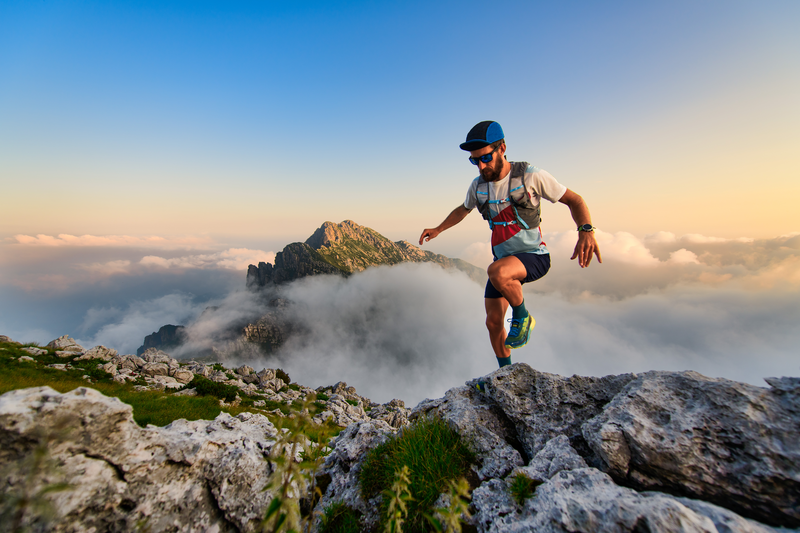
95% of researchers rate our articles as excellent or good
Learn more about the work of our research integrity team to safeguard the quality of each article we publish.
Find out more
ORIGINAL RESEARCH article
Front. Ecol. Evol. , 29 July 2021
Sec. Biogeography and Macroecology
Volume 9 - 2021 | https://doi.org/10.3389/fevo.2021.602895
This article is part of the Research Topic The Ecology, Evolution, and Preservation of Biodiversity in Amazonian Floodplain Ecosystems View all 7 articles
The Amazon River Basin, one of the world’s most threatened ecosystems, has an enormous diversity of fish species, a result of temporally and spatially complex habitat containing biogeochemically different river systems. The annual hydrologic cycle results in floodplain lakes during low water and inundates forests during high water, exposing fish to different resources and environmental conditions. The two principal river systems in the central Brazilian Amazon are blackwater, with nutrient-poor acidic water, and nutrient-rich whitewater. Although species-rich, the Amazon Basin is data-poor in terms of comparative studies on a regional scale. We analyzed data sets from independent sampling studies of pelagic fish in 16 floodplain lakes, nine whitewater (Rio Solimões) and seven blackwater (Rio Negro), in the central Amazon Basin of Brazil. Our findings suggest striking similarities in pelagic fish diversity patterns. Species richness was virtually equal (165 in whitewater and 168 in blackwater). Both species richness, and number of migratory species, per lake increased toward the confluence of the rivers in both systems in our study. The proportion of unique species was also similar in whitewater lakes and blackwater (41 and 43%, respectively), boosting total regional richness to 237 species. However, species composition in whitewater lakes was more homogenous (lower β diversity), and species composition was associated with conductivity and pH in whitewater, but with dissolved oxygen and transparency in blackwater. Therefore, regional fish diversity cannot be represented by sampling one lake or even one drainage system, but must include multiple lakes from both systems. These two systems may differ in sensitivity to anthropogenic stressors such as damming and deforestation.
The Amazon River Basin is the world’s largest freshwater ecosystem, with an aquatic surface area of 93,000 km2 (Allen and Pavelsky, 2018). This enormous ecosystem is home to an extraordinary diversity of fish. At least 2,716 fish species, including 1,696 of which are endemic to the basin, have been identified so far (Reis et al., 2016; Dagosta and Pinna, 2019). It is also one of the earth’s most endangered ecosystems, largely from ongoing anthropogenic stressors to its hydrology and biodiversity such as dam building that reduces connectivity among habitats (Kahn et al., 2014; Hurd et al., 2016; Santos et al., 2018), oil and gas development (Finer et al., 2008), overfishing of both food species and ornamental fish (Campos et al., 2015; Costa-Pereira and Galetti, 2017; Castanho et al., 2019), invasive alien species (Latini and Petrere, 2004), deforestation (Lobón-Cerviá et al., 2015), urbanization (Tregidgo et al., 2017), and droughts caused by deforestation and global warming (Castello et al., 2013; Freitas et al., 2013; Nazareno and Laurance, 2015). These problems have caused growing concern over the question of how to preserve fish species richness and also conserve fishery stocks, which are the most important sources of protein for human communities in the region (Castello et al., 2013). We therefore need to understand patterns of species distribution within this basin well enough to design ways to preserve aquatic biodiversity. Our aim is to discern how pelagic fish species diversity, which includes many species harvested for human consumption, is distributed between physically different drainage systems that flow through the central portion of the Amazon River Basin in Brazil.
The putative evolutionary causes that generated the enormous diversity of fish species in this basin, such as geologic age, productivity, adaptive radiation, and extensive allopatry have been discussed and conjectured by many authors. Recently, Albert et al. (2020) concluded that historical geologic processes periodically interrupted aquatic connectivity and created isolated areas that set the stage for habitat specialization and allopatric speciation, which was more important than adaptive radiation within habitats in explaining the development of high overall diversity. The present-day physical environment includes an annual hydrologic cycle that alternates between complete inundation of floodplain forests at high water, and formation of floodplain lakes with varying degrees of connection (or isolation) from river channels at low water. This “flood pulse” (Junk et al., 1989), in which water depth can vary 15 m or more depending on specific location along the rivers, is considered to be the dominant feature of the physical environment for fish species in this region (Junk et al., 1997; Silva et al., 2020). Floodplains occupy 73,500 km2 (Hess et al., 2015), providing important habitat for Amazonian fish, some of which remain on the floodplain all their lives, dispersing into the flooded forests to feed at high water and then returning to open water of the lakes as the water recedes. Others migrate out of the lakes to spawn in rivers when the boundaries between lakes and the river channels are erased during high water season (Fernandes, 1997).
There are two different aquatic systems in the central Amazon Basin of Brazil, based on biogeochemistry: whitewater and blackwater. Many smaller tributaries contribute flow to each of these systems, but the main blackwater artery in this region is the Rio Negro, with floodplains known as igapós, and the principle whitewater river is the Rio Solimões (Rio Amazonas west of the border with Columbia), with várzeas. The confluence of these two rivers occurs at the city of Manaus, and downriver from that point is known in Brazil as the Rio Amazonas.
Rivers in the whitewater system carry a high load of suspended nutrient-rich sediments to the floodplain during the flood season, causing the water to be the color of heavily creamed coffee and supporting highly productive terrestrial and aquatic plant communities, including floodplain forests (Furch, 1984; Junk and Piedade, 1997; Junk et al., 2011). In contrast, blackwater rivers (the color of strong tea) are very poor in nutrients and more acidic than whitewater, and thus very low in net primary productivity (Bogotá-Gregory et al., 2020). Most of the productivity in the blackwater system is in the form of allochthonous input from the adjacent forests, especially when high water floods them and allows fish close access to such forest products as vegetation, insects, and fruits (Furch, 1984; Loebens et al., 2019). In fact, it is very likely that fish were the first frugivorous vertebrates to disperse terrestrial plant seeds (ichthyochory), beginning in the Paleozoic (Horn et al., 2011).
Much of the literature reporting comparative fish species richness between these two drainage systems tends to show higher richness in whitewater than in blackwater area, although this finding is not uniform. For instance, in a survey of published comparisons of fish diversity between these two systems Crampton (2011) found that the western whitewater habitats tended to have more species than the blackwater drainage, but Saint-Paul et al. (2000) reported that blackwater fish assemblages were 10% more diverse than those in whitewater. The failure to agree on this is probably largely due to differences in how and where different studies were conducted.
Most of the published studies comparing fish diversity between systems have been limited in terms of landscape area or number of lakes, comparisons of unequal areas between blackwater and whitewater, types of habitat sampled within lakes, and sampling methodology. Much of the difficulty in making comparisons of this kind is rooted in scale dependence. Differences in species composition among lakes of the same drainage system result in high β-diversity (Freitas et al., 2014), so representation of species richness must be based on multiple lakes. Within each lake there are different habitats (e.g., open water, shoreline, and macrophyte meadows in whitewater lakes) that fragment the within-lake distribution of resident species so that all of these must be sampled (Siqueira-Souza et al., 2016). There is also a temporal scale: since fish assemblages vary in species composition among the hydrologic seasons, it is necessary to sample during both high and low water periods in order to obtain representative fish species diversity (Merona et al., 1993; Scarabotti et al., 2011; Siqueira-Souza et al., 2016). Finally, sampling methodology of many studies were inadequate to capture fish that are too small for the gill nets employed, or have behaviors that did not bring them into contact with the collection gear. Thus, the number of species sampled do not constitute total fish diversity in these studies, but are still useful for assessing species richness of selected groups.
The most common sampling strategy for fish in the basin has involved deployment of gill nets with varying mesh sizes (Saint-Paul et al., 2000), which are adequate for medium-large body size pelagic fish but not for smaller fish, or for benthic species during high water season when nets don’t reach the bottom of the lakes. A notable exception to this sampling limitation is Bogotá-Gregory et al. (2020), who used a variety of sampling gear to get a more accurate picture of total fish diversity, and arrived at the conclusion that whitewater fish had higher fish diversity than blackwater (and also than clearwater, which does not occur in our part of the Amazon Basin).
We examined patterns in the species richness of moderate-to-large size pelagic fish inhabiting floodplain lakes of the Rio Negro and Rio Solimões in the Central Amazon River Basin of Brazil, specifically located upstream from the merging of the two rivers at Manaus. Specifically, we assessed pelagic species richness in individual lakes (α-diversity), comparison of richness among lakes within each system (β-diversity), comparison of numbers of shared and unshared species between the systems, physical properties of the water associated with diversity in each system, and geographic distance of lakes from the confluence of the two rivers. We also noted those species with known migratory behavior. We were able to compare multiple unpublished data sets from several independent studies, carried out by different research teams working on local censuses, for different reasons, in either the blackwater or whitewater system, but that employed the same sampling protocol to census fish species in floodplain lakes. Further, these comparisons are from lakes in relatively close regional proximity, which reduces the potentially confounding factor of wider geographical variability within the basin (Bogotá-Gregory et al., 2020).
Fish sampling was conducted by independent teams of researchers at 16 lakes along the middle and lower reaches of the Rio Negro (seven lakes) and Rio Solimões (nine lakes) upstream of the confluence of these two rivers that forms the main channel of the Rio Amazonas (Figure 1). The lakes varied substantially in area and depth over the seasons of the hydrologic cycle, and except at low water include flooded forests at their margins, but the range of sizes (surface areas) of the whitewater and blackwater lakes we sampled did not differ between systems (Appendix 1).
Figure 1. Map of the floodplain region showing position of lakes that were sampled for fish species. The Rio Negro is the main blackwater river, flowing southeast toward the confluence with the Solimões, the main whitewater river. B, blackwater lakes; W, whitewater lakes. Numbers of lakes are in order of increasing distance from the confluence of the two rivers.
The fish assemblages of 16 Amazonian floodplain lakes were sampled by independent research groups from the Federal University of Amazonas (UFAM) and the National Institute of Amazonian Research (INPA) between 2005 and 2013 (Appendix 1). Nine of these were whitewater lakes located on the floodplain of the middle and lower reaches of the Rio Solimões, and seven were blackwater lakes along the Rio Negro (Figure 1).
All lakes in this combination of studies were typical of Amazonian floodplain lakes, and were sampled during both high and low water seasons of the hydrologic cycle for between 1 and 8 years (Appendix 1). The sampling gear consisted of floating gillnets of standardized dimensions: 20 m long by 2 m high (deep), with mesh sizes of 30, 40, 50, 60, 70, 80, 90, 100, 110, and 120 mm between opposite knots of the stretched mesh. Although samplings were carried out by independent groups, all sampling events proceeded according to the same protocol: gillnets were deployed at 0600 (daybreak) and left for 48 h at each collection site, and fish were collected from them every 6 h to minimize the predation effect (mainly foraging by piranha) on entangled fishes. Floodplain lake habitats have consistent sub-habitats: open water, floating macrophyte meadows (in whitewater; they do not occur in blackwater), and the flooded forest at the margins of the lakes except at low water (Siqueira-Souza et al., 2016). Therefore, gillnets were set at each of these sub-habitats during every sampling period. Fish collecting for all these studies was done under licenses 16889-2, 30052-1, 34446-3, and 50662-1 (Instituto Chico Mendes de Conservação da Biodiversidade–ICMBio/Brazil).
Because the lakes in our combined data set were not all sampled for the same number of years, owing to having come from different local studies that were not initially related to each other, the question arises of whether the data are biased because of potential variability in species composition between successive years. We saw no indication of this in any of our multiple-year data sets. In fact, although fish are free to move in and out of lakes during high water, which could result in random assortment among lakes, other researchers, e.g., Petry et al. (2003); Arrington and Winemiller (2006), and Fernandes et al. (2014) have noted non-random species composition, indicating affinity for a specific habitat that may qualify some regional species distributions as metapopulations (Hurd et al., 2016). Stability of species assemblages is even true for small temporary pools, and the variation in species composition declines with increasing volume of water (Espírito-Santo and Zuanon, 2017).
We constructed a rarefaction curve for each lake. Rarefaction gives us an expected number of species from a small sample drawn at random from a larger pool of individuals (Gotelli and Colwell, 2001), so the curve generated by plotting accumulated numbers of species against number of individuals sampled tells us how close we have come to representing most of the species actually present in the lake (i.e., α-diversity). Since we were specifically interested in species richness rather than relative abundance, it was important not to exclude rare species from our analyses.
We investigated the potential importance of lake size, distance between each lake and the confluence of Rios Negro and Solimões and environmental variables such as conductivity, dissolved oxygen, pH, temperature, and transparency on the taxonomic structure of local fish assemblages using Redundancy Analysis (RDA) (Ter Braak and Verdonschot, 1995). The number of fish belonging to each taxon was Hellinger-transformed prior to RDA. We tested for significance using a Monte-Carlo permutation test with 999 random permutations under the null model of no effect.
We used Moran’s I statistic (Fortin et al., 1989) to test the spatial correlation between species richness and the geographical distance between sampling sites and the confluence of Solimões and Negro River for both systems. This procedure was repeated using known migratory species richness and the same geographical distances. To test the null hypothesis that there is no influence of the geographic distance on the fish diversity we performed generalized linear models based on Poisson probability distribution (Zuur et al., 2009) using the number of species as response variable (Yi) and geographic distance between the sampled lake and the confluence of the two rivers as predictor variable (Xi). This procedure was carried out for the lakes of both systems separately.
Given the possibility that migratory species might be able to move between the two drainage systems, thus complicating the assignment of “home” habitat, we also performed generalized linear models based on Poisson probability distribution (Zuur et al., 2009) using the number of known migratory species (Appendix 2) as response variable (Yi) and geographic distance between the sampled lake and the confluence of the two rivers as predictor variable (Xi):. This procedure was carried out for the lakes of both systems separately.
All statistical analyses were carried out using R Statistical Software (R Development Core Team, 2012). RDA were done with Vegan package (Oksanen et al., 2010), GLMs were fitted using the CAR (Fox and Weisberg, 2011) and MASS packages (Ripley et al., 2013) and Moran’s I estimates were calculated using the APE package (Paradis et al., 2004).
Rarefaction curves were asymptotic, showing that the sampling effort was adequate to represent the bulk of pelagic fish species richness (Appendix 3). Fish community composition (Appendix 4) revealed that pelagic species richness was nearly identical between blackwater lakes (total S = 160) and whitewater lakes (S = 161) although there were substantial differences in species composition between the systems. Mean overall abundance of fish in blackwater lakes (1,487.28) also was not significantly different than of whitewater lakes (1,890.11) (t14 = -0.314, P = 0.723). Almost the same number of species were unique (unshared between systems) to each system: 74 or 31.6% in whitewater lakes; 73 or 31.2% in blackwater, which is reasonably consistent with overall endemism for the region (Reis et al., 2016). Thus, there were more unshared (147) than shared (87) species between these two systems, so that combined (regional) species richness was 234, substantially higher than if all species had been found in both systems (Appendix 4).
The RDA resulted in R2 = 0.666 with two significant axes [RDA1: F = 7.132, Pr(>F) = 0.01 and RDA2: F = 3.811, Pr(>F) = 0.005]. A gradient was strongly associated with axis RDA1 and indicates that species composition of lakes differed between the two systems, with blackwater lakes to the left of whitewater lakes in Figure 2. Blackwater lakes appear less homogeneous (greater β-diversity) than whitewater lakes on axis RDA2, and showed a pattern where lakes nearer the confluence of the two systems are on the lower side of the graph, and the lakes located more distant are on the upper side (see Figure 1 for position of lakes). This pattern is not as evident in whitewater lakes, although the lakes W8 and W9, located more distant of the confluence, also are on the upper side of the RDA plot. The key environmental variables that ordered the type of each lake by its fish species richness were conductivity, dissolved oxygen and pH, whereas transparency and distance from the confluence between Negro and Solimões rivers showed only a slight effect and temperature and lake area showed no effect (Table 1). Conductivity and pH were directly associated with whitewater lakes while dissolved oxygen, transparency and distance from the confluence between Negro and Solimões rivers clustered the blackwater lakes (Figure 2).
Figure 2. Redundancy Analysis (RDA) Biplot showing associations of assemblage strictures in terms of taxonomic composition by river system, blackwater system (blue circles), and whitewater system (red circles) and environmental variables, where: cond, electric conductivity; DO, dissolved oxygen; dist, distance among lakes and the confluence between Negro and Solimões rivers; tran, transparency; pH, pH.
Table 1. Results of Redundancy Analyses (RDA) for species richness and environmental variables representative of lakes in both the Negro and Solimões River drainage systems.
The null hypothesis that there is no spatial correlation between the total number of fish species and the geographic distance between the sampling sites and the confluence of Negro and Solimões rivers was rejected for the blackwater Negro River (P = 0.013) and for the whitewater Solimões River lakes (P = 0.014). Fish species richness within lakes (α diversity) declined as a function of geographic distance from the confluence of the rivers in both whitewater and blackwater (Table 2 and Figure 3).
Table 2. Results of generalized linear models between total species richness and geographic distance to the confluence of the Negro and Solimões River drainage systems.
Figure 3. Species richness in each lake (α) plotted against distance of the lake to the confluence of the two drainage systems. Lines are eye-fit just to emphasize decline in α with distance from the confluence of the river.
The total number of known migratory fish species among our collections was 40 in whitewater and 38 in blackwater, with 11.6% of migrating species unique to whitewater and 7.0% unique to blackwater (Appendix 2). As with total richness, the null hypothesis that there is no spatial correlation between the number of migratory species and the geographic distance between the sampling sites and the confluence of Negro and Solimões rivers was rejected for the blackwater Negro River lakes (P = 0.004) and for the white water Solimões River (P = 0.036). As with total richness, the number of migratory species within lakes declined as a function of geographical distance from the confluence (Table 3 and Figure 4).
Table 3. Results of generalized linear models between migratory species richness and geographic distance to the confluence of the Negro and Solimões River drainage systems.
Figure 4. Migratory species richness in each lake (α) plotted against distance of the lake to the confluence of the two drainage systems. Lines are eye-fit just to emphasize decline in α with distance from the confluence of the river.
Pelagic fish species diversity of these floodplain lakes, measured as total species richness and as mean richness/lake, was essentially equivalent. This was also true of mean fish abundance per lake between systems. The proportion of species unique to either system was close to the estimated percentage of endemic species for the entire Amazon Basin, and also considerably boosted regional species richness higher than was found in either system separately.
The pattern of diversity increasing toward the confluence of the rivers in both systems, is consistent with most earlier reports, e.g., Vannote et al. (1980) and Fernandes et al. (2004), but see Oberdorff et al. (2019) for the opposite result on a larger scale. Initially, we wondered if migratory species would move between systems and thus be counted in both systems. However, the general trend for migrants is to move from the lakes of either system to the rivers of the same system to breed as the water recedes from the floodplains, and then to return to the lakes to feed in the flooded forest during high water, while their juveniles drift from the river to the floodplain (Fernandes, 1997; Araújo-Lima and Ruffino, 2003). The many present-day migratory fish of the Amazon River Basin have some of the longest migratory routes of any freshwater fish. For instance, the catfish Brachyplatystoma rousseauxii migrates approximately 5,500 km from the Atlantic estuary of the Amazon westward to the Andean headwaters to breed (Vásquez et al., 2009; Duponchelle et al., 2016, 2021; Barthem et al., 2017; Hauser et al., 2020). Thus, migratory species should be good candidates for dispersal among different river systems. However, there are many Amazonian fish species for which migratory behavior is not yet known (Appendix 2), and the many variables associated with migratory behavior are just beginning to be analyzed (Alò et al., 2020). Further, there is still the question of how non-migratory species, many of which are shared between the two systems, got from one to the other.
The fact that different physical environmental variables were associated in RDA with species assemblages in lakes of the two systems (conductivity ad pH in whitewater; transparency and dissolved oxygen in blackwater) lacks a mechanistic explanation at present, and probably requires physiological investigation, especially of species that occur in only one of the two drainage systems. Floodplain lakes situated where the two river systems meet are open to species from both blackwater and whitewater rivers, and exhibit high variation in physical conditions (Röpke et al., 2016) that might be good transitional zones for cross colonization. This could result in higher species diversity close to the confluence. However, it also has been suggested that the substantial differences in water chemistry, such as pH and conductivity, are barriers to cross migration between systems due to chemical intolerance, particularly from the whitewater to more acid blackwater (Farias and Hrbek, 2008; Lima and Ribeiro, 2011).
Components of the floodplain landscape, including terrestrial wetland shrubs that are rooted vegetation and more permanent than floating macrophyte meadows, also have been proposed as important to maintenance of floodplain fish diversity (Freitas et al., 2018). Other studies have demonstrated that the forest cover bordering Amazonian floodplain lakes is a determinant of both the taxonomic and functional diversity of fish assemblages (Arantes et al., 2017, 2019), which indicates that the overall floodplain landscape exerts an important influence on fish diversity (Lobón-Cerviá et al., 2015; Freitas et al., 2018). Another factor is connectivity among habitats, which can stabilize regional populations and preserve diversity by inter-habitat migration and metapopulation dynamics (Arrington and Winemiller, 2006; Miyazono et al., 2010; Hurd et al., 2016).
We hope that our results will stimulate more studies on regional spatial scales, including a comparison among lakes between lakes upstream from the merging of the Solimões and Negro rivers with those downstream lakes bordering the main Amazon channel after they merge. This will require teams of researchers using the same sampling techniques concurrently in widely separated portions of the Basin. DNA analysis should allow us to compare rates and trends in speciation (Pyron and Burbrink, 2013) between the two systems. Molecular genetics has already given us fresh insight on phylogenetic trends and biogeography of some important Amazonian fishes (Farias and Hrbek, 2008; Lovejoy et al., 2010; López-Fernández et al., 2010; Lima and Ribeiro, 2011). We also need much more basic biological information for many species, such as well-defined trophic niche positions that would allow us to calculate functional diversity, and more complete information on life cycles, migratory behavior, physiological responses to water chemistry, and biogeographic distribution.
We identified several patterns in our data that reveal both similarities and differences between pelagic fish assemblages of whitewater and blackwater floodplains in the sampled region of the central Amazon River Basin of Brazil. Overall, there were surprisingly more similarities than differences.
Similarities: total number of species caught, number of shared, and unshared species (more shared than unshared between systems), number of migrating species, abundance of fish, and the decline in both α-diversity and number of migrating species with upstream distance from the confluence of the Rios Negro and Solomões.
Differences: heterogeneity of fish assemblages (β-diversity) was lower among whitewater lakes, and fish diversity was associated with different physical factors in the two systems: conductivity and pH in whitewater, and dissolved oxygen and transparency in blackwater.
Information from comparative sampling of fish in different areas and drainage systems can be important to efforts at conservation. Designing intelligent strategies for conserving dwindling fishery stocks and preserving the remaining biodiversity of the Amazon Basin may depend in part on recognition that the different systems and spatially separated areas within them have different vulnerabilities. For instance, deforestation will reduce allochthonous sources of food that are crucial to blackwater fish, depressing β diversity among lakes (Arantes et al., 2017, 2019), but may be less important to species living in productive whitewater. On the other hand, upriver damming will fragment migratory routes of some species in both systems (Barthem et al., 1991). Damming also may negatively affect whitewater floodplains more than in blackwater because in addition to reducing migration it will reduce sediment flow and prevent nutrients that support both aquatic and terrestrial productivity from reaching the lowland wetlands of the Central Amazon (Forsberg et al., 2017).
The original contributions presented in the study are included in the article/Supplementary Material, further inquiries can be directed to the corresponding author.
Ethical review and approval was not required for the animal study because the license to the samplings included a description of the fish catches to support the ethical requests.
FS-S, KY, and MS collected the data. FS-S, CF, LH, GC, and JK designed the study and methodology. CF and LH performed statistical analysis. All authors contributed to writing of the manuscript and approved the submitted.
Fish sampling for the various studies from which we compiled the data were supported by: CNPq (Grant 563073/2010-1); INCT Adapta; CF was funded by CNPq (Grant 302807/2015-2); CF, FS-S, KY, and MS were funded by FINEP (PIATAM Project); LH was funded by a Lenfest grant from Washington and Lee University, and by the Herwick Professorship Endowment. GC was also funded by a Lenfest grant. JK was funded by a Lenfest, by the Hendon Professorship Endowment, and through a fellowship from the CAPES (Grant 3344/2013).
The authors declare that the research was conducted in the absence of any commercial or financial relationships that could be construed as a potential conflict of interest.
All claims expressed in this article are solely those of the authors and do not necessarily represent those of their affiliated organizations, or those of the publisher, the editors and the reviewers. Any product that may be evaluated in this article, or claim that may be made by its manufacturer, is not guaranteed or endorsed by the publisher.
The Supplementary Material for this article can be found online at: https://www.frontiersin.org/articles/10.3389/fevo.2021.602895/full#supplementary-material
Albert, J. S., Tagliacollo, V. A., and Dagosta, F. (2020). Diversification of Neotropical freshwater fishes. Ann. Rev. Ecol. Evol. Syst. 51, 27–53.
Allen, G., and Pavelsky, T. M. (2018). Global extent of rivers and streams. Science 362, 585–588. doi: 10.1126/science.aat0636
Alò, D., Lacy, S. N., Castillo, A., Samangiego, H. A., and Marquet, P. A. (2020). The macroecology of fish migration. Global Ecol. Biogeogr. 30, 99–116. doi: 10.1111/geb.13199
Arantes, C. C., Winemiller, K. O., Asher, A., Castello, L., Hess, L. L., Petrere, M., et al. (2019). Floodplain land cover affects biomass distribution of fish functional diversity in the Amazon River. Sci. Rep. 9:16684.
Arantes, C. C., Winemiller, K. O., Petrere, M., Castello, L., Hess, L. L., and Freitas, C. E. C. (2017). Relationships between forest cover and fish diversity in the Amazon River floodplain. J. Appl. Ecol. 55, 386–395. doi: 10.1111/1365-2664.12967
Araújo-Lima, C. A. R. M., and Ruffino, M. L. (2003). “Migratory fishes of the Brazilian Amazon,” in Migratory Fishes of South America: Biology, Fisheries and Conservation Status Montreal, eds J. Carolsfeld, B. Harvey, C. Ross, and A. Baer (Canada: World Fisheries Trust).
Arrington, D. A., and Winemiller, K. O. (2006). Habitat affinity, the seasonal flood pulse, and community assembly in the littoral zone of a Neotropical floodplain river. J. North Am. Benthol. Soc. 25, 126–141. doi: 10.1899/0887-3593(2006)25[126:hatsfp]2.0.co;2
Barthem, R. B., Goulding, M., Leite, R. G., Cañas, C., Forsberg, B. R., Venticinque, E., et al. (2017). Goliath catfish spawning in the far western Amazon confirmed by the distribution of mature adults, drifting larvae and migrating juveniles. Sci. Rep. 7:e41784.
Barthem, R. B., Ribeiro, M. C. L. B., and Petrere, M. (1991). Life strategies of some long distance migratory catfish in relation to hydroelectric dams in the Amazon Basin. Biol. Conserv. 55, 339–345. doi: 10.1016/0006-3207(91)90037-a
Bogotá-Gregory, J. D., Lima, F. C. T., Correa, S. B., Silva-Oliveira, C., Jenkins, D. G., Ribeiro, F. R., et al. (2020). Biogeochemical water type influences community composition, species richness, and biomass in megadiverse Amazonian fish assemblages. Sci. Rep. 10:15349.
Campos, C. P., Garcez, R. S., Catarino, M. F., Costa, G. A., and Freitas, C. E. C. (2015). Population dynamics and stock assessment of Colossoma macropomum caught in the Manacapuru lake system (Amazon Basin. Brazil). Fish. Manag. Ecol. 22, 400–406. doi: 10.1111/fme.12139
Castanho, D. G. R., de Deus, C. P., Zuanon, J., Santorelli, S., Leitão, R. P., and Teresa, F. B. (2019). Simulation of over-exploitation of ornamental fish and its consequences for the functional structure of assemblages of Amazonian streams. Ecol. Freshw. Fish 29, 533–541. doi: 10.1111/eff.12524
Castello, L., McGrath, D. G., Hess, L. L., Coe, M. T., Lefevre, P. A., Petry, P., et al. (2013). The vulnerability of Amazon freshwater ecosystems. Conserv. Lett. 6, 217–229. doi: 10.1111/conl.12008
Costa-Pereira, R., and Galetti, M. (2017). Frugivore downsizing and the collapse of seed dispersal by fish. Biol. Cnserv. 191, 809–811. doi: 10.1016/j.biocon.2015.07.011
Crampton, W. G. R. (2011). “An ecological perspective on diversity and distributions,” in Historical Biogeography of Neotropical Freshwater Fishes, eds J. S. Albert and R. E. Reis (California: University of California Press), 165–189. doi: 10.1525/9780520948501-012
Dagosta, F. C. P., and Pinna, M. D. (2019). The Fishes of the Amazon: distribution and biogeographical patterns, with a comprehensive list of species. Bull. Am. Museum Nat. History 431, 1–163. doi: 10.1206/0003-0090.431.1.1
Duponchelle, F., Isaac, V., Doria, C., and Herrera-R, G. A. (2021). Conservation of migratory fishes in the Amazon basin. Aquatic Conserv. Mar. Freshw. Ecosyst. 31, 1087–1105. doi: 10.1002/aqc.3550
Duponchelle, F., Pouilly, M., Pecheyran, C., Hauser, M., Renno, J. R., Panfil, J., et al. (2016). Trans-Amazonian natal homing in giant catfish. J. Appl. Ecol. 53, 1511–1520. doi: 10.1111/1365-2664.12665
Espírito-Santo, H. M. V., and Zuanon, J. A. (2017). Temporary pools provide stability to fish assemblages in Amazon headwater streams. Ecol. Freshw. Fish 26, 475–483. doi: 10.1111/eff.12292
Farias, I. P., and Hrbek, T. (2008). Patterns of diversification in the discus fishes (Symphysodon spp. Cichlidae) of the Amazon basin. Mol. Phylogenet. Evol. 49, 32–43. doi: 10.1016/j.ympev.2008.05.033
Fernandes, C. C. (1997). Lateral migrations of fishes in Amazon floodplains. Ecol. Freshw. Fish 2, 36–44. doi: 10.1111/j.1600-0633.1997.tb00140.x
Fernandes, C. C., Podos, J., and Lundberg, J. G. (2004). Amazonian ecology: tributaries enhance the diversity of electric fishes. Science 305, 1960–1962. doi: 10.1126/science.1101240
Fernandes, I. M., Henriques-Silva, R., Penha, J., Zuanon, J. A., and Peres-Neto, P. R. (2014). Spatiotemporal dynamics in a seasonal metacommunity structure is predictable: the case of floodplain-fish communities. Ecography 37, 464–475.
Finer, M., Jenkins, C. N., Pimm, S. L., Keane, B., and Ross, C. (2008). Oil and gas projects in the Western Amazon: threats to wilderness, biodiversity, and indigenous peoples. PLoS One 3:e2932. doi: 10.1371/journal.pone.0002932
Forsberg, B. R., Melack, J. M., Dunne, T., Barthem, R. B., Goulding, M., Paiva, R. C. D., et al. (2017). The potential impact of new Andean dams on Amazon fluvial ecosystems. PLoS One 12:e0182254. doi: 10.1371/journal.pone.0182254
Fortin, M. J., Drapeau, P., and Legendre, P. (1989). Spatial autocorrelation and sampling design in plant ecology. Vegetatio 83, 209–222. doi: 10.1007/bf00031693
Fox, J., and Weisberg, S. (2011). An {R} Companion to Applied Regression, 2nd Edn. Thousand Oaks: SAGE Publications.
Freitas, C. E. C., Laurenson, L., Yamamoto, K. C., Forsberg, B. R., Petrere, M., Arantes, C., et al. (2018). Fish species richness is associated with the availability of landscape components across seasons in the Amazonian floodplain. PeerJ 6:e5080. doi: 10.7717/peerj.5080
Freitas, C. E. C., Siqueira-Souza, F. K., Florentino, A. C., and Hurd, L. E. (2014). The importance of spatial scales to analysis of fish diversity in Amazonian floodplain lakes and implications for conservation. Ecol. Freshw. Fish 23, 470–477. doi: 10.1111/eff.12099
Freitas, C. E. C., Siqueira-Souza, F. K., Humston, R., and Hurd, L. E. (2013). An initial assessment of drought sensitivity in Amazonian fish communities. Hydrobiologia 705, 159–171. doi: 10.1007/s10750-012-1394-4
Furch, K. (1984). “Water chemistry of the Amazon basin: the distribution of chemical elements among freshwaters,” in The Amazon: Limnology and Landscape Ecology of a Mighty Tropical River and its Basin, ed. H. Sioli (Berlin: Springer), 167–199. doi: 10.1007/978-94-009-6542-3_6
Gotelli, N. J., and Colwell, R. K. (2001). Quantifying biodiversity: procedures and pitfalls in the measurement and comparison of species richness. Ecol. Lett. 4, 379–391.
Hauser, M., Duponchelle, F., Hermann, T. W., Limbrug, K. E., Castello, L., Stewart, D. J., et al. (2020). Unmasking continental natal homing in goliath catfish from the upper Amazon. Freshw. Biol. 65, 325–336. doi: 10.1111/fwb.13427
Hess, L. L., Melack, J. M., Affonso, A. G., Barbosa, C., Gastil-Buhl, M., and Novo, E. M. (2015). Wetlands of the lowland Amazon basin: extent, vegetative cover, and dual-season inundated area as mapped with JERS-1 synthetic aperture radar. Wetlands 35, 745–756. doi: 10.1007/s13157-015-0666-y
Horn, M. H., Correa, S. B., Parolin, P., Pollux, P. J. A., Anderson, J. T., Lucas, C., et al. (2011). Seed dispersal by fishes in tropical and temperate fresh waters: the growing evidence. Acta Oecol. 37, 561–577. doi: 10.1016/j.actao.2011.06.004
Hurd, L. E., Sousa, R. G. C., Siqueira-Souza, F. K., Cooper, G. J., Kahn, J. R., and Freitas, C. E. C. (2016). Amazon floodplain fish communities: habitat connectivity and conservation in a rapidly deteriorating environment. Biol. Conserv. 195, 118–127. doi: 10.1016/j.biocon.2016.01.005
Junk, W. J., and Piedade, M. T. F. (1997). “Plant life in the floodplain with special reference to herbaceous plants,” in The Central Amazon Floodplain: Ecology of a Pulsing System, ed. W. J. Junk (Berlin: Springer-Verlag), 147–186. doi: 10.1007/978-3-662-03416-3_8
Junk, W. J., Bayley, P. B., and Sparks, R. E. (1989). “The flood pulse concept in river-floodplain systems,” in Proceedings of the International Large River Symposium (LARS), ed. D. P. Doge (Otawwa: Canadian Special Publication of Fishery and Aquatic Sciences), 110–127.
Junk, W. J., Piedade, M. T. F., Schöngart, J., Cohn-Haft, M., Adeney, J. M., and Wittmann, F. (2011). A classification of major naturally-occuring Amazonian lowland wetlands. Wetlands 31, 623–640. doi: 10.1007/s13157-011-0190-7
Junk, W. J., Soares, M. G. M., and Saint-Paul, U. (1997). “The fish,” in Ecology of a Pulsing System, ed. W. J. Junk (Berlin: Springer), 385–408.
Kahn, J. R., Freitas, C. E. C., and Petrere, M. (2014). False shades of green: the case of Brazilian Amazonian hydropower. Energies 7, 6063–6082. doi: 10.3390/en7096063
Latini, A. O., and Petrere, M. (2004). Reduction of a native fish fauna by alien species: an example from Brazilian freshwater tropical lakes. Fish. Manag. Ecol. 11, 71–79. doi: 10.1046/j.1365-2400.2003.00372.x
Lima, F. C. T., and Ribeiro, A. C. (2011). “Continental-scale tectonic controls of biogeography and ecology,” in Historical Biogeography of Neotropical Freshwater Fishes, eds J. S. Albert and R. E. Reis (California: University of California Press), 145–164. doi: 10.1525/9780520948501-011
Lobón-Cerviá, J., Hess, L. L., Melack, J. M., and Araujo-Lima, C. A. R. M. (2015). The importance of forest cover for fish richness and abundance on the Amazon floodplain. Hydrobiologia 750, 245–255. doi: 10.1007/s10750-014-2040-0
Loebens, S. C., Farias, E. U., Freitas, C. E. C., and Yamamoto, K. C. (2019). Influence of hydrological cycle on the composition and strucuture of fish assemblages in an igapó forest, Amazonas, Brazil. Boletim do Instituto de Pesca 45, e432.
Lovejoy, N. R., Willis, S. C., and Albert, J. S. (2010). “Molecular signatures of Neogene biogeographical events in the Amazon fish fauna,” in Amazonia: Landscape and Species Evolution: a Look into the Past, eds C. Hoorn and F. P. Weselingh (Oxford: Blackwell), 405–417. doi: 10.1002/9781444306408.ch25
López-Fernández, H., Winemiller, K. O., and Honeycutt, R. L. (2010). Multilocus phylogeny and rapid radiations in Neotropical cichlid fishes (Perciformes: Cichlidae: Cichlinae). Mol. Phylogenet. Evol. 55, 1070–1086. doi: 10.1016/j.ympev.2010.02.020
Merona, B., de, and Gascuel, D. (1993). The effects of flood regime and fishing effort on the overall abundance of an exploited fish community in the Amazon floodplain. Aquat. Living Resour. 6, 97–108. doi: 10.1051/alr:1993010
Miyazono, S., Aycock, J. N., Miranda, L. E., and Tietjen, T. E. (2010). Assemblage patterns of fish functional groups relative to habitat connectivity and conditions in floodplain lakes. Ecol. Freshw. Fish 19, 578–585. doi: 10.1111/j.1600-0633.2010.00438.x
Nazareno, A. G., and Laurance, W. F. (2015). Brazil’s drought: beware deforestation. Science 347:1427. doi: 10.1126/science.347.6229.1427-a
Oberdorff, T., Dias, M. S., Jézéquel, C., Albert, J. S., Arantes, C. C., Bigorne, R., et al. (2019). Unexpected fish diversity gradients in the Amazon basin. Sci. Adv. 5:eaav8681. doi: 10.1126/sciadv.aav8681
Oksanen, J., Blanchet, F. G., Friendly, M., Kindt, R., Legendre, P., and McGlinn, D. (2010). Vegan: Community Ecology Package. R Package Version 1.17-6.
Paradis, E., Claude, J., and Strimmer, K. (2004). APE: analysis of phylogenetics and evolution in R language. Bioinformatics 20, 289–290. doi: 10.1093/bioinformatics/btg412
Petry, P., Bayley, P. B., and Markle, D. F. (2003). Relationships between fish assemblages, macrophytes and environmental gradients in the Amazon River floodplain. J. Fish Biol. 63, 547–579. doi: 10.1046/j.1095-8649.2003.00169.x
Pyron, A. A., and Burbrink, F. T. (2013). Phylogenetic estimates of speciation and extinction rates for testing ecological and evolutionary hypotheses. Trends Ecol. Evol. 28, 729–736. doi: 10.1016/j.tree.2013.09.007
R Development Core Team (2012). R: a Language and Environment for Statistical Computing. Vienna: R Foundation for Statistical Computing.
Reis, R. E., Albert, J. S., Di Dario, F., Mincarone, M. M., Petry, P., and Rocha, L. A. (2016). Fish biodiversity and conservation in South America. J. Fish Biol. 89, 12–47. doi: 10.1111/jfb.13016
Ripley, B., Venables, B., Hornik, K., Gebhardt, A., and Firth, D. (2013). MASS—Modern Applied Statistics with S. 4. Available Online at: http://www.stats.ox.ac.uk/pub/MASS (accessed September 4, 2019).
Röpke, C. P., Amadio, S. A., Winemiller, K. O., and Zuanon, J. (2016). Seasonal dynamics of the fish assemblage in a floodplain lake at the confluence of the Negro and Amazon Rivers. J. Fish Biol. 89, 194–212 doi: 10.1111/jfb.12791
Saint-Paul, U., Zuanon, J., Correa, M. A. V., Garacia, M., Fabré, N. N., Berger, U., et al. (2000). Fish communities in central Amazonian white- and blackwater floodplains. Environ. Biol. Fishes 57, 235–250. doi: 10.1023/a:1007699130333
Santos, R. E., Pinto-Coelho, R. M., Fonseca, R., Simões, N. R., and Zanchi, F. B. (2018). The decline of fisheries on the Madeira River, Brazil: the high cost opf the hydroelectric dams in the Amazon Basin. Fish. Manag. Ecol. 25, 380–391. doi: 10.1111/fme.12305
Scarabotti, P. A., López, J. A., and Pouilly, M. (2011). Floodpulse and the dynamics of fish assemblage structure from Neotropical floodplain lakes. Ecol. Freshw. Fish 20, 605–618. doi: 10.1111/j.1600-0633.2011.00510.x
Silva, P. B., Arantes, C. C., Freitas, C. E. C., Petrere, M., and Ribeiro, F. R. V. (2020). Seasonal hydrology and fish assemblage structure in the floodplain of the lower Amazon river. Ecol. Freshw. Fish. 30, 162–173. doi: 10.1111/eff.12572
Siqueira-Souza, F. K., Freitas, C. E. C., Hurd, L. E., and Petrere, M. (2016). Amazon floodplain fish diversity at different scales: do time and place really matter? Hydrobiologia 776, 99–110. doi: 10.1007/s10750-016-2738-2
Ter Braak, C. J. F., and Verdonschot, P. F. M. (1995). Canonical correspondence analysis and related multivariate methods in aquatic ecology. Aquat. Sci. 57, 255–289. doi: 10.1007/bf00877430
Tregidgo, D. J., Barlow, J., Pompeu, P. S., Rocha, M. D. A., and Parry, L. (2017). Rainforest metropolis casts 1,000-km defaunation shadow. Proc. Nat. Acad. Sci. U. S. A. 114, 8655–8659. doi: 10.1073/pnas.1614499114
Vannote, R. L., Minshall, G. W., Cimmins, K. W., Sedell, J. R., and Cushing, C. E. (1980). The river continuum concept. Can. J. Fish. Aquat. Sci. 37, 130–137.
Vásquez, A. G., Alonso, J. C., Carvajal, F., Moreau, J., Nuñez, J., Renno, J. F., et al. (2009). Life-history characteristics of the large Amazonian migratory catfish Brachyplatystoma rousseauxii in the Iquitos region. Peru. J. Fish Biol. 75, 2527–2551. doi: 10.1111/j.1095-8649.2009.02444.x
Keywords: Amazon Basin, fish conservation, fish species diversity, floodplain lakes, tropical biodiversity
Citation: Siqueira-Souza FK, Hurd LE, Yamamoto KC, Soares MGM, Cooper GJ, Kahn JR and Freitas CEC (2021) Patterns of Pelagic Fish Diversity in Floodplain Lakes of Whitewater and Blackwater Drainage Systems Within the Central Amazon River Basin of Brazil. Front. Ecol. Evol. 9:602895. doi: 10.3389/fevo.2021.602895
Received: 04 September 2020; Accepted: 23 June 2021;
Published: 29 July 2021.
Edited by:
Roksana Majewska, North-West University, South AfricaReviewed by:
Luciano F. A. Montag, Federal University of Pará, BrazilCopyright © 2021 Siqueira-Souza, Hurd, Yamamoto, Soares, Cooper, Kahn and Freitas. This is an open-access article distributed under the terms of the Creative Commons Attribution License (CC BY). The use, distribution or reproduction in other forums is permitted, provided the original author(s) and the copyright owner(s) are credited and that the original publication in this journal is cited, in accordance with accepted academic practice. No use, distribution or reproduction is permitted which does not comply with these terms.
*Correspondence: Carlos E. C. Freitas, Y2VmcmVpdGFzQHVmYW0uZWR1LmJy; orcid.org/0000-0001-5406-0998
Disclaimer: All claims expressed in this article are solely those of the authors and do not necessarily represent those of their affiliated organizations, or those of the publisher, the editors and the reviewers. Any product that may be evaluated in this article or claim that may be made by its manufacturer is not guaranteed or endorsed by the publisher.
Research integrity at Frontiers
Learn more about the work of our research integrity team to safeguard the quality of each article we publish.