- 1School of Environmental Sciences, University of Guelph, Guelph, ON, Canada
- 2Centro de Investigaciones en Abejas (CIABE), Centro Universitario del Sur, Universidad de Guadalajara, Guadalajara, Mexico
- 3Departamento de Producción Agrícola, Centro de Investigaciones en Abejas (CIABE), Centro Universitario de la Costa Sur, Universidad de Guadalajara, Guadalajara, Mexico
- 4Unidad de Medicina Veterinaria y Zootecnia, Universidad Autónoma de Zacatecas, Zacatecas, Mexico
- 5Departamento de Medicina y Zootecnia en Abejas, FMVZ, UNAM, Mexico City, Mexico
- 6Departamento de Apicultura Tropical—Campus Ciencias Biológicas y Agropecuarias, Universidad Autónoma de Yucatán, Mérida, Mexico
The Africanization of honey bees (Apis mellifera L.) in the Americas is among the most extensive insect invasions in the world, with large-scale effects on the economy and ecology of a whole continent. Africanized honey bees (AHBs) are a distinctive lineage of A. mellifera, resulting of the extensive admixture between African subspecies (A. m. scutellata and A.m. adansonii) with resident European stocks of honey bees introduced into the Americas. Despite its great importance, to date, the outcome of Africanization of honey bees has not been evaluated in detail. In this article we use the case of Mexico, one of the top beekeeping countries in the world, to assess the effects of Africanization of honey bees and its outcome. There is evidence of African genes in honey bee populations across Mexico, with prevalence in the tropical areas and less so in temperate ones. The Africanization of honey bees resulted in lower honey yields per hive in temperate climates of Mexico, but this has not been assessed in the tropical regions. Mexico’s total honey production and exports at the start of the Africanization process decreased, but today, they have partially rebounded and have remained stable. As in all countries where Africanization has occurred, the defensive behavior of honey bees in Mexico increased but notably, stinging incidents involving humans have been relatively insignificant (0.23 fatalities per million people). Ecologically, AHBs seem to have posed limited impact on the native apifauna and have contributed to pollination of major economic crops, but more studies are needed to evaluate the overall effect. AHBs can be potentially more resistant to parasites and diseases and worth of note is that AHBs in Mexico resulted in a new generation of beekeepers that propelled management and selective breeding. In general, the evidence suggests that the Africanization of honey bees in Mexico has had a less severe impact than originally predicted. We suggest some lines for future directions that may help to better understand the effects, make sustainable use and ameliorate the negative characteristics of AHBs.
Introduction
The Western honey bee (Apis mellifera L.) originated in the Old World (Europe, Africa and the near East), where influenced by different selective environments, diversified into several subspecies (Ruttner, 1988). In the Americas, early European settlers introduced Western European A. m. mellifera and A. m. iberiensis, followed by later introductions of Eastern European races, mainly A. m. ligustica (Whitfield et al., 2006). Thus, up to 1956, honey bees of predominantly European-descent existed in the Americas. In that year, Brazilian researchers introduced queens of A. m. scutellata and A.m. adansonii, from South Africa and Tanzania to the state of São Paulo, Brazil, to develop a selective breeding program (Kerr, 1967). European honey bees (EHBs) kept in Brazil were not well adapted to tropical conditions, and a hybrid bee could be better suited for these regions (Kerr, 1967). An accident caused the release of pure African colonies, which interbred with locally existing EHBs, thereof, originating the so-called Africanized honey bee (AHB) through the process of Africanization (Nogueira-Neto, 1964; Rinderer and Hellmich, 1991).
The process of Africanization is one of the most dramatic invasion events by any animal species (Page, 1989; Clarke et al., 2002). In spite of many attempts to stop the advance of AHBs, they expanded rapidly and produced large feral populations, which in the course of 30 years, colonized most of the Americas displacing resident EHBs, except in temperate areas, presumably because of reduced adaptation to these environments (Rinderer and Hellmich, 1991; Schneider et al., 2004). In their northward and southward advance, AHBs disrupted beekeeping in many countries of South and Central America, in part, because the beekeeping industry was not extensively developed and only low concentrations of EHB colonies existed (Rinderer and Hellmich, 1991; Rinderer et al., 1991). In contrast, Mexico has one of the highest concentrations of managed EHB colonies worldwide, and a great diversity of climates, ecosystems and beekeeping regions (Labougle and Zozaya, 1986; Quezada-Euán, 2007). In Mexico, beekeeping is a major activity of great economic and social importance, making the country the sixth world’s largest honey producer and the third largest honey exporter (Programa Nacional para el Control de la Abeja Africana [PNCAA], 2010). The organization of Mexican beekeepers and the management techniques were more developed than in much of Central and South America (Labougle and Zozaya, 1986; Guzman-Novoa, 1996; Programa Nacional para el Control de la Abeja Africana [PNCAA], 2010). Initial predictions of the effects of Africanization were highly pessimistic, foreseeing a collapse of beekeeping. More than 60 years after the start of Africanization of honey bees, no account had been conducted on the effect and consequences of AHBs in the Americas. Mexico is a good country to assess the impact of colonization, adaptation and husbandry of this invading insect. Some of the most comprehensive studies about the defensive behavior, genetics of the Africanization process, ecology and selective breeding of honey bee populations, have been conducted in Mexico. In this review article, we document what is known about different aspects of the biology of AHB populations established in Mexico, the spread across different ecological environments, and how their behaviors and other traits have contributed to their adaptation and impact on human health, the beekeeping industry and ecosystems.
History and Genetic Makeup of AHBs in Mexico
The genetic nature of AHBs had been strongly debated (Hall and Muralidharan, 1989; Smith et al., 1989; Rinderer and Hellmich, 1991). The expansion front and feral populations of AHBs in South and Central America showed little contribution of EHBs (Smith et al., 1989). This evidence suggested that AHBs were mostly of African ancestry and that there may have been reproductive and genetic mechanisms preventing hybridization with EHBs (Harrison and Hall, 1993). However, in opposition to that view, it was argued that asymmetric hybridization and, thus, little evidence of European genes in feral populations would be expected if European colonies were vastly outnumbered by AHBs (Rinderer and Hellmich, 1991). Studies in South America revealed that the asymmetry of the process of Africanization was reinforced by several reproductive strategies of AHBs such as a larger investment in swarms per year, a significantly higher production of drones, drone and queen parasitism of EHB colonies, higher absconding rates, assortative mating and, rapid ontogenetic development of AHBs queens (Rinderer et al., 1985a,b, 1987; Hellmich, 1991; Rinderer and Hellmich, 1991; Danka et al., 1992; Schneider and McNally, 1992; Vergara et al., 1993; DeGrandi-Hoffman et al., 1998b; Taylor, 1999; Quezada-Euán and May-Itza, 2001; Schneider et al., 2004). The course of Africanization in Mexico was crucial to test those two opposing hypotheses because in Mexico, the advancing Africanized front would encounter a large population of managed EHB colonies, thus, both being for the first time in comparable numerical conditions (Hellmich and Rinderer, 1991; Taylor, 1999; Clarke et al., 2002).
AHB swarms were first detected in 1986 in the state of Chiapas, Mexico, near the border with Guatemala (Fierro et al., 1987; Moffett et al., 1987). Swarm trap lines were established between 1986 and 1987 in all states of Mexico to evaluate the movement of AHBs across the country, using morphometric and allozyme analyses (Daly and Balling, 1978; Fierro et al., 1987). Chiapas is a state clearly marked by two regions, the tropical lowlands and the temperate highlands and differences in the rate of movement of colonizing swarms were found in both climate types. Only 3% of the colonizing swarms moved at altitudes above 400–500 m, but there was a fast migration and occupation of the tropical lowlands, where swarms moved at an average of 400 km/year (Quezada-Euán, 2007). It was also evident that the large mountain ranges in the South of the country and those surrounding the high plateau posed a barrier for the movement of swarms into this region in which they were only found until 1990 (Quezada-Euán, 2007). Therefore, the Africanization of honey bees in Mexico rapidly occurred along two fronts of migrating swarms that moved from Central America across the tropical Pacific and Gulf Coast lowlands and into the Yucatan Peninsula. Notably, genetic differences were found in swarms composing both colonizing fronts. Morphometric and allozyme analysis of swarms of the Gulf coast showed that they had higher frequency of African markers compared to the ones of the Pacific coast (Labougle et al., 1989). Long-term studies of the immigrant AHBs show that swarm numbers may wane some years after initial colonization (Roubik, 1989).
The Africanization of apiaries in Mexico was first studied in the Yucatan Peninsula (Rinderer et al., 1991; Quezada-Euán et al., 1996). Managed colonies showed rapid evidence of African gene introgression, but also extensive hybridization with resident honey bees of European descent (Rinderer et al., 1991; Quezada-Euán and Paxton, 1999). Moreover, although the majority of feral colonies in Yucatan had African mitotypes (mtDNA) and wing lengths not different from those of A. m. scutellata, notably, 20% had European mtDNA and European morphometrics, which indicated a contribution of the managed EHB population to the feral AHBs (Quezada-Euán and Hinsull, 1995). The presence of European markers in the feral bee population of Yucatan was explained by the large population size of resident EHBs that existed in that region at the arrival of AHBs (Quezada-Euán and Hinsull, 1995).
Similar results have been found in the temperate regions of Northern of Mexico. There, the frequency of African and European morphotypes and mitotypes was analyzed at three different altitudes (Medina-Flores et al., 2014, 2015). It was found that the frequency of colonies with African or European mitotypes and morphotypes varied significantly between regions, with results indicating a higher degree of Africanization in warm semi-dry and subtropical regions. Conversely, the highest frequency of colonies with European morphotype and mitotype occurred in temperate regions, supporting the notion that the environment and climate influence the outcome of Africanization of honey bee colonies (Rinderer et al., 1991; Sheppard et al., 1991a). Likewise, at higher altitudes, more colonies have European genotype compared with colonies established in tropical and low-altitude regions (Quezada-Euán et al., 2003). Conversely, in tropical Veracruz, Kraus et al. (2007) did not find evidence of lower frequency of African markers in honey bee colonies at high altitudes in Veracruz, Mexico. Nonetheless, it is possible that the bees collected from feral colonies at high altitudes in their study had been derived from colonies transported by migratory beekeepers from low lands to high lands, a common practice carried out with thousands of hives every year to take advantage of different blossoms in the regions where the study by Kraus et al. (2007) was conducted.
Climate and genetic differences of the Africanization fronts (Quezada-Euán, 2007) may explain differential Africanization rates in the North of Mexico. In Northwestern Mexico, frequencies of up to 56% of European mitotypes were still found in apiaries in 2004 (Zamora et al., 2008). In contrast, in Northeastern Mexico, only 30% of the colonies sampled had European mtDNA (Silva-Contreras et al., 2019). These results support the notion that the movement of AHBs across the Eastern region was more intense and preserved better the African lineage compared with the front in the Western region (Quezada-Euán, 2007).
Recently, a comprehensive study including 500 colonies collected from the five beekeeping regions of Mexico yielded a better picture of the current genotypic composition of honey bees in the country (Domínguez-Ayala et al., 2016). About half of the colonies sampled (51.5%) had African mitotypes. The highest frequency of African mitotypes was found in the tropical beekeeping region of the Gulf coast (69.8%), followed by the Yucatan Peninsula (63.8%), and the Pacific coast region (63.1%). The lowest frequency of African mitotypes was observed in the Northern region (24.9%), where European mitotypes predominated. European mitotypes were also more frequently found in the high plateau region. Morphometric data showed a similar pattern, with larger frequencies of European morphometrics in colonies of the high plateau and North and an opposite trend in the tropics (Figure 1). Interestingly, only 8% of the samples had both European mitotype and morphology, suggesting that the majority of honey bee populations in Mexico show a degree of African gene introgression. This study confirmed that as originally proposed, the degree of Africanization is climate-driven given the differential adaptation of EHBs and AHBs to temperate and tropical environments, respectively (Sheppard et al., 1991a). It is likely that traits making AHBs successful tropical honey bees (high investment in swarms and brood, eclectic use of lodgings, reduced ability to control nest temperature, and other traits) could severely limit their progress in temperate areas (Sheppard et al., 1991a; Schneider et al., 2004).
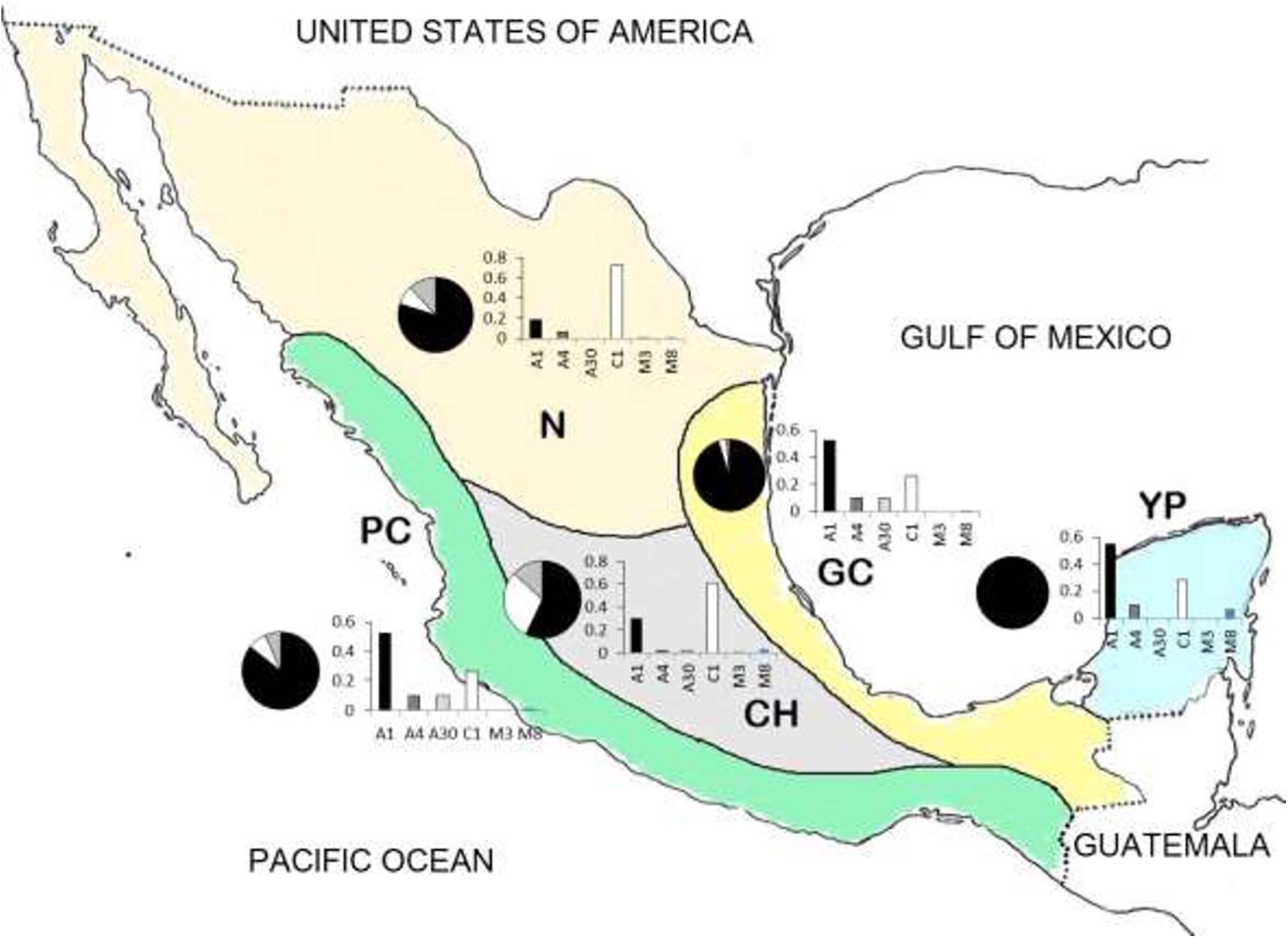
Figure 1. Map of Mexico showing the five main beekeeping regions of the country: North (N), Central highlands (CH), Pacific coast (PC), Gulf coast (GC), and Yucatan Peninsula (YP). The relative frequencies of mitotypes (bar graphs) and morphotypes (pie graphs) in each region show African in black, European in white and intermediate in gray. Letters refer to three different mitotypes of A. mellifera, namely, African (A), West-European (M), and East-European (C) (data from Domínguez-Ayala et al., 2016).
Presently, the process of Africanization of Mexico’s honey bees seems to have stabilized to a large extent (Domínguez-Ayala et al., 2016). In general, the honey bees of Mexico show evidence that hybridization has occurred extensively and that climate drives introgression of African genes into local populations. As a consequence, there are two clear populations, one mainly composed by AHBs on the tropical beekeeping regions of the Yucatan Peninsula, the Gulf of Mexico and the Pacific Coast and, another where EHBs predominate, in the temperate beekeeping regions of the high plateau and the North of the country. In general, the results of Mexico are in agreement with what has also been found in South America and the United States. Hybrid honey bee populations occur near the historic origin of AHBs in Brazil (Sheppard et al., 1991b). In Southern Texas, 23 years after the first report of AHBs, nearly 90% of the colonies had A. m. scutellata maternal ancestry, but nuclear DNA markers revealed little change in African genes compared to populations from the period 1991–2001 (Rangel et al., 2016). Thus, the general outcome of Africanization in Mexico and elsewhere indicates the existence of a hybrid swarm (Rinderer and Hellmich, 1991) with different contribution of African and European genes depending on climatic conditions.
The Ecological Impact of AHBs in Mexico
During the colonization of the Americas, AHBs expanded across some 16 million Km2 (Roubik, 1987). Most of this area was devoid of honey bees, as EHBs never established feral populations (Quezada-Euán and Hinsull, 1995; Roubik, 2000). However, by the end of last century, the tropics and subtropics contained a large population estimated in approximately one trillion colonies of honey bees of African descent from Northern Argentina to Mexico. They may consume two billion Kg of pollen and 20 billion Kg of nectar annually (Roubik, 1989). As dramatic as this may seem, compared with the rapid and hard impact on beekeeping, the influence and changes caused by the huge population of AHB colonies in the native ecosystems have been slow and difficult to detect (Roubik, 1989). There are several ways in which exotic bees can affect native bees (Goulson, 2003). These include: competition with native flower visitors for floral resources, competition with native organisms for nest sites, changes in seed set of native plants (either increases or decreases) and exotic weeds, plus transmission of parasites or pathogens to native organisms (Goulson, 2003).
The honey bee is well adapted to exploit a wide range of floral resources. Colonies are generalists and can rapidly recruit to and exploit more efficiently these resources than native bees (Roubik, 1989). Thus, a first impact could be on competition for floral resources and the displacement of native bees. Notably, although AHBs can be present in large numbers on flower patches, they do not display aggression toward other bees. Indeed, aggression is more frequent the other way around and among AHBs (Roubik and Villanueva-Gutiérrez, 2017). Rather, AHBs may displace native species by numerical advantage (Roubik, 1989). Surprisingly, long-term studies from Central America and Southern Mexico have revealed that the Neotropical bee-plant assemblages in those regions do not exist in a delicate balance and that the introduction of honey bees do not invariably upset the system (Roubik, 2000). Evidence for high resiliency of native bee populations (solitary or social) to the arriving AHBs was obtained from a long-term study in Quintana Roo, Mexico. There, native bees abandoned some resources and changed their pollen preferences to other resources after the arrival of AHBs (Roubik, 2009; Roubik and Villanueva-Gutiérrez, 2009). The native bees also shifted their foraging times to avoid competition with AHBs. Surprisingly, solitary bee abundance also increased in some years after feral AHBs were well established, so indirect benefits from the invader, such as greater pollination and abundance of certain native plants used as food by native bees could be occurring (Roubik and Villanueva-Gutiérrez, 2009). However, native bee flexibility on the use of floral resources may be constrained by the abundance and diversity of the local plant assemblage (Roubik, 2009). This means that food competition among exotic and native bees could be more intense when floral resources are limited and the resiliency of native communities could then be more compromised (Roubik, 1989, 2009). Additionally, recent studies have shown that the effect of AHBs on specialized bees and plants that depend on them may be more severe. A study from the United States revealed that the oligolectic bees Perdita meconis and Eucera quadricincta have disappeared in Southern Utah as a result of AHBs monopolizing Arctomecon humilis, the main pollen source for females of those species (Portman et al., 2018). Oligolectic bee species being unable to switch host-plants, can be left with little food needed to rear their progeny (Portman et al., 2018).
By affecting the diversity of native floral visitors, AHBs can also have an impact on the composition of local flora, but this has been seldom analyzed. One study showed that floral visitation by AHBs affects male fitness and probably fruit and seed production of the tropical tree Clusia arrudae, but without affecting the resource sought by native pollinators (Do Carmo et al., 2004). In Brazil, applying networks theory, Santos et al. (2012) found that AHBs induced significant changes in the structure of native pollination networks, mainly by making them more cohesive and monopolizing many interactions. Another network study conducted in four regions of Mexico showed that areas high in endemic species can have more specialized plant-bee interactions, and thus can be more susceptible to the effect of AHBs (Ramírez-Flores et al., 2015).
Conversely, AHBs can provide good pollination services and increase the productivity of plants and cash crops that do not require specialized pollinators. Coffee production has increased in the Americas and Mexico, probably as a result of AHBs increased floral visitation (Roubik, 2002; Vergara and Badano, 2009). On economically important Mexican crops like avocado, tomato, chilli and, physic nut, AHBs can be frequent visitors even after heavy insecticide spraying, when local apifauna wanes (Macías-Macías et al., 2009; Pérez-Balam et al., 2012; Romero and Quezada-Euán, 2013; Landaverde-González et al., 2017). Furthermore, in the light of pollinator declines (Potts et al., 2010), AHBs may act complementing the pollination services of scarce native floral visitors. One study conducted in Yucatan, Mexico, on the buzz-pollinated annatto (Bixa orellana), showed a high individual efficiency of the native stingless bee Melipona beecheii, compared with AHBs (Caro et al., 2017). However, AHBs were present in significantly higher numbers and were observed gleaning pollen previously extracted by M. beecheii, acting as a commensal. As M. beecheii is normally rare in this region of Mexico, AHBs may compensate the numeric lack of the original pollinator (Caro et al., 2017). On the Pacific coast of Mexico, temporal variation in the pollinator community and in the pollination efficiency of the main pollinators of Cucurbita moschata was found (Delgado-Carrillo et al., 2018). In the wet season, solitary native bees of the genus Peponapis were the most frequent and effective pollinators of C. moschata, whereas in the dry season, Peponapis bees were scarce. However, AHBs became the most frequent floral visitor providing an effective pollination service in this season (Delgado-Carrillo et al., 2018). Evidently, more studies are needed to understand the dynamics of AHBs with native pollinators and plants that require specialized pollination.
Nest site competition between AHBs and native stingless bees seems minimal because the former accept cavities with large openings that are not suitable for most native species and they also frequently build nests in the open (Roubik, 1989). However, the effect on other cavity nesting animals (birds and mammals) can be more severe (Efsthation and Kern, 2016). Arguably, total competition for nest sites and food could become more intense between AHBs than between the honey bees and native bees. Such competition among AHBs may be one factor contributing to curb their rate of population growth and the production of swarms (Roubik, 1989).
One less evident positive impact of honey bee Africanization in Mexico is the collateral impulse of stingless beekeeping. Before the arrival of AHBs, stingless beekeeping, an ancient activity dating from pre-Columbian times, was at the verge of extinction (Quezada-Euán et al., 2001). However, after 1986, to avoid stinging incidents, apiaries had to be relocated in the forests, further away from human settlements where they were normally kept. This opened a niche for the buildup of stingless bee backyards or meliponarios, which posed no risk to people (Quezada-Euán, 2018). New techniques started to be developed and applied, which propelled the modernization and rebirth of stingless beekeeping in Mexico (Quezada-Euán, 2018).
Native bee predators may have benefited by the sudden abundance of feral AHBs colonies. Army ants, for instance, are some of the major predators of stingless bees, but these have evolved different mechanisms to efficiently defend their colonies (Quezada-Euán, 2018). Instead, army ants frequently decimate AHBs colonies in apiaries, whilst only a few stingless bee colonies are lost in meliponarios (Quezada-Euán, 2018).
The Africanization of honey bees has resulted in extensive and more frequent interactions between honey bees and native bees (Roubik, 1989). One potential problem of such close interactions is the transmission of parasites and diseases, the dynamics of which are still not well understood. It is known that AHBs can be more resistant to some parasites like the mite Varroa destructor (Guzman-Novoa et al., 1999; Martin and Medina-Medina, 2004) that has decimated colonies of EHBs elsewhere (Guzman-Novoa et al., 2010). AHBs can also be more resistant than EHBs to viral infections (Hamiduzzaman et al., 2015). Seemingly, most honey bee parasites are not transmissible to native stingless bees and no major epidemics have been recorded in the latter (Quezada-Euán, 2018). However, several viruses found in honey bees have recently been detected in other bee species (McMahon et al., 2015; Tehel et al., 2016), raising the possibility of spill-over from AHBs to native bees. Honey bee viruses have been found in native stingless bees from Brazil (Ueira-Vieira et al., 2015) and Mexico (Guzman-Novoa et al., 2015). Surprisingly, although honey bee viruses have been found to replicate in Mexican stingless bees, no signs of disease have been found in colonies (Tapia-González et al., 2019; Morfin et al., 2020). Notably, a study conducted in the Brazilian island of Fernando de Noronha, found ubiquitous presence of deformed wing virus type C (DWV-C) in M. subnitida colonies, but rarity in A. mellifera, which suggested limited viral exchange between these two species (De Souza et al., 2019). It is possible that the so-called honey bee viruses may be generalist bee viruses or insect viruses and thus they could spread also from native bees to honey bees. More research is thus needed to clarify the issue of potential pathogen spillover between different bee species and other pollinators. AHBs seem to be also tolerant to other parasites that are damaging to EHBs, such as Nosema ceranae (Fleites-Ayil et al., 2018), and this microsporidian has also been found to multiply in M. colimana, an endemic stingless bee from Jalisco, Mexico (Macías-Macías et al., 2020). However, not much more is known about the potential virulence of honey bee pathogens in native bees. Clearly, the nature and extent of disease spillover from and between AHBs and native bees is still in early stages and deserves further investigation.
The Economic Impact of AHBs in Mexico
Honey Production
Honey production is a complex trait affected by many factors including floral resources, climate, colony populations and beekeeping practices. However, under similar environmental conditions of food resources, climate and management, differences in honey yields between colonies are directly associated to the population size of colonies, the length of life of forager bees and the foraging efficiency of bees (Woyke, 1984; Guzman-Novoa and Gary, 1993; Becerra-Guzmán et al., 2005). Therefore, differences in these traits between bee ecotypes could partially explain differences in honey production.
For reproduction rates, it is well established that AHBs reproduce at a faster rate than EHBs (Winston, 1979, 1992). For length of life and foraging efficiency, few studies have analyzed and compared AHBs and EHBs in a Mexican environment. Becerra-Guzmán et al. (2005) established colonies of both bee ecotypes in Tonatico, Mexico, and co-fostered marked AHBs and EHBs in the same colonies to study genotypic effects on their lifespan and foraging behavior. Both, EHBs and AHBs varied for length of life within a range of 20–26 days, but there was no significant difference for this trait between the two types of bees. Hive environment greatly influenced the lifespan of both bee types, indicating that genetic effects have less influence on this trait than environmental effects. By comparison, the length of life of worker bees of colonies from South America was reported to vary and no clear difference for this trait was found between EHBs and AHBs (Winston and Katz, 1981), which is similar to what Becerra-Guzmán et al. (2005) concluded in Mexico. What differed between the two bee types in the study by Becerra-Guzmán et al. (2005) was their foraging life; AHBs had shorter foraging lives (9 ± 0.4 days) than EHBs (12 ± 0.5 days), which could have a significant impact on food stores and honey yields of colonies. Additionally, work done in the same region of Mexico, showed that AHBs and EHBs did not differ in foraging force, number of total foraging trips and amount of nectar or pollen collected per individual bee, but they differed in how the foraging force was allocated. AHBs dedicated a significantly lower proportion of nectar foragers and a significantly higher proportion of pollen foragers in comparison with EHBs (Neuman, 2001). Other studies conducted in South America have reported that when different components of foraging behavior are considered altogether, such as the number of trips to flowers, as well as the quantity and quality of the nectar (sugar concentration of the nectar) transported to the hive, there are no differences between AHBs and EHBs for the amount of calories that each individual bee dedicated to the collection of nectar contributes to its colony (Rinderer et al., 1985a; Pesante et al., 1987; Rinderer and Collins, 1991). However, it has been well established that AHBs collect more pollen than EHBs because they allocate a higher proportion of their foraging force to collect pollen than bees of EHB colonies. Danka et al. (1987) compared the proportion of foragers dedicated to pollen foraging in colonies of both bee types in Venezuela, and found that more than 30% of the bees from AHB colonies performed pollen trips, while less than 15% of the bees from EHB colonies did it. Fewell and Bertram (2002) corroborated these findings in Arizona.
The consistency of results on the length of life, foraging efficiency and foraging strategy of honey bees in studies conducted in Mexico and South America, indicate that the main differences for these traits between AHBs and EHBs are longer foraging lives and higher proportions of foragers dedicated to nectar collection in EHB colonies. These differences in foraging life and foraging strategy give an advantage to EHBs over AHBs for honey production, and an advantage to AHBs for increased reproduction and colonization of new environments.
Honey production has decreased in all countries where AHBs have become established in at least half of their territory, with the exemption of Brazil (Gonçalves et al., 1991; Rinderer and Collins, 1991; Guzman-Novoa and Page, 1994b; Caron, 2001). This is one of the reasons why there is controversy about whether these bees are better suited for honey production than EHBs. The controversy has not been totally resolved because few side by side studies between colonies of the two bee eco-types have been conducted to compare them for honey yields (Kerr, 1967; Rinderer et al., 1985b; Spivak et al., 1989). Moreover, the number of colonies used in those studies (<15 per treatment) was low for valid statistical comparisons (small sample sizes) because the variation for honey yields among colonies is high (Uribe-Rubio et al., 2003). Additionally, the results of the above studies have been inconsistent. For example, Kerr (1967) found that AHBs were more productive than EHBs, Rinderer et al. (1985b) concluded that EHBs produced more honey than AHBs, and Spivak et al. (1989) did not find differences between colonies of the two bee types. Clearly, studies with a larger number of colonies conducted in different environments are necessary to obtain consistent results and to draw firm conclusions about the effect that the Africanization of honey bee populations has on honey production. Considering the above, Guzman-Novoa and Uribe-Rubio (2004) compared the honey yields of 118 EHB, 80 AHB and 84 hybrid colonies in a temperate region of Mexico. EHB colonies yielded over 30% more honey than AHB and hybrid colonies of Africanized maternal ancestry, which was significant. Hybrid bee colonies of European maternal ancestry produced slightly more honey than EHB colonies, but their yields were not significantly different. Additionally, hybrid colonies of Africanized maternal ancestry produced similar yields of honey to those of AHB colonies (Figure 2). These results demonstrate that colonies of primarily African ancestry produce less honey than colonies of primarily European ancestry. Moreover, they suggest maternal or dominance effects for honey production, and demonstrate that Africanization decreases honey yields of honey bee colonies, at least under the temperate environment of Mexico where they were tested. Uribe-Rubio et al. (2003), working in the same geographical area where the study by Guzman-Novoa and Uribe-Rubio (2004) was conducted, had previously found in a large study involving 416 colonies, that colonies having bees with African mtDNA produced significantly less honey than those having bees with European mtDNA. Therefore, it does not seem advantageous to work with populations of honey bees with a high degree of Africanization if honey yields are the primary objective of beekeeping operations. Mexican beekeepers have to work with AHBs because they are present and well adapted to most beekeeping regions in Mexico, but perhaps they can reduce the level of Africanization of colonies through selective breeding.
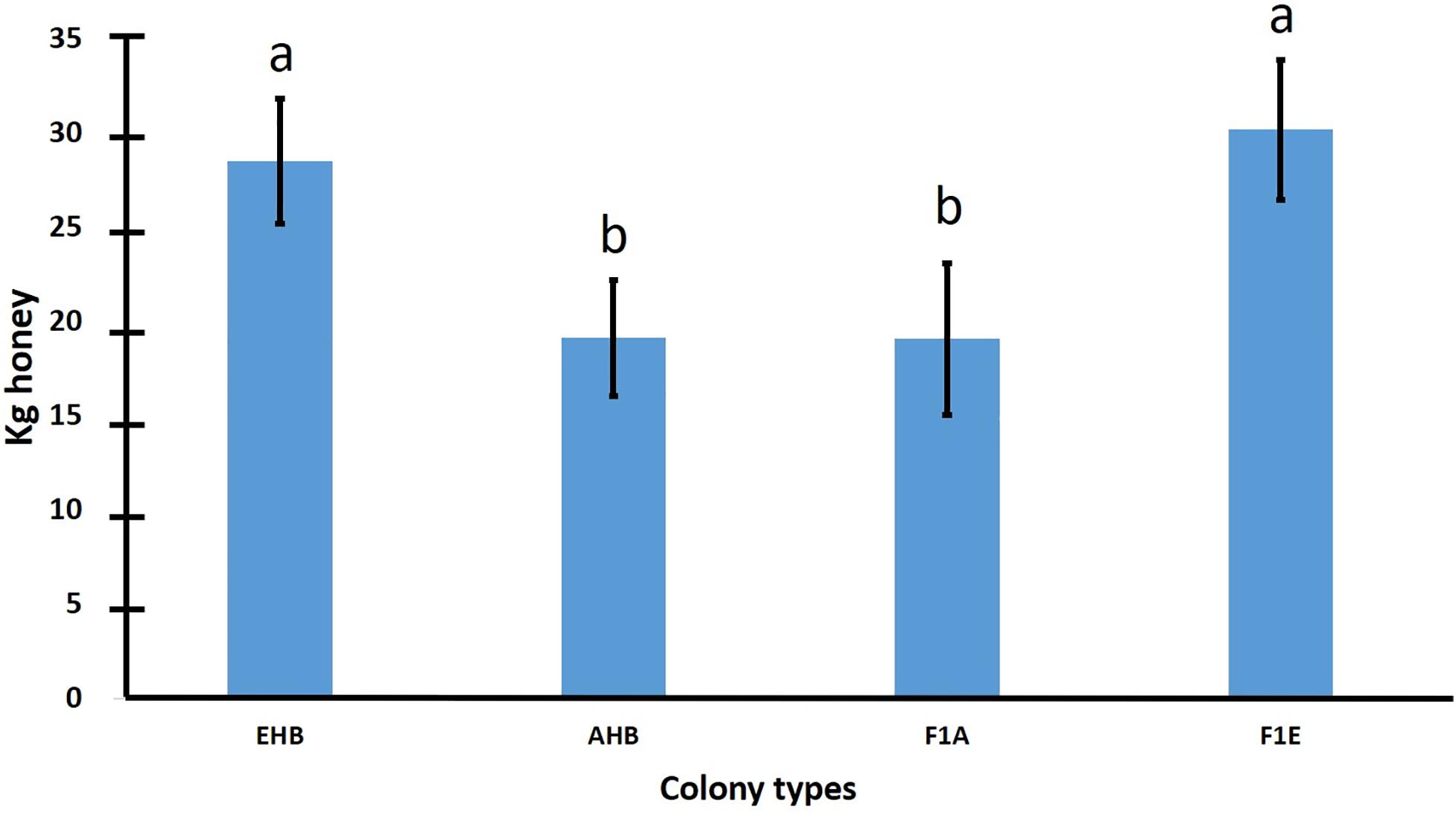
Figure 2. Mean (± SE) honey yield per hive for 282 honey bee colonies of European, Africanized and hybrid genotypes (data from Guzman-Novoa and Uribe-Rubio, 2004).
The reasons why EHB and AHB colonies vary for stored honey that can be harvested are not well known, but they may be related with the behavioral adaptations of the two bee ecotypes to different environments in the regions of the world where they evolved (Page, 1989). AHB colonies collect more pollen and less nectar compared to EHB colonies of similar strength because they allocate less bees for nectar collection than EHB colonies (Danka et al., 1987; Pesante et al., 1987). Additionally, AHB colonies tend to keep lower food stores than EHB colonies because they use more of their food resources for reproduction than EHB colonies (Pesante et al., 1987; Winston, 1992). Another factor that may explain, at least in part, why AHB colonies produce on average less honey that EHB colonies, is a higher swarming frequency (Winston, 1992). Colonies swarming before or during the nectar flow season will be less populated than colonies that do not swarm and therefore will be less productive. Also, absconding (hive abandonment), a tactic of tropical bees to avoid starvation and predation, can partially explain the lower mean honey yields per colony in Africanized areas of Mexico (Guzman-Novoa et al., 2011).
The above studies have shown that at the colony level, AHBs seem to produce less honey per hive than EHBs, at least under temperate environments in Mexico. At the country level, it is more difficult to establish the actual impact that the Africanization of honey bees has had on honey production. Nevertheless, coincidentally with the arrival and spread of AHBs in Mexico, the production of honey in the country, progressively decreased within 10 years after that event (Guzman-Novoa et al., 2011).
Even when honey production decreased in Mexico during the first decade after the arrival of AHBs, the reduction was not as severe as in Venezuela or Central America (Hellmich and Rinderer, 1991; Guzman-Novoa, 1996; Caron, 2001), probably because there is better beekeeping infrastructure in Mexico than in those countries (Guzman-Novoa, 1996, 2004; Programa Nacional para el Control de la Abeja Africana [PNCAA], 2010). In 1986, the year when AHBs arrived in Mexico, the country’s honey production was 74,613 tons, whereas honey exports exceeded 48,000 tons (Sader, 2020). Ten years after their arrival, in 1996, honey production was estimated to be under 49,000 tons, while honey exports were less than 27,000 tons, which is equivalent to 66 and 56% of the honey that was produced and exported, respectively, the year of arrival of AHBs to the country. Twenty years after the arrival of AHBs, in 2006, honey production exceeded 55,000 tons and honey exports reached 29,000 tons (Sader, 2020), which reflects a slight increase relative to 10 years before (Figures 3, 4), despite the fact that new detrimental factors have menaced the beekeeping industry of Mexico, such as the discovery of the parasitic mite V. destructor in 1992 (Chihu et al., 1992) and destructive climatic events, such as hurricanes (Guzman-Novoa et al., 2011). One decade later, in 2016, honey production statistics were similar to those of 2006. This pattern of decrease in honey production, followed by a posterior increase, is consistent with what has happened in Brazil and Venezuela. Initially, beekeepers experienced a loss of hives and production, but when they adopted new management practices and replaced or increased their lost colonies, honey production increased gradually (Hellmich and Rinderer, 1991; Rinderer and Collins, 1991; Gonçalves et al., 1991). Despite the partial increase of honey production in the last two decades, total honey production in Mexico has not been recovered to the levels prior to the Africanization of colonies. Selective breeding could contribute to increase honey production as Guzman-Novoa and Page (1999a, b) demonstrated, using local populations of AHBs in which they increased the frequency of EHB genes and colony honey yields, in an Africanized region of Mexico.
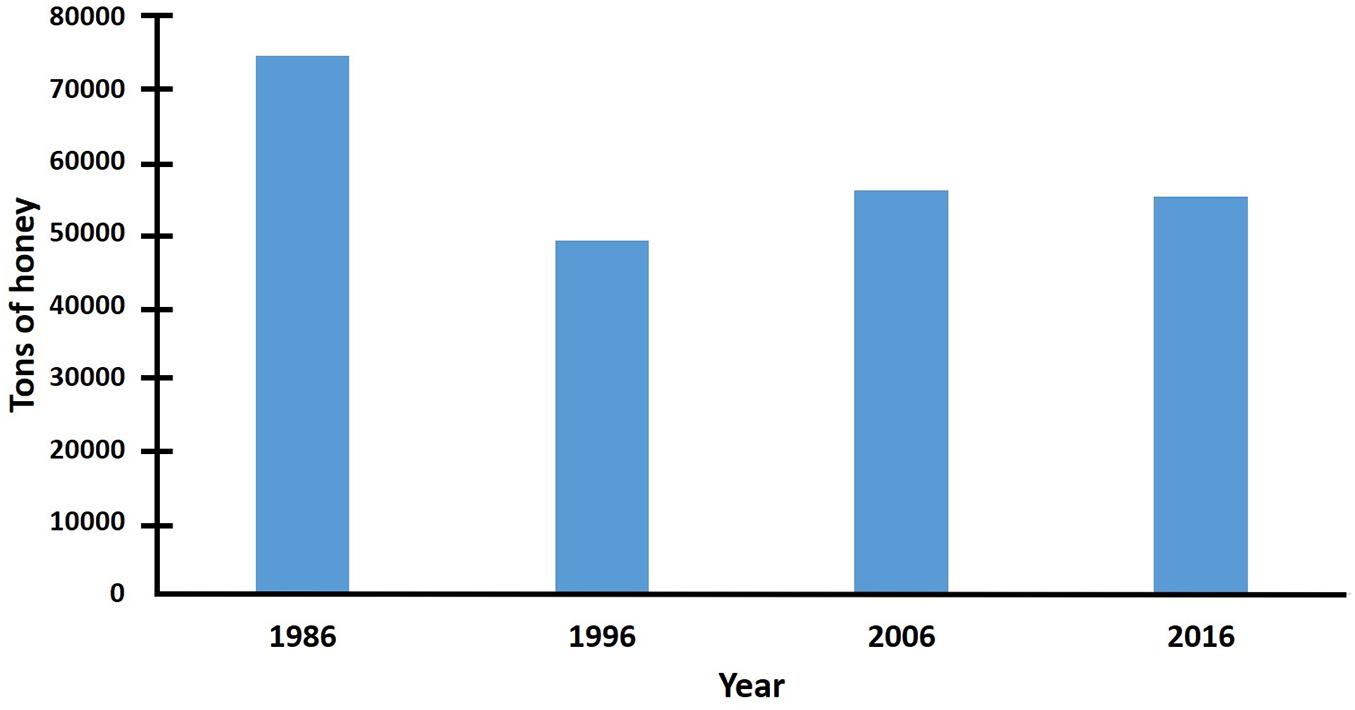
Figure 3. Honey production in Mexico the year of the arrival of AHBs (1986), 10 (1996), 20 (2006), and 30 (2016) years later (data from Sader, 2020).
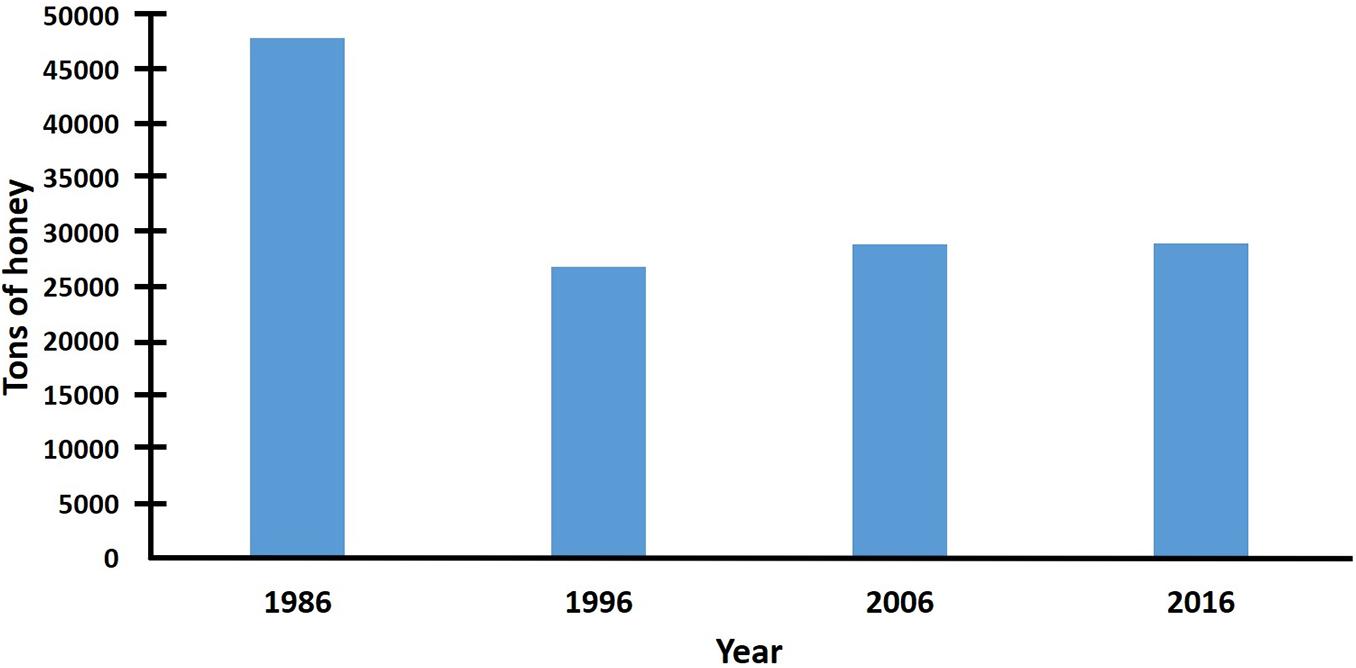
Figure 4. Honey exports in Mexico the year of the arrival of AHBs (1986), 10 (1996), 20 (2006), and 30 (2016) years later (data from Sader, 2020).
Defensive Behavior
One of the most noticeable changes in honey bee colonies as a result of Africanization, is their increased defensiveness, which occurs at a rapid pace (Quezada-Euán and Paxton, 1999). AHBs react faster, with more individuals that pursue and sting, and their defensive responses last longer compared with EHB colonies (Stort, 1975a,b; Collins et al., 1982; Collins and Rinderer, 1991; Guzman-Novoa et al., 1994, 2002a,b; Giray et al., 2000; Uribe-Rubio et al., 2003; Breed et al., 2004). Numerous studies conducted in Mexico have contributed to better understand how environmental and genetic effects, as well as worker interactions, affect the defensive behavior of AHBs, and how this behavior has impacted society and beekeeping practices.
The defensive behavior of honey bees consists of several tasks, including guarding, pursuing and stinging (Breed et al., 2004). Guarding is a specialized behavior performed by less than 40% of the bees in a colony (Moore et al., 1987; Unger and Guzman-Novoa, 2012). Guards inspect incoming bees with their antennae at the colony entrance to distinguish foreign bees and other invertebrates from nestmates. They use olfactory cues to allow nestmates enter the colony and to reject foreign bees and other invertebrates (Moore et al., 1987). When guards cannot repel intruders, particularly vertebrates, they release alarm pheromones to recruit bees from the interior of the hive to pursue and sting intruders (Breed et al., 2004). Guards thus, play an important role in impeding the entrance of invertebrates to the colony and in recruiting nestmates to defend their colony against vertebrates.
To analyze genetic and environmental effects on the guarding behavior of AHBs and EHBs in Mexico, Hunt et al. (2003a) co-fostered known numbers of individually marked AHBs and EHBs of the same age in common colonies of both genotypes, and observed their guarding behavior. They found that AHBs guarded at a younger age, in higher numbers and during more days than EHBs. On average, AHBs guarded for 5 days and EHBs for 3 days. They concluded that genotypic, environmental and genotype x environmental effects significantly increased guarding bouts in the AHB genotype. In another study, Hunt et al. (2003b) reported that when bees of the two genotypes co-fostered in a common colony were exposed to alarm pheromone components (emulating a recruiting event by guard bees), AHBs responded in greater numbers.
Soldiers (the bees recruited by guards) comprise another group of defenders that pursue intruders to deter them from the colony and sting them if they do not go away (Breed et al., 2004). The association of guarding and soldiering tasks was demonstrated by Guzman-Novoa et al. (2004), who found a correlation between individual guarding and other components of defensive behavior, such as response to alarm pheromone, pursuing tendency, and stinging. Moreover, the distance and number of bees pursuing experimenters was the component that best separated gentle and defensive colonies. The mean number of pursuing AHBs at different distances was >81 times greater than that of pursuing EHBs (Guzman-Novoa et al., 2003).
Environmental effects and worker interactions also affect the defensive behavior of honey bees (Uribe-Rubio et al., 2003, 2008, 2013). It is likely that in Africanized areas, honey bee colonies are composed of multiple worker genotypes because queens mate with many drones of different genetic origins. To emulate these conditions, colonies were assembled by co-fostering EHBs and AHBs in common colonies in Tonatico, Mexico, and their stinging responses were quantified with leather patches presented at different times. During the first 10 s of the test, 81% of the bees that stung were AHBs, but from 10 to 30 s, AHBs and EHBs were equally likely to sting. However, when tested in their own environments, bees of two of the three EHB colonies used did not sting and did not pursue in any of the eight trials conducted, whereas all three AHB colonies did (Guzman-Novoa et al., 2004). These results suggest behavioral interactions among bees of different genotypes when they share a common nest. The more defensive type of bees may affect the response thresholds of less defensive bee genotypes, recruiting them to sting. Similarly, in a previous study, changes in propensity to sting in a gentle bee genotype were demonstrated. Co-fostered EHBs were more likely to sting in colonies containing hybrid (Africanized/European) bees than in their natal nests (Guzman-Novoa and Page, 2000).
In addition to environmental influences and worker interactions, genetic effects strongly influence the defensive behavior of AHBs (Hunt et al., 2007). Their intense defensive responses are highly heritable and apparently genetically dominant (Stort, 1975a,b; Collins and Rinderer, 1991; Guzman-Novoa and Page, 1994a; DeGrandi-Hoffman et al., 1998a; Hunt et al., 1998; Guzman-Novoa et al., 2002a), but when crossed with EHBs and analyzed by type of hybrid or backcross, it was found that AHB colonies in Mexico are strongly affected by paternal effects. Paternal effects could explain why in each of four sets of crosses, bees from hybrid colonies of Africanized paternity left more stings in leather patches than bees from hybrid colonies of European paternity (Guzman-Novoa et al., 2005). Although some degree of dominance was observed for high-defensive behavior in crosses involving European paternity, most of the dominance effects appear to be the result of paternal effects (Figure 5). Paternal effects on the defensive behavior of honey bees may explain in part the ecological success of AHBs. Defensive traits are beneficial to honey bee colonies in tropical environments because they help them reduce predation (Rinderer and Hellmich, 1991). Two potential mechanisms may explain a paternal effect for defensive behavior. First, interactions between European mitochondrial genes and African nuclear alleles could result in a more defensive response. Another explanation for the observed paternal effects is that imprinting mechanisms selectively reduce expression of maternal alleles or increase expression of paternal alleles. Imprinting usually is caused by methylation that silence particular alleles depending on the sex of the parent from which the alleles were inherited (Constancia et al., 1998).
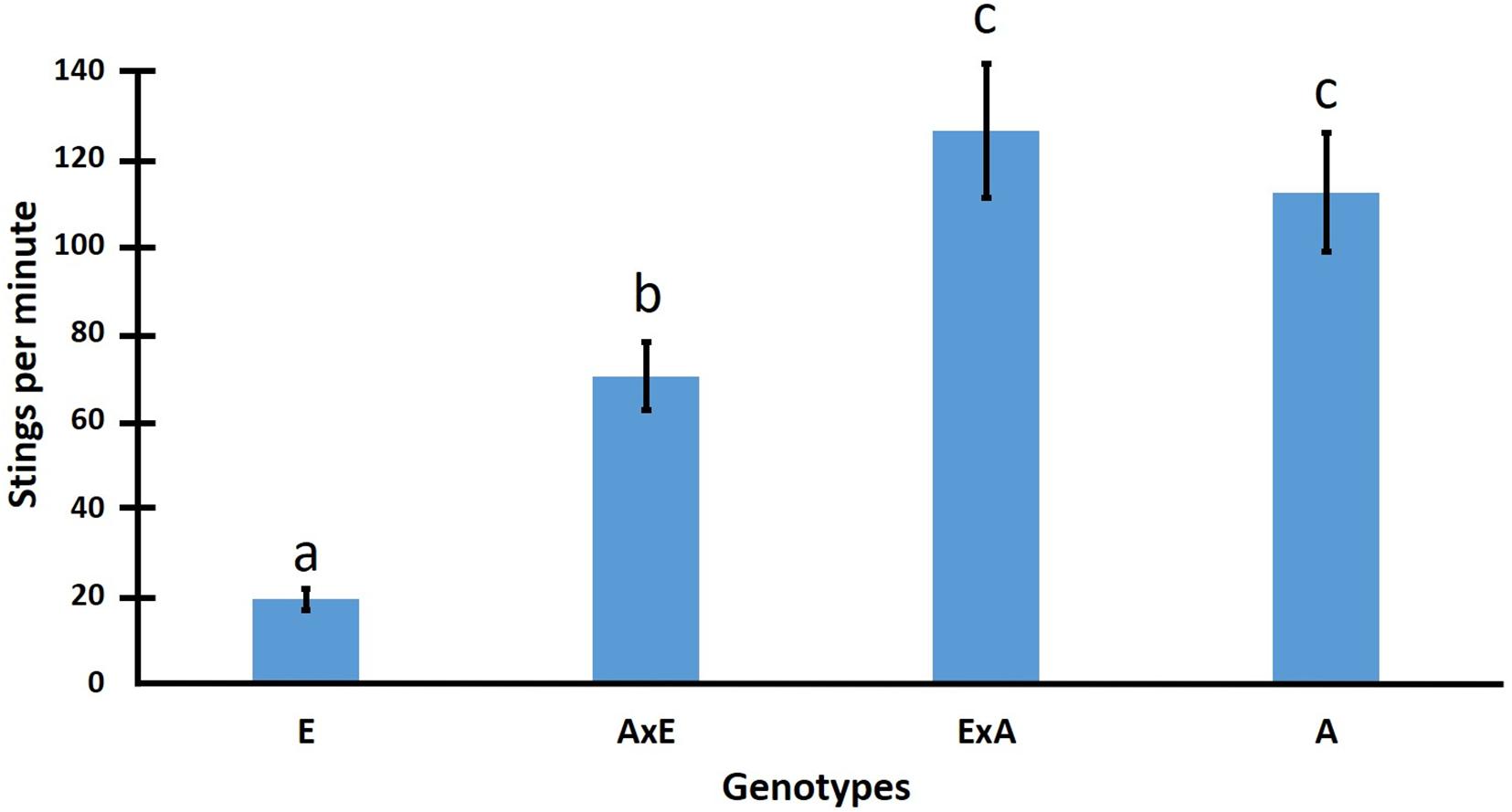
Figure 5. Mean number of stings per minute on leather patches from four years of testing for colonies of four honey bee genotypes: E (European), AxE (F1 of European paternity) ExA (F1 of Africanized paternity), A (Africanized) (data from Guzman-Novoa et al., 2005).
Five putative quantitative trait loci (QTLs), including sting-1-5 that influence honey bee defensive behavior were mapped in a population of more than 300 backcrossed colonies tested in Mexico (Hunt et al., 1998). In another study, the marker genotypes near sting-1 were associated with the tasks of guarding and fast stinging, thus confirming the effects of sting-1 on defensive behavior (Guzman-Novoa et al., 2002b). Seven additional QTLs that regulate alarm pheromone production were mapped in Mexican AHB backcrossed colonies (Hunt et al., 1999). Other genes that regulate and have influenced the evolution of defensive responses in AHBs and EHBs were identified with microarray studies conducted in Mexico (Alaux et al., 2009; Ament et al., 2012). Recent work using pooled sequencing of AHBs identified 65 loci associated to defensive behavior from colonies that diverged in defensive responses, and showed that they contained African and European alleles that interacted (Harpur et al., 2020). By comparison, the apparently gentle AHB of Puerto Rico (Rivera-Marchand et al., 2008) differs in genetic structure from AHB populations in mainland United States, probably as a consequence of local selection and evolutionary processes on the island (Galindo-Cardona et al., 2013; Avalos et al., 2017; Acevedo-Gonzalez et al., 2019). Therefore, it is possible that different sets of genes may affect the defensive behavior of AHBs in different populations as a consequence of regional selection and interaction of African and European alleles.
Clearly, the numerous studies that have been conducted in Mexico have evidenced that the defensive behavior of AHB populations in the country varies, but it is clearly more pronounced than that of EHB populations and is affected by genetic effects, environmental effects, and complex interactions between worker bees in their nests. This higher level of defensiveness of AHBs has impacted human and animal health, and the beekeeping industry of Mexico.
Impact of Defensive Behavior
For many people in Mexico, the characterization of AHBs as “killer bees” added to public awareness of honey bee defensive behavior. Unfortunately, this characterization has created a fear-driven public climate in some regions of the country that is often antagonistic to populations of honey bees, regardless of actual hazards. This is because isolated stinging incidents and human fatalities cause a great impression in people and are not easily forgotten (Guzman-Novoa et al., 2011). Beekeepers are able to take precautions in the apiary and avoid serious stinging incidents, but it is not always possible to prevent interactions between the public and managed AHBs, particularly in dense populated areas. Incidents often occur when animals or humans walk nearby apiaries that have been recently manipulated by beekeepers, and are more common just before the main nectar flow because colonies are strongly populated (Guzman-Novoa et al., 2011).
The number people killed by honey bees in Mexico between 1988 and 2009 was 21.8 per year, or 0.23 per million people (Becerril-Ángeles et al., 2013), which is relatively insignificant. By comparison, scorpion stings cause 2.87 fatalities per million people (Celis et al., 2007). Therefore, the probability of being killed by honey bee stinging incidents in Mexico is about 12 times lower than being killed by scorpion stinging incidents, and it is 3,500 times less likely to die of bee stings than to die of diabetes (Becerril-Ángeles et al., 2013). However, despite the low probability of mortality, the arrival of AHBs temporarily increased the number of human fatalities between 1988 and 1993 and then progressively decreased in the following years until 2009, the last year of recorded fatalities by honey bee stings in Mexico (Figure 6). From 1988 to 2009 there were 480 fatality cases, and more than 70% of them were people 50 years of age or older (Becerril-Ángeles et al., 2013). It is believed that the gradual decrease in the number of human fatalities between 1994 and 2009 was probably due to the sum of several factors, including more awareness in the population about the danger that approaching honey bee nests and hives represents, the elimination of more than 100,000 swarms annually by fire fighters (Becerril-Ángeles et al., 2013), the relocation of apiaries away from urban areas and main roads by beekeepers, and a higher requeening rate of honey bee colonies with gentler stock (Guzman-Novoa et al., 2011).
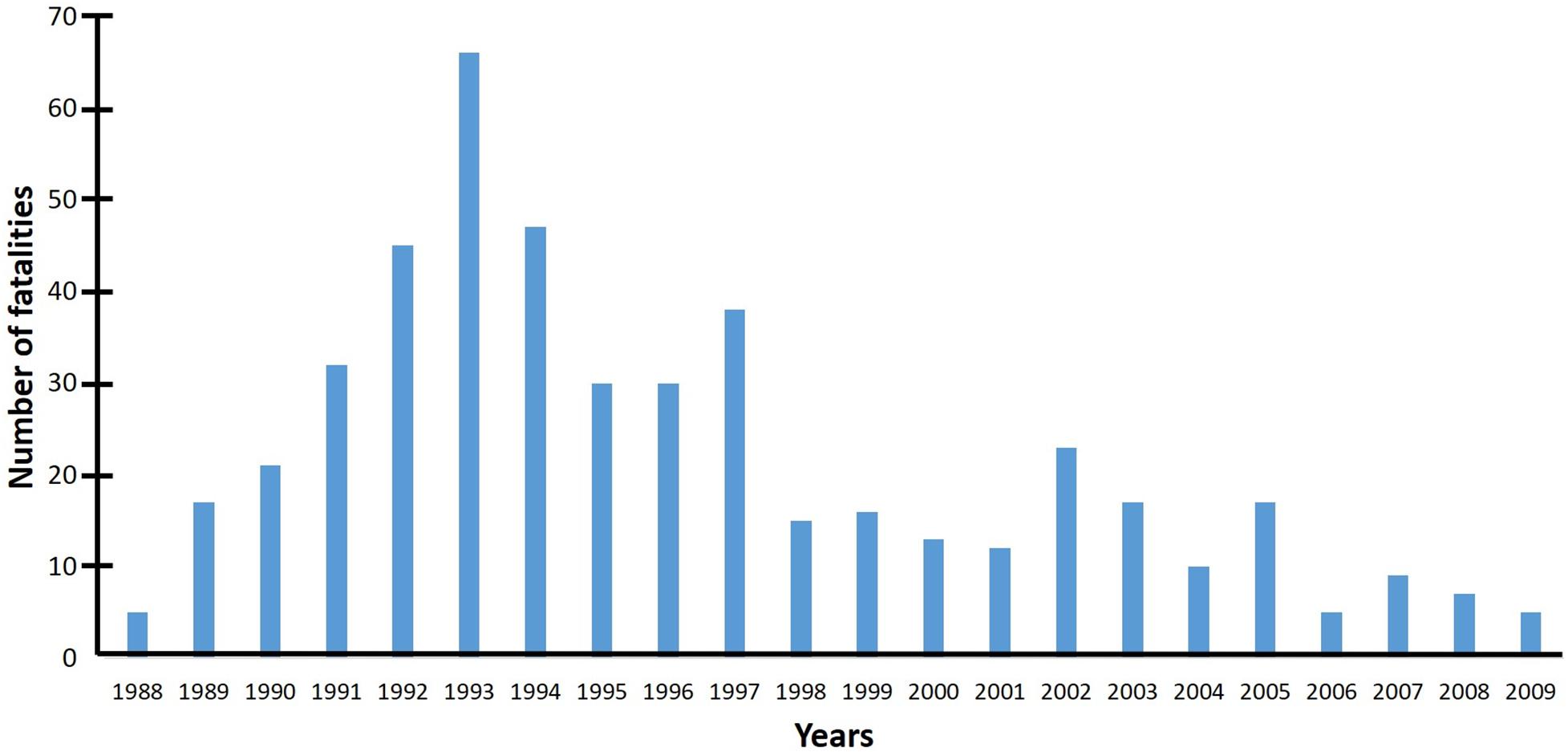
Figure 6. Number of death people from honey bee stings in Mexico between 1988 and 2009 (data from Becerril-Ángeles et al., 2013). By comparison, the probability of being killed by honey bee stinging incidents in Mexico is about 12 times lower than the probability of being killed by scorpion stinging incidents.
Management Practices and Breeding
A consequence of working with more defensive bees after the arrival of AHBs is that some beekeepers have abandoned the activity, or in the case of commercial beekeepers, reduced their colony numbers, because finding locations suitable for apiaries has become more difficult. Landowners who have fields suitable for beekeeping do not easily accept the establishment of hives within their property because they want to avoid problems related to stinging incidents (Guzman-Novoa et al., 2011). In fact, large operations of more than 5,000 hives mainly dedicated to honey production went out of business. Examples of this were “Miel Carlota,” “Acapulco Miel,” and “Veramiel” companies with more than 40,000, 20,000, and 10,000 colonies, respectively. The only large beekeeping operations remaining in Mexico after the colonization of AHBs of most beekeeping regions of the country, are those mainly dedicated to rent their colonies for crop pollination, because hive rental fees are high enough to provide profits above hive management costs (Magaña-Magaña et al., 2016). Those operations use bee stocks selected for reduced defensive behavior (Guzman-Novoa et al., 2011).
Although large commercial beekeeping operations have decreased since the arrival of AHBs, the number of small-scale and sideline beekeepers owning between 20 and 500 hives has increased, and it is expected that this trend continue (Guzman-Novoa et al., 2011). These beekeepers do not hire labor because they manage their own colonies or receive help from family members and relatives, with which labor costs and the difficulty of finding beekeeping employees do not affect them as much as large commercial companies are affected. Furthermore, their apiaries are normally located on sites close to their place of residence. Therefore, they do not have the transportation costs or the difficulties associated with finding suitable locations to place their apiaries as large commercial beekeeping companies have experienced.
Mexican beekeepers have adapted to the “new” bees by changing their management practices to better deal with some of the pronounced behaviors of AHBs, particularly their extreme defensive, swarming, and absconding behaviors. Small-scale beekeepers have adopted fewer changes than commercial beekeepers, but all have changed their management practices to some extent. According to Guzman-Novoa et al. (2011), among the practices most commonly adopted by beekeepers to manage honey bee colonies in Africanized areas of Mexico are: (1) the use of better protective equipment such as coveralls and gloves, (2) the requeening of defensive colonies with queens from gentler stock, (3) the relocation of apiaries to isolated areas to reduce the probability of stinging incidents involving humans and livestock, (4) the feeding of colonies during dearth periods to decrease colony loses due to absconding behavior, and (5) the more frequent swarming control and honey harvests.
The above practices have improved the management and productivity of honey bee colonies in Africanized areas. Requeening is an important measure because queens in tropical environments are frequently superseded. In a study conducted in an Africanized area of Mexico, the queens introduced into more than 350 colonies were monitored for 1 year. After 6 and 12 months, only 61% and less than 30% of the introduced queens, respectively, were found in the colonies (Guzman-Novoa et al., 1998). Therefore, the requeening of colonies is recommended as a minimum once a year (Guzman-Novoa et al., 2011). Fortunately, queen acceptance rates in AHB colonies are as high as those in EHB colonies (Guzman-Novoa et al., 1997). Additionally, the relocation of apiaries has decreased the number of stinging incidents (Becerril-Ángeles et al., 2013), and the last two measures have resulted in increased honey yields (Guzman-Novoa et al., 2011).
Previous studies have demonstrated that if the Africanization level of colonies is 25% or lower, bees of these colonies do not differ in stinging behavior with EHB colonies (Hellmich, 1991; Guzman-Novoa and Page, 1993, 1999a,b). Therefore, selective bee breeding could increase honey yields and decrease the defensive behavior of AHB populations by decreasing the Africanization level of colonies. Guzman-Novoa and Page (1999b) conducted a long-term selective breeding program in an open honey bee population of more than 3,000 colonies located in an Africanized area of Mexico. This program is the largest selective breeding program so far conducted in Africanized bee regions. After 5 years of selection, honey yields increased 16%, stinging behavior decreased 54%, and the length of worker wings increased >1%. Additionally, the percentage of colonies having bees with African mtDNA decreased from 28% before selection to 7% after four generations of selection. These results suggest that the level of Africanization in the selected population was reduced to some degree over time, and demonstrate that it is possible to breed gentler and more productive bees in Africanized areas without the use of instrumental insemination of queen bees. Programs like this, stimulated the implementation of other selective breeding programs for low defensive behavior (Esquivel-Rojas et al., 2015) and also queen breeders who selectively breed honey bee stock for higher honey yields and lower defensiveness, have considerably increased the number of queens they rear and sell. In fact, according to the Mexican association of queen breeders, the number of queens reared tripled in 30 years (Guzman-Novoa et al., 2011). In the Yucatan peninsula, selection of AHBs has also resulted in increased honey yields, disease resistance and lower defensiveness. Those promising results suggest that selection of AHBs, rather than the introduction of European stock seems to be the best option for tropical areas (Quezada-Euán et al., 2008; Zárate et al., 2008).
Conclusion and Further Directions
The Africanization of the honey bees of Mexico has resulted in extensive hybridization strongly driven by climatic conditions. As a result, AHB colonies are amongst the most genetically diverse A. mellifera yet recorded (Tarpy et al., 2010; Harpur et al., 2012, 2020). Notably, AHBs do not resemble anymore their original ancestors, they are genetically and biologically distinct, and might be considered a separate A. mellifera lineage well adapted to the Neotropics. Presently, the managed colonies of honey bees in Mexico show two contrasting patterns, one mainly composed by AHBs in the tropical beekeeping regions of the Yucatan peninsula, the Gulf of Mexico’s coast and the Pacific Coast, and another where EHBs predominate, in the temperate beekeeping regions of the high plateau and the North of the country. However, little is known about the composition of feral populations elsewhere in the country. Future studies of Mexican honey bees involving high-density molecular markers should be useful in revealing their population genetic structure, especially in lesser studied regions. No information exists on the patterns of introgression at nuclear loci. Thus, the level of selection that has occurred for African and European-derived genes in different regions as well as the genetic stability of such populations is still unclear. Moreover, possible AHBs-EHBs clines and hybrid zones may exist in Mexico, but this has not been studied extensively (see Kraus et al., 2007).
The Africanization of honey bees has resulted in lower honey yields per colony in temperate climates of Mexico, but how it has affected honey yields in the tropics is unknown. Therefore, side by side comparative studies of honey production and about the underlying factors that affect this trait between AHB and EHB colonies in the tropics are warranted. The defensive behavior of bees in Mexico has increased, but the impact of this behavior on stinging incidents involving humans has been relatively insignificant. A new generation of beekeepers emerged and learned how to manage AHBs to reduce their impact and to make them more productive. A corollary is that breeding programs in Mexico and elsewhere in the Neotropics, may benefit from using honey bees that are already present and better adapted to their respective tropical and temperate zones. These populations have been largely confronted to various environmental conditions and in the process are better adapted compared with foreign stock (Domínguez-Ayala et al., 2016). This is particularly relevant considering the low frequency of massive colony losses in Mexico and Latin America due to Colony Collapse Disorder and diseases, compared to what occurs in the United States and Europe (Vandame and Palacio, 2010). Moreover, the discovery and use of nuclear markers for assisted selection, should accelerate the breeding of gentler, more productive and disease resistant honey bees.
In comparison with the economic impact, in Mexico (as well as all over the Americas), the assessment of ecological impacts as a result of the Africanization of honey bees is meager. Although some positive effects have occurred, such as the increase of pollination services to some crops and the concomitant impulse of stingless beekeeping, the large-scale impact of AHBs on native ecosystems as well as on specialized bee-plant interactions is virtually unknown. Further studies should focus on ways that help maintaining an equilibrium between AHBs and native species. Better characterization of pollination mutualisms in wild and managed systems is also crucial to ensure habitat stability and agricultural production (Roubik, 2000). The evidence suggests that under limited food resources, competition can become more intense, possibly leading to limits where the systems can no longer adapt (Roubik, 2009). Therefore, one way to protect such equilibrium is by preserving and, if possible, increasing natural areas with rich floral resources, especially in regions where beekeeping is intensively practiced. Recent evidence suggests that AHBs may tolerate high levels of heat stress (Medina et al., 2018; Medina et al., 2020; Poot-Baez et al., 2020), a fact that should be considered for the sustainability of beekeeping under the threat of climate change but, comparative studies with EHBs are still needed. Research could help to better understand the basis of biological adaptations and behaviors of AHBs to develop technologies aimed at making them more productive and less defensive. AHBs are in the Americas to stay and the challenge is to make sustainable use of them while preserving the ecosystems in which they now thrive.
Author Contributions
EG-N, NM, JT-G, JM-M, FC-E, and JQ-E contributed to the original idea and structure of this manuscript. CM-F and AC-B collected the data. JQ-E and JM-M prepared the figures. AD and NM formatted the manuscript. All authors contributed to write and revise the final version of the manuscript.
Funding
CONACyT projects 291333 “Manejo sustentable de polinizadores,” 1556 “Rescate, conservación y mejoramiento genético de los recursos apícolas en México (Hymenoptera: Apidae),” and 103341 “Conservación de las abejas sin aguijón de México” supported part of the research included in this review. Publication costs were funded by a Pinchin Family grant to EG-N.
Conflict of Interest
The authors declare that the research was conducted in the absence of any commercial or financial relationships that could be construed as a potential conflict of interest.
Acknowledgments
We thank the reviewers of this article for their comments aimed at improving the quality of this manuscript.
References
Acevedo-Gonzalez, J. P., Galindo-Cardona, A., Avalos, A., Whitfield, C. W., Rodriguez, D. M., Uribe-Rubio, J. L., et al. (2019). Colonization history and population differentiation of the honey bees (Apis mellifera L.) in Puerto Rico. Ecol. Evol. 9, 1–8. doi: 10.1002/ece3.5330
Alaux, C., Sinha, S., Hasadsri, L., Hunt, G. J., Guzman-Novoa, E., DeGrandi-Hoffman, G., et al. (2009). Honey bee aggression supports a link between gene regulation and behavioral evolution. PNAS 106, 15400–15405. doi: 10.1073/pnas.0907043106
Ament, S. A., Blatti, C., Alaux, C., Wheeler, M. M., Toth, A. L., Le Conte, Y., et al. (2012). New meta-analysis tools reveal common transcriptional regulatory basis for multiple determinants of behavior. PNAS 109, 1801–1810. doi: 10.1073/pnas.1205283109
Avalos, A., Pan, H., Li, C., Acevedo-Gonzalez, J. P., Rendon, G., Fields, C. J., et al. (2017). A soft selective sweep during rapid evolution of gentle behaviour in an Africanized honeybee. Nat. Commun. 8:1550. doi: 10.1038/s41467-017-01800-0
Becerra-Guzmán, F., Guzman-Novoa, E., Correa-Benítez, A., and Zozaya, A. (2005). Length of life, age at first foraging, and foraging life of Africanized and European Honeybee (Apis mellifera L.) workers during conditions of resource abundance. J. Apic. Res. 44, 151–156. doi: 10.1080/00218839.2005.11101170
Becerril-Ángeles, M., Núñez-Velázques, M., and Arias-Martínez, M. I. (2013). Mortalidad en México relacionada con picaduras de abeja de 1988 a 2009. Rev. Alergia Mex. 60, 58–62.
Breed, M. D., Guzman-Novoa, E., and Hunt, G. J. (2004). Defensive behavior of honey bees: organization, genetics and comparisons with other bees. Annu. Rev. Entomol. 49, 271–298. doi: 10.1146/annurev.ento.49.061802.123155
Caro, A., Moo-Valle, H., Alfaro, R., and Quezada-Euán, J. J. G. (2017). Pollination services of Africanized honey bees and native Melipona beecheii to buzz-pollinated annatto (Bixa orellana L.) in the neotropics. Agric. For. Entomol. 19:274. doi: 10.1111/afe.12206
Celis, A., Gaxiola-Robles, R., Sevilla-Godínez, E., Orozco-Valerio, M. J., and Armas, J. (2007). Tendencia de la mortalidad por picaduras de alacrán en México, 1979-2003. Rev. Panam. Salud Publ. 21, 373–380.
Chihu, A. D., Rojas, L. M., and Rodríguez, S. R. (1992). “Primer reporte en México del ácaro Varroa jacobsoni, causante de la varroasis de la abeja melífera (Apis mellifera L.),” in Proceedings of the Memorias VI Sem. Am. Apic, Oaxtepéc, México, 9–11.
Clarke, K. E., Rinderer, T. E., Franck, P., Quezada-Euán, J. J. G., and Oldroyd, B. P. (2002). The Africanization of honeybees (Apis mellifera L.) of the Yucatan: a study of a massive hybridization event across time. Evolution 56, 1462–1474. doi: 10.1111/j.0014-3820.2002.tb01458.x
Collins, A. M., and Rinderer, T. E. (1991). “Genetics of defensive behavior, I,” in The “African” honey bee, eds M. Spivak, D. J. C. Fletcher, and M. D. Breed (Boulder, CO: Westview Press), 309–328. doi: 10.1201/9780429308741-16
Collins, A. M., Rinderer, T. E., Harbo, J. R., and Bolten, A. B. (1982). Colony defense by Africanized and European honey bees. Science 218, 72–74. doi: 10.1126/science.218.4567.72
Constancia, M., Pickard, B., Kelsey, G., and Reik, W. (1998). Imprinting mechanisms. Genome Res. 8, 881–900.
Daly, H. V., and Balling, S. S. (1978). Identification of Africanized honey bees in the western hemisphere by discriminant analysis. J. Kans. Entomol. Soc. 51, 857–869.
Danka, R. G., Hellmich, R. L., and Rinderer, T. E. (1992). Nest usurpation, supersedure and colony failure contribute to Africanization of commercially managed European honey bees in Venezuela. J. Apic. Res. 31, 119–123. doi: 10.1080/00218839.1992.11101272
Danka, R. G., Hellmich, R. L., Rinderer, T. E., and Collins, A. M. (1987). Diet-selection ecology of tropically and temperately adapted honey bees. Anim. Behav. 35, 1858–1863. doi: 10.1016/s0003-3472(87)80078-7
De Souza, F. S., Kevill, J. L., Correia-Oliveira, M. E., de Carvalho, C. A. L., and Martin, S. J. (2019). Occurrence of deformed wing virus variants in the stingless bee Melipona subnitida and honey bee Apis mellifera populations in Brazil. J. Gen. Virol. 100, 289–294. doi: 10.1099/jgv.0.001206
DeGrandi-Hoffman, G., Collins, A. M., Martin, J. H., Schmidt, J. O., and Spangler, H. G. (1998a). Nest defense behavior in colonies from crosses between Africanized and European honey bees (Apis mellifera L.) (Hymenoptera: Apidae). J. Insect. Behav. 11, 37–45.
DeGrandi-Hoffman, G., Watkins, J. C., Collins, A. M., Loper, G. M., Martin, J. H., and Arias, M. C. (1998b). Queen developmental time as a factor in the Africanization of European honey bee (Hymenoptera: Apidae) populations. Ann. Entomol. Soc. Am. 91, 52–58. doi: 10.1093/aesa/91.1.52
Delgado-Carrillo, O., Martén-Rodríguez, S., Ashworth, L., Aguilar, R., Lopezaraiza-Mikel, M., and Quesada, M. (2018). Temporal variation in pollination services to Cucurbita moschata is determined by bee gender and diversity. Ecosphere 9, 1–16.
Do Carmo, R. M., Franceschinelli, E. V., and de Silveira, F. A. (2004). Introduced honeybees (Apis mellifera) reduce pollination success without affecting the floral resource taken by native pollinators. Biotropica 36, 371–376. doi: 10.1111/j.1744-7429.2004.tb00329.x
Domínguez-Ayala, R., Moo-Valle, H., May-Itzá, W. J., Medina-Peralta, S., and Quezada-Euán, J. J. G. (2016). Stock composition of northern Neotropical honey bees: mitotype and morphotype diversity in Mexico (Hymenoptera: Apidae). Apidologie 47, 642–652. doi: 10.1007/s13592-015-0414-6
Efsthation, C. A., and Kern, W. H. Jr. (2016). A push-pull protocol to reduce colonization of bird nest boxes by honey bees. J. Vis. Exp. 115:53950. doi: 10.3791/53950
Esquivel-Rojas, S., Macías-Macías, J. O., Tapia-González, J. M., Contreras-Escareño, F., De León-Mantecón, M. J., and Silva-Contreras, A. (2015). Selección de abejas (Apis mellifera L) con baja defensividad y su relación con el medio ambiente en Jalisco, México. Abanico Veterinario 5, 44–50.
Fewell, J. H., and Bertram, S. M. (2002). Evidence for genetic variation in worker task performance by African and European honey bees. Behav. Ecol. Sociobiol. 52, 318–325. doi: 10.1007/s00265-002-0501-3
Fierro, M. M., Barraza, A., Maki, D. L., and Moffet, J. O. (1987). The effects of the first year of Africanization of honey bee populations in Chiapas. Mexico. Am. Bee. J. 127:845.
Fleites-Ayil, F. A., Quezada-Euán, J. J. G., and Medina-Medina, L. A. (2018). Onset of foraging and lifespan of Africanized honey bees (Apis mellifera) infected with different levels of Nosema ceranae spores in Neotropical Mexico. Apidologie 49, 781–788. doi: 10.1007/s13592-018-0602-2
Galindo-Cardona, A., Acevedo-Gonzalez, J. P., Rivera-Marchand, B., and Giray, T. (2013). Genetic structure of the gentle Africanized honey bee population (gAHB) in Puerto Rico. BMC Genet. 14:65. doi: 10.1186/1471-2156-14-65
Giray, T., Guzman-Novoa, E., Aron, C. W., Zelinsky, B., Fahrbach, S. E., and Robinson, G. E. (2000). Genetic variation in worker temporal polyethism and colony defensiveness in the honey bee, Apis mellifera. Behav. Ecol. 11, 44–55. doi: 10.1093/beheco/11.1.44
Gonçalves, L. S., Stort, A. C., and De Jong, D. (1991). “Beekeeping in Brazil,” in The “African” Honey Bee, eds M. Spivak, D. J. C. Fletcher, and M. D. Breed (Boulder, CO: Westview), 359–372. doi: 10.1201/9780429308741-18
Goulson, D. (2003). Effects of introduced bees on native ecosystems. Annu. Rev. Ecol. Evol. Syst. 34, 1–26. doi: 10.1146/annurev.ecolsys.34.011802.132355
Guzman-Novoa, E. (1996). “La Apicultura en México y Centro América,” in Proceedings of the Memorias del V Congreso Ibero Latinoamericano de Apicultura 1996, (Mercedes: Intendenc. Municip. Soriano, Central Apic. Coop. Calmer), 14–17.
Guzman-Novoa, E. (2004). Impacto de la Africanización de las abejas en México. Imagen Veterinaria 4, 22–25.
Guzman-Novoa, E., Correa-Benítez, A., Guzman, G., and Espinoza-Montaño, L. G. (2011). Colonization, impact and control of Africanized honey bees in Mexico. Veterinaria Mex. 42, 149–178.
Guzman-Novoa, E., Eccles, L., Calvete, Y., McGowan, J., Kelly, P. G., and Correa-Benítez, A. (2010). Varroa destructor is the main culprit for the death and reduced populations of overwintered honey bee (Apis mellifera) colonies in Ontario. Canada. Apidologie 41, 443–450. doi: 10.1051/apido/2009076
Guzman-Novoa, E., and Gary, N. E. (1993). Genotypic variability of components of foraging behavior in honey bees (Hymenoptera: Apidae). Ann. Entomol. Soc. Am. 83, 1152–1154.
Guzman-Novoa, E., Hamiduzzaman, M. M., Anguiano-Baez, R., Correa-Benítez, A., Castañeda-Cervantes, E., and Arnold, N. I. (2015). First detection of honey bee viruses in stingless bees in North America. J. Apic. Res. 54, 93–95. doi: 10.1080/00218839.2015.1100154
Guzman-Novoa, E., Hunt, G. J., Page, R. E. Jr., and Fondrk, M. K. (2002a). Genetic correlations among honey bee (Hymenoptera: Apidae) behavioral characteristics and wing length. Ann. Entomol. Soc. Am. 95, 402–406. doi: 10.1603/0013-8746(2002)095[0402:gcahbh]2.0.co;2
Guzman-Novoa, E., Hunt, G. J., Page, R. E. Jr., Uribe-Rubio, J. L., Prieto-Merlos, D., and Becerra-Guzmán, F. (2005). Paternal effects on the defensive behavior of honeybees. J. Hered. 96, 376–380. doi: 10.1093/jhered/esi038
Guzman-Novoa, E., Hunt, G. J., Uribe-Rubio, J. L., and Prieto-Merlos, D. (2004). Genotypic effects of honey bee (Apis mellifera) defensive behavior at the individual and colony levels: the relationship of guarding, pursuing and stinging. Apidologie 35, 15–24. doi: 10.1051/apido:2003061
Guzman-Novoa, E., Hunt, G. J., Uribe-Rubio, J. L., Smith, C., and Arechavaleta-Velasco, M. E. (2002b). Confirmation of QTL effects and evidence of genetic dominance of honey bee defensive behavior: results of colony and individual behavioral assays. Behav. Genet. 32, 95–102.
Guzman-Novoa, E., and Page, R. E. Jr. (1999a). “Breeding honey bees in Africanized areas,” in Apiculture for the 21st Century, eds R. Hoopingarner and L. Connor (Cheshire, CT: Wicwas Press), 12–14.
Guzman-Novoa, E., and Page, R. E. Jr. (1999b). Selective breeding of honey bees (Hymenoptera: Apidae) in Africanized areas. J. Econ. Entomol. 92, 521–525. doi: 10.1093/jee/92.3.521
Guzman-Novoa, E., Page, R. E. Jr., and Correa-Benítez, A. (1997). Introduction and acceptance of European queens in Africanized and European honey bee (Apis mellifera L.) colonies. Am. Bee J. 137, 667–668.
Guzman-Novoa, E., Page, R. E. Jr., and Fondrk, M. K. (1994). Morphometric techniques do not detect intermediate and low levels of Africanization in honey bee (Hymenoptera: Apidae) colonies. Ann. Entomol. Soc. Am. 87, 507–515. doi: 10.1093/aesa/87.5.507
Guzman-Novoa, E., Page, R. E. Jr., and Prieto-Merlos, D. (1998). Queen introduction, acceptance, and survival in honey bee (Hymenoptera: Apidae) colonies of a tropical. Africanized region. J. Econ. Entomol. 91, 1290–1294. doi: 10.1093/jee/91.6.1290
Guzman-Novoa, E., and Page, R. E. (1993). Backcrossing Africanized honey bee queens to European drones reduces colony defensive behavior. Ann. Entomol. Soc. Am. 86, 352–355. doi: 10.1093/aesa/86.3.352
Guzman-Novoa, E., and Page, R. E. (1994a). Genetic dominance and worker interactions affect honey bee colony defense. Behav. Ecol. 5, 91–97. doi: 10.1093/beheco/5.1.91
Guzman-Novoa, E., and Page, R. E. (1994b). The impact of Africanized bees on Mexican beekeeping. Am. Bee. J. 134, 101–106.
Guzman-Novoa, E., and Page, R. E. Jr. (2000). Pollen collection and foraging force by European and European x Africanized hybrid honey bees (Hymenoptera: Apidae) in mixed genotype colonies are similar. Ann. Entomol. Soc. Am. 93, 141–144.
Guzman-Novoa, E., Prieto-Merlos, D., Uribe-Rubio, J. L., and Hunt, G. J. (2003). Relative reliability of four field assays to test defensive behaviour of honey bees (Apis mellifera). J. Apic. Res. 42, 42–46. doi: 10.1080/00218839.2003.11101088
Guzman-Novoa, E., and Uribe-Rubio, J. L. (2004). Honey production by European. Africanized and hybrid honey bee (Apis mellifera) colonies in Mexico. Am. Bee. J. 144, 318–320.
Guzman-Novoa, E., Vandame, R., and Arechavaleta-Velasco, M. E. (1999). Susceptibility of European and Africanized honey bees (Apis mellifera L.) to Varroa jacobsoni Oud. in Mexico. Apidologie 30, 173–182. doi: 10.1051/apido:19990207
Hall, H. G., and Muralidharan, K. (1989). Evidence from mitochondrial DNA that African honey bees spread as continuous maternal lineages. Nature 339, 211–213. doi: 10.1038/339211a0
Hamiduzzaman, M. M., Guzman-Novoa, E., Goodwin, P. H., Reyes-Quintana, M., Koleoglu, G., Correa-Benítez, A., et al. (2015). Differential responses of Africanized and European honey bees (Apis mellifera) to viral replication following mechanical transmission or Varroa destructor parasitism. J. Invertebr. Pathol. 126, 12–20. doi: 10.1016/j.jip.2014.12.004
Harpur, B. A., Kadri, S. M., Orsi, R. O., Whitfield, C. W., and Zayed, A. (2020). Defense response in Brazilian honey bees (Apis mellifera scutellata x spp.) is underpinned by complex patterns of admixture. Genom. Biol. Evol. 12, 1367–1377. doi: 10.1093/gbe/evaa128
Harpur, B. A., Minae, S., Kent, C. F., and Zayed, A. (2012). Management increases genetic diversity of honey bees via admixture. Mol. Ecol. 21, 4414–4421. doi: 10.1111/j.1365-294x.2012.05614.x
Harrison, J. F., and Hall, H. G. (1993). African–European honeybee hybrids have low intermediate metabolic capacities. Nature 363, 258–259. doi: 10.1038/363258a0
Hellmich, R. L. (1991). “Continuing commercial queen production after the arrival of Africanized honey bees,” in The “African” Honey Bee, eds M. Spivak, D. J. C. Fletcher, and M. D. Breed (Boulder, CO: Westview), 187–197. doi: 10.1201/9780429308741-9
Hellmich, R. L., and Rinderer, T. E. (1991). “Beekeeping in Venezuela,” in The “African” Honey Bee, eds M. Spivak, D. J. C. Fletcher, and M. D. Breed (Boulder, CO: Westview), 399–411. doi: 10.1201/9780429308741-20
Hunt, G. J., Amdam, G. V., Schlipalius, D., Emore, C., Sardesai, N., Williams, C. E., et al. (2007). Behavioral genomics of honeybee foraging and nest defense. Naturwissenschaften 94, 247–267. doi: 10.1007/s00114-006-0183-1
Hunt, G. J., Collins, A. M., Rivera, R., Page, R. E. Jr., and Guzman-Novoa, E. (1999). Quantitative trait loci influencing honey bee alarm pheromone levels. J. Hered. 90, 585–589. doi: 10.1093/jhered/90.5.585
Hunt, G. J., Guzman-Novoa, E., Fondrk, M. K., and Page, R. E. Jr. (1998). Quantitative trait loci for honey bee stinging behavior and body size. Genetics 148, 1203–1213.
Hunt, G. J., Guzman-Novoa, E., Uribe-Rubio, J. L., and Prieto-Merlos, D. (2003a). Genotype by environment interactions in honeybee guarding behavior. Anim. Behav. 66, 459–467. doi: 10.1006/anbe.2003.2253
Hunt, G. J., Wood, K. V., Guzman-Novoa, E., Lee, H. D., Rothwell, A. P., and Bonham, C. C. (2003b). Discovery of 3-methyl-2-buten-1-yl acetate, a new alarm component in the sting apparatus of Africanized honey bees. J. Chem. Ecol. 29, 451–461.
Kerr, W. E. (1967). The history of the introduction of African Bees to Brazil. S. Afr. Bee. J. 39, 3–5.
Kraus, F. B., Franck, P., and Vandame, R. (2007). Asymmetric introgression of African genes in honeybee populations (Apis mellifera L.) in Central Mexico. Heredity 99, 233–240. doi: 10.1038/sj.hdy.6800988
Labougle, J. M., Mancera, M., and Taylor, O. R. (1989). Morphometric and ellectrophoretic study of the African honey bee in southern Mexico. Am. Bee. J. 129, 817.
Landaverde-González, P., Quezada-Euán, J. J. G., Theodorou, P., Murray, T. E., Husemann, M., Ayala, R., et al. (2017). Sweat bees on hot chillies: provision of pollination services by native bees in traditional slash-and-burn agriculture in the Yucatan peninsula of tropical Mexico. J. Appl. Ecol. 54, 1814–1824. doi: 10.1111/1365-2664.12860
Macías-Macías, J. O., Tapia-Rivera, J. C., De la Mora, A., Tapia-González, J. M., Contreras-Escareño, F., Petukhova, T., et al. (2020). Nosema ceranae causes cellular immunosuppression and interacts with thiamethoxam to increase mortality in the stingless bee Melipona colimana. Sci. Rep. 10:17021.
Macías-Macías, O., Chuc, P., Ancona-Xiu, P., Cauich, O., and Quezada-Euán, J. J. G. (2009). Contribution of native bees and Africanized honeybees (Hymenoptera: Apoidea) to Solanaceae crop pollination in tropical Mexico. J. Appl. Entomol. 133, 456–465. doi: 10.1111/j.1439-0418.2009.01399.x
Magaña-Magaña, M. A., Tavera-Cortés, M. E., Salazar-Barrientos, L. L., and Sanginés-García, J. R. (2016). Productividad de la apicultura en México y su impacto sobre la rentabilidad. Rev. Mexicana Cienc. Agrícol. 7, 1103–1115. doi: 10.29312/remexca.v7i5.235
Martin, S. J., and Medina-Medina, L. (2004). Africanized bees have a unique tolerance to Varroa mites. Trends. Parasitol. 20, 112–114. doi: 10.1016/j.pt.2004.01.001
McMahon, D. P., Fürst, M. A., Caspar, J., Theodorou, P., Brown, M. J. F., and Paxton, R. J. (2015). A sting in the spit: widespread cross-infection of multiple RNA viruses across wild and managed bees. J. Anim. Ecol. 84, 615–624. doi: 10.1111/1365-2656.12345
Medina, R. G., Paxton, R. J., De Luna, E., Fleites-Ayil, F., Medina-Medina, L. A., and Quezada-Euán, J. J. G. (2018). Developmental stability, age at onset of foraging and longevity of Africanized honey bees (Apis mellifera L.) under heat stress (Hymenoptera: Apidae). J. Therm. Biol. 74, 214–225. doi: 10.1016/j.jtherbio.2018.04.003
Medina, R. G., Paxton, R. J., Hernández-Sotomayor, S. M. T., Pech-Jimenez, C., Medina-Medina, L. A., and Quezada-Euán, J. J. G. (2020). Heat stress during development affects immunocompetence in workers, queens and drones of Africanized honey bees (Apis mellifera L.) (Hymenoptera: Apidae). J. Therm. Biol. 89:102541. doi: 10.1016/j.jtherbio.2020.102541
Medina-Flores, C. A., Guzman-Novoa, E., Hamiduzzaman, H. H., Aguilera-Soto, J., and Lopez-Carlos, M. A. (2015). Africanization of honey bees (Apis mellifera) in three climatic regions of northern Mexico. Veter. Mex. 2, 1–9. doi: 10.17221/7240-vetmed
Medina-Flores, C. A., Guzman-Novoa, E., Hamiduzzaman, M. M., Aréchiga-Flores, C. F., and López-Carlos, M. A. (2014). Africanized honey bees (Apis mellifera) have low infestation levels of the mite Varroa destructor in different ecological regions in Mexico. Genet. Mol. Res. 13, 7282–7293. doi: 10.4238/2014.february.21.10
Moffett, J. O., Maki, D. L., Andre, T., and Fierro, M. M. (1987). The Africanized bee in Chiapas. Mexico. Am. Bee. J. 127, 517–520.
Moore, A. J., Breed, M. D., and Moor, M. J. (1987). The guard honey bee: ontogeny and behavioral variability of workers performing a specialized task. Anim. Behav. 35, 1159–1167. doi: 10.1016/s0003-3472(87)80172-0
Morfin, N., Gashout, H. A., Macías-Macías, J. O., De la Mora, A., Tapia-Rivera, J. C., Tapia-González, J. M., et al. (2020). Detection, replication and quantification of deformed wing virus-A, deformed wing virus-B, and black queen cell virus in the endemic stingless bee, Melipona colimana, from Jalisco, Mexico. Int. J. Trop. Insect. Sci. doi: 10.1007/s42690-020-00320-7
Neuman, L. S. (2001). Evaluación del comportamiento de pecoreo en tres genotipos de abejas melíferas (Europeas, híbridas y Africanizadas). DVM Thesis, National Autonomous University of Mexico, Mexico.
Nogueira-Neto, P. (1964). The spread of a fierce African bee in Brazil. Bee World 45, 119–121. doi: 10.1080/0005772x.1964.11097060
Pérez-Balam, J., Quezada-Euán, J. J. G., Alfaro-Bates, R., Medina, S., McKendrick, L., Soro, A., et al. (2012). The contribution of honey bees, wasps and flies to avocado (Persea americana) pollination in southern Mexico. J. Pollinat. Ecol. 8, 42–47.
Pesante, D. G., Rinderer, T. E., and Collins, A. M. (1987). Differential nectar foraging by Africanized and European honeybees in the neotropics. J. Apic. Res. 26, 210–216. doi: 10.1080/00218839.1987.11100762
Poot-Baez, V., Medina-Hernández, R., Medina-Peralta, S., and Quezada-Euán, J. J. G. (2020). Intranidal temperature and body size of Africanized honey bees under heat waves (Hymenoptera: Apidae). Apidologie 51, 382–390. doi: 10.1007/s13592-019-00725-5
Portman, Z. M., Tepedino, V. J., Tripodi, A. D., Szalanski, A. L., and Durham, S. L. (2018). Local extinction of a rare plant pollinator in Southern Utah (USA) associated with invasion by Africanized honey bees. Biol. Invasions 20, 593–606. doi: 10.1007/s10530-017-1559-1
Potts, S. G., Biesmeijer, J. C., Kremen, C., Neumann, P., Schweiger, O., and Kunin, W. E. (2010). Global pollinator declines: trends, impacts, and drivers. Trends. Ecol. Evol. 25, 345–353. doi: 10.1016/j.tree.2010.01.007
Programa Nacional para el Control de la Abeja Africana-PNCAA. (2010). Situación actual y perspectiva de la apicultura en México. Claridades Agropecuarias 199, 3–34.
Quezada-Euán, J. J. G. (2007). A retrospective history of the expansion of Africanized honeybees in Mexico. J. Apic. Res. 46, 295–300. doi: 10.3896/ibra.1.46.4.16
Quezada-Euán, J. J. G. (2018). Stingless bees of Mexico: The Biology, Management and Conservation of An Ancient Heritage. Berlin: Springer.
Quezada-Euán, J. J. G., De Araujo-Freitas, C., Marrufo Olivares, J. C., Velásquez-Madrazo, A., and Medina-Medina, L. A. (2008). “Selección en colonias de abejas africanizadas: una alternativa viable para el trópico mexicano,” in Proceedings of the Memorias 2° Apimondia Simposio Mundial de Criadores de Abejas Reina e Inseminación Artificial, Nayarit, 15–17.
Quezada-Euán, J. J. G., and Hinsull, S. M. (1995). Evidence of continued European morphometrics and mtDNA in feral colonies of honey bees (Apis mellifera) from the Yucatan peninsula. Mexico. J. Apic. Res. 34, 161–166. doi: 10.1080/00218839.1995.11100901
Quezada-Euán, J. J. G., May-Itzá, W., de, J., and González-Acereto, J. A. (2001). Meliponiculture in Mexico: problems and perspective for development. Bee World 82, 160–167. doi: 10.1080/0005772x.2001.11099523
Quezada-Euán, J. J. G., and May-Itza, W. J. (2001). Partial seasonal isolation of African and European-derived Apis mellifera (Hymenoptera: Apidae) drones at congregation areas from subtropical Mexico. Ann. Entomol. Soc. Am. 94, 540–544. doi: 10.1603/0013-8746(2001)094[0540:psioaa]2.0.co;2
Quezada-Euán, J. J. G., and Paxton, R. J. (1999). Rapid inter-generational changes in morphology and behaviour in colonies of Africanized and European honey bees (Apis mellifera) from tropical Yucatan. Mexico. J. Apic. Res. 38, 93–104. doi: 10.1080/00218839.1999.11101000
Quezada-Euán, J. J. G., Paxton, R. J., and Echazarreta, C. M. (1996). The distribution and range expansion of the Africanized honey bees (Apis mellifera L.) in the state of Yucatan. Mexico. J. Apic. Res. 35, 49–56.
Quezada-Euán, J. J. G., Pérez-Castro, E. E., May-Itzá, W., and de, J. (2003). Hybridization between European and African-derived honeybee populations (Apis mellifera) at different altitudes in Perú. Apidologie 34, 217–225. doi: 10.1051/apido:2003010
Ramírez-Flores, V. A., Villanueva-Gutiérrez, R., Roubik, C. W., Vergara, C. H., and Lara-Rodriguez, N. (2015). Topological structure of plant-bee networks in four Mexican environments. Sociobiology 62, 56–64.
Rangel, J., Giresi, M., Pinto, M. A., Baum, K. A., Rubink, W. L., Coulson, R. N., et al. (2016). Africanization of a feral honey bee (Apis mellifera) population in South Texas: does a decade make a difference? Ecol. Evol. 6, 2158–2169. doi: 10.1002/ece3.1974
Rinderer, T. E., and Collins, A. M. (1991). “Foraging behavior and honey production,” in The “African” Honey Bee, eds M. Spivak, D. J. C. Fletcher, and M. D. Breed (Boulder, CO: Westview Press), 235–257. doi: 10.1201/9780429308741-12
Rinderer, T. E., Collins, A. M., Hellmich, R. L., and Danka, R. G. (1987). Differential drone production by Africanized and European honey bee colonies. Apidologie 18, 61–67. doi: 10.1051/apido:19870106
Rinderer, T. E., Collins, A. M., and Tucker, K. W. (1985a). Honey production and underlying nectar harvesting activities of Africanized and European honeybees. J. Apic. Res. 23, 161–167. doi: 10.1080/00218839.1985.11100666
Rinderer, T. E., and Hellmich, R. L. (1991). “The Process of Africanization,” in The “African” Honey Bee, eds M. Spivak, D. J. C. Fletcher, and M. D. Breed (Boulder CO: Westview Press), 95–117. doi: 10.1525/ahu.2004.29.1.95
Rinderer, T. E., Hellmich, R. L., Danka, R. G., and Collins, A. M. (1985b). Male reproductive parasitism: a factor in the Africanization of European honey-bee populations. Science 228, 1119–1121. doi: 10.1126/science.228.4703.1119
Rinderer, T. E., Stelzer, J. A., Oldroyd, B. P., Buco, S. M., and Rubink, W. L. (1991). Hybridization between European and Africanized honey bees in the neotropical Yucatan peninsula. Science 253, 309–311. doi: 10.1126/science.253.5017.309
Rivera-Marchand, B., Giray, T., and Guzman-Novoa, E. (2008). The cost of defense in social insects: insights from the honey bee. Entomol. Exp. Appl. 129, 1–10. doi: 10.1111/j.1570-7458.2008.00747.x
Romero, M. J., and Quezada-Euán, J. J. G. (2013). Pollinators in biofuel agricultural systems: the diversity and performance of bees (Hymenoptera: Apoidea) on Jatropha curcas in Mexico. Apidologie 44, 419–429. doi: 10.1007/s13592-013-0193-x
Roubik, D. W. (1987). “Long-term consequences of the African honey bee invasion: implications for the United States,” in Proceedings of the Africanized Honey Bee Symposium, (Park Ridge: American Farm Bureau), 46–54.
Roubik, D. W. (1989). Ecology and Natural History of Tropical Bees. Cambridge: Cambridge University Press.
Roubik, D. W. (2000). Pollination system stability in tropical America. Conserv. Biol. 14, 1235–1236. doi: 10.1046/j.1523-1739.2000.00016.x
Roubik, D. W. (2002). The value of bees to the coffee harvest. Nature 417, 708. doi: 10.1038/417708a
Roubik, D. W. (2009). Ecological impact on native bees by the invasive Africanized honey bee. Acta Biol. Colomb. 14, 115–124.
Roubik, D. W., and Villanueva-Gutiérrez, R. (2009). Invasive Africanized honey bee impact on native solitary bees: a pollen resource and trap nest analysis. Biol. J. Linn. Soc. 98, 152–160. doi: 10.1111/j.1095-8312.2009.01275.x
Roubik, D. W., and Villanueva-Gutiérrez, R. (2017). Have native Hymenoptera or Africanized bees become aggressive foragers due to resource competition? Trends Entomol. 13, 95–102.
Sader. (2020). Servicio de información y estadística agroalimentaria (SIAP). Available online at: https://www.gob.mx/siap. (accessed September 1, 2020).
Santos, G. M. M., Aguiar, C. M. L., Genini, J., Martins, C. F., Zanella, F. C. V., and Mello, M. A. R. (2012). Invasive Africanized honeybees change the structure of native pollination networks in Brazil. Biol. Invasions 14, 2369–2378. doi: 10.1007/s10530-012-0235-8
Schneider, S. S., DeGrandi-Hoffman, G., and Smith, D. R. (2004). The African honey bee: factors contributing to a successful biological invasion. Annu. Rev. Entomol. 49, 351–376. doi: 10.1146/annurev.ento.49.061802.123359
Schneider, S. S., and McNally, L. C. (1992). Factors influencing seasonal absconding in colonies of the African honey bee, Apis mellifera scutellata. Insect. Soc. 39, 403–423. doi: 10.1007/bf01240624
Sheppard, W. S., Rinderer, T. E., Mazzoli, J. A., Stelzer, J. A., and Shimanuki, H. (1991a). Gene flow between African and European derived honey bee populations in Argentina. Nature 349, 782–784. doi: 10.1038/349782a0
Sheppard, W. S., Soares, A. E. E., De Jong, D., and Shimanuki, H. (1991b). Hybrid status of honey bee populations near the historic origin of Africanization in Brazil. Apidologie 22, 643–652. doi: 10.1051/apido:19910607
Silva-Contreras, A., Martínez-González, J., Cienfuegos-Rivas, E., López-Zavala, R., Tapia-González, J., and Parra-Bracamontes, G. (2019). Africanización de colonias en Apis mellifera L. (Hymenoptera: Apidae), presente en el ADN mitocondrial. Abanico Veter. 9, 1–11.
Smith, D. R., Taylor, O. R., and Brown, W. M. (1989). Neotropical Africanized honey bees have African mitochondrial DNA. Nature 339, 213–215. doi: 10.1038/339213a0
Spivak, M., Batra, S., Segreda, F., Castro, A. L., and Ramírez, W. (1989). Honey production by Africanized and European honey bees in Costa Rica. Apidologie 20, 207–220. doi: 10.1051/apido:19890303
Stort, A. C. (1975a). Genetic study of the aggressiveness of two subspecies of Apis mellifera in Brazil. II. Time at which the first sting reached the leather ball. J. Apic. Res. 14, 171–175. doi: 10.1080/00218839.1975.11099822
Stort, A. C. (1975b). Genetic study of the aggressiveness of two subspecies of Apis mellifera in Brazil. V. Number of stings in the leather ball. J. Kans. Entomol. Soc. 48, 381–387.
Tapia-González, J. M., Morfin, N., Macías-Macías, J. O., De la Mora, A., Tapia-Rivera, J. C., Ayala, R., et al. (2019). Evidence of presence and replication of honey bee viruses among wild bee pollinators in subtropical environments. J. Invertebr. Pathol. 168:107256. doi: 10.1016/j.jip.2019.107256
Tarpy, D. R., Caren, J. R., Delaney, D. A., Sammataro, D., Finley, J., Loper, G. M., et al. (2010). Mating frequencies of Africanized honey bees in the south western USA. J. Apic. Res. 49, 302–310. doi: 10.3896/ibra.1.49.4.02
Taylor, O. R. (1999). “Displacement of European honey bee subspecies by an invading African subspecies in the Americas,” in Apiculture for the 21st Century, eds R. Hoopingarner and L. J. Connor (Cheshire, CT: Wicwas Press), 38–46.
Tehel, A., Brown, M. J. F., and Paxton, R. J. (2016). Impact of managed honey bee viruses on wild bees. Curr. Opin. Virol. 19, 16–22. doi: 10.1016/j.coviro.2016.06.006
Ueira-Vieira, C., Oliveira-Almeida, L., Correadealmeida, F., Marques, I., Brandemburgo, M. A. M., and Onetti, A. M. (2015). Scientific note on the first molecular detection of the acute bee paralysis virus in Brazilian stingless bees. Apidologie 46, 628–630. doi: 10.1007/s13592-015-0353-2
Unger, P., and Guzman-Novoa, E. (2012). Honey bee (Hymenoptera: Apidae) guarding behaviour may not be a rare task. Can. Entomol. 144, 538–541. doi: 10.4039/tce.2012.58
Uribe-Rubio, J. L., Guzman-Novoa, E., Hunt, G. J., Correa-Benítez, A., and Zozaya, J. A. (2003). The effect of Africanization on honey production, defensive behavior and size of honeybees (Apis mellifera L.) in the Mexican high plateau. Veterinaria Mex. 34, 47–59.
Uribe-Rubio, J. L., Guzman-Novoa, E., Vázquez-Peláez, C., and Hunt, G. J. (2008). Genotype, task specialization, and nest environment influence the stinging response thresholds of individual Africanized and European honeybees to electrical stimulation. Behav. Genet. 38, 93–100. doi: 10.1007/s10519-007-9177-9
Uribe-Rubio, J. L., Petukhova, T., and Guzman-Novoa, E. (2013). Genotype and task influence stinging response thresholds of honeybee (Apis mellifera L.) workers of African and European descent. Open J. Ecol. 3, 279–283. doi: 10.4236/oje.2013.34032
Vandame, R., and Palacio, M. A. (2010). Preserved honey bee health in Latin America: a fragile equilibrium due to low-intensity agriculture and beekeeping? Apidologie 41, 243–255. doi: 10.1051/apido/2010025
Vergara, C., Dietz, A., and De Leon, A. P. (1993). Female parasitism of European honey bees by Africanized honey bee swarms in Mexico. J. Apic. Res. 32, 34–40. doi: 10.1080/00218839.1993.11101285
Vergara, C. H., and Badano, E. I. (2009). Pollinator diversity increases fruit production in Mexican coffee plantations: the importance of rustic management systems. Agric. Ecosyst. Environ. 129, 117–123. doi: 10.1016/j.agee.2008.08.001
Whitfield, C. W., Behura, S. K., Berlocher, S. H., Clark, A. G., Johnston, J. S., Sheppard, W. S., et al. (2006). Thrice out of Africa: ancient and recent expansions of the honey bee. Apis Mellif. Sci. 314, 642–645. doi: 10.1126/science.1132772
Winston, M. L. (1979). Intra-colony demography and reproductive rate of the Africanized honeybee in South America. Behav. Ecol. Sociobiol. 4, 279–292. doi: 10.1007/bf00297648
Winston, M. L. (1992). Killer bees: The Africanized Honey Bee in the Americas. Massachusetts: Harvard University Press.
Winston, M. L., and Katz, S. J. (1981). Longevity of cross-fostered honey bee workers (Apis mellifera) of European and Africanized races. Canad. J. Zool. 59, 1571–1575. doi: 10.1139/z81-214
Woyke, J. (1984). Correlations and interactions between population, length of worker life and honey production by honeybees in a temperate region. J. Apic. Res. 23, 148–156. doi: 10.1080/00218839.1984.11100624
Zamora, O., Dominguez, R., Alanis-Gutierrez, L., and Quezada-Euán, J. J. G. (2008). Frequency of European and African-derived morphotypes and haplotypes in colonies of honey bees (Apis mellifera) from NW Mexico. Apidologie 39, 388–396. doi: 10.1051/apido:2008016
Keywords: Apis mellifera, Africanized honey bees, native bee, adaptation, invasive organism, impact, Mexico
Citation: Guzman-Novoa E, Morfin N, De la Mora A, Macías-Macías JO, Tapia-González JM, Contreras-Escareño F, Medina-Flores CA, Correa-Benítez A and Quezada-Euán JJG (2020) The Process and Outcome of the Africanization of Honey Bees in Mexico: Lessons and Future Directions. Front. Ecol. Evol. 8:608091. doi: 10.3389/fevo.2020.608091
Received: 18 September 2020; Accepted: 26 October 2020;
Published: 12 November 2020.
Edited by:
Tugrul Giray, University of Puerto Rico, Puerto RicoReviewed by:
Alberto Galindo-Cardona, CCT CONICET Tucuman, ArgentinaDavid Tarpy, North Carolina State University, United States
Copyright © 2020 Guzman-Novoa, Morfin, De la Mora, Macías-Macías, Tapia-González, Contreras-Escareño, Medina-Flores, Correa-Benítez and Quezada-Euán. This is an open-access article distributed under the terms of the Creative Commons Attribution License (CC BY). The use, distribution or reproduction in other forums is permitted, provided the original author(s) and the copyright owner(s) are credited and that the original publication in this journal is cited, in accordance with accepted academic practice. No use, distribution or reproduction is permitted which does not comply with these terms.
*Correspondence: Ernesto Guzman-Novoa, ZWd1em1hbkB1b2d1ZWxwaC5jYQ==