- 1Programa de Pós-Graduação em Biodiversidade Tropical, Universidade Federal do Amapá (UNIFAP), Macapá, Brazil
- 2Programa de Pós-Graduação em Biodiversidade e Meio Ambiente, Universidade Federal da Grande Dourados (UFGD), Dourados, Brazil
- 3Ecosystems and Environment Research Centre, School of Science, Engineering and Environment, University of Salford, Salford, United Kingdom
- 4Programa de Pós-Graduação em Ecologia, Universidade Federal do Rio de Janeiro (UFRJ), Rio de Janeiro, Brazil
- 5Programa de Pós-Graduação em Biologia Animal, Departamento de Ecologia, Zoologia e Genética, Instituto de Biologia, Universidade Federal de Pelotas, Pelotas, Brazil
- 6Laboratório de Mamíferos, Instituto de Pesquisas Científicas e Tecnológicas do Estado do Amapá (IEPA), Macapá, Brazil
- 7Curso de Bacharelado em Medicina Veterinária, Universidade Federal de Roraima (UFRR), Boa Vista, Brazil
- 8Companhia Independente de Policiamento Ambiental (CIPA) da Polícia Militar de Roraima (PMRR), Boa Vista, Brazil
Across the globe, millions of hectares of native vegetation have been replaced by commercial plantations, with negative consequences for biodiversity. The effects of the replacement of native vegetation with commercial plantations on the functional and phylogenetic diversity of bat assemblages remain understudied, and most studies have focused exclusively on the taxonomic component of diversity. Here, we investigate how the replacement of natural savannahs by acacia plantations affects the α- and β-diversity of bat assemblages. We sampled bats, using mist-nets at ground level, in natural forest, savannah areas and acacia plantations, in the Lavrados de Roraima in the northern Brazilian Amazon. Our results show that, in general, acacia is less diverse than native forests in terms of taxonomic and functional diversity, and is also less taxonomically diverse than the savannah matrix which it substitutes. The observed patterns of α- and β-diversity found in the present study are in large part driven by the superabundance of one generalist and opportunistic species, Carollia perspicillata, in the acacia plantations. Taken together, our results show that the replacement of areas of natural savannah by acacia plantations causes a regional loss in diversity across all diversity dimensions: taxonomic, functional and phylogenetic. However, further studies are required to fully understand the ecological and conservation implications of this landscape change.
Introduction
Across the globe, millions of hectares of native vegetation have been replaced by commercial plantations, such as rice, soybean, corn, wheat, oil palm, eucalyptus, and acacia (Lepers et al., 2005; Phalan et al., 2013; Fernandes et al., 2016; Carvalho et al., 2019). In recent decades, most of these anthropogenic landscape changes have been concentrated in tropical regions, where increasing demand for land for commercial plantations and livestock production are the key drivers of habitat loss (Boucher et al., 2011; Carvalho et al., 2019; Colli et al., 2020; Rajão et al., 2020). Brazil is one of the countries in which deforestation and the conversion of natural landscapes into agricultural landscapes has been most pronounced (Curtis et al., 2018). For example, among Brazilian biomes, the Atlantic Forest retains just 28% of its original forest cover, of which most is secondary forest and highly fragmented (Rezende et al., 2018), the Cerrado retains less than 54% of its original cover (Strassburg et al., 2017), and the Brazilian Amazon has lost 20% of its original forest cover (Cruz et al., 2020). Beyond these 20%, in recent years increasing areas of savannah within the Brazilian Amazon have been transformed into soybean, corn, eucalyptus, and acacia plantations (Mustin et al., 2017; Carvalho et al., 2019). The conversion of natural to human-modified landscapes, together with the associated fragmentation of habitats, can have irreversible negative consequences for biodiversity.
Habitat loss and fragmentation have been shown to have negative effects on tropical vertebrates (Willig et al., 2007; Coelho et al., 2014; Meyer et al., 2016; Saccol et al., 2017; Ramos Pereira et al., 2018; Aninta et al., 2019; Palmeirim et al., 2020). For bats, habitat conversion leads to decreased availability of roosts and food resources, which will affect their presence, abundance, and behaviour (Jones et al., 2009; Meyer et al., 2016). The effects of habitat conversion also lead to a decrease in the genetic diversity of populations (Collevatti et al., 2020), and loss of richness, taxonomic, functional, and phylogenetic diversity (Ramos Pereira et al., 2018; Aninta et al., 2019). However, few studies have evaluated the effect of the conversion of natural vegetation to tree plantations, such as eucalyptus, on bat assemblages (see Meyer et al., 2016; Farneda et al., 2020; Mendes and Srbek-Araujo, 2020 for a review). Those studies carried out to date have found lower species richness and diversity of bats in eucalyptus plantations than in unlogged forests (Barlow et al., 2007) and natural forests (Pina et al., 2013). As these flying mammals play a key role in maintaining forests and non-forest ecosystems through seed dispersal and insect suppression, the loss of bat species has major consequences for the functioning of these ecosystems (Treitler et al., 2016; Laurindo et al., 2019). In general, studies carried out in the Neotropics, including Brazil, show that gleaning animalivorous bats are negatively affected by landscape changes such as habitat fragmentation, whereas frugivorous and nectarivorous bats may respond positively to such changes (Delaval and Charles-Dominique, 2006; Willig et al., 2007; Meyer and Kalko, 2008; Farneda et al., 2015, 2020; Meyer et al., 2016; Oliveira et al., 2017). These differential effects on different groups of bats have manifested as changes in species composition in human-modified landscapes, with losses of species, functions and lineages (Aninta et al., 2019; Farneda et al., 2020).
The consequences of changes in natural habitats can be even more detrimental to biodiversity and ecosystem services in savannahs and open-canopy woodlands (Veldman et al., 2015), such as the Amazonian savannahs, which are not as effectively protected as other Amazonian habitats (Overbeck et al., 2015; Carvalho et al., 2019). Amazonian savannahs are a natural mosaic of vegetation with forest patches, gallery forests and palm forests of different sizes and structures immersed in a matrix of savannah (Mustin et al., 2017). So far, at least 100 bat species have been recorded in these savannahs (Aguirre, 2002; Bernard and Fenton, 2002; Loayza and Loiselle, 2009; Silva et al., 2013; Carvalho et al., 2018; Lim and Lee, 2018), with studies showing that this ecosystem has the same species richness, but higher abundance of bats than continuous forest (Bernard and Fenton, 2002; Carvalho et al., 2018). In addition, in this natural mosaic of vegetation, bats have high mobility and readily traverse the savannah matrix (Bernard and Fenton, 2003; Loayza and Loiselle, 2009), which may reflect the long history of natural fragmentation in this landscape, such that species are adapted to the configuration of forest patches in a mosaic of savannahs (Bernard and Fenton, 2003). However, very little is known regarding the effects of landscape change on any taxa in the Amazonian savannahs, and those studies that have been carried out have focused exclusively on taxonomic diversity (e.g., Piña et al., 2019), despite the availability of tools to estimate the taxonomic, functional and phylogenetic dimensions of both α- and β-diversity (Moreno et al., 2018). Indeed, for vertebrates, studies have been limited to medium and large-sized mammals, and have shown that richness and diversity are not impacted (Coelho et al., 2014; Piña et al., 2019). To the best of our knowledge, the present study is the first to examine the impacts of landscape change on bat assemblages in the Amazonian savannahs.
Here, we investigate how the replacement of natural savannah by acacia plantations affects the α- and β-diversity of bat assemblages in the northern Brazilian Amazon. Specifically, we (i) compare bat species richness and the taxonomic, functional and phylogenetic diversity dimensions between natural areas of forest and savannah and acacia plantations. In addition, we (ii) assess between-habitat differences in bat assemblage structure and species composition, and (iii) estimate the contribution of the turnover and nestedness component of β-diversity for taxonomic (TβD), functional (FβD) and phylogenetic (PβD) β-diversity among the three habitat types. We expect acacia plantations to have lower taxonomic, functional and phylogenetic diversity than natural forest and savannah areas, due to the loss of species, functions and lineages, as has been previously shown with the replacement of natural vegetation by commercial plantations in the Cerrado (Pina et al., 2013; Ramos Pereira et al., 2018). Bats have high mobility and readily traverse the savannah matrix in Amazonian savannahs (Bernard and Fenton, 2003; Loayza and Loiselle, 2009), making forest patches and savannahs taxonomically similar (e.g., Bernard and Fenton, 2002). Thus, we expect that acacia plantations will change the permeability of the savannah matrix that they replace, and that as such bat assemblage composition will be altered in such a way that taxonomic, functional and phylogenetic β-diversity will be higher between acacia plantations and forest than between savannah and forest. Due to the loss of species, functions and lineages, regarding β-diversity we expect the species richness difference component to be of greater importance than species replacement when the landscape is changed from savannah to acacia plantations.
Materials and Methods
Study Area
The study was carried out in the Serra da Lua region, municipality of Bonfim, state of Roraima, in the northern Brazilian Amazon (2°48’24.06″N and 60°21’12.85″W; 2°42’3.77″N and 60°21’18.39″W; Supplementary Figure S1). The average annual temperature in the region is 26°C, and precipitation ranges from 1,700 to 2,000 mm year–1 (Barbosa, 1997). The Serra da Lua region is part of the “Lavrados de Roraima” (also known as the Guyana savannahs), the second largest block of Amazonian savannah (Carvalho and Mustin, 2017). This region is currently threatened by the replacement of its natural habitats with commercial tree plantations, plantations of grains and pulses, pastures for domestic cattle, uncontrolled fires, and mining (Barbosa et al., 2007; Carvalho and Mustin, 2017; Carvalho et al., 2019). The study region is a mosaic of planted forests of Acacia mangium, remnants of “lavrado” (local name for savannah), forest patches and gallery forests (Supplementary Figure S1). Between the late 1980s and early 1990s, most of the natural savannahs in the area were cleared and converted to cattle pasture. However, after 8–10 years the areas were abandoned and monocultures of A. mangium were established to supply raw material to both the sawn products industry, and to energy and paper production (Toledo and Nascimento, 2019).
Our data were collected in permanent plots in four modules, established according to the RAPELD standard (Rapid Assessment Protocol for Long-term Ecological Studies; Magnusson et al., 2005) as part of the long-term ecological research of the Biodiversity Research Program (PPBio)1. Each module was comprised of two parallel trails of 5 km, 1 km apart from each other. Each trail contained five plots of 2 × 250 m (0.05 ha), totalling 10 plots per module. Out of the total of 40 permanent plots in our study area, we chose six plots in forest, four in savannah and six in acacia plantations (Supplementary Figure S1), based on maximum possible variation in age and vegetation structure within habitat types. Two additional plots in savannah were set up outside the modules, as there was no possibility of access to the savannah plots within the modules during the time this study was carried out, due to poor road and trail conditions. Therefore, a total of 18 plots were sampled, six in each habitat type: forest, savannah and acacia plantation. The age of planting in the plots in acacia plantations varied between 11 and 17 years (mean = 13.4 ± SD 2.30).
The forest is the most tree species rich habitat (286 species) in the study area and also the most complex in structure, with a high density of trees (∼3,500 stems > 1 cm diameter ha–1), widely variable in stem size, from 1 to 96 cm (mean ∼6 cm), and canopy height around 20 m with large trees of several species (> 30 cm in diameter) reaching up to 40 m in height. The acacia plantations have around six times fewer tree species (48 species) than natural forests and are less heterogeneous, with tree density (∼1,550 stems ha–1) half that of natural forests and stem diameter being less variable, ranging from 1 to 57 cm (mean ∼14 cm). The canopy is almost exclusively made up of A. mangium and is lower than the native forests (∼15 m), with few trees in the older plantations reaching 30 m in height. Forest pioneer species such as Cecropia spp. (Moraceae) and Vismia spp. (Hypericaceae) colonise the understorey of the plantations. The grassy stratum still remains in the understorey of plantations with an open canopy, but Piper spp. (Piperaceae) and several herbaceous species are abundant under more closed canopies. The savannah has a dominant grassy vegetation stratum interspersed with trees which are generally small in diameter (1–53 cm, mean ∼7 cm), short in stature (mean of ∼3 m and a few trees up to 10 m) and stem density is around 1,800 stems ha–1. More detailed botanical data for the study plots used in this study are available in Toledo and Nascimento (2019).
Bat Capture
We captured bats during one night in each plot, between July and August 2017, using nine mist-nests (12 × 3 m; 14 mm mesh size) set in the understorey. This sampling period fell within the rainy season, the time of year with the highest bat capture rates in the northern Brazilian Amazon (Carvalho et al., 2018). The mist-nets were set up at dusk, around 18:00 h, and were kept open until 00:00 h. The total sampling effort, calculated according to Straube and Bianconi (2002), was 34,992 m2∗h, being 11,664 m2∗h for each of the three vegetation types sampled.
Captured bats were removed from the nests and placed in cotton bags for later identification. Bats were sexed, weighed and identified in the field according to Lim and Engstrom (2001); Gardner (2008), López-Baucells et al. (2016), and Reis et al. (2017). Species nomenclature follows Garbino et al. (2020). For the genus Pteronotus, we consider all individuals as Pteronotus spp. because we cannot be certain if the species are P. alinotus or P. rubiginosus, recently recognised for this region (Thoisy et al., 2014; Pavan et al., 2018). All applicable institutional and/or national guidelines for the care and use of animals were followed. Also, the fieldwork, handling, and processing of all captures followed the guidelines of the American Society of Mammalogists (Sikes et al., 2016).
Functional Traits
From our capture data and the literature, we compiled data on four functional traits to estimate α- and β-functional diversity: (1) body mass, (2) diet, (3) vertical stratification, and (4) wing morphology (Supplementary Table S1). Body mass was based on the average body mass of each species captured in each habitat, excluding pregnant females and juveniles. For species that had no more than 10 individuals, we supplemented the information with body mass data from bats captured in other locations in the northern Brazilian Amazon (William Carvalho, unpublished data). Body mass was log-transformed to normalise values. Information on diet was obtained from the Ecological Register database (ecoregister.org; accessed on 15 January 2019—Alroy, 2017). Thus, we classified the species according to their specific diet as carnivores, frugivores, insectivores, nectarivores, omnivores, or sanguinivores. Vertical stratification was based on the use of vegetation stratum by bats within the forest, being divided into understorey or canopy species (Kalko and Handley, 2001; Ramos Pereira et al., 2010). Finally, for wing morphology, we used data from Tavares (2013) and Marinello and Bernard (2014) for measures of aspect ratio and relative wing loading.
Data Analysis
We restricted our analyses to phyllostomids and the mormoopid Pteronotus spp., which can be sampled adequately with understorey mist nets (Kalko et al., 1996). Firstly, we used Spearman’s correlation to test if species richness, Shannon’s and Simpson diversity and abundance varied with the age of the acacia plantations. All correlations were non-significant (p > 0.05; Supplementary Table S2), so we pooled the data of the acacia plots. Secondly, we performed a Partial Mantel test (Mantel, 1967) to assess the presence of spatial autocorrelation in the data, after accounting for the effect of habitat type on species composition. For this, we used Euclidean geographic distance, habitat type, and bat assemblage similarity among sites to test whether compositional similarity (using the Bray-Curtis index—Clarke, 1993) was explained by geographic distance. The result of the Mantel test showed no spatial autocorrelation in species composition after accounting for habitat type (r = 0.128; p = 0.113).
For all analyses, except for species richness, we considered only those species that had more than five captures (see Supplementary Table S3). We did this because species with few captures in our study, such as Artibeus concolor, Glossophaga soricina, and Gardnerycteris crenulatum, are relatively frequently captured in areas of Amazonian savannahs when higher sampling effort is employed (see Bernard and Fenton, 2002; Carvalho et al., 2018). Therefore, we cannot be sure that these species were well-sampled in our study, and as such removing them should avoid biasing our results due to our low sampling effort. After this filtering, we had to remove two plots that were sampled in the savannah, as they presented none or only one species with at least five captures. Thus, all analyses were performed using only four savannah plots.
Alpha Diversity
The α-diversity between the three habitats was compared using the estimated species richness, dominance (Simpson’s Index D), taxonomic, functional, and phylogenetic diversity. For all indices, except for dominance, we used Hill numbers (Hill, 1973). Hill numbers are defined by the q parameter, which determines the sensitivity of the measure to relative species abundances and facilitates comparison of the data (Hill, 1973; Chiu and Chao, 2014). Thus, as q increases, the diversity values become more sensitive to common species (Chiu and Chao, 2014; Li, 2018a). For Hill numbers, q = 0 is equivalent to estimated species richness (species abundance is ignored), q = 1 is equivalent to Shannon’s diversity (all species are weighted by their abundance), and q = 2 is equivalent to Simpson diversity (common species receive greater weight than rare species; Hill, 1973; Chiu and Chao, 2014; Li, 2018a). To estimate species richness (q = 0), interpolated and extrapolated species accumulation curves were constructed in the R package “iNEXT” (Hsieh et al., 2016). These curves were constructed for each plot and considering a sample size of twice the smallest sample (52 individuals—total number of individuals captured in the four analysed plots of savannah), as recommended by Chao et al. (2014). For taxonomic, functional and phylogenetic diversity, we used the R package “hillR” (Li, 2018b), considering only the values of q for Shannon’s (q = 1) and Simpson’s diversity (q = 2), as these two indices take into account species abundances. For functional diversity, Hill numbers incorporate species pairwise functional distances calculated from species traits (Chiu and Chao, 2014), while for phylogenetic diversity, Hill numbers incorporate a phylogenetic tree (Li, 2018b). For phylogenetic diversity, we used a phylogenetic tree based on the phylogeny of Jones et al. (2002). Subsequently, we used a Kruskal-Wallis test to compare species richness, taxonomic, functional, and phylogenetic diversity between the forest, savannahs and acacia plantations. Additionally, we used Dunn’s test, with Bonferroni correction, as a post hoc test to assess pairwise between-habitat differences.
Bat Assemblage Structure and Species Composition
Differences in bat assemblage composition between habitats were visualised using a Non-Metric Multidimensional Scaling (NMDS) ordination. The significance of a grouping by habitat type was assessed using analysis of similarity (ANOSIM) with the Bray-Curtis index (Clarke, 1993), applying a Bonferroni correction. To calculate the contribution of each species to the observed differences in species composition between habitats, we used the similarity percentages breakdown (SIMPER) procedure with the Bray-Curtis index (Clarke, 1993). ANOSIM, SIMPER, NMDS, Kruskal-Wallis analyses, Dunn’s test, and calculation of the Simpson’s Index D were carried out in software Past (Hammer et al., 2008).
Taxonomic, Functional, and Phylogenetic Beta Diversity
We examined the variation in taxonomic (TβD), functional (FβD), and phylogenetic (PβD) β-diversity to elucidate the ecological processes behind the differences in bat assemblages of these habitats. For this purpose, we measured total β-diversity using the Jaccard index and partitioned it into turnover and nestedness components through the β–3 and βrich indexes (Carvalho et al., 2012) and applied this framework to TβD, FβD and PβD. For TβD, the two components were species replacement (TβDTur—species turnover) and species richness difference (TβDRich—due to loss or gain of bat species). For FβD, the two components were functional replacement (FβDTur—functional turnover) and functional richness difference (FβDRich—due to loss or gain of functional traits). For PβD, the two components were lineage replacement (PβDTur—lineage turnover) and lineage richness difference (PβDRich—due to loss or gain of bat lineages). The β-diversity components (i.e., βTur + βRich) were computed using rarefaction (1,000 runs) of 104 individuals (as was done to estimate species richness—q = 0) for each plot. The analyses were conducted using the beta function of the R package “BAT” (Cardoso et al., 2015). For these estimates, we used the same functional traits (previously transformed into a functional tree) and the same phylogenetic tree mentioned above for α-diversity. Posteriorly, to visualise the similarity between habitats in terms of TβD, FβD, and PβD, we used Unweighted Pair Group Method with Arithmetic Mean (UPGMA) clustering. All necessary packages were run in R, version 4.0.2 (R Core Team, 2020).
Results
Overview
We captured a total of 528 bats belonging to 29 species, 21 genera and two families (Phyllostomidae and Mormoopidae; Supplementary Table S3). Carollia perspicillata was the most captured species in all three habitats, followed by Artibeus lituratus, Artibeus planirostris, and Artibeus cinereus (Supplementary Table S3). Seven species were exclusively captured in the acacia plantations (Chrotopterus auritus, Carollia brevicauda, Lophostoma brasiliense, Pteronotus spp., Sturnira lilium, Tonatia maresi, and Trinycteris nicefori). The forest was the second habitat with the greatest number of unique species (Artibeus concolor, Glossophaga soricina, Micronycteris hirsuta, and Vampyriscus bidens). Only one species (Lophostoma carrikeri) was found exclusively in the savannah. The acacia plantations also had the greatest number of individuals captured (385), followed by forest (75), and savannah (68).
Alpha Diversity
There was no significant difference in estimated species richness between the forest (mean ± SD—7.56 ± 3.61), savannah (6.94 ± 3.38), and acacia plantations (8.99 ± 2.92; Figure 1 and Supplementary Table S4). Taxonomic diversity was significantly lower in the acacia plantations than in forest and savannah, for both Shannon and Simpson diversity (Figure 1). Dominance was significantly higher in the acacia plantations compared to the forest and savannah (Figure 1). Additionally, functional diversity was higher for q = 1 (Shannon’s diversity) and q = 2 (Simpson’s diversity) in the forest than in the savannah and acacia plantations (Figure 1). For all other comparisons, including phylogenetic α-diversity, there were no significant differences (Figure 1 and Supplementary Table S4).
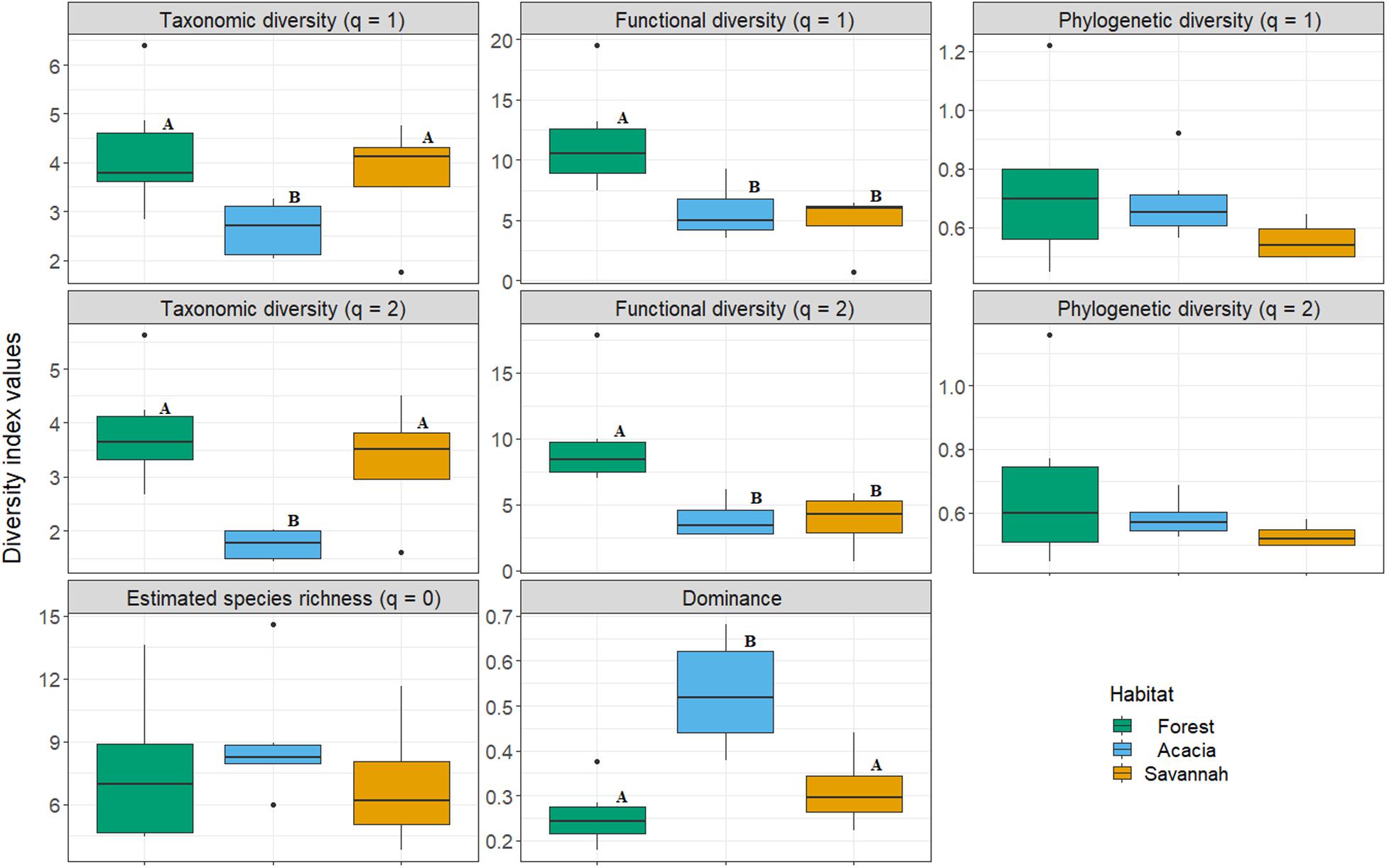
Figure 1. Comparison of taxonomic, functional, and phylogenetic diversity, as well as species richness and dominance of bat assemblages sampled in the forest, savannah and acacia plantations in the northern Brazilian Amazon in 2017. Different letters indicate a significant difference for diversity indices between habitats.
Bat Assemblage Structure and Species Composition
Considering all three habitats together, species composition differed significantly (Global R = 0.63; p < 0.01). However, this was driven by the difference between the forest and acacia plantations (R = 0.62, p < 0.01) and between the savannah and acacia plantations (R = 0.40, p = 0.04), and not between the forest and savannah, where species composition did not differ (R = 0.01, p = 1.0). These differences and similarities between species composition were further supported by the NMDS, which showed a clear separation between the acacia plantations and the other two habitats (Figure 2). Together, C. perspicillata (70%), Mesophylla macconnelli (5%), and A. planirostris (4%), contributed ∼80% to the dissimilarity between the forest and acacia plantations. All three species had higher average abundance in the acacia plantations than in the other two habitats (Figure 3). Carollia perspicillata (70%), M. macconnelli (5%), and A. lituratus (6%) contributed ∼80% to the dissimilarity between savannah and acacia plantations. Of these three species, only A. lituratus had a higher average abundance in the savannah than in the acacia plantations, with C. perspicillata and M. macconnelli having a higher average abundance in the acacia plantations (Figure 3).
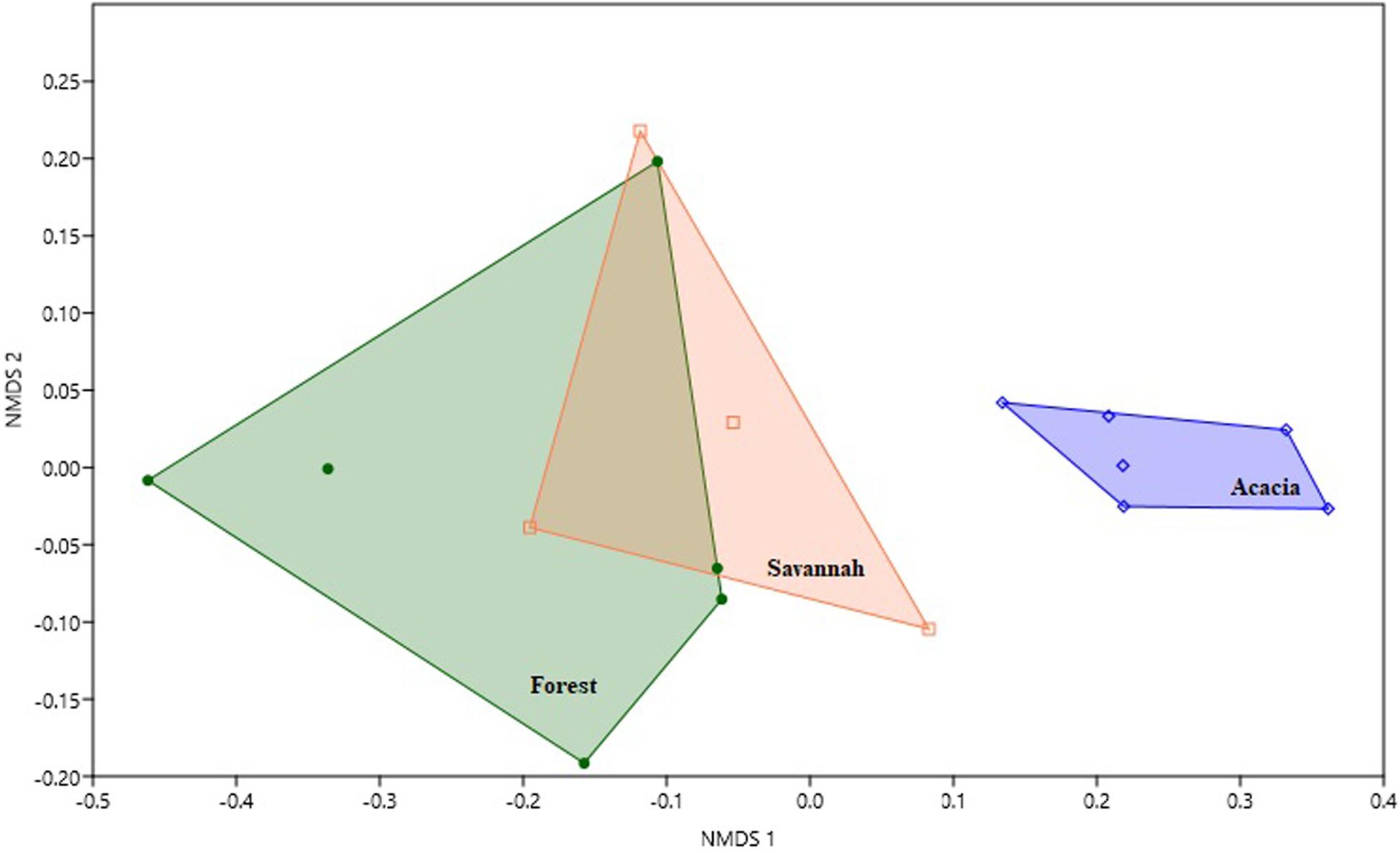
Figure 2. Non-metric multidimensional scaling (NMDS) ordination plot displaying the differences in bat species composition between the forest, savannah, and acacia plantations in an area of Amazonian savannah in the northern Brazilian Amazon. The NMDS was performed on a Bray-Curtis similarity matrix.
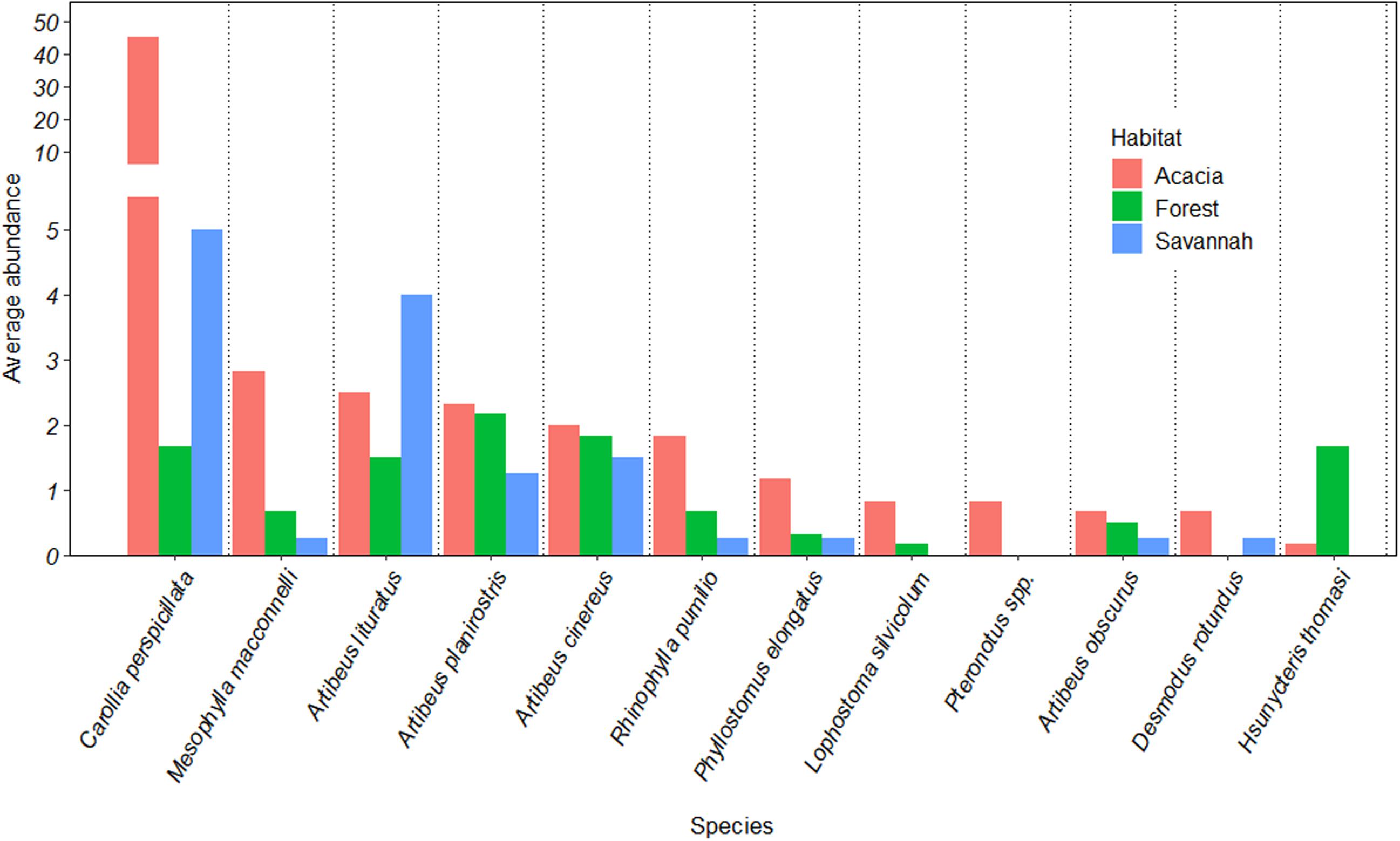
Figure 3. Average abundance of the 12 most common bat species sampled in forest, savannah and acacia plantations in an Amazonian savannah in the northern Brazilian Amazon in 2017. The mean abundance values were estimated using the similarity percentages breakdown (SIMPER) procedure. For better visualisation of the data, the y-axis was expanded below the value of 10.
Taxonomic, Functional, and Phylogenetic Beta Diversity
In terms of taxonomic diversity, the savannah and acacia plantations were slightly more similar than the savannah and forest, because the β-diversity between these two habitats was the lowest (TβD = 0.60; Figures 4, 5). The highest value of β-diversity was found between the forest and the acacia plantations (TβD = 0.77), with forest and savannah falling in between (TβD = 0.64; Figure 5). Moreover, for functional and phylogenetic β-diversity, forest and savannah attained the lowest values for β-diversity (FβD = 0.30; PβD = 0.24; Figure 5), being more similar habitats (Figure 4). The savannah and acacia plantations had intermediate values of functional and phylogenetic β-diversity (FβD = 0.53; PβD = 0.37; Figure 5), and the forest and acacia plantations the highest values (FβD = 0.59; PβD = 0.46; Figure 5).
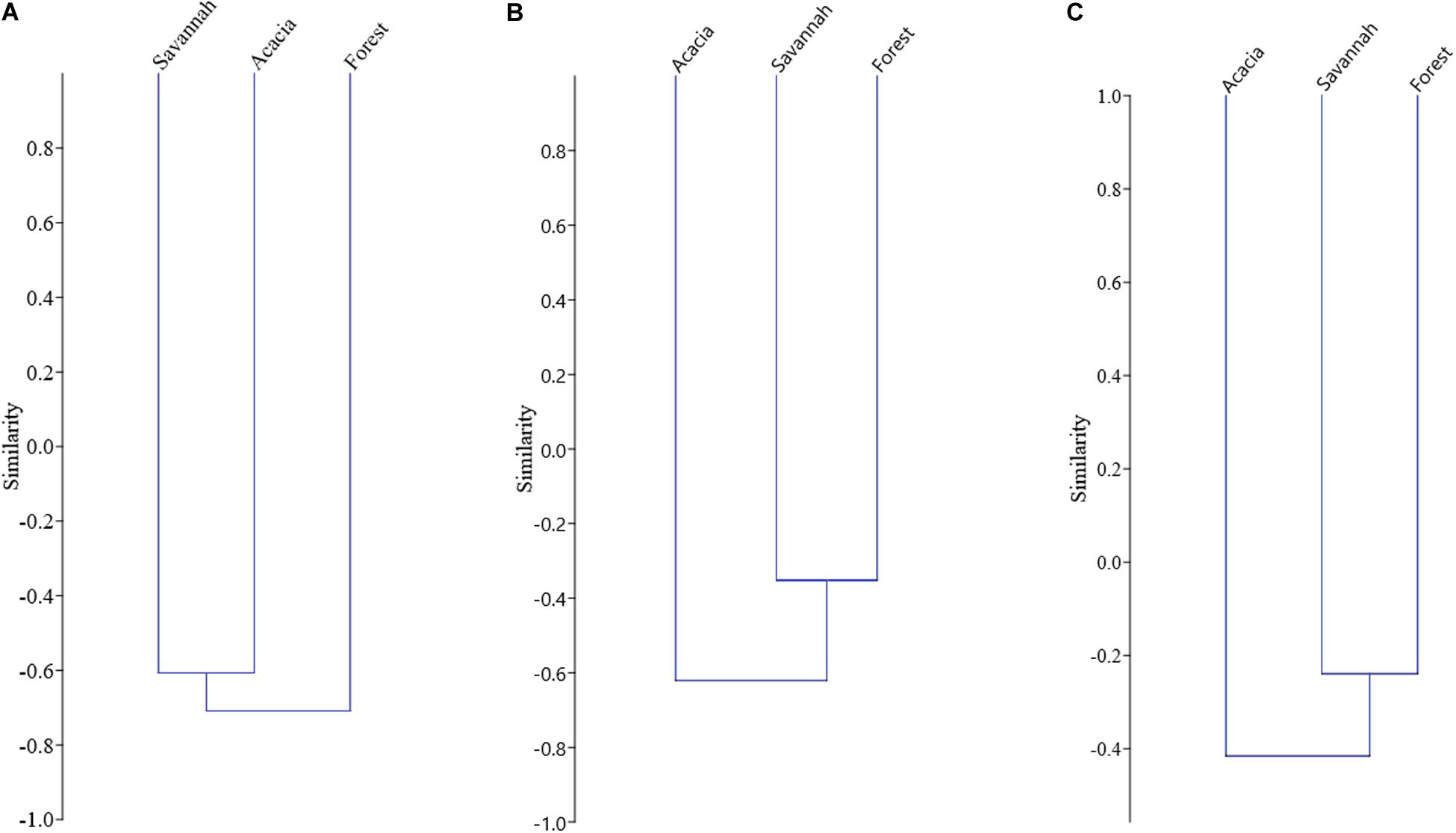
Figure 4. Unweighted Pair Group Method with Arithmetic Mean (UPGMA) clustering of values of (A) taxonomic, (B) functional, and (C) phylogenetic β-diversity for bat assemblages sampled in the forest, savannah, and acacia plantations in an Amazonian savannah in the northern Brazilian Amazonia in 2017.
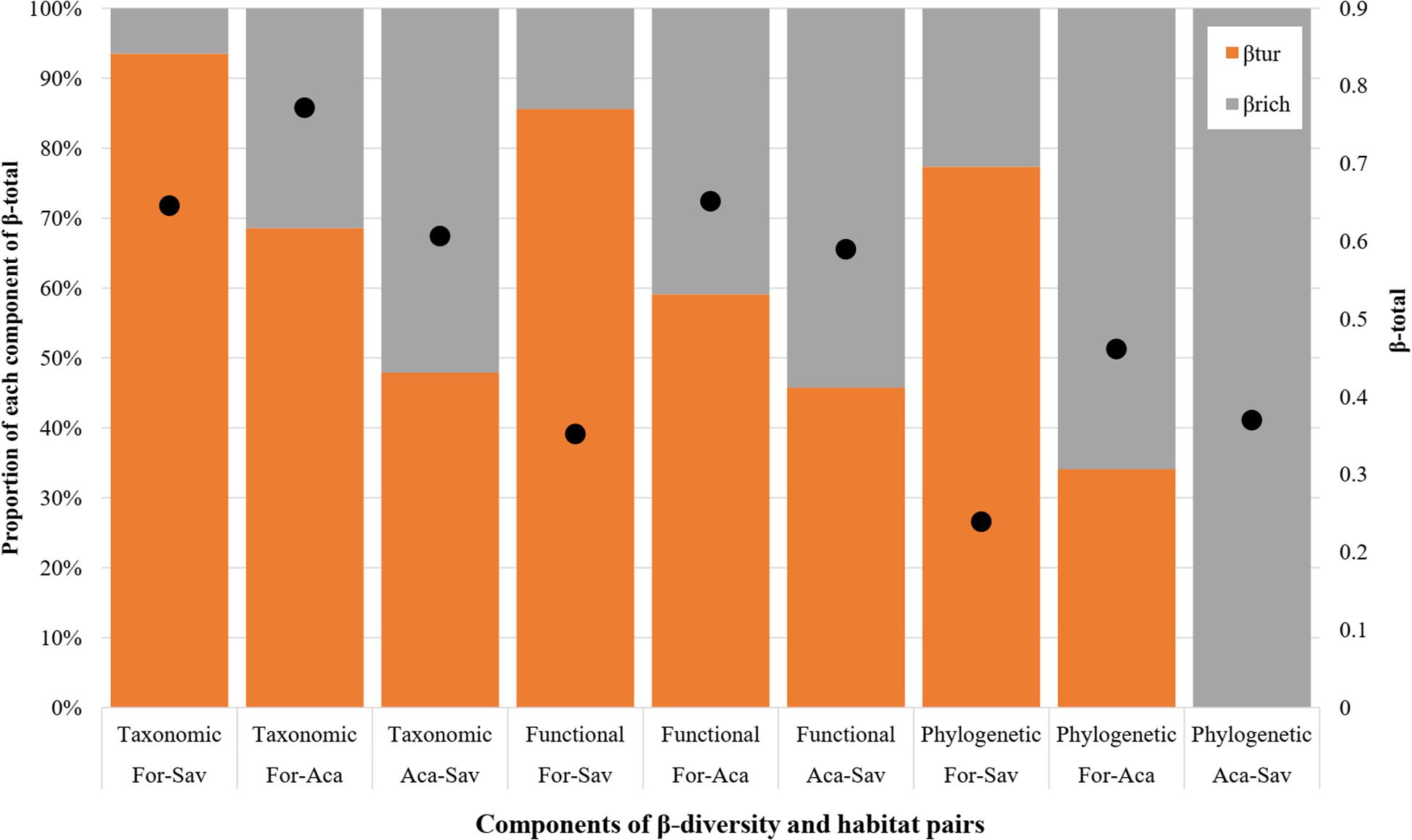
Figure 5. Values of taxonomic (TβD), functional (FβD), and phylogenetic (PβD) β-diversity and percentage of each of the components (βTur and βRich) that make up the total β-diversity for the bat assemblages sampled in the forest, savannah and acacia plantations in an Amazonian savannah in the northern Brazilian Amazonia in 2017. The black dots inside the bars represent the total β-diversity values of each partition (z-axis). For, Forest; Sav, Savannah; Aca, Acacia.
When we partitioned β-diversity, turnover (βTur) was the main component of taxonomic and functional β-diversity between the forest and the savannah, and between the forest and the acacia (Figure 5). The difference in species richness and functions (βRich) was the main component of taxonomic and functional β-diversity between the savannah and the acacia plantations (Figure 4). On the other hand, turnover was only the largest component of phylogenetic β-diversity when we compared the forest with the savannah. Between the forest and the acacia plantations, and between the savannah and the acacia plantations, the difference in lineage richness was the main component of phylogenetic β-diversity (Figure 5).
Discussion
We found that, in general, acacia is less diverse than native forests in terms of taxonomic and functional diversity, and is also less taxonomically diverse than the savannah matrix which it substitutes. This result is similar to those found in other studies that have shown lower taxonomic diversity in areas with eucalyptus plantations in the Cerrado (Brazilian savannah—Pina et al., 2013), and in the Amazon (Barlow et al., 2007), and with commercial tree plantations in other ecosystems (Phommexay et al., 2011; Syamsi, 2013). The observed patterns of α- and β-diversity found in the present study are in large part driven by the superabundance of one generalist and opportunistic species, Carollia perspicillata, in the acacia plantations, and overall the species composition in the acacia plantations differs significantly from that in both the forest and savannah habitats. Our results have direct implications for the ecology of bats in the Amazonian savannahs, as well as the conservation of these unique and threatened habitats.
The dominance of C. perspicillata, which is highly abundant in the acacia plantations, largely drives the lower taxonomic diversity of the acacia compared with the forest and savannah habitats. Carollia perspicillata is an opportunistic species that tends to consume more fruit of pioneer plant species (e.g., Piper spp. and Vismia spp.) when they are available (Fleming, 1986; Andrade et al., 2013; Cely-Gómez and Castillo-Figueroa, 2019). These pioneer plant species are found in early successional secondary forests and forest edges, and are also present in commercial forest plantations not subjected to understorey suppression (Bernhard-Reversat, 2001; Laurance et al., 2002; Toledo and Nascimento, 2019). The acacia plantations in our study area were not being managed and had a very dense undergrowth, in which pioneer plant species, such as Piper spp., Cecropia spp., Anona spp., and Vismia spp. are very abundant (see section “Materials and Methods”). Accordingly, C. perspicillata—a generalist species that can adapt very well to altered environments, using them for foraging and roosting (Fleming, 1986; Galindo-González, 2004; Castro-Luna et al., 2007; Cely-Gómez and Castillo-Figueroa, 2019)—was the most abundant species in this study, and the most common species in the acacia plantations. In the timber industry, understorey clearing is a common management practise in forest plantations. Forest plantations without understorey clearing, where management has ceased or for some other reason is less intensive than that which would usually be carried out, provide a less hostile habitat for bats and other mammals than do plantations that are managed more intensively and which have management regimes that include the suppression of understorey vegetation (Barlow et al., 2007; Pina et al., 2013, Piña et al., 2019). As such, we would expect that a comparison between native habitats and actively managed acacia plantations would show a greater difference in bat diversity, with acacia plantations being less taxonomically and functionally diverse than both forest and savannah habitats. Furthermore, the superabundance of generalist species in the acacia plantations in our study area has a series of implications for ecological interactions, seed rain, and subsequently for habitat quality for bats and other species.
Beyond the increase in abundance of generalist species such as C. perspicillata, our results also show that some more specialised species, such as gleaning animalivores Chrotopterus auritus and Lophostoma brasiliense, and the insectivorous Pteronotus spp. use the acacia plantations in the vicinity of Amazonian savannahs. These are species typically associated with intact forest environments (Fenton et al., 1992; Farneda et al., 2015), and were not captured in the natural savannah matrix. Preliminary data for the savannahs of the state of Amapá, northeastern Amazon, where the savannah matrix was replaced by eucalyptus plantations (William D. Carvalho—preliminary data) also show these gleaning animalivores using the plantations. This suggests that for some specialist, forest-associated species the substitution of savannah vegetation with commercial tree plantations may increase permeability. However, it is important to highlight that the Amazonian savannahs are a natural mosaic of vegetation with patches of forest, gallery forests and palm forests of different sizes and structures immersed in a matrix of savannah (Mustin et al., 2017), and bat species that occur in these areas are adapted to use these different types of habitats, easily traversing the landscape (Bernard and Fenton, 2003; Loayza and Loiselle, 2009). In contrast, a study in the Cerrado biome found that gleaning animalivores did not use the eucalyptus plantations (Pina et al., 2013). Further studies are required, focussing on landscape use by bats, in order to understand how the substitution of savannah vegetation with commercial tree plantations in the Amazonian savannahs affects matrix permeability and subsequently connectivity between forest patches.
Furthermore, despite the occurrence of some forest-dependent species in the acacia plantations (e.g., Lophostoma silvicola and Pteronotus spp.), assemblages in this habitat were less functionally diverse than the forest habitats for both Shannon (q = 1) and Simpson diversity (q = 2). This may again be largely driven by the superabundance of C. perspicillata in the acacia plantations, increasing the weight of shrub frugivores in the functional diversity index. This pattern, in which the acacia plantations are less functionally diverse than the forest, is also in part driven by the higher structural complexity of forests compared to acacia plantations, favouring the presence of a greater number of species with different functions, as in other Amazon regions (Farneda et al., 2018; Carrasco-Rueda and Loiselle, 2019).
Functional and phylogenetic β-diversity were always higher between the acacia and each of the natural habitats, than between the forest and the savannah. Thus, our results show that the replacement of savannah by acacia plantations increases the dissimilarity with forests. Functional β-diversity between the acacia and the forest areas is driven slightly more by turnover than richness, and phylogenetic β-diversity more by richness than turnover. However, it is the loss of functions and lineages, much more than turnover, that drives the erosion of functional and phylogenetic diversity when acacia plantations replace savannah areas. Other studies carried out in the Cerrado and Amazon biomes have already shown the loss of bat species, their functions and lineages in human-modified landscapes in comparison with areas of native vegetation (Ramos Pereira et al., 2018; Aninta et al., 2019; Farneda et al., 2020). As such, the multidimensional approach used here is powerful and goes beyond merely species richness and taxonomic diversity. By examining the functional and phylogenetic components of diversity, we are able to move away from exploring only the patterns of diversity, and can instead begin to understand how the substitution of native vegetation with plantations is impacting ecosystem processes. For example, the loss of a particular species does not necessarily have an impact on ecosystem functioning, but the loss of certain functions could have important implications for the ecosystem as a whole (Gitay et al., 1996). Similarly, while the loss of a particular species from an area may have conservation implications, when that species carries a unique evolutionary history and its loss represents the loss of a lineage, the implications for conservation are amplified (Aninta et al., 2019). In this study, by considering α- and β-taxonomic, functional, and phylogenetic diversity, we have been able to show that the replacement of areas of natural savannah by acacia plantations causes a regional loss in diversity across all diversity dimensions.
Despite the limited sampling in this study, and the use of mist nets only at ground level, our results are similar to those of other studies carried out throughout South America (Ramos Pereira et al., 2018; Farneda et al., 2020), particularly in the Amazon (Willig et al., 2007; Farneda et al., 2015; Aninta et al., 2019). Also, the sites sampled were spatially grouped by habitat, with the sites in forest very close to each other, compared with the sites in the savannah and those in acacia plantations. However, our initial spatial analysis, incorporating geographic distance, habitat type, and bat assemblage similarity, suggested that there was no influence of the sampling design on our results. We show that, although the acacia plantations partially retain the taxonomic, functional and phylogenetic diversity of the forest bat assemblages, they are significantly less taxonomically and functionally diverse than the forests themselves, and the diversity they retain may reflect their proximity to forest patches. In addition, the proximity of acacia plantations to forests can also have an indirect negative effect on forest bat assemblages, and this should be investigated by future studies. Acacia plantations seem permeable to some species of bats, providing increased availability of food for generalist species such as C. perspicillata, and potentially acting as corridors for forest-dependent species such as L. silvicola. However, the replacement of savannah by acacia plantations leads to the loss of species, functions and lineages in the bat assemblage. Furthermore, the superabundance of generalist species in our study area has a series of ecological and conservation implications. As such, taken together our results suggest that acacia plantations in the Amazon are not appropriate to use in offset programmes that aim to reforest or in forest compensation schemes. However, this type of plantation tends to be used by a wider range of species than other types of plantations, such as herbaceous crops, or pasture, and as such may be comparatively less negative for biodiversity (Barlow et al., 2007; Brockerhoff et al., 2013; Carrasco-Rueda and Loiselle, 2019). That being said, there is an alternative route for sustainable economic development in the Amazon savannahs and in the region as a whole. Given that the replacement of native savannah and forest vegetation with acacia plantations has negative impacts on bat diversity, we recommend that joint conservation and development initiatives such as The Amazon Third Way (Nobre and Nobre, 2018) should be supported and expanded. The Amazon Third Way proposes an innovative bioeconomy based on the aggregation of value to sustainably harvested non-timber forest products (NTFPs), building capacity in local communities and traditional populations, and combining traditional knowledge with state-of-the-art technology to generate jobs along the supply chain and develop the regional and national economy in a socially just and environmentally sustainable way (Nobre and Nobre, 2018). This idea is innovative, but is also based on a long history of projects that aim to support the aggregation of value to NTFPs and thus value the standing forest whilst providing sustainable and culturally appropriate livelihoods to local communities and traditional populations. In conclusion, further studies are required to fully understand the ecological and conservation implications of the conversion of native habitats to commercial tree plantations in the Amazon, but there is also a need to critically evaluate the local, regional and national social and economic impacts of this type of commercial activity and in particular to compare them to more socially, environmentally, and economically sustainable alternatives.
Data Availability Statement
The original contributions presented in the study are included in the article/Supplementary Material, further inquiries can be directed to the corresponding author/s.
Ethics Statement
The animal study was reviewed and approved by Comissão de Ética no Uso de Animais da Universidade Federal de Amapá—CEUA-UNIFAP.
Author Contributions
WC and JT originally formulated the idea. WC, KM, SS, DP, UC, and JT conducted fieldwork. WC and CM performed statistical analyses. WC, CM, BX, and KM wrote the drafts of the main manuscript and the online resources. All authors contributed critically to the manuscript and gave final approval for publication.
Funding
This work was supported by the Conselho Nacional de Desenvolvimento Científico e Tecnológico—CNPq (grant number CNPQ/Universal 459735/2014-4).
Conflict of Interest
The authors declare that the research was conducted in the absence of any commercial or financial relationships that could be construed as a potential conflict of interest.
Acknowledgments
We are grateful to F.I.T. Manejo Florestal do Brasil LTDA for allowing us to conduct this research in its farms. WC and SS were supported, respectively, by post-doctoral (PNPD/CAPES) and doctoral scholarships of the Coordenação de Aperfeiçoamento de Pessoal de Nível Superior (CAPES), Brazil. We thank IBAMA for authorisation to conduct research in the ANF (IBAMA/SISBIO permit 58887-1). We thank the two reviewers, the editor EC, and Carolina Castilho for their valuable comments and suggestions on our manuscript.
Supplementary Material
The Supplementary Material for this article can be found online at: https://www.frontiersin.org/articles/10.3389/fevo.2020.609214/full#supplementary-material
Footnotes
References
Aguirre, L. F. (2002). Structure of a Neotropical savanna bat community. J. Mamm. 83, 775–784. doi: 10.1644/1545-1542(2002)083<0775:soansb>2.0.co;2
Alroy, J. (2017). Effects of habitat disturbance on tropical forest biodiversity. Proc. Natl. Acad. Sci. U.S.A. 114, 6056–6061. doi: 10.1073/pnas.1611855114
Andrade, T. Y., Thies, W., Rogeri, P. K., Kalko, E. K., and Mello, M. A. (2013). Hierarchical fruit selection by Neotropical leaf-nosed bats (Chiroptera: Phyllostomidae). J. Mammal. 94, 1094–1101. doi: 10.1644/12-MAMM-A-244.1
Aninta, S. G., Rocha, R., López-Baucells, A., and Meyer, C. F. (2019). Erosion of phylogenetic diversity in Neotropical bat assemblages: findings from a whole-ecosystem fragmentation experiment. Biodivers. Conserv. 28, 4047–4063. doi: 10.1007/s10531-019-01864-y
Barbosa, R. I. (1997). “Distribuição das Chuvas em Roraima,” in Homem, Ambiente e Ecologia no Estado de Roraima, eds R. I. Barbosa, E. J. G. Ferreira, and E. G. Castellon (Manaus: Editora do INPA), 23–35.
Barbosa, R. I., Campos, C., Pinto, F., and Fearnside, P. M. (2007). The “Lavrados” of Roraima: biodiversity and conservation of Brazil’s Amazonian savannas. Funct. Ecosyst. Commun. 1, 30–42.
Barlow, J., Gardner, T. A., Araujo, I. S., Ávila-Pires, T. C., Bonaldo, A. B., Costa, J. E., et al. (2007). Quantifying the biodiversity value of tropical primary, secondary, and plantation forests. Proc. Natl. Acad. Sci. U.S.A. 104, 18555–18560. doi: 10.1073/pnas.0703333104
Bernard, E., and Fenton, M. B. (2002). Species diversity of bats (Mammalia: Chiroptera) in forest fragments, primary forests, and savannas in central Amazonia. Brazil. Can. J. Zool. 80, 1124–1140. doi: 10.1139/z02-094
Bernard, E., and Fenton, M. B. (2003). Bat mobility and roosts in a fragmented landscape in central Amazonia. Brazil. Biotrop. 35, 262–277. doi: 10.1111/j.1744-7429.2003.tb00285.x
Bernhard-Reversat, F. (2001). Effect of Exotic Tree Plantations on Plant Diversity and Biological Soil Fertility in the Congo Savanna: With Special Reference to Eucalypts. Bogor: Center for International Forestry Research.
Boucher, D., Elias, P., Lininger, K., May-Tobin, C., Roquemore, S., and Saxon, E. (2011). The Root of the Problem: What’s Driving Tropical Deforestation Today?. Cambridge: UCS Publications.
Brockerhoff, E. G., Jactel, H., Parrotta, J. A., and Ferraz, S. F. (2013). Role of eucalypt and other planted forests in biodiversity conservation and the provision of biodiversity-related ecosystem services. For. Ecol. Manag. 301, 43–50. doi: 10.1016/j.foreco.2012.09.018
Cardoso, P., Rigal, F., and Carvalho, J. C. (2015). BAT–Biodiversity Assessment Tools, an R package for the measurement and estimation of alpha and beta taxon, phylogenetic and functional diversity. Methods Ecol. Evol. 6, 232–236. doi: 10.1111/2041-210X.12310
Carrasco-Rueda, F., and Loiselle, B. A. (2019). Do riparian forest strips in modified forest landscapes aid in conserving bat diversity? Ecol. Evol. 9, 4192–4209. doi: 10.1002/ece3.5048
Carvalho, J. C., Cardoso, P., and Gomes, P. (2012). Determining the relative roles of species replacement and species richness differences in generating beta-diversity patterns. Glob. Ecol. Biogeogr. 21, 760–771. doi: 10.1111/j.1466-8238.2011.00694.x
Carvalho, W. D., Gomes, L. A. C., Castro, I. J., Martins, A. C., Esbérard, C. E. L., and Mustin, K. (2018). Beyond the Amazon forest: richness, abundance and flight height of bats in the understory of savannahs, campinaranas and terra firme forest. Acta Chiropt. 20, 407–419. doi: 10.3161/15081109ACC2018.20.2.011
Carvalho, W. D., and Mustin, K. (2017). The highly threatened and little known Amazonian savannahs. Nat. Ecol. Evol. 1:0100. doi: 10.1038/s41559-017-0100
Carvalho, W. D., Mustin, K., Hilário, R. R., Vasconcelos, I. M., Eilers, V., and Fearnside, P. M. (2019). Deforestation control in the Brazilian Amazon: a conservation struggle being lost as agreements and regulations are subverted and bypassed. Perspect. Ecol. Conserv. 17, 122–130. doi: 10.1016/j.pecon.2019.06.002
Castro-Luna, A. A., Sosa, I. J., and Castillo-Campos, G. (2007). Quantifying phyllostomid bats at different taxonomic levels as ecological indicators in a disturbed tropical forest. Acta Chiropt. 9, 219–228. doi: 10.3161/1733-5329(2007)9[219:qpbadt]2.0.co;2
Cely-Gómez, M. A., and Castillo-Figueroa, D. (2019). Diet of dominant frugivorous bat species in an oil palm landscape from Colombian Llanos: implications for forest conservation and recovery. Therya 10, 149–153. doi: 10.12933/therya-19-682
Chao, A., Gotelli, N. J., Hsieh, T., Sander, E. L., Ma, K., Colwell, R. K., et al. (2014). Rarefaction and extrapolation with Hill numbers: a framework for sampling and estimation in species diversity studies. Ecol. Monogr. 84, 45–67. doi: 10.1890/13-0133.1
Chiu, C.-H., and Chao, A. (2014). Distance-based functional diversity measures and their decomposition: a framework based on Hill numbers. PLoS One 9:e113561. doi: 10.1371/journal.pone.0100014
Clarke, K. (1993). Non-parametric multivariate analyses of changes in community structure. Austral Ecol. 18, 117–143. doi: 10.1111/j.1442-9993.1993.tb00438.x
Coelho, M., Juen, L., and Mendes-Oliveira, A. C. (2014). The role of remnants of Amazon savanna for the conservation of Neotropical mammal communities in eucalyptus plantations. Biodivers. Conserv. 23, 3171–3184. doi: 10.1007/s10531-014-0772-9
Collevatti, R. G., Vitorino, L. C., Vieira, T. B., Oprea, M., and Telles, M. P. C. (2020). Landscape changes decrease genetic diversity in the Pallas’ long-tongued bat. Perspect. Ecol. Conserv. 18, 169–177. doi: 10.1016/j.pecon.2020.06.006
Colli, G. R., Vieira, C. R., and Dianese, J. C. (2020). Biodiversity and conservation of the Cerrado: recent advances and old challenges. Biodivers. Conserv. 29, 1465–1475. doi: 10.1007/s10531-020-01967-x
Cruz, D. C., Benayas, J. M. R., Ferreira, G. C., Santos, S. R., and Schwartz, G. (2020). An overview of forest loss and restoration in the Brazilian Amazon. New For. doi: 10.1007/s11056-020-09777-3
Curtis, P. G., Slay, C. M., Harris, N. L., Tyukavina, A., and Hansen, M. C. (2018). Classifying drivers of global forest loss. Science 361, 1108–1111. doi: 10.1126/science.aau3445
Delaval, M., and Charles-Dominique, P. (2006). Edge effects on frugivorous and nectarivorous bat communities in a neotropical primary forest in French Guiana. Rev. Écol. 61, 343–352.
Farneda, F. Z., Meyer, C. F., and Grelle, C. E. (2020). Effects of land-use change on functional and taxonomic diversity of Neotropical bats. Biotropica 52, 120–128. doi: 10.1111/btp.12736
Farneda, F. Z., Rocha, R., López-Baucells, A., Groenenberg, M., Silva, I., Palmeirim, J. M., et al. (2015). Trait-related responses to habitat fragmentation in Amazonian bats. J. Appl. Ecol. 52, 1381–1391. doi: 10.1111/1365-2664.12490
Farneda, F. Z., Rocha, R., López-Baucells, A., Sampaio, E. M., Palmeirim, J. M., Bobrowiec, P. E., et al. (2018). Functional recovery of Amazonian bat assemblages following secondary forest succession. Biol. Conserv. 218, 192–199. doi: 10.1016/j.biocon.2017.12.036
Fenton, M. B., Acharya, L., Audet, D., Hickey, M. B., Merriman, C., Obrist, M. K., et al. (1992). Phyllostomid bats (Chiroptera: Phyllostomidae) as indicators of habitat disruption in the Neotropics. Biotropica 24, 440–446. doi: 10.2307/2388615
Fernandes, G. W., Coelho, M. S., Machado, R. B., Ferreira, M. E., Aguiar, L. D., Dirzo, R., et al. (2016). Afforestation of savannas: an impending ecological disaster. Perspect. Ecol. Conserv. 14, 146–151. doi: 10.1016/j.ncon.2016.08.002
Fleming, T. H. (1986). “Opportunism versus specialization: the evolution of feeding strategies in frugivorous bats,” in Frugivores and Seed Dispersal, eds E. Alejandro and T. H. Fleming (German: Springer), 105–118. doi: 10.1007/978-94-009-4812-9_11
Galindo-González, J. (2004). Clasificación de los murciélagos de la región de Los Tuxtlas. Veracruz, respecto a su respuesta a la fragmentación del hábitat. Acta Zool. Mex. 20, 239–243.
Garbino, G. S. T., Gregorin, R., Lima, I. P., Loureiro, L., Moras, L. M., and Moratelli, R. (2020). Updated checklist of Brazilian bats: versão 2020. Comitê da Lista de Morcegos do Brasil—CLMB. Sociedade Brasileira para o Estudo de Quirópteros (Sbeq). Available online at: https://www.sbeq.net/lista-de-especies (accessed September 22, 2020).
Gardner, A. L. (2008). Mammals of South America: Marsupials, Xenarthrans, Shrews, and Bats, Vol. 1. Chicago, IL: University of Chicago Press,Google Scholar
Gitay, H., Wilson, J. B., and Lee, W. G. (1996). Species redundancy: a redundant concept? J. Ecol. 84, 121–124. doi: 10.2307/2261706
Hammer, Ø, Harper, D., and Ryan, P. (2008). PAST: Paleontological statistics software package for education and data analysis. Paleontol. Eletron 4, 1–9.
Hill, M. (1973). Diversity and evenness: a unifying notation and its consequences. Ecology 54, 427–432. doi: 10.2307/1934352
Hsieh, T., Ma, K., and Chao, A. (2016). iNEXT: an R package for rarefaction and extrapolation of species diversity (Hill numbers). Methods Ecol. Evol. 7, 1451–1456. doi: 10.1111/2041-210X.12613
Jones, G., Jacobs, D. S., Kunz, T. H., Willig, M. R., and Racey, P. A. (2009). Carpe noctem: the importance of bats as bioindicators. Endanger. Species Res. 8, 93–115. doi: 10.3354/esr00182
Jones, K. E., Purvis, A., Maclarnon, A. N. N., Bininda-Emonds, O. R., and Simmons, N. B. (2002). A phylogenetic supertree of the bats (Mammalia: Chiroptera). Biol. Rev. 77, 223–259. doi: 10.1017/s1464793101005899
Kalko, E. K., and Handley, C. O. (2001). Neotropical bats in the canopy: diversity, community structure, and implications for conservation. Plant Ecol. 153, 319–333. doi: 10.1023/A:1017590007861
Kalko, E. K., Handley, C. O., and Handley, D. (1996). “Organization, diversity, and long-term dynamics of a neotropical bat community,” in Long-Term Studies in Vertebrate Communities, eds M. Cody and J. Smallwood (Los Angeles, CA: Academic Press), 503–553. doi: 10.1016/b978-012178075-3/50017-9
Laurance, W. F., Lovejoy, T. E., Vasconcelos, H. L., Bruna, E. M., Didham, R. K., Stouffer, P. C., et al. (2002). Ecosystem decay of Amazonian forest fragments: a 22-year investigation. Biol. Conserv. 16, 605–618. doi: 10.1046/j.1523-1739.2002.01025.x
Laurindo, R. S., Novaes, R. L., Vizentin-Bugoni, J., and Gregorin, R. (2019). The effects of habitat loss on bat-fruit networks. Biodivers. Conserv. 28, 589–601. doi: 10.1007/s10531-018-1676-x
Lepers, E., Lambin, E. F., Janetos, A. C., DeFries, R., Achard, F., Ramankutty, N., et al. (2005). A synthesis of information on rapid land-cover change for the period 1981–2000. Bioscience 55, 115–124. doi: 10.1641/0006-3568(2005)055[0115:asoior]2.0.co;2
Li, D. (2018a). hillR: taxonomic, functional, and phylogenetic diversity and similarity through Hill Numbers. J. Open Source Softw. 3:1041. doi: 10.21105/joss.01041
Li, D. (2018b). Package ‘hillR’. Available online at: https://cran.r-project.org/web/packages/hillR/hillR.pdf. (accessed May 15, 2020).
Lim, B. K., and Engstrom, M. D. (2001). Species diversity of bats (Mammalia: Chiroptera) in Iwokrama Forest, Guyana, and the Guianan subregion: implications for conservation. Biodivers. Conserv. 10, 613–657. doi: 10.1023/A:1016660123189
Lim, B. K., and Lee, T. E. Jr. (2018). Community ecology and phylogeography of bats in the Guianan Savannas of Northern South America. Diversity 10:129. doi: 10.3390/d10040129
Loayza, A. P., and Loiselle, B. A. (2009). Composition and distribution of a bat assemblage during the dry season in a naturally fragmented landscape in Bolivia. J. Mamm. 90, 732–742. doi: 10.1644/08-MAMM-A-213R.1
López-Baucells, A., Rocha, R., Bobrowiec, P., Bernard, E., Palmeirim, J., and Meyer, C. (2016). Field Guide to Amazonian Bats. Manaus: Editora INPA.
Magnusson, W. E., Lima, A. P., Luizão, R., Luizão, F., Costa, F. R. C., Castilho, C. V., et al. (2005). RAPELD: a modification of the Gentry method for biodiversity surveys in long-term ecological research sites. Biota Neotrop. 5, 19–24. doi: 10.1590/S1676-06032005000300002
Mantel, N. (1967). The detection of disease clustering and a generalized regression approach. Cancer Res. 27, 209–220.
Marinello, M., and Bernard, E. (2014). Wing morphology of Neotropical bats: a quantitative and qualitative analysis with implications for habitat use. Can. J. Zool. 92, 141–147. doi: 10.1139/cjz-2013-0127
Mendes, P., and Srbek-Araujo, A. C. (2020). Effects of land-use changes on Brazilian bats: a review of current knowledge. Mamm. Rev. doi: 10.1111/mam.12227
Meyer, C. F., and Kalko, E. K. (2008). Assemblage-level responses of phyllostomid bats to tropical forest fragmentation: land-bridge islands as a model system. J. Biogeogr. 35, 1711–1726. doi: 10.1111/j.1365-2699.2008.01916.x
Meyer, C. F., Struebig, M. J., and Willig, M. R. (2016). “Responses of tropical bats to habitat fragmentation, logging, and deforestation,” in Bats in the Anthropocene: Conservation of Bats in a Changing World, eds C. C. Voigt and T. Kingston (Berlin: Springer), 63–103. doi: 10.1007/978-3-319-25220-9_4
Moreno, C. E., Calderón-Patrón, J. M., Martín-Regalado, N., Martínez-Falcón, A. P., Ortega-Martínez, I. J., Rios-Díaz, C. L., et al. (2018). Measuring species diversity in the tropics: a review of methodological approaches and framework for future studies. Biotropica 50, 929–941. doi: 10.1111/btp.12607
Mustin, K., Carvalho, W. D., Hilário, R. R., Costa-Neto, S. V., Silva, C. R., Vasconcelos, I. M., et al. (2017). Biodiversity, threats and conservation challenges in the “Cerrado of Amapá”, an Amazonian savanna. Nat. Conserv. 22, 107–127. doi: 10.3897/natureconservation.22.13823
Nobre, I., and Nobre, C. A. (2018). “The Amazonia Third Way Initiative: The role of technology to unveil the potential of a novel Tropical biodiversity-based economy,” in Land Use - Assessing the Past, Envisioning the Future, ed. L. C. Loures (London: IntechOpen), 183–213.
Oliveira, H. F., Camargo, N. F., Gager, Y., and Aguiar, L. M. (2017). The response of bats (Mammalia: Chiroptera) to habitat modification in a Neotropical savannah. Trop. Conserv. Sci. 10, 1–14. doi: 10.1177/1940082917697263
Overbeck, G. E., Vélez-Martin, E., Scarano, F. R., Lewinsohn, T. M., Fonseca, C. R., Meyer, S. T., et al. (2015). Conservation in Brazil needs to include non-forest ecosystems. Divers. Distrib. 21, 1455–1460. doi: 10.1111/ddi.12380
Palmeirim, A. F., Santos-Filho, M., and Peres, C. A. (2020). Marked decline in forest-dependent small mammals following habitat loss and fragmentation in an Amazonian deforestation frontier. PLoS One 15:e0230209. doi: 10.1371/journal.pone.0230209
Pavan, A. C., Bobrowiec, P. E. D., and Percequillo, A. R. (2018). Geographic variation in a South American clade of mormoopid bats, Pteronotus (Phyllodia), with description of a new species. J. Mammal. 99, 624–645. doi: 10.1093/jmammal/gyy048
Phalan, B., Bertzky, M., Butchart, S. H., Donald, P. F., Scharlemann, J. P. W., Stattersfield, A. J., et al. (2013). Crop expansion and conservation priorities in tropical countries. PLoS One 8:e51759. doi: 10.1371/journal.pone.0051759
Phommexay, P., Satasook, C., Bates, P., Pearch, M., and Bumrungsri, S. (2011). The impact of rubber plantations on the diversity and activity of understorey insectivorous bats in southern Thailand. Biodivers. Conserv. 20, 1441–1456. doi: 10.1007/s10531-011-0036-x
Pina, S. M. S., Meyer, C. F. J., and Zortéa, M. (2013). A comparison of habitat use by phyllostomid bats (Chiroptera: Phyllostomidae) in natural forest fragments and Eucalyptus plantations in the Brazilian Cerrado. Chiropt. Neotrop. 19, 14–30.
Piña, T. E., Carvalho, W. D., Rosalino, L. M., and Hilário, R. R. (2019). Drivers of mammal richness, diversity and occurrence in heterogeneous landscapes composed by plantation forests and natural environments. For. Ecol. Manag. 449:117467. doi: 10.1016/j.foreco.2019.117467
R Core Team. (2020). R: A Language and Environment for Statistical Computing. Available online at: https://www.r-project.org/ (Accessed March 25, 2020)
Rajão, R., Soares-Filho, B., Nunes, F., Börner, J., Machado, L., Assis, D., et al. (2020). The rotten apples of Brazil’s agribusiness. Science 369, 246–248. doi: 10.1126/science.aba6646
Ramos Pereira, M. J., Fonseca, C., and Aguiar, L. M. (2018). Loss of multiple dimensions of bat diversity under land-use intensification in the Brazilian Cerrado. Hystrix 29, 25–32. doi: 10.4404/hystrix-00020-2017
Ramos Pereira, M. J., Marques, J. T., and Palmeirim, J. M. (2010). Vertical stratification of bat assemblages in flooded and unflooded Amazonian forests. Curr. Zool. 56, 469–478. doi: 10.1093/czoolo/56.4.469
Reis, N. R., Peracchi, A. L., Batista, C. B., and Lima, I. P. (2017). História Natural dos Morcegos Brasileiros: chave de identificação de espécies. Rio de Janeiro: Technical Books Livraria LTDA.
Rezende, C. L., Scarano, F. R., Assad, E. D., Joly, C. A., Metzger, J. P., Strassburg, B. B., et al. (2018). From hotspot to hopespot: an opportunity for the Brazilian Atlantic Forest. Perspect. Ecol. Conserv. 16, 208–214. doi: 10.1016/j.pecon.2018.10.002
Saccol, S. S. A., Bolzan, A. M. R., and Santos, T. G. (2017). In the shadow of trees: does eucalyptus afforestation reduce herpetofaunal diversity in Southern Brazil? South Am. J. Herpetol. 12, 42–56. doi: 10.2994/SAJH-D-16-00028.1
Sikes, R. S., and the Animal Care, and Use Committee of the American Society of Mammalogists. (2016). Guidelines of the American Society of Mammalogists for the use of wild mammals in research and education. J. Mammal 97, 663–688. doi: 10.1093/jmammal/gyw078
Silva, C. R., Martins, A. C. M., Castro, I. J., Bernard, E., Cardoso, E. M., Lima, D. S., et al. (2013). Mammals of Amapá State, Eastern Brazilian Amazonia: a revised taxonomic list with comments on species distributions. Mammalia 77, 409–424. doi: 10.1515/mammalia-2012-0121
Strassburg, B. B., Brooks, T., Feltran-Barbieri, R., Iribarrem, A., Crouzeilles, R., Loyola, R., et al. (2017). Moment of truth for the Cerrado hotspot. Nat. Ecol. Evol. 1:0099. doi: 10.1038/s41559-017-0099
Straube, F. C., and Bianconi, G. V. (2002). Sobre a grandeza e a unidade utilizada para estimar esforço de captura com utilização de redes-de-neblina. Chirop. Neotrop. 8, 150–152.
Tavares, V. C. (2013). Phyllostomid bat wings from Atlantic Forest bat ensembles: an ecomorphological study. Chirop. Neotrop. 19, 57–70.
Thoisy, B. D., Pavan, A. C., Delaval, M., Lavergne, A., Luglia, T., Pineau, K., et al. (2014). Cryptic diversity in common mustached bats Pteronotus cf. parnellii (Mormoopidae) in French Guiana and Brazilian Amapá. Acta Chiropt. 16, 1–13. doi: 10.3161/150811014X683228
Toledo, J. J., and Nascimento, H. E. (2019). Diversity, composition and biomass of native vascular plants regenerating under Acacia mangium Will. plantations in northern Amazonia. Rev. Bras. Bot. 42, 457–465. doi: 10.1007/s40415-019-00539-w
Treitler, J. T., Heim, O., Tschapka, M., and Jung, K. (2016). The effect of local land use and loss of forests on bats and nocturnal insects. Ecol. Evol. 6, 4289–4297. doi: 10.1002/ece3.2160
Veldman, J. W., Overbeck, G. E., Negreiros, D., Mahy, G., Le Stradic, S., Fernandes, G. W., et al. (2015). Where tree planting and forest expansion are bad for biodiversity and ecosystem services. BioScience 65, 1011–1018. doi: 10.1093/biosci/biv118
Keywords: Amazonian ecosystems, Amazonian savannahs, Chiroptera, landscape change, Lavrados de Roraima, non-forest habitats
Citation: Carvalho WD, Meyer CFJ, Xavier BS, Mustin K, Castro IJ, Silvestre SM, Pathek DB, Capaverde UD Jr, Hilário R and Toledo JJ (2020) Consequences of Replacing Native Savannahs With Acacia Plantations for the Taxonomic, Functional, and Phylogenetic α- and β-Diversity of Bats in the Northern Brazilian Amazon. Front. Ecol. Evol. 8:609214. doi: 10.3389/fevo.2020.609214
Received: 22 September 2020; Accepted: 25 November 2020;
Published: 14 December 2020.
Edited by:
Elena D. Concepción, National Museum of Natural Sciences (MNCN), SpainReviewed by:
Carolina Batista, Centro Universitário de Goiatuba (UniCerrado), BrazilSina Weier, University of the Free State, South Africa
Copyright © 2020 Carvalho, Meyer, Xavier, Mustin, Castro, Silvestre, Pathek, Capaverde, Hilário and Toledo. This is an open-access article distributed under the terms of the Creative Commons Attribution License (CC BY). The use, distribution or reproduction in other forums is permitted, provided the original author(s) and the copyright owner(s) are credited and that the original publication in this journal is cited, in accordance with accepted academic practice. No use, distribution or reproduction is permitted which does not comply with these terms.
*Correspondence: William Douglas Carvalho, wilruoca@hotmail.com