, fluvisol, tailored soils, plant restoration, soil technology, wastes
Citation: Cortinhas A, Ferreira TC, Abreu MM and Caperta AD (2021) Conservation of a Critically Endangered Endemic Halophyte of West Portugal: A Microcosm Assay to Assess the Potential of Soil Technology for Species Reintroduction. Front. Ecol. Evol. 9:604509. doi: 10.3389/fevo.2021.604509
Received: 09 September 2020; Accepted: 13 April 2021;
Published: 04 May 2021.
Edited by:
Terry D. Macfarlane, Conservation and Attractions (DBCA), AustraliaReviewed by:
Pavol Elias, Slovak University of Agriculture, SlovakiaCopyright © 2021 Cortinhas, Ferreira, Abreu and Caperta. This is an open-access article distributed under the terms of the Creative Commons Attribution License (CC BY). The use, distribution or reproduction in other forums is permitted, provided the original author(s) and the copyright owner(s) are credited and that the original publication in this journal is cited, in accordance with accepted academic practice. No use, distribution or reproduction is permitted which does not comply with these terms.
*Correspondence: Ana D. Caperta, YW5hZGVsYXVuYXlAaXNhLnVsaXNib2EucHQ=
Disclaimer: All claims expressed in this article are solely those of the authors and do not necessarily represent those of their affiliated organizations, or those of the publisher, the editors and the reviewers. Any product that may be evaluated in this article or claim that may be made by its manufacturer is not guaranteed or endorsed by the publisher.
Research integrity at Frontiers
Learn more about the work of our research integrity team to safeguard the quality of each article we publish.