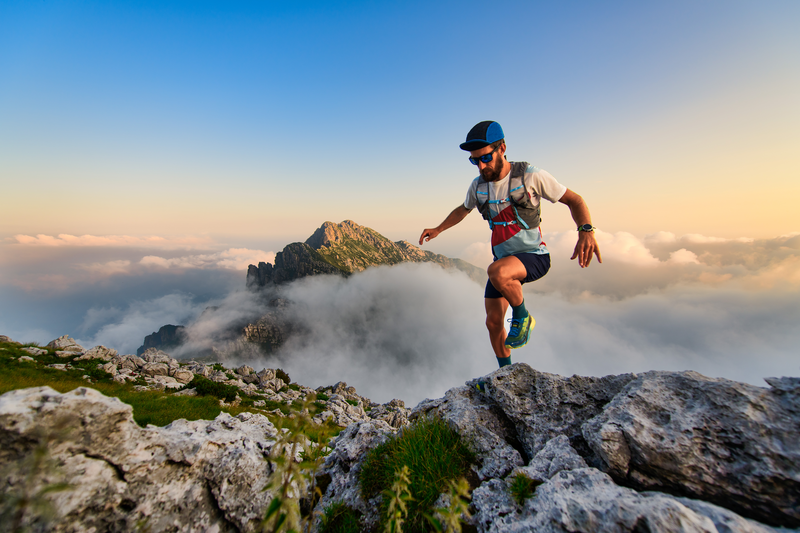
94% of researchers rate our articles as excellent or good
Learn more about the work of our research integrity team to safeguard the quality of each article we publish.
Find out more
BRIEF RESEARCH REPORT article
Front. Ecol. Evol. , 11 January 2021
Sec. Conservation and Restoration Ecology
Volume 8 - 2020 | https://doi.org/10.3389/fevo.2020.622434
This article is part of the Research Topic Conservation of European Freshwater Crayfish View all 16 articles
Procambarus clarkii is a worldwide freshwater invasive crustacean from North America and was first introduced into Europe the 1970s. Along with P. clarkii, another invasive alien species was also spreading: Aphanomyces astaci. This pathogen is listed among the 100 World’s worst invasive species and involved in the European native crayfish decline. Although both species live in freshwater ecosystems, P. clarkii can withstand brackish waters and inhabit estuarine habitats. However, the presence of A. astaci associated to North American crayfishes has never been described in brackish waters. In this study, we have investigated the presence of A. astaci in a P. clarkii population of a Mediterranean coastal lagoon in the Albufera Natural Park, Valencia, Spain introduced in 1976. Our study confirmed the presence of this pathogen, and suggests that A. astaci has been spreading for more than four decades in the mentioned estuarine environment. Mitochondrial ribosomal rnnS and rnnL indicated that the isolated pathogen belongs to d1-haplotype (i.e., D-haplogroup) typically hosted by P. clarkii. The presence of this pathogen in a brackish environment may suggest a better adaptation than other A. astaci strains to adverse conditions, such as high salinity levels. This is a matter of concern for the conservation of European native freshwater crayfish and highlights once more the risk of introducing invasive alien crustaceans.
Biological invasions represent one of the main threats to biodiversity. Some of the alien species causing these invasions are now common throughout the world and are driving the existing biodiversity toward homogenization (Piscart et al., 2011). Among the diverse ecosystems affected by invasive alien species, freshwater ecosystems are particularly rapidly altered and according to the European Union the impact of invaders accounts for billions of euros yearly (Tollington et al., 2015).
The success of freshwater invasive species seems to be due to a combination of several traits, such as aggressive behavior, higher metabolic, and growth rates, greater fecundity, omnivory, and great tolerance to different pH and salinities (Firkins, 1993; Tollington et al., 2015). In particular, freshwater crustaceans represent some of the most successful aquatic alien invaders (Hänfling et al., 2011). In European freshwater ecosystems, these represent about 53% of all macroinvertebrate invasive species, and almost half of the freshwater decapods in European waters, ca 46%, are invasive species (Karatayev et al., 2009). Five species of freshwater decapods of North American origin, i.e., the freshwater crayfish Faxonius limosus, F. virilis, Pacifastacus leniusculus, Procambarus clarkii, and P. virginalis, are included in a list of invasive alien species of the European Union concern pursuant to Regulation (EUR-lex, 2016) because of the alarming increasing impact to the flora and fauna of European ecosystems (Simberloff and Rejmánek, 2011).
Specifically, the red swamp crayfish, P. clarkii, was introduced into Europe in 1973 from Louisiana (see review by Alonso et al., 2000) and was identified as a high-risk species (Gherardi et al., 2011; Souty-Grosset et al., 2016; Oficialdegui et al., 2019). The high ecological plasticity of this species allows it to colonize most types of water bodies (Fidalgo et al., 2001; Scalici et al., 2010). Besides its omnivore condition, this invasive species is a major predator of aquatic vertebrates (i.e., amphibians and fishes) and invertebrates, and is responsible for the decline and local extinctions of many native species (Cruz and Rebelo, 2005; Geiger et al., 2005; Rodríguez et al., 2005; Casellato and Masiero, 2011). Since its introduction, P. clarkii has negatively affected freshwater ecosystems (Gherardi et al., 2011; Arce and Diéguez-Uribeondo, 2015), and native crayfish species (Diéguez-Uribeondo et al., 1997; Rezinciuc et al., 2015; Souty-Grosset et al., 2016; Martín-Torrijos et al., 2019). The latter was due to the fact that P. clarkii is a chronic carrier of the crayfish plague pathogen Aphanomyces astaci (Diéguez-Uribeondo and Söderhäll, 1993; Diéguez-Uribeondo et al., 1995; Aquiloni et al., 2010; Rezinciuc et al., 2014; Martín-Torrijos et al., 2019). This pathogenic oomycete is listed among the 100 World’s worst invasive species (Lowe et al., 2000) and has provoked a rapid decline of native European freshwater crayfishes due to a disease named crayfish plague (see review by Rezinciuc et al., 2015).
This A. astaci carrier has colonized several coastal and saline environments in Europe (Fidalgo et al., 2001; Scalici et al., 2010; Sousa et al., 2013; Meineri et al., 2014) due to its resilience. Scalici et al. (2010) demonstrated that a P. clarkii population was capable of live and reproduce in a brackish wetland in Italy, with salinity varying between 16,200 and 29,600 ppm. Procambarus clarkii’s ability consists in regulating its metabolism (Casellato and Masiero, 2011) and adapting it to saline environments by osmoregulating the ions and compatible solutes in their hemolymph. This adaptation has enabled this invasive species to live and reproduce in diverse water salinities (Fidalgo et al., 2001; Scalici et al., 2010; Casellato and Masiero, 2011; Sousa et al., 2013; Meineri et al., 2014; Bissattini et al., 2015; Vodovsky et al., 2017; Dörr et al., 2020). However, there are scarce studies focusing on the tolerance, survival and dispersion in relation to salinity conditions of the crayfish plague pathogen, that is chronically carried in the cuticle of this invasive North American crayfish species.
First studies on A. astaci physiological response and zoospore production under different salt concentrations showed that high mineral salt concentrations inhibited the growth and sporulation (Unestam, 1969a,b). Moreover, Persson and Söderhäll (1986) and Rantamäki et al. (1992) suggested that A. astaci would barely survive for long or spread under brackish water conditions. However, investigations carried out in other Aphanomyces spp. such as A. invadans (Kiryu et al., 2005), and other closely related genera such as Phytophthora or Saprolegnia (Harrison and Jones, 1975; Padgett, 1984; Hearth and Padgett, 1990; Ali, 2009; Preuett et al., 2016) suggested that some oomycetes might survive under high salinity conditions and sporulate when salinity decreases.
So far, no studies regarding presence of A. astaci in their native carriers, i.e., North American freshwater species, living under adverse conditions of high salinity, have been performed in nature. The naturalized population of P. clarkii from the shallow Mediterranean coastal lagoon of the Albufera Natural Park of Valencia, in the east coast of the Iberian Peninsula, represents an ideal opportunity to investigate the survival of A. astaci in its original host under saline conditions. This Natural Park is listed in the Ramsar List of Wetlands of International Importance (RAMSAR, 2020) and Natura 2000 site (EU, 2020), and during the past decades have suffered from several anthropogenic impacts, shaping the lake to a seriously deteriorated ecosystem (Martín et al., 2020). The invasive P. clarkii was introduced there in 1976 and since then, the decline of the native white clawed crayfish populations, Austropotamobius pallipes, in Valencia has been associated to the co-introduction of both P. clarkii and A. astaci (Galindo et al., 2000; Martín-Torrijos et al., 2019).
The detection of crayfish plague pathogen and its genetic diversity can currently accurately be performed in clinical samples (Oidtmann et al., 2004, 2006; Vrålstad et al., 2009; Makkonen et al., 2018). Therefore, this work aims to detect whether the introduced P. clarkii still carry this pathogen in a Mediterranean brackish lagoon after four decades of its introduction. This information is necessary to better understand crayfish plague epidemiology and its survival in its chronically infected carriers in different ecosystems.
The lagoon of the Albufera Natural Park (Valencia) has the surface area of 2,433 ha, and comprises brackish water (salinity 1,280–1,920 ppm). We used one baited funnel trap during 24 h to collect a total of 40 individuals of P. clarkii in December 2018 from this Natural Park. The individuals collected were transferred to aquaria in the Real Jardín Botánico (RJB-CSIC) facilities in Madrid. To test the prevalence of the pathogen A. astaci in the sampled P. clarkii, we maintained the aquaria at 17°C until the crayfish molted.
Molts were first kept in sterile distilled water for 3 days and observed for the presence of melanization spots and growing hyphae (Figure 1). If any growing hyphae were detected, we preserved part of the sample in 96% ethanol for further molecular analyses. Genomic DNA was isolated with the E.Z.N.A.® Insect DNA Kit (Omega Bio-Tek, Norcross, Georgia, United States). We performed a single round of PCR for the extracted DNA with the A. astaci diagnostic primers 42 (Oidtmann et al., 2006) and 640 (Oidtmann et al., 2004) (which amplify the ITS1 and ITS2 surrounding the 5.8S rDNA), according to the assay described by Oidtmann et al. (2006). All samples which PCR products were sequenced and matched with A. astaci (i.e., specific primers 42 and 640) were further analyzed in order to describe their genetic diversity (i.e., haplogroup and haplotype pathogen characterization). In order to do it, we amplified mitochondrial ribosomal small (rnnS) and large (rnnL) subunits as described in Makkonen et al. (2018). For all PCR reactions, we used a positive control (i.e., genomic DNA from a A. astaci SAP-Málaga5 pure culture; Makkonen et al., 2016) and a negative control (distilled Milli-Q water). Amplified products were visualized by electrophoresis in 1% agarose TAE gels stained with 0.5 μM SBYR1 Safe (Thermo Fisher Scientific, Waltham, MA, United States). Both strands of amplified PCR products (ITS, rnnS, and rnnL) were sequenced using an automated sequencer (Applied Biosystems 3730xl DNA, Macrogen, The Netherlands). Consensus sequences were assembled and edited with Geneious Prime 2019.2.1.
Figure 1. An Aphanomyces astaci growing hypha and sporangium. The sporangium is formed from a hypha (h) growing from the cuticle of a Procambarus clarkii specimen originated from Albufera Natural Park, Valencia. Primary zoospores (pz) move toward the hyphal tip to form a characteristic spore ball sporangium (s) that will eventually produce secondary zoopores, i.e., infective unit. Bar = 100 μm.
Procambarus clarkii from Albufera Natural Park exhibited characteristic melanized areas in the soft abdominal cuticle and pereiopods. Microscopically, in these melanized areas we observed hyphal growth and also sporangia of A. astaci (Figure 1).
For a total of 40 analyzed crayfish, we obtained five positive samples for A. astaci based on amplification of the nuclear ribosomal ITS region (amplified by diagnostic primers 42 and 640 for A. astaci). These five sequences (GenBank accession numbers MW332633–MW332637) were identical and showed a 99.82% similarity to other A. astaci deposited in GenBank (e.g., sequence FM999249 of the isolate SAP302). Moreover, the mitochondrial rnnS and rnnL subunits belonged to the D-haplogroup, allowing us to concatenate both regions to obtain the d1-haplotype (GenBank accession number for rnnS MW174856–MW174860 and for rnnL MW174851–MW174855).
The ability of P. clarkii to survive and reproduce in saline environments have been widely reported (Fidalgo et al., 2001; Scalici et al., 2010; Casellato and Masiero, 2011; Sousa et al., 2013; Meineri et al., 2014; Bissattini et al., 2015; Vodovsky et al., 2017; Dörr et al., 2020). However, the tolerance of A. astaci to grow in saline environments in its natural carriers, i.e., North American crayfish, have never been investigated. Panteleit et al. (2018) did not find A. astaci when tested its prevalence in nine marine decapods from the Black Sea. However, in this study, we report and describe for the first time the presence of the crayfish plague pathogen in a P. clarkii population that lives in a saline environment, a Mediterranean coastal lagoon of the Albufera Natural Park. The presence of the crayfish plague within a similar scenario, had only been reported in the Danube Delta and in the narrow-clawed crayfish (Astacus leptodactylus) (Schrimpf et al., 2012). Although these crayfish were in the vicinity of the river mouth, they might represent a real threat if their A. astaci strain could survive salinity concentrations, such as those of the Albufera Natural Park.
Procambarus clarkii was introduced in the Albufera Natural Park in 1976 (Galindo et al., 2000) and this population has survived in this lagoon until today. Our results show the presence of the pathogen A. astaci in this population, which corresponds to the same genetic group, i.e., D-haplogroup, identified in founder population of P. clarkii introduced in Spain in 1973 (Diéguez-Uribeondo et al., 1995; Makkonen et al., 2018; Martín-Torrijos et al., 2019). This lagoon is a changing environment with high salinity, accumulations of sediments, hypertrophic status, and intense daily oscillations in pH and dissolved oxygen (Martín et al., 2020). Previous studies on physiological adaptations of A. astaci isolates of the D-haplogroup showed that they can grow and sporulate at warmer temperatures than other genetic groups (Diéguez-Uribeondo et al., 1995). Physiological characteristics of this D-haplogroup may indicate a better ability to adapt to adverse conditions (e.g., higher temperatures, high salinity levels or low dissolved oxygen concentrations). Although brackish conditions are known to prevent A. astaci transmission (Rantamäki et al., 1992), the salinity of the Albufera Natural Park decreases during the months of May and September due to the rainfall and rice field irrigations. This fact appears to allow the dispersion of the pathogen by formation of the infection units of the pathogen, i.e., the swimming zoospores that infect other crayfish (Rantamäki et al., 1992). A similar effect was described by Kiryu et al. (2005) for the survival and spread of A. invadans in estuarine environments.
The resilience of both P. clarkii and A. astaci in saline environments constitutes an additional difficulty to the management of threatened populations of native European freshwater crayfish, which are susceptible to the crayfish plague pathogen, and, especially, to the native species of the Iberian Peninsula, A. pallipes. Currently, only 25 populations of this endangered native crayfish species remain in the Valencia province in small isolated highland streams (Generalitat Valenciana, 2019). Specimens of P. clarkii are continuously translocated and can transport this disease to surrounding areas, and potentially transmit it to certain decapods that might become vectors for this pathogen (e.g., the Chinese mitten crab, Eriocheir sinensis, or the semi-terrestrial Potamon potamios appear to be capable of transmitting A. astaci to the European native crayfish; Schrimpf et al., 2014; Svoboda et al., 2014). In the Albufera Natural Park, P. clarkii coexists with the blue crab, Callinectes sapidus that migrates into freshwater habitats during its live cycle (Hines et al., 1987) and since we found that P. clarkii can carry and transmit the crayfish plague pathogen, this dispersion could be favored by this invasive crab.
Therefore, this work alerts to the authorities and decision makers to rapidly develop and implement action plans to avoid the translocation from the Albufera Natural Park of P. clarkii and other potential carriers of A. astaci such as C. sapidus. Thus, future studies on salinity tolerance should be designed in order to determine the physiological adaptations of the different A. astaci genetic groups, highlighting brackish environments as favorable habitats for the maintenance of A. astaci. Moreover, transmission experiments in saline environments should be performed in order to get future insights about the biological characteristics of this pathogen and its possible transmission to other crustaceans. Furthermore, considering the current distribution of P. clarkii introductions and their negative impacts in non-European freshwater ecosystems (specifically for non-North American crayfish), these findings should be of worldwide aware and concern.
The datasets presented in this study can be found in online repositories. The names of the repository/repositories and accession number(s) can be found in the article/supplementary material.
LM-T and JD-U contributed to the design, supervision, and writing of this manuscript. AC-V conducted the sample field collection and carried out the molecular analyses (DNA extraction and haplotyping). AP conducted the sample field collection. All authors contributed to the article and approved the submitted version.
The authors declare that the research was conducted in the absence of any commercial or financial relationships that could be construed as a potential conflict of interest.
We acknowledge support of the publication fee to the Frontiers Fee Support program and the CSIC Open Access Publication Support Initiative through its Unit of Information Resources for Research (URICI). We want to thank the assistance CCEDCV-EL PALMAR (Conselleria d’Agricultura, Desenvolupament Rural, Emergència Climàtica i Transició Ecològica, Generatlitat Valenciana) for allowing the sampling and the performance of this research. We also want to thank the Master in Biodiversidad en Áreas Tropicales y su Conservación UIMP-CSIC, and María Martinez-Ríos and Gloria Casabella-Herrero from the RJB–CSIC for their technical assistance.
Ali, E. H. (2009). Antifungal activity of sodium chloride on Saprolegnia diclina and Aphanomyces sp. Acta Mycol. 44, 125–138. doi: 10.5586/am.2009.011
Alonso, F., Temiño, C., and Diéguez-Uribeondo, J. (2000). Status of the white clawed crayfish, Austropotamobius pallipes (Lereboullet, 1858) in Spain: distribution and legislation. B. Fr. Peche Piscic. 356, 31–54. doi: 10.1051/kmae:2000003
Aquiloni, L., Martín, M. P., Gherardi, F., and Diéguez-Uribeondo, J. (2010). The North American crayfish Procambarus clarkii is the carrier of the oomycete Aphanomyces astaci in Italy. Biol. Invasions 13, 359–367. doi: 10.1007/s10530-010-9828-2
Arce, J., and Diéguez-Uribeondo, J. (2015). Structural damage caused by the invasive crayfish Procambarus clarkii (Girard, 1852) in rice fields of the Iberian Peninsula: a study case. J. Fundam. Appl. Limnol. 186, 259–269. doi: 10.1127/fal/2015/0715
Bissattini, A. M., Traversetti, L., Bellavia, G., and Scalici, M. (2015). Tolerance of increasing water salinity in the red swamp crayfish Procambarus clarkii (Girard, 1852). J. Crustac. Biol. 35, 682–685. doi: 10.1163/1937240X-00002366
Casellato, S., and Masiero, L. (2011). Does Procambarus clarkii (Girard, 1852) represent a threat for estuarine brackish ecosystems of Northeastern Adriatic coast (Italy)? J. Life Sci. 5, 549–554.
Cruz, M. J., and Rebelo, R. (2005). Vulnerability of Southwest Iberian amphibians to an introduced crayfish. Procambarus clarkii. Amphib-reptil. 26, 293–303. doi: 10.1163/156853805774408577
Diéguez-Uribeondo, J., and Söderhäll, K. (1993). Procambarus clarkii as a vector for the crayfish plague fungus Aphanomyces astaci Schikora. Aquacult. Fish. Manag. 24, 761–765. doi: 10.1111/j.1365-2109.1993.tb00655.x
Diéguez-Uribeondo, J., Huang, T.-S., Cerenius, L., and Söderhäll, K. (1995). Physiological adaptation of an Aphanomyces astaci strain isolated from the freshwater crayfish Procambarus clarkii. Mycol. Res. 99, 574–578. doi: 10.1016/s0953-7562(09)80716-8
Diéguez-Uribeondo, J., Temiño, C., and Muzquiz, J. L. (1997). The crayfish plague fungus. Aphanomyces astaci in Spain. B. Fr. Peche Piscic. 347, 753–763.
Dörr, A. J. M., Scalici, M., Caldaroni, B., Magara, G., Scoparo, M., Goretti, E., et al. (2020). Salinity tolerance of the invasive red swamp crayfish Procambarus clarkii (Girard, 1852). Hydrobiologia 847, 2065–2081. doi: 10.1007/s10750-020-04231-z
EU, (2020). Thirteenth update of the list of sites of community importance for the Mediterranean Biogeographical Region. Belgium: OJEU.
EUR-lex (2016). Commission Implementing Regulation (EU) 2016/1141 of 13 July 2016 adopting a list of invasive alien species of union concern pursuant to Regulation (EU) No 1143/2014 of the European Parliament. Belgium: Official Journal of the European Union.
Fidalgo, M. L., Carvalho, A. P., and Paulo, S. (2001). Population dynamics of the red swamp crayfish, Procambarus clarkii (Girard, 1852) from the Aveiro region, Portugal (Decapoda, Cambaridae). Crustaceana 74, 369–375. doi: 10.1163/156854001300104453
Firkins, I. (1993). Environmental tolerances of three species of freshwater crayfish. [PhD thesis]. [Nottingham (United Kingdom)]: University of Nottingham.
Galindo, J., Monzó, J., and Sancho, V. (2000). Estatus del cangrejo de río (Austropotamobius pallipes) en Valencia. Dugastella 1, 9–11.
Geiger, W., Alcorlo, P., Baltanás, A., and Montes, C. (2005). Impact of an introduced crustacean on the trophic webs of Mediterranean wetlands. Biol. Invasions 7, 49–73. doi: 10.1007/1-4020-3870-4_6
Generalitat Valenciana (2019). Actuaciones de conservación del cangrejo de río en la Comunitat Valenciana. Campaña 2019. Valencia: Generalitat Valenciana.
Gherardi, F., Aquiloni, L., Diéguez-Uribeondo, J., and Tricarico, E. (2011). Managing invasive crayfish: Is there a hope? Aquat. Sci. 73, 185–200. doi: 10.1007/s00027-011-0181-z
Hänfling, B., Edwards, F., and Gherardi, F. (2011). Invasive alien crustacea: dispersal, establishment, impact and control. BioControl 56, 573–595. doi: 10.1007/s10526-011-9380-8
Harrison, J. L., and Gareth Jones, E. B. (1975). The effect of salinity on sexual and asexual sporulation of members of the Saprolegniaceae. Trans. Brit. Mycol. Soc. 65, 389–394. doi: 10.1016/s0007-1536(75)80035-0
Hearth, J. H., and Padgett, D. E. (1990). Salinity tolerance of an Aphanomyces isolate (Oomycetes) and its possible relationship to ulcerative mycosis (UM) of Atlantic menhaden. Mycologia 82, 364–369. doi: 10.1080/00275514.1990.12025892
Hines, A. H., Lipcius, R. N., and Haddon, A. M. (1987). Population dynamics and habitat partitioning by size, sex, and molt stage of blue crabs Callinectes sapidus in a subestuary of central Chesapeake Bay. Mar. Ecol. Prog. Ser. 36, 55–64. doi: 10.3354/meps036055
Karatayev, A. Y., Burlakova, L. E., Padilla, D. K., Mastitsky, S. E., and Olenin, S. (2009). Invaders are not a random selection of species. Biol. Invasions 11, 2009–2019. doi: 10.1007/s10530-009-9498-0
Kiryu, Y., Blazer, V. S., Vogelbein, W. K., Kator, H., and Shields, J. D. (2005). Factors influencing the sporulation and cystformation of Aphanomyces invadans, etiological agent of ulcerative mycosis in Atlantic menhaden. Brevoortia tyrannus. Mycologia 97, 569–575. doi: 10.1080/15572536.2006.1183278
Lowe, S., Browne, M., Boudjelas, S., and De Poorter, M. (2000). 100 of the world’s worst invasive alien species. A selection from the Global Invasive Species Database. Switzerland: The Invasive Species Specialist Group (ISSG) a specialist group of the Species Survival Commission (SSC) of the World Conservation Union (IUCN).
Makkonen, J., Jussila, J., Panteleit, J., Keller, N. S., Schrimpf, A., Theissinger, K., et al. (2018). MtDNA allow the sensitive detection and haplotyping of the crayfish plague disease agent Aphanomyces astaci showing clues about its origin and migration. Parasitology 145, 1210–1218. doi: 10.1017/S0031182018000227
Makkonen, J., Vesterbacka, A., Martin, F., Jussila, J., Dieguez-Uribeondo, J., Kortet, R., et al. (2016). Mitochondrial genomes and comparative genomics of Aphanomyces astaci and Aphanomyces invadans. Sci. Rep. 6:36089. doi: 10.1038/srep36089
Martín, M., Hernández-Crespo, C., Andrés-Doménech, I., and Benedito-Durá, V. (2020). Fifty years of eutrophication in the Albufera Lake (Valencia, Spain): causes, evolution and remediation strategies. Ecol. Eng. 155:105932. doi: 10.1016/j.ecoleng.2020.105932
Martín-Torrijos, L., Kokko, H., Makkonen, J., Jussila, J., and Diéguez-Uribeondo, J. (2019). Mapping 15 years of crayfish plague in the Iberian Peninsula: The impact of two invasive species on the endangered native crayfish. PloS One 14:e0219223. doi: 10.1371/journal.pone.0219223
Meineri, E., Rodriguez-Perez, H., Hilaire, S., and Mesleard, F. (2014). Distribution and reproduction of Procambarus clarkii in relation to water management, salinity and habitat type in the Camargue. Aquat. Conserv. 24, 312–323. doi: 10.1002/aqc.2410
Oficialdegui, F. J., Clavero, M., Sánchez, M. I., Green, A. J., Boyero, L., Michot, T. C., et al. (2019). Unravelling the global invasion routes of a worldwide invader, the red swamp crayfish (Procambarus clarkii). Freshw. Biol. 64, 1382–1400. doi: 10.1111/fwb.13312
Oidtmann, B., Geiger, S., Steinbauer, P., Culas, A., and Hoffmann, R. W. (2006). Detection of Aphanomyces astaci in North American crayfish by polymerase chain reaction. Dis. Aquat. Organ. 72, 53–64. doi: 10.3354/dao072053
Oidtmann, B., Schaefers, N., Cerenius, L., Söderhäll, K., and Hoffmann, R. W. (2004). Detection of genomic DNA of the crayfish plague fungus Aphanomyces astaci (Oomycete) in clinical samples by PCR. Vet. Microbiol. 100, 269–282. doi: 10.1016/j.vetmic.2004.01.019
Padgett, D. E. (1984). Evidence for extreme salinity tolerance in saprolegniaceous fungi (Oomycetes). Mycologia 76, 372–375. doi: 10.2307/3793118
Panteleit, J., Keller, N. S., Diéguez-Uribeondo, J., Makkonen, J., Martín-Torrijos, L., Patrulea, V., et al. (2018). Hidden sites in the distribution of the crayfish plague pathogen Aphanomyces astaci in Eastern Europe: Relicts of genetic groups from older outbreaks? J. Invertebr. Pathol. 157, 117–124. doi: 10.1016/j.jip.2018.05.006
Persson, M., and Söderhäll, K. (1986). CaCl2-Induced germination and peptidase secretion in Aphanomyces astaci. Exp. Mycol. 10, 205–213. doi: 10.1016/0147-5975(86)90005-8
Piscart, C., Kefford, B. J., and Beisel, J. N. (2011). Are salinity tolerances of non-native macroinvertebrates in France an indicator of potential for their translocation in a new area? Limnologica 41, 107–112. doi: 10.1016/j.limno.2010.09.002
Preuett, J. A., Collins, D. J., Luster, D. G., and Widmer, T. L. (2016). The effect of salinity on the survival, growth, sporulation and infection of Phytophthora ramorum. Fungal Ecol. 23, 123–130. doi: 10.1016/j.funeco.2016.08.002
Rantamäki, J., Cerenius, L., and Söderhäll, K. (1992). Prevention of transmission of the crayfish plague fungus (Aphanomyces astaci) to the freshwater crayfish Astacus astacus by treatment with MgCl2. Aquaculture 104, 11–18. doi: 10.1016/0044-8486(92)90133-6
Rezinciuc, S., Gallindo, J., Montserrat, J., and Diéguez-Uribeondo, J. (2014). AFLP-PCR and RAPD-PCR evidences of the transmission of the pathogen Aphanomyces astaci (Oomycetes) to wild populations of European crayfish from the invasive crayfish species. Procambarus clarkii. Fungal Biol. 118, 612–620. doi: 10.1016/j.funbio.2013.10.007
Rezinciuc, S., Sandoval-Sierra, J. V., Oidtmann, B., and Diéguez-Uribeondo, J. (2015). “The biology of crayfish plague pathogen Aphanomyces astaci. Current answers to most frequent questions,” in Freshwater Crayfish: A Global Overview, eds T. Kawai, Z. Faulkes, and G. Scholtz, (Boca Raton, FL: CRC Press), 182–204. doi: 10.1201/b18723-12
Rodríguez, C. F., Bécares, E., Fernández-Aláez, M., and Fernández-Aláez, C. (2005). Loss of diversity and degradation of wetlands as a result of introducing exotic crayfish. Biolog. Invasions 7, 75–85. doi: 10.1007/1-4020-3870-4_7
Scalici, M., Chiesa, S., Scuderi, S., Celauro, D., and Gibertini, G. (2010). Population structure and dynamics of Procambarus clarkii (Girard, 1852) in a Mediterranean brackish wetland (Central Italy). Biol. Invasions 12, 1415–1425. doi: 10.1007/s10530-009-9557-6
Schrimpf, A., Pârvulescu, L., Copilaş-Ciocianu, D., Petrusek, A., and Schulz, R. (2012). Crayfish plague pathogen detected in the Danube Delta-A potential threat to freshwater biodiversity in Southeastern Europe. Aquat. Invasions 7, 503–510. doi: 10.3391/ai.2012.7.4.007
Schrimpf, A., Schmidt, T., and Schulz, R. (2014). Invasive Chinese mitten crab (Eriocheir sinensis) transmits crayfish plague pathogen (Aphanomyces astaci). Aquat. Invasions 9, 203–209. doi: 10.3391/ai.2014.9.2.09
Simberloff, D., and Rejmánek, M. (2011). Encyclopedia of Biological Invasions. Berkeley, CA: University of California Press.
Sousa, R., Freitas, F. E. P., Mota, M., Nogueira, A. J. A., and Antunes, C. (2013). Invasive dynamics of the crayfish Procambarus clarkii (Girard, 1852) in the international section of the river Minho (NW of the Iberian Peninsula). Aquat. Conserv. 23, 656–666. doi: 10.1002/aqc.2323
Souty-Grosset, C., Anastácio, P. M., Aquiloni, L., Banha, F., Choquer, J., Chucholl, C., et al. (2016). The red swamp crayfish Procambarus clarkii in Europe: Impacts on aquatic ecosystems and human well-being. Limnologica 58, 78–93. doi: 10.1016/j.limno.2016.03.003
Svoboda, J., Strand, D. A., Vrålstad, T., Grandjean, F., Edsman, L., Kozák, P., et al. (2014). The crayfish plague pathogen can infect freshwater-inhabiting crabs. Freshw. Biol. 59, 918–929. doi: 10.1111/fwb.12315
Tollington, S., Turbé, A., Rabitsch, W., Groombridge, J. J., Scalera, R., Essl, F., et al. (2015). Making the EU legislation on invasive species a conservation success. Conserv. Lett. 10, 112–120. doi: 10.1111/conl.12214
Unestam, T. (1969a). On the adaptation of Aphanomyces astaci as a parasite. Physiol. Plant. 22, 221–235. doi: 10.1111/j.1399-3054.1969.tb07371.x
Unestam, T. (1969b). On the physiology of zoospore production in Aphanomyces astaci. Physiol. Plant. 22, 236–246. doi: 10.1111/j.1399-3054.1969.tb07372.x
Vodovsky, N., Patoka, J., and Kouba, A. (2017). Ecosystem of Caspian Sea threatened by pet-traded non-indigenous crayfish. Biol. Invasions 19, 2207–2217. doi: 10.1007/s10530-017-1433-1
Keywords: salinity, oomycetes, pathogen, crayfish plague, estuarine habitat, biological invasions, crayfish
Citation: Martín-Torrijos L, Correa-Villalona AJ, Pradillo A and Diéguez-Uribeondo J (2021) Coexistence of Two Invasive Species, Procambarus clarkii and Aphanomyces astaci, in Brackish Waters of a Mediterranean Coastal Lagoon. Front. Ecol. Evol. 8:622434. doi: 10.3389/fevo.2020.622434
Received: 28 October 2020; Accepted: 14 December 2020;
Published: 11 January 2021.
Edited by:
Hideyuki Doi, University of Hyogo, JapanReviewed by:
Adam Petrusek, Charles University, CzechiaCopyright © 2021 Martín-Torrijos, Correa-Villalona, Pradillo and Diéguez-Uribeondo. This is an open-access article distributed under the terms of the Creative Commons Attribution License (CC BY). The use, distribution or reproduction in other forums is permitted, provided the original author(s) and the copyright owner(s) are credited and that the original publication in this journal is cited, in accordance with accepted academic practice. No use, distribution or reproduction is permitted which does not comply with these terms.
*Correspondence: Laura Martín-Torrijos, bG10b3JyaWpvc0ByamIuY3NpYy5lcw==
Disclaimer: All claims expressed in this article are solely those of the authors and do not necessarily represent those of their affiliated organizations, or those of the publisher, the editors and the reviewers. Any product that may be evaluated in this article or claim that may be made by its manufacturer is not guaranteed or endorsed by the publisher.
Research integrity at Frontiers
Learn more about the work of our research integrity team to safeguard the quality of each article we publish.