- 1Department of Biology, University of Western Ontario, London, ON, Canada
- 2Environment and Climate Change Canada, Saskatoon, SK, Canada
- 3Facultad de Ciencias Naturales, Universidad Autónoma de Querétaro, Santiago de Querétaro, Mexico
- 4Facultad de Medicina Veterinaria y Zootecnia, Universidad Autónoma de Nuevo León, Nuevo León, Mexico
- 5Fondo Para la Conservación de la Mariposa Monarca, Zitácuaro, Mexico
Monarch butterflies (Danaus plexippus) fuel their migration and overwinter energy needs through accumulated fat stores derived from plant nectars. Determining origins of these fuels is crucial to effective conservation programs. We used stable-hydrogen (δ2H) and carbon (δ13C) isotope measurements in stored lipids of monarchs raised under laboratory conditions as a proof of principle for the isotopic spatial sourcing of stored lipids. We then applied this approach to wild specimens collected from 2015 to 2018 to infer spatial information on nectaring by fall migrants through northeast Mexico and at the Mexican overwinter sites. Migrating monarchs derived from wide geographic natal origins but lipid δ2H values from migratory cohorts were not related to natal origin. Instead, migrants exploited isotopically similar nectar sources. Distributions of lipid δ2H values in overwintering monarchs were broader and more negative by ∼40‰ suggesting more transport of lipids from higher latitudes or additional nectaring while migrating at higher elevations though northeastern to central Mexico. Our work establishes a new isotopic technique for tracking origins of stored lipids in monarchs and other migratory animals and emphasizes the importance of nectar availability in the southern portion of the range, and especially the nectar corridor through central Mexico.
Introduction
Migrant species have evolved complex physiological and behavioral mechanisms that facilitate long-distance movement, including the ability to efficiently convert dietary macromolecules to energy for immediate use or stored as lipid, an energy dense fuel (McWilliams et al., 2004). Understanding the nutritional ecology of migrant animals, especially those using stopover sites to obtain resources to fuel the next leg of their journey is crucial for the development of effective strategies for the management of migrant animal populations (Moore et al., 1993; Lennox et al., 2016).
The eastern North American population of the monarch butterfly (Danaus plexippus) is an iconic example of insect long-distance migration where millions of adults migrate from their natal sites in the United States and Canada to the overwintering sites in the high-altitude oyamel fir (Abies religiosa) forests of central Mexico. This journey is fueled by nectar (Brown and Chippendale, 1974; Brower et al., 2006) obtained at sites along the migratory pathway (Beall, 1948; Gibo and McCurdy, 1993; Brower et al., 2006). Lipid stores generally increase as the butterflies approach the wintering grounds (Brower et al., 2006). Because they rarely feed on flowers during the 4–5 month overwintering period, they must sustain themselves on lipid stores acquired prior to arrival at the winter roost sites, which provide a thermal environment conducive to lipid conservation (Alonso-Mejia et al., 1997; Williams and Brower, 2015). It was proposed that resources acquired primarily in Texas provides the bulk of the lipids required for both overwintering and subsequent reproduction in spring (Brower, 1985). However, in 2011–2012, fall monarchs in Texas had lower than average lipid reserves, due to drought conditions, yet those at the overwintering sites apparently had normal levels (reviewed by Brower et al., 2015). This difference in lipid levels would suggest that, at least in some years, monarchs actively nectar along the floral corridor in Mexico leading to and perhaps including their overwintering sites. To date, the real importance of nectaring sites along the migratory journey and especially in Mexico remains anecdotal despite their likely importance (Saunders et al., 2019).
Understanding where monarchs obtain resources to fuel both migratory flight and overwintering stores would provide new insights into the migratory strategy of this species and subsequently to overall conservation efforts (Brower et al., 2006). More and more attention is being directed to conditions experienced by migrating monarchs during the fall migration with respect to overall population demographics and conservation (Saunders et al., 2019; Taylor et al., 2019). If there is an intrinsic marker where lipids were synthesized, it could be used, retrospectively, to infer locations of key fueling sites. One approach to investigate this possibility is the use of naturally occurring stable isotopes of several elements (Hobson and Wassenaar, 2019) as stable isotope values in foodwebs can vary predictably across large spatial gradients due to a variety of biogeochemical processes and that these spatial patterns are reflected in consumers. The first studies to use stable-hydrogen isotope measurements (δ2H) to infer natal origin of any migrant species were carried out using wing chitin, a tissue that is metabolically inert following synthesis, performed on monarchs at their roost sites in central Mexico (Wassenaar and Hobson, 1998; Hobson et al., 1999). Since then, continental patterns, or isoscapes, of both precipitation δ2H and milkweed δ13C have been used to study patterns of monarch movements in North America (Miller et al., 2012; Flockhart et al., 2013, 2017; Altizer et al., 2015).
We speculate that despite the numerous metabolic pathways involved in lipid synthesis, the δ13C and δ2H values of stored neutral lipids could provide valuable provenance information of monarchs as lipids are composed primarily of carbon and hydrogen derived from plant nectars. This composition is particularly true of palmitic and oleic acids which are the most abundant stored lipids in monarchs (Cenedella, 1971; Brower et al., 2006), While a North American plant leaf water δ2H isoscape has been developed that shows a close concordance with patterns of amount-weighted precipitation δ2H (West et al., 2008, 2010), to our knowledge, no plant δ2H carbohydrate isoscapes exist. Foodwebs driven by plant primary production show well-established continental gradients in δ2H and so we expect plant nectars and the lipids synthesized from them by monarchs to follow the continent wide δ2H precipitation isoscape as demonstrated for chitins and keratins of numerous migratory taxa (Hobson and Wassenaar, 2019).
Our approach was to first demonstrate that stable isotope measurements could be used to trace nutritional origins of stored lipids and then to apply this proof of concept to wild migrating and overwintering monarchs in Mexico. We measured δ2H and δ13C in (i) lipids from individual monarchs raised in the laboratory on nectar sources comprised of isotopically known carbohydrate and water values, and (ii) wing and lipid δ2H of monarchs collected at different sites along the migratory pathway in northeastern to central Mexico and at the Mexican overwinter sites. We postulate that if the isotopic distribution of lipid δ2H values were independent of natal origin (as derived from wing δ2H) but were tightly clustered, this would suggest adults fed at geographically restricted sites that could potentially be identified. We discuss the relevance of our findings to the ultimate goal of tracing geographic origins of lipids in migratory monarchs and other insects and how our approach can have significant implications for conservation.
Materials and Methods
Laboratory Study
Eggs were obtained from adult monarchs collected in July 2019 on the Environmental Science Western Field Station (WFS) (43.07°N, 81.34°W) and reared at 25°C, 65% RH and 16L:8D. The larvae were reared in individual containers and provided pieces of washed fresh, field collected milkweed (Asclepias syriaca) leaves each day. The newly emerged adults were sexed, caged separately to deter mating, and ten individuals (five male and five female) were assigned to one of four isotopically distinct dietary treatments, an approach allowing us to directly trace isotopically contributions from dietary macromolecules to ultimate lipid stores. The isotopic values of the treatment nectars were : (1) high δ13C (C4 cane sugar: mean ± SD, −12.4 ± 0.03‰, n = 5) with high δ2H (spiked) water (182 ± 6.1‰, n = 3), (2) high δ13C with low δ2H (unspiked tap) water (−55 ± 2.0‰, n = 3), (3) low δ13C (C3 beet sugar: −26.4 ± 0/1‰, n = 5) with low δ2H water and (4) low δ13C with high δ2H water (n = 5). Deuterium spiked water was prepared by adding a quantity of 99.9 atom % heavy water (deuterium oxide) (Sigma Aldrich). The nectar, at a 15% concentration (with cane or beet sugar as the nutrient sugar source), was prepared following the methodology developed by Orley Taylor (personal communication) that avoids fermentation. Adults were fed daily ad libitum for 5 days, after which they were sacrificed, the fat body extracted using 2:1 (v:v) mixture of chloroform:methanol. The lipids were recovered following evaporation in a fume hood for > 24 h (23°C, 101.3 kPa), then frozen (−20°C) until stable isotope analyses were conducted.
Field Study
Monarchs were sampled between 2015 and 2018 (Mexican permits SGPA/DGVS/002159/18, SGPA/DGVS/002299/18.SGPA/DGVS/09159/17, SGPA/DGVS/10763/16, SGPA/DGVS/06299/15), at several sites in northeastern to central Mexico along the fall migration route and at overwintering colonies (Figure 1 and Supplementary Table S1). In 2015–2016 overwintering monarchs were collected at Sierra Chincua, El Rosario, and Piedra Herrada colonies. Evidence for isotopic change or discrimination through the period of lipid use when fasting at colonies would complicate interpretation of lipid isotope values based on timing. So, to test this, in 2017–2018 monarch lipids were sampled monthly from December to February at the Cerro Pelon colony. All monarch samples were placed in individual paper envelopes, labeled and then frozen (−20°C) and shipped to Canada for stable isotope analysis.
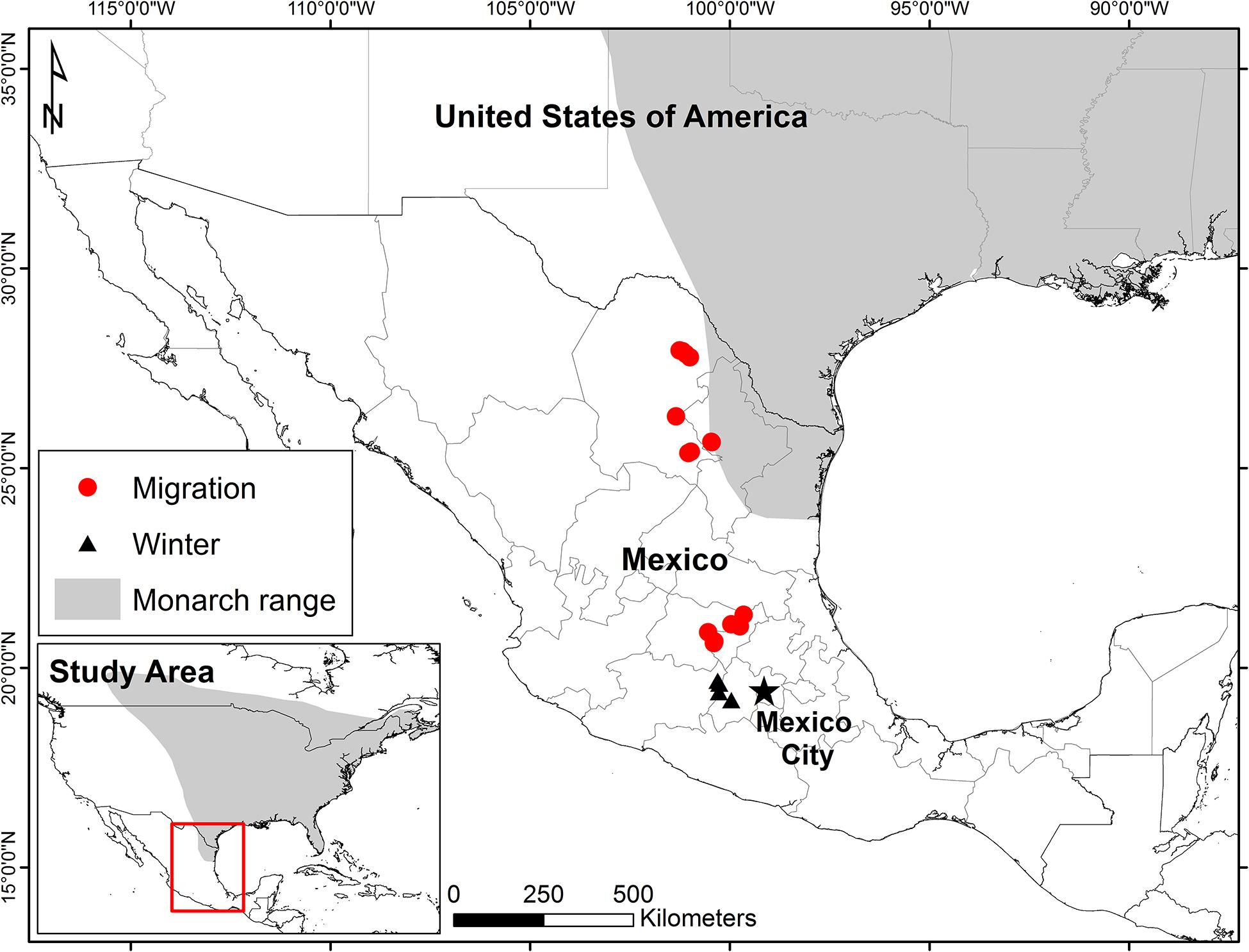
Figure 1. General study area in Northeastern Mexico. Note that the range shown in northeast Mexico includes potential non-migrants.
Stable Isotope Analyses
Samples of 0.35 ± 0.02 mg of wing were weighed into pressed silver 3.5 × 5 mm capsules, and analyzed using a Eurovector Uniprep autosampler (Milan, Italy) carousel attached to a Eurovector 3000 Elemental Analyzer, coupled to a Thermo Delta V Plus isotope ratio mass spectrometer (Bremen, Germany) in continuous flow mode with helium carrier gas. After the samples were loaded, the Uniprep autosampler (heated to 60°C) was vacuum evacuated and subsequently flushed with dry helium twice to remove adsorbed atmospheric moisture from the crushed silver capsules. Two USGS keratin standards, EC-01 (formerly CBS: Caribou Hoof Standard) and EC-02 (KHS: Kudu Horn Standard of Environment Canada) were included every ten samples. Samples were combusted at 1350°C using glassy carbon. Values of δ2H of non-exchangeable hydrogen were derived using the comparative equilibration approach of Wassenaar and Hobson (2003) and calibrated to Vienna Standard Mean Ocean Water (VSMOW) using EC-01 (± 1.9‰ 1 SD, n = 18, accepted δ2H = −197.0‰) and EC-02 (± 1.6‰, n = 17, accepted δ2H = −54.1‰). We used these former calibration standard values because our original assignment algorithms were based on these values (Hobson et al., 1999). This approach results in identical assignments if new calibration standards are used with correspondingly modified assignment algorithms (see below; Soto et al., 2017). Overall (within-run) measurement error for EC-01 and EC-02 δ2H was ∼ 2‰.
Lipid samples were analyzed in an identical fashion to wing samples but were calibrated against the USGS water standards sealed in silver tubes VSMOW2 (0‰) and USGS 46 (−235.8‰) because no international standards for animal lipids are available and we were interested only in relative lipid δ2H values (note that lipids have no exchangeable H). We compared calibration results with several other laboratory standards including the keratins CBS (−157‰) and KHS (−35.3‰) and found consistent offsets. Overall measurement error for these standards relative to the VSMOW scale and based on within-run replicate measurements was ± 3‰. For the laboratory experiment, water δ2H analyses were performed using an Off Axis Integrated Cavity Output Spectroscopy (OA-ICOS) using a Los Gatos Research DLT-100 laser spectrometer (Mountain view California). We used two calibrated reference waters (INV1 δ2H = −217.7‰ and ROD3 δ2H = −3.9‰, respectively) to normalize raw delta values to the VSMOW scale. Precisions as determined by replicate analyses of samples and reference waters were ± 1 and 0.1‰ respectively.
Concurrent δ13C and δ15N analyses were performed using a Costech Elemental Analyzer coupled to a Thermo Delta Plus XL isotope ratio mass spectrometer (Thermo Instruments, Bremen, Germany) operated in continuous flow mode with helium carrier gas, but only δ13C values are reported here. Two standards, USGS-40 and USGS-41, were included for every ten samples, and two internal laboratory standards, powdered keratin (MP Biomedicals Inc., Cat No. 90211, Lot No.9966H) and IAEA-CH-6 were included to monitor instrument drift and provide a check on accuracy over the course of each analytical session. Values of δ13C were calibrated to VPDB using USGS-40 (± 0.1‰ 1 SD, n = 8, accepted δ13C = −26.4‰) and USGS-41 (± 0.1‰, n = 8, accepted δ13C = +37.6‰). Measurement error was ± 0.1‰ for δ13C.
Assignment to Origins
We depicted the origins of wild monarchs caught in Mexico with a likelihood-based assignment method (Hobson et al., 2009b; Wunder, 2010), using the wing chitin δ2H isoscape (δ2Hw) and an amount-weighted precipitation-to-wing calibration algorithm derived by Hobson et al. (1999). This was used to convert amount-weighted mean annual precipitation δ2H (δ2Hp) isoscapes (Terzer et al., 2013; IAEA/WMO, 2015) into δ2Hw isoscapes. We used the 9.3‰ residual SD error from this regression in our assignments. We then created a spatial layer representing the known geographic range for the eastern breeding population of the monarch butterfly and used this as a mask (i.e., clip) to limit our assignment to origin analyses (Figure 2).
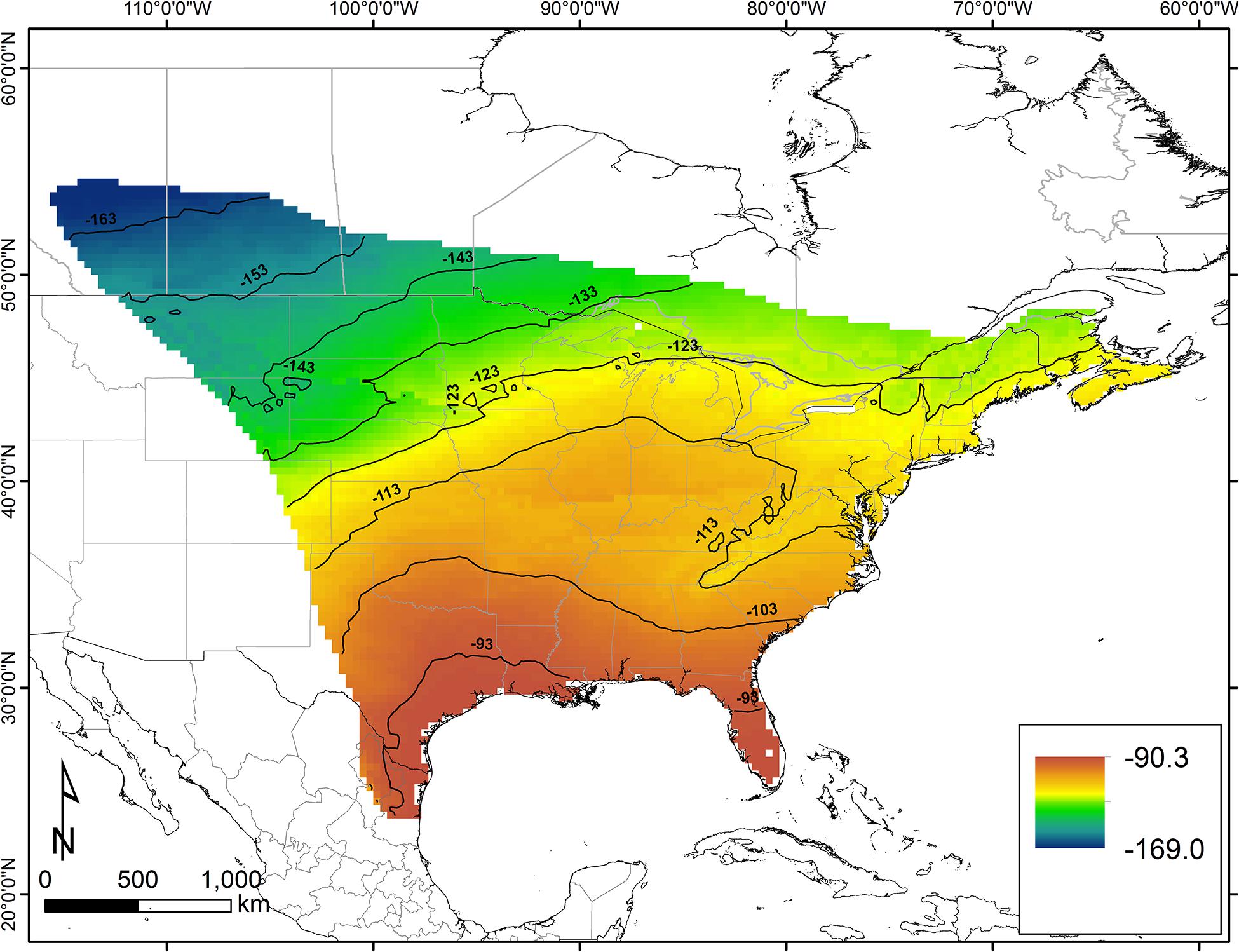
Figure 2. Predicted wing δ2H isoscpae for the eastern population of North American monarch butterfly. Surface was based on the use of a predicted amount-weighted mean growing season precipitation δ2H dataset (Terzer et al., 2013) and the precipitation-to-wing calibration algorithm of Hobson et al. (1999).
We estimated the likelihood that a cell (pixel) within the δ2Hw isoscape represented a potential origin for a sample by using a normal probability density function to estimate the likelihood function based on the observed δ2Hw, and thus depicted the likely origins of each monarch by assigning individuals to the δ2Hw isoscape one at a time. We arbitrarily chose a 2:1 odds ratio (see Van Wilgenburg et al., 2012) to include only those pixels (coded 1) with at least a 67% probability of origin vs. all others (coded 0). This resulted in a map of binary values for each assigned individual representing presence (1) or absence (0) for each cell in the isoscape. We then summed the results of individual assignments by stacking the surfaces. This approach thus provides assignment maps that show the number of individuals in a sample assigned to any given pixel.
Statistical Analyses
When data were normally distributed (Shapiro Wilk’s test), we compared isotopic data among groups using Analysis of Variance (ANOVA) and Tukey’s post-hoc comparisons with statistical significance set at p = 0.05. In cases where groups were not significantly different, they were pooled for further analysis. Simple regression was used to establish isotopic response variables in monarch tissues with isotope values of dietary treatments. The above analyses were completed in SPSS Version 24 (IBM Corp, 2016).
Geographic assignments to origin were made using functions within the R statistical computing environment (R Core Team, 2019) using scripts employing the “raster” v. 3.0-12 (Hijmans, 2020) and “maptools” v. 0.9-9 (Bivand and Lewin-Koh, 2019) packages. Thus, the final assignment surface depicted the number of individuals co-assigned at each pixel based on the odds criteria.
Results
Laboratory Experiments
No effect of sex was found for either δ13C [F(1, 37) = 0.10, p = 0.75] or δ2H [F(1, 37) = 0.035, p = 0.966] so the individual isotope data sets were pooled. The δ13C values of lipids were normally distributed (Shapiro Wilk, p > 0.05) and were significantly affected by treatment [F(3, 37) = 20.46, p < 0.001], with Tukey post-hoc analyses revealing differences based on the expected contrasts of C3 and C4 sugars (Supplementary Table S2). By regressing lipid δ13C values against sugar δ13C values for the unspiked water treatment (since tap water corresponded best to environmental waters experienced by monarchs), we obtained the relationship δ13Clipid = 0.59 ∗ δ13Csugar −15.08, indicating that nectar contributed ~59% of total carbon in the lipid.
Similarly, δ2H values of lipids were normally distributed (Shapiro Wilk, p > 0.05) and there was a significant effect of treatment [F(3, 37) = 8.96, p = 0.00017], with Tukey post-hoc analyses revealing differences based on the expected contrasts of spiked and unspiked nectar waters (Supplementary Table S2). The contribution of carbohydrate-based H to stored lipids was evaluated by examining the response in lipid δ2H with a change from beet to cane sugar under a constant unspiked water nectar source, which provided the relationship δ2Hlipid = 0.27 ∗ δ2Hsugar–279.6 indicating that sugar contributed ∼27% of the hydrogen in adult monarch lipids. A similar analysis examining the response in lipid δ2H with a change from tap water to spiked water under a constant C3 nectar sugar resulted in the relationship δ2Hlipid = 0.19 ∗ δ2Hwater–272.6 revealing that nectar water contributed about 19% of hydrogen in the fat body lipids. As determined in controlled studies involving metabolically inactive chitins (Hobson et al., 1999), our captive studies provided strong evidence for a close isotopic coupling between nectar sugars and water to synthesized lipids in monarchs. This provided the proof of concept required to interpret isotopic values in lipids of wild migrating monarchs.
Migration and Overwinter Samples
Wing δ2H values of monarchs captured during migration in northeast through central Mexico indicated that individuals potentially originated from many geographic regions of the breeding grounds. Both collection site and/or year did result in some differences in mean δ2Hw values during migration [Supplementary Table S3; F(5, 442) = 3.60, p = 0.003], with Nuevo Leon, 2017 being significantly different from both Nuevo Leon and Queretaro in 2016 (Tukey’s post hoc, p < 0.05). However, δ2Hw lipid values did not differ across samples taken during migration [Figure 3 and Supplementary Table S3, F(5, 442) = 1.74, p = 0.13]. There was no relationship between wing and lipid δ2H values (Figure 3; r2 < 0.006, p > 0.71) indicating that body lipid during migration was independent of natal origin and that the overall mean lipid δ2H values were remarkably constant regardless of wing δ2H values. For the purpose of illustration, we depicted origins of migrants for a subset of three sampling periods/locations in northeast to central Mexico (Figure 3): The first group was Queretaro and Nuevo Leon (2016) (n = 130; δ2Hw = −110.4 ± 12.4‰; δ2Hl = −324.3 ± 14.8‰; Figures 3A,B) and Queretaro and Guanajuato (2017) (n = 99; δ2Hw = −107.8 ± 13.7‰; δ2Hl = −329.5 ± 7.8‰; Figures 3C,D). The third group, Coahuila and Nuevo Leon (2017), was found to be bimodal for wing δ2H values which presents problems for isotopic assignments (Wunder, 2010) and so, based on inspection of the distribution of δ2Hw values (Supplementary Figure S1) we split this into one group with δ2Hw values < −103‰ (n = 118; δ2Hw = −116.8 ± 8.6‰, δ2Hl = −327.4 ± 20.1‰; Figures 3E,F) and the other with δ2Hw values > −103‰ (n = 98; δ2Hw = −91.9 ± 8.0‰, δ2Hl = −324.4 ± 16.1‰; Figures 3G,H).
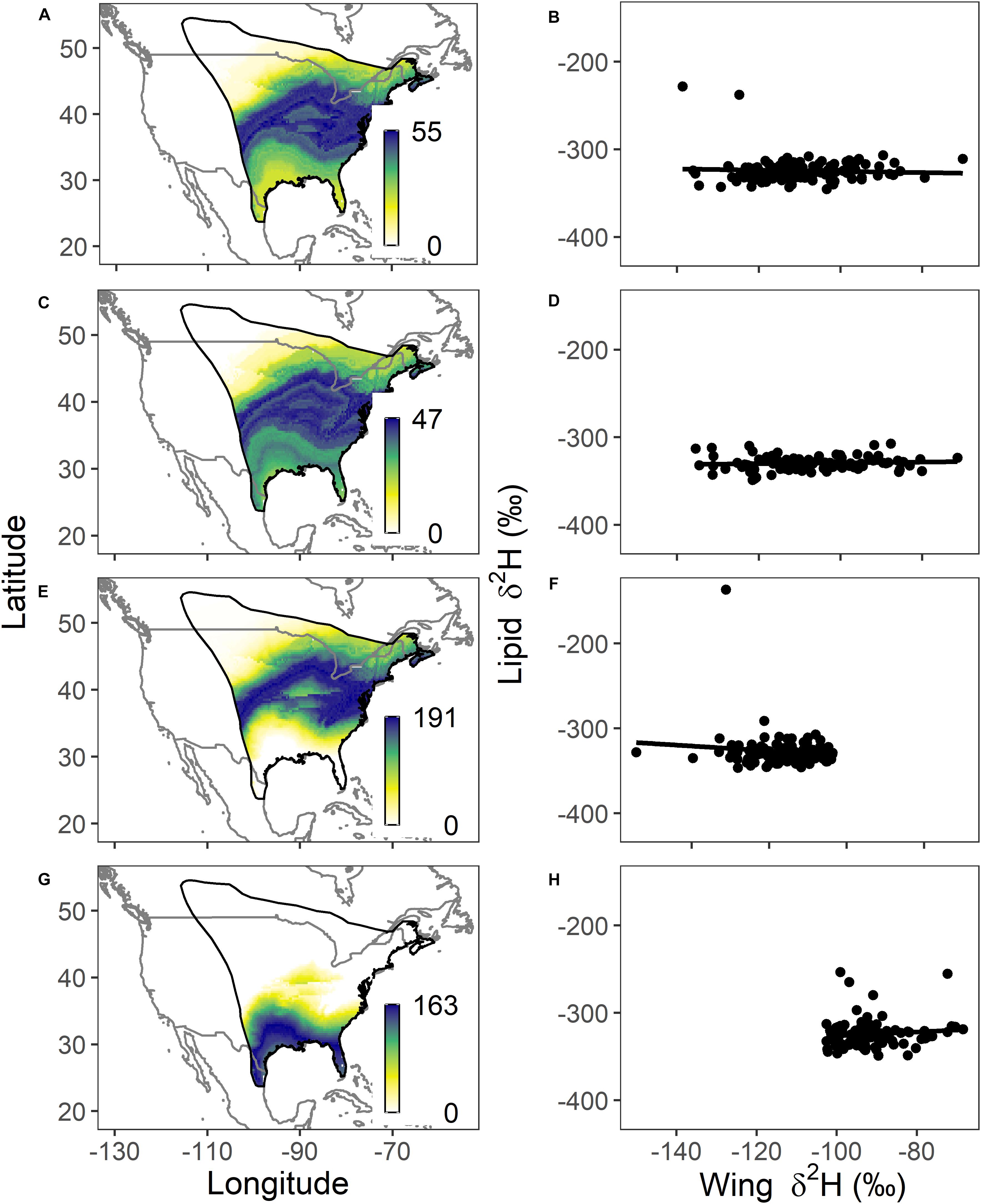
Figure 3. Depictions of potential natal locations and the relationship between lipid δ2H and wing δ2H for monarchs sampled during migration for (A,B) Queretaro and Nuevo Leon (2016) (n = 130), (C,D) Queretaro and Guanajuato (2017) (n = 99), (E,F) Coahuila and Nuevo Leon (2017) with wing δ2H < −103‰ (n = 118) and (G,H) Coahuila and Nuevo Leon (2017) with wing δ2H > −103‰ (n = 98). The legend represents the number of individuals at each pixel with wing δ2H values consistent with those predicted for that location based on a 2:1 odds ratio. In all cases, results reveal that monarchs represented diverse breeding origins but there was, in general, no relationship between natal origin and accumulated lipid stores.
A similar comparison for overwintering monarchs in 2015–2016 and 2017–2018 found that wing δ2H values differed among colonies [F(3, 87) = 9.57, p < 0.001] with two homogenous isotopic groupings (Supplementary Table S3). Lipid δ2H values for the same sample also differed among overwintering collections [F(4, 289) = 12.69, p < 0.001] and formed two homogenous isotopic groups with 2015–2016 groups El Rosario, Piedra Herrada, Sierra Chincua and the 2017–2018 Cerro Pelon group (overall mean −376.8 ± 29.6‰) showing more depleted values compared with Cerro Pelon in 2015–2016 (−331.5 ± 21.6‰). For illustrative purposes, we depicted origins of monarchs sampled in overwinter sites in the single season 2015−2016 (Figure 4): Cerro Pelon (n = 25; δ2Hw = −114.6 ± 12.2‰, δ2Hl = −340.7 ± 29.1‰; Figures 4A,B) and the combined Sierra Chincua, El Rosario and Piedra Herrada sites (n = 63; δ2Hw = −121.6 ± 39.7‰, δ2Hl = −372.0 ± 35.7‰; Figures 4C,D). Overall, across all years and sites, overwintering monarchs (n = 294) had lower lipid δ2H than migrating individuals [n = 448; migration: −326.4 ± 15.6‰; overwinter: −357.6 ± 32.4‰; F(1, 740) = 300.7, p < 0.001; Figure 5].
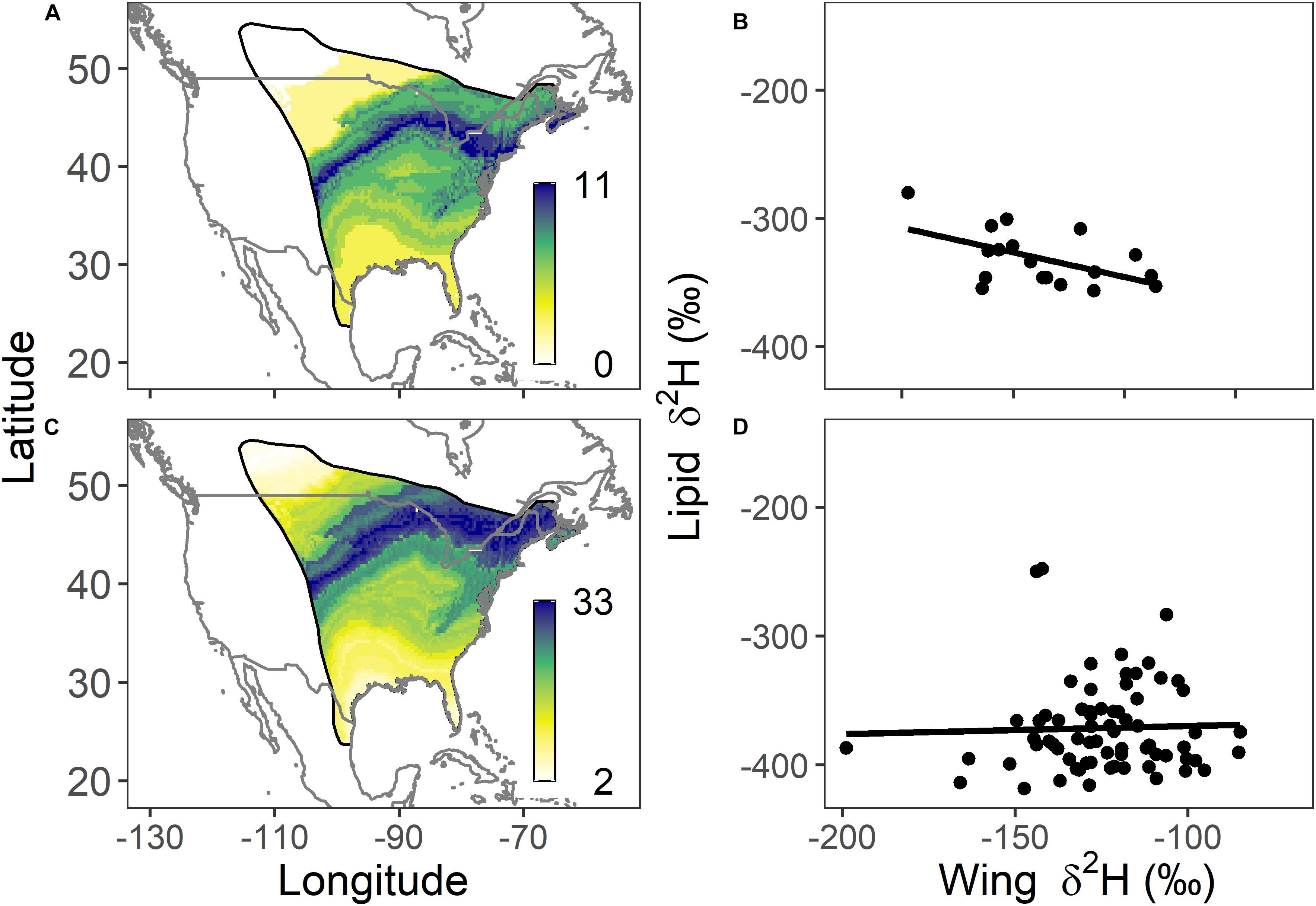
Figure 4. Depictions of potential natal locations and the relationship between lipid δ2H and wing δ2H for monarchs sampled at overwintering sites in 2015–2016: Cerro Pelon n = 25 (A,B) and Sierra Chincua n = 19; El Rosario n = 19; Piedra Herrada n = 28 (C,D). The legend represents the number of individuals at each pixel with wing δ2H values consistent with those predicted for that location based on a 2:1 odds ratio.
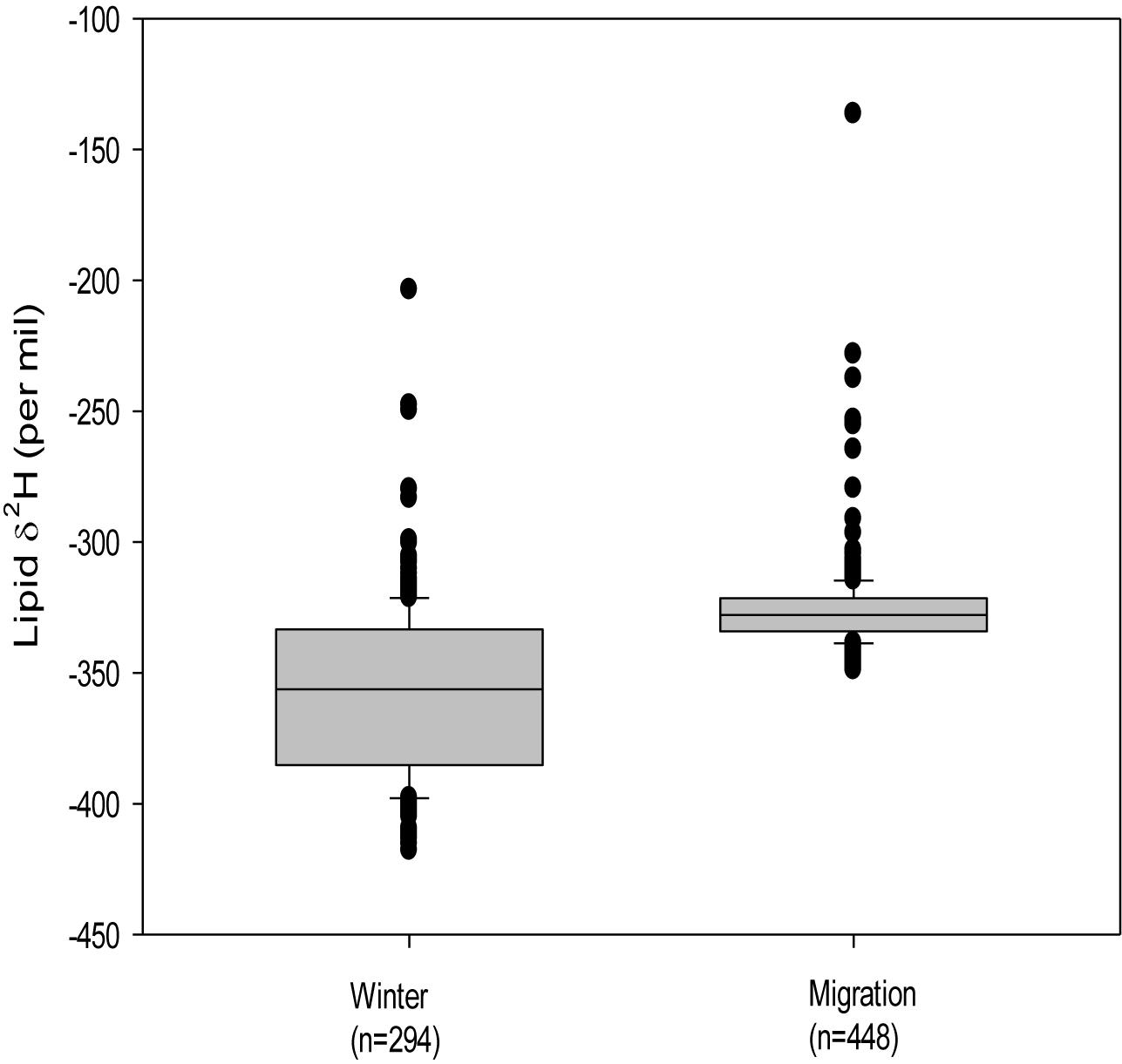
Figure 5. Difference in lipid δ2H values (‰) between migrating and overwintering monarch butterflies based on all lipid samples analyzed across sites and years (Supplementary Table S1). Not all specimens were run for wing δ2H analyses and so sample sizes may differ from those depicted in Figures 3, 4.
In 2018 at the Cerro Pelon overwinter site, there was no effect of sampling date or sex on lipid δ2H values [F(3, 84) = 1.65, p = 0.16; Supplementary Figure S2 and Supplementary Table S4]. Lipids were not quantitatively sampled for these specimens but we found no significant relationships (r2 < 0.006 in both cases) between lipid δ2H values and either dry mass or wing length.
Discussion
Our isotopic approach to investigating where and how monarch butterflies nectar to fuel the last leg of their fall migration and accumulate the necessary lipids for overwintering are encouraging and suggest a fundamentally new approach to evaluating critical nectaring strategies in this species and other animals. Our captive experiments clearly showed that stored lipids track the isotopic values of carbohydrate and water components of nectar diets. Despite a broad catchment origin of monarchs sampled across years along their Mexican migration route, monarch lipid δ2H values were not related to origin but were clustered indicating more local nectaring en route and consistent with more southern nectar sources. Lipid δ2H values of overwintering monarchs were more variable, and more negative δ2H values compared to active migrants suggests local nectaring at higher altitudes in some individuals or the selective loss of monarchs with higher lipid δ2H values detected in migration. Taken together, our results support the need to maintain nectaring corridors in the south including through northeast Mexico and in the vicinity of the overwintering sites. We encourage further isotopic research to ultimately derive means of probabilistically assigning monarch lipids to geographic origins via the establishment of species-specific “liposcapes” (Hobson and Wassenaar, 2019).
Our experimental analysis of captive monarchs allowed to accumulate lipids post emergence on manipulated nectar revealed that body lipids isotopically tracked dietary nectar and water sources. We estimated that, over the 5-day post-emergence period, about 59% of the carbon and 27% of the hydrogen in body lipids was derived from the sugar component of nectar and that the water in nectar contributed to about 19% of the hydrogen. The remaining components of body lipids were presumably derived from lipids formed at the larval stage. So, while we clearly have demonstrated that stored lipids can be quantitatively linked to dietary nectars, a prerequisite for applying stable isotope techniques to ultimately track sources of nectars for wild migrating monarchs, the larval effect in our study needs to be considered. However, for monarchs migrating in the fall and at overwintering sites, we can presume that little if any larval lipid was present. In fact, it is well known that monarchs are able to rapidly synthesize lipids when conditions permit (Beall, 1948; Brown and Chippendale, 1974).
Although nectaring occurs at wintering colonies, its prevalence depends on several factors and is generally considered to be minimal and the lipid component of body mass ranges from about 73% at arrival to 37% at departure on spring migration (Brower, 1985, reviewed by Calvert and Lawton, 1993). We investigated if there was evidence for isotopic discrimination occurring through the overwintering period at the colony of Cerro Pelon to determine if neutral lipids were differentially mobilized during the winter thereby resulting in isotopic changes in the remaining lipid stores. We found no evidence for lipid isotopic change January–March and this finding supports the potential use of stored bulk lipids in individuals at overwinter colonies as a means of inferring past nectaring history.
Why might overwintering monarchs have more depleted lipid δ2H values compared to migrants in northeast Mexico? Overwintering monarchs do require water, the degree to which depends on ambient temperature and levels of dehydration (Calvert and Lawton, 1993) and colonies are often located near a water source. This situation presents a potential hydrogen source to overwintering monarchs but lipids, consisting primarily of C-H bonds will not exchange with H from body water (Wassenaar, 2019). Rather, we suspect that some overwintering individuals had used nectar to form lipids at higher elevations, possibly close to the overwinter sites rather than at higher latitudes in the United States or Canada. Indeed, we expect higher δ2H values at lower elevations and that the altitude effect (Clark and Fritz, 1997) would result in significantly lower precipitation δ2H values at the ∼3000 m elevations of colonies along the Transvolcanic Mountain belt, in general, and the Sierra Madre Oriental region, in particular (Wassenaar et al., 2009, see also Hobson et al., 2009a). Although our migration and wintering lipid samples were collected across years, with the exception of Cerro Pelon sampled in 2015–2016, our results indicate that monarch lipids were more variable in their δ2H values at the overwinter colonies and also generally had lower lipid δ2H values (by ∼37‰) compared to those sampled during migration in northeastern Mexico. Further research is required to evaluate isotopic difference in stored lipids of monarchs during migration in northeast Mexico and those ultimately used by monarchs at overwintering colonies in order to evaluate the importance of late-stage nectaring just prior to and during the arrival phase at overwinter colonies. The prediction of more negative δ2H values of lipids at high-elevation overwintering sites compared to lower elevation migration sites from Texas and northeastern Mexico provides a potentially valuable approach to tracing the importance of flowers near the colonies.
There is much scope for future research into ways in which the stable isotope approach can be used to refine our understanding of origins of lipid synthesis in migrating monarchs and other Lepidoptera. Our captive studies using both monarchs and armyworms were necessarily of short duration (∼6 days post eclosion) and so it was not possible to entirely remove the larval influence on adult lipid stores. Ideally, these experiments would be repeated on individuals that were held on manipulated diets for long periods and then induced to go into diapause and rapidly accumulate fat stores thereby mimicking conditions experienced during migration. Regressions from such experiments would better link δ2H values of stored lipids to nectar sources integrated during migration and overwintering sites. However, because monarchs are nectar generalists (Tooker et al., 2002) and we expect plant nectar to vary considerably among and within plant species especially in water and sugar content (Abrahamczyk et al., 2017), an ultimate means of creating lipid-specific isoscapes (“liposcapes”) will be to either sample lipids of wild monarchs from known (i.e., constrained) nectaring sites on the breeding grounds prior to migration or captive monarchs allowed to nectar at outdoor pens exposed to natural precipitation (see Hobson et al., 1999). Such sampling would ideally be conducted across a large geographic gradient in precipitation δ2H. That approach has proven to be extremely successful in assigning several species of migrant birds to molt origin (Hobson and Wassenaar, 2019). Of course, stored bulk lipids can be derived from single geographic sites or represent an integration of many sites spread over large spatial gradient in environmental δ2H values. As such, in tissues like the fat body of insects, the isotope approach will always represent a probabilistic average compared to metabolically inert tissues like wing chitins. Clearly, evaluating lipid turnover rates in migrating monarchs would allow insights into expected bulk lipid isotope values. Here again, captive studies using monarchs in a “migratory phase” could expose individuals to a diet-switch (i.e., from a depleted to an enriched 2H nectar source) that would then allow the rate of lipid synthesis in the fat body to be quantified. Further manipulations could also expose individuals to different levels of flight exercise. Finally, we fully recognize that the path ahead should involve compound-specific (fatty acid) vs. bulk lipid isotopic analyses that promise to more accurately reflect metabolic pathways leading to lipid synthesis (Whiteman et al., 2019). Since stored lipids in monarchs are primarily palmitic and oleic acids (Brower et al., 2006), the compound-specific approach focused on these fatty acids should be rewarding.
We stress that our study was conducted largely through a period of drought in southern Texas and northeast Mexico and we fully expect the dynamics of lipid synthesis and storage in migrating monarchs to change depending on fall climate conditions. Such profound influences of climate on the migratory and overwinter success of monarchs (e.g., Saunders et al., 2019) means that the wing and lipid isotopic sampling of migrant and overwintering monarchs should be conducted annually or at least every few years to elucidate nectar origins. Overwhelmingly Our work provides support for maintaining nectar corridors in the southern portion of the range and especially in Mexico along the migration route1 and potentially in the immediate vicinity of the overwintering sites (Figure 6).
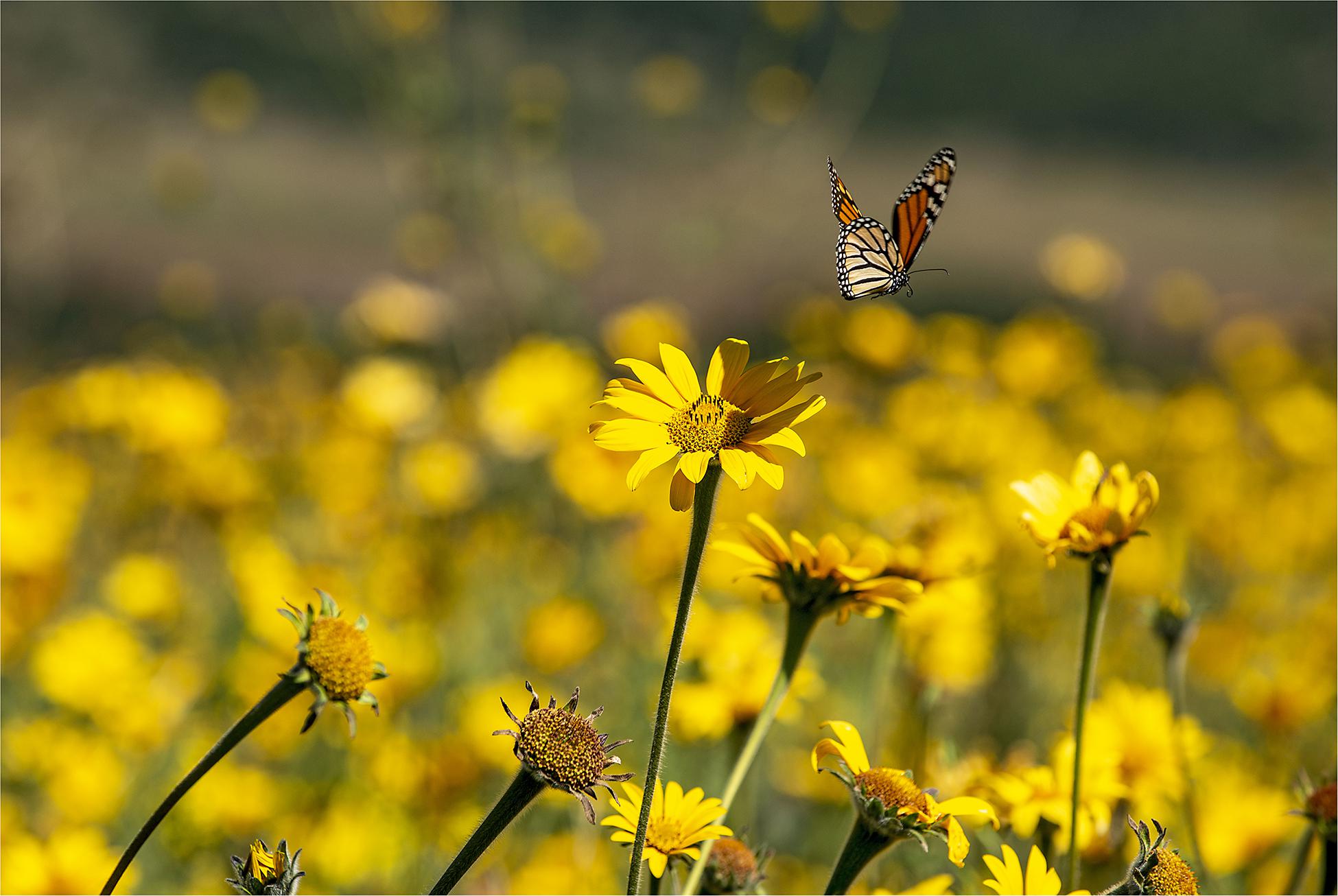
Figure 6. A migrating monarch butterfly through Nuevo Leon, Mexico. Active nectaring on flowers by migrating monarchs throughout the region is commonly observed. Photo by Omar Franco.
Data Availability Statement
The raw data supporting the conclusions of this article will be made available by the authors, without undue reservation, to any qualified researcher.
Author Contributions
KH conceived the study. KH and JM wrote the first draft. BM, OG-R, RC-T, and EG-S collected the monarch specimens. LA conducted the captive study. KK assisted with assignments and figures. All authors edited final manuscript.
Funding
This work was supported by the University of Western Ontario and an NSERC Discovery grant (2017-04430) to KH.
Conflict of Interest
The authors declare that the research was conducted in the absence of any commercial or financial relationships that could be construed as a potential conflict of interest.
Acknowledgments
Stable isotope analyses were performed by the KH at the LSIS-AFAR stable isotope facility. We thank Dr. Isabel Ramirez for assisting with sample collections that formed part of her contract with the Commission for Environmental Cooperation. We also thank Orley (Chip) Taylor and reviewer for their constructive comments on an earlier version of the manuscript.
Supplementary Material
The Supplementary Material for this article can be found online at: https://www.frontiersin.org/articles/10.3389/fevo.2020.572140/full#supplementary-material
Footnotes
References
Abrahamczyk, S., Kessler, M., Hanley, D., Karger, D. N., Muller, M. P., Knauer, A. C., et al. (2017). Pollinator adaptation and the evolution of floral néctar sugar composition. J. Evol. Biol. 30, 112–127. doi: 10.1111/jeb.12991
Alonso-Mejia, A., Rendon-Salina, E., Montesinos-Patino, E., and Brower, L. P. (1997). Use of lipid reserves by monarch butterflies overwintering in Mexico: implications for conservation. Ecol. Appl. 7, 934–947.
Altizer, S. M., Hobson, K. A., Davis, A. K., de Roode, J. C., and Wassenaar, L. I. (2015). Do healthy monarchs migrate farther? Tracking natal origins of parasitized vs. uninfected monarch butterflies overwintering in Mexico. PLoS One 10:e0141371. doi: 10.1371/journal.pone.0141371
Beall, G. (1948). The fat content of a butterfly Danaus plexippus Linn. As affected by migration. Ecology 29, 80–94. doi: 10.2307/1930346
Bivand, R. S., and Lewin-Koh, N. (2019). Maptools: Tools for Reading and Handling Spatial Objects, Version 0.8-39. Available online at: http://CRAN.R-project.org/package=maptools (accessed September 20, 2020).
Brower, L. P. (1985). “New perspectives on the migration biology of the monarch butterfly, Dnaus plexippus L,” in Migration: Mechanisms and Adaptive Significance, ed. M. A. Rankin (Ustin, TX: University of Texas), 748–785.
Brower, L. P., Fink, L. S., Kiphart, R. J., Pocius, V., Zubieta, R. R., and Ramirez, M. I. (2015). “Effect of the 2010-2011 drought on the lipid content of monarchs migrating through Texas to overwintering sites in Mexico,” in Monarchs in a Changing World: Biology and Conservation of an Iconic Butterfly, eds K. S. Oberhauser, K. R. Nail, and S. Altizer (Ithaca: Cornell University Press), 117–129.
Brower, L. P., Fink, L. S., and Walford, P. (2006). Fueling the fall migration of the monarch butterfly. Integrat. Comp. Biol. 46, 1123–1142. doi: 10.1093/icb/icl029
Brown, J. J., and Chippendale, G. M. (1974). Migration of the monarch butterfly Danaus plexippus: energy sources. J. Insect Physiol. 20, 1117–1130. doi: 10.1016/0022-1910(74)90218-2
Calvert, W. H., and Lawton, R. O. (1993). “Comparative phenology of variation in size, weight, and water content of eastern North American monarch butterflies at five overwintering sites in Mexico,” in Biology and Conservation of the Monarch Butterfly, eds S. B. Malcolm and M. P. Zalucki (Los Angeles: Natural History Museum of Los Angeles), 299–307.
Cenedella, R. J. (1971). The lipids of the female monarch butterfly, Danaus plexippus, during fall migration. Insect Biochem. 1, 244–247. doi: 10.1016/0020-1790(71)90077-1
Flockhart, D. T., Brower, L. P., Ramirez, M. I., Hobson, K. A., Wassenaar, L. I., Altizer, S., et al. (2017). Regional climate on the breeding grounds predicts variation in the natal origin of monarch butterflies overwintering in Mexico over 38 years. Glob. Chang. Biol. 23, 2565–2576. doi: 10.1111/gcb.13589
Flockhart, T., Wassenaar, L. I., Martin, T., Hobson, K. A., Wunder, M., and Norris, D. R. (2013). Tracking multi-generational colonization of the breeding grounds by monarch butterflies in eastern North America. Proc. Roy. Soc. Lond. B 280, 1–8. doi: 10.1155/2009/705780
Gibo, D. L., and McCurdy, J. A. (1993). Lipid accumulation by migrating monarch butterflies (Danaus plexippus L.). Can. J. Zool. 71, 76–82. doi: 10.1139/z93-012
Hijmans, R. J. (2020). raster: Geographic Data Analysis and Modeling, Version 2.5-8. Available online at: http://CRAN.R-project.org/package=raster (accessed September 20, 2020).
Hobson, K. A., Van Wilgenburg, S. L., Larson, K., and Wassenaar, L. I. (2009a). A feather hydrogen isoscape for Mexico. J. Geochem. Explor. 102, 63–70. doi: 10.1016/j.gexplo.2009.02.002
Hobson, K. A., and Wassenaar, L. I. (eds) (2019). Tracking Animal Migration with Stable Isotopes, 2nd Edn, London: Academic Press.
Hobson, K. A., Wassenaar, L. I., and Taylor, O. R. (1999). Stable isotopes (δD and δ13C) are geographic indicators of natal origins of monarch butterflies in eastern North America. Oecologia 120, 397–404. doi: 10.1007/s004420050872
Hobson, K. A., Wunder, M. B., Van Wilgenburg, S. L., Clark, R. G., and Wassenaar, L. I. (2009b). A Method for investigating population declines of migratory birds using stable isotopes: origins of harvested lesser scaup in North America. PLoS One 4:e7915. doi: 10.1371/journal.pone.0007915
IAEA/WMO (2015). Global Network of Isotopes in Precipitation. The GNIP Database. Available online at: http://www.iaea.org/water (accessed May 17, 2020).
IBM Corp (2016). Released 2016. IBM SPSS Statistics for Windows, Version 24.0. Armonk, NY: IBM Corp.
Lennox, R. J., Chapman, J. M., Souliere, C. M., Tudorache, C., Wikelski, M., Metcalfe, J. D., et al. (2016). Conservation physiology of animal migration. Conserv. Physiol. 4:cov072. doi: 10.1093/conphys/cov072
McWilliams, S. R., Guglielmo, C. G., Pierce, B., and Klaassen, M. (2004). Flying, fasting and feeding in birds during migration: a nutritional and physiological ecology perspective. J. Avian Biol. 35, 377–393. doi: 10.1111/j.0908-8857.2004.03378.x
Miller, N. G., Wassenaar, L. I., Hobson, K. A., and Norris, D. R. (2012). Migratory connectivity of the monarch butterfly (Danaus plexippus): patterns of spring re-colonization in eastern North America. PLoS One 7:e0031891. doi: 10.1371/journal.pone.0031891
Moore, F. R., Gauthreaux, S. A., Kerlinger, P., and Simons, T. R. (1993). “Habitat requirements during migration: Important link in conservation,” in Ecology and Management of Neotropical Migratory Birds, eds T. E. Martin and D. M. Finch (Fort Collins, CO: USDA Forest Service), 121–144.
R Core Team (2019). R: A Language and Environment for Statistical Computing, Version 3.3.2. Vienna: The R Foundation for Statistical Computing.
Saunders, S. P., Ries, L., Neupane, N., Ramírez, M. I, García-Serrano, E. U., Rendón-Salinas, E., et al. (2019). Multiscale seasonal factors drive the size of winter monarch colonies. Proc. Nat. Acad. Sci. U.S.A. 116, 8609–8614. doi: 10.1073/pnas.1805114116
Soto, D. X., Koehler, G., Wassenaar, L. I., and Hobson, K. A. (2017). Determination of stable hydrogen isotopic compositions of complex organic materials: contrasting the role of exchangeable hydrogen and residual moisture. Rapid Comm. Mass Spectrom. 31, 1193–1203.
Taylor, O. R., Lovett, J. P., Gibo, D. L., Weiser, E. L., Thogmartin, W. E., Semmens, D. J., et al. (2019). Is the timing, pace and success of the monarch migration associated with sun angle? Front. Ecol. Evol. 7:442. doi: 10.3389/fevo.2019.00442
Terzer, S., Wassenaar, L. I., Araguás-Araguás, L. J., and Aggarwal, P. K. (2013). Global isoscapes for δ18O and δ2H in precipitation: improved prediction using regionalized climatic regression models. Hydrol. Earth Syst. Sci. 17, 4713–4728. doi: 10.5194/hess-17-4713-2013
Tooker, J. F., Reagel, P. F., and Hanks, L. M. (2002). Nectar sources of dayflying lepidoptera of Central Illinois. Ann. Entomol. Soc. Am. 95, 84–96. doi: 10.1603/0013-8746(2002)095[0084:nsodfl]2.0.co;2
Van Wilgenburg, S. L., Hobson, K. A., Brewster, K. R., and Welker, J. M. (2012). Addressing uncertainty in assessing dispersal in threatened migratory species using stable hydrogen isotope analysis of feathers. Endang. Spec. Res. 16, 17–29. doi: 10.3354/esr00383
Wassenaar, L. I. (2019). “Introduction to conducting stable isotope measurements for animal migration studies,” in Tracking Animal Migration with Stable Isotopes, 2nd Edn, eds K. A. Hobson and L. I. Wassenaar (London: Academic Press), 25–51. doi: 10.1016/b978-0-12-814723-8.00002-7
Wassenaar, L. I., and Hobson, K. A. (1998). Natal origins of migratory Monarch Butterflies at wintering colonies in Mexico: New isotopic evidence. Proc. Nat. Acad. Sci. U.S.A. 95, 15436–15439. doi: 10.1073/pnas.95.26.15436
Wassenaar, L. I., and Hobson, K. A. (2003). Comparative equilibration and online technique for determination of non-exchangeable hydrogen of keratins for use in animal migration studies. Isotopes Environ. Health Stud. 39, 1–7.
Wassenaar, L. I., Van Wilgenburg, S. L., Larson, K., and Hobson, K. A. (2009). A Groundwater Isoscape (δD, δ18O) for Mexico. J. Geochem. Explor. 102, 123–136. doi: 10.1016/j.gexplo.2009.01.001
West, J. B., Kreuzer, H. W., and Ehleringer, J. R. (2010). “Approaches to plant hydrogen and oxygen isoscapes generation,” in Isoscapes, eds J. West, G. Bowen, T. Dawson, and K. Tu (Dordrecht: Springer).
West, J. B., Sobek, A., and Ehleringer, J. R. (2008). A simplified GIS approach to modeling global leaf water isoscapes. PLoS One 3:e2447. doi: 10.1371/journal.pone.0002447
Whiteman, J. P., Elliott Smith, E. A., Besser, A. C., and Newsome, S. D. (2019). A guide to using compound-specific stable isotope analysis to study the fates of molecules in organisms and ecosystems. Diversity 11, 1–18.
Williams, E. H., and Brower, L. P. (2015). “Microclimate protection of overwintering monarchs provided by Mexico’s high-elevation oyamel fir forests,” in Monarchs in a Changing World: Biology and Conservation of an Iconic Butterfly, eds K. S. Oberhauser, K. R. Nail, and S. Altizer (Ithaca: Cornell University Press), 109–116.
Keywords: lipids, overwintering, stable isotopes, deuterium, carbon-13, nectar corridors, migratory fueling
Citation: Hobson KA, García-Rubio OR, Carrera-Treviño R, Anparasan L, Kardynal KJ, McNeil JN, García-Serrano E and Mora Alvarez BX (2020) Isotopic (δ2H) Analysis of Stored Lipids in Migratory and Overwintering Monarch Butterflies (Danaus plexippus): Evidence for Southern Critical Late-Stage Nectaring Sites? Front. Ecol. Evol. 8:572140. doi: 10.3389/fevo.2020.572140
Received: 15 June 2020; Accepted: 14 September 2020;
Published: 09 October 2020.
Edited by:
Jennifer M. Gleason, University of Kansas, United StatesReviewed by:
Chip Taylor, University of Kansas, United StatesVictoria Pocius, Pennsylvania State University (PSU), United States
Copyright © 2020 Hobson, García-Rubio, Carrera-Treviño, Anparasan, Kardynal, McNeil, García-Serrano and Mora Alvarez. This is an open-access article distributed under the terms of the Creative Commons Attribution License (CC BY). The use, distribution or reproduction in other forums is permitted, provided the original author(s) and the copyright owner(s) are credited and that the original publication in this journal is cited, in accordance with accepted academic practice. No use, distribution or reproduction is permitted which does not comply with these terms.
*Correspondence: Keith A. Hobson, a2hvYnNvbjZAdXdvLmNh