- 1Centre for Environment, Fisheries and Aquaculture Science, Lowestoft, United Kingdom
- 2School of the Environment and Department of Biology, Trent University, Peterborough, ON, Canada
- 3Department of Ecology & Vertebrate Zoology, University of Łódź, Łódź, Poland
- 4Centre for Ecology, Environment and Sustainability, Bournemouth University, Bournemouth, United Kingdom
We review a model we developed in 2007 to predict the invasion potential of an introduced population, based on the relationship between juvenile growth (mean total length at age 2) and mean age-at-maturity, and test the model with data from 75 non-native populations of pumpkinseed Lepomis gibbosus in nine countries of Europe and western Asia. The database used to construct the model was derived from a mix of primary and secondary data collected between 1981 and 2016. Based on number of specimens collected or catch-per-unit-effort data, and/or comments by the original authors, populations were classified a priori as “invasive” or “non-invasive.” The plot of the proposed model placed populations in three invasiveness categories (non-invasive, transitional, invasive). Of those predicted by the model to be invasive, only 57% were correct with regard to their a priori classification, a result that was not statistically significant. For populations predicted by the model to be non-invasive, 70% were correct with regard to their a priori classification when “transitional” were grouped with non-invasive (P = 0.0024), and 64% were correct when “transitional” were excluded from the test (P = 0.12). Applications of the model to two other non-native freshwater fishes (black bullhead Ameiurus melas and crucian carp Carassius carassius) are also discussed. The lack of stronger statistical support for the model may have been the result of using life-history traits from the populations after they were introduced, as the source populations were unknown, as well as to shortcomings in the dataset that could affect a priori and modeled classifications. We conclude that such life-history models can be useful for predicting invasiveness status in non-native freshwater fishes, though the basis of the predictions, statistical or heuristic, will depend on the size and quality of the underlying life-history database.
Introduction
A major challenge confronted by environmental managers and policy makers in the development and implementation of strategies to avoid or mitigate bioinvasions is the identification of non-native species likely to become invasive and exert adverse impacts on native species and ecosystem services (Williams and Grosholz, 2008; Zengeya et al., 2017). To examine a species’ “invasiveness,” one must first acknowledge that there are numerous definitions of the term “invasive” (for a review, see Copp et al., 2005). These can be grouped into those that refer to a species that reproduces prolifically, spreads and may (or may not) exert an adverse impact (i.e., equivalent to a “weed”) and those that restrict the definition to species that exert an adverse impact. For the purposes of this paper, we rely loosely on the definition given on page 244 of the aforementioned article: “These are native or alien species that spread, with or without the aid of humans, in natural or semi-natural habitats, producing a significant change in composition, structure, or ecosystem processes, or cause severe economic losses to human activities.” However, studies that actually assess adverse impacts by freshwater fish species are in general limited to a few prominent species, e.g., common carp Cyprinus carpio Linnaeus, 1758 (reviewed in Vilizzi et al., 2015), so risk analysis considers a non-native species’ attributes, which include invasion history, the propagule pressure associated with the species’ introductions, population traits, and life-history traits.
The underlying purpose of risk analysis is to inform decision makers as to the threats posed by non-native species so that measures may be taken to avoid the introduction of potential pest species, or to control the spread as well as to limit (mitigate) the impacts of existing non-native species. The first step in this process is to identify which species are likely to be invasive, and the tools used in this non-native screening process range from purely qualitative (e.g., Kohler and Stanley, 1984) to the highly quantitative (e.g., Kolar and Lodge, 2001). The use of models to horizon scan for future invasives can aid the risk assessor in evaluating a species; perhaps the most common are climate matching models. Much more rare are models based primarily on life-history traits for predicting invasiveness (Ricciardi, 2007; see also Ricciardi and Kipp, 2008), such as the life-history-trait-based models for predicting invasiveness we proposed (Copp and Fox, 2007) for the North American centrarchid, pumpkinseed Lepomis gibbosus (Linnaeus, 1758). This model is based on the inverse relationship in female pumpkinseed between mean age-at-maturity and juvenile growth, i.e., mean total length (TL) at age 2, to identify which introduced populations in European inland waters are (or can be expected to be) invasive. We chose pumpkinseed as a test (or “model”) species because: (a) the relationship between juvenile growth and mean age-at-maturity had already been assessed in populations of this species in part of its native range (Fox, 1994); (b) an increasing amount of growth and life-history data was being acquired for pumpkinseed populations introduced to European waters with which to evaluate the use of these traits in a model to predict invasiveness; (c) many of the populations for which these data are available have been evaluated for invasiveness by one or more of the criteria listed, though with regard to definitions of the term “invasive,” the most commonly reported aspects were high density and/or prolific reproduction; and (d) population expansion is facilitated in environments with a species initially not present with “r-selected traits” like early maturity. Based on the relationship between juvenile growth and mean age-at-maturity elaborated by Fox (1994) for native pumpkinseed populations in Ontario, Canada (Figure 1A), the “model” proposed by Copp and Fox (2007) was applied to non-native pumpkinseed populations in Europe (e.g., Cucherousset et al., 2009; Ağdamar et al., 2015; Masson et al., 2015).
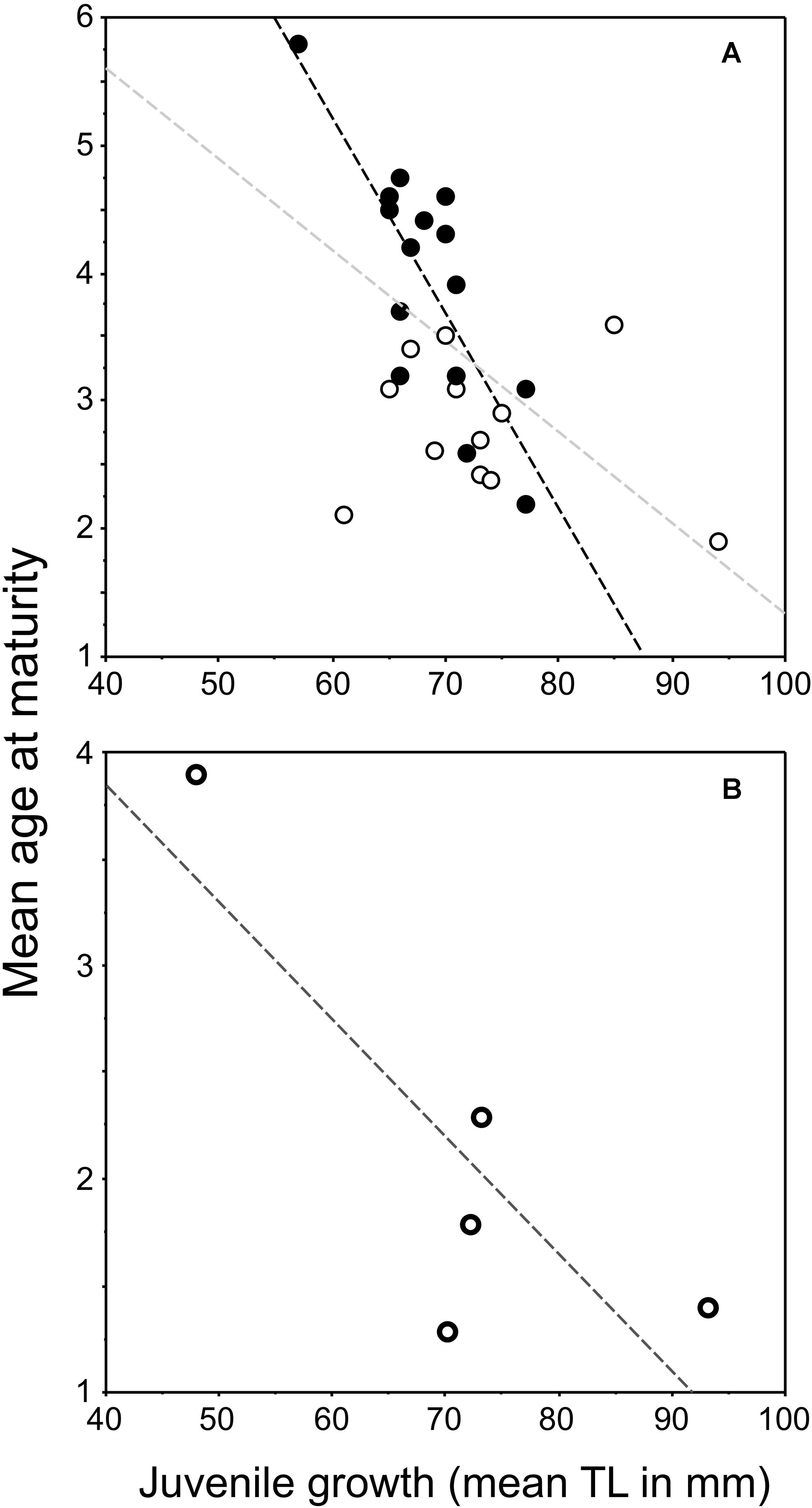
Figure 1. Plots of the relationship between juvenile growth [mean back-calculated total length (TL) at age 2] and mean age-at-maturity of female pumpkinseed: (A) redrafted values from Fig. 6 in Fox (1994) for native populations in Ontario, Canada, and (B) plot of values from Table 6 in Copp et al. (2002) for introduced populations in Europe. The regression lines in “(A)” are significant for populations from lakes containing bluegill Lepomis macrochirus (Rafinesque, 1819) (filled dots, black dashed line: y = –0.153x + 14.382, r2 = 0.646, P = 0.0002), for all lakes in Ontario (filled and open dots, gray dashed line: y = –0.071x + 8.449, r2 = 0.283; P = 0.0036), but not for the Ontario lakes where bluegill was absent (open dots, no line: y = –0.011x + 3.582, r2 = 0.029, P = 0.5977). In “(B),” the relationship for the non-native populations in Europe prior to 2000 (data from Table 6 in Copp et al., 2002) was not significant (y = –0.055x + 6.049, r2 = 0.684, df = 3, P = 0.0842) despite as similar trend that that in native populations.
However, despite there being considerable knowledge of the underlying biological response of species quantified by the model, what remains to be determined is how good this combination of traits is as a predictor of actual invasiveness in an introduced fish species or population. Thus, the aim of this review is to evaluate the application of the Copp and Fox (2007) model with regard to its potential for predicting the potential invasiveness of non-native freshwater fish populations. Also briefly discussed are two applications of this model to predict the invasiveness of the North American ictalurid catfish, black bullhead Ameiurus melas (Rafinesque, 1820) in European waters and that of the European cyprinid Carassius carassius (Linnaeus, 1758), which is not native to Great Britain (Copp et al., 2016; Tarkan et al., 2016).
Background to the Model
Model Development
With regard to pumpkinseed introduced to Europe, the first review and analysis of existing data (Copp et al., 2002) revealed that mean age-at-maturity appeared to be inversely related to the juvenile growth rate of the population (Figure 1B). This relationship, which is predicted from some life-history models (e.g., Stearns and Koella, 1986) and observable at the population level in many fish species (reviewed in Fox, 1994), was not quite significant but resembled that described by Fox (1994). This initial plot for introduced European populations comprised a single population in England (upper left of the graph, Figure 1B) and a few, mainly southern and central, European populations (Copp et al., 2002). When new data points from England (Villeneuve et al., 2005) were included in the regression, we found the relationship for introduced European pumpkinseed populations to be highly significant (Figure 2). This relationship between juvenile growth and maturity appeared to explain some of the inter-population variation in mean age-at-maturity not explained by latitude alone (Copp and Fox, 2007). And because virtually all of the populations considered to be “invasive,” i.e., those located in Southern Europe, were in the lower right of the graph, and the non-invasive English populations (Villeneuve et al., 2005; Fox et al., 2011) were situated in the upper left of the graph, we proposed the use of this relationship (Figure 2) as a predictor of the potential invasiveness of pumpkinseed populations in Europe (Copp and Fox, 2007).
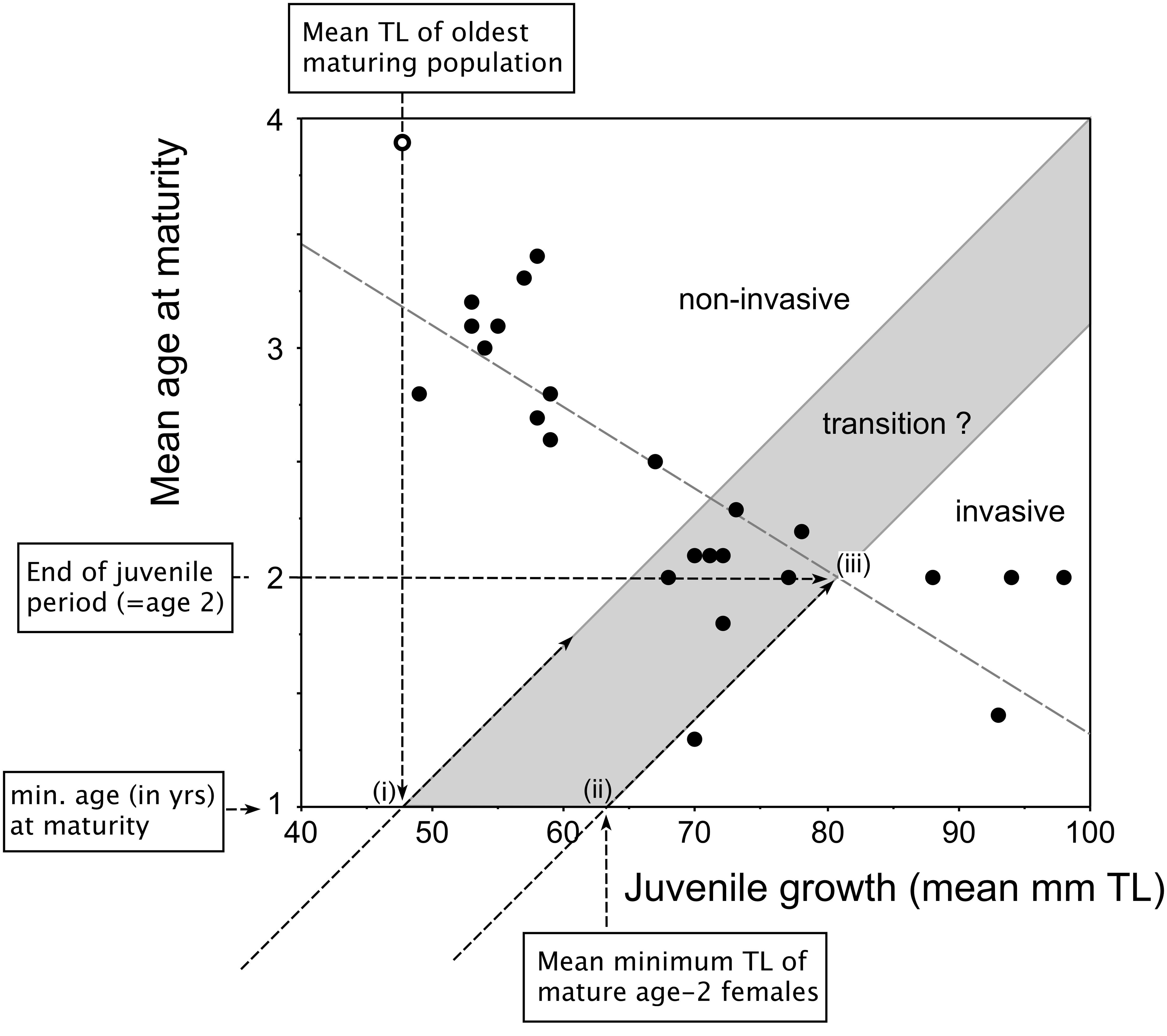
Figure 2. Redrafted plot of invasiveness prediction model proposed by Copp and Fox (2007, Fig. 6) based on the relationship between juvenile growth (in mm of back-calculated TL at age 2) and mean age-at-maturity of female pumpkinseed in the species’ non-native European range. Labeled arrows indicate the key coordinates for designation of the grayed area, which represents the populations transitioning between non-invasive populations (upper left-hand) and invasive populations (lower right-hand). See text for detailed explanation.
To delineate the upper extent of the invasive-non-invasive transition zone in this model (Figure 2), we identified “i” the mean TL at age 1 year (i.e., the minimum age at which maturity is achieved in pumpkinseed) of the oldest-maturing population and traced a 45° line from the origin through that point on the x-axis. To delineate the lower extent of the transition zone, two points were used: “ii” the mean minimum size (in TL) of mature age-2 females on the x-axis, representing their juvenile growth rate in the first year of life, and “iii” the mean size (TL) of females at the end of juvenile growth, i.e., at age 2 years. The designated zone effectively spans the second-year growth phase during which juveniles are allocating differential amounts of energy to somatic and gonadal growth as they mature into adults.
Data Sources and Model Predictions
The first evaluation of this model was undertaken by the addition of new data points (Figure 3A) acquired through the sampling of pumpkinseed populations in northerly parts of Europe (Cucherousset et al., 2009). Three further applications of the model, as presented in Cucherousset et al. (2009), include new data from England (Figure 3B), Turkey (Figure 3C), and France (Figure 3D). In England, a study of pumpkinseed dispersal and life-history traits in a small stream system examined the applicability of the model with respect to a long-established (source) population in an upstream mill-pond reservoir and a new downstream population (Figure 3B), which gained access to and established in an adjacent ornamental pond that connected temporarily to the stream during an extreme flood event during July 2007 (Fobert et al., 2013). By 2010, the new population had undergone an increase in juvenile growth and a corresponding decrease in mean age-at-maturity, relative to the source population, since gaining access to the ornamental pond (Figure 3B). This newly-established population was re-sampled during the summer of 2016 (same methods as Villeneuve et al., 2005), and these previously unpublished data comprise 130 specimens. During the 6-year period, the mean age-at-maturity of this relatively-new population decreased further by >1 year, but with a nominal increase in juvenile growth (Figure 3B), which within the model represented a transitional shift toward invasiveness.
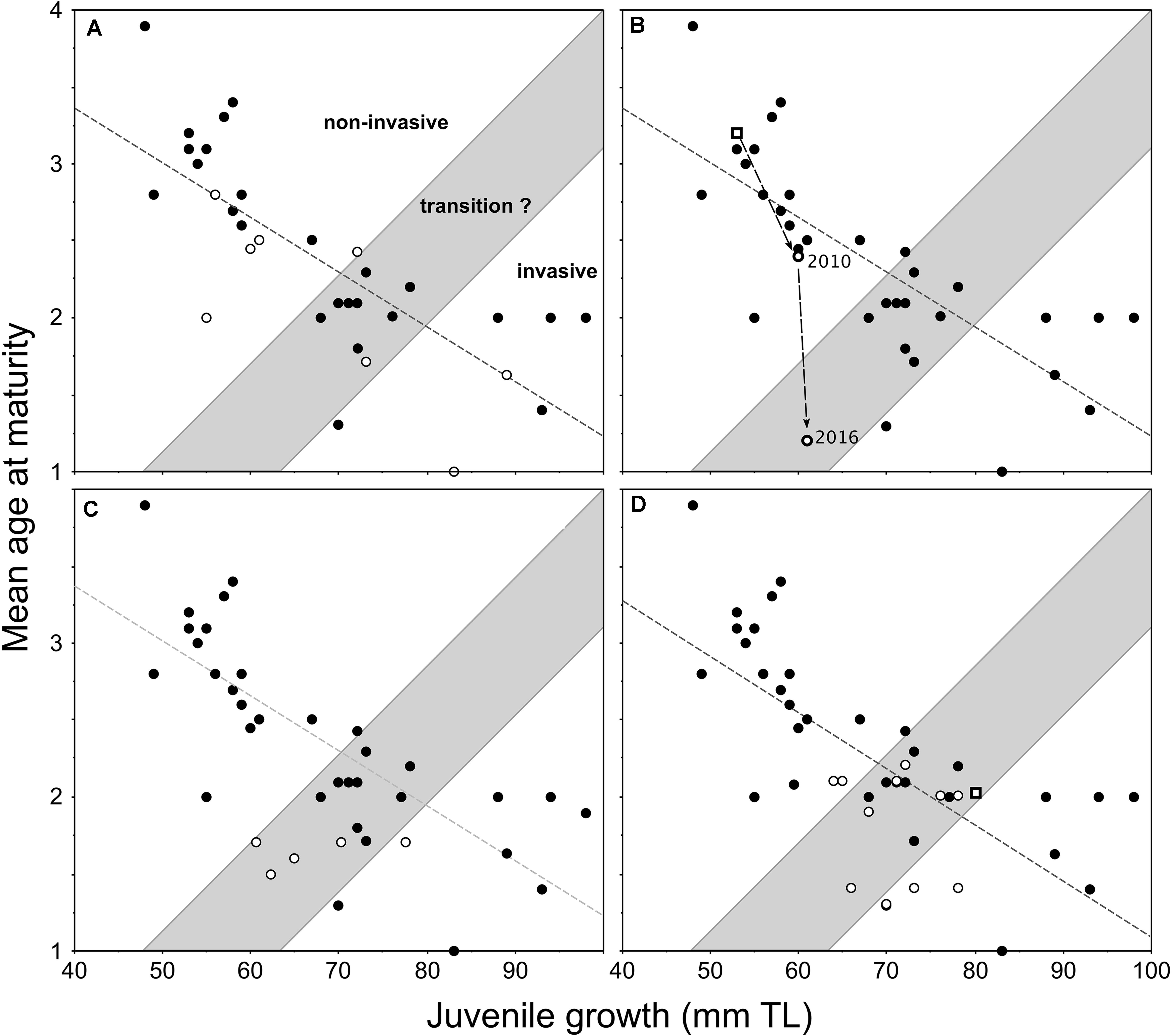
Figure 3. Redrafted plots of the applications of invasiveness prediction model proposed for female pumpkinseed in Fig. 6 of Copp and Fox (2007) with the addition of data (open circles) from populations in (A) northerly locations of Europe (Cucherousset et al., 2009); and (B) the same populations as in (A) but with the source population (from a mill-pond reservoir) and a downstream ornamental pond population, which gained access to and established in the pond during an extreme flood in July 2007 (Fobert et al., 2013); (C) the same populations as in (A) but with five established populations in Turkey (Ağdamar et al., 2015); and (D) the same populations as in (A) with various sites along the longitudinal course of the River Moselle basin, France (Masson et al., 2015) as well as a recently-established population (open square) in a small angling lake in England (Copp et al., 2017).
A separate application of the model in Turkey, described by Ağdamar et al. (2015), resulted in new data points for female pumpkinseed from five populations in artificial still waters (four reservoirs and one canal) revealed relatively similar mean ages-at-maturity across an approximate 20-mm span of juvenile growth (Figure 3C). And in France, a study of growth and reproduction in populations at various sites along the longitudinal course of the River Moselle basin showed that the life-history response of Moselle pumpkinseed populations to warmer temperatures occurred primarily in age-1 individuals (Masson et al., 2015). Three of the 11 Moselle populations, including both of those inhabiting heated waters, were predicted to be invasive, with six populations in the transition zone and only two just inside of the “non-invasive” group of populations (Figure 3D).
Test of the Model
In the various examinations of the relationship between juvenile growth and mean age-at-maturity (as per Fox, 1994) in non-native European fishes (Masson et al., 2015; Copp et al., 2016; Tarkan et al., 2016), the data points tended to further strengthen the underlying growth–maturity relationship that was the basis of the model that Copp and Fox (2007) proposed to predict pumpkinseed invasiveness. However, the ability of this model to predict invasiveness has yet to be tested. To test the model, we focused on pumpkinseed for two reasons: (1) a largest data set exists for non-native populations (Fox and Copp, 2014), and (2) a large amount of bibliographic evidence exists with which to categorise a priori the populations as either “invasive” or “non-invasive.” However, this data set was constructed from sources that date back to studies carried out in the early 1980s (Constantinescu, 1981) when bioinvasion terminology, such as invasive, pest, alien species, etc., was not used in those publications. Therefore, to determine whether or not pumpkinseed was considered to be invasive in water body, we examined available documents for evidence of the species being referred to as very abundant and widespread, or simply very abundant. If no such evidence was provided in the source paper, then we relied on information we could obtain for water bodies of the same type in the same river basin.
In the more recent studies, the published articles tended to label the study population as being “invasive” and/or the article provides more detailed information on the numbers of pumpkinseed captured from, or processed for, each population. If the total number of fish captured was available, such as studies carried out by us and our colleagues, then our databases and field notes were used to classify them a priori as invasive or non-invasive. In some cases (e.g., Spain), the source publications for pumpkinseed life-history and growth data used in our study (e.g., Gutiérrez-Estrada et al., 2000a, b) did not mention invasiveness or high abundance despite reporting large numbers of pumpkinseed captured. However, other articles on fish assemblages in the same river or lake basins refer to pumpkinseed as invasive or very abundant (e.g., Vila-Gispert et al., 2002; Prenda et al., 2006; Almeida et al., 2009; Ribeiro and Collares-Pereira, 2010; Almeida and Grossman, 2014; De Miguel et al., 2014; Rooke and Fox, 2018). For pumpkinseed in ponds and streams in England, we know from our extensive studies (e.g., Copp et al., 2002, 2010; Klaar et al., 2004; Villeneuve et al., 2005; Cucherousset et al., 2009; Fobert et al., 2013) that the species does not reproduce in streams, and that pumpkinseed densities in ponds occupied by a characteristic assemblage of native fish species are not invasive. However, in “disturbed” waters, such as newly-created (dug out) ponds, e.g., water hazards on a golf course, pumpkinseed densities were high (Fox et al., 2011) and therefore the population was classed as “invasive.”
In cases where the total number of pumpkinseed captured was not recorded, then the number of fish processed was used as an estimator of fish density, with populations for which the numbers of fish processed was ≥100 specimens, that population was classified a priori as invasive and those of fish processed <100 classified as non-invasive. In some cases, a population was known (from raw data for that population or from our colleague’s knowledge of the pumpkinseed populations) to occur in high (invasive) densities despite the number of fish processed being <100 fish. This was the case for one of the ten pumpkinseed populations examined in the River Moselle, France (Masson et al., 2015) – that the population was classified accordingly and half of the populations were classified a priori as invasive and half as non-invasive. In some cases, detailed data on pumpkinseed densities were reported. For example, a study in Netherlands of growth and life-history traits of pumpkinseed invasiveness in ponds (Van Kleef and Jongejans, 2014) provided, in the article’s supplementary information, density estimates using a standardise sampling protocol. The ponds with elevated density estimates were used to classify the populations as invasive (109–1288 fish per 100 m transect; n = 3) and non-invasive (1–69 fish per 100 m; n = 10).
True and false predictions from the model for the pumpkinseed database were tested against their classification with the aforementioned criteria. For this analysis, two separate binomial tests were conducted, one for populations predicted to be invasive and one for populations predicted to be non-invasive. Two versions of the test were conducted for the non-invasive prediction: one in which populations predicted to be transitional were grouped with those predicted to be non-invasive, and one where the transitional populations were excluded from the analysis. The rationale for including the predicted transitional populations with the predicted non-invasive populations is based on two related sources. Firstly, the implementation of non-native species policy in the management of invasive species prioritises high-risk species (“need to act” species), with low- and medium-risk species dealt with when circumstances and/or resources permit (“nice to act” species). Secondly, and inspired by the above, the calibration of assessment scores generated by risk screening tools, such as the freshwater Fish Invasiveness Screening Kit (Vilizzi et al., 2019) and the Aquatic Species Invasiveness Screening Kit (see applications cited in Li et al., 2020), has focused on the identification of the threshold between species that pose a high risk of being invasive in a given risk-assessment area and those that pose as a low or medium risk of being invasive (Vilizzi et al., 2019).
The results of our binomial test with the European pumpkinseed database do not strongly support the utility of the model for predicting invasive and non-invasive populations. For populations predicted to be invasive, only 57% (12 of 21) were correct by our classification criteria for invasive, a result that was not significant (binomial test, P = 0.33). On the other hand, 70% (39 of 56) of populations predicted to be non-invasive or transitional were correct by our criteria and significant (P = 0.0024), but not when transitional populations were excluded (64% correct, P = 0.12).
Discussion
An essential question in the use of a life-history model to predict invasiveness is whether the underlying biological state reflected in that model facilitates a species acclimation to, and invasion of, novel environments. In the present case, the basic interpretation of our model is that fast pre-maturational growth and early maturity predisposes a population to be successful in a situation when it is newly introduced, and ultimately allows it to expand not only its population, but also its distribution. Early maturity, which works with fast growth to predict invasion success in the model, is what one would expect in “r-selected” populations of MacArthur and Wilson (1967), i.e., those that may be favoured during the initial stages of a species’ establishment and spread in a novel environment because of its low density (Fox and Copp, 2014). Since fecundity is related to body size in fishes, fast juvenile growth combined with early maturity would potentially result in a large number of propagules, which is a strong predictor of invasion success (Duggan et al., 2006; Copp et al., 2007; Simberloff, 2009).
Despite these theoretical reasons why the life-history model should be a good predictor of invasion success, the model was not strongly supported in our tests with the pumpkinseed. This could be simply due to shortcomings in the dataset, which include the variety of sampling methods used (i.e., non-uniformity of sampling), the absence of density estimates for some of the populations, the extensive time interval over which the data emanates (from the 1970s onward), and differences in environmental factors that could influence the growth–maturity relationship.
Furthermore, the life-history traits used in the tests are based on samples taken in the recipient water bodies, and not the source populations that were originally introduced. This was out of necessity because, although we now have an indication of the general geographic origin of non-native European pumpkinseed populations (Yavno et al., 2020), the actual source populations were unknown. Given the likelihood that the expression of these traits was influenced by conditions in the recipient water body, the assumption that the trait mean values are reflective of the source populations is questionable, and the differences between these trait mean values in the originally introduced fish and those sampled an unknown number of years later may differ in magnitude among the water bodies sampled.
Another consideration is that life-history traits that are useful during the early stages of invasion may not be at a later phase, when population density has increased (García-Berthou, 2007; Gutowsky and Fox, 2012; Masson et al., 2016). Indeed, a preliminary assessment of changes in mean reproductive effort in non-native pumpkinseed populations found that those thought to have existed for <50 years exerted a significantly higher effort than those established prior to 1950 (Copp and Fox, 2007). As such, invasion success may depend, not only on the initial life-history traits of the introduced species or population, but also on the species’ ability to adapt its life-history traits in a later phase of the invasion. The pumpkinseed has demonstrated a high degree of life-history plasticity when newly introduced in both North America (Fox, 1994) and Europe (Fox et al., 2007). In European waters, where there are fewer pumpkinseed competitors and predators than in its native range, the life-history traits that are predicted by our model to favour invasion success appear to have been maintained over the course of these invasions (Fox and Copp, 2014). This may explain why our model worked somewhat to predict invasion potential, but it may not work at all for other species or even for pumpkinseed in other geographic regions where more potential predators and competitors are present or where thermal regimes are less favourable.
It must be highlighted that our invasion model is population-based rather than species-based, and this raises the question of whether populations of a given species, especially a highly plastic species, are likely to vary substantially from one another in invasion success. The answer to this may depend on the degree to which adaptation in different regions, or environments within regions, has genetically altered the life-history reaction norms of populations inhabiting these areas (see Hutchings et al., 2007; Fletcher et al., 2016). In the case of pumpkinseed, the wide variety of freshwater habitats it occupies over a broad native geographic range in North America and Europe suggests that its broad inter-population variation in mean age-at-maturity and growth rate (Fox, 1994; Fox and Copp, 2014) are due to adaptation and phenotypic plasticity (see Yavno et al., 2020). If that is the case and if life-history reaction norms differ across populations as a result of these adaptations, then it follows that populations will vary in performance when introduced into novel environments. Therefore, it is appropriate to make invasion success predictions for a species at the population level.
Applications of the Copp and Fox (2007) model to black bullhead (Copp et al., 2016; Jaćimović et al., 2019) and crucian carp (Tarkan et al., 2016), despite their small data sets, certainly demonstrate the same growth–maturity relationship observed in pumpkinseed (Figure 4). However, the ability of these models to predict invasiveness of the species remains to be tested. The model for non-native black bullhead was elaborated using TL at age 3 as the estimator of juvenile growth (Figure 5A), being that females in most black bullhead populations do not achieve maturity until age 3. In an application of this model to assess the impact of a viral infection on the invasiveness status of a “boom” phase population of black bullhead in a floodplain water body of the River Sava, Belgrade, Serbia (Jaćimović et al., 2019), the model should predict a sharp increase in juvenile growth with corresponding sharp decrease in mean age-at-maturity.
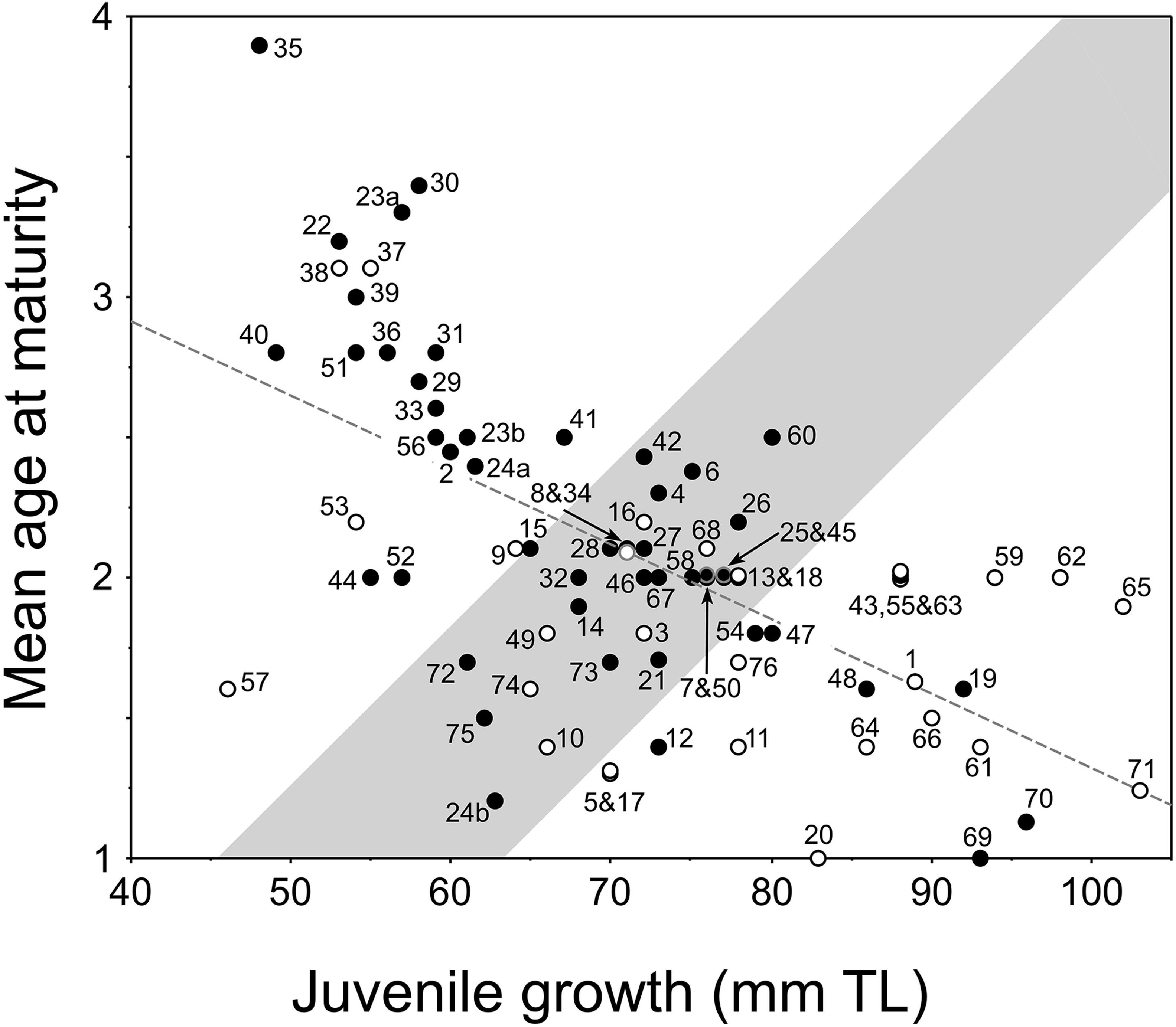
Figure 4. New plot of the relationship between juvenile growth [mean back-calculated total length (TL) at age 2] and mean age-at-maturity of female pumpkinseed in non-native European populations (i.e., Fox, 1994): y = –0.027x + 3.981, r2 = 0.39, F = 47.291, df = 74, P = 0.0001. Plot presents re-delineated transition zone due to new data (from Fox and Copp, 2014), with populations considered a priori as invasive distinguished (open dots) from those considered non-invasive (filled dots) as described and numbered (1–76) in Supplementary Table 1. In cases of overlapping values, the dots are slightly separated vertically.
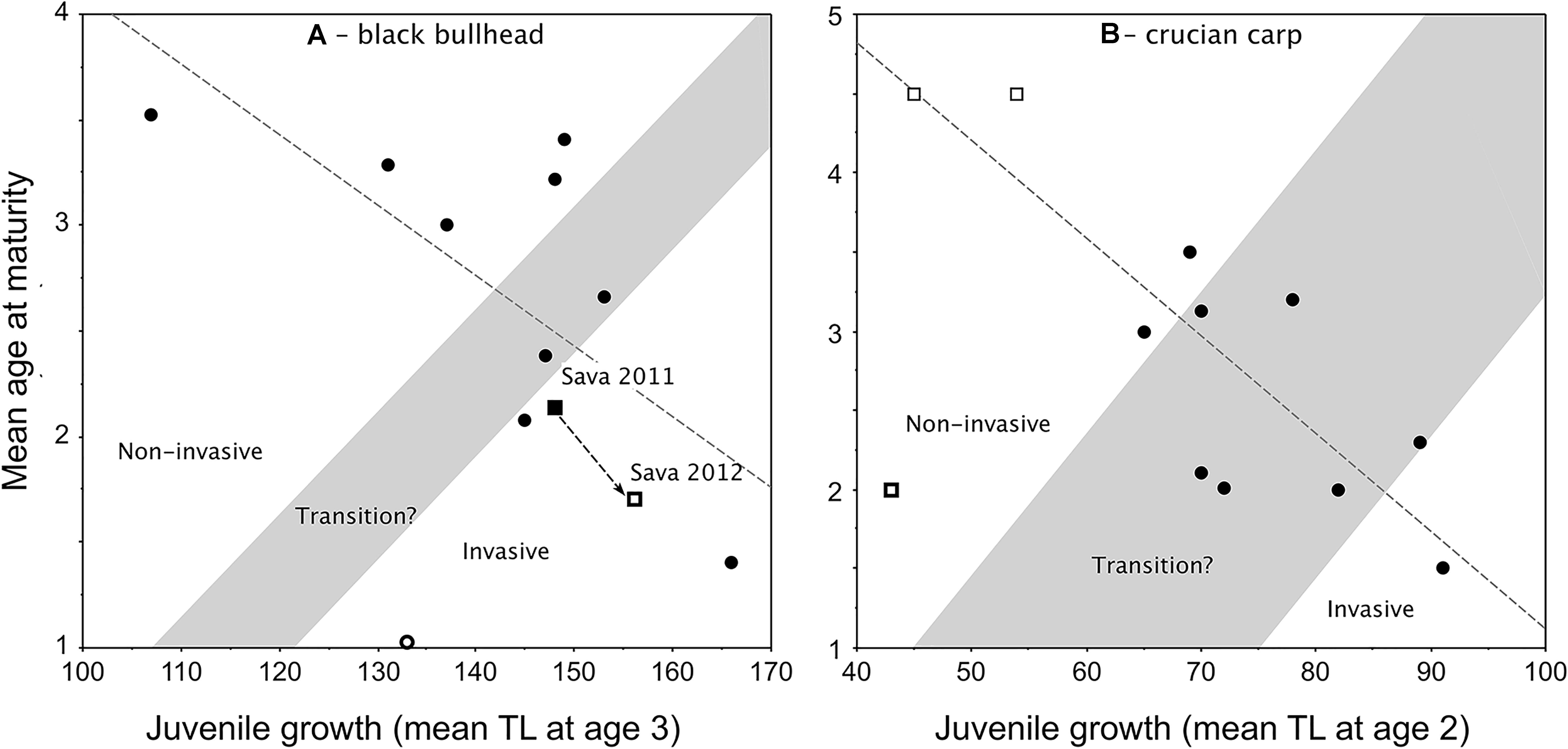
Figure 5. Redrafted plots of the Copp and Fox (2007) model applied to: (A) non-native black bullhead Ameiurus melas populations in Europe (Copp et al., 2016), with retraced transition zone as per the criteria in Figure 2 and retraced regression line (y = –0.03x + 7.441, r2 = 0.499, F = 8.956, df = 9, P = 0.0151) that includes two data points for a population in Lake Sava (Jaćimović et al., 2019), which underwent a boom (2011) and bust (2012) sequence, but ignores the extreme (female-dominated) outlier (thick-outlined open circle; Canal de Jouy, France; Copp et al., 2016); and (B) crucian carp Carassius carassius populations (Tarkan et al., 2016) from the native continental Europe (open squares) and the non-native range in England (filled dots), with the transition zone traced for the first time based on the criteria in Figure 2. The regression line (y = –0.062x + 7.296; F = 22.28, df = 9, r2 = 0.712, P = 0.0011) ignores the extreme (stunted) outlier population (thick-outlined open square; Olsztyn pond, Poland; Copp et al., 2016).
However, the observed shift reported by Jaćimović et al. (2019) between 2011 and 2012 was a slight (8 mm) increase in juvenile growth (mean TL at age 3) and a decrease in mean age-at-maturity of 0.43 years (Figure 5A). A similar chronology in a pumpkinseed population’s life-history traits was observed in England (Figure 3B), where pumpkinseed washed out from an upstream (former) mill reservoir during an extreme flood event in 2007 gained entry to a garden pond located within the stream’s flood plain (Fobert et al., 2013). Relative to the source population (thick-lined open square in Figure 3B), the new garden pond population demonstrated an increase in juvenile growth (mean = 7 mm TL) by 2010 with a corresponding decrease in mean age-at-maturity of 0.83 years (0.3 years per year). Then, after 6 years in the pond, juvenile growth had increased another 7 mm TL and mean age-at-maturity decreased 1.2 years (0.2 years per annum). This is half the annual rate of shift in the life-history traits of the River Sava black bullhead population, which could be interpreted to suggest that an established fish population responds faster to a sudden availability in resources in its existing habitat (due to a sharp drop in population density) than does a species that has just entered a new water body. For example, two native pond populations of pumpkinseed populations subjected to major winterkills (in Ontario, Canada) matured earlier and at smaller size (due to continued high mortality rates) than in years without winterkills (Fox and Keast, 1990, 1991). As such, one might expect that the removal of pumpkinseed from Meeuwven Pond (Netherlands) during sampling in 2006 (Cucherousset et al., 2009) would have led to an increase in juvenile growth and decrease in mean age-at-maturity in that population when re-sampled in 2009 (Van Kleef and Jongejans, 2014). But, over that 3-year interval, juvenile growth hardly changed (an increase of 1 mm TL) and mean age-at-maturity increased by almost a year (data points 44 and 51 in Figure 4, respectively). Experimental work with a stocked predator (northern pike Esox lucius Linnaeus, 1758) reported that pumpkinseed achieve maturity earlier and at larger size in the presence of the predator than in its absence (Beaune et al., 2019).
The application of the Copp and Fox (2007) model to crucian carp (Tarkan et al., 2016) differs from those described here (Figures 4, 5A) in that data from native continental European populations were included in the plot of non-native crucian populations in England (Figure 5B). At the time that Fig. 2 in Tarkan et al. (2016) was plotted, crucian carp were assumed to be native to England (reviewed in Copp and Sayer, 2020), though this was subsequently refuted by genetic evidence (Jeffries et al., 2017). As such, the initial plot of this model lacked delineation of a transition zone (Fig. 2 in Tarkan et al., 2016), which we have plotted here (Figure 5B) following the procedure described here above for pumpkinseed (Figure 3). Similar to pumpkinseed (Figure 3), female crucian carp in the majority of studied populations achieved maturity by age 2, and the relationship reveals a single population in England to be demonstrating the fast juvenile growth and precocious maturity that could be referred to as invasive (Figure 5B). The remaining English and native range populations (Finland and Russia) were spread across the statistically significant regression line except for one outlier (a stunted pond population at Olsztyn, Poland). Nevertheless, the relationship revealed that crucian carp populations respond well, both in terms of growth and reproduction, to the favourable environmental conditions in England. Despite the crucian carp’s non-native status in Great Britain, and in view of its decline in its native range, England is considered ideal for promoting the species’ conservation (Tarkan et al., 2016; Copp and Sayer, 2020), with at worst moderate risk to native species and ecosystems (Dodd et al., 2019).
The maturity–growth relationships in both black bullhead and crucian carp were statistically significant despite the relatively low number of sample populations (Figure 5). Adequate density estimates are not available with which to carry out tests to validate either of these two models with the same level of confidence as for pumpkinseed, but the statistical nature of this relationship suggests that both of these models offer a useful starting point for future application and testing once more populations and better density data are available for a priori classification of each population with regard its invasiveness status. That said, a key issue we have to address is “what does the relationship predict?” In the underlying relationship, mean age-at-maturity depends on, and therefore can be predicted from, juvenile growth, which is a population’s response to available resources. As previously mentioned, resource availability could potentially be greater following a sharp drop in population density, either disease or intensive predation (including removal by humans), or when introduced (human-assisted or natural dispersal) into a novel water body. The same population response is observed in the native and introduced ranges (Figure 1), which suggests that a native population of fish could be referred to as “invasive” in the same way that native terrestrial weeds are referred to as “weedy” (Copp et al., 2005). Regardless, these applications of the growth–maturity relationship as predictive models of invasiveness, can provide a heuristic means of predicting invasiveness even if their predictive power is not yet statistically significant.
In summary, our life-history model has been shown to apply to three fishes that are successful invaders in some parts, if not all, of Europe and in the case of pumpkinseed also in the extreme western extent of Asia (Ağdamar et al., 2015). However, the application of the model to predict invasion potential of pumpkinseed was only weakly supported when tested against the a priori invasiveness classification of the various populations. That said, the tests were hampered by lack of knowledge of the actual source populations for these European introductions, as well as shortcomings in the dataset that affected our classification of invasiveness. We conclude that such life-history models could potentially provide a useful means for predicting the invasiveness status of non-native freshwater fishes, though the basis of the predictions, statistical or heuristic, will depend on the size and quality of the underlying life-history database.
Author Contributions
GHC and MGF conceived the manuscript structure and content, and shared equally composition of the text. GHC prepared the draft Figures and supplementary information documents using information provided by GHC and MGF. Both authors contributed to the article and approved the submitted version.
Conflict of Interest
The authors declare that the research was conducted in the absence of any commercial or financial relationships that could be construed as a potential conflict of interest.
The handling editor declared a past co-authorship with one of the authors GHC.
Acknowledgments
This article represents a summary of research conceived within the framework of an international network initiated in 2003 as part of a NATO Science Programme “Collaborative Linkage Grant” No. LST.CLG.979499 (awarded to GHC and V. Kováč) entitled “Life-history traits as predictors in assessing risks of non-native fishes.” In this present work, GHC was supported by Cefas’ Science Excellence fund. We thank the reviewers for useful comments to the manuscript.
Supplementary Material
The Supplementary Material for this article can be found online at: https://www.frontiersin.org/articles/10.3389/fevo.2020.605287/full#supplementary-material
References
Ağdamar, S., Tarkan, A. S., Keskin, E., Top, N., Doğaç, E., and Baysal, Ö, et al. (2015). The role of environmental factors and genetic diversity on colonization success of a non-native fish, Lepomis gibbosus from western part of Turkey. Biochem. Syst. Ecol. 58, 195–203. doi: 10.1016/j.bse.2014.12.001
Almeida, D., Almodóvar, A., Nicola, G. G., and Elvira, B. (2009). Feeding tactics and body condition of two introduced populations of pumpkinseed Lepomis gibbosus: taking advantages of human disturbances? Ecol. Freshwat. Fish 18, 15–23. doi: 10.1111/j.1600-0633.2008.00317.x
Almeida, D., and Grossman, G. D. (2014). Regulated small rivers as ‘nursery’areas for invasive largemouth bass Micropterus salmoides in Iberian waters. Aquat. Conserv. Mar. Freshwat. Ecosys. 24, 805–817. doi: 10.1002/aqc.2425
Beaune, D., Castelnau, F., Sellier, Y., and Cucherousset, J. (2019). Native top-predator cannot eradicate an invasive fish from small pond ecosystems. J. Nat. Conserv. 50:125713. doi: 10.1016/j.jnc.2019.125713
Constantinescu, V. (1981). Relationship of total length, body length, weight with standard length in Lepomis gibbosus from Fundata Lake (Romania). Trav. Mus. Hist. Nat. Grigore Antipa 23, 213–223.
Copp, G. H., and Fox, M. G. (2007). “Growth and life history traits of introduced pumpkinseed (Lepomis gibbosus) in Europe, and the relevance to invasiveness potential,” in Freshwater Bioinvaders: Profiles, Distribution, and Threats, ed. F. Gherardi (Berlin: Springer), 289–306. doi: 10.1007/978-1-4020-6029-8_15
Copp, G. H., and Sayer, C. D. (2020). Demonstrating the practical impact of publications in Aquatic Conservation – the case of crucian carp Carassius carassius in the East of England. Aquat. Conserv. Mar. Freshwat. Ecosys. 2020:3353. doi: 10.1002/aqc.3353
Copp, G. H., Bianco, P. G., Bogutskaya, N., Erős, T., Falka, I., Ferreira, M. T., et al. (2005). To be, or not to be, a non-native freshwater fish? J. Appl. Ichthyol. 21, 242–262.
Copp, G. H., Britton, J. R., Wesley, K. J., and Davison, P. I. (2017). Non-native fish dispersal as a contaminant of aquatic plant consignments – a case study from England. Manag. Biol. Invas. 8, 437–442. doi: 10.3391/mbi.2017.8.3.17
Copp, G. H., Fox, M. G., and Kováč, V. (2002). Growth, morphology and life history traits of a coolwater European population of pumpkinseed Lepomis gibbosus. Arch. Hydrobiol. 155, 585–614. doi: 10.1127/archiv-hydrobiol/155/2002/585
Copp, G. H., Stakėnas, S., and Cucherousset, J. (2010). “Aliens vs. the natives: interactions between introduced Lepomis gibbosus and indigenous Salmo trutta in small streams of southern England,” in Community Ecology of Stream Fishes: Concepts, Approaches and Techniques, eds K. B. Gido and D. Jackson (Bethesda: American Fisheries Society), 347–370.
Copp, G. H., Tarkan, A. S., Masson, G., Godard, M. J., Koščo, J., Novomeská, A., et al. (2016). A review of growth and life-history traits of native and non-native European populations of black bullhead Ameiurus melas. Rev. Fish Biol. Fish 26, 441–469. doi: 10.1007/s11160-016-9436-z
Copp, G. H., Templeton, M., and Gozlan, R. E. (2007). Propagule pressure and the invasion risks of non-native freshwater fishes in Europe: a case study of England. J. Fish Biol. 71(Suppl. D), 148–159. doi: 10.1111/j.1095-8649.2007.01680.x
Cucherousset, J., Copp, G. H., Fox, M. G., Sterud, E., Van Kleef, H. H., Verreycken, H., et al. (2009). Life-history traits and potential invasiveness of introduced pumpkinseed Lepomis gibbosus populations in northwestern Europe. Biol. Invas. 11, 2171–2180. doi: 10.1007/s10530-009-9493-5
De Miguel, R. J., Oliva-Paterna, F. J., Gálvez-Bravo, L., and Fernández-Delgado, C. (2014). Fish composition in the Guadiamar River basin after one of the worst mining spills in Europe. Limnetica 33, 375–384. doi: 10.23818/limn.33.29
Dodd, J. A., Vilizzi, L., Bean, C. W., Davison, P. I., and Copp, G. H. (2019). At what spatial scale should risk screenings of translocated freshwater fishes be undertaken – river basin district or climo-geographic designation? Biol. Conserv. 230, 122–130. doi: 10.1016/j.biocon.2018.12.002
Duggan, I. C., Rixon, C. A. M., and MacIsaac, H. J. (2006). Popularity and propagule pressure: determinants of introduction and establishment of aquarium fish. Biol. Invas. 8, 393–398. doi: 10.1007/s10530-004-2310-2
Fletcher, D. H., Gillingham, P. K., Britton, J. R., Blanchet, S., and Gozlan, R. E. (2016). Predicting global invasion risks: a management tool to prevent future introductions. Sci. Rep. 6:26316. doi: 10.1038/srep26316
Fobert, E., Zięba, G., Vilizzi, L., Godard, M. J., Fox, M. G., Stakėnas, S., et al. (2013). Predicting non-native fish dispersal under conditions of climate change: case study in England of dispersal and establishment of pumpkinseed Lepomis gibbosus in a floodplain pond. Ecol. Freshwat. Fish 22, 106–116. doi: 10.1111/eff.12008
Fox, M. G. (1994). Growth, density, and interspecific influences on pumpkinseed sunfish life histories. Ecology 75, 1157–1171. doi: 10.2307/1939439
Fox, M. G., and Copp, G. H. (2014). Old world vs new world – life history alterations in a successful invader introduced across Europe. Oecologia 174, 435–446. doi: 10.1007/s00442-013-2776-7
Fox, M. G., and Keast, A. (1990). Effects of winterkill on population structure, body size, and prey consumption patterns of pumpkinseed in isolated beaver ponds. Can. J. Zool. 68, 2489–2498. doi: 10.1139/z90-348
Fox, M. G., and Keast, A. (1991). Effect of overwinter mortality on reproductive life history characteristics of pumpkinseed (Lepomis gibbosus) populations. Can. J. Fish. Aquat. Sci. 48, 1792–1799. doi: 10.1139/f91-211
Fox, M. G., Vila-Gispert, A., and Copp, G. H. (2007). Life history traits of introduced Iberian pumpkinseed (Lepomis gibbosus) relative to native populations: can differences explain colonization success? J. Fish Biol. 71 (Suppl. D), 56–69. doi: 10.1111/j.1095-8649.2007.01683.x
Fox, M. G., Villeneuve, F., and Copp, G. H. (2011). Seasonal reproductive allocation, local-scale variation and environmental influences on life history traits of introduced pumpkinseed (Lepomis gibbosus) in Southern England. Fund. Appl. Limnol. 178, 231–243. doi: 10.1127/1863-9135/2011/0178-0231
García-Berthou, E. (2007). The characteristics of invasive fish: what have we learned so far? J. Fish Biol. 71 (Suppl. D), 33–55. doi: 10.1111/j.1095-8649.2007.01668.x
Gutiérrez-Estrada, J. C., Pulido-Calvo, I., and Fernádez-Delgado, C. G. (2000a). Age-structure, growth and reproduction of the introduced pumpkinseed (Lepomis gibbosus, L. 1758) in a tribuary of the Guadalquivir River (Southern Spain). Limnetica 19, 21–29.
Gutiérrez-Estrada, J. C., Pulido-Calvo, I., and Prenda, J. (2000b). Gonadosomatic index estimates of an introduced pumpkinseed (Lepomis gibbosus) population in a Mediterranean stream, using computational neural networks. Aquat. Sci. 62, 350–363. doi: 10.1007/PL00001340
Gutowsky, L. F. G., and Fox, M. G. (2012). Intra-population variability of life-history traits and growth during range expansion of the invasive round goby, Neogobius melanostomus. Fish. Manage. Ecol. 19, 78–88. doi: 10.1111/j.1365-2400.2011.00831.x
Hutchings, J. A., Swain, D. P., Rowe, S., Eddington, J. D., Puvanendran, V., and Brown, J. A. (2007). Genetic variation in life-history reaction norms in a marine fish. Proc. Royal Soc. B 274, 1693–1699. doi: 10.1098/rspb.2007.0263
Jaćimović, M., Lenhardt, M., Krpo-Ćetković, J., Jarić, I., Gačić, Z., and Hegediš, A. (2019). Boom−bust like dynamics of invasive black bullhead (Ameiurus melas) in Lake Sava (Serbia). Fish. Manag. Ecol. 26, 153–164. doi: 10.1111/fme.12335
Jeffries, D. L., Copp, G. H., Lawson-Handley, L. J., Sayer, C. D., and Hänfling, B. (2017). Genetic evidence challenges the native status of a threatened freshwater fish (Carassius carassius) in England. Ecol. Evol. 7, 2871–2882. doi: 10.1002/ece3.2831)
Klaar, M., Copp, G. H., and Horsfield, R. (2004). Autumnal habitat use of non-native pumpkinseed Lepomis gibbosus and associations with native fish species in small English streams. Folia Zool. 53, 189–202.
Kohler, C. C., and Stanley, J. G. (1984). “A suggested protocol for evaluating proposed exotic fish introductions in the United States,” in Distribution, Biology and Management of Exotic Fishes, eds W. R. Courtney Jr. and J. R. Strauffer Jr. (London: Johns Hopkins University Press), 387–406.
Kolar, C. S., and Lodge, D. M. (2001). Progress in invasion biology: predicting invaders. Trends Ecol. Evol. 16, 199–204. doi: 10.1016/S0169-5347(01)02101-2
Li, S., Wei, H., Vilizzi, L., Zhan, A., Olden, J. D., Preston, D. L., et al. (2020). The future of legislation, policy, risk analysis, and management of non-native freshwater fishes in China. Rev. Fish. Sci. Aquacul. 2020, 1–18. doi: 10.1080/23308249.2020.1782830
MacArthur, R. H., and Wilson, E. O. (1967). Theory of Island Biogeography. Princeton, NJ: Princeton University Press, 203.
Masson, G., Valente, E., Fox, M. G., and Copp, G. H. (2015). Thermal influences on life-history traits and reproductive effort of introduced pumpkinseed sunfish Lepomis gibbosus in the River Moselle basin (northeastern France). River Res. Appl. 31, 563–575. doi: 10.1002/rra.2761
Masson, L., Brownscombe, J. W., and Fox, M. G. (2016). Fine scale spatio-temporal life history shifts in an invasive species at its expansion front. Biol. Invas. 18, 775–792. doi: 10.1007/s10530-015-1047-4
Prenda, J., Clavero, M., Blanco-Garrido, F., and Hermoso, V. (2006). Threats to the conservation of biotic integrity in Iberian fluvial ecosystems. Limnetica 25, 377–388. doi: 10.23818/limn.25.26
Ribeiro, F., and Collares-Pereira, M. J. (2010). Life-history variability of non-native centrarchids in regulated river systems of the lower River Guadiana drainage (south-west Iberian Peninsula). J. Fish Biol. 76, 522–537. doi: 10.1111/j.1095-8649.2009.02506.x
Ricciardi, A. (2007). “Predicting the number of ecologically harmful invaders in an aquatic system. Oral communication and abstract,” in Proceedings of the 15th Internati. Conf. on Aquat. Invasive Species (ICAIS) Sept. 2007, Nijmegen. 23–26.
Ricciardi, A., and Kipp, R. (2008). Predicting the number of ecologically harmful exotic species in an aquatic system. Divers. Distrib. 14, 374–380. doi: 10.1111/j.1472-4642.2007.00451.x
Rooke, A. C., and Fox, M. G. (2018). A common environment experiment reveals plastic and genetic contributions to the fast life−history strategy of an invasive fish. Ecol. Freshwat. Fish 27, 952–962. doi: 10.1111/eff.12406
Simberloff, D. (2009). The role of propagule pressure in biological invasions. Ann. Rev. Ecol. Evol. Syst. 40, 81–102. doi: 10.1146/annurev.ecolsys.110308.120304
Stearns, S. C., and Koella, J. C. (1986). The evolution of phenotypic plasticity in life-history traits: predictions of reaction norms for age and size at maturity. Evolution 40, 893–913. doi: 10.2307/2408752
Tarkan, A. S., Almeida, D., Godard, M. J., Gaygusuz, Ö, Rylands, M. S., Sayer, C. D., et al. (2016). A review and meta-analysis of growth and life-history traits of a declining European freshwater fish, crucian carp Carassius carassius. Aquat. Conserv. Mar. Freshwat. Ecosys. 26, 212–224. doi: 10.1002/aqc.2580
Van Kleef, H. H., and Jongejans, E. (2014). Identifying drivers of pumpkinseed invasiveness using population models. Aquat. Invas. 9, 315–326. doi: 10.3391/ai.2014.9.3.07
Vila-Gispert, A., García-Berthou, E., and Moreno-Amich, R. (2002). Fish zonation in a Mediterranean stream: effects of human disturbances. Aquat. Sci. 64, 163–170. doi: 10.1007/s00027-002-8064-y
Vilizzi, L., Copp, G. H., Adamovich, B., Almeida, D., Chan, J., Davison, P. I., et al. (2019). A global review and meta-analysis of applications of the Fish Invasiveness Screening Kit. Rev. Fish Biol. Fish 29, 529–568.
Vilizzi, L., Tarkan, A. S., and Copp, G. H. (2015). Experimental evidence from causal criteria analysis for the effects of common carp Cyprinus carpio on freshwater ecosystems: a global perspective. Rev. Fish Sci. Aquacul. 23, 253–290. doi: 10.1080/23308249.2015.1051214
Villeneuve, F., Copp, G. H., Fox, M. G., and Stakėnas, S. (2005). Interpopulation variation in the growth and life history traits of the introduced sunfish, pumpkinseed Lepomis gibbosus, in Southern England. J. Appl. Ichthyol. 21, 275–281. doi: 10.1111/j.1439-0426.2005.00679.x
Williams, S. L., and Grosholz, E. D. (2008). The invasive species challenge in estuarine and coastal environments: marrying management and science. Estuar. Coasts 31, 3–20. doi: 10.1007/s12237-007-9031-6
Yavno, S., Gobin, G., Wilson, C. C., Vila-Gispert, A., Copp, G. H., and Fox, M. G. (2020). New and old world phylogeography of pumpkinseed, Lepomis gibbosus (Linnaeus, 1758): the North American origin of introduced populations in Europe. Hydrobiologia 847, 345–364. doi: 10.1007/s10750-019-04095-y
Keywords: age-at-maturity, juvenile growth, non-native species, risk analysis, horizon scanning, alien species, predictive modelling
Citation: Copp GH and Fox MG (2020) Can Invasiveness in Freshwater Fishes Be Predicted From Life-History Traits? Front. Ecol. Evol. 8:605287. doi: 10.3389/fevo.2020.605287
Received: 11 September 2020; Accepted: 29 October 2020;
Published: 23 December 2020.
Edited by:
Ali Serhan Tarkan, Muğla University, TurkeyReviewed by:
Phillip Joschka Haubrock, Senckenberg Research Institute and Natural History Museum Frankfurt, GermanySean Marr, South African Institute for Aquatic Biodiversity, South Africa
Copyright © 2020 Copp and Fox. This is an open-access article distributed under the terms of the Creative Commons Attribution License (CC BY). The use, distribution or reproduction in other forums is permitted, provided the original author(s) and the copyright owner(s) are credited and that the original publication in this journal is cited, in accordance with accepted academic practice. No use, distribution or reproduction is permitted which does not comply with these terms.
*Correspondence: Gordon H. Copp, gordon.copp@cefas.co.uk