- 1State Key Laboratory of Desert and Oasis Ecology, Xinjiang Institute of Ecology and Geography, Chinese Academy of Sciences, Urumqi, China
- 2National Engineering Technology Research Center for Desert-Oasis Ecological Construction, Xinjiang Institute of Ecology and Geography, Chinese Academy of Sciences, Urumqi, China
- 3University of Chinese Academy of Sciences, Beijing, China
A grazing enclosure (GE) is one of the most effective techniques for restoring degraded rangelands by modifying the composition, abundance, and diversity of species. However, the effect of GEs on different grazing intensities and durations compared to open-grazing (OG) rangelands is not well known. We aimed to assess the effect of GE on the characteristics of plant species. We established five plots in a short-term enclosure, a long-term enclosure, and an OG treatment to examine the effect of GE on vegetation species’ height, abundance, diversity, phenology, biomass, heterogeneity, and the carrying capacity (CC) of rangeland under grazed and enclosed conditions. We found that GE significantly (P < 0.05) increased vegetation height, abundance, biomass, CC, phenological period, and species diversity. However, the increase in height, diversity, and phenological periods were not consistent with enclosure duration, and higher increments were recorded at the short-term enclosure site. The highest Shannon index value recorded at StGE (2.45) was 28.6 and 12.2% greater than the LtGE (1.75) and OG (2.15) sites, respectively. The advanced phenological periods were higher at the StGE site and showed 22.6 and 60.3% higher values than the LtGE and OG sites, respectively. The highest carrying capacities of 23.4 and 114.3% for livestock were observed at the LtGE compared to the StGE and OG grazing sites. In addition, the heterogeneity of the plants’ community was significantly higher in the long-term GE, due to the decrease in species’ diversity. In conclusion, this paper further contributes to the development of the theoretical basis on the effects of GEs and recommends strategies like rotational grazing and reseeding to be used in tandem with a GE for sustainable management of rangelands.
Introduction
Rangelands are major parts of the global ecosystem and occupy more than a quarter of Earth’s land area (Muhammad et al., 2019). The density, richness, and dominance of rangeland species depend on spatial–temporal variation (Bennie et al., 2011). The species’ composition, diversity, biomass production, and CC potential of rangelands are mainly influenced by environmental factors and ecological processes such as human disturbance, interactions between species, and seed dispersal (Shao et al., 2016; Wang et al., 2018). Conserving the diversity of rangeland species is vital to sustaining productivity and minimizing the impact of other ecosystem services (Wu et al., 2014). Grazing, as one of the most important land uses of rangelands, affects the vegetation species’ diversity, biomass, and vegetation structure (Suizi et al., 2019). Grazing animals have a great impact on the formation of vegetation community and cause changes in richness, diversity, productivity, and CC of rangelands (Peper et al., 2011). Some studies indicated that livestock grazing is seen as a promising option for maintaining and promoting grassland biodiversity (Wang et al., 2018). Many other studies have assessed the effects of grazing on forage nutritive value, forage quality, soil physicochemical properties, and biodiversity (Graff et al., 2007; Ma et al., 2019). Under appropriate grazing management, forage value, quality, quantity, and soil properties show an increasing trend or better condition, yet overgrazing has led to a decrease in forage quality, quantity, and overall degradation of rangeland (Maestre and Reynolds, 2006; Chen et al., 2015).
Different grazing species have different effects on grassland vegetation due to their specific nutritional demands, jaw anatomy, and grazing behavior (Suizi et al., 2019). The simplest and most effective method of improving degraded rangeland conditions is to restore natural vegetation by excluding grazers (Al-Rowaily et al., 2015; Zhao et al., 2019; Song et al., 2020). GE is a highly used technique for restoring degraded rangelands in arid and semi-arid rangeland (Hu et al., 2016; Suizi et al., 2019). The productivity of degraded rangeland biomass and species’ cover can be restored through the implementation of GE (Yan and Lu, 2015; Liu et al., 2018; Zhao et al., 2019). Rangeland restoration focuses on grass species’ structure, abundance, diversity, biomass, and CC (Angassa and Oba, 2008, 2010). The Borana rangeland is the largest area of savannah grazing that provides different ecosystem services, such as biodiversity and climate and water conservation (Tadesse et al., 2002; Angassa et al., 2010). It is one of the primary pastoral production areas in east Africa, including Ethiopia, mainly protected by local pastoralists and often used for herding (Abebe et al., 2006; Raiesi and Riahi, 2014). The rangelands are grazed by indigenous herbivores, such as cattle, goats, sheep, camels, mules, donkeys, and horses. These rangelands have traditionally served as the principal pastures for Borana communities and are regarded as one of the major pastoral production bases in Ethiopia (Angassa et al., 2010). Currently, much of the Borana rangelands become severely degraded, due to a combination of overgrazing, encroachment by invasive bush species, and other climatic and anthropogenic factors (Asefa et al., 2003).
Nevertheless, overgrazing, caused by an increase in the human population and domestic livestock in Borana, is widely considered to be the primary cause of rangeland degradation (Abebe et al., 2006). Under conditions of overgrazing by livestock, a significant change was observed in the composition, biomass, CC, phenology, and heterogeneity of vegetation species in rangelands (Raiesi and Riahi, 2014). Currently, to alleviate the problem of rangeland degradation in Borana, the local community and government officials are implementing the GE method to restore degraded rangeland areas by preventing grazing for either long or short periods of time. This management strategy is expected to restore vegetation and enhance rangeland health in overgrazed and degraded rangelands (Zhao et al., 2019; Song et al., 2020). This campaign has been in practice for over 15 years and highlights the question: How does this strategy manage to restore the degraded Borana rangelands and what is its effect? However, research results regarding the effect of GE on rangelands’ plant species were not consistent. Some studies have indicated that GE can have negative effects on plant species’ diversity because of litter accumulation, water change, light competition, and species exclusion (Wu and Thirgood, 2009; Porensky and Young, 2013; Wang et al., 2018), which have occurred in the mechanisms of climatic and biotic interactions. Thus, the GE has significant effects on plant phenological development. Key aspects of plant phenology, such as greening, seeding, and flowering, have profound implications for the restoration of degraded grasslands (Guo et al., 2020). Various studies have indicated that StGE shows a better increase in species’ richness and advances the plant species’ phenology, and the situation may cease as GE duration increases (Komac et al., 2015; Xiong et al., 2016). As a result, an StGE is more highly recommended for restoring degraded rangelands than an LtGE (Dong et al., 2015). During LtGE, internal changes in the enclosure area (grazing disturbance state and colonization process) have a significant role in determining species’ diversity, biomass production, and CC of grasslands in relation to the external influencing factors (Jing et al., 2017; Wang et al., 2018).
Therefore, specific studies are essential for rangelands to be properly managed and for conservation goals to be achieved. In support of these mechanisms, estimation of the general ecological effects of GE on rangeland is important to update knowledge for maintaining sustainable grassland management in the Borana rangeland. In addition, evaluating the effect of GE can be used to understand the variation of plant species’ diversity between the OG and enclosed grassland sites. Nonetheless, many studies conducted in the study area (Tadesse et al., 2002; Asefa et al., 2003; Mengistu et al., 2005; Abebe et al., 2006; Angassa et al., 2010) were limited to estimating the effects of restoration practices using GE on the species’ diversity, biomass productivity, and CC potential of the grazing rangeland. Therefore, rangeland restoration through GE methods requires the use of clear measurable data using comparable spatial methods for all species at the study site. To fill the knowledge gap mentioned above, we posed the following questions: (1) How does GE affect the characteristics and diversity of grass species in the grasslands of Borana? and (2) How does plant phenology respond to GE? In this study, we investigated the impact of GE on abundance, height, diversity, phenology, biomass, and CC potential of plant species in Borana rangeland using both grazed and enclosure (in the short term of 1–3 years, and in the long term of 6–8 years) grazing site. We have implemented the OG and enclosure treatment in the Yabelo rangeland district, as the rangeland site is an area which includes both OG and GE (LtGE and StGE) grazing sites. The objectives of this study were: (1) to assess the effect of GE on the characteristics of plant species; (2) to evaluate the impact of GE on phenological and community heterogeneity of plant species; and (3) to compare the impacts of age of enclosures and other environmental factors on plant characteristics, as well as on the efficiency of the rehabilitation of degraded rangelands. We hypothesized that GE would have a significant effect on diversity of species, evenness, phenology, heterogeneity of plant communities’ biomass production, and CC in sites of the study area.
Materials and Methods
Study Area
The study was conducted in the Yabelo district in the Borana region, Southern Ethiopia, using both OG, StGE, and LtGE sites for two consecutive years (2019 and 2020) (Figure 1 and Supplementary Table S1). It is located between 4°30′55.81″ and 5°24′36.39″ north latitude and between 7°44′14.70″ and 38°36′05.35″ east longitude (Dalle et al., 2015). The area is located 566 km south of Addis Ababa (capital city of Ethiopia), along Addis-Moyale road. The total area of the district is 15,430 km2, of which 68% (10,492 km2) is rangelands. The altitude is about 1000–1500 m, with a maximum altitude of 2000 m. Rainfall is bimodal with the main (73%) rainy season occurring between March and May, while the short (27%) rainy season occurs in September to November (Dalle et al., 2015). The two intervening dry seasons are from June to August and December to February, when forage resources are scarce (Angassa, 2014). The mean annual rainfall recorded is between 450 and 700 mm (Gemedo, 2020), while the mean annual temperature varies from 19 to 24°C with little seasonal variation. The potential evapotranspiration is 700–3000 mm (Billi et al., 2015). The main soil types in the study area include red sandy loam soil, black clay, volcanic light-colored silt clay, and silt, and are mainly used to support the growth of grazing grass species (Coppock, 1994; Gemedo, 2020). Based on the relative coverage, the dominant grass species in the investigated sites include Chrysopogon aucheri, Chloris roxburghiana, Cenchrus ciliaris, Harpachne schimperi, and Cyperus bulbosus (greater than 10% both in dry and wet seasons) (Coppock, 1994; Angassa, 2014). According to the latest census conducted in 2017, there is a reported population of 100,501 for this district, including 56,246 men and 44,255 women; 6289 or 14.2% of its population are urban dwellers. Cattle, goats, sheep, camels, mules, donkeys, and horses are the main species of livestock. Further, according to data reported by the zone livestock office, the estimated total number of herds of each species is 244,134, and the proportion in the herd and densities of each species found in the study district are cattle (104,000 or 42.6%), goats (86,039 or 35.2%), sheep (37,591 or 15.4%), camels (13,305 or 5.4%), horses (138 or 0.06%), mules (159 or 0.07%), and donkeys (2902 or 1.2%) of the total livestock population grazed in the study area. Furthermore, for the OG site, all listed species grazed year-round without rest, as pastoralist migration from one area to another is highly restricted by government policy, which is a major cause of overgrazing and degradation of Borana rangelands.
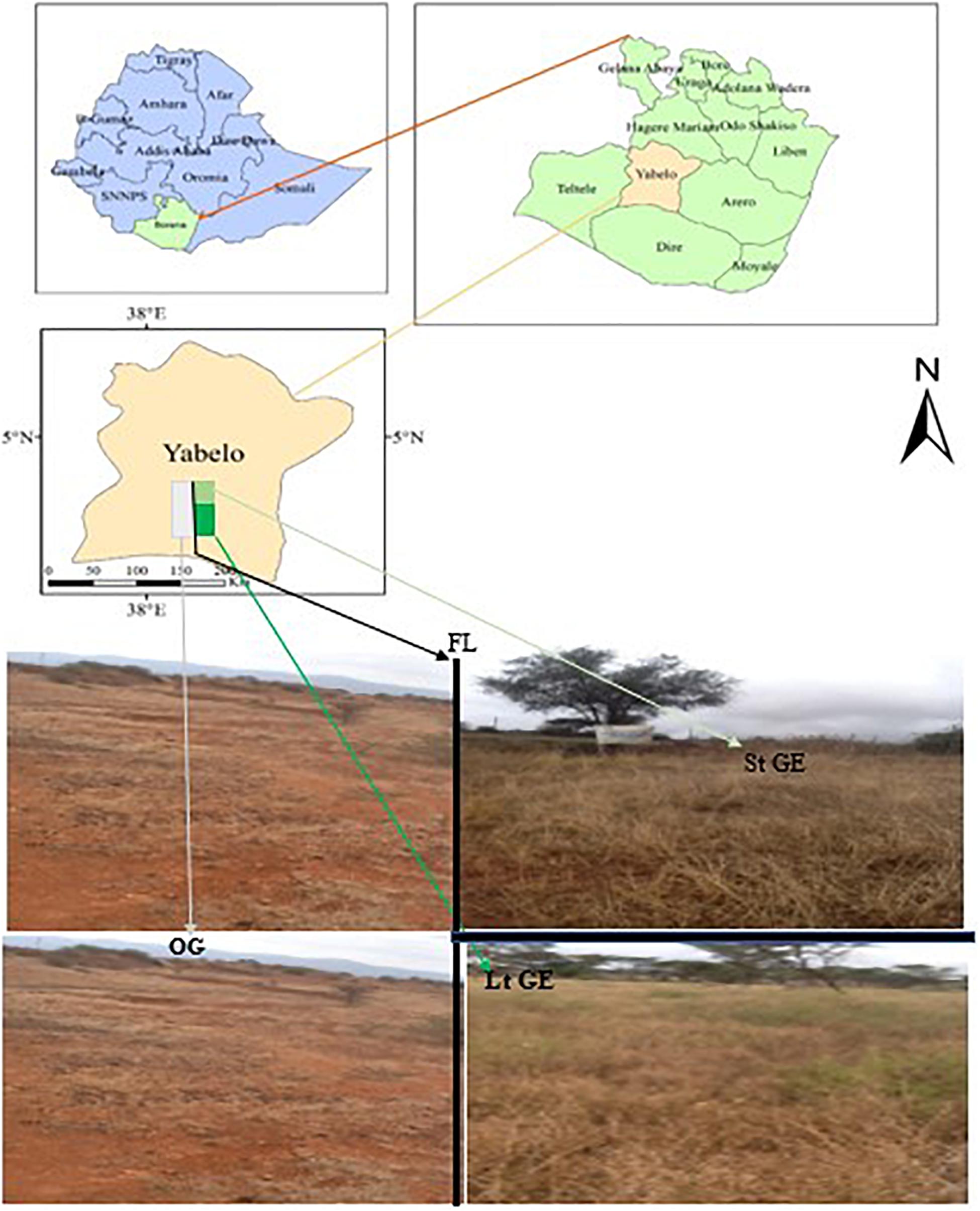
Figure 1. Location of the study area and landscape of study sites. The fence line is clearly visible with a sharp variation of vegetation density and cover inside and outside. Photos by YF. FL, fence line; StGE, short-term grazing enclosure; LtGE, long-term grazing enclosure; OG, open-grazing.
Experimental Design, Field Investigation, and Measurements
We selected a site with two treatments: an enclosed (to evaluate the effect of GE) grazing site and OG sites (as a control). Inside the enclosed grassland, livestock had been abandoned, and to compare the variations in vegetation characteristics and to evaluate the impact of GE duration, GE was divided into StGE (for the last 3 years from 2016 to 2020), locally called Dida Tuyura, and LtGE (for the last 8 years from 2011 to 2020), locally called Danbal Waccu, and was associated with non-fenced year-round OG rangeland used as control treatments, respectively. Two GE treatments with different enclosed durations (3 and 8 years) were selected and investigated within 2 km interval. The selected rangeland sampling areas of these two GE sites and the OG sites were 100 ha for each (in total two GE + two OG sites = 400 ha). The sites were selected from a homogeneous area and had similar geographical conditions like slope, elevation, and soil types. Both in the OG and enclosed rangeland sites, we established five 20 × 20 m plots at 100 m intervals; a total of 20 plots (four treatments with five plots each) were used. Then, in each plot, three 5 × 5 m subplots were randomly assigned as pseudo-replicates out of a total of 60 subplots in grazing and enclosed treatments. Finally, five 1 × 1 m quadrats in each subplot, with a total of 300 quadrats per season, were assigned by randomly casting them back side to minimize any bias resulting from selective placement in each subplot for the collection of samples of plant species over two consecutive years (Supplementary Figure S1). The total sampling of (5 plots × 3 sub-plots × 5 quadrats × 2 seasons × 2 experimental years × 3 replications = 900 for each treatment site) was conducted. Moreover, the sample collection techniques and treatment site are the same during the dry and rainy season. The size of the sampling subplots was chosen due to the vegetation sampling using a random sampling design of 5 m2, with a saturation point of species richness, and the minimum size of sampling unit was 1 m2 (Luzuriaga et al., 2012; Klink et al., 2016).
In each sampling unit, we recorded the number of species, height, and abundance for each grass vegetation species. All the above ground grass samples were harvested by using a cutter and collected in a paper bag. The fresh weight of the collected grass samples was measured in the field with a scale. Then the samples were oven-dried for 24 h at 105°C to determine the biomass. Then the dry matter (DM) measured after 24 h was converted to kilograms per hectare (kg ha–1), and the proper use factor (PUF) was taken as 30% to calculate available forage (Sintayehu, 2006; Meshesha et al., 2019). Thereafter, the DM biomass and livestock CC were determined using procedures described by Niguse (2008). Identification of the grass species was done in the field using identification keys, plates, Flora of Ethiopia books, and the Addis Ababa University national herbarium (Elmore et al., 2000; Dalle et al., 2006). The specific assessment for a detailed palatability value of grass species and soil physicochemical properties was given by a study carried out on the same site and by the same author (Supplementary Table S1) (Yeneayehu et al., 2020). Data collection on grass species sampling was performed twice per year, for two consecutive study periods of the dry season (January to February) and the rainy season (March to May), at the time where grass species were identified easily and peak biomass was recorded. In addition, the field data were collected with three replications for each season.
Data Analysis
A paired difference t-test was conducted to test the differences between the examined parameters (vegetation characteristics, biomass, species diversity, evenness, CC, and change in biomass) between enclosed and grazed plots within each treatment. Grass species diversity was assessed using the Shannon species diversity index (Shannon, 1948). H = −[Σ (pilnpi)], and Pielou evenness index (E) = H/InS was determined by calculating the frequency of each plant species (pi = proportion of individual species in each plot at which species i was recorded; S in is the total number of species in a quadrat) (Suizi et al., 2019). Species frequency, abundance, and dominance were quantified in order to evaluate the grass species’ community and abundance dynamics caused by GE (Wang et al., 2018). The RF, calculated as the frequency of individual grass species divided by the frequency of the total grass species in the sampling plot, RD, calculated as the dominance of individual grass species divided by the dominance of all grass species collected within the sampling plot, and RA, calculated as the total number of individual grass species divided by the total number of all grass species collected within the sampling plot, are described as follows (Eqs 1–3, respectively) (Van wilgen and Richardson, 2014).
CC dynamics of grazing site was calculated using Eq. 4.
where CC = carrying capacity in animal units per hectare (AUha–1), D = number of days in the year (or period to be grazed), DM = dry matter (biomass) in kgha–1, Uf = utilization factor (0.3), and r = daily DM required by one grazing animal (2.5% of bodyweight), which is 11.25 kg for an AU [450 kg grazing animal (cattle)]. To assess the effects of GE on grass species’ phenology, we established an evaluation index of the relationship change of phenological stage (EIRCP) to describe phenological differences between GE and OG sites. The index was calculated according to (Eqs 5 and 6) (Guo et al., 2020).
Psi = the phenological period of species i; APS = the average phenological period of all species inside (GE) or outside (OG) sites; and the number 4 = the maximum phenological differences between inside and outside the enclosure. If the EIRCP value is greater than 0, this tells us the grass phenology improved; if EIRCP value is less than 0, it indicates the grass phenology declined or delayed, and its value ranges between −1 and 1 (Yao et al., 2019; Guo et al., 2020). All statistical analyses were performed using the mean of different variables for the three subplots in both OG and enclosed rangeland. The impacts of GE on grass vegetation characteristics, height, biomass, diversity index, evenness, dominance index, and change of CC in the different rangeland types were tested using two-way analysis of variance (ANOVA) by the general linear model (GLM). This was employed to evaluate the effects of GE on the vegetation community, diversity, and spatial heterogeneity, i.e., to analyze the relationship between species diversity, dominance index, and dominant species heterogeneity. In the ANOVA analysis, fixed factor was Borana rangeland grazing treatments (GE and OG), while the covariates were Rs and Ds. The two covariates, Rs and Ds, were used to fit the ANOVA linear models and were not highly interacted with the fixed factor (P > 0.05). Correlation analysis was used to test the relationships between dominance index, species diversity, and dominant species heterogeneity with vegetation grass community heterogeneity. The least significant difference test was used to compare the means at P < 0.05. All statistical analyses were performed using SPSS 17.0 (SPSS Inc., Chicago, IL, United States), and all significant differences were taken at P < 0.05. The spatial heterogeneity of the grass species was calculated as the standard deviation of the total coverage of four cells (s) divided by the mean value (m) (Weigelt et al., 2008). The spatial heterogeneity of the vegetation community (and of differently dominant species) was calculated as the standard deviation of the total (and differently dominant species) coverage divided by the mean value (m) [coefficient of variation (CV)] (Weigelt et al., 2008).
Results
Effects of Grazing Enclosure on Grass Species Characteristics
Grazing enclosure had a significant effect (P < 0.05) on biomass, height, abundance, phenology, CC, and diversity of vegetation species. Comparing the GE site to the OG sites, the height and abundance of grass species increased in the GE (both at StGE and LtGE) sites (Figure 2). The average height of recorded grass species was found to be significantly higher in GE sites than OG sites, especially in the StGE site. GE generally facilitated the increment in grass species’ abundance. The response of grass species’ abundance to GE also varied with enclosure durations. GE significantly increased the abundance of LtGE compared to StGE. In addition, the effect was significant with seasonal variations. This indicates that the effect of GE showed a significant interaction with climate change factors across all grazing sites (Figure 2). In general, there was a clear trend for the differences in height and cover of plant species induced by GE. No significant (P > 0.05) differences were observed in the year-round dominant grass species index both in the GE and OG sites; however, variation was observed on the Simpson’s dominance index when the GE duration interacted with seasonal variation and during the Rs; the lowest dominance index (F = 22.5, P < 0.05) was observed at StGE. This is because of the increment of species’ diversity at StGE (Figure 3A). LtGE significantly (P < 0.05) reduced species’ diversity and increased Simpson’s dominance index. The StGE sites exhibited greater species’ diversity (21 species from total sampling quadrats or 4.2 species per plots) compared to both the LtGE (17 species from total sampling quadrants or 3.4 species per plots) and the OG (19 species from the total sampling quadrats or 3.8 species per plots) sites (Supplementary Table S2). Among the grass species, C. roxburghiana, C. aucheri, C. ciliaris, C. bulbosus, and H. schimperi had higher frequencies in the GE and OG rangelands (Supplementary Table S2) and showed decreasing patterns as the duration of the enclosures decreased and grazing time increased. The Shannon index increased significantly from the beginning to a few years of enclosure, but then declined when the enclosure duration increased, and the highest value recorded at StGE (2.45) was 28.6% and 12.2% greater than the LtGE (1.75) and OG (2.15) sites, respectively (Figure 3B). For the variation in dominance, the LtGE had no significant effect on the index of the dominant grass species but significantly reduced the diversity of the common grass species (from 16 species to 12 species) (Supplementary Table S2). The effect of GE on species’ evenness index was not significant (P > 0.05), but decreased slightly in the LtGE (Figure 3C). The highest biomass, diversity, abundance, height, and CC capacity were recorded during the rainy season at GE and OG sites, but species’ evenness and dominance in enclosures compared to areas grazed were not significant.
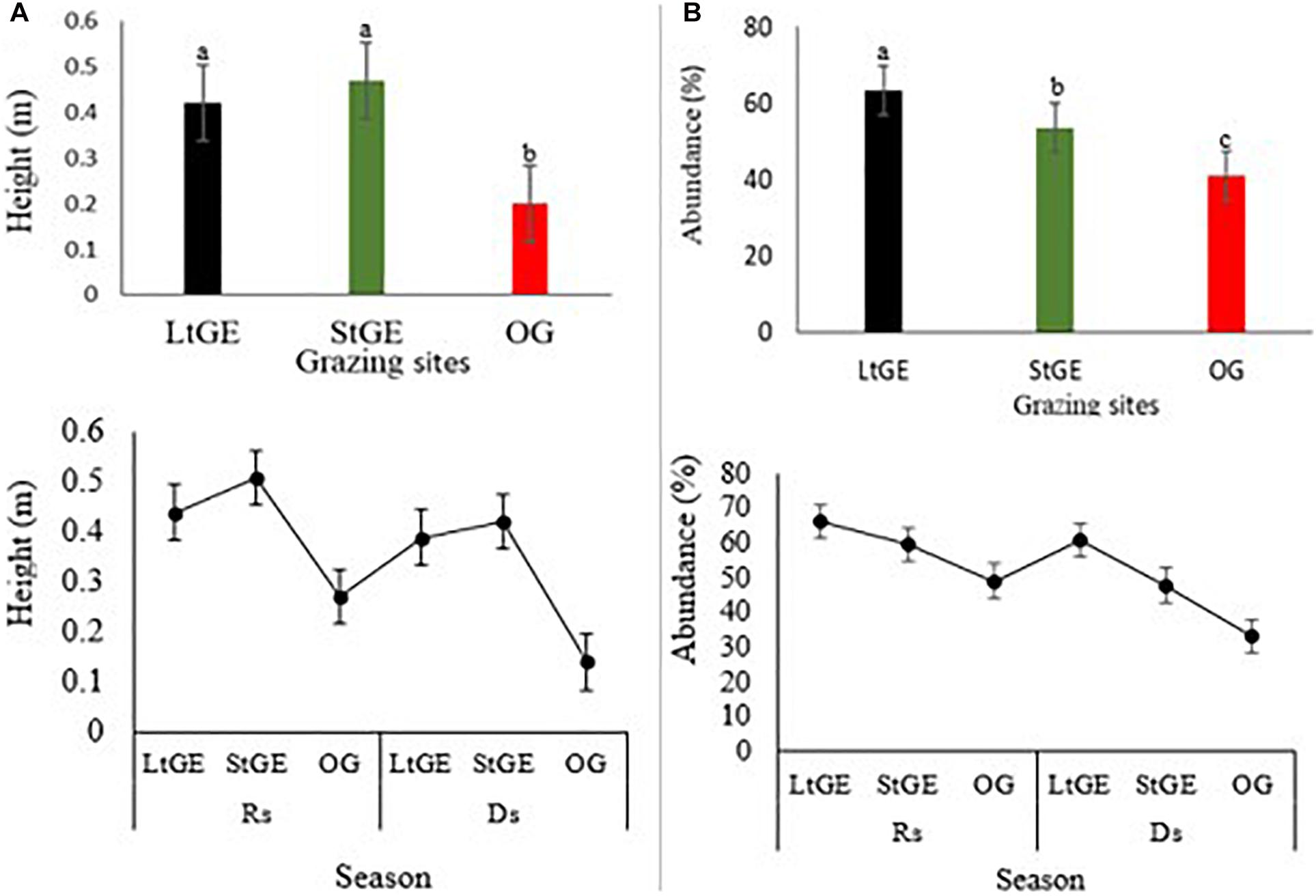
Figure 2. Response of height (A) and abundance (B) of grass species under two treatments: grazing enclosure (short and long) and open-grazing of grassland types in relation with seasonal variation. Note: LtGE, long-term grazing enclosure; StGE, short-term grazing enclosure; OG, open-grazing; Rs, rainy season; Ds, dry season. Scatter plots show seasonal variations and bar plots attached show the yearly average value of grass characteristics. Error bars are shown by standard error. Different lowercase letters within bar plots indicate differences with a significant level (P < 0.05) between the treatments.
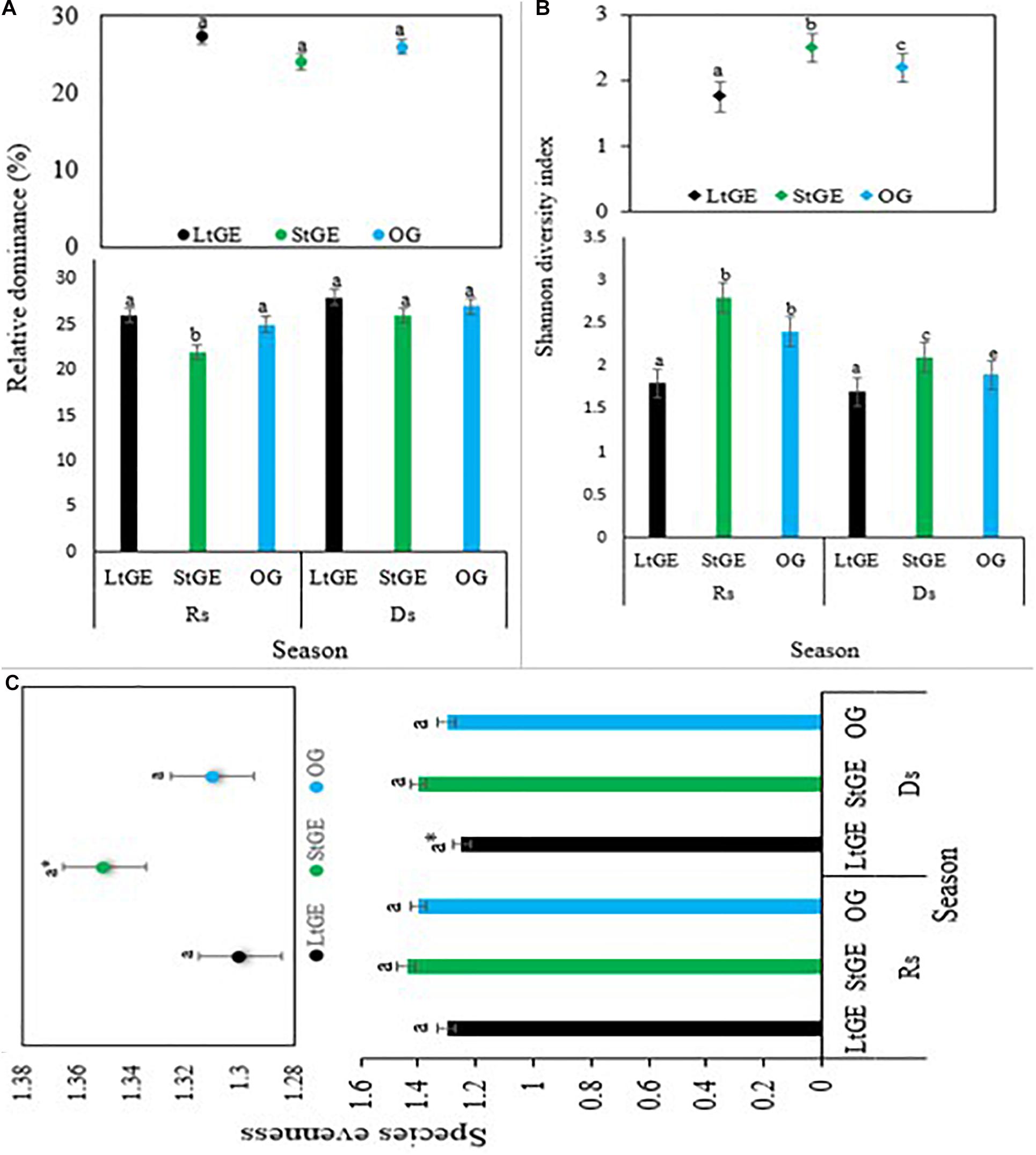
Figure 3. Species relative dominance (A), Shannon diversity (B), and species evenness (C) under treatments of grazing enclosure and open-grazing in relation with seasonal variation. Note. LtGE, long-term grazing enclosure; StGE, short-term grazing enclosure; OG, open-grazing; Rs, rainy season; Ds, dry season. a* indicates differences but not significant. Bar plots show seasonal variations and scatter plots attached show the yearly average value. Error bars are shown by standard error. Different lowercase letters within bar plots indicate differences with a significant level (P < 0.05) between the treatments.
Effects of Grazing Enclosure on Phenological Characteristics of Grass Species
Besides the height, abundance, diversity, and evenness of vegetation species, GE also had significant (P < 0.05) effects on the phenological (green up, flowering, and seeding) periods of grass species in the study sites. In general, GE advanced the phenological period of grass species, which was also observed in the Borana rangeland (Figure 4), and a better phenological period was recorded during the rainy season across all treatment sites. However, the advancement of the phenological stage of the grass showed a characteristic of decline with increasing duration of GE. An evaluation index of relationship change of phenological stages (EIRCP) describing the phenological differences between treatments recorded average values of 0.41, 0.53, and 0.21 at LtGE, StGE, and OG sites, respectively. From this point on, the advanced phenological periods were higher at the StGE site and showed 22.6 and 60.3% higher values than the LtGE and OG sites, respectively.
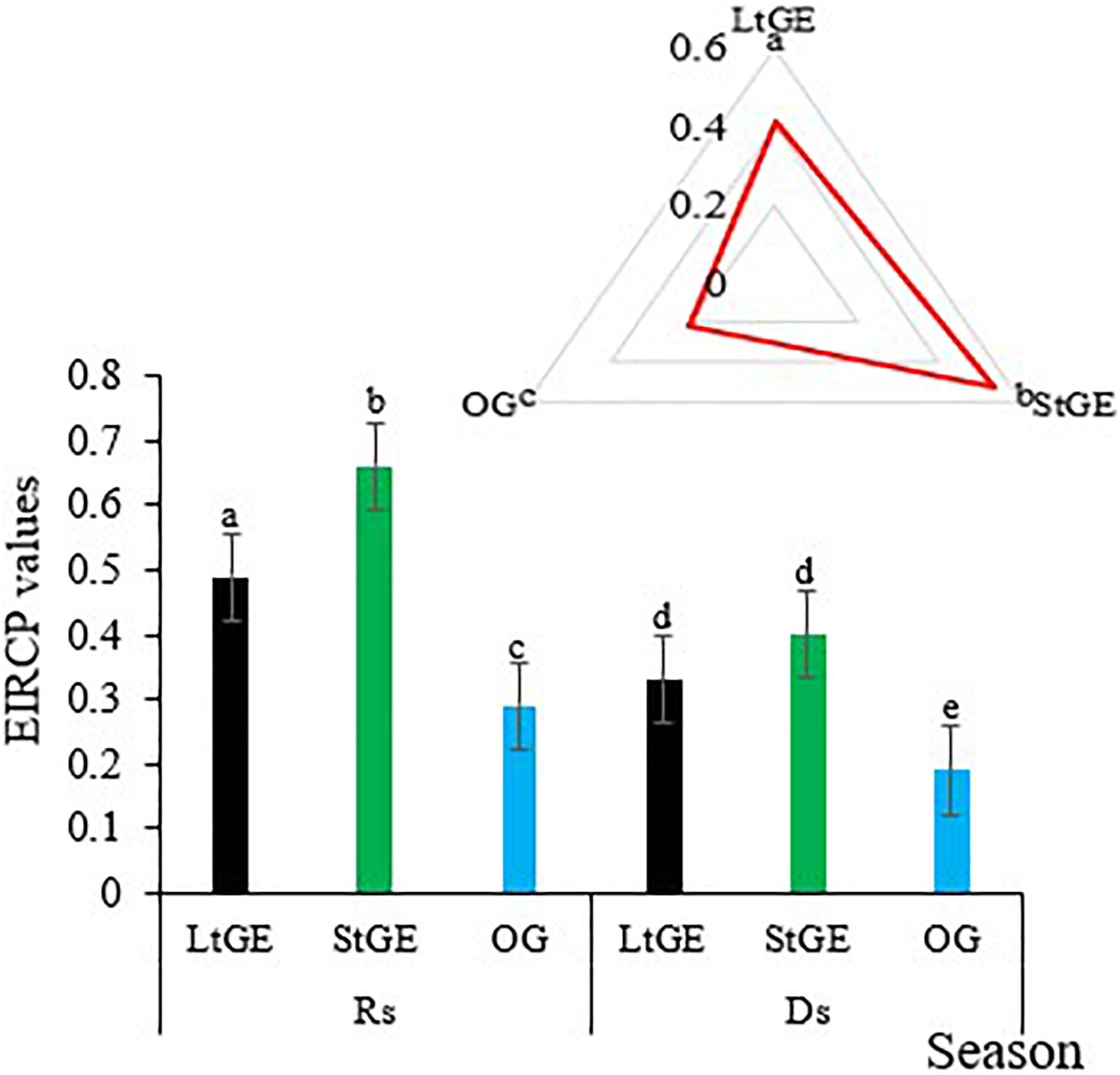
Figure 4. Grass species’ phenological difference at grazing enclosure vs. open-grazing sites. Note. LtGE, long-term grazing enclosure; StGE, short-term grazing enclosure; OG, open-grazing; Rs, rainy season; Ds, dry season. Bar plots show seasonal variations and radar plots attached show the yearly average value. Error bars are shown by standard error. Different lowercase letters within bar and radar plots indicate differences with a significant level (P < 0.05) between the treatments.
Effects of Grazing Enclosure on Grass Biomass and Carrying Capacity
The results of our findings showed significant (P < 0.05) variations in terms of grass biomass production between the GE and OG sites. The variations may be due to a decrease in grazing intensity and protection from grazing during the growth season on the GE sites. This resulted in the recovery of grass species, but in the OG site year-round grazing caused their reduction. The highest biomass was recorded in the LtGE (1100 kg ha–1), 19.5 and 53.2% higher than the StGE and the OG sites, respectively (Figure 5). Furthermore, a high accumulated biomass in the GE results in a high CC of the grazing site as compared with the OG site. Therefore, a significant difference of CC was observed between the treatment sites, and a better livestock CC status of rangeland was found at the LtGE (3.85 ha AU–1year–1 or 0.26 Au ha–1 year–1) followed by StGE (4.75 ha AU–1 year–1 or 0.21 Au ha–1 year–1) (the higher CC value indicated that the rangeland CC was low or degraded) (Figure 5). Thus, rangeland management using GE shows a better forage production and can support more livestock in a small grazing site and also reduces overgrazing and degradation of the grazing site. From this result, a high CC of 23.4 and 114.3% for livestock was observed at LtGE compared to the StGE and OG grazing sites. Regarding grass species, the dominant species (C. roxburghiana, C. aucheri, C. ciliaris, C. bulbosus, and H. schimperi) represent more than 80% of biomass production across all treatment sites.
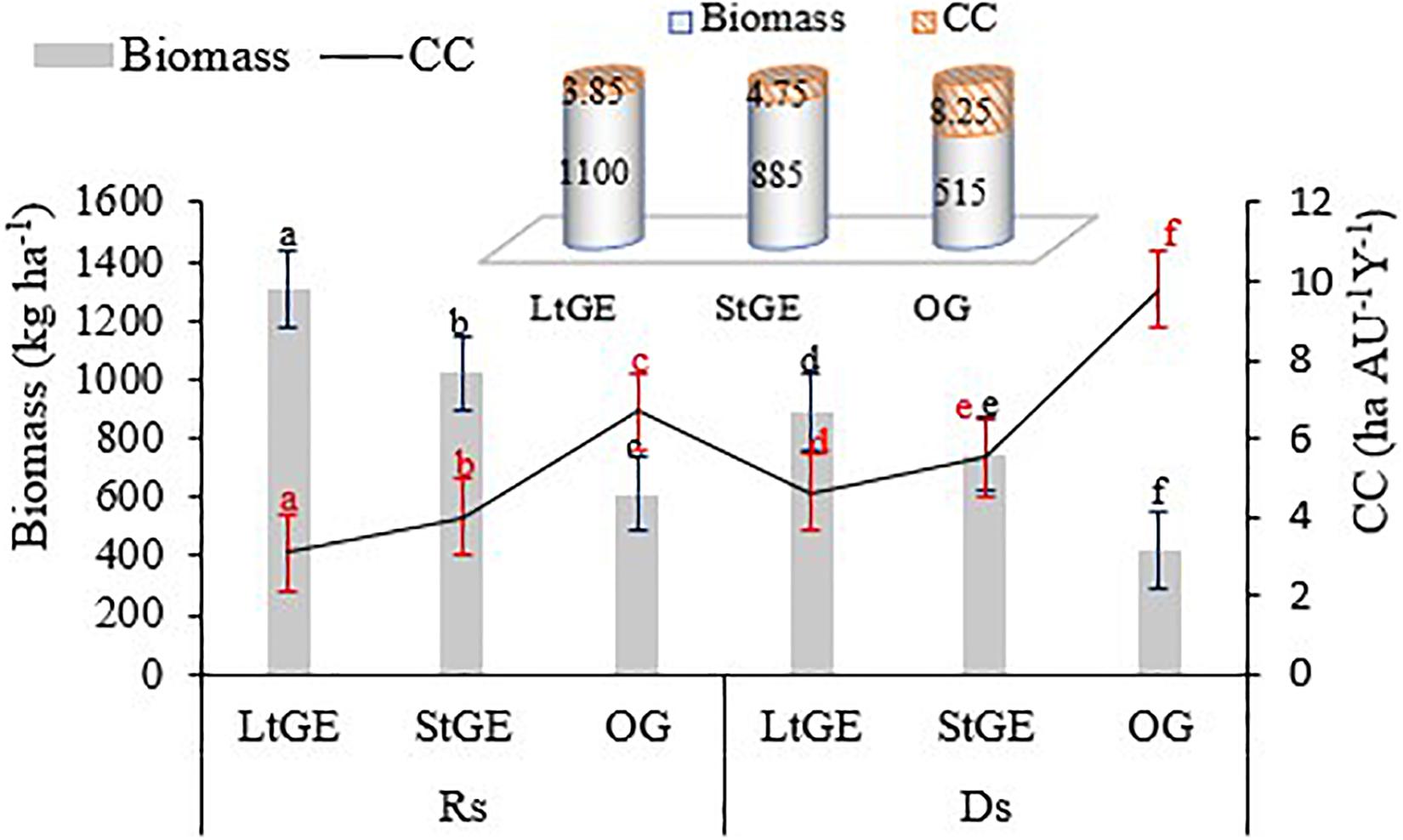
Figure 5. The grass biomass and carrying capacity potential under grazing enclosure and open-grazing conditions. Note. LtGE, long-term grazing enclosure; StGE, short-term grazing enclosure; OG, open-grazing; Rs, rainy season; Ds, dry season; CC, carrying capacity. Bar and line plots show seasonal variations and cylinder plots attached show the yearly average value. Error bars are shown by standard error. Different lowercase letters within bar and line plots indicate differences with a significant level (P < 0.05) between the treatments.
Effects of Grazing Enclosure on Grass Community Spatial Heterogeneity
The effects of GE exerted an influence on the spatial heterogeneity of grass community across the treatment sites. In general, LtGE showed an increasing and StGE a decreasing pattern of the total and dominant grass community heterogeneity compared with the OG site, but neither was significant. The simple linear regression model showed that grass community heterogeneity was negatively related with species diversity (Figure 6B), but positively related with the dominance index (Figure 6A) and dominant species spatial heterogeneity (Figure 6C). Heterogeneity of dominant grass species was a significantly negatively related with species diversity. This resulted from variations of the GE period, and livestock grazing intensity and duration became a universal driver or cause for dynamics of species diversity, richness, and dominance across the sampling site, and variation of spatial heterogeneity of species significantly depends on the dynamics of the vegetation community.
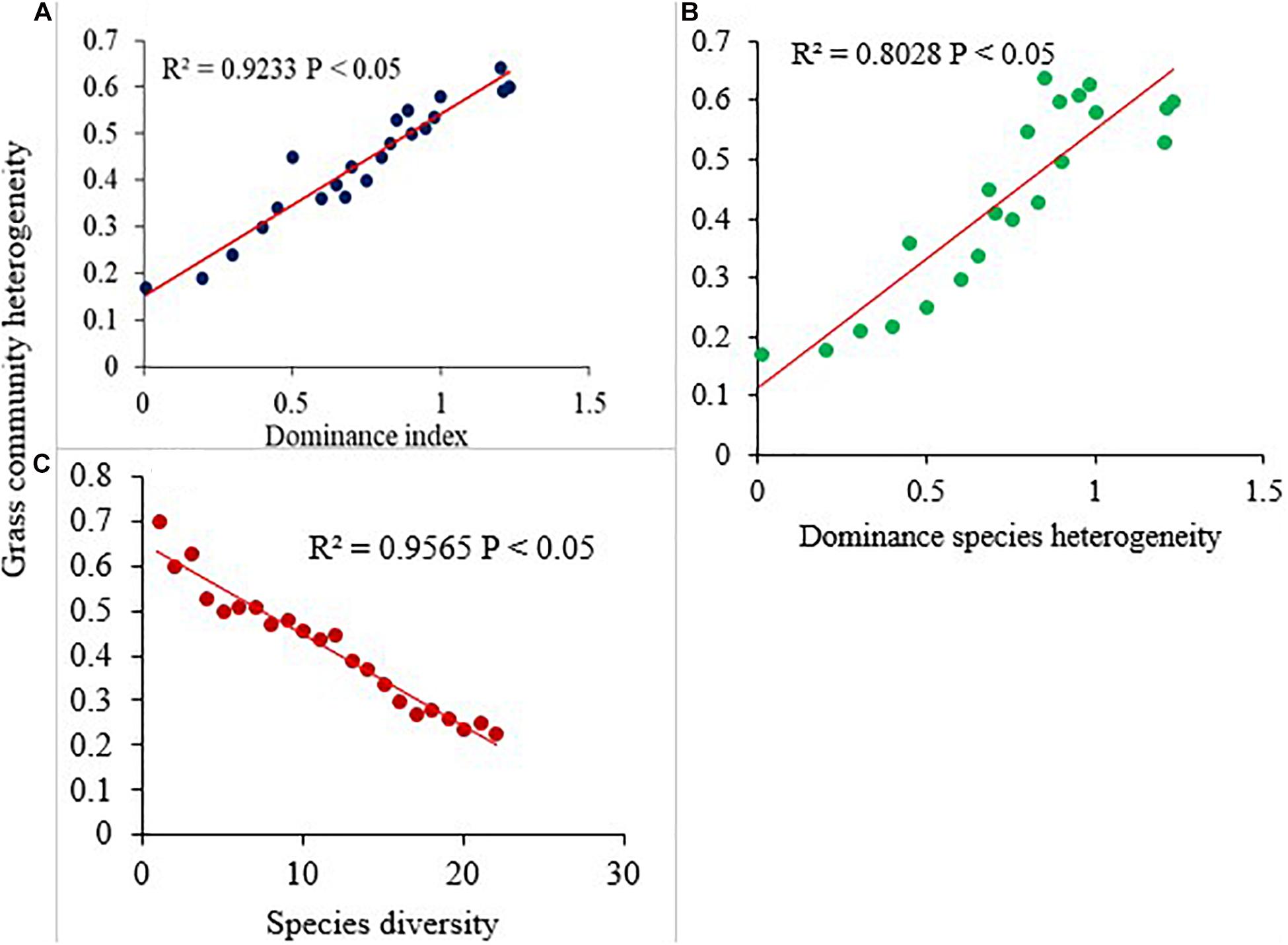
Figure 6. The relationships of dominance index (A), species diversity (B), and dominant species heterogeneity (C) with grass community heterogeneity within the study sites.
Discussion
Effects of Grazing Enclosure on Grass Species’ Composition and Characteristics
In this study, we observed that GE had an impact on height, abundance, diversity, and evenness of vegetation species, and on dominance index in the Borana rangeland site (Figures 2, 3 and Supplementary Table S2). In general, GE facilitated an increment of grass species’ height both in LtGE and StGE treatments, significantly at the StGE site (Figure 2A). This finding is in line with the results found in other studies (Li et al., 2017; Suizi et al., 2019; Guo et al., 2020). This is basically due to the decrease in livestock grazing, which allowed the recovery of species and accumulation of biomass (Muhammad et al., 2019; Song et al., 2020). We found that GE positively impact on grass species’ abundance and increased in both the long-term and the short-term enclosed areas, significantly at Lt GE areas (Figure 2B and Supplementary Table S2). This result verifies the different responses of grass species’ abundance to GE across various enclosure durations in the Borana rangeland (Angassa and Oba, 2010; Dalle et al., 2015). Grass species’ abundance was more vulnerable to grazing intensity and other environmental factors, such as seasonal variation. Such a difference in abundance of grass species between enclosure and grazing plots indicates that the rehabilitation of degraded rangeland through recovery of grass species using GE techniques is possible (Li et al., 2014; Gemedo, 2020). However, in arid and semi-arid rangelands like Borana, a change in grass abundance through GE takes many years, so we strongly recommend that other management strategies, like reseeding local grass species and fertilization or animal manure, may be better to speed up the rehabilitation rate of degraded rangeland by increasing grass abundance. This result is entirely in agreement with the data reported by Tadesse et al. (2002) and Guo et al. (2020).
The response of species’ diversity for GE was both positively and negatively significantly impacted. The highest species’ diversity was recorded in the StGE sites and at the LtGE in both Rs and Ds (Figure 3B). But there were no statistical effects on dominant species’ diversity (Figure 3A and Supplementary Table S2). Our data are consistent with the findings in other grassland ecosystems, both within Ethiopia (Niguse, 2008; Angassa et al., 2010; Meshesha et al., 2019) and in other countries (Li et al., 2014; Zhu et al., 2016; Yao et al., 2019; Guo et al., 2020), and are contradictory to the results reported by others (Zhang and Zhao, 2015; Sagar et al., 2019). The possible reason for the increasing of species’ diversity in the StGE and OG as compared to the LtGE is due to the existence of empty space and heterogeneity of habitats which favors species’ coexistence (Raiesi and Riahi, 2014). Further, the other possible reason for the lower diversity in LtGE is to prevent seeds from freely dispersing, forming climax species, which increases species’ competition for light and water resources, and thus decreases grass diversity (Zhu et al., 2016). This is in line with typical discussions of enclosure duration impact on the grass species’ diversity. Such a condition was explained by the “competitive exclusion hypothesis” (Suizi et al., 2019). As GE duration increases, the competitors gain an advantage and surpass the groups with weaker competition (Digitaria milanjiana, Bothriochloa insculpta, Dactyloctenium aegyptium, and Enteropogon somalensis) (Supplementary Table S2), and thus, become a cause for decreasing species’ evenness. As a result, in the LtGE site, species’ evenness decreased but not significantly, and this was highly observed during dry season. In general, the “intermediate disturbance hypothesis” explained that species’ diversity will increase with the intensity of appropriate grazing in a short-term enclosure, which helps to improve the ecological function of the species to promote the appropriate use of environmental resources (Wan et al., 2015). In addition, seasonal variations considerably influenced the height, abundance, diversity, and evenness of vegetation species, and during the rainy season, an increment of all mentioned grass characteristics was observed across all study treatment areas (Figures 2A,B, 3A–C).
Effects of Grazing Enclosure on Phenological Characteristics of Grass Species
Our study shows that GE had a significant impact on grass phenological advancement and tended to happen earlier in enclosed plots of sampling rangelands in terms of the whole grass community. This is because GE enhanced the growth of grass roots that increased the utilization of underground water resources (Peper et al., 2011; Guo et al., 2020). Further, we found that phenological periods of grass species decreased as the duration of GE increased and an evaluation index of relationship change of phenological stages (EIRCP) showed a decreasing pattern (Figure 4). The phenological stages’ changes were observed in all grass species in all enclosed plots, either increasing or decreasing during both rainy and dry seasons, and our data are in contrast with the data reported by Guo et al. (2020). For further study, we recommend that further evaluation of environmental factors, such as soil moisture, temperature, and microorganisms, in both GE and OG treatments in Borana grasslands, should be carried out to provide a strong conclusion on the impact of GE on grass phenology. It should also be noted that it is difficult to produce enough data using daily specific measurements of grass phenology to use for evaluating the response to GE, unless considering the exact identification of grass species for how many and at what specific times were eaten by grazing livestock (Wu and Thirgood, 2009; Hu et al., 2016).
Effects of Grazing Enclosure on Grass Biomass and Carrying Capacity
The effect of GE on grass biomass production and rangeland CC shows an increasing trend compared to the OG rangeland sites, and our findings were in agreement with the data reported in different areas of Ethiopia (Mengistu et al., 2005; Angassa et al., 2010), Kenya (Oba et al., 2001), Uganda (Lenzi-Grillini et al., 1996), and Tanzania (Mwalyosi, 2000). The reason for an increase in grass biomass in the GE sites is because of the effects of rest and recovery of grass species, and advancement of their phenological period (Wan et al., 2015). The vegetation species recorded in this study area were mainly focused on the species palatable to the livestock species that grazed in the study area; their palatability rate was identified by Mengistu et al. (2005) and Angassa et al. (2010). Further, GE increased the proportion of palatable species and decreased the unpalatable species found in the enclosed areas. The greatest impact on the proportion of palatable species was observed at the LtGE site. The GE duration also showed a significant impact on grass biomass production and this is related to the abundance of grass species (if the abundance of species high, then the biomass is also high) and our result disagrees with the data reported by Zhu et al. (2016) who stated that the age of enclosures has no effect on grass biomass. Seasonal variation had a significant impact on grass species’ productivity and indicates that inter-annual rainfall variability has a vital role in determining the availability of forage biomass in the rangeland site (Figure 5). Furthermore, biomass increased considerably during the Rs compared to the Ds in both the GE and the OG sites (Wan et al., 2015). Our result was somewhat different from this, which suggests that the duration of GE had a negative impact on grass biomass production. Contrary to the suggestions forwarded by Zhu et al. (2016) and Wang et al. (2018), the older GE sites accumulated a high grass biomass and CC potential compared to the younger GE and OG sites. The average annual CC potential was 3.85, 4.75, and 8.25 ha AU–1year–1 for the LtGE, StGE, and OG sites, respectively. This indicted that the LtGE had 23.4 and 114.3% higher CC potential compared with the StGE and OG sites, respectively (Figure 5). Generally, grass biomass production and CC showed a positive correlation with the abundance of grass species (Figure 2B and Supplementary Table S2) and did not have a significant correlation with grass species diversity (Figure 3B and Supplementary Table S2); thus, our result was opposed to the data explained by others (Klein et al., 2007; Zhu et al., 2016; Wang et al., 2018).
Effects of Grazing Enclosure on Grass Community Spatial Heterogeneity
The heterogeneity of grass communities showed an increasing trend due to GE in the study area, which is consistent with the results of a livestock exclusion experiment conducted by Adler and Lauenroth (2000) in a Colorado rangeland site. The results of our study showed that the GE duration had a positive impact on the grass species heterogeneity, which means that if the rangeland enclosed duration was high, then the grass community heterogeneity was high; this is mainly because of the decreasing of species’ diversity while GE duration increased. A simple linear regression model showed that as the species diversity increases, the grass community diversity decreased, and such observable effects of GE are consistent with our estimated hypothesis. The stability of rangeland area is more sensitive to the loss of diversity and this affects not only biomass productivity but also spatial heterogeneity (Yan and Lu, 2015; Yang et al., 2017). The stability of the grazing area is mainly affected by the stability of the dominant species (Yang et al., 2017) and in LtGE dominant species is more stable, which is also one major cause of high grass community heterogeneity (Suizi et al., 2019) (Figure 7). In this study, the LtGE caused the loss of some common species, but increased the dominant species, which led to an increase of grass community spatial heterogeneity. The evidence we have discussed here suggests that LTGE is not more effective in rehabilitation of the degradation of Borana grasslands but increases heterogeneity due to the decreased existing grass diversity, and that StGE is better for improving degraded rangeland and increasing existence grass species, but deceases heterogeneity. Our finding is similar to the results and suggestions found in other studies (Wang et al., 2018; Muhammad et al., 2019; Suizi et al., 2019; Guo et al., 2020; Song et al., 2020) and opposite to the results reported by Angassa and Oba (2008, 2010), Yan and Lu (2015), and Yao et al. (2019).
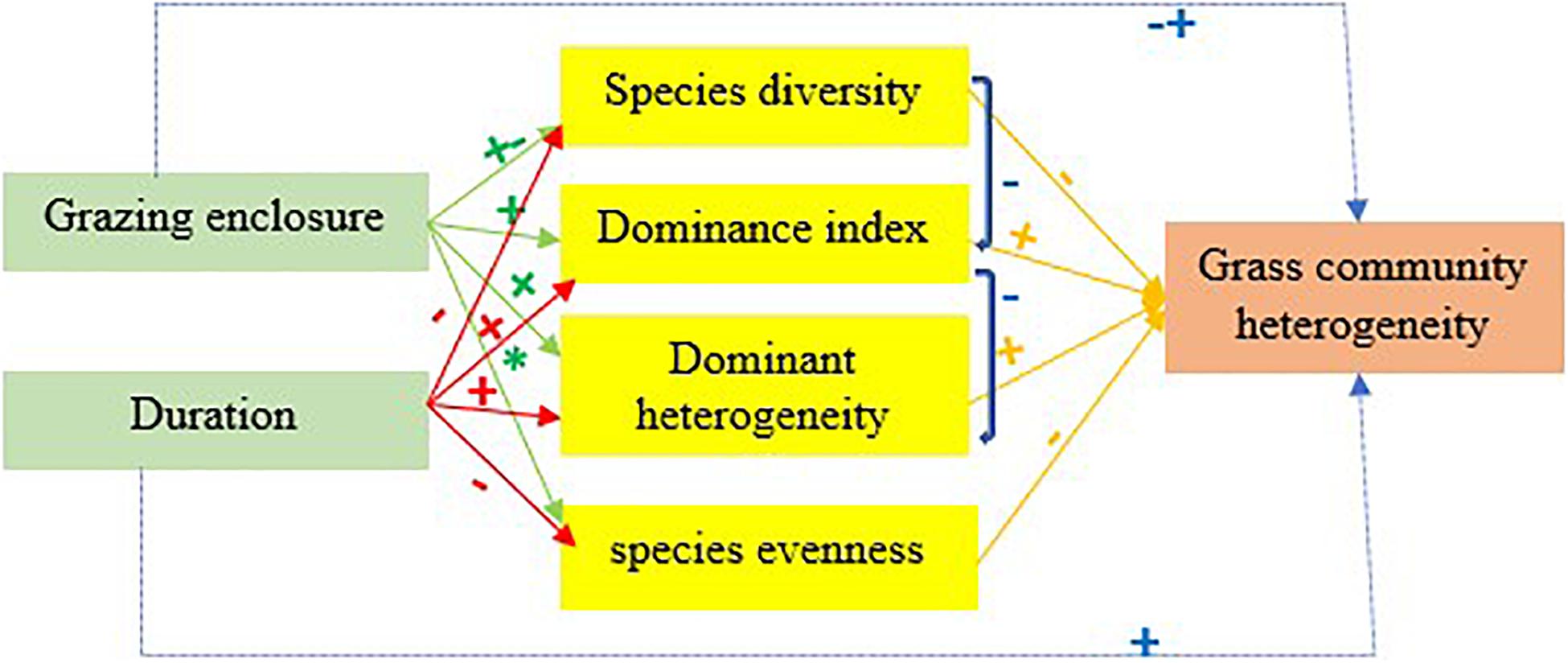
Figure 7. A conceptual model of the effects of grazing enclosure and its duration of application on grass community spatial heterogeneity. Note. + and - signs across each arrow indicate significant positive and negative impacts, and * sign indicates non-significant impacts.
Grazing Enclosure Implications for Rangeland Management Strategies
In order to overcome the effects of rangeland degradation on the Borana rangeland, GE is highly applicable at government and private levels. From a sustainable rangeland management perspective, GE is an effective approach if we apply GE for a short period of time or in the form of rotational grazing, which facilitates a better performance in restoring grass species diversity and promoting species’ coexistence (Angassa et al., 2010; Chen et al., 2015). But if the duration of GE increases, its effectiveness decreases (shows decreasing trends of vegetation diversity, the vegetation phenological periods, height, and evenness).Thus, taking into account the impacts of enclosure duration on rangeland rehabilitation practices is important for the implementation of effective and sustainable management strategies (Komac et al., 2015). Thereby, based on our current findings, using LtGE as a restoration practice is not effective; instead, StGE shows a better capacity for species existence and is also more timely. In addition, GE increased the abundance of grass species and decreased the proportion of bare areas, and also increased the N and C storage capacity through enhancing soil quality in the degraded rangelands (Zhang and Zhao, 2015). In general, our results show that GE is an effective method to rehabilitate degraded rangelands and that it is more effective if used for short time periods (Figure 2B). In the Borana zone, rapid rangeland degradation has become a bottleneck problem for the pastoral community; as a result, the local community, government officials, and other non-governmental organizations are applying the practice of GE to minimize and restore degraded rangelands. Therefore, for an effective solution to this rapid degradation of rangeland, priority should be given to the restoration of the area with a more rapid and timely result and the area selection should be based on the primary objective of techniques and status of the areas (Suizi et al., 2019).
Conclusion
In conclusion, GE was found to have a significant effect on height, abundance, biomass, phenology, diversity, spatial heterogeneity, and CC of vegetation species, yet had no significant effects on species’ evenness and yearly average dominance index in all the treatment sites of the Borana grassland. However, seasonal variation showed a significant variation in species’ dominance index and a relatively lower dominance index was observed in StGE than LtGE and OG during the Rs (Figure 3A). The effects are strongly related to the duration of the GE. The duration of GE has a positive effect on species abundance, biomass, and CC, although it has a negative effect on species’ diversity, phenology, and height of grass species and decreased the proportion of poisonous herbs and weeds. Our results indicated that the LtGE failed to improve grass diversity, height, and species’ phenological periods and changed the grass community heterogeneity in the rangeland. From this, our data indicated that the decrease in grass species’ diversity was the main reason for the increase in grass community heterogeneity in the LtGE at the Borana rangeland. Furthermore, we suggest that application of the StGE practice has restored most grass species’ diversity and maintained their stability. GE also plays a positive role in restoring degraded rangelands and the effects of GE are the highest in StGE techniques. However, for effective management practices, it is strongly recommended that short-term fencing, in the form of rotational grazing and applied reseeding technology, be considered and the use of animal manure and fertilizers should also be considered. In addition, not only management practice, but also seasonal variations have influenced rangeland productivity and diversity of grass species. In general, focusing on the impact of GE on different aspects of the grass species’ characteristics and assessing the interaction effect with other environmental factors are needed for effective and sustainable management of degraded rangeland like Borana. The results of this study may serve as a reference for further study both in the study area and in other rangelands with similar features. Finally, we recommend that including the differences in soil physico-chemical characteristics between GE and OG would be advisable to discuss the effects on rangeland condition both in the Borana and in other similar rangeland areas.
Data Availability Statement
The original contributions presented in the study are included in the article/Supplementary Material. Further inquiries can be directed to the corresponding author/s.
Author Contributions
YF collected available data and contributed to writing up, gap assessment, and design. YY, WY, and XX performed editing and proofing, provided important advice, and supervised the whole work during this project. All authors contributed to the article and approved the submitted version.
Funding
This study received financial support from the African Great Green Wall Adaptation Technical Cooperation Research and Demonstration (2018YFE0106000), Science and Technology Partnership Program, Ministry of Science and Technology of China (Grant No. KY 201702010), and International Cooperation and Exchanges NSFC (Grant No. 41861144020), as well as the support from the CAS Key Technology Talent Program.
Conflict of Interest
The authors declare that the research was conducted in the absence of any commercial or financial relationships that could be construed as a potential conflict of interest.
Acknowledgments
The authors wish to thank the Xinjiang Institute of Ecology and Geography, University of Chinese Academy of Sciences, and CAS-TWAS fellowship program for providing funding and the Ph.D. Scholarship for the first author. The authors greatly thank the local community and stakeholder of the Teltele district for providing us with help and information throughout our research.
Supplementary Material
The Supplementary Material for this article can be found online at: https://www.frontiersin.org/articles/10.3389/fevo.2020.623627/full#supplementary-material
Abbreviations
APS, average phenological period; CC, carrying capacity; EIRCP, evaluation index of relationship changes of phenological stage; GEs, grazing enclosures; LtGE, long-term grazing enclosure; OG, open-grazing; RA, relative abundance; RD, relative dominance; RF, relative frequency; StGE, short-term grazing enclosure.
References
Abebe, M. H., Oba, G., Angassa, A., and Weladji, R. B. (2006). The role of area enclosures and fallow age in the restoration of plant diversity in northern Ethiopia. Afr. J. Ecol. 44, 507–514. doi: 10.1111/j.1365-2028.2006.00664.x
Adler, P. B., and Lauenroth, W. K. (2000). Livestock exclusion increases the spatial heterogeneity of vegetation in Colorado shortgrass steppe. Appl. Veg. Sci. 3, 213–222. doi: 10.2307/1479000
Al-Rowaily, S. L., El-Bana, M. I., Al-Bakre, D. A., Assaeed, A. M., Hegazy, A. K., and Ali, M. B. (2015). Effects of open grazing and livestock exclusion on floristic composition and diversity in natural ecosystem of Western Saudi Arabia. Saudi J. Biol. Sci. 22, 430–437. doi: 10.1016/j.sjbs.2015.04.012
Angassa, A. (2014). The ecological impact of bush encroachment on the yield of grasses in the Borana rangeland ecosystem. Afr. J. Ecol. 43, 14–20. doi: 10.1111/j.1365-2028.2005.00429.x
Angassa, A., and Oba, G. (2008). Herder perceptions on impacts of range enclosures, crop farming, fire ban and bush encroachment on the Rangelands of Borana, Southern Ethiopia. Hum. Ecol. 36, 201–215. doi: 10.1007/s10745-007-9156-z
Angassa, A., and Oba, G. (2010). Effects of grazing pressure, age of enclosures and seasonality on bush cover dynamics and vegetation composition in southern Ethiopia. J. Arid Environ. 74, 111–120. doi: 10.1016/j.jaridenv.2009.07.015
Angassa, A., Oba, G., Treydte, A. C., and Wela, R. B. (2010). Role of traditional enclosures on the diversity of herbaceous vegetation in a semi-arid rangeland, southern Ethiopia. Livestock Res. Rural Dev. 22, 715–725.
Asefa, D. T., Oba, G., Weladji, R. B., and Colman, J. E. (2003). An assessment of restoration of biodiversity in degraded high mountain grazing lands in northern Ethiopia. Land Degrad. Dev. 14, 25–38. doi: 10.1002/ldr.505
Bennie, J., Anderson, K., and Wetherelt, A. (2011). Measuring biodiversity across spatial scales in a raised bog using a novel paired-sample diversity index. J. Ecol. 99, 482–490.
Billi, P., Alemu, Y. T., and Ciampalini, R. (2015). Increased frequency of flash floods in Dire Dawa, Ethiopia: change in rainfall intensity or human impact? Nat. Hazards 76, 1373–1394. doi: 10.1007/s11069-014-1554-0
Chen, J., Shiyomi, M., Wuyunna, L., Hori, Y., and Yamamura, Y. (2015). Vegetation and its spatial pattern analysis on salinized grasslands in the semiarid Inner Mongolia steppe. Grassl. Sci. 61, 121–130. doi: 10.1111/grs.12084
Coppock, L. (1994). The Borana Plateau of Southern Ethiopia: Synthesis of Pastoral Development and Change, 1980-91. Addis Ababa, ET: ILRI publications.
Dalle, G., Brigitte, L. M., and Isselstein, J. (2006). Encroachment of woody plants and its impact on pastoral livestock production in the Borana lowlands, southern Oromia, Ethiopia. Afr. J. Ecol. 44, 113–299.
Dalle, G., Maass, B. L., and Isselstein, J. (2015). Rangeland condition and trend in the semi-arid Borana lowlands, southern Oromia, Ethiopia. Afr. J. Range Forage Sci. 23, 49–58. doi: 10.2989/10220110609485886
Dong, W., Yu, L., Wu, G. L., Ding, L. M., Zheng, Y., and Hao, H. M. (2015). Effect of rest-grazing management on soil water and carbon storage in an arid grassland (China). J. Hydrol. 527, 754–760. doi: 10.1016/j.jhydrol.2015.05.036
Elmore, A. J., Mustard, J. F., Manning, S. J., and Lobell, O. B. (2000). Quantifying vegetation changein semi-arid environments. Precision and accuracy of special mixture analysis and normalizeddifference vegetation index. Remote Sen. Environ. 73, 87–102.
Gemedo, D. (2020). Evaluation of forage quantity and quality in the semi-arid Borana Lowlands, Southern Oromia, Ethiopia. Trop. Grasslands Forrajes Trop. 8, 72–85. doi: 10.17138/tgft(8)72-85
Graff, P., Aguiar, M. R., and Chaneton, E. J. (2007). Shifts in positive and negative plant interactions along a grazing intensity gradient. Ecology 88, 188–199. doi: 10.1890/0012-9658(2007)88[188:sipanp]2.0.co;2
Guo, T., Liao, H. R. L., and Tuvshintogtokhe, I. (2020). ffects of grazing exclusion by fence on vegetation characteristics and community diversity of mongolian grasslands. Appl. Ecol. Environ. Res. 18, 6995–7009. doi: 10.15666/aeer/1805_69957009
Hu, Z. M., Li, S. G., Guo, Q., Niu, S. L., Li, N. H., and He, N. P. (2016). A synthesis of the effect of grazing exclusion on carbon dynamics in grasslands in China. Glob. Chang. Biol. 22, 1385–1393. doi: 10.1111/gcb.13133
Jing, Z., Zuo, X., Xin, Z., Peng, L., Jie, L., and Yue, X. (2017). Long-term grazing effects on vegetation characteristics and soil properties in a semiarid grassland, northern china. Environ. Monit. Assess. 189:216. doi: 10.1007/s10661-017-5947-x
Klein, J. A., Harte, J., and Zhao, X. Q. (2007). Experimental warming, not grazing, decreases rangeland quality on the Tibetan plateau. Ecol. Appl. 17, 541–557. doi: 10.1890/05-0685
Klink, R. V., Nolte, S., Mandema, F. S., Lagendijk, D. D. G., Wallisdevries, M. F., Bakker, J. P., et al. (2016). Effects of grazing management on biodiversity across trophic levels the importance of livestock species and stocking density in salt marshes. Agric. Ecosyst. Environ. 235, 329–339. doi: 10.1016/j.agee.2016.11.00
Komac, B., Pladevall, C., Doménech, M., and Fanlo, R. (2015). Functional diversity and grazing intensity in sub-alpine and alpine grasslands in Andorra. Appl. Veg. Sci. 18, 75–85. doi: 10.1111/avsc.12119
Lenzi-Grillini, C. R., Viskanic, P., and Mapesa, M. (1996). Effects of 20 years of grazing exclusion in an area of the Queen Elizabeth National Park, Uganda. Afr. J. Ecol. 34, 333–341. doi: 10.1111/j.1365-2028.1996.tb00629.x
Li, Q., Zhou, D. W., Jin, Y. H., Wang, M. L., Song, Y. T., and Li, G. D. (2014). Effects of fencing on vegetation and soil restoration in a degraded alkaline grassland in northeast China. J. Arid Land. 6, 478–487. doi: 10.1007/s40333-013-0207-6
Li, W., Cao, W. X., Wang, J. L., Li, X. L., Xu, C. L., and Shi, S. L. (2017). Effects of grazing regime on vegetation structure, productivity, soil quality, carbon and nitrogen storage of alpine meadow on the Qinghai-Tibetan Plateau. Ecol. Eng. 98, 123–133. doi: 10.1016/j.ecoleng.2016.10.026
Liu, H., Mi, Z., Lin, L., Wang, Y., Zhang, Z., and Zhang, F. (2018). Shifting plant species composition in response to climate change stabilizes grassland primary production. Proc. Natl. Acad. Sci. U.S.A. 115, 4051–4056. doi: 10.1073/pnas.1700299114
Luzuriaga, A. L., Sánchez, A. M., Maestre, F. T., and Escudero, A. (2012). Assemblage of a semi-arid annual plant community: abiotic and biotic filters act hierarchically. PLoS One 7:e41270. doi: 10.1371/journal.pone.0041270
Ma, Q. Q., Chai, L. R., Hou, F. J., Chang, S. H., Ma, Y. S., Tsunekawa, A., et al. (2019). Quantifying grazing intensity using remote sensing in Alpine meadows on Qinghai-Tibetan Plateau. Sustainability 11:417. doi: 10.3390/su11020417
Maestre, F. T., and Reynolds, J. F. (2006). Spatial heterogeneity in soil nutrient supply modulates nutrient and biomass responses to multiple global change drivers in model grassland communities. Glob. Chang. Biol. 12, 2431–2441. doi: 10.1111/j.1365-2486.2006.01262.x
Mengistu, T., Teketay, D., Hulten, H., and Yemshaw, Y. (2005). The role of enclosures in the recovery of woody vegetation in degraded dryland hillsides of central and northern Ethiopia. J. Arid Environ. 60, 259–281. doi: 10.1016/j.jaridenv.2004.03.014
Meshesha, D. T., Moahmmed, M., and Yosuf, D. (2019). Estimating carrying capacity and stocking rates of rangelands in Harshin District, Eastern Somali Region, Ethiopia. Ecol. Evol. 9, 13309–13319. doi: 10.1002/ece3.5786
Muhammad, A. P., Shota, S., Maica, K. A. G., Jerrold, M. T., Hiromu, I., Momoka, N., et al. (2019). Grazing enhances species diversity in grassland communities. Sci. Rep. 9:11201.
Mwalyosi, R. B. B. (2000). Vegetation changes following land reclamation in the Kondoa Eroded Area, central Tanzania. Afr. J. Ecol. 38, 265–268. doi: 10.1046/j.1365-2028.2000.00257.x
Niguse, B. (2008). Ecological Impacts of Bush Encroachment on Rangeland Ecosystem:The Case of Hallona and Medhacho Pastoralist Associations in Borana Lowlands. M.Sc. Thesis, Addis Ababa University, Ethiopia.
Oba, G., Vetaas, O. R., and Stensteth, N. C. (2001). Relationships between biomass and plant species richness in arid-zone grazing lands. J. Appl. Ecol. 38, 836–846. doi: 10.1046/j.1365-2664.2001.00638.x
Peper, J., Jansen, F., Pietzsch, D., and Manthey, M. (2011). Patterns of plant species turnover along grazing gradients. J. Veg. Sci. 3, 457–466. doi: 10.1111/j.1654-1103.2011.01260.x
Porensky, L., and Young, T. P. (2013). Herbivory and drought interact to enhance spatial patterning and diversity in a savanna understory. Oecologia 173, 591–602. doi: 10.1007/s00442-013-2637-4
Raiesi, F., and Riahi, M. (2014). The influence of grazing exclosure on soil c stocks and dynamics, and ecological indicators in upland arid and semi-arid rangelands. Ecol. Indic. 41, 145–154. doi: 10.1016/j.ecolind.2014.01.040
Sagar, R., Li, G. Y., Singh, J. S., and Wan, S. Q. (2019). Carbon fluxes and species diversity in grazed and fenced typical steppe grassland of Inner Mongolia, China. J. Plant Ecol. 12, 199–199. doi: 10.1093/jpe/rtx069
Shao, H., Sun, X., Wang, H., Zhang, X., Xiang, Z., Tan, R., et al. (2016). A method to the impact assessment of the returning grazing land to grassland project on regional eco-environmental vulnerability. Environ. Impact Assess. Rev. 56, 155–167. doi: 10.1016/j.eiar.2015.10.006
Sintayehu, M. (2006). Changes in Land Cover and Soil Conditions for the Yabello District of the Borana Plateau, 1973-2003. Research Brief 06-06-PARIMA. GL-CRSP/University of California. Davis, CA: University of California.
Song, S., Zhum, J., Zheng, T., Tang, Z., Zhang, F., Ji, C., et al. (2020). Long-term grazing exclusion reduces species diversity but increases community heterogeneity in an Alpine Grassland. Front. Ecol. Evol. 8:66. doi: 10.3389/fevo.2020.00066
Suizi, W., Jiangwen, F., Yuzhe, L., and Lin, H. (2019). Effects of grazing exclusion on biomass growth and species diversity among various grassland types of the Tibetan Plateau. Sustainability 11:1705. doi: 10.3390/su11061705
Tadesse, G., Mohamed, S. M. A., Abyie, A., and Wagnew, A. (2002). Impact of grazing on plant species richness, plant biomass, plant attribute, and soil physical and hydrological properties of vertisol in East African Highlands. Environ. Manag. 29, 279–289. doi: 10.1007/s00267-001-0014-2
Van wilgen, B. W., and Richardson, D. M. (2014). Challenges and trade-offs in the management of invasive alien trees. Biol. Invas. 16, 721–734. doi: 10.1007/s10530-013-0615-8
Wan, W., Bai, Y., Hooper, D. U., Schönbach, P., Gierus, M., Schiborra, A., et al. (2015). Selective grazing and seasonal precipitation play key roles in shaping plant com- munity structure of semi-arid grasslands. Landsc. Ecol. 30, 1767–1782. doi: 10.1007/s10980-015-0252-y
Wang, X., Xinguo, Y., Lei, W., Lin, C., Naiping, S., Junlong, G., et al. (2018). A six-year grazing exclusion changed plant species diversity of a Stipa breviflora desert steppe community, northern China. PeerJ 6:e4359. doi: 10.7717/peerj.4359
Weigelt, A., Schumacher, J., Roscher, C., and Schmid, B. (2008). Does biodiversity increase spatial stability in plant community biomass? Ecol. Lett. 11, 338–347. doi: 10.1111/j.1461-0248.2007.01145.x
Wu, G. L., and Thirgood, S. (2009). Effect of fencing and grazing on a kobresia-dominated meadow in the Qinghai-Tibetan Plateau. Plant Soil 319, 115–126. doi: 10.1007/s11104-008-9854-3
Wu, T. N., Wu, G. L., Wang, D., and Shi, Z. H. (2014). Soil-hydrological properties response to grazing enclosure in a steppe grassland of the Loess Plateau. Environ. Earth Sci. 71, 745–752. doi: 10.1007/s12665-013-2476-y
Xiong, D. P., Shi, P. L., Zhang, X. Z., and Zou, C. B. (2016). Effects of grazing exclusion on carbon sequestration and plant diversity in grasslands of China. A meta-analysis. Ecol. Eng. 94, 647–655. doi: 10.1016/j.ecoleng.2016.06.124
Yan, Y., and Lu, X. (2015). Is grazing exclusion effective in restoring vegetation in degraded alpine grasslands in Tibet, China? PeerJ 3:e1020. doi: 10.7717/peerj.1020
Yang, Z., Zhang, Q., Su, F., Zhang, C., Pu, Z., Xia, J., et al. (2017). Daytime warming lowers community temporal stability by reducing the abundance of dominant, stable species. Glob. Chang. Biol. 23, 154–163. doi: 10.1111/gcb.13391
Yao, X. X., Wu, J. P., Gong, X. Y., Lang, X., Wang, C. L., Song, S. Z., et al. (2019). Effects of long-term fencing on biomass, coverage, density, biodiversity and nutritional values of vegetation community in an alpine meadow of the Qinghai-Tibet Plateau. Ecol. Eng. 130, 80–93. doi: 10.1016/j.ecoleng.2019.01.016
Yeneayehu, F., Xu, X. W., and Wang, Y. D. (2020). Forage composition, biomass and carrying capacity dynamics in Yabello rangeland, southern Ethiopia. Using different grazing sites. Appl. Ecol. Environ. Res. 18, 7233–7253. doi: 10.15666/aeer/1805_72337253
Zhang, Y. Y., and Zhao, W. Z. (2015). Vegetation and soil property response of short-time fencing in temperate desert of the Hexi Corridor, northwestern China. Catena 133, 43–51. doi: 10.1016/j.catena.2015.04.019
Zhao, K., Wang, Z. Y., Li, Y. Q., Zheng, T. L., and Hu, J. B. (2019). Effect of two restorations on the grassland coverage after remediation of take-abandon soil field on the Qinghai-Tibet Plateau. Pratacult. Sci. 36, 2500–2507. doi: 10.11829/j.issn.1001-0629.2019-0066
Keywords: enclosure, species diversity, heterogeneity, Borana, productivity
Citation: Fenetahun Y, Yuan Y, Xinwen X and Yongdong W (2021) Effects of Grazing Enclosures on Species Diversity, Phenology, Biomass, and Carrying Capacity in Borana Rangeland, Southern Ethiopia. Front. Ecol. Evol. 8:623627. doi: 10.3389/fevo.2020.623627
Received: 30 October 2020; Accepted: 21 December 2020;
Published: 25 January 2021.
Edited by:
Manuel Esteban Lucas-Borja, University of Castilla La Mancha, SpainReviewed by:
Stephen M. Mureithi, University of Nairobi, KenyaRodolfo Golluscio, Universidad Nacional de Buenos Aires, Argentina
Copyright © 2021 Fenetahun, Yuan, Xinwen and Yongdong. This is an open-access article distributed under the terms of the Creative Commons Attribution License (CC BY). The use, distribution or reproduction in other forums is permitted, provided the original author(s) and the copyright owner(s) are credited and that the original publication in this journal is cited, in accordance with accepted academic practice. No use, distribution or reproduction is permitted which does not comply with these terms.
*Correspondence: Wang Yongdong, wangyd@ms.xjb.ac.cn