- Aquatic Conservation and Ecology Team, Center for Bottomland Hardwoods Research, Southern Research Station, United States Department of Agriculture (USDA) Forest Service, Oxford, MS, United States
Dams are among the most prevalent and extreme alterations humans have perpetrated on fluvial systems. The dramatic physical and biological changes caused by dams have been synthesized for many aquatic faunal groups, but not for crayfishes. In addition, invasive crayfish species are an increasing threat to global biodiversity, and dams have both costs and benefits with respect to crayfish invasions. North American crayfishes have imperiled native crayfishes in Europe, largely by hosting and spreading the crayfish plague pathogen Aphanomyces astaci that is lethal to European crayfishes. The differential effects of A. astaci on North American vs. European crayfishes contribute to differences between the continents in the costs and benefits of dams. We reviewed literature on both the detrimental and beneficial effects of dams on crayfishes, with emphasis on conservation of European crayfishes. We also suggested additional potential dam effects that warrant investigation. Our review illustrates the challenges and opportunities dams create for crayfish conservation. Dams create detrimental effects to native crayfishes, including reducing suitable habitats necessary for native habitat-specialist species and creating habitats suitable for non-native habitat-generalist species; fragmenting crayfish populations; and reducing species' ability to recolonize upstream habitats. Conversely, dams can have beneficial effects by creating barriers that slow or halt upstream invasions by non-native crayfishes and spread of the crayfish plague. The complexity of the issues and the limited ecological information available highlights the need for future studies on the effects of dams on crayfishes. Crayfishes are one of the most imperiled groups of aquatic fauna globally; therefore, understanding the beneficial and detrimental effects of dams is essential for effective conservation of many crayfish species.
Introduction/Scope
Flowing water is the defining characteristic of fluvial systems, with flow influencing hydrologic structure, geomorphology, temperature regime, nutrient cycling, and the distribution and evolution of biota (e.g., Poff et al., 1997). Dams can control river flows, dramatically changing streams and creating new ecosystems (Baxter, 1977) and are among the most prevalent and extreme alterations humans have perpetrated on fluvial systems (The Heinz Center, 2002; Liermann et al., 2012; Grill et al., 2015). With dams on 59% of rivers globally and planned on another 16% (Grill et al., 2015), they have drastically altered rivers on every continent except Antarctica. Impacts range from physical alterations of stream channels and water quality to effects on most biological kingdoms.
The largest biological effects of dams often result from river fragmentation and flow regulation (Stanford and Ward, 2001; Grill et al., 2015). As of 2010, an estimated 35% of the world's river volume was heavily to severely impacted by fragmentation; 35% impacted by flow regulation; and <10% unaffected by either (Grill et al., 2015). Dams nearly always create discontinuities in the river continuum and alter aquatic communities, reducing “natural” biodiversity and facilitating invasions by non-native organisms (Stanford and Ward, 2001). They also dramatically alter stream physiochemical properties, including flow and temperature regimes, channel geomorphology, and water chemistry (Baxter, 1977) that all influence riverine communities (Stanford and Ward, 2001; Grill et al., 2015; Hanks and Hartman, 2019).
Dams are built for various purposes (e.g., water storage, flood control, navigation, hydropower, recreation) that dictate their modes of operation, with irrigation the primary purpose of an estimated 50% of the world's dams (Mulligan et al., 2020). A dam's purpose and operations, as well as its geomorphic, climatic, and biotic settings, all powerfully influence how it affects faunal communities (Stanford and Ward, 2001; The Heinz Center, 2002). For example, water storage dams in mountainous settings typically impound deep, high-volume reservoirs with long water residence times. Dams in such systems usually create insurmountable upstream migration barriers to lotic fauna, and the large reservoirs they impound may further inhibit both up- and downstream migrations. Conversely, reservoirs can also facilitate upstream invasion by flooding natural barriers (Júnior et al., 2009). Unlike water storage dams, run-of-the-river hydropower or navigation dams may impound little or no water and have negligible effects on water residence times, but instead may facilitate upstream passage and expansion of some species (Taugbøl et al., 1993; Kim and Mandrak, 2016). This is especially true of dams with locks.
As of 2019, European rivers had more than 21,000 hydropower dams, with another 8,507 planned and 278 under construction (Schwarz, 2019). Of the existing hydropower dams, 19,344 were “small,” producing 0.1–<10.0 megawatts (MW) of power, and 686 were “large” (≥50 MW). These totals excluded the considerable number of non-hydropower dams. In the Iberian Peninsula, for example, most dams are managed for irrigation or water abstraction (Schwarz, 2019). The US National Inventory of Dams (NID) included 91,457 dams, including 6,346 dams >15 m high (https://nid.sec.usace.army.mil/ords/f?p=105:113:15689164071610::NO:::, accessed 25 June 2020). The primary purposes of inventoried dams included recreation (32%), flood control (19%), irrigation (9%), and hydropower (2%). Small dams are much more abundant than large dams but are poorly quantified (Liermann et al., 2012; Grill et al., 2015; Schwarz, 2019), even though “the cumulative effects of many small dams can differ from and even exceed those of a single large dam” (Liermann et al., 2012).
The dramatic physical and biological changes caused by dams have been synthesized for many aquatic faunal groups, but not for crayfishes. Crayfishes are one of the most imperiled groups of aquatic fauna globally (Richman et al., 2015), so understanding how dams affect them is important for conservation. Dams and water management threaten an estimated 25% of at-risk crayfishes in Australia, 35% in the US, and 70% in Mexico (Richman et al., 2015). In Europe, the major threat to native crayfish persistence is the crayfish plague, caused by the oomycete Aphanomyces astaci, native to North America (Diéguez-Uribeondo, 2010; Füreder, 2016). North American crayfishes generally carry the pathogen but are relatively resistant to the crayfish plague. Their introduction to other continents also introduced the plague, which is often more devastating to the native crayfish fauna than the introduced crayfishes themselves (Reynolds, 1988; Taugbøl et al., 1993; Kozubíková et al., 2008). Due to the overwhelming impact of the crayfish plague, habitat modifications are of only intermediate concern to crayfish conservation in much of Europe (Füreder, 2016). This creates a scenario in which the costs and benefits of dams to crayfish populations may differ between the continents.
We synthesized literature on the effects of dams on crayfishes. Because the literature exploring this topic is limited, we first briefly summarized general effects of dams on riverine ecosystems and on other aquatic faunal groups that are more thoroughly studied. We then summarized effects of dams on crayfishes in non-European continents, followed by effects in Europe, including some benefits of dams for conservation of European crayfishes (Table 1). Finally, we addressed several additional effects that dams are likely to have on crayfishes, based on what is known about other faunal groups. Finally, we suggested additional dam-related research that may facilitate crayfish conservation.
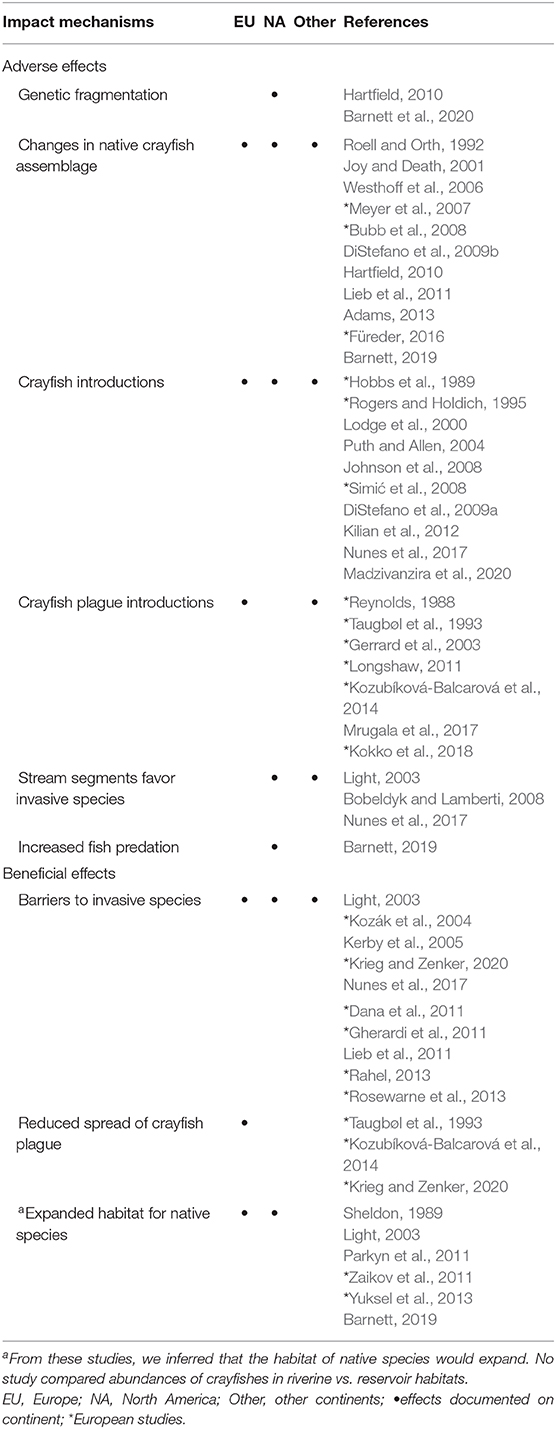
Table 1. Summary of documented effects of dams on crayfishes in Europe, North America, and other continents.
General Ecosystem Effects of Dams
Dam effects on rivers and biota differ fundamentally in three longitudinal zones: the reservoir (area converted from lotic to lentic habitat) impounded by the dam, the river segment(s) upstream of the reservoir, and the river segment(s) downstream of the dam. The spatial extent and degree of impacts in each zone vary according to geomorphology, dam height, and dam operation and often vary temporally as well. Because dam effects on riverine physiochemical properties and biological communities are synthesized elsewhere (e.g., Poff and Zimmerman, 2010; Ellis and Jones, 2013), we present only a brief synopsis of the main physiological and biotic impacts of dams.
Impacts in Reservoirs
Within a reservoir, a river's natural current velocity is greatly reduced, changing a lotic environment to a lentic one and often inundating areas that were previously floodplain or terrestrial habitats (Baxter, 1977). Thus, species adapted to lentic conditions dominate reservoirs, resulting in fewer native, habitat-specialist species and more non-native, habitat-generalist species (Johnson et al., 2008; Kanno and Vokoun, 2010; Santos et al., 2017). However, even native species tolerant of lentic conditions may have to adapt (e.g., morphologically) to new conditions in order to survive (Baxter, 1977; Haas et al., 2010). Decreased velocities in reservoirs also cause fine sediment accumulations, especially where undammed tributaries are present (Ward, 1976; Maneux et al., 2001; Schleiss et al., 2016). Fine sediments can eliminate gravel-associated species, such as some Unionid mussels and gravel-spawning salmonid fishes that require unembedded substrates for reproduction (Bates, 1962; Magilligan et al., 2016). Fine sediments can also cover and suffocate mussels or reduce their filter-feeding efficiencies (Vaughn and Taylor, 1999; McAllister et al., 2001).
The amount of water impounded differs greatly among dams (Baxter, 1977), impacting the physiochemical characteristics of the water impounded. For a run-of-the-river dam, where flow is minimally regulated, the entire water column of the reservoir may remain well-mixed thermally due to water flows throughout the reservoir and relatively shallow depths, leading to isothermal and orthograde temperature and oxygen profiles, as well as nutrient concentrations uniformly distributed throughout the water column (Worth, 1995). In storage reservoirs, where water residence times may be months or years (Maavara et al., 2014), inflows may be turbulent, and well-mixed, whereas water is thermally stratified throughout much of the reservoir. In summer, oxygen and temperature levels are highest in the epilimnion (upper layer) and lowest in the hypolimnion (bottom layer) (Cassidy, 1989). Furthermore, water storage and retention times influence reservoir temperature regimes, water quality, and other physiochemical and ecological processes (Winton et al., 2019). Changes to these processes often degrade habitats for native, riverine species and lead to communities more tolerant of anthropogenic perturbation, decreasing or eliminating native species intolerant of degraded conditions (Havel et al., 2005).
When rivers are dammed, recreational access and water-based activities often increase, which increases species introductions (Roell and Orth, 1992; Johnson et al., 2008; Adams et al., 2015). Non-native species often abound in reservoirs, due to intentional (legal or illegal) and unintentional introductions and to “the young age, increased niche availability, and high disturbance regime characteristic of most impoundments” (Johnson et al., 2008). Unintentional species introductions may occur by many means, including inadvertent transport on boats, fishing gear, or other recreational equipment (Rothlisberger et al., 2010; Cole et al., 2019) or with shipments of intentionally stocked fishes (Davies et al., 2013).
Impacts Upstream of Reservoirs
The primary physical changes to river segments upstream of reservoirs are the reduced water velocity and increased sedimentation (Graf, 2005; Hu et al., 2009). As water velocities slow, sediment deposition occurs in the river bed, river margins, and interstitial spaces, increasing water levels, flooding events, and channel migration (Baxter, 1977; Wood and Petts, 1994; Graf, 2005; Hu et al., 2009; Schleiss et al., 2016). Decreased interstitial spaces reduces shelter available to lotic species, which subsequently decreases growth and survival (Finstad et al., 2007; Magilligan et al., 2016). As in reservoirs, increased sediment deposition upstream may eliminate gravel-associated species (Bates, 1962; Magilligan et al., 2016). Sedimentation also increases the amount of invertebrates entering the drift, reducing the standing stock of benthic invertebrates and changing the abundance and composition of the remaining invertebrate community upstream of reservoirs (Jones et al., 2012). Thus, increased sedimentation and the loss of interstitial spaces can greatly reduce the abundance, reproduction, growth, and survival of numerous riverine taxa directly upstream of reservoirs. River segments upstream of reservoirs are also impacted by reservoir taxa (e.g., stocked or introduced fishes) that move into upstream river segments (Swink and Jacobs, 1983; Herbert and Gelwick, 2003; Hladík and Kubečka, 2003). These effects diminish with distance upstream of reservoirs (Ellis and Jones, 2013).
Dams and reservoirs create physical and behavioral barriers to animal movements through river networks, interrupting longitudinal connectivity and isolating upstream populations (Falke and Gido, 2006; Branco et al., 2012; Crook et al., 2015). Such river fragmentation alters patterns of ecological connectivity, potentially impacting life history strategies and habitat colonization (Pringle, 2000; Crook et al., 2015), leading to local extinction of migratory organisms (Gehrke et al., 2002; Hall et al., 2011; Liermann et al., 2012), and reducing abundances of non-migratory, upstream populations (Winston et al., 1991; Morita and Yamamoto, 2002). Inhibiting movements through river systems can also reduce gene flow among populations, decreasing population genetic diversity and population fitness (Lande and Barrowclough, 1987; Fullerton et al., 2010; Fluker et al., 2014). Because dams have fragmented riverine systems only recently relative to evolutionary timescales, the full extent of their effects on species may ultimately be much greater than currently estimated, due to time lags in population responses (Richmond et al., 2009; Abernathy et al., 2013).
Impacts Downstream of Dams
Dams and associated reservoirs can profoundly alter natural flow regimes downstream, causing subsequent changes in fauna composition (Baxter, 1977; Watters, 1996; Cumming, 2004; Graf, 2006). Some dams (e.g., hydroelectric dams) create a rapidly fluctuating downstream hydrologic regime, matching discharges to hourly water or power demands (Richter and Thomas, 2007). Such dam operation often results in a low abundance and low diversity of species downstream (Ward, 1976; Armitage, 1978; Baumgartner et al., 2020). Conversely, some dams only release high volumes of water during certain seasons, with minimum water discharged during other seasons (Graf, 2006). These dam operations lead to a high abundance, but low diversity of species (Ward, 1976; Armitage, 1978; Baumgartner et al., 2020). To meet management needs, dam managers may also stop the release of water from reservoirs or divert water from the river, completely dewatering long river segments downstream (Pringle, 2000; Perkin et al., 2015). Dry segments downstream of dams often eliminate most lotic species, especially those that are not adapted to harsh, unstable physical conditions (Anderson et al., 2006). Additionally, dams prevent movement and migration of fauna through riverine systems, which can lead to the reduced abundance or local extinction of native species downstream (Gehrke et al., 2002; Liermann et al., 2012).
Dams typically alter downstream temperature regimes, water quality, and physiochemical and ecological processes, and dams with long water-retention times often cause the greatest changes (Baxter, 1977; Watters, 1996; Maneux et al., 2001; Cumming, 2004; Maavara et al., 2014). In thermally stratified reservoirs, the seasonal timing and depth (hypolimnetic vs. epilimnetic) of water releases influence downstream water quality (Hanks and Hartman, 2019). Water released downstream from reservoirs can cool water in the summer and warm it in the winter, as well as alter oxygen and nutrient loads relative to natural levels, causing subsequent changes in downstream fauna (Baxter, 1977; Lessard and Hayes, 2003; Graf, 2005; Kunz et al., 2011; Mejia et al., 2020). Nonetheless, with distance downstream from dams and input from downstream tributaries, water conditions (e.g., thermal regime, nutrient content) often gradually returns to a more natural state (Mejia et al., 2020). Species community structure also reverts toward its pre-impoundment state with distance downstream from dams (Hanks and Hartman, 2019). Recovery distance varies by taxa and species (Voelz and Ward, 1991; Camargo and Voelz, 1998; McGregor and Garner, 2003; Phillips and Johnston, 2004b; Hanks and Hartman, 2019), with hundreds of kilometers needed for the recovery of some rare species (Vaughn and Taylor, 1999).
The reduced sediment loads carried by water released from storage reservoirs alter channel morphology downstream of dams. Reduced sedimentation creates coarser riverbeds and greater channel erosion, increasing channel incision (i.e., lowering of bed level) and width (Baxter, 1977; Chien, 1985; Wood and Petts, 1994; Gordon et al., 2004; Graf, 2005; Simon and Rinaldi, 2006). Channel incision can lower local water tables, affecting river flows and riparian conditions (Scott et al., 2000; Schilling et al., 2004). The loss of fine sediments creates riverbeds armored with large rocks and boulders (Graf, 2005) and reduces the geomorphic complexity of downstream segments, resulting in fewer sand/gravel bars and shallow-water habitats compared to unregulated rivers (Poff et al., 1997; Graf, 2006). These geomorphic changes can propagate hundreds of kilometers downstream of dams (Graf, 2006). Native species that are poorly adapted to the altered habitat conditions are decreased or eliminated, often with non-native species filling the new niches (Caiola et al., 2014).
Adverse Effects of Dams on Crayfishes
Genetic Fragmentation of Crayfish Populations
Dams and reservoirs can restrict crayfish dispersal and gene flow, genetically fragmenting populations, as demonstrated in the southern Appalachian Mountains, USA (Hartfield, 2010; Barnett et al., 2020). An older, low-head dam (>160 years, <8 m high) and three newer, large dams (36–104 years, >15 m high) reduced passage of crayfishes (Cambarus sp. and Faxonius spp.), genetically fragmenting populations (Hartfield, 2010; Barnett et al., 2020).
In impounded Alabama, USA, streams, crayfish populations shared few mitochondrial DNA (mtDNA) cytochrome oxidase subunit I gene haplotypes between segments up- and downstream of dams (Hartfield, 2010; Barnett et al., 2020), but in unimpounded streams most haplotypes were shared between up- and downstream segments (Barnett et al., 2020). Also, crayfish gene flow in most impounded streams, was one-way, downstream past dams, whereas in unimpounded streams, it was bidirectional (Barnett et al., 2020). Barnett et al. (2020) inferred that time since dam closure, reservoir size, as well as dispersal abilities and ecological tolerances of the species studied impacted the magnitude of genetic differences between impounded and unimpounded streams. For example, no gene flow occurred between up- and downstream populations in the stream with the oldest dam; only downstream gene flow occurred between populations in the largest, young dam; and both up- and downstream gene flow occurred for one species in the smallest, young dam (Barnett et al., 2020). Additionally, upstream gene flow in impounded streams was detected only for Faxonius validus (powerful crayfish), a species preferring small to medium streams; conversely, F. erichsonianus (reticulate crayfish), a species preferring medium to large streams, did not display upstream gene flow in impounded streams (Barnett et al., 2020).
One-way downstream migration isolates and reduces gene flow to upstream populations (Fagan, 2002; Fuller et al., 2015; Barnett et al., 2020). Nevertheless, the genetic diversity and effective population size of crayfish populations upstream of impoundments remained high in both impounded and unimpounded streams (Hartfield, 2010; Barnett et al., 2020), suggesting that overall loss of genetic diversity in impounded streams may be a slow process.
Genetic signatures of crayfish population fragmentation by dams in place for only a few decades suggest that substantial genetic changes are ongoing (Liermann et al., 2012; Barnett et al., 2020). Because dam disruption of gene flow in crayfishes was observed using a mtDNA marker that does not mutate at exceptionally fast rates (Hartfield, 2010; Fluker et al., 2014; Barnett et al., 2020), dams likely have had a more profound impact on crayfish population genetics than has been demonstrated to date (Lacy, 1987; Dixo et al., 2009; Hartfield, 2010; Barnett et al., 2020). The effects of genetic fragmentation caused by dams may be exacerbated in smaller crayfish populations and may heighten extinction risk of species with small natural ranges (Lodge et al., 2000; Richman et al., 2015). Although extirpation or extinction caused by dams has not been documented for crayfishes, to our knowledge, the relatively young ages of many dams makes the presence of an extinction debt likely (Tilman et al., 1994; Liermann et al., 2012). Moreover, the historic data necessary to document such extirpations is extremely limited in North America.
Changes in Native Crayfish Assemblages
Changes in resources and habitat availability can influence crayfish presence and abundance (Ellis and Jones, 2013). Shifts in relative abundances of crayfishes have been documented in streams with both small (<20 ha) and large (> 400 ha) reservoirs (Joy and Death, 2001; Westhoff et al., 2006; DiStefano et al., 2009b; Hartfield, 2010; Adams, 2013; Barnett, 2019).
Small dams altered crayfish species assemblages up- and downstream of reservoirs relative to unimpounded streams (Joy and Death, 2001; Adams, 2013). In the New Zealand Taranaki Ring Plain, the abundance of Paranephrops planifrons (northern koura), the only crayfish species collected in the region, was higher upstream of reservoirs than in unimpounded streams (Joy and Death, 2001). In Mississippi, the catch-per-unit-effort (CPUE) of F. etnieri spp. complex [Orconectes chickasawae in Adams (2013)] was lower downstream of small dams than in unimpounded streams, whereas CPUE of Procambarus spp. were either higher downstream of dams or similar between impounded and unimpounded streams (Adams, 2013). Presumably as a result of differing assemblage compositions, seasonal changes in crayfish CPUE also trended in opposite directions downstream of dams vs. in unimpounded streams (Adams, 2013). Impounded streams had higher abundances of species that were better adapted to the newly formed habitats and flow regimes (Adams, 2013).
In Alabama, crayfish density was higher in unimpounded streams than up- and downstream of large impoundments (Barnett, 2019). In unimpounded relative to impounded streams, juvenile density tended to be higher upstream and adult density higher downstream (Barnett, 2019). Higher juvenile and adult crayfish densities in unimpounded than impounded streams were correlated with more aquatic vegetation, lower top predator fish biomass, higher turbidities, higher discharge, and lower minimum temperatures (Barnett, 2019).
Impoundments reset the natural river continuum for physical and biotic variables (Ward and Stanford, 1983), interrupting longitudinal changes in assemblage structure. In Alabama, sites directly below impoundments shared similar physical and biotic characteristics with sites in headwaters, modifying habitat conditions that historically occurred downstream (Barnett, 2019). The gradual up- to downstream shifts in species composition and dominant species in unimpounded streams were not observed in impounded streams (Barnett, 2019). Instead, one or two crayfish species dominated all sites in impounded streams, with low densities of other species (Barnett, 2019). Some species [e.g., Cambarus striatus (ambiguous crayfish), F. compressus (slender crayfish)] that were common in unimpounded streams were rarely encountered in impounded streams (Adams et al., 2015; Barnett, 2019). Crayfish density, richness, and evenness increased with distance downstream of dams, indicating assemblage recovery (Barnett, 2019). Similarly, in a West Virginia river, crayfish density 15 km downstream from a dam was higher compared to just downstream of it, although no statistical comparison was made (Roell and Orth, 1992). Thus, it appears that crayfish communities, like those of fishes, mussels, and insects recover to some degree with distance downstream of dams, as tributaries enter and river conditions return to a more natural state (Voelz and Ward, 1991; Kinsolving and Bain, 1993; Camargo and Voelz, 1998; McGregor and Garner, 2003; Phillips and Johnston, 2004b). However, regardless of impoundment status, crayfish richness tends to increase with distance upstream unlike fishes (Hicks, 2003).
Impoundments have greater impacts on crayfish populations in and downstream of reservoirs than upstream of reservoirs. In Alabama streams, total crayfish CPUE was higher upstream of small reservoirs than directly below them (Hartfield, 2010). Similarly, in Pennsylvania, USA, most populations of an extremely rare crayfish (Cambarus sp.) occurred upstream of reservoirs, with the invasive F. rusticus (rusty crayfish) often abundant in and downstream of reservoirs (Lieb et al., 2011). In Missouri, USA, repeated summer trapping upstream of a reservoir produced the common crayfish F. neglectus (ringed crayfish) and two imperiled species, F. williamsi (Williams' crayfish) and F. meeki meeki (Meek's crayfish), whereas trapping within the reservoir produced the common F. neglectus and F. longidigitus (long-pincered crayfish) and only one individual of an imperiled species (DiStefano et al., 2009b). Reservoirs reduced the amount of suitable stream habitat for F. williamsi, reducing population sizes and isolating upstream populations, although populations may have been isolated even before impoundment (Westhoff et al., 2006).
Recurring themes in papers documenting changes to crayfish abundances in impounded streams were changes to stream habitats caused by dams (Joy and Death, 2001; DiStefano et al., 2009b; Adams, 2013; Barnett, 2019). These changes to stream habitats may decrease the availability of habitat types necessary for survival of some crayfish species, causing a subsequent decrease in the abundance of these crayfishes in impounded stream systems.
Crayfish Introductions to Reservoirs
Crayfishes are one of the most commonly introduced freshwater organisms because the wide niches of some crayfish species allow them to survive in diverse ecosystems (Hobbs et al., 1989; Lodge et al., 1998; Zeng et al., 2015). Unlike many aquatic species, some crayfishes can survive out of water for relatively long periods of time (Banha and Anastácio, 2014), making them more likely than many other aquatic taxa to survive unintentional translocations and overland dispersal and facilitating extensive secondary spread after initial introductions (Krieg and Zenker, 2020).
Human-mediated introductions of non-native crayfishes—and their associated diseases—tend to increase after damming, due to greater human access to and use of water bodies (Muirhead and Macisaac, 2005). For example, in a region rich with natural lakes, five aquatic invasive species, including the F. rusticus were 2.5 to 300 times more likely to occur in reservoirs than in natural lakes (Johnson et al., 2008). Non-native crayfishes are commonly introduced to reservoirs through intentional (legal or illegal; often to create new crayfisheries) or unintentional pathways (Lodge et al., 2000; Krieg and Zenker, 2020; Madzivanzira et al., 2020). Introductions of non-native crayfishes potentially also add crayfish commensals and pathogens (Longshaw, 2011; Mrugala et al., 2017; Madzivanzira et al., 2020), most notably the crayfish plague pathogen, to ecosystems (Souty-Grosset et al., 2006). Reservoir fisheries create at least three possible vectors for crayfish introductions: introduction with stocked fishes (Simić et al., 2008), introduction for fish forage (Madzivanzira et al., 2020), and release or escape of bait (DiStefano et al., 2009a). Several countries and US states have banned the transport and possession of live crayfishes as bait, but crayfish introduction through use as bait is still a concern in many places (Puth and Allen, 2004; DiStefano et al., 2009a; Kilian et al., 2012; Banha and Anastácio, 2015). Crayfishes are also introduced to reservoirs through the release or escape of aquarium, classroom, or research crayfishes (Rogers and Holdich, 1995; Lodge et al., 2000; Madzivanzira et al., 2020). Many characteristics of crayfishes sold in the aquarium trade include characteristics that promote invasion success, such as the ability to reproduce under warm aquarium conditions and a preference for lentic habitats (Chucholl and Wendler, 2017).
River Segments Hydrologically Altered by Dams Favor Invasive Species
Flow regimes are critical in structuring fluvial biotic communities (Poff and Ward, 1989; Power et al., 2008; Matthews, 2012). Native crayfishes have evolved traits and life history strategies favoring persistence under natural flow regimes that, in some systems, include extreme hydrological events (Flinders and Magoulick, 2003; Lynch et al., 2019). The numerous changes that dams cause in flow, often create environments that native, habitat-specialist species are not adapted to—or that favor native generalist or non-native species—thereby decreasing the abundance and diversity of native species and increasing biological homogenization (Bunn and Arthington, 2002; Rahel, 2002; Light, 2003; Bobeldyk and Lamberti, 2008; Nunes et al., 2017). Many invasive crayfishes have very wide physiological tolerances, flexible behaviors, high dispersal abilities, and high phenotypic plasticity allowing them to establish in the new habitats created by dams (Perry et al., 2013; Crook et al., 2015; Zeng et al., 2015). Under natural flow regimes, extreme flows can prevent, or even reverse, the establishment of invasive crayfishes (Light, 2003); however, impounded streams with more stable flow regimes (e.g., decreases in annual flow variation), sometimes allow invasive species to thrive, displacing native species through competition and predation (Rahel, 2002).
Crayfish mating, spawning, foraging, and growth are linked to flow regimes (Lowery, 1988; Mead, 2008; Barnett, 2017). Thus, changes in flow regimes can facilitate non-native crayfish invasions. In the Ontonagon River, Michigan, USA, invasive F. rusticus were abundant in stream segments closer to impoundments, where the flow regime was more stable, compared to segments further downstream (Bobeldyk and Lamberti, 2008). Similarly, in South Africa, invasive Australian crayfish Cherax quadricarinatus (Australian redclaw crayfish) were more abundant in river systems with irrigation dams than in less regulated rivers (Nunes et al., 2017). Nunes et al. (2017) inferred that less suitable habitats and higher flow velocities decreased the abundance of C. quadricarinatus in less regulated rivers. In the Lake Tahoe Basin, California, USA, invasive Pacifastacus leniusculus (signal crayfish) was present only in impounded streams (Light, 2003). Pacifastacus leniusculus was positively associated with proximity to reservoirs (both up and downstream), declining significantly in abundance upstream of reservoirs following the resumption of normal or high wet-season flows (Light, 2003). In addition, P. leniusculus moved long distances (up to 120 m/day) and occupied unimpounded streams only sporadically, leading Light (2003) to infer that they migrated into unimpounded tributaries during low-flow seasons to exploit highly productive habitats or escape fish predation but returned to the hydrologically stable reservoir before high flow seasons began. Thus, dams in the basin protected downstream reaches from high flows and allowed repeated recolonization of upstream reaches—from source populations in the reservoirs—after high-flow events (Light, 2003). Furthermore, reservoirs appear to function as invasion hubs for invasive crayfishes, by harboring source populations of invaders and increasing pathways for range expansion (e.g., irrigation canals connected to dams) that facilitate the subsequent spread and establishment of invaders into natural waterbodies (Light, 2003; Muirhead and Macisaac, 2005; Johnson et al., 2008; Nunes et al., 2017).
Increased Fish Predation on Crayfishes
Predatory fishes are often more abundant in impounded streams due to more favorable habitat conditions and fish stocking (Taylor et al., 2001; Phillips and Johnston, 2004a). Pringle (1997) noted that “until the last few decades, dams and reservoirs in the western US were often viewed as opportunities to introduce game fishes.” Because >40% of the diets of many game fish species (e.g., basses and catfishes) consists of crayfishes (Dorn and Mittelbach, 1999), increases in game fishes potentially increases predation pressure on crayfishes in reservoirs and the rivers that connect to them (Westhoff et al., 2006). Fishes introduced to reservoirs can impact entire stream systems, with the ability to freely leave the reservoirs and move to river segments upstream (Ruhr, 1956; Winston et al., 1991; Pringle, 1997), as well as potentially dispersing downstream of dams. For example, top crayfish predators (e.g., Micropterus spp.) were more abundant in impounded than unimpounded Alabama streams, and in impounded streams, top predator fish density was inversely correlated with crayfish density (Barnett, 2019).
Smaller crayfishes are more susceptible to predation than larger crayfishes. Larger crayfishes secure and retain shelter better than smaller individuals (Rabeni, 1985; Nakata and Goshima, 2003) and are less susceptible to predation by gape-limited predators, such as fishes (Stein and Magnuson, 1976; Rahel and Stein, 1988). Additionally, habitats such as tree roots, used especially by small crayfishes for protection from predators (Bohl, 1987; Smith et al., 1996; Parkyn and Collier, 2004), may become inhospitable or inaccessible due to rapidly fluctuating stream flows downstream of dams. In Alabama, the mean size of adult crayfishes was smaller in unimpounded than impounded streams due to higher densities of small-bodied species in the unimpounded streams (Barnett, 2019). The small species F. compressus, with an adult length 62% shorter than that of other species collected, occupied unimpounded, but not nearby impounded, streams. Adult crayfishes averaged 25% larger in impounded than unimpounded streams. Additionally, the density of top predator fishes was negatively correlated with juvenile crayfish density and positively correlated with adult crayfish size (Barnett, 2019).
Beneficial Effects of Dams on Crayfishes
Barriers to Invasive Species
Dams often block access to upstream river segments, thereby fragmenting populations and generally increasing conservation risks. However, in some circumstances, such movement barriers can facilitate conservation efforts by halting—or slowing—invasions by non-native species, including fishes, crayfishes, pathogens, and parasites (Ruhr, 1956; Liermann et al., 2012; Rahel, 2013; Füreder, 2016). Often, barriers may be the only means to prevent further invasion (Krieg and Zenker, 2020). In California, upstream movements by the invasive crayfish P. clarkii were stopped or greatly reduced by artificial and natural barriers, including dams, ranging from 1 to 3 m in height (Kerby et al., 2005). Interestingly, some barriers also deterred downstream movements. The presence of natural and artificial vertical barriers also appeared to stop or slow the spread of non-native P. leniusculus in Lake Tahoe Basin, California (Light, 2003). In South Africa, the upstream invasion by C. quadricarinatus in the Lomati River was apparently stopped by the Driekoppies Dam; however, an alternative explanation was that increased elevation or lower water temperatures hindered the invasion (Nunes et al., 2017). Dams can be so effective at blocking upstream animal movements that, in some cases, small dams or other artificial obstructions have been installed with the intent of blocking the upstream spread of non-native fishes, crayfishes, or diseases (Pringle, 1997; Gherardi et al., 2011; Rahel, 2013; Manfrin et al., 2019; Krieg and Zenker, 2020).
Barriers are often seen as the best method to stop the spread of invasive species (Krieg and Zenker, 2020); however, they are not wholly reliable. For example, in California, barriers were ineffective at stopping upstream invasion of P. leniusculus, but the barriers may have been breached during construction (Cowart et al., 2018). Factors influencing the effectiveness of barriers to crayfish movements include height, water velocity, angle, surface smoothness, bank characteristics (Kerby et al., 2005; Dana et al., 2011; Frings et al., 2013), and presumably, crayfish species is also important.
The tension between the conservation costs and benefits of dams can set the stage for competing conservation interests related to barrier construction or dam removals: on one hand, dams reduce population connectivity and access to habitat for native species, but on the other hand, dams may halt aquatic invasions (although as noted above, reservoirs may also increase chances of introductions). Benefits may accrue to one faunal group while costs are borne by a different group (Frings et al., 2013; Krieg and Zenker, 2020). Lieb et al. (2011) noted that neglecting to consider the role of dams in halting crayfish invasions may lead to further endangerment of rare crayfish species upstream of dams proposed for removal. They recommended (1) assessing invasion risks prior to dam removals, and (2) not removing dams located downstream of imperiled crayfishes in invasion-prone areas, even in the absence of non-natives. At the least, native crayfishes—as well as invasive and potentially invasive aquatic species—should be considered in assessments prior to dam removals. Maintaining dams to protect upstream populations from invasions may not always be an all-or-nothing choice. In some instances, dams may be retrofitted by lowering or other means so that their impacts on habitat and hydrology are reduced, while they still block invasions of certain species from downstream. Finally, barriers are likely to be less effective in low gradient habitats unless water velocities exceed the critical swimming speeds of the crayfishes (Frings et al., 2013). Most examples of dams as barriers to upstream crayfish movements have come from mountainous environments (e.g., Light, 2003; Kerby et al., 2005; Dana et al., 2011).
Expanded Habitat for Some Crayfish Species
Reservoirs can create new or expanded suitable habitat for crayfish species that are well-adapted to the reservoir's biophysical conditions. Certain crayfish species may thrive in such habitats. In Table Rock Reservoir, Missouri the native crayfish F. longidigitus, among the largest crayfishes in North America, supported a popular sport fishery (Parkyn et al., 2011). Likewise in Montana, USA, three reservoirs along the Clark Fork River supported commercial fisheries for P. leniusculus for several years, beginning in the late 1980's (Sheldon, 1989). The studies did not compare capture rates in rivers vs. reservoirs, but the bulk of harvest effort was concentrated in reservoirs. In Alabama, the dominant crayfish species, F. erichsonianus and F. validus, in lotic segments of impounded streams were also the most dominant species collected from guts of fishes in the reservoirs (Barnett, 2019). Louisiana, USA, is well-known in crayfish aquaculture circles for its high production rates of P. clarkii (red swamp crayfish), P. acutus (white river crayfish), and P. zonangulus (southern white river crayfish); in this slightly different context, crayfishes are produced in essentially very shallow reservoirs—with water pumped in from surface or groundwater sources—that are managed solely for the production of crayfishes, or of crayfishes and rice (Gillespie et al., 2012). Dams sometimes increase the habitat and resources available to crayfish species with broad niches that are adapted to lentic, or to both lentic and lotic, conditions (Light, 2003; Barnett, 2019).
Effects of Dams on Crayfishes in Europe
As elsewhere, dams appear to have both negative and positive effects on native crayfishes in Europe. Most European studies of dam effects on crayfishes examined dams as barriers to upstream dispersal of non-natives (Table 1), but we also apply the lessons from other continents to the European situation, being ever mindful that the differential effects of crayfish plague in Europe versus North America shift the balance of the costs and benefits of dams between the continents.
Negative Effects
All of the native crayfish species in Europe are adapted to both lotic and lentic waters (Reynolds and Souty-Grosset, 2011), and in general, the European crayfish fauna is better adapted to large lake ecosystems (Skurdal and Taugbøl, 2002) than are many crayfishes native to the southeastern US stream ecosystems, where several studies of dam effects on North American crayfishes were conducted (Hartfield, 2010; Adams, 2013; Barnett, 2019; Barnett et al., 2020). Reservoirs may not present the major loss of habitat to lacustrine species that they do to fluvial species, although the characteristics of reservoirs tend differ from those of lakes (Johnson et al., 2008). While reservoirs, themselves, may not create dispersal barriers to lacustrine-adapted species, dams often block upstream, and sometimes downstream, movements.
As elsewhere, European dams can imperil crayfishes by fragmenting stream systems. In an English stream, a flat-plate weir with a 25-cm drop during low flow blocked upstream, but not downstream, movements by Austropotamobius pallipes (white-clawed crayfish), illustrating that even small obstacles can fragment populations and should be assessed during conservation planning (Bubb et al., 2008). A population viability analysis on a German population of Astacus astacus (noble crayfish) provided a rare, population-level insight into conservation risks exacerbated by stream fragmentation (Meyer et al., 2007). Astacus astacus in a 400 m stream reach was bound by a dam upstream and a ford downstream (Meyer et al., 2007). The dam presented a complete barrier to upstream movements and the ford minimized downstream movements. The results suggested that the only path to long term population viability was increasing the population carrying capacity, achievable only by expanding available habitat or improving habitat quality. The most obvious solution was to remove the barriers to crayfish movements; however, such an action could increase risks of exposure to crayfish plague (see Positives below).
Other detrimental effects of European dams are likely to result from: water extraction facilitated by dams; alteration of downstream conditions (e.g., hydrology, temperature, water quality, channel morphology, and substrate); species introductions to reservoirs; or invasions facilitated by altered downstream conditions. For example, in some Mediterranean countries, water extraction is an important stressor, and in Latvia, damming, which is not regulated, dewaters streams to the detriment of some crayfish populations (Füreder, 2016). In Serbia, Astacus leptodactylus (narrow clawed crayfish), a European species with wide ecological tolerances, has been introduced to reservoirs through fish stocking (Simić et al., 2008). These unintentional introductions and the species' ability to outcompete other native species has allowed A. leptodactylus to replace A. astacus as the dominant crayfish in some river systems (Simić et al., 2008).
Healthy European crayfish populations tend to be associated with low levels of human activities such as fishing, swimming, and release of aquarium pets (Füreder, 2016), but human activities and access are often higher in reservoirs relative to rivers and even natural lakes (Roell and Orth, 1992; Johnson et al., 2008; Adams et al., 2015). To reduce the spread of invasive crayfishes and the crayfish plague, many European countries have banned the use of crayfishes as bait (Magnuson et al., 1975; DiStefano et al., 2009a; Peay, 2010) and the European Union has banned the sale, trade, transport, and release of five invasive, North American crayfishes (Regulation No 1143/2104, Commission Implementing Regulation 2016/1141). Given that crayfish plague is often introduced to water bodies via fishing gear, boats, rubber boots, or anything that remains moist (Briede, 2011), recreational boating and fishing in reservoirs is of special concern for crayfish conservation in Europe. A crayfish plague outbreak in a Czech Republic river probably originated downstream in a dense population of non-native Faxonius limosus (spiny-cheek crayfish) occupying a reservoir on the River Vlava, although it was unclear how the population was established (Kozubíková-Balcarová et al., 2014). In Ireland and Norway, crayfish plague occurred in popular angling reservoirs that lacked North American crayfishes (Reynolds, 1988; Taugbøl et al., 1993). Contaminated fishing gear or contaminated water associated with fish stocking may have caused the outbreak in Ireland (Reynolds, 1988; Gerrard et al., 2003).
Positive Effects
Dams can have benefits as well as negative effects on native, European crayfish fauna. We grouped potential benefits into two classes: (1) creation of expanded habitat for some species, and (2) creation of barriers to reduce the spread of invasive crayfishes and crayfish plague (Krieg and Zenker, 2020).
Given that the European crayfish fauna is adapted to lakes, it is unsurprising that several examples illustrate some native European species thriving in reservoirs. Austropotamobius torrentium (stone crayfish) occurred in “relatively high density” in Dospat Dam, Bulgaria (Zaikov et al., 2011). Astacus leptodactylus is native to Turkish lakes and was also widely stocked in reservoirs, where it often thrived (Yuksel et al., 2013; Kokko et al., 2018). Astacus leptodactylus stocked in the 68,731 ha Keban Dam Lake, Turkey, became the most economically important species commercially fished, yielding annual harvests of 3,000–35,000 kg from 1994 to 2013 (Yuksel et al., 2013). The population in that reservoir also appeared to be more fecund than other populations in the country (Harlioglu et al., 2004). A small sample from Keban Lake Dam taken in 2011–12 revealed no crayfish plague (Kokko et al., 2018). Nonetheless, crayfish plague was introduced to Turkey in the 1980's and is now widespread, causing periodic crayfishery collapses; however, some resistance is evidenced by latent infections (Kokko et al., 2018).
Krieg and Zenker (2020) reviewed the use of physical barriers to stop crayfish movement and concluded that barriers were the best method to stop the non-anthropogenic spread of invasive crayfishes. Thus, using dams as a barrier can benefit native crayfishes by blocking the upstream spread of invasive crayfishes and the crayfish plague pathogen (Krieg and Zenker, 2020). In three instances in the Czech Republic, dams ranging from 0.5 to 2.0 m high potentially limited the upstream spread of acute crayfish plague outbreaks (Kozubíková-Balcarová et al., 2014). In one case, boards were added to successfully fortify the barrier created by a low dam during an outbreak. Similarly in a English stream, a low-head, flow-gauging weir with relatively low water velocity reduced upstream movements by P. leniusculus (Rosewarne et al., 2013). In several Norwegian rivers, the upstream spread of the crayfish plague appeared to be stopped or slowed by dams or waterfalls (Taugbøl et al., 1993). In one example, a lock appeared to block the upstream spread of the crayfish plague until the lock was opened to allow boat passage during a plague outbreak downstream. All authors noted that dams may reduce the spread of invasive crayfishes and diseases, but they are unlikely to permanently prevent upstream invasions (Dana et al., 2011; Rosewarne et al., 2013; Kozubíková-Balcarová et al., 2014). Of course, intentional movement of crayfishes around obstructions by people will foil the best-designed barrier (Dana et al., 2011).
In Spain, three small (1.5–2.0 m high) dams were constructed in a mountain stream with the intent of protecting the “southernmost population of the endangered Austropotamobius pallipes” from invasion by P. clarkii and crayfish plague, both present downstream (Dana et al., 2011). As of publication, the dams had blocked the invasion for 4 years. In addition, the small population of P. clarkii between the two downstream dams dropped below detectable limits, facilitated by removals during sampling.
Barriers intended to block upstream movements by crayfishes, but not fishes (Frings et al., 2013), may have high potential for conservation success where crayfish plague is absent. However, in the presence of the plague, movements by fishes, water birds, mammals, boats, anglers, swimmers, etc. may spread the plague (Taugbøl et al., 1993; Oidtmann et al., 2002; Frings et al., 2013), so providing fish passage may allow transport of the plague upstream of a selective barrier, even without non-native crayfishes passing it (Frings et al., 2013).
Additional Predicted Effects of Dams on Crayfishes and Future Research Needs
Potential Effects on Burrowing Crayfishes
Fluvial floodplains are unique habitats created, in part, by their temporary connections with open water during river flooding (Opperman et al., 2010; Helms et al., 2013). Burrowing crayfishes spend all or part of their lives in burrows, and some are strictly associated with fluvial floodplains (Helms et al., 2013). When floodplains are either inundated by reservoirs or isolated from rivers due to channel incision and reduced overbank flooding downstream of dams, primary burrower populations are likely negatively affected. Channel incision may further affect such species by locally lowering the water table (Scott et al., 2000; Schilling et al., 2004), thereby requiring crayfishes to burrow deeper to reach groundwater—at least for species that tend to burrow from the floodplain rather than within stream channels. The extent of such impacts and the population responses remain unexplored. Although such effects would likely be considered detrimental in the context of conserving native burrowers, they could perhaps be beneficial in reducing population sizes of some non-native burrowing species.
Inhibition of Headcutting
The tendency of dams to inhibit upstream headcutting is potentially beneficial to upstream crayfishes in regions with highly erodible soils, lack of bedrock stream channel controls, and certain types of disturbances (e.g., channelization) that foster extreme channel incision and subsequent channel widening and headcutting (upstream spread of incision) (Simon and Rinaldi, 2006). These processes can lead to even small streams being deeply entrenched and incised, a common occurrence in the Coastal Plain of the southeastern US (Patrick et al., 1991; Hartfield, 1993). While the effects of such incision on crayfishes are poorly studied, incised streams are marked by flashy hydrographs, extremely unstable substrates, and a paucity of instream cover, all of which seem to translate to reduced crayfish densities (Adams, 2014). Dams can retard upstream channel incision, thereby helping to minimize stream headcutting and retain instream cover and stream interactions with their floodplains in ways that should benefit crayfish assemblages. Experimental weirs and small check dams have been constructed explicitly to retard channel incision and headcutting (Shields et al., 1995), but the effects on crayfish populations have not been investigated.
Managing Flows to Prevent the Spread of Invasive Species
Modification of dam operations to create bankfull or greater flows downstream may be one tool to reduce numbers of invasive crayfish downstream (Light, 2003). High water velocities or discharges have reduced densities or eliminated both P. leniusculus and P. clarkii from study stream segments (Light, 2003; Kerby et al., 2005). A similar approach used for fishes indicated that mimicking high spring flows benefitted native fish recruitment but had little impact on non-native fishes (Propst and Gido, 2004). Modifying flows in concert with vertical barriers to crayfish movements warrants further research as a tactic for halting invasions and reducing populations of some widely introduced, invasive crayfishes. However, floods may also negatively affect some native crayfishes, and increased flooding reduced modeled persistence time for an isolated population of A. astacus (Meyer et al., 2007).
Additional Research Needs
In striving to slow or halt biodiversity losses and lotic community alterations caused by anthropogenic environmental changes, more research is needed that explicitly examines the effects of dams and dam removals on both native and invasive crayfishes (Johnson et al., 2008). Research should include understanding crayfish habitat preferences, ecological tolerances, and dispersal capabilities to aid in predictions of how species will be impacted by dams. Also, assessing how various dam types (e.g., purpose, operation, size, and age) in different regional contexts affect crayfishes is key to understanding their costs and benefits to crayfish populations.
Further research on dam characteristics that block upstream crayfish migrations is needed, especially in low gradient systems. Understanding the interaction of temperature and barrier characteristics is needed (Frings et al., 2013) and may lead to barriers that could be seasonally adjusted to facilitate fish but not crayfish passage. Other non-permanent barriers (e.g., push-up or inflatable dams) could possibly be pre-installed but not raised until an invasion is imminent, such as when a downstream barrier is passed, but such an approach would require extensive testing. Frings et al. (2013) suggested that existing dams with fish ladders provide potential locations for crayfish barriers, but given that crayfish ascended an eel ladder (Welsh and Loughman, 2015), more research is needed on characteristics of fish barriers to exclude crayfishes. A fish ladder with relatively high water velocities (up to 2 ms−1) at a hydropower dam on the Elbe River prevented upstream movement of F. limosus during short-term trials, but a shipping channel in the same dam may have facilitated their upstream migration (Kozák et al., 2004). Studies are needed that evaluate alternatives to dam removals, such as reducing dam sizes—and thus, ecological impacts—while maintaining their functions as barriers to invasive crayfishes. Finally, further evaluation of the trade-offs between dams as beneficial barriers to upstream invasions versus their roles in fragmenting populations and increasing risks of novel introductions will facilitate decision making about barrier construction and dam removals.
Conclusions
In summary, this review highlights the challenges and opportunities dams create for crayfish conservation. Dams affect crayfishes differently in three zones: the reservoir and the up- and downstream fluvial river segments. Detrimental effects to native crayfishes, especially small-bodied or habitat-specialist species, can occur in each zone, with larger dams often causing greater changes to crayfish communities than smaller dams. The reduction of suitable habitats, fragmentation of crayfish populations, and reduction of a species' ability to recolonize upstream habitats may further negatively affect native populations. In addition, dams create conditions conducive to introduction of, and invasion by, non-native species that often threaten native crayfishes. Conversely, dams frequently create barriers that slow or halt upstream invasions by non-native animals, and therefore, can serve a vital role in protecting isolated, remnant populations of native crayfishes upstream. Both the ecological costs and benefits should be considered when assessing removal or installation of barriers. Although crayfish conservation concerns vary greatly between Europe and North America due to the differential effects of the crayfish plague between the continents, lessons can be learned from effects of dams on crayfishes in North America and other continents and applied to European crayfish conservation. On both continents, additional research is needed to better understand both the beneficial and detrimental effects of various types of dams on native crayfishes and is essential for effective conservation of many crayfish species.
Author Contributions
Both authors contributed to the conception and design of the manuscript, conducted literature reviews, wrote sections of the manuscript, conducted manuscript revisions, read and approved the submitted version.
Conflict of Interest
The authors declare that the research was conducted in the absence of any commercial or financial relationships that could be construed as a potential conflict of interest.
Acknowledgments
We are grateful to the two reviewers for comments that improved our manuscript. Work was supported by the USDA Forest Service, Southern Research Station. This is publication number 021 of the Center for Biodiversity & Conservation Research at the University of Mississippi.
References
Abernathy, E., McCombs, E., Siefferman, L., and Gangloff, M. M. (2013). Effect of small dams on freshwater mussel population genetics in two southeastern USA streams. Walkerana 16, 21–28. doi: 10.31931/fmbc.v16i1.2013.21-28
Adams, S. B. (2013). Effects of small impoundments on downstream crayfish assemblages. Freshw. Sci. 32, 1318–1332. doi: 10.1899/12-161.1
Adams, S. B. (2014). Crayfish use of trash versus natural cover in incised, sand-bed streams. Environ. Manage. 53, 382–392. doi: 10.1007/s00267-013-0197-3
Adams, S. B., Roghair, C., Krause, C., Warren, M. L. Jr, Cochran, J. A., Dolloff, A., et al. (2015). New crayfish species records from the sipsey fork drainage, including lewis smith reservoir (Alabama, USA): native or introduced species? Freshw. Crayfish 21, 17–32. doi: 10.5869/fc.2015.v21-1.17
Anderson, E. P., Freeman, M. C., and Pringle, C. M. (2006). Ecological consequences of hydropower development in central America: impact of small dams and water diversion on neotropical stream fish assemblages. River Res. Appl. 22, 397–411. doi: 10.1002/rra.899
Armitage, P. D. (1978). Downstream changes in the composition, numbers, and biomass of bottom fauna in the tees beelow cow green reservoir and in an unregulated tributary maize beck, in the first five years after impoundment. Hydrobiologia 58, 145–156. doi: 10.1007/BF00007996
Banha, F., and Anastácio, P. M. (2014). Desiccation survival capacities of two invasive crayfish species. Knowl. Manag. Aquat. Ecosyst. 413, 1–5. doi: 10.1051/kmae/2013084
Banha, F., and Anastácio, P. M. (2015). Live bait capture and crayfish trapping as potential vectors for freshwater invasive fauna. Limnologica 51, 63–69. doi: 10.1016/j.limno.2014.12.006
Barnett, Z. C. (2017). Habitat use and life history of the vernal crayfish, procambarus viaeviridis (Faxon, 1914), a secondary burrowing crayfish in Mississippi, USA. J. Crustacean Biol. 37, 544–555. doi: 10.1093/jcbiol/rux073
Barnett, Z. C., Adams, S. B., Ochs, C. A., and Garrick, R. C. (2020). Crayfish populations genetically fragmented in streams impounded for 36-104 years. Freshw. Biol. 65, 768–785. doi: 10.1111/fwb.13466
Barnett, Z. C. (2019). Effects of impoundments on the community assemblage and gene flow of sream crayfishes. Ph.D. thesis, Barnett: University of Mississippi.
Bates, J. M. (1962). The impact of impoundments on the mussel fauna of kentucky reservoir, tennessee river. Am. Midl. Nat. 68, 232–236. doi: 10.2307/2422648
Baumgartner, M. T., Piana, P. A., Baumgartner, G., and Gomes, L. C. (2020). Storage or run-of-river reservoirs: exploring the ecological effects of dam operation on stability and species interactions of fish assemblages. Environ. Manage. 65, 220–231. doi: 10.1007/s00267-019-01243-x
Baxter, R. M. (1977). Environmental effects of dams and impoundments. Annu. Rev. Ecol. Syst. 8, 255–283. doi: 10.1146/annurev.es.08.110177.001351
Bobeldyk, A. M., and Lamberti, G. A. (2008). A decade after invasion: evaluating the continuing effects of rusty crayfish on a michigan River. J. Great Lakes Res. 34, 265–275. doi: 10.3394/0380-1330(2008)34[265:ADAIET]2.0.CO;2
Bohl, E. (1987). Compariative studies on crayfish brooks in bavaria (Astacus astacus L., Austropotamobius torrentium Schr.). Freshw. Crayfish 7, 287–294.
Branco, P., Segurado, P., Santos, J. M., Pinheiro, P., and Ferreira, M. T. (2012). Does longitudinal connectivity loss affect the distribution of freshwater fish? Ecol. Eng. 48, 70–78. doi: 10.1016/j.ecoleng.2011.05.008
Bubb, D. H., Thom, T. J., and Lucas, M. C. (2008). Spatial ecology of the white-clawed crayfish in an upland stream and implications for the conservation of this endangered species. Aquat. Conserv. 18, 647–657. doi: 10.1002/aqc.862
Bunn, S. E., and Arthington, A. H. (2002). Basic principles and ecological consequences of altered flow refimes for aquatic biodiversity. Environ. Manage. 30, 492–507. doi: 10.1007/s00267-002-2737-0
Caiola, N., Ibáñez, C., Verdú, J., and Munné, A. (2014). Effects of flow regulation on the establishment of alien fish species: a community structure approach to biolgical validation of environmental flows. Ecol. Indic. 45, 598–604. doi: 10.1016/j.ecolind.2014.05.012
Camargo, J. A., and Voelz, N. J. (1998). Biotic and abiotic changes along the recovery gradient of two impounded rivers with different impoundment use. Environ. Monit. Assess. 50, 143–158. doi: 10.1023/A:1005712024049
Cassidy, R. A. (1989). “Water temperature, dissolved oxygen, and turbidity control in reservoir releases,” in Alternatives in Regulated River Management, (Boca Raton, FL: CRC Press), 28–62.
Chien, N. (1985). Changes in river regime after the construction of upstream reservoirs. Earth Surface Process. Landforms 10, 143–159. doi: 10.1002/esp.3290100207
Chucholl, C., and Wendler, F. (2017). Positive selection of beautiful invaders: long-term persistence and bio0invasion risk of freshwater crayfish in the pet trade. Biol. Invasions 19, 197–208. doi: 10.1007/s10530-016-1272-5
Cole, E., Keller, R. P., and Garbach, K. (2019). Risk of invasive species spread by recreational boaters remains high despite widespread adoption of conservation behaviors. J. Environ. Manag. 229, 112–119. doi: 10.1016/j.jenvman.2018.06.078
Cowart, D. A., Breedveld, K. G. H., Ellis, M. J., Hull, J. M., and Larson, E. R. (2018). Environmental DNA (eDNA) applications for the conservation of imperiled crayfish (Decapoda: Astacidea) through monitoring of invasive species barriers and relocated populations. J. Crustacean Biol. 38, 257–266. doi: 10.1093/jcbiol/ruy007
Crook, D. A., Lowe, W. H., Allendorf, F. W., Erös, T., Finn, D. S., Gillanders, B. M., et al. (2015). Human effects on ecological connectivity in aquatic ecosystems: integrating scientific approaches to support management and mitigation. Sci. Total Environ. 534, 52–64. doi: 10.1016/j.scitotenv.2015.04.034
Cumming, G. S. (2004). The impact of low-head dams on fish species richness in Wisconsin, USA. Ecol. Appl. 14, 1495–1506. doi: 10.1890/03-5306
Dana, E. D., García-de-Lomas, J., González, R., and Ortega, F. (2011). Effectiveness of dam construction to contain the invasive crayfish Procambarus clarkii in a mediterranean mountain stream. Ecol. Eng. 37, 1607–1613. doi: 10.1016/j.ecoleng.2011.06.014
Davies, G. D., Gozlan, R. E., and Britton, J. R. (2013). Can accidental introduction of non-native species be prevented by fish stocking audits? Aquat. Conserv. 23, 366–373. doi: 10.1002/aqc.2341
Diéguez-Uribeondo, J. (2010). Current techniques, approaches and knowledge in diagnosis of crayfish plague and other crayfish diseases. Knowl. Manage. Aquat. Ecosyst. 394–395. doi: 10.1051/kmae/2010004
DiStefano, R. J., Litvan, M. E., and Horner, P. T. (2009a). The bait industry as a potential vector for alien crayfish introductions: problems recognition y fisheries agencies and a Missouri evaluation. Fisheries 34, 586–597. doi: 10.1577/1548-8446-34.12.586
DiStefano, R. J., Magoulick, D. D., Imhoff, E. M., and Larson, E. R. (2009b). Imperiled crayfishes use hyporheic zone during seasonal drying of an intermittent stream. J. North Am. Benthol. Soc. 28, 142–152. doi: 10.1899/08-072.1
Dixo, M., Metzger, J. P., Morgante, J. S., and Zamudio, K. R. (2009). Habitat fragmentation reduces genetic diversity and connectivity among populations in the Brazilian Atlantic Coastal forest. Biol. Conserv. 142, 1560–1569. doi: 10.1016/j.biocon.2008.11.016
Dorn, N. J., and Mittelbach, G. G. (1999). More than predator and prey: a review of interactions between fish and crayfish. Vie Milieu 49, 229–237.
Ellis, L. E., and Jones, N. E. (2013). Longitudinal trends in regulated rivers: a review and synthesis within the context of the serial discontinuity concept. Environ. Rev. 21, 136–148. doi: 10.1139/er-2012-0064
Fagan, W. F. (2002). Connectivity, fragmentation, and extinction risk in dendritic metapopulations. Ecology 83, 3243–3249. doi: 10.1890/0012-9658(2002)083[3243:CFAERI]2.0.CO;2
Falke, J. A., and Gido, K. B. (2006). Effects of reservoir connectivity on stream fish assemblages in the great plains. Can. J. Fish. Aquat. Sci. 63, 480–493. doi: 10.1139/f05-233
Finstad, A. G., Einum, S., Forseth, T., and Ugedal, O. (2007). Shelter availability affects behviour, size-dependent and mean growth of juvenile Atlantic salmon. Freshw. Biol. 52, 1710–1718. doi: 10.1111/j.1365-2427.2007.01799.x
Flinders, C. A., and Magoulick, D. D. (2003). Effects of stream permanence on crayfish community structure. Am. Midl. Nat. 149, 134–147. doi: 10.1674/0003-0031(2003)149[0134:EOSPOC]2.0.CO;2
Fluker, B. L., Kuhajda, B. R., and Harris, P. M. (2014). The effects of riverine impoundment on genetic structure and gene flow in two stream fishes in the mobile river basin. Freshw. Biol. 59, 526–543. doi: 10.1111/fwb.12283
Frings, R. M., VaeBen, S. C. K., Grob, H., Roger, S., Schuttrumpf, H., and Hollert, H. (2013). A fish-passable barrier to stop the invasion of non-indigenous crayfish. Biol. Conserv. 159, 521–529. doi: 10.1016/j.biocon.2012.12.014
Fuller, M. R., Doyle, M. W., and Strayer, D. L. (2015). Causes and consequences of habitat fragmentation in river networks. Ann. N. Y. Acad. Sci. 1355, 31–51. doi: 10.1111/nyas.12853
Fullerton, A. H., Burnett, K. M., Steel, E. A., Flitcroft, R. L., Pess, G. R., Feist, B. E., et al. (2010). Hydrological connectivity for riverine fish: measurement challenges and research opportunities. Freshw. Biol. 55, 2215–2237. doi: 10.1111/j.1365-2427.2010.02448.x
Füreder, L. (2016). “Crayfish in Europe,” in Freshwater Crayfish: A Global Overview, eds T. Kawai, Z. Faulkes and G. Scholtz (Boca Raton, FL: CRC Press), 594–627. doi: 10.1201/b18723-26
Gehrke, P. C., Gilligan, D. M., and Barwick, M. (2002). Changes in fish communities of the shoalhaven river 20 years after construction of Tallowa Dam, Australia. River Res. Appl. 18, 265–286. doi: 10.1002/rra.669
Gerrard, C., Addy, C., Tero, C., and Kite, N. (2003). “White-clawed crayfish in the river Ise, northhamptonshire,” in Management and Conservation of Crayfish, Proceedings of a conference held in Nottingham on 7th November 2002, eds D. M. Holdich and P. J. Sibley (Bristol: Environment Agency), 112-120.
Gherardi, F., Aquiloni, L., Diéguez-Uribeondo, J., and Tricarico, E. (2011). Managing invasive crayfish: is there a hope? Aquat. Sci. 73, 185–200. doi: 10.1007/s00027-011-0181-z
Gillespie, J., Nyaupane, N., and Boucher, R. (2012). Changes in crawfish production costs and current production practices used. Aquac. Econ. Manage. 16, 250–265. doi: 10.1080/13657305.2012.704619
Gordon, N. D., McMahon, T. A., Finlayson, B. L., Gippel, C. J., and Nathan, R. J. (2004). Stream Hydrology: an Introduction for Ecologists, 2nd Edn. Chichester: John Wiley and Sons.
Graf, W. L. (2005). Geomorphology and American dams: the scientific, social, and economic context. Geomorphology 71, 3–26. doi: 10.1016/j.geomorph.2004.05.005
Graf, W. L. (2006). Downstream hydrologic and geomorphic effects of large dams on American rivers. Geomorphology 79, 336–360. doi: 10.1016/j.geomorph.2006.06.022
Grill, G., Lehner, B., Lumsdon, A. E., MacDonald, G. K., Zarfl, C., and Reidy Liermann, C. (2015). An index-based framework for assessing patterns and trends in river fragmentation and flow regulation by global dams at multiple scales. Environ. Res. Lett. 10, 1–15. doi: 10.1088/1748-9326/10/1/015001
Haas, T. C., Blum, M. J., and Heins, D. C. (2010). Morphological responses of a stream fish to water impoundment. Biol. Lett. 6, 803–806. doi: 10.1098/rsbl.2010.0401
Hall, C. J., Jordaan, A., and Frisk, M. G. (2011). The historic influence of dams on diadromous fish habitat with a focus on river herring and hydrologic longitudinal connectivity. Landsc. Ecol. 26, 95–107. doi: 10.1007/s10980-010-9539-1
Hanks, R. D., and Hartman, K. J. (2019). Evaluation of the influences of dam release types, land use, and habitat affecting abundance, richness, diversity, andcommunity structure of larval and juvenile fish. Can. J. Fish. Aquat. Sci. 76, 1388–1397. doi: 10.1139/cjfas-2017-0107
Harlioglu, M. M., Barim, Ö., Türkgülü, I., and Harlioglu, A. G. (2004). Potential fecundity of an introduced population, keban dam lake, Elazig, Turkey, of freshwater crayfish, Astacus leptodactylus leptodactylus (Esch., 1852). Aquaculture 230, 189–195. doi: 10.1016/S0044-8486(03)00430-7
Hartfield, P. (1993). “Headcuts and their effects on freshwater mussels,” in Conservation and Management of Freshwater Mussels, eds K. S. Cummings, A. C. Buchanan, and L. M. Kock (Rock Island, IL: Upper Mississippi River Conservation Committee), 131–141.
Hartfield, E. E. (2010). Consequences of low-head dams on crayfish distribution and gene flow in Alabama streams. (M.S. thesis). Auburn: Auburn University.
Havel, J. E., Lee, C. E., and Zanden, M. J. V. (2005). Do reservoirs facilitate invasions into landscapes? Bioscience 55, 518–525. doi: 10.1641/0006-3568(2005)055[0518:DRFIIL]2.0.CO;2
Helms, B. S., Budnick, W., Pecora, P., Skipper, J., Kosnicki, E., Feminella, J., et al. (2013). The influence of soil type, congeneric cues, and floodplain connectivity on the local distribution of the devil crayfish (Cambarus diogenes Girard). Freshw. Sci. 32, 1333–1344. doi: 10.1899/12-160.1
Herbert, M. E., and Gelwick, F. P. (2003). Spatial variation of headwater fish assemblages explained by hydrologic variability and upstream effects of impoundment. Copeia 2003, 273–284. doi: 10.1643/0045-8511(2003)003[0273:SVOHFA]2.0.CO;2
Hicks, B. J. (2003). Distribution and abundance of fish and crayfish in a waikato stream in relation to basin area. NZ. J. Zool. 30, 149–160. doi: 10.1080/03014223.2003.9518333
Hladík, M., and Kubečka, J. (2003). Fish migration between temperate reservoir and its main tributary. Hydrobiologia 504, 251–266. doi: 10.1023/B:HYDR.0000008525.46939.42
Hobbs, H. H. III., Jass, J. P., and Huner, J. V. (1989). A review of global crayfish introductions with particular emphasis on two North American species (Decapoda, Cambaridae). Crustaceana 56, 299–316. doi: 10.1163/156854089X00275
Hu, B., Yang, Z., Wang, H., Sun, X., Bi, N., and Li, G. (2009). Sedimentation in the three gorges dam and the future trend of changjiang (Yangtze River) sediment flux to the sea. Hydrol. Earth Syst. Sci. 13, 2253–2264. doi: 10.5194/hess-13-2253-2009
Johnson, P. T. J., Olden, J. D., and Zanden, M. J. V. (2008). Dam invaders: impoundments facilitate biological invasions into freshwaters. Front. Ecol. Environ. 6, 357–363. doi: 10.1890/070156
Jones, J. I., Murphy, J. F., Collins, A. L., Sear, D. A., Naden, P. S., and Armitage, P. D. (2012). The impact of fine sediment on macro-invertebrates. River Res. Appl. 28, 1055–1071. doi: 10.1002/rra.1516
Joy, M. K., and Death, R. G. (2001). Control of freshwater fish and crayfish community structure in Taranaki, New Zealand: dams, diadromy or habitat structure? Freshw. Biol. 46, 417–429. doi: 10.1046/j.1365-2427.2001.00681.x
Júnior, H. F. J., Tós, C. D., Agostinho, Â. A., and Pavanelli, C. S. (2009). A massive invasion of fish species after eliminating a natural barrier in the upper rio Paraná basin. Neotrop. Ichthyol. 7, 709–718. doi: 10.1590/S1679-62252009000400021
Kanno, Y., and Vokoun, J. C. (2010). Evaluating effects of water withdrawals and impoundments on fish assemblages in southern New England streams, USA. Fish. Manag. Ecol. 17, 272–283. doi: 10.1111/j.1365-2400.2009.00724.x
Kerby, J. L., Riley, S. P. D., Kats, L. B., and Wilson, P. (2005). Barriers and flow as limiting factors in the spread of an invasive crayfish (Procambarus clarkii) in southern California streams. Biol. Conserv. 126, 402–409. doi: 10.1016/j.biocon.2005.06.020
Kilian, J. V., Klauda, R. J., Widman, S., Kashiwagi, M., Bourquin, R., Weglein, S., et al. (2012). An assessment of a bait industry and angler behavior as a vector of invasive species. Biol. Invasions 14, 1469–1481. doi: 10.1007/s10530-012-0173-5
Kim, J., and Mandrak, N. E. (2016). Assessing the potential movement of invasive fishes through the Welland Canal. J. Great Lakes Res. 42, 1102–1108. doi: 10.1016/j.jglr.2016.07.009
Kinsolving, A. D., and Bain, M. B. (1993). Fish assemblage recovery along a riverine disturbance gradient. Ecol. Appl. 3, 531–544. doi: 10.2307/1941921
Kokko, H., Harlioglu, M. M., Aydin, H., Makkonen, J., Gökmen, G., Aksu, Ö., et al. (2018). Observations of crayfish plague infections in commercially important narrow-clawed crayfish populations in Turkey. Knowl. Manag. Aquat. Ecosyst. 419, 1–4. doi: 10.1051/kmae/2018001
Kozák, P., Policar, T., and Duriš, Z. (2004). Migratory ability of orconectes limosus through a fishpass and notes on its occurrence in the czech republic. Bull. Français Pêche Piscic. 372–373, 367–373. doi: 10.1051/kmae:2004010
Kozubíková, E., Petrusek, A., Duriš, Z., Martín, M. P., Diéguez-Uribeondo, J., and Oidtmann, B. (2008). The old menace is back: recent crayfish plague outbreaks in the czech republic. Aquaculture 274, 208–217. doi: 10.1016/j.aquaculture.2007.11.015
Kozubíková-Balcarová, E., Beran, L., Duriš, Z., Fischer, D., Horká, I., Svobodová, J., et al. (2014). Status and recovery of indigenous crayfish populations after recent crayfish plague outbreaks in the czech republic. Ethol. Ecol. Evol. 26, 299–319. doi: 10.1080/03949370.2014.897652
Krieg, R., and Zenker, A. (2020). A review of the use of physical barriers to stop the spread of non-indigenous crayfish species. Rev. Fish Biol. Fish. 30, 423–435. doi: 10.1007/s11160-020-09606-y
Kunz, M. J., Wüest, A., Wehrli, B., Landert, J., and Senn, D. B. (2011). Impact of a large tropical reservoir on riverine transport of sediment, carbon, and nutrients to downstream wetlands. Water Resour. Res. 47:W12531. doi: 10.1029/2011WR010996
Lacy, R. C. (1987). Loss of genetic diversity from managed populations: interacting effects of drift, mutation, immigration, selection, and population subdivision. Conserv. Biol. 1, 143–158. doi: 10.1111/j.1523-1739.1987.tb00023.x
Lande, R., and Barrowclough, G. (1987). “Effective population size, genetic variation, and their use in population management,” in Viable Populations for Conservation, ed M. E. Soule (Great Britain: University Press, Cambridge) 87–124. doi: 10.1017/CBO9780511623400.007
Lessard, J. L., and Hayes, D. B. (2003). Effects of elevated water temperature on fish and macroinvertebrate communities below small dams. River Res. Appl. 19, 721–732. doi: 10.1002/rra.713
Lieb, D. A., Bouchard, R. W., Carline, R. F., Nuttall, T. R., Wallace, J. R., and Burkholder, C. L. (2011). Conservation and management of crayfishes: lessons from pennsylvania. Fisheries 36, 489–507. doi: 10.1080/03632415.2011.607080
Liermann, C. R., Nilsson, C., Robertson, J., and Ng, R. Y. (2012). Implications of dam obstruction for global freshwater fish diversity. Bioscience 62, 539–548. doi: 10.1525/bio.2012.62.6.5
Light, T. (2003). Success and failure in a lotic crayfish invasion: the roles of hydrologic variability and habitat alteration. Freshw. Biol. 48, 1886–1897. doi: 10.1046/j.1365-2427.2003.01122.x
Lodge, D. M., Stein, R. A., Brown, K. M., Covich, A. P., Brönmark, C., Garvey, J. E., et al. (1998). Predicting impact of freshwater exotic species on native biodiversity: challenges in spatial scaling. Austr. J. Ecol. 23, 53–67. doi: 10.1111/j.1442-9993.1998.tb00705.x
Lodge, D. M., Taylor, C. A., Holdich, D. M., and Skurdal, J. (2000). Nonindigenous crayfishes threaten North American freshwater biodiversity: lessons from Europe. Fisheries 25, 7–20. doi: 10.1577/1548-8446(2000)025<0007:NCTNAF>2.0.CO
Longshaw, M. (2011). Diseases of crayfish: a review. J. Invertebrate Pathal. 106, 54–70. doi: 10.1016/j.jip.2010.09.013
Lowery, R. S. (1988). “Growth, moulting, and reproduction,” in Freshwater Crayfish: Biology, Management and Exploitation, eds D. M. Holdich and R. S. Lowery (Portland, OR: Croom Helm Ltd), 83–113.
Lynch, D. T., Leasure, D. R., and Magoulick, D. D. (2019). Flow alteration-ecology relationships in Ozark Highland streams: consequences for fish, crayfish and macroinvertebrate assemblages. Sci. Total Environ. 672, 680–697. doi: 10.1016/j.scitotenv.2019.03.383
Maavara, T., Dürr, H. H., and Van Cappellen, P. (2014). Worldwide retention of nutrient silicon by river damming: from sparse data set to global estimate. Glob. Biogeochem. Cycles 28, 842–855. doi: 10.1002/2014GB004875
Madzivanzira, T. C., South, J., Wood, L. E., Nunes, A. L., and Weyl, O. L. F. (2020). A review of freshwater crayfish introductions in Africa. Rev. Fish. Sci. Aquac. 1–24. doi: 10.1080/23308249.2020.1802405
Magilligan, F. J., Nislow, K. H., Kynard, B. E., and Hackman, A. M. (2016). Immediate changes in stream channel geomorphology, aquatic habitat, and fish assemblages following dam removal in a small upland catchment. Geomorphology 252, 158–170. doi: 10.1016/j.geomorph.2015.07.027
Magnuson, J. J., Capelli, G. M., Lorman, J. G., and Stein, R. A. (1975). “Consideration of crayfish for macrophyte control,” in Symposium on Water Quality Management Through Biological Control, eds P. L. Brezonik and J. L. Fox (Gainesville: University of Florida), 66–74.
Maneux, E., Probst, J. L., Veyssy, E., and Etcheber, H. (2001). Assessment of dam trapping efficiency from water residence time: application to fluvial sediment transport in the Adour, Dordogne, and Garonne River basins (France). Water Resour. Res. 37, 801–811. doi: 10.1029/2000WR900195
Manfrin, C., Souty-Grosset, C., Anastácio, P. M., Reynolds, J., and Giulianini, P. G. (2019). Detection and control of invasive freshwater crayfish: from traditional to innovative methods. Divers. Distrib. 11, 1–16. doi: 10.3390/d11010005
Matthews, W. J. (2012). Patterns in Freshwater Fish Ecology. New York, NY: Springer Science and Business Media.
McAllister, D. E., Craig, J. F., Davidson, N., Delany, S., and Seddon, M. (2001). “Biodiversity impacts of large dams.”, in: IUCN, UNEP, WCD: Background paper No.1.).
McGregor, S. W., and Garner, J. T. (2003). Changes in the freshwater mussel (Bivalvia: Unionidae) fauna of the bear creek system of northwest Alabama and northeast Mississippi. Am. Malacol. Bull. 18, 61–70. Available online at: https://pubs.er.usgs.gov/publication/70025377
Mead, K. S. (2008). Do antennule and aesthetasc structure in the crayfish Orconectes virilis correlate with flow habitat? Intergr. Comp. Biol. 48, 823–833. doi: 10.1093/icb/icn067
Mejia, F., Torgersen, C. E., Berntsem, E. K., Maroney, J. R., Connor, J. M., Fullerton, A. H., et al. (2020). Longitudinal, lateral, vertical, and temporal thermal heterogeneity in a large impounded river: implications for cold-water refuges. Remote Sens. 12:1386. doi: 10.3390/rs12091386
Meyer, K. M., Gimpel, K., and Brandl, R. (2007). Viability analysis of endangered crayfish populations. J. Zool. 273, 364–371. doi: 10.1111/j.1469-7998.2007.00336.x
Morita, K., and Yamamoto, S. (2002). Effects of habitat fragmentation by damming on the persistence of stream-dwelling charr populations. Conserv. Biol. 16, 1318–1323. doi: 10.1046/j.1523-1739.2002.01476.x
Mrugala, A., Kawai, T., Kozubáková-Balcarová, E., and Petrusek, A. (2017). Aphanomyces astaci presence in Japan: a threat to the endemic and endangered crayfish species in Cambaroides japonicus? Aquat. Conserv. 27, 103–114. doi: 10.1002/aqc.2674
Muirhead, J. R., and Macisaac, H. J. (2005). Development of inland lakes as hubs in an invasion network. J. Appl. Ecol. 42, 80–90. doi: 10.1111/j.1365-2664.2004.00988.x
Mulligan, M., van Soesbergen, A., and Saenz, L. (2020). GOODD, a global dataset of more than 38,000 georeferenced dams. Sci Data 7, 1–8. doi: 10.1038/s41597-020-0362-5
Nakata, K., and Goshima, S. (2003). Competition for shelter of preferred sizes between the native crayfish species Cambaroides japonicus and the alien crayfish species Pacifastacus leniusculus in Japan in relation to prior residence, sex difference, and body size. J. Crustacean Biol. 23, 897–907. doi: 10.1651/C-2411
Nunes, A. L., Zengeya, T. A., Hoffman, A. C., Measey, G. J., and Weyl, O. L. F. (2017). Distribution and establishment of the alien Australian redclaw crayfish, Cherax quadricarinatus, in South Africa and Swaziland. PeerJ 5, 1–21. doi: 10.7717/peerj.3135
Oidtmann, B., Heitz, E., Rogers, D., and Hoffmann, R. W. (2002). Transmission of crayfish plague. Dis. Aquat. Organ. 52, 159–167. doi: 10.3354/dao052159
Opperman, J. J., Luster, R., McKenney, B. A., Roberts, M., and Meadows, A. W. (2010). Ecologically functional floodplains: connectivitiy, flow regime, and scale. J. Am. Water Resourc. Assoc. 46, 211–226. doi: 10.1111/j.1752-1688.2010.00426.x
Parkyn, S. M., and Collier, K. J. (2004). Interaction of press and pulse disturbance on crayfish populations: flood impacts in pasture and forest streams. Hydrobiologia 527, 113–124. doi: 10.1023/B:HYDR.0000043189.91134.94
Parkyn, S. M., DiStefano, R. J., and Imhoff, E. M. (2011). Comparison of constructed microhabitat and baited traps in table rock reservoir, Missouri, USA. Freshw. Crayfish 18, 69–74. doi: 10.5869/fc.2011.v18.69
Patrick, D. M., Mao, L., and Ross, S. T. (1991). The Impact of Geomorphic Change on the Distribution of Bayou Darters in the Bayou Pierre System. Jackson, MS: Mississippi Department of Wildlife, Fisheries and Parks, Museum of Natural Science.
Peay, S. (2010). Invasive non-indigenous crayfish species in Europe: recommendations on managing them. Knowl. Manag. Aquat. Ecosyst. 3, 394–395. doi: 10.1051/kmae/2010009
Perkin, J. S., Gido, K. B., Cooper, A. R., Turner, T. F., Osborne, M. J., Johnson, E. R., et al. (2015). Fragmentation and dewatering transform great plains stream fish communities. Ecol. Monogr. 85, 73–92. doi: 10.1890/14-0121.1
Perry, W. L., Jacks, A. M., Fiorenza, D., Young, M., and Kuhnke, R. (2013). Effects of water velocity on the size and shape of rusty crayfish, Orconectes rusticus. Freshw. Sci. 32, 1398–1409. doi: 10.1899/12-166.2
Phillips, B. W., and Johnston, C. E. (2004a). Changes in the fish assemblage of bear creek (Tennessee River Drainage) Alabama and Mississippi: 1968-2000. Southeastern Nat. 3, 205–218. doi: 10.1656/1528-7092(2004)003[0205:CITFAO]2.0.CO;2
Phillips, B. W., and Johnston, C. E. (2004b). Fish assemblage recovery and persistence. Ecol. Freshw. Fish. 13, 145–153. doi: 10.1111/j.1600-0633.2004.00047.x
Poff, N. L., Allan, J. D., Bain, M. B., Karr, J. R., Prestegaard, K. L., Richter, B. D., et al. (1997). The natural flow regime. Bioscience 47, 769–784. doi: 10.2307/1313099
Poff, N. L., and Ward, J. V. (1989). Implications of streamflow variability and predictability for lotic community structure: a regional analysis of streamflow patterns. Can. J. Fish. Aquat. Sci. 46, 1805–1818. doi: 10.1139/f89-228
Poff, N. L., and Zimmerman, J. K. H. (2010). Ecological responses to altered flow regimes: a literature review to inform the science and management of environmental flows. Freshw. Biol. 55, 194–205. doi: 10.1111/j.1365-2427.2009.02272.x
Power, M. E., Parker, M. S., and Dietrich, W. E. (2008). Seasonal reassembly of a river food web: floods, droughts, and impacts of fish. Ecol. Monogr. 78, 263–282. doi: 10.1890/06-0902.1
Pringle, C. M. (1997). Exploring how disturbance is transmitted upstream: going against the flow. J. North Am. Benthol. Soc. 16, 425–438. doi: 10.2307/1468028
Pringle, C. M. (2000). Threats to U.S. public lands from cumulative hydrologic alterations outside of their boundaries. Ecol. Appl. 10:971. doi: 10.1890/1051-0761(2000)010[0971:TTUSPL]2.0.CO;2
Propst, D. L., and Gido, K. B. (2004). Responses of native and nonnative fishes to natural flow regime mimicry in the San Juan River. Trans. Am. Fish. Soc. 133, 922–931. doi: 10.1577/T03-057.1
Puth, L. M., and Allen, T. F. H. (2004). Potential corridors for the rusty crayfish, Orconectes rusticus, in northern Wisconsin (USA) lakes: lessons for exotic invasions. Lansc. Ecol. 20, 567–577. doi: 10.1007/s10980-004-5649-y
Rabeni, C. F. (1985). Resource partitioning by stream-dwelling crayfish: the influence of body size. Am. Midl. Nat. 113, 20–29. doi: 10.2307/2425344
Rahel, F. J. (2002). Homogenization of freshwater faunas. Annu. Rev. Ecol. Syst. 33, 291–315. doi: 10.1146/annurev.ecolsys.33.010802.150429
Rahel, F. J. (2013). Intentional fragmentation as a management strategy in aquatic systems. Bioscience 63, 362–372. doi: 10.1525/bio.2013.63.5.9
Rahel, F. J., and Stein, R. A. (1988). Complex predator-prey interactions and predator intimidation among crayfish piscivorous fish, and small benthic fish. Oecologia 75, 94–98. doi: 10.1007/BF00378819
Reynolds, J. D. (1988). Crayfish extinctions and crayfish plague in central Ireland. Biol. Conserv. 45, 279–285. doi: 10.1016/0006-3207(88)90059-6
Reynolds, J., and Souty-Grosset, C. (2011). Management of Freshwater Biodiversity: Crayfish as Bioindicators. Reynolds, GA: Cambridge University Press. doi: 10.1017/CBO9781139031790
Richman, N. I., Böhm, M., Adams, S. B., Alvarez, F., Bergey, E. A., Bunn, J. J. S., et al. (2015). Multiple drivers of decline in the global status of freshwater crayfish (Decapoda: Astacidea). Philos. Trans. R. Soc. B Biol. Sci. 370:20140060. doi: 10.1098/rstb.2014.0060
Richmond, J. Q., Reid, D. T., Ashton, K. G., and Zamudio, K. R. (2009). Delayed genetic effects of habitat fragmentation on the ecologically specialized Florida sand skink (Plestiodon reynoldsi). Conserv. Genetics 10, 1281–1297. doi: 10.1007/s10592-008-9707-x
Richter, B. D., and Thomas, G. A. (2007). Restoring environmental flows by modifying dam operations. Resilience Alliance 12:12. doi: 10.5751/ES-02014-120112
Roell, M. J., and Orth, D. J. (1992). Production of three crayfish populations in the new river of West Virginia, USA. Hydrobiologia 228, 185–194. doi: 10.1007/BF00006585
Rogers, W. D., and Holdich, D. M. (1995). Crayfish production in Britain. Freshw. Crayfish 10, 583–596.
Rosewarne, P. J., Piper, A. T., Wright, R. M., and Dunn, A. M. (2013). Do low-head riverine structures hinder the spread of invasive crayfish? Case study of signal crayfish (Pacifastacus leniusculus) movements at a flow gauging weir. Manag. Biol. Invasions 4, 273–282. doi: 10.3391/mbi.2013.4.4.02
Rothlisberger, J. D., Chadderton, W. L., McNulty, J., and Lodge, D. M. (2010). Aquatic invasive species transport via trailered boats: what is being moved, who is moving it, and what can be done. Fisheries 35, 121–132. doi: 10.1577/1548-8446-35.3.121
Ruhr, C. E. (1956). Effect of stream impoundment in Tennessee on the fish populations of tributary streams. Trans. Am. Fish. Soc. 86, 144–157. doi: 10.1577/1548-8659(1956)86[144:EOSIIT]2.0.CO;2
Santos, R. M. B., Sanches Fernandes, L. F., Cortes, R. M. V., Varandas, S. G. P., Jesus, J. J. B., and Pacheco, F. A. L. (2017). Integrative assessment of river damming impacts on aquatic fauna in a Portuguese reservoir. Sci. Total Environ. 601–602, 1108–1118. doi: 10.1016/j.scitotenv.2017.05.255
Schilling, K., Zhang, Y., and Drobney, P. (2004). Water table fluctuations near an incised stream, Walnut Creek, Iowa. J. Hydrol. 286, 236–248. doi: 10.1016/j.jhydrol.2003.09.017
Schleiss, A. J., Franca, M. J., Juez, C., and Cesare, G. D. (2016). Reservoir sedimentation. J. Hydr. Res. 54, 595–614. doi: 10.1080/00221686.2016.1225320
Schwarz (2019). Hydropower Pressure on European Rivers: The Story in Numbers. FLUVIUS, WWF, RiverWatch, EuroNatur, GEOTA.
Scott, M. L., Lines, G. C., and Auble, G. T. (2000). Channel incision and patterns of cottonwood stress and mortality along the mojave river, California. J. Arid Environ. 44, 399–414. doi: 10.1006/jare.1999.0614
Sheldon, A. (1989). A Reconnaissance of Crayfish Populations in Western Montana. Missoula, MT: The University of Montana. doi: 10.5962/bhl.title.51422
Shields, F. D. Jr., Knight, S. S., and Cooper, C. M. (1995). Rehabilitation of watersheds with incising channels. Water Resourc. Bull. 31, 971–982. doi: 10.1111/j.1752-1688.1995.tb03413.x
Simić, V., Petrović, M., and Paunović, M. (2008). Crayfish of serbia and montenegro-the population status and the level of endangerment. Crustaceana 81, 1153–1179. doi: 10.1163/156854008X374496
Simon, A., and Rinaldi, M. (2006). Disturbance, stream incision, and channel evolution: the roles of excess transport capacity and boundary materials in controlling channel response. Geomorphology 79, 361–383. doi: 10.1016/j.geomorph.2006.06.037
Skurdal, J., and Taugbøl, T. (2002). “Astacus,” in Biology of Freshwater Crayfish, ed D. M. Holdich (Ames, IA: Blackwell Scientific, Ltd), 467–510.
Smith, G. R. T., Learner, M. A., Slater, F. M., and Foster, J. (1996). Habitat features important for the conservation of the native crayfish Austropotamobius pallipes in Britain. Biol. Conserv. 75, 239–246. doi: 10.1016/0006-3207(95)00073-9
Souty-Grosset, C., Holdich, D. M., Noël, P. Y., Reynolds, J. D., and Haffner, P. (2006). Atlas of Crayfish in Europe. Paris: Muséum National d'Histoire Naturelle.
Stanford, J. A., and Ward, J. V. (2001). Revisiting the serial discontinuity concept. Regul. Rivers 17, 303–310. doi: 10.1002/rrr.659
Stein, R. A., and Magnuson, J. J. (1976). Behavioral response of crayfish to a fish predator. Ecology 57, 751–761. doi: 10.2307/1936188
Swink, W. D., and Jacobs, K. E. (1983). Influence of a kentucky flood-control reservoir on the tailwater and headwater fish populations. North Am. J. Fish. Manage. 3, 197–203. doi: 10.1577/1548-8659(1983)3<197:IOAKFR>2.0.CO;2
Taugbøl, T., Skurdal, J., and Håstein, T. (1993). Crayfish plague and management strategies in Norway. Biol. Conserv. 63, 75–82. doi: 10.1016/0006-3207(93)90076-D
Taylor, C. A., Knouft, J. H., and Hiland, T. M. (2001). Consequences of stream impoundment on fish communities in a small North American drainage. Regul. Rivers 17, 687–698. doi: 10.1002/rrr.629
The Heinz Center (2002). Dam Removal: Science and Decision Making. Washington, DC: The H. John Heinz III Center for Science, Economics, and the Environment.
Tilman, D., May, R. M., Lehman, C. L., and Nowak, M. A. (1994). Habitat desruction and the extinction debt. Lett. Nat. 371, 65–66. doi: 10.1038/371065a0
Vaughn, C. C., and Taylor, C. M. (1999). Impoundments and the decline of freshwater mussels: a case study of an extinction gradient. Conserv. Biol. 13, 912–920. doi: 10.1046/j.1523-1739.1999.97343.x
Voelz, N. J., and Ward, J. V. (1991). Biotic responses along the recovery gradient of a regulated stream. Can. J. Fish. Aquat. Sci. 48, 2477–2490. doi: 10.1139/f91-289
Ward, J. V. (1976). “Effects of flow patterns below large dams on stream benthos,” in Instream Flow Needs, eds J. F. Orsborn and C. H. Allman (Bethesda, MD: American Fisheries Society), 235–253.
Ward, J. V., and Stanford, J. A. (1983). “The serial discontinuity concept of lotic ecosystems,” in Dynamics of Lotic Ecosystems, eds T. D. Fontaine III and S. M. Bartell (Ann Arbor, MI: Ann Arbor Science), 29–42.
Watters, G. T. (1996). Small dams as barriers to freshwater mussels (Bivalvia, Unionoida) and their hosts. Biol. Conserv. 75, 79–85. doi: 10.1016/0006-3207(95)00034-8
Welsh, S. A., and Loughman, Z. J. (2015). Upstream dispersal of an invasive crayfish aided by a fish passage facility. Manage. Biol. Invasions 6, 287–294. doi: 10.3391/mbi.2015.6.3.07
Westhoff, J. T., Guyot, J. A., and DiStefano, R. J. (2006). Distribution of the imperiled williams' crayfish (Orconectes williamsi) in the white river drainage of missouri: associations with multi-scale environmental variables. Am. Midl. Nat. 156, 273–288. doi: 10.1674/0003-0031(2006)156[273:DOTIWC]2.0.CO;2
Winston, M. R., Taylor, C. M., and Pigg, J. (1991). Upstream extirpation of four minnow species due to damming of a prairie stream. Trans. Am. Fish. Soc. 120, 98–105. doi: 10.1577/1548-8659(1991)120<0098:UEOFMS>2.3.CO;2
Winton, R. S., Calamita, E., and Wehrli, B. (2019). Reviews and syntheses: dams, water quality and tropical reservoirstratification. Biogeosciences 16, 1657–1671. doi: 10.5194/bg-16-1657-2019
Wood, P. J., and Petts, G. E. (1994). Low flows and recovery of macroinvertebrates in a small regulated chalk stream. Regul. Rivers 9, 303–316. doi: 10.1002/rrr.3450090410
Worth, D. F. (1995). Gradient changes in water quality during low flows in run-of-the-river and reservoir impoundments, lower snake River, Idaho. Lake Reserv. Manag. 11, 217–224. doi: 10.1080/07438149509354202
Yuksel, F., Demirol, F., and Gunduz, F. (2013). Leslie population estimation for Turkish crayfish (Astacus leptodactylus Esch., 1823) in the Keban Dam Lake, Turkey. Turkish J. Fish. Aquat. Sci. 13, 835–839. doi: 10.4194/1303-2712-v13_5_07
Zaikov, A., Hubenova, T., Iliev, I., Vasileva, P., and Piskov, I. (2011). Study on the stone crayfish Austropotamobius torrentium (Schrank, 1803) (Crustacea: Decapoda: Astacidae) population in the dospat dam (Western Rhodope Mountains, Bulgaria). ZooNotes 18, 1–5.
Keywords: impoundment, dams, European crayfish, crayfish plague, fragmentation, dispersal, migration, non-native species
Citation: Barnett ZC and Adams SB (2021) Review of Dam Effects on Native and Invasive Crayfishes Illustrates Complex Choices for Conservation Planning. Front. Ecol. Evol. 8:621723. doi: 10.3389/fevo.2020.621723
Received: 26 October 2020; Accepted: 14 December 2020;
Published: 14 January 2021.
Edited by:
Javier Dieguez-Uribeondo, Real Jardín Botánico (RJB), SpainReviewed by:
Pavel Kozak, University of South Bohemia in Ceské Budějovice, CzechiaLeopold Füreder, University of Innsbruck, Austria
This work is authored by Zanethia C. Barnett and Susan B. Adams on behalf of the U.S. Government and, as regards Dr. Barnett, Dr. Adams, and the U.S. Government, is not subject to copyright protection in the United States. Foreign and other copyrights may apply. This is an open-access article distributed under the terms of the Creative Commons Attribution License (CC BY). The use, distribution or reproduction in other forums is permitted, provided the original author(s) and the copyright owner(s) are credited and that the original publication in this journal is cited, in accordance with accepted academic practice. No use, distribution or reproduction is permitted which does not comply with these terms.
The findings and conclusions in this publication are those of the authors, Zanethia C. Barnett and Susan B. Adams, and should not be construed to represent any official USDA or U.S. Government determination or policy; it was written and prepared by U.S. Government employees on official time.
*Correspondence: Zanethia C. Barnett, WmFuZXRoaWEuQy5CYXJuZXR0QHVzZGEuZ292