- 1Department of Agricultural Sciences, South Eastern Kenya University, Kitui, Kenya
- 2Department of Forest Sciences, University of Helsinki, Helsinki, Finland
- 3MetaMeta Research, 's Hertogenbosch, Netherlands
Degradation characterized by depleted vegetation cover is a serious environmental problem in African rangelands. It poses a serious threat to millions of pastoralists and agropastoralists who depend on livestock as a source of livelihood. Consequently, there has been a growing global interest to consolidate efforts to restore degraded ecosystems. For example, the UN decade of Ecosystem Restoration initiative aims at uniting the world behind a common goal of preventing, halting and reversing the degradation of ecosystems. Grass reseeding using native perennial species has been identified as one of the practical ecological strategies for restoring degraded African rangelands, enhancing vegetation cover and forage production. Knowledge of the multifaceted performance of African rangeland grasses in terms of morphoecological traits, interaction with weeds and water use efficiencies is however largely limited and often elusive. Perennial grasses indigenous to African rangelands Cenchrus ciliaris L. (African foxtail grass), Enteropogon macrostachyus (Hochst. Ex A. Rich.) Monro ex Benth. (Bush rye grass) and Eragrostis superba Peyr. (Maasai love grass), were established in an African semi-arid rangeland under natural conditions to fill this knowledge gap. Morphoecological plant traits: aboveground biomass (shoot, leaf and stem) production, plant densities, basal cover, tiller densities and plant height were measured 9 months after establishment. Interaction between the target grass species and weeds and water use efficiencies (WUE) were also determined. Enteropogon macrostachyus displayed significantly higher values for plant densities, tiller densities and basal cover, indices commonly used to estimate the potential of grasses for ecological restoration. Eragrostis superba produced the highest shoot biomass and water use efficiencies. This is attributed to its higher leafy biomass fraction. Higher aboveground biomass production of E. superba demonstrate its suitability for enhancing rangeland productivity. Cenchrus ciliaris suppressed the weeds. This is linked to its aggressive and allelopathic nature. In conclusion, the three perennial grasses displayed distinct morphoecological traits. In order to achieve successful seed-based restoration of degraded African rangelands using native perennial grasses, careful selection species to maximize on their unique traits is recommended. Ultimately, this selection process should match the desired restoration outcomes and subsequent use of the rangeland.
Introduction
In sub-Saharan Africa (SSA), ~65% of the total landmass is considered arid and semi-arid rangeland (Homewood, 2004). Rangelands have been defined as “land carrying natural or semi-natural vegetation which provides a habitat suitable for herds of wild or domestic ungulates. African rangelands are characterized by low erratic annual rainfall (300–600 mm), high temperatures and nutrient poor soils (Sanchez, 2002). Pastoralists are the main inhabitants of African rangelands. They traverse the vast arid and semi-arid rangelands in search of water and forage resources for their livestock. They are characterized by high levels of biodiversity and encompass a wide range of vegetation formations from grassland with or without some shrub through bush or woodland cover, to savannah woodlands. Consequently, the term “rangeland” recognizes the spatial, temporal and ecological continuities between these land cover types in arid and semi-arid SSA. Primarily, multiple perennial grasses provide a rich and reliable source of forage for grazing domestic and wild herbivores. Such rangeland grasses include Themeda triandra Forssk., Cynodon dactylon (L.) Pers. (Mapinduzi et al., 2003), Chloris roxburghiana Schult., Cenchrus ciliaris L., Enteropogon macrostachyus (Hochst. Ex A. Rich.) Monro ex Benth. Eragrostis superba Peyr. (Mnene et al., 2005), Chloris gayana Knuth., Sorghum sudanense (P.) Stapf. (Koech et al., 2016), Panicum maximum Jacq. (Ludwig et al., 2008) and Panicum coloratum L. (Macandza et al., 2004).
Land degradation, particularly desertification, has been identified as a major environmental issue in SSA rangelands (Mganga et al., 2015a). In African rangelands it is often defined and characterized by the reduction or loss of biodiversity and productivity, increasing the rate of erosion and reducing the ability to produce sustainable activities pertinent to the system of land use. This has led to the continuous reduction and/or disappearance of populations of important indigenous forage species, especially grasses (Mnene et al., 2005). In arid and semi-arid rangeland environments, the process of land degradation normally starts with the formation of smaller denuded and depleted patches that expand and link together to form large bare and degraded areas in the long-run. Increased human population increase and associated land use impacts e.g., overgrazing, are generally assumed to be the dominant factors in their perceived depletion (Cincotta et al., 2000). Indiscriminate grazing and overstocking modify vegetation composition and reduce primary productivity, particularly palatable species, thus decreasing the community resilience and instigating damaging positive feedbacks (Kinyua et al., 2010). Furthermore, degradation in African rangelands and global climatic change has exacerbated continues to pose a serious social and environmental threat leading to food insecurity, poverty, economic losses and destabilized livelihoods.
Therefore, there is need to cushion and build resilience of communities that depend on rangeland resources for their livelihoods, against such environmental vagaries and climatic shocks. Natural regeneration of depleted and denuded rangeland landscapes through plant succession processes is generally very slow, and sometimes impossible, depending on the severity of degradation. Consequently, more active restoration interventions have to be applied (Van den Berg and Kellner, 2005). Passive methods such as fencing and withdrawal of grazing herbivores alone are less effective. Degraded African rangeland environments can be relatively stable and resilient in their undesirable state. Active restoration methods such as prescribed burns, bush clearing and reseeding are key in addressing the problem of rangeland degradation in Africa. Reseeding using desirable indigenous forage species is an innovative intervention to restore denuded rangelands (Mnene et al., 2005; Kinyua et al., 2010; Mganga et al., 2015b; Koech et al., 2016) and increase the resilience of pastoral communities.
The choice of indigenous grasses used for reseeding degraded African rangelands is influenced by their forage value for livestock (Mganga et al., 2015a). Interestingly, to our knowledge, very few studies, if any, have incorporated the forage value component in evaluating indigenous grasses used for restoration programmes in African rangelands. Moreover, the classical approach used for estimating aboveground biomass estimation that aggregates leaf and stem biomass fractions conceals significant information related to the contribution of the separate biomass portions (Poorter et al., 2012). This is because: (1) biomass allocation to the leaf and stem fractions of terrestrial plants is not fixed and may vary over time, across environments and among species and (2) leaf-to-stem ratio play a significant role in ruminant diet selection and forage value determination. Subsequently, we estimated biomass yields as: (1) total aboveground biomass and (2) leaf and stem biomass fractions, respectively.
Furthermore, droughts are expected to increase in intensity and/or duration with climate change, as these changes are predicted to entail higher global mean surface temperatures or increased aridity in many regions of the world. Considering that climate variability and droughts are phenomena that characterize African rangelands, it is important to include knowledge about morphoecology and physiology of drought tolerance when evaluating perennial grasses for restoration especially in marginal rangelands with poor water holding capacity. Drought tolerance has been defined as the ability of plants to maintain metabolism and biomass production at low water potentials caused by limited water availability (Jones et al., 2015) and can be characterized by higher water use efficiency (WUE) (Mårtensson et al., 2017). Therefore, plant genotypes with high drought tolerance or WUE can contribute to yield increases and enhance rangeland restoration success. Moreover, competition for water with other users e.g., weeds, makes maximizing water use efficiency vitally important (Kiniry and Kim, 2020). This is because, arid and semiarid rangelands in Africa are infested with aggressive weeds e.g., Ipomoea kituensis that often engulfs emerging grass seedlings leading to poor establishment. Thus, reseeding with grasses adapted to the range site and highly competitive to replace the undesirable species is often the most innovative way to restore degraded rangeland landscapes.
To generate new knowledge of perennial grasses with potential for biomass production and ecological restoration, this study aimed to determine the morphoecology, water use efficiencies and grass-weed interactions of indigenous grasses commonly used to restore degraded African rangelands. Rangeland grasses indigenous to Africa namely C. ciliaris (African foxtail grass), E. superba (Maasai love grass) and E. macrostachyus (Bush rye grass) were established in a typical semi-arid rangeland in Africa. The choice of these species was primarily based on their adaptive mechanisms for survival in African rangelands and their multipurpose uses among pastoral and agropastoral communities i.e., source of livestock feed and income through the sale of seed and hay.
Materials and Methods
General Description of Location
The reseeding trial was conducted in a semi-arid rangeland at South Eastern Kenya University (Kitui County, Kenya). Crop-livestock production is the main land use system, characterized by indigenous breeds of livestock notably small East African shorthorn zebu, Red Maasai sheep and small East African goats and drought-tolerant varieties of maize, millet, sorghum, pigeon peas and beans (Mganga et al., 2015a). Rainfall pattern is bimodal, with the long rains in March-April-May and short rains in October-November-December. Long term total annual precipitation range between 300 and 800 mm. Annual temperatures averages range between 14 and 34°C, with a mean of 24°C (Schmitt et al., 2019).
On average, the soils are characterized by the following physicochemical parameters; pH (6.4), total organic C (9.7 g kg−1), total N (1.2 g kg−1), total available N (11.7 mg kg-1), available P (73 mg kg−1), K (1.1 cmol kg−1), Na (0.4 cmol kg−1), and CEC (10.1 cmol kg−1) (Yageta et al., 2019). Surface sealing properties and low infiltration rates make the soils vulnerable to erosion, particularly since intense rains come early in the growing season, when the ground is almost bare. Common tree and shrub species include Lannea triphylla (Hochst. ex A. Rich.) Engl., Commiphora africana (A.Rich.) Endl., Acacia mellifera (M. Vahl) Seigler & Ebinger and Acacia senegal (L.) Britton. The herbaceous layer was dominated by grasses such as C. roxburghiana, E. superba, C. ciliaris, E. macrostachyus, and Rhynchelytrum repens (Willd.) Zizka.
Layout and Site Preparation
A randomized complete block design (RCBD) was laid out on a semi-arid rangeland with minimal patches of native overstorey and understorey vegetation. Briefly, three (3) blocks with an area of 150 m2 (15 × 10 m) were laid horizontally adjacent to each other, and with a 2 m buffer spacing in-between blocks. Each block was further sub-divided into three (3) equal plots, each with an area of 50 m2 (5 × 10 m). Each grass species was sown in one experimental plot across the three (3) blocks. Climate data (rainfall and temperature) during the study period were obtained from the South Eastern Kenya University (SEKU) Meteorological Station.
Grasses were established from seeds in early October, 2017 prior to the onset of the short rains (October-December). Seeds were hand-sown at a constant density as monocultures along shallow ox-driven plowed microcatchments (circa 20 cm deep) at a depth of 2 cm and covered with a thin layer of soil. Spacing between the created microcatchments was around 15 cm wide. Shallow microcatchments were created to trap sufficient rainwater to prolong moisture availability and promote better germination of seeds and subsequent growth and development of the seedlings (Visser et al., 2007; Mganga et al., 2015a). Generally, the recommended seeding rate for pasture grasses indigenous to semi-arid rangelands in Kenya (i.e., 5 kg ha−1) was used for all the species (Kenya Agricultural and Livestock Research Organization, KALRO).
Data Collection and Measurements
Morphoecological plant traits (plant density, basal cover, tiller density, plant height and biomass yields) of the established grasses were measured nine (9) months, after seedling emergence. Plant densities (plants m−2) and average tiller densities per plant species were estimated in six 0.25 m2 quadrats within each plot (Cox, 1990). The height (from the base to the tip of the top leaf) of all the individual plants of the established grasses within the quadrat was measured using a 2 m ruler to the nearest centimeter. Subsequently, plant height of grass species was an average within quadrats. Percentage basal cover was estimated using the step-point method (Evans and Love, 1957). Four 10 m long line transects laid along the 10 m width were used in each of the three plots in all the three blocks. Ten measurements were taken along each transect (1 m interval) to give a total of 40 measurement points in each plot.
Thereafter, aboveground biomass production (established grasses and weeds) was determined by destructive sampling from the same six quadrats used to measure the above-mentioned parameters (i.e., plant density, basal cover, tiller density and plant height). The grasses were at a similar phenological stage (early reproductive stage). The six quadrats (0.5 × 0.5 m, 0.25 m2 area) were clipped to a stubble height of 2 cm. Freshly harvested grass yield was recorded, and then harvested biomass was placed in paper bags and oven dried at 60°C for 24 h to determine dry matter (DM) yield.
The evapotranspiration (ET) for the complete growing season of the three crops was estimated using the equation:
with precipitation (P), irrigation (I), surface run-off (S), leaching (L) and the change in soil moisture content (ΔR) according to Schoo et al. (2017). There was no grade in the experimental field and no supplemental irrigation. Consequently the surface run-off, S, and irrigation, I, were set to zero. Considering the deep ground water table (>10 m), the capillary ascent of water into the rooted soil layers was negligible. Annual rainfall received during the entire growing season was characteristically low and caused insignificant amount of leaching, L, and was also subsequently set to zero.
The water use efficiency (WUE) shoot biomass [total shoot biomass (WUEt), leaf biomass (WUEl) and stem biomass (WUEs)] were calculated as the ratio of accumulated dry matter (DM) biomass yield (Ydm) divided to cumulated evapotranspiration (ET) during the entire growing season (Kørup et al., 2018) from 1st October, 2017 until harvest 30th June, 2018. The climate data (precipitation and temperature) were collected from the local weather station
Data Processing and Statistical Analysis
The Shapiro-Wilk W test (Shapiro and Wilk, 1965) and quantile-quantile (q-q) plots were used to check for normality of the data set. Statistical analyses were performed using Software STATISTICA 10.0 StatSoft Inc. One-way ANOVA was used to test for significant differences between treatments. Tukey's honestly significance difference (HSD) post hoc test was used to separate significant differences (P < 0.05) between the grass species, at α = 0.05. All displayed results represent arithmetic means of replicates. Linear regression analysis was used to examine the relationship between the measured plant traits. Additionally, a correlation analysis between the measured morphoecological traits was conducted using R statistical environment version 4.0.3 (2020-10-10) (R Core Team, 2020) within RStudio (RStudio Team., 2018) and the correlation matrix was generated using the corrplot package (Wei et al., 2017).
Results
Rainfall and Temperature Data
Total rainfall recorded during the study period i.e., September, 2017 to August, 2018 was 326 mm. Short rains of October-December, 2017 and long rains of March-May, 2018 recorded total amounts of 105 and 198 mm, respectively (Figure 1). There were 153 rainy days in the year, with 69 and 67 rainy days for the short and long rains respectively. March 2018 (92 mm) and June 2018 (3 mm) were the wettest and driest months during the 12 months study period. Average temperature range was between 18 and 22°C. Maximum and minimum temperature ranges were between 23–28°C and 13–17°C, respectively. Highest temperatures were recorded in months before the onset of the short (October, 2017) and long (February, 2018) rainy seasons (Figure 1).
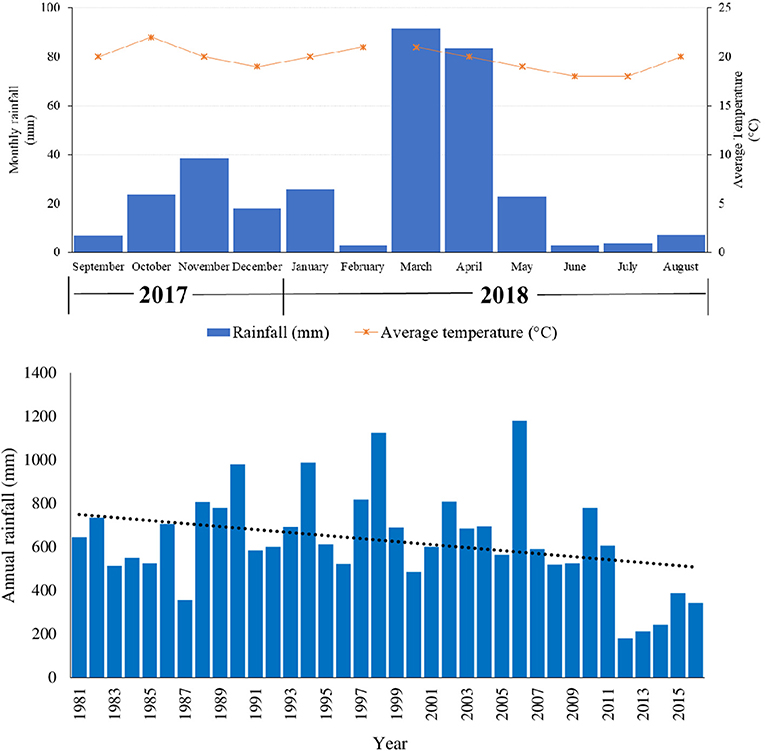
Figure 1. Monthly rainfall (mm) and average monthly temperatures (°C) during the study period and annual rainfall (1981–2016) of the study area (Sources: South Eastern Kenya University (SEKU) Meteorological Station, Kenya Meteorological Department and Kitui Meteorological Department).
Plant Morpho-Ecological Characteristics
Caryopsis weight, seed viability and the number of days for seedling emergence after sowing of the selected grass species are shown in Table 1. Enteropogon macrostachyus had the highest plant density (18 individual plants m−2). These were significantly higher compared with E. superba, which ranked lowest (10 individual plants m−2). Percent basal cover was highest in E. macrostachyus (73%), and lowest in E. superba (49%) (Figure 2). Enteropogon macrostachyus had the highest tiller density (Figure 2). Our results demonstrated a significant and positive correlation between plant densities and basal cover (R2 = 0.73). Figure 3 is a correlogram displaying the correlations (positive and negative) of the measured plant morphoecological traits of the selected perennial grasses indigenous to African rangelands. Average plant height was significantly higher in E. macrostachyus (110 cm) compared to the other established grasses, C. ciliaris (46 cm) and E. superba (76 cm), during the entire growing period. This translated to an increase in plant height of 0.41, 0.17 and 0.29 cm day−1 for E. macrostachyus, C. ciliaris and E. superba, respectively (Table 1).

Table 1. Selected characteristics of perennial African rangeland grasses used for ecological restoration.
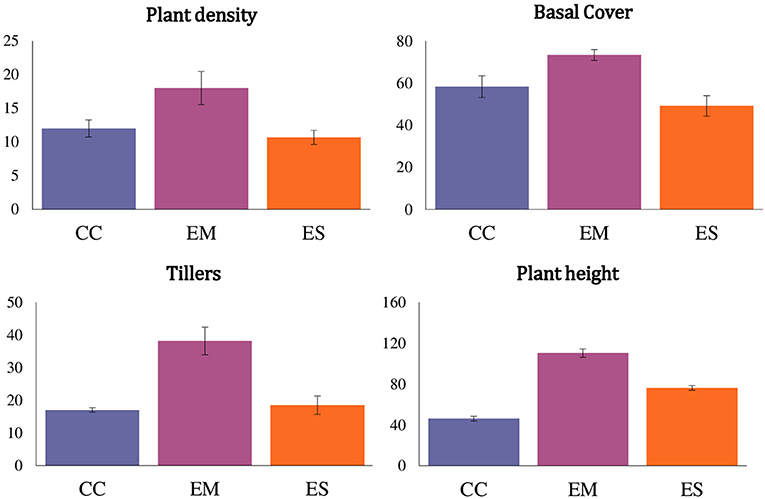
Figure 2. Plant density (plants m−2), basal cover (%), tillers (number per plant) and plant height (cm) of three African rangeland grasses used for ecological restoration 9 months after establishment. Where CC, Cenchrus ciliaris; EM, Enteropogon macrostachyus; ES, Eragrostis superba. Error bars represents standard error (± SE) of means (n = 6).
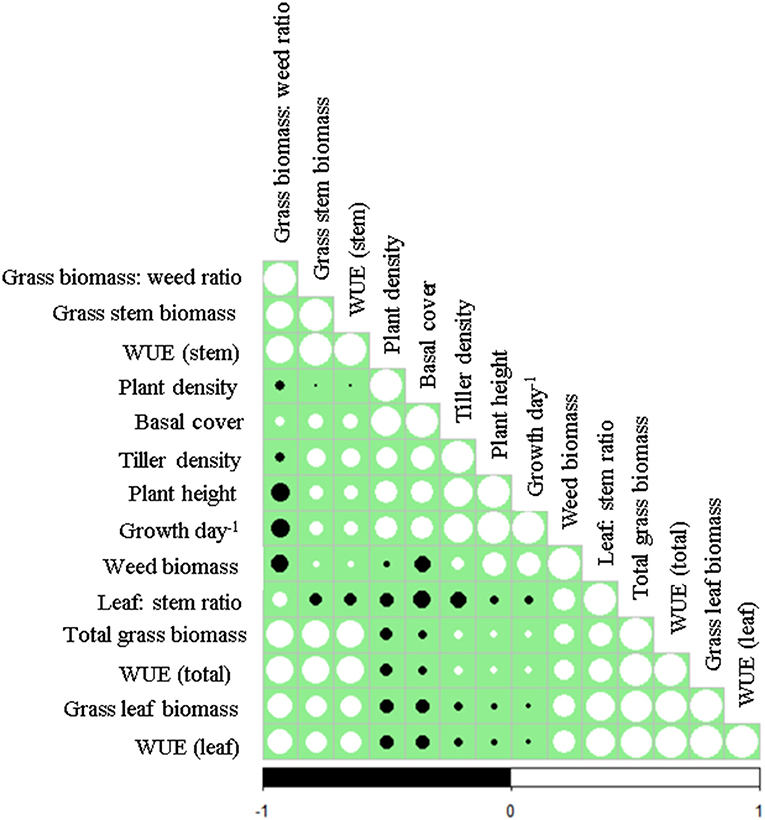
Figure 3. Correlogram of plant morphoecological traits of selected perennial grasses indigenous to African rangelands. Positive correlations are displayed in white and negative correlations in black colored circles. The size of the circles are proportional to the correlation coefficients.
Total dry matter (DM) biomass yields of the established grass species were significantly different. Nine months after establishment, E. superba (3,540 kg DM ha−1) had the highest total biomass yields. Enteropogon macrostachyus (2,600 kg DM ha−1) and C. ciliaris (2,526 kg DM ha−1), were ranked second and third, respectively (Figure 2). Eragrostis superba yielded the highest leafy biomass (2,206 kg DM ha−1), compared to C. ciliaris (1,166 kg DM ha−1) and E. macrostachyus (1,120 kg DM ha−1). This translated to significantly higher leaf-to-stem ratios in E. superba (1.59), compared to C. ciliaris (0.86) and E. macrostachyus (0.79) (Table 1). Cenchrus ciliaris significantly suppressed the weed biomass (711 kg DM ha−1) compared to E. macrostachyus (1,007 kg DM ha−1) and E. superba (1,211 kg DM ha−1) (Figure 3). Total grass biomass to weed ratio was highest in C. ciliaris (3.54 ± 0.6). Eragrostis superba (3.94 ± 0.5) and E. macrostachyus (2.58 ± 0.3) were ranked second and third, respectively (Figure 4).
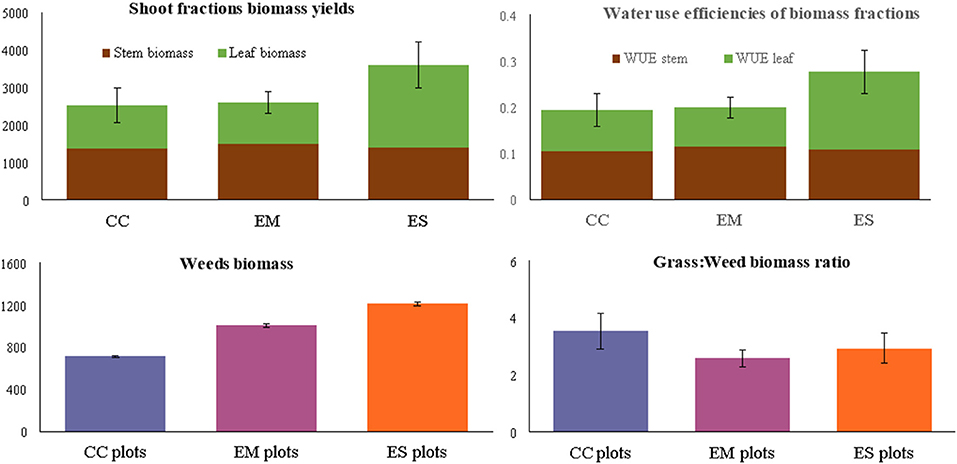
Figure 4. Biomass yields (kg DM ha−1) water use efficiencies (DM ha−1 mm−1), weed biomass (kg DM ha−1) and grass-weed biomass ratio of three African rangeland grasses used for ecological restoration 9 months after establishment. Where CC, Cenchrus ciliaris; EM, Enteropogon macrostachyus; ES, Eragrostis superba. Error bars represents standard error (± SE) of means (n = 6).
Whole Plant and Aboveground Biomass Fractions Water Use Efficiencies
Total shoot biomass WUEt of E. superba was higher (10.9 ± 1.8 kg DM ha−1 mm−1) compared to those of E. macrostachyus (7.9 ± 0.9 kg DM ha−1 mm−1) and C. ciliaris (7.7 ± 1.4 kg DM ha−1 mm−1). The WUEs of the three grasses E. superba (4.3 ± 0.7 kg DM ha−1 mm−1), E. macrostachyus (4.5 ± 0.5 kg DM ha−1 mm−1) and C. ciliaris (4.2 ± 0.8 kg DM ha−1 mm−1), were comparable. However, WUEl was significantly higher in E. superba (6.8 ± 1.5 kg DM ha−1 mm−1) compared to C. ciliaris (3.6 ± 0.8 kg DM ha−1 mm−1) and E. macrostachyus (3.4 ± 0.8 kg DM ha−1 mm−1) (Figure 4).
Discussion
The pattern of precipitation in Kenya is dominated by two mainly dry “monsoon” seasons, and two rainy seasons (bimodal pattern) associated with the movement of the Intertropical Convergence Zone (ITCZ). During the study period, the two peaks that occurred in March-May (long rains, LR) and October-December (short rains, SR) illustrated this rainfall pattern (Figure 1). Previous studies conducted in the same study area (e.g., Opiyo et al., 2011; Yageta et al., 2019) have also observed a similar rainfall pattern. Annual rainfall during the study period (326 mm) and average temperature range of between 18°C and 22°C recorded during the study period are typical of the area. Climate in semi-arid Kitui is generally hot throughout the year with temperatures ranging from 14 and 34°C and average annual precipitation is erratic and unreliable from year to year ranging between 250 and 1,050 mm (Yageta et al., 2019).
In the arid and semi-arid rangelands in Kenya, the SR are the most important and reliable (Eriksen and Lind, 2009). Consequently, in this study, sowing of the indigenous grass seeds was conducted prior to the onset of the SR. This strategy is often used in reseeding programmes in African rangelands to enhance the survival of the established grasses. This is because it ensures grasses established during the SR: (1) utilize the reliable SR for establishment, (2) experience a subsequent shorter dry spell (January-February), and (3) take advantage of LR showers between March and May. This approach contributed to the successful establishment and survival of all the seeded species in this reseeding trial. Other studies (e.g., Mganga et al., 2015a,b) have also demonstrated that timing is strongly associated with seeding success in African rangelands. Dry planting is also a traditional agronomic practice aimed at preventing critical stages of crop development from coinciding with periods of water deficit. It ensures that, the seed, once in the soil, has sufficient moisture to trigger germination and further crop development. This synchronization has contributed to the adoption of the use of indigenous grass reseeding technology for rangeland restoration among pastoralists and agropastoralists in semi-arid Kenya.
Previous studies (e.g., Xu et al., 2010; Scotton, 2019) have used plant density and cover indices as a measure of ecological restoration success. Our results demonstrate that E. macrostachyus exhibited higher plant densities and cover compared to C. ciliaris and E. superba. These observed differences could partly be linked to differences in seed size and germination (Table 1). Larger seeds of E. macrostachyus led to higher germination and faster establishment compared to C. ciliaris and E. superba. More resources in larger compared to smaller seeds enhance the successful establishment of grasses. Size of grass seed has been associated with early seedling vigor a critical phase in ecological restoration (Chang et al., 2017). Likewise, Sanderson et al. (2002) also demonstrated that seed sizes influence germination rate, emergence rate, and growth of Panicum virgatum that has been planted extensively for habitat restoration across the prairie grasslands (Chang et al., 2017). Larger seed size can be an advantage under semi-arid conditions and correlates positively with seedling survival (Veenendaal et al., 1996).
Lower plant densities and cover displayed by C. ciliaris and E. superba in this study can also be associated with seed dormancy mechanism. According to Baskin and Baskin (2004), more than 80% angiosperms occurring in arid and semi-arid drylands, produce seeds with some form of dormancy. Seed dormancy mechanism reduces recruitment failure by inhibiting germination during transient periods that are suitable for germination, but suboptimal for subsequent plant growth. This strategy optimizes the likelihood of plant survival across space and time (Childs et al., 2010). Previous studies (McIvor and Howden, 2000; Sharif-Zadeh and Murdoch, 2001; Daehler and Goergen, 2005) have demonstrated that C. ciliaris exhibits dormancy period ranging between 4 and 24 months. Similarly, Veenendaal and Ernst (1991) and Voigt and Tischler (1996) demonstrated that under field conditions, seeds of E. superba showed high seed dormancy. These previous studies strongly suggest that the observed low plant densities and cover observed in C. ciliaris and E. superba in this study can be explained by the seed dormancy mechanism. Consequently, in the context of ecological restoration, seed dormancy can hinder stand establishment and may lead to establishment failure or delay.
Higher plant densities and cover displayed by E. macrostachyus compared to C. ciliaris and E. superba suggest that E. macrostachyus is more effective than C. ciliaris and E. superba in preventing surface erosion, covering a large area of the soil, slowing down runoff and keeping the soil surface porous. Studies (e.g., Snyman and du Preez, 2005; Mohammad and Adam, 2010) have demonstrated that landscapes characterized by bare patches produced significantly more runoff than those with higher plant cover. This is because higher density of plant stems increase the hydraulic roughness for surface flow. Our results differ from those of Koech et al. (2016), who observed highest plant densities and cover in C. ciliaris compared to E. superba and E. macrostachyus, respectively. These differences can be linked to the inherent site specific edaphic (soil type, vertisol) and climatic (arid climate, annual rainfall range 220–500 mm) factors. Cenchrus ciliaris ecotypes have developed the capacity to withstand drought periods and flooding. Consequently, C. ciliaris is more likely to perform better in ecological sites dominated by heavier clay soils e.g., Vertisols, that become waterlogged (Marshall et al., 2012). Subsequently, these contradictory results demonstrate that variable outcomes of the measured plant traits are expected when the same grasses are established in different ecological sites and climatic zones.
Height is an important plant ecological strategy and major determinant of a species' ability to compete for light (Moles et al., 2009). Larger and heavier seeds of E. macrostachyus in this study provided more energy to support its early seedling growth compared to C. ciliaris and E. superba. This often translates into taller plants at subsequent phenological stages of development. Plants that achieve the greatest height in the shortest time have a competitive advantage, giving them access to most of the available light (Jefferson, 2004). Moreover, taller plants of E. macrostachyus compared to C. ciliaris and E. superba suggest its higher capacity in absorbing the kinetic energy of raindrops and subsequently protecting the ground from the explosive impact that breaks soil aggregates and weakening its structure. Rainfall simulation studies conducted in semi-arid African rangelands (e.g., Nyangito et al., 2009; Mganga et al., 2019) have demonstrated that grass height contributed significantly to reducing sediment loss in reseeded African rangelands. Moreover, taller grasses are more suitable for reducing grazing pressure because they are readily accessible to grazing herbivores. For example, cattle preferentially grazed on the taller plants as opposed to shorter plants when put out to pasture composed of layers of plants of different heights (Santos et al., 2013). Therefore, taller plants of E. macrostachyus compared to C. ciliaris and E. superba show its suitability for abating splash erosion, reducing grazing pressure and maintaining vegetation cover in reseeded African rangelands.
Enteropogon macrostachyus also displayed significantly higher tiller densities compared to C. ciliaris and E. superba. Tillers perform two significant functions during the lifespan of herbaceous plant species e.g., grasses. First, tillers in young seedlings support establishment. Rapid tillering during the early stages of grass establishment is advantageous because it increases leaf surface area for light interception. In addition to height, higher tiller densities displayed by E. macrostachyus enables it to gain competitive advantage to access most of the available light (Jewiss, 1972). Secondly, tillers are essential for the regeneration of the sward after defoliation. African rangeland grasses used for ecological restoration are prone to defoliation by grazing herbivores. This can be productive and destructive. Depending on the proportion of the leaf tissue removed from plants, defoliation reduces the photosynthetic capacity of grazed swards. Consequently, the supply of energy from photosynthesis is generally insufficient to meet the plant's demands for subsequent growth of new leaves and respiration. Mobilization of energy reserves (e.g., glucose, fructose) stored at the base of tillers supports growth of new leaves and ensures that the plant has sufficient leaf area and photosynthetic capacity to meet the energy requirements for growth and development. Subsequently, African rangeland grasses characterized by higher tiller densities, like E. macrostachyus observed in our study, have a higher capacity to regenerate and play a critical role in ecological restoration, enhancing the resilience of the ecosystems after defoliation and replenishing aboveground biomass, compared to species with much lower tiller densities e.g., C. ciliaris and E. superba. These results suggest that E. macrostachyus has evolved effective structural and functional strategies to better cope with defoliation.
Forage production is an important ecosystem service provided by African rangelands. However, biomass yield is seldomly used as a measure of successful ecological restoration. This is because of it characteristic high spatiotemporal variability. Excluding aboveground biomass production measurements could be detrimental especially when selecting grasses for the restoration of degraded African arid and semi-arid rangelands. Previous studies e.g., (Mganga et al., 2015a) have demonstrated that the choice of grass species for restoration among pastoral and agropastoral communities in east African rangelands is greatly influenced by their forage value for livestock. Our results show that E. superba was ranked lowest in the typical plant morphoecological traits used to measure restoration success (i.e., plant density and cover) compared to C. ciliaris and E. macrostachyus. However, E. superba yielded the highest dry matter biomass. This is mainly linked to its high leafy biomass fraction (Figure 4), exhibited by the higher leaf-to-stem ratios compared to C. ciliaris and E. macrostachyus. Leaf-to-stem ratio of perennial grasses provide an appropriate estimate of the proportion of structural and metabolic tissues, an important factor affecting diet selection, forage intake and quality (Annicchiarico, 2015). Reduced leaf-to-stem ratio is a major cause of the decline in forage quality with maturity. This is because leaf biomass fraction is of higher quality than stem biomass fractions, and the proportion of leaves in forage declines as the grass matures. Subsequently, higher leaf biomass fraction displayed by E. superba compared to C. ciliaris and E. macrostachyus after 9 months demonstrates its significance role in rangeland livestock production and restoration of African rangelands (Mureithi et al., 2016). Pastoral communities e.g., Pokot, Maasai, and Il Chamus have observed a higher preference for E. superba compared with C. ciliaris and E. macrostachyus, among free-ranging grazing livestock. It is no surprise that E. superba has been identified as a grass forage species to fatten livestock and improve their body condition score (BCS) among the Pokot and Il Chamus pastoral communities in Kenya (Wasonga et al., 2003; Mureithi et al., 2016).
Weeds and invasive species are a threat to the establishment of desirable plants in seed-based restoration projects Table 2 (Shackelford et al., 2019). This view is mainly attributed to the aggressive, adaptive and persistent nature of most invader plant species that colonize reseeded areas and outcompete target plant species. Yet, our finding displayed high biomass of weeds in the established E. superba and E. macrostachyus swards that also yielded higher grass biomass compared to C. ciliaris. Our results suggest a possible positive interaction between E. superba and E. macrostachyus and the weeds (Table 2). Generally, weeds are perceived to promote negative interactions leading to ecological restoration failures. However, our results demonstrated that the interaction between the target species and weeds in reseeded African rangelands could also lead to positive restoration outcomes, illustrated by high grass biomass production. Limited nutrients characteristic of African rangeland soils may have led to direct positive interactions between E. superba and weeds. This relationship is less likely to occur in nutrient rich soils where few plant species dominate in terms of biomass production and outcompete others (Cierjacks et al., 2016). However, some plant species native to African rangelands are also known to be aggressive outcompeting other plant species through various mechanisms. This characteristic is displayed by the interaction between the established C. ciliaris and weeds. Significantly lower biomass of weeds in C. ciliaris sward demonstrates its competitive and aggressive nature. Our results conform well with previous studies (Marshall et al., 2012; Bebawi et al., 2013; de Albuquerque et al., 2019) that have also displayed the aggressive nature of C. ciliaris. African foxtail grass-dominated rangeland sites exhibit fewer herbaceous species than did non-African foxtail grass sites, and species richness is negatively associated with increasing C. ciliaris biomass (Jackson, 2005). This can partly be attributed to the allelopathic nature of C. ciliaris (Jackson 2005; Mganga et al., 2015b) which can also be used as a biological method to control weeds in reseeded landscapes and a rationale for ecological restoration in African rangelands where C. ciliaris is native.
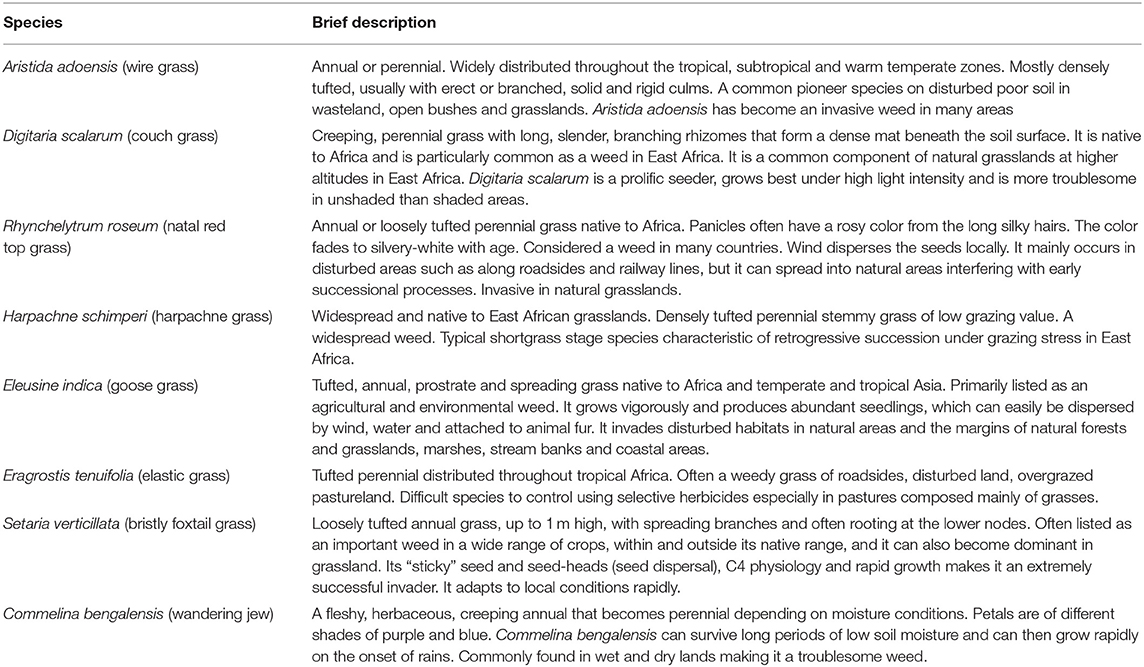
Table 2. Brief description of some of weed species occurring in the reseeded areas as described in literature.
Shoot biomass is a robust parameter for indicating WUE (Mårtensson et al., 2017). Three African rangeland grasses established in this study displayed comparable WUEt that ranged between 7 and 11 kg DM ha−1 mm−1. This is within range of WUEs of other perennial African rangeland grasses established under different water regimes and environments. For example, six perennial African rangeland grasses (C. ciliaris, E. superba, C. roxburghiana, C. gayana, E. macrostachyus, and Sorghum sudanense) established under natural rainfall in an arid rangeland in Kenya, exhibited WUEs of between 2 and 19 kg DM ha−1 mm−1 (Koech et al., 2015). Other studies e.g., Snyman (2009) have reported WUEs of between 2 and 5 kg DM ha−1 mm−1 in South African rangelands in good (T. triandra dominated), moderate (genus Eragrostis dominated) and poor (Tragus koelerioides and Aristida congesta dominated) condition. Under different irrigation regimes, WUE's of five African rangeland grasses (C. ciliaris, C. dactylon, Digitaria eriantha, P. maximum, Pennisetum clandestinum) ranged between 7 and 22 kg DM ha−1 mm−1 Marais et al. (2006). Our results and those from other studies demonstrate that perennial African rangeland grasses exhibit a wide range of WUEs when established in different environments and soil water regimes. Higher WUEl of E. superba is indicative of its capacity to utilize available water more efficiently to produce more leaf biomass compared to C. ciliaris and E. superba. Considering that African rangelands occupy landscapes characterized by rainfall variability and droughts, careful selection of plant species that maximize WUE is important for successful ecological restoration using indigenous grass reseeding.
Conclusions
Perennial grasses indigenous to African rangelands (Cenchrus ciliaris, Enteropogon macrostachyus, and Eragrostis superba) displayed unique plant morphoecological attributes that make them suitable for ecological restoration in semi-arid African rangelands. Plant densities, basal cover and tiller densities, were highest in E. macrostachyus. This shows its potential role in restoring vegetation cover in denuded African semi-arid rangeland landscapes. Eragrostis superba displayed high biomass productivity and water use efficiencies. These characteristic features suggest its suitability for livestock production and capacity to maximize water availability to enhance rangeland primary productivity. Cenchrus ciliaris aggressive nature contributed significantly to suppressing weed biomass. These findings offers valuable insights and serve as a first step in developing a deeper understanding of species-level agronomic and growth traits of three perennial grasses commonly used for restoring degraded semi-arid African rangelands. Careful selection of grasses to maximize on species specific traits is critical to achieve desirable seed-based restoration outcomes.
Data Availability Statement
The raw data supporting the conclusions of this article will be made available by the authors, without undue reservation.
Author Contributions
KM, FS, and NM conceived the ideas and designed methodology. EK, NK, GM, TK, and AN collected the data. KM, LB, and SW analyzed the data. KM and LB led the writing of the manuscript. All authors reviewed and edited the manuscript.
Funding
Financial support for this research project was provided by the NWO-WOTRO Netherlands Organization for Scientific Research and Science for Global Development under the Food and Business Applied Research Fund (ARF), 2016. Budget No. 3350, W 08.270.348. The paper was finalized with the support of the Green Future Farming Program.
Conflict of Interest
The authors declare that the research was conducted in the absence of any commercial or financial relationships that could be construed as a potential conflict of interest.
Acknowledgments
Thanks to the staff at the South Eastern University research farm where this reseeding trial was conducted. We dedicate this publication to our co-author NM who passed on during the implementation of this research project. See research project links here https://www.nwo.nl/en/projects/w-08270348 and https://knowledge4food.net/research-project/arf3-kenya-rofip/.
References
Annicchiarico, P. (2015). Alfafa forage yield and leaf/stem ration: narrow-sense heritability, genetic correlation and parent selection procedures. Euphytica 205, 409–420. doi: 10.1007/s10681-015-1399-y
Baskin, J. M., and Baskin, C. C. (2004). A classification system for seed dormancy. Seed Sci. Res. 14, 1–16. doi: 10.1079/SSR2003150
Bebawi, F. F., Campbell, S. D., and Mayer, R. J. (2013). Can competition with pasture be used to manipulate bellyache bush (Jatropha gossypiifolia L.) population biology? Rangeland J. 35, 393–401. doi: 10.1071/RJ13011
Chang, H., Alexander, H. M., Mutegi, E., and Snow, A. A. (2017). Habitat restoration and native grass conservation: a case study of switchgrass (Panicum virgatum). Restor. Ecol. 26, 506–515. doi: 10.1111/rec.12599
Childs, D. Z., Metcalf, C. J. E., and Rees, M. (2010). Evolutionary bet-hedging in the real world: empirical evidence and challenges revealed by plants. Proc. R Soc. B 277, 3055–3064. doi: 10.1098/rspb.2010.0707
Cierjacks, A., Pommeranz, M., Schulz, K., and Almeida-Cortez, J. (2016). Is crop yield related to weed species diversity and biomass in coconut and banana fields of northeastern Brazil? Agric. Ecosyst. Environ. 220, 175–183. doi: 10.1016/j.agee.2016.01.006
Cincotta, R. P., Wisnewski, J., and Engelman, R. (2000). Human population in the biodiversity hotspots. Nature 404, 990–992. doi: 10.1038/35010105
Daehler, C. C., and Goergen, E. M. (2005). Experimental restoration of an indigenous Hawaiian grassland after invasion by buffel grass (Cenchrus ciliaris). Restor. Ecol. 13, 380–389. doi: 10.1111/j.1526-100X.2005.00047.x
de Albuquerque, F. S., Maćias-Rodríguez, M. Á., Búrquez, A., and Astudillo-Scalia, Y. (2019). Climate change and the potential of buffelgrass (Cenchrus ciliaris L., Poaceae) in biotic communities of southwest United States and northern Mexico. Biol. Invasions 21, 3335–3347. doi: 10.1007/s10530-019-02050-5
Eriksen, S., and Lind, J. (2009). Adaptation as a political process: adjusting to drought and conflict in Kenya's drylands. Environ. Manage. 43, 817–835. doi: 10.1007/s00267-008-9189-0
Evans, R. A., and Love, R. M. (1957). The step-point method of sampling. A practical tool in range research. J. Range Manage. 10, 208–212. doi: 10.2307/3894015
Homewood, K. M. (2004). Policy, environment and development in African rangelands. Environ. Sci. Policy 7, 125–143. doi: 10.1016/j.envsci.2003.12.006
Jackson, J. (2005). Is there a relationship between herbaceous species richness and buffel grass (Cenchrus ciliaris)? Aust. Ecol. 30, 505–517. doi: 10.1111/j.1442-9993.2005.01465.x
Jefferson, L. V. (2004). Implications of plant density on the resulting community structure of mine site land. Restor. Ecol. 12, 429–438. doi: 10.1111/j.1061-2971.2004.00328.x
Jewiss, O. R. (1972). Tillering in grasses – its significance and control. Grass Forage Sci. 27, 65–82. doi: 10.1111/j.1365-2494.1972.tb00689.x
Jones, M. B., Finnan, J., and Hodkinson, T. R. (2015). Morphological and physiological traits or higher biomass production in perennial rhizomatous grasses grown on marginal land. Glob. Change Biol. Bioenergy 7, 375–385. doi: 10.1111/gcbb.12203
Kiniry, J. R., and Kim, S. (2020). A review of modeled water use efficient of highly productive perennial grasses useful for bioenergy. Agronomy 10:328. doi: 10.3390/agronomy10030328
Kinyua, D., McGeoch, L. E., Georgiadis, N., and Truman, P. Y. (2010). Short-term and long-term effects of soil ripping, seeding and fertilisation on the restoration of a tropical rangeland. Restor. Ecol. 18, 226–233. doi: 10.1111/j.1526-100X.2009.00594.x
Koech, O. K., Kinuthia, R. N., Karuku, G. N., Mureithi, S. M., and Wanjogu, R. (2015). Water use efficiency of six rangeland grasses under varied soil moisture content levels in the arid Tana River county, Kenya. Afr. J. Environ. Sci. Tech. 9, 632–640. doi: 10.5897/AJEST2015.1917
Koech, O. K., Kinuthia, R. N., Karuku, G. N., Mureithi, S. M., and Wanjogu, R. K. (2016). Irrigation levels affects biomass yields and morphometric characteristics of range grasses in arid rangelands of Kenya. Springer Plus 5:1640. doi: 10.1186/s40064-016-3309-8
Kørup, K., Lærke, P. E., Baadsgaard, H., Andersen, M. N., Kristensen, K., Münnich, C., et al. (2018). Biomass production and water use efficiency in perennial grasses during and after drought stress. Glob. Change Biol. Bioenergy 10, 12–27. doi: 10.1111/gcbb.12464
Ludwig, F., De Kroon, H., and Prins, H. H. T. (2008). Impacts of savanna trees on forage quality for a large African herbivore. Oecologia 155, 487–496. doi: 10.1007/s00442-007-0878-9
Mårtensson, L.-M., Carlsson, G., Prade, T., Kørup, K., Lærke, P. E., and Jensen, E. S. (2017). Water use efficiency and shoot biomass production under water limitation is negatively correlated to the discrimination against 13C in the C3 grasses Dactylis glomerata, Festuca arundinacea and Phalaris arundinacea. Plant Physiol. Biochem. 113, 1–5. doi: 10.1016/j.plaphy.2017.01.021
Macandza, V. A., Owen-Smith, N., and Cross, P. C. (2004). Forage selection by African buffalo in the late dry season in two landscapes. South Afr. J. Wildlife Res. 34, 113–121. Available online at: https://hdl.handle.net/10520/EJC117195
Mapinduzi, A. L., Oba, G., Weladji, R. B., and Colman, J. E. (2003). Use of indigenous ecological knowledge of the Maasai pastoralists for assessing rangeland biodiversity in Tanzania. Afr. J. Ecol. 41, 329–336. doi: 10.1111/j.1365-2028.2003.00479.x
Marais, D., Rethman, N., and Annandale, J. (2006). Dry matter yield and water use efficiency of five perennial subtropical grasses at four levels of water availability. Afr. J. Range Forage Sci. 23, 165–169. doi: 10.2989/10220110609485900
Marshall, V. M., Lewis, M. M., and Ostendorf, B. (2012). Buffel grass (Cenchrus ciliaris) as an invader and threat to biodiversity in arid environments: a review. J. Arid Environ. 78, 1–12. doi: 10.1016/j.jaridenv.2011.11.005
McIvor, J. G., and Howden, S. M. (2000). Dormancy and germination characteristics of herbaceous species in the seasonally dry tropics of northern Australia. Austr. Ecol. 25, 213–222. doi: 10.1046/j.1442-9993.2000.01026.x
Mganga, K. Z., Musimba, N. K. R., and Nyariki, D. M. (2015b). Competition indices of three perennial grasses used to rehabilitate degraded semi-arid rangelands in Kenya. Rangeland J. 37, 489–495. doi: 10.1071/RJ15023
Mganga, K. Z., Musimba, N. K. R., Nyariki, D. M., Nyangito, M. M., and Mwang'ombe, A. W. (2015a). The choice of grass species to combat desertification in semi-arid rangelands is greatly influenced by their forage value for livestock. Grass Forage Sci. 70, 161–167. doi: 10.1111/gfs.12089
Mganga, K. Z., Nyariki, D. M., Musimba, N. K. R., and Mwang'ombe, A. W. (2019). “Indigenous grasses for rehabilitating degraded african drylands,” in Agriculture and Ecosystem Resilience in Sub Saharan Africa. Climate Change Management, eds Y. Bamutaze, S. Kyamanywa, B. Singh, G. Nabanoga, and R. Lal (Cham: Springer), 53–68. doi: 10.1007/978-3-030-12974-3_3
Mnene, W. N., Hanson, J., Ekaya, W. N., Kinyamario, J. I., Mweki, P., Lall, G., et al. (2005). Genetic variation between ecotypic populations of Chloris roxburghiana grass detected through RAPD analysis. Afr. J. Range Forage Sci. 22, 107–115. doi: 10.2989/10220110509485868
Mohammad, A. G., and Adam, M. A. (2010). The impact of vegetative cover type on runoff and soil erosion under different land uses. Catena 81, 97–103. doi: 10.1016/j.catena.2010.01.008
Moles, A. T., Warton, D. I., Warman, L., Swenson, N. G., et al. (2009). Global patterns in plant height. J. Ecol. 97, 923–932. doi: 10.1111/j.1365-2745.2009.01526.x
Mureithi, S. M., Verdoodt, A., Njoka, J. T., Gachene, C. K. K., and van Ranst, E. (2016). Benefits derived from rehabilitating a degraded semi-arid rangeland in communal enclosures, Kenya. Land Degrad. Dev. 27, 1853–1862. doi: 10.1002/ldr.2341
Nyangito, M. M., Musimba, N. K. R., and Nyariki, D. M. (2009). Hydrologic properties of grazed perennial swards in semiarid southeastern Kenya. Afr. J. Environ. Sci. Tech. 3, 026–033. doi: 10.5897/AJEST08.181
Opiyo, F. E. O., Mureithi, S. M., and Ngugi, R. K. (2011). The influence of water availability on pastoralist's resource use in Mwingi and Kitui districts in Kenya. J. Hum. Ecol. 35, 43–52. doi: 10.1080/09709274.2011.11906389
Poorter, H., Niklas, K. J., Reich, P. B., Oleksyn, J., Poot, P., and Mommer, L. (2012). Biomass allocation to leaves, stems and roots: meta-analyses of interspecific variation and environmental control. New Phytol. 193, 30–50. doi: 10.1111/j.1469-8137.2011.03952.x
R Core Team (2020). R: A Language and Environment for Statistical Computing. Vienna, Austria: R Foundation for Statistical Computing.
Sanchez, P. A. (2002). Ecology – soil fertility and hunger in Africa. Science 295, 2019–2220. doi: 10.1126/science.1065256
Sanderson, M. A., Skinner, R. H., and Elwinger, G. F. (2002). Seedling development and field performance of prairiegrass grazing bromegrass and orchardgrass. Crop Sci. 42, 224–230. doi: 10.2135/cropsci2002.2240
Santos, M. E. R., Silveira, M. C. T., Gomes, V. M., da Fonseca, D. M., Sousa, B. M. L., and Santos, A. D. (2013). Pasture height at the beginning of deferment as a determinant of signal grass structure and potential selectivity by cattle. Acta Scientiarum Anim. Sci. 35, 379–385. doi: 10.4025/actascianimsci.v35i4.20421
Schmitt, C. B., Kisangau, D., and Matheka, K. W. (2019). Tree diversity in a human modified riparian forest landscape in semi-arid Kenya. Forest Ecol. Manage. 433, 645–655. doi: 10.1016/j.foreco.2018.11.030
Schoo, B., Wittich, K. P., Böttcher, U., Kage, H., and Schittenhelm, S. (2017). Drought tolerance and water-use efficiency of biogas crops: a comparison of cup plant, maize and lucerne-grass. J. Agron. Crop Sci. 203, 117–130. doi: 10.1111/jac.12173
Scotton, M. (2019). Mountain grassland restoration: effects of sowing rate, climate and soil on plant density and cover. Sci. Total Environ. 651, 3090–3098. doi: 10.1016/j.scitotenv.2018.10.192
Shackelford, N., Murray, S. M., Bennett, J. R., Lilley, P. L., Starzomski, B. M., and Standish, R. J. (2019). Ten years of pulling: ecosystem recovery after long-term weed management in Garry oak savanna. Conserv. Sci. Pract. 1:e92. doi: 10.1111/csp2.92
Shapiro, S. S., and Wilk, M. B. (1965). An analysis of variance test for normality (complete samples). Biometrika 52, 591–611. doi: 10.1093/biomet/52.3-4.591
Sharif-Zadeh, F., and Murdoch, A. J. (2001). The effects of temperature and moisture on after-ripening of Cenchrus ciliaris seeds. J. Arid Environ. 49, 823–831. doi: 10.1006/jare.2001.0820
Snyman, H. A. (2009). Root studies of grass species in a semi-arid South Africa along a degradation gradient. Agric. Ecosyst. Environ. 130, 100–108. doi: 10.1016/j.agee.2008.12.003
Snyman, H. A., and du Preez, C. C. (2005). Rangeland degradation in semi-arid South Africa—II: influence on soil quality. J. Arid Environ. 60, 483–507. doi: 10.1016/j.jaridenv.2004.06.005
Van den Berg, L., and Kellner, K. (2005). Restoring degraded patches in a semi-arid rangeland of South Africa. J. Arid Environ. 61, 497–511. doi: 10.1016/j.jaridenv.2004.09.024
Veenendaal, E. M., and Ernst, W. H. O. (1991). Dormancy patterns in accession of caryopses from savanna grass species in South Eastern Botswana. Acta Bot. Neerlandica 40, 297–309. doi: 10.1111/j.1438-8677.1991.tb01560.x
Veenendaal, E. M., Ernst, W. H. O., and Modise, G. S. (1996). Reproductive effort and phenology of seed production of savanna grasses with different growth form and life history. Vegetatio 123, 91–100. doi: 10.1007/BF00044891
Visser, N., Morris, C., Hardy, M. B., and Botha, J. C. (2007). Restoring bare patches in the Nama-Karoo of South Africa. Afr. J. Range Forage Sci. 24, 87–96. doi: 10.2989/AJRFS.2007.24.2.5.159
Voigt, P. W., and Tischler, C. R. (1996). Effect of seed treatment on germination and emergence of 3 warm-season grasses. J. Range Manag. 50, 170–174. doi: 10.2307/4002376
Wasonga, V. O., Ngugi, R. K., and Kitalyi, A. (2003). Traditional range condition and trend assessment: lessons from Pokot and Il Chamus pastoralists of Kenya. Anthropologist 5, 79–87. doi: 10.1080/09720073.2003.11890783
Wei, T., Simko, V., Levy, M., Xie, Y., Jin, Y., and Zemla, J. (2017). Corrplot Package: Visualization of a Correlation Matrix. Available online at: https://github.com/taiyun/corrplot (accessed November 20, 2020).
Xu, Z., Wan, S., Zhu, G., Ren, H., and Han, X. (2010). The influence of historical land use and water availability on grassland restoration. Restor. Ecol. 18, 217–225. doi: 10.1111/j.1526-100X.2009.00595.x
Keywords: biomass fractions, drylands, forage grasses, leaf:stem ratio, desertification, nature based solution
Citation: Mganga KZ, Kaindi E, Ndathi AJN, Bosma L, Kioko T, Kadenyi N, Musyoki GK, Wambua S, van Steenbergen F and Musimba NKR (2021) Plant Morphoecological Traits, Grass-Weed Interactions and Water Use Efficiencies of Grasses Used for Restoration of African Rangelands. Front. Ecol. Evol. 8:613835. doi: 10.3389/fevo.2020.613835
Received: 03 October 2020; Accepted: 08 December 2020;
Published: 07 January 2021.
Edited by:
Miriam Muñoz-Rojas, University of New South Wales, AustraliaReviewed by:
Thomas Monaco, Forage and Range Research, United StatesMoses Nyangito, University of Nairobi, Kenya
Stephen M. Mureithi, University of Nairobi, Kenya
Copyright © 2021 Mganga, Kaindi, Ndathi, Bosma, Kioko, Kadenyi, Musyoki, Wambua, van Steenbergen and Musimba. This is an open-access article distributed under the terms of the Creative Commons Attribution License (CC BY). The use, distribution or reproduction in other forums is permitted, provided the original author(s) and the copyright owner(s) are credited and that the original publication in this journal is cited, in accordance with accepted academic practice. No use, distribution or reproduction is permitted which does not comply with these terms.
*Correspondence: Kevin Z. Mganga, kmganga@seku.ac.ke; kevin.mganga@helsinki.fi