- 1Département de biologie, Université de Moncton, Moncton, NB, Canada
- 2Department of Ecology and Evolutionary Biology, University of Toronto, Toronto, ON, Canada
- 3Department of Biology, Mount Allison University, Sackville, NB, Canada
Perceived predation risk can elicit strong behavioral responses in potential prey. During nest building, songbirds exhibit anti-predator behaviors under experimental conditions. Here, we hypothesized that females of two ground-nesting songbird species, the Ovenbird (Seiurus aurocapilla) and the Hermit Thrush (Catharus guttatus), would use naturally available cues of predation risk when selecting their nest site, thereby avoiding activity hotspots of Eastern Chipmunks (Tamias striatus), a predator on songbird nests and fledglings. Chipmunks are highly vocal, thus providing cues of their presence. We mapped chipmunk detections and songbird nests over four successive years in study plots located in mature deciduous forest of New Brunswick, Canada. Chipmunk activity varied by an order of magnitude among study plots and years. Nests were built further away from chipmunk detections than expected by chance in some, but not all, plot-year combinations. When comparing study plots, the proportion of nests built within hotspots of chipmunk activity was four times lower in the two plots where chipmunk activity was highest. Yet, we did not find clear evidence that chipmunk avoidance provided fitness benefits, possibly because this behavior procured little protection at high chipmunk densities. The persistence of this avoidance behavior in our focal species of ground-nesting songbirds might be linked to the benefits it procures at intermediate chipmunk densities.
Introduction
Variation in perceived predation risk can have profound effects on prey behavior or life-history traits, including vigilance, movement patterns, habitat use, growth rate, reproductive effort and, ultimately, population dynamics (Preisser et al., 2005; LaManna et al., 2015; Dröge et al., 2017). Although individuals responding to perceived predation risk can increase their probability of survival or make more strategic investment in reproduction (Zanette, 2011; LaManna and Martin, 2016), these responses still incur a cost in terms of access to resources and mates, or energy investment in defense mechanisms or structures (Preisser et al., 2005). Yet, the persistence of such behaviors in prey populations suggests that they are adaptive.
Natural selection favors individuals making optimal decisions when selecting their breeding territory (Clark and Shutler, 1999; Germain and Arcese, 2014). Among other factors, perceived predation risk would be expected to influence habitat selection. In songbirds, nest predation is the main cause of nesting failure (Martin, 1992) and, thus, it should represent a strong agent of natural selection because nest provisioning and care for nestlings require high-energy investments on the part of adults. Indeed, renesting can have negative effects on female body condition (Morris et al., 2015) and potentially on their fitness, through carry-over effects (Saino et al., 2017). Several studies have suggested that birds avoid areas where nest predators are abundant when selecting their breeding territory (e.g., Morton, 2005; Fontaine and Martin, 2006; Bonnington et al., 2015). Birds might also respond to predation risk by gathering information on nest predator abundance when selecting a nest site within their territory (e.g., Forstmeier and Weiss, 2002; Emmering et al., 2018). Schmidt et al. (2006) have documented fine-scale (ca. 225 m2) spatial patterns in the relationship between a nest predator (White-footed Mouse, Peromyscus leucopus) and its prey (nests of Veery, Catharus fuscescens). Other studies addressing songbird nest placement in response to nest predator abundance have been conducted at broader spatial scales (e.g., 2 ha, Kearns and Rodewald, 2017; 0.09 ha, Emmering et al., 2018) or have specifically investigated shifts in nest height (Peluc et al., 2008).
Nest site selection may or may not be adaptive, depending on the study system. There are many examples of apparently adaptive nest placement relative to vegetation composition (Martin, 1998) or structure (Martin and Roper, 1988; Poulin et al., 2008). Yet, in other systems, preferred nest sites may not necessarily be those where nest survival rate is highest (Martin, 1998; Latif et al., 2011). Low congruence between habitat selection and fitness might reflect fluctuations in the density of nest predators (Schmidt et al., 2008), year-to-year changes in habitat quality due to precipitation and their effects on microhabitat and food (Zanette et al., 2006), differences in selection pressures acting on nest versus fledgling survival (Streby et al., 2014), attraction to human-modified environments (Hollander et al., 2011), or the limited availability of good-quality nest sites (Holmes et al., 1996). Nonetheless, birds have been shown to behave proactively to reduce nest predation risk under natural conditions, for example through the selection of safer nest sites (Hansson et al., 2000; Chalfoun and Martin, 2009), through habitat selection at broader spatial scales (LaManna and Martin, 2016), or via a shift in their latitudinal distribution (McKinnon et al., 2010). Birds may also actively seek safer nest sites among those accessible to nest predators (Forstmeier and Weiss, 2004; Schmidt et al., 2006) based on available cues of predator presence or abundance (Eggers et al., 2006; Emmering and Schmidt, 2011).
Here, we performed an observational study to determine whether the location of songbird nests was related to fine-scale variation in nest predator activity. Rodents, including chipmunks, are major nest predators and can account for up to 75% of all nest predation events (Hethcoat and Chalfoun, 2015). The present study was conducted in a northern hardwood forest of eastern Canada and focused on Eastern Chipmunk (Tamias striatus), a known nest predator on ground- and shrub-nesting passerines (King and DeGraaf, 2006; Fiola et al., 2017). This species has also been shown to kill Ovenbird (King et al., 2006; Haché et al., 2014), Cerulean Warbler (Setophaga cerulea, Raybuck et al., 2020), and Wood Thrush (Hylocichla mustelina, Schmidt et al., 2008) fledglings and, therefore, is a highly probable predator on other thrush species. We also have photographic evidence of a chipmunk predation event on an Ovenbird nest (Fiola et al., 2017). In this study, we aimed to determine whether ground-nesting songbird females respond proactively to nest predation risk in the absence of artificial enhancement of stimuli. We hypothesized that females would assess nest predation risk when choosing a nest site.
In many migratory bird species, males arrive first from spring migration. Hence, they would be expected to select a breeding territory characterized, among other parameters, by low nest predation risk. However, the timing of male return from spring migration may not match the activity patterns of some nest predators. For example, in our study area of northern New Brunswick, Canada, it would seem almost impossible for male Ovenbirds (Seiurus aurocapilla) and Hermit Thrush (Catharus guttatus), the two most common ground-nesting songbird species in our study plots, to assess nest predation risk because above-ground activity and vocalizations of Eastern Chipmunks are minimal until the last week of May. Male Ovenbirds settle on a territory in mid-May and they appear to use private information (their previous year’s reproductive success) and location cues provided by conspecifics to select their territory (Thériault et al., 2012). Successful males were more likely to return to the same plot the following year than unsuccessful ones (Haché and Villard, 2010), whereas unbanded males immigrating into a plot seemed to rely on location cues, as they were never found to settle into a site before the first returning male (Thériault et al., 2012). Hermit Thrushes settle on territory slightly earlier than Ovenbirds, but less is known about the cues they use to select their territory.
Ovenbird females arrive on the breeding grounds 9–14 days after males (Porneluzi et al., 2020) and then, presumably, proceed to choose the best territory or the best male (Germain and Arcese, 2014). They may also be able to assess nest predation risk, using this information to select the safest territory available and to determine the safest location within the chosen territory. Indeed, in our study area, the emergence of Eastern Chipmunks typically occurred between Ovenbird territory settlement and nest building, although the precise timing varied as a function of the previous year’s seed crop (Bergeron et al., 2011). Since chipmunk activity can fluctuate up to one order of magnitude between years (e.g., Fiola et al., 2017), females must use cues from the current year to make adaptive choices to reduce nest predation risk, and experimental evidence suggests that they do (Emmering and Schmidt, 2011). In a playback experiment, the latter authors found that nest-building females of two ground-nesting species actively avoided speakers broadcasting Eastern Chipmunk vocalizations. Similarly, Morton (2005) observed that Ovenbirds preferred to settle in lower-quality habitat, because prime habitat was characterized by high chipmunk density. Emmering et al. (2018) also observed that Ovenbirds built their nests away from clusters of chipmunk activity.
We mapped the nests of the two most common ground-nesting songbird species in our study plots, Hermit Thrush and Ovenbird. Ovenbirds prefer deciduous and mixedwood stands with an open understory (Porneluzi et al., 2020), like Eastern Chipmunks (Bowers, 1995), whereas Hermit Thrushes use a broader range of stand types (Dellinger et al., 2020). In both songbird species, females build the nest alone. We predicted that nests would be placed in areas characterized by lower Eastern Chipmunk activity relative to the surroundings (coldspots). In our study plots, the number of Eastern Chipmunk detections was an order of magnitude higher than those of two other nest predators: Red Squirrel (Tamiasciurus hudsonicus) and Blue Jay (Cyanocitta cristata) (Supplementary Table 1). Barred Owl (Strix varia) and American Marten (Martes americana), both generalist predators also known to depredate songbird nests, were detected in some of our study plots but at even lower densities. Hereafter, we focused our attention on eastern chipmunks. This species was not only the most abundant diurnal nest predator, but also the most vocal, and its relatively small home range size (0.04–0.3 ha, Lacher and Mares, 1996; 0.5–0.8 ha, Gharnit et al., unpublished data) relative to the territory size of the focal songbird species (Ovenbird: ca. 1 ha, Haché and Villard, 2010; Hermit Thrush: 0.7 ha, Dellinger et al., 2020), made it possible for songbird females to avoid its hotspots of activity to some extent when selecting their nest sites. In the same study area, we found that nest survival in Ovenbirds was negatively related to an index of Eastern Chipmunk activity (Fiola et al., 2017). Here, we predicted that daily nest success of either focal species will be negatively related to chipmunk activity at the nest.
Materials and Methods
This study was conducted in a northern hardwood forest of northwestern New Brunswick, Canada (47°23′ N, 67°40′ W). For the purposes of this study, we focused on two of the five plot pairs selected by Haché and Villard (2010) to quantify the demographic response of songbirds to timber harvesting. During the winter of 2006–2007, one plot of each pair was treated through selection harvesting (30–40% basal area removal, plots B and D). The average distance between paired plots was 4.2 ± 1.0 km (mean ± SD), and 23.8 ± 9.1 km among pairs. All four 25-ha study plots (A,B,C,D) were located in well-drained sites on glacial till deposits and dominated by shade-tolerant deciduous tree species: Sugar Maple (Acer saccharum), Yellow Birch (Betula alleghaniensis), and American Beech (Fagus grandifolia) (Table S1 in Fiola et al., 2017). The four plots were selected based on their relatively high abundances of Eastern Chipmunks, as determined from detections during avian spot mapping (Poulin et al., 2010). Because selection harvesting had no significant effect on Eastern Chipmunk activity in those study plots (Poulin et al., 2010), we did not include harvest treatment in our models. We recorded Eastern Chipmunk locations and songbird nest positions from 2012 to 2015.
Field Survey Methods
Songbird Surveys
We mapped all visual or aural detections of songbirds using a standard spot-mapping method. Observers walked slowly along transects located 100 m apart and recorded all individuals of all species detected within a 50-m band on either side of the transect line. Each detection was recorded on a detailed map of the grid (scale: 1 cm = 25 m), including a 25-m buffer all around. Each year (25 May–30 June), we conducted eight spot mapping visits per plot between 0600 and 0900. Surveys were only conducted under favorable weather conditions (no precipitation nor strong winds). Territories were drawn based on a minimum of two detections separated by at least 10 days (Bibby et al., 2000). In the case of Ovenbirds, territorial males were individually identified using color band combinations, which helped to confirm nest success.
Nest Searching and Monitoring
We performed intensive nest searches within mapped Ovenbird territories and around locations where any sign of reproductive activity of the focal species had been detected during spot mapping. First, we followed Ovenbird males in each territory to determine their pairing status (i.e., presence of a female or signs of reproductive activity). Once a female was observed, we followed her to detect cues suggesting the presence of a nest (e.g., agitated behavior, individuals carrying nest material or food). We also followed males carrying food and we performed intensive searches in areas where we had lost sight of them. For Ovenbirds, nest searching was conducted every 2–3 days in each territory.
Hermit Thrush nests were found incidentally while spot mapping birds or nest predators, or when conducting intensive nest searching within Ovenbird territories. Study plots were thoroughly surveyed, and nest-searching methods were consistent among plots and years. In our study area, the timing of Hermit Thrush nest building preceded that of Ovenbirds by ca. one week (MLF, AV, MAV, personal observation).
All nests were monitored every 2–3 days until depredated, abandoned, or all young had fledged. All nests found (n = 104) were included in the analyses, and their fate was recorded as abandoned, depredated, or successful. A nest was considered abandoned if it was undamaged (before eggs were laid or if the eggs were left untouched), but there were no signs of adult activity at or around the nest after three visits. A predation event was recorded when a nest was found empty or partially empty after eggs had been laid and prior to the expected fledging date.
Chipmunk Surveys
We estimated nest predator activity using an intensive spot-mapping protocol. To map nest predator locations, one observer walked slowly and as silently as possible along transects located 50 m apart and recorded all visual or aural detections within a 25 m band on either side of the transect line, for a total of 5.5 km and a duration of 3.5–4 h (Supplementary Figure 2). This band width was selected to maximize detectability. Each detection was recorded on a detailed map of the grid (scale: 1 cm = 25 m), including a 25 m buffer all around. Most of the detections were visual, sometimes following an aural detection. We recorded detections of Eastern Chipmunks, Red Squirrels, and Blue Jays. Because Eastern Chipmunks were by far the most common of the three, with a number of detections per visit an order of magnitude higher (Supplementary Table 1), we only used Eastern Chipmunk detections in the analyses. Each plot was surveyed between 900 and 1300, a period encompassing the peak of Eastern Chipmunk activity (Snyder, 1982). We conducted six surveys per plot each year, between 23 May and 21 June, except in 2013, when only five visits were completed due to unusually cold weather at the end of May. Because Eastern Chipmunks are less active in cold weather (i.e., below 15°C, Dunford, 1972), we assumed the later start would not substantially affect the activity index recorded that year, as we also observed a later start in the Ovenbird nest building phase. Nest predator survey dates were chosen to coincide with the peak in construction of first nests or renests by female Ovenbirds (Supplementary Figure 3). Surveys were only conducted when weather conditions did not interfere with detection (no precipitation nor strong winds). When analyzing each year separately, there was no observer effect on the number of Eastern Chipmunk detections (Fiola et al., 2017). Although the spot mapping method we used to estimate Eastern Chipmunk activity does not yield an abundance estimate like capture-mark-recapture techniques, it provides an estimate of visual and aural cues available to songbirds.
All survey protocols were approved by Université de Moncton’s Animal Care Committee. All manipulations were performed in accordance with the guidelines and regulations of the Canadian Council on Animal Care.
Statistical Analyses
We first calculated the 50 and 90% isopleths to determine where most chipmunks were detected in each site for each year using the isopleth function of Hawthorne’s Geospatial Modelling Environment with ArcGIS. We tested whether bird nests showed evidence for positive or negative association with 50% isopleths of chipmunk activity using a bivariate Ripley’s K-function (Dale and Fortin, 2014). An isopleth is a contour line that delineates area where a concentration of locations can be found. Here, we selected 50%, a value commonly used in animal ecology (e.g., Wilson et al., 2010). Bivariate Ripley’s K-function is a point-event method that determines the scales at which two types of events (here, bird nest locations and chipmunk detections) are either spatially aggregated or segregated from each other. For these analyses, we used the coordinates of each chipmunk detection comprised within the 50% isopleth. Observed Ripley’s K values are computed at increasing radius r and are depicted as the black line in the plots of Ripley’s K against the radii, r). Then, to assess the significance of Ripley’s K value calculated at increasing window sizes, we used the null hypothesis of complete spatial randomness (CSR) based on a Poisson distribution. We then used 99% confidence envelopes based on the CSR randomizations to determine the spatial relationship between the two types of points. Observed Ripley’s K values (i.e., black line) that are outside the confidence envelope (in gray) indicate either spatial aggregation, when they are above the envelope, or spatial segregation when they fall below the envelope. Bivariate Ripley’s K-function was performed for each plot and year separately because both nest and chipmunk locations varied over time and space.
We then estimated chipmunk activity using the kernel density function (Dale and Fortin, 2014) for each plot and for each year. Kernel density function was computed using fixed Gaussian kernels to map hotspots of chipmunk detections at a 10 m resolution scale for an area of 1km × 1 km using the kde (kernel density estimation) function of the Hawthorne’s Geospatial Modelling Environment with ArcGIS. We used the estimated value of chipmunk activity at each nest for our analyses on daily nest survival rate.
Daily nest survival rate was calculated using logistic-exposure models (Shaffer, 2004) and binomial distribution. To determine whether chipmunk activity influenced fledging success of songbird nests, we modeled daily nest survival rate as a function of chipmunk activity (kernel value at the nest location), year, songbird species, plot, and their interactions (fixed factors) with generalized linear models. Year was included as a fixed factor in our models as previous research in the same study plots and during the same years showed strong fluctuations in daily nest survival rate in Ovenbirds, which was negatively correlated with an index of chipmunk activity.
In 2012, chipmunk activity peaked in plots A and B, so there were virtually no coldspots in chipmunk activity that birds could use to build their nests; in 2013, chipmunk activity was virtually non-existent (Fiola et al., 2017; also Figure 1). Hence, we reasoned that the benefit of nesting away from hotspots of chipmunk activity would be highest in years with intermediate chipmunk activity (2014, 2015) and therefore, we tested the same set of models for those years only. We used AICc to assess the fit of each model. Models were considered equivalent when ΔAICc < 2.0 (Burnham and Anderson, 2002). We obtained p-values for each fixed factor using likelihood ratio tests of the model with the fixed factor of interest against the model without the fixed factor of interest. Parameter estimation was achieved using residual maximum likelihood.
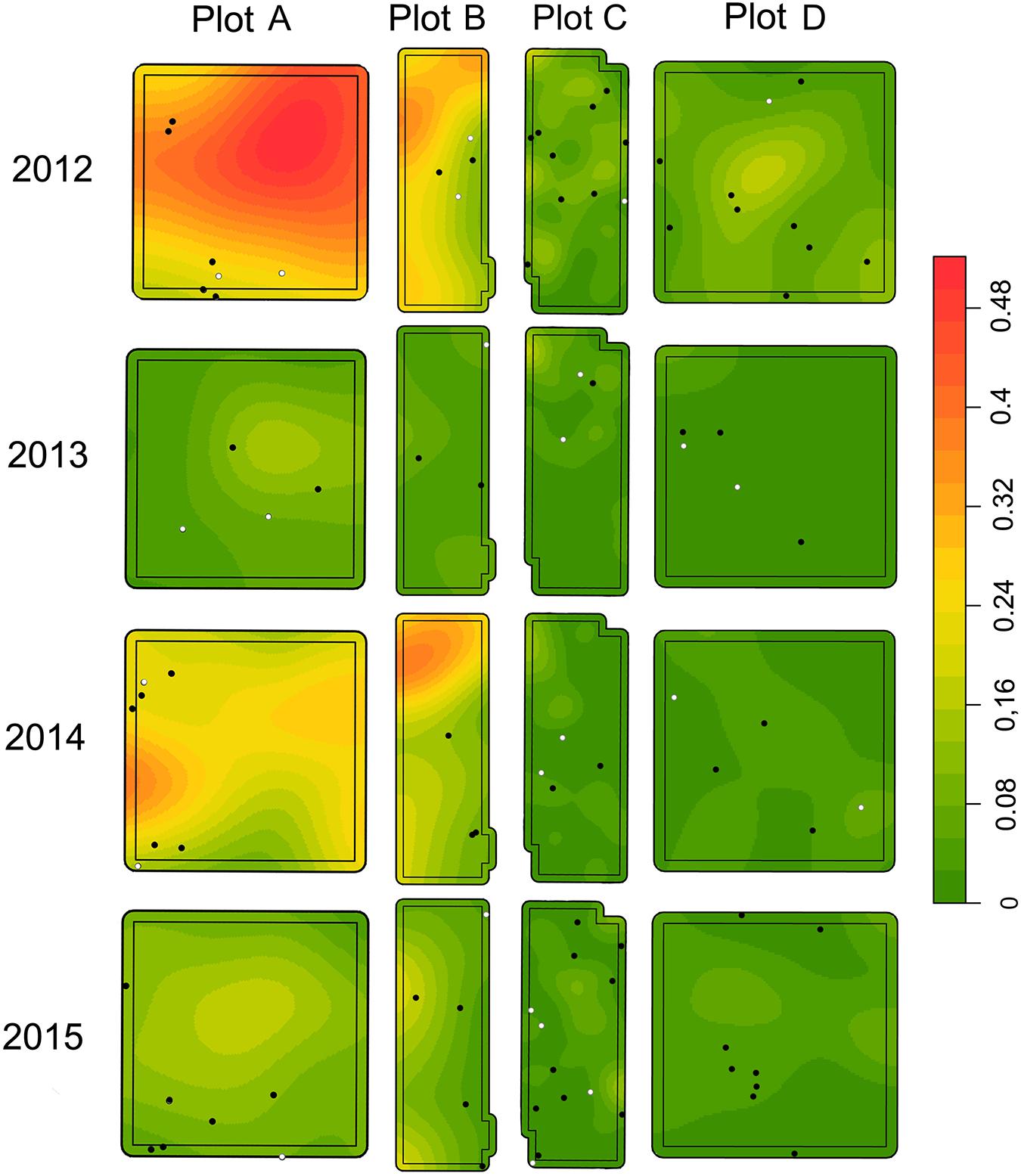
Figure 1. Spatiotemporal variability in Eastern Chipmunk activity. Kernel densities of eastern chipmunk detections (expressed as number of detections per hectare) in each study plot (25 ha) and year. Note that plot size was adjusted to allow direct comparisons. Black and white dots represent the locations of Ovenbird and Hermit Thrush nests, respectively.
Bivariate Ripley’s K statistics and generalized linear model were obtained with R version 3.2.2 (R Core Team, 2015) using spatstat (Baddeley and Turner, 2005) and AICcmodavg packages (Mazerolle, 2015).
Results
Over the four study years, there was considerable spatiotemporal heterogeneity in Eastern Chipmunk activity (Figure 1 and Supplementary Figure 1). The number of detections was highest in 2012, and consistently higher in one pair of study plots (A–B; Figure 1). Plots A and B were characterized by the presence of geophytes (Erythronium americanum, Dicentra canadensis, Claytonia caroliniana), whose rhizomes are consumed by Eastern Chipmunks (Careau et al., 2013). The nests of the two focal bird species were built further away from hotspots of chipmunk activity than expected by chance, but only in some plot-year combinations (Figure 2): in plots B and C (all years combined) and in plot A when Eastern Chipmunk activity was highest (2012). In the pair of plots that had a higher number of chipmunk detections (i.e., plots A and B), five out of 46 nests (10.9%) were built within the 50% isopleth of chipmunk activity, whereas in the pair of plots with the lower number of chipmunk detections (i.e., plots C and D), 24 out of 58 nests (41.4%) were built within the 50% isopleth of chipmunk activity (Figure 1 and Supplementary Figure 1).
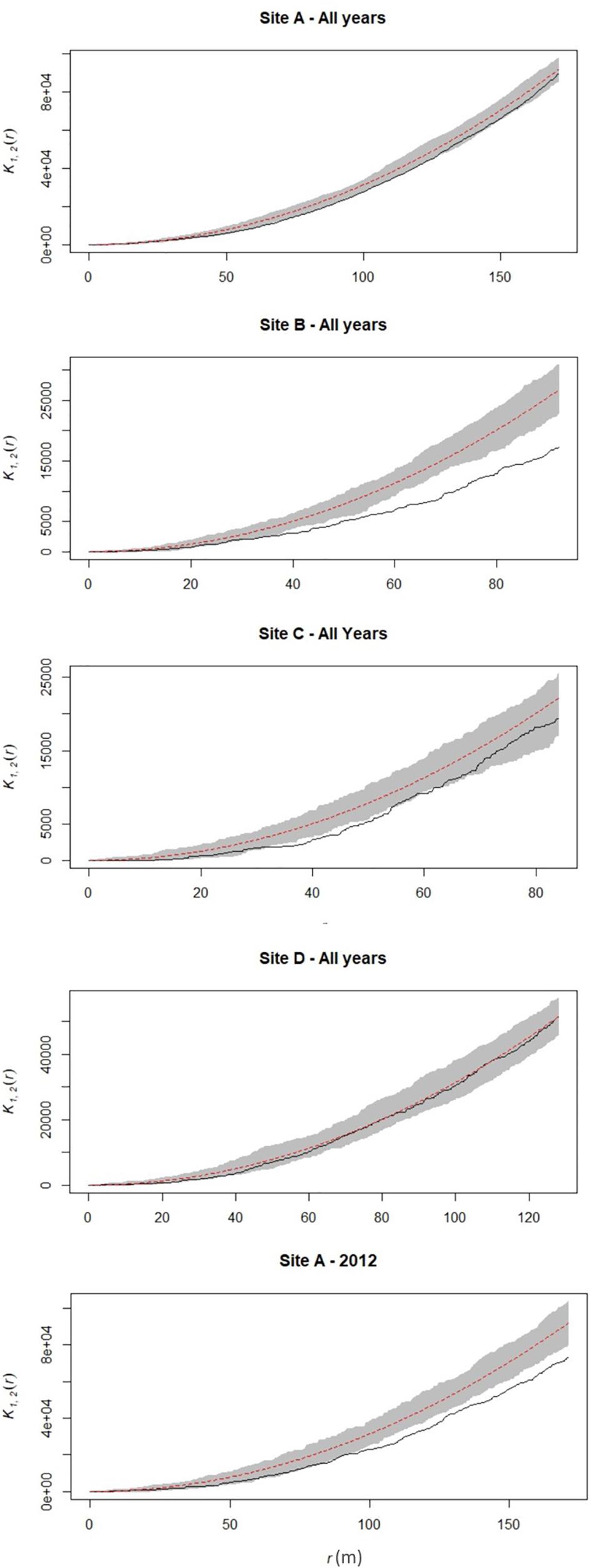
Figure 2. Spatial aggregation between the Eastern Chipmunk locations and the Hermit Thrush and Ovenbird nests. The gray 99% confidence envelope and red line indicate the null hypothesis of complete spatial randomness (CSR). The black line indicates the observed Bivariate Ripley K values against Euclidean distance r. When the black line is below the confidence envelope, it indicates a significant negative spatial aggregated pattern.
When considering all study years, the four best models predicting daily nest survival rate for the two focal songbird species included (1) the additive effects of chipmunk activity and songbird species (ΔAIC = 0.00, Model Weight = 0.30), (2) the effect of songbird species (ΔAIC = 0.19, Model Weight = 0.27), (3) the additive effects of songbird species and study plot (ΔAIC = 0.55, Model Weight = 0.23), and (4) the additive effects of chipmunk activity, songbird species, and study plot (ΔAIC = 0.16, Model Weight = 0.16; Supplementary Tables 2–6). When restricting the analysis to years of intermediate chipmunk activity, the best model included chipmunk activity, study plot and year (ΔAIC = 0.00, Model Weight = 0.67; Supplementary Tables 7–8). However, parameter estimate values for chipmunk activity indicated that this variable had a weak influence on daily nest survival rate (Supplementary Tables 3, 6) when considering all study years, and was only marginally non-significant during years with intermediate chipmunk activity (p = 0.056, Supplementary Table 8). Songbird species was the main factor influencing daily nest survival rate: Ovenbird nests tended to have a higher survival rate than those of Hermit Thrush (Supplementary Tables 3–6), possibly reflecting differences in nest detectability by chipmunks, the relative efficiency of nest defense, or both. During years characterized by intermediate chipmunk activity, daily nest survival rate was significantly lower in the study plot where chipmunk activity was highest (i.e., Plot A, p = 0.049). Across the two years, daily nest survival rate was higher in 2015 (p = 0.010, Supplementary Table 8).
Discussion
This study provides some evidence for the avoidance of a nest predator by songbird females in a natural environment, where cues of predator activity vary over space and time. Eastern Chipmunk activity was indeed highly dynamic, with a peak in 2012 and clear activity hotspots in high abundance years. Some of those hotspots were spatially persistent over time, probably reflecting the long-term use of specific burrow networks. In 2013, there were virtually no detections in most of our plots, even in those where Eastern Chipmunk activity had been very high the previous year (i.e., plots A and B). That year, the density of Sugar Maple seedlings was the lowest we recorded over the 2012–2015 period, suggesting a low seed crop the previous year (Fiola et al., 2017). Sugar Maple seeds are an important component of Eastern Chipmunk diet and during periods of mast failure, Eastern Chipmunks may skip reproduction and spend more time in their burrow relying on hoarded food accumulated in the previous year (Munro et al., 2008; Bergeron et al., 2011). This may explain the low activity level we recorded in 2013. In 2014 and 2015, both the density of Sugar Maple seeds and Eastern Chipmunk activity were intermediate between these two extremes (Fiola et al., 2017). This spatial heterogeneity in chipmunk activity provided opportunities for songbirds to build their nests in areas with lower predation risk. However, these areas are not risk-free as other nest predators have larger home ranges that encompass those of songbirds.
Our focal species of ground-nesting songbirds showed a tendency to avoid hotspots of Eastern Chipmunk activity, but only in plot-year combinations where chipmunk activity was relatively high. Additionally, most of the nests were built outside of the core areas of chipmunk activity, especially in plots with the highest chipmunk activity. This avoidance of chipmunk activity by Ovenbirds has been documented at lower spatial resolutions (Morton, 2005; Emmering et al., 2018) and experimentally tested using playbacks of chipmunk and control (frog) vocalizations (Emmering and Schmidt, 2011), but not at the fine resolution considered in the present study. In our study region, nest survival rates were exceedingly low in years of peak Eastern Chipmunk activity, but they remained high and relatively stable during years with low or intermediate activity (Fiola et al., 2017). Abundance of seed-eating nest predators such as Eastern Chipmunk and White-footed Mice (Peromyscus leucopus) are known to fluctuate sometimes across huge ecoregions (Haynes et al., 2009) in response to mast cycles (Schmidt et al., 2008; Haynes et al., 2009). Yet, despite wide fluctuations in nest predator abundance, behavioral responses to nest predation risk associated with Eastern Chipmunk did not appear to substantially reduce daily nest survival rate at the finer spatial resolution we considered in this study. Kelly et al. (2017) have reported similar results in Ovenbirds, and so did Bouffard et al. (2020) using artificial nests.
How can we explain the persistence of an anti-nest predator behavior in the absence of clear fitness benefits for the individuals involved? Because nest predation risk may vary dramatically between years through fluctuations in nest predator populations or activity (Fiola et al., 2017; see also Schmidt et al., 2008), the ability to assess the risk associated with Eastern Chipmunks may become irrelevant in an environment that is virtually free of them, as was the case in 2013 in our study area, or in plot-years where coldspots of Eastern Chipmunk activity are extremely restricted, as in plot A in 2012 (Figures 1, 3 and Supplementary Figure 1). Behavioral plasticity in nest site selection has been shown in Dusky Warblers (Phylloscopus fuscatus), individuals being more likely to choose safer nest sites, but only during years when Siberian Chipmunk (Tamias sibiricus) densities were high (Forstmeier and Weiss, 2004). Yet, the avoidance of hotspots of nest predator activity would not be expected to persist in a population unless females experience at least one year of intermediate to high Eastern Chipmunk activity during their lifetime, when there should be a fitness advantage to use this strategy. When nest predation risk reaches a peak, it must also be spatially heterogeneous so that females can find “safe havens” in which to build their nest. In our study area, Eastern Chipmunk activity varied by an order of magnitude between years and it peaked approximately every five years (Fiola et al., 2017). In our Ovenbird population, apparent survival rate was 2.7–2.9 years, with a maximum lifespan of at least 11 years (Vernouillet et al., 2014). As for Hermit Thrush, some individuals have been shown to reach at least 9 years of age (Dellinger et al., 2020). Hence, females of both focal species living at least 3 to 5 years would be expected to encounter years of high or intermediate Eastern Chipmunk activity during their lifetime.
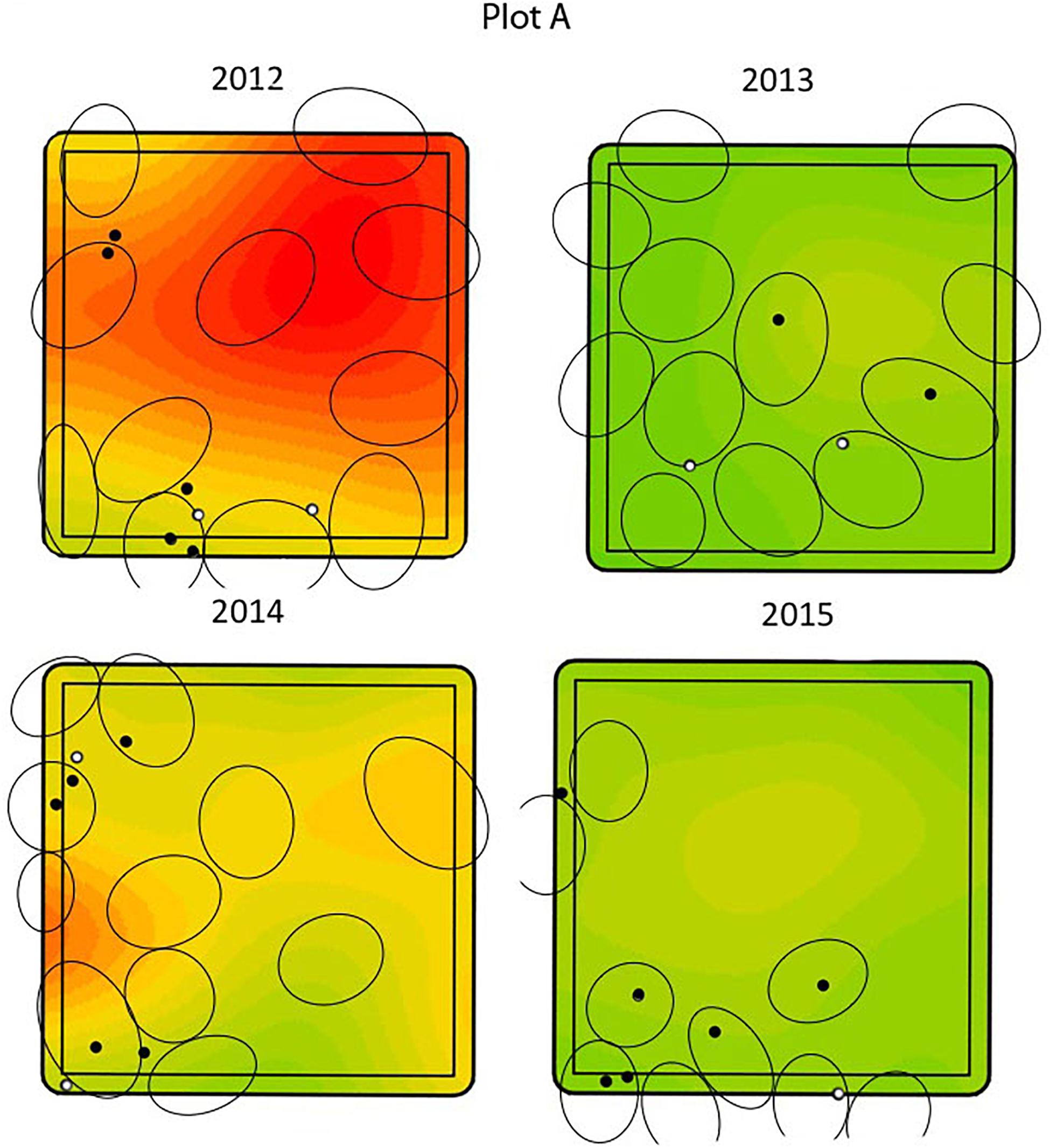
Figure 3. Distribution of Ovenbird territories, kernel densities of Eastern Chipmunk activity, and songbird nests found in study plot A. See Figure 1 for meaning of color coding. Ovenbird territory locations are shown by ellipses. Black dots represent Ovenbird nests and white dots, Hermit Thrush nests.
Avoidance of chipmunk activity hotspots may have persisted because it increases nest survival rate in years with intermediate chipmunk densities, but statistical power may have been too limited to detect such an effect. By pooling the nests of the two species of ground-nesting songbirds, we assumed that in spite of their body size difference [mean body mass: 30.1 g for Hermit Thrush (Dellinger et al., 2020); ca. 22 g for Ovenbird (Porneluzi et al., 2020)], they did not differ in their ability to defend their nests against Eastern Chipmunks. That assumption was based on video-monitoring data suggesting that nest defense by adult Ovenbirds and Hermit Thrushes against White-footed Mice is not successful (King and DeGraaf, 2006; Porneluzi et al., 2020). Yet, our results suggest otherwise. Bird species influenced daily nest survival rate, pointing toward differences in nest detectability by, or efficacy of nest defense against chipmunks (see also Emmering et al., 2018 for a comparison between Ovenbird and Veery nests, and Linder and Bollinger, 1995 for artificial Ovenbird-like nests). Ovenbird nests are shaped as a Dutch oven (Porneluzi et al., 2020), whereas Hermit Thrush build open-cup nests (Dellinger et al., 2020) that may be more easily detected by nest predators. Alternatively, Ovenbirds may be more efficient at selecting nest sites with lower nest predation risk than Hermit Thrushes (Schmidt and Ostfeld, 2003; Kelly et al., 2017). However, we did not have enough nests to evaluate avoidance of chipmunk activity for each bird species separately.
Unlike males, females may assess nest predation risk at two different spatial scales. First, upon their arrival from spring migration, when selecting a territory and a mate. Safer territories, i.e., those with a lower perceived nest predation risk, should be favored (Bonnington et al., 2015; Schmidt et al., 2015). Then, females can assess fine-scale nest predation risk and select a spatial refugium (coldspot of chipmunk activity), when available, before building their nest. In our study system, we suspect that females play the major role in nest predator avoidance, because complete avoidance of Eastern Chipmunk activity is unlikely at the scale of a male’s territory (Ovenbird: ca. 1 ha, Haché and Villard, 2010; Hermit Thrush: 0.7 ha, Dellinger et al., 2020; Figure 3).
Our results are broadly consistent with experimental evidence on songbird nest site selection as a function of nest predation risk (Eggers et al., 2006; Emmering and Schmidt, 2011), and are consistent with those of a field study (Emmering et al., 2018). Nest site selection may vary as a function of the main nest predator in the system. Female songbirds have been shown to “eavesdrop” on vocalizations of a nest predator and, in turn, to adjust the location of their nest (Emmering and Schmidt, 2011) or its degree of concealment by vegetation (Eggers et al., 2006) in response to perceived nest predation risk. Cues provided by nest predators, when available, may thus be an important component of nest site selection in songbirds.
A mismatch between nest predator abundance and actual nest predation risk has been reported elsewhere (Hollander et al., 2015; Kearns and Rodewald, 2017). For instance, at the scale of our study area, the survival rate of Ovenbird nests was negatively related to Eastern Chipmunk activity (Fiola et al., 2017), suggesting that eavesdropping on Eastern Chipmunk vocalizations can be potentially adaptive, even though we did not find evidence for this at the scale of our study plots. Activity indices have previously been used in studies investigating songbird nest survival rates (Schmidt et al., 2006; Hethcoat and Chalfoun, 2015). Our activity index may not have provided an accurate estimate of Eastern Chipmunk density, but our objective was to obtain a good approximation of the cues available to female songbirds when selecting their nest site.
In many fields of ecology, manipulative experiments are considered as the gold standard when attempting to isolate factors underlying observed phenomena. Here, we performed an observational study to examine a phenomenon previously described using manipulative experiments. We aimed to determine whether avoidance of nest predators by nest-building female songbirds could be observed in a system where access to public information is highly variable through space and time, in contrast with most manipulative experiments. Our results suggest that ground-nesting songbirds do indeed make use of the information provided by a nest predator. The fact that Eastern Chipmunks may depredate nests at all stages of nesting and even in the first days post-fledging might explain why nest-building females respond to the auditory and visual cues given by this predator. Such predator-prey dynamics may be taking place over very large spatial scales, as Eastern Chipmunks strongly respond to Sugar Maple mast-seeding events, at least where this tree species dominates the canopy (Fiola et al., 2017). Mast seed production by Sugar Maple appears to be spatially autocorrelated over a very large area ranging at least from our study area in New Brunswick to New Hampshire (Cleavitt and Fahey, 2017) and eastern Québec. Large-scale, spatially autocorrelated mast seeding has also been reported in a wide range of other deciduous and coniferous tree species and its influence of songbird productivity and predator-prey dynamics can be profound (e.g., Schmidt et al., 2008; Hill et al., 2019).
Data Availability Statement
All datasets generated for this study are included in the article/Supplementary Material.
Ethics Statement
The animal study was reviewed and approved by Simon Lamarre, president, Dept de biologie, Université de Moncton.
Author Contributions
M-AV and AV conceived the project. M-AV supervised the research. AV, M-LF, and M-AV designed the methods and performed the experiment. AV, M-LF, and M-JF conducted the statistical analyses. All authors wrote the manuscript.
Funding
This work was supported by grants from the Natural Sciences and Engineering Research Council of Canada (DG 2017-05102), New Brunswick Wildlife Trust Fund, and New Brunswick Innovation Foundation to M-AV., by a NSERC-J.D. Irving Ltd Industrial Postgraduate Scholarship to AV, and by NBIF-STGM and FESR scholarships to M-LF, as well as NSERC (DG 2019-5134) to M-JF.
Conflict of Interest
The authors declare that the research was conducted in the absence of any commercial or financial relationships that could be construed as a potential conflict of interest.
Acknowledgments
We thank all field assistants who participated in data collection and J. Boisvert for her help with Kernel analyses. M. Allard, M. J. Mazerolle, G. Moreau, D. Swain, and M. Desrochers provided valuable advice on statistical analyses. D. Kirk made helpful comments on the manuscript. We are also indebted to G. Adams, C. Michaud, P. Poitras, and B. Quirion (J.D. Irving Limited) for logistical support.
Supplementary Material
The Supplementary Material for this article can be found online at: https://www.frontiersin.org/articles/10.3389/fevo.2020.571456/full#supplementary-material
References
Baddeley, A., and Turner, R. (2005). spatstat: an R package for analyzing spatial point patterns. J. Stat. Soft. 12, 1–42.
Bergeron, P., Réale, D., Humphries, M. M., and Garant, D. (2011). Anticipation and tracking of pulsed resources drive population dynamics in eastern chipmunks. Ecology 92, 2027–2034. doi: 10.1890/11-0766.1
Bibby, C. J., Burgess, N. D., Hill, D. A., and Mustoe, S. H. (2000). Bird Census Techniques, 2nd Edn. London: Academic Press.
Bonnington, C., Gaston, K. J., and Evans, K. L. (2015). Ecological traps and behavioural adjustments of urban songbirds to fine-scale spatial variation in predator activity. Anim. Conserv. 18, 529–538. doi: 10.1111/acv.12206
Bouffard, J., Garant, D., and Bergeron, P. (2020). Dynamics of ground-nest egg depredation by rodents in a mixed-wood forest. Can. J. Zoo. 98, 47–54. doi: 10.1139/cjz-2019-0141
Bowers, M. A. (1995). Use of space and habitats by the Eastern Chipmunk, Tamias striatus. J. Mamm. 76, 12–21. doi: 10.2307/1382310
Burnham, K. P., and Anderson, D. R. (2002). Model Selection and Multimodel Inference: A Practical Information-Theoretic Approach, 2nd Edn. New York, NY: Springer.
Careau, V., Réale, D., Garant, D., Pelletier, F., Speakman, J. R., and Humphries, M. M. (2013). Context-dependent correlation between resting metabolic rate and daily energy expenditure in wild chipmunks. J. Exp. Biol. 216, 418–426. doi: 10.1242/jeb.076794
Chalfoun, A. D., and Martin, T. E. (2009). Habitat structure mediates predation risk for sedentary prey: experimental tests of alternative hypotheses. J. Anim. Ecol. 78, 497–503. doi: 10.1111/j.1365-2656.2008.01506.x
Clark, R., and Shutler, D. (1999). Avian habitat selection: pattern from process in nest-site use by ducks? Ecology 80, 272–287. doi: 10.1890/0012-9658(1999)080[0272:ahspfp]2.0.co;2
Cleavitt, N. L., and Fahey, T. J. (2017). Seed production of sugar maple and American beech in northern hardwood forests, New Hampshire, USA. Can. J. For. Res. 47, 985–990. doi: 10.1139/cjfr-2017-0096
Dale, M., and Fortin, M.-J. (2014). Spatial Analysis: A Guide for Ecologists, 2nd Edn. Cambridge: Cambridge University Press.
Dellinger, R., Wood, P. B., Jones, P. W., and Donovan, T. M. (2020). “Hermit Thrush, catharus guttatus, version 1.0,” in The Birds of the World, ed. A. F. Poole (Ithaca, NY: Cornell Laboratory of Ornithology), doi: 10.2173/bow.herthr.01
Dröge, E., Creel, S., Becker, M. S., and M’soka, J. (2017). Risky times and risky places interact to affect prey behaviour. Nat. Ecol. Evol. 1, 1123–1128. doi: 10.1038/s41559-017-0220-9
Dunford, C. (1972). Summer activity of eastern chipmunks. J. Mamm. 53, 176–180. doi: 10.2307/1378838
Eggers, S., Griesser, M., Nystrand, M., and Ekman, J. (2006). Predation risk induces changes in nest-site selection and clutch size in the Siberian Jay. Proc. Royal Soc. B 273, 701–706. doi: 10.1098/rspb.2005.3373
Emmering, Q. C., and Schmidt, K. A. (2011). Nesting songbirds assess spatial heterogeneity of predatory chipmunks by eavesdropping on their vocalizations. J. Anim. Ecol. 80, 1305–1312. doi: 10.1111/j.1365-2656.2011.01869.x
Emmering, Q. C., Kelly, J. K., Ostfeld, R. S., and Schmidt, K. A. (2018). Variation in coexisting birds to exploit spatial heterogeneity in small mammal activity. J. Av. Biol. 49:e01946.
Fiola, M.-L., Vernouillet, A., and Villard, M.-A. (2017). Linking songbird nest predation to seedling density: sugar maple masting as a resource pulse in a forest food web. Ecol. Evol. 7, 10733–10742. doi: 10.1002/ece3.3581
Fontaine, J. J., and Martin, T. E. (2006). Habitat selection responses of parents to offspring predation risk: an experimental test. Am. Nat. 168, 811–818. doi: 10.2307/4122252
Forstmeier, W., and Weiss, I. (2002). Effects of nest predation by the Siberian chipmunk Tamias sibiricus on success of the dusky warbler Phylloscopus fuscatus breeding. Zool. Z. 81, 1367–1370.
Forstmeier, W., and Weiss, I. (2004). Adaptive plasticity in nest-site selection in response to changing predation risk. Oikos 104, 487–499. doi: 10.1111/j.0030-1299.1999.12698.x
Germain, R. R., and Arcese, P. (2014). Distinguishing individual quality from habitat preference and quality in a territorial passerine. Ecology 95, 436–445. doi: 10.1890/13-0467.1
Haché, S., Bayne, E. M., and Villard, M.-A. (2014). Postharvest regeneration, sciurid abundance, and postfledging survival and movements in an Ovenbird population. Condor 116, 102–112. doi: 10.1650/condor-13-002-r2.1
Haché, S., and Villard, M.-A. (2010). Age-specific response of a migratory bird to an experimental alteration of its habitat. J. Anim. Ecol. 79, 897–905.
Hansson, B., Bensch, S., and Hasselquist, D. (2000). Patterns of nest predation contribute to polygyny in the great reed warbler. Ecology 81, 319–328. doi: 10.1890/0012-9658(2000)081[0319:ponpct]2.0.co;2
Haynes, K. J., Liehbold, A. M., Fearer, T. M., Wang, G., Norman, G. W., and Johnson, D. M. (2009). Spatial synchrony propagates through a forest food web via consumer-resource interactions. Ecology 90, 2974–2983. doi: 10.1890/08-1709.1
Hethcoat, M. G., and Chalfoun, A. D. (2015). Towards a mechanistic understanding of human-induced rapid environmental change: a case study linking energy development, nest predation and predators. J. Appl. Ecol. 52, 1492–1499. doi: 10.1111/1365-2664.12513
Hill, J. M., Lloyd, J. D., McFarland, K. P., and Rimmer, C. C. (2019). Apparent survival of a range-restricted montane forest bird species is influenced by weather throughout the annual cycle. Avian Conserv. Ecol. 14:16. doi: 10.5751/ACE-01462-140216
Hollander, F. A., Van Dyck, H., San Martin, G., and Titeux, N. (2011). Maladaptive habitat selection of a migratory passerine bird in a human-modified landscape. PLoS One 6:e25703. doi: 10.1371/journal.pone.0025703
Hollander, F. A., Van Dyck, H., San Martin, G., and Titeux, N. (2015). Nest predation deviates from nest predator abundance in an ecologically trapped bird. PLoS One 10:e0144098. doi: 10.1371/journal.pone.0144098
Holmes, R. T., Sherry, T. W., and Marra, P. P. (1996). Habitat-specific demography of breeding Black-throated Blue Warblers (Dendroica caerulescens): implications for population dynamics. J. Anim. Ecol. 65, 183–195. doi: 10.2307/5721
Kearns, L., and Rodewald, A. D. (2017). Does nest predator activity predict the location and survival of songbirds nests in urbanizing landscapes? Condor 119, 745–760. doi: 10.1650/condor-17-23.1
Kelly, J. K., Schmidt, K. A., and Ostfeld, R. S. (2017). Not all nesting guild members are alike: nest predators and conspecific abundance differentially influence nest survival in the ground-nesting Ovenbird (Seiurus aurocapilla) and Veery (Catharus fuscescens). Wilson J. Ornith. 129, 112–121. doi: 10.1676/1559-4491-129.1.112
King, D. I., and DeGraaf, R. M. (2006). Predators at bird nests in a northern hardwood forest in New Hampshire. J. Field Ornithol. 77, 239–243. doi: 10.1111/j.1557-9263.2006.00048.x
King, D. I., DeGraaf, R. M., Smith, M.-L., and Buonaccorsi, J. P. (2006). Habitat selection and habitat-specific survival of fledgling ovenbirds. J. Zool. 269, 414–421. doi: 10.1111/j.1469-7998.2006.00158.x
Lacher, T. E., and Mares, M. A. (1996). Availability of resources and use of space in eastern chipmunks. Tamias striatus. J. Mamm. 77, 833–849. doi: 10.2307/1382689
LaManna, J. A., Hemenway, A. B., Boccadori, V., and Martin, T. E. (2015). Bird species turnover is related to changing predation risk along a vegetation gradient. Ecology 96, 1670–1680. doi: 10.1890/14-1333.1
LaManna, J. A., and Martin, T. E. (2016). Costs of fear: behavioural and life-history responses to risk and their demographic consequences vary across species. Ecol. Lett. 19, 403–413. doi: 10.1111/ele.12573
Latif, Q. S., Heath, S. K., and Rotenberry, J. T. (2011). An ‘ecological trap’ for yellow warbler nest microhabitat selection. Oikos 120, 1139–1150. doi: 10.1111/j.1600-0706.2010.18835.x
Linder, E. T., and Bollinger, E. T. (1995). Depredation of artificial ovenbird nests in a forest patch. Wilson Bull. 107, 169–174.
Martin, T. E. (1992). “Breeding productivity considerations: what are the appropriate habitat features for management?,” in Ecology and Conservation of Neotropical Migrant Landbirds, eds J. M. Hagan and D. W. Johnston (Washington: Smithsonian Institution Press), 455–473.
Martin, T. E. (1998). Are microhabitat preferences of coexisting species under selection and adaptive? Ecology 79, 656–670. doi: 10.1890/0012-9658(1998)079[0656:ampocs]2.0.co;2
Martin, T. E., and Roper, J. J. (1988). Nest predation and nest-site selection of a western population of the Hermit Thrush. Condor 90, 51–57. doi: 10.2307/1368432
Mazerolle, M. J. (2015). AICcmodavg: Model Selection and Multimodel Inference Based on (Q)AIC(c). R Package Version 2.0-3. Available at: http://CRAN.Rproject.org/package=AICcmodavg (accessed August 6, 2020).
McKinnon, L., Smith, P. A., Nol, E., Martin, J. L., Doyle, F. I., Abraham, K. F., et al. (2010). Lower predation risk for migratory birds at high latitudes. Science 327, 326–327. doi: 10.1126/science.1183010
Morris, D. L., Faaborg, J., Washburn, B. E., and Millspaugh, J. J. (2015). Predator-induced renesting and reproductive effort in Indigo Buntings: more work for less pay? Conserv. Phys. 3:cou063. doi: 10.1093/conphys/cou063
Morton, E. S. (2005). Predation and variation in breeding habitat use in the Ovenbird, with special reference to breeding habitat selection in northwestern Pennsylvania. Wilson Ornith. Soc. 117, 327–335. doi: 10.1676/04-135.1
Munro, D., Thomas, D. W., and Humphries, H. H. (2008). Extreme suppression of aboveground activity by afood-storing hibernator, the eastern chipmunk (Tamias striatus). Can. J. Zool. 86, 364–370. doi: 10.1139/z08-008
Peluc, S. I., Sillett, T. S., Rotenberry, J. T., and Ghalambor, C. K. (2008). Adaptive phenotypic plasticity in an island songbird exposed to a novel predation risk. Behav. Ecol. 19, 830–835. doi: 10.1093/beheco/arn033
Porneluzi, P., Van Horne, M., and Donovan, T. M. (2020). “Ovenbird, Seiurus aurocapilla version 1.0,” in The Birds of the World, ed. A. F. Poole (Ithaca, NY: Cornell Laboratory of Ornithology), doi: 10.2173/bow.ovenbi1.01
Poulin, J.-F., Villard, M.-A., Edman, M., Goulet, P. J., and Ericksson, A.-M. (2008). Thresholds in nesting habitat requirements of an old forest specialist, the Brown Creeper (Certhia americana), as conservation targets. Biol. Conserv. 141, 1129–1137. doi: 10.1016/j.biocon.2008.02.012
Poulin, J.-F., Villard, M.-A., and Haché, S. (2010). Short-term demographic response of an old forest specialist to experimental selection harvesting. Ecoscience 17, 20–27. doi: 10.2980/17-1-3297
Preisser, E. L., Bolnik, D. I., and Benard, M. F. (2005). Scared to death? The effects of intimidation and consumption in predator-prey interactions. Ecology 86, 501–509. doi: 10.1890/04-0719
R Core Team (2015). R: A Language and Environment for Statistical Computing. Vienna: R Foundation for Statistical Computing.
Raybuck, D. W., Larkin, J. L., Stoleson, S. H., and Boves, T. J. (2020). Radio-tracking reveals insight into survival and dynamic habitat selection of fledgling Cerulean Warblers. Condor 122:duz063.
Saino, N., Ambrosini, R., Caprioli, M., Romano, A., Romano, M., Rubolini, D., et al. (2017). Sex-dependent carry-over effects on timing of reproduction and fecundity of a migratory bird. J. Anim. Ecol. 86, 239–249. doi: 10.1111/1365-2656.12625
Schmidt, K. A., Johansson, J., Kristensen, N., Massol, F., and Jonzén, N. (2015). Consequences of information use in breeding habitat selection on the evolution of settlement time. Oikos 124, 69–80. doi: 10.1111/oik.01483
Schmidt, K. A., and Ostfeld, R. S. (2003). Songbird populations in fluctuating environments: predator responses to pulsed resources. Ecology 84, 406–415. doi: 10.1890/0012-9658(2003)084[0406:spifep]2.0.co;2
Schmidt, K. A., Ostfeld, R. S., and Smyth, K. N. (2006). Spatial heterogeneity in predator activity, nest survivorship, and nest-site selection in two forest thrushes. Oecologia 148, 22–29. doi: 10.1007/s00442-005-0340-9
Schmidt, K. A., Rush, S. A., and Ostfeld, R. S. (2008). Wood thrush nest success and post-fledging survival across a temporal pulse of small mammal abundance in an oak forest. J. Anim. Ecol. 77, 830–837. doi: 10.1111/j.1365-2656.2008.01378.x
Shaffer, T. L. (2004). A unified approach to analyzing nest success. Auk 121, 526–540. doi: 10.1642/0004-8038(2004)121[0526:auatan]2.0.co;2
Streby, H. M., Refsnider, J. M., Peterson, S. M., and Anderson, D. E. (2014). Retirement investment theory explains patterns in songbird nest-site choice. Proc. Royal Soc. B 281:20131834. doi: 10.1098/rspb.2013.1834
Thériault, S., Villard, M.-A., and Haché, S. (2012). Habitat selection in site-faithful ovenbirds and recruits in the absence of experimental attraction. Behav. Ecol. 23, 1289–1295. doi: 10.1093/beheco/ars119
Vernouillet, A., Villard, M.-A., and Haché, S. (2014). ENSO, nest predation risk, food abundance, and male status fail to explain annual variation in the apparent survival rate of a migratory songbird. PLoS One 9:e113844. doi: 10.1371/journal.pone.0113844
Wilson, R. R., Hooten, M. B., Strobel, B. N., and Shivik, J. A. (2010). Accounting for individuals, uncertainty, and multiscale clustering in core area estimation. J. Wildl. Manag. 74, 1343–1352. doi: 10.1111/j.1937-2817.2010.tb01256.x
Zanette, L. (2011). Perceived predation risk reduces the number of offspring songbirds produce per year. Science 334, 1398–1401. doi: 10.1126/science.1210908
Keywords: avian productivity, behavioral response, landscapes of fear, resource pulses, northern hardwoods forest, mast-seeding events, predator–prey relationships
Citation: Vernouillet A, Fortin M-J, Fiola M-L and Villard M-A (2020) Do Female Songbirds Avoid a Mammalian Nest Predator When Selecting Their Nest Site? Front. Ecol. Evol. 8:571456. doi: 10.3389/fevo.2020.571456
Received: 10 June 2020; Accepted: 29 October 2020;
Published: 19 November 2020.
Edited by:
Guy Gilles Beauchamp, Independent Researcher, Montreal, CanadaReviewed by:
Chelsey Paquette, Bishop’s University, CanadaPatrick Bergeron, Bishop’s University, Canada
Copyright © 2020 Vernouillet, Fortin, Fiola and Villard. This is an open-access article distributed under the terms of the Creative Commons Attribution License (CC BY). The use, distribution or reproduction in other forums is permitted, provided the original author(s) and the copyright owner(s) are credited and that the original publication in this journal is cited, in accordance with accepted academic practice. No use, distribution or reproduction is permitted which does not comply with these terms.
*Correspondence: Marc-André Villard, bXZpbGxhcmRAbXRhLmNh