- 1Department of Biological Sciences, Virginia Tech, Blacksburg, VA, United States
- 2School of Plant and Environmental Sciences, Virginia Tech, Blacksburg, VA, United States
Perhaps more than any other ecological discipline, invasion biology has married the practices of basic science and the application of that science. The conceptual frameworks of population regulation, metapopulations, supply-side ecology, and community assembly have all to some degree informed the regulation, management, and prevention of biological invasions. Invasion biology needs to continue to adopt emerging frameworks and paradigms to progress as both a basic and applied science. This need is urgent as the biological invasion problem continues to worsen. The development of metacommunity theory in the last two decades represents a paradigm-shifting approach to community ecology that emphasizes the multi-scale nature of community assembly and biodiversity regulation. Work on metacommunities has demonstrated that even relatively simple processes at local scales are often heavily influenced by regional-scale processes driven primarily by the dispersal of organisms. Often the influence of dispersal interacts with, or even swamps, the influence of local-scale drivers like environmental conditions and species interactions. An emphasis on dispersal and a focus on multi-scale processes enable metacommunity theory to contribute strongly to the advancement of invasion biology. Propagule pressure of invaders has been identified as one of the most important drivers facilitating invasion, so the metacommunity concept, designed to address how dispersal-driven dynamics affect community structure, can directly address many of the central questions of invasion biology. Here we revisit many of the important concepts and paradigms of biological invasions—propagule pressure, biotic resistance, enemy release, functional traits, neonative species, human-assisted transport,—and view those concepts through the lens of metacommunity theory. In doing so, we accomplish several goals. First, we show that work on metacommunities has generated multiple predictions, models, and the tools that can be directly applied to invasion scenarios. Among these predictions is that invasibility of a community should decrease with both local controls on community assembly, and the dispersal rates of native species. Second, we demonstrate that framing biological invasions in metacommunity terms actually unifies several seemingly disparate concepts central to invasion biology. Finally, we recommend several courses of action for the control and management of invasive species that emerge from applying the concepts of metacommunity theory.
Invasion as a Metacommunity Problem
By its very nature, invasion biology demands a partnership between the basic and applied aspects of ecology and environmental science. However, the field has also struggled since its inception to find generalizable concepts and approaches that both enhance our fundamental understanding of invasive species that can also be leveraged to aid in invasive species management. Much of the work in invasion biology has focused on identifying, characterizing, and limiting the spread of problem invaders (Pyšek and Richardson, 2007, 2010). This invader-focused perspective is often driven by policy, regulation, and funding, and has produced a huge amount of insight into the biology and impacts of invasive species (Vilà et al., 2011; Crystal-Ornelas and Lockwood, 2020). However, despite the successes of invader-driven research, this perspective is ultimately limited because it overlooks an important aspect of invasions: invasion is a community ecology problem (Shea and Chesson, 2002; Gallien and Carboni, 2017). Viewing invasions in isolation rather than in the community context within which invasion occurs risks missing or misidentifying many of the determinants that dictate invasion success and the emergence of problem invaders (Pearson et al., 2018). A mechanistic understanding of the process of invasion and the effects invasions produce in native ecosystems requires a community assembly context, and modern community assembly theory is built within the framework of metacommunity ecology.
Humans have moved species both intentionally and accidentally for millennia. These actions have provided resources, sustenance, and quality of life for humankind, but an unintended consequence of these actions is a global mixing of life that has resulted in unprecedented biotic homogenization affecting every landscape and ecosystem on Earth. For example, exotic mussels (Cárdenas et al., 2020) and vascular plants (Chwedorzewska et al., 2015) have now been identified on Antarctica, long considered immune or isolated from invasion. Similar concerns have now been raised about the inevitable likelihood of the melting Artic suffering a similar fate (Ricciardi et al., 2017). The ecological and economic impacts of invasive species are diverse, and in some cases irreversible, including extinction of native species, increasing intensity and frequency of wildfires, and alteration of nutrient and water cycles (e.g., Vilà et al., 2011). Despite decades of research and management, the rate of introductions of new species continues to rise across all taxonomic groups (Seebens et al., 2018), suggesting that invasion and their resulting ecosystem transformations are the new normal. Addressing this element of global change requires a robust conceptual framework that is broadly applicable across diverse species and ecosystems, and readily translates to applied management, because unlike many subdisciplines in ecology, invasion biology is both a basic and applied science.
The proportion of introduced species that are ultimately considered invasive, i.e., cause negative impacts, is a small fraction, estimated at <1% (Williamson, 1996), though the veracity of this number has been questioned (Jarić and Cvijanović, 2012). The small number of introduced species that become invasive presents a perplexing challenge that has driven decades of research: What is it about these select few species that result in problem invasions? There are two intertwined aspects of invasive species that have to be considered simultaneously: the biogeographic aspect that deals primarily with the distribution and abundance of non-native species (Richardson and Pyšek, 2006), and the impact of those species since invaders are often defined by agencies like the International Union for the Conservation of Nature by their impacts. These aspects can be difficult to reconcile. The overwhelming majority of work that followed focused on (i) what makes a species invasive (invasiveness), and (ii) what makes communities susceptible to invasion (invasibility) (Richardson and Pyšek, 2006). What resulted were dozens of hypotheses, each seeking to explain invasiveness and invasibility (Catford et al., 2009), and testing these hypotheses greatly enhanced our understanding of individual invasive species, but left the field fragmented chasing non-generalizable explanations (Hulme et al., 2013).
The frustration for investigators produced by this piecemeal approach, and resulting equivocal evidence (e.g., Jeschke et al., 2012), has led to the development of multiple syntheses (e.g., Catford et al., 2009), and conceptual frameworks (e.g., Barney and Whitlow, 2008). These approaches identified common themes that cut across diverse species and systems. While no unifying framework has emerged that is broadly applicable and lends itself to leverage improved management, due to the diversity of invaders and the communities and contexts to which they are introduced, several important trends have emerged. For example, Catford et al. (2009) evaluated the overlapping aspects of >20 individual hypotheses and found that they cluster around common elements: ecosystem invasibility defined by the abiotic and biotic characteristics of the system, and propagule pressure—all of which are modified by human interactions. This synthesis largely reflected the original conception of invasiveness and invasibility, modified to reflect the dominant influence of propagule pressure, or the number and frequency of introduction events, which has long been recognized as a through-line concept in invasion biology (Lockwood et al., 2005; Colautti et al., 2006). Despite major advances in our understanding of invasive species, our search for a conceptual synthesis continues with a large emphasis on increasingly sophisticated analyses (Enders et al., 2020). Here we suggest that approaching biological invasions as a community ecology problem (e.g., Shea and Chesson, 2002) and that applying a metacommunity lens will aid the development of a generalizable and readily applicable framework for invasion biology. Such improvements would enhance our basic understanding of invasion success, while also affording enhanced mitigation strategies—an important goal to address the biological invasion crisis.
The Intersection Between Invasion Biology and Metacommunity Ecology
Over the previous two decades, metacommunity ecology has produced a paradigm shift in community ecology by emphasizing that community assembly is dictated not only by local niche factors like environmental conditions and species interactions, but that dispersal between local communities can also be a major driving force in community assembly (Leibold et al., 2004; Holyoak et al., 2005). The goals of metacommunity ecology closely align with current needs invasion biology in two identifiable ways:
The Importance of Dispersal in Community Assembly and Membership
While dispersal has long been recognized as important in structuring ecological communities (e.g., MacArthur and Wilson, 1967; Lewin, 1986), metacommunity theory recognized that dispersal can be a more powerful driver of community assembly and structure than previously thought. Though much of the history of community ecology has been devoted to local niche-based controls on community structure, work on metacommunities has demonstrated that dispersal can be as or more influential on structuring communities than niche-based processes, and that there is often an important interaction between local and regional processes. This influence of dispersal has been documented in a wide range of both theoretical (e.g., Mouquet and Loreau, 2003; Calcagno et al., 2006) and empirical (e.g., Cottenie, 2005; Werner et al., 2007; Heino and Mykrä, 2008; Brown and Swan, 2010; Chase, 2010) studies.
A Multi-Scale Perspective on Community Assembly
Metacommunity ecology strongly emphasizes the role of multi-scale factors in driving community assembly and membership by making a distinction between “local” and “regional” processes (Figure 1). Local processes are traditional niche-based processes that limit species distributions and abundances like species interactions, environmental conditions, and localized disturbances. Regional processes are those driven by dispersal between local communities. Thus, a metacommunity is defined as a set of local communities linked by the regional process of dispersal between those localities (Figure 1). One major goal of metacommunity research is to estimate the relative influences of local vs. regional factors on community composition, as well as to examine the local x regional interaction (Logue et al., 2011; Brown et al., 2017). Along with this focus on multiple scales came tools designed to describe multi-scale assemblages; chief among those are the descriptors of diversity, α, β, and γ. α-diversity is the diversity of a local community (or average of all local communities), usually defined by species richness or a diversity index like Shannon or Simpson. γ-diversity is the accumulated diversity of the entire metacommunity, and β-diversity is the turnover in species composition between local communities, often interpreted as the number of distinct communities on a landscape (more detail on diversity metrics can be found in the glossary; Chao et al., 2012). These diversity parameters are intimately related to one another, and γ-diversity can be mathematically partitioned into independent contributions of α and β, usually in the form of α × β = γ, though other valid partitioning formulations exist (Jost, 2007; Chao et al., 2012). For example, in Figure 1, using species richness as a measure of α, γ = 3, α = 2.5, and β = 1.2.
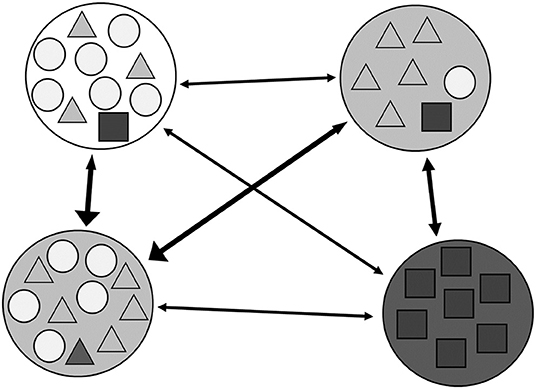
Figure 1. Schematic representation of metacommunity dynamics. Circles indicate habitat patches (i.e., local communities) where increased shading represents increased local filtering on community composition. Shapes represent individuals of a species and congruent shading of species and patches represents a match between species and habitat. Arrows represent dispersal between local communities, with the thickness of the arrow shaft indicating overall dispersal rate between communities and size of the arrow head indicating possible asymmetry in dispersal.
Because of a close alignment between focal questions in both metacommunities and invasion biology, metacommunity ecology is well-positioned to assist in the study of invasive species. The emphasis on propagule pressure in invasion biology (Simberloff, 2009) is closely mirrored by the emphasis on dispersal in metacommunity ecology (Leibold et al., 2004). Likewise, the need for a multi-scale perspective in invasion biology has been recognized and strongly advocated because the success of invasions is as reliant on the properties of the invaded community as it is on the properties of a specific invader (Shea and Chesson, 2002; Gallien and Carboni, 2017). These two foci also merge because it is dispersal among local communities that dictates assembly patterns in a metacommunity, and dispersal of native species may be as important in determining invasion outcomes as the dispersal of invasives (Howeth, 2017).
How Metacommunity Theory Can Assist in Addressing Invasive Species Questions
Decades of research in invasion biology have identified two key factors that are central to invasive species establishment and success: propagule pressure, and the characteristics of the community into which invaders are introduced (Simberloff, 2009). As suggested in Howeth et al. (2010), these factors in invasion biology directly parallel the regional and local forces addressed by metacommunity frameworks and suggest that invasions could benefit from a multi-scale concept. The multi-scale emphasis of metacommunity ecology can address invasive species spread and establishment in a realistic way by incorporating the movement of an invader from patch to patch across a landscape rather than focusing exclusively on a single-patch. Unlike single patch studies, which have dominated invasive species study systems (Box 1), a multi-scale approach can reveal details about the dynamics and limitations of invasive species. For example, Figure 2 depicts the mechanisms and limitations of spread of an invader through a metacommunity, illustrating the multi-scale roles of dispersal and limitation through local community conditions. As Figure 2 illustrates, the degree of spread of an invader is not just a product of local conditions or propagule pressure, but also depends on the interaction between those factors (Figure 2, spread from A to C).
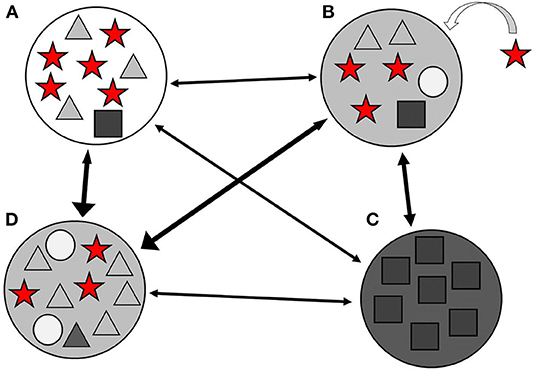
Figure 2. Schematic representation of the mechanisms and limitations of spread of an invasive species through a metacommunity. The invasive species is depicted in red and initially propagates into patch A. Spread to patch B is facilitated by the more permissive local conditions and lack of local filtering. Despite a moderate amount of environmental filtering in patch C, establishment is facilitated by substantial dispersal from A to C. Finally, the invader fails to establish in patch D despite relatively strong dispersal because of impermissive local conditions.
Box 1. Metacommunity theory and invasion biology.
To estimate the extent to which metacommunity theory is currently being utilized in the field of invasion science we surveyed all published articles in the >20 year catalog of the primary journal in the field, Biological Invasions. We surveyed the titles, abstracts, and keywords of all 4,280 articles published in Biological Invasions from 1999 to January 2020 for 13 of the most common concepts and terms in metacommunity theory. These terms were selected for either their general usage in metacommunity parlance (e.g., dispersal), or because they identified one of the major metacommunity paradigms (species sorting, mass effects, patch dynamics; Leibold et al., 2004). Many metacommunity terms were used (9/13), with “dispersal” (9.9% of papers), and “propagule pressure” (3.3% of papers) the most common. It is not surprising that “spread” related terms were the most common, as spread dynamics is one of the defining characters of invasive species (Richardson et al., 2000). However, aside from these common movement-related invasive species terms, only the metacommunity concepts “patch” (1.1%) and the generic “meta” (0.6%) were included in 25 or more papers over >20 years. This suggests that metacommunity theory has played little role in the field to date.
Number of articles including metacommunity terms in the journal Biological Invasions from 1999-(early) 2020 (N = 4,280 articles).
Metacommunity theory can also address the human aspects of invasive species by partitioning the human influence on the process of invasion into influences on either local or regional processes (see below for more detail about the human element in invasions). For example, human movement of a species that contributes to invasion directly affects dispersal, i.e., a regional process, while human modification of the environment primarily affects local processes. Clearly many examples of human activity won't fit easily into a facile local/regional dichotomy, but as illustrated before, metacommunity theory also emphasizes the interaction between local and regional processes.
A formidable body of theory has grown out of metacommunity ecology, complemented by an abundance of empirical tests of its efficacy. Metacommunity theory offers predictions about how biodiversity changes both locally and regionally (α and γ diversities) with rates of dispersal (e.g., Mouquet and Loreau, 2003; Gravel et al., 2011; Büchi and Vuilleumier, 2014), the consequences of local environmental filtering strength for community assembly (e.g., Chase, 2003; Sokol et al., 2011; Datry et al., 2016), how and why turnover (β-diversity) occurs across a landscape and what these patterns of β-diversity indicate about how communities are formed and maintained (e.g., Brown and Swan, 2010; Heino, 2011; Jamoneau et al., 2012), and how disturbance can affect metacommunity processes at both local and regional scales (e.g., Urban, 2004; Vanschoenwinkel et al., 2013; O'Neill, 2016). All of these efforts are largely directed at understanding the factors that control community composition and dynamics, including how they affect the entry of new species into a metacommunity. Accompanying the construction of this conceptual framework has been the development of methods and analyses for predicting and quantifying metacommunity patterns (e.g., Legendre et al., 1997; Nekola and White, 1999; Leibold and Mikkelson, 2002; Peres-Neto et al., 2006; Oksanen et al., 2020). Taken together, the conceptual framework of modern metacommunity ecology and the accompanying methodologies that have developed to support it offer a prodigious set of relevant tools for the study of invasions.
We will explore the application of metacommunity theory, approaches, and analyses to invasion biology, paying particular attention to elements that can increase understanding of invader establishment, spread, and effects, thereby enhancing mitigation and management. Our goal is to both broaden the conceptual frameworks and toolkits for invasion biologists, but also to highlight that biological invasions present excellent systems in which to explore metacommunity concepts. To meet these objectives, we provide a glossary of invasion and metacommunity terms, survey the invasion literature for metacommunity concepts, explore examples of invasion concepts and their metacommunity counterparts, identify where application of metacommunity concepts allows novel exploitation for invasive species management, and conclude with development of novel hypotheses.
Invasion Concepts in a Metacommunity Framework
Foundational to the exchange of ideas across disciplines or sub-disciplines is common vocabulary (Holbrook, 2013). To elucidate the parallels and opportunities for explicit incorporation of invasions in a metacommunity framework, we explore several important invasion biology concepts; first from a traditional invasion biology perspective, then through the lens of metacommunity ecology. Topics were chosen based on reviews and syntheses to be representative of broad invasion topics as examples, and not to be an exhaustive survey. We identified several invasion biology concepts that are regularly discussed as base elements of the field of invasion biology, including propagule pressure, biotic resistance, enemy release, functional traits, and human influences (Catford et al., 2009; Gurevitch et al., 2011; Enders et al., 2020).
Propagule Pressure
Invasion Biology Perspective
Biological invasions are the result of intentional or accidental introduction of species propagules to locations outside their historical range. Propagule pressure is the number and magnitude of introduction events of a single species to a location (Lockwood et al., 2005). This composite measure of the number of individuals (or propagules) introduced to a location has been shown to be integral to the success of invading species at all stages of invasion, including transport, colonization, establishment, and landscape spread (Theoharides and Dukes, 2007). The role of propagule pressure in invasion success cannot be overstated–all syntheses, reviews, and conceptual frameworks (including our analysis of invasion literature in Box 1) have consistently identified the important role that propagule pressure plays in successful invasion, and a practical understanding of propagule pressure has been described as “…probably the biggest challenge facing invasion ecologists” (Richardson, 2004). Simply put, successful invasion is dependent on successful movement of viable propagules.
In their search for general principles, Colautti et al. (2006) found few consistencies aside from the strong predictive power of propagule pressure, stating that “propagule pressure should serve as the basis of a null model for studies of biological invasions when inferring process from patterns of invasion.” As stated above, when Catford et al. (2009) reduced redundancies in the multitude of individual invasion hypotheses they found propagule pressure to be one of a tripartite of common elements. In his review of propagule pressure in invasion, Simberloff (2009) concluded that increasing the number of introduced propagules likely minimizes demographic stochasticity, while increasing the number of introduction events buffers against environmental stochasticity, hence partially explaining the mechanism of propagule pressure as fundamental to invasion success. In an under-appreciated proposal, Davis (2009) formalized the probability of invasion as a function of propagule pressure (N) and the probability of establishment of each propagule (P):
Davis' (2009) equation suggests that introduced species can successfully establish even with low individual propagule establishment potential, perhaps because of environmental mismatching or genetic bottlenecks, through a proportional exponential increase in the number of introduced propagules. In other words, successful invasion can result from either few introductions of propagules with high establishment potential, or large numbers of low establishment potential propagules. Increasing the number of introduced individuals, especially through multiple introduction events has been repeatedly found to increase invasion success, particularly when it increases genetic diversity (Simberloff, 2009). Though Barney et al. (2016) found that propagule pressure is not always a guarantor of establishment success, particularly in the face of strong biotic resistance of the receiving community. As with most aspects of biological invasions, successful establishment and spread is a result of complex interactions of many factors (e.g., Barney and Whitlow, 2008). Despite the universal importance of propagule pressure in invasion, it is still largely viewed within single patches, or as a driver of genetic variation in introduced populations.
Metacommunity Perspective
Propagule pressure is closely aligned with the concept of dispersal in a metacommunity. While “dispersal” is a more general term (Glossary) that can be used to describe a variety of movements of organisms, its realized meaning in most metacommunity applications is almost identical to propagule pressure, i.e., both movement and establishment. The major factor that differentiates metacommunity ecology from traditional niche-based ecology is dispersal and how its consideration leads to a multi-scale perspective on biodiversity dynamics (Leibold et al., 2004; Holyoak et al., 2005; Logue et al., 2011). For example, metacommunity approaches have demonstrated that dispersal-driven dynamics lead to higher biodiversity in more productive environments (Chase, 2010), that spatial structuring of local communities interacts with dispersal to affect community assembly (Sokol et al., 2015; Resetarits and Silberbush, 2016), and that position of a local community within a river network metacommunity often predicts how influential dispersal-driven dynamics are to community composition (Tornwall et al., 2017; Tonkin et al., 2018). This congruence between the concepts of propagule pressure and dispersal in a metacommunity suggests that the theory, predictions, and tools of metacommunity theory can be readily applied to biological invasions. However, given the 2 distinct aspects of invasive species—the biogeographic aspect and the impact—Metacommunity ecology is best positioned to deal with invaders in a biogeographic sense, as members of a metacommunity of organisms.
Many of the predictions of metacommunity ecology are based on either dispersal rates, or the connectivity between local communities of the metacommunity. In the latter case, connectivity is actually a proxy for dispersal since dispersal is generally expected to decrease with distance between local communities (Nekola and White, 1999; Chase et al., 2005; Altermatt and Fronhofer, 2018). Some of the most foundational predictions of metacommunity ecology regard how α, β, and γ-diversities change within a metacommunity across a gradient of dispersal (Mouquet and Loreau, 2003; Chase et al., 2005; Swenson et al., 2012; Matias et al., 2013). Likewise, dispersal is predicted to directly influence how communities assemble. Classic-niche based processes control community assembly at low rates of dispersal or connectivity, but become less important as dispersal increases and dynamics driven by propagule pressure overwhelm local niche-based processes (Mouquet and Loreau, 2003; Leibold et al., 2004; Chase et al., 2005). Importantly, these predictions have also received strong empirical support from subsequent studies (e.g., Cadotte, 2006; Hunt and Bonsall, 2009; Steiner et al., 2011; Carrara et al., 2012; Frisch et al., 2012; Heino et al., 2015), including some studies of invasive species. For example, Smith et al. (2020) demonstrated that human-mediated repeated introductions, even over long distances, allowed a widespread invasive plant to overcome genetic and environmental constraints. Both theoretical and empirical studies have extended these concepts to more complex metacommunities by using connectivity within networks to precisely map connectivity and predict how α, β, and γ-diversities will change at multiple scales in the metacommunity (e.g., Economo and Keitt, 2008; Brown and Swan, 2010; Carrara et al., 2012; Moritz et al., 2013). Indeed, some frameworks for invasion ecology also emphasize the role of invader propagule pressure in a community context, most notably Catford et al.'s PAB framework (Propaule pressure, Abiotic characteristics, Biotic characteristics; Catford et al., 2009), and the invasiability predictive framework presented in Hui and Richardson (2017).
One potential mismatch between current metacommunity approaches and the focus of invasive species work is that metacommunity models tend to consider the dispersal rates of communities as a whole rather than with a focus on individual species like an invader. However, some metacommunity models allow for flexibility or individuality in species' dispersal rates (Sokol, 2016; Sokol et al., 2017), and these models could be easily adapted for the purposes of generating predictions regarding invasives. However, we submit that the tendency of metacommunity work to focus on whole communities rather than individual species should be adopted by invasion ecology, particularly with regard to understanding the community context into which an invader is moving (see below for development of this idea).
Biotic Resistance
Invasion Biology Perspective
It has long been thought that not all communities are equally susceptible to invasion, a result of the unique combination of abiotic and biotic properties comprising individual ecosystems. The susceptibility of a community to invasion is termed invasibility, while the communities that are less susceptible to invasion are said to have “biotic resistance.” The concept of biotic resistance harks back to Darwin and the “father” of invasion biology Charles Elton. The general concept is that resident species with long co-evolutionary histories are best adapted to a locality and will exclude invaders through a combination of competitive exclusion and niche filling (Elton, 1958; Levine et al., 2004). Thus, Elton's proposal was that communities with large numbers of native species will better resist invasion than communities with fewer natives. However, there has also been contrasting evidence that supports a concept of Biotic Acceptance, essentially a “rich get richer” paradigm in which species rich communities are more susceptible to invasion because the environment is favorable for both natives and invasives (Stohlgren et al., 2006).
A growing body of research shows equivocal evidence for Biotic Resistance, finding both positive and negative relationships between exotic and native species richness (Fridley, 2010), which was largely attributed to the scale and method (experimental vs. observation) at which the study was conducted (Shea and Chesson, 2002). In an attempt to reconcile this seeming “invasion paradox,” Fridley et al. (2007) conclude that native-rich communities are more invasible than native-poor communities, and that threats to native residents will accelerate further invasion. However, a recent expansive meta-analysis found strong support that more native-rich communities supported fewer exotic species across a wide range of communities and ecoregions (Beaury et al., 2020).
Expanding the scope of propagule pressure, Lockwood et al. (2009) propose the concept of colonization pressure, or the number of species introduced to a single location, as a key explanatory parameter describing exotic species richness. Similar to propagule pressure, they argue that the probability of exotic species establishing is proportional to the number that are introduced. This concept unites both propagule/colonization pressure and biotic resistance because there is always an element of stochasticity in colonization by non-native species, and increasing the number of chances at successful invasion ultimately results in higher numbers of invasions. Thus, propagule pressure and community composition of the target community in invasions are inherently integrated concepts that interact in a complex network, partially determining the success of introduced species.
Metacommunity Perspective
One of the major goals of metacommunity ecology is to understand how communities are assembled and the mechanisms underlying the distributions of species. Classical ecological approaches have largely attempted to explain the presence of a species in a particular locale using local niche-based factors like environmental conditions and species interactions, often with the implicit assumption that presence of a species indicates favorable conditions (e.g., Hutchinson, 1959; MacArthur, 1970). This “overwhelming emphasis on localness” (Lawton, 1999) dominated ecological thought for decades and continues to be the standard paradigm for much of ecology, environmental science, and conservation. Approaches that focus on localness have also formed the foundational basis for invasion biology (Peterson, 2003), and local niche-based mechanisms lie at the heart of the Biotic Resistance hypothesis. To be clear, these niche-based processes are fundamental to understanding community assembly, and density-dependent interactions between species often play a strong role in dictating community composition, even when dispersal is high (Thompson et al., 2020). However, metacommunity theory recognizes that species presence in a local community is the product of multiple factors, both local and regional, and that the occurrence of a species does not always reflect favorable local niche-based conditions (Leibold et al., 2004).
The application of metacommunity theory to the topic of biotic resistance begins with the recognition that species presence or absence from a local community is not dictated by local factors alone. In some ways, invasion biology has already made this acknowledgment through its emphasis on the importance of propagule pressure. However, from a metacommunity perspective, propagule pressure isn't necessarily just a vehicle for the introduction of a species to a site, after which local factors take over. Rather, propagule pressure is a dynamic force of community assembly that interacts with local factors like density-dependent population growth and species interactions, and it is this interplay that dictates a species inclusion or exclusion from any locality (Thompson et al., 2020). In other words, the mechanisms through which an invader successfully establishes in a local community will depend on the assembly mechanisms of that community. In a species sorting metacommunity (glossary), propagule pressure is just a vehicle for arrival of an invader, and successful establishment occurs though local density-dependent interactions like environmental suitability and interactions with currently residing species. This process of community membership being limited by local factors like environmental conditions and species interactions is collectively termed “species sorting” in metacommunity parlance. However, in metacommunities where assembly follows a patch dynamics (glossary) or mass effects (glossary) pattern, dispersal will play a more active role in establishment and maintenance of local species composition. Additionally, these latter paradigms emphasize multi-scale dynamics that will not be obvious by a focus on a single local patch. In fact, a recent experiment in which native species reduced invader propagule pressure because invader dispersal was density-dependent clearly demonstrates the interaction between local-scale competition and propagule pressure of invaders (Legault et al., 2020).
Much of invasion biology has been based on single-patch approaches (Box 1), and seeking local explanations in cases when dynamics are actually driven by regional-scale processes could be potentially confounding to studies of invasive species establishment, resulting in erroneous, or nonsensical conclusions. Thus, applying multi-scale approaches to invasion has the potential to reveal mechanistic insights that would be difficult or impossible to derive from single-patch studies.
One particularly effective example of this multi-scale approach applies community coexistence theory (Chesson, 2000) in a spatial context to create a framework for understanding successes and failures of invaders by viewing invasion as a successional process (Hui and Richardson, 2017). The framework uses two traits of invaders to categorize possible outcomes: the fitness differences between natives and invaders, and the degree to which the invader niche overlaps those of resident species, and suggests that, early in the process of invasion, a Neutral Paradigm (glossary) is more likely to dictate community assembly than niche-based processes due to the more stochastic nature of propagule pressure. The framework identifies not only three possible outcomes—coexistence, invader excluded, invader excluding residents—but also the rates at which these outcomes are expected to materialize (Hui and Richardson, 2017).
The ability of a species to invade an established local community has long been a focus of community ecology. In fact, a metric known as the invasibility criterion is often used as a definitive test of the ability of species to coexist in communities (MacArthur, 1972; Holt, 1977; Chesson, 2000). In this case, coexistence is distinguished from co-occurrence, though they are often incorrectly used interchangeably (Siepielski and McPeek, 2010). True coexistence doesn't simply imply that two species appear together; both species must both be able to persist together indefinitely (Holt, 1977; Blanchet et al., 2020). The invasibility criterion stipulates that in order for a species to truly coexist with other species in a community, it must be able to increase its abundance when rare, in the presence of other community members (Chesson, 2000; Siepielski and McPeek, 2010). The name of the criterion clearly derives from the scenario of a species invading, at low abundance, a locality within an extant community. Therefore, one potential mechanism of biotic resistance is that interactions with other species in a community cause an invader to fail the invasibility criterion. However, metacommunity approaches demonstrate that even if a species fails to meet the invasibility criterion, the species may still co-occur and even spread to new patches if dispersal of an invader is high, and their dynamics more resemble a mass effect or patch dynamic paradigm than a species sorting (i.e., niche based) paradigm. Thus, presence of an invader does not necessarily imply that a species is coexisting with native species, nor that an invader is even gratified by local conditions, shifting the burden of proof for biotic resistance (or acceptance) to a demonstration of local control on community membership.
Enemy Release
Invasion Biology Perspective
Perhaps the most commonly cited explanation for the success of invasive species is escape from their suite of herbivores, pathogens, predators, and other biotic limitations in the introduced range. Termed Enemy Release (Keane and Crawley, 2002), this hypothesis serves as the basis for classical biological control whose modus operandi is to introduce native enemies of the invader into the introduced range to limit population growth, as well as the basis for a range of additional hypotheses expanding on this basic tenet (e.g., Evolution of Increased Competitive Ability, Blossey and Notzold, 1995). Enemy release is also an element of biotic resistance broadly interpreted, comprising elements of an ecosystem that may limit exotic species success.
In a meta-analysis, Levine et al. (2004) found little evidence that resident competitors, their diversity, and the resident suite of limiting herbivores and facilitating soil fungal communities curb exotic species introductions, but do play a role in limiting population growth once established, and thus may contribute to invaders failing the Invasibility criterion. Evidence for enemy release remains weak, with some studies showing strong support for release from plant pathogens and viruses (Mitchell and Power, 2003), while a meta-analysis showed reduced herbivore damage especially of specialists (Liu and Stiling, 2006) and higher insect diversity on native plants (Meijer et al., 2016). As with most aspects of invasion biology, testing outside the plant kingdom remains scant (e.g., Roy et al., 2011), precluding broad, empirically-supported, general statements.
Metacommunity Perspective
As with Biotic Resistance, the Enemy Release hypothesis highlights local factors as a mechanism for success or failure of an invasion. In the case of Enemy Release, local filters on community membership that are created by natural enemies present in the home range of an invader are absent in the colonized community. From a metacommunity perspective, Enemy Release differs from Biotic Resistance because it necessarily invokes complex trophic structure, with invaders in their natural ranges serving as a food resource. However, the majority of metacommunity theory was developed around concepts of competitive metacommunities (e.g., Mouquet and Loreau, 2003; Chase et al., 2005; Mouquet et al., 2005) and the incorporation of more complex trophic structure into metacommunity frameworks is a relatively new advance (Leibold and Chase, 2018; Guzman et al., 2019). Despite the relative youth of multi-trophic metacommunity concepts, there are already considerable insights that may benefit invasion biology.
As with the Biotic Resistance Hypothesis, the appeal to strictly local mechanistic explanations misses the potential for larger-scale, dispersal-driven dynamics. While release from natural enemies in a local community may explain some of the successes and failures of invasion, dispersal-driven dynamics of both the invader, and the metacommunity into which it is invading, may play a larger role than local-scale species interactions. Predators and parasites move; prey move, and plants disperse to new areas. The dynamics produced by those movements on a landscape can rarely be captured in single-patch studies. Simulation models show a complex interplay between trophic level persistence and the spatial properties of a metacommunity, illustrating that overlooking the spatial properties of metacommunity trophic construction risks missing key mechanisms that shape predator-prey dynamics (Baiser et al., 2013; Guzman et al., 2019). Simulation models that included spatial dynamics also accurately represented realistic patterns in focal systems like pitcher-plant communities (Baiser et al., 2013). Dispersal in metacommunities has also been demonstrated, both theoretically and empirically, to provide a sort of “spatial insurance” that buffers communities against perturbations by promoting rescue effects and resource complementarity (Loreau et al., 2003; Limberger et al., 2019), and experiments have confirmed that these same spatial insurance effects can lower the invasibility of local communities when invasive species are the “perturbation” (Howeth, 2017). Intermediate levels of dispersal in a metacommunity also maximize food web linkages and species diversity, the latter of which has been shown to be a deterrent for invaders (Beaury et al., 2020).
These early results from a metacommunity approach to food webs have clear implications for the Enemy Release Hypothesis: spatial properties of metacommunities can have large effects on food-web dynamics, and therefore, for how robust a mechanism Enemy Release is likely to be in a particular metacommunity. A strategy for exploring the effect of Enemy Release in a metacommunity context is to examine the dynamics of multi-trophic metacommunities with vs. without predators/parasites. Of particular interest is that intermediate rates of dispersal maximize community diversity and therefore, resistance of a (meta)community to invasion (Beaury et al., 2020). As with Biotic Resistance, a mechanism like Enemy Release that is predicated solely on local effects may have some successes, but is likely missing important mechanistic links in a metacommunity context where dispersal of natives is moderate to high.
One way in which metacommunity theory can be more finely tuned to address issues of invasive species is that most metacommunity models and theory include the general assumption that either dispersal is a stochastic, probabilistic process with regards to which species colonize and at what rate, or dispersal is probabilistically equivalent across all species in a metacommunity (e.g., Loreau et al., 2003; Dallas et al., 2019). However, dispersal has been demonstrated to be distinctly non-random in both food webs (Melián et al., 2015) and in metacommunities (Lowe and McPeek, 2014). Specifically in an invasion context, non-random dispersal can benefit invaders who show distinct “dispersal syndromes” that facilitate invasion (Cote et al., 2017). However, a number of metacommunity models and simulations, allow for flexible dispersal kernels in models, including freely-available simulation packages (e.g., Sokol, 2019) and these platforms can provide an excellent tool for generating and evaluating hypotheses about multi-trophic Enemy Release effects.
Functional Traits
Invasion Biology Perspective
Increasing attention is being paid to functional traits, the morpho-physio-phenological traits that impact fitness (Violle et al., 2007), broadly in community ecology, and especially in invasion biology. Drenovsky et al. (2012) expand the functional trait framework beyond those that affect individual fitness, to include those that play a role in invader abundance and impacts, two defining elements of biological invasions. This attention to traits that influence performance and fitness affords an opportunity to add quantifiable elements in complex systems, again in a search to understand the mechanisms resulting in invasiveness.
In a meta-analysis of plant invaders, Van Kleunen et al. (2010b) found that invasive plants had significantly higher values than natives for six performance-related traits. A complementary analysis found that invasive species show higher phenotypic plasticity than native species across a range of traits (Davidson et al., 2011). A similar approach is being applied to ecosystems by viewing not just the richness/diversity of the community, but the functional trait diversity of the community and its relationship to ecosystem processes (Diaz and Cabido, 2001). This functional trait diversity approach also informs community susceptibility to invasion through “trait space,” the uniqueness of invader traits relative to the breadth of resident traits (Funk et al., 2008). Despite these advances, many challenges remain to fully realize and generalize a functional trait approach in invasion biology and community and ecosystem processes (Funk and Wolf, 2016).
Metacommunity Perspective
Functional traits have played a large role in metacommunity investigation in much the same way they have in invasion biology, and they have been employed in a variety of ways. Many studies use community trait indices as a metric in place of, or in addition to, taxonomic identity to examine how communities are assembled from a functional perspective (Ackerly and Cornwell, 2007; Cadotte et al., 2013; Biswas et al., 2016; Falster et al., 2017; Tolonen et al., 2018), while others have used traits as evidence supporting various community assembly paradigms (e.g., Brown and Swan, 2010; Sokol et al., 2011; Zhang et al., 2015). Trait metrics have also shown predictable relationships with other diversity metrics like α, β, and γ diversity (Patrick and Brown, 2018). Fourth Corner Analysis is a common method in metacommunity studies that simultaneously relates data on species abundances, spatial data, and traits to test trait-environment relationships in a spatial context (Legendre et al., 1997; Dray and Legendre, 2008; Peres-Neto et al., 2012), a method that could prove particularly useful in deciphering the mechanics of invasions by elucidating the relationships between community composition, functional traits, and spatial dynamics driven by dispersal.
The combination of species traits and the multi-scale perspective of metacommunity ecology has great potential for producing insights about the success and effects of invasions. A rapidly growing literature suggests that invader traits, rather than simply species identities, are the best predictors of invasion success (Berg and Ellers, 2010; Van Kleunen et al., 2010a; Davidson et al., 2011; Drenovsky et al., 2012), particularly because traits are best suited for defining the trade-offs that allow invasion success in a particular target community. For example, while high propagule pressure is often associated with invasion success, high propagule pressure alone cannot ensure successful invasion in some systems due to ecological mismatches or innate biotic resistance that ultimately depend on the local-scale traits of the invader like competitive ability and predator/herbivore resistance (Barney et al., 2016). Some investigations have even focused on the composition of target communities in an effort to predict what traits would allow invasion into that system (Moles et al., 2008). Metacommunity approaches can add a layer of explanatory power to these investigations by considering how these same trait combinations of natives and invaders play out in a more complex spatial environment. In a metacommunity context, the trade-offs defined by species traits like competitive ability and propagule pressure exist not just at the local scale, but also within the whole metacommunity. Thus, regional properties—like the regional abundance of invaders or habitat heterogeneity across patches within the metacommunity—can interact with species traits and exert a strong influence over invasion success and effects in any single local community.
A number of perspectives on trade-offs in a multi-scale environment have emerged from the metacommunity literature (e.g., Kneitel and Chase, 2004; Hillebrand et al., 2008). Of these perspectives, Hillebrand et al.'s (2008) investigation of dominance effects is of particular significance. Hillebrand et al. define “dominance” by the distribution of traits within a community, with “dominance” characterized by a lack of trait evenness (2008), and they distinguish two distinct scenarios for dominant species: locally dominant but regionally rare, and both locally and regionally dominant (Hillebrand et al., 2008). From the perspective of the invader, in the case of locally dominant and regionally rare, invader persistence depends on an invader possessing trait combinations that allow it to occupy a local niche and exceed the invasion criterion. However, in a locally and regionally dominant scenario, invader persistence may occur in less advantageous patches through mass effects which can rescue an invader from local extinction. The local/regional dominance perspective can also address biotic resistance of a local community. Theoretically, low dominance in a native community should result in lower invasibility since a higher evenness in community traits should mean that a greater number of niches are filled in community niche space (Hillebrand et al., 2008). These theoretical predictions have been supported empirically (Mwangi et al., 2007; Zavaleta and Hulvey, 2007) with at least one study demonstrating that invasion is actually facilitated by dominance in the native community (Smith et al., 2004).
Human Influence
Invasion Perspective
Unlike many aspects of classic ecology, humans are a fundamental element of invasion. Not only are humans the initial source of invasion, through direct or indirect action, but affect invasion nearly all stages of invasion (Theoharides and Dukes, 2007). As Catford et al. (2009) noted, humans also modify the biotic and abiotic environment of the receiving environment through both direct (e.g., habitat destruction) or indirect (e.g., climate change) actions. And of course, humans are the source of propagule introduction through a diverse variety of pathways. Thus, there can be no realistic discussion of invasive species without considering the influences that humans exert on the specific species that invade ecosystems, the movement of species across large and small spatial distances, and the mechanisms of their invasion.
Metacommunity Perspective
From a metacommunity perspective, humans influence both local and regional processes. Local influence is felt primarily through habitat modification and land-use change, though in more built environments, humans may also exert a strong direct influence over the local species pool through horticultural and agricultural practices (e.g., Knapp et al., 2012; Johnson et al., 2015). Humans also affect regional-scale processes by influencing the dispersal of invasives and augmenting the regional species pool (e.g., Gilroy et al., 2017). Both sets of effects can be accommodated by metacommunity approaches which have a rich history of analytical methods designed to partition the influences of diverse effects on community composition (Leibold and Mikkelson, 2002; Peres-Neto et al., 2006). Despite the recognition of the role humans play in invasion, explicit incorporation of that role is rarely measured as an explicit component of the community assembly process. Assuming human influence can be accurately measured, metacommunity theory and tools provide a number of mechanisms for incorporation of those effects into empirical research, either through implicit incorporation of human elements as covariates in the local/regional partitioning of metacommunity drivers, or though explicit incorporation using methods like variation partitioning (Peres-Neto et al., 2006). These approaches may show not only the clear, direct effect of human agency in invasion, but also reveal much about the interaction between human influence on local conditions like environmental conditions and land use change, and regional factors like human movement of species and introduction of non-natives to the regional species pool.
From a conceptual metacommunity perspective, human involvement in invasions has one major effect on community processes: decoupling the tradeoffs that promote coexistence in communities. Tradeoffs have formed a central tenet of community ecology for decades (MacArthur, 1972), and species coexistence in a community is thought to depend on tradeoffs between traits of species with overlapping niche space (MacArthur, 1972; Tilman, 1982, 2000; Chesson and Huntly, 1997; Grover, 1997). Trade-offs are negatively correlated fitness-related traits in a species, developed through the serially optimizing force of natural selection, constrained by the physiological limits of organic life (Garland, 2014). Tradeoffs impose constraints that prohibit the evolution of “superspecies” and allow for coexistence of species through the employment of alternative tradeoff strategies (Kneitel and Chase, 2004). Common trade-offs in communities include growth rate vs. carry capacity (Pianka, 1970; Boyce, 1984), differential resource use (MacArthur, 1972; Tilman, 1982), predator/herbivore tolerance vs. competitive ability (Holt et al., 1994; Leibold, 1996), and competition vs. colonization (Levins and Culver, 1971; Hastings, 1980). The influence of humans on invasive species effectively decouples traits involved in tradeoffs in two distinct ways. The most obvious is the alleviation of dispersal limitation. For example, given a classic competition vs. colonization tradeoff, a species with high competitive ability would have low rates of colonization/propagule pressure. If that limitation on colonization is removed via human agency, then a species' competitive ability is left unchecked. Humans can also alter the other side of the equation, competitive ability, by selectively promoting or eliminating species. Intentional cultivation of invasive species is common, particularly in built environments and agricultural settings (Johnson and Swan, 2014), and horticulture is a major vehicle for the introduction of invasive species (Reichard and White, 2001). Surveys from non-governmental organizations suggest that possibly as many as 83% of invasive plants in the United States had horticultural origins (Niemiera and Von Holle, 2009). Promoting a species' competitive ability can also occur without intent through the indirect effects of land use changes that favor invasives (e.g., Lenda et al., 2018). In a metacommunity of interconnected patches, the intentional cultivation of invasive species can also have trait-decoupling effects that range far beyond a local patch because maintenance of invasives in the regional species pool not only subverts local competitive hierarchies, but also facilitates the dispersal of invaders into non-target patches. A recent publication from urban ecosystems demonstrates how human activity can be incorporated in a metacommunity framework to holistically describe the factors that structure communities in cities (Andrade et al., 2020). Another prominent example of human activity altering the course of invasions through both local and regional effects is the “bridgehead effect” in which invasions beget additional invasions (Lombaert et al., 2010). While first descriptions of the bridgehead effect postulated that adaptation in the initially invading population was the driver of subsequent invasions, further analysis suggests that a more parsimonious explanation is that human agency either (1) subverts limitations of the competition-colonization tradeoff structure to allow establishment of an invasive population that continues to spread, or (2) that the structure of networks of human transport result in multiple introductions that can exacerbate one another (Bertelsmeier and Keller, 2018), and these mechanisms have been supported by subsequent empirical investigations (e.g., Oficialdegui et al., 2019).
Emergent Invasion Predictions Via Metacommunity Approaches
Translating invasion terms and concepts into the vocabulary of metacommunity theory is a practical first step toward a metacommunity approach to invasive species. However, simply rephrasing well-known concepts has little utility unless new and interesting principles, predictions, and hypotheses emerge. A recurring theme in this paper–and especially in this section—is the importance of a multi-scale perspective. Just as metacommunity theory explicitly incorporates both local and regional scales, invasions should be similarly viewed, moving beyond the focus on single-patch studies that do not incorporate interconnectedness and regional processes. Likewise, we continue to focus not only on a particular invasive species and their traits, but rather on the community into which an invader is attempting to colonize. More so, expanding our approach to the total suite of exotic and native species within and among patches that interact with each other, as well as the local environment. This perspective has been encouraged by previous researchers (e.g., Shea and Chesson, 2002; Preston et al., 2012; Gallien and Carboni, 2017), but its adoption has been sluggish. Here we present several ideas and testable hypotheses that result from a metacommunity approach to invasions, and suggest approaches through which these ideas can be tested. This list is by no means exhaustive and serves more as an illustration of what a metacommunity approach to invasions may accomplish rather than a comprehensive list of ideas.
The Relationship Between Local Sorting Strength and Invasion Success
Two major invasion hypotheses, Biotic Resistance and Enemy Release, implicate local sorting strength in determining invasion success. However, a metacommunity approach strongly suggests that local sorting strength is often only part of the equation when it comes to community assembly, and that regional dispersal-driven factors can be just as important, or more important in structuring communities (Leibold et al., 2004). Previous work on metacommunities has demonstrated that when local sorting strength is the primary driver of community assembly, resultant communities tend to be far more predictable and deterministic (Chase, 2003, 2007). The converse of that result is that when communities are significantly influenced by dispersal-driven dynamics, resulting communities are more stochastic in structure and more permissible to the introduction of new species (Sokol et al., 2017; Brown et al., 2018). Taken together, these suggest a powerful hypothesis about the relationship between local sorting strength and the invasibility of a community: probability of invasion decreases as the importance of local sorting strength increases (Figure 3A).
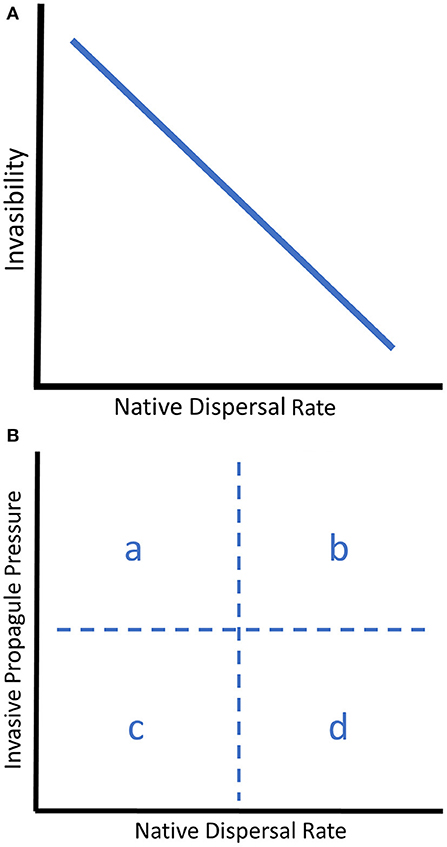
Figure 3. Hypothesized relationships between dispersal and invasibility. (A) Hypothesized effect of native dispersal rate of species in a community on the invasibility of that locality. (B) Four invasion scenarios defined by propagule pressure of a potential invader and dispersal rates of native species. Scenario a represents the highest potential for invasion while scenario d represents the lowest potential for invasion. Scenarios b and c have intermediate potential for invasion and specific outcomes will largely depend on specifics of the local community.
This hypothesis is both imminently testable and useful. A rich history of metacommunity investigation provides both the conceptual foundation and analytical tools for evaluating the relative contributions of local sorting strength and dispersal for community assembly. Experimental approaches are the most powerful for evaluating assembly mechanisms because they allow for direct evaluation of the outcome of assembly processes (e.g., Cadotte, 2006; Chase, 2007; Steiner et al., 2013; Resetarits and Silberbush, 2016; Tornwall et al., 2017; Brown et al., 2018), and when experimental approaches are practical, they are encouraged. Experimental approaches use replicated communities to examine the repeatability of species compositions after assembly, with high levels of repeatability being an indicator of deterministic, niche-based local control (Chase, 2007; Swan and Brown, 2017; Brown et al., 2018). However, experimental approaches may prove intractable, since available data are often the work of surveys outside of a designed context. Nevertheless, these sorts of survey data have been employed in a wide range of metacommunity studies to great effect. While methods to evaluate the relative balance of local vs. regional effects in assembling communities are numerous, two common methodologies are distance-decay and variation partitioning. Distance-decay studies examine how similarity between multiple local communities changes as a function of environmental distance and physical distance (Nekola and White, 1999). Stronger relationships with environmental parameters indicate more local, niche-based control, while strong relationships with distance indicate dispersal-driven dynamics (Chase et al., 2005). These methods have been used to evaluate diverse ranges of communities including stream invertebrates, frogs, microbiota, plants, lentic algae, and internal parasites (Morlon et al., 2008; Brown and Swan, 2010; Diniz-Filho et al., 2012; Warburton et al., 2016; He et al., 2020). Variation partitioning is a method based on constrained ordination that simultaneously uses community composition data (taxonomic or trait-based), spatial data, and environmental data across multiple sites to evaluate the factors that most strongly structure communities (Borcard et al., 1992; Peres-Neto et al., 2006). Similar to distance-decay relationships, the degree of local vs. regional control is inferred based on the relative influences of environmental and spatial variables, but in the case of variation partitioning, statistical significance of the various partitions can be simultaneously evaluated (Peres-Neto et al., 2006). Variation partitioning has been used in a wide range of systems in both an exploratory (e.g., Grönroos et al., 2013; Sokol et al., 2013) and a hypothesis-testing framework (e.g., Cottenie and de Meester, 2003; Resetarits and Silberbush, 2016). It is also worth noting that while the most common predictor matrices in variation partitioning are spatial and environmental variables, other predictor matrices can be incorporated into the analysis if appropriate, such as phylogenetic (Perez Rocha et al., 2018) and functional trait data (De Bie et al., 2012). Any or all of these approaches can be used effectively to evaluate the drivers of community assembly and evaluate risk of invasion.
To be clear, even without assessing the impact of an invasive species, we posit that a community's degree of sorting strength may be a useful predictor of invasion success in that community. Invasive species need not necessarily be involved in the studies, since the focus is on what structures the pre-invasion community, so these studies can be used as assays to evaluate the potential for invasion before invasion occurs in a locality. One caveat for all of these approaches is that they necessarily involve the evaluation of multiple patches within a metacommunity. In metacommunity ecology, there are no single-patch studies since regional scale processes cannot be evaluated from a single locality. Again, this point underscores a major argument of this contribution: that invasion biology can benefit greatly from larger scale or multi-site studies. Different sets of community processes occurring at different scales may also help explain discrepancies in results of studies on Enemy Release and Biotic Resistance. The search for strictly local explanations of species' membership in communities is destined to produce negative or confounded results when assembly is strongly influenced by regional, dispersal-driven dynamics. In fact, the vast majority of studies of community assembly in metacommunities find that, when evaluating the relative influences of local vs. regional processes in structuring communities, there is rarely an either/or explanation and more often than not, the two sets of forces interact (e.g., Forbes and Chase, 2002; Cottenie et al., 2003; De Bie et al., 2012; Göthe et al., 2013). Thus, for both Biotic Resistance and Enemy Release hypotheses, an interaction between local and regional processes is likely, and mechanistic understanding of successful invasion into a community will likely be elusive without considering both sets of forces.
The Importance of Native Dispersal in Invasion Resistance
Higher rates of dispersal do not always result in stochastic community assembly. When local sorting strength is high and dispersal rates are moderate, dispersal can “fuel” deterministic processes by providing a steady source of colonists that are then sorted into largely deterministic communities by local forces (Cottenie and De Meester, 2004). Under this scenario, high rates of native dispersal may also act as a deterrent to invasion by maximizing local species richness (Cadotte, 2006; Grainger and Gilbert, 2016; Figure 3A). Howeth (2017) tested this scenario using zooplankton mesocosms and the invasive cladoceran zooplankton, Daphnia lumholtzi, and found that, while holding environmental conditions constant across treatments, experimentally increasing dispersal of native species decreased rates of invasion of D. lumholtzi. There appear to be two non-exclusive mechanisms contributing to this effect. The first was that higher rates of dispersal produced rescue effects (Brown and Kodric-Brown, 1977) that allowed locally extinct native species to rapidly recolonize. Secondarily, rescue effects resulted in higher species richness of natives in higher-dispersal treatments which resulted in higher niche complementarity, reduced niche space available for the invader, and lower rates of invasion. In the highest dispersal treatments (equating to highest propagule pressure), D. lumholtzi had negative growth rates, indicating that native dispersal had resulted in failure of the invader to meet the Invasibility criterion and therefore not be able to coexist with native species (Howeth, 2017).
However, a high rate of dispersal (i.e., high propagule pressure) is also a mechanism that allows many invasive species to colonize and eventually dominate native communities (Simberloff, 2009). Therefore, operationalizing a native dispersal mechanism for invasion resistance will necessarily involve simultaneous consideration of dispersal of both natives and invaders. Figure 3B illustrates four bookend scenarios when considering both native and invasive dispersal. Assuming similar local environmental conditions across all four scenarios, scenario a—high invader propagule pressure and low native dispersal—would present the highest invasion risk, while scenario d would present the lowest risk of invasion (high native and low invasive dispersal), with scenarios b and c posing intermediate risk.
Native-Invasive Diversity Relationships
One of the most controversial and frustrating topics in invasion biology has been the relationship between native and invasive diversity (the so-called Invasion Paradox; Fridley et al., 2007). The two extreme positions in this controversy are Biotic Resistance, and Biotic Acceptance. We previously defined and discussed Biotic Resistance, and the prediction that emerges from Biotic Resistance is that diversity of native and invasive species should be negatively correlated on a landscape. On the other hand, Biotic Acceptance posits that environmental conditions that are good for natives are also good for invasives, and therefore their abundances should be positively correlated (Stohlgren et al., 2006). While these predictions are straightforward, testable, and easily distinguishable, the issue of scale has complicated a seemingly simple question. At smaller spatial scales, particularly in experimental studies, evidence for Biotic Resistance has been substantial (e.g., Levine et al., 2004; Fridley et al., 2007). In contrast, studies incorporating larger spatial scales have found considerable evidence for Biotic Acceptance (e.g., Stohlgren et al., 2006; Iannone et al., 2016). This difference in results across spatial scales has long been a source of debate for invasion biologists (Shea and Chesson, 2002; Fridley et al., 2007), though some recent work has claimed to resolve this debate in favor of Biotic Resistance by incorporating covariates not included in prior analyses (Beaury et al., 2020), and a global meta-analysis of observational data found no paradox across scales (Peng et al., 2019).
Here we pose another potential explanation for the discrepancy in results across scales, i.e., that different metacommunity processes are being evaluated at different scales. This explanation operates in tandem with our prediction that invasion success will decrease with local sorting strength. At smaller spatial scales—particularly in experiments, which often have limited spatial extent (e.g., Chen et al., 2010; Sandel and Corbin, 2010)—local factors that contribute to sorting strength are readily observable and, in the case of experiments, “localness” may even be strictly maintained. These circumstances create the highest probability of observing local control over community composition, and thus, a decreased probability of invasion success (Figure 5). Larger scale studies tend to be observational. As such, both the lack of control of local conditions and the ability to observe dispersal-driven, regional-scale effects are inherent in study design. Taking these points together, we suggest that the scale related discrepancy in results of native-invasive relationships may be an artifact of study designs, that localized studies, especially experiments, are more likely to identify local sorting strength as a driver of community assembly, while larger scale, less controlled studies capture the dispersal-driven regional processes that are fundamental to many metacommunities (Figure 4).
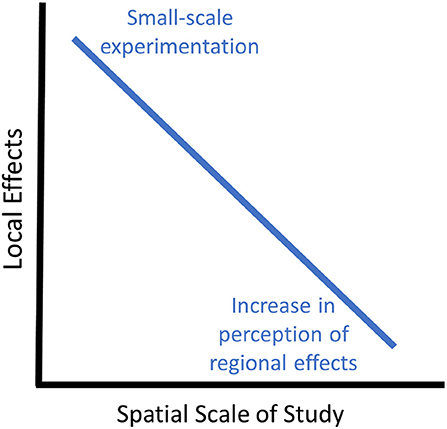
Figure 4. Hypothesized explanation for scale discrepancies in native-invasive relationships. Studies at small spatial scales are only able to perceive local effects and often encourage exacerbated local effects through study design or experimental control. Given the hypothesized relationship between local sorting strength and invasibility (Figure 5), signs of Biotic Resistance are frequently detected. Studies at larger spatial scales or spanning multiple spatial scales are better able to perceive regional-scale effects on community assembly and are more likely to observe positive relationships between native biodiversity and invader biodiversity, especially in heterogeneous landscapes.
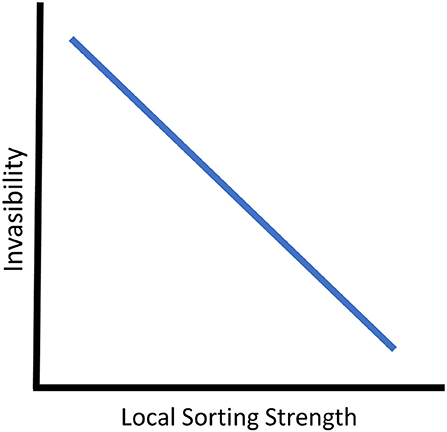
Figure 5. Hypothesized relationship between local sorting strength and invasibility of a local community.
Does this potential explanation offer an answer as to whether Biotic Resistance or Biotic Acceptance should be the dominant paradigm? No. Rather, this idea emphasizes the multi-scale processes that are involved in community assembly and suggests that the search for a single, unvarying answer regarding native-invasive relationships is illogical. As predicted in our first hypothesis, invasibility will likely depend on the relative balance of local sorting strength and dispersal-driven dynamics in any single metacommunity. However, this idea does present testable predictions and potential solutions for resolving this “Invasion Paradox” (Fridley et al., 2007). One imminently testable prediction is that the variability in native-invasive relationships should increase with the spatial scale of study because increasing incorporation of regional-scale effects will introduce stochasticity (Leibold et al., 2004; Chase and Myers, 2011). Another way to address this idea is through controlled experiments or designed studies that span scales and can thus be evaluated for both local and regional community dynamics. In the case of direct experimentation, either dispersal or local conditions could be manipulated across a spatial array of study sites. However, similar goals can be accomplished in well-designed survey studies that use observation of communities across spatially arranged sites.
Applications of a Metacommunity Approach to Mitigate Invasions
One of the most intriguing outcomes of integrating metacommunity concepts into invasion biology is the possibility for improved management, which has been a long-term goal of the field. Invasion biologists have long posited that enhanced understanding of basic invasion biology, ecology, and the underlying causes and consequences of invasiveness would collectively lead to our ability to mitigate the current invasion threat and limit future invasion. There are certainly success stories, including weed risk assessment (WRA, Pheloung et al., 1999) and fish invasiveness scoring kit (FISK, Copp et al., 2005), which were designed to identify invasive weed and fishes, pre-introduction, to minimize new invaders. Despite these limited successes, the number of new species introductions continues seemingly unabated (Seebens et al., 2017), necessitating continued development of theory and practice that mitigate current invasion impacts and limit future spread of invaders. We contend that application of the metacommunity concepts outlined above present several key opportunities to enhance invasive species management.
Dispersal Disruption of Invasive Species
Dispersal and propagule pressure are fundamental aspects of invasion that most clearly lend themselves to mitigation. Since invasion is contingent on movement of propagules, disrupting the production and movement of propagules would result in immediate benefits. For example, with emergence of devastating herbicide resistant agricultural weeds, many of which are spread through seed and equipment contamination, many farmers are adopting a “no weed seed” approach that is designed to prevent weed seed production, diversifying weed management tactics, eliminating pollen production, and equipment sanitation (e.g., Norsworthy et al., 2012; Riar et al., 2016). However, effective disruption must consider the interconnectedness of patches, as it is clear that regional propagule dispersal can “rescue” local extinction. Thus, propagules should be managed in a broader spatial context.
Dispersal disruption is also a powerful, yet underutilized tool, to mitigate the spread of invasive species. This technique is currently being recommended for limiting the spread of wavyleaf basketgrass in the Mid-Atlantic US through land management practices and limitations on access to hikers and hunters. The seeds of wavyleaf basketgrass can stick to animals and clothes, easily transporting propagules over large distances (Beauchamp, 2014). Thus, dispersal disruption tactics of avoiding infested areas during seed production, or thoroughly cleaning clothes and equipment have been recommended (Swearingen et al., 2014). This tactic is particularly useful when the introduction/dispersal pathway of the invasive species is known. For example, live bait was identified as a common dispersal pathway in many freshwater systems, and has been targeted to reduce use and release of exotic live bait (Kilian et al., 2012). Recent work has also demonstrated variability in the efficacy of propagule interception using simulation modeling (Latombe et al., 2020). Of course, the management of propagules is not new, but the metacommunity perspective warrants clear identification of patch interconnectedness and dispersal pathways, identifying invasive species functional traits enhancing fitness, as well as an understanding of the dispersal of resident native species as well as invaders and parsing the effects on community dynamics of each.
Community Risk Assessment
Perhaps the most unique management suggestion that emerges from this work is using assessment of native communities as a way to evaluate invasion risk. Risk assessment is not new to invasion biology, and has been used with no small degree of success (Keller et al., 2007). However, current risk assessments focus on the particular invasive species of interest, rather than on the community into which an invader may attempt to colonize. Our invasibility x sorting strength prediction (Figure 5), derived from principles of metacommunity ecology, suggests that understanding the assembly mechanisms of an extant metacommunity will provide strong evidence for how invasible that community will be. Communities strongly influenced by regional forces like dispersal should be more vulnerable to invasion, while metacommunities more influenced by local controls should resist invasion. As previously described, metacommunity ecology provides a powerful conceptual framework and a large range of analytical tools for making such evaluations. We should stress, however, that while our prediction emerges as a natural consequence of metacommunity theory, it is still just a prediction and requires empirical evaluation prior to any attempts to operationalize it as a management strategy.
Resilient Communities
The relationship between native and exotic richness remains empirically equivocal, but tactics that enhance native species abundance, dispersal, and connectivity are likely to enhance community resilience to invasive species. Management actions that encourage native species may be more effective than eradicating invasions, the latter of which is relatively rare and often expensive and limited to small infestations (Rejmanek and Pitcairn, 2002). Managing for native-rich assemblages should be holistic and multi-scale in nature to manage for both local and regional effects. Management efforts that focus only on local effects (e.g., species/environment interactions) are destined to be ineffective if community assembly is driven by regional scale properties.
One example of application of this approach is the ecologically-based invasive plant management on rangelands, which Krueger-Mangold et al. (2006) describe as incorporating strategies that encourage desirable plant communities and simultaneously disfavor invasive species. This “successional-based” strategy incorporates many of the elements of metacommunity approaches including local and regional processes, species functional traits, and propagule dispersal. Building resilient communities should comprise enhancing local conditions that favor native species and disfavor invasive species, limiting invasive species propagule production, and enhancing native species connectivity. Achieving this will be an inherently system and community-specific approach, and will require knowledge of species functional traits, local environmental conditions, and regional connectedness and processes.
We have predicted—and empirical evidence suggests (Krueger-Mangold et al., 2006)—that native dispersal can be a deterrent to invasions (Figure 3). Considering how this prediction can be applied to management, the clear theme that emerges is that managing to support native species may be as effective as attempting to directly reduce invasives. This idea is not new and several previous researchers have suggested that the management of natives may be the most effective way to manage invasions (e.g., Sheley et al., 1996; Krueger-Mangold et al., 2006). However, a nuance suggested by our prediction is that facilitating dispersal of natives should be a priority. This proposal could be viewed as the native equivalent of the invasion cliff posited by Davis (2009) above, with native success being proportional to propagule load. How can native dispersal be encouraged? One possible answer is habitat connectivity. Enhanced connectivity has long been associated with higher local diversity in both theoretical and empirical studies (e.g., Horn and MacArthur, 1972; Brown and Kodric-Brown, 1977; Tilman, 1994; Holyoak and Lawler, 1996; Hanski, 1998; Chesson, 2000; Amarasekare and Nisbet, 2001), and conservation efforts have found success in managing biodiversity through increasing connectivity, at least when connectivity management is successfully implemented (reviewed in Correa Ayram et al., 2016; Keeley et al., 2019). However, connectivity management should also be mindful of the effects of the interaction between connectivity and habitat heterogeneity in which high connectivity can lead to biotic homogenization, especially when environments are relatively homogeneous (Forbes and Chase, 2002; Strecker and Brittain, 2017). In addition, we also want to inject a note of caution. While managing for dispersal abilities of native species should be an effective means of mitigating invasive species' impacts, we do not advocate for human-enhanced dispersal of native species. Such activities could have a number of unintended consequences, including the unintentional movement of invasives. But perhaps more importantly, enhanced dispersal of natives has the potential to repeat the mistakes created by human involvement in invasive species, i.e., decoupling tradeoffs that naturally limit the distributions and abundances of species.
Nativity and Range-Expanding Species
An emerging issue in invasion biology is the rapid increase in species that expand their ranges as a result of human-induced environmental change (Essl et al., 2019). These species, termed “neonative” by Essl et al. (2019), are distinguished from other invasive species in that they expand their ranges without the aid of direct human agency, but do so as an indirect consequence of human induced environmental change. While the concept of neonativity has been criticized and declared a non-useful concept for a number of reasons, even its critics acknowledge that a large number of species of this type exist, and that many are problematic (Wilson, 2020). Vocabulary is often a sticking point in science, and the vocabulary of many concepts has been hotly debated, from community stability (Grimm and Wissel, 1997), to definitions of β-diversity (Anderson et al., 2011), to how to define an ecosystem (O'Neill, 2001). Invasion biology is no exception to this common mania (Richardson et al., 2000; Colautti and MacIsaac, 2004; Colautti and Richardson, 2009).
Defining invasive species will always be a necessity, particularly for regulation and management, but we suggest that a shift of focus from specific invasive species to communities of interest will move the conversation from identifying and defining invaders, to examining effects of invaders. While this perspective of invasives as just another species in a community may seem shortsighted and to downplay the importance of invaders, we contend that invaders are important for their effects on communities, not solely for their identities as invaders. It would also be unjust to suggest that metacommunity approaches can't or don't single out particular species for their effects in a metacommunity. One common prediction in many metacommunity models is that high rates of dispersal will allow competitively dominant species to reach all local communities in a metacommunity, resulting in biotic homogenization (Mouquet and Loreau, 2003; Mouquet et al., 2005), a prediction that has also been empirically verified in a number of studies (e.g., Forbes and Chase, 2002; Livingston et al., 2012). Thus, while a major focus of an investigation of an invaded metacommunity may be on the community as a whole, invaders can still be singled out and examined for their specific effects.
Conclusion
Invasion Biology has long been a field that sits at the intersection of basic and applied science and has been exemplary in the pursuit of converting theory and concept to practice. However, invasion is a community ecology problem, and community ecology has experienced a paradigm shift in recent years. Metacommunity theory and approaches have revolutionized the field of community ecology, but their adoption into the field of Invasion Biology has been slow. Here we have illustrated how some of the most fundamental concepts of Invasion Biology can be viewed through a metacommunity lens, demonstrated that this change in perspective can produce useful predictions regarding invasions, and illustrated how those concepts can be directly applied to management. Likewise, we encourage the metacommunity scientists to examine invasions as interesting and useful study systems to explore their theory and concepts. We stress that our treatment of invasion concepts is not exhaustive and serve more as illustration of potential than a comprehensive guide to the metacommunity ecology of invasions. Two pervasive themes that we repeatedly visit are the potential benefits of focusing on communities rather than on specific invaders, and the need for a multi-scale approach to Invasion Biology. Our hope is that this contribution will catalyze thought and provide a starting point for investigations that successfully wed Invasion Biology with metacommunity approaches.
Data Availability Statement
The original contributions presented in the study are included in the article/supplementary materials, further inquiries can be directed to the corresponding author/s.
Author Contributions
BB and JB were involved in conceptual development, writing, and editing. Both authors contributed to the article and approved the submitted version.
Funding
JB was partially supported by the College of Agriculture and Life Sciences. This work was partially a result of the Virginia Tech Invasive Species Working Group.
Conflict of Interest
The authors declare that the research was conducted in the absence of any commercial or financial relationships that could be construed as a potential conflict of interest.
Acknowledgments
We greatly appreciate the feedback from David Haak, Meryl Mims, and the reviewers whose comments on a previous draft greatly improved the outcome.
References
Ackerly, D. D., and Cornwell, W. K. (2007). A trait-based approach to community assembly: partitioning of species trait values into within- and among-community components. Ecol. Lett. 10, 135–145. doi: 10.1111/j.1461-0248.2006.01006.x
Altermatt, F., and Fronhofer, E. A. (2018). Dispersal in dendritic networks: ecological consequences on the spatial distribution of population densities. Freshw. Biol. 63, 22–32. doi: 10.1111/fwb.12951
Amarasekare, P., and Nisbet, R. M. (2001). Spatial heterogeneity, source-sink dynamics, and the local coexistence of competing species. Am. Nat. 158, 572–584. doi: 10.1086/323586
Anderson, M. J., Crist, T. O., Chase, J. M., Vellend, M., Inouye, B. D., Freestone, A. L., et al. (2011). Navigating the multiple meanings of β diversity: a roadmap for the practicing ecologist. Ecol. Lett. 14, 19–28. doi: 10.1111/j.1461-0248.2010.01552.x
Andrade, R., Franklin, J., Larson, K. L., Swan, C. M., Lerman, S. B., Bateman, H. L., et al. (2020). Predicting the assembly of novel communities in urban ecosystems. Landsc. Ecol. doi: 10.1007/s10980-020-01142-1
Baiser, B., Buckley, H. L., Gotelli, N. J., and Ellison, A. M. (2013). Predicting food-web structure with metacommunity models. Oikos 122, 492–506. doi: 10.1111/j.1600-0706.2012.00005.x
Barney, J. N., Ho, M. W., and Atwater, D. Z. (2016). Propagule pressure cannot always overcome biotic resistance: the role of density-dependent establishment in four invasive species. Weed Res. 56, 208–218. doi: 10.1111/wre.12204
Barney, J. N., and Whitlow, T. H. (2008). A unifying framework for biological invasions: the state factor model. Biol. Invasions 10, 259–272. doi: 10.1007/s10530-007-9127-8
Beaury, E. M., Finn, J. T., Corbin, J. D., Barr, V., and Bradley, B. A. (2020). Biotic resistance to invasion is ubiquitous across ecosystems of the United States. Ecol. Lett. 23, 476–482. doi: 10.1111/ele.13446
Berg, M., and Ellers, J. (2010). Trait plasticity in species interactions: a driving force of community dynamics. Evol. Ecol. 24, 617–629. doi: 10.1007/s10682-009-9347-8
Bertelsmeier, C., and Keller, L. (2018). Bridgehead effects and role of adaptive evolution in invasive populations. Trends Ecol. Evol. 33, 527–534. doi: 10.1016/j.tree.2018.04.014
Biswas, S. R., Mallik, A. U., Braithwaite, N. T., and Wagner, H. H. (2016). A conceptual framework for the spatial analysis of functional trait diversity. Oikos 125, 192–200. doi: 10.1111/oik.02277
Blanchet, F. G., Cazelles, K., and Gravel, D. (2020). Co-occurrence is not evidence of ecological interactions. Ecol. Lett. 23, 1050–1063. doi: 10.1111/ele.13525
Blossey, B., and Notzold, R. (1995). Evolution of increased competitive ability in invasive nonindigenous plants: a hypothesis. J. Ecol. 83, 887–889. doi: 10.2307/2261425
Borcard, D., Legendre, P., and Drapeau, P. (1992). Partialling out the spatial component of ecological variation. Ecology 73, 1045–1055. doi: 10.2307/1940179
Boyce, M. S. (1984). Restitution of r- and K-selection as a model of density-dependent natural selection. Annu. Rev. Ecol. Syst. 15, 427–447. doi: 10.1146/annurev.es.15.110184.002235
Brown, B. L., Sokol, E. R., Skelton, J., and Tornwall, B. (2017). Making sense of metacommunities: dispelling the mythology of a metacommunity typology. Oecologia 183, 643–652. doi: 10.1007/s00442-016-3792-1
Brown, B. L., and Swan, C. M. (2010). Dendritic network structure constrains metacommunity properties in riverine ecosystems. J. Anim. Ecol. 79, 571–580. doi: 10.1111/j.1365-2656.2010.01668.x
Brown, B. L., Wahl, C., and Swan, C. M. (2018). Experimentally disentangling the influence of dispersal and habitat filtering on benthic invertebrate community structure. Freshw. Biol. 63, 48–61. doi: 10.1111/fwb.12995
Brown, J. H., and Kodric-Brown, A. (1977). Turnover rates in insular biogeography: effect of immigration on extinction. Ecology 58, 445–449. doi: 10.2307/1935620
Büchi, L., and Vuilleumier, S. (2014). Coexistence of specialist and generalist species is shaped by dispersal and environmental factors. Am. Nat. 183, 612–624. doi: 10.1086/675756
Cadotte, M., Albert, C. H., and Walker, S. C. (2013). The ecology of differences: assessing community assembly with trait and evolutionary distances. Ecol. Lett. 16, 1234–1244. doi: 10.1111/ele.12161
Cadotte, M. W. (2006). Metacommunity influences on community richness at multiple spatial scales: a microcosm experiment. Ecology 87, 1008–1016. doi: 10.1890/0012-9658(2006)87[1008:MIOCRA]2.0.CO;2
Calcagno, V., Mouquet, N., Jarne, P., and David, P. (2006). Coexistence in a metacommunity: the competition-colonization trade-off is not dead. Ecol. Lett. 9, 897–907. doi: 10.1111/j.1461-0248.2006.00930.x
Cárdenas, L., Leclerc, J.-C., Bruning, P., Garrido, I., Détrée, C., Figueroa, A., et al. (2020). First mussel settlement observed in Antarctica reveals the potential for future invasions. Sci. Rep. 10:5552. doi: 10.1038/s41598-020-62340-0
Carrara, F., Altermatt, F., Rodriguez-Iturbe, I., and Rinaldo, A. (2012). Dendritic connectivity controls biodiversity patterns in experimental metacommunities. Proc. Natl. Acad. Sci. U.S.A. 109, 5761–5766. doi: 10.1073/pnas.1119651109
Catford, J. A., Jansson, R., and Nilsson, C. (2009). Reducing redundancy in invasion ecology by integrating hypotheses into a single theoretical framework. Divers. Distribut. 15, 22–40. doi: 10.1111/j.1472-4642.2008.00521.x
Chao, A., Chiu, C.-H., and Hsieh, T. C. (2012). Proposing a resolution to debates on diversity partitioning. Ecology 93, 2037–2051. doi: 10.1890/11-1817.1
Chase, J. M. (2003). Community assembly: when should history matter? Oecologia 136, 489–498. doi: 10.1007/s00442-003-1311-7
Chase, J. M. (2007). Drought mediates the importance of stochastic community assembly. Proc. Natl. Acad. Sci. U.S.A. 104, 17430–17434. doi: 10.1073/pnas.0704350104
Chase, J. M. (2010). Stochastic community assembly causes higher biodiversity in more productive environments. Science 328, 1388–1391. doi: 10.1126/science.1187820
Chase, J. M., Amarasekare, P., Cottenie, K., Gonzalez, A., Holt, R. D., Holyoak, M., et al. (2005). “Competing theories for competitive metacommunities,” in Metacommunities: Spatial Dynamics and Ecological Communities, eds M. Holyoak, M. A. Leibold, and R. D. Holt (Chicago, IL; London: University of Chicago Press), 335–354.
Chase, J. M., and Myers, J. A. (2011). Disentangling the importance of ecological niches from stochastic processes across scales. Philos. Trans. R. Soc. Lond. B Biol. Sci. 366, 2351–2363. doi: 10.1098/rstb.2011.0063
Chen, H., Qian, H., Spyreas, G., and Crossland, M. (2010). BIODIVERSITY RESEARCH: native-exotic species richness relationships across spatial scales and biotic homogenization in wetland plant communities of Illinois, USA. Divers. Distribut. 16, 737–743. doi: 10.1111/j.1472-4642.2010.00679.x
Chesson, P., and Huntly, N. (1997). The roles of harsh and fluctuating conditions in the dynamics of ecological communities. Am. Nat. 150, 519–553. doi: 10.1086/286080
Chesson, P. L. (2000). Mechanisms of maintenance of species diversity. Annu. Rev. Ecol. Syst. 31, 343–366. doi: 10.1146/annurev.ecolsys.31.1.343
Chwedorzewska, K. J., Giełwanowska, I., Olech, M., Molina-Montenegro, M. A., Wódkiewicz, M., and Galera, H. (2015). Poa annua L. in the maritime antarctic: an overview. Polar Rec. 51, 637–643. doi: 10.1017/S0032247414000916
Colautti, R. I., Grigorovich, I. A., and MacIsaac, H. J. (2006). Propagule pressure: a null model for biological invasions. Biol. Invasions 8, 1023–1037. doi: 10.1007/s10530-005-3735-y
Colautti, R. I., and MacIsaac, H. J. (2004). A neutral terminology to define ‘invasive’ species. Divers. Distribut. 10, 135–141. doi: 10.1111/j.1366-9516.2004.00061.x
Colautti, R. I., and Richardson, D. M. (2009). Subjectivity and flexibility in invasion terminology: too much of a good thing? Biol. Invasions 11, 1225–1229. doi: 10.1007/s10530-008-9333-z
Copp, G. H., Garthwaite, R., and Gozlan, R. E. (2005). Risk identification and assessment of non-native freshwater fishes: a summary of concepts and perspectives on protocols for the UK. J. Appl. Ichthyol. 21, 371–373. doi: 10.1111/j.1439-0426.2005.00692.x
Correa Ayram, C. A., Mendoza, M. E., Etter, A., and Salicrup, D. R. P. (2016). Habitat connectivity in biodiversity conservation: a review of recent studies and applications. Progr. Phys. Geogr. 40, 7–37. doi: 10.1177/0309133315598713
Cote, J., Brodin, T., Fogarty, S., and Sih, A. (2017). Non-random dispersal mediates invader impacts on the invertebrate community. J. Anim. Ecol. 86, 1298–1307. doi: 10.1111/1365-2656.12734
Cottenie, K. (2005). Integrating environmental and spatial processes in ecological community dynamics. Ecol. Lett. 8, 1175–1182. doi: 10.1111/j.1461-0248.2005.00820.x
Cottenie, K., and de Meester, L. (2003). Connectivity and cladoceran species richness in a metacommunity of shallow lakes. Freshw. Biol. 48, 823–832. doi: 10.1046/j.1365-2427.2003.01050.x
Cottenie, K., and De Meester, L. (2004). Metacommunity structure: synergy of biotic interactions as selective agents and dispersal as fuel. Ecology 85, 114–119. doi: 10.1890/03-3004
Cottenie, K., Michels, E., Nuytten, N., and DeMeester, L. (2003). Zooplankton metacommunity structure: regional vs. local processes in highly interconnected ponds. Ecology 84, 991–1000. doi: 10.1890/0012-9658(2003)084[0991:ZMSRVL]2.0.CO;2
Crystal-Ornelas, R., and Lockwood, J. L. (2020). The ‘known unknowns’ of invasive species impact measurement. Biol. Invasions 22, 1513–1525. doi: 10.1007/s10530-020-02200-0
Dallas, T., Melbourne, B. A., and Hastings, A. (2019). When can competition and dispersal lead to checkerboard distributions? J. Anim. Ecol. 88, 269–276. doi: 10.1111/1365-2656.12913
Datry, T., Bonada, N., and Heino, J. (2016). Towards understanding the organisation of metacommunities in highly dynamic ecological systems. Oikos 125, 149–159. doi: 10.1111/oik.02922
Davidson, A. M., Jennions, M., and Nicotra, A. B. (2011). Do invasive species show higher phenotypic plasticity than native species and, if so, is it adaptive? A meta-analysis. Ecol. Lett. 14, 419–431. doi: 10.1111/j.1461-0248.2011.01596.x
De Bie, T., De Meester, L., Brendonck, L., Martens, K., Goddeeris, B., Ercken, D., et al. (2012). Body size and dispersal mode as key traits determining metacommunity structure of aquatic organisms. Ecol. Lett. 15, 740–747. doi: 10.1111/j.1461-0248.2012.01794.x
Diaz, S., and Cabido, M. (2001). Vive la différence: plant functional diversity matters to ecosystem processes. Trends Ecol. Evol. 16, 646–655. doi: 10.1016/S0169-5347(01)02283-2
Diniz-Filho, J. A. F., Siqueira, T., Padial, A. A., Rangel, T. F., Landeiro, V. L., and Bini, L. M. (2012). Spatial autocorrelation analysis allows disentangling the balance between neutral and niche processes in metacommunities. Oikos 121, 201–210. doi: 10.1111/j.1600-0706.2011.19563.x
Dray, S., and Legendre, P. (2008). Testing the species traits-environment relationships: the fourth-corner problem revisited. Ecology 89, 3400–3412. doi: 10.1890/08-0349.1
Drenovsky, R. E., Grewell, B. J., D'Antonio, C. M., Funk, J. L., James, J. J., Molinari, N., et al. (2012). A functional trait perspective on plant invasion. Ann. Bot. 110, 141–153. doi: 10.1093/aob/mcs100
Economo, E. P., and Keitt, T. H. (2008). Species diversity in neutral metacommunities: a network approach. Ecology Letters 10, 52–62. doi: 10.1111/j.1461-0248.2007.01126.x
Elton, C. S. (1958). The Ecology of Invasions by Animals and Plants. Methuen, MA; London: Chapman and Hall Ltd. doi: 10.1007/978-1-4899-7214-9
Enders, M., Havemann, F., Ruland, F., Bernard-Verdier, M., Catford, J. A., Gómez-Aparicio, L., et al. (2020). A conceptual map of invasion biology: integrating hypotheses into a consensus network. Glob. Ecol. Biogeogr. 29, 978–991. doi: 10.1111/geb.13082
Essl, F., Dullinger, S., Genovesi, P., Hulme, P. E., Jeschke, J. M., Katsanevakis, S., et al. (2019). A conceptual framework for range-expanding species that track human-induced environmental change. BioScience. 69, 908–919. doi: 10.1093/biosci/biz101
Falster, D. S., Brännström, Å., Westoby, M., and Dieckmann, U. (2017). Multitrait successional forest dynamics enable diverse competitive coexistence. Proc. Natl. Acad. Sci. U.S.A. 114, E2719–E2728. doi: 10.1073/pnas.1610206114
Forbes, A. E., and Chase, J. M. (2002). The role of habitat connectivity and landscape geometry in experimental zooplankton metacommunities. Oikos 96, 433–440. doi: 10.1034/j.1600-0706.2002.960305.x
Fridley, J. D. (2010). “Biodiversity as a bulwark against invasion: conceptual threads since elton,” in Fifty Years of Invasion Ecology, ed D. M. Richardson (Oxford: Blackwell), 121–130. doi: 10.1002/9781444329988.ch10
Fridley, J. D., Stachowicz, J. J., Naeem, S., Sax, D. F., Seabloom, E. W., Smith, M. D., et al. (2007). The invasion paradox: reconciling pattern and process in species invasions. Ecology 88, 3–17. doi: 10.1890/0012-9658(2007)88[3:TIPRPA]2.0.CO;2
Frisch, D., Cottenie, K., Badosa, A., and Green, A. J. (2012). Strong spatial influence on colonization rates in a pioneer zooplankton metacommunity. PLoS ONE 7:e40205. doi: 10.1371/journal.pone.0040205
Funk, J. L., Cleland, E. E., Suding, K. N., and Zavaleta, E. S. (2008). Restoration through reassembly: plant traits and invasion resistance. Trends Ecol. Evol. 23, 695–703. doi: 10.1016/j.tree.2008.07.013
Funk, J. L., and Wolf, A. A. (2016). Testing the trait-based community framework: do functional traits predict competitive outcomes? Ecology 97, 2206–2211. doi: 10.1002/ecy.1484
Gallien, L., and Carboni, M. (2017). The community ecology of invasive species: where are we and what's next? Ecography 40, 335–352. doi: 10.1111/ecog.02446
Gilroy, J. J., Avery, J. D., and Lockwood, J. L. (2017). Seeking international agreement on what it means to be “Native”. Conserv. Lett. 10, 238–247. doi: 10.1111/conl.12246
Göthe, E., Angeler, D. G., and Sandin, L. (2013). Metacommunity structure in a small boreal stream network. J. Anim. Ecol. 82, 449–458. doi: 10.1111/1365-2656.12004
Grainger, T. N., and Gilbert, B. (2016). Dispersal and diversity in experimental metacommunities: linking theory and practice. Oikos 125, 1213–1223. doi: 10.1111/oik.03018
Gravel, D., Canard, E., Guichard, F., and Mouquet, N. (2011). Persistence increases with diversity and connectance in trophic metacommunities. PLoS ONE 6:e19374. doi: 10.1371/journal.pone.0019374
Grimm, V., and Wissel, C. (1997). Babel, or the ecological stability discussions: an inventory and analysis of terminology and a guide for avoiding confusion. Oecologia 109, 323–334. doi: 10.1007/s004420050090
Grönroos, M., Heino, J., Siqueira, T., Landeiro, V. L., Kotanen, J., and Bini, L. M. (2013). Metacommunity structuring in stream networks: roles of dispersal mode, distance type, and regional environmental context. Ecol. Evol. 3, 4473–4487. doi: 10.1002/ece3.834
Gurevitch, J., Fox, G. A., Wardle, G., Inderjit, M., and Taub, D. (2011). Emergent insights from the synthesis of conceptual frameworks for biological invasions. Ecol. Lett. 14, 407–418. doi: 10.1111/j.1461-0248.2011.01594.x
Guzman, L. M., Germain, R. M., Forbes, C., Straus, S., O'Connor, M. I., Gravel, D., et al. (2019). Towards a multi-trophic extension of metacommunity ecology. Ecol. Lett. 22, 19–33. doi: 10.1111/ele.13162
Hastings, A. (1980). Disturbance, coexistence, history, and competition for space. Theor. Popul. Biol. 18, 363–373. doi: 10.1016/0040-5809(80)90059-3
He, S., Soininen, J., Chen, K., and Wang, B. (2020). Environmental factors override dispersal-related factors in shaping diatom and macroinvertebrate communities within stream networks in China. Front. Ecol. Evol. 8:141. doi: 10.3389/fevo.2020.00141
Heino, J. (2011). A macroecological perspective of diversity patterns in the freshwater realm. Freshw. Biol. 56, 1703–1722. doi: 10.1111/j.1365-2427.2011.02610.x
Heino, J., Melo, A. S., Siqueira, T., Soininen, J., Valanko, S., and Bini, L. M. (2015). Metacommunity organisation, spatial extent and dispersal in aquatic systems: patterns, processes and prospects. Freshw. Biol. 60, 845–869. doi: 10.1111/fwb.12533
Heino, J., and Mykrä, H. (2008). Control of stream insect assemblages: roles of spatial configuration and local environmental factors. Ecol. Entomol. 33, 614–622. doi: 10.1111/j.1365-2311.2008.01012.x
Hillebrand, H., Bennett, D. M., and Cadotte, M. W. (2008). Consequences of dominance: a review of evenness effects on local and regional ecosystem processes. Ecology 89, 1510–1520. doi: 10.1890/07-1053.1
Holbrook, J. B. (2013). What is interdisciplinary communication? Reflections on the very idea of disciplinary integration. Synthese 190, 1865–1879. doi: 10.1007/s11229-012-0179-7
Holt, R. D. (1977). Predation, apparent competition and the structure of prey communities. Theor. Popul. Biol. 12, 197–229. doi: 10.1016/0040-5809(77)90042-9
Holt, R. D., Grover, J., and Tilman, D. (1994). Simple rules for interspecific dominance in systems with exploitative and apparent competition. Am. Nat. 144, 741–771. doi: 10.1086/285705
Holyoak, M., and Lawler, S. P. (1996). Persistence of an extinction-prone predator-prey interaction through metapopulation dynamics. Ecology 77, 1867–1879. doi: 10.2307/2265790
Holyoak, M., Leibold, M. A., and Holt, R. D. (2005). Metacommunities: Spatial Dynamics and Ecological Communities, 1 Edn. Chicago, IL and London: University of Chicago Press.
Horn, H. S., and MacArthur, R. H. (1972). Competition among fugitive species in a harlequin environment. Ecology 53, 749–752. doi: 10.2307/1934797
Howeth, J. G. (2017). Native species dispersal reduces community invasibility by increasing species richness and biotic resistance. J. Anim. Ecol. 86, 1380–1393. doi: 10.1111/1365-2656.12733
Howeth, J. G., Derry, A. M., and Reitzel, A. M. (2010). “Metacommunity biology as an eco-evolutionary framework for understanding exotic invasion in aquatic ecosystems,” in Eco-DAS VIII Symposium. American Society of Limnology and Oceanography (American Society of Limnology and Oceanography), 93–109.
Hubbell, S. P. (2001). The Unified Neutral Theory of Biodiversity and Biogeography. Princeton, NJ: Princeton University Press.
Hui, C., and Richardson, D. M. (2017). “Chapter 8: Community Assembly and Succession,” in Invasion Dynamics (Oxford: Oxford University Press), 191–221. doi: 10.1093/acprof:oso/9780198745334.003.0008
Hulme, P. E., Pyšek, P., Jarošík, V., Pergl, J., Schaffner, U., and Vilà, M. (2013). Bias and error in understanding plant invasion impacts. Trends Ecol. Evol. 28, 212–218. doi: 10.1016/j.tree.2012.10.010
Hunt, J. J. F. G., and Bonsall, M. B. (2009). The effects of colonization, extinction and competition on co-existence in metacommunities. J. Anim. Ecol. 78, 866–879. doi: 10.1111/j.1365-2656.2009.01532.x
Hutchinson, G. E. (1959). Homage to santa rosalia or why are there so many kinds of animals? Am. Nat. 63, 145–159. doi: 10.1086/282070
Iannone, B. V., Potter, K. M., Hamil, K.-A. D., Huang, W., Zhang, H., Guo, Q., et al. (2016). Evidence of biotic resistance to invasions in forests of the Eastern USA. Landsc. Ecol. 31, 85–99. doi: 10.1007/s10980-015-0280-7
Jamoneau, A., Chabrerie, O., Closset-Kopp, D., and Decocq, G. (2012). Fragmentation alters beta-diversity patterns of habitat specialists within forest metacommunities. Ecography 35, 124–133. doi: 10.1111/j.1600-0587.2011.06900.x
Jarić, I., and Cvijanović, G. (2012). The tens rule in invasion biology: measure of a true impact or our lack of knowledge and understanding? Environ. Manage. 50, 979–981. doi: 10.1007/s00267-012-9951-1
Jeschke, J., Gómez Aparicio, L., Haider, S., Heger, T., Lortie, C., Pyšek, P., et al. (2012). Support for major hypotheses in invasion biology is uneven and declining. NeoBiota 14, 1–20. doi: 10.3897/neobiota.14.3435
Johnson, A. L., and Swan, C. M. (2014). “Drivers of vegetation species diversity and composition in urban ecosystems,” in Urban Wildlife Conservation: Theory and Practice, eds R. A. McCleery, C. E. Moorman, and M. N. Peterson (Boston: Springer), 75–90. doi: 10.1007/978-1-4899-7500-3_5
Johnson, A. L., Tauzer, E. C., and Swan, C. M. (2015). Human legacies differentially organize functional and phylogenetic diversity of urban herbaceous plant communities at multiple spatial scales. Appl. Vegetation Sci. 18, 513–527. doi: 10.1111/avsc.12155
Jost, L. (2007). Partitioning diversity into independent alpha and beta components. Ecology 88, 2427–2439. doi: 10.1890/06-1736.1
Keane, R. M., and Crawley, M. J. (2002). Exotic plant invasions and the enemy release hypothesis. Trends Ecol. Evol. 17, 164–170. doi: 10.1016/S0169-5347(02)02499-0
Keeley, A. T. H., Beier, P., Creech, T., Jones, K., Jongman, R. H. G., Stonecipher, G., et al. (2019). Thirty years of connectivity conservation planning: an assessment of factors influencing plan implementation. Environ. Res. Lett. 14:103001. doi: 10.1088/1748-9326/ab3234
Keller, R. P., Lodge, D. M., and Finnoff, D. C. (2007). Risk assessment for invasive species produces net bioeconomic benefits. Proc. Natl. Acad. Sci. U.S.A. 104, 203–207. doi: 10.1073/pnas.0605787104
Kilian, J. V., Klauda, R. J., Widman, S., Kashiwagi, M., Bourquin, R., Weglein, S., et al. (2012). An assessment of a bait industry and angler behavior as a vector of invasive species. Biol. Invasions 14, 1469–1481. doi: 10.1007/s10530-012-0173-5
Knapp, S., Dinsmore, L., Fissore, C., Hobbie, S. E., Jakobsdottir, I., Kattge, J., et al. (2012). Phylogenetic and functional characteristics of household yard floras and their changes along an urbanization gradient. Ecology 93, S83–S98. doi: 10.1890/11-0392.1
Kneitel, J. M., and Chase, J. M. (2004). Trade-offs in community ecology: linking spatial scales and species coexistence. Ecol. Lett. 7, 69–80. doi: 10.1046/j.1461-0248.2003.00551.x
Krueger-Mangold, J. M., Sheley, R. L., and Svejcar, T. J. (2006). Toward ecologically-based invasive plant management on rangeland. Weed Sci. 54, 597–605. doi: 10.1614/WS-05-049R3.1
Latombe, G., Essl, F., and McGeoch, M. A. (2020). The effect of cross-boundary management on the trajectory to commonness in biological invasions. NeoBiota 62, 241–267. doi: 10.3897/neobiota.62.52708
Legault, G., Bitters, M. E., Hastings, A., and Melbourne, B. A. (2020). Interspecific competition slows range expansion and shapes range boundaries. Proc. Natl. Acad. Sci. U.S.A. 117, 26854–26860. doi: 10.1073/pnas.2009701117
Legendre, P., Galzin, R., and Harmelin-Vivien, M. L. (1997). Relating behavior to habitat: solutions to the fourth-corner problem. Ecology 78, 547–562. doi: 10.2307/2266029
Leibold, M. A. (1996). A graphical model of keystone predators in food webs: trophic regulation of abundance, incidence, and diversity patterns in communities. Am. Nat. 147, 784–812. doi: 10.1086/285879
Leibold, M. A., and Chase, J. M. (2018). Metacommunity Ecology. Princeton, NJ; Oxford: Princeton University Press. doi: 10.1515/9781400889068
Leibold, M. A., Holyoak, M., Mouquet, N., Amarasekare, P., Chase, J. M., Hoopes, M. F., et al. (2004). The metacommunity concept: a framework for multi-scale community ecology. Ecol. Lett. 7, 601–613. doi: 10.1111/j.1461-0248.2004.00608.x
Leibold, M. A., and Mikkelson, G. M. (2002). Coherence, species turnover, and boundary clumping: elements of meta-community structure. Oikos 97, 237–250. doi: 10.1034/j.1600-0706.2002.970210.x
Lenda, M., Knops, J. H., Skórka, P., Moroń, D., and Woyciechowski, M. (2018). Cascading effects of changes in land use on the invasion of the walnut juglans regia in forest ecosystems. J. Ecol. 106, 671–686. doi: 10.1111/1365-2745.12827
Levine, J. M., Adler, P. B., and Yelenik, S. G. (2004). A meta-analysis of biotic resistance to exotic plant invasions. Ecol. Lett. 7, 975–989. doi: 10.1111/j.1461-0248.2004.00657.x
Levins, R., and Culver, D. (1971). Regional coexistence of species and competition between rare species. Proc. Natl. Acad. Sci.U.S.A. 68, 1246–1248. doi: 10.1073/pnas.68.6.1246
Limberger, R., Pitt, A., Hahn, M. W., and Wickham, S. A. (2019). Spatial insurance in multi-trophic metacommunities. Ecol. Lett. 22, 1828–1837. doi: 10.1111/ele.13365
Liu, H., and Stiling, P. (2006). Testing the enemy release hypothesis: a review and meta-analysis. Biol. Invasions 8, 1535–1545. doi: 10.1007/s10530-005-5845-y
Livingston, G., Matias, M., Calcagno, V., Barbera, C., Combe, M., Leibold, M. A., et al. (2012). Competition–colonization dynamics in experimental bacterial metacommunities. Nat. Commun. 3:1234. doi: 10.1038/ncomms2239
Lockwood, J. L., Cassey, P., and Blackburn, T. (2005). The role of propagule pressure in explaining species invasions. Trends Ecol. Evol. 20, 223–228. doi: 10.1016/j.tree.2005.02.004
Lockwood, J. L., Cassey, P., and Blackburn, T. M. (2009). The more you introduce the more you get: the role of colonization pressure and propagule pressure in invasion ecology. Div. Distr. 15, 904–910.
Logue, J. B., Mouquet, N., Peter, H., and Hillebrand, H. (2011). Empirical approaches to metacommunities: a review and comparison with theory. Trends Ecol. Evol. 26, 482–491. doi: 10.1016/j.tree.2011.04.009
Lombaert, E., Guillemaud, T., Cornuet, J.-M., Malausa, T., Facon, B., and Estoup, A. (2010). Bridgehead effect in the worldwide invasion of the biocontrol harlequin ladybird. PLoS ONE 5:e9743. doi: 10.1371/journal.pone.0009743
Loreau, M., Mouquet, N., and Gonzalez, A. (2003). Biodiversity as spatial insurance in heterogeneous landscapes. Proc. Natl. Acad. Sci. U.S.A. 100, 12765–12770. doi: 10.1073/pnas.2235465100
Lowe, W. H., and McPeek, M. A. (2014). Is dispersal neutral? Trends Ecol. Evol. 29, 444–450. doi: 10.1016/j.tree.2014.05.009
MacArthur, R. H. (1970). Species packing and competitive equilibria for many species. Theor. Popul. Biol. 1, 1–11. doi: 10.1016/0040-5809(70)90039-0
MacArthur, R. H., and Wilson, E. O. (1967). The Theory of Island Biogeography. Princeton, NJ: Princeton University Press.
Matias, M. G., Mouquet, N., and Chase, J. M. (2013). Dispersal stochasticity mediates species richness in source–sink metacommunities. Oikos 122, 395–402. doi: 10.1111/j.1600-0706.2012.20479.x
Meijer, K., Schilthuizen, M., Beukeboom, L., and Smit, C. (2016). A review and meta-analysis of the enemy release hypothesis in plant-herbivorous insect systems. PeerJ 4:e2778. doi: 10.7717/peerj.2778
Melián, C. J., Krivan, V., Altermatt, F., Starý, P., Pellissier, L., and Laender, F. D. (2015). Dispersal dynamics in food webs. Am. Nat. 185, 157–168. doi: 10.1086/679505
Mitchell, C. E., and Power, A. G. (2003). Release of invasive plants from fungal and viral pathogens. Nature 421, 625–627. doi: 10.1038/nature01317
Moles, A. T., Gruber, M. A. M., and Bonser, S. P. (2008). A new framework for predicting invasive plant species. J. Ecol. 96, 13–17. doi: 10.1111/j.1365-2745.2007.01332.x
Moritz, C., Meynard, C. N., Devictor, V., Guizien, K., Labrune, C., Guarini, J. M., et al. (2013). Disentangling the role of connectivity, environmental filtering, and spatial structure on metacommunity dynamics. Oikos 122, 1401–1410. doi: 10.1111/j.1600-0706.2013.00377.x
Morlon, H., Chuyong, G., Condit, R., Hubbell, S., Kenfack, D., Thomas, D., et al. (2008). A general framework for the distance-decay of similarity in ecological communities. Ecol. Lett. 11, 904–917. doi: 10.1111/j.1461-0248.2008.01202.x
Mouquet, N., Hoopes, M. F., and Amarasekare, P. (2005). “The world is patchy and heterogeneous! Trade-off and source-sink dynamics in competitive metacommunities,” in Metacommunities: Spatial Dynamics and Ecological Communities, eds M. Holyoak, M. A. Leibold, and R. D. Holt (Chicago, IL; London: University of Chicago Press), 237–262.
Mouquet, N., and Loreau, M. (2003). Community patterns in source-sink metacommunities. Am. Nat. 162, 544–557. doi: 10.1086/378857
Mwangi, P. N., Schmitz, M., Scherber, C., Roscher, C., Schumacher, J., Scherer-Lorenzen, M., et al. (2007). Niche pre-emption increases with species richness in experimental plant communities. J. Ecol. 95, 65–78. doi: 10.1111/j.1365-2745.2006.01189.x
Nekola, J. C., and White, P. S. (1999). The distance decay of similarity in biogeography and ecology. J. Biogeogr. 26, 867–878. doi: 10.1046/j.1365-2699.1999.00305.x
Niemiera, A. X., and Von Holle, B. (2009). Invasive Plants–A Horticultural Perspective. Virginia Cooperative Extension. doi: 10.1002/9780470767986.ch8
Norsworthy, J. K., Ward, S. M., Shaw, D. R., Llewellyn, R. S., Nichols, R. L., Webster, T. M., et al. (2012). Reducing the risks of herbicide resistance: best management practices and recommendations. Weed Sci. 60, 31–62. doi: 10.1614/WS-D-11-00155.1
Oficialdegui, F. J., Clavero, M., Sánchez, M. I., Green, A. J., Boyero, L., Michot, T. C., et al. (2019). Unravelling the global invasion routes of a worldwide invader, the red swamp crayfish (Procambarus clarkii). Freshw. Biol. 64, 1382–1400. doi: 10.1111/fwb.13312
Oksanen, J., Blanchet, F. G., Friendly, M., Kindt, R., Legendre, P., McGlinn, D. J., et al. (2020). Vegan: Community Ecology Package. R package version 2. 5–7.
O'Neill, B. J. (2016). Community disassembly in ephemeral ecosystems. Ecology 97, 3285–3292. doi: 10.1002/ecy.1604
O'Neill, R. V. (2001). Is it time to bury the ecosystem concept? (With full military honors, of course!). Ecology 82, 3275–3284. doi: 10.1890/0012-9658(2001)082[3275:IITTBT]2.0.CO;2
Patrick, C. J., and Brown, B. L. (2018). Species pool functional diversity plays a hidden role in generating β-diversity. Am. Nat. 191, E159–E170. doi: 10.1086/696978
Pearson, D. E., Ortega, Y. K., Eren, Ö., and Hierro, J. L. (2018). Community assembly theory as a framework for biological invasions. Trends Ecol. Evol. 33, 313–325. doi: 10.1016/j.tree.2018.03.002
Peng, S., Kinlock, N. L., Gurevitch, J., and Peng, S. (2019). Correlation of native and exotic species richness: a global meta-analysis finds no invasion paradox across scales. Ecology 100:e02552. doi: 10.1002/ecy.2552
Peres-Neto, P. R., Legendre, P., Dray, S., and Borcard, D. (2006). Variation partitioning of species data matrices: estimateion and comparision of fractions. Ecology 87, 2614–2625. doi: 10.1890/0012-9658(2006)87[2614:VPOSDM]2.0.CO;2
Peres-Neto, P. R., Leibold, M. A., and Dray, S. (2012). Assessing the effects of spatial contingency and environmental filtering on metacommunity phylogenetics. Ecology 93, S14–S30. doi: 10.1890/11-0494.1
Perez Rocha, M., Bini, L. M., Domisch, S., Tolonen, K. T., Jyrkänkallio-Mikkola, J., Soininen, J., et al. (2018). Local environment and space drive multiple facets of stream macroinvertebrate beta diversity. J. Biogeogr. 45, 2744–2754. doi: 10.1111/jbi.13457
Peterson, A. T. (2003). Predicting the geography of species' invasions via ecological niche modeling. Q. Rev. Biol. 78, 419–433. doi: 10.1086/378926
Pheloung, P. C., Williams, P. A., and Halloy, S. R. (1999). A weed risk assessment model for use as a biosecurity tool evaluating plant introductions. J. Environ. Manage. 57, 239–251. doi: 10.1006/jema.1999.0297
Preston, D. L., Henderson, J. S., and Johnson, P. T. J. (2012). Community ecology of invasions: direct and indirect effects of multiple invasive species on aquatic communities. Ecology 93, 1254–1261. doi: 10.1890/11-1821.1
Pyšek, P., and Richardson, D. M. (2007). “Traits associated with invasiveness in alien plants: where do we stand?,” in Biological Invasions, ed W. Nentwig (Berlin; Heidelberg: Springer), 97–125. doi: 10.1007/978-3-540-36920-2_7
Pyšek, P., and Richardson, D. M. (2010). Invasive species, environmental change and management, and health. Annu. Rev. Environ. Resour. 35, 25–55. doi: 10.1146/annurev-environ-033009-095548
Reichard, S. H., and White, P. (2001). Horticulture as a pathway of invasive plant introductions in the united states: most invasive plants have been introduced for horticultural use by nurseries, botanical gardens, and individuals. Bioscience 51, 103–113. doi: 10.1641/0006-3568(2001)051[0103:HAAPOI]2.0.CO;2
Rejmanek, M., and Pitcairn, M. J. (2002). “When is eradication of exotic pest plants a realistic goal?,” in Turning the Tide: The Eradication of Invasive Species. the IUCN Species Survival Commission, eds C. R. Veitch and M. N. Clout (Auckland: IUCN), 414.
Resetarits, W. J., and Silberbush, A. (2016). Local contagion and regional compression: habitat selection drives spatially explicit, multiscale dynamics of colonisation in experimental metacommunities. Ecol. Lett. 19, 191–200. doi: 10.1111/ele.12553
Riar, M. K., Carley, D. S., Zhang, C., Schroeder-Moreno, M. S., Jordan, D. L., Webster, T. M., et al. (2016). Environmental influences on growth and reproduction of invasive Commelina benghalensis. Int. J. Agron. 2016:5679249. doi: 10.1155/2016/5679249
Ricciardi, A., Blackburn, T. M., Carlton, J. T., Dick, J. T. A., Hulme, P. E., Iacarella, J. C., et al. (2017). Invasion science: a horizon scan of emerging challenges and opportunities. Trends Ecol. Evol. 32, 464–474. doi: 10.1016/j.tree.2017.03.007
Richardson, D. M. (2004). Plant invasion ecology — dispatches from the front line. Divers. Distribut. 10, 315–319. doi: 10.1111/j.1366-9516.2004.00129.x
Richardson, D. M., and Pyšek, P. (2006). Plant invasions: merging the concepts of species invasiveness and community invasibility. Progr. Phys. Geogr. 30, 409–431. doi: 10.1191/0309133306pp490pr
Richardson, D. M., Pyšek, P., Rejmánek, M., Barbour, M. G., Panetta, F. D., and West, C. J. (2000). Naturalization and invasion of alien plants: concepts and definitions. Diversity Distributions 6, 93–107. doi: 10.1046/j.1472-4642.2000.00083.x
Roy, H. E., Lawson Handley, L. J., Schönrogge, K., Poland, R. L., and Purse, B. V. (2011). Can the enemy release hypothesis explain the success of invasive alien predators and parasitoids? BioControl 56, 451–468. doi: 10.1007/s10526-011-9349-7
Sandel, B., and Corbin, J. D. (2010). Scale, disturbance and productivity control the native-exotic richness relationship. Oikos 119, 1281–1290. doi: 10.1111/j.1600-0706.2010.18230.x
Seebens, H., Blackburn, T. M., Dyer, E. E., Genovesi, P., Hulme, P. E., Jeschke, J. M., et al. (2017). No saturation in the accumulation of alien species worldwide. Nat. Commun. 8:14435.
Seebens, H., Blackburn, T. M., Dyer, E. E., Genovesi, P., Hulme, P. E., Jeschke, J. M., et al. (2018). Global rise in emerging alien species results from increased accessibility of new source pools. Proc. Natl. Acad. Sci. U.S.A. 115, E2264–E2273. doi: 10.1073/pnas.1719429115
Shea, K., and Chesson, P. (2002). Community ecology theory as a framework for biological invasions. Trends Ecol. Evol. 17, 170–176. doi: 10.1016/S0169-5347(02)02495-3
Sheley, R. L., Svejcar, T. J., and Maxwell, B. D. (1996). A theoretical framework for developing successional weed management strategies on rangeland. Weed Technol. 10, 766–773. doi: 10.1017/S0890037X00040793
Shmida, A., and Wilson, M. V. (1985). Biological determinants of species diversity. J. Biogeogr. 12, 1–20.
Siepielski, A. M., and McPeek, M. A. (2010). On the evidence for species coexistence: a critique of the coexistence program. Ecology 91, 3153–3164. doi: 10.1890/10-0154.1
Simberloff, D. (2009). The role of propagule pressure in biological invasions. Annu. Rev. Ecol. Evol. Syst. 40, 81–102. doi: 10.1146/annurev.ecolsys.110308.120304
Smith, A. L., Hodkinson, T. R., Villellas, J., Catford, J. A., Csergo, A. M., Blomberg, S. P., et al. (2020). Global gene flow releases invasive plants from environmental constraints on genetic diversity. Proc. Natl. Acad. Sci. U.S.A. 117, 4218–4227. doi: 10.1073/pnas.1915848117
Smith, M. D., Wilcox, J. C., Kelly, T., and Knapp, A. K. (2004). Dominance not richness determines invasibility of tallgrass prairie. Oikos 106, 253–262. doi: 10.1111/j.0030-1299.2004.13057.x
Sokol, E. R. (2016). A Tutorial on Metacommunity Simulations (MCSim) for R. Available online at: http://rpubs.com/sokole/159425 (accessed December 12, 2019)
Sokol, E. R. (2019). MCSim: An R Package for Metacommunity Simulations. Available online at: https://github.com/sokole/MCSim/releases/tag/v0.4.9
Sokol, E. R., Benfield, E. F., Belden, L. K., and Valett, H. M. (2011). The assembly of ecological communities inferred from taxonomic and functional composition. Am. Nat. 177, 630–644. doi: 10.1086/659625
Sokol, E. R., Brown, B. L., and Barrett, J. E. (2017). A simulation-based approach to understand how metacommunity characteristics influence emergent biodiversity patterns. Oikos 126, 723–737. doi: 10.1111/oik.03690
Sokol, E. R., Brown, B. L., Carey, C. C., Tornwall, B., Swan, C. M., and Barrett, J. E. (2015). Linking management to biodiversity in built ponds using metacommunity simulations. Ecol. Modell. 296, 36–45. doi: 10.1016/j.ecolmodel.2014.10.022
Sokol, E. R., Hoch, J. M., Gaiser, E., and Trexler, J. C. (2013). Metacommunity structure along resource and disturbance gradients in everglades wetlands. Wetlands 34, 135–146. doi: 10.1007/s13157-013-0413-1
Steiner, C. F., Stockwell, R. D., Kalaimani, V., and Aqel, Z. (2011). Dispersal promotes compensatory dynamics and stability in forced metacommunities. Am. Nat. 178, 159–170. doi: 10.1086/660835
Steiner, C. F., Stockwell, R. D., Kalaimani, V., and Aqel, Z. (2013). Population synchrony and stability in environmentally forced metacommunities. Oikos 122, 1195–1206. doi: 10.1111/j.1600-0706.2012.20936.x
Stohlgren, T. J., Jarnevich, C., Chong, G. W., and Evangelista, P. H. (2006). Scale and plant invasions: a theory of biotic acceptance. Preslia 78, 405–426.
Strecker, A. L., and Brittain, J. T. (2017). Increased habitat connectivity homogenizes freshwater communities: historical and landscape perspectives. J. Appl. Ecol. 54, 1343–1352. doi: 10.1111/1365-2664.12882
Swan, C. M., and Brown, B. L. (2017). Metacommunity theory meets restoration: isolation may mediate how ecological communities respond to stream restoration. Ecol. Appl. 27, 2209–2219. doi: 10.1002/eap.1602
Swearingen, J., Blattery, B., Reshetiloff, K., and Zwicker, S. (2014). Plant Invaders of Mid-Atlantic Natural Areas, 4th Edn. Washington, DC: National Park Service and U.S. Fish and Wildlife Service, 168.
Swenson, N. G., Erickson, D. L., Mi, X., Bourg, N. A., Forero-Montaña, J., Ge, X., et al. (2012). Phylogenetic and functional alpha and beta diversity in temperate and tropical tree communities. Ecology 93, S112–S125. doi: 10.1890/11-1180.1
Theoharides, K. A., and Dukes, J. S. (2007). Plant invasion across space and time: factors affecting nonindigenous species success during four stages of invasion. N. Phytol. 176, 256–273. doi: 10.1111/j.1469-8137.2007.02207.x
Thompson, P. L., Guzman, L. M., De Meester, L., Horváth, Z., Ptacnik, R., Vanschoenwinkel, B., et al. (2020). A process-based metacommunity framework linking local and regional scale community ecology. Ecol. Lett. 23, 1314–1329. doi: 10.1111/ele.13568
Tilman, D. (1982). Resource Competition and Community Structure. Princeton, NJ: Princeton University Press. doi: 10.1515/9780691209654
Tilman, D. (1994). Competition and biodiversity in spatially structured habitats. Ecology 75, 2–16. doi: 10.2307/1939377
Tilman, D. (2000). Causes, consequences and ethics of biodiversity. Nature 405, 208–211. doi: 10.1038/35012217
Tolonen, K. T., Cai, Y., Vilmi, A., Karjalainen, S. M., Sutela, T., and Heino, J. (2018). Environmental filtering and spatial effects on metacommunity organisation differ among littoral macroinvertebrate groups deconstructed by biological traits. Aquatic. Ecol. 52, 119–131. doi: 10.1007/s10452-018-9649-4
Tonkin, J. D., Heino, J., and Altermatt, F. (2018). Metacommunities in river networks: the importance of network structure and connectivity on patterns and processes. Freshw. Biol. 63, 1–5. doi: 10.1111/fwb.13045
Tornwall, B. M., Swan, C. M., and Brown, B. L. (2017). Manipulation of local environment produces different diversity outcomes depending on location within a river network. Oecologia 184, 663–674. doi: 10.1007/s00442-017-3891-7
Urban, M. C. (2004). Disturbance heterogeneity determines freshwater metacommunity structure. Ecology 85, 2971–2978. doi: 10.1890/03-0631
Van Kleunen, M., Dawson, W., Schlaepfer, D., Jeschke, J. M., and Fischer, M. (2010a). Are invaders different? A conceptual framework of comparative approaches for assessing determinants of invasiveness. Ecol. Lett. 13, 947–958. doi: 10.1111/j.1461-0248.2010.01503.x
Van Kleunen, M., Weber, E., and Fischer, M. (2010b). A meta-analysis of trait differences between invasive and non-invasive plant species. Ecol. Lett. 13, 235–245. doi: 10.1111/j.1461-0248.2009.01418.x
Vanschoenwinkel, B., Buschke, F., and Brendonck, L. (2013). Disturbance regime alters the impact of dispersal on alpha and beta diversity in a natural metacommunity. Ecology 94, 2547–2557. doi: 10.1890/12-1576.1
Vilà, M., Espinar, J. L., Hejda, M., Hulme, P. E., Jarošík, V., Maron, J. L., et al. (2011). Ecological impacts of invasive alien plants: a meta-analysis of their effects on species, communities and ecosystems. Ecol. Lett. 14, 702–708. doi: 10.1111/j.1461-0248.2011.01628.x
Violle, C., Navas, M.-L., Vile, D., Kazakou, E., Fortunel, C., Hummel, I., et al. (2007). Let the concept of trait be functional! Oikos 116, 882–892. doi: 10.1111/j.0030-1299.2007.15559.x
Warburton, E. M., Kohler, S. L., and Vonhof, M. J. (2016). Patterns of parasite community dissimilarity: the significant role of land use and lack of distance-decay in a bat–helminth system. Oikos 125, 374–385. doi: 10.1111/oik.02313
Werner, E. E., Yurewicz, K. L., Skelly, D. K., and Relyea, R. A. (2007). Turnover in an amphibian metacommunity: the role of local and regional factors. Oikos 116, 1713–1725. doi: 10.1111/j.0030-1299.2007.16039.x
Wilson, J. R. U. (2020). Definitions can confuse: why the “Neonative” neologism is bad for conservation. Bioscience 70, 110–111. doi: 10.1093/biosci/biz159
Zavaleta, E. S., and Hulvey, K. B. (2007). Realistic variation in species composition affects grassland production, resource use and invasion resistance. Plant Ecol. 188, 39–51. doi: 10.1007/s11258-006-9146-z
Zhang, H., Qi, W., John, R., Wang, W., Song, F., and Zhou, S. (2015). Using functional trait diversity to evaluate the contribution of multiple ecological processes to community assembly during succession. Ecography 38, 1176–1186. doi: 10.1111/ecog.01123
Glossary
Alien Species
Species that have established a range outside of their historical ranges as a result of human transportation.
Biotic Acceptance
A paradigm suggesting that positive correlations between native species richness and invasive species richness occur because environmental conditions that are good for natives are also good for invasives. Often presented as a counter to the Biotic Resistance Hypothesis (Stohlgren et al., 2006).
Biotic Resistance
A paradigm suggesting that the susceptibility of a community to invasion is influenced by its biotic composition across trophic levels, and that higher levels of species richness generally deter invasion (Elton, 1958; Levine et al., 2004). There has remained equivocal evidence for the relationship between native and exotic richness.
Dispersal
Generalized term in metacommunity ecology that incorporates both movement and establishment of species. Viewed as the major “regional” process of metacommunity theory (Leibold et al., 2004).
Diversity Metrics (α, β, γ)
Common metrics used for describing metacommunities at multiple spatial scales. α = the diversity of a single local community or, when multiple local communities are being considered, the average diversity of localities. Frequently measured using either species richness or common diversity indices (e.g., Shannon, Simpson). γ = total diversity in a region, measured in the same way as α, but aggregated across all local communities in a region. β = turnover in composition between the local communities within a region. β-diversity can be measured in a number of different ways, including dissimilarity indices (e.g., Jaccard, Bray-Curtis). However, truly partitioning γ-diversity into independent α and β components (i.e., measures of β do not depend on α) is generally accomplished through α × β = γ (Jost, 2007), though other valid partitionings also exist (Jost, 2007; Chao et al., 2012).
Enemy Release
Invasive species are often thought to have been introduced to new ranges that lack the suite of (often specialist) predators, diseases, etc. that limited size and population growth in their native range (Keane and Crawley, 2002).
Invasibility Criterion
According to ecological theory, the definitive criterion for co-existence of species in a community; states that to truly coexist with other species in a community, the species must be able to increase its abundance when it is rare (MacArthur, 1972; Chesson, 2000). This criterion distinguishes coexistence from co-occurrence of species in a community (Siepielski and McPeek, 2010). The name of the criterion derives from a scenario in which a species invades an extant community at low abundances.
Invasive Species
Non-native species to that location, often as a direct or indirect result of human action. Invasive species can cause a multitude of ecological, economic, and human health impacts and are distinguished from alien species in that they have demonstrable negative impacts on communities, ecosystems, or ecosystem services.
Local and Regional (in Metacommunity Terms)
Metacommunity concepts categorize the factors that control community assembly and composition into two parts: local and regional. Local effects are traditional niche-associated effects like environmental conditions and species interactions. Regional effects are those effects driven primarily by the dispersal of organisms between local communities of the metacommunity (Leibold et al., 2004).
Metacommunity Paradigms (Species Sorting, Patch Dynamics, Mass Effects, Neutrality)
Four paradigms are strongly associated with metacommunity theory. Species Sorting = classic niche-based paradigm in which community composition is controlled by local environmental conditions and species interactions (Whittaker, 1962). Patch Dynamics = paradigm in which composition of local communities is driven by a landscape patchwork of local extinction/colonization dynamics. A key aspect of Patch Dynamics is the assumed tradeoff between competitive ability and dispersal ability (Levins and Culver, 1971; Levin, 1974). Mass Effects = paradigm that recognizes that local effects are important determinants of community composition, but that the regional effect of high dispersal rates may swamp out the influence of local factors (Brown and Kodric-Brown, 1977; Shmida and Wilson, 1985). Neutrality = paradigm that considers the traits of species to be inconsequential in determining species composition of a community; composition is the product of random extinction and probabilistic colonization of species. Often considered to be a null model of metacommunity effects (Bell, 2001; Hubbell, 2001). An important recognition is that these four paradigms were historically developed independently, outside of the scope of metacommunity theory. As such, they are not mutually exclusive in terms of mechanisms or predictions, and do not represent the entire inference space of metacommunity theory (Brown et al., 2017).
Neonative
Species expanding their range as a result of anthropogenically-based environmental changes. A controversial neologism (Wilson, 2020) meant to capture the fuzzy category of often native species that are shifting ranges, complicating management and policy.
Propagule Pressure
The number and size of introduction events of a single species to a location. Often considered one of the most important and fundamental elements of biological invasions (Simberloff, 2009). The concept of propagule pressure is closely paralleled by “dispersal” in metacommunity theory.
Rescue Effects
In a metacommunity, when a species goes extinct at a locality, it can be “rescued” by recolonization from other local communities (Brown and Kodric-Brown, 1977).
Sorting Strength and Ecological Filters
Sorting strength is a measure of the influence local factors have on species composition of the local community. The name derives from the Species Sorting paradigm of metacommunity theory. Sorting strength is often conceived as a set of ecological filters (e.g., environmental conditions, local competition, or predation) that exclude species from a particular local community.
Keywords: biotic resistance, community ecology, dispersal, invasive species, invasibility, propagule pressure, mass effect hypothesis, sorting strength
Citation: Brown BL and Barney JN (2021) Rethinking Biological Invasions as a Metacommunity Problem. Front. Ecol. Evol. 8:584701. doi: 10.3389/fevo.2020.584701
Received: 17 July 2020; Accepted: 09 December 2020;
Published: 13 January 2021.
Edited by:
Miguel Cañedo-Argüelles, University of Barcelona, SpainReviewed by:
David Mark Richardson, Stellenbosch University, South AfricaGuillaume Latombe, University of Vienna, Austria
Copyright © 2021 Brown and Barney. This is an open-access article distributed under the terms of the Creative Commons Attribution License (CC BY). The use, distribution or reproduction in other forums is permitted, provided the original author(s) and the copyright owner(s) are credited and that the original publication in this journal is cited, in accordance with accepted academic practice. No use, distribution or reproduction is permitted which does not comply with these terms.
*Correspondence: Bryan L. Brown, c3RvbmVmbHkmI3gwMDA0MDt2dC5lZHU=
†These authors have contributed equally to this work