- Department of Biology, University of Nevada, Reno, Reno, NV, United States
As global land surfaces are being converted to urban areas at an alarming rate, understanding how individuals respond to urbanization is a key focus for behavioral ecology. As a critical component of avian parental care, incubating adults face a tradeoff between maintaining an optimal thermal environment for the developing embryos while meeting their own energetic demands. Urban habitats are biotically and abiotically different from their rural counterparts, i.e., in food availability, predator compositions, and the thermal environment. Therefore, urban birds may face different incubation challenges than their natural counterparts. We measured incubation behavior of rural and urban house wrens, Troglodytes aedon, with temperature loggers throughout the 12-day period. We found that urban females had more incubation bouts of shorter duration and spent less total time incubating per day than rural females. Results could provide evidence of behavioral shifts of wrens in cities, which have implications for the evolution of parental care. Our findings contribute to our understanding of the behavioral traits needed for city life and possible environmental pressures driving urban adaptations.
Introduction
The growth and spread of urban areas is one of the most extensive of all anthropogenic effects (Johnson and Munshi-South, 2017). Landscapes are rapidly changing to become more urbanized (Chen et al., 2020), and these effects are expected to exponentially increase as more than two-thirds of the world’s population is projected to live in urban areas by 2050 (United Nations, 2018). Urban environments present a unique set of challenges to wildlife which have led to changes in wildlife behavior, physiology, morphology, and fitness (Hall and Warner, 2018; Ouyang et al., 2019; Reynolds et al., 2019). Despite urban environmental challenges, some species continue to colonize and thrive in urban environments (urban exploiters; Sepp et al., 2018). Understanding how and why urban exploiters thrive in urban environments is a major goal in evolutionary ecology (Ouyang et al., 2018). Avian systems are ideal to study effects of urbanization, as their behavior is easily observed, they readily colonize new habitats, and can act as bioindicators of urban pollutants (Bonier et al., 2007; Zhang and Ma, 2011; Sol et al., 2013; Marzluff, 2017).
Behavioral flexibility and innovation represent the first line of defense against novel challenges (Mckinney, 2002, 2006; Shochat et al., 2006; Sih et al., 2011; Sol et al., 2013). Environmental alterations in cities, such as reduced and fragmented natural vegetation, novel building structures, human disturbance, new predator compositions, and elevated light, noise and heavy metal pollution, can all affect behavioral phenotypes of urban adapters (Gorissen et al., 2005; Shochat et al., 2010; Clucas and Marzluff, 2012; Sol et al., 2013; Seress and Liker, 2015). The urban heat island, for example, is a phenomenon in which cities are several degrees hotter than the natural environments from which they are built (Landsberg, 1981; Arnfield, 2003; Peng et al., 2012). Urban habitats may also have lower food availability for avian wildlife, especially in nutritious food for insectivorous birds (Seress et al., 2018, 2020; Baldan and Ouyang, 2020). Furthermore, predator compositions can vary across the urban-rural gradient and between artificial and natural nests (Rodewald and Kearns, 2011; Vincze et al., 2017; Eötvös et al., 2018). Studying behavioral differences between urban wildlife and their natural counterparts gives us the opportunity to understand the environmental pressures driving adaptations needed for urban living.
While wildlife have shifted life history traits to compensate for changes in their environments, important aspects of avian parental care that ensure embryonic development, such as incubation, may be affected as well. For eggs to successfully hatch, the egg-adult unit in contact incubation is critical (Deeming, 2002c). An essential aspect of avian parental care is behaviorally maintaining and creating a thermal environment suitable for offspring development (Skutch, 1962; Deeming, 2002b). Avian embryos require a narrow species-specific thermal range for optimal development in which any prolonged period outside the limits result in embryonic development abnormalities (reviewed in Durant et al., 2013). Even small variations in the thermal environment can influence offspring phenotypes, such as growth rate, immune function, and survival (Pérez et al., 2008; Ardia et al., 2010; Nord and Nilsson, 2011; Durant et al., 2013; Ospina, 2017; Merrill et al., 2020).
Furthermore, female-only incubation is especially constrained (Deeming, 2002b; Nord and Williams, 2015). The length and frequency of female incubation behavior are affected by ambient temperature, food availability, and presence of predators (Skutch, 1962; Conway and Martin, 2000a,b; Londoño et al., 2008). Seeing as these abiotic and biotic factors also differ between urban and rural areas, incubating adults should adjust their incubation strategies to maintain optimal embryonic development in the face of urban environmental changes. Behavioral adjustments in incubation, such as more and shorter bouts, can lead to reduced attentiveness and have been reported when adults are exposed to elevated ambient temperatures in both experimental (Ton et al., 2021) and field studies (Haftorn, 1979; Conway and Martin, 2000a; Londoño et al., 2008; Álvarez and Barba, 2014; McClintock et al., 2014; Amininasab et al., 2016; Batisteli et al., 2020). Elevated risk of predation has been shown to lengthen both incubation and recess bouts so as to decrease activity at the nest (Martin and Ghalambor, 1999; Conway and Martin, 2000b). Additionally, low food abundance may increase the energetic expense of foraging trips and result in more frequent and longer recesses (Conway and Martin, 2000b; Londoño et al., 2008). Therefore, the incubation life history stage requires the female to face a tradeoff between maintaining her own energetic needs, e.g., self-maintenance, and maintaining an optimal thermal environment during incubation (Conway and Martin, 2000a; Deeming, 2002a). With avian incubation tightly linked to various climactic conditions, these behaviors are critically sensitive to climate changes (Mainwaring, 2015).
It is likely that a variety of urban factors will affect incubation behaviors, leading to differences in offspring development and/or energy balance in the female. However, there are currently no studies that we are aware of that have investigated differences in incubation strategies between urban and non-urban birds. Here, we used thermal buttons in nests of both urban and rural house wrens (Troglodytes aedon) to test whether their strategies would differ and if these differences match the trends in their thermal environments. House wrens are small cavity nesters that readily use nest boxes in both urban and rural locations with female only incubation (Ouyang et al., 2019). Whether due to less food availability, increased thermal environment or decreased natural predators in the urban environment, we predicted that incubation bouts would be shorter in urban birds. Overall, we predicted that due to the combined factors of the urban environment, female wrens would spend less time on the nest incubating eggs compared to rural wrens.
Materials and Methods
Study Site Descriptions
This study was conducted from May to August 2019 at one urban location in Reno, NV, United States, and one rural location in Sparks, NV, United States. The urban site was a city park (39°30′3 ″N, 119°50′ 00″W) and the rural site was at the University of Nevada, Reno Agricultural Experimental Station, a university owned agricultural farm (39°30′ 50″N, 119°51′ 43″W). These two sites differed in urbanization score and environmental traits (see Baldan and Ouyang, 2020 for a detailed description of the field sites). Briefly, an urbanization score was estimated following the validated methods of Seress et al. (2014) in which land use was estimated by scoring vegetation abundance, building density, and paved surfaces from aerial images of each site composed of 100 × 100 m cells. The urban site has a higher urbanization score compared to the rural site with more cells containing increased building density and paved surfaces as well as decreased vegetation density (Baldan and Ouyang, 2020).
Incubation Behavior
We checked nest boxes daily to monitor nest building, egg laying, and initiation of incubation (x = 8 urban, 7 rural nests). On the first day of incubation, determined as the first day no further egg was laid and eggs felt warm to the touch, we placed an iButton temperature logger (Model Thermochron TCS, OnSolution, Baulkham Hills, New South Wales, Australia; accuracy: ±0.5°C, resolution: 0.5°C) within the center of the nest cup among the eggs. iButtons were placed in the same location in the nest cup and remained for the entire duration of incubation until chicks hatched. No iButtons were found moved or removed. We also measured ambient temperature at our sites using iButtons. Two ambient iButtons were attached to the outer underside of two nest boxes at each site evenly spaced out from one another across the site. We validated these temperatures with weather stations located near our sites. At the urban site, the weather station was 0.67 km from the location of our boxes (Weather Underground, Southwest Reno – KNVRENO251) and at the rural site, the weather station was located at the breeding site [Western Regional Climate Center – Desert Research Institute – Reno, Nevada, Sparks (UNR) Nevada].
Maternal incubation behavior was measured from a time series of temperature data collected by iButton temperature data loggers (Cooper and Mills, 2005). iButtons have been previously shown to accurately measure incubation and recess bouts of female incubation behavior (Nord and Cooper, 2019). Temperature loggers recorded a temperature measurement every 2 min throughout the entire 12-day incubation period.
We used the combination of both Rhythm and Raven Pro software programs (Cooper and Mills, 2005) to compute incubation bouts and duration while taking ambient temperatures into account. We analyzed daytime incubation behavior determined by daily sunrise and sunset times and included days 2 through 11 of the 12-day incubation period to ensure analysis of only full incubation days (there was no individual variation in incubation period: all birds had an incubation period of 12 days). The Rhythm program (1.1, Cooper and Mills, 2005) was used to identify incubation bouts and recesses considering a bout as a rise in nest temperature of more than 2°C with the rise in temperature trend lasting more than 2 min (Amininasab et al., 2016). We used the Raven Pro program (1.6, Cooper and Mills, 2005) to visually inspect the selected bouts and manually edit the bouts Rhythm selected or missed by either keeping selected bouts as is, extending the period to the observed start of recess, or deleting the selected bout. We normally observed a pattern in which a noticeable rise in temperature of an incubation bout was followed by some period of stability. Raven Pro plotted the ambient temperatures along with iButton incubation temperatures to more accurately determine when a female was on or off the nest. This data allowed us to calculate the number of incubation bouts per day, average incubation bout duration in minutes per day, and total minutes of incubation per day throughout the incubation period. This study was carried out in accordance with recommendations of the Institutional Animal Care and Use Committee of the University of Nevada, Reno.
Statistical Analysis
For statistical analyses, all models were run with R version 3.6.1 (R Core Team, 2019). Using the lme4 package, we performed a generalized linear mixed-effects model (GLMM) using the glmer function and linear mixed models (LMM) using the lmer function. We used the lmerTest package to report all p-values. All final models met assumptions of normality and homoscedasticity of residual errors. Significance was taken at α = 0.05. We used the cohen.d function in the effsize package to calculate all effect sizes. Females at the rural site started incubating at earlier Julian dates than urban females (coef = 0.1, s.e. = 0.05, z-value = 2.2, p = 0.03). Therefore, to avoid collinearity, we did not include Julian date in the models. We subsetted our data to include only data in which Julian dates overlapped at both sites and ensured that our reported results were consistent with this subset dataset.
For all models, we included the interaction of site and incubation day as a fixed effect and nest ID as a random effect. When the interaction was not significant, we removed the interaction term and tested the fixed effects independently. Including clutch size and egg mass was insignificant in all models and thus removed. In this study, neither clutch size nor egg mass differed between the urban and rural birds (clutch size: t = 1.4, df = 13.0, p = 0.2; egg mass: df = 21.7, t = −0.8, p = 0.4). Hatching success also did not differ between the urban and rural birds (t = −0.9, df = 12.2, p = 0.4). We used a GLMM with a Poisson distribution to test if the number of incubation bouts differed between the urban and rural site. A LMM was used to test the difference in average bout duration between the two sites. To meet linear model assumptions, we transformed average incubation duration, which was skewed to the right, by taking the reciprocal. We used a LMM to test if total daily incubation duration differed between the two sites. For this model, we removed two statistical outliers to ensure the statistical assumption of normality of residuals. We note that our results remain the same whether or not we include these outliers.
Results
We had complete incubation recordings (11 days per nest) for eight urban and seven rural nests. Urban females had more incubation bouts per day than rural females (Figure 1A and Table 1) with a large effect size (Cohen’s D = −1.52). Urban mothers had on average shorter incubation bouts than rural mothers (Figure 1B and Table 1) with a large effect size (Cohen’s D = −1.88). There was a significant interaction between site and day for average incubation duration (Table 1). As the incubation period progressed, urban females decreased average bout duration while rural females’ average bouts duration was maintained (Figure 1B). Additionally, rural females spent more total time incubating per day than urban females (Figure 1C and Table 1) with a large effect size (Cohen’s D = 1.51). There was a significant interaction between site and day for the total incubation duration (Table 1). Rural females increased total incubation duration as incubation period progressed but urban females did not (Figure 1C).
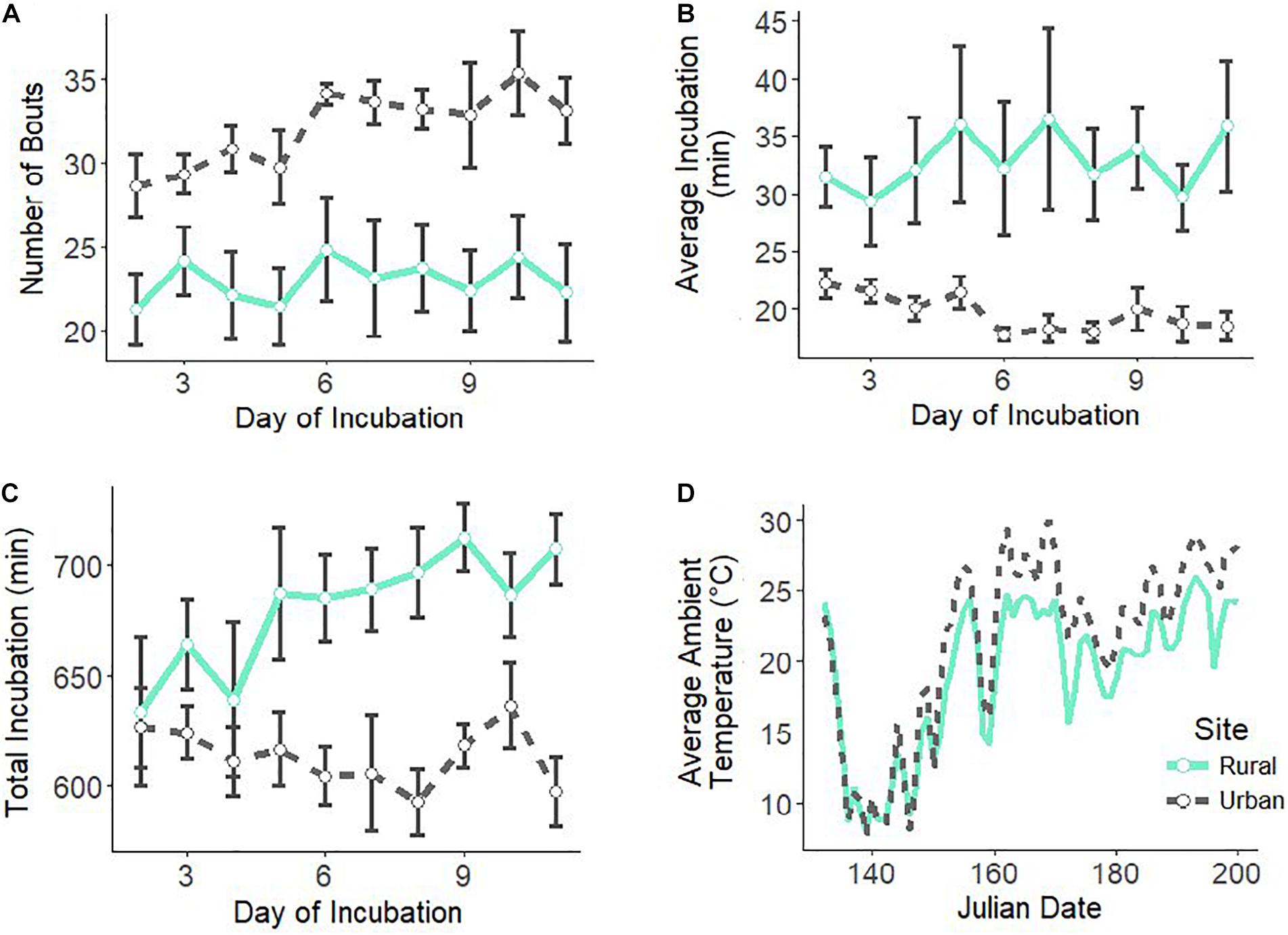
Figure 1. Female house wren incubation behaviors over the 11 days of incubation, including number of incubation bouts (A), average incubation bout duration (B), and total incubation duration (C). Ambient daytime temperatures are shown for both sites throughout the breeding season (D). Urban site and females are in black, dotted lines and rural site and females are in green, solid lines. Shown are means ± 1 SE.
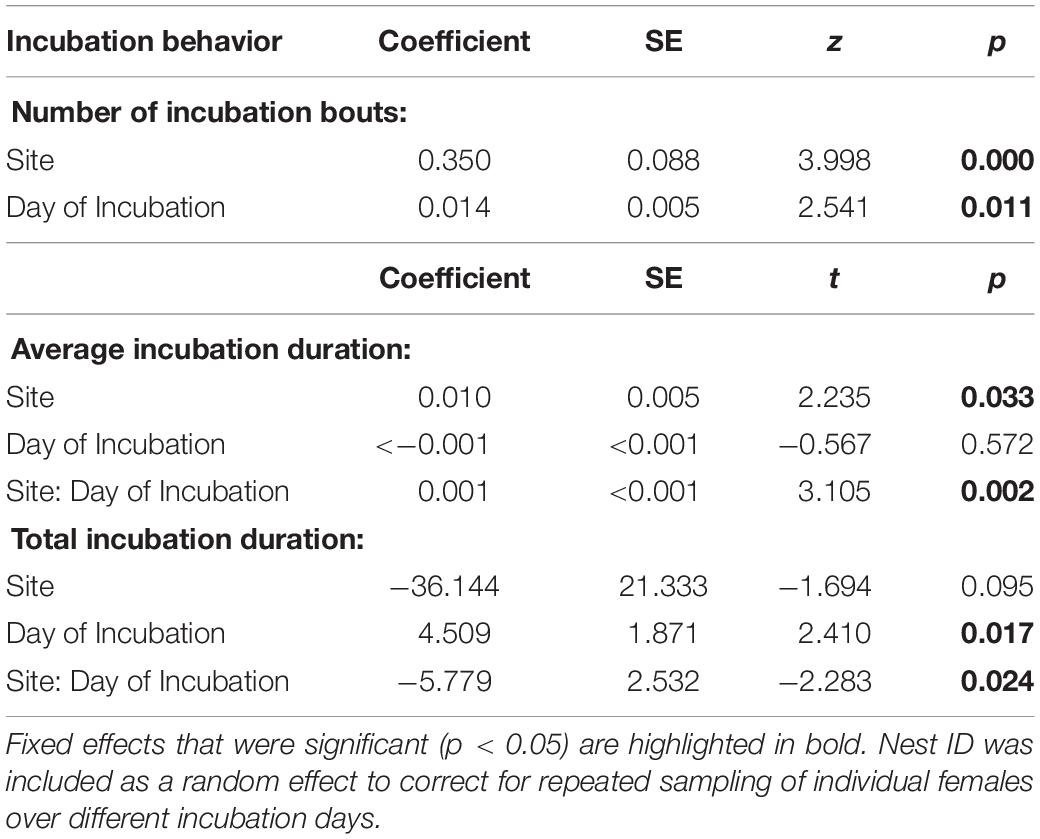
Table 1. Results from generalized linear mixed model (number of incubation bouts) and linear mixed models (average and total incubation duration) analyzing differences in incubation behavior between urban and rural house wren females.
Discussion
We measured incubation behavior of urban and rural house wrens to determine whether differences in the urban environment influenced parental behavior during incubation. We found that urban females had more and shorter daily incubation bouts leading to less total time spent incubating eggs over the entire incubation period. However, our study is limited in its lack of site replicates. Therefore, while the results are promising, we caution the extrapolation of our results to other urban and rural areas that may differ in environmental pressures.
Urban house wren females had a higher number of incubation bouts per day (on average nine more bouts per day), meaning females were on and off the eggs more often compared to rural females. Incubation is an extremely energetically costly aspect of parental care (Vleck, 1981; Thomson et al., 1998; Tinbergen and Williams, 2002) in which parents must cope with varying environmental conditions and adjust the balance between incubation and self-maintenance (Conway and Martin, 2000b; Nord and Williams, 2015). Cities often experience higher disturbance from increase traffic noise (Mulholland et al., 2018), human disturbance (Markovchick-Nicholls et al., 2008), and new predator compositions (Rodewald and Kearns, 2011), which all may force females to leave the nest more often.
Each incubation bout was also shorter in duration for urban females by on average 13 min. The “energetic-bottleneck” hypothesis states that incubation effort is dependent on energy availability acquired through self-maintenance (Yom-Tov and Hilborn, 1981). Therefore, female’s incubation effort can depend on a variety of factors including food availability, foraging efficiency, and amount of time allowed off the nest to forage. In species such as house wrens in which females incubate alone, but are provisioned by mates to some extent, incubation limits time for foraging (Skutch, 1962; Jones, 1987; Monaghan and Nager, 2002). Urban environments pose challenges of reduced nutritious food and limited food availability (Chamberlain et al., 2009), which is mirrored in this study (Baldan and Ouyang, 2020). If birds are faced with lower foraging efficiency and abundance of food in urban habitats, females may need to take shorter incubation bouts and longer foraging bouts to meet their own energetic demands (Conway and Martin, 2000b). Lack of predation risk can be another reason we see these differences (Thompson, 2007). While longer incubation and recess bouts would limit nest activity and decrease risk of predation (Conway and Martin, 2000b), urban birds may move on and off the nest more often with shorter bouts because they experience lower risk of predation in urbanized habitats (J Heppner per obs, Vincze et al., 2017; Eötvös et al., 2018).
As the number of shortened bouts increases, these behaviors in urban females all lead to a total shortened period of incubation by an average of 67 min. Temperature is one of the most critical aspects of successful incubation, and the urban heat island, a byproduct of urbanization in which cities have higher temperatures than surrounding natural lands, could have lasting effects. Our urban site had an average of 2.3°C higher temperatures than our rural site (Figure 1D). The majority of studies have reported that incubating adults respond to elevated temperatures by decreasing incubation effort due to reduced thermostatic demand (Kendeigh, 1952; Haftorn, 1979; Conway and Martin, 2000a,b; Londoño et al., 2008; Camfield and Martin, 2009; Álvarez and Barba, 2014; Amininasab et al., 2016). For example, urban pale breasted thrush (Turdus leucomelas) that built their nests on buildings decreased nest attentiveness as compared to ones that built nests on trees because buildings increase nest temperatures (Batisteli et al., 2020). In a recent laboratory experiment, elevated ambient temperatures decreased female incubation attentiveness in zebra finches (Taeniopygia guttata) (Ton et al., 2021). Therefore, elevated ambient temperatures in urban habitats may be one environmental pressure driving differences in incubation behavior of urban birds.
Incubating adults will adjust their incubation patterns to maximize fitness, for example when they are energetically constrained (Skutch, 1962; Deeming, 2002a). Thus, we propose two possible hypotheses as to the mechanisms driving the observed behavioral changes. The first is that urban females decrease nest attentiveness because increased ambient temperatures allow them the opportunity (reduced thermal costs). Experiencing higher ambient temperature in urban areas may allow females to compensate for reduced foraging efficiency by spending more time off the nest foraging for themselves (Conway and Martin, 2000a,b; Londoño et al., 2008). Higher temperatures in nest boxes overnight may reduce the thermostatic costs of incubating adults, allowing them to have higher energy stores (Bryan and Bryant, 1999), which may permit incubating adults to decrease nest attentiveness and increase time off the nest. Further studies investigating nighttime allocation of energy is warranted to disentangle the temporal distribution of female energetic expenditure during incubation. An alternative but non-mutually exclusive explanation is that urban birds decrease incubation effort because they are required to spend more time foraging due to lower availability of food to acquire the needed energy for incubation. This possibility could come at a cost to the offspring in terms of hatching success (Charmantier et al., 2017). However, we saw no difference in hatching success between our urban and rural nests, possibly suggesting a reduced cost of incubation for urban females. Egg and microclimate temperature within the nest would be needed to fully understand the effects of ambient temperature on these behaviors.
As the incubation period progressed, urban females spent less time on average incubating during a bout while this duration stayed relatively constant for rural females (Figure 1B). Additionally, while total incubation time in rural birds increased over the course of the period, urban females remained relatively constant (Figure 1C). Why would urban and rural birds differ in intensity of these behaviors as incubation day progresses? Temperate birds such as house wrens, normally display a rapid increase in nest attentiveness once the full clutch is laid and maintain this level as hatching approaches (Kendeigh, 1952; Skutch, 1962), which is observed in our rural females (Figure 1C). As food availability is lower at our urban site, it supports why urban females do not mirror the expected increase in incubation time of their rural counterparts and have a lower, yet consistent, incubation effort as more time may need to be spent foraging for food. More experimental studies are needed to disentangle the directionality and duration of these behavioral differences in urban and rural female incubation.
While there is growing evidence for behavioral adjustments in urban environments, it is difficult to conclude whether these shifts are caused by plasticity or evolutionary processes (Sol et al., 2013). Birds in particular are able to tolerate new conditions and environments due to behavioral, physiological and ecological flexibility (Bonier et al., 2007). The individuals that colonize urban environments may possess more behavioral plasticity in their incubation strategies (Haftorn, 1979; Sol et al., 2013; McClintock et al., 2014; Marzluff, 2017; Simmonds et al., 2017). Adjusting incubation behavior can help avian wildlife cope with novel environmental challenges, ensure an optimal thermal environment for the developing embryos, and allow birds to inhabit urbanized areas. As incubation temperature affects offspring fitness (Hepp et al., 2015; Ospina, 2017), behavioral alternations can also be a mechanism producing phenotypic differences in urban birds. Urban heat island effects, combined with previously described ambient temperature and predation effects (Conway and Martin, 2000b), may together drive selective forces in urban birds.
Urbanization poses a suite of new challenges which influence life-time fitness (Conway and Martin, 2000b; McDonnell and Hahs, 2015; Sepp et al., 2018). While urbanization has changed the biotic and abiotic environment, the characteristics that distinguish the persistence of urban wildlife are poorly understood (Bonier et al., 2007). Our study suggests that urbanization may be linked to an essential aspect of avian reproduction and parental care. However, our study is correlative, and experimental studies are needed to test whether this shift in behavior is due to plasticity or adaptation. Our study also only had one urban and one rural site; therefore, these results may not be representative of other urbanized landscapes. Due to this limitation, we caution from general interpretations and highly urge further studies across multiple urban to rural gradients to better understand the patterns between urbanization and behavioral shifts. However, this study gives an initial insight to altered behaviors due to environmental change. By studying trait differences between urban and non-urban wildlife, we can better understand urbanization’s effects on wildlife behavior and how these parental behaviors affect offspring phenotypes in a changing landscape.
Data Availability Statement
The original contributions presented in the study are included in the article/Supplementary Material, further inquiries can be directed to the corresponding author/s.
Ethics Statement
The animal study was reviewed and approved by the Institutional Animal Care and Use Committee of the University of Nevada, Reno.
Author Contributions
JH and JO conceived the study. JO secured funding for the study. JH collected and analyzed the data and wrote the manuscript. Both authors revised, edited, and approved the manuscript before submission.
Funding
JO is funded by the National Science Foundation (OIA-1738594) and the National Institute of Health (P20 GM103650).
Conflict of Interest
The authors declare that the research was conducted in the absence of any commercial or financial relationships that could be construed as a potential conflict of interest.
Acknowledgments
We thank the Caughlin Ranch homeowner’s association for the use of their sites. We also thank Ryan Fung for his indispensable help in the field.
Supplementary Material
The Supplementary Material for this article can be found online at: https://www.frontiersin.org/articles/10.3389/fevo.2021.590069/full#supplementary-material
References
Álvarez, E., and Barba, E. (2014). Behavioural responses of great tits to experimental manipulation of nest temperature during incubation. Ornis Fenn. 91, 220–230.
Amininasab, S. M., Kingma, S. A., Birker, M., Hildenbrandt, H., and Komdeur, J. (2016). The effect of ambient temperature, habitat quality and individual age on incubation behaviour and incubation feeding in a socially monogamous songbird. Behav. Ecol. Sociobiol. 70, 1591–1600. doi: 10.1007/s00265-016-2167-2
Ardia, D. R., Pérez, J. H., and Clotfelter, E. D. (2010). Experimental cooling during incubation leads to reduced innate immunity and body condition in nestling tree swallows. Proc. R. Soc. B Biol. Sci. 277, 1881–1888. doi: 10.1098/rspb.2009.2138
Arnfield, A. J. (2003). Two decades of urban climate research: a review of turbulence, exchanges of energy and water, and the urban heat island. Int. J. Climatol. 23, 1–26. doi: 10.1002/joc.859
Baldan, D., and Ouyang, J. Q. (2020). Urban resources limit pair coordination over offspring provisioning. Sci. Rep. 10:15888. doi: 10.1038/s41598-020-72951-2
Batisteli, A. F., de Souza, L. B., Santieff, I. Z., Gomes, G., Soares, T. P., Pini, M., et al. (2020). Buildings promote higher incubation temperatures and reduce nest attentiveness in a Neotropical thrush. Ibis (Lond. 1859) 163, 79–89. doi: 10.1111/ibi.12863
Bonier, F., Martin, P. R., and Wingfield, J. C. (2007). Urban birds have broader environmental tolerance. Biol. Lett. 3, 670–673. doi: 10.1098/rsbl.2007.0349
Bryan, S. M., and Bryant, D. M. (1999). Heating nest-boxes reveals an energetic constraint on incubation behaviour in great tits, Parus major. Proc. R. Soc. B Biol. Sci. 266, 157–162. doi: 10.1098/rspb.1999.0616
Camfield, A. F., and Martin, K. (2009). The influence of ambient temperature on horned lark incubation behaviour in an alpine environment. Behaviour 146, 1615–1633. doi: 10.1163/156853909X463335
Chamberlain, D. E., Cannon, A. R., Toms, M. P., Leech, D. I., Hatchwell, B. J., and Gaston, K. J. (2009). Avian productivity in urban landscapes: a review and meta-analysis. Ibis (Lond. 1859). 151, 1–18. doi: 10.1111/j.1474-919X.2008.00899.x
Charmantier, A., Demeyrier, V., Lambrechts, M., Perret, S., and Grégoire, A. (2017). Urbanization is associated with divergence in pace-of-life in great tits. Front. Ecol. Evol. 5:53. doi: 10.3389/fevo.2017.00053
Chen, G., Li, X., Liu, X., Chen, Y., Liang, X., Leng, J., et al. (2020). Global projections of future urban land expansion under shared socioeconomic pathways. Nat. Commun. 11:537. doi: 10.1038/s41467-020-14386-x
Clucas, B., and Marzluff, J. M. (2012). Attitudes and actions toward birds in Urban areas: human cultural differences influence bird behavior. Auk 129, 8–16. doi: 10.1525/auk.2011.11121
Conway, C. J., and Martin, T. E. (2000a). Effects of ambient temperature on avian incubation behavior. Behav. Ecol. 11, 178–188. doi: 10.1093/beheco/11.2.178
Conway, C. J., and Martin, T. E. (2000b). Evolution of passerine incubation behavior: influence of food, temperature, and nest predation. Evolution (N. Y). 54, 670–685. doi: 10.1111/j.0014-3820.2000.tb00068.x
Cooper, C. B., and Mills, H. (2005). New software for quantifying incubation behavior from time-series recordings. J. F. Ornithol. 76, 352–356. doi: 10.1648/0273-8570-76.4.352
Deeming, D. C. (2002a). Avian Incubation: Behaviour, Environment, and Evolution. Oxford: Oxford University Press.
Deeming, D. C. (2002b). “Behaviour patterns during incubation,” in Avian Incubation: Behaviour, Environment, and Evolution, ed. D. C. Deeming (Oxford: Oxford University Press), 63–87.
Deeming, D. C. (2002c). “Importance and evolution of incubation in avian reproduction,” in Avian Incubation: Behaviour, Environment, and Evolution, ed. D. C. Deeming (Oxford: Oxford University Press), 1–7. doi: 10.1093/acprof:oso/9780198718666.003.0001
Durant, S. E., Hopkins, W. A., Hepp, G. R., and Walters, J. R. (2013). Ecological, evolutionary, and conservation implications of incubation temperature-dependent phenotypes in birds. Biol. Rev. 88, 499–509. doi: 10.1111/brv.12015
Eötvös, C. B., Magura, T., and Lövei, G. L. (2018). A meta-analysis indicates reduced predation pressure with increasing urbanization. Landsc. Urban Plan. 180, 54–59. doi: 10.1016/j.landurbplan.2018.08.010
Gorissen, L., Snoeijs, T., Van Duyse, E., and Eens, M. (2005). Heavy metal pollution affects dawn singing behaviour in a small passerine bird. Oecologia 145, 504–509. doi: 10.1007/s00442-005-0091-7
Haftorn, S. (1979). Incubation and Regulation of Egg Temperature in the Willow Tit Parus montanus. Ornis Scand. (Scandinavian J. Ornithol.) 10, 220–234. doi: 10.2307/3676066
Hall, J. M., and Warner, D. A. (2018). Thermal spikes from the urban heat island increase mortality and alter phyhysiology of lizard embryos. J. Exp. Biol. 221:jeb181552. doi: 10.1242/jeb.181552
Hepp, G. R., Durant, S. E., and Hopkins, W. A. (2015). “Influences of incubation temperature on offspring phenotype and fitness in birds,” in Nests, Eggs, and Incubation, eds D. C. Deeming and S. J. Reynolds (Oxford: Oxford University Press), 171–178. doi: 10.1093/acprof:oso/9780198718666.003.0014
Johnson, M. T. J., and Munshi-South, J. (2017). Evolution of life in urban environments. Science 358:eaam8327. doi: 10.1126/science.aam8327
Jones, G. (1987). Time and energy constraints during incubation in free-living swallows (Hirundo rustica): an experimental study using precision electronic balances. J. Anim. Ecol. 56:229. doi: 10.2307/4812
Kendeigh, S. C. (1952). Parental Care and its Evolution in Birds. Illinois Biological Monographs. Urbana: The University of Illinois Press.
Landsberg, H. E. (1981). The Urban Climate. urban Clim. New York: Academic Press. doi: 10.1016/0304-4009(83)90022-0.
Londoño, G. A., Levey, D. J., and Robinson, S. K. (2008). Effects of temperature and food on incubation behaviour of the northern mockingbird, Mimus polyglottos. Anim. Behav. 76, 669–677. doi: 10.1016/j.anbehav.2008.05.002
Mainwaring, M. C. (2015). “Nest construction and incubation in a changing climate,” in Nests, Eggs, and Incubation, eds D. C. Deeming and S. J. Reynolds (Oxford: Oxford University Press), 65–74. doi: 10.1093/acprof:oso/9780198718666.003.0006
Markovchick-Nicholls, L., Regan, H. M., Deutschman, D. H., Widyanata, A., Martin, B., Noreke, L., et al. (2008). Relationships between human disturbance and wildlife land use in urban habitat fragments. Conserv. Biol. 22, 99–109. doi: 10.1111/j.1523-1739.2007.00846.x
Martin, T. E., and Ghalambor, C. K. (1999). Males feeding females during incubation. I. Required by microclimate or constrained by nest predation? Am. Nat. 153, 131–139. doi: 10.1086/303153
Marzluff, J. M. (2017). A decadal review of urban ornithology and a prospectus for the future. Ibis (Lond. 1859). 159, 1–13. doi: 10.1111/ibi.12430
McClintock, M. E., Hepp, G. R., and Kennamer, R. A. (2014). Plasticity of incubation behaviors helps Wood Ducks (Aix sponsa) maintain an optimal thermal environment for developing embryos. Auk 131, 672–680. doi: 10.1642/auk-14-57.1
McDonnell, M. J., and Hahs, A. K. (2015). Adaptation and Adaptedness of organisms to urban environments. Annu. Rev. Ecol. Evol. Syst. 46, 261–280. doi: 10.1146/annurev-ecolsys-112414-054258
Mckinney, M. L. (2006). Urbanization as a major cause of biotic homogenization. Biol. Conserv. 127, 247–260. doi: 10.1016/j.biocon.2005.09.005
Merrill, L., Ospina, E. A., Santymire, R. M., and Benson, T. J. (2020). Egg incubation temperature affects development of innate immune function in nestling American Robins (Turdus migratorius). Physiol. Biochem. Zool. 93, 1–12. doi: 10.1086/705361
Monaghan, P., and Nager, R. G. (2002). Why don’t birds lay more eggs? Qual. Saf. Heal. Care 11:6. doi: 10.1136/qhc.11.1.6
Mulholland, T. I., Ferraro, D. M., Boland, K. C., Ivey, K. N., Le, M. L., LaRiccia, C. A., et al. (2018). Effects of experimental anthropogenic noise exposure on the reproductive success of secondary cavity nesting birds. Integr. Comp. Biol. 58, 967–976. doi: 10.1093/icb/icy079
Nord, A., and Cooper, C. B. (2019). Night conditions affect morning incubation behaviour differently across a latitudinal gradient. Int. J. Avian Sci. 162, 827–835. doi: 10.1111/ibi.12804
Nord, A., and Nilsson, J. Å (2011). Incubation temperature affects growth and energy metabolism in blue tit nestlings. Am. Nat. 178, 639–651. doi: 10.1086/662172
Nord, A., and Williams, J. B. (2015). “The energetic costs of incubation,” in Nests, Eggs, and Incubation, eds D. C. Deeming and J. S. Ronalds (Oxford: Oxford University Press).
Ospina, E. A. (2017). Incubation Temperature Impacts Growth, Physiology and Survival in Nestlings of an Open-Cup Nesting Passerine. M.S. Thesis, University of Illinois at Urbana, Urbana, IL.
Ouyang, J. Q., Baldan, D., Munguia, C., and Davies, S. (2019). Genetic inheritance and environment determine endocrine plasticity to urban living. Proc. R. Soc. B Biol. Sci. 286:20191215. doi: 10.1098/rspb.2019.1215
Ouyang, J. Q., Isaksson, C., Schmidt, C., Hutton, P., Bonier, F., and Dominoni, D. (2018). A new framework for urban ecology: an integration of proximate and ultimate responses to anthropogenic change. Integr. Comp. Biol. 58, 915–928. doi: 10.1093/icb/icy110
Peng, S., Piao, S., Ciais, P., Friedlingstein, P., Ottle, C., Bréon, F. M., et al. (2012). Surface urban heat island across 419 global big cities. Environ. Sci. Technol. 46, 696–703. doi: 10.1021/es2030438
Pérez, J. H., Ardia, D. R., Chad, E. K., and Clotfelter, E. D. (2008). Experimental heating reveals nest temperature affects nestling condition in tree swallows (Tachycineta bicolor). Biol. Lett. 4, 468–471. doi: 10.1098/rsbl.2008.0266
R Core Team (2019). R: A Language and Environment for Statistical Computing. Vienna: R Foundation for Statistical Computing.
Reynolds, J. S., Ibáñez-Álamo, J. D., Sumasgutner, P., and Mainwaring, M. C. (2019). Urbanisation and nest building in birds: a review of threats and opportunities. J. Ornithol. 160, 841–860. doi: 10.1007/s10336-019-01657-8
Rodewald, A. D., and Kearns, L. J. (2011). Shifts in dominant nest predators along a rural-to-urban landscape gradient. Condor 113, 899–906. doi: 10.1525/cond.2011.100132
Sepp, T., McGraw, K. J., Kaasik, A., and Giraudeau, M. (2018). A review of urban impacts on avian life-history evolution: does city living lead to slower pace of life? Glob. Chang. Biol. 24, 1452–1469. doi: 10.1111/gcb.13969
Seress, G., Hammer, T., Bókony, V., Vincze, E., Preiszner, B., Pipoly, I., et al. (2018). Impact of urbanization on abundance and phenology of caterpillars and consequences for breeding in an insectivorous bird. Ecol. Appl. 28, 1143–1156. doi: 10.1002/eap.1730
Seress, G., and Liker, A. (2015). Habitat urbanization and its effects on birds. Acta Zool. Acad. Sci. Hungaricae 61, 373–408. doi: 10.17109/AZH.61.4.373.2015
Seress, G., Lipovits, Á, Bókony, V., and Czúni, L. (2014). Quantifying the urban gradient: a practical method for broad measurements. Landsc. Urban Plan 131, 42–50. doi: 10.1016/j.landurbplan.2014.07.010
Seress, G., Sándor, K., Evans, K. L., and Liker, A. (2020). Food availability limits avian reproduction in the city: an experimental study on great tits Parus major. J. Anim. Ecol. 89, 1570–1580. doi: 10.1111/1365-2656.13211
Shochat, E., Lerman, S., and Fernández-Juricic, E. (2010). “Birds in urban ecosystems: population dynamics, community structure, biodiversity, and conservation,” in Urban Ecosystem Ecology, eds J. Aitkenhead-Peterson and A. Volder (Madison: ASA-CSSA-SSSA), 75–86. doi: 10.2134/agronmonogr55.c4
Shochat, E., Warren, P. S., Faeth, S. H., McIntyre, N. E., and Hope, D. (2006). From patterns to emerging processes in mechanistic urban ecology. Trends Ecol. Evol. 21, 186–191. doi: 10.1016/j.tree.2005.11.019
Sih, A., Ferrari, M. C. O., and Harris, D. J. (2011). Evolution and behavioural responses to human-induced rapid environmental change. Evol. Appl. 4, 367–387. doi: 10.1111/j.1752-4571.2010.00166.x
Simmonds, E. G., Sheldon, B. C., Coulson, T., and Cole, E. F. (2017). Incubation behavior adjustments, driven by ambient temperature variation, improve synchrony between hatch dates and caterpillar peak in a wild bird population. Ecol. Evol. 7, 9415–9425. doi: 10.1002/ece3.3446
Sol, D., Lapiedra, O., and González-Lagos, C. (2013). Behavioural adjustments for a life in the city. Anim. Behav. 85, 1101–1112. doi: 10.1016/j.anbehav.2013.01.023
Thompson, F. R. (2007). Factors affecting nest predation on forest songbirds in North America. Ibis (Lond. 1859). 149, 98–109. doi: 10.1111/j.1474-919X.2007.00697.x
Thomson, D. L., Monaghan, P., and Furness, R. W. (1998). The demands of incubation and avian clutch size. Biol. Rev. 73, 293–304. doi: 10.1111/j.1469-185X.1998.tb00032.x
Tinbergen, J. M., and Williams, J. B. (2002). “Energetics of incubation,” in Avian Incubation: Behaviour, Environment, and Evolution, ed. D. C. Deeming (Oxford: Oxford University Press), 299–313.
Ton, R., Hurley, L. L., and Griffith, S. C. (2021). Higher experimental ambient temperature decreases female incubation attentiveness in Zebra Finches (Taeniopygia guttata) and lower effort yields negligible energy savings. Int. J. Avian Sci. doi: 10.1111/ibi.12922
United Nations (2018). World Urbanization Prospects. Department of Economic and Social Affairs, Population Division. New York, NY: United Nations.
Vincze, E., Seress, G., Lagisz, M., Nakagawa, S., Dingemanse, N. J., and Sprau, P. (2017). Does urbanization affect predation of bird nests? A meta-analysis. Front. Ecol. Evol. 5:29. doi: 10.3389/fevo.2017.00029
Vleck, C. M. (1981). Energetic cost of incubation in the zebra finch. Con 83, 229–237. doi: 10.2307/1367313
Yom-Tov, Y., and Hilborn, R. (1981). Energetic constraints on clutch size and time of breeding in temperate zone birds. Oecologia 48, 234–243. doi: 10.1007/BF00347969
Keywords: behavioral flexibility, heat island, food availability, parental care, plasticity, avian reproduction, anthropogenic effects, thermal buttons
Citation: Heppner JJ and Ouyang JQ (2021) Incubation Behavior Differences in Urban and Rural House Wrens, Troglodytes aedon. Front. Ecol. Evol. 9:590069. doi: 10.3389/fevo.2021.590069
Received: 31 July 2020; Accepted: 04 February 2021;
Published: 24 February 2021.
Edited by:
David Andrew Luther, George Mason University, United StatesReviewed by:
Sydney Frances Hope, Virginia Tech, United StatesSarah Foltz, Radford University, United States
Copyright © 2021 Heppner and Ouyang. This is an open-access article distributed under the terms of the Creative Commons Attribution License (CC BY). The use, distribution or reproduction in other forums is permitted, provided the original author(s) and the copyright owner(s) are credited and that the original publication in this journal is cited, in accordance with accepted academic practice. No use, distribution or reproduction is permitted which does not comply with these terms.
*Correspondence: Jennifer J. Heppner, amhlcHBuZXJAbmV2YWRhLnVuci5lZHU=