- 1Department of Geoscience, Faculty of Natural Sciences, Aarhus University, Aarhus, Denmark
- 2Department of Geology, UiT, CAGE-Center for Arctic Gas Hydrate, Environment and Climate, Arctic University of Norway, Tromsø, Norway
Increasingly, strontium (Sr) isotopes are used to distinguish locals and migrants in prehistoric studies, by measuring 87Sr/86Sr in human remains and comparing these values to the distribution of the bioavailable 87Sr/86Sr in the study area, often in surface water. However, it has recently been shown that agricultural lime can have a substantial impact on the 87Sr/86Sr ratio and strontium concentration in surface water in areas where soils are low- to non-calcareous. Agricultural lime is rich in strontium with low 87Sr/86Sr ratios, such that interpretations of prehistoric migration based on surface waters affected by agricultural lime often overestimate the number of migrants in a given area. However, the impact of agricultural lime was questioned in a new study, which argues that strontium derived from agricultural lime is retained in the topsoil of the fields and therefore do not contaminate the surface water. In the present study and in a companion study in this volume, we show that strontium derived from agricultural lime is highly mobile in soils, and so contaminate surface waters extensively. We also show that the 87Sr/86Sr ratios are consistently higher in waters from “pristine areas” (where no agricultural lime has been applied within a distance of 150 m from the sample locality) than in water from farmland, thus confirming that it is of vital importance for accurate mapping of isoscapes to avoid sampling waters contaminated by agricultural lime. Our new measurements of 87Sr/86Sr ratios in central Jutland, Denmark, raise the highest measured values to 0.7186. High values between 0.7140 and 0.7156 occur repeatedly and it is apparent that nearly all prehistoric human finds in Jutland, previously believed to have journeyed from afar are more likely of local origin. Furthermore, we show that carbonate-rich areas along the coast of southwest Zealand carry high 87Sr/86Sr values (0.7112–0.7132), where we would expect low values. This surprising result indicates that nearly all humans buried at the Viking Age site, Trelleborg could well have originated locally, in contrast to past studies, which have suggested that about 50% of the burials were of individuals who came from afar.
Introduction
The strontium isotopic signature 87Sr/86Sr has been used to evaluate origin and mobility in prehistoric humans with considerable success (e.g., Ericson, 1985; Bentley, 2006; Montgomery, 2010; Guede et al., 2017; Knipper et al., 2017; Blank et al., 2018; Madgwick et al., 2019). Strontium signatures measured in human remains are compared to isotope maps (i.e., isoscapes or baselines) of the natural distribution of the biologically available 87Sr/86Sr in the study area. In many investigations, these maps are constructed from 87Sr/86Sr measured in present-day surface waters, but other proxies such as surface soils, plants, and fossilized- and living animals are also used (Capo et al., 1998; Evans et al., 2010; Price et al., 2011, 2012; Frei and Price, 2012; Maurer et al., 2012; Grimstead et al., 2017).
The accuracy of the isoscape is critical to the success of the method, and thus, new isoscapes are constantly published and older isoscapes improved, either by increasing the number of sample sites or by omitting sites deemed to have been contaminated, just as new mathematical methods for analyzing the strontium isotopic distribution in an area are regularly introduced (Bataille and Bowen, 2012; Bataille et al., 2018).
Today, the most wide-ranging isoscapes examine the distribution of strontium isotopes on continental scales (Widga et al., 2017; Bataille et al., 2018, 2020; Hoogewerff et al., 2019). The purpose of these isoscapes is primarily to create overviews, and they are generally not sufficiently detailed to allow for the evaluation of prehistoric mobility of individuals. The isoscapes published for Great Britain (Evans et al., 2010, 2018), France (Willmes et al., 2018), the Netherlands (Kootker et al., 2016), and Denmark (Frei and Frei, 2011; Frei and Price, 2012) were constructed from a much higher density of samples. For example, the Danish isoscape of Frei and Frei (2011) is based on close to 200 water samples covering an area of 43,000 km2, and in 2013, Frei and Frei published a baseline for the Danish island of Bornholm (588 km2) based on 51 samples. The isoscape for mainland Denmark has subsequently served as a reference map in several studies of prehistoric provenance and mobility ranging in time from the Neolithic to the early Middle age (e.g., Frei et al., 2015, 2017a,b; Felding et al., 2020; Van der Sluis et al., 2020). In practice, these large-scale isoscapes are typically combined with- or replaced by local isoscapes developed for specific mobility studies in the areas surrounding the archaeological sites under study (Price et al., 2002; Bentley, 2006; Slovak and Paytan, 2011; Maurer et al., 2012). The density of sample localities may be quite high in these studies. For example, Maurer et al. (2012) collected water samples from eighteen localities within a radius of 4 km from their archaeological targets.
The 87Sr/86Sr signature of a particular lake or stream is influenced by numerous factors, of which the underlying geology typically is the most important (Willmes et al., 2018). This means that a higher number of sample localities are needed in geologically heterogeneous areas than in more homogeneous ones (Grimstead et al., 2017: Bataille et al., 2020). The surface deposits across Denmark consist largely of glaciogenic weathering products from the Scandinavian shield carried to Denmark by ice during the last two major glaciations. The siliciclastic deposits are mixed with Cretaceous chalk and Early Paleocene limestones eroded in the Kattegat and Baltic Sea areas, where these carbonates are exposed (Figure 1). According to Frei and Price (2012), the content of strontium in the Danish soils is strongly dominated by contributions from these carbonates, which are rich in strontium with a low isotopic signature.
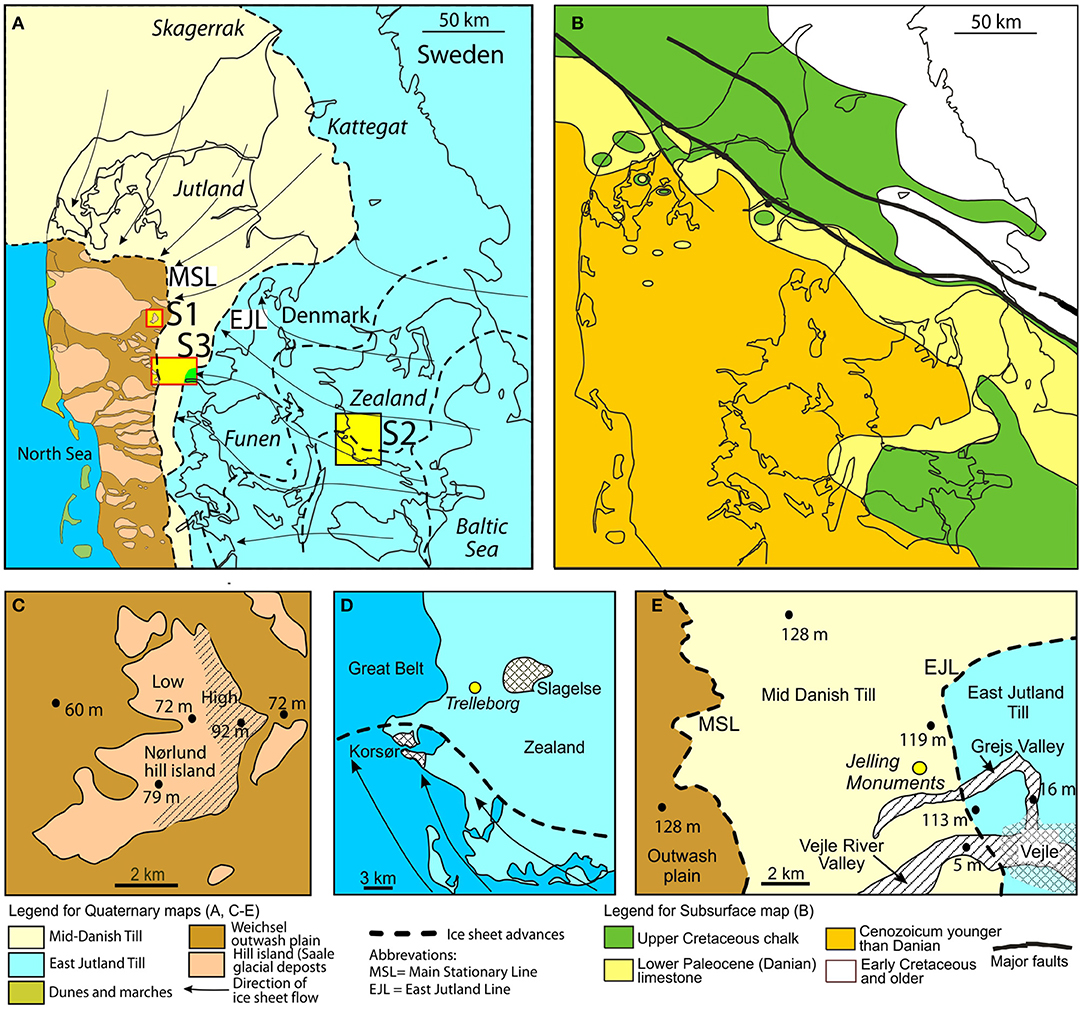
Figure 1. Simplified geological maps of Denmark with study areas depicted. (A) Map showing main features of the Quaternary glacial deposits. The Main Stationary Line (MSL) and the East Jutland Line (EJL) indicate the maximum extent of the Weichselian ice sheet in Denmark and the maximum extent of the East Jutland Advance, respectively. The two lines subdivide Jutland into three glaciogenic provinces: West Jutland, Central Jutland, and East Jutland. The distribution in West Jutland of hill islands (Saale glacial deposits) and Weichselian outwash plains is indicated. Arrows indicate direction of ice movement during advances to the Main Stationary Line and East Jutland Line. Locations of study areas S1–S3 are shown. Map is based on Krüger (1983); Madsen et al. (1992), Kjær et al. (2003), and Smed (2013). (B) Map showing the distribution of pre-Quaternary deposits in Denmark. Note distribution of Cretaceous chalk and Lower Paleocene limestone. Map is based on Håkansson and Pedersen (1992). (C) Map showing main features of study area S1, Nørlund hill island. (D) Map showing main features of study area S2, SWZealand. (E) Map showing main features of study area S3, Jelling.
Frei and Frei (2011) published 87Sr/86Sr values for 192 samples of surface water from Danish streams and lakes ranging from 0.70787 to 0.71282. From these values they calculated an isoscape for Denmark spanning from 0.708 to 0.711 (mean = 0.7096 ± 0.0015; 2σ). According to Price et al. (2011) and Frei and Price (2012), this relatively narrow range is the result of a rather simple and homogenous glacial geology. Nevertheless, in a recent study, Thomsen and Andreasen (2019) found a much higher variability within three areas of central and western Jutland. Within an area of a few square kilometers, these authors measured values ranging from 0.70847 to 0.71495, which greatly exceeded the total range in the isoscape for Denmark published by Frei and Frei (2011). The high values were encountered in water bodies from pristine areas, that is areas that have been sheltered from the influence of agricultural activity, and, in particular, from the effect of agricultural lime. Thomsen and Andreasen (2019) concluded that the narrow range observed by Frei and Frei (2011) was due to the fact that most of their samples came from areas that were affected by farming, here, in particular, by an extensive use of agricultural lime. The significance of the results of Thomsen and Andreasen (2019) has since been questioned (Frei et al., 2020), but in this paper and in an accompanying paper by Andreasen and Thomsen, 2021 in this volume, we present results that confirm and expand upon the central findings of Thomsen and Andreasen (2019) and that do not support the interpretations of Frei et al. (2020).
The main goal of the present study is to further investigate the high variability in the strontium signature and strontium concentration in water samples from pristine areas observed by Thomsen and Andreasen (2019). The high variability seems surprising since the surficial geological deposits of Denmark appear relative homogeneous consisting predominantly of siliciclastic glaciogenic sediments transported by ice from central Scandinavia during the Saalian and Weichselian glaciations and mixed with various amount of calcium carbonate mainly from chalk.
For the investigation, we selected three areas representing contrasting geological environments. Study area 1 (70 km2) comprises mainly non-calcareous water- or aeolian-sorted sandy deposits. The deposits of study area 2 (300 km2) are very different composed of strongly calcareous clayey tills. Study areas 1 and 2 are geologically relatively homogeneous. Study area 3 (200 km2) is much more heterogeneous as it can be subdivided into several geological zones ranging from pure non-calcareous sand in the west to calcareous clay in the east. The goal of the sampling strategy was to capture the lithological variability within each area as closely as possible, and to correlate the strontium results with the changing geology. The new elevated 87Sr/86Sr values for central Jutland and western Zealand has significant consequences for previous interpretations of prehistoric migrations in Denmark. This is exemplified by four case studies.
Distribution of Glaciogenic Deposits in Denmark
The landscapes and surface deposits of Denmark are almost ubiquitously formed by glacial activity during the Saalian and Weichselian glaciations. The Main Stationary Line marks the maximum extent of the Weichselian glaciation in Denmark, and dates to ca. 22.000 years (Figure 1A). It subdivides the country into two distinctly different geological provinces (Krüger, 1983; Houmark-Nielsen, 1987, 2003; Larsen and Kronborg, 1994; Kjær et al., 2003; Houmark-Nielsen et al., 2005) (Figure 1A). The landscapes west of the Main Stationary Line are dominated by flat outwash plains composed of sandy, often cross-bedded, meltwater deposits. Protruding through the outwash plains are numerous older, higher-lying landscape features, termed “hill islands” in the Danish geologic literature. They are generally remnants of the Saale glaciation. The hill islands consist of tills and meltwater deposits, with occasional outcrops of Quaternary marl and of Miocene sand, but their geology is not always well-understood (Krüger, 1983; Houmark-Nielsen et al., 2005). Apart from local marl deposits, the upper 10 m or more of both the outwash plains and hill island deposits are generally non-calcareous.
The landscapes east of the Main Stationary Line are referred to the Mid Danish Till. This unit consists of rather sandy tills mixed with local meltwater deposits. The upper 2–10 m is often non-calcareous (Madsen et al., 1992). After a major ice retreat, a new advance started about 19,000–18,000 years ago. This event is termed the East Jutland Advance (Houmark-Nielsen, 2003; Smed, 2013) (Figure 1A). The maximum extent of this new advance is marked by a number of terminal moraines forming the East Jutland Line. The East Jutland advance came from the southeast through the Baltic Sea depression and the deposits are both more clayey and more calcareous than the Mid Danish Till, which came from a more northeasterly direction (Houmark-Nielsen, 1987; Kjær et al., 2003) (Figure 1A).
After 18,000 years BP, the ice retreated rapidly from the East Jutland Line. Around 17,000 years, the general retreat was interrupted by several small advances, which, on the islands of Funen and Zealand, are marked by distinct terminal and lateral moraines (Houmark-Nielsen et al., 2005; Smed, 2013) (Figure 1A).
Distribution and Origin of the Natural Calcium Carbonate Component
The glacial deposits of western and central Jutland are low- to non-calcareous, while the deposits of eastern Jutland and the islands of Funen and Zealand are strongly calcareous. The reason for this change is primarily due to differences in the amount of lime, picked up by the various ice advances. The natural calcareous part of the glaciogenic sediments of Denmark consists predominantly of Cretaceous chalk and early Paleocene (Danian) limestone eroded by the advancing glaciers as they passed Skagerrak, Kattegat, the western Baltic Sea and eastern Denmark, where these deposits are exposed immediately below the glacial sediments (Kjær et al., 2003) (Figure 1B). The relatively soft Cretaceous and Paleocene carbonates consist mainly of well-preserved calcareous nannofossils (coccoliths) mixed with a diverse fauna of calcareous invertebrates. The chalk and limestone deposits disintegrate easily when subjected to normal weathering and are smeared into the siliciclastic sediments. These soft carbonates are mixed with smaller amounts of hard fragments of Ordovician and Silurian limestone transported primarily from central Scandinavia (Kjær et al., 2003).
Natural Strontium Sources
The natural strontium content of surface waters in Denmark are almost exclusively the result of a mixing of strontium from two major sources. The first source is the siliciclastic weathering products brought to Denmark during the Quaternary (Frei and Frei, 2011; Price et al., 2011). The origin of these deposits is the granitic and gneissic bedrocks of central Scandinavia. The 87Sr/86Sr signature in surface waters from pure siliciclastic deposits sampled in pristine areas is relatively high, ranging between 0.7108 and 0.7186, whereas the concentration is low, ranging from 0.002 to 0.064 (Thomsen and Andreasen, 2019; this study). The second source is the upper Cretaceous chalk and Lower Paleocene limestone, with minor contributions of Palaeozoic limestone (see above). Cretaceous and Paleocene carbonates are rich in strontium (~800–1,000 ppm) with a low 87Sr/86Sr ratio (~0.70785; Frei and Frei, 2011; Thomsen and Andreasen, 2019).
Agricultural Lime
About 57% of the total Danish area is farmland under plow, and nearly all of this land is regularly treated with agricultural lime, typically about 500 kg/ha/year (Thomsen and Andreasen, 2019). Agricultural lime is used to ameliorate the acidity of the soils and improve crop yield. It is most intensively applied in central and western Jutland, where the soil is naturally low- to non-calcareous.
In Denmark, agricultural lime and natural lime are basically the same product, namely chalk and limestone from the Upper Cretaceous and Lower Paleocene. The natural lime was eroded into the soil during the glaciations (Figures 1A,B). The agricultural lime is quarried from the same deposits in northeastern Jutland and eastern Zealand. The strontium content of agricultural lime and natural lime is therefore basically the same. We here disregard the Palaeozoic limestone fragments that contributes to the carbonate content in the glacial deposits, but are absent in the agricultural lime.
Thomsen and Andreasen (2019) showed that in western Jutland the 87Sr/86Sr ratio was distinctly higher in pristine surface water (0.7108–0.7150, N = 37), than in surface water from farmland (0.7091–0.7110, N = 16). The study also indicated that the majority of ponds, lakes, and streams in Denmark are contaminated by agricultural lime, such that their waters cannot be used in the construction of strontium isoscapes. The effect of agricultural lime was particularly strong in central and western Jutland, where the soil is naturally low- to non-calcareous. In eastern Jutland and on the islands of Funen and Zealand, where the natural content of lime is high, the effect was small and difficult to trace.
Materials and Methods
Sampling of Individual Samples
Nearly all samples were collected from ponds and small lakes. Only a few samples were taken in streams and larger lakes, as these water bodies in Denmark nearly always have been in contact with farmland and therefore are contaminated. The distinction between pristine (water bodies from non-agricultural areas) and non-pristine surface waters (water bodies from agricultural areas) was made during the planning of the field trips. For a pond to qualify as a pristine there must be a distance of at least 150 m between the pond and the nearest farmland (Thomsen and Andreasen, 2019 and references therein).
Sample sites were selected from studies of new and old topographical maps and aerial photographs in combination with the geological maps of Smed (1978). In principle, all selected water bodies were sampled. However, a few samples were not analyzed as the water bodies turned out to be contaminated (e.g., by bird feed, building waste).
Water samples were collected in acid-cleaned 250 ml polyethylene bottles. During field sampling, bottles were filled twice with sample water before final sampling. Most samples were taken 15–20 cm below the surface. However, a few samples were taken at a depth of 40–50 cm. Samples were stored in a cool room at 4 °C. The data for all analyzed samples are given in Table 1.
Strontium Isotope Analyses
Twenty ml of water were dried down and digested overnight in 2 ml of aqua regia, dried down again, and dissolved in nitric acid for strontium separation chemistry. Strontium was separated from the sample matrix using Eichrom (TrisKem International) Sr spec resin and analyzed for isotopic composition using a Nu Plasma II multicollector ICP-MS (inductively coupled plasma mass spectrometry) at the Department of Geoscience, Aarhus University. The four stable isotopes of strontium (84Sr, 86Sr, 87Sr, and 88Sr) were measured simultaneously, as were isotopes of Kr, Rb, Y, and doubly charged REE, to monitor and correct for interferences. Masses 82, 83, 84, 85, 86, 87, 88, and 89 were measured simultaneously, as were half-masses 83.5, 84.5, 85.5, 86.5, and 87.5. Baselines were determined by on-peak zero, and each sample run consisted of 400 s of peak time. Data were fractionation-corrected using the exponential law and normalized to NBS SRM 987 (87Sr/86Sr = 0.71025); and samples of Holocene foraminifera (Baculogypsina sphaerulata) expected to give a modern-day seawater Sr isotope value (87Sr/86Sr = 0.70917, Dia et al., 1992) were processed along with the samples as a secondary standard. Three samples of foraminifera analyzed throughout the analytical campaign gave 87Sr/86Sr = 0.709173 ± 0.000005 (2σ). Reproducibility of individual measurement of samples is estimated to be 0.0025% (0.000018, 2σ) based on repeat analyses of standards and samples. The Sr isotopic compositions of the analyzed samples are given in Table 1.
Selection of Study Areas
The main purpose of the present study is to investigate local variability in the strontium signature and strontium concentration in surface water from a region with a relatively simple glacial geology. We selected three study areas representing some of the most characteristic glacial landscape types in Denmark (Figure 1A). Sampling density was generally limited by the number of pristine water bodies making it impossible to capture the full geological variability. However, it was possible to sample all geological facies types and often with several samples.
Study Area S1. Nørlund Hill Island
The Nørlund hill island was selected to represent the Saalian hill island terrains, which, to our knowledge, have never been investigated for strontium. The Nørlund hill island was chosen because of its manageable size (30 km2; 70 km2 including surrounding outwash plains), because it has never been cultivated for farming, and because it contains several water bodies (ponds to small lakes) available for sampling (Figures 1A,C). Some of the larger water bodies in the central part of the island had to be omitted, because they are contaminated by feces from thousands of whooper swans, who uses them as a refuge, after feeding on nearby farmland (according to information from local forester). To the east, the hill island rises 20–25 m above the outwash plain (Figure 1C). The terrain falls gradually toward the west and the western delimitation is difficult to establish with certainty. This difficulty is enhanced by the fact that the sediments of the hill island and the outwash plain are very similar, composed of medium to coarse sand with some gravel. We also sampled three small isolated marl pits that were in use during the early part of the nineteenth century.
The hill island has periodically been subject to intense sand drift, and substantial areas are covered by sand dunes. The age of the sand dunes is poorly known, but most of them are probably of late glacial age. Today, about 1/3 of the Nørlund hill island is heathland, while 2/3 is coniferous forests. This is in contrast to the surrounding outwash plains, which for the most part are intensively farmed.
Study Area S2, SW Zealand
The costal terrain of southwest Zealand seems typical for the highly calcareous clayey tills that dominate eastern Jutland, and the islands of Funen and Zealand (Madsen et al., 1992; Kjær et al., 2003; Balstrøm et al., 2013) (Figures 1A,D). The main reason for selecting this particular region is that it is home for the Trelleborg Viking Fortress, where numerous human remains have been excavated and analyzed for their strontium content (Price et al., 2011) (Figure 1D).
This study area (~300 km2) is the largest of the three areas selected for this investigation, but, geologically, it is also the simplest, as it contains only a single landscape type, namely hilly moraines dominated by calcareous tills (Houmark-Nielsen et al., 2005; Smed, 2013). Nevertheless, it is not completely homogeneous. Parallel to the coastline at a distance of 4–10 km runs a series of low ridges marking a lateral moraine (Milthers, 1931; Smed, 1978, 2013) (see dashed line in Figures 1A,D). The ridges are the result of a late Weichselian glacier advance from the southeast dated to about 17,000 years (Houmark-Nielsen et al., 2005).
SW Zealand is densely farmed limiting the number of available pristine water bodies. This investigation of study area 2 should, therefore, be considered as only preliminary.
Study Area S3, Jelling and the North Side of the Vejle River Valley
The Jelling area was selected as a study area, because it constitutes one of the most complex glaciogenic landscapes in Denmark (Figures 1A,E). Although the selected area is only about 220 km2, it is intersected by two important glaciogenic boundaries in the country, namely the Main Stationary Line, which marks the maximum extent of the Fennoscandian ice sheet during the last glaciation, and the East Jutland Line, which marks the maximum extent of the East Jutland Advance (Figures 1A,E). The outwash plain in front of the Main Stationary Line and the Mid Danish Till behind the line date to about 22,000 years (Kjær et al., 2003). The East Jutland Line dates to about 19,000–18,000 years (see Geological Setting). The landscape west of the Main Stationary Line is dominated by flat, sandy, non-calcareous outwash plains. The Mid Danish Till between the Main Stationary Line and the East Jutland Line is rather sandy, and in the upper layers often low- to non-calcareous. This is in contrast to the tills of the East Jutland Advance, which are clayey and calcareous (Kjær et al., 2003). The area is intersected by several late Glacial and Holocene erosional valleys of which the Vejle River Valley and the Grejs Valley are the largest (Figure 1E).
Results and Interpretations
In presenting the data, we informally use the terms “high” and “low” 87Sr/86Sr values.” “High” values are used for signatures above the maximum 87Sr/86Sr ratio of 0.711 in the baseline for Denmark of Frei and Frei (2011), while “low” values are used for signatures below 0.711.
Study Area S1. Nørlund Hill Island and Surrounding Outwash Plains
A total of 33 ponds and lakes have been sampled and analyzed from Study Area S1 (Figure 2 and Table 1). Sixteen of these are from the hill island, while seventeen are from the surrounding outwash plains (Figure 2). Nine of the samples from the outwash plain are pristine; eight are from farmland. All sixteen samples from the hill island are pristine (Figure 2 and Table 1). The 87Sr/86Sr signature in the samples from the hill island varies from 0.70892 to 0.71855, while the concentration varies from 0.002 to 0.151 (Figures 3, 4A). The 87Sr/86Sr signatures from the central part of the hill island show relatively low variability ranging from 0.71103 to 0.71271. The lowest 87Sr/86Sr signatures (0.70892, 0.70980, and 0.70937) and highest Sr concentrations (0.036–0.151 mg/l), are found in the water from the three abandoned marl pits (Figures 3, 4A). The highest values (0.71324–0.71855) are from a relatively small area, which forms a peninsula on the western side of the hill island (Figure 3). The pristine 87Sr/86Sr values from the outwash plains vary from 0.71097 to 0.71338. The values from the farmland are distinctly lower, ranging from 0.70829 to 0.71011 (Figure 4A).
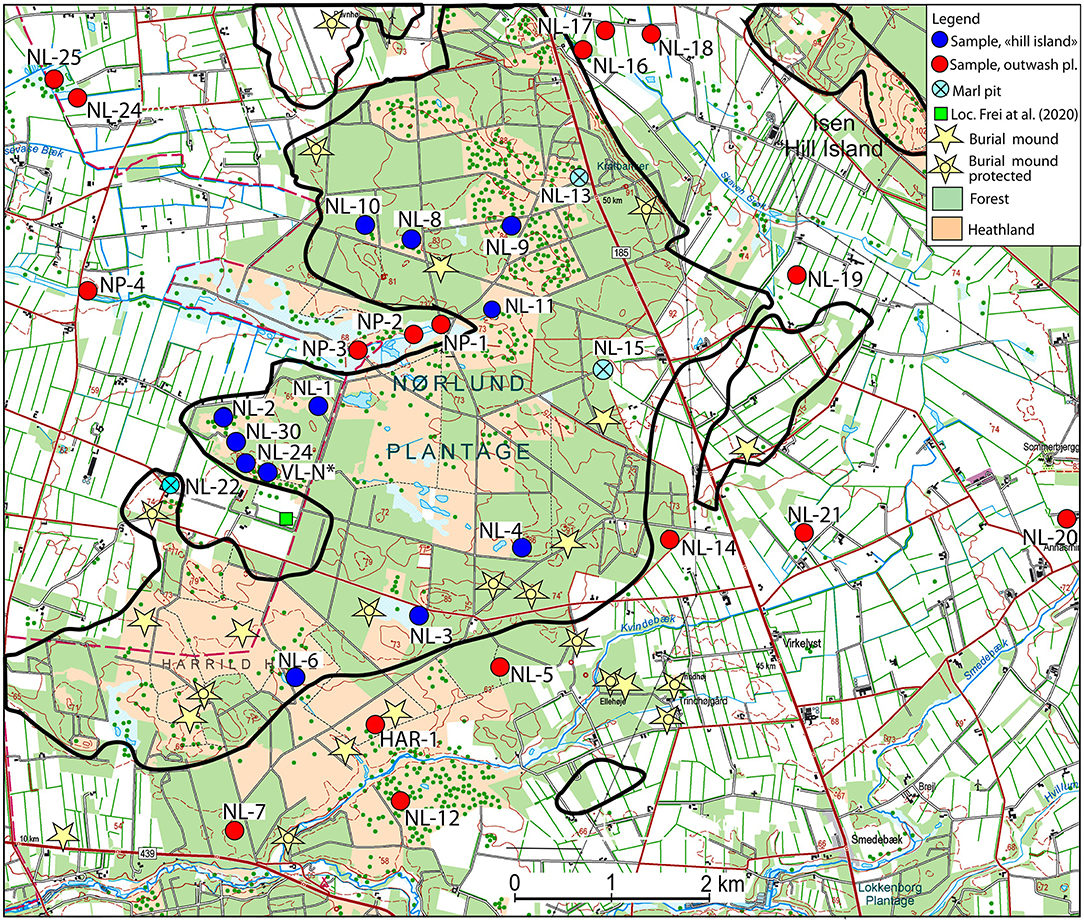
Figure 2. Modern topographical map of study area S1, the Nørlund hill island and surrounding outwash plains. The outline of the “hill island” is transferred from Figure 3 and indicated with a black line. Sample locations are indicated by colored dots. Sample number and detailed information on location and laboratory data is given in Table 1. Yellow stars mark location of preserved prehistoric burial mounds. See Figure 1A for location of study area. Location of the site investigated by Frei et al. (2020) is marked by a green square. Map is based on free data from “Styrelsen for Dataforsyning og Effektivisering, topografisk kort, WMS-tjeneste”.
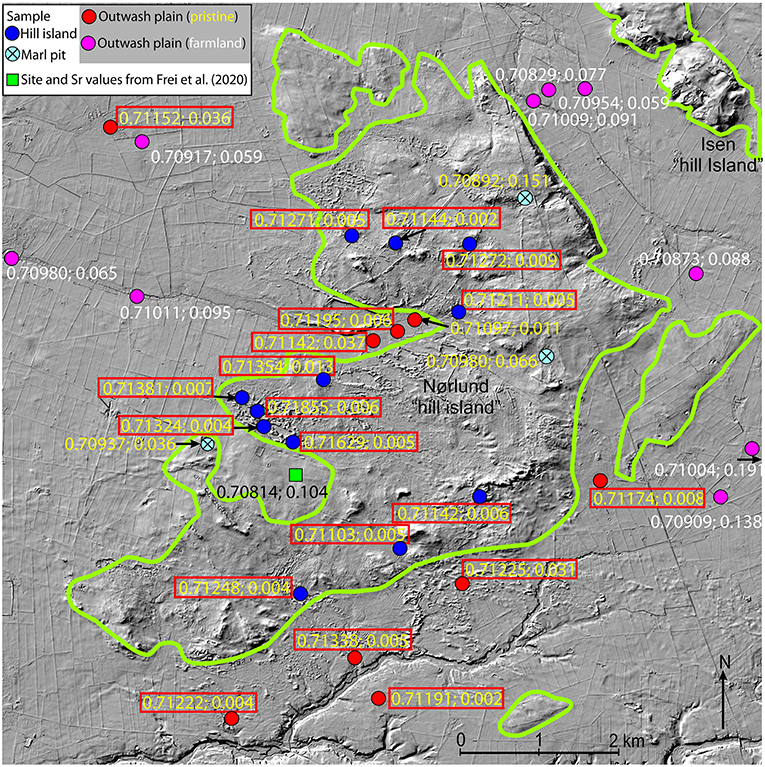
Figure 3. Hillshade map of study area S1, the Nørlund hill island, and surrounding outwash plains. The outline of the “hill island” is indicated by a green line. Sample locations are indicated by colored dots (for color code see legend and Figure 4). For numbering of sample stations see Figure 2. 87Sr/86Sr ratio and strontium concentration are shown for each sample. Sample stations with 87Sr/86Sr ratios exceeding the maximum value of 0.711 in the baseline for Denmark (Frei and Frei, 2011) are highlighted with a red box. Location of the site investigated by Frei et al. (2020) is marked by a green square. Map is based on free data from “Styrelsen for Dataforsyning og Effektivisering, skyggekort, WMS-tjeneste”.
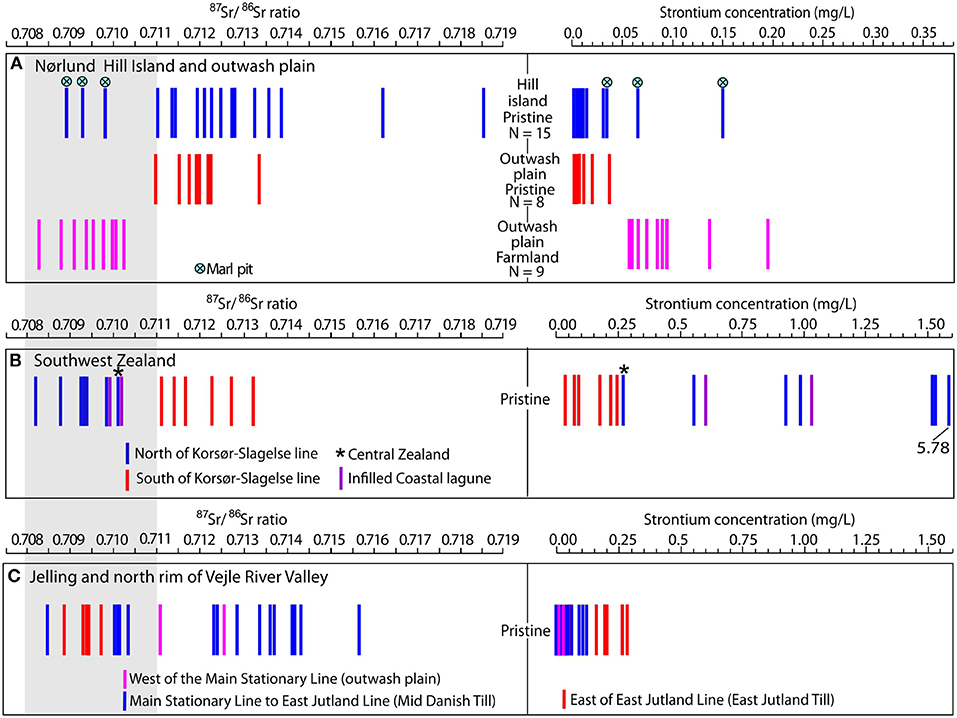
Figure 4. 87Sr/86Sr ratios and strontium concentrations for all surface water samples in each of the three study areas: (A) S1; Nørlund hill island, (B) S2; Southwest Zealand and (C) S3; Jelling and Vejle River Valley. Each sample is represented by two vertical bars; one for the 87Sr/86Sr ratio and one for the strontium concentration. The samples of study area S1 in (A) are subdivided into two main groups: Samples from the hill island and samples from the outwash plain. The samples from the outwash plain are further subdivided into pristine sample and samples from farmland. For study area S2 (B) and S3 (C) we have analyzed only pristine samples. In study area S2, we distinguish between samples from within the glacial advance at 17,000 years (see Figure 5, red and purple arrows) and samples from outside of this advance) (Figure 5, blue arrows). In study area S3, we mark the samples according to their geographical location (see Figure 7). Gray box to the left marks the 87Sr/86Sr isoscape for Denmark of Frei and Frei (2011). N = number of samples in each category.
One surprising discovery from the Nørlund hill island study is the very high maximum 87Sr/86Sr values of 0.71629 and 0.71855. The large span between the lowest ratio (0.70829) and highest (0.71855) was also unexpected, particularly as the total area of the hill island is only about 30 km2. Twelve out of fifteen samples from the hill island exhibit higher 87Sr/86Sr values than the maximum value in the isoscape for the whole of Denmark, created by Frei and Frei (2011). As they also exhibit low Sr concentrations (0.002–0.031 mg/l), there can be no doubt that these samples are from non-calcareous surface waters, and that the surface deposits of most of the hill island are non-calcareous.
The only pristine samples with 87Sr/86Sr values within the baseline of Frei and Frei (2011) are from the marl pits (Figure 4A). These small pits (diameter 30–<60 m) are surrounded by non-calcareous sandy deposits and appear isolated. The marl has previously been dug for soil improvement by nearby farms, but the pits have been abandoned for many decades. The marl is regarded to be of Saalian age. The Sr concentration in the marl-pit water is relatively high (0.036–0.151 mg/l), in accordance with the high content of calcium carbonate in these deposits (Figure 3).
According to historic maps (Høje Målebordsblade, 1842–99), the Nørlund hill island has not been under plow in historic times. The same is true for the outwash plain south of the hill island (Figure 2), which despite having a different origin and a different age, exhibits the same 87Sr/86Sr values and the same Sr concentrations as the samples from the hill island (Figure 3). The situation is different for the outwash plains to the east, north, and west. During the last 150 years, these plains have been increasingly cultivated, and today only small remnants of pristine land remain (Figure 2). From the outwash plains, we collected nine water samples from farmland and five from pristine land. The 87Sr/86Sr values of the pristine samples resemble the majority of samples from the hill island, with high 87Sr/86Sr values and low Sr concentrations whereas the values from the farmland samples show the opposite pattern and resemble the samples from the three marl pits (Figures 3, 4A and Table 1). This indicate that the farmland samples like the marl pit samples are affected by calcium carbonate. The only difference between them is that in the marl accumulations, the calcium carbonate is natural, whereas it is anthropogenically added agricultural lime in the farmland.
The results from the Nørlund hill island underline the main conclusion of Thomsen and Andreasen (2019) that agricultural lime in areas with low- to non-calcareous soils can significantly alter the isotopic composition and concentrations of the strontium in the surface waters (Figure 4A).
The highest 87Sr/86Sr values (0.71324–0.71855) on the Nørlund hill island are found in a series of shallow lakes and ponds located on the low peninsula on the western side of the hill island (Figures 2, 3). During the spring and summer of the year 2020, we dug a number of small pits a few hundred meters from the lakes and met groundwater at increasing depth of 70–100 cm over time (63.3–63.0 m above sea level). The groundwater table in the excavations coincides with and fluctuates simultaneously with the water level in the lakes, which is consistent with a groundwater source for the lake waters. The 86Sr/87Sr ratio of the groundwater from the excavations is high (0.71629) and close to the average signature value of the surrounding lakes. A groundwater control of the lakes on the peninsula is in accordance with several investigations, which have found that the water in many lakes and ponds on the sandy soils of central and western Jutland is dominated by groundwater or is a mixture of groundwater and surface water (Refsgaard et al., 2002). If and to what extent groundwater is infiltrating the ponds and lakes on the low-lying central part of the hill island is uncertain, just as it is uncertain what causes the difference between the relative low signatures values here and the high values on the peninsula.
Study Area S2. Southwest Zealand
Southwest Zealand is densely farmed and has a relatively low percentage of pristine areas (Figure 5). In the northern part of the study area, north of the main road between the cities of Korsør and Slagelse, the samples are from large low-lying bogs (Figure 5). In the southern part, the samples are from deciduous forests. All water bodies investigated from southwest Zealand are classified as pristine.
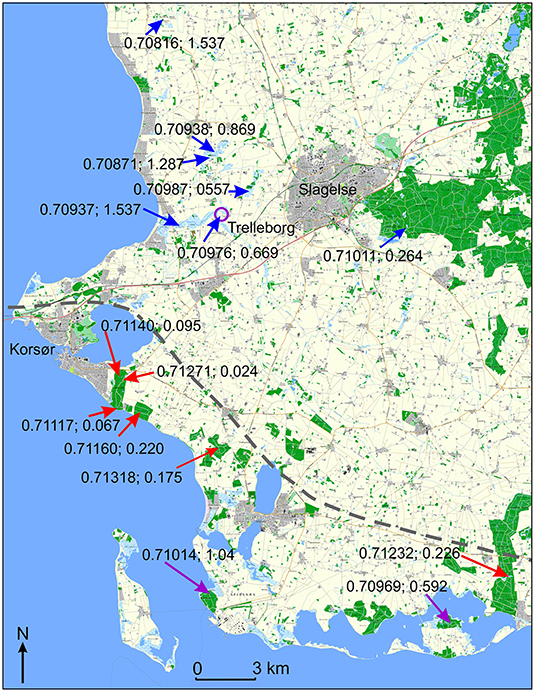
Figure 5. Map of study area S2, southwestern Zealand, showing location of sample stations. 87Sr/86Sr ratio and strontium concentration are shown for each sample. Sample number and detailed information on sample location and laboratory data are given in Table 1. The dashed line indicates the position of a lateral moraine associated with a glacial advance from the southeast dated to about 17,000 years (Houmark-Nielsen et al., 2005). Samples taken within the tills of the glacial advance are marked with red arrows. Samples taken in low-lying lagoonal deposits are marked with purple arrows. Samples taken outside of the advance are marked with blue arrows. For location of study area and full extent of the glacial advance see Figure 1A. Map is based on free data from “Styrelsen for Dataforsyning og Effektivisering, GeoDanmark natur og friluftskort, WMS-tjeneste”.
The 87Sr/86Sr signature of the bogs north of Korsør ranges from 0.70816 to 0.70938, while Sr concentrations vary from 0.557 to 5.738 (Figure 5, blue arrows). The low 87Sr/86Sr signature values, the high Sr concentrations and the overall low variability of the data indicate a strong influence of calcium carbonate on strontium, which is in good agreement with the typical high percentages of calcium carbonate in the tills of eastern Zealand (Madsen et al., 1992; Kjær et al., 2003, Smed, 2013). However, the eight samples obtained south of Korsør show a very different pattern. Geographically and isotopically, these samples fall into two groups. The first group consists of the six samples (TR-11, KS-1, KS-2, KLA-1, AP-1 and RUDE-1) taken in the hilly moraine landscape behind the coastline (Figure 5, red arrows, Table 1). Their 87Sr/86Sr values range from 0.71117 to 0.71318, while their Sr concentrations range from 0.024 to 0.226 (Figure 4B). The second group consists of the two samples taken in forests growing on infilled low-lying lagoons (elevation ~1 m) (Figure 5, purple arrows). They exhibit relatively low 87Sr/86Sr values (STIG-1=0.71014 and GLA-1=0.70969) (Figure 4B). The six samples from the moraine landscape show an unusual combination of both high 87Sr/86Sr values and relatively high Sr concentrations. Due to the influence of lime in the soil, high 87Sr/86Sr values are generally correlated with low Sr concentration and vice versa. However, a similar combination occurs on the Danish island of Bornholm (Frei and Frei, 2013), where the content of lime in the soil is low. The high Sr concentrations here are apparently caused by a higher content of radiogenic Sr-rich minerals in the soil. A similar explanation may be suggested for the tills along the western coast of Zealand. The area was affected by one of the last re-advances during the deglaciation (see Figure 1A). This advance could have brought material from the central part of the Baltic depression, where Bornholm is located, to the southwest coast of Zealand.
The low 87Sr/86Sr values in the two samples from the low-lying coastal areas are consistent with a marine influence as also indicated by unusually high concentrations of Na. In STIG-1, the Na concentration is nearly 30 times the average of the investigated surface water from Zealand; in GLA-1 it is a little lower. High concentrations of Na are a good indicator of an influence by seawater, which has a high concentration of Sr around 8 mg/L and a 87Sr/86Sr signature of 0.70917 (Dia et al., 1992).
The number of samples obtained south of Korsør is relatively low due to a scarcity of pristine areas. Nevertheless, the 87Sr/86Sr values of the analyzed water bodies are consistently high, indicating that the moraine landscape along the southwest coast of Zealand was dominated by high 87Sr/86Sr ratios. How far inland these high values continue has not been investigated, but a sample taken in the forests south of the town of Slagelse, ca. 17 km from the Great Belt show a low 87Sr/86Sr signature resembling the values from the northern part of the study area (Figures 4B, 5).
In conclusion, a substantial part of the moraine landscapes along the southwest coast of Zealand carries 87Sr/86Sr ratios above the maximum value in the isoscape for Denmark of Frei and Frei (2011) (Figure 4B). The archaeological implications of these findings are significant as discussed in the last chapter.
Study Area S3. Jelling and the North Side of the Vejle River Tunnel Valley
A total of 26 samples were analyzed from the Jelling area and the Vejle River Valley (Figure 6). Spatially, the samples are distributed irregularly, and they cannot be expected to show the full range of 87Sr/86Sr values and Sr concentrations in the area. The limiting factor is primarily the number and distribution of ponds and lakes with pristine surface waters, as the central area around Jelling is densely farmed (Figure 6). To supplement the data from this study, we include data from a neighboring area to the south investigated by Thomsen and Andreasen (2019).
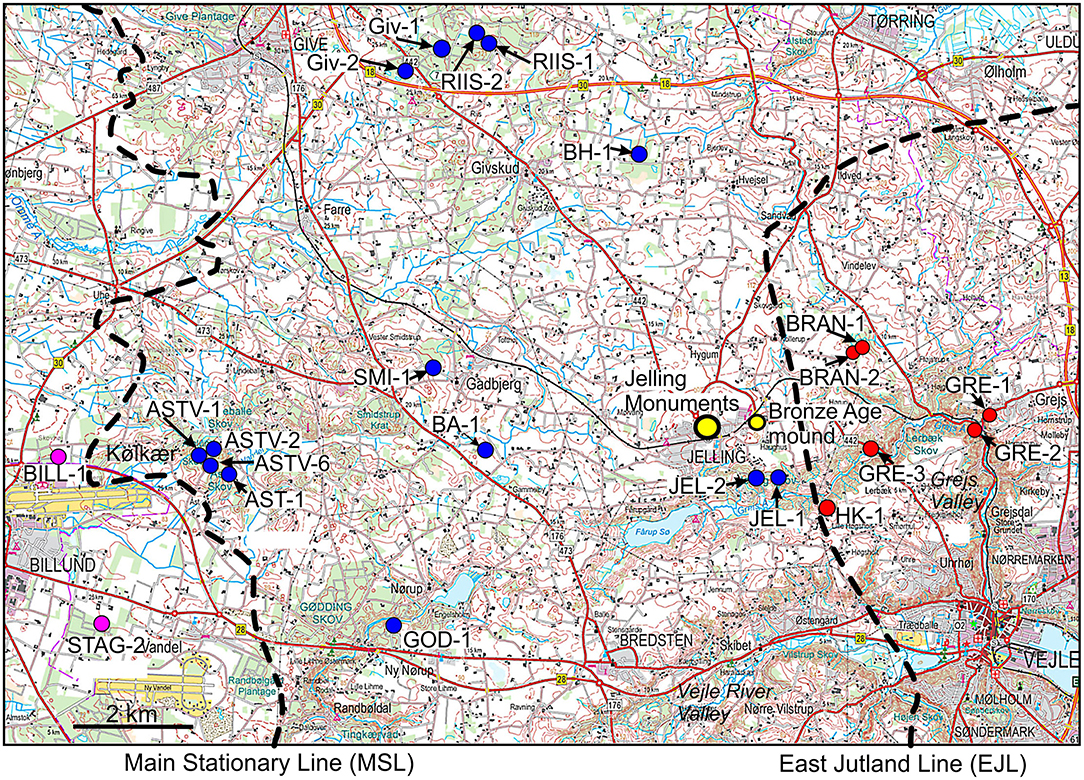
Figure 6. Modern topographical map of study area S3, Jelling. Sample location and sample number are indicated. Detailed information on location of samples and laboratory data are given in Table 1. For location of study area see Figure 1A. Location of the Jelling Viking Monuments and a recently analyzed Bronze Age burial (Felding et al., 2020) are indicated. Map is based on free data from “Styrelsen for Dataforsyning og Effektivisering, topografisk kort, WMS-tjeneste”.
The two 87Sr/86Sr values from the sandy plains west of the Main Stationary Line (Figure 7) (0.71102 and 0.71254) are typical for the outwash plains of Jutland. This is evidenced by a comparison with the results from Study area S1 and with 24 analyses of pristine waters from outwash plains at Egtved southwest of the study area, which show a range from 0.70929 to 0.71495 (Figure 8) (Thomsen and Andreasen, 2019).
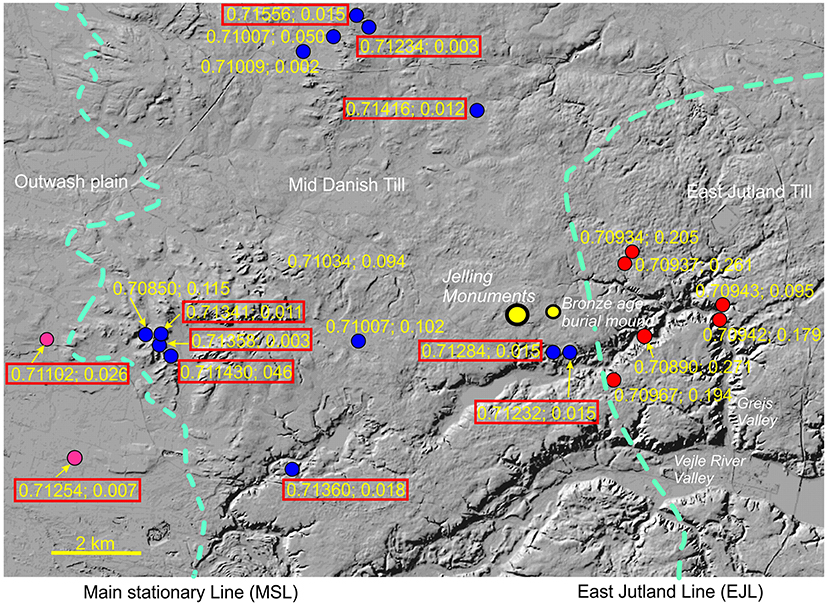
Figure 7. Hillshade map of the study area S3 (Jelling). Location of sample stations is indicated by colored dots. For numbering of sample stations see Figure 6. 87Sr/86Sr ratio and strontium concentration are shown for each sample. Sample stations with 87Sr/86Sr ratios exceeding the maximum value of 0.711 in the baseline for Denmark (Frei and Frei, 2011) are highlighted with a red box. Map is based on free data from “Styrelsen for Dataforsyning og Effektivisering, skyggekort, WMS-tjeneste”.
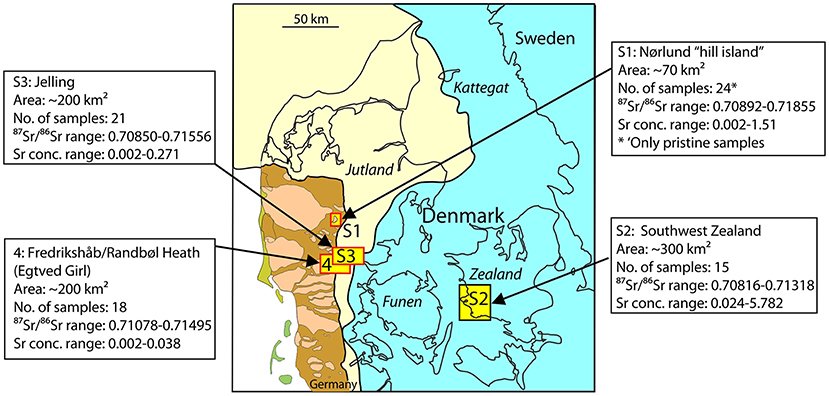
Figure 8. Statistical summary of 87Sr/86Sr ratios and strontium concentration for each of the three study areas. Location of study areas is indicated on Quaternary geological map. Site 4 is based on data from Thomsen and Andreasen (2019).
The central part of the study area is occupied by the Mid Danish Till. This till became exposed shortly after 22,000 years, when the ice-sheet rapidly withdrew from the Main Stationary Line (MSL) (Figure 1A). The till is complex and sometimes clayey and calcareous, but is mostly sandy and non-calcareous, especially in the upper few meters, possibly due to leaching (Smed, 1978; Madsen et al., 1992). Low-lying areas are often covered by small local outwash plains developed, as the ice sheet returned to the East Jutland Line about 18,000 years ago.
The 87Sr/86Sr values for the eighteen samples from the Mid Danish Till are highly variable, ranging from 0.70850 to 0.71556, with a mean 87Sr/86Sr value of 0.71170 (Figures 4C, 7). The geological complexity of the area is reflected in the 87Sr/86Sr data, where nearby ponds may have highly different 87Sr/86Sr values. This is exemplified in four ponds and lakes near Give, which have values ranging from 0.71007 to 0.71556, though they are located just 1 km apart (Figures 6, 7). The reason for these large differences is likely geological. According to landscape studies by Smed (1978), the ponds showing low values are located on a clayey till, whereas the ponds producing high values are located on a local outwash plain.
Similar contrasts are characteristic for the area around Kulkær Creek, in the western part of the study area (Figures 6, 7). This creek runs in a relatively steep gully cut into a higher-lying glacial landscape during the Holocene. Two ponds from the surrounding hills produce high values of 0.71358 and 0.71341, respectively, as compared to the low values found in ponds and spring near the bottom of the gully (around 0.70850) (Figure 7). The distribution of high and low 87Sr/86Sr values here may be due to leaching that dissolved the calcium carbonate from the top few meters of the soils atop the plateau. Great contrasts are also found in the Jelling area. Ponds from the upland along the edge of the Grejs Valley about 1 km south of the Jelling Monuments show high values (0.71284 and 0.71232). About 1 km east of these two ponds, we enter the calcareous East Jutland Till (Figures 6, 7). We have analyzed samples from six water bodies (streams and ponds) from this till and they all produce low 87Sr/86Sr values (0.70890–0.70967) and high concentrations (0.095–0.271) in accordance with the overall high content of calcareous material in this unit (Madsen et al., 1992; Balstrøm et al., 2013). The homogeneity of this till is underlined by the fact that we in the Grejs Valley area find the same values in ponds and springs from the upper rim to the bottom of the valley (Figure 7).
In summary, the 87Sr/86Sr distribution in the Mid Danish Till is characterized by great variability and contrasts. Water bodies with87Sr/86Sr values ranging from 0.708 to 0.710 can be found just a few hundred meters from water bodies ranging from 0.713 to 0.716 (Figure 7). This is in accordance with the geology of The Danish Till, which also is rich in contrasts, both in terms of the calcium carbonate content, but also in the composition of the non-calcareous siliciclastic component, which may vary from coarse-grained sandy gravel to fine grained clays with scattered stones (Smed, 1978; Thomsen and Andreasen, 2019).
Discussion
Sampling Media
Numerous methods have been used to estimate the local bioavailable 87Sr/86Sr range comprising both geological and biological materials (Bentley, 2006; Montgomery et al., 2007; Evans et al., 2010, 2018; Maurer et al., 2012). Based on a location in central Germany, Maurer et al. (2012) compared a large number of materials (ground vegetation, tree leaves, rock leachates, water, soil extracts, as well as modern and fossil animals) for best estimation of local bioavailable ratios when dealing with origin and movements of past populations. Of the many proxies investigated by Maurer et al. (2012), water and plant samples appear to provide the most accurate 87Sr/86Sr isoscapes. Principally, however, archaeological teeth enamel of rodents and selected mammals may provide an even better proxy Kootker et al. (2016). Unfortunately, they are rarely preserved in acidic environments (Kootker et al., 2016), and they are also absent or poorly preserved in acidic soils in central Jutland (Tjelldén et al., 2016), where two of our three study areas are located. Frei and Price (2012) published an isoscape for Denmark based on modern bones picked from owl pellets (mostly from rodents) mixed with a few archeological animal remains. The 87Sr/86Sr values show a narrow range (0.709–0.7108) and a homogeneous distribution pattern very similar to the isoscape of Frei and Frei (2011). The similarity of the two isoscapes suggests that strontium derived from modern animals may also be affected by agricultural liming.
In the present study, we prefer water for two reasons. Firstly, it allows us to directly compare our 87Sr/86Sr values with existing values. Secondly, water permits us to obtain Sr concentration data to use in modeling, which is difficult in plants, where the concentration may vary within a single plant and also between species (Poszwa et al., 2004). Strontium concentration data are often essential in order to understand the geological background for the spatial variations in 87Sr/86Sr values. This is exemplified in Figure 9, where we plot strontium concentration vs. 87Sr/86Sr ratios in water samples from Zealand (Study area S2) and Jutland (Study area S3), respectively. The plot indicates that the strontium in the two areas have a chalk/limestone source in common whereas the siliciclastic sources are different (see Results and interpretation, Study area S2).
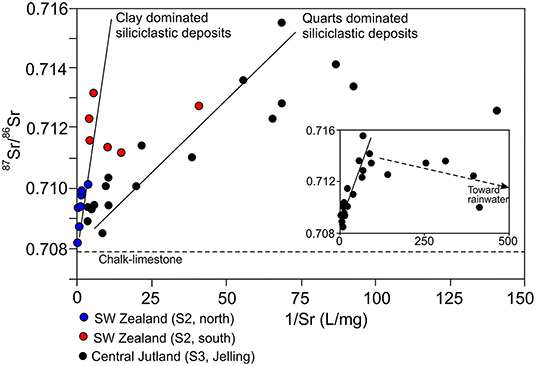
Figure 9. Strontium isotope ratio vs. inverse strontium concentration for pristine samples from SW Zealand (study area S2, blue and red dots) and central Jutland (study area S3, black dots). Samples from SW Zealand define a mixing line with steep slope (high Sr concentration) between a calcareous end-member dominant in the northern samples and a siliciclastic end-member dominant in the southern samples. Samples from central Jutland define a mixing line with a shallower slope (lower Sr concentration) indicating different compositions of the siliciclastic end-members in the two study areas. A few samples, particularly in Jutland (insert), fall off the mixing line and trend toward dilution with rainwater [87Sr/86Sr = 0.7092 and 1/Sr = 1,000–10,000 (Frei and Frei, 2011; Thomsen and Andreasen, 2019)].
Agricultural Lime Affects Natural 87Sr/86Sr Ratios
The reliability of the 87Sr/86Sr method to discriminate between prehistoric local and non-local individuals depends very much on the accuracy of the isoscapes used in the interpretation (Price et al., 2002; Frei and Frei, 2011; Maurer et al., 2012). These maps are intended to reflect the distribution of 87Sr/86Sr ratios in the past, but most are based on modern proxies such as surface waters and plants, which may be subjected to contamination by modern-day humans. Frei and Frei (2011) published a baseline for Denmark promoting a rather homogeneous 87Sr/86Sr range of 0.708–0.711, excepting the island of Bornholm. This range has since been adopted as a reference in numerous studies of prehistoric human mobility in Denmark (see below). However, the range of the baseline of Frei and Frei (2011) was extended when Thomsen and Andreasen (2019) obtained ratios of up to 0.71495 within areas of central and western Jutland. Moreover, Thomsen and Andreasen (2019) demonstrated that the main reason for the homogeneous baseline of Frei and Frei (2011) is that most of their samples were obtained from lakes and streams that were affected by additions of agricultural lime.
Frei et al. (2020) argued against Thomsen and Andreasen (2019), suggesting that strontium derived from agricultural lime is permanently retained in the organic-rich topsoil and therefore have no effect on the strontium content in the pore- and groundwater below or in groundwater-supported surface water. This conclusion is based on a single borehole bored in 2014 and located on the outwash plain immediately west of the Nørlund hill island (see Figures 1, 2). The site is regularly treated with agricultural lime and according to Frei et al. (2020), the content of strontium in the topsoil corresponds to about 50 years of normal liming (= 500 kg/ha/year). Frei et al. (2020) consider the site as representative for farm fields on the outwash plains of western Jutland. However, the groundwater (groundwater level 61.5 m above sea level) at the site is actually rich in strontium with a clear carbonate affinity (87Sr/86Sr ratio = 0.708135, Sr concentration = 0.104 ppm). According to Frei et al. (2020), the source of the strontium in the groundwater is not agricultural lime, but fragments of undissolved natural carbonate “relicts.” These should be present almost everywhere in the subsurface Quaternary deposits of western Jutland (Frei et al., 2020). The groundwater and groundwater-supported water bodies investigated by us, several of which are located only a few hundred meters from the site of Frei et al. (2020), should therefore also exhibit low strontium signatures and high concentrations. However, values comparable to those of Frei et al. (2020) were only found in water bodies in close contact with farmland and affected by agricultural lime plus at the three small, localized natural occurrences of marl (Figures 2, 3). All our samples from areas unaffected by agricultural lime show high 87Sr/86Sr values (up to 0.71855) and low Sr concentrations (down to 0.002 mg/l), indicative of absence of an input from lime (Figure 3). Thus, it seems unlikely that the source of the low 87Sr/86Sr ratios in the pore- and groundwater at the site of Frei et al. (2020) are subsurface carbonate relicts. The most plausible strontium source is agricultural lime. It is here important to convey that the investigated site in 2014 was not an ordinary farm field, as indicated by the authors, but a test field to investigate CO2 storage in soil (Jessen et al., 2014a,b). In the course of testing, no <48 t/ha of agricultural lime was spread across the test site in 2012–2013, before the samples used in Frei et al. (2020) were collected in 2014. We suggest that this lime, which incidentally corresponds to ~96 years of standard liming, is the main source for the high strontium content in the topsoil and in the pore- and groundwater at the site of Frei et al. (2020). This conclusion is in agreement with new studies, which indicate that strontium is not retained in the topsoil of the outwash plains of western Jutland, but rapidly transported into the pore and groundwater below (Andreasen and Thomsen, this volume).
Agricultural Lime Outside of Denmark
Agricultural lime is applied to farmland to reduce acidity in the soil and improve growth conditions for plants (Goulding, 2016). Agricultural lime is essential in non- to low-calcareous regions. It is also used in regions with calcareous soil to counteract the effect of leaching. Soils comparable to the Danish deposits described here occur widespread on most continents. In Europe, they are especially common along the rim of the Weichselian and Saalian ice sheets from Ukraine in the east to Ireland in the west (Böse et al., 2012; Ballabio et al., 2016; Price et al., 2017). In Europe and North America, the application of lime generally varies from 200 to 1,000 kg/ha/year (Adams, 1984; Goulding, 2016). These levels are comparable to the rates of agricultural liming in Jutland, suggesting that the use of lime in these countries would have a similar effect on the strontium content in the environment.
That agricultural lime can have a significant effect on even large river systems is exemplified in a study from the Ohio River system, eastern USA (Oh and Raymond, 2006). In two of the most intensively farmed watersheds from this system (~85% farmland), 29% of the riverine bicarbonate export in the years 1971–1987 could be attributed to agricultural lime. Furthermore, 30% of the observed difference in bicarbonate export between an agricultural and a forested watershed could be directly related to liming. Average yearly supply of lime in the investigated area is estimated to have been about 200–280 kg/ha (Oh and Raymond, 2006).
Archaeological Implications
Together, the three study areas analyzed in this paper plus the similarly sized area analyzed in Thomsen and Andreasen (2019) make up <1.8% of Denmark (Figure 8). This is clearly insufficient for proposing a new isoscape for Denmark. However, the data give 87Sr/86Sr ranges for three areas from the central part Jutland and one from western Zealand that cautiously may be used in prehistoric provenance studies from these regions. Here we are only concerned with the maximum isotopic values; the minimum value in Denmark will nearly always be close the value of chalk (0.7078) and it is rarely critical for Danish interpretations. The maximum 87Sr/86Sr values in surface water from the three study areas from Jutland are 0.71629 and 0.71855 for Study area S1, 0.71556 for Study area S2, and 0.71495 for the Egtved area (area 4 in Figure 8). Based on these values, we tentatively suggest that archaeological objects from the central part of Jutland with 87Sr/86Sr values up to 0.716 (or 0.715 for a more conservative estimate) most likely are of local origin. For SW Zealand, we propose a value 0.71318 corresponding to the maximum value found so far in Study area S2.
Accepting that 87Sr/86Sr values up to 0.716 (or more conservative 0.715) are obtainable in central Jutland and that values up 0.71328 are obtainable in western Zealand, it appears that many provenance and migration interpretations of prehistoric artifacts and individuals from Denmark warrant revision (e.g., Frei et al., 2017b, 2019; Nielsen et al., 2020; Van der Sluis et al., 2020). Below we will comment in more detail on four investigations, which are within the realm of our study areas.
Egtved and Skrydstrup Females
The iconic Bronze Age females from Egtved and Skrydstrup were re-interpreted in 2019 on the basis of new 87Sr/86Sr data from the vicinity of the burial mounds (Thomsen and Andreasen, 2019). Frei et al. (2015) and Frei et al. (2017b) obtained 87Sr/86Sr ranges for the Egtved Girl of 0.71028–0.71255 and for the Skrydstrup Woman of 0.7086–0.7138, based on samples from hair, nail and teeth. Frei et al. (2015) and (Frei et al., 2017b) compared these 87Sr/86Sr values to the isoscapes of Frei and Frei (2011) (max 87Sr/86Sr - 0.708–711) and concluded that both females were born and raised outside of Denmark, probably in central Europe, and that they first came to Denmark as teenagers. In 2019, Thomsen and Andreasen found high values in several pristine water bodies in the vicinity of the two burial sites indicating that the high values measured in their remains could have been obtained locally, thus challenging the interpretation that they originated from elsewhere.
Jelling Male
Felding et al. (2020) analyzed the 87Sr/86Sr ratios in six teeth from two Bronze age males buried in eastern Jutland, Denmark. The 87Sr/86Sr values of the three teeth from the eastern-most skeleton were all within the baseline range of Frei and Frei (2011) and the individual was determined to be of local origin. The inhabitant of the western-most grave (VKH 3418) near Jelling, (see Study area S3 in Figures 1, 6, 7) was determined to be different, as two of the three molars yielded values (0.71151 and 0.71128) above the isoscape of Frei and Frei (2011), indicating that this male may be of non-local origin and may have moved from a place outside present-day Denmark to the region of Jelling during his youth (Felding et al., 2020). However, these ratios are well below the values we have obtained from pristine waters in the Jelling area, which have 87Sr/86Sr ratios up to 0.71556 (Figures 6, 7). In fact, we have measured 87Sr/86Sr ratios of 0.71232 and 0.71284 in two ponds located <1 km from the investigated grave (Figure 7). The 87Sr/86Sr ratios obtained in the remains of the Jelling male could therefore have been obtained locally, challenging the interpretation that he originated elsewhere outside of present-day Denmark.
Origin of Bronze Age Wool Textiles
The results of a strontium isotope analysis of 42 pieces of wool textiles from the Danish Bronze Age suggests that at least 75% of the tested textiles were produced outside of present-day Denmark (Frei et al., 2017a) when compared to the isoscape of Frei and Frei (2011). In the present study, we propose a maximum 87Sr/86Sr value for central Jutland of 0.716 (or more conservative 0.715). Applying these figures, we calculated that only about 12% (or 21% using 0.715) of the wool textiles are of non-local origin.
Trelleborg Graveyard
Finally, we propose a re-interpretation of the Viking Age individuals buried at the Trelleborg fortress (Figure 5) located in western Zealand within Study Area S2. Price et al. (2011) analyzed remains of 48 individuals from a graveyard near the fortress for their 87Sr/86Sr compositions. Based on a maximum isoscape value for Denmark of 0.7108 (Price et al., 2011), the conclusion of the study was that just 16 out of 48 individuals were of local origin, while 32 individuals came from afar, possibly Norway and Poland. However, as all sites from coastal southwest Zealand investigated in this study show values above the maximum value of Price et al. (2011) with 0.71318 as the highest, it appears that all but four individuals could well be of local, southwest Zealandic origin. Of the remaining four, a young female with a 87Sr/86Sr value of 0.71542 and buried with a silver chest (Price et al., 2011) may possibly be from the Jelling region, as we have a 87Sr/86Sr value of 0.71556 just 10 km north of the Jelling Monuments (Figure 7). During this period, Jelling served as the royal seat for King Harold Bluetooth, and a connection between Jelling and the prominent contemporaneous fortress at Trelleborg is to be expected.
Concluding Remarks
Our expanded study of the high local variability seen in some of the most characteristic glaciogenic environments in Denmark confirms the central conclusions of Thomsen and Andreasen (2019) that isoscapes of 87Sr/86Sr ratios based on surface waters should be constructed exclusively from data from pristine areas. This implies that all data from water that are or have been exposed to agricultural lime should be avoided, especially in areas having low- to non-calcareous soils. Streams should be avoided once they have been in contact with farmland. Yet, even though the distinction between pristine and farmland samples may be of less significance in areas with highly calcareous soils, it is still advisable in such areas to collect pristine samples. This is exemplified by the high 87Sr/86Sr values observed along the southwest coast of Zealand (Study Area S2) where the tills are classified as calcareous (Madsen et al., 1992). Had we used an approach in which sampling was concentrated on major lakes and streams, we would probably have missed these high values, with significant consequences for the interpretation of the Viking Age individuals buried at Trelleborg. For this reason, a lower number of pristine samples should always take precedence over a higher number of randomly collected samples, in spite of the fact that a high sampling density normally would increase the accuracy of the resulting isoscape.
In Denmark, agricultural lime generally lowers the natural 87Sr/86Sr values of streams in water bodies in contact with farmland. The range of 87Sr/86Sr isoscapes becomes narrower with lower maximum values. Interpretation of prehistoric migration based on modern surface waters affected by agricultural lime therefore tends to overestimate the number of migrants in a given area. In areas, where the natural 87Sr/86Sr ratio mostly is below that of the applied agricultural lime, the situation may be reverse.
Data Availability Statement
The original contributions presented in the study are included in the article, further inquiries can be directed to the corresponding author/s.
Author Contributions
The study was devised by ET and RA. Sampling was planned and carried out by ET and TR. Sample processing and analyses as well as the initial geochemical interpretation was done by RA. ET planned and wrote the first draft of the manuscript and was responsible for the overall interpretation, continuously assisted by RA and TR. All authors contributed to the final version of the manuscript and approved the submitted version.
Conflict of Interest
The authors declare that the research was conducted in the absence of any commercial or financial relationships that could be construed as a potential conflict of interest.
Acknowledgments
We are grateful to C. Heilmann-Clausen, S. B. Andersen, C. Kronborg, A. Clemmensen, J. Thomsen, and S. Thomsen for enthusiastic assistance during fieldwork. We are grateful to L. Rasmussen, and forester H. Jensen, and several farmers and landowners from the three study areas, in particular J. Mølby and H. Pedersen for support and advice. We are grateful to E. J. Rosenberg, who improved the manuscript. Part of the research was supported by the Research Council of Norway through its Centers of Excellence funding scheme, grant number 223259 and Aarhus University Research Foundation (AUFF) NOVA grant E-2019-9-27.
References
Adams, F. (ed.) (1984). Soil Acidity and Liming, 2nd Edn, Agronomy 12. American Society of Agronomy/Crop Science Society of America/Soil Science Society of America, Madison, Wisconsin.
Andreasen, R., and Thomsen, E. (2021). Strontium is released rapidly from agricultural lime implications for provenance and migration studies. Front. Ecol. Evolut. 8:588422.
Ballabio, C., Panagos, P., and Monatanarella, L. (2016). Mapping topsoil physical properties at European scale using the LUCAS database. Geoderma 26, 110–123. doi: 10.1016/j.geoderma.2015.07.006
Balstrøm, T., Breuning-Madsen, H., Krüger, J., Jensen, N. H., and Greve, M. H. (2013). A statistically based mapping of the influence of geology and land use on soil pH. A case study from Denmark. Geoderma 192, 453–462. doi: 10.1016/j.geoderma.2012.08.024
Bataille, C. P., and Bowen, G. J. (2012). Mapping 87Sr/86Sr variations in bedrock and water for large scale provenance studies. Chem. Geol. 304–305, 39–52. doi: 10.1016/j.chemgeo.2012.01.028
Bataille, C. P., Crowley, B. E., Wooller, M. J., and Bowen, G. J. (2020). Advances in global bioavailable strontium isoscapes. Palaeogeogr. Palaeoclimatol. Palaeoecol. 555:109849. doi: 10.1016/j.palaeo.2020.109849
Bataille, C. P., von Holstein, I. C. C., Laffoon, J. E., Willmes, M., Liu, X. -M., and Davies, G. R. (2018). A bioavailable strontium isoscape for Western Europe: a machine learning approach. PLoS ONE 13:e019738. doi: 10.1371/journal.pone.0197386
Bentley, R. A. (2006). Strontium isotopes from the earth to the archaeological skeleton: a review. J. Archaeol. Meth. Theor. 13, 135–187. doi: 10.1007/s10816-006-9009-x
Blank, M., Sjögren, K. -G., Knipper, C., Frei, K. M., and Storå, J. (2018). Isotope values of the bioavailable strontium in inland southwestern Sweden - A baseline for mobility studies. PLoS ONE 13:e0204649. doi: 10.1371/journal.pone.0204649
Böse, M., Lüthgens, C., Lee, J. R., and Rose, J. (2012). Quaternary glaciations of northern Europe. Quat. Sci. Rev. 44, 1–25. doi: 10.1016/j.quascirev.2012.04.017
Capo, R. C., Stewart, B. W., and Chadwick, O. A. (1998). Strontium isotopes as tracers of ecosystem processes: theory and methods. Geoderma 82, 197–225. doi: 10.1016/S0016-7061(97)00102-X
Dia, A. N., Cohen, A. S., O'Nions, R. K., and Shackleton, N. J. (1992). Seawater Sr isotope variation over the past 300 kyr and influence of global climate cycles. Nature 356, 786–788. doi: 10.1038/356786a0
Ericson, J. E. (1985). Strontium isotope characterisation in the study of prehistoric human ecology. J. Hum. Evol. 14, 503–504. doi: 10.1016/S0047-2484(85)80029-4
Evans, J. A., Chenery, C. A., Mee, K., Cartwright, C. E., Lee, K. A., Marchant, A. P., et al. (2018). Biosphere Isotope Domains GB (V1): Interactive Website. British Geological Survey. (Interactive Resource).
Evans, J. A., Montgomery, J., Wildman, G., and Bouton, G. (2010). Spatial variations in biosphere 87Sr/86Sr in Britain. J. Geol. Soc. 167, 1–4. doi: 10.1144/0016-76492009-090
Felding, L., Reiter, S. S., Frei, K. M., and Vandkilde, H. (2020). Male social roles and mobility in the early nordic bronze age. A Perspective from SE Jutland. Danish J. Archaeol. 9, 1–167. doi: 10.7146/dja.v9i0.117955
Frei, K. M., Bergerbrant, S., Sjögren, K. -G., Jørkov, M. L., Lynnerup, N., Harvig, L., et al. (2019). Mapping human mobility during the third and second millennia BC in present-day Denmark. PLoS ONE 14:e0219850. doi: 10.1371/journal.pone.0219850
Frei, K. M., and Frei, R. (2011). The geographic distribution of strontium isotopes in Danish surface waters base for provenance studies in archaeology, hydrology and agriculture. Appl. Geochem. 26, 326–340. doi: 10.1016/j.apgeochem.2010.12.006
Frei, K. M., Mannering, U., Kristiansen, K., Allentoft, M. E., Wilson, A. S., Skals, I. S., et al. (2015). Tracing the dynamic life story of a Bronze Age Female. Sci. Rep. 5:10431. doi: 10.1038/srep10431
Frei, K. M., Mannering, U., Van den Berghe, I., and Kristiansen, K. (2017a). Bronze age wool: provenance and dye investigations of Danish textiles. Antiquity 91, 640–654. doi: 10.15184/aqy.2017.64
Frei, K. M., and Price, T. D. (2012). Strontium isotopes and human mobility in prehistoric Denmark. Archaeol. Anthropol Sci. 4, 103–114. doi: 10.1007/s12520-011-0087-7
Frei, K. M., Villa, C., Jørkov, M. L., Allentoft, M. E., Kaul, F., Ethelberg, P., et al. (2017b). A matter of months: High precision migration chronology of a Bronze Age female. PLoS ONE 12:e0178834. doi: 10.1371/journal.pone.0178834
Frei, R., and Frei, K. M. (2013). The geographic distribution of Sr isotopes from surface waters and soil extracts over the island of Bornholm (Denmark) – a base for provenance studies in archaeology and agriculture. Appl. Geochem 38, 147–160. doi: 10.1016/j.apgeochem.2013.09.007
Frei, R., Frei, K. M., and Jessen, S. (2020). Shallow retardation of the strontium isotope signal of agricultural liming – implications for isoscapes used in provenance studies. Sci. Total Environ. 706:135710. doi: 10.1016/j.scitotenv.2019.135710
Goulding, K. W. T. (2016). Soil acidification and the importance of liming agricultural soils with particular reference to the United Kingdom, Soil Use Manag. 32, 390–399. doi: 10.1111/sum.12270
Grimstead, D., Nugent, S., and Whipple, J. (2017). Why a standardization of strontium isotope baseline environmental data is needed and recommendations for methodology. Advan. Archaeol. Practice 5, 184–195. doi: 10.1017/aap.2017.6
Guede, I., Ortega, L. A., Cruz Zuluaga, M., Alonso-Olazabal, A., Murelaga, X., Pina, M., et al. (2017). Isotope analyses to explore diet and mobility in a medieval Muslim population at Tauste (NE Spain). PLoS ONE 12:e0176572. doi: 10.1371/journal.pone.0176572
Høje Målebordsblade (1842–1899). Available online at: http://miljoegis.mim.dk/spatialmap
Hoogewerff, J. A., Reimann, C., Ueckermann, H., Frei, R., Frei, K. M., van Aswegen, T., et al. (2019). Bioavailable 87Sr/86Sr in European soils: a baseline for provenancing studies. Sci. Total Environ. 672, 1033–1044. doi: 10.1016/j.scitotenv.2019.03.387
Houmark-Nielsen, M. (1987). Pleistocene stratigraphy and glacial history of the central part of Denmark. Bull. Geol. Soc. Denmark 36, 1–189.
Houmark-Nielsen, M. (2003). Signature and timing of the Kattegat Ice Stream: onset of the LGM-sequence in the southwestern part of the Scandinavian Ice Sheet. Boreas 32, 227–241. doi: 10.1111/j.1502-3885.2003.tb01439.x
Houmark-Nielsen, M., Krüger, J., and Kjær, K. H. (2005). De seneste 150.000 år i Danmark. Geoviden 2, 1–19. Available online at: https://www.geocenter.dk/wp-content/uploads/2018/07/Geoviden_2_2005.pdf
Jessen, S., Jakobsen, R., Postma, D., Looms, M., and Larsen, F. (2014a). Carbon Transfer Across the Vadose Zone: Inhibition by 20th Century Acid Rain? Available online at: https://co2gs.geus.net/xpdf/WP5-Carbon_transfer_across_the_vadose_zone.pdf
Jessen, S., Postma, D., Jakobsen, R., Looms, M. C., and Larsen, F. (2014b). Inhibition of carbon transfer across the vadose zone by 20th century rain. Geophys. Res. Abstracts 16:EGU2014-6340.
Kjær, K. H., Houmark-Nielsen, M., and Richardt, N. (2003). Ice-flow patterns and dispersal of erratics at the southwestern margin of the last Scandinavian ice sheet: imprint after palaeo-ice streams. Boreas 32, 130–148. doi: 10.1111/j.1502-3885.2003.tb01434.x
Knipper, C., Mittnik, A., Massy, K., Kociumaka, C., Kucukkalipci, I., Maus, M., et al. (2017). Female exogamy and gene pool diversification at the transition from the Final Neolithic to the Early Bronze Age in central Europe. PNAS 114, 10083–10088. doi: 10.1073/pnas.1706355114
Kootker, L. M., Van Lanen, R. J., Kars, H., and Davies, G. R. (2016). Strontium isoscapes in the Netherlands. Spatial variations in 87Sr/86Sr as a proxy for paleomobility. J. Archaeol. Sci. Rep. 6:15. doi: 10.1016/j.jasrep.2016.01.015
Krüger, J. (1983). “Glacial morphology and deposits in Denmark,” in Glacial Deposits in North-west Europe, eds J. Ehlers and A. A. Balkema (Rotterdam), 181–191.
Larsen, G, and Kronborg, C. (1994). Geologisk set. Det mellemste Jylland. Miljøministeriet, Skov og Naturstyrelsen og Geografforlaget, 1–272.
Madgwick, R., Lamb, A. L., Sloane, H., Nederbragt, A. J., Albarella, U., Pearson, M. P., et al. (2019). Multi-isotope analysis reveals that feasts in the Stonehenge environs and across Wessex drew people and animals from throughout Britain. Sci. Adv. 5:eaau6078. doi: 10.1126/sciadv.aau6078
Madsen, H. B., Nørr, A. H., and Holst, K., Aa. (1992). Atlas over Danmark, S.1, B.3, Copenhagen: C. A. Reitzel Publisher.
Maurer, A. -F., Galer, S. J. G., Knipper, C., Beierlein, L., Nunn, E. V., Peters, D. et al. (2012). Bioavailable 87Sr/86Sr in different environmental samples. Effects of anthropogenic contamination and implications for isoscapes in past migration studies. Sci. Total Environ. 233, 216–229. doi: 10.1016/j.scitotenv.2012.06.046
Milthers, V. (1931). Israndens Tilbagerykning fra Østjylland til Sjælland-Fyn, belyst ved Ledeblokke. Medd. Dansk Geol. Foren. 8, 1–70.
Montgomery, J. (2010). Passports from the past: investigating human dispersals using strontium isotope analysis of tooth enamel. Ann. Hum. Biol. 37, 325–346. doi: 10.3109/03014461003649297
Montgomery, J., Evans, J. A., and Cooper, R. E. (2007). Resolving archaeological populations with Sr-isotope mixing models. Appl. Geochem. 22, 1502–1514. doi: 10.1016/j.apgeochem.2007.02.009
Nielsen, B. H., Christensen, T. E., Moltsen, C., Frei, A. K., Reiter, S, Mortensen, M. F., et al. (2020). Late Neolithic Stenildgård grave: Re-excavated re-analysed and re-interpreted. Acta Archaeologica 9, 121–145. doi: 10.1111/j.1600-0390.2020.12224.x
Oh, N. H., and Raymond, P. A. (2006). Contribution of agricultural liming to riverine bicarbonate export and CO2 sequestration in the Ohio River basin. Global Biogeochem. Cycles 20:GB3012. doi: 10.1029/2005GB002565
Poszwa, A., Ferry, B., Dambrine, E., Pollier, B., Wickman, T., Loubet, M., et al. (2004). Variations of bioavailable Sr concentration and 87Sr/86Sr ratio in boreal forest ecosystems. Role of biocycling, mineral weathering and depth of root uptake. Biogeochemistry 67, 1–20. doi: 10.1023/B:BIOG.0000015162.12857.3e
Price, T. D., Burton, J. H., and Bentley, R. A. (2002). The characterization of biologically available strontium isotope ratios for the study of prehistoric migration. Archaeometry 44, 117–135. doi: 10.1111/1475-4754.00047
Price, T. D., Frei, K. M, Dobat, A. S., Lynnerup, N., and Bennike, P. (2011). Who was in Harold Bluetooth's army? Stronium isotope investigation of the cemetary at the Viking Age fortress at Trelleborg, Denmark. Antiquity 85, 476–489. doi: 10.1017/S0003598X00067880
Price, T. D., Meiggs, D., Weber, M. -J., and Pike-Tay, A. (2017). The migration of Late Pleistocene reindeer: Isotopic evidence from northern Europe. Archaeol. Anthropol. Sci. 9, 371–394. doi: 10.1007/s12520-015-0290-z
Price, T. D., Nielsen, J. N., Frei, K. M., and Lynnerup, N. (2012). Sebbersund: isotopes and mobility in an 11th-12th c. AD Danish churchyard. J. Archaeological Sci. 39, 3714–3720. doi: 10.1016/j.jas.2012.06.015
Refsgaard, J. C., Henriksen, H. J., Nilsson, B., Rasmussen, P., Kronvang, B., Skriver, J., et al. (2002). Vidensstatus for sammenhængen mellem tilstanden i grundvand og overfladevand. Arbejdsrapport fra Miljøstyrelsen 21, 1–110. Available online at: https://www2.mst.dk/udgiv/publikationer/2002/87-7972-157-5/pdf/87-7972-158-3.pdf
Slovak, N. M., and Paytan, A. (2011). “Applications of Sr isotopes in archaeology,” in Handbook of Environmental Isotope Geochemistry. ed M. Baskaran (Berlin; Heidelberg: Springer-Verlag), 743–768. doi: 10.1007/978-3-642-10637-8_35
Smed, P. (2013). Weichsel istiden på Sjælland. Geologisk Tidsskrift 1–42, ISSN 2245-7097, Copenhagen.
Thomsen, E., and Andreasen, R. (2019). Agricultural lime disturbs natural strontium isotope variations: implications for provenance and migration studies. Sci. Adv. 5:eaav8083. doi: 10.1126/sciadv.aav8083
Tjelldén, A. K. E., Matthiesen, H., Petersen, L. M. M., Søe, N. E., and Kristiansen, S. M. (2016). In situ preservation solutions for deposited Iron Age human bones in Alken Enge, Denmark. Conservat. Manage. Archaeol. Sites 18, 126–138. doi: 10.1080/13505033.2016.1182768
Van der Sluis, L. G., Daly, J. S., Frei, K. M., and Reimer, P. J. (2020). Mobility and diet in Prehistoric Denmark: strontium isotope analysis and incremental stable isotope analysis of human remains from the Limfjord area. Danish J. Archaeol. 9, 1–29. doi: 10.7146/dja.v9i0.116301
Widga, C. J., Walker, J. D., and Boehm, A. (2017). Variability in Bioavailable 87Sr/86Sr in the North American Midcontinent. Open Quaternary 3, 1–7. doi: 10.5334/oq.32
Keywords: 87Sr/86Sr, agricultural lime, prehistoric human migration, Bronze Age, Viking Age, glacial deposit, surface water chemistry
Citation: Thomsen E, Andreasen R and Rasmussen TL (2021) Homogeneous Glacial Landscapes Can Have High Local Variability of Strontium Isotope Signatures: Implications for Prehistoric Migration Studies. Front. Ecol. Evol. 8:588318. doi: 10.3389/fevo.2020.588318
Received: 28 July 2020; Accepted: 18 December 2020;
Published: 15 January 2021.
Edited by:
Joshua H. Miller, University of Cincinnati, United StatesReviewed by:
Jason Laffoon, Leiden University, NetherlandsIan Moffat, Flinders University, Australia
Copyright © 2021 Thomsen, Andreasen and Rasmussen. This is an open-access article distributed under the terms of the Creative Commons Attribution License (CC BY). The use, distribution or reproduction in other forums is permitted, provided the original author(s) and the copyright owner(s) are credited and that the original publication in this journal is cited, in accordance with accepted academic practice. No use, distribution or reproduction is permitted which does not comply with these terms.
*Correspondence: Erik Thomsen, ZXJpay50aG9tc2VuJiN4MDAwNDA7Z2VvLmF1LmRr