- 1Research Group Insect-Fungus Symbioses, Department of Animal Ecology and Tropical Biology, University of Würzburg, Würzburg, Germany
- 2Chair of Forest Entomology and Protection, University of Freiburg, Freiburg, Germany
- 3Southern Research Station, USDA Forest Service, Pineville, LA, United States
Fungus-farming is known from attine ants, macrotermites, and ambrosia beetles (Scolytinae, Platypodinae). Farming ant and termite societies are superorganismal and grow fungal cultivars in monocultures. Social organization of ambrosia beetle groups and their farming systems are poorly studied, because of their enigmatic life within tunnel systems inside of wood. Ambrosia beetle-fungus symbioses evolved many times independently in both the beetles and their fungal cultivars. Observations suggest that there is evolutionary convergence between these lineages, but also a high variation in the degree of sociality and the modes of fungiculture. Using a laboratory observation technique, I here tried to give insights into the social system and fungus symbiosis of the sugar-cane borer, Xyleborus affinis Eichhoff (Scolytinae: Curculionidae), a currently poorly studied ambrosia beetle. The study revealed a cooperatively breeding system characterized by delayed dispersal of adult daughters, alloparental brood care by larvae and adults, and about half of the totipotent adult daughters laying eggs within the natal nest. Most interesting, there was a tendency of egg-laying females to engage more commonly in mutually beneficial behaviors than non-egg-layers. Fungus gardens covering gallery walls composed of five different filamentous fungi. A Raffaelea isolate was predominant and together with an unidentified fungus likely served as the main food for adults and larvae. Three isolates, a Mucor, a Fusarium and a Phaeoacremonium isolate were most abundant in the oldest gallery part close to the entrance; Mucor, Fusarium and the Raffaelea isolate in diseased individuals. Additionally, there was correlative evidence for some fungal isoaltes influencing beetle feeding and hygienic behaviors. Overall, X. affinis is now the second ambrosia beetle that can be classified as a cooperative breeder with division of labor among and between adults and larvae.
Introduction
Advanced fungus agriculture by insects has evolved once in ants, once in termites and more than a dozen times independently in wood-boring weevils (Mueller et al., 2005; Hulcr and Stelinski, 2017; Vanderpool et al., 2018; Biedermann and Vega, 2020). The latter are jointly termed ambrosia beetles, even though they are a polyphyletic group and their mutualisms with fungi evolved multiple times independently (in both beetles and fungi; Hulcr and Stelinski, 2017) and every beetle-fungus combination may have a different farming system (Harrington, 2005; Six, 2012; Hulcr and Stelinski, 2017; Biedermann and Vega, 2020). In the best studied ambrosia beetle lineage, the Xyleborini (Curculionidae: Scolytinae), fungus-farming evolved along with social behavior and in some cases division of labor between and within developmental stages and adults (Mueller et al., 2005; De Fine Licht and Biedermann, 2012; Biedermann and Rohlfs, 2017; Nuotclá et al., 2019; Biedermann and Vega, 2020). At least one Xyleborini species classifies as cooperatively breeding (Xyleborinus saxesenii; Biedermann and Taborsky, 2011; Biedermann et al., 2012), which is defined in ambrosia beetles by adult daughters delaying dispersal from the natal nest and engaging in in hygienic brood care and fungus-farming tasks, while the mother is still present and breeding (Peer and Taborsky, 2007; Biedermann and Taborsky, 2011; Nuotclá et al., 2019). Some of the daughters may co-breed with the mother (Biedermann et al., 2012). They may disperse anytime to found their own nests (Biedermann et al., 2009). The remainder of described social systems in Xyleborini comprise maternal care and some kind of communal breeding (all daughters that stay co-breed with their mother in Ambrosiophilus spp.; Kirkendall et al., 2015; Kasson et al., 2016).
The morphology of ambrosia beetle galleries (i.e., tunnel systems within wood, where they nest) is highly variable (Kirkendall et al., 2015). Most common types in Xyleborini are brood-chambers as in Xyleborinus or Xylosandrus species and branching tunnel systems as in Xyleborus and Euwallacea species (Roeper, 1995; Biedermann, 2012). As adults, brood and fungus gardens are spatially more separated in branching tunnels than in communal chambers, gallery morphology has probably a major influence on social interactions and modes of farming. Cooperative breeding has been reported from X. saxesenii with a brood-chamber type of breeding system (Biedermann and Taborsky, 2011; Biedermann et al., 2012), but the social and farming behaviors of species that construct branching tunnel systems remain enigmatic. The sugar-cane borer Xyleborus affinis (Xyleborini, Scolytinae, Curcilionidae) is one of those species making branching tunnel systems.
Healthy fungus gardens of ants, termites, and ambrosia beetles harbor a rich community of fungi and bacteria, even though typically one or rarely two main cultivar fungi dominate (Francke-Grosmann, 1967; Haanstad and Norris, 1985; Kajimura and Hijii, 1992; Mueller et al., 2005; Rodrigues et al., 2008; Biedermann et al., 2013; Saucedo-Carabez et al., 2018; Skelton et al., 2018; Biedermann and Vega, 2020). In ambrosia beetles, these food fungi are transmitted by adult females from the natal to a newly established nest either within the gut (Francke-Grosmann, 1975) or more commonly within a specialized cuticular pouch, the mycetangium (Francke-Grosmann, 1956, 1967; Hulcr and Stelinski, 2017). The whole mycobiome and how monocultures of cultivar fungi are established by the beetles remains poorly studied (Hulcr and Stelinski, 2017; Biedermann and Vega, 2020). In the cooperatively breeding X. saxesenii hygienic behaviors are induced in response to fungal pathogens (Biedermann and Taborsky, 2011; Nuotclá et al., 2019). Furthermore, removal of beetles from galleries does lead to the immediate outgrowth of cultivars by non-mutualistic, weedy fungi that are present in a resting form in the fungus gardens of all ambrosia beetles studied (Schneider-Orelli, 1913; Francke-Grosmann, 1956; Norris, 1993).
My study species, X. affinis, is best known for its damages in sugar-cane plantations, it is reported to also attack more than 248 other woody plant species (Schedl K. E., 1963). Galleries are founded by single females within a stressed or recently dead plant and may be inhabited by one to several generations of beetle offspring if the nesting substrate is durable enough (Schneider, 1987). While boring an entrance tunnel perpendicular into the wood, foundresses inseminate tunnel walls with spores of Raffaelea ambrosia fungus mutualists [possibly Raffaelea arxii, which might be the primary food fungus (Saucedo-Carabez et al., 2018)] they carry in oral mycetangia [i.e., fungal-spore pockets (Francke-Grosmann and Schedl, 1960; Schneider, 1987)]. If successful, layers of conidiophores with nutritional conidiospores by the Raffaelea fungi line tunnel walls and the foundresses start to lay eggs. By feeding solely on the fungi, larvae pass through three instars followed by pupation (Biedermann et al., 2009). When reaching adulthood many daughters do not disperse immediately, but remain in the natal nest, where they may help in gallery expansion and hygiene, brood and fungus care, and possibly co-breed. Delayed dispersers have been found to loose reserves in the natal nest either by egg-laying and/or investments in helping (Biedermann et al., 2011). Thus, initial tunnel systems with a length of about 20–30 cm after one generation have been found to expand over 6 m (within 4 years) by the work of several consecutive overlapping generations (Schneider, 1987). Staying and consecutive breeding within one gallery is possible because X. affinis (i) produces its own fungal food and (ii) is an inbreeding species with regular brother-sister mating and a haplodiploid sex-determination system. Haplodiploidy enables founder females to assign optimal brood sex-ratios (by laying unfertilized eggs that develop into males and fertilized eggs that develop into females), which are strongly female biased (Schedl K. E., 1963; Biedermann et al., 2009). Eighty-five percent of laboratory galleries contain only a single male, which is hatching first and obviously capable to fertilize several dozens of sisters (Roeper et al., 1980). Males are flightless and may disperse only by foot, which they do after all female offspring matured (Biedermann, 2010).
Here I used artificial observation tubes that contained entire colonies of reproducing X. affinis beetles and their fungus gardens (a) to determine the social system of an ambrosia beetle breeding in a branching tunnel system and (b) to describe the fungal community of gardens in relation to the presence and the behaviors of beetles and their larvae. Furthermore, I asked whether (c) larvae and adult offspring engage in alloparental brood care and fungus maintenance, (d) decisions of adult females to help, to breed and to disperse relate to the number of potential beneficiaries, the number of potential competitors and depend on the location within the nest (old vs. freshly excavated gallery parts), and (e) ovary status affects the propensity to engage in cooperative behaviors.
Materials and Methods
Beetle Collection, Laboratory Breeding and Phenology
Females used for breeding in this study were directly collected from oak logs in Pineville, LA, United States (123 ft asl; 31°20′, 92°24′) in June 2007. Carried in sterile glass vials, they were immediately brought to the laboratory and used for artificial rearing: Females were surface-sterilized (by washing them first for a few seconds with 95% ethanol and then with distilled water) and afterward singly placed on prepared sterile artificial medium in separate glass tubes (18 mm diameter × 150 mm length; Bellco Glass, Vineland, NJ, United States) that were covered by sterile plastic caps (Bellco Glass kap-uts, Vineland, NJ, United States). Artificial medium had been drying for 5 days before the placement of beetles and consisted of an autoclaved mixture of 0.35 g streptomycin, 1 g Wesson’s salt mixture, 5 g yeast, 5 g casein, 5 g starch, 10 g sucrose, 20 g agar, 75 g oak tree sawdust (freshly grounded and oven dried), 2.5 ml wheat germ oil, 5 ml 95% ethanol and 500 ml deionized water (for details on the preparation see Biedermann et al., 2009). After introduction of the beetles, tubes were stored at room temperature (∼23°C) in darkness (wrapped in paper, but light could shine on the entrance). This way, females start digging tunnels as if in wood and often build them adjacent to the glass of the tube. Thus, this technique allows to observe behavior of adults and larvae in the tunnels when the paper is removed (Biedermann et al., 2009). At 23°C a fungal layer and first eggs appear in successfully founded galleries around 10 days after gallery foundation. The first adults hatch about a month later and females remain in the natal nest for at least a week before they disperse by crawling onto the surface of the media, where they try to fly off (and where they can be collected for consecutive rearing). Peak productivity is around 60 days after gallery foundation. At that time, offspring of all stages are present together and the first adult daughters start to disperse. In the laboratory, more offspring will develop typically for another 20–30 days until the medium dries out and all individuals leave the gallery (Roeper et al., 1980; Biedermann et al., 2011).
Behavioral Observations, Dissections of Galleries and of Female Ovaries
Here I used a pool of 23 successfully founded laboratory galleries. Tunnels were visible in 16 galleries, which were used for behavioral observations. Fungi were isolated from 15 galleries. Both behavior and fungal communities were determined for eight galleries among those (for details on these galleries see Supplementary Table S1). I started the treatment at about the peak of gallery productivity, when study galleries ranged between 51 and 60 days of age (after gallery foundation) and adult daughters started to disperse. In the 3 days before the treatment, I conducted daily behavioral observations of all visible individuals (N = 16 galleries). For each individual I noted the developmental stage and sex, its location within the gallery (main tunnel, side tunnel, brood tunnel; see Figure 1) and its behavior at the time of observation [i.e., “scan observation” technique (Biedermann and Taborsky, 2011)]. Larvae were classified by size in either 1st or 2nd/3rd instars. After pupation, females first have a brownish coloration and turn black when fully sclerotized. Light brown females were termed tenerals and dark brown females adults. Males are discernable from females by their smaller body size small horns on the pronotum. I differentiated between the larval behaviors allogrooming, fungus cropping, cannibalism, locomotion, being pushed by an adult female and inactivity, and the teneral/adult behaviors shuffling frass, blocking, digging, allogrooming, self-grooming, fungus cropping, cannibalism, locomotion, pushing larva, inactivity and the male mating (attempt) (for details see Table 1). For a video of shuffling frass by adults see Supplementary Material.
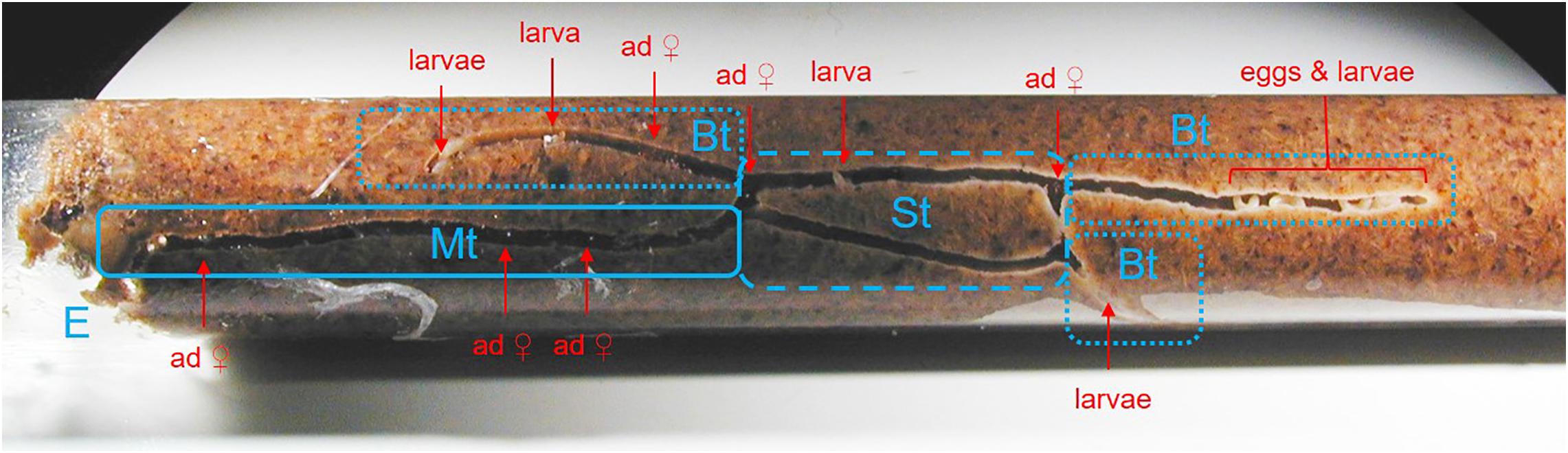
Figure 1. Morphology of a X. affinis laboratory gallery in artificial medium at about 60 days after its foundation. Note the species-characteristic tunnel system with the four compartments: E, entrance; Mt, main tunnel; St, side tunnel; Bt, brood tunnel. A white fungal layer and white larvae are visible within the Sts and the Bts. Several adult beetles are sitting in the Mt and a few in the Sts and the Bts.
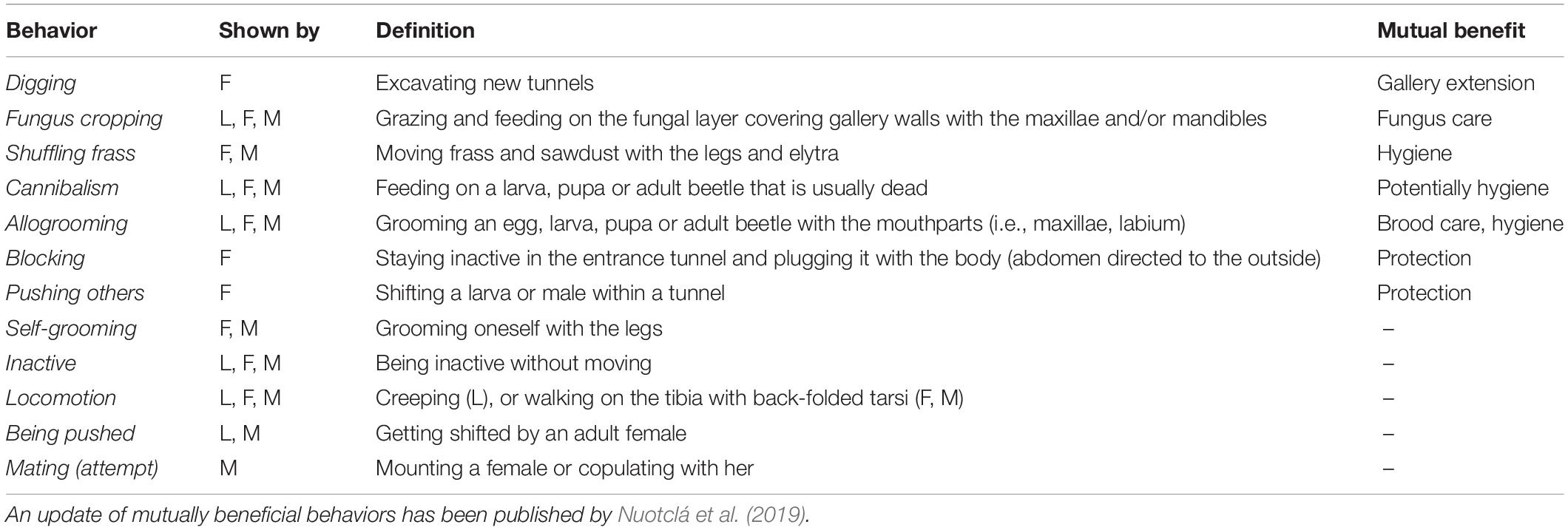
Table 1. Ethogram of the observed behaviors of X. affinis larvae (L), females (F), and males (M) (modified from Biedermann and Taborsky, 2011).
Most galleries are not fully visible from the outside. Therefore, to assess the number of individuals within a gallery and the reproductive status of adult females, I broke the glass of eight galleries at an age between 55 and 61 days, when the first generation of offspring matured and just started to disperse (Supplementary Table S1). All developmental stages (eggs, 1st instar larvae, 2nd/3rd instar larvae, pupae and adults) from within the nests were counted and all 49 females from within nests as well as 14 dispersing females were preserved in 95% ethanol until dissection. For all the 49 females from within nests, I noted their location within the nest and for 21 of these females it was even possible to record their behavior immediately before the glass of the tube was destroyed. I dissected female ovaries on microscope slides within some drops of phosphate-buffered saline (PBS) buffer solution under a binocular (6.4×–40× magnification). Females were decapitated, their wings removed and ovaries were exposed by dorsal opening of the abdomen with high precision tweezers and a scissor. I discriminated between non-developed (the four ovarioles are all thin or not present), developed (four ovarioles present, but not thickened and no oocytes visible) and egg-carrying ovaries (one to two of the four ovarioles are thickened and contain one to two oocytes); numbers of oocytes (i.e., developing eggs) were noted (for figure of ovaries see Fischer, 1954).
Fungal Isolations and Identification of Filamentous Fungal Isolates
On the fourth day of the treatment (i.e., 55 to 64 days after gallery foundation), I brought some of the galleries (N = 15) to a sterile bench, carefully broke the glass of the tubes, and took 12 fungus samples each from the main tunnel, the side tunnel and the brood tunnel with a forceps that was flame-sterilized after each sample. Four samples were placed on malt agar plates (MA: 25 g malt extract, 20 g agar, 1 L deionized H2O), four samples on cycloheximide-streptomycin malt agar plates (CSMA: 10 g malt extract, 15 g agar, 20 ml filter sterilized CSMA stock solution containing 2 mg of cycloheximide and 1 mg streptomycin, 1 L deionized H2O) and the last four on benomyl malt agar plates (BMA: 10 g malt extract, 15 g agar, 1 ml filter sterilized BMA stock solution containing 1 mg benomyl dissolved in dimethylsulfoxid, 1 L deionized H2O). MA is unselective, CSMA is selective for Ophiostomatoid fungi (e.g., Raffaelea species; Harrington, 1981) and BMA for Basidiomycetes (Ross et al., 1992). Samples were spotted in the center of the plates. Afterward, I fully dissected the whole gallery system and counted all eggs, larvae, pupae, adult females and males (see above).
For obtaining fungal isolates from female cadavers (N = 4), I put diseased adult females (from within nests) singly in 1.5 ml Eppendorf tubes with 1 ml PBS buffer. Bodies were crushed with a pestle and vortexed within the PBS buffer for 5 s. Afterward 1:1000 and 1:10000 dilution series were generated and 100 ul of both dilutions were plated out on the three media above. Presence/absence of fungal isolates on these plates was recorded 10 days later.
Isolated fungi were first sorted into groups based on cultural growth and morphological colony characteristics (i.e., morphology and color of the mycelium) and these were compared with a database of fungal isolates from bark and ambrosia beetles available at the Southern Research Station of the USDA Forest Service. Afterward, I brought pieces of fungal tissue on microscope slides with drops of PBS buffer and examined their spore structures. Confirmation and final determination of four of the five fungal isolates was done using internet sources and the illustrated key to identify genera of imperfect fungi by Barnett and Hunter (1998). Representative samples were stored for DNA sequencing, but material got lost through a failure of a refrigerator and could neither be revived nor successfully sequenced. Therefore, only morphological, genus-level identifications are available.
Statistical Analyses
If not stated differently, all statistical analyses were done using generalized estimating equations (GEEs) in R [lmer; Version 2.12.1 (R-Development-Core-Team, 2008)]. GEEs are an extension of generalized linear models with an exchangeable correlation structure of the response variable within a cluster, which allows for controlling the variation between observations from a single gallery. This was necessary because of variation in sample sizes between galleries (as for the fungal community analyses a few plates without microbial growth had to be excluded).
Using all 12 samples taken from each location (main, side, brood tunnel) of each gallery (independent variable), I estimated the frequency and presence (yes/no) of each fungal isolate (dependent variables) by controlling for medium (MA, CSMA, BMA; fixed factors) and gallery of origin (random factor). I also analyzed how number of eggs, larvae and adult females (dependent variable) were affected by the location within the gallery (fixed factor) and the presence of the different fungi (fixed factor) by controlling for gallery of origin (random factor). Differences in the frequency of male observations between locations within the gallery were tested using Fisher’s exact test, because of the small number of males observed. In a third series of models, I analyzed whether frequencies of larval and adult behaviors (dependent variable) were influenced by the location within the gallery (fixed factor).
By correlating behavior with fungal communities, I first determined whether the frequency of larval and adult behaviors (dependent variable) were affected by the presence of the different fungi (fixed factor), by controlling for location within the gallery (fixed factor) and gallery of origin (random factor). Second, I modeled whether the frequency of larval and adult fungus cropping and shuffling frass (fixed factors) and gallery of origin (random factor) affected isolation frequency of each fungus (dependent variable). Finally, I used logistic regression models (GLMs with binary response variable) to model if the proportion of egg-laying and dispersing females and females with developed ovaries (dependent variables) were affected by the proportion of immatures relative to adults in the nest, the proportion of different female reproductive groups relative to all females inside the nest, and the proportion of dispersing relative to staying females (fixed factors).
Results
Overlapping Generations, Factors Influencing Reproductive Division of Labor and Alloparental Care by Adult Daughters
Between day 55 and 61, when the first generation of offspring matured and started to disperse, galleries (N = 8) contained on average 33.6 (±10 SE) eggs, 7.1 (±2.2) larvae, 5.4 (±3) pupae, 0.3 (±0.3) immature females, 6.8 (±0.9) adult females and 0.9 (±0.1) adult males. Of the adult females a mean of 26.8% (±9.7) had non-developed ovaries, 19.2% (±7.9) had developed ovaries, and 54.1% (±13.3) were laying eggs (Supplementary Table S1). All 14 dispersing adult females that were dissected had either non-developed (N = 9) or developed ovaries (N = 5).
Egg-laying was unequally distributed among females and there was no fixed proportion of females laying eggs per gallery: First, egg numbers were independent of the number of potential egg-layers (i.e., females with developed ovaries and egg-laying females) (GLM: p > 0.05) and second the proportion of egg-laying females were negatively affected by the proportion of females inside the nest with non-developed ovaries (GLM: p = 0.016; Supplementary Table S2) and developed ovaries (p = 0.031). The proportion of females with developed ovaries correlated negatively with the proportion of females with non-developed ovaries (p = 0.013) and with the proportion of egg-layers (p = 0.042), which might suggest that development of ovaries is triggered by the opportunity to breed. The propensity of females to disperse was reduced with increasing numbers of larvae dependent on brood care (p = 0.019), but independent of the relative proportion of immature offspring to adult females inside the nest as well as the proportion of female egg-layers and females with non-developed ovaries. In contrast, proportion of female dispersers correlated negatively with proportion of females with developed ovaries, possibly suggesting that ovary development typically leads to either dispersal or egg-laying in the natal nest (see above).
Both potential egg-layers and females with non-developed ovaries engaged in alloparental care (i.e., allogrooming, fungus cropping and shuffling frass) at the natal nest. However, despite the small sample size, there was a trend for adult females with non-developed ovaries (N = 11) to engage less in these mutually beneficial behaviors immediately before galleries were dissected, than females potentially laying eggs (Fisher’s exact test: p = 0.085, N = 21; Figure 2). Additionally, potential egg-layers tended to be found more frequently close to the brood (i.e., in the side-tunnel) than in the main tunnel compared to females with non-developed ovaries (Chi2-test: χ2 = 2.94, df = 1, p = 0.087, N = 93).
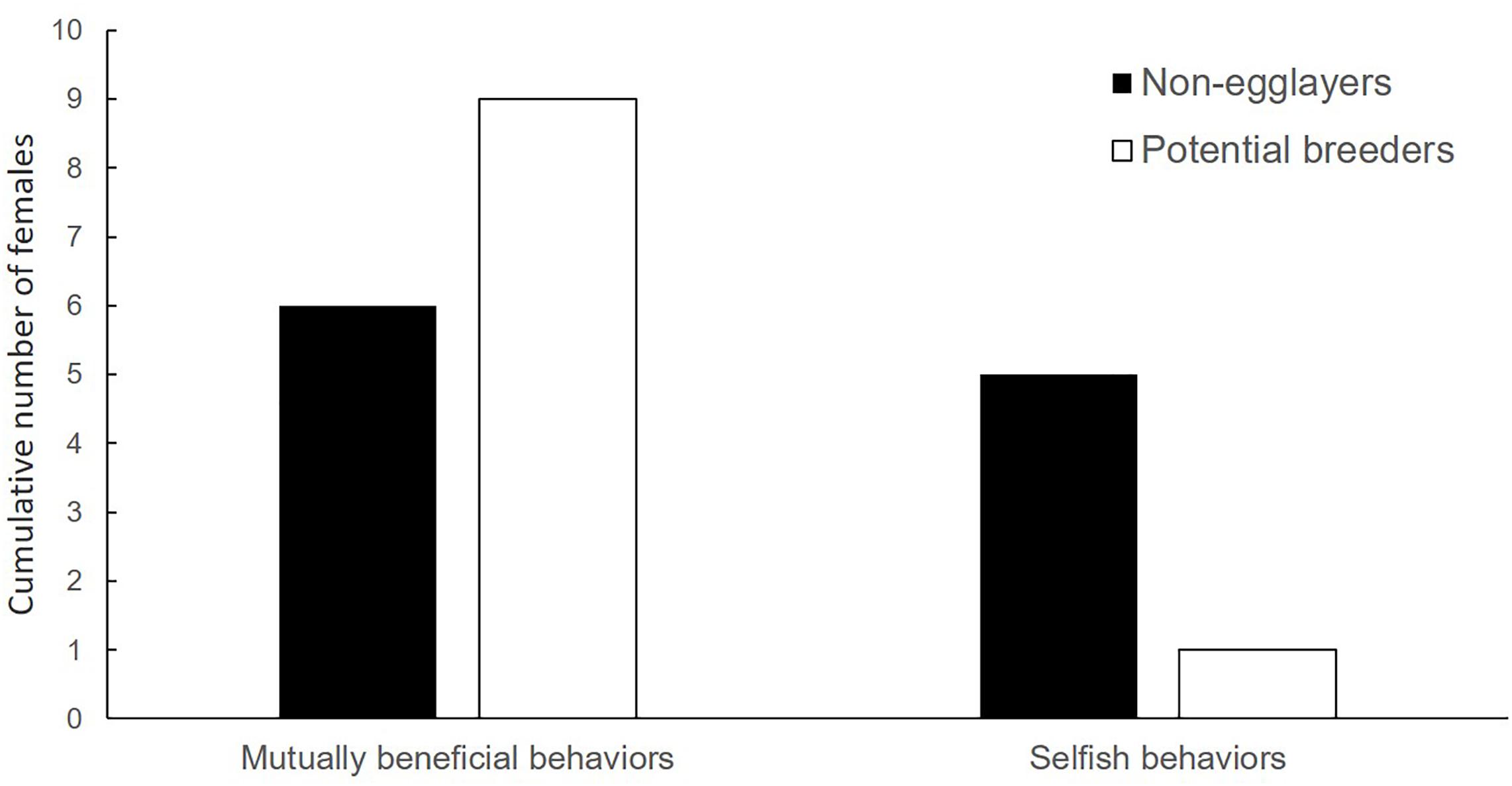
Figure 2. Reproductive status in relation to adult female behavior. All females engage in mutually beneficial behaviors. However, relative to selfish behaviors, females with fully developed or egg-carrying ovaries (potential breeders) tended to engage more often in mutually beneficial behaviors (see Table 1) than females with non-developed ovaries (non-egg-layers) (Fisher’s exact test: p = 0.085, N = 21).
Offspring and Adult Numbers and Their Behaviors in Relation to the Three Gallery Compartments
Eggs and pupae were only found in the brood tunnels of the galleries. Larvae were most commonly found in the brood tunnels, followed by the side tunnels (GEE: p = 0.03; Supplementary Table S3, Figure 3), and the main tunnel (p < 0.001). When present in the main tunnel they only showed locomotion, which was also often observed in the side tunnel, but only rarely in the brood tunnel (p < 0.001; Supplementary Table S4). Larval allogrooming and fungus cropping were most common in the brood tunnel (p < 0.05). Larval cannibalism was only present at low rates in the side tunnel.
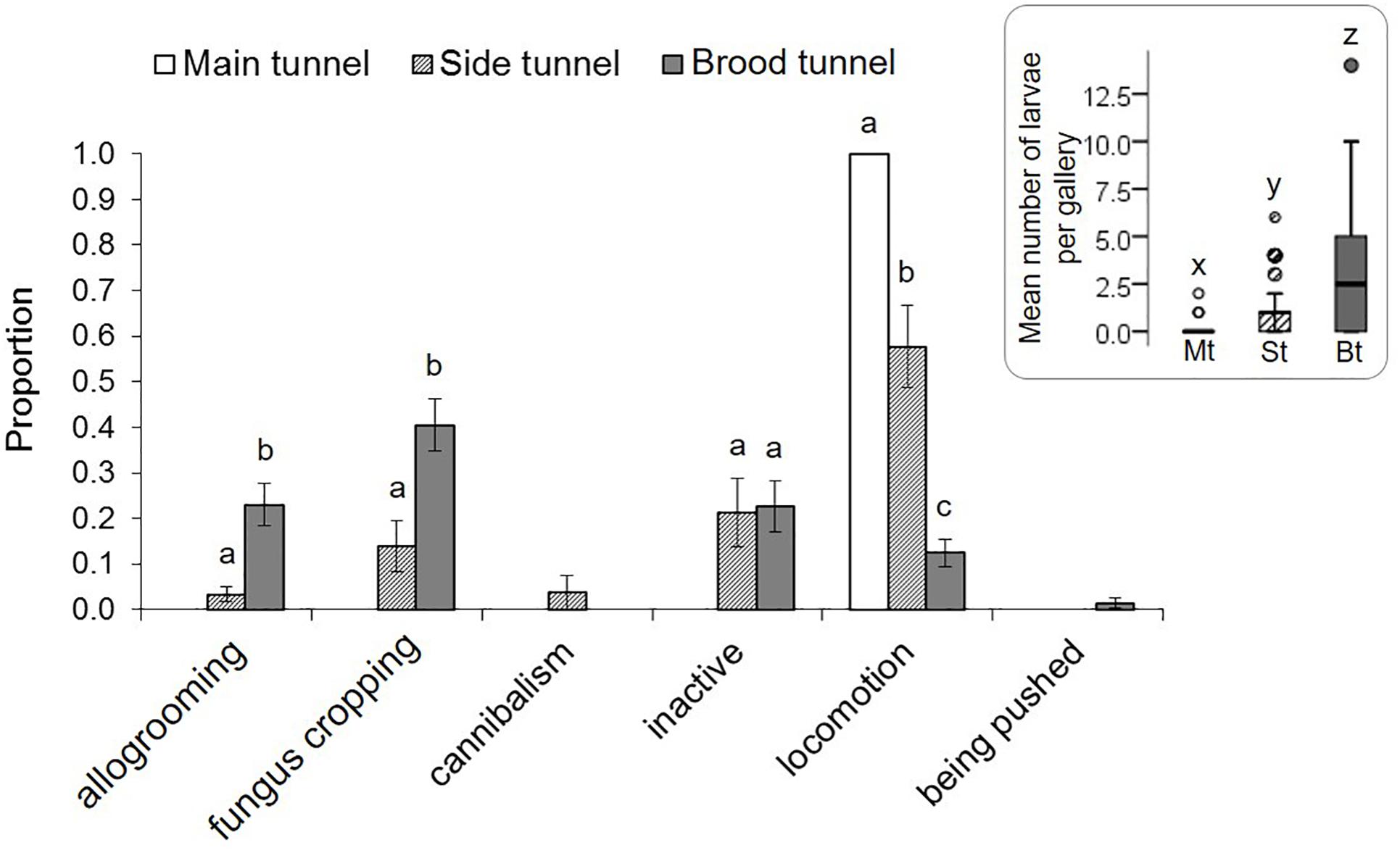
Figure 3. Behaviors of X. affinis larvae in the three gallery compartments. Mean ± SE; different letters denote significant differences (p < 0.05; for exact values see Supplementary Table S4) in pairwise comparisons between compartments. The Mean (±SE) numbers of larvae observed in the three compartments are given in the box on the right.
Adult females and males were least common in the brood tunnels (p < 0.05; Supplementary Table S3, Figure 4). Males tended to stay in the side tunnels (p = 0.064). The most common adult female behaviors were shuffling frass and fungus cropping, with the last most common in the brood tunnel (p = 0.001; Supplementary Table S5, Figure 4). Inactivity tended to be expressed most often in the main tunnel (p < 0.066). Blocking was, by definition, only found in the main tunnel, whereas digging, cannibalism and pushing larva was never expressed there. All other female behaviors were equally common in all three gallery compartments (p > 0.05). Adult males spent their time mainly with fungus cropping (27% of their time), locomotion (25%), inactivity (16%), followed by attempting to mate (11%), and allogrooming (11%). Self-grooming, mating, being pushed and shuffling frass were only rarely shown by males (<4%).
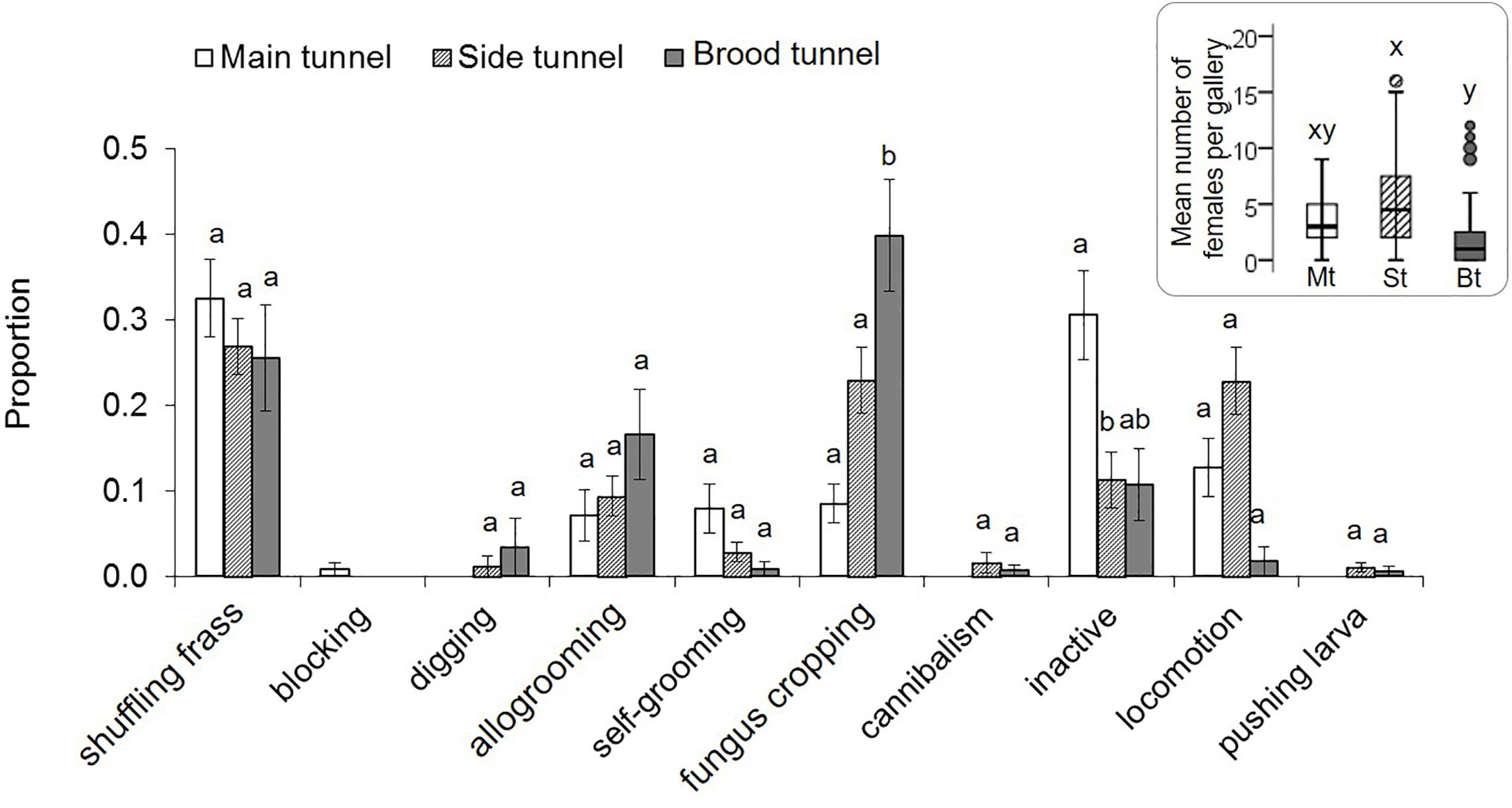
Figure 4. Behaviors of X. affinis adult females in the three gallery compartments. Mean ± SE; different letters denote significant differences (p < 0.05; for exact values see Supplementary Table S5) in pairwise comparisons between compartments. The Mean (±SE) numbers of adult females observed in the three compartments are given in the box on the right.
Fungal Isolations
Our isolations revealed five isolates of filamentous fungi associated with galleries of X. affinis: a Fusarium, a Mucor, a Phaeoacremonium, a Raffaelea and an unidentified filamentous fungus (“Unknown”) isolate. The Mucor isolate was identified by its fluffy aerial mycelium and the black sporangiophores. The Raffaelea isolate was identified by its characteristic growth on plates (filamentous on the outside of the culture, yeast-like in the center) and the morphology of conidiophores and the budding conidiospores. The Fusarium isolate was identified by its characteristic sickle-shaped conidia. The identity of the Phaeoacremonium isolate was confirmed by macro- and micro-comparisons with a morphologically nearly identical Phaeoacremonium rubrigenum isolate in the fungal database of the USDA Forest Service. The Raffaelea isolate was the most common fungus isolated.
Overall, Raffaelea, Phaeoacremonium and Unknown were more commonly detected on CSMA than MA (GEEs: p = 0.017–0.001; Supplementary Table S6), which shows that these three isolates are resistant to the antibiotic cycloheximide. Interestingly they grew also better on BMA than MA (p = 0.007–0.001). The opposite was found for Mucor and Fusarium, which were most frequently isolated from MA followed by BMA and CSMA (p < 0.01).
Fungal Diversity in Relation to Gallery Compartment and Age of Gallery
The Raffaelea was isolated from all compartments of all galleries, and was most commonly detected in the brood tunnels (GEEs: p = 0.003–0.001; Supplementary Table S6, Figure 5 and Supplementary Figure S1), which strongly suggests its nutritional role for X. affinis. Fusarium, Mucor, and Unknown were present in about 60% of all galleries. Unknown and Fusarium were isolated equally often from all compartments (p > 0.05), whereas Mucor and Phaeoacremonium occurred more frequently near the entrance than the brood (p = 0.003–0.006). Raffaelea, Fusarium and Mucor were strongly associated with old galleries (i.e., isolation rate > 60%; Supplementary Figure S1). The other two fungi were detected in <20% within these samples. Also dead females harbored Raffaelea, Fusarium and Mucor; the latter in >60% of diseased individuals.
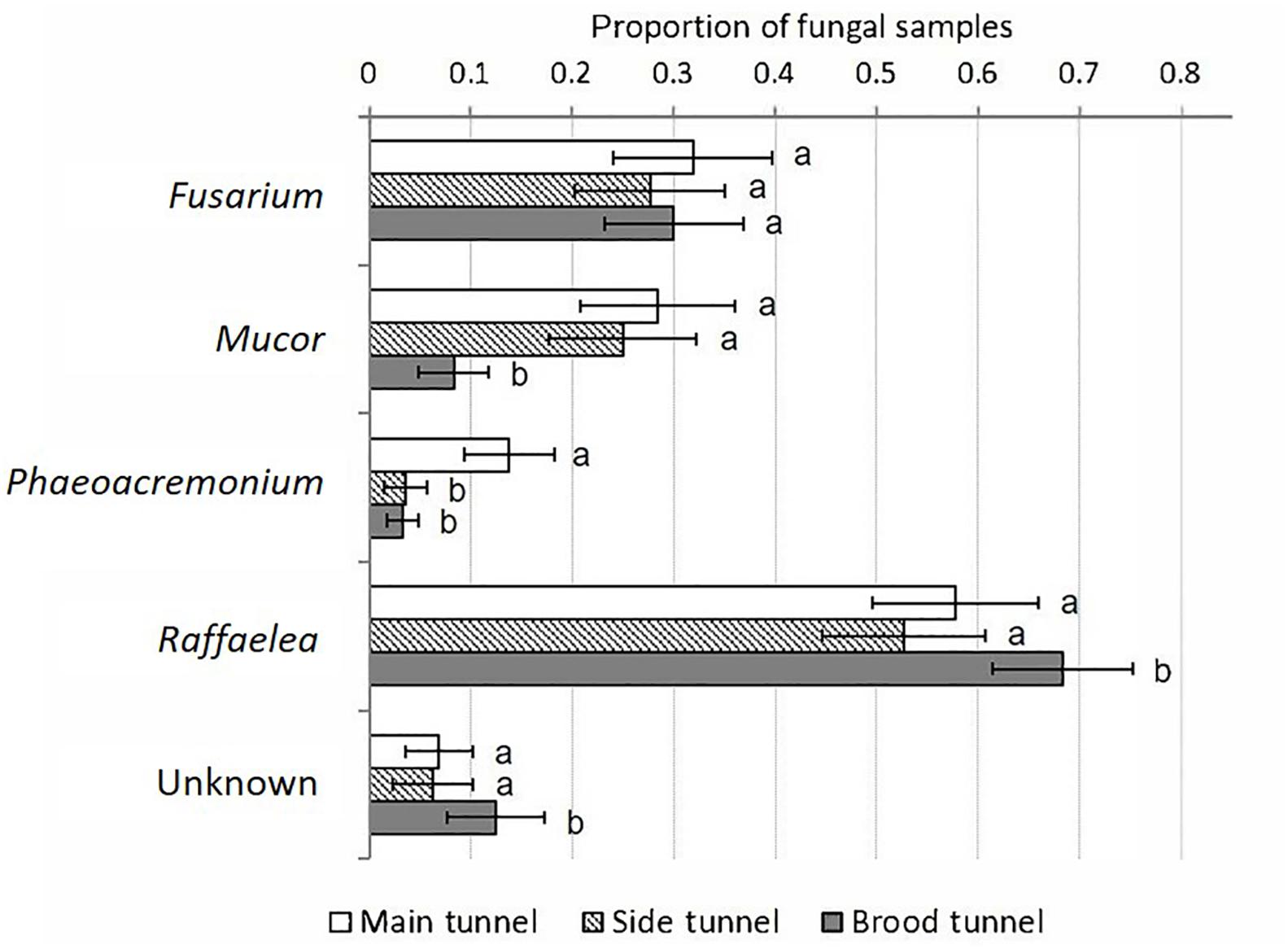
Figure 5. Percentage of fungal morphospecies isolated relative to the total number of samples taken per gallery compartment. Mean ± SE; N = 12 samples per compartment; main tunnel – eight galleries, side tunnel – six galleries, brood tunnel – seven galleries. Different letters denote significant differences (p < 0.05; for exact values see Supplementary Table S6) in pairwise comparisons between compartments.
Influence of Fungal Species on Beetle Productivity and Their Farming Behaviors
The regular presence of Raffaelea in samples from all compartments of all galleries supports its essential function for the beetles, but inhibited testing the influence of its presence on beetle productivity and behaviors. It is possibly the only fungus with a nutritional role for the brood, because egg and larval numbers were both not affected by any other isolate (GEEs: p > 0.05; Supplementary Table S3). In contrast, adult female numbers were negatively affected by the presence of Phaeoacremonium (p = 0.005). Adult females increased fungus cropping frequency in the presence of Unknown (p = 0.038; Supplementary Table S7, Figure 6), increased shuffling frass (p = 0.048) and general activity levels in the presence of Fusarium (p = 0.02) and decreased activity levels in the presence of Phaeoacremonium (p = 0.002). None of the fungal isolates influenced the activity of larvae (i.e., their fungus cropping and shuffling frass behaviors; p > 0.05; data not shown).
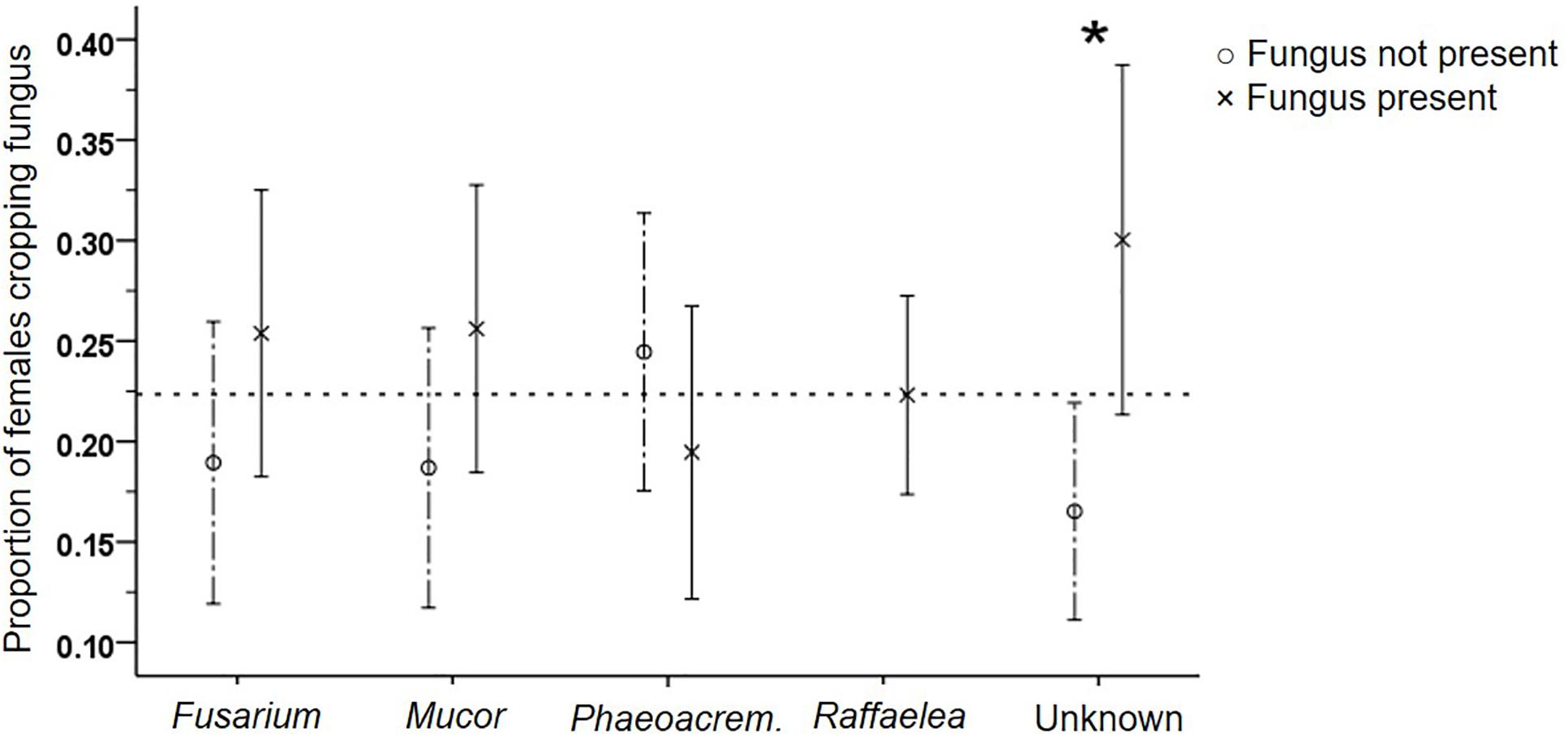
Figure 6. Effect of the presence of the five different fungi on the frequency of adult female fungus cropping. Mean (±SE) frequencies, for fungus present or not per gallery, are given. Raffaelea was always present. *p < 0.05 (for exact values see Supplementary Table S7).
Potential Influence of Larval and Adult Farming Behaviors on the Isolation Frequency of the Fungi
Raffaelea was less commonly isolated from those galleries with higher activity levels of larvae in the 3 days before the fungal isolations (p < 0.05; Supplementary Table S8). Isolation frequency of Unknown was positively correlated with larval fungus cropping (p = 0.004). Adult fungus cropping, shuffling frass or activity levels showed no significant correlation with the isolation frequency of the five fungi. There was, however, a very weak trend for a higher isolation frequency of Mucor and Phaeoacremonium from those galleries with lower activity levels of adults in the days before (p < 0.12).
Discussion
This study is the first to correlate ambrosia beetle behavior with egg-laying, location within the gallery and frequency of different fungal symbionts. Ambrosia beetle behavior normally cannot be observed within solid wood, so here I reared beetles in artificial medium in glass tubes (Biedermann et al., 2009). That gave the chance for individual behavioral observations of females immediately before dissection of ovaries and targeted fungal samplings, in combination with behavioral observations. So for each of the three X. affinis gallery compartments – brood tunnel, side tunnel, and main tunnel – I was able to give an overview of the number of larval and adult inhabitants, their respective behaviors (including egg-laying) and the diversity of filamentous fungi. The social system was similar to that of other Xyleborini ambrosia beetles (Table 2) and there was correlative evidence for active management of fungus gardens by the beetles, i.e., behavioral changes in response to the fungal composition of their ambrosia gardens.
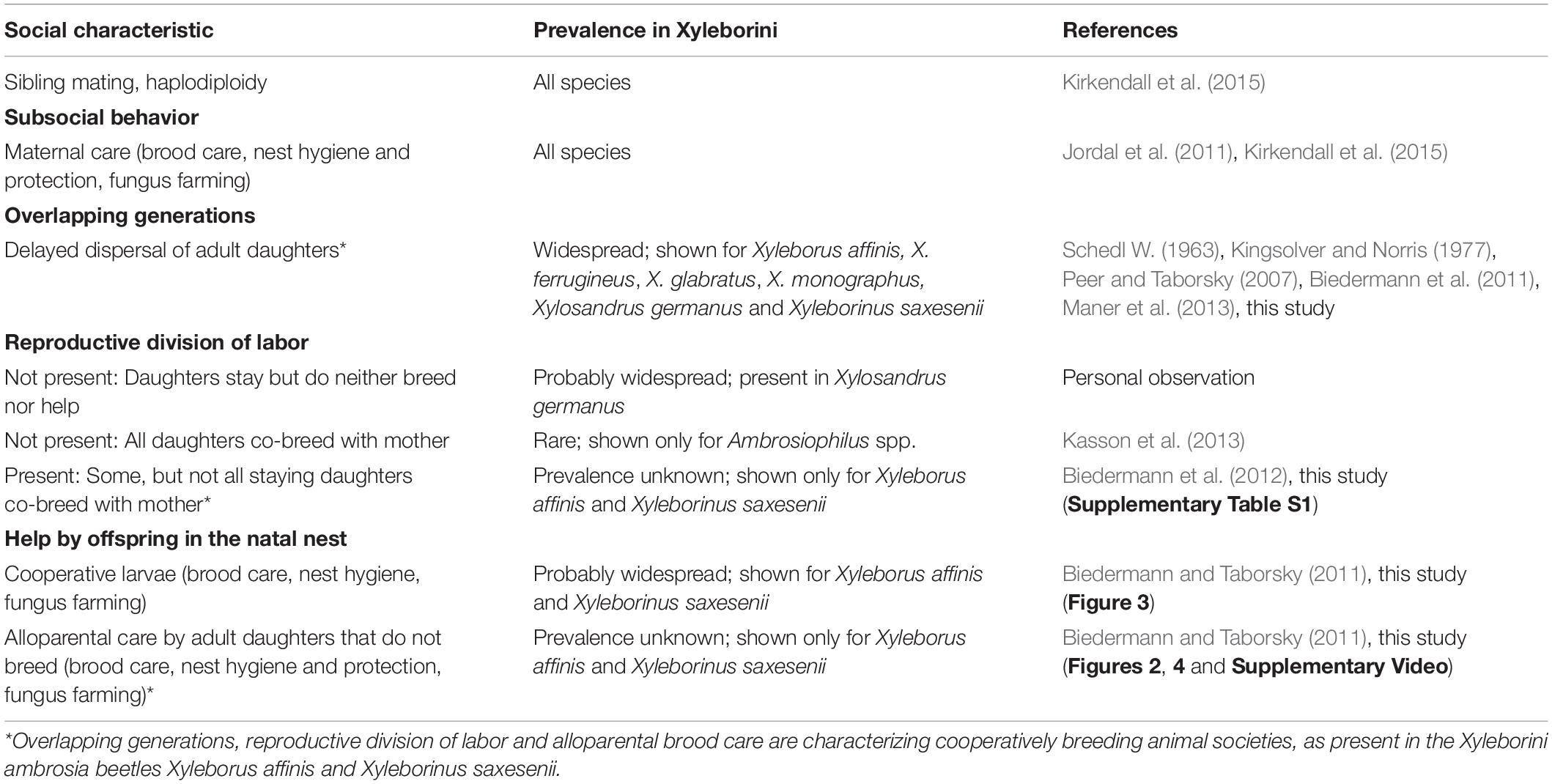
Table 2. Overview of social traits found in the ambrosia beetle tribe Xyleborini (Curculionidae: Scolytinae).
Observations and female dissections revealed that in X. affinis some adult daughters delay dispersal, help in hygienic tasks, brood and fungus care (Figure 2; see Supplementary Video) and on average half of them reproduce in the natal gallery together with their mother (Supplementary Table S1). This social system is termed cooperative breeding or facultative eusociality (Boomsma and Gawne, 2017) and has already been described from the ambrosia beetle Xyleborinus saxesenii (Biedermann and Taborsky, 2011) (Table 2). Development, offspring numbers, number of egg-layers and dispersed females after 60 days almost equals the numbers reported from Biedermann et al. (2012) that used also the same rearing media. Even though we currently lack behavioral studies on other ambrosia beetle species, data on development and delayed dispersal of adult offspring indicate that cooperatively breeding can be possibly found also in some other Xyleborinus and Xyleborus species, even if it is probably not widespread (Schedl W., 1963; Kingsolver and Norris, 1977; Gagne and Kearby, 1979; Maner et al., 2013).
Xyleborus affinis galleries contained high numbers of individuals of all age groups at around day 60, which is shortly after adult daughters start to disperse from galleries (Biedermann et al., 2011). Multiple egg-layers were already present and on average, about half of the adult daughters that had remained in the natal nest had started to lay eggs. Similar numbers been reported for X. saxesenii (Biedermann et al., 2012). In contrast to X. saxesenii, the propensity of females to disperse did not correlate with brood depending on care (i.e., eggs, larvae, pupae). Although all adult females participate in social tasks in the natal nest, egg-laying females were more inclined to do so. This higher investment of egg-laying individuals than of non-reproducing helpers into brood care is typical for social groups that have not taken the transition to superorganismality (i.e., obligate eusociality; Boomsma and Gawne, 2017). Reproducing females in the equally cooperatively breeding X. saxesenii, for example, typically protect the gallery entrance against the introduction of predators, which is the most risky task in an ambrosia beetle society (Biedermann and Taborsky, 2011). By contrast, in superorganismal societies sterile workers are not only overtaking the most dangerous tasks, but usually all non-reproductive tasks that need to be done (Wilson, 1971; Bourke, 2011; Boomsma and Gawne, 2017).
Observations of the three gallery compartments (Figure 1) revealed a spatial organization of developmental stages and behavioral tasks. Brood tunnels were surrounded by thick layers of fungal growth and inhabited mostly eggs, pupae and larvae, whereas males and females capable of laying eggs (i.e., developed ovaries or ones containing eggs) tended to be found in the side tunnels. Males were waiting in the side tunnels for freshly emerging females to mate. There was a trend for females with undeveloped ovaries to be found in the main tunnels. This compartmentalization of developmental stages reflects the spatial fidelity found in ants, where brood and adults are also unequally distributed within the nest (Mersch et al., 2013). Not surprisingly, this compartmentalization is also reflected by a spatial division of labor in the sense that most fungus cropping and allogrooming are found in the brood tunnels, whereas shuffling frass, inactivity and locomotion are found most often in the food-deficient side and main tunnels (Figures 2, 3 and Supplementary Video). This compartmentalization of age groups and the resulting spatial division of labor has not been reported for any other ambrosia beetle and is probably caused by breeding in tunnels instead of brood chambers (where the fungal food resource and all age groups occur together; Biedermann and Taborsky, 2011; Biedermann et al., 2012). Given that all Xyleborus species make tunnel systems (Roeper, 1995; Kirkendall et al., 2015), it is likely that compartmentalization is present in other species of this genus. In the future, some more comparative analyses of brood-chamber- and branching-tunnel-building species need to be done, to check if gallery morphology allows inferences on the social structures of the societies.
This is the first study to look at fungal communities of X. affinis using artificial laboratory cultures. Five different morphospecies were identified: a Raffaelea, a Fusarium, a Phaeoacremonium, a Mucor and an Unknown fungus. This diversity of fungi is much lower than culture independent studies from X. affinis in the field reported (Kostovcik et al., 2014; Saucedo-Carabez et al., 2018), but also not surprising as many environmental microbes are excluded by the lab rearing. This study was not successful to determine a primary mutualistic fungus. Here I present correlative evidence that Raffaelea and Unknown are putative nutritional mutualists of X. affinis. Raffaelea formed thick ambrosia layers on the walls of the side and brood tunnels, which were fed upon by larvae and adults. Raffaelea species produce nutritional conidiospores and have been proven to be primary cultivars in other Xyleborus and Xyleborinus species (Francke-Grosmann, 1967; Roeper, 1996; Biedermann et al., 2013; Hulcr and Stelinski, 2017; Saucedo et al., 2017; Saucedo-Carabez et al., 2018). Saucedo-Carabez et al. (2018) predominately isolated Raffaelea arxii from field galleries of X. affinis, next to unfrequent R. lauricola and R. subfusca. R. arxii might therefore be the primary mutualist of X. affinis that I here could not determine to the species level. A recent metabarcoding study also confirmed the ubiquitous presence and nutritional role of a Raffaelea species for X. affinis (Ibarra-Juarez et al., 2020). Unknown may function as a secondary food source because it was particularly common in brood tunnels and its presence had a positive influence on adult female feeding.
It is unlikely that the other three fungal isolates, Mucor, Fusarium, and Phaeoacremonium, have a mutualistic role for the beetles. All three were predominantly found in the main tunnels, old galleries and associated with dead individuals. Presence of Fusarium also correlated with more active adult females and hygienic shuffling activity. Phaeoacremonium had negative correlative effects on the number of adult females present. Mucor species are typical saprotrophs (Ritz, 1995), whereas Fusarium and Phaeoacremonium species are often plant pathogens (Larignon and Dubos, 2000; Ma et al., 2013) and might profit from transmission by ambrosia beetles. One clade of Fusarium species is known as primary mutualists of Euwallacea ambrosia beetles, however (Kasson et al., 2013; O’Donnell et al., 2015; Freeman et al., 2016; Aoki et al., 2019). Fungi in this clade have typically thickened conidiospores, which I did not see in the isolate found in this study. Clearly, molecular identifications and experimental manipulations are needed to determine the roles suggested here.
I found some significant correlations between ambrosia beetle behavior and presence of specific fungi. Whether these behavioral changes are actually caused by the fungi is unclear, however. Ambrosia beetles are termed “farmers,” but currently there is very little data proving that the beetles indeed actively manage fungal communities in the sense that they have means to promote beneficial fungi over antagonistic fungi. The strongest evidence comes from a recent study on X. saxesenii by Nuotclá et al. (2019), who found that injection of Aspergillus pathogens in laboratory galleries may induce adult (allo-)grooming and - over time - reduced Aspergillus spore loads (Nuotclá et al., 2019). Previously it had been shown that X. saxesenii larvae are able to suppress the growth of not-identified fungal pathogens (Biedermann and Taborsky, 2011). Mechanistically, beetles may suppress fungal pathogens by application of antibiotic producing bacteria. Application of oral secretions containing bacteria have been observed (Cardoza et al., 2006) and bacteria selectively inhibiting fungal pathogens by producing cycloheximide (which does not harm Raffaelea species) have been isolated from X. affinis and X. saxesenii laboratory galleries (Grubbs et al., 2011, 2019).
In summary, X. affinis lives in a branching gallery in a cooperatively breeding social system with multiple egg-layers and division of labor between larvae and adults. Adult daughters have been shown previously to lose reserves during the delayed dispersal period (Biedermann et al., 2011). Future studies have to determine if some daughters lay eggs before dispersal, which may be possible as we found that one third of the dispersing females had developed ovaries. A similar social system has been described for X. saxesenii, which builds brood chambers, so interactions between adults and larvae are more common there (Biedermann and Taborsky, 2011; Biedermann et al., 2012). X. affinis is predominately associated with one or two fungal mutualists, of which the predominating one is a Raffaelea fungus (maybe R. arxii). This species has been reported by Saucedo-Carabez et al. (2018) and confirms the mutualism between the genus Xyleborus and Raffaelea species. Even though two recent articles suggest that yeasts (e.g., Ambrosiozyma) are additional mutualists of X. affinis and other Xyleborus species (Saucedo-Carabez et al., 2018; Ibarra-Juarez et al., 2020), their mutualisms appear less promiscuous then the first metabarcoding study on Xyleborus field galleries suggested (Kostovcik et al., 2014). More experimental work on the fitness effects of single fungal species on the beetles is clearly needed to test how specific the ambrosia beetle-fungus symbioses are (see e.g., Skelton et al., 2019). Finally, it is important to mention that our findings from the Xyleborini lineage should not be extrapolated to other independently evolved ambrosia beetle-fungus symbioses (Kirkendall et al., 2015; Hulcr and Stelinski, 2017). There is some evidence that the many independent origins of the ambrosia symbiosis in beetles differ considerably in their behaviors, social systems and ways of farming (Biedermann and Vega, 2020). We are just at the beginning of getting a small glimpse in the diversity of these fascinating beetle farmers.
Data Availability Statement
The datasets generated for this study are available on request to the corresponding author.
Author Contributions
PB conceived the study, performed all analyses, and wrote the manuscript.
Funding
The research was supported by a cooperative agreement with the USDA Forest Service. The author was funded by the Austrian Academy of Sciences (ÖAW; DOC fellowship) and by the German Research Foundation (DFG; Emmy Noether grant no. BI 1956/1-1). This publication was funded by the University of Würzburg in the funding programme Open Access Publishing. Funders had no role in research design or decision to submit.
Conflict of Interest
The authors declare that the research was conducted in the absence of any commercial or financial relationships that could be construed as a potential conflict of interest.
Acknowledgments
I am grateful to Kier D. Klepzig for the invitation to a research stay at the Southern Research Station and for introducing me in fungal isolation techniques. I also thank Stacy Blomqvist and Eric Ott for collecting X. affinis in the field and starting the first laboratory galleries. This manuscript was part of the doctoral thesis of PB (Biedermann, 2012).
Supplementary Material
The Supplementary Material for this article can be found online at: https://www.frontiersin.org/articles/10.3389/fevo.2020.518954/full#supplementary-material
References
Aoki, T., Smith, J. A., Kasson, M. T., Freeman, S., Geiser, D. M., Geering, A. D. W., et al. (2019). Three novel ambrosia Fusarium clade species producing clavate macroconidia known (F. floridanum and F. obliquiseptatum) or predicted (F. tuaranense) to be farmed by Euwallacea spp. (Coleoptera: Scolytinae) on woody hosts. Mycologia 111, 919–935. doi: 10.1080/00275514.2019.1647074
Barnett, H. L., and Hunter, B. B. (1998). Illustrated Genera of Imperfect Fungi. St. Paul, MN: American Phytopathological Society Press.
Biedermann, P. H. W. (2010). Observations on sex ratio and behavior of males in Xyleborinus saxesenii Ratzeburg (Scolytinae, Coleoptera). ZooKeys 56, 253–267. doi: 10.3897/zookeys.56.530
Biedermann, P. H. W. (2012). The Evolution of Cooperation in Ambrosia Beetles. Ph.D. thesis, University of Bern, Bern.
Biedermann, P. H. W., Klepzig, K. D., and Taborsky, M. (2009). Fungus cultivation by ambrosia beetles: behavior and laboratory breeding success in three Xyleborine species. Environ. Entomol. 38, 1096–1105. doi: 10.1603/022.038.0417
Biedermann, P. H. W., Klepzig, K. D., and Taborsky, M. (2011). Costs of delayed dispersal and alloparental care in the fungus-cultivating ambrosia beetle Xyleborus affinis Eichhoff (Scolytinae: Curculionidae). Behav. Ecol. Sociobiol. 65, 1753–1761. doi: 10.1007/s00265-011-1183-5
Biedermann, P. H. W., Klepzig, K. D., Taborsky, M., and Six, D. L. (2013). Abundance and dynamics of filamentous fungi in the complex ambrosia gardens of the primitively eusocial beetle Xyleborinus saxesenii Ratzeburg (Coleoptera: Curculionidae, Scolytinae). FEMS Microbiol. Ecol. 83, 711–723. doi: 10.1111/1574-6941.12026
Biedermann, P. H. W., Peer, K., and Taborsky, M. (2012). Female dispersal and reproduction in the ambrosia beetle Xyleborinus saxesenii Ratzeburg (Coleoptera; Scolytinae). Mitt. Dtsch. Ges. Allg. Angew. Entomol. 18, 231–235.
Biedermann, P. H. W., and Rohlfs, M. (2017). Evolutionary feedbacks between insect sociality and microbial management. Curr. Opin. Insect Sci. 22, 92–100. doi: 10.1016/j.cois.2017.06.003
Biedermann, P. H. W., and Taborsky, M. (2011). Larval helpers and age polyethism in ambrosia beetles. Proc. Natl. Acad. Sci. U.S.A. 108, 17064–17069. doi: 10.1073/pnas.1107758108
Biedermann, P. H. W., and Vega, F. E. (2020). Ecology and evolution of insect–fungus mutualisms. Annu. Rev. Entomol. 65, 431–455. doi: 10.1146/annurev-ento-011019-024910
Boomsma, J. J., and Gawne, R. (2017). Superorganismality and caste differentiation as points of no return: how the major evolutionary transitions were lost in translation. Biol. Rev. 93, 28–54. doi: 10.1111/brv.12330
Bourke, A. F. G. (2011). Principles of Social Evolution. Oxford: Oxford University Press. doi: 10.1093/acprof:oso/9780199231157.001.0001
Cardoza, Y. J., Klepzig, K. D., and Raffa, K. F. (2006). Bacteria in oral secretions of an endophytic insect inhibit antagonistic fungi. Ecol. Entomol. 31, 636–645. doi: 10.1111/j.1365-2311.2006.00829.x
De Fine Licht, H. H., and Biedermann, P. H. W. (2012). Patterns of functional enzyme activity in fungus farming ambrosia beetles. Front. Zool. 9:13. doi: 10.1186/1742-9994-9-13
Fischer, M. (1954). Untersuchungen über den kleinen Holzbohrer (Xyleborus saxeseni). Pflanzenschutzberichte 12, 137–180.
Francke-Grosmann, H. (1956). Hautdrüsen als Träger der Pilzsymbiose bei Ambrosiakäfern. Z. Ökol. Morphol. Tiere 45, 275–308. doi: 10.1007/bf00430256
Francke-Grosmann, H. (1967). “Ectosymbiosis in wood-inhabiting beetles,” in Symbiosis, ed. S. M. Henry (New York, NY: Academic Press), 141–205. doi: 10.1016/b978-1-4832-2758-0.50010-2
Francke-Grosmann, H. (1975). Zur epizoischen und endozoischen Übertragung der symbiotischen Pilze des Ambrosiakäfers Xyleborus saxeseni (Coleoptera: Scolitidae). Entomol. Ger. 1, 279–292.
Francke-Grosmann, H., and Schedl, W. (1960). Ein orales Übertragungsorgan der Nährpilze beiXyleborus mascarensis Eichh. (Scolytidae). Naturwissenschaften 47, 405–405. doi: 10.1007/BF00631270
Freeman, S., Sharon, M., Dori-Bachash, M., Maymon, M., Belausov, E., Maoz, Y., et al. (2016). Symbiotic association of three fungal species throughout the life cycle of the ambrosia beetle Euwallacea nr. fornicatus. Symbiosis 68, 115–128. doi: 10.1007/s13199-015-0356-9
Gagne, J. A., and Kearby, W. H. (1979). Life history, development, and insect-host relationships of Xyleborus celsus (Coleoptera: Scolytidae) in Missouri. Can. Entomol. 111, 295–304. doi: 10.4039/ent111295-3
Grubbs, K. J., Biedermann, P. H. W., Suen, G., Adams, S. M., Moeller, J. A., Klassen, J. L., et al. (2011). Genome sequence of Streptomyces griseus strain XylebKG-1, an ambrosia beetle - associated Actinomycete. J. Bacteriol. 193, 2890–2891. doi: 10.1128/JB.00330-11
Grubbs, K. J., Surup, F., Biedermann, P. H. W., McDonald, B. R., Klassen, J. L., Carlson, C. M., et al. (2019). Cycloheximide-producing Streptomyces associated with Xyleborinus saxesenii and Xyleborus affinis fungus-farming ambrosia beetles. bioRxiv [Preprint]. doi: 10.1101/511493
Haanstad, J. O., and Norris, D. M. (1985). Microbial symbiotes of the ambrosia beetle Xyletorinus politus. Microb. Ecol. 11, 267–276. doi: 10.1007/bf02010605
Harrington, T. C. (1981). Cycloheximide sensitivity as a taxonomic character in Ceratocystis. Mycologia 73, 1123–1129. doi: 10.2307/3759682
Harrington, T. C. (2005). “Ecology and evolution of mycophagous bark beetles and their fungal partners,” in Ecological and Evolutionary Advances in Insect-Fungal Associations, eds F. E. Vega and M. Blackwell (New York, NY: Oxford University Press), 257–291.
Hulcr, J., and Stelinski, L. L. (2017). The ambrosia symbiosis: from evolutionary ecology to practical management. Annu. Rev. Entomol. 62, 285–303. doi: 10.1146/annurev-ento-031616-035105
Ibarra-Juarez, L. A., Burton, M. A., Biedermann, P. H. W., Cruz, L., Desgarennes, D., Ibarra-Laclette, E., et al. (2020). Evidence for succession and putative metabolic roles of fungi and bacteria in the farming mutualism of the ambrosia beetle Xyleborus affinis. mSystems 5:e00541-20. doi: 10.1128/mSystems.00541-20
Jordal, B. H., Sequeira, A. S., and Cognato, A. I. (2011). The age and phylogeny of wood boring weevils and the origin of subsociality. Mol. Phylogenet. Evol. 59, 708–724. doi: 10.1016/j.ympev.2011.03.016
Kajimura, H., and Hijii, N. (1992). Dymamics of the fungal symbionts in the gallery system and the mycangia of the ambrosia beetle, Xylosandrus mutilatus (Blandford) (Coleoptera, Scolytidae). Ecol. Res. 7, 107–117. doi: 10.1007/bf02348489
Kasson, M. T., O’Donnell, K., Rooney, A. P., Sink, S., Ploetz, R. C., Ploetz, J. N., et al. (2013). An inordinate fondness for Fusarium: phylogenetic diversity of fusaria cultivated by ambrosia beetles in the genus Euwallacea on avocado and other plant hosts. Fungal Genet. Biol. 56, 147–157. doi: 10.1016/j.fgb.2013.04.004
Kasson, M. T., Wickert, K. L., Stauder, C. M., Macias, A. M., Berger, M. C., Simmons, D. R., et al. (2016). Mutualism with aggressive wood-degrading Flavodon ambrosius (Polyporales) facilitates niche expansion and communal social structure in Ambrosiophilus ambrosia beetles. Fungal Ecol. 23, 86–96. doi: 10.1016/j.funeco.2016.07.002
Kingsolver, J. G., and Norris, D. M. (1977). The interaction of the female ambrosia beetle, Xyleborus ferrugineus (Coleoptera: Scolytidae), with her eggs in relation to the morphology of the gallery system. Entomol. Exp. Appl. 21, 9–13. doi: 10.1111/j.1570-7458.1977.tb02650.x
Kirkendall, L. R., Biedermann, P. H. W., and Jordal, B. H. (2015). “Evolution and diversity of bark and ambrosia beetles,” in Bark Beetles: Biology and Ecology of Native and Invasive Species, eds F. E. Vega and R. W. Hofstetter (Amsterdam: Elsevier), 85–156. doi: 10.1016/b978-0-12-417156-5.00003-4
Kostovcik, M., Bateman, C. C., Kolarik, M., Stelinski, L. L., Jordal, B. H., and Hulcr, J. (2014). The ambrosia symbiosis is specific in some species and promiscuous in others: evidence from community pyrosequencing. ISME J. 9, 126–138. doi: 10.1038/ismej.2014.115
Larignon, P., and Dubos, B. (2000). Preliminary studies on the biology of Phaeoacremonium. Phytopathol. Mediterr. 39, 184–189.
Ma, L.-J., Geiser, D. M., Proctor, R. H., Rooney, A. P., O’Donnell, K., Trail, F., et al. (2013). Fusarium pathogenomics. Annu. Rev. Microbiol. 67, 399–416. doi: 10.1146/annurev-micro-092412-155650
Maner, M. L., Hanula, J. L., and Braman, S. K. (2013). Gallery productivity, emergence, and flight activity of the redbay ambrosia beetle (Coleoptera: Curculionidae: Scolytinae). Environ. Entomol. 42, 642–647. doi: 10.1603/EN13014
Mersch, D. P., Crespi, A., and Keller, L. (2013). Tracking individuals shows spatial fidelity is a key regulator of ant social organization. Science 340, 1090–1093. doi: 10.1126/science.1234316
Mueller, U. G., Gerardo, N. M., Aanen, D. K., Six, D. L., and Schultz, T. R. (2005). The evolution of agriculture in insects. Annu. Rev. Ecol. Evol. Syst. 36, 563–595. doi: 10.1146/annurev.ecolsys.36.102003.152626
Norris, D. M. (1993). Xyleborus ambrosia beetles - a symbiotic ideal extreme biofacies with evolved polyphagous privileges at monophagous prices. Symbiosis 14, 229–236.
Nuotclá, J. A., Biedermann, P. H. W., and Taborsky, M. (2019). Pathogen defence is a potential driver of social evolution in ambrosia beetles. Proc. Biol. Sci. 286:20192332. doi: 10.1098/rspb.2019.2332
O’Donnell, K., Sink, S., Libeskind-Hadas, R., Hulcr, J., Kasson, M. T., Ploetz, R. C., et al. (2015). Discordant phylogenies suggest repeated host shifts in the Fusarium - Euwallacea ambrosia beetle mutualism. Fungal Genet. Biol. 82, 277–290. doi: 10.1016/j.fgb.2014.10.014
Peer, K., and Taborsky, M. (2007). Delayed dispersal as a potential route to cooperative breeding in ambrosia beetles. Behav. Ecol. Sociobiol. 61, 729–739. doi: 10.1007/s00265-006-0303-0
R-Development-Core-Team (2008). R: A Language and Environment for Statistical Computing. Vienna: R Foundation for Statistical Computing.
Ritz, K. (1995). Growth responses of some soil fungi to spatially heterogeneous nutrients. FEMS Microbiol. Ecol. 16, 269–279. doi: 10.1111/j.1574-6941.1995.tb00291.x
Rodrigues, A., Bacci, M. Jr., Mueller, U. G., Ortiz, A., and Pagnocca, F. C. (2008). Microfungal “weeds” in the leafcutter ant symbiosis. Microb. Ecol. 56, 604–614. doi: 10.1007/s00248-008-9380-0
Roeper, R. A. (1995). Patterns of mycetophagy in Michigan ambrosia beetles. Mich. Acad. 272, 153–161.
Roeper, R. A. (1996). Ambrosia beetles of the continental United States and Canada and status of knowlegde of their associated primary symbiotic fungi. Newsl. Mich. Entomol. Soc. 41, 12–14.
Roeper, R. A., Treeful, L. M., Foote, R. A., and Bunce, M. A. (1980). In vitro culture of the ambrosia beetle Xyleborus affinis (Coleoptera: Scolytidae). Great Lakes Entomol. 13, 33–35.
Ross, D. W., Fenn, P., and Stephen, F. M. (1992). Growth of southern pine beetle associated fungi in relation to the induced wound response in loblolly pine. Can. J. For. Res. 22, 1851–1859. doi: 10.1139/x92-242
Saucedo, J. R., Ploetz, R. C., Konkol, J. L., Ángel, M., Mantilla, J., Menocal, O., et al. (2017). Nutritional symbionts of a putative vector, Xyleborus bispinatus, of the laurel wilt pathogen of avocado, Raffaelea lauricola. Symbiosis 75, 29–38. doi: 10.1007/s13199-017-0514-3
Saucedo-Carabez, J. R., Ploetz, R. C., Konkol, J. L., Carrillo, D., and Gazis, R. (2018). Partnerships between ambrosia beetles and fungi: lineage-specific promiscuity among vectors of the laurel wilt pathogen, Raffaelea lauricola. Microb. Ecol. 76, 925–940. doi: 10.1007/s00248-018-1188-y
Schedl, W. (1963). Biologie des gehöckerten Eichenholzbohrers, Xyleborus monographus Fab. (Scolytidae, Coleoptera). Z. Angew. Entomol. 53, 411–428. doi: 10.1111/j.1439-0418.1963.tb02902.x
Schedl, K. E. (1963). Scolytidae und Platypodidae Afrikas. II. Revista de Entomologia de Moçambique 5, 1–594.
Schneider, I. (1987). Verbreitung, Pilzübertragung und Brutsystem des Ambrosiakäfers Xyleborus affinis im Vergleich mit X. mascarensis (Col., Scolytidae). Entomol. Ger. 12, 267–275. doi: 10.1127/entom.gen/12/1987/267
Schneider-Orelli, O. (1913). Untersuchungen über den pilzzüchtenden Obstbaumborkenkäfer Xyleborus (Anisandrus) dispar und seinen Nährpilz. Centralbl. Bakt. Paras. 38, 25–110.
Six, D. L. (2012). Ecological and evolutionary determinants of bark beetle - fungus symbioses. Insects 3, 339–366. doi: 10.3390/insects3010339
Skelton, J., Johnson Andrew, J., Jusino Michelle, A., Bateman Craig, C., Li, Y., and Hulcr, J. (2019). A selective fungal transport organ (mycangium) maintains coarse phylogenetic congruence between fungus-farming ambrosia beetles and their symbionts. Proc. Biol. Sci. 286:20182127. doi: 10.1098/rspb.2018.2127
Skelton, J., Jusino, M. A., Li, Y., Bateman, C., Thai, P. H., Wu, C., et al. (2018). Detecting symbioses in complex communities: the fungal symbionts of bark and ambrosia beetles within Asian pines. Microb. Ecol. 76, 839–850. doi: 10.1007/s00248-018-1154-8
Vanderpool, D., Bracewell, R. R., and McCutcheon, J. P. (2018). Know your farmer: Ancient origins and multiple independent domestications of ambrosia beetle fungal cultivars. Mol. Ecol. 27, 2077–2094. doi: 10.1111/mec.14394
Keywords: cooperative breeding, bark beetle, insect agriculture, symbiosis, fungus community, social behavior, fungus-farming, mutualism
Citation: Biedermann PHW (2020) Cooperative Breeding in the Ambrosia Beetle Xyleborus affinis and Management of Its Fungal Symbionts. Front. Ecol. Evol. 8:518954. doi: 10.3389/fevo.2020.518954
Received: 10 December 2019; Accepted: 29 September 2020;
Published: 04 November 2020.
Edited by:
Heikki Helanterä, University of Oulu, FinlandReviewed by:
Duur Kornelis Aanen, Wageningen University and Research, NetherlandsMichael Poulsen, University of Copenhagen, Denmark
Veronica M. Sinotte, University of Copenhagen, Denmark, in collaboration with reviewer MP
Copyright © 2020 Biedermann. This is an open-access article distributed under the terms of the Creative Commons Attribution License (CC BY). The use, distribution or reproduction in other forums is permitted, provided the original author(s) and the copyright owner(s) are credited and that the original publication in this journal is cited, in accordance with accepted academic practice. No use, distribution or reproduction is permitted which does not comply with these terms.
*Correspondence: Peter H. W. Biedermann, peter.biedermann@uni-wuerzburg.de