- Department of Biology, California State University, San Bernardino, CA, United States
Urban environments pose different selective pressures than natural ones, leading to changes in animal behavior, physiology, and morphology. Understanding how animals respond to urbanization could inform the management of urban habitats. Non-avian reptiles have important roles in ecosystems worldwide, yet their responses to urbanization have not been as comprehensively studied as those of mammals and birds. However, unlike mammals and birds, most reptiles cannot easily move away from disturbances, making the selective pressure to adapt to urban environments especially strong. In recent years, there has been a surge in research on the responses of lizards to urbanization, yet no formal synthesis has determined what makes an urban lizard, in other words, which phenotypic traits are most likely to change with urbanization and in which direction? Here, we present a qualitative synthesis of the literature and a quantitative phylogenetic meta-analysis comparing phenotypic traits between urban and non-urban lizard populations. The most robust finding from our analysis is that urban lizards are larger than their non-urban counterparts. This result remained consistent between sexes and taxonomic groups. Hence, lizards that pass through the urban filter have access to better resources, more time for foraging, and/or there is selection on attaining a larger body size. Other results included an increase in the diameters of perches used and longer limb and digit lengths, although this may be a result of increased body size. Urban lizards were not bolder, more active or exploratory, and did not differ in immune responses than non-urban populations. Overall, studies are biased to a few geographic regions and taxa. More than 70% of all data came from three species of anoles in the family Dactyloidae, making it difficult to generalize patterns to other clades. Thus, more studies are needed across multiple taxa and habitats to produce meaningful predictions that could help inform conservation and management of urban ecological communities.
Introduction
We live in the Anthropocene, an epoch in which humans are now the major driver of global environmental change (Lewis and Maslin, 2015; Biermann et al., 2016). Urbanization is one of the most extreme forms of human-induced environmental change and has progressed rapidly in recent years. In 2007, for the first time in human history, more people were living in cities than in rural areas, and it is expected that more than 65% of the human population will be urban by the year 2050 (United Nations, 2019). Cities drastically change the abiotic and biotic features of the environment, creating strong, and sometimes novel, selection pressures on native flora and fauna. Impervious surface cover (e.g., concrete) is greatly increased in cities which affects the water cycle, albedo, and temperature, often leading to the “urban heat island” effect (Grimm et al., 2008; Hulley, 2012). Urban habitats tend to be more open, with fewer trees (LaMontagne et al., 2015) and with human-constructed substrates that are more broad and smooth than natural substrates (Winchell et al., 2018). Habitat fragmentation due to urbanization could lead to depressed diversity and abundance of native species, including a reduction in natural predator populations, altering the ecological relationships within communities (McKinney, 2008; Dobbs et al., 2017). These anthropogenic impacts are apparent in cities around the world, demonstrating the potential to use these systems as replicated natural experiments to study the ecological and evolutionary implications of urbanization on wildlife (Johnson and Munshi-South, 2017).
Urban selection pressures, like those listed above, have led to shifts in behavioral, morphological, and physiological responses in animals. Urban animals tend to be more bold in response to reduced predation pressure and a habituation to human presence (Møller, 2009; Samia et al., 2015; Symonds et al., 2016). Urban birds and frogs shift their singing/calling frequencies to reduce overlap with anthropogenic sounds (Roca et al., 2016), while urban mammals have become more nocturnal to reduce overlap of activity times with humans (Gaynor et al., 2018). In response to the urban heat island effect, many species, from ants to anoles, have evolved higher physiological tolerance of high temperatures (Angilletta et al., 2007; Brans et al., 2017; Campbell-Staton et al., 2020). Shifts in morphological traits are also commonly reported; for instance urban insects are generally smaller in body size (Merckx et al., 2018; Eggenberger et al., 2019), urban bats and birds tend to have higher wing aspect ratios (Croci et al., 2008; Voigt and Kingston, 2015; Santini et al., 2019), and urban birds show changes in beak depth and length (Giraudeau et al., 2014; Miller et al., 2018) compared to their non-urban counterparts. These are just a few examples of differences in animal phenotypes that have been and continue to be documented in urban environments.
Shifts in animal phenotypes associated with urbanization occur at higher rates than those due to other forms of anthropogenic disturbances (Alberti et al., 2017). Many species and populations have demonstrated remarkable abilities to survive and reproduce within habitats that have been drastically altered by humans, yet not all have the capabilities to do so (i.e., many species are filtered out; Hamer and McDonnell, 2008; Schochet et al., 2016; Silva et al., 2016; Santini et al., 2019). While it is possible for rapid evolution to occur on timescales that are relevant to urbanization, shifts in phenotypic traits are more likely the result of phenotypic plasticity (Kinnison and Hendry, 2001; Miranda et al., 2013; Nelson et al., 2015), and/or spatial sorting of individuals across urban-rural landscapes whereby individuals with certain traits are more likely to disperse into urban habitats while individuals lacking these traits remain in non-urban habitats (Berthouly-Salazar et al., 2012; Piano et al., 2017). Additionally, some phenotypes, like body size, might not change due to selection, but rather are a consequence of shifting resource types or availabilities. In most cases, observed changes in phenotypes cannot yet be specifically attributed to changes in gene frequency or expression (McDonnell and Hahs, 2015), although some studies find support for rapid genetic responses to urban environments [reviewed in Johnson and Munshi-South (2017)]. Understanding how organisms respond to urbanization will allow us to predict which species, populations, or individuals are most vulnerable, providing valuable information for curbing the loss of wildlife during the Anthropocene (Dirzo et al., 2014).
Reviews, syntheses, and multi-species assessments on the phenotypic responses of animals to urbanization have largely centered around birds (Chamberlain et al., 2009; Evans et al., 2011; Seress and Liker, 2015; Kettel et al., 2018; Martin and Bonier, 2018) and mammals (Benítez-López et al., 2010; Ordeñana et al., 2010; Saito and Koike, 2013; Voigt and Kingston, 2015; Łopucki and Kitowski, 2017). Yet, an increase in research on non-avian reptiles (henceforth “reptiles”) has the potential to expand our knowledge on wildlife responses to urbanization, which could better inform the management and conservation of ecological communities in urban areas. Similar to mammals and birds, reptiles are mostly terrestrial vertebrates that serve important roles in the ecosystems where they occur (de Miranda, 2017). However, the functional significance of reptiles often differs substantially from that of mammals and birds due to their different thermoregulatory strategies (Nowak et al., 2008; Jessop et al., 2020). In turn, their responses to urbanization are likely to differ. Unlike mammals and birds, reptiles tend to have relatively low dispersal abilities, and so they may be more likely to experience selection from rapid human-induced habitat changes. As ectotherms, reptiles might be more sensitive to the urban thermal environment than mammals or birds. They also utilize habitats differently (e.g., climbing on human-made structures) and are likely susceptible to different suites of predators and parasites.
Research on the effects of urbanization on reptiles has increased considerably in the last 5 to 10 years. A handful of recent reviews have attempted to synthesize this nascent literature (French et al., 2018; Lapiedra, 2018; Putman et al., 2019b). While many unique reptilian responses to urbanization have been documented, directionality seems to be largely variable (likely due to the heterogeneity of urban environments and differences among species) with very few overall trends. Behaviorally, urban species seem to display more neophilic and risk-taking behavior, however, where domestic predators (especially cats) are present, flight initiation distance tends to increase (French et al., 2018; Putman et al., 2019b). Some species have shown higher rates of morphological asymmetry and increased limb length and body size, often accompanying changes in habitat use (e.g., perch selection and tendency to jump between perches vs. moving about on the same perch; French et al., 2018; Lapiedra, 2018). All authors agree that significant gaps in the literature persist regarding the mechanisms that drive specific responses within urban systems, the relationships between responses and fitness, and the relationships between responses at different levels of ecological organization.
The majority of research within the field of urban herpetology has focused on lizards (all clades within the order Squamata excluding Serpentes and Amphisbaenia), likely because lizards have historically been used as models in research on behavior, ecology, and evolution. Lizards are also ideal for comparative studies as they are widely distributed, physiologically and morphologically diverse, tolerant of experimental procedures and handling, and the life histories of many species are well-understood (Camargo et al., 2010). While many individual studies on various urban lizard populations have been investigated, no generalizations can be drawn as to what makes an urban lizard because no quantitative synthesis at the global scale has been conducted. A recent meta-analysis found that human-induced habitat modifications have had negative impacts on reptile populations worldwide (Doherty et al., 2020), yet information regarding traits of successful species will be useful in predicting other species' responses and for the holistic management of urban ecological communities. In order to fill this information gap, here we qualitatively review the state of the literature within this field of urban herpetological research and take a comparative phylogenetic approach to analyze published data on the effects of urbanization on the physiological, morphological, or behavioral traits in lizards worldwide.
Methods
Literature Search
Our goal was to determine the impacts of urbanization on behavioral, physiological, and/or morphological traits in lizards. We performed a literature search on 23 May 2019, using the University of California, Los Angeles institutional subscriptions. Our search contained all years of publication. Within Web of Science, we searched the Core Collection, BIOSIS Previews, and Zoological Record. We used the following search terms: (lizard* OR gecko* OR agama* OR chameleon* OR iguan* OR anol* OR tegu* OR whiptail* OR skink* OR dragon*) AND (urban* OR suburb* OR anthropogenic OR city OR cities OR village* OR town* OR municipalit* OR neighborhood* OR industrializ* OR “human impact” OR “human built” OR “human modified” OR “human altered”) AND (behavior OR morphology OR physiology). We also searched ProQuest for unpublished data in dissertations and theses, and verified that the data presented in these were not already published in the peer-reviewed literature. In ProQuest, we searched on title and abstract only (no full text) using the same search terms as above. Finally, when conference proceedings were found, we searched to see if the research had been published in the peer-reviewed literature. Three reviews were found and we reviewed their references to verify that the searches above did not miss any additional relevant publications.
Study Selection
The study selection process followed PRISMA guidelines (Moher et al., 2009, Figure 1). The initial search yielded 1,700 unique records, including two from published reviews. We removed duplicates, which narrowed down the total to 1,336 records. Next, we reviewed papers on their titles and abstracts. We set criteria based on PICOs (population, intervention, comparator, outcome) of traditional systematic reviews to decide whether studies met eligibility for being included in our syntheses. Articles were deemed relevant if the study population was a lizard species, if the study compared at least one urban and one non-urban population of the same species (intervention of urbanization and comparator of urban “treatment” vs. non-urban “control”), and if the study quantified a behavioral, morphological, or physiological trait (outcome). We were only interested in papers that focused solely on urbanization, and not other forms of anthropogenic disturbances such as logging or agriculture. We recognize the lack of globally universal criteria used to define urban areas from rural areas and so we relied on authors' descriptions of their study sites along with the objectives of their study (i.e., if the goal was to compare an urban population with a non-urban one) to determine relevance for inclusion. In five cases we had to determine ourselves whether a study fit our comparator inclusion criterion. For this, we used a liberal definition of urbanization that is related to the functional characteristics of urban areas, such as the existence of buildings, paved roads, electrical and sewage systems, and other urban infrastructure [see United Nations (2019)]. We considered a lizard population urban if it occurred in an area with these urban functional characteristics regardless of the human population density or density of buildings. Non-urban lizard populations occurred in areas that lacked such characteristics. We acknowledge that this added heterogeneity to our dataset, and we tried to account for this in the analyses below.
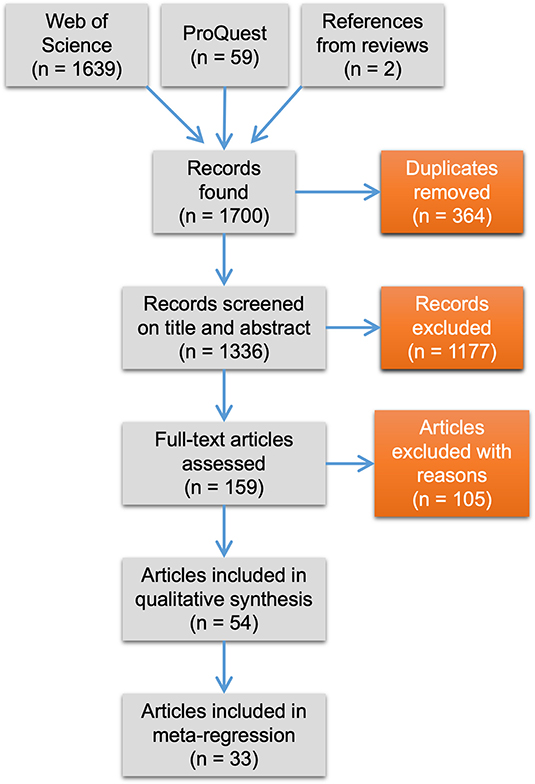
Figure 1. PRISMA flow diagram showing number of articles retrieved from the literature search and retained or excluded during the study selection process.
The removal of studies lacking an urban to non-urban comparator narrowed down our total to 159 papers (Supplementary Table 1 shows all relevant studies and reasons for exclusion). Full text screening was then conducted using the same inclusion criteria as above. We emailed authors when we could not access their articles or when data were not presented in a way useful for a meta-analysis (i.e., no means reported). Seven out of 14 authors that were emailed replied and graciously supplied the missing information. Finally, we also excluded studies not published in the English language as this is the only language we are proficient in. In all, 54 articles were available for data extraction (Supplementary Tables 1, 2). We used these 54 articles to perform a qualitative synthesis of current foci and practices in urban lizard research. In this synthesis, we included information on focal species and traits studied, and information on the study attributes listed below.
Data Extraction
For all studies, we extracted the raw means and variances (e.g., standard deviation) of the measured phenotypic trait(s). We used the WebPlotDigitizer (https://automeris.io/WebPlotDigitizer/) to extract data presented in figures. We also scored the following variables: (1) taxonomic information such as family, genus, and species, (2) sex of population studied (as female, male, or both), (3) name of urban locality (e.g., city or metropolitan area) to account for differences associated with year and/or intensity of urbanization, and (4) study attributes including location, duration, and number of replicate sites. Some papers reported results on more than one species, quantified multiple phenotypic traits, quantified traits under different treatments, and/or reported results from multiple localities (e.g., different cities), and each of these were considered as different entries in the database (i.e., there can be multiple entries per article). When papers reported separate traits by sex (e.g., body sizes of males and of females separately), these were also considered different entries. We only included results from adult or sub-adult lizards as only a handful of studies focused on hatchling or juvenile traits (i.e., these studies were not included in the quantitative analysis).
We extracted data on behavioral, morphological, or physiological traits. Studies focused on various traits within each of these broad phenotypic categories, listed in Figure 2 and Supplementary Table 2. Traits were further refined based on specific assays used to estimate them; for instance, limb length can be measured multiple ways including total hind limb length, femur length, tibia/fibula length, forelimb length, etc. We made an effort to create biologically meaningful categories and erred on the side of splitting traits based on assays used to measure them instead of lumping means from multiple assays together. As an example, we split boldness into three categories (escape responses, willingness to be exposed, and latency to respond to stimuli) as we felt each of these represent bold behaviors under different circumstances or motivational states.
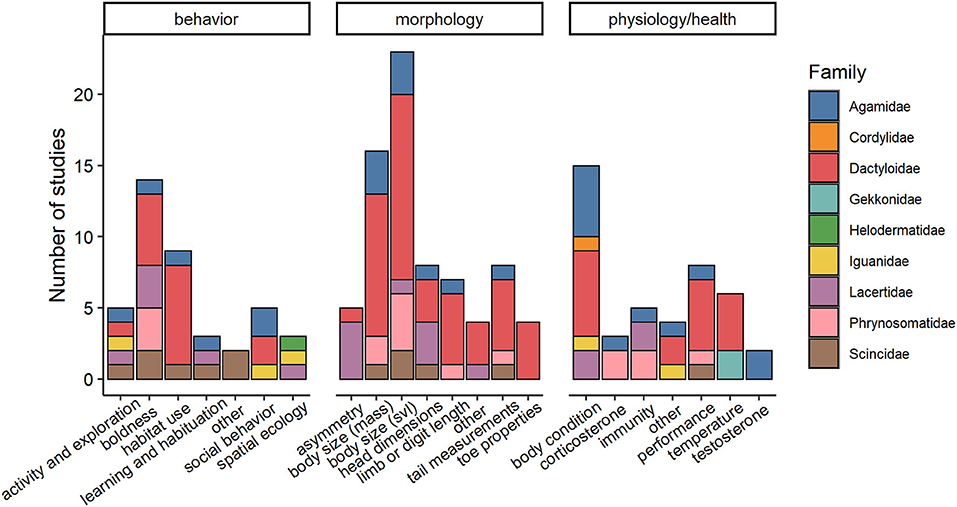
Figure 2. Number of studies on urban lizards categorized by type of phenotypic trait and by family (N = 54 articles; each article may have more than one study). Only a few behavioral, morphological, and/or physiological traits have been quantified and within only nine lizard families. Snout-vent-length, a measure of lizard body length, is abbreviated to svl.
Statistical Analyses
We performed a phylogenetic meta-analysis, specifically a meta-regression, to statistically evaluate the effects of urbanization on lizard traits. In order to be included in this analysis, a phenotypic trait category had to contain a minimum of 10 estimates (i.e., 10 effect sizes) as this sample size allows for correct quantification of between-study variance (Nakagawa et al., 2017). These included 18 traits: 5 measures of behavior, 10 measures of morphology (mainly different assays of limb and digit lengths), and 3 measures of physiology/health (Supplementary Table 3 shows the full database). To examine the mean effects of urbanization on these traits, we calculated the standardized mean difference (SMD) as Hedges' g (Hedges, 1981). Although the log response ratio (lnRR) is another statistic of effect size commonly used in ecology and evolutionary biology (Borenstein et al., 2009), we could not compute this because our database contained instances of means with opposing signs. When applicable, we adjusted the signs of effect size estimates so that positive values indicate a positive shift in the trait with urbanization (e.g., when boldness is measured as latency to emerge from refuge lower values indicate higher boldness).
We followed the methods of Mallen-Cooper et al. (2019) for the statistical analyses. We used the “metaphor” package in R (Viechtbauer, 2010; R version 3.6.1) to perform a meta-regression to evaluate heterogeneity among effect sizes. We fitted a multilevel model with the rma.mv function. We included study (article) and id (different effect sizes) as random factors to account for between-study effects and within-study effects, respectively. We added urban locality (e.g., city or metropolitan area) as a random effect to account for variation attributable to time since and/or intensity of urbanization. We also added species and phylogeny as random effects. To control for potential non-independence from phylogenetic relatedness, we used a correlation matrix derived from an ultrametric tree of squamate reptiles from Pyron et al. (2013) assuming a Brownian-motion model of evolution (Supplementary Figure 1). Inclusion of these five random effects helps identify additional sources of variance besides sampling error, and hence they are considered variance components in the model.
We included phenotypic trait as a moderator variable (fixed effect), and we calculated true intercepts and standard errors for each trait so that results reflect group means rather than contrasts to a reference group. We did this by running the model without an intercept so that all dummy variables are included as predictors. We used confidence intervals to evaluate statistical significance; a result was deemed significant if the interval did not include zero. Publication biases were evaluated using a funnel plot, Egger's test for asymmetry, and the trim and fill method (Nakagawa and Santos, 2012). A post-model fitting check was also performed to assess whether the full model was overparameterized. We created profile plots of the restricted log-likelihood as a function of each variance component (random effects; Konstantopoulos, 2011).
In addition to the above model, we ran models with reduced data sets to evaluate the robustness of the results. First, we recognized that multiple studies had measured the same traits from the same individuals multiple ways or under multiple treatments contributing to pseudoreplication in the database. As examples, Winchell et al. (2016) measured lower forelimb length as both the length of the ulna and length of the radius (from the same individual), and Lapiedra et al. (2017) measured boldness in the same individuals as latency to appear out of a refuge, latency to emerge from the refuge, and latency to climb on a perch. In all these instances, the estimates were highly correlated. Although we included study as a random effect to partially account for this, this still does not entirely remove this source of non-independence. Thus, when studies reported multiple estimates of the same trait from the same set of individuals, we only included one of these estimates and removed the others. We selected to include the estimate that was measured most-closely to the majority of the other traits in the same phenotypic category. For instance, we selected to include data on ulna length from Winchell et al. (2016) and remove data on radius length because most of the other studies within the lower forelimb category reported estimates of ulna length. This process lead to the removal of 74 effect sizes (12%) from the database, 16% from activity and exploration, 10% from boldness (exposure), 28% from boldness (latency), 7% from digit length (forelimb), 33% from digit length (hind limb), 11% from immunity, 26% from limb length (radius/ulna), 23% from limb length (tibia/fibula), and 38% from performance (Supplementary Table 4 shows the reduced database).
Furthermore, we performed subgroup analyses to determine the influence of particular groupings on the results. We were specifically interested in the effects of sex (i.e., were responses more or less pronounced in males?). Unfortunately, the only trait for which we could evaluate sex differences in responses was for body size (measured as snout-vent-length, svl). All other traits did not have adequate sample sizes of effect sizes, especially for female lizards (65% of all effect sizes came from males and 21% of effect sizes were from the sexes pooled). Thus, we ran a meta-regression model on body size (svl) effect sizes and set sex as the moderator variable (removing estimates from the sexes pooled so that we could assess males and females separately). We included the same random effects as above.
We also assessed the influence of Anolis lizards (family Dactyloidae), which contributed to ~75% of all effect sizes in the database, in driving the results. We removed all effect sizes associated with Anolis lizards and ran this reduced meta-regression model with trait as a moderator and the same random effects as above. However, this model only included the traits of body size (svl) and immunity as the removal of Anolis effect sizes made most traits unsuitable for analysis due to too small of sample sizes. Thus, in all, we could only look at the influence of sex and taxonomic bias using the body size (svl) trait data.
Results
Qualitative Synthesis
The number of studies published on lizards in urban environments has substantially grown in just the past 5 years (Figure 3). Yet, studies have been narrowly focused on only 22 different species in 9 families (Figure 2) even though lizards represent ~9% of all vertebrate life on Earth (Uetz, 2020). The vast majority of studies have been conducted on anoles in the family Dactyloidae. Specifically, Anolis cristatellus was the most studied species with roughly half of the studies on this species occurring in Miami, Florida, where this species is invasive. The remaining studies on this species occurred in Puerto Rico, where it is native. Studies have been geographically biased (Figure 4). Studies conducted in Puerto Rico and mainland United States contributed the most effects toward our analysis (111 and 105 effect sizes, respectively), with studies in Australia contributing the third most effects with only 20 effect sizes. Thus, we lack data from most continents even though lizards occur on every one except Antarctica. Effects from male lizards outnumbered effects from females and mixed sex samples combined (168, 44, and 62 effect sizes, respectively). Body size (as svl) was the most commonly reported phenotype with 40 effects from 21 of the studies, and morphology, in general, was the most commonly reported phenotype followed by behavior (192 and 62 effect sizes, respectively). Number of replicate sites used in each study ranged from 1–7, with an average of 2.5 urban sites, and non-urban replicates were slightly fewer with an average of 2.4 sites. Most studies were conducted in a single year (61%), but the overall range was 1–9 years.
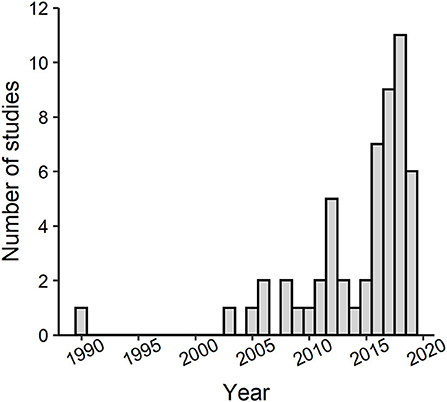
Figure 3. Histogram showing the number of studies produced per year for the past 30 years (each bar represents a single year) that have investigated effects of urbanization on lizard phenotypic traits. The bar associated with 2019 does not include all studies from that year because our literature search was conducted in May of 2019.
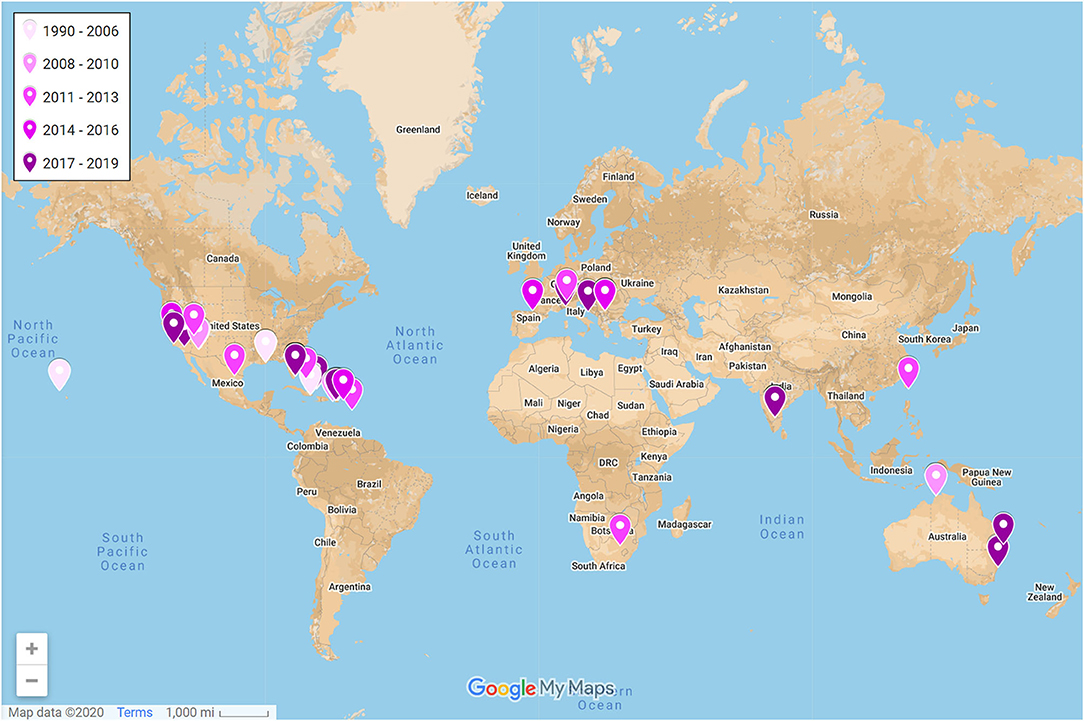
Figure 4. Geographic locations of studies on urban lizards included in the qualitative synthesis (N = 54). Points represent averaged centroids of urban replicate localities. Tags are colored by publication year; darker tags are more recent (range: 1990–2019). A full interactive evidence atlas can be found here: https://www.google.com/maps/d/edit?mid=1SAgOD-kWvbQfOXB9_Om-vQVAgk1udo8c&usp=sharing.
Quantitative Meta-Analysis
The effects of urbanization have led to significant increases in lizard body size (svl and mass) and limb and digit lengths, and a significant increase in the diameters of perches used (Figure 5A, Table 1). There was a marginal increase in running performance (sprint speed) with urbanization, but this was not significant in the reduced model when pseudoreplicated effect sizes were removed (Figure 5B, Table 1). Urbanization had large effects (SMD > 0.8) on body mass and the diameters of perches used, and medium effects (SMD > 0.5) on measures of limb length (except for humerus length), on measures of digit length, and on body length (svl). Urbanization did not affect two estimates of boldness (willingness to be exposed and latency to respond to stimuli), perch height, activity and exploration, body condition, or immune responses of lizards.
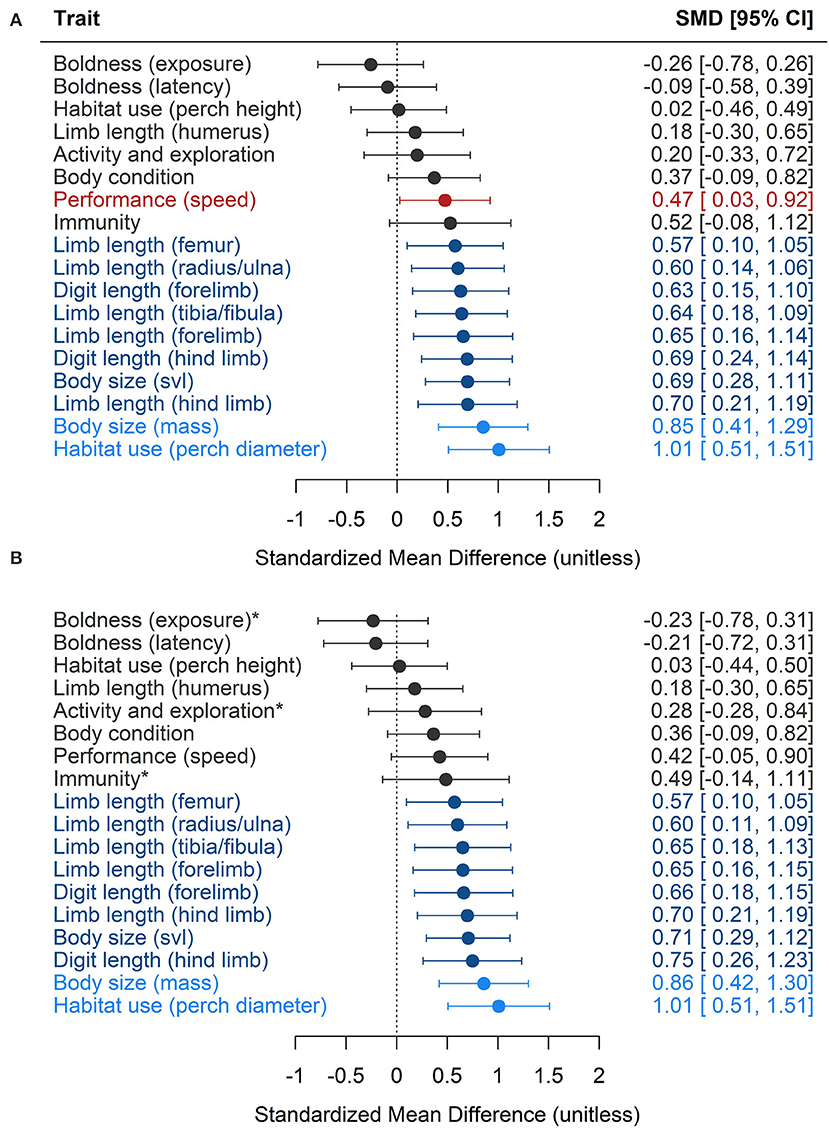
Figure 5. The effect of urbanization on lizard phenotypic traits in (A) the full model with all recorded effect sizes (n = 348), and (B) the reduced model with pseudoreplicated effect sizes removed (n = 274). Significant results are shown in blue (dark blue indicating medium effects, SMD > 0.5, and light blue indicating large effects, SMD > 0.8) and error bars represent 95% confidence intervals. One trait, running performance, is highlighted in red as it reached significance in the full model, but not in the reduced model. Traits in the reduced model that failed to meet the minimum sample size of 10 effect sizes are indicated with asterisks. Snout-vent-length, a measure of lizard body length, is abbreviated to svl.
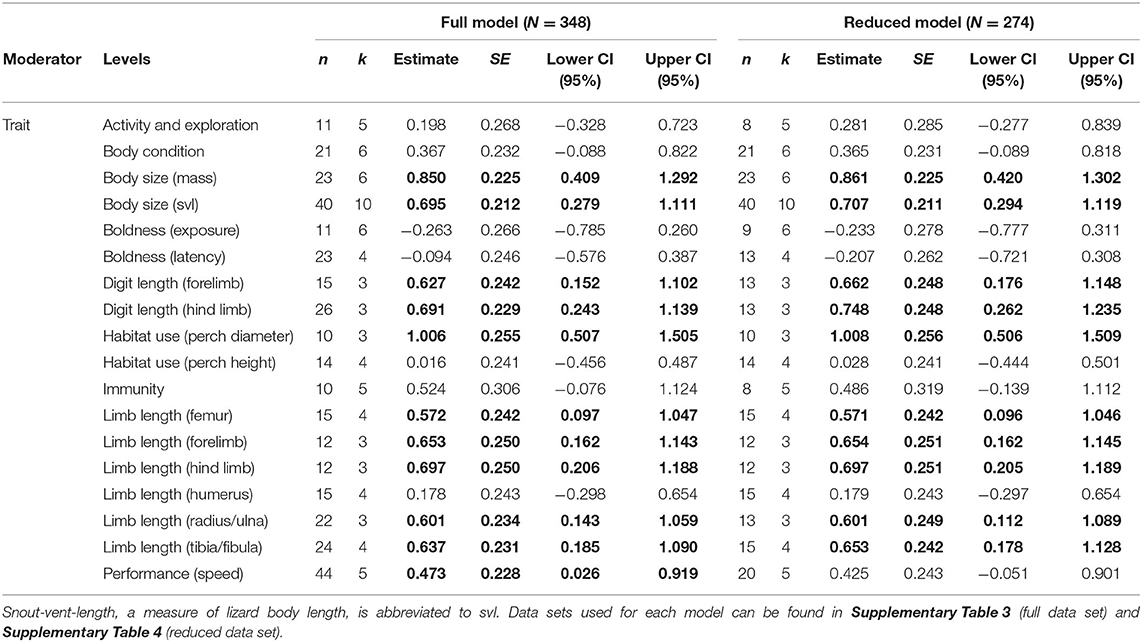
Table 1. Summaries of the meta–regression models with significant results shown in bold (n, number of effect sizes; k, number of lizard species).
We found high heterogeneity (I2 = 95.2%) in our meta-regression model. The effects of urbanization on lizard traits were largely unrelated to phylogenetic relatedness of the lizard species ( < 0.01%), and were similar within species ( = 5.7%), but urban locality displayed high variance ( = 59.9%), along with high variance at the effect size level ( = 18.7%). There was moderate between-study variance ( = 11.0%). When assessing publication bias, we found that although the Egger's test revealed asymmetry in our data set (z = 3.290, P = 0.001), the trim and fill function indicated that no studies were missing from the left-hand side of the funnel plot (P = 0.500, Supplementary Figure 2). All five profile plots for the variance components peaked at the respective parameter (REML) estimates and the log-likelihoods quickly decreased as the values of the components moved away from the estimates, indicating that the variance components were identifiable and our model was not overparameterized (Supplementary Figure 3).
When effect sizes from Anolis lizards were removed from the database, results on body size (svl) and immunity still held, with non-anoline lizards showing an increase in body size with urbanization (estimate ± SE: 0.594 ± 0.241, 95% CI: 0.122–1.066) and no shift in immune responses (estimate ± SE: 0.355 ± 0.269, 95% CI: −0.172–0.881). We found no effect of sex on body size: urbanization leads to larger body sizes in both female (estimate ± SE: 0.579 ± 0.252, 95% CI: 0.085–1.074) and male lizards (estimate ± SE: 0.703 ± 0.206, 95% CI: 0.300–1.107).
Discussion
Our knowledge on the effects of urbanization in lizards is still in its infancy. We show that studies are biased toward certain taxa, certain geographical locations, and we lack data on most phenotypic traits to conduct meaningful evaluations. Reptiles are important members of ecosystems worldwide, and with over 6,600 species, lizards make up more than 60% of all reptiles (Uetz, 2020). Yet, our synthesis shows that only 22 lizard species have been studied in the context of urbanization. Although not all lizard species filter into urban habitats (e.g., Shea, 2010), the paucity of studies on a variety of species is likely not due to lack of availability. An additional limitation in synthesizing data across urbanized areas is the differences in criteria used to define what is “urban.” Indeed, some studies were conducted in small villages whereas others were in large cities. The inclusion of urban locality as a random effect displayed high variance in the meta-regression model ( = 59.9%), suggesting that such differences among urban environments are important at influencing phenotypic responses. Finally, we also found that most studies are conducted in a single year and so we also lack data on seasonal or yearly variations in traits or information on how traits might change over time. In all, more research will be needed to produce meaningful generalizations on the effects of urbanization on this diverse group of vertebrates.
The most robust finding from our quantitative analysis is that urban lizards are larger than their non-urban counterparts. This finding remained robust even when accounting for sex and when removing data associated with anoles (family Dactyloidae). Our study therefore adds to the growing literature on shifts in animal body size associated with urbanization. Nonetheless, lizards do not respond in the same way as other animal groups. Almost all studies on urban invertebrates show a decline in body size with urbanization (Ulrich et al., 2008; Brans et al., 2017; Merckx et al., 2018; Eggenberger et al., 2019) and this is thought to be driven by the urban heat island effect because animals with larger surface area to volume ratios are favored under warmer conditions (Scheffers et al., 2016). Birds show a similar pattern with a general decrease in body size with urbanization (Kark et al., 2007; Liker et al., 2008; Meillère et al., 2015, 2017; Biard et al., 2017), although not all studies support this (Evans et al., 2009). Although these smaller body sizes could be due to nutritional deficits (Meillère et al., 2017), one study found that the difference in body size between urban and rural birds persisted even under laboratory conditions with access to the same amount of food (Liker et al., 2008). Thus, this could also be an evolutionary response to urban heat islands. Urban lizards have been found to physiologically respond to the increased temperature of cities (Campbell-Staton et al., 2020), but our results on body size contradict the findings on other urban taxa. Our results are more in line with those for mammals: larger species (Santini et al., 2019) or moderately-sized species (Bateman and Fleming, 2012) are more likely to be found within urban habitats. For mammals, body size promotes dispersal and ranging abilities, and so larger species do better in cities because they can access widely spaced habitat patches (Santini et al., 2013). However, most lizards do not have large home ranges like mammals, and it is unlikely that an increase in dispersal due to larger body size would benefit them in urban habitats. Thus, we find that different animal taxa respond in different ways and perhaps for different reasons. This information will be useful for holistically managing or conserving urban ecological communities.
An increase in lizard body size with urbanization could be the result of multiple non-mutually exclusive hypotheses, yet few studies have specifically tested these. First, urban lizards might have access to higher quality, more abundant, and/or more stable resources. This has been shown for other urban vertebrates such as birds, which benefit from supplemental feeding by humans through bird feeders (Robb et al., 2008). Although, it is unlikely that most humans are actively providing food for lizards as most consume invertebrate prey. However, lizard prey could become stable, abundant and concentrated around anthropogenic sources such as areas of human rubbish (Gross, 2015) or artificial lights (Perry et al., 2008), both of which attract different types of invertebrates. Iglesias et al. (2012) found support, through quantification of invertebrate prey abundance, for more stable resource availability in urban areas compared to natural areas. They also found that urban Lophognathus temporalis lizard populations consumed more food, on average, than non-urban populations. Changes in the types and diversity of available prey could also contribute to increased body size with urbanization. Indeed, studies show differential diets between urban and non-urban lizard populations with some species experiencing an increase in dietary breath (de Carvalho et al., 2007), while others showing a narrowing of diet (Balakrishna et al., 2016). Third, lizards might spend more time foraging in urban habitats because of a reduction in overall predation risk that occurs with urbanization (Eötvös et al., 2018). Based on risk-allocation theory, lizards can devote more time and energy toward foraging when predation risk is lower (Lima and Dill, 1990; Ydenberg, 2010). Conversely, a shift toward larger body sizes might be an evolutionary response to escape predation. Individuals of larger size are generally less susceptible to predation than smaller individuals because of enhanced locomotor performance (Irschick, 2000), greater bite force (defensive retaliation; Herrel and O'Reilly, 2006), or because they are released from being prey for small-bodied predators (Vitt, 2000). Cats have been shown to be a major predator of herpetofauna in urban areas (Loyd et al., 2013; Kitts-Morgan, 2015), and larger lizards might be better at escaping cat predation for the above-mentioned reasons. In all, these hypotheses remain untested and therefore, our meta-analysis indicates multiple directions for future research.
We also found that urban lizards use wide perches, most likely because they are climbing on human-made structures such as buildings and walls. They also tend to have longer limbs and digits than their non-urban counterparts. Previous studies have shown that longer limbs and toes, at least in anoles, enhance performance on broad and smooth substrates, such as concrete (Kolbe et al., 2016; Winchell et al., 2018). However, the increase in limb and toe lengths that we found might also be a result of increased body size as most of the means in our database were not scaled to body size (as reported by the authors). Also, these three phenotypic traits (perch width, limb lengths, and digit lengths) were measured mainly within a single lizard family, Dactyloidae, on just a few species of arboreal anoles. Because of this, we could not perform subgroup sensitivity analyses to determine the robustness of these findings beyond this taxonomic group or to determine whether non-arboreal lizards respond in similar ways. Thus, we are hesitant to make broad generalizations regarding the effect of urbanization on these traits. It remains to be determined how lizard species from other families will respond. For example, a study on non-anole lizards (Sceloporus occidentalis) in our database found a reduction in limb lengths with urbanization (Sparkman et al., 2018). A more recent study, not included in our analysis because it was published after our literature search, also found the same pattern of reduced limb and toe lengths in urban populations of S. occidentalis (Putman et al., 2019a). Sceloporus occidentalis is less arboreal than most anoles and this may explain these conflicting results. In all, our qualitative synthesis and meta-analysis indicate that research on lizard species outside of Dactyloidae, and on lizard taxa that vary in life history and in ecomorphological characteristics, will be valuable in making generalizable predictions, which we are unable to do at this time based on the current state of the literature.
Other than habitat use (perch width), we failed to find consistent shifts in behavioral responses with urbanization. For the two personality traits, activity/exploration and boldness, we did not detect a general effect of urbanization, even though urban populations of other taxa display consistent shifts (Lowry et al., 2013; Sol et al., 2013). Previous research and a global quantitative synthesis have shown urban animals to be more bold in terms of escape responses such as flight initiation distance (FID) (Møller et al., 2015; Samia et al., 2015). We had an inadequate sample size to test for this in our data set; only a handful of studies have compared FID between urban and non-urban lizard populations. It is possible that we did not find patterns as strong as those for morphological traits because behaviors are more difficult to quantify in a standardized manner across studies. Even though we attempted to group behaviors based on assays used for quantification, such as latency to respond to stimuli or willingness to be exposed, other factors could influence these traits such as whether traits were measured in the lab or field, lizard body temperature, and even the season or time of testing. Morphological traits, such as body length or limb length, are less sensitive to such factors. Clearly more research is needed to determine whether boldness, exploration, activity, sociability, or other personality traits generally associate with urbanization in lizards.
Our meta-analysis reveals various phenotypic shifts in lizards due to urbanization, yet we are unable to determine whether these are associated with underlying genetic changes. A few studies assessed the heritability of traits through common garden rearing of offspring from urban and natural habitats (Winchell et al., 2016; Hall and Warner, 2017; Tiatragul et al., 2017). Other studies (not included in our analysis) have shown strong morphological and genetic differentiation among lizard populations living in urban parks in a single city (Littleford-Colquhoun et al., 2017), and tolerance of higher temperatures (in response to the urban heat island effect) in urban Anolis cristatellus populations has been linked to a single gene polymorphism (Campbell-Staton et al., 2020). Together, these studies provide support for rapid evolution of traits due to urbanization, a phenomenon that appears to induce greater rates of phenotypic change than other forms of human disturbance (Alberti et al., 2017). More studies are needed though to determine the exact mechanisms driving phenotypic change as shifts could also be due to phenotypic plasticity or differential sorting of individuals into urban habitats. This information is important because populations could have limits to plasticity (van Baaren and Candolin, 2018) and sorting of individuals could reduce genetic diversity, limiting urban populations' abilities to respond to additional environmental disturbances (Barrett and Schluter, 2008).
Finally, we also lack support for whether the phenotypic shifts associated with urban living improve fitness within these anthropogenic habitats. It is possible that larger body sizes correlate with larger clutch or egg sizes (Dunham and Miles, 1985). Yet, even though we have documented some shifts in lizard phenotypes due to urbanization, these responses can also be maladaptive, creating evolutionary and ecological traps (Robertson et al., 2013; Hale and Swearer, 2016), or they could be insufficient to overcome novel selection pressures leading to extirpation of populations over time (Sinervo et al., 2010). A few species in our dataset are invasive at the locations where they were studied (e.g., Anolis cristatellus and A. sagrei), and these species might have higher fitness in urban areas because their evolutionary histories are often associated with humans and anthropogenic habitats (Hufbauer et al., 2012). We did not test for the effects of invasive status in our model, but further studies on this would improve our understanding of interspecific differences in responses to urbanization. Hall and Warner (2017) found that the large body size of urban invasive female anoles was associated with higher body condition and fecundity as these females starting laying eggs earlier in the season than females from non-urban habitats. Lucas and French (2012) also found urban populations of Uta stansburiana had higher fecundity (larger clutches and eggs) than non-urban populations, but this was at the cost of reduced survival. These studies provide partial support that lizards are responding adaptively to urbanization, yet Tiatragul et al. (2017) found that urban anole embryos were not more robust to urban thermal environments than non-urban embryos, suggesting a lack of adaptation. In sum, we will need much more research to determine the fitness impacts of phenotypic responses, which could set priorities for conservation management of urban populations.
Conclusions
Our synthesis of the current urban lizard literature reveals large data gaps in various areas including geographic, taxonomic, and phenotypic extent. Even so, our meta-analysis reveals a robust finding of an increase in lizard body size with urbanization, although we still do not know the mechanism(s) driving this phenotypic shift. We demonstrate that this response differs from that of other urban animal groups, namely invertebrates and birds. Further research is needed to understand whether documented shifts in phenotypic traits are adaptive and/or great enough to withstand the pressures of urban life. In sum, our study provides a path forward for future studies and contributes to the growing literature on animal responses to rapid human-induced habitat change.
Data Availability Statement
The original contributions presented in the study are included in the article/Supplementary Materials, further inquiries can be directed to the corresponding author/s.
Author Contributions
BP conceived of the study and performed the literature search and study selection process. BP and ZT extracted the data from studies. ZT conducted the qualitative synthesis and BP conducted the phylogenetic meta-analysis. Both authors contributed to writing the article.
Conflict of Interest
The authors declare that the research was conducted in the absence of any commercial or financial relationships that could be construed as a potential conflict of interest.
Acknowledgments
We thank all the authors who contributed data to our synthesis, Sarah Berryman and Nina Fresco for help with data extraction, and Matthew Holding for help with obtaining and trimming the phylogenetic tree used in our analysis.
Supplementary Material
The Supplementary Material for this article can be found online at: https://www.frontiersin.org/articles/10.3389/fevo.2020.580745/full#supplementary-material
References
Alberti, M., Correa, C., Marzluff, J. M., Hendry, A. P., Palkovacs, E. P., Gotanda, K. M., et al. (2017). Global urban signatures of phenotypic change in animal and plant populations. Proc. Natl. Acad. Sci. U.S.A. 114, 8951–8956. doi: 10.1073/pnas.1606034114
Angilletta, M. J., Wilson, R. S., Niehaus, A. C., Sears, M. W., Navas, C. A., and Ribeiro, P. L. (2007). Urban physiology: city ants possess high heat tolerance. PLoS ONE 2:e258. doi: 10.1371/journal.pone.0000258
Balakrishna, S., Batabyal, A., and Thaker, M. (2016). Dining in the city: dietary shifts in Indian rock agamas across an urban-rural landscape. J. Herpetol. 50, 423–428. doi: 10.1670/14-073
Barrett, R. D. H., and Schluter, D. (2008). Adaptation from standing genetic variation. Trends Ecol. Evol. 23, 38–44. doi: 10.1016/j.tree.2007.09.008
Bateman, P. W., and Fleming, P. A. (2012). Big city life: carnivores in urban environments. J. Zool. 287, 1–23. doi: 10.1111/j.1469-7998.2011.00887.x
Benítez-López, A., Alkemade, R., and Verweij, P. A. (2010). The impacts of roads and other infrastructure on mammal and bird populations: a meta-analysis. Biol. Conserv. 143, 1307–1316. doi: 10.1016/j.biocon.2010.02.009
Berthouly-Salazar, C., van Rensburg, B. J., Le Roux, J. J., van Vuuren, B. J., and Hui, C. (2012). Spatial sorting drives morphological variation in the invasive bird, Acridotheris tristis. PLoS ONE 7:e38145. doi: 10.1371/journal.pone.0038145
Biard, C., Brischoux, F., Meillère, A., Michaud, B., Nivière, M., Ruault, S., et al. (2017). Growing in cities: an urban penalty for wild birds? A study of phenotypic differences between urban and rural great tit chicks (Parus major). Front. Ecol. Evol. 5:79. doi: 10.3389/fevo.2017.00079
Biermann, F., Bai, X., Bondre, N., Broadgate, W., Arthur Chen, C. T., Dube, O. P., et al. (2016). Down to earth: contextualizing the anthropocene. Glob. Environ. Change 39, 341–350. doi: 10.1016/j.gloenvcha.2015.11.004
Borenstein, M., Hedges, L. V., Higgins, J. P. T., and Rothstein, H. R. (2009). Introduction to Meta-Analysis. Chichester: John Wiley & Sons, Ltd
Brans, K. I., Jansen, M., Vanoverbeke, J., Tüzün, N., Stoks, R., and De Meester, L. (2017). The heat is on: genetic adaptation to urbanization mediated by thermal tolerance and body size. Glob. Chang. Biol. 23, 5218–5227. doi: 10.1111/gcb.13784
Camargo, A., Sinervo, B., and Sites, J. W. (2010). Lizards as model organisms for linking phylogeographic and speciation studies. Mol. Ecol. 19, 3250–3270. doi: 10.1111/j.1365-294X.2010.04722.x
Campbell-Staton, S. C., Winchell, K. M., Rochette, N. C., Fredette, J., Maayan, I., Schweizer, R. M., et al. (2020). Parallel selection on thermal physiology facilitates repeated adaptation of city lizards to urban heat islands. Nat. Ecol. Evol. 4, 652–658. doi: 10.1038/s41559-020-1131-8
Chamberlain, D. E., Cannon, A. R., Toms, M. P., Leech, D. I., Hatchwell, B. J., and Gaston, K. J. (2009). Avian productivity in urban landscapes: a review and meta-analysis. Ibis 151, 1–18. doi: 10.1111/j.1474-919X.2008.00899.x
Croci, S., Butet, A., and Clergeau, P. (2008). Does urbanization filter birds on the basis of their biological traits? Condor 110, 223–240. doi: 10.1525/cond.2008.8409
de Carvalho, A. L. G., da Silva, H. R., de Araújo, A. F. B., Alves-Silva, R., and da Silva-Leite, R. R. (2007). Feeding ecology of Tropidurus torquatus (Wied) (Squamata, Tropiduridae) in two areas with different degrees of conservation in Marambaia Island, Rio de Janeiro, Southeastern Brazil. Rev. Bras. Zool. 24, 222–227. doi: 10.1590/S0101-81752007000100029
de Miranda, E. B. P. (2017). The plight of reptiles as ecological actors in the tropicss. Front. Ecol. Evol. 5:159. doi: 10.3389/fevo.2017.00159
Dirzo, R., Young, H. S., Galetti, M., Ceballos, G., Isaac, N. J. B., and Collen, B. (2014). Defaunation in the anthropocene. Science 345, 401–406. doi: 10.1126/science.1251817
Dobbs, C., Nitschke, C., and Kendal, D. (2017). Assessing the drivers shaping global patterns of urban vegetation landscape structure. Sci. Total Environ. 592, 171–177. doi: 10.1016/j.scitotenv.2017.03.058
Doherty, T. S., Balouch, S., Bell, K., Burns, T. J., Feldman, A., Fist, C., et al. (2020). Reptile responses to anthropogenic habitat modification: a global meta-analysis. Glob. Ecol. Biogeogr. 29, 1265–1279. doi: 10.1111/geb.13091
Dunham, A. E., and Miles, D. B. (1985). Patterns of covariation in life history traits of squamate reptiles: the effects of size and phylogeny reconsidered. Am. Nat. 126, 231–257. doi: 10.1086/284411
Eggenberger, H., Frey, D., Pellissier, L., Ghazoul, J., Fontana, S., and Moretti, M. (2019). Urban bumblebees are smaller and more phenotypically diverse than their rural counterparts. J. Anim. Ecol. 88, 1522–1533. doi: 10.1111/1365-2656.13051
Eötvös, C. B., Magura, T., and Lövei, G. L. (2018). A meta-analysis indicates reduced predation pressure with increasing urbanization. Landsc. Urban Plan. 180, 54–59. doi: 10.1016/j.landurbplan.2018.08.010
Evans, K. L., Chamberlain, D. E., Hatchwell, B. J., Gregory, R. D., and Gaston, K. J. (2011). What makes an urban bird? Glob. Chang. Biol. 17, 32–44. doi: 10.1111/j.1365-2486.2010.02247.x
Evans, K. L., Gaston, K. J., Sharp, S. P., Mcgowan, A., and Hatchwell, B. J. (2009). The effect of urbanisation on avian morphology and latitudinal gradients in body size. Oikos 118, 251–259. doi: 10.1111/j.1600-0706.2008.17092.x
French, S. S., Webb, A. C., Hudson, S. B., and Virgin, E. E. (2018). Town and country reptiles: a review of reptilian responses to urbanization. Integr. Comp. Biol. 58, 948–966. doi: 10.1093/icb/icy052
Gaynor, K. M., Hojnowski, C. E., Carter, N. H., and Brashares, J. S. (2018). The influence of human disturbance on wildlife nocturnality. Science 360, 1232–1235. doi: 10.1126/science.aar7121
Giraudeau, M., Nolan, P. M., Black, C. E., Earl, S. R., Hasegawa, M., and McGraw, K. J. (2014). Song characteristics track bill morphology along a gradient of urbanization in house finches (Haemorhous mexicanus). Front. Zool. 11:83. doi: 10.1186/s12983-014-0083-8
Grimm, N. B., Faeth, S. H., Golubiewski, N. E., Redman, C. L., Wu, J., Bai, X., et al. (2008). Global change and the ecology of cities. Science 319, 756–760. doi: 10.1126/science.1150195
Gross, S. D. (2015). Carrion-associated arthropods in rural and urban environments. (Dissertation), Purdue University, West Lafayette, IN (USA).
Hale, R., and Swearer, S. E. (2016). Ecological traps: current evidence and future directions. Proc. R. Soc. London B. 283:20152647. doi: 10.1098/rspb.2015.2647
Hall, J. M., and Warner, D. A. (2017). Body size and reproduction of a non-native lizard are enhanced in an urban environment. Biol. J. Linn. Soc. 122, 860–871. doi: 10.1093/biolinnean/blx109
Hamer, A. J., and McDonnell, M. J. (2008). Amphibian ecology and conservation in the urbanising world: a review. Biol. Conserv. 141, 2432–2449. doi: 10.1016/j.biocon.2008.07.020
Hedges, L. V. (1981). Distribution theory for Glass's estimator of effect size and related estimators. J. Educ. Stat. 6, 107–128. doi: 10.3102/10769986006002107
Herrel, A., and O'Reilly, J. C. (2006). Ontogenetic scaling of bite force in lizards and turtles. Physiol. Biochem. Zool. 79, 31–42. doi: 10.1086/498193
Hufbauer, R. A., Facon, B., Ravigne, V., Turgeon, J., Foucaud, J., et al. (2012). Anthropogenically induced adaptation to invade (AIAI): contemporary adaptation to human-altered habitats within the native range can promote invasions. Evol. Appl. 5, 89–101. doi: 10.1111/j.1752-4571.2011.00211.x
Hulley, M. E. (2012). “The urban heat island effect: Causes and potential solutions,” in Metropolitan Sustainability, ed F. Zeman (Cambridge: Woodhead Publishing), 79–98.
Iglesias, S., Tracy, C., Bedford, G., and Christian, K. (2012). Habitat differences in body size and shape of the Australian agamid lizard, Lophognathus temporalis. J. Herpetol. 46, 297–303. doi: 10.1670/11-084
Irschick, D. J. (2000). Effects of behaviour and ontogeny on the locomotor performance of a West Indian lizard, Anolis lineatopus. Funct. Ecol. 14, 438–444. doi: 10.1046/j.1365-2435.2000.00447.x
Jessop, T. S., Ariefiandy, A., Forsyth, D. M., Purwandana, D., White, C. R., Benu, Y. J., et al. (2020). Komodo dragons are not ecological analogs of apex mammalian predators. Ecology 101:e02970. doi: 10.1002/ecy.2970
Johnson, M. T. J., and Munshi-South, J. (2017). Evolution of life in urban environments. Science 358:eaam8327. doi: 10.1126/science.aam8327
Kark, S., Iwaniuk, A., Schalimtzek, A., and Banker, E. (2007). Living in the city: can anyone become an “urban exploiter”? J. Biogeogr. 34, 638–651. doi: 10.1111/j.1365-2699.2006.01638.x
Kettel, E. F., Gentle, L. K., Quinn, J. L., and Yarnell, R. W. (2018). The breeding performance of raptors in urban landscapes: a review and meta-analysis. J. Ornithol. 159, 1–18. doi: 10.1007/s10336-017-1497-9
Kinnison, M. T., and Hendry, A. P. (2001). The pace of modern life II: from rates of contemporary microevolution to pattern and process. Genetica 112–113, 145–164. doi: 10.1007/978-94-010-0585-2_10
Kitts-Morgan, S. E. (2015). Sustainable ecosystems: domestic cats and their effect on wildlife populations. J. Anim. Sci. 93, 848–859. doi: 10.2527/jas.2014-8557
Kolbe, J. J., Battles, A. C., and Aviles-Rodriguez, K. J. (2016). City slickers: poor performance does not deter Anolis lizards from using artificial substrates in human-modified habitats. Funct. Ecol. 30, 1418–1429. doi: 10.1111/1365-2435.12607
Konstantopoulos, S. (2011). Fixed effects and variance components estimation in three-level meta-analysis. Res. Synth. Methods. 2, 61–76. doi: 10.1002/jrsm.35
LaMontagne, J. M., Kilgour, R. J., Anderson, E. C., and Magle, S. (2015). Tree cavity availability across forest, park, and residential habitats in a highly urban area. Urban Ecosyst. 18, 151–167. doi: 10.1007/s11252-014-0383-y
Lapiedra, O. (2018). Urban behavioral ecology: lessons from Anolis lizards. Integr. Comp. Biol. 58, 939–947. doi: 10.1093/icb/icy109
Lapiedra, O., Chejanovski, Z., and Kolbe, J. J. (2017). Urbanization and biological invasion shape animal personalities. Glob. Chang. Biol. 23, 592–603. doi: 10.1111/gcb.13395
Lewis, S. L., and Maslin, M. A. (2015). Defining the anthropocene. Nature 519, 171–180. doi: 10.1038/nature14258
Liker, A., Papp, Z., Bókony, V., and Lendvai, Á. Z. (2008). Lean birds in the city: body size and condition of house sparrows along the urbanization gradient. J. Anim. Ecol. 77, 789–795. doi: 10.1111/j.1365-2656.2008.01402.x
Lima, S. L., and Dill, L. M. (1990). Behavioral decisions made under the risk of predation: a review and prospectus. Can. J. Zool. 68, 619–640. doi: 10.1139/z90-092
Littleford-Colquhoun, B. L., Clemente, C., Whiting, M. J., Ortiz-Barrientos, D., and Frere, C. H. (2017). Archipelagos of the Anthropocene: rapid and extensive differentiation of native terrestrial vertebrates in a single metropolis. Mol. Ecol. 26, 2466–2481. doi: 10.1111/mec.14042
Łopucki, R., and Kitowski, I. (2017). How small cities affect the biodiversity of ground-dwelling mammals and the relevance of this knowledge in planning urban land expansion in terms of urban wildlife. Urban Ecosyst. 20, 933–943. doi: 10.1007/s11252-016-0637-y
Lowry, H., Lill, A., and Wong, B. B. M. (2013). Behavioural responses of wildlife to urban environments. Biol. Rev. 88, 537–549. doi: 10.1111/brv.12012
Loyd, K. A. T., Hernandez, S. M., Carroll, J. P., Abernathy, K. J., and Marshall, G. J. (2013). Quantifying free-roaming domestic cat predation using animal-borne video cameras. Biol. Conserv. 160, 183–189. doi: 10.1016/j.biocon.2013.01.008
Lucas, L. D., and French, S. S. (2012). Stress-induced tradeoffs in a free-living lizard across a variable landscape: consequences for individuals and populations. PLoS ONE 7:e49895. doi: 10.1371/journal.pone.0049895
Mallen-Cooper, M., Nakagawa, S., and Eldridge, D. J. (2019). Global meta-analysis of soil-disturbing vertebrates reveals strong effects on ecosystem patterns and processes. Glob. Ecol. Biogeogr. 28, 661–679. doi: 10.1111/geb.12877
Martin, P. R., and Bonier, F. (2018). Species interactions limit the occurrence of urban-adapted birds in cities. Proc. Natl. Acad. Sci. U.S.A. 115, E11495–E11504. doi: 10.1073/pnas.1809317115
McDonnell, M. J., and Hahs, A. K. (2015). Adaptation and adaptedness of organisms to urban environments. Annu. Rev. Ecol. Evol. Syst. 46, 261–280. doi: 10.1146/annurev-ecolsys-112414-054258
McKinney, M. L. (2008). Effects of urbanization on species richness: a review of plants and animals. Urban Ecosyst. 11, 161–176. doi: 10.1007/s11252-007-0045-4
Meillère, A., Brischoux, F., Henry, P. Y., Michaud, B., Garcin, R., and Angelier, F. (2017). Growing in a city: consequences on body size and plumage quality in an urban dweller, the house sparrow (Passer domesticus). Landsc. Urban Plan. 160, 127–138. doi: 10.1016/j.landurbplan.2016.12.014
Meillère, A., Brischoux, F., Parenteau, C., and Angelier, F. (2015). Influence of urbanization on body size, condition, and physiology in an urban exploiter: a multi-component approach. PLoS ONE 10:e0135685. doi: 10.1371/journal.pone.0135685
Merckx, T., Souffreau, C., Kaiser, A., Baardsen, L. F., Backeljau, T., Bonte, D., et al. (2018). Body-size shifts in aquatic and terrestrial urban communities. Nature 558, 113–116. doi: 10.1038/s41586-018-0140-0
Miller, C. R., Latimer, C. E., and Zuckerberg, B. (2018). Bill size variation in northern cardinals associated with anthropogenic drivers across North America. Ecol. Evol. 8, 4841–4851. doi: 10.1002/ece3.4038
Miranda, A. C., Schielzeth, H., Sonntag, T., and Partecke, J. (2013). Urbanization and its effects on personality traits: a result of microevolution or phenotypic plasticity? Glob. Chang. Biol. 19, 2634–2644. doi: 10.1111/gcb.12258
Moher, D., Liberati, A., Tetzlaff, J., Altman, D. G., Altman, D., Antes, G., et al. (2009). Preferred reporting items for systematic reviews and meta-analyses: the PRISMA statement. PLoS Med. 6:e1000097. doi: 10.1371/journal.pmed.1000097
Møller, A. P. (2009). Successful city dwellers: a comparative study of the ecological characteristics of urban birds in the western palearctic. Oecologia 159, 849–858. doi: 10.1007/s00442-008-1259-8
Møller, A. P., Tryjanowski, P., Diaz, M., Kwiecinski, Z., Indykiewicz, P., Mitrus, C., et al. (2015). Urban habitats and feeders both contribute to flight initiation distance reduction in birds. Behav. Ecol. 26, 861–865. doi: 10.1093/beheco/arv024
Nakagawa, S., Noble, D. W. A., Senior, A. M., and Lagisz, M. (2017). Meta-evaluation of meta-analysis: Ten appraisal questions for biologists. BMC Biol. 15:18. doi: 10.1186/s12915-017-0357-7
Nakagawa, S., and Santos, E. S. A. (2012). Methodological issues and advances in biological meta-analysis. Evol. Ecol. 26, 1253–1274. doi: 10.1007/s10682-012-9555-5
Nelson, J. A., Atzori, F., and Gastrich, K. R. (2015). Repeatability and phenotypic plasticity of fish swimming performance across a gradient of urbanization. Environ. Biol. Fishes 98, 1431–1447. doi: 10.1007/s10641-014-0369-x
Nowak, E. M., Theimer, T. C., and Schuett, G. W. (2008). Functional and numerical responses of predators: where do vipers fit in the traditional paradigms? Biol. Rev. Camb. Philos. Soc. 83, 601–620. doi: 10.1111/j.1469-185X.2008.00056.x
Ordeñana, M. A., Crooks, K. R., Boydston, E. E., Fisher, R. N., Lyren, L. M., Siudyla, S., et al. (2010). Effects of urbanization on carnivore species distribution and richness. J. Mammal. 91, 1322–1331. doi: 10.1644/09-MAMM-A-312.1
Perry, G., Buchanan, B. W., Fisher, R. N., Salmon, M., and Wise, S. E. (2008). “Effects of night lighting on urban reptiles and amphibians,” in Urban Herpetology, eds J. C. Mitchell, R. E. Jung Brown, and B. Bartholomew (Society for the Study of Amphibians and Reptiles) (Salt Lake City), 239–256.
Piano, E., De Wolf, K., Bona, F., Bonte, D., Bowler, D. E., Isaia, M., et al. (2017). Urbanization drives community shifts towards thermophilic and dispersive species at local and landscape scales. Glob. Chang. Biol. 23, 2554–2564. doi: 10.1111/gcb.13606
Putman, B. J., Gasca, M., Blumstein, D. T., and Pauly, G. B. (2019a). Downsizing for downtown : limb lengths, toe lengths, and scale counts decrease with urbanization in western fence lizards (Sceloporus occidentalis). Urban Ecosyst. 22, 1071–1081. doi: 10.1007/s11252-019-00889-z
Putman, B. J., Samia, D. S. M., Cooper, W. E., and Blumstein, D. T. (2019b). “Impact of human-induced environmental changes on lizard behavior: insights from urbanization,” in Behavior of Lizards, eds V. L. Bels and A. Russell (Boca Raton: CRC Press), 375–396.
Pyron, R., Burbrink, F., and Wiens, J. (2013). A phylogeny and revised classification of Squamata, including 4161 species of lizards and snakes. BMC Evol. Biol. 13:93. doi: 10.1186/1471-2148-13-93
Robb, G. N., McDonald, R. A., Chamberlain, D. E., and Bearhop, S. (2008). Food for thought: supplementary feeding as a driver of ecological change in avian populations. Front. Ecol. Environ. 6, 476–484. doi: 10.1890/060152
Robertson, B. A., Rehage, J. S., and Sih, A. (2013). Ecological novelty and the emergence of evolutionary traps. Trends Ecol. Evol. 28, 552–560. doi: 10.1016/j.tree.2013.04.004
Roca, I. T., Desrochers, L., Giacomazzo, M., Bertolo, A., Bolduc, P., Deschesnes, R., et al. (2016). Shifting song frequencies in response to anthropogenic noise: a meta-analysis on birds and anurans. Behav. Ecol. 27, 1269–1274. doi: 10.1093/beheco/arw060
Saito, M., and Koike, F. (2013). Distribution of wild mammal assemblages along an urban-rural-forest landscape gradient in warm-temperate East Asia. PLoS ONE 8:e65464. doi: 10.1371/journal.pone.0065464
Samia, D. S. M., Nakagawa, S., Nomura, F., Rangel, T. F., and Blumstein, D. T. (2015). Increased tolerance to humans among disturbed wildlife. Nat. Commun. 6:8877. doi: 10.1038/ncomms9877
Santini, L., Di Marcc, M., Visconti, P., Baiserc, D., Boitanl, L., and Rondinini, C. (2013). Ecological correlates of dispersal distance in terrestrial mammals. Hystrix 24, 181–186. doi: 10.4404/hystrix-24.2-8746
Santini, L., González-Suárez, M., Russo, D., Gonzalez-Voyer, A., von Hardenberg, A., and Ancillotto, L. (2019). One strategy does not fit all: determinants of urban adaptation in mammals. Ecol. Lett. 22, 365–376. doi: 10.1111/ele.13199
Scheffers, B. R., de Meester, L., Bridge, T. C. L., Hoffmann, A. A., Pandolfi, J. M., Corlett, R. T., et al. (2016). The broad footprint of climate change from genes to biomes to people. Science 354:aaf7671. doi: 10.1126/science.aaf7671
Schochet, A. B., Hung, K. L. J., and Holway, D. A. (2016). Bumble bee species exhibit divergent responses to urbanisation in a Southern California landscape. Ecol. Entomol. 41, 685–692. doi: 10.1111/een.12343
Seress, G., and Liker, A. (2015). Habitat urbanization and its effects on birds. Acta Zool. Acad. Sci. Hungaricae 61, 373–408. doi: 10.17109/AZH.61.4.373.2015
Shea, G. M. (2010). “The suburban terrestrial reptile fauna of Sydney - winners and losers,” in The Natural History of Sydney, eds D. Lunney, P. Hutchings, and D. Hochuli (Mosman: Royal Zoological Society of NSW), 154–197.
Silva, C. P., Sepúlveda, R. D., and Barbosa, O. (2016). Nonrandom filtering effect on birds: species and guilds response to urbanization. Ecol. Evol. 6, 3711–3720. doi: 10.1002/ece3.2144
Sinervo, B., Mendez-de-la-Cruz, F., Miles, D. B., Heulin, B., Bastiaans, E., Villagran-Santa Cruz, M., et al. (2010). Erosion of lizard diversity by climate change and altered thermal niches. Science 328, 894–899. doi: 10.1126/science.1184695
Sol, D., Lapiedra, O., and Gonzalez-Lagos, C. (2013). Behavioural adjustments for a life in the city. Anim. Behav. 85, 1101–1112. doi: 10.1016/j.anbehav.2013.01.023
Sparkman, A., Howe, S., Hynes, S., Hobbs, B., and Handal, K. (2018). Parallel behavioral and morphological divergence in fence lizards on two college campuses. PLoS ONE 13:e191800. doi: 10.1371/journal.pone.0191800
Symonds, M. R. E., Weston, M. A., van Dongen, W. F. D., Lill, A., Robinson, R. W., and Guay, P. J. (2016). Time since urbanization but not encephalisation is associated with increased tolerance of human proximity in birds. Front. Ecol. Evol. 4:117. doi: 10.3389/fevo.2016.00117
Tiatragul, S., Kurniawan, A., Kolbe, J. J., and Warner, D. A. (2017). Embryos of non-native anoles are robust to urban thermal environments. J. Therm. Biol. 65, 119–124. doi: 10.1016/j.jtherbio.2017.02.021
Uetz, P. (2020). Species Statistics August 2019. Available online at: http://www.reptile-database.org/db-info/SpeciesStat.html (accessed July 5, 2020).
Ulrich, W., Komosiński, K., and Zalewski, M. (2008). Body size and biomass distributions of carrion visiting beetles: do cities host smaller species? Ecol. Res. 23, 241–248. doi: 10.1007/s11284-007-0369-9
United Nations (2019). World Urbanization Prospects: The 2018 Revision. New York, NY: United Nations.
van Baaren, J., and Candolin, U. (2018). Plasticity in a changing world: behavioural responses to human perturbations. Curr. Opin. Insect Sci. 27, 21–25. doi: 10.1016/j.cois.2018.02.003
Viechtbauer, W. (2010). Conducting meta-analyses in R with the metafor. J. Stat. Softw. 36, 1–48. doi: 10.18637/jss.v036.i03
Vitt, L. J. (2000). Ecological consequences of body size in neonatal and small-bodied lizards in the Neotropics. Herpetol. Monogr. 14, 388–400. doi: 10.2307/1467053
Voigt, C. C., and Kingston, T. (2015). Bats in the Anthropocene: Conservation of Bats in a Changing World. New York, NY: Springer.
Winchell, K. M., Maayan, I., Fredette, J. R., and Revell, L. J. (2018). Linking locomotor performance to morphological shifts in urban lizards. Proc. R. Soc. B. 285:20180229. doi: 10.1098/rspb.2018.0229
Winchell, K. M., Reynolds, R. G., Prado-Irwin, S. R., Puente-Rolon, A. R., and Revell, L. J. (2016). Phenotypic shifts in urban areas in the tropical lizard Anolis cristatellus. Evolution 70, 1009–1022. doi: 10.1111/evo.12925
Keywords: ectotherm, evidence synthesis, human induced environmental change, meta-regression, urban ecology, urban evolution
Citation: Putman BJ and Tippie ZA (2020) Big City Living: A Global Meta-Analysis Reveals Positive Impact of Urbanization on Body Size in Lizards. Front. Ecol. Evol. 8:580745. doi: 10.3389/fevo.2020.580745
Received: 06 July 2020; Accepted: 22 October 2020;
Published: 27 November 2020.
Edited by:
David Andrew Luther, George Mason University, United StatesReviewed by:
Anthony Herrel, Muséum National d'Histoire Naturelle, FranceYlenia Chiari, George Mason University, United States
Copyright © 2020 Putman and Tippie. This is an open-access article distributed under the terms of the Creative Commons Attribution License (CC BY). The use, distribution or reproduction in other forums is permitted, provided the original author(s) and the copyright owner(s) are credited and that the original publication in this journal is cited, in accordance with accepted academic practice. No use, distribution or reproduction is permitted which does not comply with these terms.
*Correspondence: Breanna J. Putman, breanna.putman@csusb.edu