- 1Department of Bioscience, Faculty of Science and Technology, Aarhus University, Roskilde, Denmark
- 2Sovon Dutch Centre for Field Ornithology, Den Burg, Netherlands
- 3Department of Environmental and Marine Biology, Åbo Akademi University, Turku, Finland
- 4Novia University of Applied Sciences, Ekenäs, Finland
- 5Nature and Game Management Trust Finland, Degerby, Finland
- 6Utklippan Bird Station, Jämjö, Sweden
- 7Department of Bioscience, Aarhus University, Rønde, Denmark
Spatio-temporal variation in population dynamics of migratory populations is shaped by exposure to different environments during the annual cycle. Hence, exposure to similar environments should translate into synchrony in vital rates. Despite a wide-ranging breeding population, the Baltic/Wadden Sea flyway population of eiders (Somateria m. mollissima) shares wintering grounds in the southern Baltic Sea, inner Danish waters and the Wadden Sea; different colonies within this flyway population are therefore likely to exhibit some degree of synchrony in vital rates. Here we used capture-recapture-recovery data to investigate the impact of hunting, winter climate (the North-Atlantic Oscillation Index), winter temperature, nitrogen runoff, autumn-winter body condition of blue mussels Mytilus spp., natural predation and epidemic disease (avian cholera) on annual survival of adult females in ten study colonies distributed between Netherlands and Finland. Moreover, we tested how the degree of similarity in spatial winter distributions affected the degree of similarity in annual survival among colonies. None of the covariates universally affected female survival. While the quality of blue mussels in the wintering area explained almost 40% of the variation in survival of eiders breeding on Christiansø in the south-western Baltic Sea, incidence of epidemic disease explained >60% in two affected colonies. Furthermore, the spatial winter distribution did not appreciably influence annual survival rates in these 10 colonies. The lack of universal effects of spatial winter distribution and winter conditions on survival suggests that local breeding conditions may be more important, and hence prime targets for conservation efforts. Better monitoring of e.g., food quality, predation pressure and epidemic disease at the time of breeding could be the key to better understand the population dynamics in this endangered flyway population.
Introduction
Spatio-temporal variation in vital rates of migratory populations is driven by spatial heterogeneity and temporal fluctuations in the environment (Harris et al., 2005; Grosbois et al., 2009; Frederiksen et al., 2014). When environmental patterns operate at large spatial scales they are likely to cause a “Moran effect” on population growth due to their large-scale spatial autocorrelation of environmental attributes (Moran, 1953). As a consequence, similar patterns of variation in survival can be expected for animals that share wintering or breeding areas. Joint analysis of long-term demographic datasets from multiple sites thus constitutes an obvious potential for examining the importance of different environmental drivers in different sub-populations (Frederiksen et al., 2005). Knowledge about spatial variation in the environment is an important prerequisite for management as targeted actions can be better prioritized. Ideally, effective and sustainable management of migratory populations should apply empirical data to evaluate relevant natural and anthropogenic drivers causing population dynamics to change in space and time. Thorough evaluations of how potential drivers affect vital rates and thus population dynamics require both long-term demographic data as well as time series of temporally matching and ecologically relevant covariate data (Frederiksen et al., 2014). Long-term demographic data are in most cases either scarce or absent as they rely on continuous, long-term efforts, but are nevertheless available for some long-lived species of particular management interest such as seabirds. Moreover, single-site studies of demographic rates might fail to represent the larger population at a scale appropriate to management (Öst et al., 2016).
Populations of many migratory bird species are now being managed at the level of the flyway, i.e., a network of sub-populations with a partially or fully overlapping distribution during the staging or wintering period (Boere et al., 2006). In theory, the degree of auto-correlation in environmental conditions and range overlap affect the potential for environmental factors to synchronize local demographic rates (Lande et al., 1999; Engen et al., 2005). Across the range of a given flyway population some environmental drivers might either act to synchronize or de-synchronize vital rates by operating at different times during the annual cycle. For instance, partitioning of global- and local scale variation in annual survival of four Atlantic puffin (Fratercula arctica) colonies identified sea-surface temperature as an important driver at both the global and local-scale, causing synchrony as well as de-synchrony among the colonies (Grosbois et al., 2009). However, there are also examples of the same factor having a very different impact on different seabird populations, despite these birds being extremely wide-ranging. For instance, while the decline of one wandering albatross (Diomedea exulans) colony in the Indian Ocean could be ascribed to longline fishing, bycatch was not causing the decline in an Atlantic colony (Tuck et al., 2001). At a much smaller scale, neighboring populations of seabirds might as well express surprisingly different patterns of variation in demographic rates and ultimately in population dynamics (Öst et al., 2016). This was evident in a comparative demographic study of neighboring colonies of both European storm petrels (Hydrobates pelagicus) and balearic shearwaters (Puffinus mauretanicus) in the Mediterranean. In both species adult survival differed markedly between the two neighboring study sites presumably due to very local factors such as predation, small-scale habitat features, individual quality or age structure (Tavecchia et al., 2008). Given that effects of environmental factors can be highly variable in different sub-populations, successful flyway management should be based on joint or integrated information from several representative sites.
Throughout the past two decades, the declining Baltic/Wadden Sea flyway population of eiders has invoked attention and increased concern (e.g., Desholm et al., 2002; Ekroos et al., 2012a), recently warranting classification as endangered within the European Union (BirdLife International, 2015). Nonetheless, no studies so far have evaluated to what extent environmental conditions at the wintering or breeding areas affect survival in this flyway population. In contrast, potential mechanisms behind this large-scale decline have mostly been inferred from studies of single colonies. For instance, outbreaks of avian cholera have been suggested to significantly affect survival of nesting females in a Danish colony (Tjørnløv et al., 2013), whereas the decline of an eider colony in the south-western Baltic Sea has been linked to long-term changes in nutrients and mussel stocks (Laursen and Møller, 2014). In the western Gulf of Finland, survival of nesting females was considerably lower in open versus forested habitats, likely as a result of higher relative predation rates caused by white-tailed eagles (Haliaeetus albicilla) and American mink (Neovison vison) (Ekroos et al., 2012b). Interestingly, small-scale spatial variation in female survival in this area was found to cause the observed difference in growth rates of two large, geographically separated eider colonies (Öst et al., 2016).
Other studies have focused on the scale of regions. For instance, mass mortality of wintering eiders in the Dutch Wadden Sea has been linked to a reduction in sublittoral mussel stocks resulting from a declining nutrient supply and from overexploitation by commercial fisheries (Camphuysen et al., 2002; Ens, 2006). Mass mortality has also been reported from other regions. Based on observations of dead birds, Christensen et al. (1997) estimated that 35–90% of the female eiders breeding in five Danish colonies died during an avian cholera epizootic in 1996. Large-scale effects on breeding eider populations have also been recorded in the central and southern Baltic Sea. In 2008 an extensive spring bloom of the potentially toxic flagellate (Prymnesium polylepis) affected prey availability at pre-breeding foraging sites and caused extensive non-breeding in that year (Larsson et al., 2014). At the larger scale, several single-site studies have rejected winter climate, a large-scale and potentially synchronizing attribute of the environment, as a driver of variation in female survival, but this remains yet to be investigated at the level of multiple colonies of mixed geographical origin (Hario et al., 2009; Ekroos et al., 2012b; Tjørnløv et al., 2013). Finally, spring counts of eiders migrating into the Baltic Sea suggests that the flyway population sex ratio has shifted toward an increasing male bias (Lehikoinen et al., 2008; Berg, 2014; Ramula et al., 2018), which can mainly be attributed to sex-specific survival of prime reproductive-age individuals (≥5-year-old) during the breeding season (Ramula et al., 2018). An evaluation of hunting at the flyway scale suggests that in recent decades hunting has been sustainable and well-regulated (Tjørnløv et al., 2019), ultimately resulting in a ban on hunting females in Denmark (where most hunting takes place) from 2014 onward (Christensen and Hounisen, 2014).
The diversity of proposed explanations underlying the large-scale decline of the Baltic/Wadden Sea eider population hints toward influence of several factors operating at different spatial scales. Aiming to scale up, this study compiles an extensive long-term capture-recapture-recovery dataset including encounter histories of >22,000 adult females from ten different colonies along the flyway, and tests how the spatial winter distribution and a range of potential environmental drivers affect their rates of survival. We expected that similarity in winter distribution of the study colonies would translate into a similar pattern of annual variation in survival rates across colonies, synchronized by one or more covariates operating during the winter season. In addition, we expected colony-specific survival to be affected by de-synchronizing attributes of the environment operating at the scale of single colonies or regions.
Materials and Methods
Species and Study Area
The eider is a long-lived species of sea duck characterized by high rates of adult survival and low reproductive output. It has a wide northern hemisphere distribution (Goudie et al., 2000), separated into discrete flyway populations. These flyway populations typically share wintering grounds, whereas spring migration separates birds into minor units, i.e., local sub-populations (Desholm et al., 2002). After pair formation in late winter males follow their mates toward the breeding grounds and are thus widely dispersed, whereas females exhibit extreme fidelity to their natal colony (Greenwood, 1980).
Eiders winter in large flocks in shallow, coastal areas, feeding primarily on blue mussels Mytilus spp., other molluscs and crustaceans (Bagge et al., 1973). Spring migration toward the breeding sites is fueled by food resources collected on the wintering grounds and staging areas. At the breeding sites, females forage extensively until the early phase of egg-laying (Senechal et al., 2011; Hobson et al., 2015; Jaatinen et al., 2016). This implies that foraging conditions at both wintering and breeding sites contribute to sustain body condition of the females during incubation (Jaatinen et al., 2016). From the late phase of egg-laying until hatching of the young, females do not feed, but are known to regularly recess incubation, presumably in order to drink (Hellmann, 2017). The fasting of females during incubation results in an annual minimum of endogenous resources, which may compromise their immune and antioxidant defenses (Fenstad et al., 2016; Neggazi et al., 2016). In fact, even females in an apparently good body condition can fall victim to disease, such as avian cholera (Christensen et al., 1997). In addition, females are at their highest risk of predation during the incubation period, as they tend to remain on the nest, relying solely on their crypsis to avoid predators (Ekroos et al., 2012b).
Mark-Recapture-Recovery Data
We used long-term capture-recapture-recovery data from ten different eider colonies (Figure 1) belonging to the Baltic-Wadden Sea flyway population. Encounter histories were compiled and prepared in a Live-Dead format for Program MARK (White and Burnham, 1999; Cooch and White, 2014). For a combined analysis of these data, we prepared one joint dataset with all colonies included as separate groups. As computation time of the joint model was rather excessive, the potential effect of available drivers of variation in survival was tested within colony-specific models. In total, encounter histories of >22,000 eider females were compiled covering the period 1970–2015, i.e., 46 years (see Supplementary Material).
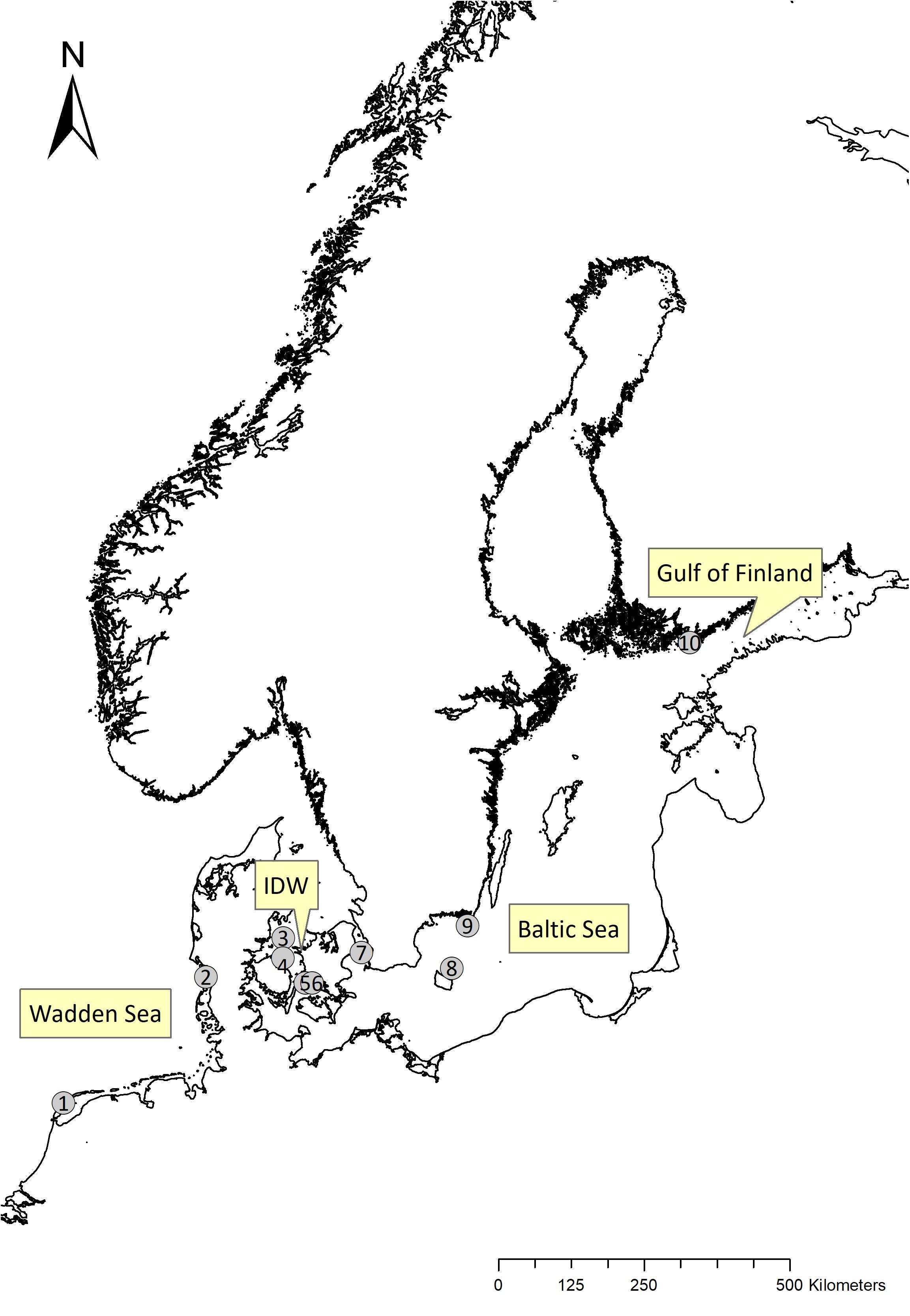
Figure 1. Study colonies with available long-term capture-recapture-recovery data on breeding female Eiders. 1: Vlieland (NL), 2: Mandø (DK), 3: Stavns Fjord (DK), 4: Hindsholm (DK), 5: Helleholm, Agersø (DK), 6: Næbbet, Stigsnæs (DK), 7: Saltholm (DK), 8: Christiansø (DK), 9: Utklippan (S), 10: Tvärminne (FIN). Important and highlighted sea areas: Wadden Sea, IDW (Inner Danish waters), Baltic Sea and Gulf of Finland.
Drivers of Variation in Female Survival
We focused mainly on potential drivers of variation in female survival in the ten study colonies operating at the largely shared wintering area around Denmark. We identified environmental and anthropogenic covariates that were likely to either directly or indirectly affect survival of female Eiders. We tested for an effect of winter climate, as represented by the December-March North Atlantic Oscillation (NAO) index (Hurrell, 1995) and winter water temperature (°C) measured in inner Danish waters [data from Hansen (2015)]. In the corresponding analysis for the females at Vlieland (colony 1, Figure 1), which do not migrate, we used winter water temperature data from Den Helder, Netherlands (SeaDataNet, 2018). Both covariates reflect winter severity, albeit at different spatial scales; winter severity may affect body mass, reproduction and recruitment of bivalves, the main prey of eiders (Honkoop and Beukema, 1997; van Beusekom et al., 2009; Carstensen et al., 2011; Waldeck and Larsson, 2013). For instance, Waldeck and Larsson (2013) showed in an experimental study that Baltic blue mussels exposed to an elevated temperature regime during winter had considerably lower body mass compared to mussels exposed to cold water. The reduced body mass of mussels exposed to elevated winter water temperatures was likely due to a negative energy balance, associated with feeding activity during a time of the year when physiological dormancy should be optimal (Waldeck and Larsson, 2013). In line with this hypothesis, a stable-isotope study on nutrient allocation for egg-production in Finnish female Eiders indicated more extensive use of endogenous resources following a cold winter, which is consistent with more favorable feeding conditions at the wintering grounds (Hobson et al., 2015).
We also tested for an effect of nitrogen run-off into Danish marine areas [data from Hansen (2015)], as phytoplankton and thus blue mussels rely on nitrogen for growth. Data from commercial fishery of mussels (Kristensen and Hoffmann, 2000; Laursen et al., 2009) also allowed direct testing of the effect of autumn-winter blue mussel body condition on annual female survival between 1997 and 2013. We used data from the Danish Wadden Sea to test for an effect of blue mussel body condition on survival at Vlieland, whereas for the other colonies we used annual averages over several widely distributed stations located within the wintering area. Furthermore, we tested the effect of hunting pressure estimated as a killrateh, i.e., the probability of dying due to hunting within a year (Tjørnløv et al., 2019). Moreover, we identified 1984, 1985, 1996, 2001, 2003, 2008, and 2009 as years with strikes of avian cholera, and tested how outbreaks of epidemic disease in a given colony affected survival of the females nesting there. In the western Gulf of Finland, we investigated the effect of predation, mainly induced by white-tailed eagles and American mink (Öst et al., 2018), on female survival. Annual predation indices were calculated as the total number of incubating females found killed by predators inside all the sub-colonies at Tvärminne, divided by the total number of nesting attempts in each year (including active, depredated and abandoned nests, and nests which had already hatched at the time of monitoring) (Öst et al., 2011; Ekroos et al., 2012b). Because vulnerability is likely to depend on vegetation cover, and female survival varies considerably between open and forested habitats in this colony (Ekroos et al., 2012b), we also computed annual habitat-specific indices in addition to the annual global indices.
While some of these factors affect survival instantaneously (hunting, predation and to some extent disease), other factors may operate at a much slower pace (winter climate, winter water temperature, nutrient runoff), either due to retention in the environment or due to the time it takes for these effects to cascade up to higher trophic levels. This encouraged us to also test for a 1-year lagged effect of these potentially slow-pace drivers.
Statistical Analysis
We used the Burnham model parameterization implemented in Program MARK (White and Burnham, 1999) to estimate probabilities of survival (S), recapture (p), recovery (r), and site fidelity (F). Survival (S) is the probability of true annual survival, recapture (p) is the probability of recapturing an individual, given that it is alive and has not left the sampling area permanently, recovery (r) is the combined probability that a dead individual is recovered and its ring reported, and site fidelity (F) is the probability of remaining in the sampling area given that the bird remains alive. Moreover, the site fidelity parameter (F) is also affected by heterogeneity in the recapture probability (p) which is commonly found in mark-recapture data on nesting eiders (Hario et al., 2009; Tjørnløv et al., 2013). Most importantly, including recoveries in the analysis of annual survival can be estimated without bias due to emigration (true survival). Due to the mixing of birds in wintering areas, we specified models with common recovery probabilities across sites. In addition, we also specified models with a different probability of recovery for birds from Vlieland, as we speculated these to be influenced by long-term, systematic beached bird surveys in the Dutch Wadden Sea (Camphuysen and Heubeck, 2001). Finally, site fidelity (F) was assumed constant and either similar or different between colonies. Akaike’s Information Criterion adjusted for small sample sizes (AICc) was used to select between candidate models (Burnham and Anderson, 2002). Potential violations of model assumptions were evaluated by means of the Median c-hat overdispersion coefficient estimated for the most general model. An estimate of annual variation in survival was derived by means of the variance components procedure in MARK, which decomposes the total variance into process (sigma2) and sampling variance (Gould and Nichols, 1998).
For simplicity, we tested effects of covariates within colony-specific capture-recapture-recovery models rather than within the common framework. Exceptions were the two colonies at Hindsholm and Næbbet (colony 4 and 6, Figure 1), as these datasets by themselves did not contain enough information to reliably estimate all required parameters. Effects of covariates on survival at Hindsholm were tested within the joint capture-recapture-recovery model, whereas Næbbet was combined with the closely located colony of Helleholm (colony 5, Figure 1).
The effect of covariates on female survival was tested using analysis of deviance (Skalski et al., 1993; Grosbois et al., 2008) implemented in Program MARK. Testing was performed exclusively on standardized covariates (mean = 0, SD = 1) which facilitated a direct comparison of effect sizes. To avoid the risk of erroneous inferences due to multiple comparisons, we corrected p-values for multiple testing using the function “p.adjust,” method “holm” implemented in the R package “stats.”
Relationship Between Survival and Location of Wintering Site
We assigned recoveries to one of seven wintering areas (1) south North Sea, (2) central North Sea, (3) north North Sea, (4) north Kattegat, (5) south Kattegat, (6) west Baltic Sea, (7) east Baltic Sea. From the proportion of recoveries from each colony found in each area, we calculated a distance matrix of Bray-Curtis dissimilarities between study colonies (Bray and Curtis, 1957). Similar distance matrices were computed with respect to survival over three different time intervals (1975–1981, 1985–2002, and 1997–2011) during which survival estimates from at least three colonies were available. Finally, we used a Mantel test to test the relationship between choice of wintering area and survival (Legendre and Legendre, 1998). This test did not account for potentially different recovery probabilities in the seven wintering areas.
Results
Fully time-dependent capture-recapture-recovery models resulted in issues of parameter identifiability. We therefore constrained either recovery rates (r), site fidelity rates (F), or both, and formulated a set of reduced candidate models.
Over-dispersion of the most general joint capture-recapture-recovery model including all study colonies was modest (median c-hat ± 95% CI = 1.23 ± 0.03). Quasi Akaike’s Information Criterion corrected for small sample sizes (QAICc) selected a model with time-dependent and colony-specific survival (S) and recapture (p) probabilities, a common linear trend across populations on the rate of recovery (r) (except Vlieland which was set constant over time), and colony-specific and constant rates of site fidelity (F). AICc weights indicated that this model was clearly better than all other candidate models (Table 1) and received all the support (AICc weight = 1).

Table 1. Model selection of the set of joint capture-recapture-recovery candidate models, where g × t is the interaction between group (g) i.e., colony and time (t), T is a linear trend over time and VL is the abbreviation for Vlieland.
As suggested by our most parsimonious model adult female survival was highly variable between study colonies and over years (Figure 2).
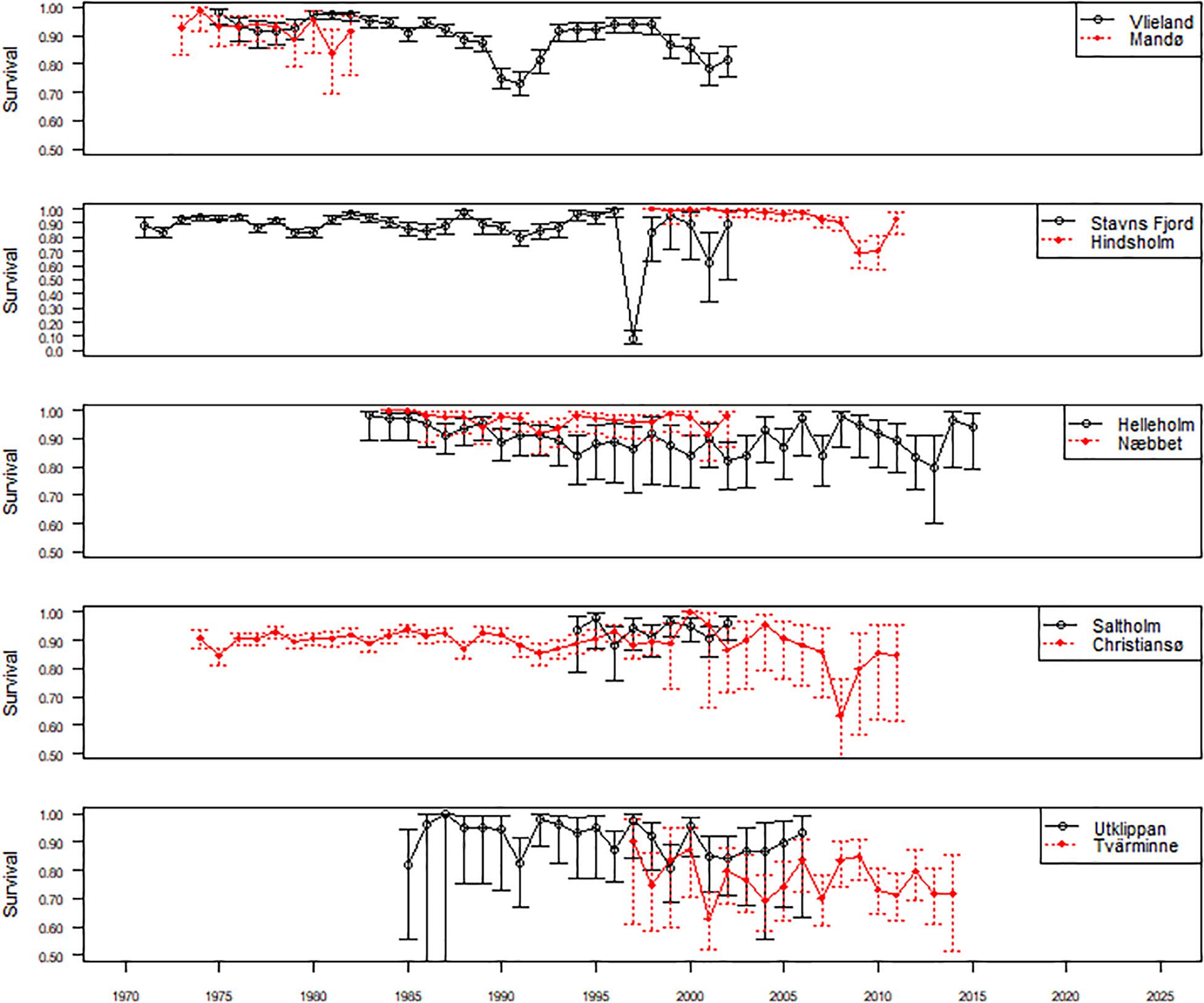
Figure 2. Colony-specific estimates of adult female survival from the most parsimonious joint capture-recapture-recovery model i.e., S(g × t) p(g × t) r(T, VL) F(g).
In the Dutch Wadden Sea, female survival was >0.92 in most years but somewhat lower between the late 1980s and early 1990s and again from the late 1990s to the early 2000s. At Mandø (colony 2, Figure 1) in the Danish Wadden Sea (early 1970s to early 1980s), female survival was marginally higher i.e., >0.93 and remarkably stable. There was, however, considerable inter-colony variation in survival of different breeding populations in the inner Danish waters. At Stavns Fjord (colony 3, Figure 1), year-to-year variation in survival was high (Table 2), driven by a considerable drop in survival from 1996 to 1997 and a minor drop from 2001 to 2002. At Hindsholm, a major drop in survival lasted for two consecutive years (2008–2010). Mean survival of the two closely located colonies Helleholm (colony 5, Figure 1) and Næbbet off south-western Zealand was 0.90 and 0.97, respectively. Likewise, mean survival was high (0.94) and extremely stable at Saltholm in Øresund between Denmark and Sweden (colony 7, Figure 1). In the south-western Baltic Sea at Christiansø and Utklippan (colony 8 and 9, Figure 1), survival was also high but dropped to 0.68 at Christiansø in 2008. Interestingly, further north-east at Tvärminne in the Gulf of Finland (colony 10, Figure 1), mean survival was only 0.77, which is considerably lower than at any of the other study colonies.
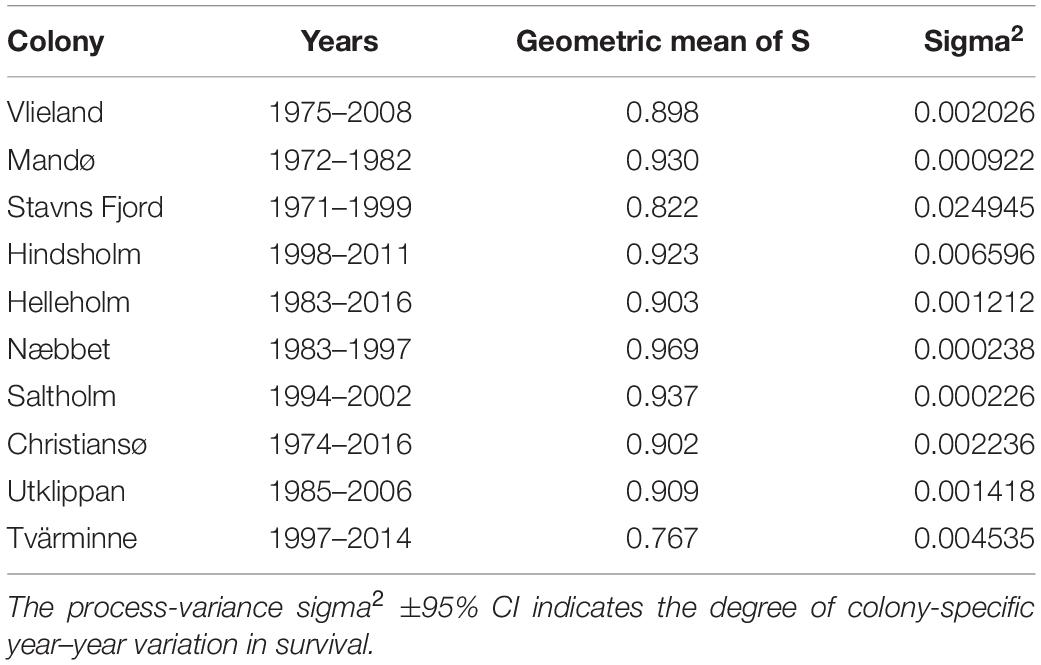
Table 2. Colonies, time series of survival of adult eider females estimated by colony-specific capture-recapture-recovery models and their geometric means.
The recovery rate (r) for the breeding population at Vlieland was estimated as 0.26, while it for the other populations declined over time (1971–2016) from 0.34 to 0.08. The probability of a breeder to stay at the same colony, i.e., the site fidelity (F), ranged between 0.86 and 0.99 with a mean ± sd of 0.95 ± 0.04.
Effects of Environmental Covariates
None of the tested environmental covariates had a universal effect on survival across colonies (see Supplementary Material for all results of effect sizes). Rather, we found effects to be predominantly colony-specific. For instance, survival in the Vlieland colony in the Dutch Wadden Sea was negatively affected by local winter water temperature in the previous year (effect size = −0.46 ± 0.07, R2 = 0.38, p = 0.001, Figure 3). At two sites in inner Danish waters, major drops in survival coincided with outbreaks of avian cholera: at Stavns Fjord in 1996 (effect size = −0.86 ± 0.18, R2 = 0.61, p = 0.001) and at Hindsholm in 2008 and 2009 (effect size = −0.85 ± 0.14, R2 = 0.75, p = 0.001), respectively.
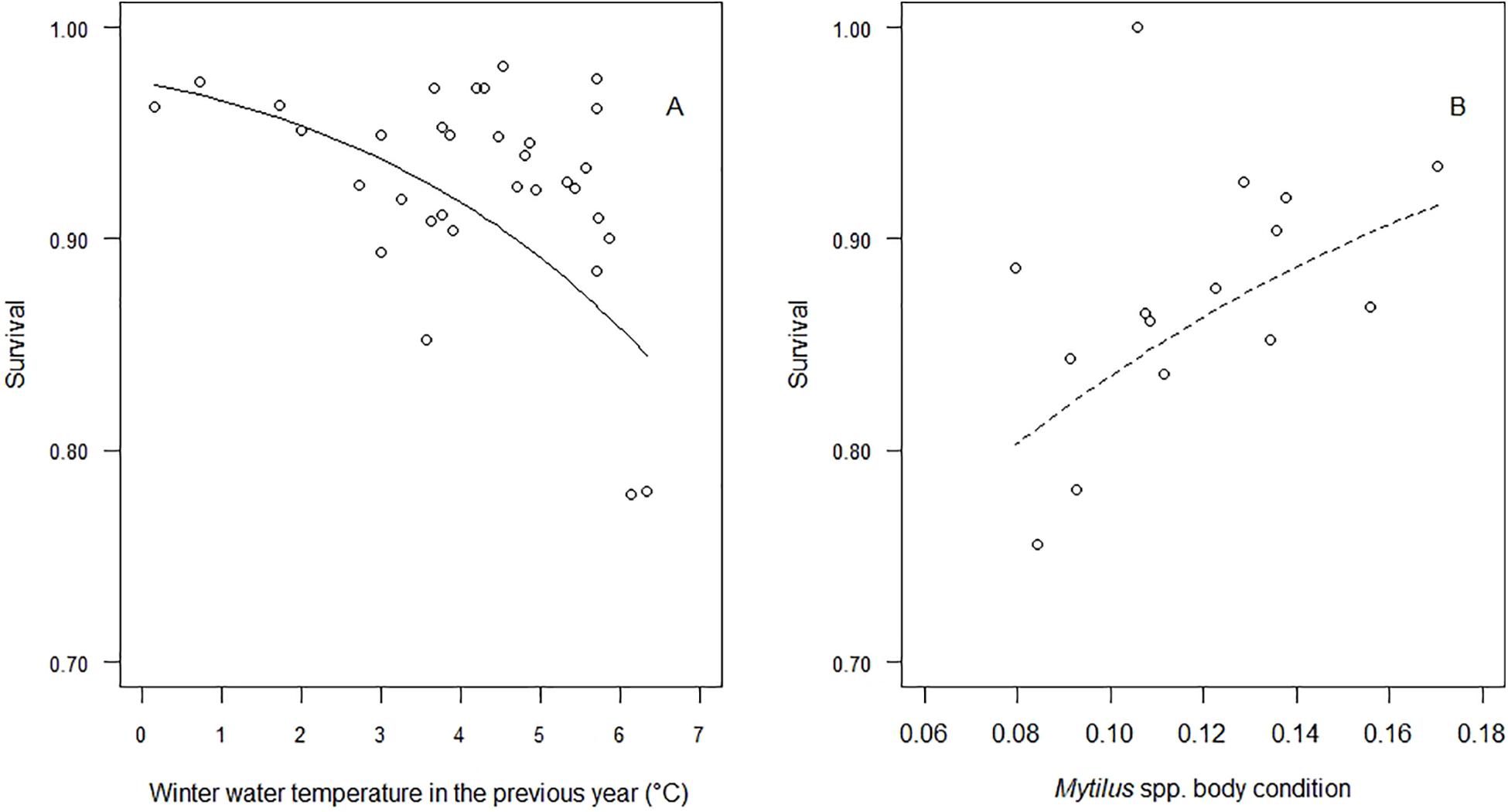
Figure 3. Relationship between environmental covariates and annual survival of female eiders nesting in the Vlieland (panel A) and Christiansø (panel B) colonies. Winter water temperature is from the station den Helder, Netherlands, whereas the autumn-winter body condition of blue mussels is averaged over several stations across the flyway wintering area.
Moreover, winter NAO in the previous year and in the same year, respectively, positively affected survival at Stavns Fjord (1-year delayed effect, effect size = 0.52 ± 0.07, R2 = 0.34, p = 0.01) and Saltholm (direct effect, effect size = 0.47 ± 0.26, R2 = 0.76, p = 0.04).
Finally, we found an effect of blue mussel body condition on survival of females nesting at Christiansø in the southwestern Baltic Sea but significance could not be retained when correcting for multiple testing (effect size = 0.27 ± 0.14, R2 = 0.39, p = 0.093, Figure 3).
Effects of Wintering Area
Similarities in survival among colonies were not related to similarities in spatial winter distribution (Figure 4, dissimilarity matrices in Supplementary Material) in any of the three time periods (Early period: mantel r < 0, p > 0.33, Middle period: mantel r < 0, p > 0.48, Late period: mantel r > 0, p > 0.35).
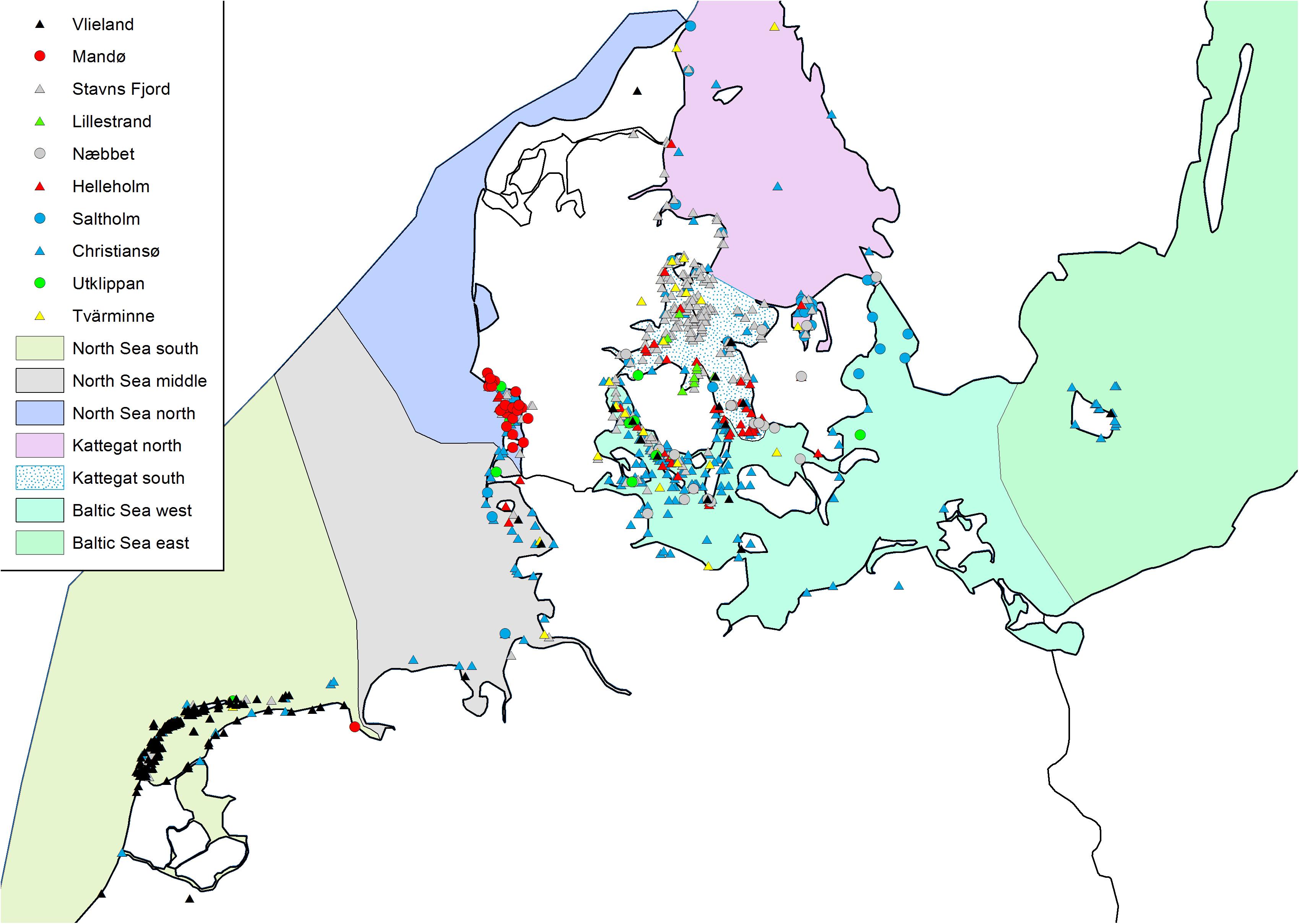
Figure 4. Location of winter (November–February) recoveries of dead female eiders ringed at the study colonies and recovered during the years 1970–2015. The proportion of recoveries in each defined area was used to test for an effect of spatial winter distribution on female survival.
Discussion
We showed that female survival in this flyway population was driven by global as well as local-scale year-to-year variation in the environment, and that variation in survival was not related to the use of a specific wintering location. Perhaps the most salient finding was that none of the tested covariates had a universal effect on survival across study colonies, despite the high overlap in winter distribution. In contrast, we found that effects varied considerably even between closely located colonies. This finding suggests that there may be no universal driver of variation in female survival operating in the largely shared wintering area, suggesting a higher importance of environmental conditions at the breeding grounds. In addition, annual variation in survival was generally modest except in colonies with confirmed outbreaks of epidemic disease.
Interestingly, however, female survival of the northernmost Eider colony at Tvärminne, western Gulf of Finland, was markedly lower in comparison to the other colonies, which is in line with another study from the same colony (Ekroos et al., 2012b). The consistently lower female survival at Tvärminne is driven by high predation rates, as discussed below.
In the Dutch Wadden Sea, annual variation in survival of the largely resident Eider population at Vlieland was driven by local winter temperature in the previous year. A likely ecological explanation behind this pattern is a bottom-up effect of winter temperature on bivalves, the most important prey of eiders. Experimental studies have shown that mild winter temperatures result in a faster over-winter decline of the flesh content of key prey-species e.g., blue mussels Mytilus spp. and the common cockle Cerastoderma edule followed by a slower growth in the following spring (Honkoop and Beukema, 1997; Waldeck and Larsson, 2013). Surprisingly, we did not find evidence of a direct effect of blue mussel body condition on female survival at Vlieland. Another consequence of exceptionally mild winters is the reproductive failure of bivalves resulting in shrinkage of sublittoral mussel stocks, the most important food source for diving ducks (Beukema et al., 1993). Baltic Sea blue mussels have also been shown to suffer from warmer and less saline waters (Westerbom et al., 2019) both of which are predicted outcomes of ongoing climate change (Meier et al., 2012). In the Dutch Wadden Sea, a study by Ens (2006) found a significant negative relationship between the sublittoral stock of mussels and the number of dead Eiders reported by systematic beached bird surveys. We did, however, not see a similar effect when tested against formally estimated survival rates of females breeding at Vlieland.
Mass mortality events caused by avian cholera has occurred in multiple Danish colonies in different years since the mid 1990s. A strong outbreak in 1996 wiped out most of the colony in Stavns Fjord, which was also hit by a minor outbreak in 2001 (Christensen et al., 1997; Pedersen et al., 2003). During late winter and early spring 1996, large numbers of dead Eiders washed ashore along the coasts of inner Danish waters. In addition, up to 95% of all nesting females in Stavns Fjord were later found dead on their nests or around drinking pools. Mass mortality in 1996 was also reported from five other colonies in the region and bacteriological analysis of necropsies confirmed avian cholera (Pasteurella multocida) as the cause of death of both wintering and breeding birds (Christensen et al., 1997). However, extension of the time series beyond 1998 was only feasible within the joint capture-recapture-recovery model and hence, we did not formally test for an effect on survival of epidemic disease in 2001. However, the low estimate of survival from 2001 to 2002 suggests that mortality was higher than usual (Figure 2). Although the bacterium should not be able to persist in the wild for very long (Blanchong et al., 2006), isolates of P. multocida from these two outbreaks were identical suggesting that the same bacteriological strain had survived and remained undetected for several years (Pedersen et al., 2003). Another outbreak of avian cholera in 2008 and 2009 further south in the colony of Hindsholm is also the likely result of disease transmission from chronically infected survivors from earlier outbreaks. In fact, the first documented incidence of avian cholera in Netherlands in 1984 was followed by a presumed avian cholera outbreak at Hov Røn in inner Danish waters in 1985 (Christensen et al., 1997). This series of epidemic events occurring at a higher frequency in recent years and mainly affecting breeding females might constitute a serious threat to a population, which has become increasingly male-biased (Lehikoinen et al., 2008; Ramula et al., 2018). An aggravating circumstance is the periodic depletion of body reserves, which is likely to affect immunological resistance and mutual proximity, for instance, when sharing drinking pools becomes a critical feature for the vulnerable females. As a result of the recent population decline lower densities in the colonies may cause less susceptibility to disease and may entail a positive population effect through compensations in adult female survival. However, the most recent comprehensive survey of the Baltic/Wadden Sea flyway population showed that total breeding numbers had dropped throughout the flyway population since 2000, this was not the case for Denmark and Germany (Ekroos et al., 2012a).
The effect of winter NAO, as a measure of winter climatic conditions, on survival was not universal, but two Danish colonies expressed a positive effect. A diverse survival response to winter climatic conditions in Arctic and sub-Arctic Eider populations have recently been ascribed to differences in migratory tactics (Guéry et al., 2017). While a direct survival response to winter NAO was observed in two migratory populations, the effect was delayed by 2–3 years in a sedentary population. Given the diverse migratory behavior of our ten study colonies, ranging from nearly resident to partly and fully migratory, a heterogeneous survival response to winter climate is in line with the findings of Guéry et al. (2017).
In the south-western Baltic Sea, female survival in the Christiansø colony was positively related to the autumn-winter body condition of blue mussels. After conservative adjustment to account for multiple testing, the effect was, however, no longer statistically significant. Nonetheless, an otherwise clear relationship (Figure 3) is likely disturbed by an influential outlier in 1 year when survival was estimated as 1. The observed relationship suggests that body condition of the main prey in autumn-winter positively affects body condition of female eiders and ultimately their ability to survive from 1 year to the next. When body condition of blue mussels is low, Eiders have to dive more frequently in order to consume the same amount of energy. Presumably, the observed negative effect of winter water temperature in the previous year on survival in the Dutch Wadden Sea is mediated through a similar pathway, i.e., through condition of the prey affecting the predator. Although the concentration of nutrients in the marine environment has been suggested to control population size in the colony of Christiansø (Laursen and Møller, 2014), presumably through a similar mechanism, our results cannot confirm such an effect, at least not when it comes to adult female survival.
At Tvärminne, western Gulf of Finland, female survival was consistently lower compared to the other colonies. If this colony is spatially representative, consistently lower survival in this region could have very large effects at the flyway scale as Finland hosts >70% of the entire flyway breeding population (Desholm et al., 2002). However, at Söderskär further east in the Gulf of Finland, survival was markedly higher than at Tvärminne (Hario et al., 2009) and comparable to the other colonies included in our study. The primary cause for the lower survival at Tvärminne is likely more intense predation on incubating females (Ekroos et al., 2012b). In contrast, Söderskär is situated farther away from the core distribution area of the white-tailed eagle in Finland but since survival estimates are not available since 2007 a recent comparison in survival between the two colonies is not feasible. Surprisingly, there was no evidence that female survival had declined over time at Tvärminne, despite a recent analysis reporting 14% and 11% annual increases over the past 23 years in predation-induced mortality caused by white-tailed eagles and American mink, respectively (Öst et al., 2018).
While intriguing at first sight, this result must be evaluated against behavioral and physiological adaptations of female Eiders exposed to increasing predation risk. Thus, there has been a steep increase in the incidence of skipping breeding based on extensive monitoring of color-ringed individuals in this colony, suggesting that breeding propensity has decreased from ca 95% in 2004 to only ca 54% in 2016 (Öst et al., 2018). This response is characteristic of long-lived species and implies that females promote their own survival at the expense of current reproduction under high predation regimes. Therefore, whennon-breeding is frequent, the observed proportion of killed females at their nests is likely to overestimate the true proportion of killed individuals of the entire breeding pool. Furthermore, the shift toward increasing non-breeding at Tvärminne has been accompanied by a concomitant increase in the mean body condition among the females that do breed (Öst et al., 2018). This result implies that the individuals opting to breed under the currently prevailing unfavorable conditions (high predation risk) are on average of higher quality. Good body condition of female Eiders should translate into better survival prospects, as previously shown in the Tvärminne colony (Ekroos et al., 2012b).
Despite the large number of study colonies with long-term demographic data, availability of temporally overlapping and relevant covariate data was found to be limiting. Longer time series of temporally matching data would have contributed to a more robust effect-analysis of potential drivers of variation in female survival. Systematic collection of data on pre-breeding feeding conditions near the colonies and breeding-related incidences of predation and disease seem relevant foci for future monitoring efforts.
Conclusion
In conclusion, our results indicate that female survival in this flyway population is significantly affected by attributes of the environment at both wintering and breeding grounds. Drivers of annual variation in female survival were largely colony-specific and related to either feeding conditions during winter or to epidemic disease. In addition, consistently lower survival at Tvärminne in the Gulf of Finland relative to the other colonies is presumably driven by more intense predation in this colony. The lack of universal effects strongly suggests that inter-colony variation should be addressed in future management of this flyway population of eiders. Ideally, within an adaptive management framework, continuous evaluations of the influence of environmental drivers at different spatial scales might be one of the keys to successful conservation.
Data Availability Statement
The raw data supporting the conclusions of this article will be made available by the authors, without undue reservation.
Ethics Statement
Ethical review and approval was not required for the animal study because bird ringing is exempt from animal welfare legislation in the countries in which the research took place.
Author Contributions
RT and MF conceived the study. RT developed the statistical models with input from MF. RT collated the mark-recapture data from numerous contributors including BE, MÖ, KJ, PK, RL, and TC. RT analyzed the data and led the writing of the manuscript. All authors contributed critically to the drafts and gave final approval for publication.
Funding
MÖ was funded by the Swedish Cultural Foundation in Finland. KJ was supported by a grant by Sophie von Julins Foundation to the Nature and Game Management Trust Finland.
Conflict of Interest
PK was employed as Academy Research Fellow (Academy of Finland project 309992).
The remaining authors declare that the research was conducted in the absence of any commercial or financial relationships that could be construed as a potential conflict of interest.
Acknowledgments
This study could not have been achieved without the great long-term efforts of the many volunteers, students, and researchers engaged in local monitoring schemes who have kindly made their data available to us. In particular, we wish to thank a number of deceased people who have made an invaluable contribution to this study by ringing and recapturing eiders in the colonies over an impressive period of time: Peter Lyngs and Niels-Erik Franzmann (Christiansø), Henning Noer (Stavns Fjord and Saltholm), Lars Hansen (Hindsholm), and Kees Swennen (Vlieland). We also wish to thank several ringing offices for their collaboration and contribution with data; Copenhagen Bird Ringing Centre, Swedish Bird Ringing Centre, Stockholm, Finnish Museum of Natural History, Helsinki, and Vogeltrekstation, Arnhem, Netherlands. Last but not least, we want to thank the Danish Nature Agency and 15. Juni Fonden for funding this research.
Supplementary Material
The Supplementary Material for this article can be found online at: https://www.frontiersin.org/articles/10.3389/fevo.2020.566154/full#supplementary-material
References
Bagge, P., Lemmetyinen, R., and Raitis, T. (1973). Spring food of some diving waterfowl in southwestern Finnish archipelago. Oikos 15, 146–150.
Berg, P. (2014). The ratio of females and immature males among migrating Common Eiders Somateria mollissima in southern Denmark, in spring 2013 and 2014. Dansk Ornitol. Foren. Tidsskrift 2014, 207–213.
Beukema, J. J., Essink, K., Michaelis, H., and Zwarts, L. (1993). Year-to-year variability in the biomass of macrobenthic animals on tidal flats of the Wadden Sea - how predictable is this food source for birds. Netherlands J. Sea Res. 31, 319–330. doi: 10.1016/0077-7579(93)90051-s
BirdLife International (2015). European Red List of Birds. Luxembourg: Office for Official Publications of the European Communities.
Blanchong, J. A., Samuel, M. D., Goldberg, D. R., Shadduck, D. J., and Lehr, M. A. (2006). Persistence of Pasteurella multocida in wetlands following avian cholera outbreaks. J. Wildl. Dis. 42, 33–39. doi: 10.7589/0090-3558-42.1.33
Boere, G. C., Galbraith, C. A., and Stroud, D. A. (eds) (2006). Waterbirds Around the World. Edinburgh: The Stationery Office.
Bray, J. R., and Curtis, J. T. (1957). An ordination of the upland forest communities of southern Wisconsin. Ecol. Monogr. 27, 326–349.
Burnham, K. P., and Anderson, D. R. (2002). Model Selection and Multimodel Inference. A Practical Information-Theoretic Approach, 2nd Edn. New York, NY: Springer.
Camphuysen, C. J., Berrevoets, C. M., Cremers, H., Dekinga, A., Dekker, R., Ens, B. J., et al. (2002). Mass mortality of common eiders (Somateria mollissima) in the Dutch Wadden Sea, winter 1999/2000: starvation in a commercially exploited wetland of international importance. Biol. Conserv. 106, 303–317. doi: 10.1016/s0006-3207(01)00256-7
Camphuysen, C. J., and Heubeck, M. (2001). Marine oil pollution and beached bird surveys: the development of a sensitive monitoring instrument. Environ. Pollut. 112, 443–461. doi: 10.1016/s0269-7491(00)00138-x
Carstensen, J., Sanchez-Camacho, M., Duarte, C. M., Krause-Jensen, D., and Marba, N. (2011). Connecting the dots: responses of coastal ecosystems to changing nutrient concentrations. Environ. Sci. Technol. 45, 9122–9132. doi: 10.1021/es202351y
Christensen, T. K., Bregnballe, T., Andersen, T. H., and Dietz, H. H. (1997). Outbreak of Pasteurellosis among wintering and breeding common eiders Somateria mollissima in Denmark. Wildlife Biol. 3, 125–128. doi: 10.2981/wlb.1997.008
Christensen, T. K., and Hounisen, J. P. (2014). Managing hunted populations through sex-specific season lengths: a case of the Common Eider in the Baltic-Wadden Sea flyway population. Eur. J. Wildlife Res. 60, 717–726. doi: 10.1007/s10344-014-0840-1
Cooch, E. G., and White, G. C. (2014). Program MARK - A Gentle Introduction. Fort Collins, CO: Colorado State University.
Desholm, M., Christensen, T. K., Scheiffarth, G., Hario, M., Andersson, Å, Ens, B., et al. (2002). Status of the Baltic/Wadden sea population of the common eider Somateria m. mollissima. Wildfowl 53, 167–203.
Ekroos, J., Fox, A. D., Christensen, T. K., Petersen, I. K., Kilpi, M., Jonsson, J. E., et al. (2012a). Declines amongst breeding Eider Somateria mollissima numbers in the Baltic/Wadden Sea flyway. Ornis Fennica 89, 81–90.
Ekroos, J., Öst, M., Karell, P., Jaatinen, K., and Kilpi, M. (2012b). Philopatric predisposition to predation-induced ecological traps: habitat-dependent mortality of breeding eiders. Oecologia 170, 979–986. doi: 10.1007/s00442-012-2378-9
Engen, S., Lande, R., Sæther, B., and Bregnballe, T. (2005). Estimating the pattern of synchrony in fluctuating populations. J. Anim. Ecol. 74, 601–611. doi: 10.1111/j.1365-2656.2005.00942.x
Ens, B. J. (2006). “The conflict between shellfisheries and migratory waterbirds in the Dutch Wadden Sea,” in Waterbirds Around the World, eds G. C. Boere, C. A. Galbraith, and D. A. Stroud (Edingburgh: The Stationary Office), 806–811.
Fenstad, A. A., Moody, A. J., Öst, M., Jaatinen, K., Bustnes, J. O., Moe, B., et al. (2016). Antioxidant responses in relation to persistent organic pollutants and metals in a low- and a high-exposure population of Seabirds. Environ. Sci. Technol. 50, 4817–4825. doi: 10.1021/acs.est.6b00478
Frederiksen, M., Harris, M. P., and Wanless, S. (2005). Inter-population variation in demographic parameters: a neglected subject? Oikos 111, 209–214. doi: 10.1111/j.0030-1299.2005.13746.x
Frederiksen, M., Lebreton, J. D., Pradel, R., Choquet, R., and Gimenez, O. (2014). Identifying links between vital rates and environment: a toolbox for the applied ecologist. J. Appl. Ecol. 51, 71–81. doi: 10.1111/1365-2664.12172
Goudie, R. I., Robertson, G. J., and Reed, A. (2000). “Common eider (Somateria mollissima),” in The Birds of North America, eds A. Poole and F. Gill (Philadelphia: Birds of North America), 31.
Gould, W. R., and Nichols, J. D. (1998). Estimation of temporal variability of survival in animal populations. Ecology 79, 2531–2538. doi: 10.1890/0012-9658(1998)079[2531:eotvos]2.0.co;2
Greenwood, P. J. (1980). Mating systems, philopatry and dispersal in birds and mammals. Anim. Behav. 28, 1140–1162. doi: 10.1016/s0003-3472(80)80103-5
Grosbois, V., Gimenez, O., Gaillard, J. M., Pradel, R., Barbraud, C., Clobert, J., et al. (2008). Assessing the impact of climate variation on survival in vertebrate populations. Biol. Rev. 83, 357–399. doi: 10.1111/j.1469-185x.2008.00047.x
Grosbois, V., Harris, M. P., Anker-Nilssen, T., McCleery, R. H., Shaw, D. N., Morgan, B. J. T., et al. (2009). Modeling survival at multi-population scales using mark-recapture data. Ecology 90, 2922–2932. doi: 10.1890/08-1657.1
Guéry, L., Descamps, S., Pradel, R., Hanssen, S. A., Erikstad, K. E., Gabrielsen, G. W., et al. (2017). Hidden survival heterogeneity of three Common eider populations in response to climate fluctuations. J. Anim. Ecol. 86, 683–693. doi: 10.1111/1365-2656.12643
Hario, M., Mazerolle, M. J., and Saurola, P. (2009). Survival of female common eiders Somateria m. mollissima in a declining population of the northern Baltic Sea. Oecologia 159:747. doi: 10.1007/s00442-008-1265-x
Harris, M. P., Anker-Nilssen, T., McCleery, R. H., Erikstad, K. E., Shaw, D. N., and Grosbois, V. (2005). Effect of wintering area and climate on the survival of adult Atlantic puffins Fratercula arctica in the eastern Atlantic. Mar. Ecol. Prog. Ser. 297, 283–296. doi: 10.3354/meps297283
Hellmann, J. (2017). Haahkanaaraan (Somateria mollissima) Pitkäaikaisen Paaston Fysiologiset Vaikutukset. MSc thesis, University of Helsinki, Helsinki.
Hobson, K. A., Jaatinen, K., and Öst, M. (2015). Differential contributions of endogenous and exogenous nutrients to egg components in wild Baltic Common Eiders (Somateria mollissima): a test of alternative stable isotope approaches. Auk 132, 624–633. doi: 10.1642/auk-14-294.1
Honkoop, P. J. C., and Beukema, J. J. (1997). Loss of body mass in winter in three intertidal bivalve species: an experimental and observational study of the interacting effects between water temperature, feeding time and feeding behaviour. J. Exp. Mar. Biol. Ecol. 212, 277–297. doi: 10.1016/s0022-0981(96)02757-8
Hurrell, J. W. (1995). Decadal trends in the North Atlantic Oscillation: regional temperatures and precipitation. Science 269, 676–679. doi: 10.1126/science.269.5224.676
Jaatinen, K., Öst, M., and Hobson, K. A. (2016). State-dependent capital and income breeding: a novel approach to evaluating individual strategies with stable isotopes. Front. Zool. 13:24. doi: 10.1186/s12983-016-0157-x
Kristensen, P. S., and Hoffmann, E. (2000). Fishery for Blue Mussels in Danish waters 1989–1999, 72-00. Charlottenlund: Danish Fishery Research Institute.
Lande, R., Engen, S., and Sæther, B. (1999). Spatial scale of population synchrony: environmental correlation versus dispersal and density regulation. Am. Nat. 154, 271–281. doi: 10.2307/2463650
Larsson, K., Hajdu, S., Kilpi, M., Larsson, R., Leito, A., and Lyngs, P. (2014). Effects of an extensive Prymnesium polylepis bloom on breeding eiders in the Baltic Sea. J. Sea Res. 88, 21–28. doi: 10.1016/j.seares.2013.12.017
Laursen, K., Asferg, K. S., Frikke, J., and Sunde, P. (2009). Mussel fishery affects diet and reduces body condition of Eiders Somateria mollissima in the Wadden Sea. J. Sea Res. 62, 22–30. doi: 10.1016/j.seares.2009.02.004
Laursen, K., and Møller, A. P. (2014). Long-term changes in nutrients and mussel stocks are related to numbers of breeding eiders somateria mollissima at a large baltic colony. PLoS One 9:e0095851. doi: 10.1371/journal.pone.0095851
Legendre, P., and Legendre, L. (1998). Numerical Ecology. Second English edition. Amsterdam: Elsevier.
Lehikoinen, A., Christensen, T. K., Öst, M., Kilpi, M., Saurola, P., and Vattulainen, A. (2008). Large-scale change in the sex ratio of a declining eider Somateria mollissima population. Wildlife Biol. 14, 288–301. doi: 10.2981/0909-6396(2008)14[288:lcitsr]2.0.co;2
Meier, H. E. M., Andersson, H. C., Arheimer, B., Blenckner, T., Chubarenko, B., Donnelly, C., et al. (2012). Comparing reconstructed past variations and future projections of the Baltic Sea ecosystem-first results from multi-model ensemble simulations. Environ. Res. Lett. 7:034005. doi: 10.1088/1748-9326/7/3/034005
Moran, P. A. P. (1953). The statistical analysis of the Canadian Lynx cycle.2. Synchronization and meteorology. Austral. J Zool. 1, 291–298. doi: 10.1071/zo9530291
Neggazi, S. A., Noreikiene, K., Öst, M., and Jaatinen, K. (2016). Reproductive investment is connected to innate immunity in a long-lived animal. Oecologia 182, 347–356. doi: 10.1007/s00442-016-3657-7
Öst, M., Lehikoinen, A., Jaatinen, K., and Kilpi, M. (2011). Causes and consequences of fine-scale breeding dispersal in a female-philopatric species. Oecologia 166, 327–336. doi: 10.1007/s00442-010-1855-2
Öst, M., Lindén, A., Karell, P., Ramula, S., and Kilpi, M. (2018). To breed or not to breed: drivers of intermittent breeding in a seabird under increasing predation risk and male bias. Oecologia 188, 129–138. doi: 10.1007/s00442-018-4176-5
Öst, M., Ramula, S., Lindén, A., Karell, P., and Kilpi, M. (2016). Small-scale spatial and temporal variation in the demographic processes underlaying the large-scale decline of eiders in the Baltic Sea. Population Ecol. 58, 121–133. doi: 10.1007/s10144-015-0517-y
Pedersen, K., Dietz, H. H., Jorgensen, J. C., Christensen, T. K., Bregnballe, T., and Andersen, T. H. (2003). Pasteurella multocida from outbreaks of avian cholera in wild and captive birds in Denmark. J. Wildl. Dis. 39, 808–816. doi: 10.7589/0090-3558-39.4.808
Ramula, S., Öst, M., Linden, A., Karell, P., and Kilpi, M. (2018). Increased male bias in eider ducks can be explained by sex-specific survival of prime-age breeders. PLoS One 13:e0195415. doi: 10.1371/journal.pone.0195415
SeaDataNet (2018). Pan-European Infrastructure for Ocean and Marine Data Management. London: SeaDataNet.
Senechal, E., Bety, J., Gilchrist, H. G., Hobson, K. A., and Jamieson, S. E. (2011). Do purely capital layers exist among flying birds? Evidence of exogenous contribution to arctic-nesting common eider eggs. Oecologia 165, 593–604. doi: 10.1007/s00442-010-1853-4
Skalski, J. R., Hoffmann, A., and Smith, S. G. (1993). “Testing the significance of individual- and cohort-level covariates in animal survival studies,” in Marked Individuals in the Study of Bird Population, eds J.-D. Lebreton and P. M. North (Basel: Birkhäuser Verlag).
Tavecchia, G., Minguez, E., Leon, D., Louzao, M., and Oroi, D. (2008). Living close, doing differently: small-scale asynchrony in demography of two species of seabirds. Ecology 89, 77–85. doi: 10.1890/06-0326.1
Tjørnløv, R. S., Humaidan, J., and Frederiksen, M. (2013). Impacts of avian cholera on survival of common eiders Somateria mollissima in a danish colony. Bird Study 60, 321–326. doi: 10.1080/00063657.2013.798261
Tjørnløv, R. S., Pradel, R., Choquet, R., Christensen, T. K., and Frederiksen, M. (2019). Consequences of past and present harvest management in a declining flyway population of common eiders Somateria mollissima. Ecol. Evol. 9, 12515–12530. doi: 10.1002/ece3.5707
Tuck, G. N., Polacheck, T., Croxall, J. P., and Weimerskirch, H. (2001). Modelling the impact of fishery by-catches on albatross populations. J. Appl. Ecol. 38, 1182–1196. doi: 10.1046/j.0021-8901.2001.00661.x
van Beusekom, J. E. E., Loebl, M., and Martens, P. (2009). Distant riverine nutrient supply and local temperature drive the long-term phytoplankton development in a temperate coastal basin. J. Sea Res. 61, 26–33. doi: 10.1016/j.seares.2008.06.005
Waldeck, P., and Larsson, K. (2013). Effects of winter water temperature on mass loss in Baltic blue mussels: implications for foraging sea ducks. J. Exp. Mar. Biol. Ecol. 444, 24–30. doi: 10.1016/j.jembe.2013.03.007
Westerbom, M., Mustonen, O., Jaatinen, K., Kilpi, M., and Norkko, A. (2019). Population dynamics at the range margin: implications of climate change on sublittoral blue mussels (Mytilus trossulus). Front. Mar. Sci. 6:292. doi: 10.3389/fmars.2019.00292
Keywords: female survival, capture-recapture, environmental covariate, spatiotemporal variation, wintering area, colony-specific
Citation: Tjørnløv RS, Ens BJ, Öst M, Jaatinen K, Karell P, Larsson R, Christensen TK and Frederiksen M (2020) Drivers of Spatiotemporal Variation in Survival in a Flyway Population: A Multi-Colony Study. Front. Ecol. Evol. 8:566154. doi: 10.3389/fevo.2020.566154
Received: 27 May 2020; Accepted: 17 September 2020;
Published: 15 October 2020.
Edited by:
Umesh Srinivasan, Princeton University, United StatesReviewed by:
Marcelo Bertellotti, Centro Para el Estudio de Sistemas Marinos (CONICET), ArgentinaAttila D. Sándor, University of Agricultural Sciences and Veterinary Medicine of Cluj-Napoca, Romania
Copyright © 2020 Tjørnløv, Ens, Öst, Jaatinen, Karell, Larsson, Christensen and Frederiksen. This is an open-access article distributed under the terms of the Creative Commons Attribution License (CC BY). The use, distribution or reproduction in other forums is permitted, provided the original author(s) and the copyright owner(s) are credited and that the original publication in this journal is cited, in accordance with accepted academic practice. No use, distribution or reproduction is permitted which does not comply with these terms.
*Correspondence: Rune S. Tjørnløv, cnN0QGJpb3MuYXUuZGs=