- 1Laboratório de Etologia, Desenvolvimento e Interação Social (LEDIS), Department of Experimental Psychology, Institute of Psychology, University of São Paulo, São Paulo, Brazil
- 2Núcleo de Etologia e Evolução (NuEVo), Department of Zoology, Institute of Biology, Federal University of Bahia, Salvador, Brazil
- 3Laboratório de Evolução e Biogeografia (LEBI), Department of Zoology, Institute of Biology, Federal University of Bahia, Salvador, Brazil
- 4Department of Zoology, National Institute of Science and Technology in Interdisciplinary and Transdisciplinary Studies in Ecology and Evolution (INCT IN-TREE), Institute of Biology, Federal University of Bahia, Salvador, Brazil
- 5Sylvius Laboratory, Faculty of Science, Institute of Biology Leiden, Leiden University, Leiden, Netherlands
Anthropogenic noise can interfere with animal behavior through masking of acoustic communication. In response to masking, animals may change their acoustic signals as an apparent adjustment strategy, but this may have a drawback on signal quality. Songs and calls may show noise-dependent changes in frequency and duration, which may yield some masking avoidance, but may also constrain other acoustic parameters that might carry information about the sender. In the present study, we investigated whether noise-dependent reduction in frequency bandwidth or song duration restricted syllable diversity or song elaboration in a Neotropical songbird, the bananaquit (Coereba flaveola). We show that bananaquits sing higher frequency songs, of narrower bandwidth, in noisier territories, independent of variation in territory density, without significant variation in song duration. We also show that songs with higher minimum frequencies, narrower bandwidths, and shorter durations have on average a lower number of syllable types and higher syllable rates. This finding is in line with an acoustic restriction and may reflect a functional trade-off between audibility and signal value: higher frequencies may be more audible but less elaborate songs may weaken the message of sender quality. Consequently, noise pollution may not only alter avian communities, but also shape acoustic diversity and processes of sexual selection in urban environments.
Introduction
Anthropogenic noise has increased in natural and human-altered habitats (Mennitt et al., 2015; Buxton et al., 2017), where it might negatively affect vocally communicating animals. The elevated noise levels can cause acoustic interference by masking the functional variation in frequency and amplitude of vocal signals, for example in frogs, birds, and mammals (Wollerman and Wiley, 2002; Lohr et al., 2003; Erbe et al., 2016). Acoustic overlap in time and frequency with anthropogenic noise may decrease the detectability and recognizability of animal vocalizations and thereby disrupt or alter communication (Barber et al., 2010; Parris and McCarthy, 2013; Templeton et al., 2016). The masking problems are typically biased to the lower frequencies of animal signals, because anthropogenic noise is biased to lower frequencies (Halfwerk et al., 2011a; Lazerte et al., 2017). As a consequence, anthropogenic noise may negatively impact vocal function and undermine survival and reproductive success (Halfwerk et al., 2011b; Potvin and MacDougall-Shackleton, 2015; Kleist et al., 2018), for example through reduced foraging efficiency while avoiding predation, and less success in territory defense and mate attraction (Quinn et al., 2006; Halfwerk et al., 2011a; Kleist et al., 2016).
Evidence for a detrimental impact of anthropogenic noise via interference of acoustic communication has been reported in a variety of studies. Ambient noise levels may for example affect courtship: female great tits (Parus major) and female canaries (Serinus canaria) were found to respond less to the low-frequency songs of males in experimentally elevated noisy conditions than in ambient control conditions (Halfwerk et al., 2011a; Huet des Aunay et al., 2014). Ambient noise levels may also undermine communication about predation risk. Savannah sparrows (Passerculus sandwichensis) delay feeding visits to their nestlings when hearing conspecific alarm calls or predators. This behavior is likely reducing predation for both parents and nestlings, but feeding latencies declined under noisy conditions despite the presence of alarm calls or predator songs (Antze and Koper, 2018). A solution to the problems, at least to some extent, would be to adjust acoustic signals in such a way that would reduce masking by anthropogenic noise.
Several types of noise-dependent vocal adjustments could make signals more audible under noisy conditions. For bird songs, the vocal adjustments include modifications of the minimum frequency, changes in duration, amplitude and syllable rate, and altered timing of vocal activity (Brumm, 2004; Potvin and Mulder, 2013; Gil et al., 2014). Specifically, city birds have been reported to sing higher-pitched, longer and more intense vocalizations than birds of the same species from more quiet territories in rural areas (Slabbekoorn and Den Boer-Visser, 2006; Brumm and Zollinger, 2011; Ríos-Chelén et al., 2013). Such song adjustments may decrease the masking effect of the typical low-frequencies of anthropogenic noise and increase song audibility (Brumm and Slabbekoorn, 2005; Pohl et al., 2012). Spectral and temporal adjustments, however, could also lead to vocal restrictions on signal efficiency, as they may prevent the use of specific syllables with potentially high signal value (Halfwerk et al., 2011a, b; Huet des Aunay et al., 2014) or reduce available repertoire size, which may also signal some sender quality (Buchanan and Catchpole, 2000; Kipper et al., 2006).
There is indeed some evidence that spectral and temporal shifts under noisy conditions may impose inherent restrictions on syllable diversity. Montague et al. (2012) found that European robins (Erithacus rubecula) increased the minimum frequency of their songs in response to an elevation in ambient noise levels, which was associated with a synchronous decrease in frequency bandwidth, song duration and syllable length, as well as a decrease in the number of different syllable types. Montague et al. (2012) argued that birds may respond with adjustments in song structure to masking noise, but that acoustic plasticity may be restricted by mechanistic correlations among different song parameters. If such restrictions to acoustic variety affect song function, birds face a trade-off between audibility and signal quality (Slabbekoorn and Ripmeester, 2008; Gross et al., 2010; Slabbekoorn, 2013; Luther et al., 2016), regardless of whether acoustic changes are a direct response to elevated noise levels, or an indirect consequence of a noise-dependent change in another parameter.
Song frequency use or syllable diversity may also be affected by factors other than ambient noise. For some bird species, population density can increase in human-altered habitats due to the increased availability of food resources and decreased presence of predators (Tomiałojć, 1998; Chace and Walsh, 2006; Ciach and Fröhlich, 2017). High territory density may induce competition among males and change their singing behavior (Dabelsteen and Pedersen, 1990; Ripmeester et al., 2010; Narango and Rodewald, 2015). Territory density may therefore be correlated to motivational variation in temporal components of song, such as syllable length, syllable rate, number of syllables and song length (Hamao et al., 2011; Narango and Rodewald, 2015). However, it has also been shown that territorial density can correlate with song frequency use. With higher territory densities, great tits (Parus major) were found to sing with higher minimum frequencies (Hamao et al., 2011), and European blackbirds (Turdus merula) were found to sing with higher peak frequencies (Ripmeester et al., 2010). Therefore, territory density, varying between urban and rural populations, may be an alternative explanation for noise-dependent song variation and thereby a confounding variable that should be taken into account.
The neotropical songbird bananaquit (Coereba flaveola) is an excellent model system to study noise-dependent song variation and to test for potential signaling trade-offs due to inherent acoustic correlations among song parameters. Bananaquits exhibit quite complex vocalizations, including relatively large song repertoires and high singing rates (Wunderle et al., 1992), a variety of high- and low-pitched syllable types, while song diversity and elaboration has been reported to vary among birds from different areas (Hilty and Christie, 2018). Furthermore, they are relatively abundant and have urban and rural distributions where they breed and sing in a variety of microhabitats with variable ambient noise levels. The bananaquit is typically also used to human presence and thereby very suitable to approach for song recordings and analyses of geographic variation and correlation to environmental variables.
In this study, we recorded bananaquit songs in urban territories with variable ambient noise levels to correlate song variation to noise, taking territory density into account. We aimed to answer the following questions: (1) do bananaquit songs have higher minimum and maximum frequencies, do they have narrower frequency bandwidths, and are their songs shorter, in noisier territories? (2) is territory density a confounding variable and also correlated to song frequency use or duration? and (3) does any potential noise-dependent song structure restrict song elaboration, yielding less and lower diversity in syllables? If so, we would provide more insight into how noise pollution may alter conditions for sexual selection and evolutionary change in urban bird species, as well as shape species and song diversity of urban bird communities.
Materials and Methods
Subject Species and Study Area
Bananaquits (Coereba flaveola) are small nectivorous birds with a downward curved bill that occur across the Neotropics, from Southern Mexico to Northern Argentina and the Caribbean islands. They breed in a wide variety of habitats, predominantly at low elevation, including city gardens, urban parks, disturbed areas, and forest borders, in which they can experience a variety of high and low traffic loads (Hilty and Christie, 2018). They are largely monomorphic and appear in the study area with a gray back, black crown and cheek, white eyebrow, light-gray throat, and a bright yellow belly (this plumage varies geographically). Bananaquits are persistent singers and breed throughout the year (Hilty and Christie, 2018). The song is a relatively short series of high-pitched syllables, with more or less repeated sound elements that are often repeated in stereotypic fashion. They can sing during all parts of the day, including the rush hours (personal observation). Their high singing rate comes together with large song repertoires of 120 –340 songs per male, which are mostly produced by the addition and/or deletion of notes at the end of the songs (Wunderle et al., 1992). Although each male is able to produce large amounts of song variation, territory neighbors hardly share similar song types (Wunderle et al., 1992).
We conducted our study in urban parks and built-up areas in the city of Salvador, Bahia, Brazil, between January of 2016 and March of 2018. We visited localities 400 m to 25 km apart (Figure 1). Our sampling covered a range of environmental noise levels from 40 to 73 decibels (dBA). We recorded bananaquits, for example, in the relatively quiet areas of the tropical sand dunes (“restinga”) of “Parque das Dunas,” at the borders of the Atlantic forest fragments of “Jardim Botânico,” “Parque de Pituaçu,” “Vale Encantado,” and “Parque Zoobotânico Getúlio Vargas,” and in urban gardens of “Jardim da Saudade” and the Federal University of Bahia. Noisier bananaquit territories were recorded in busier, urban areas—i.e., main avenues with high concentration of concrete buildings and low quantities of natural trees and gardens—which occur alongside Atlantic forest fragments, such as “Coelba,” at the borders of the sand dunes, and in urban gardens such as at the “Garibaldi,” and “Dique” localities. We consider our sampling design as a contiguous population in urbanized area with high heterogeneity in terms of vegetation, buildings and noise level. All territories, localities and respective ambient noise levels are represented in the map (Figure 1), which was generated using “ggmap” packge in R TM software (Kahle and Wickham, 2013).
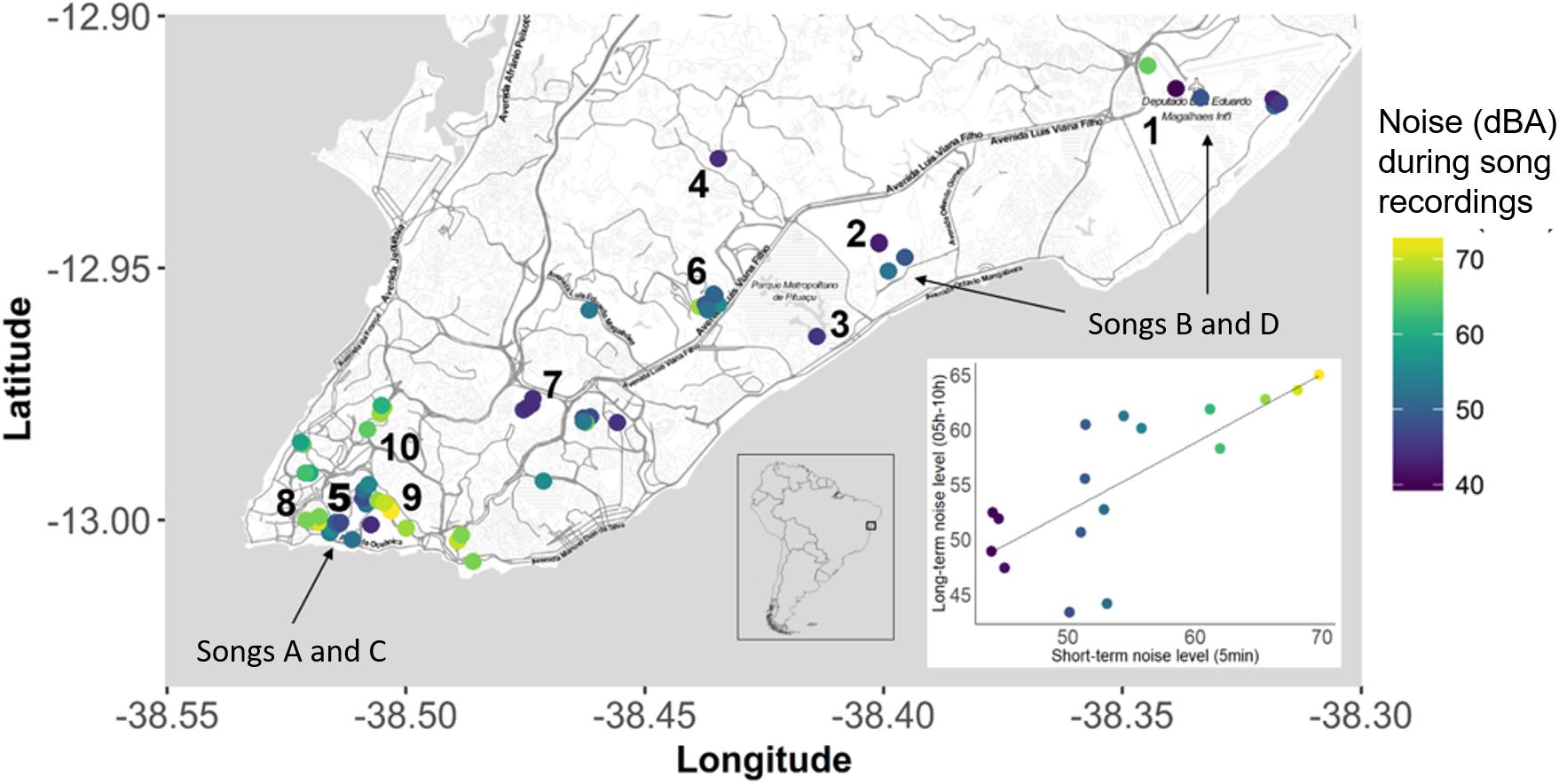
Figure 1. Map representing the distribution of the recorded birds in the city of Salvador, Bahia, Brazil, and the variation of noise levels along sampled territories and in the correlational graph (long-term and short-term noise recordings). The color of the dots represents the intensity of the environmental noise (in decibels, A-curve), measured during the recording of bananaquit songs. In a continuous colored scale, purple dots represent quieter territories and yellow dots the noisier territories. Indicated by numbers are example of quieter and noisier localities and their respective habitat types: Tropical sand dunes of (1) “Parque da Dunas,” Atlantic forest fragments of (2) “Vale Encantado,” (3) “Parque de Pituaçu,” (4) “Jardim Botânico,” (5) “Parque Zoobotânico Getúlio Vargas”, and (6) “Coelba,” Urban Gardens of (7) “Jardim da Saudade,” and (8) Federal University of Bahia; Main avenues in (6) “Coelba,” (9) “Garibaldi,” and (10) “Dique.” Map made with ggmap package (Kahle and Wickham, 2013).
Song Recordings and Noise Measurements
We recorded bananaquit songs with a Sennheiser TM (Wedemark, Germany) shotgun directional microphone (ME67 + K6) connected to a Sony TM (Tokyo, Japan) PCM-D50 digital recorder. Song recordings were taken in WAV format, at a sampling rate of 44.1 kHz. We recorded the birds mostly between 05:00 and 10:00, from a distance of 5–10 m to the focal bird, and each bird was recorded only once. To improve signal-to-noise ratio and the quality of the recordings in noisy locations, we positioned the microphone as close as possible to the bird and as far as possible from the noise source. We positioned the direction of the microphone in parallel to the direction of the noise source as the sides of the microphone are the least sensitive. Additionally, we positioned a barrier, like a car or a wall, between the noise source and the microphone when possible (c.f. Slabbekoorn, 2012).
We measured the ambient noise level in each territory where we were able to record sufficient bananaquit songs. We assessed the sound levels of ambient noise for 5 min, starting at the beginning of song recording, using a sound pressure level meter with data logger Skill-Tec TM (São Paulo, São Paulo, Brazil) SKDEC–02 (A-weighting, slow response, range 30–130 dB, 1s interval). We positioned the equipment upwards, kept vertically on a tripod at 150 cm from the ground. The 5 min sample was integrated into a single, average sound level (dBA) as the ambient noise measure per territory.
The variation in sound levels within territories across the time period of sampling may undermine comparisons across territories (Slabbekoorn and Peet, 2003; Arroyo-Solís et al., 2013). This potential problem was checked with repeated sound level measurements over the morning in a subset of bananaquit territories. We averaged 10–5 min samples per locality recorded between 05:00 and 10:00, sampled at the start of each half hour. These long-term averages were compared to the nearest short-term territory samples by a simple regression, where the long-term averages represented the independent variable and the nearest short-term samples the dependent variable.
Song Processing and Measurements
The song recordings were high-pass filtered between 0.5 and 2.5 kHz using the software Audacity TM v. 2.1.2 (Carnegie Mellon University, Pittsburgh, Pennsylvania, United States). We used spectrogram observation to adjust filter limits for each individual recording, to remove as much ambient noise as possible, without removing any trace of song. The omission of low-frequency background noise renders a distinct presence of the target songs in the amplitude wave, which supported the measurement of song duration on the spectrogram. We generated song spectrograms and waveforms with Raven TM PRO software (Cornell Laboratory of Ornithology, Ithaca, NY, United States) version 1.5. The chosen parameters for the calculation of spectrograms were Hann windows, DFT size 512 samples, and an overlap of 50%.
We measured spectral and temporal variables for each song of a bout of a total of 1 –13 songs per individual. The spectral and temporal song parameters were: minimum, maximum and peak frequency (log10Hz), frequency bandwidth (log10Hz) and song duration (s). We assessed these measures by cursor placement and the automated writing to file procedure of the Raven TM PRO software. There are advantages and disadvantages to the method of cursor placement compared to a fully objective automated measurement technique by amplitude cut-off points relative to the peak amplitude in the song (Verzijden et al., 2010; Zollinger et al., 2012; Ríos-Chelén et al., 2016). The potential issues about effect and artifact size are addressed in the discussion (c.f. Verzijden et al., 2010). We log10 transformed the frequency measurements for each recorded song, before performing any averages (for the graphics) and statistical analyses, as this better reflects pitch perception in birds and should therefore provide a biologically more relevant t-test (Cardoso, 2013). For frequency bandwidth (log10Hz), we first log-transformed maximum and minimum frequency measurements and then computed frequency bandwidth as the difference between the two.
An observer bias is possible to some extent for some of the measurements (e.g., minimum and maximum frequency), as we had clear directional expectations about noise-dependent song variation (c.f. Brumm et al., 2017). However, our recording strategy in the field, to optimize signal-to-noise ratio, reduced direct audibility of current noise level during song measurements. Furthermore, noise level fluctuations in time also reduced the link between noise conditions at specific recording times and average noise levels. Consequently, song analyses were largely blind to variation in the relative noise level among territories, which should prevent problems of observer bias.
We also quantified four measurements of song elaboration, related to the number of repeated song units and the acoustic variety among these units, as done in several studies for different species (Garamszegi and Moller, 2004; Soma and Garamszegi, 2011; Hill et al., 2017). Also the measurements were done for each song of a bout of 1–13 songs per individual. We quantified: (1) number of syllables (sound units per song), (2) number of syllable types (different sound units per song), (3) syllable rate (number of syllables sung per second); and (4) number of phrases. Phrases in bananaquits may concern trills formed by repetitions of the same syllable, fixed combinations of note complexes, or stereotypic sequences of different syllables (Thompson et al., 1994). These measures of song elaboration have been shown to play a role in communication related to sexual and territorial advertisement (Hoi-Leitner et al., 1995; Catchpole and Leisler, 1996; Soma and Garamszegi, 2011; Hill et al., 2017).
Territory Density Assessment
We assessed the number of singing bananaquits within a range of 100 m of a target territory. Within this distance, birds are expected to properly hear each other and face relevant territorial interactions (Ripmeester et al., 2010; Hamao et al., 2011; Narango and Rodewald, 2015). Bananaquits may hear more neighbors than human observers, due to a perceptual focus on conspecific song features and advantageous perch heights. However, it is important to have a measure of territory density that is sampled in a standardized way and which adequately reflects variation among territories in density related competition.
Statistical Analysis
We tested whether spectral and temporal song measurements (minimum, maximum, peak frequency, frequency bandwidth and song duration) were influenced by the noise level measures associated with individual song recordings. We also tested for a confounding impact by social competition by interplaying these spectral, temporal and elaboration song measurements to territory density. Finally, we tested whether the noise-dependent spectral variation in songs found in the first tests affected the song elaboration. We added song duration as a covariate to these analyses, as song duration can affect our measures of song elaboration. We used linear mixed models (LMM) throughout by selecting the “lme4” function (Bates et al., 2015) of R software (R Core Team, 2017). Each model contained one spectral, temporal or elaboration song measurement as the dependent variable, noise or territory density as independent variable, and individual as a random factor. To test the interplay between song spectral measurements and song elaboration, each model contained one elaboration measurement of the song as the dependent variable (syllable types, number of syllables, number of phrases and syllable rate), one spectral characteristic of the songs and song duration as covariate independent variables (minimum, maximum frequency and frequency bandwidth), and individual as a random factor. For ordinal variables as number of syllables, we used a Poisson distribution and for numeric variables as spectral and temporal measurements, we used a Gaussian distribution. Using the same software and “lme4” function, we performed linear mixed models to investigate whether there was an interplay between the spectral variables minimum, maximum frequency and frequency bandwidth, the number of neighbors, song duration, and the song elaboration measurements.
Results
We recorded songs and assessed ambient noise levels in 65 bananaquit territories and measured the territory density of 37 of these in terms of the number of singing male neighbors audible to the human observer. Three out of four of the spectral song measurements were significantly related to the ambient noise level. Song minimum and maximum frequencies were higher in noisier territories, with noise-related differences in minimum frequency being more prominent than differences in maximum frequency (Table 1 and Figures 2, 3). As a consequence, we found that also the frequency bandwidth was significantly related to noise level, with a narrower frequency range in noisier conditions (Table 1). Peak frequency and song duration were not influenced by the noise level (Table 1). The spectral, temporal and elaboration variables that were related to the ambient noise level were not related to territory density (Table 2). The short-term noise measurements, sampled in a 5-min period during song recordings, associated well with the long-term noise assessments, based on ten 5-mi periods spread out over the 5-h period of song recordings between 5.00 and 10.00 h in the morning (r2 = 0.5598, t = 4.367, p = 0.0005, N = 17, Figure 1).
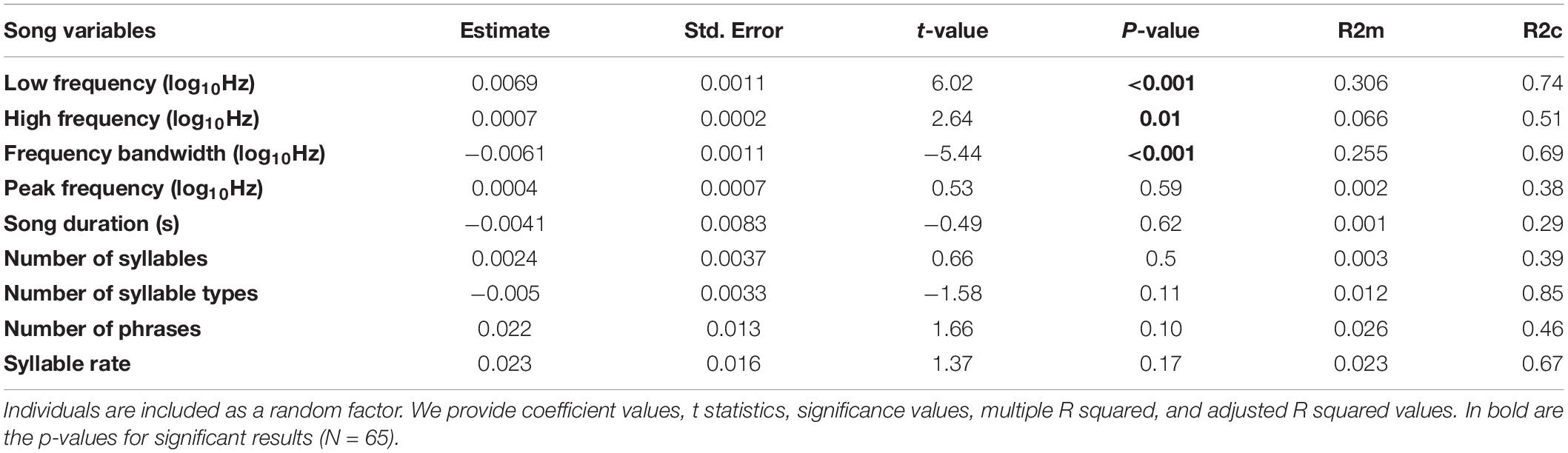
Table 1. Fitted linear mixed models of the spectral or temporal or elaboration song variables on the noise level.
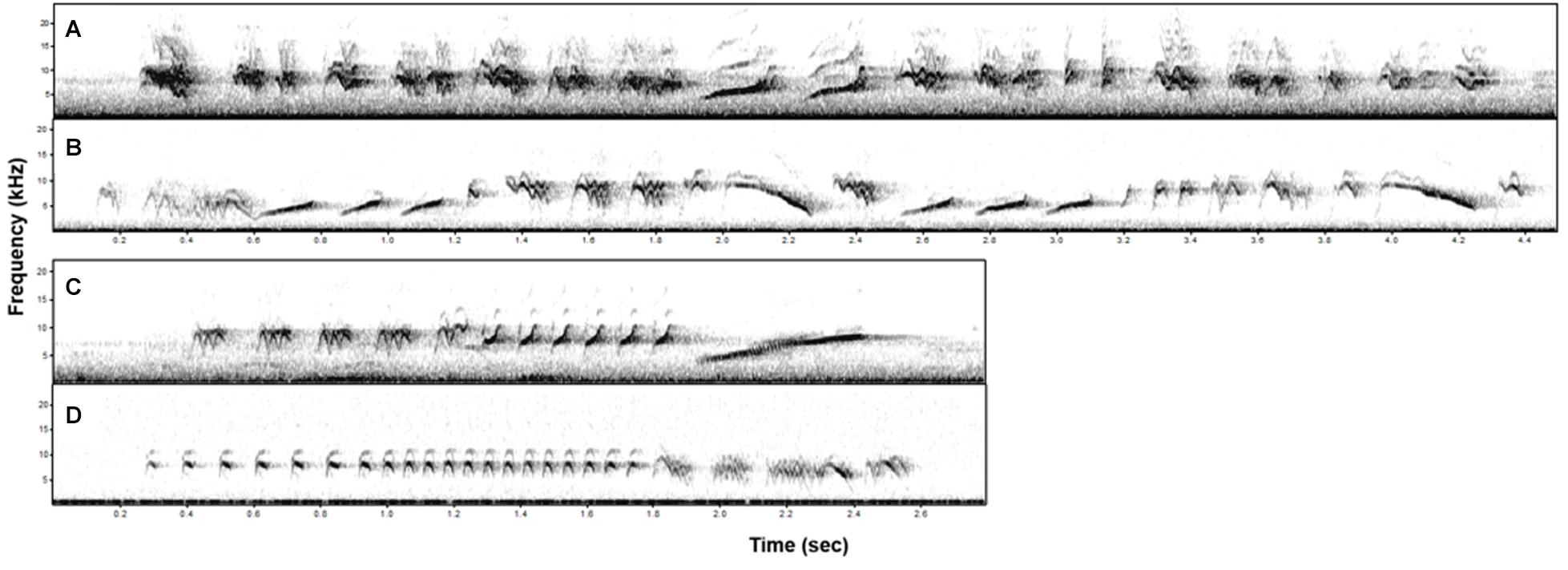
Figure 2. Spectrograms of four bananaquit songs differing in levels of elaboration, i.e., in the number of different syllable types. More (A,B) and less (C,D) elaborate songs occur both in the most geographically distant locations.
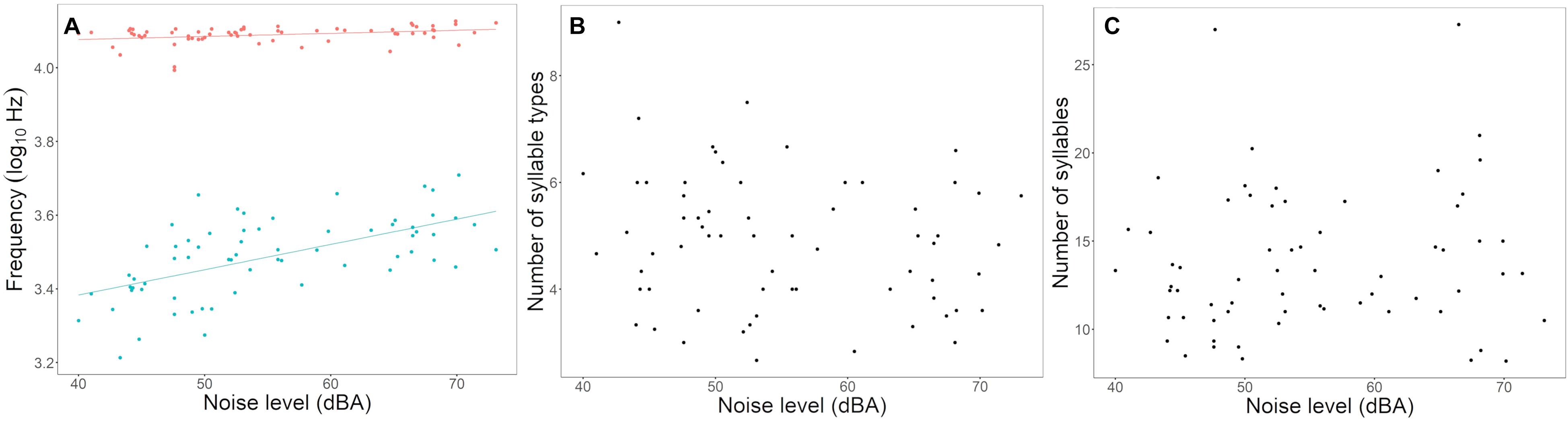
Figure 3. Relationship between noise level and bananaquit song variables. In noisier situations, bananaquits sing higher songs (A), do not change the song syllable length (B), nor the syllable diversity (C). The lines represent the fitted linear model for significant results. In graph A, blue lines and dots represent the minimum frequency and the red line and dots represent the maximum frequency.
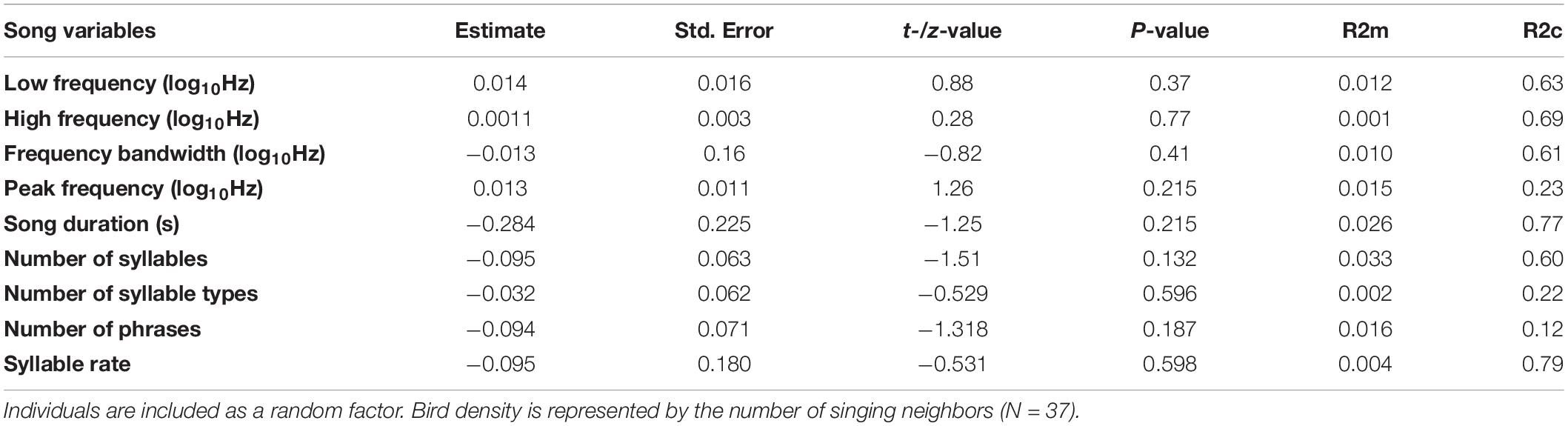
Table 2. Fitted linear mixed models of the spectral or temporal or elaboration song variables on the bird density.
The frequency variation in minimum frequency and frequency bandwidth and also song duration were significantly related to measures of song elaboration (Table 3). The minimum song frequency was negatively correlated to the number of different syllable types, while the frequency bandwidth and song duration were positively related to the number of syllable types. This means that a narrower frequency bandwidth, driven by a higher minimum frequency, together with a shorter duration, lead to less syllable diversity (Table 3 and Figure 4). The minimum and maximum song frequency were positively related, and the frequency bandwidth and song duration negatively related to syllable rate. This means that songs with higher minimum and maximum frequencies had a narrower frequency bandwidth and shorter duration, which yielded higher rates of syllables sang per second (Table 3 and Figure 4). Finally, spectral measurements did not correlate to the number of syllables or phrases. Here, song duration was just related to the number of syllables and phrases. This means that shorter songs naturally had less total number of syllables and phrases.
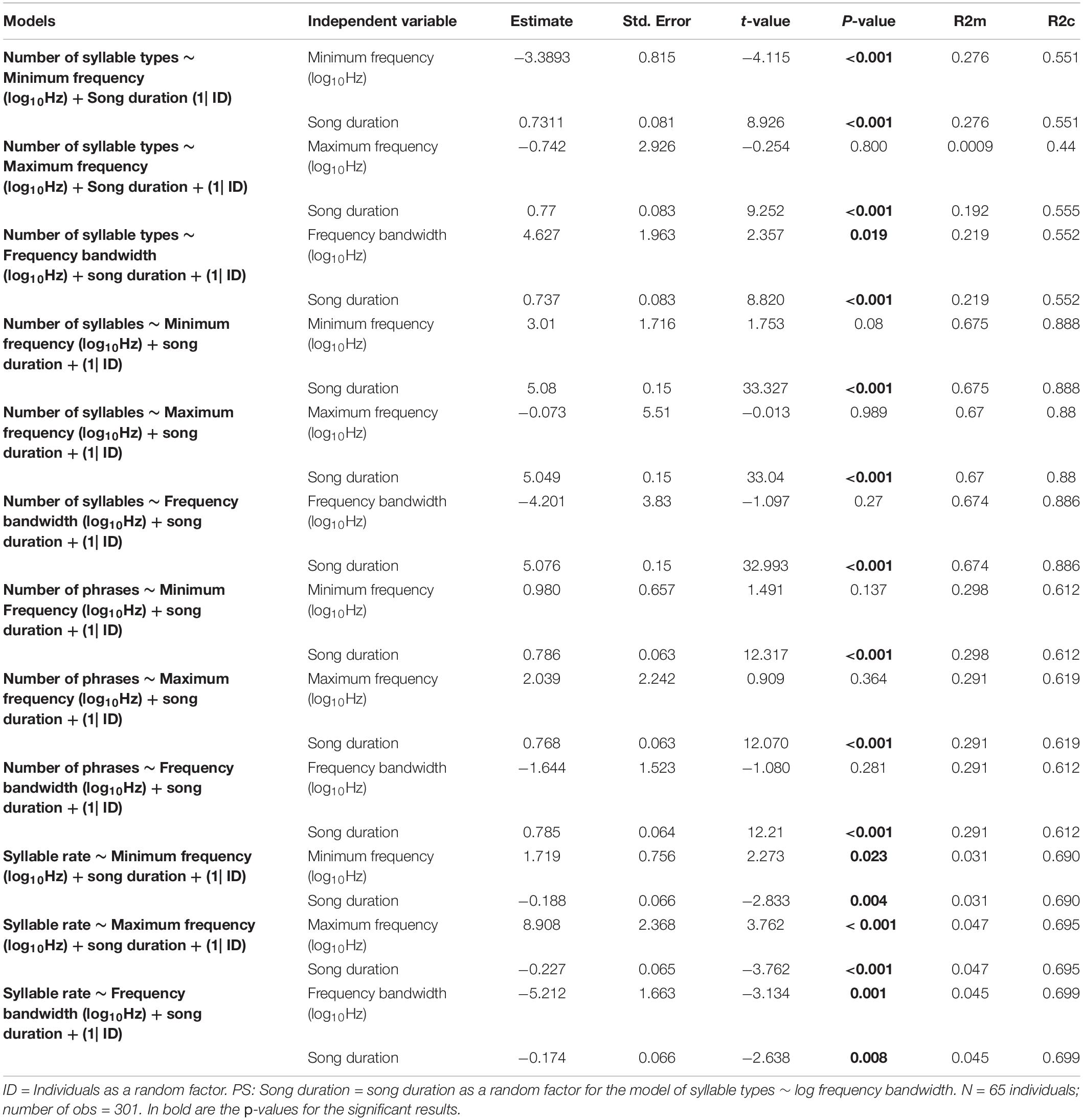
Table 3. Fitted linear mixed models of the elaboration song measurements on the spectral song variables and song duration.
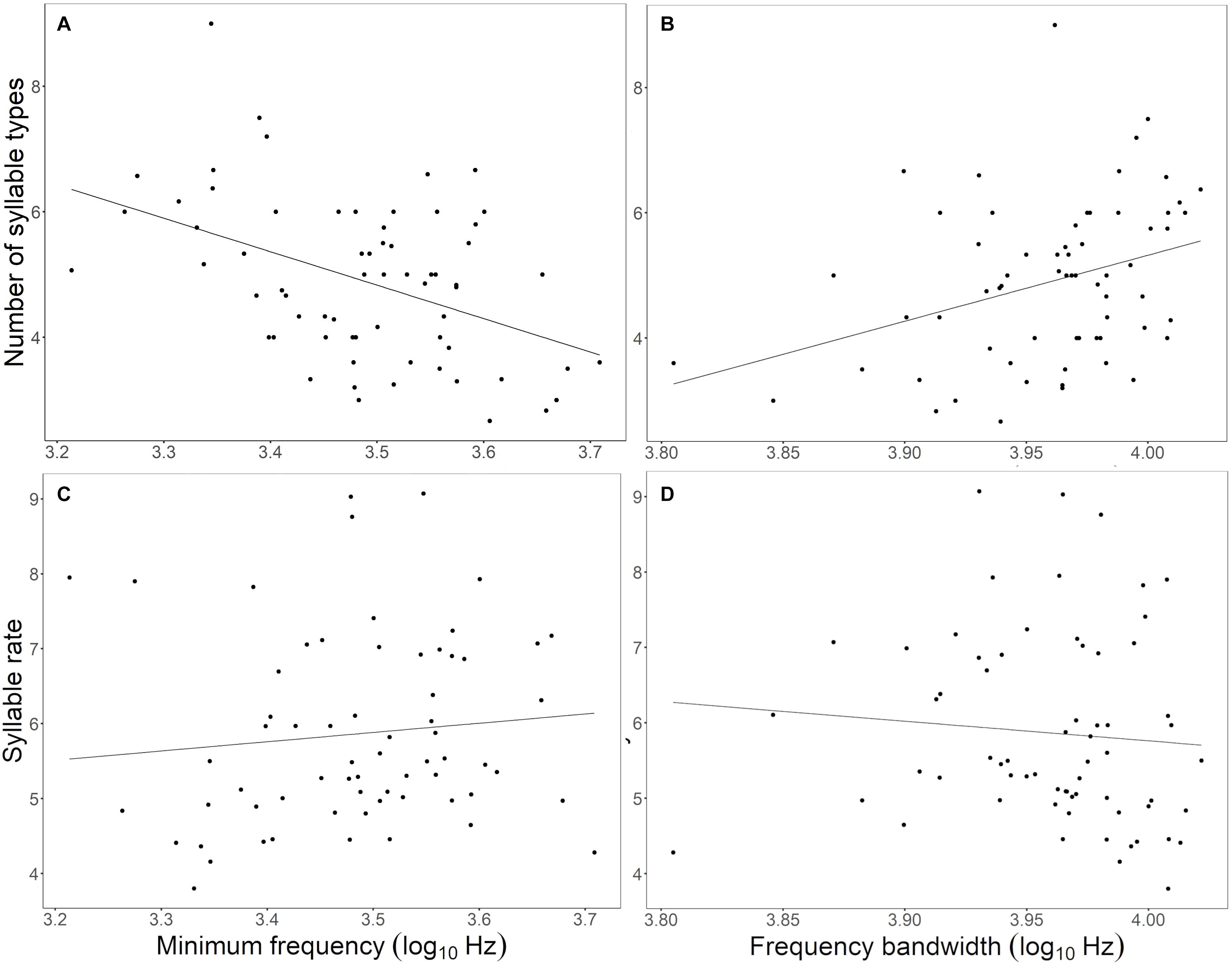
Figure 4. Phenotypic restriction of the song syllable diversity (i.e., number of different syllable types) and syllable rate (i.e., syllables per second) by the increase of the minimum song frequency (A,B) and the decrease of the frequency bandwidth (C,D). The black lines represent the fitted linear model for significant results.
Discussion
We investigated whether bananaquit songs exhibited noise-dependent variation in spectral and temporal parameters and whether higher minimum song frequencies, narrower frequency bandwidths, or shorter songs would restrict song elaboration. Bananaquit songs varied spectrally with noise levels among individuals from different territories, but song duration did not. We found significantly higher minimum and maximum frequencies and significantly narrower frequency bandwidth in noisier territories. The elevated minimum song frequency was more prominent than the noise-dependent maximum frequency, which is in line with a masking avoidance strategy against traffic noise biased to low frequencies. The noise-dependent spectral variation was not affected by territory density, and appears to restrict song elaboration. Despite being not related to the noise levels, song duration was related to frequency bandwidth and song elaboration: higher, narrower, and shorter songs showed less diversity in the number of different syllable types in a song.
Trade-Off Between Audibility and Signal Quality
The noise-dependent changes in frequencies can make bananaquit songs more audible under noisy conditions, but at the same time make them of lower signal value to the singer (Slabbekoorn, 2013; Luther et al., 2016). Bananaquits sang with higher minimum and maximum frequencies, but as the minimum frequency shift was more prominent, songs also became of narrower frequency bandwidth in noisier territories. The different extents of change in minimum and maximum frequencies makes a mechanistic coupling of noise-dependent amplitude and frequency use (Verzijden et al., 2010) less likely as an explanation for the higher sound frequencies in bananaquit songs from noisy territories (Cardoso and Atwell, 2011). Spectral masking avoidance of low-frequency traffic noise (c.f. Slabbekoorn and Peet, 2003) may therefore be a likely explanation, as, for example, great tit females responded more, and male black-capped chickadees (Poecile atricapillus) responded faster, to high than to low frequency songs in noisy situations (Halfwerk et al., 2011a; Lazerte et al., 2017). Furthermore, since bananaquit song repertoires are large and versatile, consisting largely of removals or additions of different syllables to the song (Wunderle et al., 1992), it is possible that individuals avoided the masking effect of noise by removing low-frequency syllables from their songs. However, a higher song frequency may also affect signal value, as in some species low-frequency songs have been shown to signal male size and may play a critical role in mate attraction and competitor deterrence (Brown et al., 1996; Linhart et al., 2012; Luther and Magnotti, 2014).
A decrease in frequency bandwidth may also benefit audibility through improvement of signal-to-noise ratio by spectral concentration of signal energy (c.f. Hanna et al., 2011). At the same time, more narrow bandwidths may limit performance features related to combinations of bandwidth and trill rate (c.f. Podos, 1997) and make a song shorter. Songs with restricted bandwidths have also been shown to elicit lower response levels from territorial rivals and to exhibit lower vocal performance than songs with broader frequency bandwidths (Luther et al., 2016; Davidson et al., 2017). For male bananaquits, narrow song bandwidths with short durations, and possibly lower response levels from territorial neighbors (Winandy et al., 2021), could negatively impact the dynamics of territorial defense, while this species has high levels of intrasexual competition over small territory sizes that are constantly being disputed (Wunderle et al., 1992). These data on the production side of communication indicate that bandwidth reductions may negatively impact signal function and thereby reduce mating opportunities and territory defense efficiency.
On top of the direct consequences of noise-dependent spectral changes, we found a correlated reduction of song elaboration that may also affect signal value. In European robins, a noise-dependent increase in minimum song frequency also correlated to a decrease in the number of different syllable types in a song (Montague et al., 2012). This association of spectral restriction and decline in syllable diversity, now found in multiple species, may be related to an elimination of low-frequency syllables and result from inherent acoustic restrictions due to physical constraints. Alternatively, lower syllable diversity may also emerge through the production of more repetitive trills of the same syllables, as syllable rate increased in songs of narrower frequency bandwidth (as illustrated by the example in Figure 2 and also see Figure 4). The increase in syllable rates could also be explained by the fact that song duration was also related to the song elaboration. Shorter songs may have had higher syllable rates because there was less time for singing the relevant syllables. This could be a counter strategy to cope with noisy conditions in itself (Shannon and Weaver, 1949; Wiley, 1994), as suggested, for noise-dependent repetition rates in chaffinches (Fringilla coelebs) of Europe (Brumm and Slater, 2006) and urban silvereyes (Zosterops lateralis) of Australia (Potvin and Parris, 2012).
The noise-dependent song variation found for the bananaquits can therefore represent a functional trade-off in several ways. The spectral adjustments and increased redundancy may make their songs more audible in the noisy territories. However, the reduced syllable diversity may also affect perceived repertoire size, which may undermine the abilities of singing bananaquits to signal sender quality (Buchanan and Catchpole, 2000; Kipper et al., 2006). Kagawa and Soma (2013) found for example that larger and heavier Java sparrows (Lonchura oryzibora) sang more elaborate songs, i.e., with more note types. Whether a detrimental effect on signal content due to reduced syllable diversity applies to bananaquits remains to be tested and could be done by playback experiments (Winandy et al., 2021).
Methodological Validation
Accurate measurements of noise-dependent song frequency variation may be hindered by the fading song amplitude at spectral extremes and the presence of noise around these same frequencies (Verzijden et al., 2010; Zollinger et al., 2012; Ríos-Chelén et al., 2016). Fully objective automated measurements using amplitude cut-off points, relative to the peak amplitude in the song, avoid this problem but compromise on the assessment of the actual song frequency range. Measurements by cursor placement are the most precise determination of spectral song extremes, but may suffer from observer bias (c.f. Brumm et al., 2017) and may introduce a noise-dependent artifact (Verzijden et al., 2010).
For the current study, we selected spectral measurements by cursor placement and we believe that observer bias is not a problem (see section “Materials and Methods”), as any possible artifact is of a much smaller scale than the effect size in our results. Bananaquit songs show, for example, variation over a range of 3,467 Hz in the minimum frequency between the highest and lowest songs and the noise-dependent shift in this parameter concerns about 1,500 Hz over the sampled range of noise levels, from 40 to 70 dB (A). This is a large effect size compared to other descriptive studies on noise-dependent song variation (Nemeth et al., 2012; Slabbekoorn et al., 2012) and far beyond the artifact size in studies that determined this experimentally for measurements on song playbacks with variable noise levels for chiffchaffs (Phylloscopus collybita): 49 Hz (Verzijden et al., 2010), and red-winged blackbirds (Agelaius phoeniceus): 615 Hz (Ríos-Chelén et al., 2017).
As a final methodological issue, we here briefly address territory density as a potentially confounding variable. Bird densities may increase in cities, for example, by an increase in the availability of nesting sites and food resources (Shochat, 2004). Higher densities may affect urban song variation through altered levels of interaction and agitation that potentially covary with variation in noise levels (Ripmeester et al., 2010; Hamao et al., 2011; Narango and Rodewald, 2015). It is possible that bananaquit densities are higher in more urbanized (and consequently noisier) areas as the species relies on nectar, a very common resource especially in urban parks with a selection of ornamental flowers (Kaluza et al., 2016). However, high densities of bananaquits can indeed be seen in foraging clusters at particular localities, but this seems less so during singing behavior and territorial defense (personal observations). In our study, we also did not find any relationship between our territory density measure and spectral or elaboration features of bananaquit songs. We therefore consider the noise-dependent song variation to be independent of this potentially confounding factor for our species.
Conclusion
Neotropical bananaquits present another case of noise-dependent variation in song frequency use, correlated with a decline in song elaboration. We found that bananaquits sang higher minimum and maximum song frequencies, with most prominent changes in minimum frequencies, and a corresponding narrower frequency range in noisier situations. This noise-dependent song frequency use results in masking avoidance of low-frequency traffic noise at least to some extent. Songs in more noisy territories were also shorter, more repetitive and less diverse in terms of the number of different syllable types, which may reduce signal value for mate attraction and territorial defense. Consequently, these findings are congruent with a functional trade-off in song acoustic structure and suggest that urban birds face a compromise between audibility and signal quality. We believe our findings indicate that anthropogenic noise may not only alter the song features birds sing in urban areas, but that these changes can also affect fundamental processes of sexual selection that may undermine individual fitness and the fate of populations.
Data Availability Statement
The raw data supporting the conclusions of this article will be made available by the authors, without undue reservation.
Ethics Statement
Ethical review and approval was not required for the animal study because this study involved only sound recordings of free ranging animals, in their natural habitats, without any interference of their natural behavior or any kind of manipulation of variables. Therefore, an ethical document was not required in my country.
Author Contributions
GW, RF, RS, and RM collected and analyzed the data. GW constructed the figures and tables. All authors contributed equally to the conception and writing of the manuscript.
Funding
This work was supported by Fundação de Amparo à Pesquisa do Estado da Bahia (grant no RED0045/2014 to HB-F) and Coordenação de Aperfeiçoamento de Pessoal de Nível Superior (CAPES)—Finance Code 001 (grant nos. 1514172, 88881.189177/2018–01 to GW).
Conflict of Interest
The authors declare that the research was conducted in the absence of any commercial or financial relationships that could be construed as a potential conflict of interest.
Acknowledgments
We thank to the organizations which provided funding for this study: Fundação de Amparo à Pesquisa do Estado da Bahia (FAPESB, RED0045/2014) for the overall financial support and Coordenação de Aperfeiçoamento de Pessoal de Nível Superior (CAPES)—Finance Code 001, for the Ph.D. scholarship (n° 1514172) and internship grant (Programa de Doutorado Sanduíche no Exterior/Processo n° 88881.189177/2018–01) given to GW. We also thank to the Post-Graduate Program in Experimental Psychology, University of São Paulo (USP) and Núcleo de Etologia e Evolução (NuEVo), Federal University of Bahia (UFBA), for the structural and educational support. Finally, we thank all colleagues who helped and motivated us during data collection and processing.
References
Antze, B., and Koper, N. (2018). Noisy anthropogenic infrastructure interferes with alarm responses in Savannah sparrows (Passerculus sandwichensis). R. Soc. Open Sci. 5:172168. doi: 10.1098/rsos.172168
Arroyo-Solís, A., Castillo, J. M., Figueroa, E., López−Sánchez, J. L., and Slabbekoorn, H. (2013). Experimental evidence for an impact of anthropogenic noise on dawn chorus timing in urban birds. J. Avian Biol. 44, 288–296. doi: 10.1111/j.1600-048x.2012.05796.x
Barber, J. R., Crooks, K. R., and Fristrup, K. M. (2010). The costs of chronic noise exposure for terrestrial organisms. Trends Ecol. Evol. 25, 180–189. doi: 10.1016/j.tree.2009.08.002
Bates, D., Mächler, M., Bolker, B., and Walker, S. (2015). Fitting linear mixed-effects models using lme4. J. Stat. Softw. 67, 1–48. doi: 10.18637/jss.v067.i01
Brown, W. D., Wideman, J., Andrade, M. C., Mason, A. C., and Gwynne, D. T. (1996). Female choice for an indicator of male size in the song of the black−horned tree cricket, Oecanthus nigricornis (Orthoptera: Gryllidae: Oecanthinae). Evolution 50, 2400–2411. doi: 10.2307/2410708
Brumm, H. (2004). The impact of environmental noise on song amplitude in a territorial bird. J. Anim. Ecol. 73, 434–440. doi: 10.1111/j.0021-8790.2004.00814.x
Brumm, H., and Slabbekoorn, H. (2005). Acoustic communication in noise. Adv. Stud. Behav. 35, 151–209.
Brumm, H., and Slater, P. J. (2006). Ambient noise, motor fatigue, and serial redundancy in chaffinch song. Behav. Ecol. Sociobiol. 60, 475–481. doi: 10.1007/s00265-006-0188-y
Brumm, H., and Zollinger, S. A. (2011). The evolution of the lombard effect: 100 years of psychoacoustic research. Behaviour 148, 1173–1198. doi: 10.1163/000579511x605759
Brumm, H., Zollinger, S. A., Niemelä, P. T., and Sprau, P. (2017). Measurements artefacts lead to false positives in the study of birdsong in noise. Methods Ecol. Evol. 8, 1617–1625. doi: 10.1111/2041-210x.12766
Buchanan, K. L., and Catchpole, C. K. (2000). Song as an indicator of male parental effort in the sedge warbler. Proc. R. Soc. B Bio. Sci. 267, 321–326. doi: 10.1098/rspb.2000.1003
Buxton, R. T., McKenna, M. F., Mennitt, D., Fristrup, K., Crooks, K., Angeloni, L., et al. (2017). Noise pollution is pervasive in U.S. protected areas. Science 356, 531–533. doi: 10.1126/science.aah4783
Cardoso, G. C. (2013). Using frequency ratios to study vocal communication. Anim. Behav. 85, 1529–1532. doi: 10.1016/j.anbehav.2013.03.044
Cardoso, G. C., and Atwell, J. W. (2011). On the relationship between loudness and increased snog frequency of urban birds. Anim. Behav. 82, 831–836. doi: 10.1016/j.anbehav.2011.07.018
Catchpole, C. K., and Leisler, B. (1996). Female aquatic warblers (Acrocephalus paludicola) are attracted by playback of longer and more complicated songs. Behaviour 133, 1153–1164. doi: 10.1163/156853996x00341
Chace, J. F., and Walsh, J. J. (2006). Urban effects on native avifauna: a review. Landsc. Urban Plan. 74, 46–69. doi: 10.1016/j.landurbplan.2004.08.007
Ciach, M., and Fröhlich, A. (2017). Habitat type, food resources, noise and light pollution explain the species composition, abundance and stability of a winter bird assemblage in an urban environment. Urban Ecosyst. 20, 547–559. doi: 10.1007/s11252-016-0613-6
Dabelsteen, T., and Pedersen, S. B. (1990). Song and information about aggressive responses of blackbirds, Turdus merula: evidence from interactive playback experiments with territory owners. Anim. Behav. 40, 1158–1168. doi: 10.1016/s0003-3472(05)80182-4
Davidson, B. M., Antonova, G., Dlott, H., Barber, J. R., and Francis, C. D. (2017). Natural and anthropogenic sounds reduce song performance: insights from two emberizid species. Behav. Ecol. 28, 974–982. doi: 10.1093/beheco/arx036
Erbe, C., Reichmuth, C., Cunningham, K., Lucke, K., and Dooling, R. (2016). Communication masking in marine mammals: a review and research strategy. Mar. Pollut. Bull. 103, 15–38. doi: 10.1016/j.marpolbul.2015.12.007
Garamszegi, L. Z., and Moller, A. P. (2004). Extrapair paternity and the evolution of bird song. Behav. Ecol. 15, 508–519. doi: 10.1093/beheco/arh041
Gil, D., Honarmand, M., Pascual, J., Pérez-Mena, E., and Garcia, C. M. (2014). Birds living near airports advance their dawn chorus and reduce overlap with aircraft noise. Behav. Ecol. 26, 435–443. doi: 10.1093/beheco/aru207
Gross, K., Pasinelli, G., and Kunc, H. P. (2010). Behavioral plasticity allows short-term adjustment to a novel environment. Am. Nat. 176, 456–464. doi: 10.1086/655428
Halfwerk, W., Bot, S., Buikx, J., van der Velde, M., Komdeur, J., Ten Cate, C., et al. (2011a). Low-frequency songs lose their potency in noise urban conditions. Proc. Natl. Acad. Sci. U.S.A. 108, 14549–14554. doi: 10.1073/pnas.1109091108
Halfwerk, W., Holleman, L. J. M., and Slabbekoorn, H. (2011b). Negative impacts of traffic noise on avian reproductive success. J. Appl. Ecol. 48, 210–219. doi: 10.1111/j.1365-2664.2010.01914.x
Hamao, S., Watanabe, M., and Mori, Y. (2011). Urban noise and male density affect songs in the great tit Parus major. Ethol. Ecol. Evol. 23, 111–119. doi: 10.1080/03949370.2011.554881
Hanna, D., Blouin-Demers, G., Wilson, D. R., and Mennill, D. J. (2011). Anthropogenic noise affects song structure in red-winged blackbirds (Agelaius phoeniceus). J. Exp. Biol. 214, 3549–3556. doi: 10.1242/jeb.060194
Hill, S. D., Pawley, M. D., Anderson, M. G., and Ji, W. (2017). Higher song complexity and intruder pressure at dawn in a vocally complex songbird. Emu 118, 147–157. doi: 10.1080/01584197.2017.1380503
Hilty, S., and Christie, D. A. (2018). “Bananaquit (Coereba flaveola),” in Handbook of the Birds of the World Alive, eds J. del Hoyo, A. Elliott, J. Sargatal, D. A. Christie, and E. de Juana (Barcelona: Lynx Edicions).
Hoi-Leitner, M., Nechtelberger, H., and Hoi, H. (1995). Song rate as a signal for nest site quality in blackcaps (Sylvia atricapilla). Behav. Ecol. Sociobiol. 37, 399–405. doi: 10.1007/s002650050207
Huet des Aunay, G., Slabbekoorn, H., Nagle, L., Passas, F., Nicolas, P., and Draganoiu, T. I. (2014). Urban noise undermines female sexual preferences for low-frequency songs in domestic canaries. Anim. Behav. 87, 67–75. doi: 10.1016/j.anbehav.2013.10.010
Kagawa, H., and Soma, M. (2013). Song performance and elaboration as potential indicators of male quality in Java sparrows. Behav. Processes 99, 138–144. doi: 10.1016/j.beproc.2013.07.012
Kahle, D., and Wickham, H. (2013). ggmap: spatial visualization with ggplot2. R J. 5, 144–161. doi: 10.32614/rj-2013-014
Kaluza, B. F., Wallace, H., Heard, T. A., Klein, A. M., and Leonhardt, S. D. (2016). Urban gardens promote bee foraging over natural habitats and plantations. Ecol. Evol. 6, 1304–1316. doi: 10.1002/ece3.1941
Kipper, S., Mundry, R., Sommer, C., Hultsch, H., and Todt, D. (2006). Song repertoire size is correlated with body measures and arrival date in common nightingales, Luscinia megarhynchos. Anim. Behav. 71, 211–217. doi: 10.1016/j.anbehav.2005.04.011
Kleist, N. J., Guralnick, R. P., Cruz, A., and Francis, C. D. (2016). Anthropogenic noise weakens territorial response to intruder’s songs. Ecosphere 7:e01259. doi: 10.1002/ecs2.1259
Kleist, N. J., Guralnick, R. P., Cruz, A., Lowry, C. A., and Francis, C. D. (2018). Chronic anthropogenic noise disrupts glucocorticoid signalling and has multiple effects on fitness in an avian community. Proc. Natl. Acad. Sci. U.S.A. 115, E648–E657. doi: 10.1073/pnas.1709200115
Lazerte, S. E., Slabbekoorn, H., and Otter, K. A. (2017). Territorial black-capped chickadee males respond faster to high-than to low-frequency songs in experimentally elevated noise conditions. PeerJ 5:e3257. doi: 10.7717/peerj.3257
Linhart, P., Slabbekoorn, H., and Fuchs, R. (2012). The communicative significance of song frequency and song length in territorial chiffchaffs. Behav. Ecol. 23, 1338–1347. doi: 10.1093/beheco/ars127
Lohr, B., Wright, T. F., and Dooling, R. J. (2003). Detection and discrimination of natural calls in masking noise by birds: estimating the active space of a signal. Anim. Behav. 65, 763–777. doi: 10.1006/anbe.2003.2093
Luther, D., and Magnotti, J. (2014). Can animals detect differences in vocalizations adjusted for anthropogenic noise? Anim. Behav. 92, 111–116. doi: 10.1016/j.anbehav.2014.03.033
Luther, D. A., Phillips, J., and Derryberry, E. P. (2016). Not so sexy in the city: urban birds adjust songs to noise but compromise vocal performance. Behav. Ecol. 27, 332–340. doi: 10.1093/beheco/arv162
Mennitt, D. J., Fristrup, K. M., and Nelson, L. (2015). A spatially explicit estimate of environmental noise exposure in the contiguous United States. J. Acoust. Soc. Am. 137, 2339–2340. doi: 10.1121/1.4920539
Montague, M. J., Danek-Gontard, M., and Kunc, H. P. (2012). Phenotypic plasticity affects the response of a sexually selected trait to anthropogenic noise. Behav. Ecol. 24, 343–348. doi: 10.1093/beheco/ars169
Narango, D. L., and Rodewald, A. D. (2015). Urban-associated drivers of song variation along a rural-urban gradient. Behav. Ecol. 27, 608–616. doi: 10.1093/beheco/arv197
Nemeth, E., Zollinger, S. A., and Brumm, H. (2012). Effect sizes and the integrative understanding of urban bird song (A reply to Slabbekoorn et al.). Am. Nat. 180, 146–152. doi: 10.1086/665994
Parris, K. M., and McCarthy, M. A. (2013). Predicting the effect of urban noise on the active space of avian vocal signals. Am. Nat. 182, 452–464. doi: 10.1086/671906
Podos, J. (1997). A performance constraint on the evolution of trilled vocalizations in a songbird family (Passeriformes: Emberizidae). Evolution 51, 537–551. doi: 10.1111/j.1558-5646.1997.tb02441.x
Pohl, N. U., Leadbeater, E., Slabbekoorn, H., Klump, G. M., and Langemann, U. (2012). Great tits in urban noise benefit from high frequencies in song detection and discrimination. Anim. Behav. 83, 711–721. doi: 10.1016/j.anbehav.2011.12.019
Potvin, D., and MacDougall-Shackleton, S. A. (2015). Traffic noise affects embryo mortality and nestling growth rates in captive zebra finches. J. Exp. Zool. A Ecol. Genet. Physiol. 323, 722–730. doi: 10.1002/jez.1965
Potvin, D., and Parris, K. M. (2012). Song convergence in multiple urban populations of silvereyes (Zosterops lateralis). Ecol. Evol. 2, 1977–1984. doi: 10.1002/ece3.320
Potvin, D. A., and Mulder, R. A. (2013). Immediate, independent adjustment of call pitch and amplitude in response to varying background noise by silvereyes (Zosterops lateralis). Behav. Ecol. 24, 1363–1368. doi: 10.1093/beheco/art075
Quinn, J. L., Whittingham, M. J., Butler, J. S., and Cresswell, W. (2006). Noise, predation risk compensation and vigilance in the chaffinch Fringilla coelebs. J. Avian Biol. 37, 601–608. doi: 10.1111/j.2006.0908-8857.03781.x
R Core Team (2017). R: A Language and Environment for Statistical Computing. Vienna: R Foundation for Statistical Computing.
Ríos-Chelén, A. A., Lee, G. C., and Patricelli, G. L. (2016). A comparison between two ways to measure minimum frequency and an experimental test of vocal plasticity in red-winged blackbirds in response to noise. Behaviour 153, 1445–1472. doi: 10.1163/1568539x-00003390
Ríos-Chelén, A. A., McDonald, A. N., Berger, A., Perry, A. C., Krakauer, A. H., and Patricelli, G. L. (2017). Do birds vocalize at higher pitch in noise, or is it a matter of measurement? Behav. Ecol. Sociobiol. 71:29. doi: 10.1007/s00265-016-2243-7
Ríos-Chelén, A. A., Quirós-Guerrero, E., Gil, D., and Garcia, C. (2013). Dealing with urban noise: vermilion flycatchers sing longer songs in noisier territories. Behav. Ecol. Sociobiol. 67, 145–152. doi: 10.1007/s00265-012-1434-0
Ripmeester, E. A. P., Mulder, M., and Slabbekoorn, H. (2010). Habitat-dependent acoustic divergence affects playback response in urban and forest population of the European blackbird. Behav. Ecol. 21, 876–883. doi: 10.1093/beheco/arq075
Shannon, C. E., and Weaver, W. (1949). The Mathematical Theory of Communication. Champaign, IL: University of Illinois Press.
Shochat, E. (2004). Credit or debit? Resource input changes population dynamics of city−slicker birds. Oikos 106, 622–626. doi: 10.1111/j.0030-1299.2004.13159.x
Slabbekoorn, H. (2012). “Measuring behavioural changes to assess anthropogenic noise impact on singing birds,” in Proceedings of Measuring Behavior, eds A. J. Spink, F. Grieco, O. E. Krips, L. W. S. Loijens, L. P. J. J. Noldus, and P. H. Zimmerman (Utrecht: Noldus Information Technology), 28–31.
Slabbekoorn, H. (2013). Songs of the city: noise-dependent spectral plasticity in the acoustic phenotype of urban birds. Anim. Behav. 85, 1089–1099. doi: 10.1016/j.anbehav.2013.01.021
Slabbekoorn, H., and Den Boer-Visser, A. (2006). Cities change the songs of birds. Curr. Biol. 16, 2326–2331. doi: 10.1016/j.cub.2006.10.008
Slabbekoorn, H., and Peet, M. (2003). Birds sing at a higher pitch in urban noise. Nature 424, 267–267. doi: 10.1038/424267a
Slabbekoorn, H., and Ripmeester, E. A. (2008). Birdsong and anthropogenic noise: implications and applications for conservation. Mol. Ecol. 17, 72–83. doi: 10.1111/j.1365-294x.2007.03487.x
Slabbekoorn, H., Yang, X. J., and Halfwerk, W. (2012). Birds and anthropogenic noise: singing higher may matter: (A comment on Nemeth and Brumm, “Birds and anthropogenic noise: are urban songs adaptive?”). Am. Nat. 180, 142–145. doi: 10.1086/665991
Soma, M., and Garamszegi, L. Z. (2011). Rethinking birdsong evolution: meta-analysis of the relationship between song complexity and reproductive success. Behav. Ecol. 22, 363–371. doi: 10.1093/beheco/arq219
Templeton, C. N., Zollinger, S. A., and Brumm, H. (2016). Traffic noise drowns out great tit alarm calls. Curr. Biol. 26, R1167–R1176.
Thompson, N. S., Ledoux, K., and Moody, K. (1994). A system for describing bird song units. Bioacoustics 5, 267–279. doi: 10.1080/09524622.1994.9753257
Tomiałojć, L. (1998). Breeding densities in some urban versus non-urban habitats: the Dijon case. Acta Ornithol. 33, 159–171.
Verzijden, M. N., Ripmeester, E. A. P., Ohms, V. R., Snelderwaard, P., and Slabbekoorn, H. (2010). Immediate spectral flexibility in singing chiffchaffs during experimental exposure to highway noise. J. Exp. Biol. 213, 2575–2581. doi: 10.1242/jeb.038299
Wiley, R. H. (1994). “Errors, exaggeration, and deception in animal communication,” in Behavioral Mechanisms in Ecology, ed. L. Real (Chicago, IL: University of Chicago Press), 157–189.
Winandy, G. S. M., Japyassú, H. F., Izar, P., and Slabbekoorn, H. (2021). Noise-related song variation affects communication: bananaquits adjust vocally to playback of elaborate or simple songs. Front. Ecol. Evol. 8:570431. doi: 10.3389/fevo.2020.570431
Wollerman, L., and Wiley, R. H. (2002). Background noise from a natural chorus alters female discrimination of male calls in a neotropical frog. Anim. Behav. 63, 15–22. doi: 10.1006/anbe.2001.1885
Wunderle, J. M. Jr., Cortes, R. A., and Carromero, W. (1992). Song characteristics and variation in a population of bananaquits on Puerto Rico. Condor 94, 680–691. doi: 10.2307/1369252
Keywords: behavioral plasticity, noise-dependent adjustments, song complexity, song quality, song elaboration
Citation: Winandy GSM, Félix RP, Sacramento RA, Mascarenhas R, Batalha-Filho H, Japyassú HF, Izar P and Slabbekoorn H (2021) Urban Noise Restricts Song Frequency Bandwidth and Syllable Diversity in Bananaquits: Increasing Audibility at the Expense of Signal Quality. Front. Ecol. Evol. 9:570420. doi: 10.3389/fevo.2021.570420
Received: 07 June 2020; Accepted: 11 May 2021;
Published: 07 June 2021.
Edited by:
David Andrew Luther, George Mason University, United StatesReviewed by:
Gonçalo C. Cardoso, University of Porto, PortugalChristopher B. Sturdy, University of Alberta, Canada
Copyright © 2021 Winandy, Félix, Sacramento, Mascarenhas, Batalha-Filho, Japyassú, Izar and Slabbekoorn. This is an open-access article distributed under the terms of the Creative Commons Attribution License (CC BY). The use, distribution or reproduction in other forums is permitted, provided the original author(s) and the copyright owner(s) are credited and that the original publication in this journal is cited, in accordance with accepted academic practice. No use, distribution or reproduction is permitted which does not comply with these terms.
*Correspondence: Gabrielle S. M. Winandy, Z2FicmllbGxlLndpbmFuZHlAZ21haWwuY29t