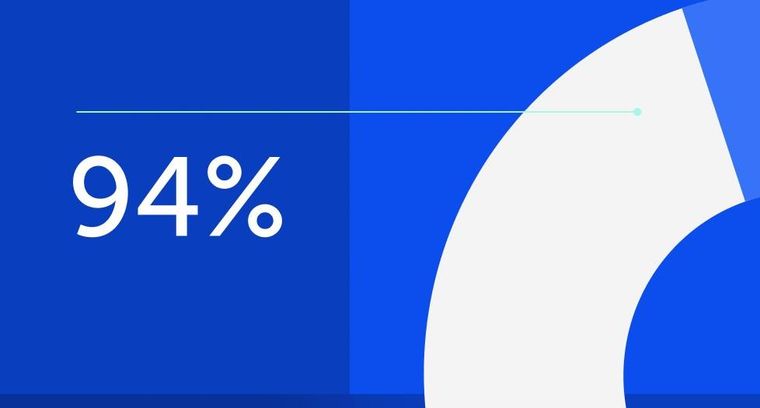
94% of researchers rate our articles as excellent or good
Learn more about the work of our research integrity team to safeguard the quality of each article we publish.
Find out more
PERSPECTIVE article
Front. Ecol. Evol., 08 April 2021
Sec. Urban Ecology
Volume 9 - 2021 | https://doi.org/10.3389/fevo.2021.603757
This article is part of the Research TopicHistorical Legacies of Land Use in Cities; Parks, Open Spaces and Potential for Green Infrastructure- Ideas of City Nature in an Urbanizing PlanetView all 10 articles
Many of the world’s major cities have implemented tree planting programs based on assumed environmental and social benefits of urban forests. Recent studies have increasingly tested these assumptions and provide empirical evidence for the contributions of tree planting programs, as well as their feasibility and limits, for solving or mitigating urban environmental and social issues. We propose that current evidence supports local cooling, stormwater absorption, and health benefits of urban trees for local residents. However, the potential for urban trees to appreciably mitigate greenhouse gas emissions and air pollution over a wide array of sites and environmental conditions is limited. Consequently, urban trees appear to be more promising for climate and pollution adaptation strategies than mitigation strategies. In large part, this is due to space constraints limiting the extent of urban tree canopies relative to the current magnitude of emissions. The most promising environmental and health impacts of urban trees are those that can be realized with well-stewarded tree planting and localized design interventions at site to municipal scales. Tree planting at these scales has documented benefits on local climate and health, which can be maximized through targeted site design followed by monitoring, adaptive management, and studies of long-term eco-evolutionary dynamics.
Urban trees in parks, yards, streets, and remnant parcels have been features of urban design and landscape architecture for centuries (Arnold, 1980), and are still integral components of civic spaces that are well-recognized for their public value. Urban trees are purported to have a number of environmental benefits, such as pollution absorption (Nowak et al., 2006, 2018), stormwater mitigation (Bartens et al., 2009), atmospheric cooling (Shashua-Bar and Hoffman, 2000), reduced energy use (Akbari et al., 1997; Akbari, 2002; Donovan and Butry, 2009; Hsieh et al., 2018), and habitat provision (Burghardt et al., 2009). In addition, studies have indicated that proximity to urban vegetation may increase property values (Sander et al., 2010; Li and Saphores, 2012; Escobedo et al., 2015), facilitate recuperation after stress and illness (Ulrich, 1983; Ulrich et al., 1991; Li and Sullivan, 2016), and reduce mental fatigue (Houlden et al., 2018). There is a substantial literature indicating that trees provide benefits for municipalities and their residents, and this perception, in part, has motivated local, regional and global initiatives that promote the planting of urban trees (McDonald et al., 2016).
However, there is also an increasing empirical understanding of the limits of tree planting as a nature-based solution to climate change and pollution. Urban forest dynamics, species composition, soil dynamics, and the costs of planting and managing designed spaces are important variables in urban forest outcomes, and must inform urban planting practices for successful planning and management (Oldfield et al., 2013). In addition, recent empirical studies highlight that spatial and temporal scales heavily influence the extent of environmental and social impacts of urban trees. Some of the purported benefits of urban forests require that trees are planted on large spatial scales beyond municipal boundaries, and maintained over the long term, to ensure effectiveness (Salmond et al., 2016). However, trees may provide other ecosystem services even when planting is constrained by the relatively limited spaces in dense cities. In this perspective, we refer to mitigation as strategies that aim to reduce climate change and pollution, and adaptation as strategies that aim to modify cities to help residents cope with climate change and pollution (Laukkonen et al., 2009). Many environmental benefits attributed to urban trees fall into one of these two categories. In addition, we discuss the evidence pertaining to both positive and negative impacts of trees on human health in the context of the extent of urban tree planting.
Early studies of urban tree ecosystem services emphasized rates of carbon (C) sequestration and air pollution reduction (Nowak and Crane, 2000; McPherson et al., 2005; Ray, 2005). Tools such as iTree1 translated the relatively scant data on urban tree processes available at the time—primarily estimates of biomass and dry deposition rates—into easily understood, municipal-scale metrics, such as tons of pollution absorbed (Tallis et al., 2011), energy savings (McPherson, 1993), C sequestered (Nowak, 1993), and total monetary value (McPherson, 1992; Nowak et al., 2002). However, since these tools were originally developed, additional empirical studies of the influence of trees on pollution concentrations have reported negligible or inconsistent impacts (Setälä et al., 2013; Han et al., 2020), or even increases in the residence time of particulate and NO2 concentrations in the atmosphere in the presence of tree canopies (Tong et al., 2015; Viippola et al., 2018). When atmospheric mixing is low, pollutants may be concentrated under tree canopies (Salmond et al., 2013), and when atmospheric mixing is high, studies have shown no discernable effect of the presence of trees on urban pollutant concentrations (Figure 1). In addition, trees may exacerbate rates of asthma due to the release of allergens and this is seldom accounted for in assessments of the impacts on trees on public health (Lovasi et al., 2013). In recent comprehensive reviews, Eisenman et al. (2019) and Xing and Brimblecombe (2020) both concluded that as a result of the many influences of trees on atmospheric composition besides dry deposition rates, current empirical evidence does not support the assumption that trees significantly and consistently reduce pollution concentrations.
Figure 1. In Lahti, Finland, researchers found that atmospheric particle deposition (the mass of particles deposited on the sampler) was significantly lower at urban sites with trees than in open areas. However, this difference did not result in significant differences in atmospheric PM 2.5 or PM 10 concentrations (particulate matter <2.5 and <10 μm in diameter) at any of their measurement sites, as shown above. This distinction is important because while many studies have inferred that trees will improve human health based on the influence of trees on particle deposition, it is atmospheric concentrations that actually impact human health directly. Error bars show SD; data from Setälä et al. (2013).
Similarly, for C sequestration, tree inventories coupled with allometric equations have been commonly used to estimate CO2 stored in urban trees in units of mass (Nowak and Crane, 2002; Nowak et al., 2013). However, to assess the potential of trees to enable climate mitigation, a systems-level approach is needed to compare C sequestration in urban forests to local fossil fuel emissions (Hutyra et al., 2014). Most studies of urban forest C sequestration attempt to estimate either tree biomass (McHale et al., 2007; Hutyra et al., 2011; Strohbach and Haase, 2012; Timilsina et al., 2014) or the rate of change in biomass over time (Net Primary Production, NPP) (Bialecki et al., 2018; Sonti et al., 2019; Trlica et al., 2020). Soil C dynamics should also be assessed as part of urban C balance, as soil C may either contribute to sequestration if organic matter is accumulating, or release C to the atmosphere if heterotrophic respiration reduces organic matter concentrations (Pouyat et al., 2002, 2006; Decina et al., 2016). But in either case, the spatial extent of urban trees and soils is quite limited relative to the magnitude of fossil fuel emissions. Cities are highly heterotrophic and expend orders of magnitude more C than they fix in photosynthesis (Collins et al., 2000). In most modern cities, fossil fuel combustion exceeds NPP per unit land area by at least an order of magnitude (Pataki et al., 2011). Hence, urban tree growth typically offsets municipal C emissions by only 0–3% annually (Pataki et al., 2009; Escobedo et al., 2010; Liu and Li, 2012; Baró et al., 2015; Velasco et al., 2016; Lindén et al., 2020), even before accounting for the energy needed to produce, transport, irrigate, prune, and fertilize urban trees (Roy et al., 2012).
This is fundamentally a problem of scale. Pollution and greenhouse gas (GHG) emissions in modern cities are disproportionately large relative to the extent of urban trees. Globally, forests are an important contributor to the C cycle because they occupy about a third of the land surface (FAO and UNEP, 2020). However, cities occupy less than 1% of the global land surface (Zhou et al., 2015; Liu et al., 2018), and within cities tree cover is highly variable but seldom equivalent to closed canopy forests (Nowak and Greenfield, 2018). Consequently, urban trees are most effective at providing ecosystem services that operate at local scales, such as parcels or urban forest/neighborhood patches.
For example, trees may improve human thermal comfort locally both through evaporatively cooling and humidifying urban air, and through shading, i.e., the interception of radiation by large canopies (Shashua-Bar et al., 2011; Chen and Ng, 2012; Rupp et al., 2015; Middel et al., 2016; Wang et al., 2018; Zhao et al., 2018). Wang et al. (2018) utilized the Weather Research and Forecasting Model (WRF) and found that all urban trees in the contiguous United States lower air temperature by about 3°C across urban areas compared to scenarios in which cities contained no trees at all. This is likely an upper limit on municipal-scale cooling effects, as this study evaluated the impact of all existing urban trees. Salmond et al. (2016) pointed out that most studies of urban forest-climate interactions are regional scale modeling that use WRF or other simulation tools, and there is generally less information about cooling effects from empirical measurement at local scales. Nevertheless, cooling has been found in empirical studies as a function of canopy cover. Jung et al. (2021) showed that the capacity of urban trees to mitigate land surface temperatures is non-linear and depends on the specific land cover type and level of development. Santamouris et al. (2017) found that across a wide range of studies, the median reduction in local air temperature by cooling interventions involving urban trees was as high as 1.5°C when including modeling scenarios that largely eliminated built cover, and 0.6°C across more realistic urban conditions. By intensively sampling urban air temperature across land cover gradients, Ziter et al. (2019) estimated urban cooling of up 2°C at spatial scales of 60–90 m in radius at very high canopy cover close to 100%.
Therefore, localized cooling with dense tree planting offers the potential for microclimate adaptation through highly targeted design interventions that focus on parks, bus stops, pathways, school yards, community centers and other pedestrian urban gathering spaces that significantly impact thermal comfort. In addition, the reduction of stormwater runoff from impervious surfaces may also facilitate climate change adaptation. Canopy interception, i.e., rainfall that is intercepted by the tree canopy and evaporates from the leaves, may constitute a significant fraction of water inputs from low intensity, short duration rainfall events, depending on tree species (Xiao et al., 1998; Xiao and McPherson, 2002; Asadian and Weiler, 2009; Nytch et al., 2019). Urban greenspaces typified by pervious, non-sealed soils can also absorb and store considerable amounts of rainwater in the soil. For example, urban catchments in two Finnish cities with 30–40% of permeable soils (parks and other green infrastructure) stored more than 1,000 m3 of rain water per ha per year, measured as the difference between the amount of water entering the catchment and discharge. The result was a substantial reduction in urban runoff volume and considerable improvement of the quality of runoff water (Valtanen et al., 2014a,b). In these cities, about 70–80% of rainfall occurs in low intensity (<10 mm) events, which are the conditions under which adding pervious areas of soil to cities is impactful for stormwater mitigation. Consequently, urban forests and other greenspaces with trees and permeable soils can help ameliorate the modified urban microclimate and hydrological cycle and mitigate heat and flooding locally.
Cities are limited in their extent globally. Space allocated to urban trees is also constrained. Urban spatial configurations that have been designed for dense human populations, movement, and social interactions leave limited options for accommodating biophysical processes like water uptake and evapotranspiration, nutrient uptake, wet and dry deposition, gas exchange, and C sequestration at the scale required to offset urban pollution. Hence cities are difficult to retrofit to accommodate greening strategies even as we discover their specific value. For example, it would be quite difficult, if not impossible, to reduce stormwater runoff by 50% using green infrastructure in a city that is 90% impervious, particularly if we can only allocate 1–2% of the land surface to greening strategies.
The spatial scale and distribution of urban greenspace reflects a range of socioeconomic dynamics and historical planning decisions made by city governments (Grove et al., 2014; Locke et al., 2020). Recent studies investigating the socioeconomic determinants of urban vegetation have predominantly shown a correlation between wealth and both vegetation cover and diversity, although this relationship is far from universal and may depend on other factors (Kendal et al., 2012; Szantoi et al., 2012; Schwarz et al., 2015; Watkins et al., 2017; Kuras et al., 2020). Trees may also present liabilities like tree falls, root damage to infrastructure, pollen allergies, and maintenance concerns. These disservices will necessarily constrain the location of trees in the built environment (Pataki, 2021). Temporal dynamics, including tree demographics, host-pathogen interactions, extinctions, and other population and evolutionary processes also influence the extent to which tree planting efforts influence urban conditions. A multitude of both anthropogenic and biophysical factors influence tree planting rates, removal, and mortality at the landscape scape, and some cities and neighborhoods are on trajectories of decreasing tree density and canopy cover (Roman et al., 2014; Ossola and Hopton, 2018; Hilbert et al., 2019). Low species diversity, poor site conditions, and planting palettes that are no longer suited to changing climatic conditions and pathogens present additional vulnerabilities for urban tree populations (Laćan and McBride, 2008; Berland and Elliott, 2014; Ordóñez and Duinker, 2014). There is increasing evidence of urban-driven evolutionary change in urban forest dynamics that interacts with ecosystem function over the long term (Johnson and Munshi-South, 2017). For example, evolutionary changes in traits that influence C cycling such as plant root traits, stomatal conductance, leaf nutrient stoichiometry, and soil microbial C cycling traits, have been shown to have significant implications for C sequestration (Monroe et al., 2018). While evolutionary processes may operate at long time scales for long-lived organisms such as trees, they have been shown to be very rapid for the microbial communities that influence plant fitness (Lau and Lennon, 2012). Both demographic and evolutionary processes are highly complex in urban forests that contain varying proportions of planted and naturally dispersed and regenerated trees (Nowak, 2012). Therefore, urban greening strategies must account for both spatial and temporal dynamics of planted urban trees and urban forest patches in defining planting strategies and management targets.
Maintaining urban tree populations at small spatial scales appears to significantly influence human health. Recent reviews have utilized the World Health Organization’s definition of health to evaluate the effects of either trees specifically, or greenspace more generally, on physical, mental, and social well-being (Nesbitt et al., 2017; van den Bosch and Ode Sang, 2017; Kondo et al., 2018; Wolf et al., 2020). Several aspects of physical health have been shown to be correlated with aspects of urban “greenery,” such as mortality, longevity, and heart rates, and weight changes (Nesbitt et al., 2017; Kondo et al., 2018). There are also numerous studies relating aspects of mental health to the prevalence of vegetation (Bratman et al., 2012; Nesbitt et al., 2017; Houlden et al., 2018). Notably, some studies distinguish the effects of trees, or species of trees, from other vegetation and some do not (Nesbitt et al., 2017). As a result, the precise mechanisms linking trees, biodiversity, and the different components of health: physical, mental, and social, remain uncertain (Lee and Maheswaran, 2010; Aerts et al., 2018). However, mechanistic responses of human health to actual and perceived biodiversity have been generally categorized as those that cause or reduce harm, and restore or build capacity for physical and mental health (Marselle et al., 2021). Research has shown that the amount of greenspace, as well as the distance urban dwellers have to travel to that greenspace, can influence the benefits of trees on public health (Annerstedt van den Bosch et al., 2016), but few studies have attempted to understand the type and amount of exposure to trees that confers health benefits (Shanahan et al., 2015; Jiang et al., 2016; Zhang et al., 2017). Finally, the relationship between the prevalence and types of urban trees and social health remains largely unexplored (Dinnie et al., 2013; Nesbitt et al., 2017; Jennings and Bamkole, 2019), but may be impactful at small spatial scales and through particular configurations, such as community gardens and the associated place attachment (Petrovic et al., 2019).
The health effects of trees may be a critically important aspect of urban forest benefits, even in dense cities with relatively limited space to support urban forests. This is because it is very possible that exposure to small plantings, parks, and views of urban forests may be effective in improving human health. That is, unlike pollution mitigation, small-scale plantings may have large impacts on health. Though some urban residents avoid large wooded areas due to safety concerns (Klein and Felson, 2021), many make varied use of small greenspaces (Peschardt et al., 2012). Indeed, some urban residents are highly deprived of virtually any access to nature, such that even modest additions have been shown to have measurable positive effects (Nadkarni et al., 2017). These social and public health functions of small greenspaces have been further highlighted by the COVID-19 pandemic, which in many cities restricted recreation to small local parks (Kleinschroth and Kowarik, 2020). Similar to the call for “dose-response” relationships between exposure to nature and human health (Jiang et al., 2016), there is a great need for additional quantitative and qualitative studies of the health impacts of urban tree plantings at different spatial and temporal scales, applicable to the realistic conditions and constraints of adding and maintaining trees in dense cities.
Going forward, a central question across many disciplines and stakeholders is: “What types of urban spaces promote social, economic, and environmental sustainability and prosperity?” As cities struggle to address myriad social, economic, and environmental problems, it is important to identify the specific social and environmental goals that can be achieved by the location, density, and extent of tree planting. Hence, the spatiotemporal dimensions of urban forest dynamics are a critical research uncertainty. For some ecosystem services, such as C sequestration, the ecological dynamics of C uptake by trees are well understood, but the direct impacts on atmospheric CO2 concentrations and climate depend on the spatial extent of urban trees, their demographic and population dynamics, and the interactions between productivity, heterotrophic respiration, and soil C dynamics at large spatial and temporal scales. Conversely, the reciprocal impacts of the atmosphere, including urban pollution and heat, on urban forest ecological processes are also in need of additional empirical measurements (Meineke et al., 2016). For other ecosystems services, the interactions between tree and soil processes and the built environment will determine the net influence of urban forests. For example, the effects of buildings, street design, and urban morphology on atmospheric dispersion, and their interactions with tree canopies, play a significant role in atmospheric pollutant concentrations (Han et al., 2020). Integrating trees into the built environment may also facilitate interventions to increase active transportation (Tsai et al., 2019; Young et al., 2020). Overall, we conclude that is difficult to significantly offset urban GHG and atmospheric pollution with localized tree planting, given the magnitude of emissions in modern cities. However, trees have localized effects on climate, thermal comfort, human health, and habitat for other species that may be impactful at the site scale.
The public health impacts of urban trees have been particularly difficult to characterize because collaborative, interdisciplinary approaches are needed to ascertain the nature of these impacts. Assessing the effects of planting interventions may require techniques such as virtual and/or real life walks or progressions through different types and scales of landscape designs (Berman et al., 2008). Browning et al. (2020) suggested that a lack of randomized treatments and experimental controls has hampered our understanding of the influence of natural landscapes on cognitive and mental health. There have also been disciplinary gaps and barriers between urban ecological and forestry studies and health scientists that continue to limit progress in linking trees and health (Eisenman et al., 2019). The next phase of planning and managing tree planting programs will require collaborative teams of natural scientists, social scientists, and practitioners from the health sciences including epidemiologists, ecopsychologists, and clinicians to evaluate the specific dimensions—including forest composition, biodiversity, soil health, and spatiotemporal dynamics—that interact with human health.
The study of socioecological interactions in cities provides a framework for generating and organizing place- and site-specific data across the many disciplines involved in planning and designing urban spaces. Furthermore, the growing field of ecological design and urban-focused landscape ecology provides hands-on approaches to planning and managing urban flora and fauna (Beck, 2013; Felson et al., 2013a, Figure 2). The COVID-19 pandemic has provided an unparalleled opportunity to re-configure urban landscapes in ways that integrate trees, forest patches, and green corridors into the built environment with evidence-based ecological designs. There may be unique opportunities to expand tree canopies in abandoned or re-zoned urban lands if remote work becomes commonplace on a permanent basis, causing redistributions of commercial and/or residential land uses (Boyd, 2020; Eltarabily and Elghezanwy, 2020; Ferrini and Gori, 2021). There are previous examples of “urban shrinkage” that resulted in reconceptualized urban greenspaces, open space networks, or forest regrowth (Kowarik and Körner, 2005; Nassauer and Raskin, 2014; Frazier and Bagchi-Sen, 2015; Haase et al., 2018). Where this is feasible, cost effective relative to other land use priorities, and implemented with resources to maintain tree planting over decadal timescales, extensive urban forests have observable environmental and social benefits. However, cities have competing demands for space that include pressing human needs for affordable housing, renewable energy generation, and food production, among other uses. Nevertheless, we suggest that even small-scale and temporary tree planting may have specific benefits. To maximize these benefits, it is essential to establish collaborative teams working through the design process to influence the direction of the built environment (Felson et al., 2013b). Focusing on comprehensive and phased planning alongside targeted site design and monitoring for the specific attributes of trees that contribute to climate adaptation and human health may be most effective for integrating urban forests into sustainability strategies.
Figure 2. The MillionTrees NYC Initiative uses the model of designed experiments to engage ecologists and designers in the creation of a long term and large-scale urban green infrastructure research program. Adapted from Felson et al. (2013a,b).
DP conceived of the manuscript and organized the text and manuscript. AF, MM, HS, and TW contributed images and figures. All authors contributed equally to writing the text. All authors contributed to the article and approved the submitted version.
DP was supported by National Science Foundation grant CNH 1924288 and the Fulbright Global Scholar program. HS was supported by the Academy of Finland grant 315987.
The authors declare that the research was conducted in the absence of any commercial or financial relationships that could be construed as a potential conflict of interest.
Aerts, R., Honnay, O., and Van Nieuwenhuyse, A. (2018). Biodiversity and human health: mechanisms and evidence of the positive health effects of diversity in nature and green spaces. Br. Med. Bull. 127, 5–22. doi: 10.1093/bmb/ldy021
Akbari, H. (2002). Shade trees reduce building energy use and CO2 emissions from power plants. Environ. Pollut. 116, S119–S126.
Akbari, H., Kurn, D. M., Bretz, S. E., and Hanford, J. W. (1997). Peak power and cooling energy savings of shade trees. Energy Build. 25, 139–148.
Annerstedt van den Bosch, M., Mudu, P., Uscila, V., Barrdahl, M., Kulinkina, A., Staatsen, B., et al. (2016). Development of an urban green space indicator and the public health rationale. Scand. J. Public Health 44, 159–167. doi: 10.1177/1403494815615444
Asadian, Y., and Weiler, M. (2009). A new approach in measuring rainfall interception by urban trees in coastal British Columbia. Water Qual. Rese. J. 44, 16–25. doi: 10.2166/wqrj.2009.003
Baró, F., Haase, D., Gómez-Baggethun, E., and Frantzeskaki, N. (2015). Mismatches between ecosystem services supply and demand in urban areas: a quantitative assessment in five European cities. Ecol. Ind. 55, 146–158.
Bartens, J., Day, S. D., Harris, J. R., Wynn, T. M., and Dove, J. E. (2009). Transpiration and root development of urban trees in structural soil stormwater reservoirs. Environ. Manag. 44, 646–657. doi: 10.1007/s00267-009-9366-9
Berland, A., and Elliott, G. P. (2014). Unexpected connections between residential urban forest diversity and vulnerability to two invasive beetles. Landscape Ecol. 29, 141–152. doi: 10.1007/s10980-013-9953-2
Berman, M. G., Jonides, J., and Kaplan, S. (2008). The cognitive benefits of interacting with nature. Psychol. Sci. 19, 1207–1212. doi: 10.1111/j.1467-9280.2008.02225.x
Bialecki, M. B., Fahey, R. T., and Scharenbroch, B. (2018). Variation in urban forest productivity and response to extreme drought across a large metropolitan region. Urban Ecosyst. 21, 157–169. doi: 10.1007/s11252-017-0692-z
Boyd, I. (2020). After Corona – rebuilding habitats for humans. Cities Health (in press), 1–4. doi: 10.1080/23748834.2020.1788756
Bratman, G. N., Hamilton, J. P., and Daily, G. C. (2012). The impacts of nature experience on human cognitive function and mental health. Ann. N. Y. Acad. Sci. 1249, 118–136. doi: 10.1111/j.1749-6632.2011.06400.x
Browning, M. H. E. M., Saeidi-Rizi, F., McAnirlin, O., Yoon, H., and Pei, Y. (2020). The role of methodological choices in the effects of experimental exposure to simulated natural landscapes on human health and cognitive performance: a systematic review. Environ. Behav. (in press), 0013916520906481. doi: 10.1177/0013916520906481
Burghardt, K. T., Tallamy, D. W., and Gregory Shriver, W. (2009). Impact of native plants on bird and butterfly biodiversity in suburban landscapes. Conserv. Biol. 23, 219–224. doi: 10.1111/j.1523-1739.2008.01076.x
Chen, L., and Ng, E. (2012). Outdoor thermal comfort and outdoor activities: a review of research in the past decade. Cities 29, 118–125. doi: 10.1016/j.cities.2011.08.006
Collins, J. P., Kinzig, A., Grimm, N. B., Fagan, W. F., Hope, D., Wu, J., et al. (2000). A new urban ecology: modeling human communities as integral parts of ecosystems poses special problems for the development and testing of ecological theory. Am. Sci. 88, 416–425.
Decina, S. M., Hutyra, L. R., Gately, C. K., Getson, J. M., Reinmann, A. B., Short Gianotti, A. G., et al. (2016). Soil respiration contributes substantially to urban carbon fluxes in the greater Boston area. Environ. Pollut. 212, 433–439. doi: 10.1016/j.envpol.2016.01.012
Dinnie, E., Brown, K. M., and Morris, S. (2013). Community, cooperation and conflict: negotiating the social well-being benefits of urban greenspace experiences. Landsc. Urban Plan. 118, 103–111. doi: 10.1016/j.landurbplan.2013.07.011
Donovan, G. H., and Butry, D. T. (2009). The value of shade: estimating the effect of urban trees on summertime electricity use. Energy Build. 41, 662–668. doi: 10.1016/j.enbuild.2009.01.002
Eisenman, T. S., Churkina, G., Jariwala, S. P., Lovasi, G. S., Pataki, D. E., Weinberger, K., et al. (2019). Urban trees, air quality, and asthma: an interdisciplinary review. Landsc. Urban Plan. 187, 47–59.
Eltarabily, S., and Elghezanwy, D. (2020). Post-pandemic cities - the impact of COVID-19 on cities and urban design. Arch. Res. 10, 75–84.
Escobedo, F., Varela, S., Zhao, M., Wagner, J. E., and Zipperer, W. (2010). Analyzing the efficacy of subtropical urban forests in offsetting carbon emissions from cities. Environ. Sci. Policy 13, 362–372. doi: 10.1016/j.envsci.2010.03.009
Escobedo, F. J., Adams, D. C., and Timilsina, N. (2015). Urban forest structure effects on property value. Ecosyst. Serv. 12, 209–217. doi: 10.1016/j.ecoser.2014.05.002
FAO, and UNEP (2020). The State of the World’s Forests 2020. Forests, Biodiversity and People. Rome: FAO, doi: 10.4060/CA8642EN
Felson, A. J., Bradford, M. A., and Terway, T. M. (2013a). Promoting Earth Stewardship through urban design experiments. Front. Ecol. Environ. 11:362–367. doi: 10.1890/130061
Felson, A. J., Pavao-Zuckerman, M., Carter, T., Montalto, F., Shuster, B., Springer, N., et al. (2013b). Mapping the design process for urban ecology researchers. BioScience 63, 854–865.
Ferrini, F., and Gori, A. (2021). Cities after COVID-19: how trees and green infrastructure can help shaping a sustainable future. Ri Vista (in press).
Frazier, A. E., and Bagchi-Sen, S. (2015). Developing open space networks in shrinking cities. Appl. Geogr. 59, 1–9. doi: 10.1016/j.apgeog.2015.02.010
Grove, J. M., Locke, D. H., and O’Neil-Dunne, J. P. M. (2014). An ecology of prestige in New York City: examining the relationships among population density, socio-economic status, group identity, and residential canopy cover. Environ. Manag. 54, 402–419.
Haase, A., Wolff, M., and Rink, D. (2018). “From shrinkage to regrowth: the nexus between urban dynamics, land use change and ecosystem service provision,” in Urban Transformations: Sustainable Urban Development Through Resource Efficiency, Quality of Life and Resilience Future City, eds S. Kabisch, F. Koch, E. Gawel, A. Haase, S. Knapp, K. Krellenberg, et al. (Cham: Springer International Publishing), 197–219. doi: 10.1007/978-3-319-59324-1_11
Han, D., Shen, H., Duan, W., and Chen, L. (2020). A review on particulate matter removal capacity by urban forests at different scales. Urban For. Urban Green. 48:126565. doi: 10.1016/j.ufug.2019.126565
Hilbert, D. R., Roman, L. A., Koeser, A. K., Vogt, J., and van Doorn, N. S. (2019). Urban tree mortality: a literature review. Arboric. Urban For. 45, 167–200.
Houlden, V., Weich, S., de Albuquerque, J. P., Jarvis, S., and Rees, K. (2018). The relationship between greenspace and the mental wellbeing of adults: a systematic review. PLoS One 13:e0203000. doi: 10.1371/journal.pone.0203000
Hsieh, C.-M., Li, J.-J., Zhang, L., and Schwegler, B. (2018). Effects of tree shading and transpiration on building cooling energy use. Energy Build. 159, 382–397. doi: 10.1016/j.enbuild.2017.10.045
Hutyra, L. R., Duren, R., Gurney, K. R., Grimm, N., Kort, E. A., Larson, E., et al. (2014). Urbanization and the carbon cycle: current capabilities and research outlook from the natural sciences perspective. Earth’s Future 2, 473–495. doi: 10.1002/2014EF000255
Hutyra, L. R., Yoon, B., and Alberti, M. (2011). Terrestrial carbon stocks across a gradient of urbanization: a study of the Seattle. WA region. Glob. Change Biol. 17, 783–797. doi: 10.1111/j.1365-2486.2010.02238.x
Jennings, V., and Bamkole, O. (2019). The relationship between social cohesion and urban green space: an avenue for health promotion. Int. J. Environ. Res. Public Health 16:452. doi: 10.3390/ijerph16030452
Jiang, B., Li, D., Larsen, L., and Sullivan, W. C. (2016). A dose-response curve describing the relationship between urban tree cover density and self-reported stress recovery. Environ. Behav. 48, 607–629. doi: 10.1177/0013916514552321
Johnson, T. J., and Munshi-South, J. (2017). Evolution of life in urban environments. Science 358:eaam8327.
Jung, M. C., Dyson, K., and Alberti, M. (2021). Urban landscape heterogeneity influences the relationship between tree canopy and land surface temperature. Urban For. Urban Green. 57:126930. doi: 10.1016/j.ufug.2020.126930
Kendal, D., Williams, N. S. G., and Williams, K. J. H. (2012). Drivers of diversity and tree cover in gardens, parks and streetscapes in an Australian city. Urban For. Urban Green. 11, 257–265. doi: 10.1016/j.ufug.2012.03.005
Klein, W., and Felson, A. (2021). Engaging the unengaged: understanding resident’s perceptions of social access to familiar neighborhood places. Urban For. Urban Green. 59:126991.
Kleinschroth, F., and Kowarik, I. (2020). COVID-19 crisis demonstrates the urgent need for urban greenspaces. Front. Ecol. Environ. 18:318–319. doi: 10.1002/fee.2230
Kondo, M. C., Fluehr, J. M., McKeon, T., and Branas, C. C. (2018). Urban green space and its impact on human health. Int. J. Environ. Res. Public Health 15:445. doi: 10.3390/ijerph15030445
Kowarik, I., and Körner, S. (eds) (2005). Wild Urban Woodlands: New Perspectives for Urban Forestry. Berlin: Springer-Verlag, doi: 10.1007/b138211
Kuras, E. R., Warren, P. S., Zinda, J. A., Aronson, M. F. J., Cilliers, S., Goddard, M. A., et al. (2020). Urban socioeconomic inequality and biodiversity often converge, but not always: a global meta-analysis. Landsc. Urban Plan. 198:103799. doi: 10.1016/j.landurbplan.2020.103799
Laćan, I., and McBride, J. R. (2008). Pest vulnerability matrix (PVM): a graphic model for assessing the interaction between tree species diversity and urban forest susceptibility to insects and diseases. Urban For. Urban Green. 7, 291–300. doi: 10.1016/j.ufug.2008.06.002
Lau, J. A., and Lennon, J. T. (2012). Rapid responses of soil microorganisms improve plant fitness in novel environments. PNAS 109, 14058–14062. doi: 10.1073/pnas.1202319109
Laukkonen, J., Blanco, P. K., Lenhart, J., Keiner, M., Cavric, B., and Kinuthia-Njenga, C. (2009). Combining climate change adaptation and mitigation measures at the local level. Habitat Int. 33, 287–292. doi: 10.1016/j.habitatint.2008.10.003
Lee, A. C. K., and Maheswaran, R. (2010). The health benefits of urban green spaces: a review of the evidence. J. Public Health 33, 212–222. doi: 10.1093/pubmed/fdq068
Li, D., and Sullivan, W. C. (2016). Impact of views to school landscapes on recovery from stress and mental fatigue. Landsc. Urban Plan. 148, 149–158. doi: 10.1016/j.landurbplan.2015.12.015
Li, W., and Saphores, J.-D. (2012). A spatial hedonic analysis of the value of urban land cover in the multifamily housing market in Los Angeles. CA. Urban Stud 49, 2597–2615. doi: 10.1177/0042098011429486
Lindén, L., Riikonen, A., Setälä, H., and Yli-Pelkonen, V. (2020). Quantifying carbon stocks in urban parks under cold climate conditions. Urban For. Urban Green. 49:126633. doi: 10.1016/j.ufug.2020.126633
Liu, C., and Li, X. (2012). Carbon storage and sequestration by urban forests in Shenyang. China. Urban For. Urban Green. 11, 121–128. doi: 10.1016/j.ufug.2011.03.002
Liu, X., Hu, G., Chen, Y., Li, X., Xu, X., Li, S., et al. (2018). High-resolution multi-temporal mapping of global urban land using Landsat images based on the Google Earth Engine Platform. Remote Sens. Environ. 209, 227–239. doi: 10.1016/j.rse.2018.02.055
Locke, D., Hall, B., Grove, J. M., Pickett, S. T. A., Ogden, L. A., Aoki, C., et al. (2020). Residential Housing Segregation and Urban tree Canopy in 37 US Cities. Charlottesville, VA: Center for Open Science.
Lovasi, G. S., O’Neil-Dunne, J. P. M., Lu, J. W. T., Sheehan, D., Perzanowski, M. S., Macfaden, S. W., et al. (2013). Urban tree canopy and asthma, wheeze, rhinitis, and allergic sensitization to tree pollen in a New York City birth cohort. Environ. Health Perspect. 121, 494–500. doi: 10.1289/ehp.1205513
Marselle, M. R., Hartig, T., Cox, D. T. C., de Bell, S., Knapp, S., Lindley, S., et al. (2021). Pathways linking biodiversity to human health: a conceptual framework. Environ. Int. 150:106420. doi: 10.1016/j.envint.2021.106420
McDonald, R., Kroeger, T., Boucher, T., Longzhu, R., Salem, J., Adams, S., et al. (2016). Planting Healthy Air: A Global Analysis of the Role of Trees in Addressing Particulate Matter Pollution and Extreme Heat. Arlington, VA: The Nature Conservancy.
McHale, M. R., McPherson, E. G., and Burke, I. C. (2007). The potential of urban tree plantings to be cost effective in carbon credit markets. Urban For. Urban Green. 6, 49–60.
McPherson, E. G. (1992). Accounting for for benefits and costs of urban greenspace. Landsc. Urban Plan. 22, 41–51.
McPherson, E. G. (1993). Evaluating the cost effectiveness of shade trees for demand-side management. Electr. J. 6, 57–65.
McPherson, E. G., Simpson, J. R., Peper, P. F., Maco, S. E., and Xiao, Q. (2005). Municipal forest benefits and costs in five U.S. cities. J. For. 104, 411–416.
Meineke, E., Youngsteadt, E., Dunn, R. R., and Frank, S. D. (2016). Urban warming reduces aboveground carbon storage. Proc. R. Soc. B Biol. Sci. 283:20161574. doi: 10.1098/rspb.2016.1574
Middel, A., Selover, N., Hagen, B., and Chhetri, N. (2016). Impact of shade on outdoor thermal comfort—a seasonal field study in Tempe. Arizona. Int. J. Biometeorol. 60, 1849–1861. doi: 10.1007/s00484-016-1172-5
Monroe, J. G., Markman, D. W., Beck, W. S., Felton, A. J., Vahsen, M. L., and Pressler, Y. (2018). Ecoevolutionary dynamics of carbon cycling in the Anthropocene. Trends Ecol. Evol. 33, 213–225. doi: 10.1016/j.tree.2017.12.006
Nadkarni, N. M., Hasbach, P. H., Thys, T., Crockett, E. G., and Schnacker, L. (2017). Impacts of nature imagery on people in severely nature-deprived environments. Front. Ecol. Environ. 15:395–403. doi: 10.1002/fee.1518
Nassauer, J. I., and Raskin, J. (2014). Urban vacancy and land use legacies: a frontier for urban ecological research, design, and planning. Landsc. Urban Plan. 125, 245–253. doi: 10.1016/j.landurbplan.2013.10.008
Nesbitt, L., Hotte, N., Barron, S., Cowan, J., and Sheppard, S. R. J. (2017). The social and economic value of cultural ecosystem services provided by urban forests in North America: a review and suggestions for future research. Urban For. Urban Green. 25, 103–111. doi: 10.1016/j.ufug.2017.05.005
Nowak, D., and Crane, D. E. (2000). “The urban forest effects (UFORE) model: quantifying urban forest structure and functions,” in Integrated Tools for Natural Resources Inventories in the 21st Century, Gen. Tech. Rep. NC-212, eds M. Hansen and T. Burk (St. Paul, MN: U.S. Dept. of Agriculture), 714–720.
Nowak, D. J. (2012). Contrasting natural regeneration and tree planting in fourteen North American cities. Urban For. Urban Green. 11, 374–382. doi: 10.1016/j.ufug.2012.02.005
Nowak, D. J., and Crane, D. E. (2002). Carbon storage and sequestration by urban trees in the USA. Environ. Pollut. 116, 381–389.
Nowak, D. J., Crane, D. E., and Dwyer, J. F. (2002). Compensatory value of urban trees in the United States. J. Arboric. 28, 194–199.
Nowak, D. J., Crane, D. E., and Stevens, J. C. (2006). Air pollution removal by urban trees and shrubs in the United States. Urban For. Urban Green. 4, 115–123.
Nowak, D. J., and Greenfield, E. J. (2018). US urban forest statistics, values, and projections. J. For. 116, 164–177. doi: 10.1093/jofore/fvx004
Nowak, D. J., Greenfield, E. J., Hoehn, R. E., and Lapoint, E. (2013). Carbon storage and sequestration by trees in urban and community areas of the United States. Environ. Pollut. 178, 229–236. doi: 10.1016/j.envpol.2013.03.019
Nowak, D. J., Hirabayashi, S., Doyle, M., McGovern, M., and Pasher, J. (2018). Air pollution removal by urban forests in Canada and its effect on air quality and human health. Urban For. Urban Green. 29, 40–48. doi: 10.1016/j.ufug.2017.10.019
Nytch, C. J., Meléndez-Ackerman, E. J., Pérez, M.-E., and Ortiz-Zayas, J. R. (2019). Rainfall interception by six urban trees in San Juan. Puerto Rico. Urban Ecosyst. 22, 103–115. doi: 10.1007/s11252-018-0768-4
Oldfield, E. E., Warren, R. J., Felson, A. J., and Bradford, M. A. (2013). Challenges and future directions in urban afforestation. J. Appl. Ecol. 50, 1169–1177. doi: 10.1111/1365-2664.12124
Ordóñez, C., and Duinker, P. N. (2014). Assessing the vulnerability of urban forests to climate change. Environ. Rev. 22. doi: 10.1139/er-2013-0078
Ossola, A., and Hopton, M. E. (2018). Measuring urban tree loss dynamics across residential landscapes. Sci. Total Environ. 612, 940–949. doi: 10.1016/j.scitotenv.2017.08.103
Pataki, D. E. (2021). “Ecosystem disservices from urban nature,” in The Routledge Handbook of Urban Ecology, eds I. Douglas, P. M. L. Anderson, D. Goode, M. C. Houck, D. Maddox, H. Nagendra, et al. (London: Routledge).
Pataki, D. E., Carreiro, M. M., Cherrier, J., Grulke, N. E., Jennings, V., Pincetl, S., et al. (2011). Coupling biogeochemical cycles in urban environments: ecosystem services, green solutions, and misconceptions. Front. Ecol. Environ. 9:27–36. doi: 10.1890/090220
Pataki, D. E., Emmi, P. C., Forster, C., Mills, J., Pardyjak, E. R., Peterson, T. R., et al. (2009). An integrated approach to improving fossil fuel emissions scenarios with urban ecosystems studies. Ecol. Comp. 6, 1–14.
Peschardt, K. K., Schipperijn, J., and Stigsdotter, U. K. (2012). Use of Small Public Urban Green Spaces (SPUGS). Urban For. Urban Green. 11, 235–244. doi: 10.1016/j.ufug.2012.04.002
Petrovic, N., Simpson, T., Orlove, B., and Dowd-Uribe, B. (2019). Environmental and social dimensions of community gardens in East Harlem. Landsc. Urban Plan. 183, 36–49. doi: 10.1016/j.landurbplan.2018.10.009
Pouyat, R., Groffman, P., Yesilonis, I., and Hernandez, L. (2002). Soil carbon pools and fluxes in urban ecosystems. Environ. Pollut. 116, S107–S118.
Pouyat, R. V., Yesilonis, I. D., and Nowak, D. J. (2006). Carbon storage by urban soils in the United States. J. Environ. Qual. 35, 1566–1575.
Ray, E. B. (2005). “Using CITYgreen and high resolution multi-spectral imagery to analyze the urban ecosystem,” in Managing Watersheds for Human and Natural Impacts, (Williamsburg, Virginia: American Society of Civil Engineers). Available online at: http://ascelibrary.org/doi/abs/10.1061/40763%28178%2914
Roman, L. A., Battles, J. J., and McBride, J. R. (2014). The balance of planting and mortality in a street tree population. Urban Ecosyst. 17, 387–404. doi: 10.1007/s11252-013-0320-5
Roy, S., Byrne, J., and Pickering, C. (2012). A systematic quantitative review of urban tree benefits, costs, and assessment methods across cities in different climatic zones. Urban For. Urban Green. 11, 351–363.
Rupp, R. F., Vásquez, N. G., and Lamberts, R. (2015). A review of human thermal comfort in the built environment. Energy Build. 105, 178–205. doi: 10.1016/j.enbuild.2015.07.047
Salmond, J. A., Tadaki, M., Vardoulakis, S., Arbuthnott, K., Coutts, A., Demuzere, M., et al. (2016). Health and climate related ecosystem services provided by street trees in the urban environment. Environ. Health 15:S36. doi: 10.1186/s12940-016-0103-6
Salmond, J. A., Williams, D. E., Laing, G., Kingham, S., Dirks, K., Longley, I., et al. (2013). The influence of vegetation on the horizontal and vertical distribution of pollutants in a street canyon. Sci. The Total Environ. 443, 287–298. doi: 10.1016/j.scitotenv.2012.10.101
Sander, H., Polasky, S., and Haight, R. G. (2010). The value of urban tree cover: a hedonic property price model in Ramsey and Dakota Counties. Minnesota, USA. Ecol. Econ. 69, 1646–1656.
Santamouris, M., Ding, L., Fiorito, F., Oldfield, P., Osmond, P., Paolini, R., et al. (2017). Passive and active cooling for the outdoor built environment – Analysis and assessment of the cooling potential of mitigation technologies using performance data from 220 large scale projects. Solar Energy 154, 14–33. doi: 10.1016/j.solener.2016.12.006
Schwarz, K., Fragkias, M., Boone, C. G., Zhou, W., McHale, M., Grove, J. M., et al. (2015). Trees grow on money: urban tree canopy cover and environmental justice. PLoS One 10:e0122051. doi: 10.1371/journal.pone.0122051
Setälä, H., Viippola, V., Rantalainen, A.-L., Pennanen, A., and Yli-Pelkonen, V. (2013). Does urban vegetation mitigate air pollution in northern conditions? Environ. Pollut. 183, 104–112. doi: 10.1016/j.envpol.2012.11.010
Shanahan, D. F., Lin, B. B., Bush, R., Gaston, K. J., Dean, J. H., Barber, E., et al. (2015). Toward improved public health outcomes from Urban nature. Am. J. Public Health 105, 470–477. doi: 10.2105/AJPH.2014.302324
Shashua-Bar, L., and Hoffman, M. E. (2000). Vegetation as a climatic component in the design of an urban street. Energy Build. 31, 221–235. doi: 10.1016/S0378-7788(99)00018-3
Shashua-Bar, L., Pearlmutter, D., and Erell, E. (2011). The influence of trees and grass on outdoor thermal comfort in a hot-arid environment. Int. J. Climatol. 31, 1498–1506. doi: 10.1002/joc.2177
Sonti, N. F., Hallett, R. A., Griffin, K. L., and Sullivan, J. H. (2019). White oak and red maple tree ring analysis reveals enhanced productivity in urban forest patches. For. Ecol. Manag. 453:117626. doi: 10.1016/j.foreco.2019.117626
Strohbach, M. W., and Haase, D. (2012). Above-ground carbon storage by urban trees in Leipzig, Germany: analysis of patterns in a European city. Landsc. Urban Plan. 104, 95–104. doi: 10.1016/j.landurbplan.2011.10.001
Szantoi, Z., Escobedo, F., Wagner, J., Rodriguez, J. M., and Smith, S. (2012). Socioeconomic factors and urban tree cover policies in a subtropical Urban forest. GISci. Remote Sens. 49, 428–449. doi: 10.2747/1548-1603.49.3.428
Tallis, M., Taylor, G., Sinnett, D., and Freer-Smith, P. (2011). Estimating the removal of atmospheric particulate pollution by the urban tree canopy of London, under current and future environments. Landsc. Urban Plan. 103, 129–138.
Timilsina, N., Staudhammer, C. L., Escobedo, F. J., and Lawrence, A. (2014). Tree biomass, wood waste yield, and carbon storage changes in an urban forest. Landsc. Urban Plan. 127, 18–27. doi: 10.1016/j.landurbplan.2014.04.003
Tong, Z., Whitlow, T. H., MacRae, P. F., Landers, A. J., and Harada, Y. (2015). Quantifying the effect of vegetation on near-road air quality using brief campaigns. Environ. Pollut. 201, 141–149. doi: 10.1016/j.envpol.2015.02.026
Trlica, A., Hutyra, L. R., Morreale, L. L., Smith, I. A., and Reinmann, A. B. (2020). Current and future biomass carbon uptake in Boston’s urban forest. Sci. Total Environ. 709:136196. doi: 10.1016/j.scitotenv.2019.136196
Tsai, W.-L., Yngve, L., Zhou, Y., Beyer, K. M. M., Bersch, A., Malecki, K. M., et al. (2019). Street-level neighborhood greenery linked to active transportation: a case study in Milwaukee and Green Bay. WI, USA. Landsc. Urban Plann. 191:103619. doi: 10.1016/j.landurbplan.2019.103619
Ulrich, R., Simons, R., Losito, B., Fiorito, E., Miles, M., and Zelson, M. (1991). Stress recovery during exposure to natural and urban environments. J. Environ. Psychol. 11, 201–230. doi: 10.1016/S0272-4944(05)80184-7
Ulrich, R. S. (1983). View through a window may influence recovery from surgery. Science 224, 420–421.
Valtanen, M., Sillanpää, N., and Setälä, H. (2014a). Effects of land use intensity on stormwater runoff and its temporal occurrence in cold climates. Hydrol. Process. 28, 2639–2650. doi: 10.1002/hyp.9819
Valtanen, M., Sillanpää, N., and Setälä, H. (2014b). The effects of urbanization on runoff pollutant concentrations, loadings and their seasonal patterns Under Cold Climate. Water Air Soil Pollut. 225:1977. doi: 10.1007/s11270-014-1977-y
van den Bosch, M., and Ode Sang, Å (2017). Urban natural environments as nature-based solutions for improved public health – A systematic review of reviews. Environ. Res. 158, 373–384. doi: 10.1016/j.envres.2017.05.040
Velasco, E., Roth, M., Norford, L., and Molina, L. T. (2016). Does urban vegetation enhance carbon sequestration? Landsc. Urban Plan. 148, 99–107. doi: 10.1016/j.landurbplan.2015.12.003
Viippola, V., Whitlow, T. H., Zhao, W., Yli-Pelkonen, V., Mikola, J., Pouyat, R., et al. (2018). The effects of trees on air pollutant levels in peri-urban near-road environments. Urban For. Urban Green. 30, 62–71. doi: 10.1016/j.ufug.2018.01.014
Wang, C., Wang, Z.-H., and Yang, J. (2018). Cooling effect of urban trees on the built environment of contiguous United States. Earth’s Future 6, 1066–1081. doi: 10.1029/2018EF000891
Watkins, S. L., Mincey, S. K., Vogt, J., and Sweeney, S. P. (2017). Is planting equitable? An examination of the spatial distribution of nonprofit urban tree-planting programs by canopy cover, income, race, and ethnicity. Environ. Behav. 49, 452–482. doi: 10.1177/0013916516636423
Wolf, K. L., Lam, S. T., McKeen, J. K., Richardson, G. R. A., van den Bosch, M., and Bardekjian, A. C. (2020). Urban trees and human health: a scoping review. Int. J. Environ. Res. Public Health 17:4371. doi: 10.3390/ijerph17124371
Xiao, Q., and McPherson, E. G. (2002). Rainfall interception by Santa Monica’s municipal urban forest. Urban Ecosyst. 6, 291–302. doi: 10.1023/B:UECO.0000004828.05143.67
Xiao, Q., McPherson, E. G., Simpson, J. R., and Ustin, S. L. (1998). Rainfall interception by Sacramento’s urban forest. J. Arboric. 24, 235–244.
Xing, Y., and Brimblecombe, P. (2020). Trees and parks as “the lungs of cities.”. Urban For. Urban Green. 48:126552.
Young, D. R., Cradock, A. L., Eyler, A. A., Fenton, M., Pedroso, M., Sallis, J. F., et al. (2020). Creating built environments that expand active transportation and active living across the United States: a policy statement from the American Heart Association. Circulation 142, e167–e183. doi: 10.1161/CIR.0000000000000878
Zhang, L., Tan, P. Y., and Diehl, J. A. (2017). A conceptual framework for studying urban green spaces effects on health. J. Urban Ecol. 3, 212–222. doi: 10.1093/jue/jux015
Zhao, Q., Sailor, D. J., and Wentz, E. A. (2018). Impact of tree locations and arrangements on outdoor microclimates and human thermal comfort in an urban residential environment. Urban For. Urban Green. 32, 81–91. doi: 10.1016/j.ufug.2018.03.022
Zhou, Y., Smith, S. J., Zhao, K., Imhoff, M., Thomson, A., Bond-Lamberty, B., et al. (2015). A global map of urban extent from nightlights. Environ. Res. Lett. 10:054011. doi: 10.1088/1748-9326/10/5/054011
Keywords: urban ecology, forestry, sustainability, policy, climate mitigation, climate adaptation, ecosystem services, ecosystem disservices
Citation: Pataki DE, Alberti M, Cadenasso ML, Felson AJ, McDonnell MJ, Pincetl S, Pouyat RV, Setälä H and Whitlow TH (2021) The Benefits and Limits of Urban Tree Planting for Environmental and Human Health. Front. Ecol. Evol. 9:603757. doi: 10.3389/fevo.2021.603757
Received: 07 September 2020; Accepted: 24 February 2021;
Published: 08 April 2021.
Edited by:
Galina Churkina, Potsdam Institute for Climate Impact Research (PIK), GermanyReviewed by:
Stephan Pauleit, Technical University of Munich, GermanyCopyright © 2021 Pataki, Alberti, Cadenasso, Felson, McDonnell, Pincetl, Pouyat, Setälä and Whitlow. This is an open-access article distributed under the terms of the Creative Commons Attribution License (CC BY). The use, distribution or reproduction in other forums is permitted, provided the original author(s) and the copyright owner(s) are credited and that the original publication in this journal is cited, in accordance with accepted academic practice. No use, distribution or reproduction is permitted which does not comply with these terms.
*Correspondence: Diane E. Pataki, ZGlhbmUucGF0YWtpQHV0YWguZWR1
Disclaimer: All claims expressed in this article are solely those of the authors and do not necessarily represent those of their affiliated organizations, or those of the publisher, the editors and the reviewers. Any product that may be evaluated in this article or claim that may be made by its manufacturer is not guaranteed or endorsed by the publisher.
Research integrity at Frontiers
Learn more about the work of our research integrity team to safeguard the quality of each article we publish.