- 1Department of Functional and Evolutionary Ecology, Estación Experimental de Zonas Áridas (EEZA–CSIC), Almería, Spain
- 2Department of Zoology, Facultad de Ciencias, Universidad de Granada, Granada, Spain
- 3Unidad Asociada (CSIC): Coevolución: Cucos, Hospedadores y Bacterias Simbiontes, Facultad de Ciencias, Universidad de Granada, Granada, Spain
Research on the mechanisms involved in host location by parasites is of paramount importance and may aid in developing protective measures against them. This topic attains far-reaching repercussions for human and animal welfare regarding parasites transmitting vector-borne pathogens, such as blood-feeding flies. Very few studies have evaluated the effect of bird-derived cues on attraction of vectors in field conditions. We here explored the attraction of different groups of blood-feeding flies (mosquitoes, blackflies and biting midges) to auditory cues produced by begging hoopoe (Upupa epops) nestlings, and to three chemical cues derived from hoopoe nestlings or nests (uropygial secretion, symbiotic bacteria isolated from the secretion, and nest material) in the field. We deployed insect traps baited with the different stimuli at the beginning and at the end of the hoopoe breeding-season in four different habitats. Abundance of blood-feeding flies varied depending on habitat and sampling period. Begging auditory cues of nestling hoopoes did not affect abundance of flies. However, chemical stimuli affected abundance of mosquitoes, which were less abundant in traps baited with bacteria or with nest material than in control traps. Abundance of biting midges in traps also depended on the chemical stimulus but in interaction with sampling period or habitat. Fewer biting midges were collected in traps baited with bacteria and with secretion in the habitats where abundance of biting midges is higher. Our results suggest that uropygial secretion of hoopoes, and symbiotic bacteria living in this secretion, may repel blood-feeding flies from their nests.
Introduction
Parasitism is one the most important selection pressures influencing evolution across most taxa (Combes, 2001; Poulin, 2011). Thus, understanding the processes underlying and governing parasite-host interactions is paramount from ecological, epidemiological and evolutionary points of view (Schmid-Hempel, 2011; Webster and Cardé, 2017; Chandrasegaran et al., 2020). For efficient parasitism, the parasite first needs to establish contact with the host. The study of factors mediating attraction of the parasite to locate and colonize a host have long been searched for (e.g., Sutcliffe, 1986, 2010; Allan et al., 1987), especially for some selected parasite types of medical and veterinary importance (Mullen and Durden, 2002). However, it is recognized that after decades of research, our comprehension of these factors is still limited and incomplete for many parasite taxa (Tomás et al., 2008b; Tomás and Soler, 2016; Webster and Cardé, 2017; Veiga et al., 2020).
Birds and their ectoparasites continue to be fruitful model systems to shed light on many aspects of parasite-host interactions (Loye and Zuk, 1991; Clayton and Moore, 1997). Among all ectoparasites of birds, our knowledge on free-living blood-feeding flies still lies far behind that of nest-dwelling ectoparasites, mainly due to the more ephemeral contact with their host of the former, which poses subsequent challenges for their sampling and study (Tomás et al., 2008a; López-Rull and Macías-García, 2015). Yet among all sort of blood-feeding flies affecting birds, three dipteran families have received the most attention by researchers, mainly because of their nearly world-wide distribution and of their importance in the transmission of vector-borne pathogens. These vectors are commonly named mosquitoes, blackflies and biting midges.
Mosquitoes (Culicidae) are the best-known family of blood-feeding flies due to their major importance as vectors of human diseases (Foster and Walker, 2002). They are also vectors of infection for birds all over the world, being the main responsible for transmission of malaria parasites of the genus Plasmodium, and of several viruses (Naugle et al., 2004; Valkiûnas, 2005; Becker et al., 2010). Despite being relatively large-sized and reaching high abundances in many areas, the direct impact on wild birds caused by blood-sucking and associated nuisances of these mainly crepuscular or nocturnal insects is scarcely known. Blackflies (Simuliidae) are small flies present in all continents except Antarctica. Blackflies can build up huge populations specially in boreal ecosystems (Malmqvist et al., 2004). These mainly diurnal flies can cause nestling mortality (Hunter et al., 1997; Smith et al., 1998), complete nest failures (Solheim et al., 2013) and even provoke mass desertions of bird colonies (Bukaciński and Bukacińska, 2000). They are known vectors of filarial nematodes, bacteria, viruses and haemosporidians such as Leucocytozooon or Haemoproteus malarial parasites (Adler and McCreadie, 2002; Adler et al., 2004). Biting midges of the genus Culicoides (Ceratopogonidae) are tiny flies also globally distributed that can reach huge abundances in certain areas, especially in tropical and subtropical regions (Mullen, 2002). They are mainly crepuscular or nocturnal, and have detrimental effects on nestling birds (Tomás et al., 2008b). Biting midges are known to transmit viruses, filarial worms, and several malarial parasites of the genus Haemoproteus and Plasmodium (Borkent, 2005; Valkiûnas, 2005; Martínez-de la Puente et al., 2011a).
Host seeking by these blood-feeding insects relies on the detection of host associated cues, which include body heat, carbon dioxide (CO2), and different odors (Lehane, 2005; Takken and Knols, 2010; Cardé, 2015; Lazzari, 2019; Castaño-Vázquez et al., 2020; Greppi et al., 2020). Long range detection of hosts is assumed to rely on chemical cues, which are combined with heat and visual cues when in closer proximity to hosts (Sutcliffe, 1986; Allan et al., 1987; Gibson and Torr, 1999; Cardé, 2015; Greppi et al., 2020). It has also been suggested that ectoparasites might use bird host auditory cues for host location (Tomás and Soler, 2016), though this hypothesis has not yet been tested. Members of several ectoparasite groups locate their hosts by means of host auditory cues (Tomás and Soler, 2016). These include Sarcophagidae and Tachinidae parasitoid flies attracted to calling crickets and cicadas (Lehmann, 2003; Farris et al., 2009), and also Chaoboridae phantom midges, Corethrellidae frog-biting midges and Culicidae mosquitoes attracted to frog calls (Borkent, 2008; Toma et al., 2019). Anecdotal evidence further suggests that Corethrellidae and mosquitoes can be attracted to bird calls or songs (Camp, 2006; Bartlett-Healy et al., 2008; see Tomás and Soler, 2016). Overall, current knowledge on the array and specificity of cues used by blood-feeding flies to locate hosts is still incomplete for many parasite groups. Even different related parasites may not be affected by the same array of cues for host location, a possibility that has received little attention. Regarding chemical cues derived from birds that may be involved in host detection by blood-feeding flies, several odor sources have been investigated. For instance, mosquitoes (Cooperband et al., 2008) and biting midges (Fernandes-Rios et al., 2020) are attracted to traps baited with bird feces. Yet, odors emitted or derived from the uropygial gland of birds have been repeatedly suggested as major candidates involved in attraction of biting flies. Thus, extracts of uropygial glands of crows Corvus brachyrhynchus and common loons Gavia immer have been shown to attract mosquitoes (Russell and Hunter, 2005) and blackflies (Fallis and Smith, 1964; Bennett et al., 1972), respectively. Birds continuously rub their uropygial secretion onto their plumage and skin, which confers them specific odor signatures. This may explain that mosquitoes and blackflies are also attracted to chemical cues derived from bird feathers, in combination with CO2 (Allan et al., 2006) or with visual cues provided by bird decoys (Weinandt et al., 2012), respectively. Mosquitoes are also attracted to different chemical compounds prevalent in the odorant profile of birds (and humans) (Syed and Leal, 2009). In contrast, other studies have not found attraction of blood-feeding flies to uropygial secretions of birds. Biting midges and blackflies were not attracted to uropygial secretions of blue tits Cyanistes caeruleus and feral pigeons Columba livia (Martínez-de la Puente et al., 2011b), while mosquitoes were not attracted to uropygial secretions of house sparrows Passer domesticus (Garvin et al., 2018a; Díez-Fernández et al., 2019, 2020). Alternatively, certain odors released or derived from the uropygial gland may be employed by birds as repellents of ectoparasites (Moyer et al., 2003).
The hoopoe (Upupa epops) can be regarded as a quite special case in relation to its uropygial secretion. The uropygial gland of this bird is unusually large and, only in reproducing females and nestlings, produces a dark and pungent, malodorous secretion (Soler et al., 2008; Martín-Vivaldi et al., 2009, 2010). The hoopoe (and two related species) is unique in harboring symbiotic Enterococci bacteria in their uropygial secretion, which produce antibiotic substances (Soler et al., 2008). Enterococcus faecalis is by far the most prevalent bacteria present when aerobically culturing hoopoe uropygial secretions (Soler et al., 2008; Ruiz-Rodríguez et al., 2012). Analysis of bacterial abundance by different methodologies revealed consistently high bacterial loads in uropygial secretions (Soler et al., 2008; Rodríguez-Ruano et al., 2018), and similar bacterial community composition in birds from different nests (Rodríguez-Ruano et al., 2018). This secretion is rubbed into plumage and eggs through preening (Soler et al., 2014; Martínez-García et al., 2015), and it has been shown to increase hatching success (Martín-Vivaldi et al., 2014). The uropygial gland microbiome is partially acquired from the environment, and nest material is thought to be a source of bacteria (Martín-Vivaldi et al., 2018; Díaz-Lora et al., 2019; Campos-Cerda and Bohannan, 2020). Hoopoes do not add materials to the cavity, so nest materials mainly comprise rotten wood from cavity walls, old nest debris and feces and prey remains (Martín-Vivaldi et al., 2016). Besides the antimicrobial function, uropygial secretions may play a role in sexual selection (Whittaker et al., 2011; Amo et al., 2012; Díaz-Lora et al., 2020) or deterring predators (Jacob and Ziswiler, 1982) or ectoparasites (Soler et al., 2010).
The abundance and composition of vector and host species in the community vary across time and space, geographical variability being influenced for instance by environmental variables (e.g., Ferraguti et al., 2018; van Hoesel et al., 2019; Nourani et al., 2020). Vector species composition and abundance may affect host investment in antiparasite or immunological defenses (e.g., Moyer et al., 2002). Both factors, variation in vector species and in host traits, might in turn influence the cues employed by blood-feeding flies to locate their avian hosts in any given habitat (Webster and Cardé, 2017). It is important therefore to take into account seasonal and spatial variability when researching on host location mechanisms by vectors.
In this study, we assessed the potential role of auditory and chemical cues of hoopoes in the attraction of blood-feeding flies to insect traps in the field. Specifically, we assessed the potential role of nestling begging auditory cues, and of chemical cues released by the uropygial secretion, by bacteria living in the uropygial secretion, and by the nest substrate. We expected that these auditory and chemical cues were used by blood-feeding flies for host location and acted as attractants. Alternatively, traps baited with these auditory or chemical stimuli may render fewer captures if these stimuli acted as repellents for blood feeding ectoparasitic flies.
Materials and Methods
Study Area
The study was carried out during the breeding season of 2019 in the Hoya de Guadix (37° 18′ N, 38° 11′ W), located in the south east of Spain (e.g., Martín-Vivaldi et al., 1999). The experiment was performed in four different habitats representative of the main variability found in the study area. These habitats were: (1) irrigated (irrigated crops where fruit trees, including olive trees, as well as vegetables and greens are grown); (2) oak savannah (Mediterranean savannah, with scattered holm-oak (Quercus ilex) trees); (3) steppe (high-altitude arid plateaux with scarce vegetation represented mainly by a few Retama sphaerocarpa and crops of Prunus dulcis); and 4) pine forest (Pinus pinaster and P. halepensis orchards scattered within the arid plateaux). Distance between these sampled locations at different habitats ranges from 3.5 to 15 km. Proximity to waterbodies suitable for oviposition of vectors (small irrigation channels and ponds) is higher in the irrigated habitat, intermediate in the savannah and pines habitat, and lower in the steppe habitat. Hoopoe nests are scattered in the area, with higher densities in the pines habitat, intermediate in the savannah and irrigated habitat, and lower densities in the steppe.
Sampling of Blood-Feeding Flies
We carried out two samplings of vectors of three consecutive days each. The first sampling (May 4th–May 7th) was conducted coinciding when most bird species in the area start reproduction, and the second sampling (June 3rd–June 6th) was conducted when most birds in the area are raising nestlings. Weeks with similar and stable weather, and with no rain, were selected for both sampling periods. For each of the sampling periods and in each of the four habitats, we installed four CDC miniature downdraft suction traps (model 1212; John W. Hock, Gainesville, FL) but with the lights removed to focus exclusively on attraction or repulsion caused by the tested stimuli (see Russell and Hunter, 2005). The traps were placed at 1.5 m above ground, in the shadow provided by vegetation cover, and were operated for 24 h periods (Silver, 2008a; Becker et al., 2010). Distance between the four traps within each habitat was 25 m. Location of the traps was the same in both sampling periods. Traps were baited with a source of CO2 and a combination of chemical and auditory stimulus. Dry ice was used as a source of CO2. For that, 800 g of dry ice maintained in a cool box were used in each trap per 24 h, to ensure the continued emission of CO2 from a standard cannula (ref. G8581, Entomopraxis, Barcelona, Spain) located close to the fan of the trap (Veiga et al., 2018).
Auditory Stimulus
As auditory stimulus, we played back a recording of begging calls of hoopoe nestlings. This recording was constructed with 20 sequences of begging, each lasting between 0.5 and 2 min, recorded from five nests (four sequences from each nest) when nestlings were 2/3–3/3 grown. These sequences were played back in random order but alternated with a 2 min silence, thus simulating a maximum of one provisioning visit by a parent every 2.5 min. This 60 min-recording was played back in a MP3 device at 65 dB (measured at 20 cm of the speaker, which was hung close to the trap fan entrance) in a continuous loop for 6 h (the duration of the battery of the MP3 device). A speaker with no sound was used as the control auditory treatment. Traps were deployed at 19:00 h and collected insects for 24 h periods. All traps were checked in the morning, to replace batteries and MP3 devices, so that auditory stimuli were displayed for 12 of the 24 h periods.
Chemical Stimuli
As chemical stimuli, we used one of three different products derived from hoopoe nestlings (or nests) presented in a Petri dish inside a black and opaque cotton bag hung close to the fan entrance of the trap (an empty Petri dish was used as Control treatment): (1) Secretion: malodorous uropygial secretion of hoopoe nestlings. The secretion of nestlings when 2/3–3/3 grown was collected from active nests in the area following the protocol described in Soler et al. (2008). Briefly, the secretion was collected using an automatic 1–10 μl micropipette with a previously autoclaved tip that was gently introduced into the opening of the papilla of the uropygial gland and directly pipetted the secretion. The collected secretion was introduced in sterile microcentrifuge tubes that were kept frozen at −20°C until usage. For the experiment, the secretion of all nestlings in a nest was collected with a cotton swab that was then fixed inside a Petri dish (see Russell and Hunter, 2005). Secretion from different nests was used for different traps and days. (2) Nest: nest material and debris collected the day before the experiment from active hoopoe nests and kept at 4°C until usage. The amount needed to fill a Petri dish was used. Nest material from different nests was used for different traps and days. (3) Bacteria: a culture from our laboratory collection of the bacterium Enterococcus faecalis (MRR-10.3), isolated from hoopoe uropygial secretions. The bacterium was grown on Brain-Heart Infusion (BHI) medium at 37°C for 12 h and then BHI containing bacteria was spread onto TSA (Tryptic Soy Agar) plates by streaking technique and incubated again at 37°C for 24 h before being used as stimulus.
Within each habitat, two traps were baited with one of the three chemical treatments and two traps were controls, while one trap with each treatment was presented also with sound stimulus and the other with control sound (Table 1). After 24 h of sampling, the chemical treatment corresponding to the area was changed with a second chemical treatment. Again the next day, the third chemical treatment was applied to the area. Therefore, both auditory and chemical stimuli were always presented with their respective control treatments, while the chemical experimental treatment was changed from the first to the third day within the same sampling period, so that all habitats had the three chemical treatments in three consecutive days (Table 1). The order of treatment presentation and whether each trap within each habitat had control or experimental stimuli were randomly assigned. Thus, by assuming that abundance of blood-feeding flies does not differ within the same sampling period, we got a full factorial design of the auditory and chemical stimuli presented.
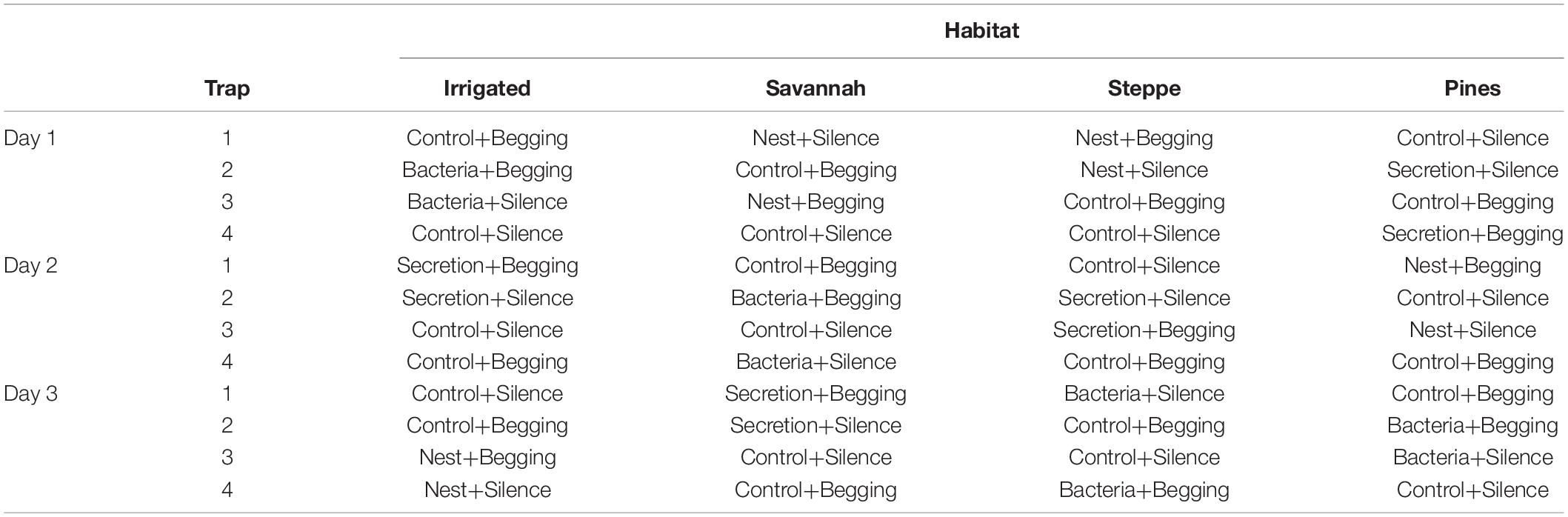
Table 1. Assignment of hoopoe stimuli (chemical + auditory) to traps for capturing blood-feeding flies in relation to day of sampling and habitat in Guadix, south eastern Spain.
Statistical Analyses
Abundance of mosquitoes, blackflies and biting midges were Box-Cox transformed before analyses. A multivariate analysis of variance (MANOVA) was used to first explore the potential effects of habitat and sampling period (as categorical predictors) on abundance of blood-feeding flies, with abundance of mosquitoes, blackflies and biting midges as dependent variables. Similar MANOVAs were used to explore differences in abundance of these blood-feeding flies between auditory and chemical stimuli separately, with habitat and sampling period as categorical predictors. Two-order interactions between sampling period, habitat and treatment (either auditory or chemical stimuli) were also explored. In all analyses, main effects were explored in models that did not include the interactions, while the interactions were estimated in models that included main effects. Fisher LSD tests were used for post hoc comparisons between the different experimental treatments and the control treatment. Analyses were conducted with Statistica 12 (StatSoft Inc, 2007). Residuals of the models were checked for normality. Means ± 95% confidence intervals (CI) are shown.
Results
Abundance of Blood-Feeding Flies
Mosquitoes were collected in 41 out of 96 traps (42.7%), ranging from 0 to 18 individuals, with an average of 3.1 ± 1.3 mosquitoes (excluding traps with no captures). Blackflies were collected in 64 out of 96 traps (66.7%), ranging from 0 to 242 individuals, with an average of 20.9 ± 10.5 blackflies. Biting midges (Culicoides spp.) were collected in 40 out of 96 traps (41.7%), ranging from 0 to 24 individuals, with an average of 5.1 ± 1.8 biting midges. Seventy-eight traps captured at least one blood-feeding fly, while 18 traps rendered zero captures. For the three groups of blood-feeding flies, most traps rendered a few vectors or none at all, while others have many, which resulted in typical aggregated right-skewed distributions of parasite abundance.
Habitat and Seasonal Differences
Abundance of blood-feeding flies varied depending on habitat and sampling period (Table 2). Blackflies, but not mosquitoes or biting midges, were more abundant at the beginning than at the end of the bird breeding season (Table 3 and Figure 1). Seasonal differences for these three blood-sucking vectors however depended on habitat types (Table 3). Mosquitoes were more abundant in the irrigated habitat, mainly during the second sampling period in early June (Table 2). The lower abundance of mosquitoes was detected in the steppe habitat (Table 2). Blackflies were more abundant in pine forest and in oak savannah, but mainly during the first sampling period, at early May (Table 2). Again, independent of the sampling period, the lower abundances were detected in the steppe habitat (Table 2). Biting midges were also more abundant in the pine forest and, during the second sampling period, they were also abundant in the irrigated habitat (Table 2).
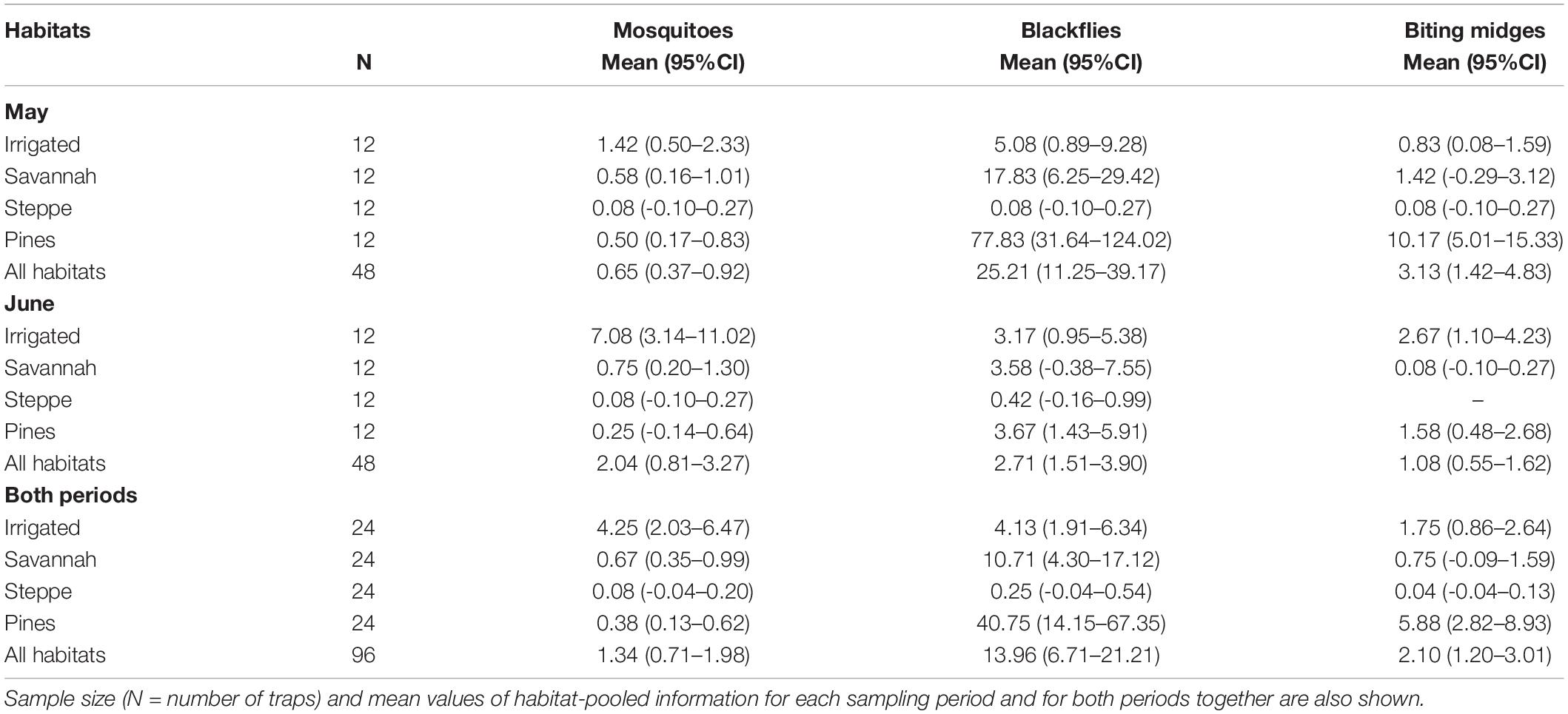
Table 2. Abundance of mosquitoes, blackflies, and biting midges [mean and 95% confidence intervals (CI)] collected in traps in four different habitats (irrigated, oak savannah, steppe and pine forest) and in two sampling periods (May and June).
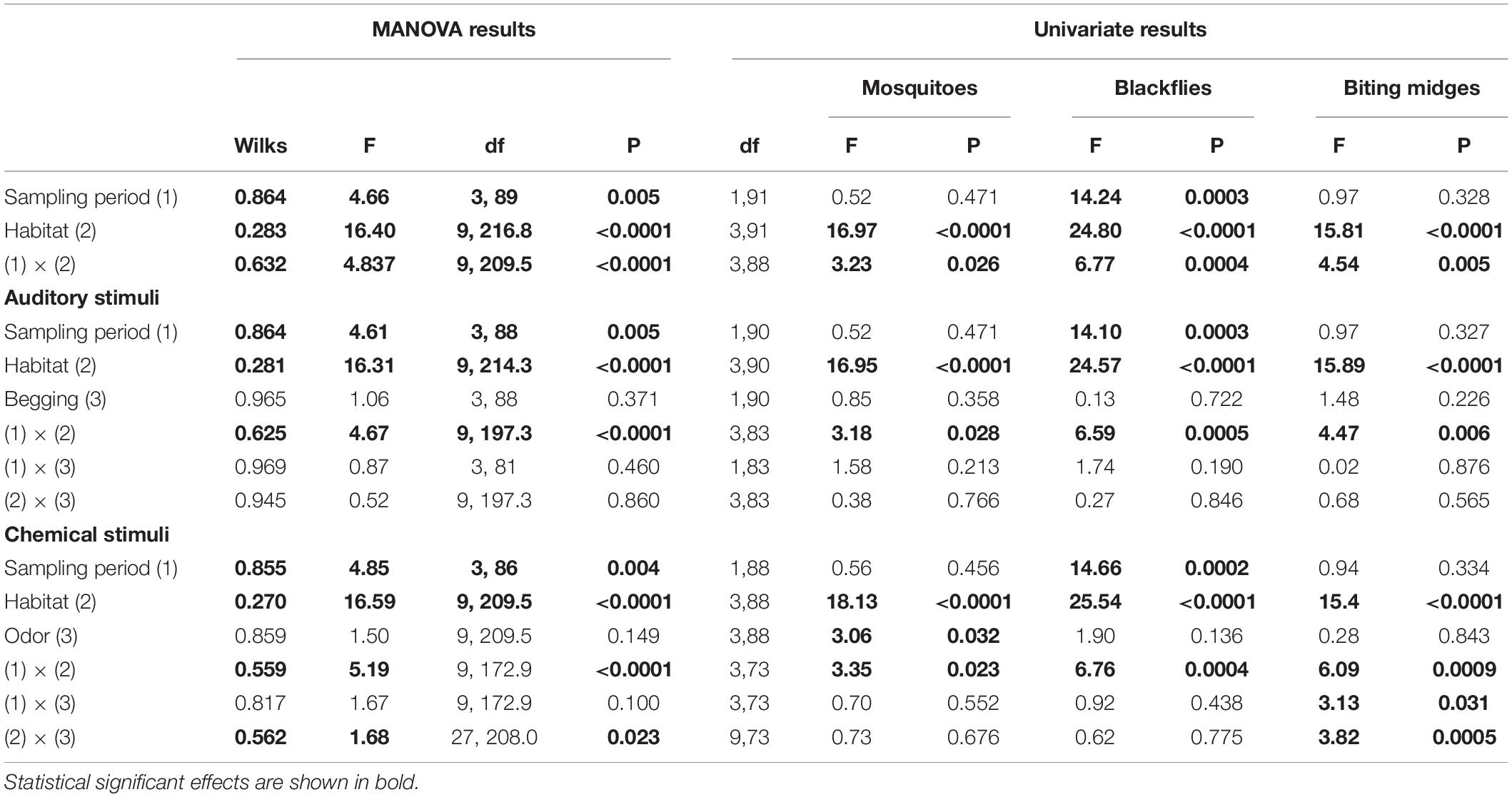
Table 3. Results from multivariate and univariate ANOVA exploring the effects of sampling period and habitat, together with the experimental effect of auditory and chemical stimuli, on abundance of blood-feeding flies collected in traps.
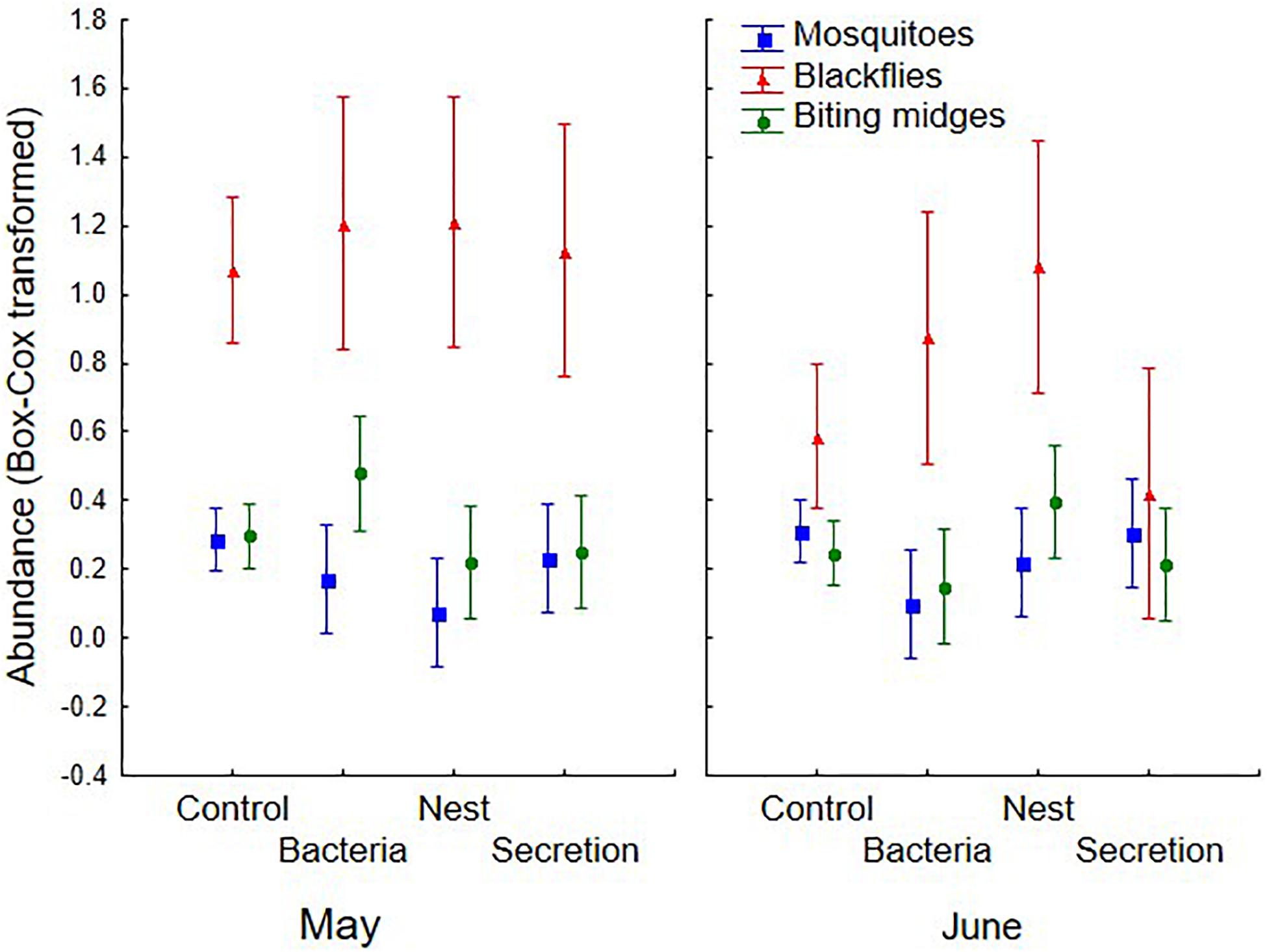
Figure 1. Abundance of blood-feeding flies (mosquitoes, blackflies, and biting midges) collected in traps baited with different chemical stimuli derived from hoopoe nestlings or nests (uropygial secretion, nest material, or bacteria found in the secretion), or without stimulus (control), and in two sampling periods (May and June). Mean and 95% confidence intervals (CI) are shown.
Effects of Auditory and Chemical Stimuli
Auditory stimuli did not affect abundance of blood-feeding flies in the traps after controlling for the effects of habitat and sampling period, neither as main effect nor in interaction with habitat or sampling period (Table 3). Chemical stimuli however affected abundance of mosquitoes and biting midges in the traps, but did not affect blackfly attraction (Table 3). Mosquitoes were less abundant in traps baited with bacteria or nest material odors than in control traps (Fisher LSD tests: bacteria vs. control: p = 0.016; nest vs. control: p = 0.026), and this effect was more apparent in the irrigated habitat (main effect in Table 3 and Figure 2). Abundance of mosquitoes did not differ between traps baited with the secretion and control traps (p = 0.670). Thus, bacteria from hoopoe secretion as well as nest material may repel mosquitoes from hoopoe nests. Moreover, abundance of biting midges depended on the used olfactory stimulus but in interaction with either sampling period or habitat (Table 3). Abundance of biting midges did not differ between control traps and traps baited with any of the olfactory stimuli, in first and second sampling periods (all p > 0.05). During the first sampling period, biting midges were more abundant in traps baited with bacteria stimulus than in those with nest material, while the opposite was found during the second sampling period (Figure 1). The effects of olfactory stimuli on biting midge abundance also depended on the habitat (Table 3). Traps baited with bacteria in the irrigated habitat, and with secretion in the pine forest, significantly captured less biting midges than control traps (bacteria vs. control in irrigated: p = 0.019; secretion vs. control in pines: p = 0.008; Figure 2). However, in the pine forest, traps baited with nest material captured more biting midges than control traps (p = 0.015). Given that biting midges were more abundant in the irrigated and pine habitats (Table 2), these results suggest that uropygial secretion of hoopoes, and symbiotic bacteria living in this secretion, might repel biting midges from their nests depending on habitat. Chemical cues released by nest material instead may attract biting midges to hoopoe nests, also depending on habitat.
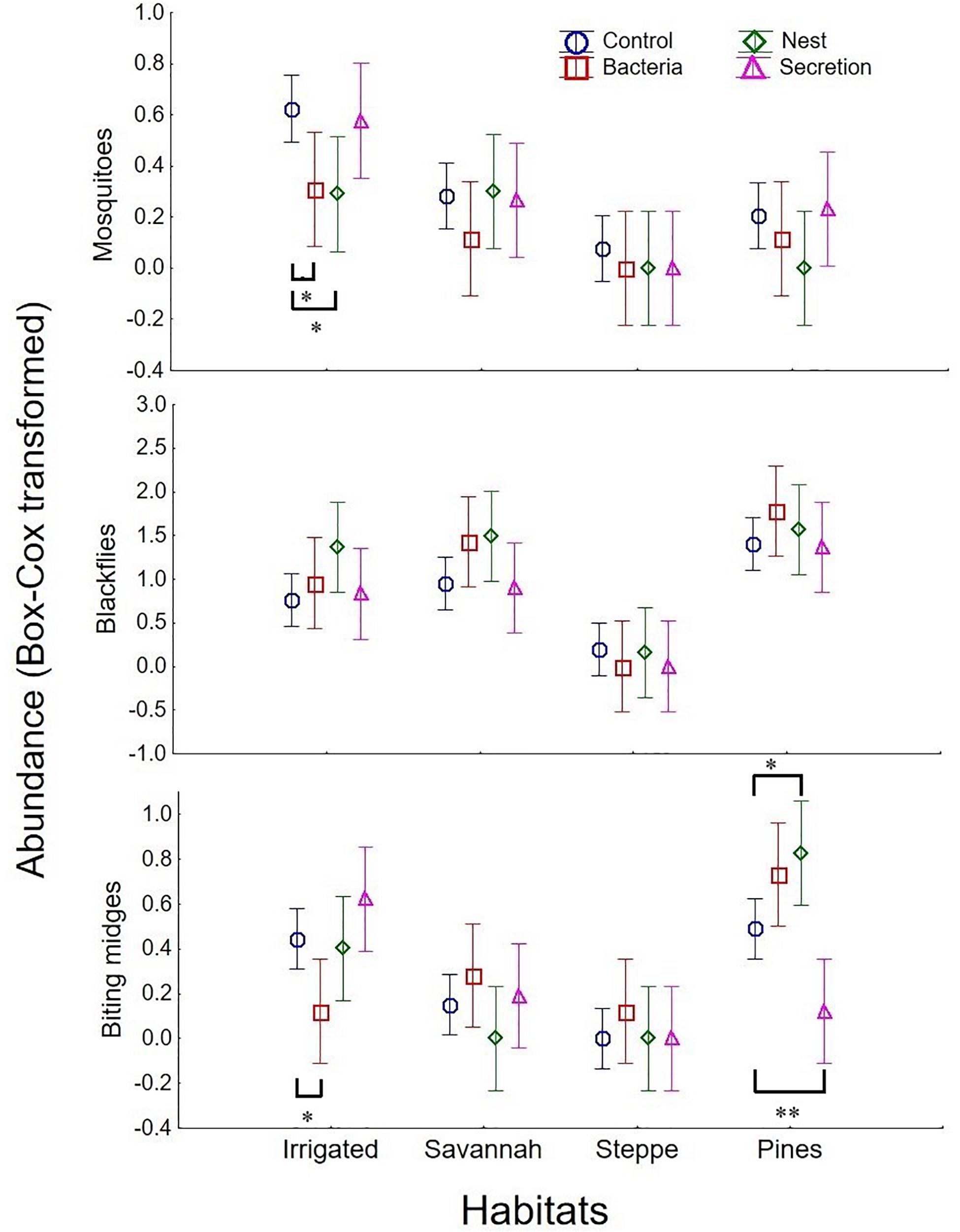
Figure 2. Abundance of blood-feeding flies (mosquitoes, blackflies, and biting midges) collected in traps baited with different chemical stimuli derived from hoopoe nestlings or nests (uropygial secretion, nest material, or bacteria found in the secretion), or without stimulus (control), and in four different habitats (irrigated, oak savannah, steppe and pine forest). Mean and 95% confidence intervals (CI) are shown. Statistically significant differences between the different chemical stimuli and the control in each habitat are also shown (*p < 0.05; **p < 0.01).
Discussion
Research on the factors and mechanisms involved in host location by ectoparasites is of paramount importance and may aid in developing protective measures against these parasites (Webster and Cardé, 2017; Chandrasegaran et al., 2020). This topic attains far-reaching repercussions for human and animal welfare regarding those parasites transmitting vector-borne pathogens, such as blood-feeding flies which include mosquitoes, blackflies and biting midges (Mullen and Durden, 2002; World Health Organization [WHO], 2020). We have found seasonal and habitat differences in abundance of blood-feeding flies that also varied depending on the vector considered.
Blackflies were more abundant at the beginning than at the end of the birds breeding season. When sampled inside avian nest-boxes in an oak forest in central Spain, both blackflies and biting midges were more abundant at nestling than at incubation stages, as well as in later than in early nests (Tomás et al., 2008a, 2012; Martínez-de la Puente et al., 2009a). Besides differences in sampling method, if we assume that obtained abundances reflect real abundances in our study area, this discrepancy in seasonal patterns may be partly due to habitat differences. Abundance of certain blood-feeding flies such as blackflies is greater at forested habitats (Martin et al., 1994). In accordance with this, we have found that blood-feeding flies are more abundant in the pines and irrigated habitats, and less abundant in the steppe habitat, which is less forested. This pattern might also match the relative abundance of hoopoes in the different habitats.
Current knowledge on dispersal patterns in blood-feeding flies in general is rather poor (e.g., Veiga et al., 2020). Apparently, dispersal of blackflies is concentrated within a few kilometers of the natal waters (Baldwin et al., 1975). Nevertheless, blackflies, by being active during the day when air convection is higher than during the night, may disperse over longer distances than other blood-feeding flies with more restricted nocturnal activity patterns (Service, 1980). This may explain that blackflies were the most abundant blood-feeding flies in the steppe habitat, which is situated further from potential oviposition sites. However, abundance of blackflies (and biting midges) in nests was not directly related with distance to water sources (i.e., oviposition sites) in another area (Tomás et al., 2008a). Besides dispersal behavior, environmental conditions may also explain the observed habitat differences in abundance of blood-feeding flies. Their activity is affected by weather variables including wind speed, temperature and humidity (e.g., Service, 1980; Garvin and Greiner, 2003; Martínez-de la Puente et al., 2009a), some of which may show marked differences among sampled habitats, even though distance among them is relatively small.
It has been suggested that ectoparasites might take advantage of auditory cues of their hosts for host location, likely in combination with other host cues (Tomás and Soler, 2016). The present study represents the first formal test of this hypothesis. However, we did not find that auditory cues of begging hoopoe nestlings attract mosquitoes, blackflies or biting midges to traps, even though auditory cues were presented in combination with CO2 and with several chemical stimuli. We cannot rule out the possibility that sound plays a role in host detection under different experimental conditions in other host-ectoparasite systems (Tomás and Soler, 2016). This hypothesis was mainly postulated for ectoparasites detecting auditory cues of begging offspring. Besides the potential attraction of auditory cues derived from begging nestlings, other host auditory cues may be detected by different ectoparasites (Tomás and Soler, 2016). In the past, for example, sound of wing beats of female mosquitoes was evaluated as attractant for increasing trap catches of various species of mosquitoes (Bidlingmayer, 1967; Silver, 2008b), so further research is necessary to fully test the hypothesis.
Regarding olfactory cues, we have found that different chemical stimuli affected attraction of blood-feeding flies to traps. First, fewer mosquitoes were collected in traps baited with bacteria and with nest material than in control traps. This effect was especially apparent in the irrigated habitat, where mosquitoes were more abundant. Second, abundance of biting midges collected in traps varied in relation to chemical stimuli, but in interaction with sampling period and with habitat. Depending on the sampling period, either traps baited with bacteria or with nest material collected fewer biting midges. This suggests that the main odor source in nests that is relevant for host detection by vectors might change during the breeding period of the birds. However, fewer biting midges were collected in traps baited with bacteria in the irrigated habitat and with uropygial secretion in the pines habitat compared to control traps. On the other hand, in the pines habitat, more biting midges were collected in traps baited with nest material than in control traps. It is noteworthy that the larger abundances of biting midges were detected in the irrigated and the pines habitats. Thus, results suggest that the uropygial secretion of hoopoes, as well as the chemical cues delivered by symbiotic bacteria living in this secretion, are not attractants for blood-feeding flies but might instead act as repellents for mosquitoes and biting midges.
Although all chemical stimuli came from hoopoes, they did not have the same effects attracting or repelling blood-feeding flies. Blackflies were not apparently affected by any of the stimuli presented, and CO2 may be a major attractant for this group (Sutcliffe, 2010). Nest material repelled mosquitoes, but attracted biting midges in the pines habitat. Uropygial secretion repelled biting midges in the pines habitat, while a culture of Enterococci isolated from hoopoe secretion repelled both mosquitoes and biting midges in the irrigated habitat. That the effect of the different chemical stimuli varies depending on the habitat may be explained by habitat differences in the abundance (or species composition) within these different blood-feeding flies. Additionally, it might suggest that different vector species may follow different cues for host location (e.g., Mullens and Gerry, 1998; Russell and Hunter, 2005; Allan et al., 2006; Gerry et al., 2009). Identifying blood-feeding flies to species level would be a next step to shed light on these possibilities. In addition, visual and olfactory associative learning of the vectors may affect host preferences (Takken and Verhulst, 2013). Uropygial secretion of hoopoes is special in harboring Enterococci symbiotic bacteria, especially in females during reproduction and in nestlings (Soler et al., 2008; Martín-Vivaldi et al., 2009, 2010). Hoopoes spread this secretion onto their plumage, brood patch and nest contents when preening (Martín-Vivaldi et al., 2014, 2018; Soler et al., 2014), while the nest environment is also an important source of bacteria (Ruiz-Rodríguez et al., 2014; Martínez-García et al., 2015, 2016; Rodríguez-Ruano et al., 2015; Martín-Vivaldi et al., 2018; Díaz-Lora et al., 2019). Therefore, multiple sources interact to create the microbiome assembly of hoopoe nesting environments. This microbiome may be a major component of chemical cues produced (Caro et al., 2015), with a prominent role of the nest, the parents and the offspring in integrating what has been termed the nidobiome (Soler et al., 2016; Campos-Cerda and Bohannan, 2020).
Different odors emitted by birds have been evaluated as attractants of vectors, from feces that attract mosquitoes (Cooperband et al., 2008) and biting midges (Fernandes-Rios et al., 2020), to bird feathers and skin that attract mosquitoes (Allan et al., 2006) and blackflies (Lowther and Wood, 1964; Weinandt et al., 2012). It has been also shown that mosquitoes are attracted to birds (Cornet et al., 2013a,b; Yan et al., 2018), or to odor from birds (Díez-Fernández et al., 2020), infected by avian malaria over non-infected or less infected birds (but see Tomás et al., 2008b; Martínez-de la Puente et al., 2009b; Lalubin et al., 2012 for contrasting results). However, chemical cues from the uropygial secretion apparently are not responsible for this mosquito response (Díez-Fernández et al., 2020).
Only a handful of studies have evaluated the influence of uropygial secretion of birds in host location by blood-feeding flies, and experimental research in field conditions is also scarce. Overall, the influence of uropygial secretion in host location remains controversial. Several studies have found that uropygial secretion attracts mosquitoes (Russell and Hunter, 2005; Garvin et al., 2018b) and blackflies (Fallis and Smith, 1964; Lowther and Wood, 1964; Bennett et al., 1972), either alone or in combination with other stimuli. However, other studies have failed to find any attractant effect (Martínez-de la Puente et al., 2011b; Garvin et al., 2018a; Díez-Fernández et al., 2019, 2020) or have found attraction to the secretion only in certain bird or mosquito species, while not in other bird (Garvin et al., 2018b) or mosquito species (Russell and Hunter, 2005). On the other hand, it has even been suggested that uropygial secretion may confer protection against ectoparasites such as lice (Moyer et al., 2003). Our results would suggest that uropygial secretion and derived odors may protect hoopoes from blood-feeding flies and the pathogens they vector by having a repellent or masking effect impairing host detection. Likewise, further evidence suggesting that uropygial secretions may act as repellent of blood-feeding flies comes from the finding that house sparrows with larger uropygial glands are less infected by a vector-borne malarial parasite (Magallanes et al., 2016). However, this relationship may be driven by overlooked third variables, because for instance gland size is positively correlated with body condition (Magallanes et al., 2016; Moreno-Rueda, 2017).
Evidence of chemical deterrents of ectoparasites in birds is reduced to a handful of bird species, and it does not include uropygial secretions but compounds produced in the skin or feathers (reviewed by Dumbacher and Pruett-Jones, 1996; Moyer and Clayton, 2004; Rajchard, 2007, 2010; Caro et al., 2015). Crested auklets (Aethia cristatella) emit a characteristic citrusy odor, which is associated from specialized wick feathers in the interscapular region. These chemical compounds are effective in repelling or killing lice, ticks and mosquitoes (Douglas et al., 2004, 2005a; Douglas, 2013; but see Douglas et al., 2005b). Similarly, the feathers and skin of birds of the genus Pitohui and Ifrita from New Guinea contain a potent toxin that has been suggested to deter lice (Dumbacher et al., 1992, 2000; Mouritsen and Madsen, 1994; Poulsen, 1994; Dumbacher, 1999). Hoopoe uropygial secretion is special among birds as well. Besides the known adaptive functions of uropygial secretion, our results suggest a role in protection against ectoparasites that deserves further research, also to ascertain to what degree it can be generalized to other bird species.
Data Availability Statement
Data used in this paper are available from the CSIC Institutional Repository at: http://dx.doi.org/10.20350/digitalCSIC/12618.
Author Contributions
GT, CZ-M, and JS conceived and designed the research. GT, CZ-M, MB, and JS conducted fieldwork. GT, CZ-M, MM-V, MB, CR-C, and JS contributed to reagents and materials. GT analyzed the parasite samples. JS and GT analyzed the data. GT and JS wrote the article. All authors contributed to revisions.
Funding
Financial support was provided by grants from the Spanish Ministerio de Economía, Industria y Competitividad (CGL2017-89063-P and CGL2017-83103-P). GT was also supported by the Ramón y Cajal Program. We acknowledge support of the publication fee by the CSIC Open Access Publication Support Initiative through its Unit of Information Resources for Research (URICI).
Conflict of Interest
The authors declare that the research was conducted in the absence of any commercial or financial relationships that could be construed as a potential conflict of interest.
Acknowledgments
The study was conducted according to relevant Spanish national (Decreto 105/2011, 19 de abril) and regional guidelines. All necessary permits were provided by Consejería de Medio Ambiente de la Junta de Andalucía, Spain (Ref: SGYB/FOA/AFR/CFS and SGMN/GyB/JMIF). This research benefited from facilities provided by the city hall of Guadix. We thank Francisco Valera and Jesús Veiga for advice on CO2 supply, and Belinda Nzo for kindly sewing collector bags, for insect traps. We appreciate the constructive comments of the two reviewers.
References
Adler, P. H., Currie, D. C., and Wood, D. M. (2004). The Black Flies (Simuliidae) of North America. Ithaca, NY: Cornell University Press.
Adler, P. H., and McCreadie, J. W. (2002). “Black flies (Simuliidae),” in Medical and Veterinary Entomology, eds G. Mullen, and L. Durden, (San Diego, CA: Academic Press), 185–202. doi: 10.1016/b978-012510451-7/50013-x
Allan, S. A., Bernier, U. R., and Kline, D. L. (2006). Laboratory evaluation of avian odors for mosquito (Diptera: Culicidae) attraction. J. Med. Entomol. 43, 225–231. doi: 10.1093/jmedent/43.2.225
Allan, S. A., Day, J. F., and Edman, J. D. (1987). Visual ecology of biting flies. Annu. Rev. Entomol. 32, 297–316. doi: 10.1146/annurev.en.32.010187.001501
Amo, L., Avilés, J. M., Parejo, D., Peña, A., Rodríguez, J., and Tomás, G. (2012). Sex recognition by odour and variation in the uropygial gland secretion in starlings. J. Anim. Ecol. 81, 605–613. doi: 10.1111/j.1365-2656.2011.01940.x
Baldwin, W. F., West, A. S., and Gomery, J. (1975). Dispersal patterns of black flies (Diptera: Simuliidae) tagged with 32P. Can. Entomol. 107, 113–118. doi: 10.4039/ent107113-2
Bartlett-Healy, K., Crans, W., and Gaugler, R. (2008). Phonotaxis to amphibian vocalizations in Culex territans (Diptera: Culicidae). Ann. Entomol. Soc. Am. 101, 95–103. doi: 10.1603/0013-8746(2008)101[95:ptavic]2.0.co;2
Becker, N., Petric, D., Zgomba, M., Boase, C., Dahl, C., Madon, M., et al. (2010). Mosquitoes and Their Control, 2nd Edn, Berlin: Springer-Verlag.
Bennett, G. F., Fallis, A. M., and Campbell, A. G. (1972). The response of Simulium (Eusimulium) Euryadminiculum (Davies) (Diptera: Simuliidae) to some olfactory and visual stimuli. Can. J. Zool. 50, 793–800. doi: 10.1139/z72-108
Bidlingmayer, W. L. (1967). A comparison of trapping methods for adult mosquitoes: species response and environmental influence. J. Med. Entomol. 4, 200–220. doi: 10.1093/jmedent/4.2.200
Borkent, A. (2005). “The biting midges, the Ceratopogonidae (Diptera),” in Biology of Disease Vectors, 2nd Edn, ed. W. Marquardt, (Burlington, MA: Elsevier), 113–126.
Borkent, A. (2008). The frog-biting midges of the world (Corethrellidae: Diptera). Zootaxa 1804:456.
Bukaciński, D., and Bukacińska, M. (2000). The impact of mass outbreaks of black flies (Simuliidae) on the parental behaviour and breeding output of colonial common gulls (Larus canus). Ann. Zool. Fennici 37, 43–49.
Camp, J. V. (2006). Host Attraction and Host Selection in the Family Corethrellidae (Wood and Borkent) (Diptera). Statesboro, GA: Georgia Southern University.
Campos-Cerda, F., and Bohannan, B. J. M. (2020). The nidobiome: a framework for understanding microbiome assembly in neonates. Trends Ecol. Evol. 35, 573–582. doi: 10.1016/j.tree.2020.03.007
Cardé, R. T. (2015). Multi-cue integration: how female mosquitoes locate a human host. Curr. Biol. 25, R793–R795.
Caro, S. P., Balthazart, J., and Bonadonna, F. (2015). The perfume of reproduction in birds: chemosignaling in avian social life. Horm. Behav. 68, 25–42. doi: 10.1016/j.yhbeh.2014.06.001
Castaño-Vázquez, F., Merino, S., Cuezva, S., and Sánchez-Moral, S. (2020). Nest gasses as a potential attraction cue for biting flying insects and other ectoparasites of cavity nesting birds. Front. Ecol. Evol. 8:258. doi: 10.3389/fevo.2020.00258
Chandrasegaran, K., Lahondère, C., Escobar, L. E., and Vinauger, C. (2020). Linking mosquito ecology, traits, behavior, and disease transmission. Trends Parasitol. 36, 393–403. doi: 10.1016/j.pt.2020.02.001
Clayton, D. H., and Moore, J. (1997). Host-Parasite Evolution: General Principles and Avian Models. Oxford: Oxford University Press.
Combes, C. (2001). Parasitism: The Ecology and Evolution of Intimate Interactions. Chicago: University of Chicago Press.
Cooperband, M. F., McElfresh, J. S., Millar, J. G., and Cardé, R. T. (2008). Attraction of female Culex quinquefasciatus Say (Diptera: Culicidae) to odors from chicken feces. J. Insect Physiol. 54, 1184–1192. doi: 10.1016/j.jinsphys.2008.05.003
Cornet, S., Nicot, A., Rivero, A., and Gandon, S. (2013a). Both infected and uninfected mosquitoes are attracted toward malaria infected birds. Malar. J. 12, 1–8.
Cornet, S., Nicot, A., Rivero, A., and Gandon, S. (2013b). Malaria infection increases bird attractiveness to uninfected mosquitoes. Ecol. Lett. 16, 323–329. doi: 10.1111/ele.12041
Díaz-Lora, S., Martín-Vivaldi, M., Juárez García-Pelayo, N., Azcárate García, M., Rodríguez-Ruano, S. M., Martínez-Bueno, M., et al. (2019). Experimental old nest material predicts hoopoe Upupa epops eggshell and uropygial gland microbiota. J. Avian Biol. 50:jav.02083. doi: 10.1111/jav.02083
Díaz-Lora, S., Pérez-Contreras, T., Azcárate-García, M., Martínez-Bueno, M., Soler, J. J., and Martín-Vivaldi, M. (2020). Hoopoe Upupa epops male feeding effort is related to female cosmetic egg colouration. J. Avian Biol. 51:jav.02433. doi: 10.1111/jav.02433
Díez-Fernández, A., Martínez-de la Puente, J., Gangoso, L., Ferraguti, M., Soriguer, R., and Figuerola, J. (2019). House sparrow uropygial gland secretions do not attract ornithophilic nor mammophilic mosquitoes. Med. Vet. Entomol. 34, 225–228. doi: 10.1111/mve.12401
Díez-Fernández, A., Martínez-de la Puente, J., Gangoso, L., López, P., Soriguer, R., Martín, J., et al. (2020). Mosquitoes are attracted by the odour of Plasmodium-infected birds. Intern. J. Parasitol. 50, 569–575. doi: 10.1016/j.ijpara.2020.03.013
Douglas, H. D. (2013). Colonial seabird’s paralytic perfume slows lice down: an opportunity for parasite-mediated selection? Intern. J. Parasitol. 43, 399–407. doi: 10.1016/j.ijpara.2013.01.004
Douglas, H. D., Co, J. E., Conner, W. E., and Jones, T. H. (2004). Interspecific differences in Aethia spp. auklet odorants and evidence for chemical defense against ectoparasites. J. Chem. Ecol. 30, 1921–1935. doi: 10.1023/b:joec.0000045586.59468.de
Douglas, H. D., Co, J. E., Jones, T. H., Conner, W. E., and Day, J. F. (2005a). Chemical odorant of colonial seabird repels mosquitoes. J. Med. Entomol. 42, 647–651. doi: 10.1093/jmedent/42.4.647
Douglas, H. D., Malenke, J. R., and Clayton, D. H. (2005b). Is the citrus-like plumage odorant of crested auklets (Aethia cristatella) a defense against lice? J. Ornithol. 146, 111–115. doi: 10.1007/s10336-004-0065-2
Dumbacher, J. P. (1999). Evolution of toxicity in pitohuis: I. Effects of homobatrachotoxin on chewing lice (order Phthiraptera). Auk 116, 957–963. doi: 10.2307/4089675
Dumbacher, J. P., Beehler, B. M., Spande, T. F., Garraffo, H. M., and Daly, J. W. (1992). Homobatrachotoxin in the genus Pitohui: chemical defense in birds? Science 258, 799–801. doi: 10.1126/science.1439786
Dumbacher, J. P., and Pruett-Jones, S. (1996). Avian chemical defense. Curr. Ornithol. 13, 137–174. doi: 10.1007/978-1-4615-5881-1_4
Dumbacher, J. P., Spande, T. F., and Anddaly, J. W. (2000). Batrachotoxin alkaloids from passerine birds: a second toxic bird genus (Ifrita kowaldi) from New Guinea. Proc. Natl. Acad. Sci. U.S.A. 97, 12970–12975. doi: 10.1073/pnas.200346897
Fallis, A. M., and Smith, S. M. (1964). Ether extracts from birds and CO2 as attractants for some ornithophilic simuliids. Can. J. Zool. 42, 723–730. doi: 10.1139/z64-069
Farris, H. E., Oshinsky, M. L., Forrest, T. G., and Hoy, R. R. (2009). Auditory sensitivity of an acoustic parasitoid (Emblemasoma sp., Sarcophagidae, Diptera) and the calling behavior of potential hosts. Brain Behav. Evol. 72, 16–26. doi: 10.1159/000139458
Fernandes-Rios, A., Chagas-Costa, J. P., Marinho-de Brito, A. V., Dos Santos-Coelho, T., Abreu-Bandeira, M. D. C., and Macário-Rebêlo, J. M. (2020). Effect of vertebrate feces on the attraction of Culicoides (Diptera: Ceratopogonidae) in an Amazonian mangrove. J. Vector Ecol. 45, 127–134. doi: 10.1111/jvec.12380
Ferraguti, M., Martínez-de la Puente, J., Bensch, S., Roiz, D., Ruiz, S., Viana, D. S., et al. (2018). Ecological determinants of avian malaria infections: an integrative analysis at landscape, mosquito and vertebrate community levels. J. Anim. Ecol. 87, 727–740. doi: 10.1111/1365-2656.12805
Foster, W. A., and Walker, E. D. (2002). “Mosquitoes (Culicidae),” in Medical and Veterinary Entomology, eds G. Mullen and L. Durden, (San Diego, CA: Academic Press), 203–262.
Garvin, M. C., Austin, A., Boyer, K., Gefke, M., Wright, C., Pryor, Y., et al. (2018a). Attraction of Culex pipiens to house sparrows is influenced by host age but not uropygial gland secretions. Insects 9:127. doi: 10.3390/insects9040127
Garvin, M. C., Austin, A. L., Stracker, N. H., Slowinski, S. P., Rutter, J. E., Butler, M., et al. (2018b). Attraction of Culex pipiens to uropygial gland secretions does not explain feeding preference for American robins. J. Vector Ecol. 43, 110–116. doi: 10.1111/jvec.12290
Garvin, M. C., and Greiner, E. C. (2003). Ecology of Culicoides (Diptera: Ceratopogonidae) in southcentral Florida and experimental Culicoides vectors of the avian hematozoan Haemoproteus danilewskyi Kruse. J. Wildl. Dis. 39, 170–178. doi: 10.7589/0090-3558-39.1.170
Gerry, A. C., Sarto I Monteys, V., Moreno-Vidal, J. O., Francino, O., and Mullens, B. A. (2009). Biting rates of Culicoides midges (Diptera: Ceratopogonidae) on sheep in northeastern Spain in relation to midge capture using UV light and carbon dioxide-baited traps. J. Med. Entomol. 46, 615–624. doi: 10.1603/033.046.0329
Gibson, G., and Torr, S. J. (1999). Visual and olfactory responses of haematophagous Diptera to host stimuli. Med. Vet. Entomol. 13, 2–23. doi: 10.1046/j.1365-2915.1999.00163.x
Greppi, C., Laursen, W. J., Budelli, G., Chang, E. C., Daniels, A. M., van Giesen, L., et al. (2020). Mosquito heat seeking is driven by an ancestral cooling receptor. Science 367, 681–684. doi: 10.1126/science.aay9847
Hunter, D. B., Rohner, C., and Currie, D. C. (1997). Mortality in fledgling great horned owls from black fly hematophaga and leucocytozoonosis. J. Wildl. Dis. 33, 486–491. doi: 10.7589/0090-3558-33.3.486
Jacob, J., and Ziswiler, V. (1982). “The uropygial gland,” in Avian Biology, Vol. VII, eds D. S. Famer, J. R. King, and K. C. Parker, (New York, NY: Academic Press), 359–362.
Lalubin, F., Bize, P., van Rooyen, J., Christe, P., and Glaizot, O. (2012). Potential evidence of parasite avoidance in an avian malarial vector. Anim. Behav. 84, 539–545. doi: 10.1016/j.anbehav.2012.06.004
Lazzari, C. R. (2019). The thermal sense of blood-sucking insects: why physics matters. Curr. Opin. Insect Sci. 34, 112–116. doi: 10.1016/j.cois.2019.05.006
Lehane, M. J. (2005). The Evolution of Blood-Sucking in Insects. Cambridge: Cambridge University Press.
Lehmann, G. U. C. (2003). Review of biogeography, host range and evolution of acoustic hunting in Ormiini (Insecta, Diptera, Tachinidae), parasitoids of night-calling bushcrickets and crickets (Insecta, Orthoptera, Ensifera). Zool. Anzeig. 242, 107–120. doi: 10.1078/0044-5231-00091
López-Rull, I., and Macías-García, C. (2015). “Control of invertebrate occupants of nests,” in Nests, Eggs, and Incubation: New Ideas About Avian Reproduction, eds D. C. Deeming and S. J. Reynolds, (Oxford: Oxford University Press), 82–96. doi: 10.1093/acprof:oso/9780198718666.003.0008
Lowther, J. K., and Wood, D. M. (1964). Specificity of a black fly, Simulium euryadminiculum Davies, toward its host, the common loon. Can. Entomol. 96, 911–913. doi: 10.4039/ent96911-6
Loye, J. E., and Zuk, M. (1991). Bird-Parasite Interactions. Ecology, Evolution and Behaviour. Oxford: Oxford University Press.
Magallanes, S., Møller, A. P., García-Longoria, L., de Lope, F., and Marzal, A. (2016). Volume and antimicrobial activity of secretions of the uropygial gland are correlated with malaria infection in house sparrows. Parasit. Vec. 9:232.
Malmqvist, B., Adler, P. H., Kuusela, K., Merritt, R. W., and Wotton, R. S. (2004). Black flies in the boreal biome, key organisms in both terrestrial and aquatic enviroments: a review. Ecoscience 11, 187–200. doi: 10.1080/11956860.2004.11682824
Martin, F. R., McCreadie, J. W., and Colbo, M. H. (1994). Effect of trap site, time of day, and meteorological factors on abundance of host-seeking mammalophilic black flies (Diptera: Simuliidae). Can. Entomol. 126, 283–289.
Martínez-de la Puente, J., Martínez, J., Rivero-de Aguilar, J., Herrero, J., and Merino, S. (2011a). On the specificity of avian blood parasites: revealing specific and generalist relationships between haemosporidians and biting midges. Mol. Ecol. 20, 3275–3287. doi: 10.1111/j.1365-294x.2011.05136.x
Martínez-de la Puente, J., Rivero-de Aguilar, J., del Cerro, S., Argüello, A., and Merino, S. (2011b). Do secretions from the uropygial gland of birds attract biting midges and black flies? Parasitol. Res. 109, 1715–1718. doi: 10.1007/s00436-011-2436-y
Martínez-de la Puente, J., Merino, S., Lobato, E., Rivero-de Aguilar, J., del Cerro, S., Ruiz-de Castañeda, R., et al. (2009a). Does weather affect biting fly abundance in avian nests? J. Avian Biol. 40, 653–657. doi: 10.1111/j.1600-048x.2009.04726.x
Martínez-de la Puente, J., Merino, S., Tomás, G., Moreno, J., Morales, J., Lobato, E., et al. (2009b). Factors affecting Culicoides species composition and abundance in avian nests. Parasitology 136, 1033–1041. doi: 10.1017/s0031182009006374
Martínez-García, Á, Martín-Vivaldi, M., Rodríguez-Ruano, S. M., Peralta-Sánchez, J. M., Valdivia, E., and Soler, J. J. (2016). Nest bacterial environment affects microbiome of hoopoe eggshells, but not that of the uropygial secretion. PLoS One 11:e0158158. doi: 10.1371/journal.pone.0158158
Martínez-García, Á, Soler, J. J., Rodríguez-Ruano, S. M., Martínez-Bueno, M., Martín-Platero, A. M., Juárez-García, N., et al. (2015). Preening as a vehicle for key bacteria in hoopoes. Microb. Ecol. 70, 1024–1033. doi: 10.1007/s00248-015-0636-1
Martín-Vivaldi, M., Doña, J., Romero Masegosa, J., and Soto Cárdenas, M. (2016). “Abubilla - Upupa epops,” in Enciclopedia Virtual de los Vertebrados Españoles, eds A. Salvador and M. B. Morales, (Madrid: Museo Nacional de Ciencias Naturales).
Martín-Vivaldi, M., Palomino, J. J., Soler, M., and Soler, J. J. (1999). Determinants of reproductive success in the hoopoe (Upupa epops), a hole-nesting non-passerine bird with asynchronous hatching. Bird Study 46, 205–216. doi: 10.1080/00063659909461132
Martín-Vivaldi, M., Peña, A., Peralta-Sánchez, J. M., Sánchez, L., Ananou, S., Ruiz-Rodríguez, M., et al. (2010). Antimicrobial chemicals in hoopoe preen secretions are produced by symbiotic bacteria. Proc. R. Soc. B 277, 123–130. doi: 10.1098/rspb.2009.1377
Martín-Vivaldi, M., Ruiz-Rodríguez, M., Soler, J. J., Peralta-Sánchez, J. M., Méndez, M., Valdivia, E., et al. (2009). Seasonal, sexual and developmental differences in hoopoe Upupa epops preen gland morphology and secretions: evidence for a role of bacteria. J. Avian Biol. 40, 191–205. doi: 10.1111/j.1600-048x.2009.04393.x
Martín-Vivaldi, M., Soler, J. J., Martínez-García, Á, Arco, L., Juárez-García-Pelayo, N., Ruiz-Rodríguez, M., et al. (2018). Acquisition of uropygial gland microbiome by hoopoe nestlings. Microb. Ecol. 76, 285–297. doi: 10.1007/s00248-017-1125-5
Martín-Vivaldi, M., Soler, J. J., Peralta-Sánchez, J. M., Arco, L., Martín-Platero, A. M., Martínez-Bueno, M., et al. (2014). Special structures of hoopoe eggshells enhance the adhesion of symbiont-carrying uropygial secretion that increase hatching success. J. Anim. Ecol. 83, 1289–1301. doi: 10.1111/1365-2656.12243
Moreno-Rueda, G. (2017). Preen oil and bird fitness: a critical review of the evidence. Biol. Rev. 92, 2131–2143. doi: 10.1111/brv.12324
Mouritsen, K. N., and Madsen, J. (1994). Toxic birds: defence against parasites? Oikos 69, 357–358. doi: 10.2307/3546161
Moyer, B. R., and Clayton, D. H. (2004). “Avian defences against ectoparasites,” in Insect and Bird Interactions, eds H. F. van Emdenand and M. Rothschild, (Andover: Intercept), 243–259. doi: 10.1002/9783527802883.ch12
Moyer, B. R., Drown, D. M., and Clayton, D. H. (2002). Low humidity reduces ectoparasite pressure: implications for host life history evolution. Oikos 97, 223–228. doi: 10.1034/j.1600-0706.2002.970208.x
Moyer, B. R., Rock, A. N., and Clayton, D. H. (2003). Experimental test of the importance of preen oil in rock doves (Columba livia). Auk 120, 490–496. doi: 10.1642/0004-8038(2003)120[0490:etotio]2.0.co;2
Mullen, G. R. (2002). “Biting midges (Ceratopogonidae),” in Medical and Veterinary Entomology, eds G. Mullen and L. Durden, (San Diego, CA: Academic Press), 163–183. doi: 10.1016/b978-012510451-7/50012-8
Mullen, G. R., and Durden, L. A. (2002). Medical and Veterinary Entomology. San Diego, CA: Academic Press.
Mullens, B. A., and Gerry, A. C. (1998). Comparison of bait cattle and carbon dioxide-baited suction traps for collecting Culicoides variipennis sonorensis (Diptera: Ceratopogonidae) and Culex quinquefasciatus (Diptera: Culicidae). J. Med. Entomol. 35, 245–250. doi: 10.1093/jmedent/35.3.245
Naugle, D. E., Aldridge, C. L., Walker, B. L., Cornish, T. E., Moynahan, B. J., Holloran, M. J., et al. (2004). West Nile virus: pending crisis for greater sage-grouse. Ecol. Lett. 7, 704–713. doi: 10.1111/j.1461-0248.2004.00631.x
Nourani, L., Zakeri, S., and Djadid, N. D. (2020). Dynamics of prevalence and distribution pattern of avian Plasmodium species and its vectors in diverse zoogeographical areas-A review. Infect. Genet. Evol. 81:104244. doi: 10.1016/j.meegid.2020.104244
Poulsen, B. O. (1994). Poison in Pitohui birds: against predators or ectoparasites? EMU 94, 128–129. doi: 10.1071/mu9940128
Rajchard, J. (2007). Intraspecific and interspecific chemosignals in birds: a review. Vet. Med. 52, 385–391. doi: 10.17221/2000-vetmed
Rajchard, J. (2010). Biologically active substances of bird skin: a review. Vet. Med. 55, 413–421. doi: 10.17221/2981-vetmed
Rodríguez-Ruano, S. M., Martín-Vivaldi, M., Martín-Platero, A. M., López-López, J. P., Peralta-Sánchez, J. M., Ruiz-Rodríguez, M., et al. (2015). The Hoopoe’s uropygial gland hosts a bacterial community influenced by the living conditions of the bird. PLoS One 10:e0139734. doi: 10.1371/journal.pone.0139734
Rodríguez-Ruano, S. M., Martín-Vivaldi, M., Peralta-Sánchez, J. M., García-Martín, A. B., Martínez-García, Á, Soler, J. J., et al. (2018). Seasonal and sexual differences in the microbiota of the hoopoe uropygial secretion. Genes 9, 1–18.
Ruiz-Rodríguez, M., Soler, J. J., Martín-Vivaldi, M., Martín-Platero, A. M., Méndez, M., Peralta-Sánchez, J. M., et al. (2014). Environmental factors shape the community of symbionts in the hoopoe uropygial gland more than genetic factors. Appl. Environ. Microbiol. 80, 6714–6723. doi: 10.1128/aem.02242-14
Ruiz-Rodríguez, M., Valdivia, E., Martín-Vivaldi, M., Martín-Platero, A. M., Martínez-Bueno, M., Méndez, M., et al. (2012). Antimicrobial activity and genetic profile of Enterococci isolated from hoopoes uropygial gland. PLoS One 7:e41843. doi: 10.1371/journal.pone.0041843
Russell, C. B., and Hunter, F. F. (2005). Attraction of Culex pipiens/restuans (Diptera: Culicidae) mosquitoes to bird uropygial gland odors at two elevations in the Niagara region of Ontario. J. Med. Entomol 42, 301–305. doi: 10.1603/0022-2585(2005)042[0301:aocrdc]2.0.co;2
Schmid-Hempel, P. (2011). Evolutionary Parasitology: The Integrated Study of Infections, Immunology, Ecology and Genetics. Oxford: Oxford University Press.
Service, M. W. (1980). Effects of wind on the behaviour and distribution of mosquitoes and blackflies. Int. J. Biometeorol. 24, 347–353. doi: 10.1007/bf02250577
Silver, J. B. (2008b). “Sampling adults with visual attraction traps, sound traps and other miscellaneous attraction traps,” in Mosquito Ecology: Field Sampling Methods, ed. J. B. Silver, (Dordrecht: Springer), 1027–1048.
Smith, R. N., Cain, S. L., Anderson, S. H., Dunk, J. R., and Williams, E. S. (1998). Blackfly-induced mortality of nestling red-tailed hawks. Auk 115, 368–375.
Soler, J. J., Martínez-García, Á, Rodríguez-Ruano, S. M., Martínez-Bueno, M., Martín-Platero, A. M., Peralta-Sánchez, J. M., et al. (2016). Nestedness of hoopoes’ bacterial communities: symbionts from the uropygial gland to the eggshell. Biol. J. Linnea. Soc. 118, 763–773. doi: 10.1111/bij.12772
Soler, J. J., Martín-Vivaldi, M., Peralta-Sánchez, J. M., Arco, L., and Juárez-García-Pelayo, N. (2014). Hoopoes color their eggs with antimicrobial uropygial secretions. Naturwissenschaften 101, 697–705. doi: 10.1007/s00114-014-1201-3
Soler, J. J., Martín-Vivaldi, M., Peralta-Sánchez, J. M., and Ruiz-Rodríguez, M. (2010). Antibiotic-producing bacteria as a possible defence of birds against pathogenic microorganisms. Open Ornithol. J. 3, 93–100. doi: 10.2174/1874453201003010093
Soler, J. J., Martín-Vivaldi, M., Ruiz-Rodríguez, M., Valdivia, E., Martín-Platero, A. M., Martínez-Bueno, M., et al. (2008). Symbiotic association between hoopoes and antibiotic-producing bacteria that live in their uropygial gland. Funct. Ecol. 22, 864–871. doi: 10.1111/j.1365-2435.2008.01448.x
Solheim, R., Jacobsen, K.-O., Øien, I. J., Aarvak, T., and Polojärvi, P. (2013). Snowy Owl nest failures caused by blackfly attacks on incubating females. Ornis Norveg. 36, 1–5. doi: 10.15845/on.v36i0.394
StatSoft Inc, (2007). Statistica (Data Analysis Software System), Ver. 12. Available online at: https://www.statsoft.de/en/software (accessed September 20, 2020).
Sutcliffe, J. F. (1986). Black fly host location: a review. Can. J. Zool. 64, 1041–1053. doi: 10.1139/z86-156
Sutcliffe, J. F. (2010). “Black fly interactions with their hosts,” in Olfaction in Vector-Host Interactions. Ecology and Control of Vector-Borne Diseases, Vol. 2, eds W. Takken, and B. G. J. Knols, (Wageningen: Wageningen Academic Publishers), 247–264.
Syed, Z., and Leal, W. S. (2009). Acute olfactory response of Culex mosquitoes to a human- and bird-derived attractant. Proc. Natl. Acad. Sci. U.S.A. 106, 18803–18808.
Takken, W., and Knols, B. G. J. (2010). Olfaction in Vector-Host Interactions: Ecology and Control of Vector-Borne Diseases. Wageningen: Wageningen Academic Publishers.
Takken, W., and Verhulst, N. O. (2013). Host preferences of blood-feeding mosquitoes. Annu. Rev. Entomol. 58, 433–453.
Toma, T., Takara, T., Miyagi, I., Futami, K., and Higa, Y. (2019). Mosquitoes and frog-biting midges (Diptera: Culicidae and Corethrellidae) attracted to traps with natural frog calls and synthesized sounds at Iriomote Island, Ryukyu Archipelago, Japan. Med. Entomol. Zool. 70, 221–234.
Tomás, G., Merino, S., Martínez-de la Puente, J., Moreno, J., Morales, J., and Lobato, E. (2008a). A simple trapping method to estimate abundances of blood-sucking flying insects in avian nests. Anim. Behav. 75, 723–729.
Tomás, G., Merino, S., Martínez-de la Puente, J., Moreno, J., Morales, J., and Lobato, E. (2008b). Determinants of abundance and effects of blood-sucking flying insects in the nest of a hole-nesting bird. Oecologia 156, 305–312.
Tomás, G., Merino, S., Martínez-de la Puente, J., Moreno, J., Morales, J., Lobato, E., et al. (2012). Interacting effects of aromatic plants and female age on nest-dwelling ectoparasites and blood-sucking flies in avian nests. Behav. Process. 90, 246–253.
van Hoesel, W., Marzal, A., Magallanes, S., Santiago-Alarcón, D., Ibáñez-Bernal, S., and Renner, S. C. (2019). Management of ecosystems alters vector dynamics and haemosporidian infections. Sci. Rep. 9:11.
Veiga, J., Martínez-de la Puente, J., Vaclav, R., Figuerola, J., and Valera, F. (2018). Culicoides paolae and C. circumscriptus as potential vectors of avian haemosporidians in an arid ecosystem. Parasit. Vect. 11:524.
Veiga, J., Václav, R., and Valera, F. (2020). The effect of parasite density on host colonisation success by a mobile avian ectoparasite. Ecol. Entomol. 45, 867–875. doi: 10.1111/een.12864
Webster, B., and Cardé, R. T. (2017). Use of habitat odour by host-seeking insects. Biol. Rev. 92, 1241–1249.
Weinandt, M. L., Meyer, M., Strand, M., and Lindsay, A. R. (2012). Cues used by the black fly, Simulium annulus, for attraction to the common loon (Gavia immer). J. Vector Ecol. 37, 359–364.
Whittaker, D. J., Richmond, K. M., Miller, A. K., Kiley, R., Burns, C. B., Atwell, J. W., et al. (2011). Intraspecific preen oil odor preferences in dark-eyed juncos (Junco hyemalis). Behav. Ecol. 22, 1256–1263.
Keywords: begging, Ceratopogonidae, Culicidae, ectoparasite repellent, Enterococcus faecalis, host location mechanisms, Simuliidae, uropygial gland
Citation: Tomás G, Zamora-Muñoz C, Martín-Vivaldi M, Barón MD, Ruiz-Castellano C and Soler JJ (2020) Effects of Chemical and Auditory Cues of Hoopoes (Upupa epops) in Repellence and Attraction of Blood-Feeding Flies. Front. Ecol. Evol. 8:579667. doi: 10.3389/fevo.2020.579667
Received: 03 July 2020; Accepted: 14 September 2020;
Published: 30 September 2020.
Edited by:
Jenny C. Dunn, University of Lincoln, United KingdomReviewed by:
William Jones, Uppsala University, SwedenAlejandro Cantarero, University of Turku, Finland
Copyright © 2020 Tomás, Zamora-Muñoz, Martín-Vivaldi, Barón, Ruiz-Castellano and Soler. This is an open-access article distributed under the terms of the Creative Commons Attribution License (CC BY). The use, distribution or reproduction in other forums is permitted, provided the original author(s) and the copyright owner(s) are credited and that the original publication in this journal is cited, in accordance with accepted academic practice. No use, distribution or reproduction is permitted which does not comply with these terms.
*Correspondence: Gustavo Tomás, Z3RvbWFzQGVlemEuY3NpYy5lcw==