- 1Department of Biology and Chemistry, Faculty of Chemistry, Biology, Geography, West University of Timisoara, Timisoara, Romania
- 2Department of Mechanics and Strength of Materials, Politehnica University of Timisoara, Timisoara, Romania
- 3Department of Informatics, Faculty of Mathematics and Computer Science, West University of Timisoara, Timisoara, Romania
- 4Institute of Biology Bucharest, Romanian Academy, Bucharest, Romania
- 5Faculty of Biology, University of Bucharest, Bucharest, Romania
- 6Department of Genetics, Evolution and Environment, University College London, London, United Kingdom
- 7Department of Functional Sciences and Pathophysiology, Center for Translational Research and Systems Medicine, “Victor Babeş” University of Medicine and Pharmacy, Timisoara, Romania
Multiple causes can determine the disturbance of natural equilibrium in a population of a species, with a common one being the presence of invasive competitors. Invasives can drive native species to the resettlement of the trophic position, changing reproduction strategies or even daily normal behaviours. Here, we investigated the hypothesis that more effective anatomical features of an intruder (Faxonius limosus) come with increased boldness behaviour, contributing to their invasion success in competition against the native species (Pontastacus leptodactylus). We tested the boldness of specimens representing the two species by video-based assessment of crayfish individuals’ attempts to leave their settlement microenvironment. The experiment was followed by a series of measurements concerning chelae biometry, force and muscle energetics. The native species was less expressive in terms of boldness even if it had larger chelae and better muscular tissue performance. In contrast, because of better biomechanical construction of the chelae, the invasive species was capable of twice superior force achievements, which expectedly explained its bolder behaviour. These findings suggest that, in interspecific agonistic interactions, the behaviour strategy of the invasive crayfish species is based on sheer physical superiority, whereas the native crayfish relies on intimidation display.
Introduction
Biological invasions are one of the most challenging issues for nowadays conservationists (Nentwig et al., 2018; Flood et al., 2020). The successful establishment of alien species in a new environment is generally driven by intrinsic mechanisms (Sullivan et al., 2017; Pacioglu et al., 2020) and extrinsic pathways (Light, 2003; Essl et al., 2015). Multiple perspectives have been approached to understand and depict the processes behind successful invasions, such as ecological (Lodge, 1993; South et al., 2020), economical (Perrings et al., 2002), or pathological (Strauss et al., 2012) ones. Eco-behavioural perspectives might allow more insights into the complex process of biological invasions. Here, we addressed the hypothesis that a successful crayfish invader is driven by its own bolder behaviour against shyness of the native resident. To test this, we used behaviour, morpho-mechanical and bioenergetic approaches.
Boldness, considered as the propensity of an animal to engage in risky activities (White et al., 2013), is giving the animals a presumed confidence against the unknown, associated with high diel activity and exploration initiatives (Chapple et al., 2012; Juette et al., 2014). The expression of boldness in agonistic confrontation is aggressivity and bite force superiority (Usio et al., 2001; De Meyer et al., 2019), and this behaviour was found associated with successful biological invasions (Ferrari et al., 2018). Analysing behavioural interactions between invasive and native residents may provide useful information for understanding the mechanisms behind the spreading success of invasives (Carere and Gherardi, 2013).
Relatively equal in body size (Richardson, 2019), the native narrow-clawed crayfish Pontastacus leptodactylus Eschscholtz 1823 and the invasive spiny-cheek crayfish Faxonius limosus (Rafinesque, 1817) are two crayfish species with a long interaction history in Europe (Holdich et al., 2009). The native species is a typical K-strategist, whereas the invasive crayfish shows characteristics of r-strategists, demonstrating plasticity and significant improvement of reproduction (i.e., increased fecundity) in the active front of invasion (Pârvulescu et al., 2015). Experimentally, it was demonstrated that the invasive species dominates the native one, even in highly imbalanced confrontations (e.g., smaller invasive vs. larger native specimens) for food and shelter (Lele and Pârvulescu, 2017). Crayfish chelae are used for gripping (Ion et al., 2020) and pinching (van der Meijden et al., 2010), or showing sheer superiority (Graham and Angilletta, 2020), hence are important in both intra- and interspecific interactions. Prolonged competition either drove the native species to local extinction or resource partitioning between species; recent investigations point to natural selection of heavier (i.e., stronger and combative) specimens in recovering populations after the invasion is established, compared to the non-invaded ones (Haubrock et al., 2019; Pacioglu et al., 2020).
In this study, we aimed to dig inside the bio-mechanical mechanisms behind a confrontation between two crayfish species, testing the hypothesis that more effective chela weapons came with increased boldness behaviour of the invasive species compared to a native one. The invasive F. limosus and the native P. leptodactylus were used as a models for multiple comparisons, including muscle metabolic capacity and fundamental for chelae function (Guderley and Couture, 2005).
Materials and Methods
Study Specimens and Morphological Measurements
To avoid biases caused by any sexual influences during the experiment, this study involved intermolt adult males only, collected from the most recent known invasion front in the Lower Danube (near Orşova, Romania, GPS 44.689064°N/22.500242°E), where both investigated species have been coexisting for at least 5 years (Pârvulescu et al., 2015; Pacioglu et al., 2020). This study omitted those specimens injured or showing obvious discrepancies between the left and the right chelae.
The geometric dimensions of the specimens were then acquired using a digital calliper of 0.1-mm accuracy. A total of nine dimensions were measured for every individual: the total length of the crayfish [TL (mm)], chela length, thickness and width [CL, CT, and CW (mm)] for left and right cheliped and also the length of the movable finger (dactylus, DL). Using the three dimensions of the chela, its volume was computed (CV) as an approximated elliptical cylinder, were the ellipse semi-axes were the half of the chela length and height, while the height of the cylinder was the chela width.
After measurements, each specimens’ chelae were detached for further investigation. The muscle of the propodus was extracted and oven-dried for 48 h at 60°C to obtain the dry muscle mass [DMM (g)]. The chela (propodus and dactylus) cuticle was also collected and dried in the same setup, obtaining the dry cuticle, and dry dactylus mass [DCM and DDM (g)]. These variables were measured by using a precision balance (Kern & Sohn GmbH, Balingen, Germany) of 0.001-g accuracy.
Experimental Set-Up for Boldness Assessment
In total, 73 specimens of P. leptodactylus and 78 of F. limosus were involved in the experiments. Aiming to obtain best results on the assessment of boldness behaviour, we acclimated crayfish prior to the experiments in the experimental arena (20-L water tank), for each trial, with ad libitum food and shelter but lacking the ramp (described below). The arena was placed in a second (larger) tank (60 L) to retain the successfully escaped individuals. Both tanks were equipped with a submersed filtering pump for cleaning and oxygenating water. A ramp, facilitating the escape from the inside of the arena, was built from adherent (ceramic) material inclined at 30°, with a length of 45 cm, with half of this length being submersed and half dry, making the crossing conditions more difficult. Such an aerial ramp simulates an environment that is potentially risky, more prone to predatory attacks; hence, approaching and crossing it by the crayfish can be used as a measure of the individual’s boldness. For a better understanding of the experimental setup, we refer readers to a video sample in the Supplementary Material. This set-up was placed into the area on the third day of the settlement period, allowing the individuals to freely explore the ramp and opening the way to a new environment in the larger tank. The experiment started once the ramp was inserted and lasted 24 h for each trial and was video recorded. The ethogram for each specimen consisted of the binary variable (0/1) of successful attempts to escape (further referred to as “sa” variable) in both day, and night, and, if the case, the duration (s) of the sa (time completed ramp crossing, referred as “tcrs” variable). To avoid any bias in crayfish activity caused by temperature (Seebacher and Wilson, 2006; Zhao and Feng, 2015), we maintained the environmental temperature for all the experiments at ∼20°C.
To obtain the first insights into the differences between the two crayfish species’ behaviour, exploratory data analysis was performed. We investigated if there was a difference between the sa of the two species. Crayfish individuals that have completed the crossing action were selected and their tcrs were compared using the non-parametric Mann-Whitney U test. In the same respect, we tested if the TL of the analysed crayfish influenced the crossing success, using a logistic regression model.
Force Measurement Device and Procedure
Pinching force measurements were conducted in vivo using a 1,400 g/0.1 g loading cell custom-made for measuring the pressure exerted by the tip of the crayfish chela. The transducer was connected to an Arduino Uno board, and the data were acquired using a sampling frequency of 6 Hz. On the transducer, two rigid metallic levers were attached for providing pinching elements for the chelae. To convert the electrical parameter of the transducer (mV) in mechanical force (N), the device calibration was conducted using five different weights: 100, 250, 500, 750, and 1,000 g, reading the corresponding voltage values. For measurement consistency, the transducer’s levers were placed at the tips of the chelae of all individuals. Also, the longitudinal direction of the chela and the levers were always as collinear as possible. The time span of the measurement was an average of 60 s for each investigated chela (a.k.a. claw).
The same 151 (73 P. leptodactylus and 78 F. limosus) crayfish male specimens used for previous experiments were used. While measuring the force values, some crayfish demonstrated high activity, generating several pinching cycles in the recorded time interval. On the other hand, some individuals manifested less activity and, therefore, fewer pinching cycles or just one was acquired. To obtain a representative biomechanical behaviour for each individual, the cycles were isolated and processed (Figure 1).
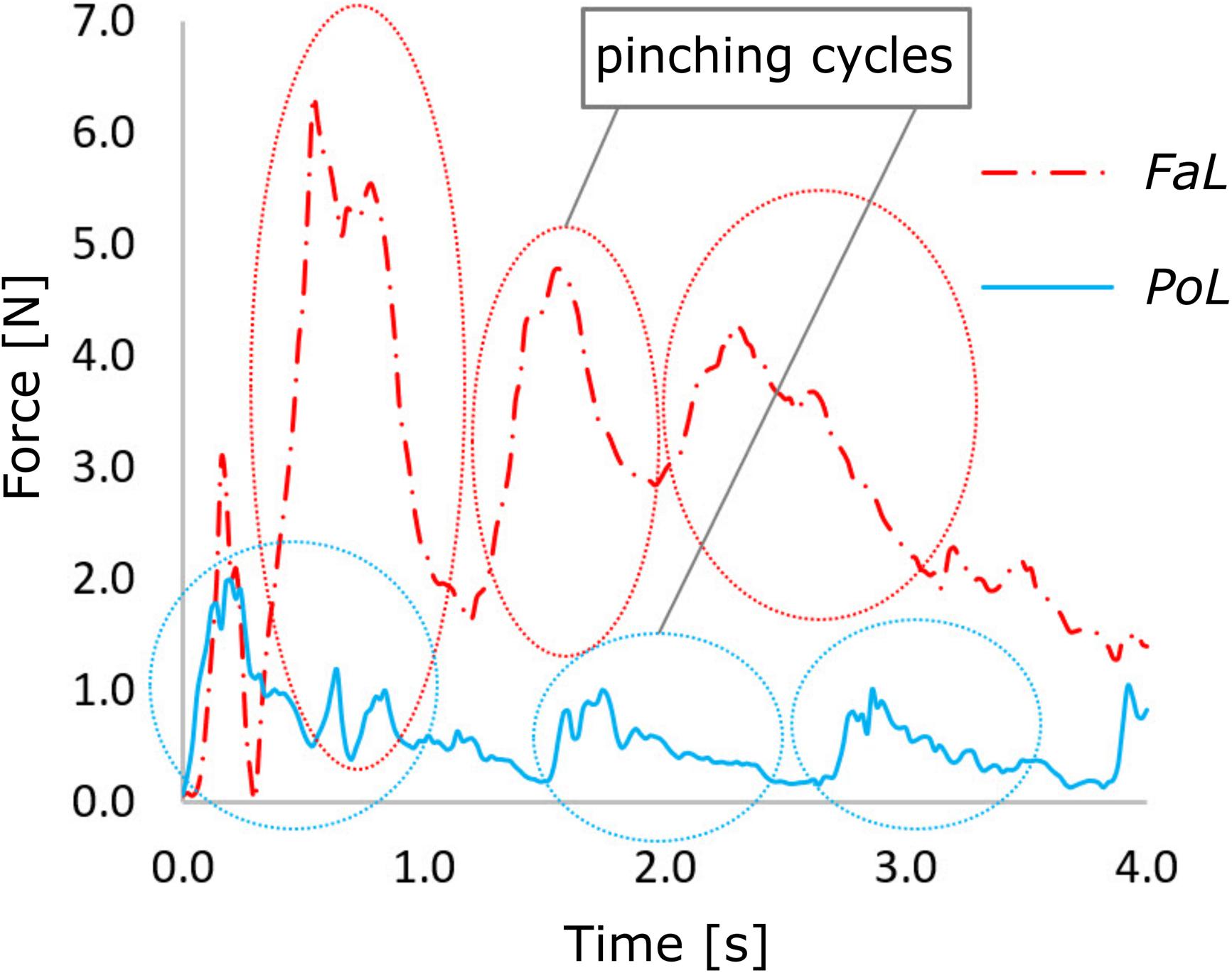
Figure 1. Pinching force cycles of two representative individuals of Pontastacus leptodactylus and Faxonius limosus.
Based on the pinching cycles, the absolute maximum force [Fmax (N)] was extracted for each crayfish of both species and used in raw data graphic representation. After extracting the pinching cycles from all active individuals, the force-time series were averaged for each single crayfish. Three parameters were directly extracted: average of maximum forces [FAv (N)], average contraction time [Ct (s)], and average releasing time [Rt (s)]. In addition, average loading velocity [LV (N/s)], average releasing velocity [RV (N/s)], and the energy of the pinching cycle [EN (N⋅s)] were computed. The LV represents the speed of the closing movement from zero force to maximum force and was computed as a numerical derivative of force in respect to time, using Eq. (1). A similar equation was used for computing RV, which represents the speed of releasing the grip from the maximum force value to zero or to an inflexion point from where the force increases again. The EN is an integrative parameter of force in respect to time and characterises the mechanical effort spent to complete a pinching cycle. It was computed by numerical integration using Eq. (2), were the two terms under the sum represent the area under the curve split in two elemental components of the area: a rectangle and a triangle. Here, Δt represents the time interval according to the sampling rate and Fi+1 and Fi represent consecutive values of instantaneous force.
Relationship Between Chelae Forces and Body Properties
To obtain the relationship between the body properties and the forces that crayfish produce, various multiple linear regression architectures were tested. The data variables considered as a continuous model outcome were as follows: FAv, EN, LV, RV, Rt, and Ct, and the predictive variables were modelled in two groups: based on the body size (TL, CL, CT, CW, DL, and CV) and based on the chelae weight (DMM, DCM, and DDM). Because crayfish are ambidextrous in the usage of chelae (Lele and Pârvulescu, 2019), we chose to group the associated data to perform the statistical analyses.
Due to the large multicollinearity among the predictive variables, stepwise regression was performed to remove the redundant ones. The predictors that were found to be significant based on the 0.05 cut-off in the final models were interpreted as those that have an effect on the specific outcome. Finally, the t-test was applied to investigate the difference between the two species for the biometric variables that were significant in the stepwise regression model describing the chelae forces outcomes at least for one of the species.
Mitochondrial High-Resolution Respirometry (HRR) Analysis
Aerobic metabolic capacity was measured as oxygen consumption of isolated mitochondria in the two groups of crayfish, the native crayfish group (P. leptodactylus, N = 6) and the invasive crayfish group (F. limosus, N = 7). Mitochondria were isolated by differential centrifugation at 4°C, according to a previously described method (Liu et al., 2013). Briefly, 1.5–2.5 g of muscle collected from the crayfish chela was cut into small pieces and homogenised in 30 mL isolation buffer (320 mM Sucrose, 10 mM Tris, 10 mM EGTA, 0.5% BSA, pH 7.3), using a tissue homogeniser (Glas-Col 099C K5424 CE). Homogenisation was performed at minimal speed (two 40 s periods/1 min interval), and the tissue homogenate was further centrifuged (Rotina centrifuge 38R) for 10 min at 2,000 g; the supernatant was centrifuged for 10 min at 12,000 g. Finally, the resulting mitochondrial rich pellet was re-suspended in 0.25 mL of isolation buffer in a glass homogeniser, kept on ice and used within 4 h in respiratory rate measurements. The mitochondrial protein concentration was further evaluated according to the Biuret method (Gornall et al., 1949).
Oxygen consumption was measured at 37°C with the Oxygraph-2k (Oroboros Instruments, Austria). The mitochondria (0.1 mg protein/mL) were incubated in 2 mL of incubation medium containing 0.5 mM EGTA, 3 mM MgCl2.6H2O, 60 mM K-lactobionate, 20 mM taurine, 10 mM KH2P04, 20 mM HEPES, 110 mM sucrose, 1 g/L BSA, essentially fatty acid free + 280 U/mL catalase lyophilised powder, 2,000–5,000 units/mg protein (pH 7.1, 37°C). The Substrate-Uncoupler-Inhibitor Titration (SUIT) protocol adapted from Duicu et al. (2013) was as follows: chamber A: GMSTATE 2 + ADPOXPHOS + cyt c + OmySTATE 4 + FCCPUncoupled state + AmaROX and chamber B: S(Rot)STATE 2 + ADPOXPHOS + cyt c + OmySTATE 4 + FCCP Uncoupled state + AmaROX. The protocol consisted of the following steps and the corresponding respiratory states: (i) addition of 10 mM glutamate/G and 2 mM malate/M (CI substrates) and 10 mM succinate/S (CII substrate) + 0.5 μM rotenone/Rot (CI inhibitor): STATE 2 (i.e., basal respiration); (ii) addition of 5 mM ADP as a measure of the maximal oxidative phosphorylation capacity: OXPHOS capacity; (iii) addition of 10 μM cytochrome c/cyt c to assess the intactness of the outer mitochondrial membrane; (iv) inhibition of ATP synthase by oligomycin/Omy (2 μg/ml): STATE 4 (i.e., basal respiration); (v) FCCP titration (0.5 μM steps), to obtain the Uncoupled respiration, (vi) inhibition of respiration with 2.5 μM antimycin A/Ama (Residual OXygen consumption): ROX state.
For high-resolution respirometry (HRR) experiments, the independent two-side Mann-Whitney U test was applied. Significance was tested based on the 0.05 cut-off, i.e., if the p-value was less than 0.05, the null hypothesis of two groups being equal was rejected. Also, the means and standard deviations (mean ± SD) are reported.
Results
Relationship Between Chelae Forces and Body Properties
After applying the stepwise regression for P. leptodactylus (Table 1), only TL had a significant influence on the FAv outcome. Regarding the outcome EN, no predictor was significant. The TL and CL were significant for the LV outcome. The same predictors, TL and CL, had a significant effect on the RV outcome. A significant relationship with the outcome Ct was found for CW. No significant relationships were found between chela weight and force variables (Table 2).
The results for F. limosus (Tables 1, 2) obtained after stepwise regression indicate that CL CW, DMM, and DCM have a significant influence on the FAv outcome. For the EN, the same predictors were significant. The CW was significantly related to outcome LV. For the outcome RV, no predictor was significant, whereas for outcome Ct, the variables CL, DMM, and DCM were significant.
To understand the differences in the results obtained for the two investigated species, the body variables that were significant (TL, CL, CW, DMM, and DCM) for at least one of the force variables were compared using the two-sample t-test, as all of the variables are normally distributed. Testing the one-sided hypothesis that the mean values of P. leptodactylus are greater than those of F. limosus, the values for TL, CL, CW, DMM, and DCM were significant. To visualise those significant differences, we refer readers to Figures 2A–E.
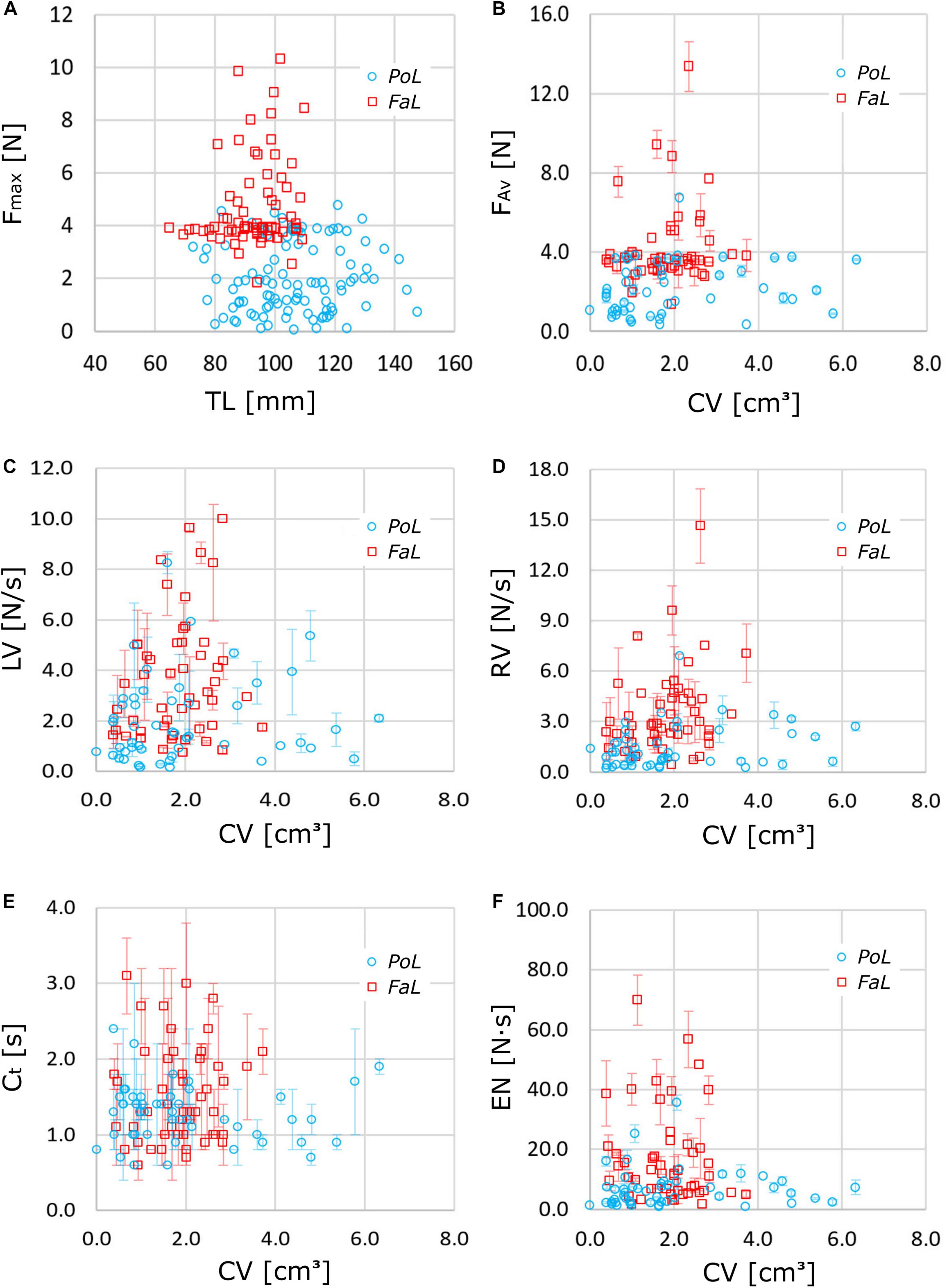
Figure 2. Boxplots showing the difference between P. leptodactylus and F. limosus for the statistically relevant variables: (A) total length (TL), (B) chela length (CL), (C) chela width (CW), (D) dry chela muscle mass (DMM), and (E) dry chela cuticle mass (DCM). Values represent means ± SD.
Boldness Assessment
Of all tested specimens, 5.8% of P. leptodactylus and 29.6% of F. limosus completely crossed the arena ramp. The Mann-Whitney U test was significant (P < 0.05) in the tcrs of the two species; F. limosus managed to cross the arena ramp much faster than P. leptodactylus. Analysis of the TL of the crayfish revealed no influence on the complete crossing success, with the logistic regression model (Table 3) showing no significant relationship between TL and the binary sa variable (0/1).

Table 3. Parameter estimates for logistic regression relationship models between crayfish total length (TL) and the binary successful attempts to escape (sa variable) to assess boldness behaviour.
The results differed significantly between day and night sa of the arena ramp for both species (P < 0.05), with successful crossing being encountered exclusively during the night in native species, whereas 7.9% of the successful crossings for the invasive species were recorded in daylight.
Force Assessment
Raw data analysis revealed that the invasive species F. limosus exhibited higher Fmax and FAv compared to P. leptodactylus, with some individuals producing more than double values (Figures 3A,B). The maximum limit of the force recorded for P. leptodactylus represented the lower limit for F. limosus. A body size in the range of 90 to 100 mm TL seemed to be suitable for the highest force development in F. limosus, while no force-length relation could be observed for P. leptodactylus. The LV, a measure of how quickly the maximum force is achieved, appeared to be similar for the two investigated species (Figure 3C); for a CV of around 2 cm3, the maximum LV was achieved in both crayfish species. The average RV (Figure 3D) showed a higher releasing rate for F. limosus, indicating that muscle relaxation occurs much faster for F. limosus. Most individuals of both species exhibited a releasing velocity of around 3 N/s. Larger Ct values could be observed for F. limosus (Figure 3E). Because no obvious difference in loading velocity was identified between the species, the larger Ct might be determined by the higher force values produced by F. limosus. The EN (Figure 3F) was also higher for F. limosus, a normal evolution of this parameter since the forces and contraction times were also larger. The faster releasing rate does, however, not compensate for the other two parameters. Higher mechanical energy was recorded in both species for smaller CV values.
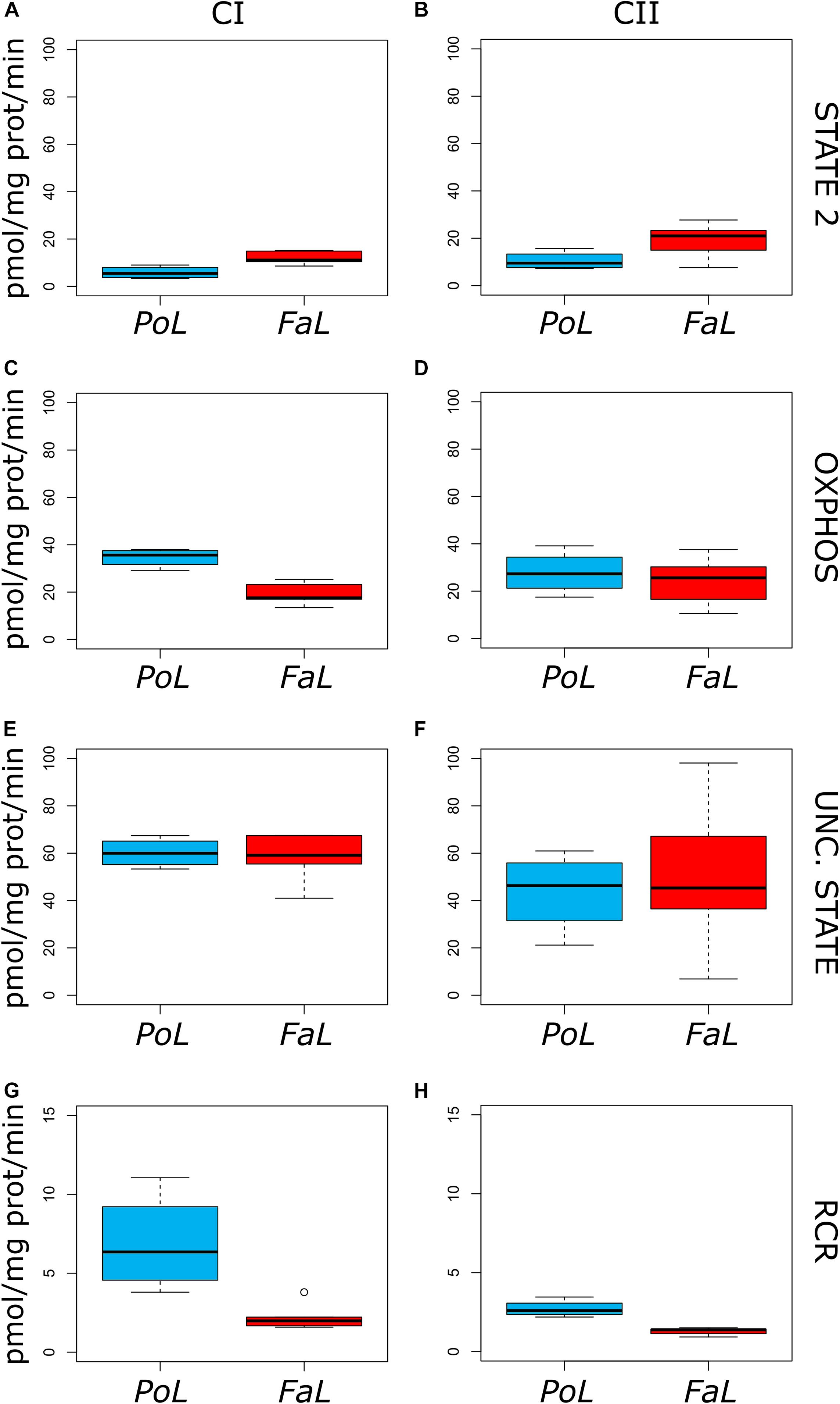
Figure 3. Raw data of force and biometrical parameters: (A) maximum pinching force (Fmax) vs. total length (TL), (B) average of maximum pinching forces (FAv) vs. chela volume (CV), (C) average loading velocity (LV) vs. CV, (D) average releasing velocity (RV) vs. CV, (E) contraction time (Ct) vs. CV, and (F) energy of the pinching cycle (EN) vs. CV.
HRR Assessment
The results of HRR measurements in the analysed groups are presented in Figure 4. To calculate the respiratory rates, we used the Oroboros software Datlab. Mitochondrial respiration was corrected for oxygen flux due to the instrumental background and ROX (Gnaiger, 2014).
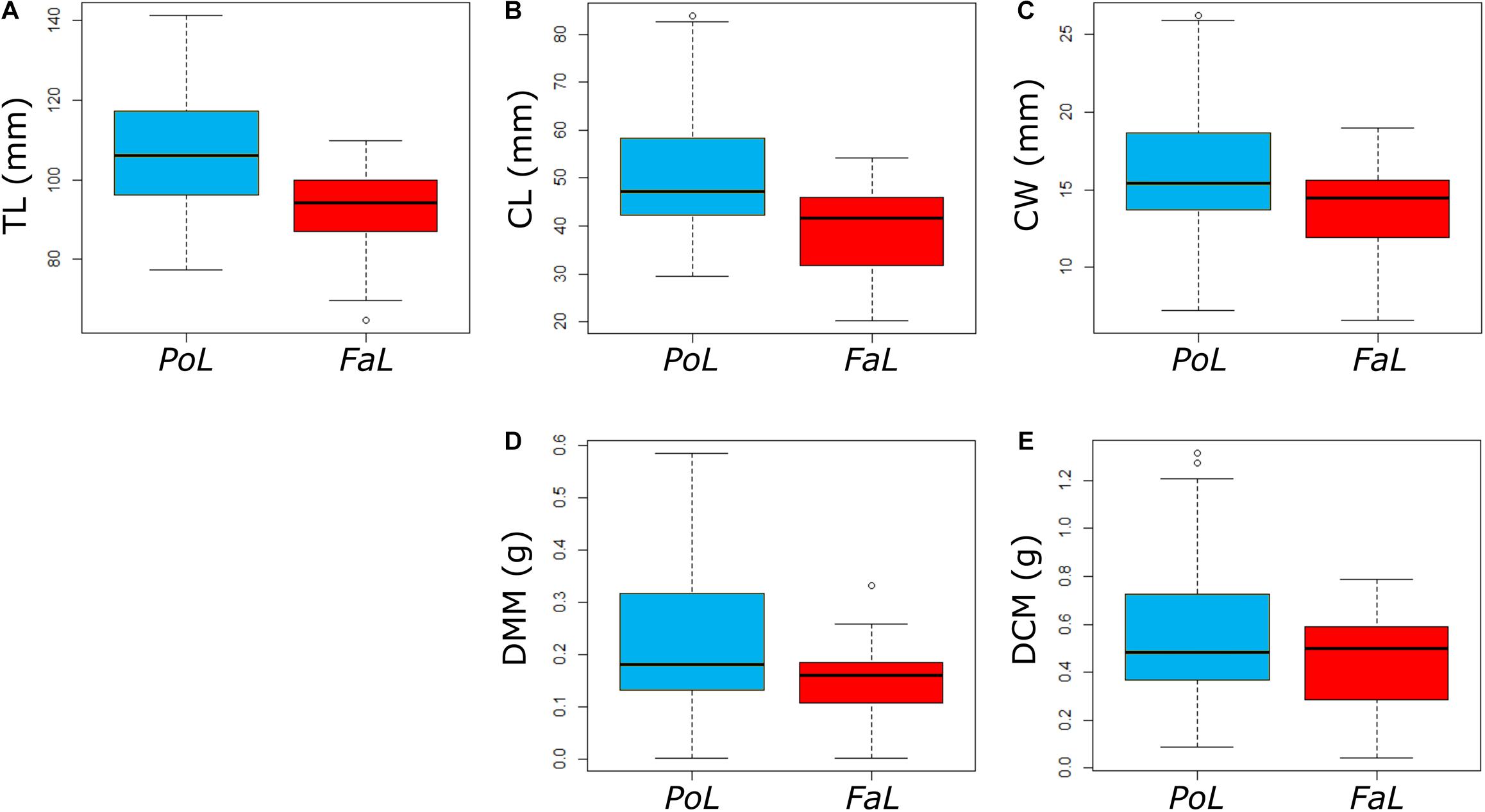
Figure 4. High-resolution respirometry data for CI-supported (A,C,E,G) and CII-supported (B,D,F,H) respiration in Pontastacus leptodactylus and Faxonius limosus. Values represent means ± SD.
Based on a previous study, STATE 2 (i.e., basal respiration corresponding to mitochondria oxidising substrates when the membrane is fully energised) is determined by the intrinsic proton conductivity of the inner membrane, which dissipates the ΔΨ (Harris and Das, 1991). In mitochondria respiring on glutamate + malate, we found a statistically significant increase in basal respiratory state in F. limosus vs. P. leptodactylus groups (Figure 4A): STATE 2 12.04 ± 2.8 vs. 5.8 ± 2.6 pmol.s–1.mL–1 (P < 0.05). Addition of ADP stimulates oxidative phosphorylation (OXPHOS capacity), which induces a drop in membrane potential and a subsequent increase in respiration (Harris and Das, 1991). In our results, OXPHOS was significantly lower in the F. limosus group energised with CI substrates (Figure 4C): 19.33 ± 4.8 vs. 34.6 ± 3.9 pmol.s–1.mL–1 (P < 0.05). Addition of FCCP, a protonophore, induces the inhibition of oxidative phosphorylation due to dissipation of the membrane potential, leading to the increase in respiration (i.e., phosphorylation and respiration become uncoupled) (Harris and Das, 1991). In mitochondria energised with glutamate + malate, the Uncoupled State (Figure 4E) was similar in F. limosus vs. P. leptodactylus groups: 58.1 ± 10.9 vs. 60.15 ± 6.2 pmol.s–1.mL–1 (P NS).
In the presence of CII-dependent substrate, we observed the same trend of mitochondrial respiratory rates in F. limosus vs. P. leptodactylus groups, albeit in a lesser (not significant) degree as compared to CI-supported respiration: STATE 2 (Figure 3B) 19 ± 7.1 vs. 10.5 ± 3.8 pmol.s–1.mL–1 (P NS), OXPHOS (Figure 4D) 23.9 ± 9.7 vs. 27.8 ± 9.1 pmol.s–1.mL–1 (P NS) and the Uncoupled State (Figure 4F) 51.7 ± 30.6 vs. 43.7 ± 16.9 pmol.s–1.mL–1 (P NS).
We further calculated the Respiratory Control Ratio (RCR) as the OXPHOS/State 2 ratio. Under both experimental conditions, RCR was significantly decreased (P < 0.05) in the F. limosus group (Figures 4G,H) as a direct reflection of the STATE 2 increase and the inhibition of ADP phosphorylation.
Discussion
The Competitors’ Bio-Mechanical Performance
The invasive crayfish F. limosus was more confident than the native P. leptodactylus, taking more risks as demonstrated by our laboratory experiments. The native P. leptodactylus appeared to be more shy, probably explaining other findings such as its limited access to shelter and food resources (Lele and Pârvulescu, 2017; Pacioglu et al., 2020). The species F. limosus exhibited superior values of every parameter except those related to body size, emphasising the differences also observed from a biomechanical point of view. The average of maximum forces applied by F. limosus revealed pinching forces of 2.3 times higher than for P. leptodactylus. This advantage becomes even more relevant in the context of the total body length of individuals, which did not influence the mechanism of pinching force development. However, it may contribute to the body stability condition of the larger species during a fight (Zeil and Hemmi, 2006; Bywater et al., 2008; Malavé et al., 2018; Kuo et al., 2019).
Force production is a physiological attribute of the adductor muscle, which in turn is proportional to the chelae volume. On the other hand, force transmission depends on the length of the mobile dactylus of the chelae, and therefore, both aspects should be taken into account. The reaction force measured at the tip of the claw can be explained by the lever mechanism developed by the muscle force through the physiological distances, as depicted in Figure 5. The value of the reaction force at the tip of the claw is directly influenced by the muscular force value and its insertion point, while being negatively influenced by the length of the claw. This is caused by the effect or the moment of torque developed by the forces and physiological distances. Therefore, for a constant muscle force, as the claw became longer the reaction force will be smaller. This biomechanical behaviour may explain the smaller pinching forces of P. leptodactylus species, which possesses longer claws compared to F. limosus. This disadvantage is apparently neither compensated by the observed better muscular energetic efficiency in P. leptodactylus nor by the increased physiological distance between the fulcrum and the adductor insertion point.
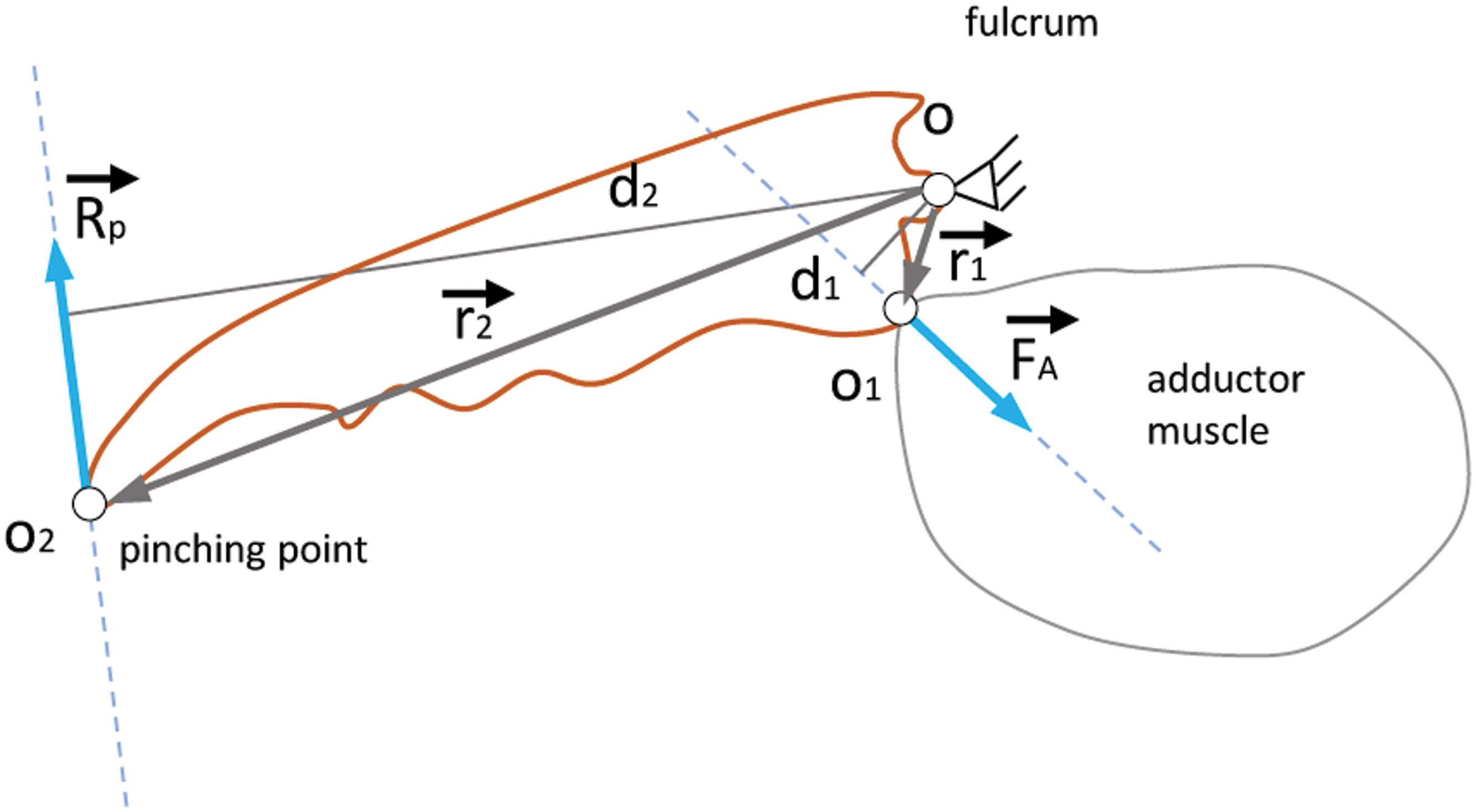
Figure 5. Mechanism of force production and transmission. The equation that governs the biomechanical equilibrium is where Rp is the reaction force at the tip of the claw, FA is the adductor muscle force and d1 and d2 are the lever arms.
Investigations on pinching cycle energy revealed higher values for F. limosus, which leads us to infer that this crayfish species not only produces higher forces but is also capable of sustaining this force for an extended period of time compared to the native P. leptodactylus. The energy of the pinching cycle will create a significant advantage for the invasive in clash with the native species, since the application of a force for a longer time allows crack growth and propagation up to the fracture point in any biological (Egan et al., 2015) or non-living structures (Linul et al., 2020). The biomechanical parameters (biometrics and forces) make the invader prone to win most of the fights, as revealed in other studies regarding confrontations between these two species for food and shelter (Lele and Pârvulescu, 2017). The agonistic behaviour strategy of the invasive crayfish species appears to be based on physical superiority, whereas the native crayfish works on intimidation display.
The Competitors’ Chelae Muscular Performance
There is evidence that metabolic capacity changes with thermal acclimation in crayfish (Seebacher and Wilson, 2006), and this might counteract threats from invasive species. We found that RCR, an indicator of the efficacy of oxidative ATP production (Montaigne et al., 2011), was significantly higher in native crayfish, and mitochondrial respiration rates, using the physiologically relevant substrate mixtures (i.e., glutamate + malate), significantly differed between F. limosus and P. leptodactylus when compared to using succinate (+rotenone) as the substrate. Thereby, an important finding of our study is that mitochondrial respiration in crayfish chelae is substrate-dependent, with a preference for CI substrates.
Another finding in our experiments was that the STATE 2 respiration rate was increased in the F. limosus group under both experimental conditions (with a significant difference in CI-supported respiration, see Figure 4), indicating partial mitochondrial uncoupling; yet, mitochondrial uncoupling was not complete since mitochondrial respiration further increased after the addition of ADP (Figure 4). Mild uncoupling of mitochondrial respiration, leading to the decrease in membrane potential, could yet be beneficial since it prevents the excessive generation of reactive oxygen species (Skulachev, 1998).
Finally, a major indicator of mitochondrial function is RCR, which represents a measure of the efficiency of oxidative phosphorylation. Accordingly, another important finding of our study was that RCR in the F. limosus group was significantly lower in mitochondria energised with both substrate types. This result, together with the finding of OXPHOS inhibition, indicates that the chelae muscle mitochondria of this species use oxygen less efficiently compared to P. leptodactylus. Literature data suggest that dominance can modulate behavioural dynamics through a better synchronisation of biomechanical activities (Alcala et al., 2019).
Further Coexistence Scenario
Boldness is often related to intense activity and exploration and less neophobic behaviour (Chapple et al., 2012), with bolder individuals being more likely to be found in crowded environments (Evans et al., 2010), and often associated with invasive species ready to assume more risks (Hazlett et al., 2003; Pintor et al., 2008; Reisinger et al., 2017). Invasive crayfish encounter, along their paths, many different habitats, with changing environments and biological associations. Our data detected a bolder behaviour of invasives in novel environments over the native crayfish, supporting the findings that animals in prolonged highly changeable environments display less or no neophobia (Modlinska and Stryjek, 2016; Meuthen et al., 2019). Moreover, these results confirm a similar behaviour pattern of invasive species, such as cane toads, in introduced habitats (Candler and Bernal, 2014).
The fighting strategies (both inter- and intraspecific) of different species vary (Fořt et al., 2019), and although body size and chelae display are important in the initial assessment of the competitor, these are no longer good indicators of the outcome once the fight is initiated, as there is evidence that these are unreliable signals of actual chelae strength (Walter et al., 2011; Angilletta and Wilson, 2012). Consequently, species aggressivity (Fořt et al., 2019) or even sheer force, as measured for F. limosus in our study, influences the individual’s rank. Equally important, prior research substantiated the idea that social rank position and boldness level in animals are at least connected by the same influencing factors (Boogert et al., 2006; Mettler and Shivik, 2007). In our case, superior chela force seems determinant in dominance and, therefore, in bolder behaviour, both being advantageous for the invasive species.
The behavioural expression of boldness is influenced by various factors such as resource availability, predation-risk cues or even temperature (Hazlett et al., 2003; Seebacher and Wilson, 2006; Juette et al., 2014; Zhao and Feng, 2015; Reisinger et al., 2017). Studies primarily investigating the trophic position of these two crayfish species, in the same habitat of the Lower Danube, revealed that the invasive F. limosus outcompetes the native P. leptodactylus, pushing the latter to a lower position (Pacioglu et al., 2019, 2020). Still, in this context, recovering pockets (i.e., sub-populations) have been documented, where males of P. leptodactylus have a larger chelae size compared to those populations that did not encounter the pressure of invasive species competition (Pacioglu et al., 2020). The reduced genetic structure of these recovering populations (Pacioglu et al., 2020) led to the assumption of a kind of resettlement of both crayfish species’ competition over time, after invasion. Apparently, the native species selectively increased its weapon in size in the detriment of force (Seebacher and Wilson, 2006), whereas the invasive species may already have passed through the basic establishment period of a self-sustaining population in the invasion dynamics (Song et al., 2006; Blackburn et al., 2011; Hudina et al., 2015), documented as resulting in the increase of its biological (i.e., foraging and reproductive) conditions (Pârvulescu et al., 2015; Pacioglu et al., 2020). This would be an encouraging scenario for these two species to further reach a reasonable equilibrium of their coexistence.
Data Availability Statement
The raw data supporting the conclusions of this article will be made available by the authors upon request, without undue reservation.
Ethics Statement
All applicable International, National, and Institutional Guidelines for the care and use of animals were followed. No protected or rare species were involved in this study. After the specimens were analysed, they were euthanised by freezing to −20°C (Oidtmann et al., 2002) to avoid any environmental contamination with the widespread crayfish plague pathogen Aphanomyces astaci (Ungureanu et al., 2020).
Author Contributions
LP conceived the experiments. AEP conducted the laboratory investigations. IM provided assistance for measurement device. MCI, MS, and MV conducted the biometric measurements. DIS processed and interpreted the biomechanical data. MDM and OMA conducted and interpreted the muscle energetic investigations. KM statistically analysed the results. LP, DIS, MDM, OMA, and MCI wrote the first version of the manuscript. All authors read and approved the final manuscript.
Funding
This work was partially supported by a grant of the Romanian National Authority for Scientific Research and Innovation (UEFISCDI), project code PN-III-P4-ID-PCE-2020-1187. MCI was supported by the Institute of Biology Bucharest, Romanian Academy, project no. RO1567-IBB04/2020.
Conflict of Interest
The authors declare that the research was conducted in the absence of any commercial or financial relationships that could be construed as a potential conflict of interest.
Acknowledgments
Andrei Năstase was acknowledged for providing crayfish specimens for this study, Alexandra Almăjan for supporting laboratory processes, and Coşa Alexandru for data processing and computation of derived biomechanical parameters. We thank the two reviewers whose valuable suggestions significantly improved the manuscript.
Supplementary Material
The Supplementary Material for this article can be found online at: https://www.frontiersin.org/articles/10.3389/fevo.2021.581247/full#supplementary-material
References
Alcala, R. S., Caliva, J. M., Flesia, A. G., Marin, R. H., and Kembro, J. M. (2019). Aggressive dominance can decrease behavioral complexity on subordinates through synchronization of locomotor activities. Commun. Biol. 2:467. doi: 10.1038/s42003-019-0710-1
Angilletta, M. J. J., and Wilson, R. S. (2012). Cryptic asymmetry: unreliable signals mask asymmetric performance of crayfish weapons. Biol. Lett. 8, 551–553. doi: 10.1098/rsbl.2012.0029
Blackburn, T. M., Pyšek, P., Bacher, S., Carlton, J. T., Duncan, R. P., Jarošík, V., et al. (2011). A proposed unified framework for biological invasions. Trends Ecol. Evol. 26, 333–339. doi: 10.1016/j.tree.2011.03.023
Boogert, N. J., Reader, S. M., and Laland, K. N. (2006). The relation between social rank, neophobia and individual learning in starlings. Anim. Behav. 72, 1229–1239. doi: 10.1016/J.ANBEHAV.2006.02.021
Bywater, C. L. Jr., Angilletta, M. J., and Wilson, R. S. (2008). Weapon size is a reliable indicator of strength and social dominance in female slender crayfish (Cherax dispar). Funct. Ecol. 22, 311–316. doi: 10.2307/20142807
Candler, S., and Bernal, X. E. (2014). Differences in neophobia between cane toads from introduced and native populations. Behav. Ecol. 26, 97–104. doi: 10.1093/beheco/aru162
Carere, C., and Gherardi, F. (2013). Animal personalities matter for biological invasions. Trends Ecol. Evol. 28, 5–6. doi: 10.1016/j.tree.2012.10.006
Chapple, D. G., Simmonds, S. M., and Wong, B. B. M. (2012). Can behavioral and personality traits influence the success of unintentional species introductions? Trends Ecol. Evol. 27, 57–64. doi: 10.1016/J.TREE.2011.09.010
De Meyer, J., Irschick, D. J., Vanhooydonck, B., Losos, J. B., Adriaens, D., and Herrel, A. (2019). The role of bite force in the evolution of head shape and head shape dimorphism in Anolis lizards. Funct. Ecol. 33, 2191–2202. doi: 10.1111/1365-2435.13438
Duicu, O. M., Mirica, S. N., Gheorgheosu, D. E., Privistirescu, A. I., Fira-Mladinescu, O., and Muntean, D. M. (2013). Ageing-induced decrease in cardiac mitochondrial function in healthy rats. Can. J. Physiol. Pharmacol. 91, 593–600. doi: 10.1139/cjpp-2012-0422
Egan, P., Sinko, R., Leduc, P. R., and Keten, S. (2015). The role of mechanics in biological and bio-inspired systems. Nat. Commun. 6:7418. doi: 10.1038/ncomms8418
Essl, F., Bacher, S., Blackburn, T. M., Booy, O., Brundu, G., Brunel, S., et al. (2015). Crossing frontiers in tackling pathways of biological invasions. Bioscience 65, 769–782. doi: 10.1093/biosci/biv082
Evans, J., Boudreau, K., and Hyman, J. (2010). Behavioural syndromes in Urban and rural populations of song sparrows. Ethology 116, 588–595. doi: 10.1111/j.1439-0310.2010.01771.x
Ferrari, M. C. O., Brown, G. E., and Chivers, D. P. (2018). Understanding the effect of uncertainty on the development of neophobic antipredator phenotypes. Anim. Behav. 136, 101–106. doi: 10.1016/j.anbehav.2017.11.024
Flood, P. J., Duran, A., Barton, M., Mercado-Molina, A. E., and Trexler, J. C. (2020). Invasion impacts on functions and services of aquatic ecosystems. Hydrobiologia 847, 1571–1586. doi: 10.1007/s10750-020-04211-3
Fořt, M., Hossain, M. S., Kouba, A., Buřič, M., and Kozák, P. (2019). Agonistic interactions and dominance establishment in three crayfish species non-native to Europe. Limnologica 74, 73–79. doi: 10.1016/J.LIMNO.2018.11.003
Gnaiger, E. (2014). Mitochondrial Pathways and Respiratory Control. An Introduction to OXPHOS Analysis, ed. E. Gnaiger (Innsbruck: MiPNet Publications).
Gornall, A. G., Bardawill, C. J., and David, M. M. (1949). Determination of serum proteins by means of the biuret reaction. J. Biol. Chem. 177, 751–766.
Graham, Z. A., and Angilletta, M. J. (2020). Claw size predicts dominance within and between invasive species of crayfish. Anim. Behav. 166, 153–161. doi: 10.1016/j.anbehav.2020.06.021
Guderley, H., and Couture, P. (2005). Stickleback fights: Why do winners win? Influence of metabolic and morphometric parameters. Physiol. Biochem. Zool. 78, 173–181. doi: 10.1086/425204
Harris, D. A., and Das, A. M. (1991). Control of mitochondrial ATP synthesis in the heart. Biochem. J. 280, 561–573. doi: 10.1042/bj2800561
Haubrock, P. J., Balzani, P., Azzini, M., Inghilesi, A. F., Veselý, L., Guo, W., et al. (2019). Shared histories of co-evolution may affect trophic interactions in a freshwater community dominated by alien species. Front. Ecol. Evol. 7:355. doi: 10.3389/fevo.2019.00355
Hazlett, B. A., Burba, A., Gherardi, F., and Acquistapace, P. (2003). Invasive species of crayfish use a broader range of predation-risk cues than native species. Biol. Invasions 5, 223–228. doi: 10.1023/A:1026114623612
Holdich, D. M., Reynolds, J. D., Souty-Grosset, C., and Sibley, P. J. (2009). A review of the ever increasing threat to European crayfish from non-indigenous crayfish species. Knowl. Manag. Aquat. Ecosyst. 394-395:11. doi: 10.1051/kmae/2009025
Hudina, S., Žganec, K., and Hock, K. (2015). Differences in aggressive behaviour along the expanding range of an invasive crayfish: an important component of invasion dynamics. Biol. Invasions 17, 3101–3112. doi: 10.1007/s10530-015-0936-x
Ion, M. C., Puha, A. E., Suciu, T., and Pârvulescu, L. (2020). Get a grip: unusual disturbances drive crayfish to improvise. Behaviour 157, 101–120. doi: 10.1163/1568539x-00003583
Juette, T., Cucherousset, J., and Cote, J. (2014). Animal personality and the ecological impacts of freshwater non-native species. Curr. Zool. 60, 417–427. doi: 10.1093/czoolo/60.3.417
Kuo, J. H., Chang, Y. T., Chen, Y. J., and Hsu, Y. (2019). Influence of previous agonistic interactions with conspecifics on contest decisions. Ethology 125, 660–668. doi: 10.1111/eth.12919
Lele, S.-F., and Pârvulescu, L. (2017). Experimental evidence of the successful invader Orconectes limosus outcompeting the native Astacus leptodactylus in acquiring shelter and food. Biologia 72, 877–885. doi: 10.1515/biolog-2017-0094
Lele, S.-F., and Pârvulescu, L. (2019). Crayfish chelae usage suggests predominantly ambidextrous habitude. Crustaceana 92, 257–267. doi: 10.1163/15685403-00003886
Light, T. (2003). Success and failure in a lotic crayfish invasion: the roles of hydrologic variability and habitat alteration. Freshw. Biol. 48, 1886–1897. doi: 10.1046/j.1365-2427.2003.01122.x
Linul, E., Marsavina, L., and Stoia, D. I. (2020). Mode I and II fracture toughness investigation of Laser-Sintered Polyamide. Theor. Appl. Fract. Mech. 106, 102497. doi: 10.1016/j.tafmec.2020.102497
Liu, Z.-M., Wang, G.-Z., Wu, L.-S., Zeng, Z.-S., and Chen, X.-L. (2013). Seasonal change in mitochondrial function and metabolic enzyme activity of different populations of the mud crab, Scylla paramamosain, from China. Aquaculture 376-379, 68–75. doi: 10.1016/J.AQUACULTURE.2012.11.007
Lodge, D. M. (1993). Biological invasions: lessons for ecology. Trends Ecol. Evol. 8, 133–137. doi: 10.1016/0169-5347(93)90025-K
Malavé, B. M., Styga, J. M., and Clotfelter, E. D. (2018). Size, shape, and sex-dependent variation in force production by crayfish chelae. J. Morphol. 279, 312–318. doi: 10.1002/jmor.20773
Mettler, A. E., and Shivik, J. A. (2007). Dominance and neophobia in coyote (Canis latrans) breeding pairs. Appl. Anim. Behav. Sci. 102, 85–94. doi: 10.1016/J.APPLANIM.2006.03.012
Meuthen, D., Ferrari, M., Lane, T., and Chivers, D. (2019). High background risk induces risk allocation rather than generalized neophobia in the fathead minnow. Behav. Ecol. 30, 1416–1424. doi: 10.1093/beheco/arz094
Modlinska, K., and Stryjek, R. (2016). Food neophobia in wild rats (Rattus norvegicus) inhabiting a changeable environment—a field study. PLoS One 11:e0156741. doi: 10.1371/journal.pone.0156741
Montaigne, D., Marechal, X., Preau, S., Baccouch, R., Modine, T., Fayad, G., et al. (2011). Doxorubicin induces mitochondrial permeability transition and contractile dysfunction in the human myocardium. Mitochondrion 11, 22–26. doi: 10.1016/j.mito.2010.06.001
Nentwig, W., Bacher, S., Kumschick, S., Pyšek, P., and Vilà, M. (2018). More than “100 worst” alien species in Europe. Biol. Invasions 20, 1611–1621. doi: 10.1007/s10530-017-1651-6
Oidtmann, B., Heitz, E., Rogers, D., and Hoffmann, R. (2002). Transmission of crayfish plague. Dis. Aquat. Organ. 52, 159–167. doi: 10.3354/dao052159
Pacioglu, O., Theissinger, K., Alexa, A., Samoilă, C., Sîrbu, O. I., Schrimpf, A., et al. (2020). Multifaceted implications of the competition between native and invasive crayfish: a glimmer of hope for the native’s long-term survival. Biol. Invasions 22, 827–842. doi: 10.1007/s10530-019-02136-0
Pacioglu, O., Zubrod, J. P., Schulz, R., Jones, J. I., and Pârvulescu, L. (2019). Two is better than one: combining gut content and stable isotope analyses to infer trophic interactions between native and invasive species. Hydrobiologia 839, 25–35. doi: 10.1007/s10750-019-03990-8
Pârvulescu, L., Pîrvu, M., Moroşan, L.-G., and Zaharia, C. (2015). Plasticity in fecundity highlights the females’ importance in the spiny-cheek crayfish invasion mechanism. Zoology 118, 424–432. doi: 10.1016/J.ZOOL.2015.08.003
Perrings, C., Williamson, M., Barbier, E. B., Delfino, D., Dalmazzone, S., Shogren, J., et al. (2002). Biological invasion risks and the public good: an economic perspective. Conserv. Ecol. 6:1. doi: 10.5751/ES-00396-060101
Pintor, L. M., Sih, A., and Bauer, M. L. (2008). Differences in aggression, activity and boldness between native and introduced populations of an invasive crayfish. Oikos 117, 1629–1636. doi: 10.1111/j.1600-0706.2008.16578.x
Reisinger, L. S., Elgin, A. K., Towle, K. M., Chan, D. J., and Lodge, D. M. (2017). The influence of evolution and plasticity on the behavior of an invasive crayfish. Biol. Invasions 19, 815–830. doi: 10.1007/s10530-016-1346-4
Richardson, A. (2019). Body size in freshwater crayfish: an intercontinental comparison. Freshw. Crayfish 24, 43–47. doi: 10.5869/fc.2019.v24-1.43
Seebacher, F., and Wilson, R. S. (2006). Fighting fit: thermal plasticity of metabolic function and fighting success in the crayfish Cherax destructor. Funct. Ecol. 20, 1045–1053. doi: 10.1111/j.1365-2435.2006.01194.x
Skulachev, V. P. (1998). Uncoupling: new approaches to an old problem of bioenergetics. Biochim. Biophys. Acta Bioenerg. 1363, 100–124. doi: 10.1016/S0005-2728(97)00091-1
Song, C. K., Herberholz, J., and Edwards, D. H. (2006). The effects of social experience on the behavioral response to unexpected touch in crayfish. J. Exp. Biol. 209, 1355–1363. doi: 10.1242/jeb.02126
South, J., Madzivanzira, T. C., Tshali, N., Measey, J., and Weyl, O. L. F. (2020). In a pinch: mechanisms behind potential biotic resistance toward two invasive crayfish by native African freshwater crabs. Front. Ecol. Evol. 8:72. doi: 10.3389/fevo.2020.00072
Strauss, A., White, A., and Boots, M. (2012). Invading with biological weapons: the importance of disease-mediated invasions. Funct. Ecol. 26, 1249–1261. doi: 10.1111/1365-2435.12011
Sullivan, L. L., Li, B., Miller, T. E. X., Neubert, M. G., and Shaw, A. K. (2017). Density dependence in demography and dispersal generates fluctuating invasion speeds. Proc. Natl. Acad. Sci. U.S.A. 114, 5053–5058. doi: 10.1073/pnas.1618744114
Ungureanu, E., Mojžišová, M., Tangerman, M., Ion, M. C., Parvulescu, L., and Petrusek, A. (2020). The spatial distribution of Aphanomyces astaci genotypes across Europe: introducing the first data from Ukraine. Freshw. Crayfish 25, 77–87. doi: 10.5869/fc.2020.v25-1.077
Usio, N., Konishi, M., and Nakano, S. (2001). Species displacement between an introduced and A ‘vulnerable’ crayfish: the role of aggressive interactions and shelter competition. Biol. Invasions 3, 179–185. doi: 10.1023/A:1014573915464
van der Meijden, A., Herrel, A., and Summers, A. (2010). Comparison of chela size and pincer force in scorpions; getting a first grip. J. Zool. 280, 319–325. doi: 10.1111/j.1469-7998.2009.00628.x
Walter, G. M., van Uitregt, V. O., and Wilson, R. S. (2011). Social control of unreliable signals of strength in male but not female crayfish, Cherax destructor. J. Exp. Biol. 214, 3294–3299. doi: 10.1242/jeb.056754
White, J. R., Meekan, M. G., McCormick, M. I., and Ferrari, M. C. O. (2013). A comparison of measures of boldness and their relationships to survival in young fish. PLoS One 8:e68900. doi: 10.1371/journal.pone.0068900
Zeil, J., and Hemmi, J. M. (2006). The visual ecology of fiddler crabs. J. Comp. Physiol. A Neuroethol. Sens. Neural Behav. Physiol. 192, 1–25. doi: 10.1007/s00359-005-0048-7
Keywords: behaviour, competition, Faxonius limosus, invasive species, Pontastacus leptodactylus
Citation: Pârvulescu L, Stoia DI, Miok K, Ion MC, Puha AE, Sterie M, Vereş M, Marcu I, Muntean MD and Aburel OM (2021) Force and Boldness: Cumulative Assets of a Successful Crayfish Invader. Front. Ecol. Evol. 9:581247. doi: 10.3389/fevo.2021.581247
Received: 08 July 2020; Accepted: 20 January 2021;
Published: 10 February 2021.
Edited by:
Japo Jussila, University of Eastern Finland, FinlandReviewed by:
Pierre Horwitz, Edith Cowan University, AustraliaMarco Gamba, University of Turin, Italy
Copyright © 2021 Pârvulescu, Stoia, Miok, Ion, Puha, Sterie, Vereş, Marcu, Muntean and Aburel. This is an open-access article distributed under the terms of the Creative Commons Attribution License (CC BY). The use, distribution or reproduction in other forums is permitted, provided the original author(s) and the copyright owner(s) are credited and that the original publication in this journal is cited, in accordance with accepted academic practice. No use, distribution or reproduction is permitted which does not comply with these terms.
*Correspondence: Lucian Pârvulescu, bHVjaWFuLnBhcnZ1bGVzY3VAZS11dnQucm8=; orcid.org/0000-0002-1528-1429