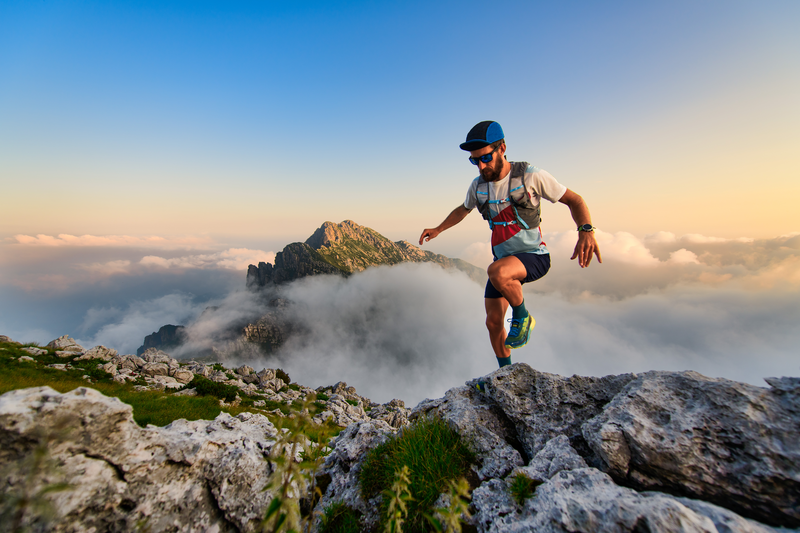
95% of researchers rate our articles as excellent or good
Learn more about the work of our research integrity team to safeguard the quality of each article we publish.
Find out more
ORIGINAL RESEARCH article
Front. Ecol. Evol. , 30 April 2021
Sec. Conservation and Restoration Ecology
Volume 9 - 2021 | https://doi.org/10.3389/fevo.2021.586948
This article is part of the Research Topic Trait-Based Plant Community Assembly, Ecological Restoration, and the Biocontrol of Invasive Exotic Plant Species View all 12 articles
Selecting appropriate native species for the biological control of invasive exotic plants is a recurring challenge for conservationists, ecologists, and land managers. Recently developed trait-based approaches may be an effective means of overcoming this challenge. However, we lack a protocol and software platform that can be used to quickly and effectively select potential native plant species for performing biological control of the invasive exotic plant species. Here, our study introduces a protocol and a software program that can be used for trait-based selection of appropriate native plant species for performing biocontrol of invasive exotic plant species. In particular, we illustrate the effectiveness of this software program and protocol by identifying native species that can be used for the biological control of Leucaena leucocephala (Lam.) de Wit, a highly invasive plant species found in many parts of the world. Bougainvillea spectabilis was the only native species selected by our software program as a potential biocontrol agent for L. leucocephala. When separately planting 4 seedlings of B. spectabilis and two unselected species (Bombax ceiba, and Ficus microcarpa) as neighbors of each individual of L. leucocephala for 3 years, we found that B. spectabilis, which was functionally similar to the invasive L. leucocephala, significantly limited the invasion of the latter, while the unselected native plant species could not. That was because all the seedling of B. spectabilis survived, while half seedlings of unselected species (B. ceiba and F. microcarpa) died, during the experimental period when planted with L. leucocephala seedlings. Moreover, the growth of L. leucocephala was restricted when planted with B. spectabilis, in contrast B. ceiba and F. microcarpa did not influence the growth of L. leucocephala. Overall, our software program and protocol can quickly and efficiently select native plant species for use in the biological control of invasive exotic plant species. We expect that this work will provide a general protocol to perform biological control of many different types of invasive exotic plant species.
Invasive exotic plant species greatly impact global native plant diversity and ecosystem functioning (Pyšek et al., 2012; Barney et al., 2013). Chemical and mechanical methods have long been used to quickly remove invasive plant species from natural ecosystems (Paynter and Flanagan, 2004; Flory and Clay, 2009). However, these methods are extremely expensive, requiring large amounts of time, money, and resources (Seastedt, 2015). Furthermore, these efforts can often fail, due to a high risk of re-invasion (Kettenring and Adams, 2011). As a result, many ecologists and invasion biologists have instead suggested using suitable native plant species as biological control agents. Such efforts may be a more effective and/or more cost-effective way of preventing the invasion of exotic plant species (Moran et al., 2005; Seastedt, 2015; van Wilgen et al., 2020).
It has been shown that functional traits can explain why and how some exotic plant species successfully invade ecosystems outside their native range (Küster et al., 2008; van Kleunen et al., 2010; Hulme et al., 2013; Ostertag et al., 2015). That is because, functional traits that help to better adapt (e.g., having higher growth rate) to the local environment, and are also functionally distinctive from the native dominant plant species, can successfully facilitate invasive exotic plant species to outperform the native dominant plant species, thereby to successfully invade the local plant ecosystem (Funk et al., 2008). Thus, trait-based methods can be useful for selecting appropriate native species for use as biological control agents (Funk et al., 2008; Laughlin, 2014; Ostertag et al., 2015). Recently, Laughlin (2014) proposed such a trait-based native species selection framework for ecological restoration and preventing further invasions. Similarly, Wang et al. (2020) were able to use these methods to select suitable plant species in the restoration of a highly degraded tropical coral island in China. Here, we seek to examine the effectiveness of trait-based native species selection framework in performing the biocontrol of invasive exotic plant species in a tropical seasonal forest.
van Kleunen et al. (2010) used a meta-analysis to examine trait differences between exotic and native plant species, and identified six types of functional traits that capture key characteristics of invasive exotic plant species. As such, these functional traits can be utilized for the selection of native plant species for use in biocontrol efforts. Here, we introduce a trait-based native plant species protocol (TBNPSP) that can identify native candidate species for biocontrol agents of invasive exotic plant species. Our TBNPSP is based on the theory of limiting similarity, whereby due to competitive exclusion, invasive plant species that are functionally similar to native plant species cannot invade a native plant community (MacArthur and Levins, 1967). Consequently, mixing invasive exotic plant species with functionally similar native plant species may be an effective method for performing biological control of exotic invasive species (D’Antonio and Chambers, 2006; Funk et al., 2008).
Here, we quantify the effectiveness of our model by testifying whether the native species selected by our program can be used for the biological control of L. leucocephala, a highly invasive plant species found in many parts of the world (Richardson and Rejmánek, 2011). Due to its high nitrogen-fixing ability, fast-growth rate, and tolerance to drought, L. leucocephala has been used widely in the restoration of degraded forest ecosystems worldwide (Goel and Behl, 2002; Wolfe and van Bloem, 2012; Ishihara et al., 2018; Liu et al., 2018; Peng et al., 2019). For example, 20 years of limestone mining associated with the cement industry degraded a 0.2 km2 area of tropical forest located in Baopoling hill (BPL), Sanya city, China, to bare rock that could hardly support any plant life. In 2015, the local government initiated a large-scale reforestation project in this area. Monocultures of L. leucocephala were first planted at the top of BPL in an attempt to quickly restore vegetative cover. Within 2 years, however, Leucaena leucocephala has become highly invasive in the BPL, and no other plant species have been able to establish or survive. Moreover, the species has invaded the adjacent undisturbed old-growth tropical forests. The reforestation project on BPL thus provides a perfect system for testing the utility of TBNPSP for restoration effort. Specifically, we judge whether the native plant species selected by our TBNPSP can indeed prevent the invasion of L. leucocephala by the following two standards. First, we quantify whether functional traits of selected native species should be very similar to L. leucocephala, but dissimilar to the unselected species? Second, we test whether mixture of selected native species and L. leucocephala can indeed restrict the growth rate and survival rate of L. leucocephala, whereas mixture of unselected native species and L. leucocephala cannot achieve this function.
Invasive exotic plant species can finish successfully invasion, only when it can not only better adapt (e.g., having higher growth rate) to the local environment than the native dominant plant species, but also are functionally distinctive from the native dominant plant species. Thus, selecting plant species which are functionally similar to invasive exotic plant species among the native dominant plant species can help biocontrol of invasive exotic plant species.
Functional traits used for selecting native plant species for biocontrol of invasive exotic species should capture key characteristics of the invasive exotic species. If there is no information on the characteristics of the invasive exotic species, users can apply the six different functional traits identified by van Kleunen et al. (2010) as a start point (see Table 1).
Table 1. Six functional trait that are known to reflect the common characteristics of invasive exotic plant species (van Kleunen et al., 2010).
Based on the trait-based community framework and limiting similarity theory, Funk et al. (2008) suggested building native communities with traits similar to potential invader to limit exotic species invasion. To verify this hypothesis, we developed a software program to select native plant species for biocontrol of functionally similar invasive species. Briefly, for a series of candidate species, the software platform calculates the similarity index as a measure of the functional trait similarity between each of the candidate native plant species and the invasive exotic plant species, and ranks them from the highest to the lowest according to the similarity index. A higher similarity index indicates higher similarity between the native plant species and the invasive exotic plant species, therefore suggesting better performance of the native species in the biocontrol of the invasive species. This software program uses the maximum entropy model developed by Shipley et al. (2006) to obtain a set of native plant species that are most functionally similar to the invasive plant species. Our species selection function was integrated into a web-based software platform named the “Plant Species Selection Platform” (Wang et al., 2021). Input data for the program are trait values of the invasive species and the potential native species. The program outputs text and figure results showing similarity ranks between native and invasive species. The software was written in the R language1, and the main page of the platform is shown in Supplementary Figure 1.
Planting invasive exotic plant species with candidate native plant species selected for and selected against biocontrol by the software program, respectively, to determine whether native species selected for biocontrol are efficient in limiting the invasion of invasive exotic species.
We tested whether our TBNPSP protocol can indeed select suitable species to perform biocontrol of an invasive exotic plant species (L. leucocephala) on Baopoling hill (BPL, 110°58′01″E, 19°38′48″N), which is a limestone hill in Sanya City, Hainan island, China that has an elevation of 300 m (Luo et al., 2020; Figure 1). The site has a tropical monsoon oceanic climate with a mean annual temperature of 28°C. The average annual precipitation in Sanya city is 1,500 mm, with approximately 91% of the precipitation occurring between June and October (Luo et al., 2018). The vegetation of the study area is classified as tropical monsoon broad-leaf forest. Limestone mining associated with the cement industry lasted from 1995 till 2015, resulting in a 0.2 km2 extremely degraded area covered by bare rock (Figure 1A). Outside of this degraded area is the undisturbed old-growth tropical monsoon forest (Figure 1A). In year 2015 we invested 3 million USA dollars to performing reforestation to recover this 0.2 km2 extremely degraded area. At the beginning, we used explosive and excavator to remove the top of the degraded area to reconstruct the slope and soil layers to perform reforestation (Figures 1B,C). For preventing any of the local people’s misunderstanding that we are blasting the hill, L. leucocephala is first used to quickly perform the reforestation in 2015 (Figure 1D). Only within 2 years, L. leucocephala has become highly invasive and no other plant species can survive and grow around L. leucocephala (Figure 1E). Moreover, L. leucocephala has successfully invaded the adjacent undisturbed old-growth tropical forests (Figure 1F).
Figure 1. Map of the study site (Baopoling hill) and the surrounding landscape, including the 0.2 km2 area of extremely degraded land and the undisturbed old growth forest (A) and the detail processes for describing why and how Leucaena leucocephala not only becomes highly invasive in Baopoling hill, but also has successfully invaded the undisturbed old growth forest (B–F).
For getting the local dominant native plant species, in 2017, thirty plots, each of 20 × 20 m2 that were arranged along five parallel transects in the adjacent undisturbed old-growth forest, with 300 m intervals between adjacent plots. Within each plot, all freestanding plants were measured and identified to species. Here, we used the 11 dominant native plant species (relative abundance range from 6 to 10%) (Bougainvillea spectabilis, Bombax ceiba, and Ficus microcarpa, Bridelia tomentosa, Radermachera frondosa, Lepisanthes rubiginosa, Rhaphiolepis indica, Pterospermum heterophyllum, Fissistigma oldhamii, Psychotria rubra, and Cudrania cochinchinensis) as our biological control agents.
It has been found that high growth rates and drought stress tolerance help L. leucocephala outcompete native species in multiple places (Wolfe and van Bloem, 2012; Chiou et al., 2016; Barros et al., 2020). Previous work also suggests that traits that are highly associated with fast-growth (i.e., maximum photosynthesis rate, stomatal conductance and transpiration) and drought tolerance (i.e., leaf turgor loss point) are key characteristics facilitating the invasion of L. leucocephala in the BPL (Luo et al., 2020). As such, we expect that these traits can be used to select native plant species to perform biocontrol of this invasive species. To verify this, in the peak of both the wet (July) and the dry (February) seasons in 2018, we measured maximum photosynthesis rate, stomatal conductance, transpiration, and leaf turgor loss point for both L. leucocephala and the 11 candidate native plant species. We collected 20 fully expanded and healthy leaves from five individuals from each of the 12 species in February 2017 (the peak of the dry season) for trait measurement. For avoiding intraspecific variances in functional traits measurements, samples were collected from individuals that had a diameter at breast height (DBH) close to the species mean value (Supplementary Table 1), which was obtained from an extensive vegetation sample conducted at the site. Leaf samples were used to measure a suite of functional traits related to plant growth (i.e., maximum photosynthesis rate (Amass; μmol m–2 s–1), stomatal conductance (SC; mmol m–2 s–1), transpiration (TR; μmol m–2 s–1), and drought stress tolerance (TLP, Mpa). These traits were listed and introduced in Table 2. Traits were measured following the methods described in Zhang et al. (2018), and the detailed procedures for measuring these functional traits can be found in the Supplementary Materials.
Inputting all measured traits for L. leucocephala and all candidate native plant species into our developed software program to automatically select native plant species for biocontrol of L. leucocephala.
It was showed that mixed plantations of Eucalyptus camaldulensis and Eucalyptus citriodora can restrict the growth of L. leucocephala, making them effective biocontrol agents of L. leucocephala (Liu et al., 2018). Here, we planted L. leucocephala with our candidate native plant species selected for and selected against biocontrol by our software program, respectively, to determine (1) whether native species selected for biocontrol are efficient in limiting the invasion of L. leucocephala; and (2) whether those native species selected against biocontrol perform inferiorly to those selected for. To accomplish these, we measured the average height and diameter at breast height (DBH) of all individuals of mono-planted L. leucocephala in BPL in 2017. We first tried to purchase seedlings of L. leucocephala and each of the 11 candidate native plant species with the same average height and DBH as mono-planted L. leucocephala in BPL from a local market. We planted monocultures of L. leucocephala (MLL) seedlings 3 m apart and treated it as a control in a nursery nearby. Mixture of L. leucocephala and native species were created in the same nursery as below. We first regularly planted 25 individuals of L. leucocephala, and then regularly planted 4 seedlings of a specific native species as neighbors of each individual of L. leucocephala. In 2020, we collected survival status (death or survival), and the height and DBH of each survival seedling. Detail statistic methods for analyzing whether selected native plant species can prevent L. leucocephala. were shown as below:
First, we used our native plant species selection software to select native plant species that were functionally similar/dissimilar to L. leucocephala. We then verified the results of the species selection via manual test. To do so, we performed a Wilcoxon signed-rank test to compare differences in the traits (i.e., photosynthesis rate, stomatal conductance, transpiration rate, and leaf turgor loss point) between L. leucocephala and each of the 11 native species to test: (1) whether native plant species selected by our program are functionally similar to L. leucocephala; and (2) whether native species that were not selected by our program are functionally dissimilar to L. leucocephala. Finally, we compared the differences in average height and DBH among individuals of L. leucocephala growing in the monoculture, and those individuals growing with selected and unselected native species to test whether selected native species can limit the growth of L. leucocephala, whereas unselected native species cannot.
Bougainvillea spectabilis was the only species selected by our software program as a potential biocontrol agent for L. leucocephala, having a similarity index value of 0.99 (Figure 2). Similarity index values for the remaining 10 native tree species ranged from 0.001 to 0.01, indicating that they were extremely and functionally dissimilar to L. leucocephala (Figure 2). The Wilcoxon signed-rank test further confirmed that B. spectabilis was highly similar to L. leucocephala, whereas the remaining 10 native plant species were all extremely dissimilar to L. leucocephala. There was no difference in the measured four traits (maximum photosynthesis rate, stomatal conductance, transpiration rate and leaf turgor loss point) between B. spectabilis and L. leucocephala (p > 0.05, Figure 3). In contrast, L. leucocephala had much higher Amass, SC and TR, and much lower TLP, than the remaining native species (p < 0.05, Figure 3).
Figure 2. Native plant species selection result based on our native plant species selection program. This result uses a similarity index to determine the functional trait similarity between each of the 11 candidate native plant species (i.e., BS, Bougainvillea spectabilis; BC, Bombax ceiba; FM, Ficus microcarpa; BT, Bridelia tomentosa; RF, Radermachera frondosa; LR, Lepisanthes rubiginosa; RI, Rhaphiolepis indica; PH, Pterospermum heterophyllum; FO, Fissistigma oldhamii; PR, Psychotria rubra; CC, Cudrania cochinchinensis) and the invasive exotic plant species (Leucaena leucocephala). A higher similarity index indicates higher similarity between the native plant species and the invasive exotic plant species.
Figure 3. Differences in the four functional traits (i.e., transpiration rate, TR; maximum Photosynthesis rate, Amass; stomatal conductance, SC; and leaf turgor loss point, TLP) between the invasive exotic plant species L. leucocephala, and each of the 11 native plant species (i.e., B. spectabilis, B. ceiba and F. microcarpa, B. tomentosa, R. frondosa, L. rubiginosa, R. indica, P. heterophyllum, F. oldhamii, P. rubra, and C. cochinchinensi). *** indicates P < 0.05 and NS (short for non-significant) indicates P > 0.05 based on Wilcoxon signed-rank test.
Among the 10 unselected native candidate species, we were only able to purchase seedlings of B. ceiba, and F. microcarpa in the local market. Thus, we followed the mixing planting design in Figure 4 to first monocultures of L. leucocephala (MLL) seedlings 3 m apart and treated it as a control in a nursery nearby. We then regularly planted 25 individuals of L. leucocephala, then regularly planted 4 seedlings of the selected native plant species (B. spectabilis) and unselected native plant species (B. ceiba, and F. microcarpa) as neighbors of each individual of L. leucocephala. After 3 years’ plantation, we found that all 100 B. spectabilis seedlings survived with L. leucocephala seedlings. In contrast, only 40–45 seedlings of B. ceiba and F. microcarpa survived when planted with L. leucocephala, respectively. The average height and DBH of monocultured L. leucocephala were 1.4 times greater than individuals planted with B. spectabilis (p < 0.05, Figures 5A,D). In contrast, there was no significant difference in the average height or DBH of L. leucocephala when planted with B. ceiba (LL2) or F. microcarpa (LL3) (p > 0.05, Figures 5A,D). The average height and DBH of L. leucocephala were 33% (p < 0.05, Table 1 and Figures 5B,E), and 17–23% (p < 0.05, Figures 5B,E) lower than the selected native species (B. spectabilis) and unselected species (B. ceiba, and F. microcarpa), respectively. In addition, the average height and DBH of B. spectabilis were 1.3 times greater than L. leucocephala in the monoculture (p < 0.05, Figures 5C,F). In summary, the survival and growth of L. leucocephala was restricted when planted with B. spectabilis, whereas B. ceiba and F. microcarpa did not significantly influence the survival and growth of L. leucocephala.
Figure 4. The design of L. leucocephala monocultures (MLL), mixed plantation of B. spectabilis and L. leucocephala, mixed plantation of B. ceiba and L. leucocephala, and mixed plantation of F. microcarpa and L. leucocephala. MLL is made of 25 monocultured seedlings of L. leucocephala at 3 m spacing. BS vs. LL1 is mixing 100 B. spectabilis seedings with 25 L. leucocephala seedlings at 3 × 3 m spacing, with 4 B. spectabilis seedings surrounding one L. leucocephala seedling. Mixed plantation of B. ceiba and L. leucocephala, and mixed plantation of F. microcarpa and L. leucocephala uses the same mixing design as mixed plantation of B. spectabilis and L. leucocephala.
Figure 5. Differences in average height and diameter at breast height (DBH) among monocultured L. leucocephala (MLL), L. leucocephala that is mixed with selected native species (B. spectabilis) (LL1), L. leucocephala mixed with unselected native species (B. ceiba and F. microcarpa) (LL2 and LL3, respectively) and selected and unselected native species (B. spectabilis, B. ceiba, and F. microcarpa) on Baopoling hill after 3 years of planting. (A,D) Demonstrate the differences in average height and DBH between individuals of L. leucocephala in LL1 and MLL (LL1 vs. MLL), between LL2 and MLL (LL2 vs. MLL) and between LL3 and MLL (LL3 vs. MLL). (B,E) represent the differences in average height and DBH between L. leucocephala in LL1 and B. spectabilis (BS vs. LL1), between L. leucocephala in LL2 and B. ceiba (BC vs. LL2) and between L. leucocephala in LL3 and F. microcarpa (FM vs. LL3). (C,F) show the differences in average height and DBH between L. leucocephala in MLL and BS, between MLL and BC and between MLL and FM. Detail seedlings planting design are provided in Figure 4. *** indicates P < 0.05 and NS (short for non-significant) indicates P > 0.05 based on Wilcoxon signed-rank test.
Here we introduce a trait-based native plant species selection protocol and a software program that can be used for selection of appropriate native plant species for performing biocontrol of invasive exotic plant species.
Using L. leucocephala as an example, we verified the power of our protocol and the software program in selecting potential biocontrol agents based on specific functional trait targets. By using the selected four functional traits (maximum photosynthesis rates, stomatal conductance, transpiration rate, and leaf turgor loss points), B. spectabilis was the only species selected by our software program as a potential biocontrol agent for L. leucocephala. By comparing the differences in these four traits between L. leucocephala and each of the 11 native plant species, we verified the results of our software program that B. spectabilis was the only species that was functionally similar to L. leucocephala. Interestingly, the remaining 10 native plant species were extremely dissimilar to L. leucocephala.
As expected, B. spectabilis, which is functionally similar to the invasive L. leucocephala, significantly limited the invasion of the latter, while the unselected native plant species could not. All the seedling of B. spectabilis survived, while half seedlings of unselected species (B. ceiba and F. microcarpa) died, during the experimental period when planted with L. leucocephala seedlings. Moreover, the growth of L. leucocephala was restricted when planted with B. spectabilis, in contrast B. ceiba and F. microcarpa did not influence the growth of L. leucocephala. Ultimately, our results demonstrated the power of our software program in selecting appropriate native plant species for the biological control of invasive exotic plant species.
Although the widely used traditional trial-and-error method could also be applied for selecting appropriate native species for the biocontrol of the invasive species, it is time and effort consuming because it may need to conduct a large experiment for several years. Moreover, such methods are likely limited to a small number of species because the number of treatments and replicates will exponentially increase with the number of candidate species (Giardina et al., 2007; Funk et al., 2008; Hall et al., 2011; Williams, 2011; Kanowski, 2014). Here we showed that, using our protocol, selecting appropriate native species as biological control agents can be fulfilled easily and efficiently. Most importantly, a large number of candidate native species can be considered simultaneously, given the availability of specific functional traits targeted for the biocontrol of the invasive exotic plant species.
We noted that merely using growth rates as indictor for the biocontrol of L. leucocephala may be not fully sufficient. Ecological factors that structure plant communities, determine growth and survival of individual plants, and affect plant evolution (e.g., root and shoot competition, light competition, soil heterogeneity), biomass, the number of fruits or seeds, roots development merits future survey. In addition, it is very necessary to quantify whether B. ceiba and F. microcarpa seedling mortality is due to competition with L. leucocephala in future.
In summary, we developed a protocol that includes the following steps for selecting suitable native plant species for biocontrol. First, identify functional traits that can capture key characteristics of the invasive exotic species. If there is no information on the characteristics of the invasive exotic species, users can apply the six different functional traits identified by van Kleunen et al. (2010) as a start point (see Table 2). Second, a potential native plant species pool should be selected using communities found in an adjacent area to the study site or similar habitats. Third, careful measurements of the selected functional traits should be performed for both exotic and native species. Fourth, input trait data to the native plant species selection software platform to select functionally similar native plant species. Finally, mix the selected native plant species with populations of the invasive exotic plant species, with the hope that they will act as biocontrol agents to reduce the growth and spread of the invasive species. Taken together, we demonstrated that our native plant species selection protocol and software program can quickly and efficiently select native plant species for use in the biological control of invasive exotic plant species. We expect that this work provides a general protocol to help conservationists and land managers perform biological control of various types of invasive exotic plant species. However, we have to admit our current results can only prove the native plant species selection software program and protocol is feasible and effective for biocontrol of tree species. Usually, many invasive exotic plant species in forest ecosystem are vine and herbaceous species, but not tree species. Thus, future researches are still necessary to testify the effectiveness of our native plant species selection software program and protocol in preventing the invasion of vine and herbaceous species.
The raw data supporting the conclusions of this article will be made available by the authors, without undue reservation.
HZ, KJ, YZ, JC, TDL, and CW designed the research. HZ, KJ, YZ, YTX, HJG, JC, TDL, and CW performed the research. HZ, KJ, YZ, JC, TDL, and CW analyzed the data. HZ, KJ, YZ, JC, TDL, and CW wrote the manuscript. All authors contributed to the article and approved the submitted version.
Scientific Research Project of Ecological Restoration of Baopoling Mountain in Sanya, National Natural Science Foundation of China (Grant Nos. 31770469, 41905094, 31961143023, and 41430529), the Strategic Priority Research Program of the Chinese Academy of Sciences (Grant No. XDA23080302), and the National Science and Technology Basic Work Project (Grant No. 2015FY1103002).
The authors declare that the research was conducted in the absence of any commercial or financial relationships that could be construed as a potential conflict of interest.
The Supplementary Material for this article can be found online at: https://www.frontiersin.org/articles/10.3389/fevo.2021.586948/full#supplementary-material
Barney, J. N., Tekiela, D. R., Dollete, E. S., and Tomasek, B. J. (2013). What is the “real” impact of invasive plant species? Front. Ecol. Environ. 11:322–329. doi: 10.1890/120120
Barros, V., Melo, A., Santos, M., Nogueira, L., Frosi, G., and Santos, M. G. (2020). Different resource-use strategies of invasive and native woody species from a seasonally dry tropical forest under drought stress and recovery. Plant Physiol. Biochem. 147, 181–190. doi: 10.1016/j.plaphy.2019.12.018
Chiou, C.-R., Chen, Y.-J., Wang, H. H., and Grant, W. E. (2016). Predicted range expansion of the invasive plant Leucaena leucocephala in the Hengchun peninsula. Taiwan. Biol. Invasions 18, 381–394. doi: 10.1007/s10530-015-1010-4
D’Antonio, C. M., and Chambers, J. C. (2006). “Using ecological theory to manage or restore ecosystems affected by invasive plant species,” in Foundations of Restoration Ecology, eds D. A. Falk, M. A. Palmer, and J. B. Zedler (Covelo, CA: Island Press), 260–279.
Flory, S., and Clay, K. (2009). Invasive plant removal method determines native plant community responses. J. Appl. Ecol. 46, 434–442. doi: 10.1111/j.1365-2664.2009.01610.x
Funk, J. L., Cleland, E. E., Suding, K. N., and Zavaleta, E. S. (2008). Restoration through reassembly: plant traits and invasion resistance. Trends Ecol. Evol. 23, 695–703. doi: 10.1016/j.tree.2008.07.013
Giardina, C. P., Litton, C. M., Thaxton, J. M., Cordell, S., Hadway, L. J., and Sandquist, D. R. (2007). Science Driven restoration: a candle in a demon haunted World—Response to cabin. Restor. Ecol. 15, 171–176. doi: 10.1111/j.1526-100X.2007.00227.x
Goel, V. L., and Behl, H. M. (2002). Selection of Leucaena species for afforestation and amelioration of sodic soils. Land Degrad. Dev. 13, 387–393. doi: 10.1002/ldr.518
Hall, J. S., Ashton, M. S., Garen, E. J., and Jose, S. (2011). The ecology and ecosystem services of native trees: implications for reforestation and land restoration in Mesoamerica. For. Ecol. Manag. 261, 1553–1557. doi: 10.1016/j.foreco.2010.12.011
Hulme, P. E., Pyšek, P., Jarošík, V., Pergl, J., Schaffner, U., and Vila, M. (2013). Bias and error in understanding plant invasion impacts. Trends Ecol. Evol. 28, 212–218. doi: 10.1016/j.tree.2012.10.010
Ishihara, K. L., Honda, M. D., Bageel, A., and Borthakur, D. (2018). “Leucaena leucocephala: a leguminous tree suitable for eroded habitats of Hawaiian islands,” in Ravine Lands: Greening for Livelihood and Environmental Security, eds J. C. Dagar and A. K. Singh (Berlin: Springer), 413–431. doi: 10.1007/978-981-10-8043-2
Kanowski, J. (2014). Restoration Ecology: The New Frontier, 2nd Edn, Vol. 15, eds J. Van Andel and J. Aronson, Oxford: Blackwell Publishing, 1–7.
Kettenring, K. M., and Adams, C. R. (2011). Lessons learned from invasive plant control experiments: a systematic review and meta-analysis. J. Appl. Ecol. 48, 970–979. doi: 10.1111/j.1365-2664.2011.01979.x
Küster, E. C., Kühn, I., Bruelheide, H., and Klotz, S. (2008). Trait interactions help explain plant invasion success in the German flora. J. Ecol. 96, 860–868. doi: 10.1111/j.1365-2745.2008.01406.x
Laughlin, D. C. (2014). Applying trait-based models to achieve functional targets for theory-driven ecological restoration. Ecol. Lett. 17, 771–784. doi: 10.1111/ele.12288
Liu, F., Gao, C., Chen, M., and Li, K. (2018). Above- and below-ground biomass relationships of Leucaena leucocephala (Lam.) de Wit in different plant stands. PLoS One 13:e0207059. doi: 10.1371/journal.pone.0207059
Luo, H. X., Dai, S. P., Li, M. F., and Xi, Z. H. (2018). The climate characteristics of 302 Hainan Island from 1959-2015. Jiangsu Agric. Sci. 46, 261–268. doi: 10.15889/j.issn.1002-1302.2018.15.066
Luo, J. H., Cui, J., Jiang, K., Tan, Z. Y., He, Q. F., Zhang, H., et al. (2020). Invariably fast-growing from wet to dry season and high drought stress tolerance in the dry season make Leucaena leucocephala successfully invade tropical forest. Trop Conserv. Sci. 13, 1–7.
MacArthur, R., and Levins, R. (1967). The limiting similarity, convergence, and divergence of coexisting species. Am. Nat. 101, 377–385. doi: 10.1086/282505
Moran, V. C., Hoffmann, J. H., and Zimmermann, H. G. (2005). Biological control of invasive alien plants in South Africa: necessity, circumspection, and success. Front. Ecol. Environ. 3:71–77. doi: 10.2307/3868513
Ostertag, R., Warman, L., Cordell, S., and Vitousek, P. M. (2015). Using plant functional traits to restore Hawaiian rainforest. J. Appl. Ecol. 52, 805–809. doi: 10.1111/1365-2664.12413
Paynter, Q., and Flanagan, G. J. (2004). Integrating herbicide and mechanical control treatments with fire and biological control to manage an invasive wetland shrub, mimosa pigra. J. Appl. Ecol. 41, 615–629. doi: 10.1111/j.0021-8901.2004.00931.x
Peng, S. H., Wang, H. H., and Kuo, Y. L. (2019). Methods for preventing the invasion of Leucaena leucocephala in Coastal Forests of the Hengchun Peninsula. Taiwan. Taiwan J. For. Sci. 34, 99–112.
Pyšek, P., Jarošík, V., Hulme, P. E., Pergl, J., Hejda, M., Schaffner, U., et al. (2012). A global assessment of invasive plant impacts on resident species, communities and ecosystems: the interaction of impact measures, invading species’ traits and environment. Glob. Change Biol. 18, 1725–1737. doi: 10.1111/j.1365-2486.2011.02636.x
Richardson, D. M., and Rejmánek, M. (2011). Trees and shrubs as invasive alien species-a global review. Divers. Distrib. 17, 788–809. doi: 10.1111/j.1472-4642.2011.00782.x
Seastedt, T. R. (2015). Biological control of invasive plant species: a reassessment for the Anthropocene. New Phytol. 205, 490–502. doi: 10.1111/nph.13065
Shipley, B., Vile, D., and Garnier, É (2006). From plant traits to plant communities: a statistical mechanistic approach to biodiversity. Science 314, 812–814. doi: 10.1126/science.1131344
van Kleunen, M., Weber, E., and Fischer, M. (2010). A meta-analysis of trait differences between invasive and non-invasive plant species. Ecol. Lett. 13, 235–245. doi: 10.1111/j.1461-0248.2009.01418.x
van Wilgen, B. W., Raghu, S., Sheppard, A. W., and Schaffner, U. (2020). Quantifying the social and economic benefits of the biological control of invasive alien plants in natural ecosystems. Curr. Opin. Insect Sci. 38, 1–5.
Wang, C., Jian, S., Ren, H., Yan, J., and Liu, N. (2021). A web-based software platform for restoration-oriented species selection based on plant functional traits. Front. Ecol. Evol. 9:570454. doi: 10.3389/fevo.2021.570454
Wang, C., Zhang, H., Liu, H., Jian, S., Yan, J., and Liu, N. (2020). Application of a trait-based species screening framework for vegetation restoration in a tropical coral island of China. Funct. Ecol. 34, 1193–1204. doi: 10.1111/1365-2435.13553
Williams, B. K. (2011). Adaptive management of natural resources—framework and issues. J. Environ. Manag. 92, 1346–1353. doi: 10.1016/j.jenvman.2010.10.041
Wolfe, B. T., and van Bloem, S. J. (2012). Subtropical dry forest regeneration in grass-invaded areas of Puerto Rico: understanding why Leucaena leucocephala dominates and native species fail. For. Ecol. Manag. 267, 253–261. doi: 10.1016/j.foreco.2011.12.015
Keywords: biocontrol invasion, invasive exotic plant species, Leucaena leucocephala, native plant species, trait-based native plant species selection software platform, tropical seasonal forest
Citation: Zhang H, Jiang K, Zhao Y, Xing YT, Ge HJ, Cui J, Liu TD and Wang C (2021) A Trait-Based Protocol for the Biological Control of Invasive Exotic Plant Species. Front. Ecol. Evol. 9:586948. doi: 10.3389/fevo.2021.586948
Received: 24 July 2020; Accepted: 08 April 2021;
Published: 30 April 2021.
Edited by:
Qinfeng Guo, United States Forest Service (USDA), United StatesReviewed by:
Riccardo Motti, Università degli Studi di Napoli Federico II, ItalyCopyright © 2021 Zhang, Jiang, Zhao, Xing, Ge, Cui, Liu and Wang. This is an open-access article distributed under the terms of the Creative Commons Attribution License (CC BY). The use, distribution or reproduction in other forums is permitted, provided the original author(s) and the copyright owner(s) are credited and that the original publication in this journal is cited, in accordance with accepted academic practice. No use, distribution or reproduction is permitted which does not comply with these terms.
*Correspondence: Chen Wang, Y2hlbi53YW5nQHNjYmcuYWMuY24=
†These authors have contributed equally to this work
Disclaimer: All claims expressed in this article are solely those of the authors and do not necessarily represent those of their affiliated organizations, or those of the publisher, the editors and the reviewers. Any product that may be evaluated in this article or claim that may be made by its manufacturer is not guaranteed or endorsed by the publisher.
Research integrity at Frontiers
Learn more about the work of our research integrity team to safeguard the quality of each article we publish.