- 1Evolutionary Ecology Laboratories, Department of Biology, Brigham Young University, Provo, UT, United States
- 2Departamento de Ecología y Recursos Naturales, Facultad de Ciencias, Universidad Nacional Autónoma de México, Ciudad Universitaria, Ciudad de México, Mexico
- 3Monte L. Bean Life Science Museum, Brigham Young University, Provo, UT, United States
Life-history traits are directly linked to fitness, and therefore, can be highly adaptive. Livebearers have been used as models for understanding the evolution of life histories due to their wide diversity in these traits. Several different selective pressures, including population density, predation, and resource levels, can shape life-history traits. However, these selective pressures are usually considered independently in livebearers and we lack a clear understanding of how they interact in shaping life-history evolution. Furthermore, selective pressures such as interspecific competition are rarely considered as drivers of life-history evolution in poeciliids. Here we test the simultaneous effects of several potential selective pressures on life-history traits in the livebearing fish Poeciliopsis prolifica. We employ a multi-model inference approach. We focus on four known agents of selection: resource availability, stream velocity, population density, and interspecific competition, and their effect on four life-history traits: reproductive allocation, superfetation, number of embryos, and individual embryo size. We found that models with population density and interspecific competition alone were strongly supported in our data and, hence, indicated that these two factors are the most important selective agents for most life-history traits, except for embryo size. When population density and interspecific competition increase there is an increase in each of the three life-history traits (reproductive allocation, superfetation, and number of embryos). For individual embryo size, we found that all single-agent models were equivalent and it was unclear which selective agent best explained variation. We also found that models that included population density and interspecific competition as direct effects were better supported than those that included them as indirect effects through their influence on resource availability. Our study underscores the importance of interspecific competitive interactions on shaping life-history traits and suggests that these interactions should be considered in future life-history studies.
Introduction
Life-history traits can be highly adaptive (Roff, 2002; Chapuis et al., 2017), and can evolve in response to a variety of selective pressures, both biotic and abiotic (Johnson and Bagley, 2011). Some demonstrated selective agents known to affect the evolution of life histories are population density (Reznick et al., 2002, 2012), predation (Martin, 1995; Johnson and Belk, 2001; Reznick et al., 2001; Roff, 2002; Chapuis et al., 2017), resource availability (Reznick and Yang, 1993; Grether et al., 2001; Roff, 2002; Pérez-Mendoza et al., 2014; Zani and Stein, 2018), and other environmental effects, such as water flow, elevation, toxicity, etc. (Badyaev and Ghalambor, 2001; Ghalambor et al., 2004; Zúñiga-Vega et al., 2007; Riesch et al., 2010, 2014; Johnson and Bagley, 2011; Banet et al., 2016; Heins and Baker, 2017; Santi et al., 2019). The family Poeciliidae has a staggering diversity in reproductive adaptations and heterogeneity in life-history traits, emerging as a model system for life-history research (Pollux et al., 2009). A wealth of knowledge has been accumulated in several species of this family (Pollux et al., 2009; Johnson and Bagley, 2011), however, most studies tend to focus on a single selective factor, although it is clear that life-history traits can be affected and shaped simultaneously by several factors (Moore et al., 2016).
Studies that have examined how several factors affect life-history traits in poeciliids have shown that life histories respond in a predictable and repeatable way to certain selective agents, but not to all of them (Moore et al., 2016). For example, in several livebearing fish species predation drives life-history evolution in a predictable and repeatable manner, but resource availability has less consistent effects (Johnson and Belk, 2001; Reznick et al., 2001; Moore et al., 2016). However, the response to other selective factors can depend on the population studied (Moore et al., 2016). For some populations, it is clear that several pressures may be acting in concert (Johnson, 2002; Moore et al., 2016), whereas in others one factor may be dominant. Nevertheless, we still have a lack of studies that allow us to draw general predictions about responses to several selective pressures, and which selective pressures have primacy in wild populations.
Here, we evaluate several hypotheses about the relative importance of biotic and abiotic factors in shaping four life-history traits using a multi-model inference approach in a species of livebearing fishes (Johnson and Bagley, 2011). We focus on four well known life-history traits: superfetation (number of simultaneous broods carried by a female), individual embryo size, number of embryos (across all broods; Frías-Alvarez and Zúñiga-Vega, 2016), and reproductive allotment. We build specific hypotheses of how each factor could affect the evolution of the life-history traits using the wealth of knowledge available for poeciliids (Johnson and Bagley, 2011). However, some selective agents have only received modest consideration in this type of study (interspecific competition and stream velocity; Johnson and Bagley, 2011). Given this modest inclusion, it is unknown how important interspecific competition and stream velocity are in comparison to other more commonly studied selective agents in livebearers. Thus, we model four hypothetical drivers of life-history variation: resource availability, population density, stream velocity, and interspecific competition (see Table 1 for hypotheses), each of which is a potential agent of life-history evolution in livebearing fishes (Poeciliidae; Johnson and Bagley, 2011). We compare these putative selective agents to evaluate the relative importance of each on the evolution of life histories of Poeciliopsis prolifica and test if the effect of each selective agent is in accordance with previous hypotheses (Table 1).
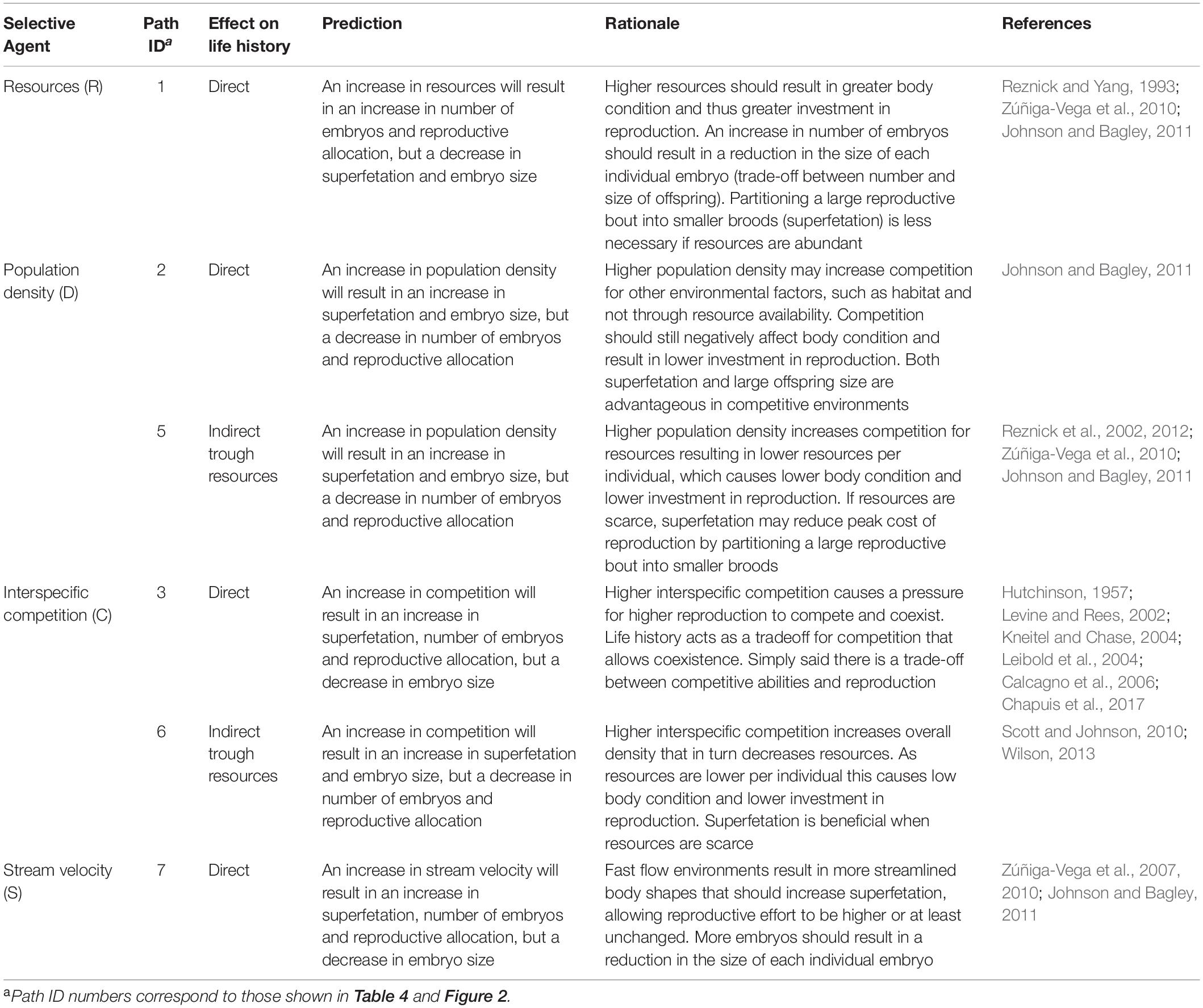
Table 1. Potential effects of the four putative selective agents on the life history of P. prolifica.
Materials and Methods
Study System and Collection Sites
Poeciliopsis prolifica is distributed through northwestern Mexico on the Pacific slope from the Rio Yaqui, Sonora south to near Las Varas, Nayarit (Miller et al., 2005). Populations exist under a variety of environmental conditions that include differences in fish density, stream velocity, fish community structure, and habitat characteristics. This provides a range of selective conditions under which populations might evolve local adaptations. We collected 298 P. prolifica females using hand-held seine nets (1.3 m × 5 m; 8 mm mesh size) from 12 populations (Figure 1) during the dry season (Table 2; Permits FAUT-0117, DGOPA/1864/210205/-0765, and DGOPA-005/16). All individuals were euthanized and preserved in alcohol following guidelines of the ethics committee of Brigham Young University (although Ethics Committee approval is not required according to national law in Mexico).
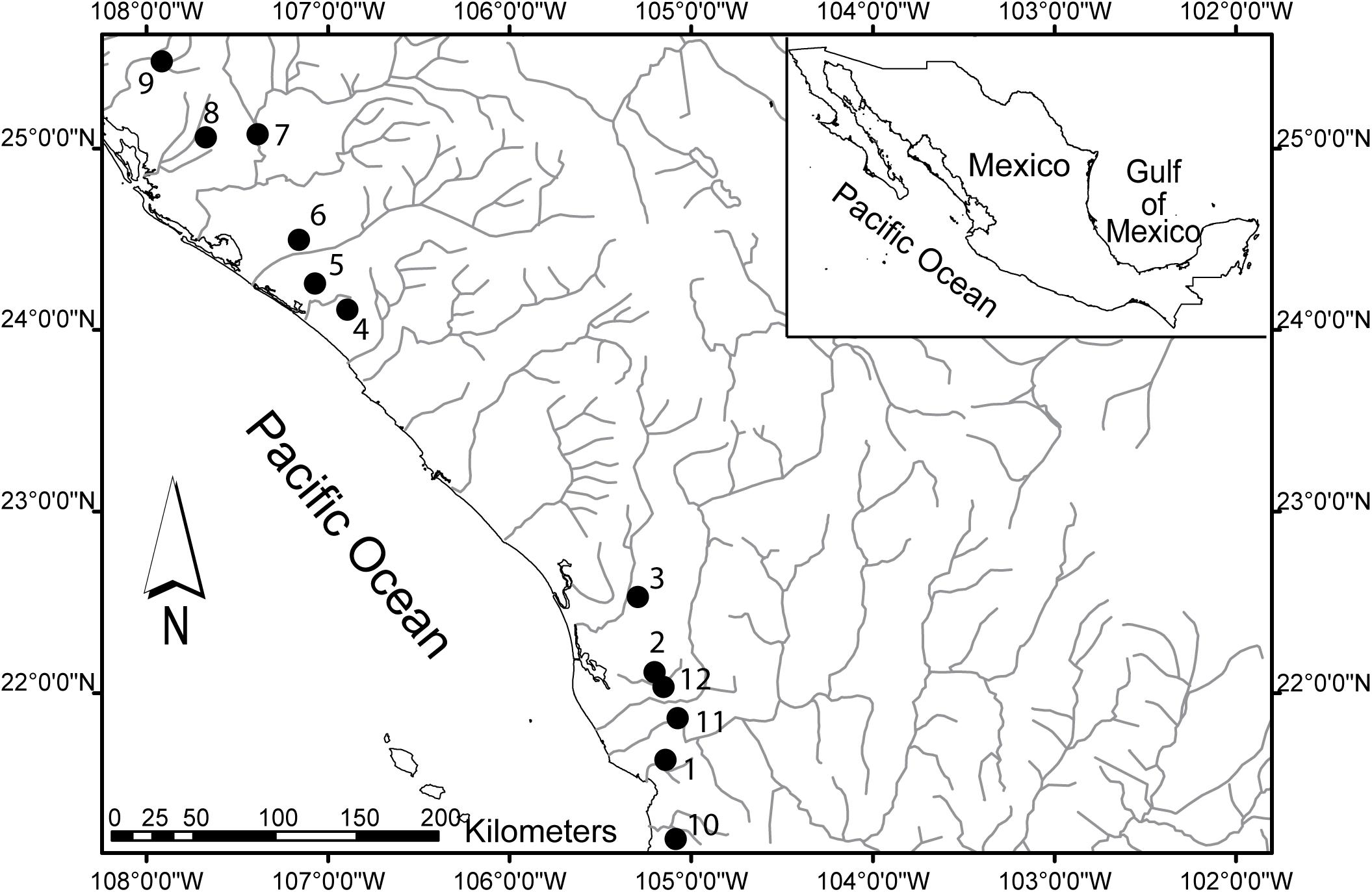
Figure 1. Map showing the localities sampled for Poeciliopsis prolifica. Inset map shows Mexico and the two states (Nayarit and Sinaloa) that were sampled. Numbers correspond to location ID (also found in Table 2).
For each locality, we quantified four environmental parameters that represent four putative selective agents: interspecific competition, resource availability, stream velocity, and population density (Table 2). All selective agents are predicted to affect life-history traits, either directly or indirectly (Johnson and Bagley, 2011). In this study, we assume that these four selective agents are unmeasured factors that are correlated with measurable environmental characteristics. To characterize the selective agent we attempt to identify environmental parameters that are correlated with the unmeasured true selective agent. We then estimate the correlation between observed characters and unobserved factors through path analysis.
We estimated relative population density of P. prolifica by calculating the average number of P. prolifica fish observed per seining attempt, a standardized catch per unit effort (CPUE) found to be positively correlated with actual population density (Johnson, 2002). CPUE can allow comparison of localities with different seining efforts and has been used in several studies as a proxy to population density (Kobza et al., 2004; Cruz et al., 2020). We estimated interspecific competition as the number of co-occurring species of the same genus. P. prolifica can co-occur with up to three species of the same genus: P. viriosa, P. latidens, and P. presidionis (Mateos et al., 2002; Miller et al., 2005). Thus, competition will have four factors from no competitors to three co-occurring competitors (0,1,2,3). To our knowledge there are no published accounts of the degree of similarity or the niche overlap among these four species. However, general accounts of these four species indicated that they are ecologically similar—they all inhabit the mid-water column in streams and small rivers, they are similar in body form, and they are omnivorous, consuming plant, and animal matter (Miller et al., 2005). Furthermore, we collected these species in the same microhabitat and it has been shown that P. prolifica body shape converges with that of its congeners when co-occurring due to interspecific competition (Roth-Monzón et al., 2020). Hence, we conclude that due to previous evidence, their close phylogenetic relationship, and ecological similarity there is high potential for competitive interactions. We used number of co-occurring species as an indicator of level of interspecific competition in our analysis. We chose number of co-occurring species to focus on answering the effect of the addition of species in life-history traits. Furthermore, it is a known measure that has affected P. prolifica (Roth-Monzón et al., 2020). Additionally, number of competitors is a general measure that can characterize interspecific competition throughout several years. We found no piscivorous predators in the localities sampled. However, in all locations, we found another species of livebearer (Poecilia butleri). We also found one location with a very low density (only 16 individuals collected) of an introduced livebearer (Gambusia affinis).
We use stream slope to characterize stream velocity. We calculated stream slope in ArcMap 10.6 (Enviromental Systems Research Institute [ESRI]., 2014), as the difference between upper elevation and lower elevation of a 2-km segment of stream for each locality sampled. It is known that stream velocity increases as stream slope increases, thus this indirect measure should be a good proxy for stream velocity (Gore and Banning, 2017). We recognize that there is variation among sites in the number of pools and riffles, regardless of the overall slope across the focal 2-km segment of the stream. Frequently, poeciliids use pools of stagnant water for foraging activities and social interactions (Mazzoni et al., 2011). However, these pools are highly dynamic, some of them dry out, and new ones are constantly formed. Thus, our proxy for stream velocity does not represent these changing microhabitats, which may be temporarily available to fish depending on the amount of daily precipitation or water runoff, but instead represents an estimate of the general water velocity that fish experience all throughout the year. Individuals that inhabit a steep river may indeed search for pools, but they certainly deal with fast currents when moving among pools. In contrast, individuals that inhabit a stream that runs through a plain landscape will never experience fast water currents, not even when moving between pools. Therefore, selection for more streamlined phenotypes with enhanced swimming abilities, which in turn impose constraints on reproductive allotment (Zúñiga-Vega et al., 2010), must be overall stronger in streams located in steep terrains than in streams located in relatively plain areas.
We estimated resource availability measuring canopy cover with a hand-held densiometer. Canopy cover is an indicator of primary productivity (Grether et al., 2001; Zimmermann and Death, 2002; Kiffney et al., 2004; Schiesari, 2006). High canopy cover indicates lower primary productivity that has been correlated with lower secondary productivity such as invertebrates, thus being a reliable measure of resources in a stream (Grether et al., 2001; Zimmermann and Death, 2002; Kiffney et al., 2004; Schiesari, 2006). Canopy cover has been a good proxy for resource availability in other studies with streams similar to ours (Grether et al., 2001; Collins et al., 2015). Two populations had missing data on canopy cover (localities four and seven); to avoid the exclusion of these in the analysis we used aerial images from Google Earth to calculate percent cover in ImageJ. This approach has been used before and found to strongly correlate with field measurements of canopy cover (Inskeep et al., 2011). In our localities, we also found a strong correlation between our field measurements of canopy cover and our calculations of canopy cover from aerial images (R2 = 0.76, P = 0.010). To understand if competition, resources, stream slope, and population density covaried, we conducted a pairwise correlation tests. We found no significant correlations among these putative selective agents (Table 3).
Life-History Traits
We quantified four different life-history traits: superfetation (number of simultaneous broods carried by a female), individual embryo size, number of embryos (across all broods; Frías-Alvarez and Zúñiga-Vega, 2016), and reproductive allotment. We choose these traits because the four selective pressures of interest are thought to affect them, and clear predictions can be made of their effects (see Table 1). We classified embryo development stage using the 11-stage scale developed by Haynes (1995). We defined a brood as all the embryos that share the same developmental stage (Haynes, 1995). We measured individual embryo size by drying an entire brood of offspring in a desiccating oven for 48 h at 55°C and dividing the brood dry mass by the number of embryos in the brood. To avoid non-independence in individual embryo size due to the fact that females can have more than one brood, we only considered the brood at the most advanced developmental stage of each female for the calculation of individual embryo size. We obtained female somatic dry mass by drying the female soma (minus the intestinal tract and offspring) for 48 h at 55°C. For reproductive allocation, we used the total dry mass of all broods of each female relative to somatic dry mass of the female following Tomkins and Simmons, 2002.
To account for the effect of maternal body size differences on each life-history trait, we adjusted each trait by an analysis of covariance (ANCOVA). We adjusted individual embryo size by including maternal somatic dry mass and developmental stage as covariates, to obtain comparable “size-free” and “stage-free” least squares means for analysis. We only adjusted superfetation, number of embryos, and reproductive allocation by using maternal somatic dry mass as a covariate. To meet assumptions of normality for the ANCOVAs, we used the following transformations on the life-history traits: superfetation and total number of embryos were square root transformed, whereas embryo size and reproductive allocation were log transformed. Hence, the comparison among populations were done using the adjusted least squares means generated by the ANCOVA models allowing us to compare “size-free” and “stage-free” life-history traits.
Before using the adjusted life histories in a model selection approach, we tested for population differences in all four life-history traits by employing a multivariate analysis of covariance (MANCOVA). Likewise, we included maternal somatic dry mass and stage of development as covariates in the MANCOVA. We found significant differences among the 12 populations of P. prolifica in all life-history traits (F11,1136 = 14.75, P ≤ 0.001), so we proceeded with the model selection approach using the adjusted least squares means. All of these analyses were implemented in R software (R Core Team., 2020).
Model Selection
We generated a set of 14 candidate models that represent competing biological hypotheses of the way the four putative selection agents could act to shape life-history traits (Figure 2, Table 4, and Supplementary Figure 1). We did not include all possible interactions between factors because we wanted to include only those that represent plausible hypotheses taken from theory (Table 1). We used a structural equation modeling approach (i.e., path analysis) because it allowed us to assess both direct and indirect effects of the selective agents. Assessing indirect effects is important in our study because some selective agents (e.g., competition and population density) are usually only considered in previous studies as acting through indirect effects (Scott and Johnson, 2010; Reznick et al., 2012).
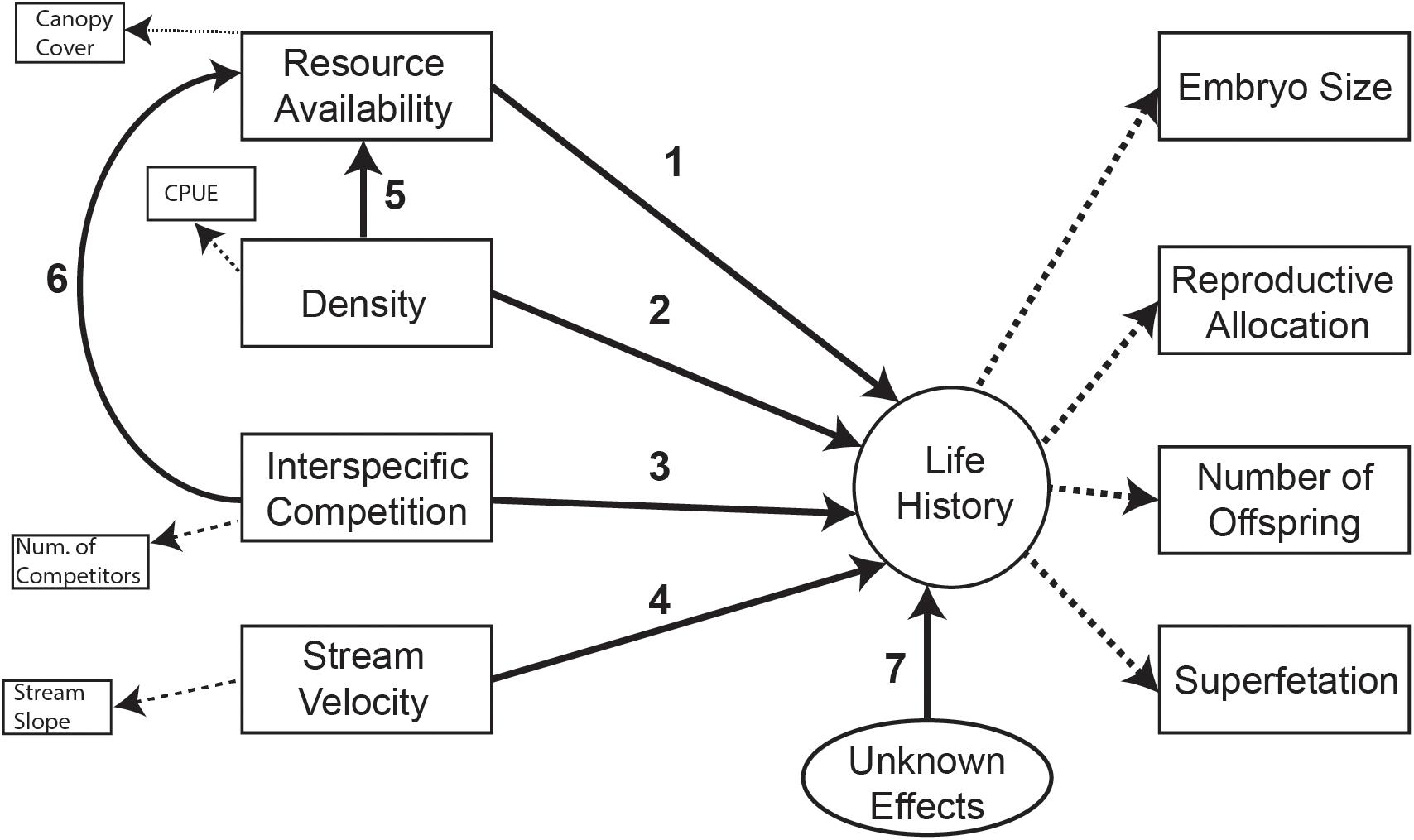
Figure 2. Path diagram of the global model with both direct and indirect effects of four putative selective agents on life histories. Numbers above each path line correspond to path ID (also found in Table 1 and Table 4).
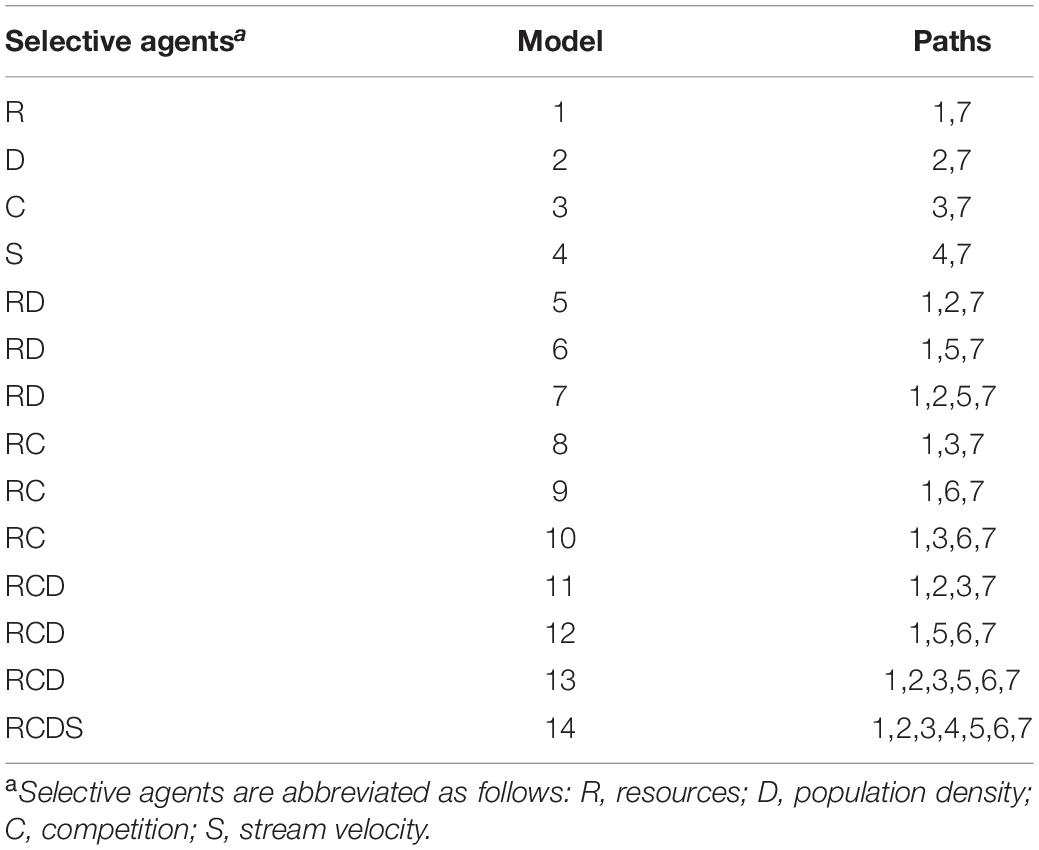
Table 4. List of the a priori 14 candidate models of the effect of the four putative selective agents.
We know that life-history traits can co-vary and its theory suggests that it may evolve as an integrated suite of traits (Fisher, 1930; Reznick, 1985). This assumption is important as it affects whether life histories can be evaluated as a collective strategy or if each life-history trait should be considered separately. To assess this assumption we used a confirmatory factor analysis that allowed us to test if a single factor (i.e., a single life-history variable) could adequately summarize all four life-history traits measured (Burnham and Anderson, 2002). During this confirmatory analysis, we found a negative error for reproductive allocation. We determined that the negative error was due to sample variance (Van Driel, 1978; Chen et al., 2001), thus we restricted the error to a small positive number (0.01) following Van Driel (1978). Fixing the error term as a small positive number allowed us to continue running a factor analysis and retain the potential for error in the measurement of reproductive allocation. We found that three of the four life-history traits appear to behave as an integrated suite of traits in P. prolifica, as they all had a positive association and loadings greater than 0.50 in the calculated life-history suite (Figure 3), corroborating the idea that certain life-history traits evolve in an integrated fashion (Fisher, 1930; Reznick, 1985). These three correlated life-history traits were number of embryos, reproductive allocation, and superfetation and, hence, our life-history suite is a combination of these three variables in such a way that large positive values of this variable correspond to females that produce many embryos, high reproductive allocation, and several simultaneous broods. In turn, large negative values of our life-history suite indicate females with the opposite set of traits (fewer embryos, low reproductive allocation, and fewer simultaneous broods). In contrast, embryo size had a small loading and very little variation explained by this life-history suite. Thus, our life-history suit was not a good reflection of embryo size, so for all the models we treated embryo size separately. We therefore proceeded to test the different hypotheses through a multi-model inference approach with two life histories measures as response: the life-history suite (which is a combined measure of number of embryos, reproductive allocation, and superfetation) and embryo size alone.
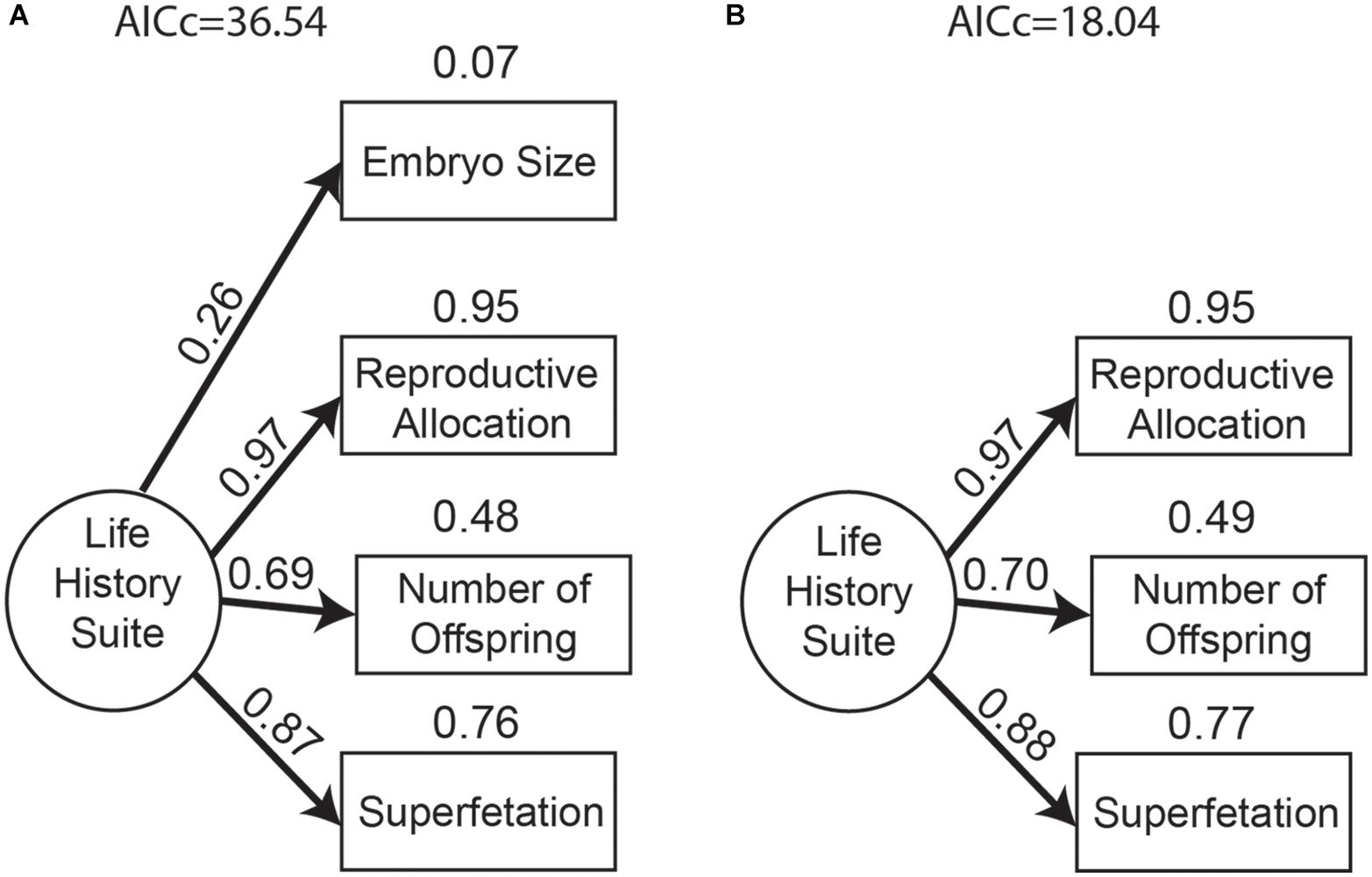
Figure 3. Path diagrams of confirmatory factor analysis for (A) all life-history traits as a single measure and (B) excluding embryo size. Path diagram shows loadings above each path and proportion of variance accounted for (R2) above each life-history trait.
We ran all 14 candidate models in path analysis using the software Amos (Arbuckle, 2013). All models were run using a maximum likelihood estimator. For each model, we generated an Akaike Information Criteria score (corrected for small sample sizes; AICc). We used AICc scores to identify models that best fit the data. Models in which AICc scores differ by less than two are generally considered indistinguishable (Burnham and Anderson, 2002; Burnham et al., 2011). We also calculated the model-averaged standard total effect for each selective agent using all 14 models and their associated AIC weights. The model-averaged standard total effect represents the amount of change in either the life-history suite or embryo size given a standard deviation unit change in each of the selective agent included in the models.
Results
Population density and interspecific competition alone were the best predictors of number of embryos, reproductive allocation, and superfetation in P. prolifica. For this life-history suite, the two models with population density and interspecific competition alone as direct effects had lower AICc scores than all other models. However, it was not possible to distinguish between these two models as they differed by less than two AICc score units (Table 5). For embryo size, all models that included a single selective agent had the lowest AICc values and were equivalent in terms of AICc (Table 6).
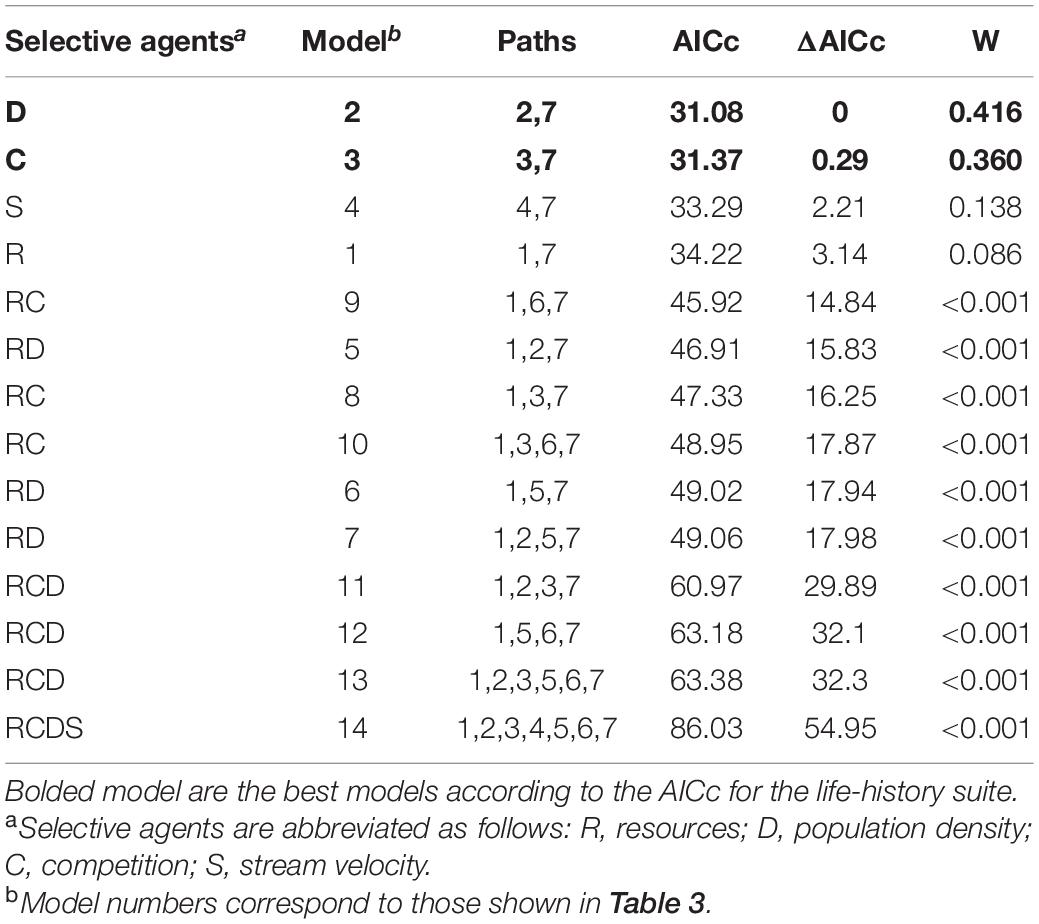
Table 5. List of the a priori 14 candidate models of the effect of the four putative selective agents on the life-history suite with corresponding AICc values, ΔAICc, and AICc weight (W).
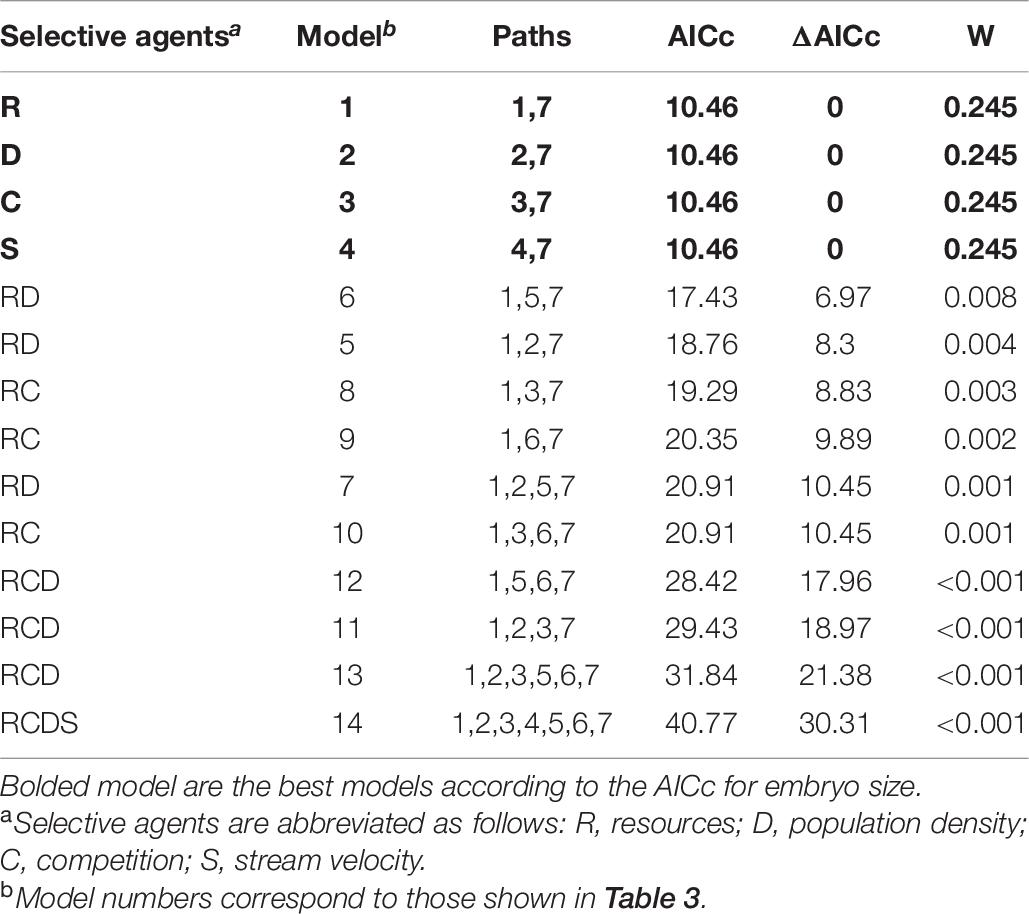
Table 6. List of the a priori 14 candidate models of the effect of the four putative selective agents on embryo size with corresponding AICc values, ΔAICc, and AICc weight (W).
Although the two models with population density and interspecific competition alone were undistinguishable based on AICc scores for the life-history suite, they differed in the strength of their effects on life histories. Population density had a stronger effect than interspecific competition (Figure 4), but both models revealed a positive influence of these two environmental factors on the life-history suite. In other words, increasing population density or interspecific competition results in an increase in all three life-history traits (number of embryos, RA, and superfetation).
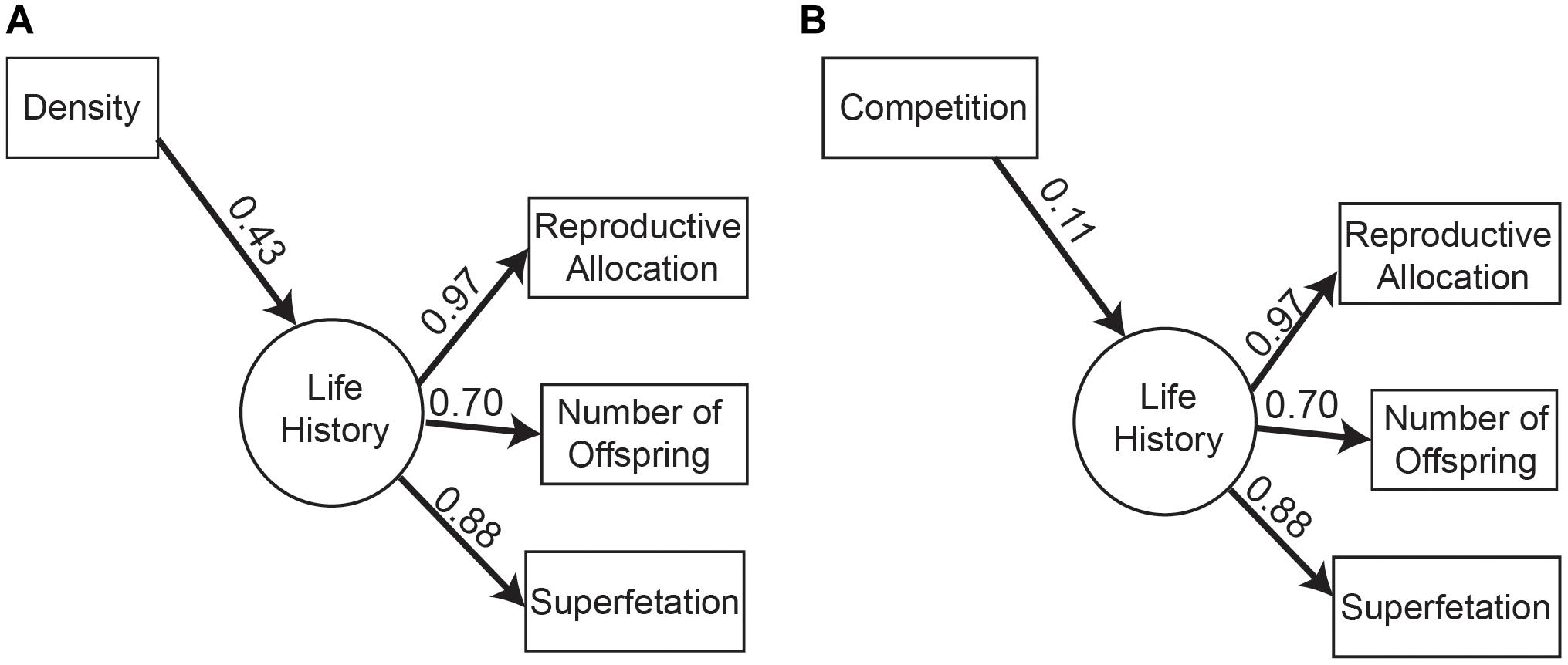
Figure 4. Path diagrams of best models for the life-history suite showing (A) population density and (B) competition as direct effect on the life histories. Path diagram shows model-averaged standard total effect above the path line.
For embryo size, all selective pressures, except interspecific competition, had the predicted effect from theory (Table 1 and Figure 5). This means that embryo size increased as population density increased (Figure 5A), and decreased as resources and stream gradient increased (Figures 5B,D). In contrast, interspecific competition had a positive effect on embryo size (Figure 5C). This said, the model-averaged standard total effect was generally low for all four selective agents suggesting the strength of these selective pressures was overall weak on embryo size (Figure 5).
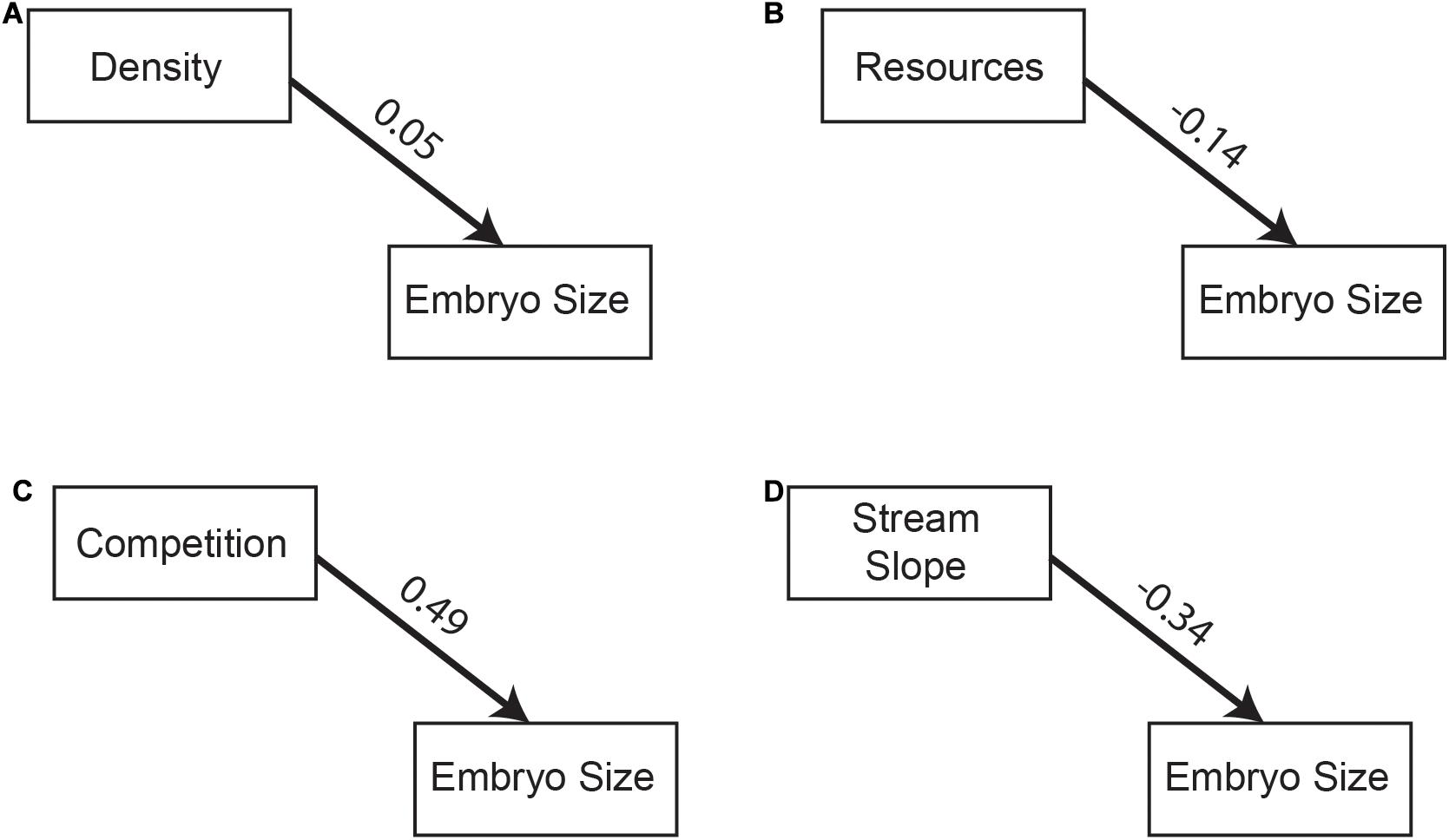
Figure 5. Path diagrams of best models for embryo size showing (A) population density, (B) resource availability, (C) competition, and (D) stream slope as direct effect on embryo size. Path diagram shows model-averaged standard total effect above the path line.
Discussion
Overall we found mixed results, for embryo size selective agents were indistinguishable in their ability to predict life-history variation as in a previous study with the livebearing fish Brachyrhaphis rhabdophora (Johnson, 2002). However, for the other three life-history traits studied two selective agents independently, competition and population density, were equally likely to explain the variation in P. prolifica.
As interspecific competition increased, we found an increase in number of embryos, reproductive allocation, and superfetation. These results are consistent with theoretical predictions suggesting that poor competitive abilities can be compensated by a large reproductive effort (Hutchinson, 1957; Levine and Rees, 2002; Kneitel and Chase, 2004; Leibold et al., 2004; Calcagno et al., 2006; Chapuis et al., 2017). Hence, our findings indicate that P. prolifica may have low competitive abilities, but is likely better at reproduction. However, we did not measure competitive abilities which will be needed to confirm the hypothesis of a trade-off between reproduction and competitive abilities (Kneitel and Chase, 2004; Calcagno et al., 2006). Nevertheless, a trade-off is the most likely explanation for the observed positive association between the life-history suite and interspecific competition, otherwise a decrease in number of embryos and reproductive allocation would be expected (Scott and Johnson, 2010). Furthermore, interspecific competition was also positively associated with embryo size, meaning that for P. prolifica an increase in interspecific competition causes an increase in all four life-history traits, which also supports a trade-off between competitive ability and reproduction. Noticeably, the fact that intense competition can increase both number and size of offspring indicates that the common trade-off between these two life-history traits, which has been previously documented in other poeciliids (e.g., Poeciliopsis gracilis and P. infans; Frías-Alvarez et al., 2014), does not occur in P. prolifica.
Population density was also positively associated with each of the life-history traits evaluated here (reproductive allocation, number of embryos, superfetation, and embryo size). However, for embryo size the effect of population density was small (Figure 5). Nevertheless, results are still somewhat puzzling for the three other life-history traits in that increasing population density is expected to result in a decrease in number of embryos and in reproductive allocation (Johnson and Bagley, 2011; Moore et al., 2016). Higher population density should result in a reduction in per capita resource availability, resulting in lower reproductive allocation and number of embryos, but higher superfetation (Table 1). Two possibilities could account for our observations. First, competition in our system may not be through competition for available food resources as appears to be the case in other studies (Reznick, 1989; Reznick et al., 2002). In other words, life-history traits in P. prolifica may be shifting to improve competitive ability in a context different from competition for resources, such as microhabitat (Table 1). Second, most studies examining the effects of population density on life history have been conducted in species that lack superfetation (e.g., Smith, 2007; Reznick et al., 2012). The ability to carry multiple broods simultaneously in P. prolifica might confer advantages that alter the effect of population density on life history (Zúñiga-Vega et al., 2017). For example, higher superfetation is related to higher reproductive allocation in other livebearing fishes (Zúñiga-Vega et al., 2017), and it has been suggested that superfetation allows these species to overcome morphological constraints in terms of reproductive investment (Frías-Alvarez and Zúñiga-Vega, 2016; Zúñiga-Vega et al., 2017). Thus, it is possible that species that superfetate can have more offspring without the typical trade-off relative to non-superfetating species (Olivera-Tlahuel et al., 2015). This could allow for the positive relationship between population density and the life-history traits observed in our study.
Resource availability was not a good predictor of the life history suite, despite the fact this is a known selective agent for other poeciliids (Zúñiga-Vega et al., 2010; Johnson and Bagley, 2011). One possibility is that food resources are simply not limiting in this system and therefore play a lesser role in shaping life histories. Moreover, food resources availability can change through time (Reznick and Yang, 1993) and can be affected by several abiotic factors (i.e., substrate disturbance, stream velocity, etc., Zimmermann and Death, 2002; Hall et al., 2015) and it is possible that when we sampled did not capture the effects of resource availability. Another explanation could be that canopy cover, although a useful surrogate for primary productivity in streams, does not adequately reflect the resource dynamics in this system. If so, then future studies could focus on the inclusion of different measures to construct a more direct estimate of resource availability.
We were similarly surprised to find that stream velocity did not predict life history in our system, given that P. prolifica is a superfetating species and stream velocity is known to affect this life-history-trait in other superfetating poeciliid fish species (Zúñiga-Vega et al., 2007, 2010). One possible reason for this is that our use of stream slope may not capture the actual water velocity that these populations encounter. It is possible that P. prolifica females spend their majority of time in pools, rather than in the current where stream slope would be a better predictor of flow rate (Croft et al., 2003). Thus, measuring water velocity in the particular pool where individuals are observed could better account for the stream velocity that the species is experiencing. Additionally, stream velocity is a complex measure that can vary in time and space, especially depending on space complexity and heterogeneity (Lake, 2000; Palmer et al., 2010). Several measures may be needed to truly account for stream velocity variability, and basic information about species-specific microhabitat preferences could aid in considering what is the best measure for a particular species. It is also important to note that the effect of stream velocity on superfetation has not been conclusive, as some studies have found positive effects (Zúñiga-Vega et al., 2007) while others have found no effect (Frías-Alvarez and Zúñiga-Vega, 2016; Zúñiga-Vega et al., 2017). More research will be needed to understand how generally stream velocity can affect life histories. Finally, stream velocity is potentially a gradient in each population due to spatial heterogeneity. This gradient may cause individual fish to experience high variability in the direction and magnitude of selection. Adaptation to stream velocity could only occur in environments that are consistent in streamflow allowing selection time to act. Furthermore, the effect of stream velocity may not be detectable on those populations that experience gradients (either spatially or temporally) with a single point in time and several collections will be needed to reflect this variability.
The effect of all selective pressures on embryo size were small in P. prolifica. It is unclear why this is the case. It is possible that the selective agents that we examined here are simply weak in their ability to shape embryo size. Alternatively, embryo size may be a trait with little variation among populations such as has been previously observed in other poeciliids (e.g., Poecilia butleri; Zúñiga-Vega et al., 2011), perhaps as a result of genetic constraints. Despite the modest responses of offspring size to the putative selective agents, the pattern of change observed here was consistent in the direction predicted by theory for all selective agents (Table 1).
We found that the effect of interspecific competition was generally weaker than the effect of population density in predicting life history. This supports a body of theory that argues that intraspecific competition in general should be stronger than interspecific competition (Chesson, 2000, 2013; Adler et al., 2018). Although this was true for the majority of life-history traits examined here, this was not the case for embryo size where interspecific competition was stronger than the effects of population density. We note that studies which consider interspecific competition as a selective pressure on life histories of livebearers are uncommon (Scott and Johnson, 2010; Chapuis et al., 2017). Hence, our findings point to a promising area for future research, especially in understanding the interplay of ecological interactions both within and among species.
Finally, it is interesting to note that both population density and interspecific competition best explained our data when included as direct effects on life histories. Usually, when competition (both intraspecific and interspecific) is considered in life-history research, it is usually explained in terms of its indirect effect on life history mediated through food resource availability (Reznick and Yang, 1993; Scott and Johnson, 2010; Johnson and Bagley, 2011; Wilson, 2013). However, this was not the case in our study. Several possibilities could account for this result. First, food resource availability may not be as important in our system as in others; and it is possible that competition could be acting through some other environmental variable, such as habitat use. Second, this could simply be an artifact of the model selection framework used in our analysis. Models with indirect effects required more parameters, and consequently these models are penalized for the inclusion of additional parameters. Hence, the models may not be favored not because indirect effects are unimportant, but because they are not sufficiently important to offset the cost of including them in the model. When comparing models with direct and indirect effects at comparable nesting (models 5 vs. 6, 8 vs. 9, and 11 vs. 12), most were indistinguishable (Table 4 and Table 5), so penalization for number of parameters may play an important aspect on preference for direct and indirect effects. Finally, it is possible that competition indeed has a strong direct effect on life history in P. prolifica. If this is the case, we need to consider how competition could act as a direct selective agent on life histories, an area where we still lack a strong theoretical foundation to make predictions.
Data Availability Statement
The raw data supporting the conclusions of this article will be made available by the authors, without undue reservation.
Ethics Statement
The animal study was reviewed and approved by the Brigham Young University Ethics Committee.
Author Contributions
AJR-M, JJ, and MB conceived the study and interpreted the data analysis. AJR-M and JJZ-V collected all samples and processed samples. AJR-M collected and analyzed the data, and wrote the first draft of the manuscript. All authors contributed to manuscript corrections, editing, and improved the final manuscript.
Funding
This research was partially funded by a BYU Graduate Student Research Grant and the Vern Parish Fund from the American Livebearer Association awarded to AJR-M. AJR-M acknowledges support from CONACYT scholarship and a High Impact Doctoral Research Assistantship from BYU.
Conflict of Interest
The authors declare that the research was conducted in the absence of any commercial or financial relationships that could be construed as a potential conflict of interest.
Acknowledgments
We thank A. Mendoza-Hernández and H. Camarillo for help on fieldwork collection. T. Williams provided useful comments to this manuscript.
Supplementary Material
The Supplementary Material for this article can be found online at: https://www.frontiersin.org/articles/10.3389/fevo.2020.608046/full#supplementary-material
References
Adler, P. B., Smull, D., Beard, K. H., Choi, R. T., Furniss, T., Kulmatiski, A., et al. (2018). Competition and coexistence in plant communities: intraspecific competition is stronger than interspecific competition. Ecol. Lett. 21, 1319–1329. doi: 10.1111/ele.13098
Badyaev, A. V., and Ghalambor, C. K. (2001). Evolution Of Life Histories Along Elevational Gradients: Trade-Off Between Parental Care And Fecundity. Ecology 82, 2948–2960. doi: 10.1007/BF01647286
Banet, A. I., Svendsen, J. C., Eng, K. J., and Reznick, D. N. (2016). Linking reproduction, locomotion, and habitat use in the Trinidadian guppy (Poecilia reticulata). Oecologia 181, 87–96. doi: 10.1007/s00442-015-3542-9
Burnham, K. P., and Anderson, R. D. (2002). Model selection and inference: a practical information-theoretic approach. New York, NY: Springer.
Burnham, K. P., Anderson, D. R., and Huyvaert, K. P. (2011). AIC model selection and multimodel inference in behavioral ecology: some background, observations, and comparisons. Behav. Ecol. Sociobiol. 65, 23–35. doi: 10.1007/s00265-010-1029-6
Calcagno, V., Mouquet, N., Jarne, P., and David, P. (2006). Coexistence in a metacommunity: the competition–colonization trade-off is not dead. Ecol. Lett. 9, 897–907. doi: 10.1086/320865
Chapuis, E., Lamy, T., Pointier, J.-P., Juillet, N., Ségard, A., Jarne, P., et al. (2017). Bioinvasion Triggers Rapid Evolution of Life Histories in Freshwater Snails. Am. Nat. 190, 694–706. doi: 10.1086/693854
Chen, F., Bollen, K. A., Paxton, P., Curran, P. J., and Kirby, J. B. (2001). Improper Solutions in Structural Equation Models. Sociolog. Methods Res. 29, 468–508. doi: 10.1007/BF02293865
Chesson, P. (2000). Mechanisms of Maintenance of Species Diversity. Annu. Rev. Ecol. Syst. 31, 343–366. doi: 10.1146/annurev.ecolsys.31.1.343
Chesson, P. (2013). “Species Competition and Predation,” in Encyclopedia of Sustainability Science and Technology, ed. R. A. Meyers (New York, NY: Springer), 223–309. doi: 10.1007/978-1-4419-0851-3
Collins, S. M., Kohler, T. J., Thomas, S. A., Fetzer, W. W., and Flecker, A. S. (2015). The importance of terrestrial subsidies in stream food webs varies along a stream size gradient. Oikos 125, 674–685. doi: 10.1111/j.1365-2435.2011.01865.x
Croft, D. P., Albanese, B., Arrowsmith, B. J., Botham, M., Webster, M., and Krause, J. (2003). Sex-biased movement in the guppy (Poecilia reticulata). Oecologia 137, 62–68. doi: 10.1007/s00442-003-1268-6
Cruz, D. O., Kingsford, R. T., Suthers, I. M., Rayner, T. S., Smith, J. A., and Arthington, A. H. (2020). Connectivity but not recruitment: Response of the fish community to a large-scale flood on a heavily regulated floodplain. Ecohydrology 13:1042. doi: 10.1002/rra.1061
Frías-Alvarez, P., and Zúñiga-Vega, J. J. (2016). Superfetation in live-bearing fishes is not always the result of a morphological constraint. Oecologia 181, 645–658. doi: 10.1007/s00442-015-3477-1
Frías-Alvarez, P., Garcia, C. M., Vázquez-Vega, L. F., and Zúñiga-Vega, J. J. (2014). Spatial and temporal variation in superfoetation and related life history traits of two viviparous fishes: Poeciliopsis gracilis and P. infans. Naturwissenschaften 101, 1085–1098. doi: 10.1007/s00114-014-1247-2
Ghalambor, C. K., Reznick, D. N., and Walker, J. A. (2004). Constraints on Adaptive Evolution: The Functional Trade-Off between Reproduction and Fast-Start Swimming Performance in the Trinidadian Guppy (Poecilia reticulata). Am. Nat. 164, 38–50. doi: 10.1086/421412
Gore, J. A., and Banning, J. (2017). “Discharge Measurements and Streamflow Analysis,” in Methods in Stream Ecology, eds F. R. Hauer and G. A. Lamberti (Cambridge,MA: Academic Press), 49–70. doi: 10.1016/b978-0-12-416558-8.00003-2
Grether, G. F., Millie, D. F., Bryant, M. J., Reznick, D. N., and Mayea, W. (2001). Rain Forest Canopy Cover, Resource Availability, And Life History Evolution In Guppies. Ecology 82, 1546–1559. doi: 10.1890/0012-96582001082[1546:RFCCRA]2.0.CO;2
Hall, R. O., Tank, J. L., Baker, M. A., Rosi-Marshall, E. J., and Hotchkiss, E. R. (2015). Metabolism, Gas Exchange, and Carbon Spiraling in Rivers. Ecosystems 19, 73–86. doi: 10.1007/s10021-015-9918-1
Haynes, J. L. (1995). Standardized Classification of Poeciliid Development for Life-History Studies. Copeia 1995, 147–154. doi: 10.2307/1446809
Heins, D. C., and Baker, J. A. (2017). Streamflow environment predicts divergent life history phenotypes among populations of the Blacktail Shiner Cyprinella venusta: Temporal stability of a large-scale pattern. Ecol. Freshw Fish 27, 453–459. doi: 10.1111/j.0030-1299.2007.15763.x
Inskeep, D., Wagner, A., and Buchanan, B. A. (2011). Estimating Canopy Cover Using Aerial Photography For A Mixed Conifer Zone, Northern, New Mexico. Proc. Am. Soc. Mining Reclam. 2011, 306–315. doi: 10.21000/JASMR11010306
Johnson, J. B. (2002). Divergent life histories among populations of the fish Brachyrhaphis rhabdophora: detecting putative agents of selection by candidate model analysis. Oikos 96, 82–91. doi: 10.1034/j.1600-0706.2002.960109.x
Johnson, J. B., and Bagley, J. C. (2011). “Ecological drivers of life-history evolution,” in Ecology and evolution of poeciliid fishes, eds J. P. Evans, A. Pilastro, and I. Schlupp (Chicago, IL: Universtiy of Chicago Press), 38–49.
Johnson, J. B., and Belk, M. C. (2001). Predation environment predicts divergent life-history phenotypes among populations of the livebearing fish Brachyrhaphis rhabdophora. Oecologia 126, 142–149. doi: 10.1007/s004420000504
Kiffney, P. M., Richardson, J. S., and Bull, J. P. (2004). Establishing light as a causal mechanism structuring stream communities in response to experimental manipulation of riparian buffer width. J. North Am. Bentholog. Soc. 23, 542–555. doi: 10.1899/0887-3593(2004)023<0542:elaacm>2.0.co;2
Kneitel, J. M., and Chase, J. M. (2004). Trade-offs in community ecology: linking spatial scales and species coexistence. Ecol. Lett. 7, 69–80. doi: 10.1046/j.1461-0248.2003.00551.x
Kobza, R. M., Trexler, J. C., Loftus, W. F., and Perry, J. (2004). Community structure of fishes inhabiting aquatic refuges in a threatened Karst wetland and its implications for ecosystem management. Biol. Conserv. 116, 153–165. doi: 10.1016/S0006-3207(03)00186-1
Lake, P. S. (2000). Disturbance, patchiness, and diversity in streams. J. North Am. Bentholog. Soc. 19, 573–592. doi: 10.2307/1468118
Leibold, M. A., Holyoak, M., and Mouquet, N. (2004). The metacommunity concept: a framework for multi-scale community ecology. Ecology 7, 601–613. doi: 10.1111/j.1461-0248.2004.00608.x
Levine, J. M., and Rees, M. (2002). Coexistence and Relative Abundance in Annual Plant Assemblages: The Roles of Competition and Colonization. Am. Nat. 160, 452–467. doi: 10.1086/342073
Martin, T. E. (1995). Avian Life-History Evolution in Relation to Nest Sites, Nest Predation, and Food. Ecol. Monogr. 65, 101–127. doi: 10.2307/2937160
Mateos, M., Sanjur, O. I., and Vrijenhoek, R. C. (2002). Historical Biogeography Of The Livebearing Fish Genus Poeciliopsis (Poeciliidae: Cyprinodontiformes). Evolution 56, 972–984. doi: 10.1111/j.0014-3820.2002.tb01409.x
Mazzoni, R., Novaesm, V. C., and Iglesias-Rios, R. (2011). Microhabitat use by Phalloceros harpagos Lucinda (Cyprinodontiformes:Poeciliidae) from a coastel stream from Southeast Brazil. Neotropical. Ichthyol. 9, 665–672. doi: 10.1590/s1679-62252011005000027
Miller, R. R., Minckley, W. L., and Norris, S. M. (2005). Freshwater Fishes of México. Chicago, IL: University of Chicago Press.
Moore, M. P., Riesch, R., and Martin, R. A. (2016). The predictability and magnitude of life-history divergence to ecological agents of selection: a meta-analysis in livebearing fishes. Ecol. Lett. 19, 435–442. doi: 10.1086/282956
Olivera-Tlahuel, C., Ossip-Klein, A. G., Espinosa-Pérez, H. S., and Zúñiga-Vega, J. J. (2015). Have superfetation and matrotrophy facilitated the evolution of larger offspring in poeciliid fishes? Biol. J. Linnean Soc. 116, 787–804. doi: 10.1111/bij.12662
Palmer, M. A., Menninger, H. L., and Bernhardt, E. (2010). River restoration, habitat heterogeneity and biodiversity: a failure of theory or practice? Freshwater Biol. 55, 205–222. doi: 10.1007/BF00032721
Pérez-Mendoza, H. A., Zúñiga-Vega, J. J., Martorell, C., Zurita-Gutiérrez, Y. H., Solano-Zavaleta, I., Hernández-Rosas, A. L., et al. (2014). Patterns of spatio-temporal variation in the survival rates of a viviparous lizard: the interacting effects of sex, reproductive trade-offs, aridity, and human-induced disturbance. Popul. Ecol. 56, 605–618. doi: 10.1111/j.1365-2435.2006.01180.x
Pollux, B. J. A., Pires, M. N., Banet, A. I., and Reznick, D. N. (2009). Evolution of Placentas in the Fish Family Poeciliidae: An Empirical Study of Macroevolution. Annu. Rev. Ecol. Evol. Syst. 40, 271–289. doi: 10.1146/annurev.ecolsys.110308.120209
R Core Team. (2020). R: A Language And Environment For Statistical Computing. R Foundation for Statistical Computing. Vienna: R Core Team.
Reznick, D. (1985). Costs of Reproduction: An Evaluation of the Empirical Evidence. Oikos 44, 257–267. doi: 10.2307/3544698
Reznick, D. N. (1989). Life-History Evolution in Guppies .2. Repeatability of Field Observations and the Effects of Season on Life Histories. Evolution 43, 1285–1297. doi: 10.2307/2409363
Reznick, D. N., and Yang, A. P. (1993). The Influence of Fluctuating Resources on Life History: Patterns of Allocation and Plasticity in Female Guppies. Ecology 74, 2011–2019. doi: 10.2307/1940844
Reznick, D. N., Bassar, R. D., Travis, J., and Helen Rodd, F. (2012). Life-History Evolution In Guppies VIII: The Demographics Of Density Regulation In Guppies (Poecilia reticulata). Evolution 66, 2903–2915. doi: 10.1111/j.1461-0248.2009.01393.x
Reznick, D., Bryant, M. J., and Bashey, F. (2002). r- and K-selection revisited: The role of population regulation in life-history evolution. Ecology 83, 1509–1520. doi: 10.1890/0012-9658(2002)083[1509:raksrt]2.0.co;2
Reznick, D., Butler, M. J. IV, and Rodd, H. (2001). Life-History Evolution in Guppies. VII. The Comparative Ecology of High- and Low-Predation Environments. Am. Nat. 157, 126–140. doi: 10.1086/318627
Riesch, R., Plath, M., and Schlupp, I. (2010). Toxic hydrogen sulfide and dark caves: life-history adaptations in a livebearing fish (Poecilia mexicana, Poeciliidae). Ecology 91, 1494–1505. doi: 10.1890/09-1008.1
Riesch, R., Plath, M., Schlupp, I., Tobler, M., and Brian Langerhans, R. (2014). Colonisation of toxic environments drives predictable life-history evolution in livebearing fishes (Poeciliidae). Ecol. Lett. 17, 65–71. doi: 10.1111/ele.12209
Roth-Monzón, A. J., Belk, M. C., Zúñiga-Vega, J. J., and Johnson, J. B. (2020). Beyond Pairwise Interactions: Multispecies Character Displacement in Mexican Freshwater Fish Communities. Am. Nat. 195, 983–996. doi: 10.1086/708513
Santi, F., Bierbach, D., Schartl, M., and Riesch, R. (2019). Life histories of guppies (Poecilia reticulata Peters, 1869; Poeciliidae) from the Pitch Lake in Trinidad. Car. J. Sci. 49:255. doi: 10.18475/cjos.v49i2.a13
Schiesari, L. (2006). Pond canopy cover: a resource gradient for anuran larvae. Freshwater Biol. 51, 412–423. doi: 10.2307/1447379
Scott, L. E., and Johnson, J. B. (2010). Does sympatry predict life history and morphological diversification in the Mexican livebearing fish Poeciliopsis baenschi? Biol. J. Linnean Soc. 100, 608–618. doi: 10.1111/j.1095-8312.2010.01452.x
Smith, C. C. (2007). Independent effects of male and female density on sexual harassment, female fitness, and male competition for mates in the western mosquitofish Gambusia affinis. Behav. Ecol. Sociobiol. 61, 1349–1358. doi: 10.1080/08927014.1995.9522966
Tomkins, J. L., and Simmons, L. W. (2002). Measuring relative investment: a case study of testes investment in species with alternative male reproductive tactics. Anim. Behav. 63, 1009–1016. doi: 10.1006/anbe.2001.1994
Van Driel, O. (1978). On the various causes of improper solutions in maximum likelihood analysis. Psychometrika 43, 225–243. doi: 10.1007/bf02293865
Wilson, A. J. (2013). Competition as a source of constraint on life history evolution in natural populations. Heredity 112, 70–78. doi: 10.1038/hdy.2013.7
Zani, P. A., and Stein, S. J. (2018). Field and laboratory responses to drought by Common Side-blotched Lizards (Uta stansburiana). J. Arid Environ. 154, 15–23. doi: 10.1016/j.jaridenv.2018.03.001
Zimmermann, E. M., and Death, R. G. (2002). Effect of substrate stability and canopy cover on stream invertebrate communities. N. Zealand J. Mar. Freshwater Res. 36, 537–545. doi: 10.1146/annurev.ento.43.1.271
Zúñiga-Vega, J. J., Macías-Garcia, C., and Johnson, J. B. (2010). “Hypothesis to Explain the Evolution of Superfetation in Viviparous Fishes,” in Viviparous Fishes II, eds M. C. Uribe and H. J. Grier (Mexico: New Life Publications), 242–253.
Zúñiga-Vega, J. J., Olivera-Tlahuel, C., and Molina-Moctezuma, A. (2017). Superfetation increases total fecundity in a viviparous fish regardless of the ecological context. Acta Oecologica 84, 48–56. doi: 10.1016/j.actao.2017.08.002
Zúñiga-Vega, J. J., Reznick, D. N., and Johnson, B. J. (2007). Habitat predicts reproductive superfetation and body shape in the livebearing fish Poeciliopsis turrubarensis. Oikos 116, 995–1005. doi: 10.1111/j.2007.0030-1299.15763.x
Keywords: resource availability, interspecific competition, density, life history, poeciliids, stream velocity
Citation: Roth-Monzón AJ, Belk MC, Zúñiga-Vega JJ and Johnson JB (2021) What Drives Life-History Variation in the Livebearing Fish Poeciliopsis prolifica? An Assessment of Multiple Putative Selective Agents. Front. Ecol. Evol. 8:608046. doi: 10.3389/fevo.2020.608046
Received: 18 September 2020; Accepted: 14 December 2020;
Published: 11 January 2021.
Edited by:
Wayne Iwan Lee Davies, Umeå University, SwedenReviewed by:
Ingo Schlupp, University of Oklahoma, United StatesEugenia Zandona, Rio de Janeiro State University, Brazil
Copyright © 2021 Roth-Monzón, Belk, Zúñiga-Vega and Johnson. This is an open-access article distributed under the terms of the Creative Commons Attribution License (CC BY). The use, distribution or reproduction in other forums is permitted, provided the original author(s) and the copyright owner(s) are credited and that the original publication in this journal is cited, in accordance with accepted academic practice. No use, distribution or reproduction is permitted which does not comply with these terms.
*Correspondence: Andrea J. Roth-Monzón, rothmonzon@gmail.com
†Present address: Andrea J. Roth-Monzón, Department of Ecology and Evolutionary Biology, University of Connecticut, Mansfield, CT, United States