- Illinois Natural History Survey, Prairie Research Institute, University of Illinois, Champaign, IL, United States
Global climate change and warming are altering hemispheric and local weather patterns. Altered weather patterns have great potential to affect the phenology of life history events, such as the initiation of breeding in organisms that reproduce seasonally. Long-distance migratory birds may be particularly challenged by changes in local weather on breeding grounds because they arrive from distant locations and must commence breeding when conditions are appropriate. Here we explore the effects of local temperature on first egg dates and annual productivity in a long-distance Neotropical migratory songbird, the prothonotary warbler Protonotaria citrea. We present results from a 20-year (1994 to 2013) study documenting the detailed nesting activities of a color-marked population (average of 155 individual females each year) of warblers in southern Illinois, United States. The warblers typically arrive in April and start breeding in late April and May in our study system. We tested for an effect of local average April daily temperature and female age on first egg dates, total number of offspring produced per female, and the probability of fledging two broods. We found that warmer April temperatures promoted earlier first egg dates and higher average annual productivity in the warblers. On average, older females had earlier first egg dates than 1-year-old females, but both age groups responded similarly to local April temperatures. The reproductive gains associated with earlier first egg dates in warmer years stemmed from an increased probability of successfully fledging two broods, suggesting that earlier first egg dates do not currently create a mismatch with food (insect) resources. Earliest arrival dates of warblers to the region of our study system were not affected by local April temperatures, suggesting that females vary their first egg date based on conditions they experience/assess after their arrival. Whereas these birds currently adjust the timing of their breeding and actually produce more offspring in warmer years, continued global warming may eventually upset the current balance between arrival dates, food resources, and the commencement of nesting.
Introduction
Natural selection favors timing of reproduction that maximizes fitness. Favorable environmental conditions, availability of mates, and pulses in necessary food resources are some of the main factors shaping where and when to reproduce (Perrins, 1970; Bronson, 1985; Daan and Tinbergen, 1997; Verhulst and Nilsson, 2008). Animal migrations are behavioral and physiological wonders that have evolved to take advantage of geographically disparate resources that affect the timing and location of breeding events (Milner-Gulland et al., 2011; Winkler et al., 2014; Shaw, 2016). The breadth of taxa that migrate is expansive and includes insects, reptiles, fish, mammals and birds (Williams, 1957; MacKeown, 1984; Dingle, 1996; Holland et al., 2006; Newton, 2010; Southwood and Avens, 2010). Bird migrations cover thousands of kilometers, with many species moving between equatorial latitudes where they spend time on non-breeding grounds, and northern and southern temperate latitudes where they breed during hemispheric summers (Berthold, 2001; Newton, 2010). These temperate breeding seasons in migratory birds occur annually during pulses and peaks in food resources (often insects) that are enough to support both resident and migratory species as they attempt to reproduce (Alerstam, 1990; Greenberg and Marra, 2005; Thorup et al., 2017). The onset of long-distance migration to breeding grounds in passerines (i.e., songbirds) is controlled by endogenous circannual rhythms which are synchronized by changes in day length (i.e., photoperiod; Gwinner, 2003; Gwinner and Helm, 2003; Kumar et al., 2010). Once begun, it then takes days to weeks of flying and refueling (at stopover sites) for these migrating birds to reach their breeding grounds (Bowlin et al., 2005). Therefore, long-distance migrants are constrained in their ability to adjust their departure dates from non-breeding grounds based on what conditions are like on their breeding grounds (Both and Visser, 2001; Miller-Rushing et al., 2008; Fraser et al., 2013).
Global average temperatures are increasing, with significant rates of increase in the past half century (Hurrell and Trenberth, 2010; IPCC, 2013), and the potential for global climate change (warming) to disrupt or otherwise affect the timing of breeding in migratory birds is substantial (Dunn and Winkler, 2010; Visser et al., 2010; Cohen et al., 2018). Migratory birds are thought to be particularly vulnerable because they may not be able to adjust their breeding phenology as global temperatures increase (Strode, 2003; Mills, 2005; Miller-Rushing et al., 2008; Both et al., 2010). To the contrary, a growing body of research is finding that warming in temperate latitudes associated with global climate change is advancing the timing of clutch initiation in several species of migratory birds (e.g., Dunn and Winkler, 1999, 2010; Both et al., 2004; Mazerolle et al., 2011; Tomotani et al., 2018). Earlier nesting may be a byproduct of temperature-related advances in plant and insect phenologies experienced by birds upon arrival at breeding grounds (Townsend et al., 2013; Shave et al., 2019). However, earlier breeding can cause reductions in reproductive success if the timing of breeding creates a mismatch between seasonal pulses in critical resources (Both et al., 2010) (i.e., food) and peak demand for feeding young (Visser and Gienapp, 2019). For example, a long-term study of pied flycatcher Ficedula hypoleuca in the Netherlands found that the flycatchers started nesting earlier in response to global warming, but not enough to match earlier peaks in caterpillar abundance at some locations (Both and Visser, 2001). Flycatcher population declines in areas where the greatest mismatches occurred have been attributed to this dynamic (Both et al., 2006). Another study looking at arrival dates of 117 migratory bird species over five decades found that ecological mismatch was a general pattern among migratory birds breeding in northern Europe, possibly contributing to population declines (Saino et al., 2011). These mismatches can reduce individual fitness and population viability, and therefore drive evolution by favoring those individuals well-matched to peak resource availability (reviewed in Visser and Gienapp, 2019).
Resident (i.e., non-migratory) bird species are thought to be less susceptible to trophic mismatches than migratory species (Monkkönen et al., 1990; Both et al., 2010; Parejo, 2016). One reason is that the timing of nesting is plastic in some resident species, allowing individuals to adjust their breeding based on spring phenology (Charmantier et al., 2008). In addition, during the spring, non-migratory birds are already near where they are going to breed, making it easier for them to respond to warmer or colder springs (Pulido and Widmer, 2006; Knudsen et al., 2011). For example, in a population of year-round resident song sparrows Melospiza melodia in North America individuals initiated breeding earlier in warm springs, and produced more offspring as a result (Wilson and Arcese, 2003). In another example, a 47-year study of great tits Parus major breeding in nest-boxes in the United Kingdom found that mean first egg dates and caterpillar phenology both had advanced by about 14 days on the same study site (Charmantier et al., 2008). But not all resident bird species avoid mismatches. For example, a separate study of great tits over a 23-year period in the Netherlands found that the timing of reproduction had not advanced in step with warming temperatures and earlier peaks in availability of food for the young (Visser et al., 1998). In addition, one recent study of 21 British songbirds spanning 18 years found no evidence that the relationship between productivity and relative amount of mismatch varied with migratory distance status (Franks et al., 2018).
While resident species may by and large avoid mismatches, not all migratory birds suffer reduced fitness when breeding phenology is altered by warmer spring temperatures. In some instances, advanced breeding of migratory birds in warmer years resulted in increased reproductive output. For example, black-throated blue warblers Setophaga caerulescens in New Hampshire United States initiated breeding earlier in warmer springs, which increased the probability that individuals attempted a second brood and led to higher annual fecundity (Townsend et al., 2013). Similarly, in a study of reed warblers Acrocephalus scirpaceus in Poland, first egg dates were earlier in warmer springs, which increased the length of the breeding season and resulted in more opportunities to renest (Halupka et al., 2008). Both studies also noted that the abundance and diversity of insects available for consumption were not diminished or mismatched in warmer years. Therefore, mismatches may be less likely in environments and for species where food is relatively constant or abundant throughout the breeding season (Dunn et al., 2011). This highlights the importance of documenting how local weather (e.g., temperature) experienced on the breeding grounds by newly arriving migratory birds influences both the commencement of nesting (i.e., first egg dates) and reproductive output of individuals when looking for the presence of a mismatch.
Beyond the potential for there to be a trophic mismatch, migratory birds nesting earlier on temperate breeding grounds may experience local weather conditions (e.g., temperature and precipitation) that affect when and how successfully they reproduce (Dunn and Winkler, 2010). Unusual weather events, in general, tend to negatively impact breeding birds (Shipley et al., 2020). For example, some migratory species may start breeding earlier because of warm weather on breeding grounds, only to have subsequent colder and/or wetter weather cause adult mortality, or reduce reproductive success by decreasing incubation efficiency and nestling provisioning (Brown and Brown, 2000; Conway and Martin, 2000; Coe et al., 2015; Cox et al., 2019; Shipley et al., 2020). This is particularly true for aerial insectivores such as barn swallows Hirundo rustica and tree swallows Tachycineta bicolor where cold temperatures and precipitation can cause short-term food (insect) shortages linked to inadequate incubation and poor nestling growth (Coe et al., 2015; Cox et al., 2019), and for barn and cliff swallows Petrochelidon pyrrhonota where adults can starve when activity of flying insects is reduced by periods of cold and/or wet weather lasting four or more days (Brown and Brown, 2000). Breeding earlier at temperate latitudes, therefore, comes with some added weather-related risks.
Prothonotary warblers Protonotaria citrea are long-distance Neotropical migrants that breed seasonally at temperate latitudes in the eastern United States. The warblers are insectivorous but not highly specialized on one guild of insect (Petit et al., 1990a,b; Dodson et al., 2016; Petit, 2020), and they occur in bottomland and swamp forests where there is an abundant and diverse invertebrate biomass continuously emerging during the warbler’s breeding season (Petit and Petit, 1996; Heinrich et al., 2013; Batzer et al., 2016; Dodson et al., 2016). Previous work on these warblers in our study system found no support for food to limit reproductive output, even when densities were manipulated to increase substantially (Hoover et al., 2020). One previous study of prothonotary warblers in the eastern part of their breeding range found changes in local spring temperatures not to have an effect on mean nest initiation dates, and for warmer spring temperatures to increase mean probability of attempting a second brood when older females were more abundant in the population being studied (Bulluck et al., 2013). Here we take a somewhat different approach by studying the effects of local spring temperatures on individual females’ first egg dates, annual reproductive output, and the probability of fledging second broods. Given that the warblers breed in a food-rich habitat, it is possible that any local-temperature-driven annual adjustments in when nesting is first initiated would not necessarily have a negative effect on overall reproductive output.
Here, we investigate the effects of local temperature on first egg dates, annual productivity, and the probability of fledging two broods in prothonotary warblers using data collected during a 20-year period (1994-2013). Because local temperature trends are not always similar to global warming trends (Hansen et al., 2001), we compared our local April temperature data trend to the Annual Global Land Temperature Anomaly data trend (NOAA National Centers for Environmental information, Climate at a Glance: Global Time Series, published January 2020, retrieved on February 1, 2020 from https://www.ncdc.noaa.gov/cag/) for the period 1983 to 2019 to determine if local April temperatures reflected global temperature changes. The warblers arrive at our study system during April and commence nesting in late April and May (Hoover, 2001). Thus, we predicted that higher local average April daily temperatures (local April temperature hereafter) would result in earlier first egg dates in our study system. Based on prior observations (Hoover, 2001), we expected that, within a given year, older (>1 year old) females would have earlier first egg dates than the 1-year-old females that were breeding for the first time. To determine whether warmer spring temperatures were a cost or benefit to warbler productivity, we also tested for effects of local April temperature on the season-long reproductive output of the warblers and, related to that, the probability that females successfully fledged two broods. Finally, to determine if arrival dates of migrating warblers to the general area where our study took place were associated with local April temperatures, we compared earliest arrival dates (using eBird) to local April temperatures for the period 1999 to 2019.
Materials and Methods
From 1994 to 2013, we studied the breeding ecology of individually marked prothonotary warblers on 14 discrete (i.e., separated from each other by >1 km) forested wetland study sites within a 192 km2 portion of the Cache River watershed (centered at 37.294°N, 88.975°W) in southern Illinois, United States. The warblers migrate from non-breeding grounds in southern Central America and northern South America to breeding grounds in the eastern half of the United States from the Gulf of Mexico to the northern tier states and southern Ontario Canada (Petit, 2020). Prothonotary warblers are obligate secondary cavity nesters that breed over or near standing water in bottomland hardwood and swamp forests (Petit and Petit, 1996). They defend exclusive territories, are socially monogamous, readily accept nest boxes (Fleming and Petit, 1986), and adults exhibit high site fidelity between breeding seasons (Hoover, 2003a). Females build nests and incubate eggs, and both sexes provision nestlings and fledglings. During the breeding season, the warbler’s diet is a diverse assemblage of insects including caterpillars (order Lepidoptera), flies and midges (order Diptera), spiders (class Arachnida), mayflies (order Ephemeroptera), and dragonflies (order Odonata) mostly gleaned from leaves, twigs, and branches (Petit et al., 1990a,b; Dodson et al., 2016; Petit, 2020). The warblers often attempt a second brood and on rare occasions fledge three broods in a single breeding season (Hoover, 2003a; Bulluck et al., 2013; Petit, 2020).
We monitored warblers that used artificial nest boxes placed on trees or metal conduit, located 50 to 100 m apart, within the 14 study sites (Hoover, 2003a). Prothonotary warblers used nest boxes almost exclusively (Hoover, 2003a; Hoover et al., 2020) and the nest boxes mimicked natural cavities in terms of nest predation and cowbird parasitism (Hoover, 2001). Nest boxes were monitored every 3–5 days from mid-April to early August each year. This frequency of nest monitoring allowed us to determine when egg laying and incubation commenced, clutch size, and hatching success. Nests were typically visited two to three days prior to fledging and warbler nestlings were banded with a uniquely numbered aluminum leg band (U. S. Geological Survey). The number of warbler fledglings assigned to each nesting attempt was the number of nestlings present at that pre-fledging visit so long as the nest was intact and the adult warblers were seen with, or behaved as though they had, recently fledged warblers in their territory the very next visit post-fledging (Hoover, 2003b, 2006). Warbler nests are often parasitized by brown-headed cowbirds Molothrus ater (Hoover, 2003b; Hoover and Reetz, 2006), and we noted the number of cowbird eggs, nestlings and fledglings associated with each nesting attempt.
We were unable to document arrival dates of individual warblers to our study system. Nearly all females in a given year were not observed until they were building a nest in a given nest box. We captured all adult birds that used nest boxes and banded each with a unique combination of a single numbered aluminum leg band and multiple colored plastic leg bands. We captured and/or re-sighted (for those already banded) birds to identify the individual male and female associated with each nesting attempt each year. We captured females while they were incubating by placing our hand over the opening of an active nest box, trapping the female inside. Females exited the nest box into a clear plastic bag placed over the opening and were removed from the bag immediately for processing. We captured males by placing a male decoy warbler paired with a playback of a warbler song in front of a mist-net within each male’s territory. Upon capture of adults, we measured body mass (g), wing chord length (mm), and tarsus length (mm) of each individual. For individuals captured for the first time, we used these measurements along with plumage characteristics to determine their age [second-year (SY; i.e., 1 year old and entering their first breeding season) vs. after-second-year (ASY; i.e., ≥2 years old) (see Kowalski, 1986; Pyle et al., 1987). We also knew the age of warblers we had banded as nestlings that returned in subsequent years to breed in our study system. For nearly every individual female breeding on our study sites in a given year we were able to document her first egg date, age, total number of offspring produced, and whether or not she fledged two broods.
Temperature Data
Our focus for comparison with average first egg dates and reproductive output of prothonotary warblers was the local temperature during the month of April when warblers arrive at our study system. Local temperature data for 1994 to 2013 were obtained using archived weather data from a nearby weather station (∼ 30 km south of study system; https://www.wunderground.com/history/monthly/us/ky/west-paducah/KPAH/date). We used the mean value of average daily temperature (°C) measurements from the entire month of April each year as our metric of local temperature because the warblers initiate nesting in late April and May. To simply determine that our local April temperatures trended similarly to global temperatures we considered a longer run of years (1983 to 2019), and used the above source for the local temperatures and Annual Global Land Temperature Anomaly data from the National Oceanic and Atmospheric Administration (NOAA National Centers for Environmental information, Climate at a Glance: Global Time Series, published January 2020, retrieved on February 1, 2020 from https://www.ncdc.noaa.gov/cag/). These global data are the departure of annual global land temperature (for a given year) from the average of 1901–2000 values (values are °C; positive and negative values represent warming and cooling, respectively).
First Arrival Data
To determine if there was a relationship between local April temperatures and earliest arrival dates of migrating warblers to southern Illinois during 1999 to 2019, we used data from eBird (https://ebird.org/map). We selected the months of March-April for each year and noted the earliest reported sighting(s) along the Gulf Coast (from Houston, Texas to Tallahassee, Florida) and in the southern Illinois region (just north of the Ohio River) where our study took place. These arrival dates are those of male prothonotary warblers, which often arrive earlier than females or are more likely to be observed because they are more conspicuous than females (Hoover, 2001; Petit, 2020). We lack detailed arrival dates for females breeding on our study sites, thus we used this approach with eBird data to determine whether local earliest arrival dates and local temperatures were correlated, whether local earliest arrival dates and those for along the Gulf Coast of the United States were correlated, and whether first eggs dates were correlated with local first arrival dates.
Statistical Analysis
We used SAS 9.4 software (SAS Institute, 2013) to construct and fit all models. Prior to fitting all models, we examined the residuals of response variables to check for normality. Values reported in the results section are means ± SE unless otherwise indicated. We report the type-III fixed effects test statistics and p-values and set α = 0.05. We first used simple linear regression (i.e., the ‘genmod’ procedure) analyses to determine if any trends (i.e., increasing, decreasing, or no trend) existed between our local April temperatures or Annual Global Land Temperature Anomaly values and year (1983 to 2019).
We next used a general linear mixed model (i.e., the ‘mixed’ procedure) to examine the influence of local April temperatures on warbler first egg dates. Fixed effects included local April temperature and female age (category: 1-year-old versus older). Random effects included Female ID as a random intercept with year as the random slope, and study site as a separate random intercept. Female age was included as a fixed effect because after-second-year (older) females tend to arrive and begin nesting earlier than second-year (1-year-old) females (Hoover, 2001; Petit, 2020). Warblers are relatively long-lived (i.e., up to 13 years, unpublished data) and exhibit high site fidelity between years (Hoover, 2003a) thus we expected numerous data points for each individual across years. Because we studied females across 14 distinct study sites within our study area, we included study site as a random effect to account for variation among study sites.
We used a generalized linear mixed model (i.e., the ‘glimmix’ procedure) with a negative binomial distribution and log-link function and a Laplace approximation to determine whether local spring temperatures influenced the number of warbler fledglings produced per female per year (annual productivity hereafter). Fixed effects included local average April temperature and female age. Random effects included a random intercept of female ID with year as a random slope, and a random intercept of study site with the categorical term tree/poles as a repeated effect. The tree/pole variable described whether most boxes within a given study site in a given year were installed on trees or metal conduit. Throughout the course of this study we used metal conduit to reduce nest predation for various unrelated experiments and thus wanted to control for this effect in our modeling of annual productivity. We incorporated the additional tree/pole categorical variable as a repeated measure on study site as most study sites had both categories represented at some point throughout the study. We also know that cowbirds reduce warbler productivity in very predictable ways (Hoover, 2003b), thus prior to constructing our model we used a regression analysis to determine if the average number of cowbird nestlings per female warbler per year was affected by local April temperatures. The average number of cowbird nestlings per female warbler per year was not affected by local April temperatures (F1,19 = 0.24, P = 0.63, r2 = 0.01) so we did not consider brood parasitism by cowbirds further in this study.
Previous research in our study system demonstrated that females that nest earlier are more likely to attempt second broods (Hoover et al., 2020). Thus, local warmer April temperatures could also increase the probability of females successfully fledging two broods and explain any increases in productivity we observed. To test this prediction, we used a generalized linear mixed model (i.e., the ‘glimmix’ procedure) with a binomial distribution and logit-link function using a Laplace approximation, the fledging of two broods (no, yes) as the response variable, and female age and local April temperatures as fixed effects. Random effects again included a random intercept of female ID with year as a random slope, and a random intercept of study site with the categorical term tree/poles as a repeated effect.
In all mixed models where we used random effects with a repeated measure or random slope design, we tested three separate variance-covariance structures to see which structure best fit the data. For this, we compared variance components (i.e., default in SAS), unstructured, and compound symmetry structures and then compared the AICc values to find the best variance-covariance structure given the data. In all cases, the default variance components structure provided the lowest AICc value and thus the best fit. In all models where we compared the variance-covariance structures, the outcomes for our variables of interest were qualitatively the same. Lastly, we used simple linear regression analyses to determine if the earliest warbler spring arrival in southern Illinois (based on eBird.org) was influenced by local April temperature or earliest arrival dates of warblers to the Gulf Coast states; and whether first egg dates were associated with earliest arrival dates to southern Illinois.
Results
During the period 1994 to 2013 we obtained 3,136 first egg dates, annual productivity values, and double-brooding statuses, from 2,017 individual female prothonotary warblers nesting in our study system. Individual female warblers were sampled in anywhere from 1 to 6 different years during the study. Annual values for local average April daily temperature, mean first egg dates, and mean annual productivity are provided in Table 1.
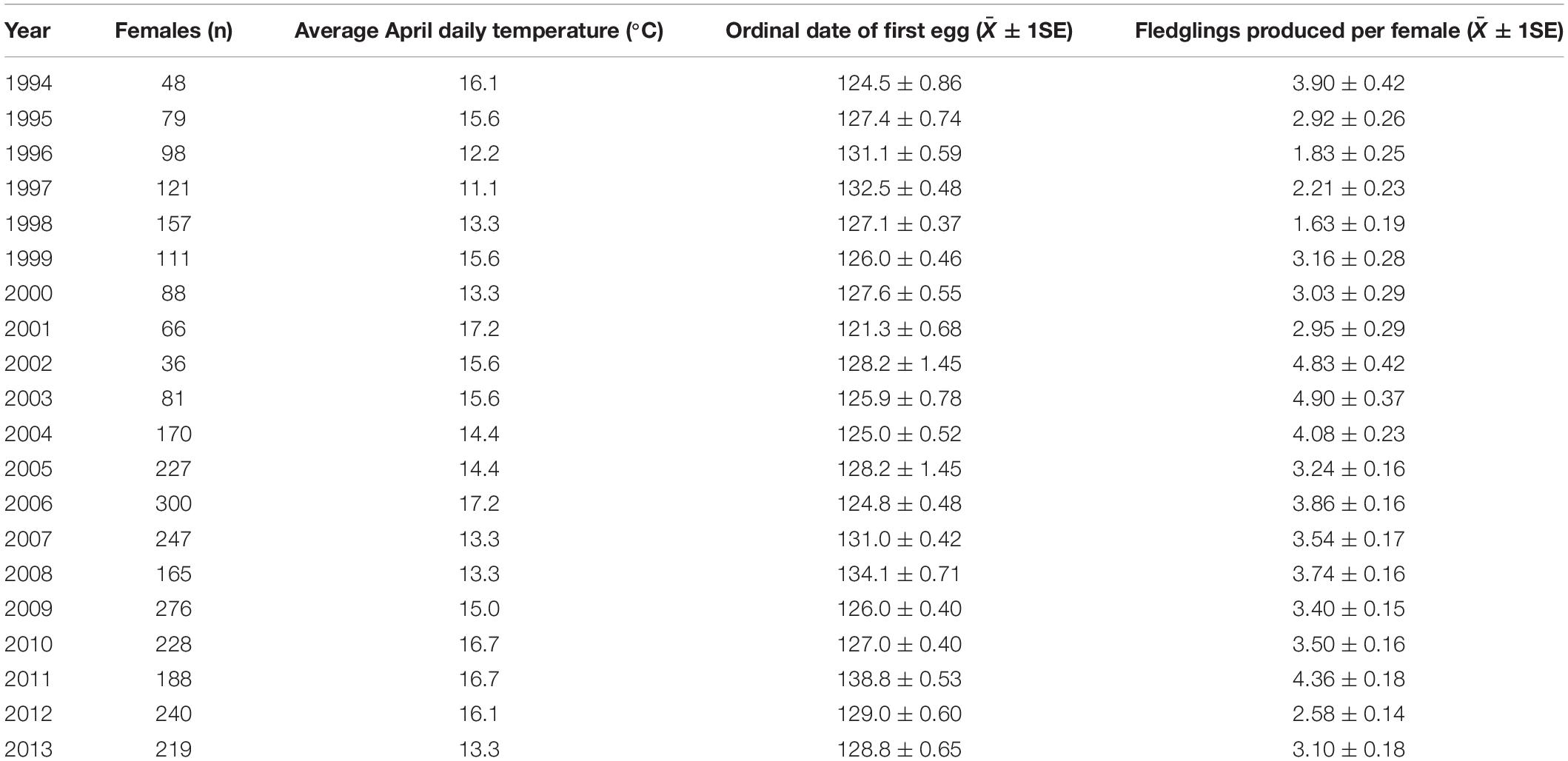
Table 1. Summary data for prothonotary warblers nesting in the Cache River watershed in southern Illinois, United States during 1994–2013. Ordinal date 121 = 1 May.
During the period 1983 to 2019, both the local April temperature (F1,35 = 17.2, P < 0.001, r2 = 0.33) and the Annual Global Land Temperature Anomaly (F1,35 = 156.8, P < 0.001, r2 = 0.82) increased significantly with year (Figure 1). This shows that our local temperature pattern was similar to temperature trends at a global scale during the same time period.
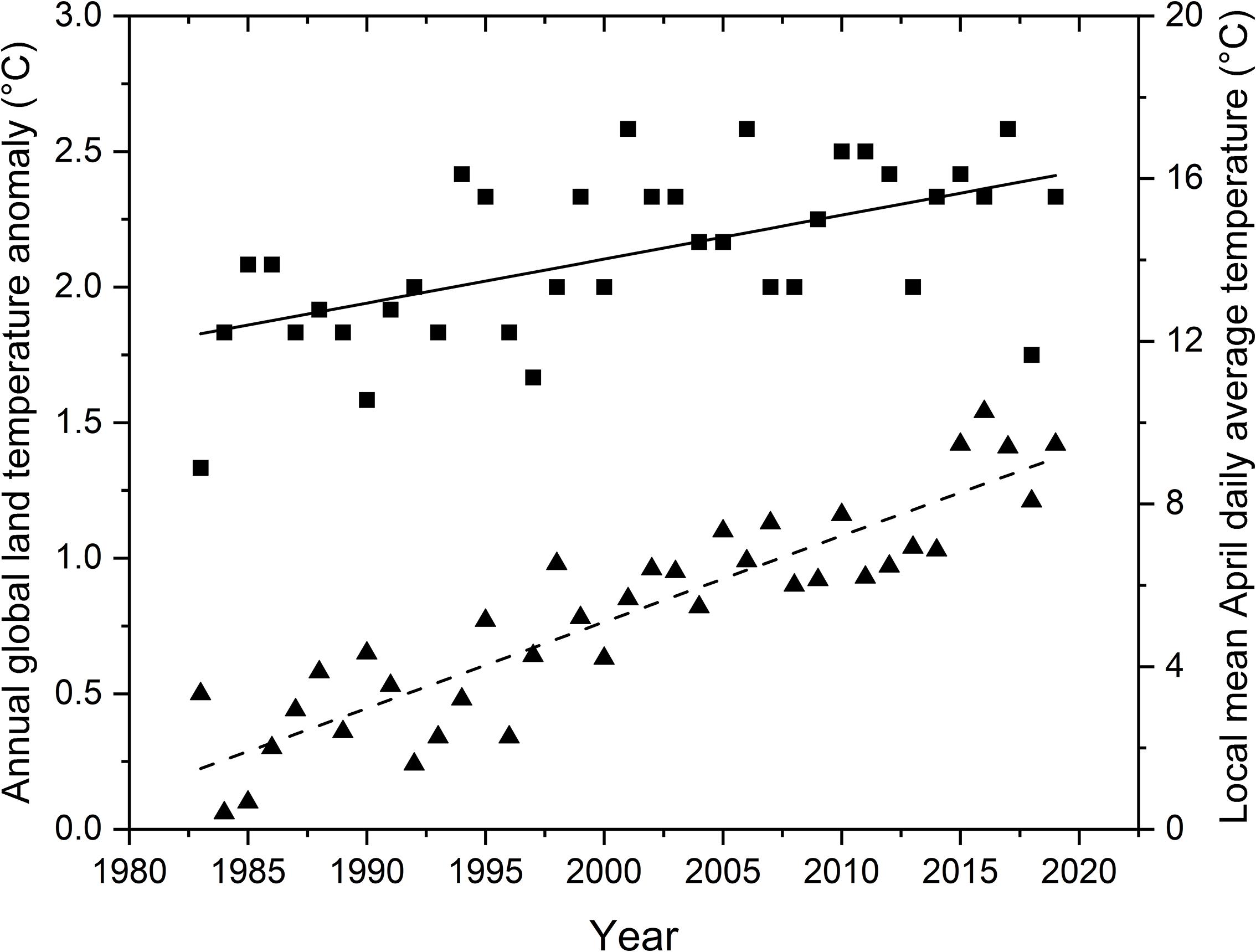
Figure 1. Local April temperatures for southern Illinois, United States (squares and solid line) and Annual Global Land Temperature Anomaly values (triangles and dashed line) significantly increased during 1983 to 2019. Shown are the data points for each year and best fit lines for the regressions of the local and global parameters on year.
The range of values for first egg dates across all individual females was ordinal dates 107 (17 April) to 157 (6 June). Both average April daily temperature and female age had significant effects on first egg dates of prothonotary warblers. First egg dates became earlier as local April temperatures increased (F1,3045 = 100.8, P < 0.001) and were earlier for older females (i.e., >1-year-old) compared to 1-year-olds (F1,3125 = 153.7, P < 0.001) (Figure 2). First egg dates for older females, on average, were approximately 4 days earlier than young females in a given year. Across the spectrum of local April temperatures occurring during our study, mean first egg dates varied by approximately 9 days, from older females in years with warmest April temperatures to young females in years that were coldest (Figure 2).
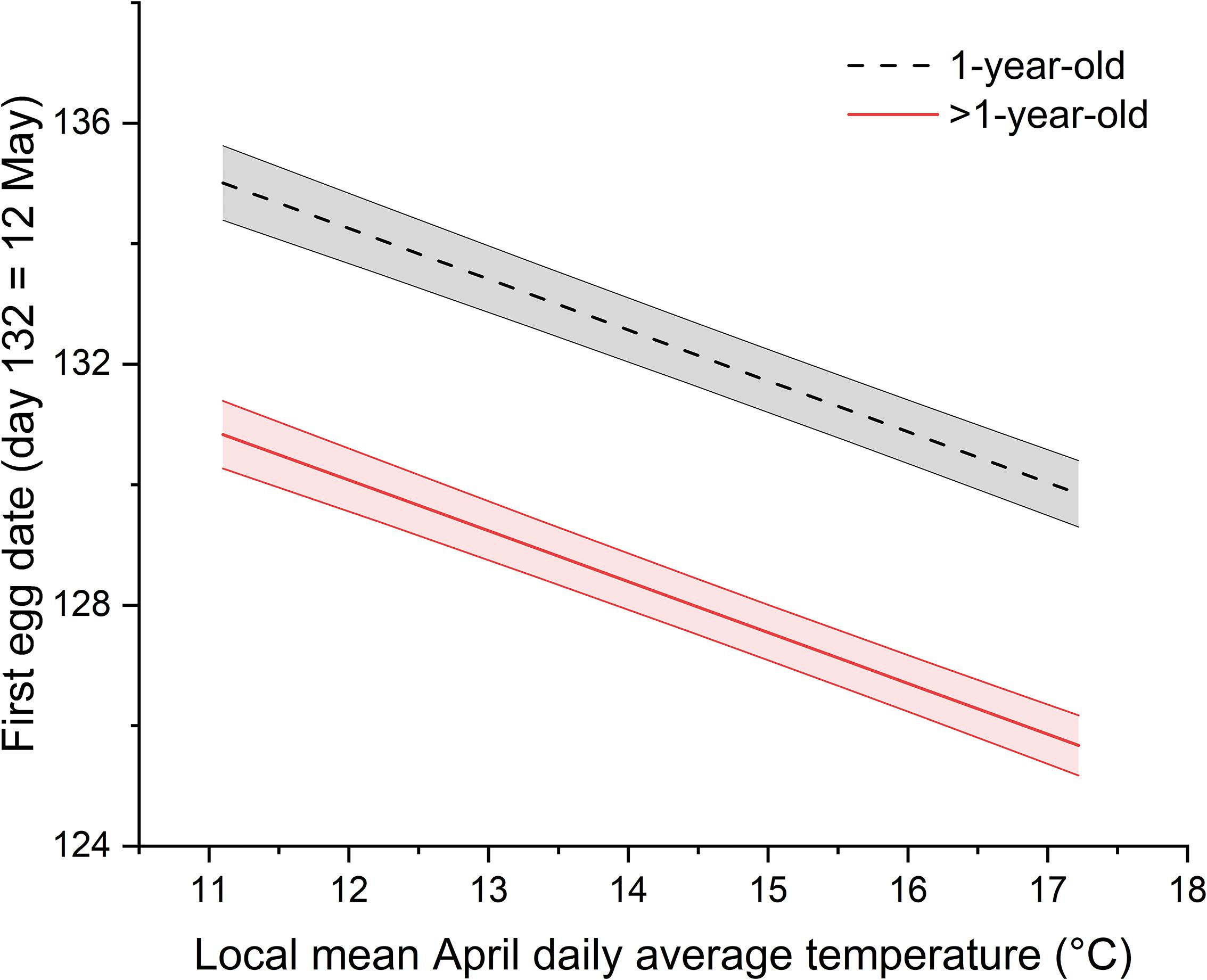
Figure 2. First egg dates of prothonotary warblers are earlier when local April temperatures are warmer and for older females in the Cache River watershed, IL, United States during 1994 to 2013. Shown are model output best fit lines including random effects held at their mean values. Shaded regions around best fit lines are ± 1 SE.
Across all individual females in our study, the range for annual productivity was 0 to 14 warbler fledglings produced. Annual productivity of female warblers was affected significantly by average April daily temperature, female age, and when sites had boxes on metal conduit. The number of warbler fledglings produced increased as local April temperatures increased (F1,1097 = 22.8, P < 0.001) and was greater for older females (i.e., >1-year old) compared to 1-year-olds (F1,1097 = 19.9, P < 0.001) (Figure 3). For young and older females alike, reproductive output increased by approximately 0.7 fledglings across the range of April temperatures experienced, with older females producing on average 0.5 more fledglings per year compared to young females (Figure 3). As we expected, females nesting on sites where most nest boxes were installed on metal conduit produced 0.63 ± 0.23 (SE) more warbler fledglings in a given year on average compared to those nesting where most nest boxes were on trees (Z = 2.8, P = 0.003). In addition, females were more likely to produce two broods in warmer years (F1,3133 = 11.0, P < 0.001) (Figure 4). On average, older females were nearly twice as likely as younger females to produce two broods (F1,3133 = 25.9, P < 0.001).
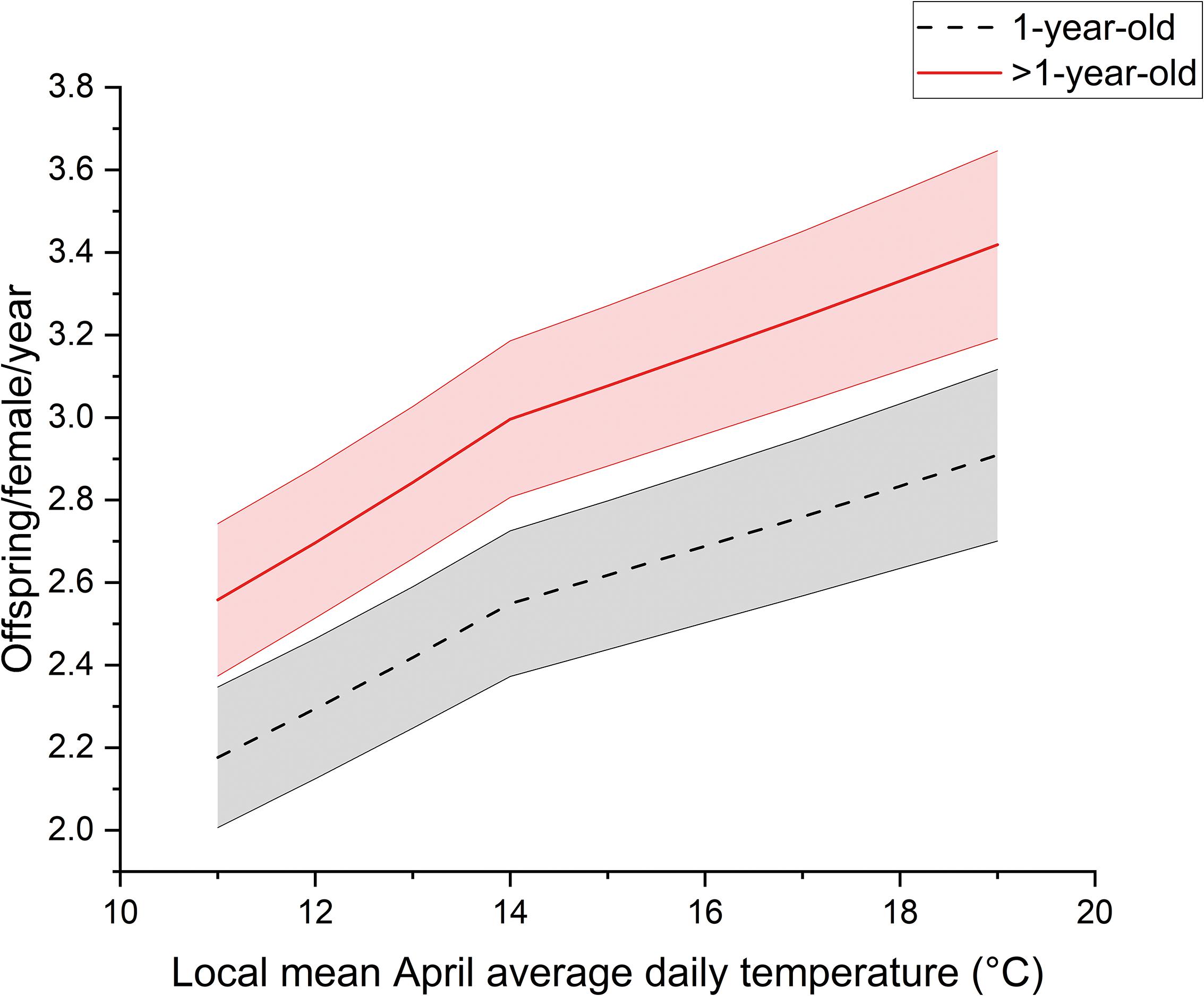
Figure 3. Fledglings produced per female prothonotary warbler per year increased with local April temperatures and female age in the Cache River watershed, IL, United Sttaes during 1994 to 2013. Shown are model output best fit lines including random effects held at their mean values. Shaded regions around best fit lines are ± 1 SE.
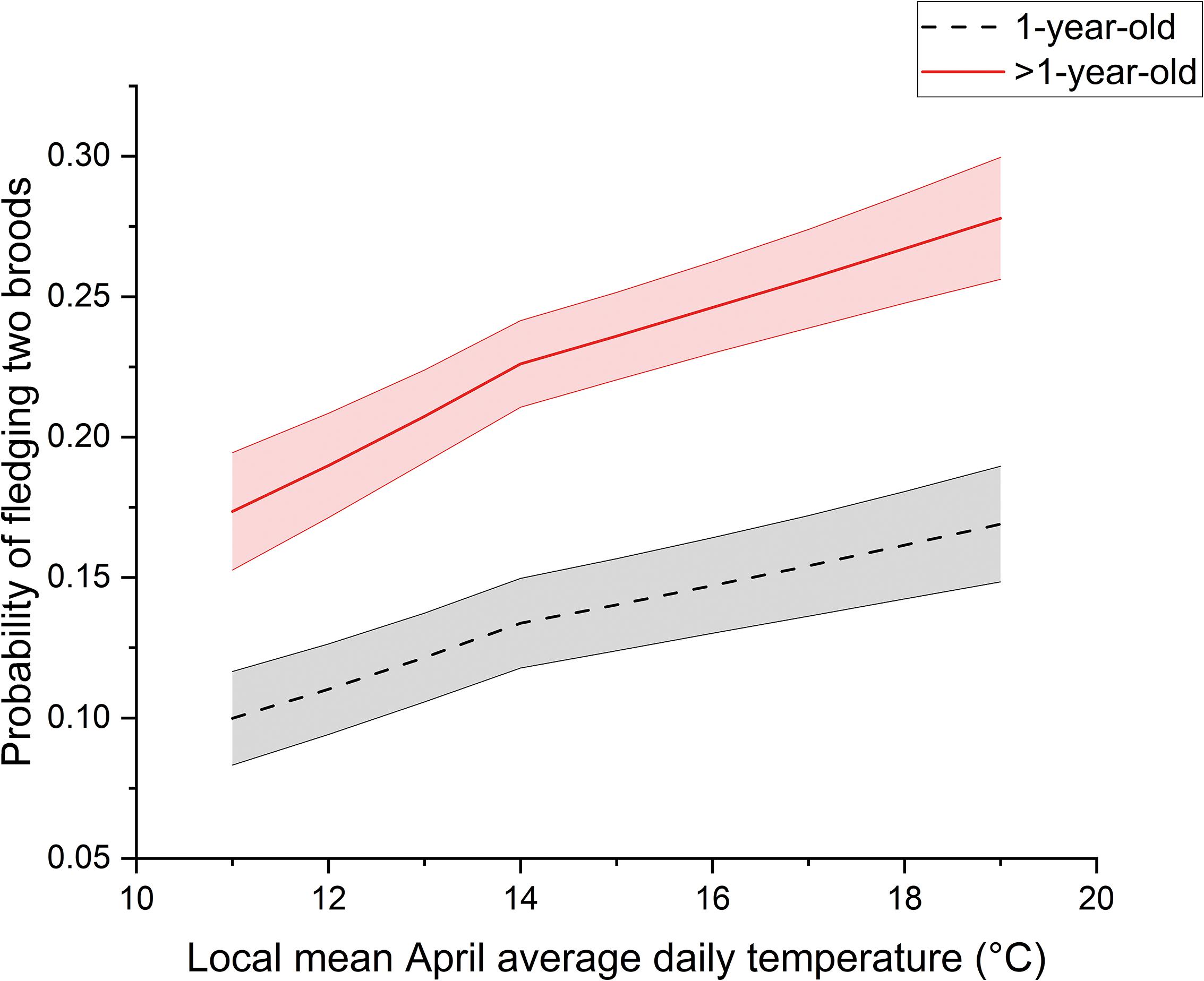
Figure 4. The probability of producing two broods increased with local April temperatures and female age in the Cache River watershed, IL, United States during 1994 to 2013. Shown are model output best fit lines including random effects held at their mean values. Shaded regions around best fit lines are ± 1 SE.
Earliest male arrival dates to southern Illinois based on eBird data during the period 1999 to 2019 were not related to local April daily temperatures (F1,19 = 0.4, P = 0.51, r2 = 0.02) (Figure 5). Arrival dates for warblers in southern Illinois tended to be earlier when arrivals to the Gulf Coast states were later (F1,19 = 3.6, P = 0.07, r2 = 0.16). During the period 1999 to 2013, first egg dates were not related to earliest local arrival dates (F1,13 = 0.08, P = 0.79, r2 = 0.01).
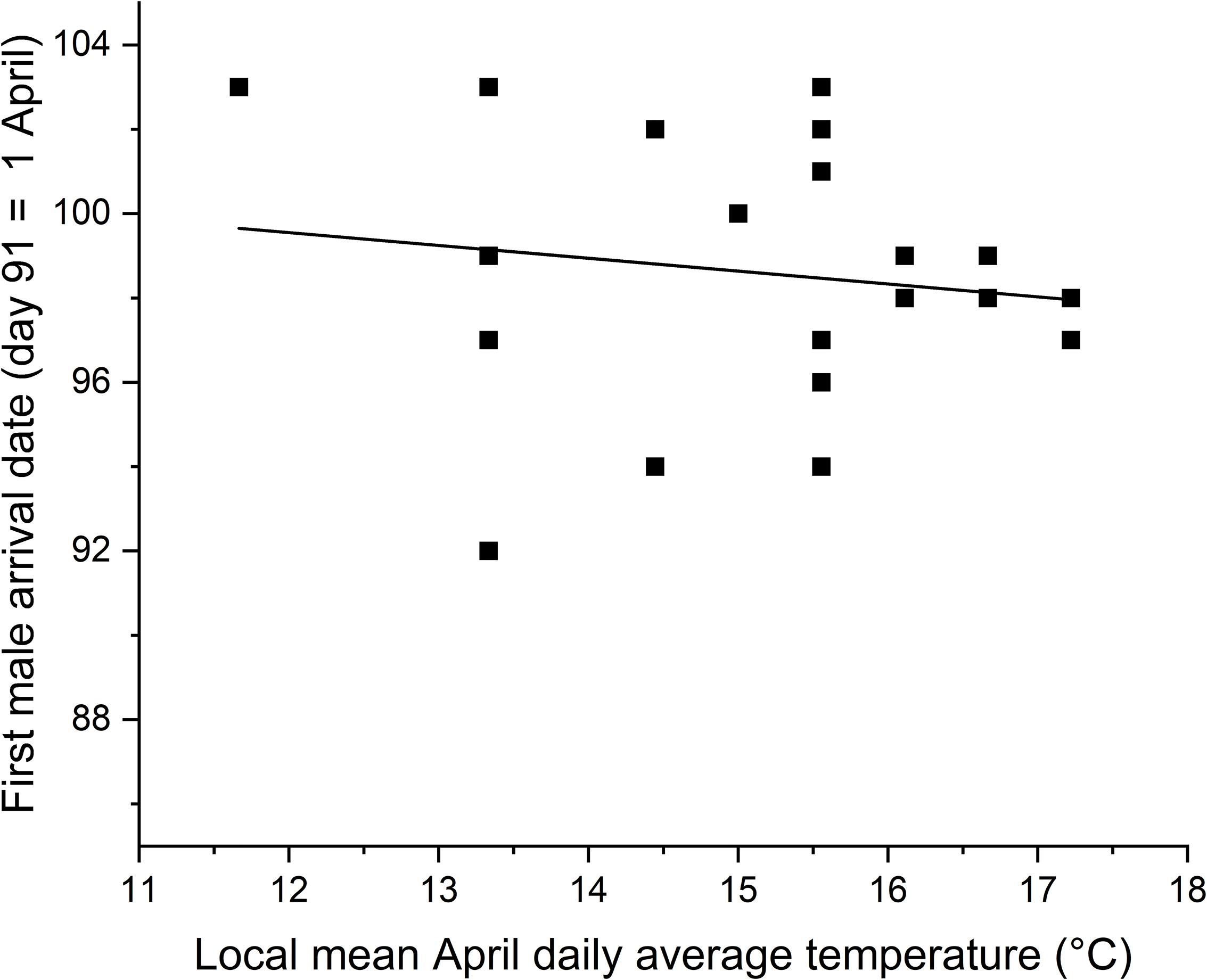
Figure 5. Earliest male prothonotary warbler arrival dates to southern Illinois, United States were not affected by local April temperatures during 1999 to 2019. Shown are the data points for each year and the best fit line for the regression of arrival dates on local April temperatures.
Discussion
We found that similar to global warming trends, local April temperatures in southern Illinois are also increasing over time. Prothonotary warblers in our study system began nesting earlier and produced more offspring in years when local April temperatures were higher. As expected, older females started earlier and produced more offspring than first time breeders likely because of the earlier arrival dates of non-yearlings. The result of higher annual productivity in warmer years when nesting begins earlier suggests that the local warming trend has not yet created a mismatch between the timing of nesting and availability of food resources. We also found that local April temperatures did not influence earliest warbler arrival dates in southern Illinois. Taken together, these results show that prothonotary warblers are flexible in their timing of breeding and that current warming trends may prove beneficial to our local population at least in the short term.
Warblers with earlier first egg dates had higher annual productivity in our study even under scenarios where predators were not excluded. The likely reason for this is that earlier breeding associated with warmer temperatures resulted in an increased probability of fledging two broods within a given breeding season. An increase in attempting second broods previously had been documented in our study system (Hoover et al., 2020), as well as in a study of prothonotary warblers in Virginia, United States (Bulluck et al., 2013). Similar patterns of increased double brooding, or increased chances of successfully producing a replacement brood, as a byproduct of nesting earlier in warmer years has also been found in other species (Halupka et al., 2008; Monroe et al., 2008;, Verhulst and Nilsson 2008; Townsend et al., 2013). A study of 20 bird species in Denmark found warmer temperatures to lengthen the breeding season for those species capable of producing multiple broods (Møller et al., 2010), and increase the probability of individuals to attempt second broods. Other examples include research on black-throated blue warblers in New Hampshire, United States (Townsend et al., 2013) and reed warblers in Poland (Halupka et al., 2008), where individuals breeding earlier in warmer years had higher annual fecundity because they were more likely to attempt multiple broods (both studies) or multiple re-nests (reed warblers), and both studies noted that double-brooding and re-nesting were supported by ample and diverse insects (i.e., food) being available. To produce more offspring requires abundant food resources throughout the breeding season, and abundant insects are a hallmark of the bottomland and swamp forests where the prothonotary warblers nest (Petit and Petit, 1996; Heinrich et al., 2013; Batzer et al., 2016; Dodson et al., 2016). Food resources (insects) in the forested wetlands and swamps where the warblers prefer to nest can even be supplemented by the terrestrial habitats found in bottomland forest ecosystems (Nakano and Murakami, 2001; Baxter et al., 2005). Differences in warbler clutch sizes likely contributed little to the trend in annual productivity we report here because the vast majority of warbler first clutches contain five warbler eggs in any given year (Hoover, 2001, 2003b; Hoover et al., 2020). In terms of age effects, even though the young female warblers on average started later and produced fewer total fledglings than the older females, the general effects of local April temperatures on first egg dates and annual productivity in young females were similar to the older females (Figures 2–4).
Advanced first egg dates of migratory birds in warmer years may be a byproduct of earlier arrival dates. However, global warming has no effect on photoperiod and should have little effect on when migratory birds using these cues depart for their breeding grounds. Indeed, the ability of long-distance migrant bird species to adjust first egg dates (i.e., breeding phenology) in response to environmental factors is thought to be constrained by limitations associated with the timing of migration (Strode, 2003; Miller-Rushing et al., 2008, Both et al., 2010). Yet, several studies have documented earlier arrival of migratory birds to breeding grounds in years when temperatures on breeding grounds were warmer, or even when temperatures on non-breeding grounds were warmer prior to spring migration (Cotton, 2003; Usui et al., 2017). In a meta-analysis covering 73 published studies and 413 species, Usui et al. (2017) found a robust pattern where earlier arrivals in warmer years were not dependent on whether temperatures represented changes at breeding, passage or non-breeding sites, or whether arrival was to the breeding or passage grounds. In another meta-analysis of arrival dates of 20 migratory bird species breeding in Oxfordshire, United Kingdom, Cotton (2003) found that arrival dates became earlier with increases in a continental-scale temperature measurement taken from their non-breeding grounds (winter sub-Saharan African temperature anomaly), suggesting that some migratory birds may begin their migrations earlier when it is warmer on the non-breeding grounds. Counter to these patterns of earlier arrival in warmer years, arrival dates of several species of long-distance migratory wood warblers to breeding grounds in Illinois (100-year period) and Minnesota (40-year period) United States were not correlated with local spring temperatures (Strode, 2003). Earliest arrival dates of prothonotary warblers to southern Illinois, as determined using eBird data, varied among years but showed no relation to local April temperatures. Differences in arrival dates may explain, however, why older females in our study system had earlier first egg dates on average. Female warblers are relatively cryptic (no singing and not boldly colored) during the breeding season (Petit, 2020). While we do not have good data on specific arrival dates of individuals across years in our study system, we have noticed that the vast majority of the earliest females we happen to observe in the spring prior to the commencement of nesting are banded individuals captured in a previous year (Hoover, 2001), which places them in the older age category.
While the aim of our investigation here was to document whether or not local spring temperatures affected first egg dates and season-long reproductive output in prothonotary warblers, how and why the warblers nest earlier and produce more offspring in warmer years remains to be determined. Even if arrival dates were constrained by departure dates from wintering areas, there could still be flexibility in when breeding begins after arriving on breeding grounds. It is possible that arrival dates are similar across years but latency to laying first eggs is shortened in years when local temperatures are warmer and lengthened when cooler (Both and Visser, 2001; Visser et al., 2004). Arrival dates for individual females are a critical missing piece. To adequately document age-related patterns in arrival dates and whether arrival dates contribute to explaining earlier first egg dates when temperatures are warmer, we need to collect additional detailed information on arrival dates and settlement patterns of individual females in our study system in years with warmer and colder springs. These data for individual females, particularly those that breed across several years in our study system, would allow us to better understand whether evolutionary changes versus changes due to phenotypic plasticity (i.e., individual flexibility) are influencing the pattern between first egg dates and local April temperatures we observed (Charmantier et al., 2008; reviewed in Charmantier and Gienapp, 2014). If individuals are flexible in when they lay their first egg relative to local temperatures, then population mean first egg dates may trend earlier as temperatures trend warmer over several years, but still vary considerably between consecutive years that are warmer and colder (Charmantier et al., 2008). Our results suggest that individual females in our study population are flexible in their ability to adjust first eggs dates from year to year, depending on the local temperature, but specific data to verify this are still needed.
In a number of songbird species, breeding earlier in the season can have numerous benefits including producing offspring that are larger at fledging, and are more likely to be recruited into the breeding population (Wheelwright et al., 2003;, Müller et al. 2005, Tarof et al., 2011). In our study system, warbler nestlings fledging earlier in the season have a higher first-year survival rate and likelihood of local recruitment than those produced later (McKim-Louder et al., 2013). Tomotani et al. (2018) found a similar pattern in a population of European pied flycatchers and attributed the higher recruitment of fledglings from earlier nesting attempts to fledglings having more time to develop prior to migrating to non-breeding grounds. The warbler young produced in our system return locally to breed (McKim-Louder et al., 2013) and may therefore be fairly well-adapted to local conditions. It remains to be seen if continued warming and even earlier fledging translates into similar or even higher recruitment rates, or possibly alters natal dispersal patterns that could contribute to expected shifts in species’ breeding ranges (Parmesan and Yohe, 2003; Visser et al., 2009; Langham et al., 2015; Stephens et al., 2016).
Along with earlier nesting, higher local temperatures can alter plant phenology (i.e., earlier bud break and leaf out) (Schwartz et al., 2006; Richardson et al., 2013) and cause earlier insect emergence (Roy and Sparks, 2000; Both et al., 2006; Charmantier et al., 2008; Hodgson et al., 2011; Jamieson et al., 2012; but see Primack et al., 2009). The greatest demand for resources occurs when birds are feeding nestlings/fledglings (Nilsson, 1994; van Noordwijk et al., 1995; Both et al., 2009) and it is therefore advantageous for birds to initiate nesting so that peaks in insect availability match the demands of provisioning a brood or broods. Mismatches occur when environmental factors such as warming temperatures cause a misalignment of peak food demand and peak food availability (Thomas et al., 2001; Visser and Both, 2005; reviewed in Both, 2010; Dunn and Winkler, 2010). Had earlier breeding by the warblers in warmer years resulted in a mismatch with food resources, we would have expected to see a decrease in season-long reproductive output, rather than our observed increase. While we did not measure insect abundance, or how it varies seasonally and in relation to temperature, recent work in our system (Hoover et al., 2020), where densities of breeding warblers were manipulated higher, found no evidence for reproductive output to be food limited. In that study we found no significant effects of density or a density by date interaction on measures of reproductive output within a breeding season even with nearest-neighbor densities (i.e., pairs within 200 m of focal pair) exceeding 20 pairs. We suspect that abundant food (insect) resources known to occur in bottomland forest ecosystems (Baxter et al., 2005; Batzer et al., 2016), in conjunction with the diverse diet of the warblers (Petit, 2020), reduces the risk that temperature-related shifts in their first egg dates might result in a mis-match. It is also possible that the emergence and abundance of relevant insects is shifted earlier in warmer years, similar to warbler first egg dates and what has been found in black-throated blue warblers in New Hampshire, United States (Townsend et al., 2013), as well as in great tits in the United Kingdom (Charmantier et al., 2008). Regardless, there are much more data needed from our study system to fully understand the dynamics between spring temperatures, arrival dates of individual females, and plant, insect and breeding phenologies.
Species or individuals not able to adjust the timing of their own breeding to match changes in resource peaks, or that tend to specialize on few types of food resources may be especially vulnerable to resource mismatches associated with global warming (Both et al., 2006; Møller et al., 2008; Both, 2010; Charmantier and Gienapp, 2014). Prothonotary warblers do not belong to either of these categories of vulnerable species. Similarly, breeding earlier in warmer years with no apparent cost to reproduction has been documented in many other migratory bird species including reed warblers (Halupka et al., 2008), tree swallows (Dunn et al., 2011), purple martins Progne subis (Shave et al., 2019), and black-throated blue warblers (Townsend et al., 2013). In each of these study systems, authors suggested that earlier breeding was not costly, in part, because food resources (i.e., insects) were available enough to accommodate it.
Apart from food availability, it is possible that continued global warming could hasten late-season dewatering of forested wetlands and swamps that are home to prothonotary warblers, thereby reducing the suitability of the habitat or exposing later season nests to increased nest predation (Hoover, 2006). Global warming could also exacerbate the threats prothonotary warblers already face from the loss, fragmentation, and hydrologic degradation of bottomland forest habitats (Hoover, 2009; Robinson and Hoover, 2011). In addition, while breeding was the focus of our study and is essential to species persistence, it is only one stage in the complicated life cycles of migratory birds.
The overall effects of global warming remain particularly difficult to predict for migratory species because they have complex habitat needs throughout their life cycles and complex physiological adaptations that coincide with timing of migrations (Winkler et al., 2014). For example, the effects of global climate change on the quality and distribution of suitable habitats may be very different for migratory pathways, stopover locations and non-breeding grounds used by migrants, potentially undermining the timing of events that have evolved to get migrants to their destination when resources are adequate to meet needs. Thus, much more information is needed, particularly for migratory species, on how global warming affects other annual cycle stages beyond reproduction (Hostetler et al., 2015; Culp et al., 2017; Tomotani et al., 2018). The prothonotary warbler is one example where warming temperatures currently are providing fitness benefits on local breeding grounds. The mobility of migratory species in general may make them more capable of adapting to a changing environment provided that any changes in the phenology of their life stages match any changes in the phenology of critical resources. With substantial warming in the Northern Hemisphere during the warbler’s breeding season projected to continue in the coming decades (Vogel et al., 2019), it remains to be seen if and when a tipping point may be reached whereby earlier nesting no longer conveys a reproduction advantage.
Data Availability Statement
The datasets generated and analyzed for this study can be found in the Illinois Data Bank https://doi.org/10.13012/B2IDB-6482573_V1.
Ethics Statement
The studies involving prothonotary warblers were conducted in compliance with Animal Research Protocols N6C107/7093, N8C046, 07040, 07224, 10011, 10013, and 10173 (each approved by the Institutional Animal Care and Use Committee of the University of Illinois at Urbana-Champaign, United States), Illinois State Lands and Nature Preserves Permits, United States Fish and Wildlife Service Permit number MB815400-1, and United States Geological Survey Banding Permit number 06507.
Author Contributions
JH and WS formulated the questions, collected the data, supervised research and analyzed the data. Both authors contributed to the article and approved the submitted version.
Funding
Financial support for this work was provided by the United States Fish and Wildlife Service (INT 1448-0003-95-1007 and Neotropical Migratory Bird Conservation Act funds), the Illinois Department of Natural Resources (Conservation 2000 Grant and State Wildlife Grant), the Nature Conservancy in Illinois, the National Fish and Wildlife Fund, The R.J. Kosie Fund, Wings Over the Americas, the University of Illinois, Champaign County and Decatur Audubon Societies, Illinois Ornithological Society, American Ornithologists’ Union, Sigma Xi, North American Bluebird Society, and the American Museum of Natural History. None of the funders had any influence on the content of the submitted or published manuscript. None of the funders require approval of the final manuscript to be published.
Conflict of Interest
The authors declare that the research was conducted in the absence of any commercial or financial relationships that could be construed as a potential conflict of interest.
Acknowledgments
The tremendous efforts of dedicated field assistants and graduate students greatly improved this research. We are grateful to the members of the Cache River Joint Venture (the United States Fish and Wildlife Service, The Nature Conservancy in Illinois, and the Illinois Department of Natural Resources) for providing assistance with the logistics of conducting the field research. The Avian Ecology Group at the University of Illinois provided fruitful discussions. Last, we are thankful for the editorial staff and two reviewers whose input greatly improved the manuscript.
References
Batzer, D., Gallardo, B., Boulton, A., and Whiles, M. (2016). “Invertebrates of temperate-zone river floodplains,” in Invertebrates in Freshwater Wetlands: An International Perspective on Their Ecology, eds D. Batzer and D. Boix (Cham: Springer), 451–492. doi: 10.1007/978-3-319-24978-0_13
Baxter, C. V., Fausch, K. D., and Saunders, W. C. (2005). Tangled webs: reciprocal flows of invertebrate prey link streams and riparian zones. Freshwater Biol. 50, 201–220. doi: 10.1111/j.1365-2427.2004.01328.x
Berthold, P. (2001). Bird Migration: A General Survey, 2nd Edn. New York, NY: Oxford University Press.
Both, C. (2010). “Food availability, mistiming, and climatic change,” in Effects of Climate Change on Birds, eds A. P. Møller, W. Fielder, and P. Berthold (Oxford, UK: Oxford University Press), 129–147.
Both, C., and Visser, M. E. (2001). Adjustment to climate change is constrained by arrival date in a long-distance migrant bird. Nature 411, 296–298. doi: 10.1038/35077063
Both, C., Artemyev, A. V., Blaauw, B., Cowie, R. J., Dekhuijzen, A. J., Eeva, T., et al. (2004). Large-scale geographical variation confirms that climate change causes birds to lay earlier. Proc. R. Soc. B 271, 1657–1662. doi: 10.1098/rspb.2004.2770
Both, C., Bouwhuis, S., Lessells, C. M., and Visser, M. E. (2006). Climate change and population declines in a long-distance migratory bird. Nature 441, 81–83. doi: 10.1038/nature04539
Both, C., van Asch, M., Bijlsma, R. G., van den Burg, A. B., and Visser, M. E. (2009). Climate change and unequal phenological changes across four trophic levels: constraints or adaptations? J. Anim. Ecol. 78, 73–83. doi: 10.1111/j.1365-2656.2008.01458.x
Both, C., van Turnhout, C. A. M., Bijlsma, R. G., Siepel, H., van Strien, A. J. V., and Foppen, R. P. B. (2010). Avian population consequences of climate change are most severe for long-distance migrants in seasonal habitats. Proc. R. Soc. B 277, 1259–1266. doi: 10.1098/rspb.2009.1525
Bowlin, M. S., Cochran, W. W., and Wikelski, M. (2005). Biotelemetry of New World thrushes during migration: physiology, energetics and orientation in the wild. Integr. Comp. Biol. 45, 295–304. doi: 10.1093/icb/45.2.295
Bronson, F. H. (1985). Mammalian reproduction: an ecological perspective. Biol. Reprod. 32, 1–26. doi: 10.1095/biolreprod32.1.1
Brown, C., and Brown, M. B. (2000). Weather-mediated natural selection on arrival time in cliff swallows (Petrochelidon pyrrhonota). Behav. Ecol. Sociobiol. 47, 339–345. doi: 10.1007/s002650050674
Bulluck, L., Huber, S., Viverette, C., and Blem, C. (2013). Age-specific responses to spring temperature in a migratory songbird: older females attempt more broods in warmer springs. Ecol. Evol. 3, 3298–3306. doi: 10.1002/ece3.673
Charmantier, A., and Gienapp, P. (2014). Climate change and timing of avian breeding and migration: evolutionary versus plastic responses. Evol. Appl. 7, 15–28. doi: 10.1111/eva.12126
Charmantier, A., McCleery, R. H., Cole, L. R., Perrins, C., Kruuk, L. E. B., and Sheldon, B. C. (2008). Adaptive phenotypic plasticity in response to climate change in a wild bird population. Science 320, 800–803. doi: 10.1126/science.1157174
Coe, B. H., Beck, M. L., Chin, S. Y., Jachowski, C., and Hopkins, W. A. (2015). Local variation in weather conditions influences incubation behavior and temperature in a passerine bird. J. Avian Biol. 46, 385–394. doi: 10.1111/jav.00581
Cohen, J. M., Lajeunesse, M. J., and Rohr, J. R. (2018). A global synthesis of animal phenological responses to climate change. Nat. Clim. Change 8, 224–228. doi: 10.1038/s41558-018-0067-3
Conway, C. J., and Martin, T. E. (2000). Effects of ambient temperature on avian incubation behavior. Behav. Ecol. 11, 178–188. doi: 10.1093/beheco/11.2.178
Cotton, P. A. (2003). Avian migration phenology and global climate change. Proc. Natl. Acad. Sci. U S A. 100, 12219–12222. doi: 10.1073/pnas.1930548100
Cox, A., Robertson, R., Lendvai, Á, Everitt, K., and Bonier, F. (2019). Rainy springs linked to poor nestling growth in a declining avian aerial insectivore Tachycineta bicolor. Proc. R. Soc. B 286:20190018. doi: 10.1098/rspb.2019.0018
Culp, L. A., Cohen, E. B., Scarpignato, A. L., Thogmartin, W. E., and Marra, P. P. (2017). Full annual cycle climate change vulnerability assessment for migratory birds. Ecosphere 8:e01565. doi: 10.1002/ecs2.1565
Daan, S., and Tinbergen, J. M. (1997). “Adaptation of life histories,” in Behavioural Ecology: An Evolutionary Approach, 4th Edn, eds J. R. Krebs and N. B. Davies (Oxford, UK: Oxford University Press), 311–333.
Dodson, J. C., Moy, N. J., and Bulluck, L. P. (2016). Prothonotary warbler nestling growth and condition in response to variation in aquatic and terrestrial prey availability. Ecol. Evol. 6, 7462–7474. doi: 10.1002/ece3.2400
Dunn, P. O., and Winkler, D. W. (1999). Climate change has affected the breeding date of tree swallows throughout North America. Proc. R. Soc. B 266, 2487–2490. doi: 10.1098/rspb.1999.0950
Dunn, P. O., and Winkler, D. W. (2010). “Effects of climate change on timing of breeding and reproductive success in birds,” in Effects of Climate Change on Birds, eds A. P. Møller, W. Fielder, and P. Berthold (Oxford, UK: Oxford University Press), 113–128.
Dunn, P. O., Winkler, D. W., Whittingham, L. A., Hannon, S. J., and Robertson, R. J. (2011). A test of the mismatch hypothesis: How is timing of reproduction related to food abundance in an aerial insectivore? Ecology 92, 450–461. doi: 10.1890/10-0478.1
Fleming, W. J., and Petit, D. R. (1986). Modified milk carton nest box for studies of prothonotary warblers. J. Field Ornithol. 57, 313–315.
Franks, S. E., Pearce−Higgins, J. W., Atkinson, S., Bell, J. R., Botham, M. S., Brereton, T., et al. (2018). The sensitivity of breeding songbirds to changes in seasonal timing is linked to population change but cannot be directly attributed to the effects of trophic asynchrony on productivity. Glob. Change Biol. 24, 957–971. doi: 10.1111/gcb.13960
Fraser, K. C., Silverio, C., Kramer, P., Mickle, N., Aeppli, R., and Stutchbury, B. J. M. (2013). A trans-hemispheric migratory songbird does not advance spring schedules or increase migration rate in response to record-setting temperatures at breeding sites. PLoS One 8:e64587. doi: 10.1371/journal.pone.0064587
Greenberg, R., and Marra, P. P. (eds) (2005). Birds of Two Worlds. Baltimore, MD: Johns Hopkins University Press.
Gwinner, E. (2003). Circannual rhythms in birds. Curr. Opin. Neurobiol. 13, 770–778. doi: 10.1016/j.conb.2003.10.010
Gwinner, E., and Helm, B. (2003). “Circannual and circadian contributions to the timing of avian migration,” in Avian Migration, eds P. Berthold, E. Gwinner, and E. Sonnenschein (Heidelberg: Springer), 81–95. doi: 10.1007/978-3-662-05957-9_5
Halupka, L., Dyrcz, A., and Borowiec, M. (2008). Climate change affects breeding of reed warblers Acrocephalus scirpaceus. J. Avian Biol. 39, 95–100. doi: 10.1111/j.0908-8857.2008.04047.x
Hansen, J., Ruedy, R., Stao, M., Imhoff, M., Lawrence, W., Easterling, D., et al. (2001). A closer look at United States and global surface temperature change. J. Geophys. Res. 106, 23947–23963. doi: 10.1029/2001JD000354
Heinrich, K. K., Whiles, M. R., and Roy, C. (2013). Cascading ecological responses to an in-stream restoration project in a Midwestern river. Restor. Ecol. 22, 72–80. doi: 10.1111/rec.12026
Hodgson, J. A., Thomas, C. D., Oliver, T. H., Anderson, B. J., Brereton, T. M., and Crone, E. E. (2011). Predicting insect phenology across space and time. Glob. Change. Biol. 17, 1289–1300. doi: 10.1111/j.1365-2486.2010.02308.x
Holland, R. A., Wikelski, M., and Wilcove, D. S. (2006). How and why do insects migrate? Science 313, 794–796. doi: 10.1126/science.1127272
Hoover, J. P. (2001). An Experimental Study of Behavioral Responses to Nest Predation and Brood Parasitism in a Migratory Songbird.Dissertation. Urbana: University of Illinois at Urbana-Champaign.
Hoover, J. P. (2003a). Decision rules for site fidelity in a migratory songbird, the prothonotary warbler. Ecology 84, 416–430. doi: 10.1890/0012-9658(2003)084[0416:DRFSFI]2.0.CO;2
Hoover, J. P. (2003b). Multiple effects of brood parasitism reduce the reproductive success of prothonotary warblers, Protonotaria citrea. Anim. Behav. 65, 923–934. doi: 10.1006/anbe.2003.2155
Hoover, J. P. (2006). Water depth influences nest predation for a wetland-dependent bird in fragmented bottomland forests. Biol. Conserv. 127, 37–45. doi: 10.1016/j.biocon.2005.07.017
Hoover, J. P. (2009). Effects of hydrologic restoration on birds breeding in forested wetlands. Wetlands 29, 563–573. doi: 10.1672/08-75.1
Hoover, J. P., and Reetz, M. J. (2006). Brood parasitism increases provisioning rate, and reduces offspring recruitment and adult return rates, in a cowbird host. Oecologia 149, 165–173. doi: 10.1007/s00442-006-0424-1
Hoover, J. P., Davros, N. M., Schelsky, W. M., and Brawn, J. D. (2020). Local neighbor density does not influence reproductive output in a secondary cavity-nesting songbird. The Auk: Ornithol. Adv. 137, 1–15. doi: 10.1093/auk/ukaa002
Hostetler, J. A., Sillett, T. S., and Marra, P. P. (2015). Full-annual-cycle population models for migratory birds. Auk Ornithol. Adv. 132, 433–449. doi: 10.1642/AUK-14-211.1
Hurrell, J. W., and Trenberth, K. E. (2010). “Climate change,” in Effects of Climate Change on Birds, eds A. P. Møller, W. Fiedler, and P. Berthold (London, UK: Oxford University Press), 9–38.
IPCC (2013). “Climate Change 2013,” in The Physical Science Basis. Contribution of Working Group I to the Fifth Assessment Report of the Intergovernmental Panel on Climate Change, eds T. F. Stocker, D. Qin, G.-K. Plattner, M. Tignor, S. K. Allen, J. Boschung, et al. (Cambridge, UK: Cambridge University Press).
Jamieson, M. A., Trowbridge, A. M., Raffa, K. F., and Lindroth, R. L. (2012). Consequences of climate warming and altered precipitation patterns for plant-insect and multitrophic interactions. Plant Physiol. 160, 1719–1727. doi: 10.1104/pp.112.206524
Knudsen, E., Lindén, A., Both, C., Jonzén, N., Pulido, F., Saino, N., et al. (2011). Challenging claims in the study of migratory birds and climate change. Biol. Rev. 86, 928–946. doi: 10.1111/j.1469-185X.2011.00179.x
Kowalski, M. P. (1986). Weights and measurements of Prothonotary Warblers from southern Indiana, with a method of aging males. N. Am. Bird Band. 11, 129–131.
Kumar, V., Wingfield, J. C., Dawson, A., Ramenofsky, M., Rani, S., and Bartell, P. (2010). Biological clocks and regulation of seasonal reproduction and migration in birds. Physiol. Biochem. Zool. 83, 827–835. doi: 10.1086/652243
Langham, G. M., Schuetz, J. G., Distler, T., Soykan, C. U., and Wilsey, C. (2015). Conservation status of North American birds in the face of future climate change. PLoS One 10:e0135350. doi: 10.1371/journal.pone.0135350
Mazerolle, D. F., Sealy, S. G., and Hobson, K. A. (2011). Interannual flexibility in breeding phenology of a Neotropical migrant songbird in response to weather conditions at breeding and wintering areas. Ecoscience 18, 18–25. doi: 10.2980/18-1-3345
McKim-Louder, M. I., Hoover, J. P., Benson, T. J., and Schelsky, W. M. (2013). Juvenile survival in a Neotropical migratory songbird is lower than expected. PLoS One 8:e56059. doi: 10.1371/journal.pone.0056059
Miller-Rushing, A. J., Lloyd-Evans, T. L., Primack, R. B., and Satzinger, P. (2008). Bird migration times, climate change, and changing population sizes. Glob. Change Biol. 14, 1959–1972. doi: 10.1111/j.1365-2486.2008.01619.x
Mills, A. M. (2005). Changes in the timing of spring and autumn migration in North American migrant passerines during a period of global warming. Ibis 147, 259–269. doi: 10.1111/j.1474-919X.2005.00380.x
Milner-Gulland, E. J., Fryxell, J. M., and Sinclair, A. R. E. (2011). Animal Migration: A Synthesis. New York, NY: Oxford University Press. doi: 10.1093/acprof:oso/9780199568994.001.0001
Møller, A. P., Flensted-Jensen, E., Klarborg, K., Mardal, W., and Nielsen, J. T. (2010). Climate change affects the duration of the reproductive season in birds. J. Anim. Ecol. 79, 777–784. doi: 10.1111/j.1365-2656.2010.01677.x
Møller, A. P., Rubolini, D., and Lehikoinen, E. (2008). Populations of migratory bird species that did not show a phenological response to climate change are declining. Proc. Natl. Acad. Sci. U S A. 105, 16195–16200. doi: 10.1073/pnas.0803825105
Monkkönen, M., Helle, P., and Soppela, K. (1990). Numerical and behavioral-responses of migrant passerines to experimental manipulation of resident tits (Parus spp.) – heterospecific attraction in Northern breeding bird communities. Oecologia 85, 218–225. doi: 10.1007/BF00319404
Monroe, A. P., Hallinger, K. K., Brasso, R. L., and Cristol, D. A. (2008). Occurrence and implications of double brooding in a southern population of tree swallows. Condor 110, 382–386. doi: 10.1525/cond.2008.8341
Müller, M., Pasinelli, G., Schiegg, K., Spaar, R., and Jenni, L. (2005). Ecological and social effects on reproduction and local recruitment in the red-backed shrike. Oecologia 143, 37–50. doi: 10.1007/s00442-004-1770-5
Nakano, S., and Murakami, M. (2001). Reciprocal subsidies: Dynamic interdependence between terrestrial and aquatic food webs. Proc. Natl. Acad. Sci. U S A. 98, 166–170. doi: 10.1073/pnas.98.1.166
Nilsson, J.-Å (1994). Energetic bottle-necks during breeding and the reproductive cost of being too early. J. Anim. Ecol. 63, 200–208. doi: 10.2307/5595
Parejo, D. (2016). Informational mismatches: a neglected threat of climate change to interspecific interactions. Front. Ecol. Evol. 4:31. doi: 10.3389/fevo.2016.00031
Parmesan, C., and Yohe, G. (2003). A globally coherent fingerprint of climate change impacts across natural systems. Nature 421, 37–42. doi: 10.1038/nature01286
Perrins, C. M. (1970). The timing of birds’ breeding seasons. Ibis 112, 242–255. doi: 10.1111/j.1474-919X.1970.tb00096.x
Petit, L. J. (2020). “Prothonotary warbler (Protonotaria citrea), version 1.0,” in Birds of the World, eds A. F. Poole and F. B. Gill (Ithaca, NY: Cornell Lab of Ornithology), doi: 10.2173/bow.prowar.01
Petit, L. J., and Petit, D. R. (1996). Factors governing habitat selection by prothonotary warblers: field tests of the Fretwell-Lucas models. Ecol. Monogr. 66, 367–387. doi: 10.2307/2963523
Petit, L. J., Petit, D. R., Petit, K. E., and Fleming, W. J. (1990a). Intersexual and temporal variation in foraging ecology of prothonotary warblers during the breeding season. Auk 107, 133–145.
Petit, L. J., Petit, D. R., Petit, K. E., and Fleming, W. J. (1990b). Annual variation in the foraging ecology of prothonotary warblers during the breeding season. Auk 107, 146–152.
Primack, R. B., Ibanez, I., Higuchi, H., Lee, S. D., Wilson, A. M., et al. (2009). Spatial and interspecific variability in phenological responses to warming temperatures. Biol. Conserv. 142, 2569–2577. doi: 10.1016/j.biocon.2009.06.003
Pulido, F., and Widmer, M. (2006). Are long-distance migrants constrained in their evolutionary response to environmental change? Causes of variation in the timing of autumn migration in a blackcap (S. atricapilla) and two garden warbler (Sylvia borin) populations. Ann. N.Y. Acad. Sci. 1046, 228–241. doi: 10.1196/annals.1343.020
Pyle, P., Howell, S. N. G., Yunick, R. P., and DeSante, D. F. (1987). Identification Guide to North American Passerines. Bolinas, CA: Slate Creek Press.
Richardson, A. D., Keenan, T. F., Migliavacca, M., Ryu, Y., Sonnentag, O., and Toomey, M. (2013). Climate change, phenology, and phenological control of vegetation feedbacks to the climate system. Agric. and Forest Meteorol. 169, 156–173. doi: 10.1016/j.agrformet.2012.09.012
Robinson, S. K., and Hoover, J. P. (2011). “Does forest fragmentation and loss generate sources, sinks, and ecological traps in migratory songbirds?,” in Sources, Sinks and Sustainability, eds J. Liu, V. Hull, A. Morzillo, and J. A. Wiens (Cambridge, UK: Cambridge University Press), 423–449. doi: 10.1017/CBO9780511842399.022
Roy, D. B., and Sparks, T. H. (2000). Phenology of British butterflies and climate change. Glob. Change Biol. 6, 407–416. doi: 10.1046/j.1365-2486.2000.00322.x
Saino, N., Ambrosini, R., Rubolini, D., Von Hardenberg, J., Provenzale, A., Hüppop, K., et al. (2011). Climate warming, ecological mismatch at arrival and population decline in migratory birds. Proc. R. Soc. B 278, 835–842. doi: 10.1098/rspb.2010.1778
SAS Institute (2013). SAS® software, Version 9.4 for Windows. Copyright©. SAS and all other SAS Institute Inc. product or service names are registered trademarks or trademarks of SAS Institute Inc. Cary, NC: SAS Institute Inc.
Schwartz, M. D., Ahas, R., and Aasa, A. (2006). Onset of spring starting earlier across the Northern Hemisphere. Glob. Change Biol. 12, 343–351. doi: 10.1111/j.1365-2486.2005.01097.x
Shave, A., Garroway, C. J., Siegrist, J., and Fraser, K. C. (2019). Timing to temperature: egg-laying dates respond to temperature and are under stronger selection at northern latitudes. Ecosphere 10:e02974. doi: 10.1002/ecs2.2974
Shaw, A. K. (2016). Drivers of animal migration and implications in changing environments. Evol. Ecol. 30, 991–1007. doi: 10.1007/s10682-016-9860-5
Shipley, J. R., Twining, C. W., Taff, C. C., Vitousek, M. N., Flack, A., and Winkler, D. W. (2020). Birds advancing lay dates with warming springs face greater risk of chick mortality. Proc. Natl. Acad. Sci. U S A. 2020:202009864. doi: 10.1073/pnas.2009864117
Southwood, A., and Avens, L. (2010). Physiological, behavioral, and ecological aspects of migration in reptiles. J. Comp. Physiol. B 180, 1–23. doi: 10.1007/s00360-009-0415-8
Stephens, P. A., Mason, L. R., Green, R. E., Gregory, R. D., Sauer, J. R., Alison, J., et al. (2016). Consistent response of bird populations to climate change on two continents. Science 352, 84–87. doi: 10.1126/science.aac4858
Strode, P. K. (2003). Implications of climate change for North American wood warblers (Parulidae). Glob. Change Biol. 9, 1137–1144. doi: 10.1046/j.1365-2486.2003.00664.x
Tarof, S. A., Kramer, P. M., Hill, J. R., Tautin, J., and Stutchbury, B. J. M. (2011). Brood size and late breeding are negatively related to juvenile survival in a neotropical migratory songbird. Auk 128, 716–725. doi: 10.1525/auk.2011.11087
Thomas, D. W., Blondel, J., Perret, P., Lambrechts, M. M., and Speakman, J. R. (2001). Energetic and fitness costs of mismatching resource supply and demand in seasonally breeding birds. Science 291, 2598–2600. doi: 10.1126/science.1057487
Thorup, K., Tøttrup, A. P., Willemoes, M., Klaassen, R. H. G., Strandberg, R., and Vega, M. L. (2017). Resource tracking within and across continents in long-distance bird migrants. Sci. Adv. 3:e1601360. doi: 10.1126/sciadv.1601360
Tomotani, B. M., van der Jeugd, H., Gienapp, P., de la Hera, I., Pilzecker, J., Teichmann, C., et al. (2018). Climate change leads to differential shifts in the timing of annual cycle stages in a migratory bird. Glob. Change Biol. 24, 823–835. doi: 10.1111/gcb.14006
Townsend, A. K., Sillett, T. S., Lany, N. K., Kaiser, S. A., Rodenhouse, N. L., Webster, M. S., et al. (2013). Warm springs, early lay dates, and double brooding in a North American migratory songbird, the black-throated blue warbler. PLoS One 8:e59467. doi: 10.1371/journal.pone.0059467
Usui, T., Butchart, S. H. M., and Phillimore, A. B. (2017). Temporal shifts and temperature sensitivity of avian spring migratory phenology: a phylogenetic meta-analysis. J. Anim. Ecol. 86, 250–261. doi: 10.1111/1365-2656.12612
van Noordwijk, A. J., McCleery, R. H., and Perrins, C. M. (1995). Selection for the timing of Great Tit breeding in relation to caterpillar growth and temperature. J. Anim. Ecol. 64, 451–458. doi: 10.2307/5648
Verhulst, S., and Nilsson, J.-Å (2008). The timing of birds’ breeding seasons: a review of experiments that manipulated timing of breeding. Phil. Trans. R. Soc. B 363, 399–410. doi: 10.1098/rstb.2007.2146
Visser, M. E., and Both, C. (2005). Shifts in phenology due to global climate change: the need for a yardstick. Proc. R. Soc. B 272, 2561–2569. doi: 10.1098/rspb.2005.3356
Visser, M. E., and Gienapp, P. (2019). Evolutionary and demographic consequences of phenological mismatches. Nat. Ecol. Evol. 3, 879–885. doi: 10.1038/s41559-019-0880-8
Visser, M. E., Both, C., and Lambrechts, M. M. (2004). Global climate change leads to mistimed avian reproduction. Adv. Ecol. Res. 35, 89–110. doi: 10.1016/S0065-2504(04)35005-1
Visser, M. E., Caro, S. P., van Oers, K., Schaper, S. V., and Helm, B. (2010). Phenology, seasonal timing and circannual rhythms: towards a unified framework. Phil. Trans. R. Soc. B 365, 3113–3127. doi: 10.1098/rstb.2010.0111
Visser, M. E., Perdeck, A. C., van Balen, J. H., and Both, C. (2009). Climate change leads to decreasing bird migration distances. Glob. Change Biol. 15, 1859–1865. doi: 10.1111/j.1365-2486.2009.01865.x
Visser, M. E., van Noordwijk, A. J., Tinbergen, J. M., and Lessells, C. M. (1998). Warmer springs lead to mistimed reproduction in great tits (Parus major). Proc. R. Soc. B 265, 1867–1870. doi: 10.1098/rspb.1998.0514
Vogel, M. M., Zscheischler, J., Wartenburger, R., Dee, D., and Seneviratne, S. I. (2019). Concurrent 2018 hot extremes across Northern Hemisphere due to human-induced climate change. Earth Fut. 7, 692–703. doi: 10.1029/2019EF001189
Wheelwright, N. T., Tice, K. A., and Freeman-Gallant, C. R. (2003). Post-fledging parental care in savannah sparrows: sex, size, and survival. Anim. Behav. 65, 435–443. doi: 10.1006/anbe.2003.2086
Williams, C. B. (1957). Insect Migration. Annu. Rev. Entomol. 2, 163–180. doi: 10.1146/annurev.en.02.010157.001115
Wilson, S., and Arcese, P. (2003). El Nino drives timing of breeding but not population growth in the song sparrow (Melospiza melodia). Proc. Natl. Acad. Sci. U S A. 100, 11139–11142. doi: 10.1073/pnas.1931407100
Keywords: first egg dates, global warming, local temperature effects, long-distance migratory bird, prothonotary warbler, Protonotaria citrea, reproductive output
Citation: Hoover JP and Schelsky WM (2020) Warmer April Temperatures on Breeding Grounds Promote Earlier Nesting in a Long-Distance Migratory Bird, the Prothonotary Warbler. Front. Ecol. Evol. 8:580725. doi: 10.3389/fevo.2020.580725
Received: 06 July 2020; Accepted: 10 November 2020;
Published: 02 December 2020.
Edited by:
Stuart Peter Sharp, Lancaster University, United KingdomReviewed by:
Helen Chmura, University of Alaska Fairbanks, United StatesKevin C. Fraser, University of Manitoba, Canada
Copyright © 2020 Hoover and Schelsky. This is an open-access article distributed under the terms of the Creative Commons Attribution License (CC BY). The use, distribution or reproduction in other forums is permitted, provided the original author(s) and the copyright owner(s) are credited and that the original publication in this journal is cited, in accordance with accepted academic practice. No use, distribution or reproduction is permitted which does not comply with these terms.
*Correspondence: Jeffrey P. Hoover, j-hoover@illinois.edu