- 1Red de Biología y Conservación de Vertebrados, Instituto de Ecología, Xalapa, Mexico
- 2Center for Conservation Genomics, Smithsonian Conservation Biology Institute, Washington, DC, United States
- 3Center for Vector Biology, Rutgers University, New Brunswick, NJ, United States
Vector-host interactions play a central role in the transmission of vector-borne diseases. Determining the factors that affect vector attraction to vertebrate hosts is critical to understand disease ecological dynamics. Using malaria as the primary model, we reviewed studies that examined whether pathogen infection and host traits affect mosquito attraction. We found contradictory results examining mosquito attraction to birds infected with Plasmodium parasites, presumably because of widely variable experimental approaches. We summarize current experimental findings and propose standardized experimental approaches for future studies.
Introduction
Insect vectors have often been considered passive vessels that carry pathogens without exerting much selection. However, vectors may suffer high pathogen-related mortalities (Valkiūnas et al., 2014; Gutiérrez-López et al., 2019a,b), and vectors selection of vertebrate hosts to obtain blood meals is not random or solely based on host availability, they follow general and specific host cues such as carbon dioxide and volatile body chemicals (Takken and Verhulst, 2013). Interestingly, both host choice and host attractiveness may be manipulated by the parasite in order to increase its transmission success (i.e., manipulation hypothesis; Heil, 2016).
The process by which pathogens manipulate vectors’ behavior can be indirect during the development in a vertebrate host (e.g., increased attractiveness of infected hosts to uninfected vectors), and/or direct during the cycle inside the vectors (e.g., increase in attraction or feeding rates toward uninfected hosts; Gandon, 2018). These changes have profound implications for pathogen transmission. For instance, uninfected vectors highly attracted toward infected hosts would initially increase transmission in local populations (Gandon, 2018). Generalist mosquito species feeding on birds and mammals (depending on host availability; Abella-Medrano et al., 2018) play important roles in the transmission of zoonotic pathogens (e.g., Culex pipiens transmitting West Nile virus (WNV) and Usutu (USUV) virus among birds, equids, and humans; Brugman et al., 2018; Rochlin et al., 2019). Thus, investigating drivers of mosquito host selection is essential to understand the dynamics of vertebrate-vector-pathogen systems.
In recent years avian haemosporidian parasites have received attention as model systems to understand the ecology and evolution of vector-borne parasites (Rivero and Gandon, 2018). In particular because they are widespread, infect a large proportion of bird species worldwide, and affect bird fitness and survival (Atkinson and Van Riper, 1991; Valkiūnas, 2005; Palinauskas et al., 2020). Thus, by focusing on avian malaria, here we provide an overview of the factors affecting host selection by mosquitoes concentrating on Culex mosquitoes, which transmit human pathogens, bird pathogens, and zoonotic pathogens that use birds as reservoirs (WNV, USUV). Although Culex mosquitoes have a broad range of hosts, some are predominantly mammophilic and others are ornithophilic (e.g., Culex theileri vs. Cx. pipiens), yet their feeding behaviors depend on the species and population of origin, which also affects the parasites they transmit (Santa-Ana et al., 2006; Farajollahi et al., 2011; Rochlin et al., 2019).
Birds are used as models to analyze factors within species that affect host attractiveness to mosquitoes, which include mosquito and bird pathogen infection status, differences in the production of natural chemicals by birds (uropygial gland secretion and volatile compounds), host sex, and interactions among some of these factors (Table 1). We took advantage of studies on human and mice malaria systems that also addressed some of these factors to suggest next steps for studies on bird attractiveness to mosquitoes. We found discrepancies among studies, which may be due to differences in study design (Table 1). Therefore, we propose standardized experimental approaches to help identify generalities and contingencies in pathogen-vector-host interactions using avian malaria as a model (Figure 1).
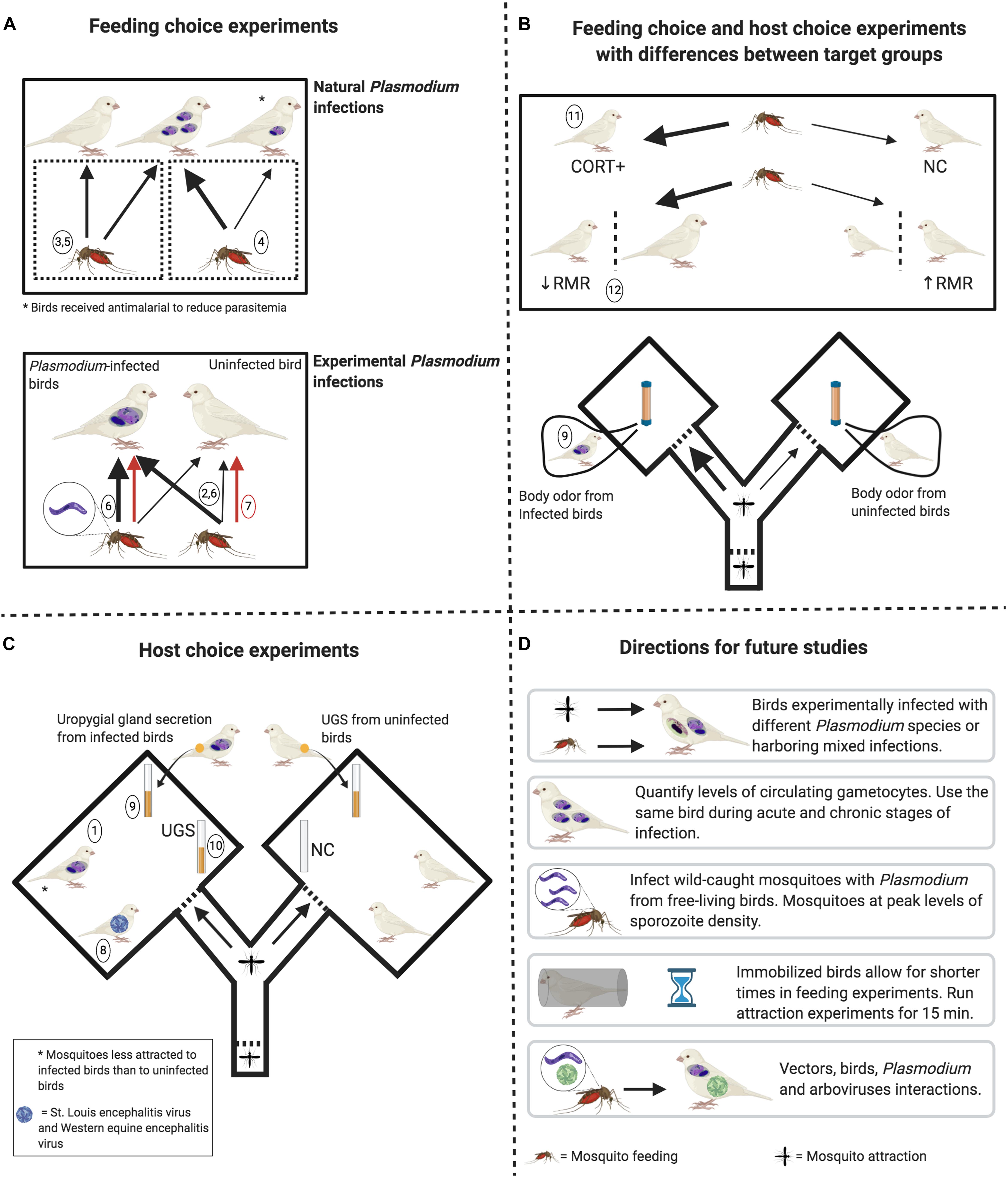
Figure 1. Experimental designs to determine the factors potentially affecting mosquito feeding behavior and attraction toward birds. (A) Upper pane: mosquitoes do not feed more on naturally infected birds than on uninfected birds. However, feeding rates are higher on birds with higher levels of circulating parasites (thick black arrow) when compared to birds with reduced levels of parasites after antimalarial treatment (thin black arrow). Lower pane: uninfected mosquitoes feed more on birds experimentally infected with Plasmodium relictum (thick black arrow) when compared to uninfected birds. Infective mosquitoes (harboring sporozoites) may (thick black arrow) or may not (red arrows) feed more on infected birds than on uninfected ones. (B) Upper pane: Mosquitoes feed more (thick black arrows) on birds with higher levels of corticosterone (CORT+) and feed more on birds with lower resting metabolic rate (RMR) and with higher body mass. Lower pane: Mosquitoes are more attracted toward whole-body odor from birds naturally infected with Plasmodium parasites. (C) No mosquito preference in relation to bird arboviral infection and to uropygial gland secretion (UGS) from Plasmodium-infected birds when compared to UGS from uninfected birds. No mosquito preference toward UGS when compared to vials containing no stimuli. Mosquitos were less attracted to birds naturally infected with Plasmodium. (D) Open questions that can be addressed in future studies, always keeping in mind to standardize bird and mosquito cohorts in terms of age and sex. Numbers in circles refer to specific studies indicated in Table 1 (see Source column). Figure created with BioRender.com.
Current Experimental Approaches
In general, laboratory experiments assess mosquito attraction using dual-choice olfactometers, or they assess feeding choice by placing mosquitoes inside cages containing target host groups. Olfactometers expose mosquitoes to olfactory host cues (CO2 and volatile compounds) and prevent mosquitoes from using visual cues or differences in body temperature for host detection (Lalubin et al., 2012; De Moraes et al., 2014; Díez-Fernández et al., 2020a). This approach is useful for studies using chemical stimuli collected from hosts and has the advantage to protect hosts from being bitten by mosquitoes. Host attractiveness in single-choice system can be measured as the number of mosquitoes approaching a host in one end of an enclosure or in a tunnel in relation to the number of mosquitoes released at the opposite end (Cator et al., 2013; Batista et al., 2014).
Feeding rates can be measured by comparing the proportion of mosquitoes taking a blood meal from single-housed (Cornet et al., 2019) or pair-housed hosts. In the latter, two hosts from different groups (e.g., control vs. experimental group) are placed inside the same cage where mosquitoes are released and host choice is assessed via microsatellite testing (Yan et al., 2018b) or by molecular sexing methods (Cornet et al., 2013a; Yan et al., 2018a) using engorged mosquitoes to determine blood source. Overall, studies varied in duration and type (attraction assays using olfactometers vs. feeding choice inside cages), with feeding experiments used either immobilized or non-immobilized birds (Table 1). These differences, therefore, may explain discrepancies in the general findings.
Indirect and Direct Manipulation of Vector’s Behavior by Pathogens
Pathogens can modify conditions within vertebrate hosts or within vectors to increase transmission rates from infected to uninfected hosts. These indirect and direct vector manipulations, respectively, may affect host-seeking behaviors, feeding behaviors, or both (Gandon, 2018).
Infected Hosts and Mosquito Attraction
Most studies tested whether hosts infected with Plasmodium parasites are more attractive to uninfected mosquitoes or whether uninfected mosquitoes feed more on infected hosts (indirect vector manipulation). Some of these studies also tested whether hosts with higher number of circulating gametocytes (sexual parasite stage that is infective to mosquitoes) were more attractive to mosquitoes even when compared with hosts with low levels of gametocytes (Table 1).
Avian Malaria
Cornet et al. (2013a, b) found that laboratory-reared Cx. pipiens fed more on domestic canaries (Serinus canaria) infected with a laboratory strain of Plasmodium relictum (SGS1) when compared to uninfected birds. This effect was detected in birds undergoing chronic infections (24–26 days post infection – dpi), but not in birds undergoing the acute phase of the infection (10 dpi; Cornet et al., 2013b). Mosquitoes fed more on birds with a higher hematocrit, a measure that can be interpreted as a higher blood quality (Cornet et al., 2013a).
Four avian malaria studies used wild caught Cx. pipiens and free-living birds infected with Plasmodium spp. and uninfected birds to assess vector manipulation in more natural systems (Table 1). One set of experiments conducted by Yan et al. (2018b) compared infected birds with their counterparts treated with primaquine to reduce parasitemia (the number of circulating parasites). They found mosquitoes fed more on infected birds than on treated birds, which provided experimental support for the vector manipulation hypothesis. Similarly, mosquitoes were more attracted to body odors isolated from infected birds when compared to uninfected birds (Díez-Fernández et al., 2020b). However, this effect was not observed when using uropygial gland secretion collected from the same bird groups. Other experiments found that mosquitoes were either more attracted to uninfected birds (Lalubin et al., 2012) or that infection status did not change mosquito feeding rates when compared to control groups (Yan et al., 2018b; Gutiérrez-López et al., 2019a).
From the discussed studies, two used dual-choice olfactometers to assess mosquito attraction to birds or to chemical compounds collected from birds, while three studies released mosquitoes inside cages containing single birds or pairs of infected and uninfected birds that were immobilized in most cases (except Yan et al., 2018b; Table 1). Observed contrasting results addressing the host manipulation hypothesis may be the result of differences in host, vector and parasites used and/or due to differences in experimental settings.
Human and Mice Malaria
Most studies on human malaria found that natural Plasmodium infections are associated with higher host attractiveness to vectors. Three out of four studies found that Anopheles mosquitoes were more attracted to humans harboring microscopically detectable gametocytes when compared to uninfected people and to people with only asexual stages detectable in the blood stream (Lacroix et al., 2005; Batista et al., 2014; Busula et al., 2017). The fourth study showed that socks worn by children parasitized by Plasmodium falciparum were more attractive to mosquitoes when compared with socks worn by the same children after the completion of antimalarial treatment (Robinson et al., 2018). A study during its first trial using feet odors found that samples from individuals infected with P. falciparum were less attractive to mosquitoes when compared to cotton pads from the same individuals after antimalaria treatment and compared to odors from uninfected people; the second trial did not find any difference in mosquito attraction (de Boer et al., 2017).
Two studies using mice malaria found that Plasmodium chabaudi infection increased Anopheles stephensi feeding rates (Ferguson et al., 2003) and attraction (De Moraes et al., 2014) when compared to uninfected hosts. This latter study found this effect only during the initial chronic stage, which was associated to microscopically observable levels of circulating gametocytes. These studies on mammals also show contrasting results, precluding generalizations and strengthening the fact of having a standard initial experimental design.
Infected Mosquitoes and Host-Feeding
Pathogens can influence host-seeking and feeding behaviors during their development inside mosquitoes. Because mosquitoes only become infective a few days or even weeks after blood feeding on an Plasmodium-infected host (i.e., once sporozoites are found in salivary glands; Valkiūnas et al., 2013; Palinauskas et al., 2016), changes in host-seeking and feeding behaviors may be displayed differentially according to vector infective stage rather than infection status only.
When mosquitoes were simultaneously exposed to both Plasmodium-infected and uninfected birds, both infective and uninfected mosquitoes fed more frequently on Plasmodium-infected birds (Cornet et al., 2013a). When exposed to uninfected birds, uninfected mosquitoes fed faster than infective mosquitoes, but the final feeding rate after the 3 h the experiment lasted was identical between both groups (Cornet et al., 2019). This demonstrates that experiment duration can affect results, because the authors would have observed higher feeding rates of uninfected mosquitoes if experiments had lasted only 1 h.
Mosquitoes that fed on Plasmodium yoelii-infected mice were less attracted to hosts during the oocyst stage (non-infective) than during the sporozoite stage, when they engaged in higher rates of host-seeking behavior when compared to negative controls (Cator et al., 2013). However, these changes were also observed in mosquitoes that were not infected after feeding on infected mice. Therefore, the observed behavioral changes may be due to Plasmodium infections and to unspecific immune stimuli in the challenged mosquitoes.
Laboratory reared Anopheles gambiae harboring sporozoites of a laboratory strain of P. falciparum were more attracted to human odor when compared to infective mosquitoes exposed to negative-controls (Smallegange et al., 2013). In contrast, three different species of wild caught Anopheles infected with P. falciparum from natural infections had similar attraction rates to humans when compared with uninfected mosquitoes (Nguyen et al., 2017).
Chemical Compounds Studied in Relation to Vector Attraction
Differences in mosquito attraction are related to dissimilarities in chemical volatiles produced by each host, which can be modified by pathogen infections. Díez-Fernández et al. (2020b) were the first to show that whole-body odor from Plasmodium-infected birds are more attractive to mosquitoes when compared to uninfected birds. De Moraes et al. (2014) demonstrated that mice displayed different odor profiles when infected with Plasmodium chabaudi and became more attractive to mosquitoes after the acute phase of infection. Mice produced fewer volatile chemicals during the acute phase of infection and attracted less mosquitoes. In humans, increased mosquito attraction toward Plasmodium-infected children was associated to changes in skin odor profile driven by increases in the production of aldehydes (Robinson et al., 2018). Children infected with P. falciparum in Malawi produced a different chemical breath composition than uninfected children (Schaber et al., 2018), which mosquitos can use as a cue to find their hosts (Kelly et al., 2015). Thus, the chemical volatiles emitted by hosts and how they change during infection likely constitute the most important factor determining vector attraction.
Birds’ uropygial (or preen) gland produces some antimicrobial and antifungal chemicals that protect the plumage and skin of birds; they also provide defense against ectoparasites and haemosporidian infection (Salibian and Montalti, 2009; Magallanes et al., 2016). Some studies suggest that uropygial gland secretions can attract vectors (Russell and Hunter, 2005), but others suggest no increased attraction toward such secretions (Díez-Fernández et al., 2020a) or toward common chemicals from uropygial glands (Allan et al., 2006) when compared to negative controls (no stimuli). The amount of volatile compounds of uropygial glands differ among adult, fledging and nestling house sparrows (Passer domesticus), but these differences did not modify mosquito attraction in dual-choice olfactometer assays (Garvin et al., 2018a). Plasmodium infection did not change the composition of the volatile fraction of uropygial gland secretion in house sparrows when compared to the uninfected group, and this may explain the similar mosquito attraction toward the secretion from both bird groups (Díez-Fernández et al., 2020b). However, other study showed that exposure to Plasmodium altered the wax ester composition of the secretion in song sparrows (Melospiza melodia), regardless of whether infections were cleared or not (Grieves et al., 2018). Therefore, more research is needed to cover the whole array of chemicals produced by the uropygial gland and their effect on different vector species and families (Martínez-de la Puente et al., 2020). Particularly considering that parasites from different Haemosporida genera (e.g., Haemoproteus, Leucocytozoon) are transmitted by different Diptera families, which have both different life cycles and ecologies (Ibáñez-Bernal et al., 2020).
Studies Focused on Other Host Attributes
Physiological differences (e.g., hormones) and differential exposure between males and females due to sex-specific physiology (e.g., immunosuppression due to breeding activities), behavior (e.g., nesting), morphology (e.g., body size), and pathogen infections can influence mosquito attraction (Darbro et al., 2007; Cozzarolo et al., 2019). For instance, Cx. pipiens were more attracted to female Great tits (Parus major; Lalubin et al., 2012). Ochlerotatus caspius fed more on female than on male jackdaws (Corvus monedula), but no difference was observed when using Cx. pipiens. In addition, these two mosquito species did not show sex-biased feeding preference toward house sparrows (Gutiérrez-López et al., 2019a). Cozzarolo et al. (2019) did not find any mosquito feeding preference between sexes, but they used nestlings instead of adults (see also Simpson et al., 2009). Higher feeding rate on female birds by wild-caught Culex restuans has been reported during the nesting period, suggesting the role of seasonality in this process (Egizi et al., 2014), but this has yet to be investigated under laboratory conditions. Yan et al. (2018a) found that mosquitos fed less on birds with higher resting metabolic rate, which authors interpreted as related to bird activity, where birds with higher metabolic rates are more active and thus display more defensive behaviors than more lethargic individuals. Finally, elevated levels of corticosterone increased bird attractiveness to mosquitoes despite the fact that these birds displayed increased anti-mosquito behaviors when compared to the control group (Gervasi et al., 2016). Increases in corticosterone levels may lead to Plasmodium resurgence in the blood stream in chronically infected birds with an increase in parasitemia (Applegate and Beaudoin, 1970). Therefore, the interaction between elevated stress hormone levels and parasitemia may synergistically increase bird attractiveness to mosquitoes, but this has yet to be tested. Different results across studies may be due to differences in life history traits of bird species, which may in turn influence exposure to vectors and species-specific physiological trade-offs. Hence, we suggest that fair comparisons and generalizations must be done across species (both hosts and vectors) with similar life history traits.
Summary of Current Evidence and Implications for Future Studies
We found that fewer studies assessed direct effects of avian and mammalian Plasmodium on mosquito host choice (infective mosquitoes) when compared to the number of studies on indirect effects on mosquitoes (infected vertebrate hosts). Rearing mosquitoes under laboratory conditions over many generations may alter some genetic traits involved in their responsiveness to host cues (Takken and Verhulst, 2013), but nothing is known about whether genetic bottlenecks would change as a function of host-vector-parasite lineage assemblages (Gutiérrez-López et al., 2020). Although using hosts (birds and mice), mosquitoes and pathogens maintained in laboratories has provided fundamental tests of hypothesis related to the vector manipulation by pathogens, experiments mimicking natural conditions using wild caught mosquitoes are essential to fill in gaps for real world situations.
Free-living birds are infected with genetic lineages that vary in their pathological effects as a function of host species and parasite lineage (Palinauskas et al., 2011; Himmel et al., 2020). Assays using experimental infections have focused on domestic canaries infected with a P. relictum strain (SGS1) kept in the laboratory over dozens of passages, while assays using free-living birds have used three avian species naturally infected with a high diversity of parasites. In Gutiérrez-López et al. (2019a), 21 of 23 jackdaws exposed to wild-caught Cx. pipiens were naturally infected with P. relictum (SGS1), and yet these authors did not find differences in mosquito feeding rates between infected and uninfected birds. On the other hand, laboratory-reared mosquitoes fed more on canaries experimentally infected with a laboratory strain of P. relictum SGS1 (Cornet et al., 2013a,b).
The use of naturally infected birds has the limitation of confounding the bird’s infection stage during mosquito exposure. This is because in birds the Plasmodium acute stage of infection usually finishes within a month post infection. After this period surviving birds enter the chronic stage of infection, in which they display low parasitemia for long periods or even for their entire life (Valkiūnas, 2005). In many of the studies, free-living birds were undergoing chronic infections when exposed to mosquitoes and parasitemia was not evaluated. Interestingly, mosquitoes were more attracted to chronically infected birds when compared to acute infection stages in experimental settings (Cornet et al., 2013b), which may be interpreted as a parasite avoidance strategy because high parasitemia in the host is associated to high vector mortality (Gutiérrez-López et al., 2019b).
Birds and mosquitoes can be co-infected by different genetically related or unrelated parasites (e.g., co-infection between Plasmodium parasites and WNV; Hughes et al., 2010; Medeiros et al., 2014). Co-infections by different Plasmodium spp. and other related haemosporidians are often found in wild birds (e.g., Valkiūnas et al., 2006). Yet, evidence for the effects of multiple infections on vector feeding behavior and on fitness of both bird hosts (e.g., Marzal et al., 2008) and vectors (e.g., Gutiérrez-López et al., 2020) is still very limited (see Martínez-de la Puente et al., 2020). To complicate matters further, if mosquitoes are more attracted to birds co-infected with Plasmodium and arboviruses than to birds infected with arboviruses only, avian malaria may indirectly increase the transmission of zoonotic pathogens. Future studies, therefore, should perform feeding or attraction assays using birds experimentally infected with strains of different Plasmodium species with as few laboratory passages as possible, and also using different parasite groups (e.g., malaria and arbovirus). In addition, stage of infection (acute vs. chronic) and gametocyte levels (parasitemia) should be taken into consideration in studies using both natural and experimental infections. Furthermore, mosquitoes captured from the wild should be favored over populations kept in the laboratory for many generations. This would provide more real settings to understand host attractiveness to free-moving mosquitoes in nature.
Important issues to consider when planning experiments are:
(1) will birds be free or immobilized? Free birds may reflect natural conditions, but immobilized birds will increase vector sample size and reduce vector mortality by birds’ behavioral defenses. (2) Will birds be visible to vectors or just odor cues will be investigated? Our opinion is that light levels should reflect those from the natural conditions during which vector activity peaks, which depends on vector species. (3) What would be the duration of the experiment? Immobilizing birds allows for shorter feeding experiments (1–3 h), while 15 min seems to be a common and reasonable time for attraction experiments. Ideally, researchers must know the natural feeding ecology of the studied vector in order to make an informed decision on the duration of experiments. Finally, (4) co-infections by different Plasmodium lineages or different parasite genera (e.g., Plasmodium/Haemoproteus) that are ubiquitous in nature, or even by Haemosporidians and arboviruses (e.g., WNV and USUV) should be considered. Understanding factors that change mosquito attraction to birds may have implications for human health as well, since highly ornithophilic mosquitoes such as Cx. pipiens, Cx. perexiguus, and Cx. restuans are vectors of some arboviruses that may affect humans, such as WNV and USUV (e.g., Kilpatrick et al., 2006; Vázquez et al., 2011; Brugman et al., 2018). Thus, further studies are necessary to understand the range of effects that multiple infections may have on host-vector contact rates and, hence, on the dynamics of parasite transmission. Our review highlights the need to design and implement general standard experimental procedures to obtain comparable results, which can help to generalize findings across studies and to identify contingencies (Figure 1) that later will allow drawing generalizations by, for instance, conducting meta-analyses.
Author Contributions
Both authors have made a substantial, direct and intellectual contribution to the work, and approved it for publication.
Funding
DS-A has been supported by Consejo Nacional de Ciencia y Tecnología (CONACYT, project number Ciencia Básica 2011-01-168524 and project number Problemas Nacionales 2015-01-1628). FCF was supported by National Science Foundation grant 1717498 as part of the joint NSF-NIH-USDA Ecology and Evolution of Infectious Diseases program.
Conflict of Interest
The authors declare that the research was conducted in the absence of any commercial or financial relationships that could be construed as a potential conflict of interest.
Acknowledgments
We thank Sergio Ibáñez-Bernal, Daniela A. Dutra, Dina M. Fonseca, the editor Laura Gangoso and the three reviewers for their comments that highly improved the quality of this review.
References
Abella-Medrano, C. A., Ibáñez-Bernal, S., Carbó-Ramírez, P., and Santiago-Alarcon, D. (2018). Blood-meal preferences and avian malaria detection in mosquitoes (Diptera: Culicidae) captured at different land use types within a neotropical montane cloud forest matrix. Parasitol. Int. 67, 313–320. doi: 10.1016/j.parint.2018.01.006
Allan, S. A., Bernier, U. R., and Kline, D. L. (2006). Laboratory evaluation of avian odors for mosquito (Diptera: Culicidae) attraction. J. Med. Entomol. 43, 225–231. doi: 10.1093/jmedent/43.2.225
Applegate, J. E., and Beaudoin, R. L. (1970). Mechanism of spring relapse in avian malaria: effect of gonadotropin and corticosterone. J. Wildl. Dis. 6, 443–447. doi: 10.7589/0090-3558-6.4.443
Atkinson, C. T., and Van Riper, I. I. I. C. (1991). “Pathogenicity and epizootiology of avian haematozoa: Plasmodium, Leucocytozoon and Haemoproteus,” in Bird-Parasite Interacctions, eds J. E. Loye and M. Zuk (Oxford: Oxford University Press), 19–48.
Batista, E. P., Costa, E. F., and Silva, A. A. (2014). Anopheles darlingi (Diptera: Culicidae) displays increased attractiveness to infected individuals with Plasmodium vivax gametocytes. Parasit. Vector. 7:251. doi: 10.1186/1756-3305-7-251
Brugman, V. A., Hernández-Triana, L. M., Medlock, J. M., Fooks, A. R., Carpenter, S., and Johnson, N. (2018). The role of Culex pipiens L. (Diptera: Culicidae) in virus transmission in Europe. Int. J. Environ. Res. Public Health 15:389. doi: 10.3390/ijerph15020389
Burkett-Cadena, N. D., Ligon, R. A., Liu, M., Hassan, H. K., Hill, G. E., Eubanks, M. D., et al. (2010). Vector-host interactions in avian nests: do mosquitoes prefer nestlings over adults? Am. J. Trop. Med. Hyg. 83, 395–399. doi: 10.4269/ajtmh.2010.10-0048
Busula, A. O., Bousema, T., Mweresa, C. K., Masiga, D., Logan, J. G., Sauerwein, R. W., et al. (2017). Gametocytemia and attractiveness of Plasmodium falciparum-infected kenyan children to Anopheles gambiae mosquitoes. J. Infect. Dis. 216, 291–295. doi: 10.1093/infdis/jix214
Cator, L. J., George, J., Blanford, S., Murdock, C. C., Baker, T. C., Read, A. F., et al. (2013). ‘Manipulation’ without the parasite: altered feeding behaviour of mosquitoes is not dependent on infection with malaria parasites. Proc. R. Soc. B 280:20130711. doi: 10.1098/rspb.2013.0711
Cornet, S., Nicot, A., Rivero, A., and Gandon, S. (2013a). Both infected and uninfected mosquitoes are attracted toward malaria infected birds. Malar. J. 12:179. doi: 10.1186/1475-2875-12-179
Cornet, S., Nicot, A., Rivero, A., and Gandon, S. (2013b). Malaria infection increases bird attractiveness to uninfected mosquitoes. Ecol. Lett. 16, 323–329. doi: 10.1111/ele.12041
Cornet, S., Nicot, A., Rivero, A., and Gandon, S. (2019). Avian malaria alters the dynamics of blood feeding in Culex pipiens mosquitoes. Malar. J. 18, 82. doi: 10.1186/s12936-019-2690-5
Cozzarolo, C.-S., Sironi, N., Glaizot, O., Pigeault, R., and Christe, P. (2019). Sex-biased parasitism in vector-borne disease: vector preference? PLoS One 14:e0216360. doi: 10.1371/journal.pone.0216360
Darbro, J. M., Dhondt, A. A., Vermeylen, F. M., and Harrington, L. C. (2007). Mycoplasma gallisepticum infection in house finches (Carpodacus mexicanus) affects mosquito blood feeding patterns. Am. J. Trop. Med. Hyg. 77, 488–494. doi: 10.4269/ajtmh.2007.77.488
de Boer, J. G., Robinson, A., Powers, S. J., Burgers, S. L. G. E., Caulfield, J. C., Birkett, M. A., et al. (2017). Odours of Plasmodium falciparum-infected participants influence mosquito-host interactions. Sci. Rep. 7:9283. doi: 10.1038/s41598-017-08978-9
De Moraes, C. M., Stanczyk, N. M., Betz, H. S., Pulido, H., Sim, D. G., Read, A. F., et al. (2014). Malaria-induced changes in host odors enhance mosquito attraction. Proc. Natl. Acad. Sci. U.S.A. 111, 11079–11084. doi: 10.1073/pnas.1405617111
Díez-Fernández, A., Martínez-de la Puente, J., Gangoso, L., Ferraguti, M., Soriguer, R., and Figuerola, J. (2020a). House sparrow uropygial gland secretions do not attract ornithophilic nor mammophilic mosquitoes. Med. Vet. Entomol. 2020:12401. doi: 10.1111/mve.12401
Díez-Fernández, A., Martínez-de la Puente, J., Gangoso, L., López, P., Soriguer, R., Martín, J., et al. (2020b). Mosquitoes are attracted by the odour of Plasmodium-infected birds. Int. J. Parasitol. 50, 569–575. doi: 10.1016/j.ijpara.2020.03.013
Egizi, A. M., Farajollahi, A., and Fonseca, D. M. (2014). Diverse host feeding on nesting birds may limit early-season West Nile virus amplification. Vector Borne Zoonotic Dis. 14, 447–453. doi: 10.1089/vbz.2013.1536
Farajollahi, A., Fonseca, D. M., Kramer, L. D., and Marm Kilpatrick, A. (2011). “Bird biting” mosquitoes and human disease: a review of the role of Culex pipiens complex mosquitoes in epidemiology. Infect. Genet. Evol. 11, 1577–1585. doi: 10.1016/j.meegid.2011.08.013
Ferguson, H. M., Rivero, A., and Read, A. F. (2003). The influence of malaria parasite genetic diversity and anaemia on mosquito feeding and fecundity. Parasitology 127, 9–19. doi: 10.1017/s0031182003003287
Gandon, S. (2018). Evolution and manipulation of vector host choice. Am. Nat. 192, 23–34. doi: 10.1086/697575
Garvin, M. C., Austin, A., Boyer, K., Gefke, M., Wright, C., Pryor, Y., et al. (2018a). Attraction of Culex pipiens to house sparrows is influenced by host age but not uropygial gland secretions. Insects 9:127. doi: 10.3390/insects9040127
Garvin, M. C., Austin, A. L., Stracker, N. H., Slowinski, S. P., Rutter, J. E., Butler, M., et al. (2018b). Attraction of Culex pipiens to uropygial gland secretions does not explain feeding preference for American robins. J. Vect. Ecol. 43, 110–116. doi: 10.1111/jvec.12290
Gervasi, S. S., Burkett-Cadena, N., Burgan, S. C., Schrey, A. W., Hassan, H. K., Unnasch, T. R., et al. (2016). Host stress hormones alter vector feeding preferences, success, and productivity. Proc. R. Soc. B 283:20161278. doi: 10.1098/rspb.2016.1278
Grieves, L. A., Kelly, T. R., Bernards, M. A., and MacDougall-Shackleton, E. A. (2018). Malarial infection alters wax ester composition of preen oil in songbirds: results of an experimental study. Auk: Ornithol. Adv. 135, 767–776. doi: 10.1642/AUK-17-242.1
Griffing, S. M., Kilpatrick, A. M., Clark, L., and Marra, P. P. (2007). Mosquito landing rates on nesting American robins (Turdus migratorius). Vect. Borne Zoonot. Dis. 7, 437–443. doi: 10.1089/vbz.2006.0560
Gutiérrez-López, R., Martínez-de la Puente, J., Gangoso, L., Soriguer, R., and Figuerola, J. (2019a). Effects of host sex, body mass and infection by avian Plasmodium on the biting rate of two mosquito species with different feeding preferences. Parasit. Vect. 12:87. doi: 10.1186/s13071-019-3342-x
Gutiérrez-López, R., Martínez-de la Puente, J., Gangoso, L., Yan, J., Soriguer, R., and Figuerola, J. (2019b). Experimental reduction of host Plasmodium infection load affects mosquito survival. Sci. Rep. 9:8782. doi: 10.1038/s41598-019-45143-w
Gutiérrez-López, R., Martínez-de la Puente, J., Gangoso, L., Soriguer, R., and Figuerola, J. (2020). Plasmodium transmission differs between mosquito species and parasite lineages. Parasitology 147, 441–447. doi: 10.1017/S0031182020000062
Heil, M. (2016). Host manipulation by parasites: cases, patterns and remaining doubts. Front. Ecol. Evol. 4:80. doi: 10.3389/fevo.2016.00080
Himmel, T., Harl, J., Pfanner, S., Nedorost, N., Nowotny, N., and Weissenböck, H. (2020). Haemosporidioses in wild Eurasian blackbirds (Turdus merula) and song thrushes (T. philomelos): an in situ hybridization study with emphasis on exo-erythrocytic parasite burden. Malar. J. 19:69. doi: 10.1186/s12936-020-3147-6
Hughes, T., Irwin, P., Hofmeister, E., and Paskewitz, S. M. (2010). Occurrence of avian Plasmodium and West Nile virus in Culex species in Wisconsin. J. Am. Mosq. Control Assoc. 26, 24–31. doi: 10.2987/09-5893.1
Ibáñez-Bernal, S., Rivera-García, K. D., and Abella-Medrano, C. A. (2020). “Introduction to the taxonomy and general biology of Diptera (Insecta) involved in the transmission of avian haemosporida,” in Avian Malaria and Related Parasites in the Tropics - Ecology, Evolution and Systematics, eds D. Santiago-Alarcon and A. Marzal (Cham: Springer).
Kelly, M., Su, C.-Y., Schaber, C., Crowley, J. R., Hsu, F.-F., Carlson, J. R., et al. (2015). Malaria parasites produce volatile mosquito attractants. mBio 6:e00235-15.
Kilpatrick, A. M., Kramer, L. D., Jones, M. J., Marra, P. P., and Daszak, P. (2006). West Nile virus epidemics in North America are driven by shifts in mosquito feeding behavior. PLoS Biol. 4:e82. doi: 10.1371/journal.pbio.0040082
Lacroix, R., Mukabana, W. R., Gouagna, L. C., and Koella, J. C. (2005). Malaria infection increases attractiveness of humans to mosquitoes. PLoS Biol. 3:e298. doi: 10.1371/journal.pbio.0030298
Lalubin, F., Bize, P., van Rooyen, J., Christe, P., and Glaizot, O. (2012). Potential evidence of parasite avoidance in an avian malarial vector. Anim. Behav. 84, 539–545. doi: 10.1016/j.anbehav.2012.06.004
Magallanes, S., Møller, A. P., García-Longoria, L., de Lope, F., and Marzal, A. (2016). Volume and antimicrobial activity of secretions of the uropygial gland are correlated with malaria infection in house sparrows. Parasit. Vect. 9:232. doi: 10.1186/s13071-016-1512-1517
Martínez-de la Puente, J., Díez-Fernández, A., Soriguer, R., Rambozzi, L., Peano, A., Meneguz, P., et al. (2020). Are malaria-infected birds more attractive to mosquito vectors. Ardeola 68, 251–264. doi: 10.13157/arla.68.1.2021.fo1
Marzal, A., Bensch, S., Reviriego, M., Balbontin, J., and De Lope, F. (2008). Effects of malaria double infection in birds: one plus one is not two. J. Evol. Biol. 21, 979–987. doi: 10.1111/j.1420-9101.2008.01545.x
Medeiros, M. C. I., Anderson, T. K., Higashiguchi, J. M., Kitron, U. D., Walker, E. D., Brawn, J. D., et al. (2014). An inverse association between West Nile virus serostatus and avian malaria infection status. Parasit. Vect. 7:415. doi: 10.1186/1756-3305-7-415
Nguyen, P. L., Vantaux, A., Hien, D. F., Dabiré, K. R., Yameogo, B. K., Gouagna, L.-C., et al. (2017). No evidence for manipulation of Anopheles gambiae, An. coluzzii and An. arabiensis host preference by Plasmodium falciparum. Sci. Rep. 7:9415. doi: 10.1038/s41598-017-09821-x
Palinauskas, V., Martínez-de la Puente, J., Hernández-Soto, R. S., and Marzal, A. (2020). “Experimental Parasitology and Ecoimmunology: concepts and opportunities in avian Haemosporidian studies,” Avian Malaria and Related Parasites in the Tropics, eds D. Santiago-Alarcon and A. Marzal (Cham: Springer).
Palinauskas, V., Valkiūnas, G., Bolshakov, C. V., and Bensch, S. (2011). Plasmodium relictum (lineage SGS1) and Plasmodium ashfordi (lineage GRW2): the effects of the co-infection on experimentally infected passerine birds. Exp. Parasitol. 127, 527–533. doi: 10.1016/j.exppara.2010.10.007
Palinauskas, V., Žiegytė, R., Iezhova, T. A., Ilgūnas, M., Bernotienė, R., and Valkiūnas, G. (2016). Description, molecular characterisation, diagnostics and life cycle of Plasmodium elongatum (lineage pERIRUB01), the virulent avian malaria parasite. Int. J. Parasitol. 46, 697–707. doi: 10.1016/j.ijpara.2016.05.005
Rivero, A., and Gandon, S. (2018). Evolutionary ecology of avian malaria: past to present. Trends Parasitol. 34, 712–726. doi: 10.1016/j.pt.2018.06.002
Robinson, A., Busula, A. O., Voets, M. A., Beshir, K. B., Caulfield, J. C., Powers, S. J., et al. (2018). Plasmodium-associated changes in human odor attract mosquitoes. Proc. Natl. Acad. Sci. U.S.A. 115, E4209–E4218. doi: 10.1073/pnas.1721610115
Rochlin, I., Faraji, A., Healy, K., and Andreadis, T. G. (2019). West Nile virus mosquito vectors in North America. J. Med. Entomol. 56, 1475–1490. doi: 10.1093/jme/tjz146
Russell, C. B., and Hunter, F. F. (2005). Attraction of Culex pipiens/restuans (Diptera: Culicidae) mosquitoes to bird Uropygial gland odors at two elevations in the niagara region of Ontario. J. Med. Entomol. 42, 301–305. doi: 10.1093/jmedent/42.3.301
Salibian, A., and Montalti, D. (2009). Physiological and biochemical aspects of the avian uropygial gland. Braz. J. Biol. 69, 437–446. doi: 10.1590/s1519-69842009000200029
Santa-Ana, M., Khadem, M., and Capela, R. (2006). Natural infection of Culex theileri (Diptera: Culicidae) with Dirofilaria immitis (Nematoda: Filarioidea) on Madeira Island. Portugal J. Med. Entomol. 43, 104–106. doi: 10.1093/jmedent/43.1.104
Schaber, C. L., Katta, N., Bollinger, L. B., Mwale, M., Mlotha-Mitole, R., Trehan, I., et al. (2018). Breathprinting reveals malaria-associated biomarkers and mosquito attractants. J. Infect. Dis. 217, 1553–1560. doi: 10.1093/infdis/jiy072
Scott, T. W., Lorenz, L. H., and Edman, J. D. (1990). Effects of house sparrow age and arbovirus infection on attraction of mosquitoes. J. Med. Entomol. 27, 856–863. doi: 10.1093/jmedent/27.5.856
Simpson, J. E., Folsom-O’Keefe, C. M., Childs, J. E., Simons, L. E., Andreadis, T. G., and Diuk-Wasser, M. A. (2009). Avian host-selection by Culex pipiens in experimental trials. PLoS One 4:e7861. doi: 10.1371/journal.pone.0007861
Smallegange, R. C., van Gemert, G. J., van de Vegte-Bolmer, M., Gezan, S., Takken, W., Sauerwein, R. W., et al. (2013). Malaria infected mosquitoes express enhanced attraction to human odor. PLoS One 8:e63602. doi: 10.1371/journal.pone.0063602
Spanoudis, C. G., Andreadis, S. S., Bray, D. P., Savopoulou-Soultani, M., and Ignell, R. (2020). Behavioural response of the house mosquitoes Culex quinquefasciatus and Culex pipiens molestus to avian odours and its reliance on carbon dioxide. Med. Vet. Entomol. 34, 129–137. doi: 10.1111/mve.12429
Takken, W., and Verhulst, N. O. (2013). Host preferences of blood-feeding mosquitoes. Annu. Rev. Entomol. 58, 433–453. doi: 10.1146/annurev-ento-120811-153618
Valkiūnas, G. (2005). Avian Malaria Parasites and Other Haemosporidia, 1st Edn, Boca Raton, FL: CRC Press.
Valkiūnas, G., Bensch, S., Iezhova, T. A., Križanauskienë, A., Hellgren, O., and Bolshakov, C. V. (2006). Nested cytochrome b polymerase chain reaction diagnostics underestimate mixed infections of avian blood haemosporidian parasites: microscopy is still essential. J. Parasitol. 92, 418–422. doi: 10.1645/ge-3547rn.1
Valkiūnas, G., Kazlauskienë, R., Bernotienë, R., Bukauskaitë, D., Palinauskas, V., and Iezhova, T. A. (2014). Haemoproteus infections (Haemosporida, Haemoproteidae) kill bird-biting mosquitoes. Parasitol. Res. 113, 1011–1018. doi: 10.1007/s00436-013-3733-4
Valkiūnas, G., Kazlauskienë, R., Bernotienë, R., Palinauskas, V., and Iezhova, T. A. (2013). Abortive long-lasting sporogony of two Haemoproteus species (Haemosporida, Haemoproteidae) in the mosquito Ochlerotatus cantans, with perspectives on haemosporidian vector research. Parasitol. Res. 112, 2159–2169. doi: 10.1007/s00436-013-3375-6
Vázquez, A., Ruiz, S., Herrero, L., Moreno, J., Molero, F., Magallanes, A., et al. (2011). West Nile and Usutu viruses in mosquitoes in Spain, 2008-2009. Am. J. Trop. Med. Hyg. 85, 178–181. doi: 10.4269/ajtmh.2011.11-0042
Vogels, C. B. F., Fros, J. J., Pijlman, G. P., van Loon, J. J. A., Gort, G., and Koenraadt, C. J. M. (2017). Virus interferes with host-seeking behaviour of mosquito. J. Exp. Biol. 220, 3598–3603. doi: 10.1242/jeb.164186
Yan, J., Broggi, J., Martínez-de la Puente, J., Gutiérrez-López, R., Gangoso, L., Soriguer, R., et al. (2018a). Does bird metabolic rate influence mosquito feeding preference? Parasit. Vector. 11:110. doi: 10.1186/s13071-018-2708-9
Keywords: behavioral manipulation, vector attraction, experimental parasitology, arboviruses, avian malaria, Culicidae, Diptera
Citation: Santiago-Alarcon D and Ferreira FC (2020) Does Plasmodium Infection Affect Mosquito Attraction? Front. Ecol. Evol. 8:582943. doi: 10.3389/fevo.2020.582943
Received: 13 July 2020; Accepted: 21 October 2020;
Published: 09 November 2020.
Edited by:
Laura Gangoso, Consejo Superior de Investigaciones Científicas (CSIC), SpainReviewed by:
Rafael Gutiérrez López, University of Porto, PortugalLeanne Grieves, McMaster University, Canada
Jiayue Yan, University of Illinois at Urbana-Champaign, United States
Copyright © 2020 Santiago-Alarcon and Ferreira. This is an open-access article distributed under the terms of the Creative Commons Attribution License (CC BY). The use, distribution or reproduction in other forums is permitted, provided the original author(s) and the copyright owner(s) are credited and that the original publication in this journal is cited, in accordance with accepted academic practice. No use, distribution or reproduction is permitted which does not comply with these terms.
*Correspondence: Diego Santiago-Alarcon, diego.santiago@inecol.mx; onca77@gmail.com; Francisco C. Ferreira, franciscocarlosfj@gmail.com