- Department of Entomology and Nematology, Fort Lauderdale Research and Education Center, University of Florida, Fort Lauderdale, FL, United States
Hybridization of two different species is an important mechanism to have gene flows between species. Recently, mating of two economically important invasive species of subterranean termites (Coptotermes formosanus and Coptotermes gestroi) have been observed in the field and hybrids colonies have been established in the laboratory. It was previously reported that incipient colonies (~1 year old) of hybrid Coptotermes species contained more termites than colonies of parental species, showing hybrid vigor. In this study, colony vigor and individual termite vigor were investigated in juvenile colonies (~2 year old), using colony growth parameters and the movement activity of individual termites as proxies for the evaluation of hybrid vigor beyond the initial colony foundation. After 2 years from colony foundations, hybrid colonies showed no more hybrid vigor. In addition, movement activity of termites in hybrid colonies was significantly slower than in termites from conspecific colonies. It is suggested that a reduction in the molting rates of individuals in hybrid colonies may have a negative impact on their physiology and their movement activity. These possible changes in physiology may affect the movement of individuals, and accumulation of these inefficient termites in hybrid colonies may contribute to the loss of hybrid vigor at 2 years of age in hybrid colonies.
Introduction
Sexual reproduction between two different species or relatively distant lineages can potentially allow for hybridization events, where it was estimated that up to 25% of plant species and 10% of animal species may have the ability to hybridize (Mallet, 2005, 2007). Hybridization events may result in various outcomes, such as gene flow between species, individuals with increase genetic heterogeneity, which sometimes results in evolutionary novelties and hybrid vigor (Rhymer and Simberloff, 1996; Seehausen, 2004; Mallet, 2005, 2007; Seehausen et al., 2008; Abbott et al., 2013). However, hybridization often results in low hybrid fitness, which primarily result from low fertility, low vigor, non-adapted traits or genetic incompatibilities. Although hybridization events have been reported in various insect groups, hybridization in social insects such as ants, bees, wasps and termites can provide unique opportunities to study the phenotypic consequences of novel genetic combinations from the individual to the colony level (Feldhaar et al., 2008).
Hybridization in social insects has primarily been documented in haplodiploid hymenopterans, such as ants and bees. Consequences of hybridization in these cases have often included negative effects such as lack of viable F1 (Seifert, 1999), infertile individuals (Pearson, 1983; Douwes and Stille, 1991; Umphrey and Danzmann, 1998; Umphrey, 2006) and reduced fertilities in F1 (Plateaux, 1979; Julian et al., 2002; Schwander et al., 2007). In other cases, hybridization resulted in enhanced traits, such as the hybrids of two fire ant species (Solenopsis) that outcompeted native species in disturbed areas (James et al., 2002; Gibbons and Simberloff, 2005). It was also reported that hybridization of honey bees (Apis), Africanized honey bees (hybrids between European and African honey bees) not only displayed intermediate traits of both, but also showed high levels of defensive behavior (Guzmán-Novoa et al., 2002; Alaux et al., 2009), causing significant problems to human society, beekeeping and agriculture (Winston, 1992; Scott Schneider et al., 2004).
In termites, hybridization events have been reported in only a few species, in the genera Coptotermes (Chouvenc et al., 2015; Su et al., 2017), Reticulitermes (Lefebvre et al., 2008), Zootermopsis (Aldrich and Kambhampati, 2009), Pseudacanthotermes (Connétable et al., 2012), and Nasutitermes (Hartke and Rosengaus, 2011). Although termites and social Hymenoptera showed evolutionary convergence, they differ in key biological characteristics as termites are social roaches with a hemimetabolous development, where most individuals retain juvenile form and keep molting to the next larval instar until they differentiate into reproductives or soldiers, excluding workers in derived family Termitidae (Nalepa, 2010, 2011, 2015; Chouvenc and Su, 2014; Roisin, 2016). Colony members that have to periodically engage in molting rely on their nesmates to molt successfully (Xing et al., 2013), and for the re-acquisition of their obligatory gut mutualist (Nalepa, 2015, 2017). Because of the fundamental differences in developmental pathways and the recurrent molting requirement in termites, consequences of hybridization events may differ from those of holometabolous social hymenopterans, where all functional individuals are adults that undergo complete metamorphosis.
Of the 3,000 described termite species, Coptotermes formosanus Shiraki [the Formosan subterranean termite (FST)], and the C. gestroi (Wasmann) [Asian subterranean termite (AST)], are the most destructive structural pests, and both species are successful invaders (Rust and Su, 2012; Evans et al., 2013; Chouvenc et al., 2016). Coptotermes formosanus distributed from subtropics to warm temperate regions, is endemic to southern China and Taiwan, and have invaded Japan and parts of the Southeastern United States (Su, 2003; Chouvenc et al., 2016). However, C. gestroi primarily found in the tropics, is native to southeast Asia and has been introduced to many parts of the neotropics (Evans et al., 2013; Su et al., 2017). These two species are allopatric in their native ranges, and it was estimated that both species have evolved separately 15–20 million years ago (Bourguignon et al., 2016).
As a result of human activities and climate change, FST and AST are now sympatric in three locations: Taiwan, Hawaii and southeastern Florida (Chouvenc et al., 2015; Su et al., 2017). Since 2013, simultaneous dispersal flight events were observed in Florida (Chouvenc et al., 2015, 2017b) and heterospecific mating behavior were observed, as AST males readily initiated tandem with females of FST (Chouvenc et al., 2015). From such pairings, hybrid colonies from the two possible mating combinations (♀AST × ♂FST and ♀FST × ♂AST) were successfully established in the laboratory, showing that there were no pre-zygotic barriers for the production of a viable F1 hybrid brood (Chouvenc et al., 2015, 2017a). Hybrid colonies after 1 year of foundation showed relatively high hybrid vigor by displaying faster colony growth compared to colonies founded with conspecific pairs of FST and AST (Chouvenc et al., 2015). In addition, individuals from hybrid colonies displayed a wider temperature range survivorship than parental species, suggesting that hybrid colonies have the potential to survive in a geographic range combining the tropical, subtropical to temperate region distribution of the two parental species (Patel et al., 2018).
Although heterospecific pairings were observed in the field, it is unknown if such matings can result in successfully hybrid colony establishment in the field, and if such colonies could produce fertile F1 alates. Laboratory colonies therefore allows for the biological characterization of such hybrids. In this study, we investigated the consequences of hybridization compared to parental species after 2 years of colony foundation from the individual to colony level, to determine if the hybrid colony vigor previously observed in 1 year-old colony (Chouvenc et al., 2015) is maintained as colonies grow. To test this hypothesis, we used 2 year-old colonies to measure their biomass (overall colony growth), the movement behavior of individual termites, and the molting rate of workers in the two heterospecific mating combinations (hybrids) and of the two conspecific colonies (parents).
Materials and Methods
Colony Foundations and Rearing
Alates (winged imagoes) of C. formosanus and C. gestroi were collected in Broward County (Florida, USA) during dispersal flight events using a light trap (Chouvenc et al., 2015). Rearing units were comprised of 6 g of organic soil moistened with distilled water at the bottom of a plastic cylindrical vials (8 cm height × 2.5 cm diameter). Four spruce pieces (Picea sp.) were placed on top of the soil and a 3% agar solution (5 cm high) was poured in. A small vertical hole was made through the agar using a piece (8 × 0.5 × 0.5 cm) of wood to serve as royal chamber for the mated pair. After the agar solidified, a male and a female of each species were introduced into each rearing unit, then covered with its lid, which pierced with a safety pin to have four tiny holes to allow air exchange. Four mating combinations were prepared: hybrid_F (HF; ♀C. formosanus × ♂C. gestroi), hybrid_G (HG; ♀C. gestroi × ♂C. formosanus), C. formosanus (FST; ♀C. formosanus × ♂C. formosanus), and C. gestroi (AST; ♀C. gestroi × ♂C. gestroi). After 1 year, colonies were transferred to ~1.5 L plastic boxes (17 × 12 × 7 cm) containing moist organic soil and wood pieces. Distilled water and new wood pieces were provisioned periodically. All rearing units and experiments were carried out at 28 ± 1°C and 80 ± 3% RH.
Experiment 1: Colony Census
Two year-old colonies of the four different mating types (i.e., HF, HG, FST, and AST) were processed to measure total biomass of the colony and each castes as a proxy variable for overall colony growth. All colonies had well-developed carton material and had the presence of larvae and younger worker instars, confirming reproductive activity. To collect all termites from the container, the wood, the carton and debris were progressively removed. In the process, termites were collected using aspirators, however, when termites were mixed with nest material, the sorting process of Tamashiro et al. (1973) was used. This procedure was repeated multiple times until all termites were collected in the colonies and separated from nest materials.
Total biomass of the colony and body mass of queen, king, 100 workers, and 20 soldiers were measured from three colonies of each mating combination. For each colony, 100 workers and 20 soldiers were randomly collected three times for measurements. Mass of 100 workers and 20 soldiers were then divided by their numbers to measure individuals mass. Statistical differences of the variables were tested with a permutation analysis of variance (ANOVA) at α = 0.05 with mating combinations as a factor, and pairwise comparisons were applied for post-hoc analysis. In test, number of permutation was 9,999.
Experiment 2: Individual Behavior Assay
Artificial tunnels within a planar arena were used to observe termite movement behavior (Figure 1). The bottom and top layers were made of transparent plexiglass (9 × 16 cm, 5 mm in height), separated by gray middle layer made of plexiglass (9 × 16 cm, 2 mm in height). The surface of the bottom layer was sanded to provide traction for termite movement (Roughness 60, CAMI grit designation) and tunnels were cut out of the middle layer. Releasing chambers were provided at both ends of the arena on the top layer. The tunnel widths were 3 mm, and the distance between releasing chambers was 5 cm.
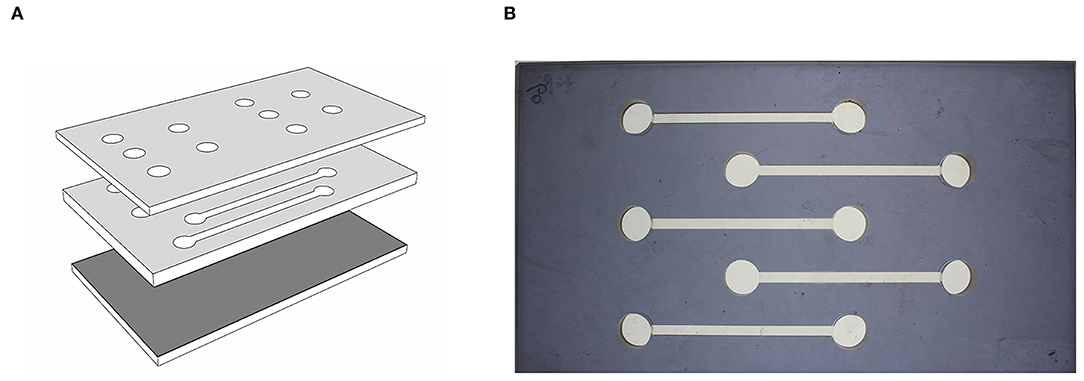
Figure 1. Experimental arena used in behavioral assay. (A) The planar arena composed of three layers (top, middle and bottom layer). The top layer contain introduction hole, middle layer produce tunnel and bottom layer. (B) Top view of the planar arena.
Workers (undifferentiated larval of the 4th instar) of similar body size were randomly selected from each mating combination (HF, HG, FST, and AST). For each assay, a termite was introduced into each tunnel, such that five workers were tested simultaneously (Figure 1). Termites were introduced into the releasing chamber on the left side for consistency across the experiment, covered with a transparent coverslip, and were left for 10 min for acclimatization before recording. A digital camcorder (SONY CX-700, Tokyo, Japan) was mounted on top of the arena for 10 min video recording. At the end of each experiment, Arenas were dismantled and washed with detergents, 95% ethanol and distilled water three times to prevent confounding effects of potential trail-following pheromones. Fifteen workers from each colony and three different colonies of origin were tested for each mating combination, for a total of 45 workers in each of HF, HG, FST, and AST mating combinations.
Videos were analyzed using a processing software, virtualdub (http://www.virtualdub.org/) and two variables were obtained. “Passing time” was defined as the time taken by a termite to move through the entire tunnel length, from one chamber to the other. “Stop time” was defined as the time taken by a termite from the moment it entered one of the chamber and the moment it left the chamber. Passing times and stop times of each termite were measured for 10 min, and all of its instances of passing time and stop time were averaged, yielding a single value for passing time and stop time, respectively, for each termite. The passing time and stop time (variables) were compared among mating combinations using a permutation ANOVA and pairwise comparison as post-hoc at α = 0.05. In the permutation ANOVA, the number of permutation was 9,999. In addition, rank distribution of passing and stop times were subjected to curve fitting with linear, logarithmic, power and exponential curves.
Experiment 3: Molting Ratio Experiment
Whole colonies (two-years-old) of HF, HG, FST and AST were processed and termites were placed in plastic boxes (17.5 × 12.5 × 7 cm3) containing moistened sand (depth: 0.5 cm) at the bottom. Following the method of Raina et al. (2008) and modified by Xing et al. (2013) to identify termites in pre-molt and/or pre-ecdysis period, three cellulose filter papers (diameter: 9 cm) stained with Nile Blue A (0.1% wt:wt) were used as a dietary marker. Termites in the pre-molt and/or pre-ecdysis period stop feeding to void their guts prior to ecdysis and will not be stained. After 5 days, numbers of white termites and blue termites in the colonies were counted to calculate the percentages of termites, defined by number of white workers divided by total number of workers, in both pre-molt and pre-ecdysis stages. Also, mortality during the treatment of Nile Blue A was measured. In experiment 3, 3 different colonies of HF, HG, FST and AST were tested. Data were arcsine transformed and subjected to an one-way ANOVA (α = 0.05) with mating combination as a factor, followed by Tukey's HSD for post-hoc analysis. All statistical analyses were carried out using PAST version 3 (Hammer et al., 2001) and IBM SPSS version 20 (IBM Corp.).
Results
Experiment 1. Colony Census
We found no significant difference in total biomass (F = 2.176, df = 3,8, p = 0.171, body mass of queen (F = 0.928, df = 3,8, p = 0.489), and of king (F = 2.073, df = 3,8, p = 0.170) (Table 1). Statistical differences were detected in body mass of workers (F = 6.228, df = 3, 8, p = 0.026) and of soldiers (F = 5.707, df = 3,8, p = 0.014), revealing some degree of variability of biological traits among mating combinations (Table 1). FST workers displayed significantly higher individual body weight than AST, but neither hybrids (HF and HG) was different from FST nor AST, presented as intermediate trait. The average individual mass of soldiers was highest in HG and lowest in AST.
Experiment 2: Individual Behavior Assay
In individual movement bioassays, both FST and AST workers displayed significantly faster passing time (F = 14.916, df = 3,176, p < 0.001) than hybrid workers HF and HG (Figure 2A). In addition, HF and HG workers had statistically longer stop time than AST workers (F = 6.019, df = 3,176, p < 0.001), with FST being intermediate.
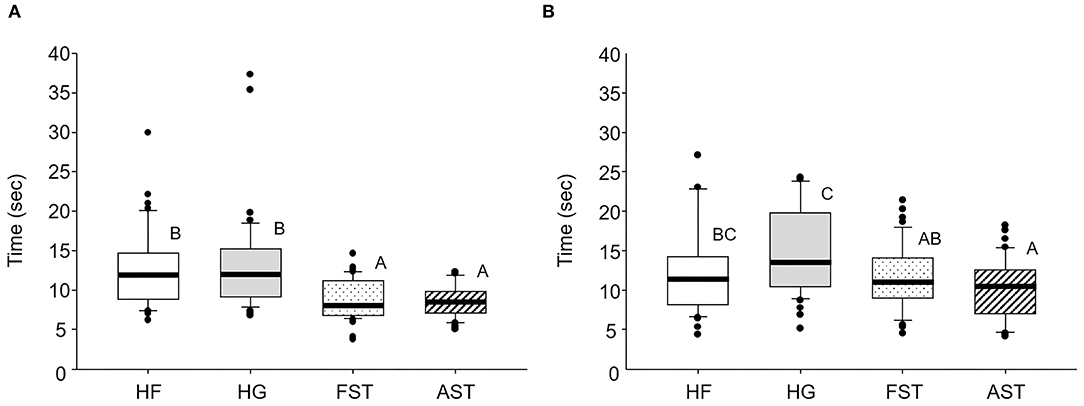
Figure 2. Box plots of movement activities of 2 hybrids and conspecific colonies. (A) Passing time and (B) stop time. Different upper caste letters indicates statistical difference according to Tukey's HSD (α = 0.05). Line on the box represents mean and circles above and below box indicate outliers. HF (♀ AST × ♂ FST), HG (♀ FST × ♂ AST), FST (♀ FST × ♂ FST), and AST (♀ AST × ♂ AST). FST, Formosan subterranean termites; Coptotermes formosanus Shiraki; AST, Asian subterranean termites; C. gestroi (Wasmann). In each colony, 15 termites were tested and 3 different colonies were used (N: 15 × 3 = 45).
Exponential curve had the best fit for rank distribution of passing time for both hybrid species (HF: R2 = 0.965, F = 1,189.828, p < 0.001; HG: R2 = 0.885, F = 332.139, p < 0.001), whereas linear curves were the best fit for both FST and AST (FST: R2 = 0.955, F = 902.215, p < 0.001; AST: R2 = 0.983, F = 2421.442, p < 0.001) (Figure 3A). Such observation revealed that hybrid mating combination were slower on average than parental species and there was the presence of several outliers within the workers of both hybrid mating combinations with extremely slow individuals. In addition, similar patterns were observed in stop time that exponential curves showed the highest R2 value among tested functions for both hybrids (HF: R2 = 0.875, F = 300.007, p < 0.001; HG: R2 = 0.932, F = 586.752, p < 0.001), however, rank distribution of both FST and AST were fitted onto linear curves (FST: R2 = 0.957, F = 952.564, p < 0.001; AST: R2 = 0.968, F = 1321.236, p < 0.001) (Figure 3B), suggesting that hybrids species spend more time for stop.
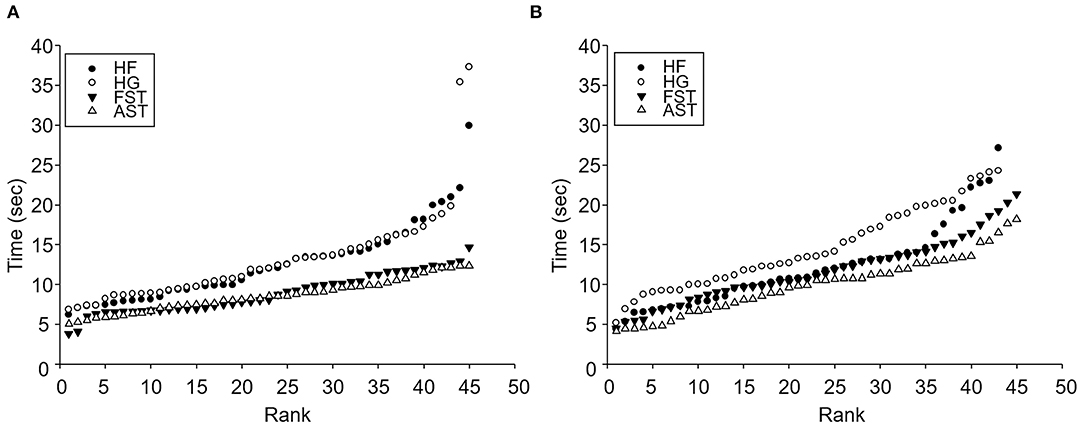
Figure 3. Rank distribution of movement activities of 2 hybrids and conspecific colonies. (A) Passing time and (B) Stop time. Black circles, blank circles, black triangles, and blank triangles are HF, HG, FST, and AST. HF (♀ AST × ♂ FST), HG (♀ FST × ♂ AST), FST (♀ FST × ♂ FST), and AST (♀ AST × ♂ AST). FST, Formosan subterranean termites; Coptotermes formosanus Shiraki; AST, Asian subterranean termites; C. gestroi (Wasmann).
Experiment 3: Molting Ratio Experiment
The mortality during Nile Blue A treatments was not significantly differed across mating types (F = 1.171, df =3,8, p = 0.379) and it was from 5 to 9% in 5 days. The percentages of termites in pre-molt fasting stage, right before molting, was significantly higher in FST and AST, 11.97 ± 1.98 and 10.46 ± 3.43%, respectively, than those of HF and HG 4.31 ± 1.98 and 2.34 ± 0.35%, respectively (F = 10.535, df =3,8, p < 0.01) (Figure 4).
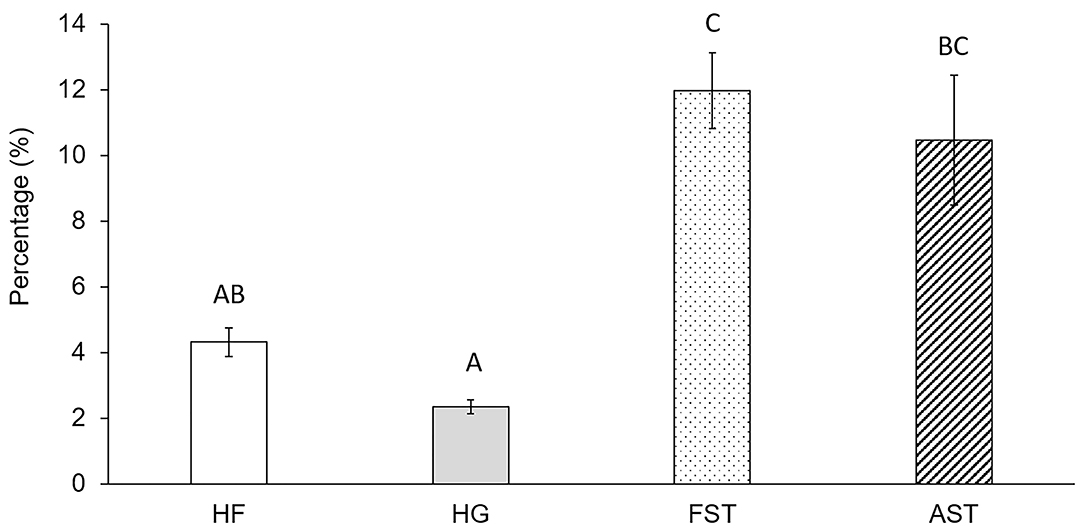
Figure 4. Percentage of termites in pre-molting stage. Different upper case letters represent statistically different group according to Tukey's HSD (α = 0.05). HF (♀ AST × ♂ FST), HG (♀ FST × ♂ AST), FST (♀ FST × ♂ FST), and AST (♀ AST × ♂ AST). FST, Formosan subterranean termites; Coptotermes formosanus Shiraki; AST, Asian subterranean termites; C. gestroi (Wasmann). For each mating types, 3 different colonies were used.
Discussion
Hybrid Coptotermes displayed faster growth during the 1st year after colony foundations than parent colonies (Chouvenc et al., 2015), but current study revealed that by year two, such differences in colony growth were no longer observed, and all mating combinations displayed similar colony size. Workers and soldiers in hybrid combination were approximately intermediate in body size and cuticular hydrocarbon profiles when compared to those of the parental species (Chouvenc and Su, 2017). These observations confirm that hybrid termites can display some intermediate phenotypic traits of their parental species.
We initially expected to obtain hybrid colonies with even larger colonies than parental species at 2 years of development. Our current study, however, showed no difference in growth rate between hybrid and parent species, which indicates that something happened in hybrid colonies between 1 and 2-year of colony growth that potentially slowed down their development. The movement assay revealed that hybrid workers were slower than workers of parental species. In addition, hybrid colonies contained a number of outlying individuals that were extremely slow, when compared with workers in parental species. These results therefore suggest that some individuals within hybrid colonies may suffer a reduced mobility, potentially impacting their overall efficiency and reducing their contribution toward the function of the colony, and ultimately reducing the vigor of the colony.
The physiological changes are currently unknown that underlie the slow movement of workers in hybrids colonies. Chouvenc and Su (2014) indicated that during this time frame of colony development, workers progressively have the ability to successfully molt into older instar, which implies an accumulation of older and more efficient workers within the colony. Molting in termite workers is a critical part of their development as their physiology require them to molt regularly (Korb and Hartfelder, 2008; Xing et al., 2013; Kakkar et al., 2016). Roisin (2016) argues that periodical molting in termite workers allow for individual to regularly shed an aging cuticle and dull mandibles, to a new, thicker cuticle and sharp mandibles. The intermolt period in a termite worker must therefore be bound in time and molting should be taking place at an optimal time to keep workers as efficient as possible within their molting cycle. This intermolt period is critical which is about 45 days in C. formosanus (Kakkar et al., 2016) and the molting takes usually 6 days in C. formosanus, as they stop feeding to void their guts around 6 days before ecdysis (Xing et al., 2013).
Our last experiment therefore aimed at investigating the molting rates of workers at the colony level, to determine if hybrid worker would molt more or less often than parental species, which would potentially impact the efficiency of their active intermolt period and their mobility. Daily molting frequencies of C. formosanus are determined to be ~2% of all workers per day at 28°C (Kakkar et al., 2016), and in this study we confirmed such molting frequency in C. formosanus (2%/day), with similar molting frequencies in C. gestroi. However, the molting frequency in both hybrids were drastically lower (0.6%/day). The result implies that the intermolt period in hybrid workers is possibly longer than in parental species, resulting in the accumulation of individuals that have passed their optimal molting time with a reduced efficiency. Consequently, many worker termites in 2-year-old hybrid colonies may progressively lose the efficiency of their contribution to the colony, and potentially become a burden in the colony, ultimately slowing down the growth of the colony.
We here argue that, if hybrid termites of two Coptotermes species display a less than optimal molting cycle, with potential complication in their development and their efficiency, it may be a significant hurdle for a potential establishment of hybrid colonies in the field and further potential gene flow among species. The molting process in termite workers is such a fundamental aspect of their biology (Nalepa, 2011) that a potential dysfunctional gene expression within hybrid termites could result in the accumulation of low vigor individuals, and raise questions about the potential production of functional and fertile alates in hypothetic mature colonies. However, it is challenging to observe the fertility of F1 because it will take at least 5–8 years for a colony to reach maturity and produce winged adults (Chouvenc and Su, 2014).
Unlike social Hymenoptera, termites are diploid social insects, so hybridization will impacts on both females and males. In social Hymenoptera, such as ants, bees and wasps, hybridization can only affect female phenotypes because males develop from an unfertilized egg (Feldhaar et al., 2008). Currently, the effects on females and males in the hybridization of termites are unknown. Nevertheless, hybridization of termites can provide a framework to understand gene flow between males and females to their offspring. Only a few studies have reported hybridization of termites with cases of introgressive hybrids in the genus Reticulitermes (Lefebvre et al., 2008) and Zootermopsis (Aldrich and Kambhampati, 2009). Viable hybrids were observed from pairing Nasutitermes corniger (Motschulsky) and N. ephratae (Holmgren) in the laboratory (Hartke and Rosengaus, 2011). In heterospecific pairs, courtship and nest construction behavior as phenotypes of the colony were similar to conspecific pairs, and survival of heterospecific pairs was determined by females of pairings rather than males (Hartke and Rosengaus, 2011). There was no observable hybrid vigor, since each combination produced offspring either as similar as or less than conspecific pairs 60 days after colony foundation (Hartke and Rosengaus, 2011).
As pointed out by Chouvenc and Su (2017), hybrid colonies can provide unique opportunities and contribute not only to understanding of theoretical and fundamental questions, such as mode of transmission of endosymbiotic communities, but also practical aspects, such as controls against hybridization of two destructive subterranean termites. Future, hybridization between FST and AST is needed to address foraging activities, physiology and genetics.
In conclusion, it is premature to conclude consequences of hybridization of two destructive subterranean termites, since the life-cycle of these species takes more than 5 years. We only investigated 2-year-old hybrids and parental species in order to study consequences of hybrid vigor after the report by Chouvenc et al. (2015). Hybridization has significant effects on both the individual and colony level, and these effects are rather negative in terms of movement behavior and physiology, with no hybrid vigor after 2 years from colony foundation.
Data Availability Statement
The raw data supporting the conclusions of this article will be made available by the authors, without undue reservation.
Author Contributions
S-BL and TC developed the idea and designed experiments. S-BL conducted experiments and the statistical analyses. S-BL and JP collected the data. S-BL, N-YS, and TC interpreted the results and discussed the direction of the manuscript. All authors contributed to the writing and approved the manuscript.
Funding
This work was supported in part by the USDA National Institute of Food and Agriculture, Hatch project number FLA-FTL-005865, and by a NSF-DEB grant agreement No. 1754083.
Conflict of Interest
The authors declare that the research was conducted in the absence of any commercial or financial relationships that could be construed as a potential conflict of interest.
Acknowledgments
We are grateful to Reina Tong (University of Florida) for providing helps in colony processing and review of the first draft. We also thank to two reviewers for constructive comments to improve the previous version of the manuscript.
Abbreviations
AST, Asian subterranean termite; FST, Formosan subterranean termite; HF, Hybrid F (♀C. formosanus × ♂C. gestroi); HG, Hybrid G (♀C. gestroi × ♂C. formosanus).
References
Abbott, R., Albach, D., Ansell, S., Arntzen, J. W., Baird, S. J. E., Bierne, N., et al. (2013). Hybridization and speciation. J. Evol. Biol. 26, 229–246. doi: 10.1111/j.1420-9101.2012.02599.x
Alaux, C., Sinha, S., Hasadsri, L., Hunt, G. J., Guzmán-Novoa, E., DeGrandi-Hoffman, G., et al. (2009). Honey bee aggression supports a link between gene regulation and behavioral evolution. Proc. Natl. Acad. Sci. U.S.A. 106, 15400–15405. doi: 10.1073/pnas.0907043106
Aldrich, B. T., and Kambhampati, S. (2009). Preliminary analysis of a hybrid zone between two subspecies of Zootermopsis nevadensis. Insectes Soc. 56:439. doi: 10.1007/s00040-009-0041-1
Bourguignon, T., Lo, N., Šobotník, J., Sillam-Dussès, D., Roisin, Y., and Evans, T. A. (2016). Oceanic dispersal, vicariance and human introduction shaped the modern distribution of the termites reticulitermes, heterotermes and coptotermes. Proc. R. Soc. B Biol. Sci. 283:20160179. doi: 10.1098/rspb.2016.0179
Chouvenc, T., Helmick, E., and Su, N.-Y. (2015). Hybridization of two major termite invaders as a consequence of human activity. PLoS ONE 10:e0120745. doi: 10.1371/journal.pone.0120745
Chouvenc, T., Li, H., Austin, J., Bordereau, C., Bourguignon, T., Cameron, S. L., et al. (2016). Revisiting coptotermes (Isoptera: Rhinotermitidae): a global taxonomic road map for species validity and distribution of an economically important subterranean termite genus. Syst. Entomol. 41, 299–306. doi: 10.1111/syen.12157
Chouvenc, T., Osorio, S., Chakrabarti, S., Helmick, E., Li, H.-F., and Su, N.-Y. (2017a). Assessment of genetic markers for the determination of Coptotermes formosanus × Coptotermes gestroi (Isoptera: Rhinotermitidae) F1 hybrids. Florida Entomol. 100, 657–659. doi: 10.1653/024.100.0325
Chouvenc, T., Scheffrahn, R. H., Mullins, A. J., and Su, N.-Y. (2017b). Flight phenology of two Coptotermes species (Isoptera: Rhinotermitidae) in Southeastern Florida. J. Econ. Entomol. 110, 1693–1704. doi: 10.1093/jee/tox136
Chouvenc, T., and Su, N.-Y. (2014). Colony age-dependent pathway in caste development of Coptotermes formosanus Shiraki. Insectes Soc. 61, 171–182. doi: 10.1007/s00040-014-0343-9
Chouvenc, T., and Su, N.-Y. (2017). Testing the role of cuticular hydrocarbons on intercolonial agonism in two subterranean termite species (Coptotermes) and their hybrids. Insectes Soc. 64, 347–355. doi: 10.1007/s00040-017-0552-0
Connétable, S., Robert, A., Bordereau, C., Connetable, S., Robert, A., and Bordereau, C. (2012). Dispersal flight and colony development in the fungus-growing termites Pseudacanthotermes spiniger and P. militaris. Insectes Soc. 59, 269–277. doi: 10.1007/s00040-011-0216-4
Douwes, P., and Stille, B. (1991). Hybridization and variation in the Leptothorax tuberum group (Hymenoptera: Formicidae). J. Zool. Syst. Evol. Res. 29, 165–175. doi: 10.1111/j.1439-0469.1991.tb01629.x
Evans, T. A., Forschler, B. T., and Grace, J. K. (2013). Biology of invasive termites: a worldwide review. Annu. Rev. Entomol. 58, 455–474. doi: 10.1146/annurev-ento-120811-153554
Feldhaar, H., Foitzik, S., and Heinze, J. (2008). Lifelong commitment to the wrong partner: hybridization in ants. Philos. Trans. R. Soc. B Biol. Sci. 363, 2891–2899. doi: 10.1098/rstb.2008.0022
Gibbons, L., and Simberloff, D. (2005). Interaction of hybrid imported fire ants (Solenopsis invicta × S. richteri) with native ants at baits in southeastern Tennessee. Southeast. Nat. 4, 303–320. doi: 10.1656/1528-7092(2005)004[0303:IOHIFA]2.0.CO;2
Guzmán-Novoa, E., Hunt, G. J., Uribe, J. L., Smith, C., and Arechavaleta-Velasco, M. E. (2002). Confirmation of QTL effects and evidence of genetic dominance of honeybee defensive behavior: results of colony and individual behavioral assays. Behav. Genet. 32, 95–102. doi: 10.1023/A:1015245605670
Hammer, Ø., Harper, D., and Ryan, P. (2001). PAST: Paleontological statistics software package for education and data analysis. Palaeontol. Electr. 4:9.
Hartke, T. R., and Rosengaus, R. B. (2011). Heterospecific pairing and hybridization between Nasutitermes corniger and N. ephratae. Naturwissenschaften 98:745. doi: 10.1007/s00114-011-0823-y
James, S. S., Pereira, R. M., Vail, K. M., and Ownley, B. H. (2002). Survival of imported fire ant (Hymenoptera: Formicidae) species subjected to freezing and near-freezing temperatures. Environ. Entomol. 31, 127–133. doi: 10.1603/0046-225X-31.1.127
Julian, G. E., Fewell, J. H., Gadau, J., Johnson, R. A., and Larrabee, D. (2002). Genetic determination of the queen caste in an ant hybrid zone. Proc. Natl. Acad. Sci. U.S.A. 99, 8157–8160. doi: 10.1073/pnas.112222099
Kakkar, G., Chouvenc, T., Osbrink, W., and Su, N. Y. (2016). Temporal assessment of molting in workers of Formosan subterranean termites (Isoptera: Rhinotermitidae). J. Econ. Entomol. 109, 2175–2181. doi: 10.1093/jee/tow180
Korb, J., and Hartfelder, K. (2008). Life history and development-a framework for understanding developmental plasticity in lower termites. Biol. Rev. 83, 295–313. doi: 10.1111/j.1469-185X.2008.00044.x
Lefebvre, T., Châline, N., Limousin, D., Dupont, S., and Bagnères, A.-G. (2008). From speciation to introgressive hybridization: the phylogeographic structure of an island subspecies of termite, Reticulitermes lucifugus corsicus. BMC Evol. Biol. 8:38. doi: 10.1186/1471-2148-8-38
Mallet, J. (2005). Hybridization as an invasion of the genome. Trends Ecol. Evol. 20, 229–237. doi: 10.1016/j.tree.2005.02.010
Nalepa, C. A. (2010). Altricial development in wood-feeding cockroaches: the key antecedent of termite eusociality, in Biology of Termites: A Modern Synthesis eds Bignell, D.E., Roisin, Y., and Lo, N., (Dordrecht: Springer), 69–95.
Nalepa, C. A. (2011). Body size and termite evolution. Evol. Biol. 38, 243–257. doi: 10.1007/s11692-011-9121-z
Nalepa, C. A. (2015). Origin of termite eusociality: Trophallaxis integrates the social, nutritional, and microbial environments. Ecol. Entomol. 40, 323–335. doi: 10.1111/een.12197
Nalepa, C. A. (2017). What kills the hindgut flagellates of lower termites during the host molting cycle? Microorganisms 5:82. doi: 10.3390/microorganisms5040082
Patel, J. S., Tong, R. L., Chouvenc, T., and Su, N.-Y. (2018). Comparison of temperature-dependent survivorship and wood-consumption rate among two invasive subterranean termite species (Blattodea: Rhinotermitidae: Coptotermes) and their hybrids. J. Econ. Entomol. 112, 300–304. doi: 10.1093/jee/toy347
Pearson, B. (1983). Hybridisation between the ant species Lasius niger and Lasius alienus: the genetic evidence. Insectes Soc. 30, 402–411. doi: 10.1007/BF02223971
Plateaux, L. (1979). Ovarian polymorphism of queens in Leptothorax ants, interspecific variations-inferiority of interspecific hybrids. Arch. Zool. Exp. Gen. 120, 381–398.
Raina, A., Park, Y. I., and Gelman, D. (2008). Molting in workers of the Formosan subterranean termite Coptotermes formosanus. J. Insect Physiol. 54, 155–161. doi: 10.1016/j.jinsphys.2007.08.015
Rhymer, J. M., and Simberloff, D. (1996). Extinction by hybridization and introgression. Annu. Rev. Ecol. Syst. 27, 83–109. doi: 10.1146/annurev.ecolsys.27.1.83
Roisin, Y. (2016). What makes the cost of brood care important for the evolution of termite sociality? its insignificance. Ecol. Entomol. 41, 31–33. doi: 10.1111/een.12278
Rust, M. K., and Su, N.-Y. (2012). Managing social insects of urban importance. Annu. Rev. Entomol. 57, 355–375. doi: 10.1146/annurev-ento-120710-100634
Schwander, T., Cahan, S. H., and Keller, L. (2007). Characterization and distribution of Pogonomyrmex harvester ant lineages with genetic caste determination. Mol. Ecol. 16, 367–387. doi: 10.1111/j.1365-294X.2006.03124.x
Scott Schneider, S., DeGrandi-Hoffman, G., and Smith, D. R. (2004). The African honey bee: factors contributing to a successful biological invasion. Annu. Rev. Entomol. 49, 351–376. doi: 10.1146/annurev.ento.49.061802.123359
Seehausen, O. (2004). Hybridization and adaptive radiation. Trends Ecol. Evol. 19, 198–207. doi: 10.1016/j.tree.2004.01.003
Seehausen, O. L. E., Takimoto, G., Roy, D., and Jokela, J. (2008). Speciation reversal and biodiversity dynamics with hybridization in changing environments. Mol. Ecol. 17, 30–44. doi: 10.1111/j.1365-294X.2007.03529.x
Seifert, B. (1999). Interspecific hybridisations in natural populations of ants by example of a regional fauna (Hymenoptera, Formicidae). Insectes Soc. 46, 45–52. doi: 10.1007/s000400050111
Su, N.-Y. (2003). Overview of the global distribution and control of the Fornmosan subterranean termite. Sociobiology 41, 7–16.
Su, N.-Y., Chouvenc, T., and Li, H.-F. (2017). Potential hybridization between two invasive termite species, Coptotermes formosanus and C. Gestroi (Isoptera: Rhinotermitidae), and its biological and economic implications. Insects 8:14. doi: 10.3390/insects8010014
Tamashiro, M., Fujii, J. K., and Lai, P-Y. (1973). A simple method to observe, trap, and prepare large numbers of subterranean termites for laboratory and field experiments. Environ. Entomol. 2, 721–722.
Umphrey, G. J. (2006). Sperm parasitism in ants: selection for interspecific mating and hybridization. Ecology 87, 2148–2159. doi: 10.1890/0012-9658(2006)87[2148:SPIASF]2.0.CO;2
Umphrey, G. J., and Danzmann, R. G. (1998). Electrophoretic evidence for hybridization in the ant genus Acanthomyops (Hymenoptera: Formicidae). Biochem. Syst. Ecol. 26, 431–440. doi: 10.1016/S0305-1978(97)00124-5
Winston, M. L. (1992). Killer Bees. The Africanized Honey Bee in the Americas. Cambridge, MA: Harvard University Press.
Keywords: C. formosanus, hybrid vigor, subterranean termite, invasive species, C. gestroi, hybridization
Citation: Lee S-B, Chouvenc T, Patel J and Su N-Y (2020) Altered Mobility and Accumulation of Inefficient Workers in Juvenile Hybrid Termite Colonies. Front. Ecol. Evol. 8:589762. doi: 10.3389/fevo.2020.589762
Received: 31 July 2020; Accepted: 19 November 2020;
Published: 10 December 2020.
Edited by:
Alberto Arab, Federal University of ABC, BrazilReviewed by:
Judith Korb, University of Freiburg, GermanyChristophe Lucas, UMR7261 Institut de Recherche sur la Biologie de L'insecte (IRBI), France
Copyright © 2020 Lee, Chouvenc, Patel and Su. This is an open-access article distributed under the terms of the Creative Commons Attribution License (CC BY). The use, distribution or reproduction in other forums is permitted, provided the original author(s) and the copyright owner(s) are credited and that the original publication in this journal is cited, in accordance with accepted academic practice. No use, distribution or reproduction is permitted which does not comply with these terms.
*Correspondence: Sang-Bin Lee, bHNiNTE2MkB1ZmwuZWR1