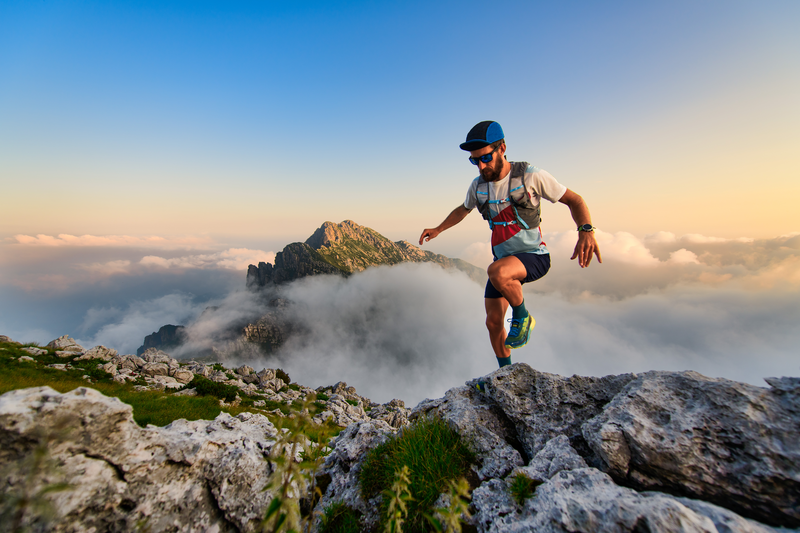
95% of researchers rate our articles as excellent or good
Learn more about the work of our research integrity team to safeguard the quality of each article we publish.
Find out more
ORIGINAL RESEARCH article
Front. Ecol. Evol. , 22 October 2020
Sec. Urban Ecology
Volume 8 - 2020 | https://doi.org/10.3389/fevo.2020.501502
This article is part of the Research Topic Patterns and Drivers of Urban Biodiversity View all 8 articles
Recreational urban parks support diverse assemblages of plants that through their functions, contribute beneficial services to billions of individuals throughout the world. Drivers of vegetation-derived services in parks are complex, as climate and park management interact with the functioning of multiple species of vegetation types. Yet, informal observations suggest that recreational parks are constructed consistently to specific principles of landscape design. Here we ask: what are the patterns of functional traits and vegetation diversity in cities of varying climate in the United States, and how do these patterns result in a consistent typology of recreational park? We hypothesized that increased aridity would exclude species not adapted to warm, dry climates, thereby reducing local, or alpha, taxonomic diversity and shifting community composition. However, a similar preference of park managers in the United States for suites of service-based functional traits leads to similarity of mean values of services traits in recreational parks among cities, regardless of climate differences. We tested this hypothesis by surveying lawn species, comprised of herbaceous turf and spontaneous plants, and woody species in fifteen recreational parks across Baltimore MD, Riverside CA, and Palm Springs CA, three cities that contain multiple parks but differ in regional climate. With increasing aridity, taxonomic alpha diversity decreased and plant physiology shifted, yet no differences were observed among most service-based functional traits. Among the cities surveyed, no significant differences were observed in functional dispersion of woody and spontaneous species or most service-based traits. Taxonomic composition differed in each city for all vegetation types, while suites of service-based traits differed between Baltimore and the two more arid cities of Riverside and Palm Springs. Our results suggest that across the United States, service-based functional traits are consistent, even when arising from unique compositions and abundances of species in recreational parks. We interpret these results as an interaction between climate and the preferences of recreation park managers for services, creating a pattern of vegetation diversity where taxonomic alpha and beta diversity vary among regions while specific suites of services remain available.
Urban vegetation comprises novel ecosystems by bringing together native and non-native plant species of no natural analog (Hobbs et al., 2014; Aronson et al., 2017). In cities across climates and cultures, one finds novel vegetation arrangements within recreational parks (Threlfall et al., 2016; Weems, 2016; Talal and Santelmann, 2019). Recreational parks are a public form of green infrastructure that provides services to individuals through access to recreation, cooler temperatures, and improvement to overall health (Bolund and Hunhammar, 1999; Larson L. R. et al., 2016; Ayala-Azcárraga et al., 2019). These urban greenspaces contain multiple species of trees planted to provide esthetic benefits and shade as well as large expanses of green turf for recreation and gathering (Tinsley et al., 2002; Pataki et al., 2013; Talal and Santelmann, 2019). However, specific services provided to individuals and neighborhoods by planted vegetation may be contingent on the diversity of species and vegetation function (Larson K. L. et al., 2016; Vieira et al., 2018).
Examining patterns of taxonomic and functional diversity among communities allows us to understand the drivers behind local species assembly and functioning (Anderson et al., 2011; Johnson et al., 2015). What drives urban diversity is a blend of biophysical and social factors (Padullés Cubino et al., 2018). Climate and management preferences can determine the expression of plant functional traits in parks, from the physiological of leaves to esthetic characteristics preferred by people (McCarthy et al., 2011; Pataki et al., 2013; Avolio et al., 2018). Vegetation physiological function has also been linked to the provisioning of ecosystem service-based traits (Pataki et al., 2013; Swan et al., 2016). The influence of climate and management preference can vary depending on the type of vegetation studied (Nielsen et al., 2014). A meta-analysis of species richness in urban parks found out of ten studies focused on vascular plants, seven studies restricted results to only woody species (Nielsen et al., 2014). Other recent studies in urban green systems focus only on trees (Avolio et al., 2015; Gillespie et al., 2017; Kendal et al., 2018), or herbaceous turf and weedy species (Knapp et al., 2012; Wheeler et al., 2017; Padullés Cubino et al., 2018). To understand the causes of vegetation diversity in an urban plant community, we need to know how regional (climate) and local (management) influence different vegetation types in a specific urban community (Aronson et al., 2016), such as recreational parks.
Building on the framework of environmental filtering of communities (Ackerly, 2003; Spasojevic and Suding, 2012; Kraft et al., 2015), extreme aridity reduces physiological niche space, thus reducing taxonomic diversity and shifting physiological function of park vegetation (Spasojevic et al., 2014; Kraft et al., 2015; Pearse et al., 2018). Cities of extreme climates generally have lower species richness of urban trees and lawn vegetation compared to milder climates (Jenerette et al., 2016; Padullés Cubino et al., 2018). Within lawn communities, these patterns may arise due to differences in irrigation practices across cities, as well as differences in turf varietals (Kendal et al., 2018; Pincetl et al., 2019). Cities in more arid climates, such as Los Angeles and Phoenix, irrigate their lawns, while more mesic cities have both irrigated and non-irrigated lawns (Wheeler et al., 2017). Warmer climates also allow land managers to plant species of turf which use the C4 photosynthetic pathway, which can maintain productivity under high temperatures and aridity, while C3 turf species are more physiologically restricted, making them a poor choice for hot and dry cities environments (Wherley and Sinclair, 2009; Beard, 2013). Furthermore, lawn communities are often colonized by spontaneously growing herbaceous species that has a greater range of functional properties compared to turf, potentially allowing more spontaneous species to pass through environmental filters that limit turf species (Robinson and Lundholm, 2012).
While taxonomic composition and physiological functions are strongly driven by climate, similar preferences for suites of traits related to specific service-based functions among urban residents may decrease functional diversity of urban plant communities (Fukami et al., 2005; Larson K. L. et al., 2016). The service-based traits chosen for recreational parks reflect how park managers design park vegetation, where the park tree composition can provide services such as shade and visual esthetics not found in the local native communities (Avolio et al., 2018). Lawns provide similar services across cities, such as vibrant greenness and resiliency to active use (Larson K. L. et al., 2016). However, the effect of preference in parks on service-based traits can differ depending on the plant community and type of trait. In warmer cities, there can be an increase in service-based traits relating to showy flowers and fruits, however across a gradient of aridity, differences in tree heights or leaf areas have not been identified (Jenerette et al., 2016; Pearse et al., 2018).
Service-based functional traits can have distributions less limited by climate compared to ecophysiological traits. For example, esthetic flower production is a valued service trait not limited by climate due to seasonally available flowering species in most regions (Konijnendijk et al., 2005; Threlfall et al., 2016; Goodness, 2018). However, esthetic fall foliage is prevalent in broadleaf deciduous species, which are likely to be found mostly in biomes like temperate deciduous forests. Preferences allow for similarity of esthetic flower traits across climates while having fewer effects on traits of esthetic fall foliage. Alternatively, spontaneous vegetation is not actively planted, making its dispersal more limited than horticultural vegetation (De Wet and Harlan, 1975). After undergoing selection by dispersal and environmental filters, the selected traits of spontaneous species in recreational parks are those that can overcome mowing, weeding, and physical dispersal barriers. Overall, these at times independent variations of taxonomic, ecophysiological, and service-based diversity implies multiple drivers may determine the pattern of diversity in urban park vegetation.
While visually one can see that recreational parks appear similar in form to one another regarding their design, the question remains who controls that typology and the diversity found within? Urban park tree diversity has been found to respond to different physical and social drivers compared to residential yards and street trees (Kendal et al., 2012). What vegetation is found in recreational parks is ultimately a function of the park managers who decide what is planted. A recent study based in Portland OR found the reasons behind vegetation design in recreational parks are often a function of economics, maintenance of the park environment from human disturbance, and a desire to provide beneficial services for visitors (Talal and Santelmann, 2020). Similar management desires for economy of maintenance were found in surveys of park managers in Hong Kong (Chan et al., 2014). The differences between park visitor preferences and manager preferences can often be due to budget constraints (Baur et al., 2013), where park visitors may desire an increase in colorful foliage and more flowers, managers are looking for ways to improve maintenance on a restricted budget (Talal and Santelmann, 2020). While park managers may have similar goals in different countries, the species diversity found in parks is often determined by national and local horticultural trends (Pincetl et al., 2013; Nielsen et al., 2014; Roman et al., 2018), as well as regional climate (Jenerette et al., 2016).
To better understand how climate and management preferences influence the distributions of recreation park diversity, we ask: how do park biodiversity, community composition, and values of service-based traits differ among three mid-sized United States cities of varying climates? We predict that alpha diversity will vary among recreational parks in arid and mesic cities due to the physiological tolerances of vegetation, and that each city will have a unique taxonomic beta diversity. Functional diversity and service provisioning, however, will not differ across climates owing to the preference of park managers for a specific typology of vegetation composition and provided services within recreational parks. To resolve the gap spanning multiple vegetation types within the same community, we focus on three different vegetation types, including horticultural woody species and lawn communities comprised of turfgrass and spontaneous herbaceous vegetation. By quantifying patterns in taxonomic and functional diversity and their relationship to services provisioning in recreational parks, we describe how broad-scale climatic variables interact with the preferences of recreational park managers to influence park vegetation diversity and function.
We assessed climate and park managers preference as drivers of park vegetation biodiversity by sampling 15 recreational parks located in three cities within the United States: Baltimore MD, Riverside CA, and Palm Springs CA (Figure 1 and Table 1). Cities were selected to represent a gradient of aridity (measured as the vapor pressure deficit, VPD). Baltimore (mean summer VPD: 0.89 kPa) is in a temperate humid climate characterized by the regular precipitation events throughout the growing season. Riverside (mean summer VPD: 2.7 kPa) is in a Mediterranean climate of intermediate aridity and Palm Springs (mean summer VPD: 6.2 kPa) is in a desert climate of extreme aridity, and both cities are characterized by seasonal precipitation during winter and early spring with very little precipitation during summer and fall. Within Riverside and Palm Springs, urban vegetation health is maintained through regular irrigation throughout the year. In each city, we selected five recreational parks. We focused only on highly managed recreational parks, rather than natural parks or parks with remnant natural areas, to highlight effects of park managers’ preferences for recreation. Recreational parks are characterized by large lawn areas with multiple species of planted, maintained woody species. Lawns, along with spontaneous species found within the lawn, are the only herbaceous species present in the park and no area is unmanaged. To test whether species-area relationships influence diversity (Nielsen et al., 2014; van der Maarel and Franklin, 2015), our parks represented a range of areas, from 10,000 to 300,000 m2. Park size was determined via ArcGIS 10.6 (ESRI, Redlands, CA, United States) polygon delineation (Table 1). Our field sampling protocol was designed to collect all taxonomic data for the five parks within each region over 7 days of field work in each city. To assess climate as a driver of physiological function, parks were sampled during mid-afternoon in peak summer months. Our experimental protocol is designed to be transferrable to other cities to characterize recreational park vegetation can within a approximately 5 days.
Figure 1. Study area of cities of interest. Five recreational parks were sampled in each city. All parks are representative of a recreational park typology, comprising expansive lawns and individually planted trees. Images are sourced from Google Earth Pro.
We assessed entire woody species assemblages in each park. We sampled herbaceous species by generating four randomized points within the mowed lawn areas of each selected park. From each point, we randomly placed a 0.5 × 0.5 m quadrat in each cardinal direction. We calculated the total cover of all species in the quadrat using a modified Braun-Blanquet method (Braun-Blanquet, 1932; van der Maarel, 2007), which included the following cover classes: (0) absent, (1) >1% (individual), (2) 1–5%, (3) 5–10%, (4) 10–25%, (5) 25–50%, (6) 50–75%, and (7) 75–100% cover. This methodology resulted in a total of 16 sample quadrats per park. Sports fields within parks (e.g., baseball diamonds, soccer fields, etc.) were not included in quadrat surveys. We identified all herbaceous species in each quadrat and classified them as turf or spontaneous species.
Our research protocol is designed for maximum repeatability, even by researchers without access to physiology wet labs. To that end we collected functional data in silico, using trait databases and primary literature. To incorporate functional diversity metrics into our analysis of recreational park species, we used the BIEN database (Maitner et al., 2018), the Global Wood Density Database (Chave et al., 2009) and primary literature to assemble average ecophysiological (EP) traits for each sampled species (Table 2). Following Westoby’s leaf-height-seed plant ecology strategy scheme, we included traits that relate to a holistic ecological strategy: Specific Leaf Area, Seed Dry Mass, and Height (Westoby, 1998). To incorporate traits that relate to hydraulic architecture in stems, we included wood density as an additional trait (Swenson and Enquist, 2007; Chave et al., 2009) for woody species. To analyze influences of park manager preference, we included a series of service-based traits for woody species (ES). These traits are based on desired attributes found in nursery stock (Avolio et al., 2018). We included traits reflecting shade and fruit provisioning, as well as flower, fruit, foliage, and bark esthetics. While there are many service-based traits found in horticultural vegetation, such as carbon capture (Pataki et al., 2006), pollution and dust mitigation (Wang et al., 2019), and microclimate regulation (Shiflett et al., 2017), we chose to focus on traits that were more associated with attributes preferred by urban residents and urban land managers (Pataki et al., 2013; Larson K. L. et al., 2016). We used horticultural sources to determine ES traits for each woody species (Table 2). ES traits were analyzed as presence/absence, where if a horticulture record listed a trait as associated with the species, that trait was given a value of 1 and if no record of the traits was found, we recorded a value of 0. We only used species that had records for all trait factors for subsequent analyses. As there was an unequal number of species containing all ES and all EP traits, we separated woody species into two categories to capture all species with a full spectrum of service-based traits, and all species with a full spectrum of EP traits.
Table 2. Functional traits used for analysis, grouped into “Service-based trait” and “EcoPhysiological” suites.
We analyzed herbaceous species as a lawn, encompassing all herbaceous species, as well as separated by functional groups of turf species or spontaneous species. As turf and spontaneous species were all herbaceous, we did not include wood density as an EP trait and focused only on those traits in the Leaf-Height-Seed strategy. ES traits were not calculated for turf or spontaneous species. Turf is selected based on its ability to persist; thus if turf is present a singular selectable service is being provided (Christians et al., 2016). Spontaneous species are not preferentially selected for planting. We filled gaps in trait data through searches of primary sources (Table 2).
We conducted all statistical analyses in R (R Core Team. 2019). For each vegetation type we created a site by species matrix, each site being one sampled recreational park. Taxonomic alpha diversity of vegetation types in parks was represented by the Shannon-Wiener index calculated in the vegan package in R (Oksanen et al., 2008). Statistical significance was determined using one-way ANOVA calculated for each cover type; differences among cities were determined with a Tukey’s HSD post hoc test. Taxonomic beta diversity between any two parks was calculated using Brey-Curtis distance, and visualized through Principle Coordinates Analysis (PCoA). Within-city beta diversity was defined as the Euclidean distance to centroid, and significance was determined through a PERMANOVA, while differences in composition were determined using a pairwise PERMANOVA in the RVaideMemorie package in R (Maxime, 2019). To examine differences in beta diversity, we calculated dissimilarity matrices between all parks in the study, using both taxonomic and functional characteristics. Functional beta diversity was calculated using Gowers distance. Any trait distribution that did not fit a normal distribution was log-transformed and all trait data were z-transformed before any multivariate analysis was completed. Using PERMANOVA, we tested for compositional shifts in taxonomic and functional diversity for all vegetation types, and visualized results by the PCoA. The dissimilarity matrices allowed us to calculate homogeneity of variance through PERMANOVA and we identified compositional shifts in taxonomic and functional diversity.
Functional alpha diversity was defined as the functional dispersion (FDis) of each park. FDis is an abundance-weighted measure of the distribution of functional traits in a community and quantifies the range of functional strategies within a community (Laliberte and Legendre, 2010). To quantify individual functional metrics, we calculated community weighted trait means (CWM) for each trait. CWM values provide a single-trait metric that is weighted by species abundances within a sampled community (Zuo et al., 2016). FDis and CWM analyses were completed using the FD package in R (Laliberté et al., 2015).
Across all cities, a total of 187 woody species, 9 turf species, and 44 spontaneous species were identified. Of the 187 woody species, we obtained physiological traits from databases for 107 species and service-based traits for 178 species. Woody species with no available trait information were removed from functional analyses. We obtained EP traits for all turf varieties and 36 of the 44 spontaneous species. Turf species included cool- and warm-season grasses that commonly comprise grass seed mixtures for lawns: Festuca arundinacea (C3), Poa annua (C3), Agrostis palustris (C3), Lolium perenne (C3), Poa pratense (C3), Paspalum notatum (C4), Buchloe dactyloides (C4), Stenotaphrum secundatum (C4), Eremochloa ophiuroides (C4), and Zoysia spp. (C4).
Taxonomic and functional alpha diversity was first analyzed to determine if park area acted as a significant driver. Park area was not correlated with either taxonomic or functional alpha diversity. We then conducted subsequent analyses investigating city-wide climate as a primary driver of diversity metrics.
For both woody and turf vegetation, taxonomic alpha diversity, measured as Shannon-Weiner diversity, was lower in recreational parks of Palm Springs compared to the more mesic city of Baltimore (Woody species EP traits, p = 0.011; woody species ES traits, p = 0.021; turf species, p = 0.008). Differences in Shannon diversity of woody vegetation between Riverside and either Baltimore or Palm Springs were not detected. Differences in lawn taxonomic diversity were observed between the mesic Baltimore and the two drier cities of Riverside (p = 0.050) and Palm Springs (p = 0.010). However, when isolating turf and spontaneous species, the turf in Palm Springs was different compared to the other two cities (Palm Springs – Riverside p = 0.024, Palm Springs – Baltimore p = 0.008) and no differences were found when comparing spontaneous species assemblages (p = 0.115) (Figure 2A).
Figure 2. Metrics of alpha diversity for each vegetation type (mean and standard deviation). Asterix refer to significant differences between groups tested through ANOVA with a post hoc Tukey HSD test (*0.001 < p < 0.05). Herbaceous species are both spontaneous and turf species (A) Taxonomi.c alpha diversity was measured by Shannon-Weiner Diversity, (B) Functional alpha diversity (FDis) of woody species, (C) FDis of herbaceous type species. FDis was measured by Functional Dispersion, an abundance weighted metric of functional trait ranges.
Functional alpha diversity, FDis, of both EP and ES traits in woody species were not significantly different among the three cities (EP p = 0.487; ES p = 0.659) (Figure 2A). Likewise, FDis of all lawn species and only spontaneous species were not significantly different across cities (p = 0.247). Within turf species, there was a significant difference in city-scale FDis means (p = 0.034). No significant difference of FDis means was found between Baltimore (mean FDis = 0.206) and Riverside (mean FDis = 0.200), however Palm Springs (mean FDis = 0.047) values were significantly lower than Baltimore (p = 0.050) (Figure 2C).
Woody EP traits all showed shifts in CWM across cities. The three traits associated with the Leaf-Height-Seed strategy all decreased from Baltimore to Riverside and Palm Springs (SLA: Baltimore – Riverside p < 0.001, Baltimore - Palm Springs p < 0.001; Height: Baltimore – Palm Springs p = 0.002; Seed Dry Mass: Baltimore – Riverside p < 0.001, Baltimore – Palm Springs p < 0.001). Wood density increased from Baltimore to Riverside and Palm Springs (Baltimore – Riverside p = 0.031, Baltimore – Palm Springs p = 0.001) (Figure 3A). Overall values of woody ES traits display little dissimilarity among cities. Shade and fruit provisioning, and esthetic traits of flowers, fruit and bark were not different across our study cities. In each city, the highest value for any service-based trait was that of shade provisioning. The lone service functional trait which exhibited differences among cities was that of esthetic fall foliage; more trees associated with the fall foliage trait were found in Baltimore compared to Riverside and Palm Springs (Baltimore – Riverside p < 0.001, Baltimore – Palm Springs p < 0.001) (Figure 3B).
Figure 3. Community weighted means (CWM) of woody functional traits (mean and standard deviation). Asterix refer to significant differences between groups tested through ANOVA with a post hoc Tukey HSD test (*0.001 < p < 0.05, ***p < 0.0001). (A) Traits related to ecophysiological characteristics; (B) Traits related to service-based functional trait characteristics.
Within lawn species, only seed dry mass differed across cities, with Palm Springs having significantly lower values than Baltimore (p = 0.031). Similar results were observed in spontaneous species, where seed dry mass was higher in Baltimore compared to Riverside and Palm Springs (Baltimore-Riverside, p = 0.016; Baltimore-Palm Springs, p = 0.012). Turf species in Riverside showed larger specific leaf area compared to Baltimore (p = 0.021), while vegetation height in all herbaceous vegetation groups was not different among cities (Figure 4).
Figure 4. CWM of ecophysiological traits of herbaceous, turf, and spontaneous species (mean and standard deviation). Asterix refer to significant differences between groups tested through ANOVA with a post hoc Tukey HSD test (∗p < 0.05, ∗∗p < 0.001, ∗∗∗p < 0.0001). Observe that y-axis varies for each trait type.
All cities displayed a significant difference in taxonomic composition from each other except in lawn species composition between Riverside and Palm Springs (Figure 5A, p values displayed in Table 3). In a pairwise PERMANOVA, we found no difference between all lawn and only spontaneous functional composition among cities; woody ES and EP and turf composition were significantly different in Baltimore compared to both Riverside and Palm Springs but not between the two more arid cities (Figure 5 and Table 3B). No differences were found in the distance to centroid of taxonomic compositions among the cities or vegetation types. Regarding functional composition, only woody physiological composition showed a significant difference in variance around the centroid between parks in Baltimore and Palm Springs (Table 3A).
Figure 5. Principal Coordinates Analysis of Beta Diversity for (A) Taxonomic Diversity, and (B) Functional Diversity, for all vegetation types. Point represent a sampled park and are colored and labeled based on city of location. Difference of points is calculated as bray-curtis distance. Ellipses are one standard deviation of the data.
Table 3. (A) P-values for homogeneity of multivariate dispersions, by group and post hoc results. (B) P-values for difference between centroids of groups, ANOVA and post hoc.
The decrease of taxonomic alpha diversity with increasing aridity is consistent with our hypothesis of climate filtering. In arid cities like Palm Springs, the number of woody and turf grass species that can withstand physiological stress is limited compared to the horticultural species found in more mesic cities (Pearse et al., 2018; Figure 2). In recreational parks, taxonomic diversity is further filtered by park planners selecting species that provide specific desired services (Talal and Santelmann, 2019). The increased selection pressure of park management combined with extreme aridity could explain why we found a decrease in diversity while other studies found a positive correlation between species diversity of all urban trees and warmer climates (Jenerette et al., 2016). Conclusions based on broad sampling of urban vegetation differ when confining studies to a specific type of green infrastructures, such as residential lawns or recreational parks as in this study, where management practices regarding planting and watering are more specialized (Pearse et al., 2018). As increasingly arid climates appear to reduce species diversity within recreational parks, differences in climate also result in unique taxonomic and functional composition in recreational parks across cities (Figure 5).
All vegetation types in recreation parks have unique compositions among our study cities. We hypothesized that to achieve desired services, the taxonomic composition will vary with minimal effect on the values of service-based traits. Unique taxonomic compositions can vary across cities due to climate-driven changes in the availability of urban vegetation (Jenerette et al., 2016), temporally through variability of nursery offerings (Pincetl et al., 2013), or depending on local consumer preferences (Conway and Vander Vecht, 2015; Roman et al., 2018). While many species found in recreational parks are not native to the region (Wheeler et al., 2017; Talal and Santelmann, 2019), the lower Shannon diversity in Palm Springs and the difference in composition among all cities, explain how horticultural vegetation physiologically responds to the native climate.
For recreational parks to maintain healthy vegetation, planted vegetation must be able to functionally acclimate to regional climate. Lower specific leaf area is often connected to adaptations that confer tolerance to drought, such as reduced wilting and ability to maintain photosynthesis in extremely arid environments (Poorter et al., 2009; De Micco and Aronne, 2012). The higher wood density seen in arid urban park trees can lower cavitation risk, and mortality caused by extreme aridity may be better avoided by these species than by species of lower wood densities (Wright et al., 2004; Savi et al., 2015). However, resistance to drought is likely a co-benefit as park managers are more concerned with planting for ease of maintenance and economic reasons, and high wood density is associated with long lived trees which would need to fewer replacements (Díaz et al., 2015; Talal and Santelmann, 2020). This ecological tradeoff between stress tolerance and growth is also found in the trait of seed dry mass. Species adapted to arid regions generally have seeds with lower dry mass to prevent desiccation before precipitation brings on germination (Westoby, 1998). Recreational park tree species found in arid regions exhibit adaptations to reduce heat and drought stress by having lower specific leaf area, seed dry mass, and higher wood density. Variation in functional traits (Figure 3A) among cities will result in park visitors in Baltimore experiencing a different functional composition than in Riverside and Palm Springs (Figure 5B).
The photosynthetic pathway of C4 turfgrasses allows for continuous transpiration during extreme heat and aridity, and in a well-irrigated Palm Springs park there is plenty of water for turf to maintain productivity. In a mild city like Baltimore, there are better conditions for C3 turf to highly productive year-round. However, while the physiological traits of the C3 turf in Baltimore and the C4 turf in Palm Springs were similar, the range of trait values was smaller in Palm Springs. This result is indicative of fewer varieties of C4 turf grass for planting compared to C3 varieties in nurseries throughout the country (Trammell et al., 2019).
Spontaneous species are not subject to the same environmental or preferential drivers as turf species (Niinemets and Peñuelas, 2008; Knapp et al., 2012). Yet, many varieties of spontaneous species persist in cities and are of similar functional type (Wheeler et al., 2017), resulting in similar physiological traits and taxonomic diversity. Spontaneous species maintained distinct compositions among cities, while the turf species were less dissimilar (Figure 5A). However, unlike turf composition, spontaneous functional composition was not significantly different across cities. For spontaneous species to be found within a recreational park lawn, the species must disperse and germinate within the climate of the city, and then establish despite management and recreational activities enacted on lawns (Abu-Dieyeh and Watson, 2005; Anderson and Minor, 2019). Adaptations to regional climate may explain the variety in lawn taxonomy that we observed, while the adaptation to intense human impacts could explain the lack of difference in functional composition.
Depending on the specific functional trait, management preference or climate can be a greater influence on park plants. The functional responses specific leaf area in turf and seed mass in spontaneous species are potential evidence of aridity creating regions of unique taxonomic and functional compositions (Figure 4). Regarding suites of service-based traits, Baltimore’s climate allows for a greater abundance of species with the highly valued trait of esthetic fall foliage, as evidenced by the Maryland Department of Natural Resources’ weekly fall updates tracking the changing of the leaves (Maryland Department of Natural Resources 2019). This unique difference in a single climate driven trait can drive significant changes in total service-based trait composition in recreational parks (Figure 5B). Similarly, while fruit production is not a preferential service-based trait by park managers, we did find a greater abundance of fruit trees in the Palm Springs and Riverside regions, which could be indicative to the legacy of citrus in southern Californian agriculture (Farmer, 2013).
In recreational parks, woody species are generally planted to provide valued service traits of shade, and esthetic appreciation (Pataki et al., 2013; Avolio et al., 2018) and turf is planted to provide the service of vegetative greenness and recreational play areas (Ayala-Azcárraga et al., 2019). Park trees are spaced far enough apart for esthetics to be appreciated while providing copious but fragmented shaded areas, creating a physical arrangement of vegetation where similar service traits are available across cities (Goodness, 2018; Talal and Santelmann, 2019). While regional aridity is correlated with park vegetation physiology, the preference for specific values of ES traits is generally similar across urban regions. Similarity of service-based traits in recreational parks allows park visitors, who value certain services, to have similar experiences in cities regardless of regional horticulture. While our study cities exhibited a unique functional composition of woody EP and ES traits, we found no differences in FDis or compositional variation (Figure 2B and Table 3A). We suggest this pattern represents an example of park managers’ preference for a similar arrangement of function in recreation parks across cities, despite a regionally varying species diversity. Parks in Palm Springs achieve a comparable range of functional breadth to milder cities while exhibiting a unique taxonomic and function composition and a significantly smaller taxonomic diversity (Figures 2, 3, 5).
One of the defining characteristics of woody species in recreational parks is that they are not a monoculture; there is generally a variety of tree species (Jim and Chen, 2008; Nielsen et al., 2014). Moreover, surveys of urban residents have identified specific highly valued service-based traits in trees, such as shade, beauty, and fruit production (Avolio et al., 2018). Variation in the desires of urban residents result in a large variety of species that can provide these specific services (Avolio et al., 2018; Talal and Santelmann, 2020). Therefore, a wide variety of woody species will result in a similar FDis of physiological traits, even when the distribution of species is different. While parks may provide varying amounts of services, the similarity of dispersion of service-based traits in cities with differing Shannon diversity implies management practices that select for a specific range of service functions in a park.
Woody species may not be a monoculture, but our results show that planted turf acts like a plantation. We interpret this result as evidence that the highest value service trait is that it remains green and usable for recreation and is easily maintained (Christians et al., 2016; Larson K. L. et al., 2016). If a minimal number of species available can achieve this goal, there is little need to expand planting to species with unique functions. Maintenance regimes can be standardized to the similar taxa, which fits with the stated desires of park managers to minimize maintenance costs (Chan et al., 2014; Talal and Santelmann, 2020). There are other varieties of native grasses or sedges that are viable in regions of high aridity, yet these varieties are not commonly cultivated as turf.
Interestingly, we did not find significant relationships between park area and alpha diversity, either taxonomic or functional. This is in contradiction to the recent review of diversity drivers in urban parks (Nielsen et al., 2014). The discrepancy between our study and current literature could arise from our focus on urban recreational parks, where the entire park area is actively managed only for recreation (Weems, 2016). Conversely, the review by Nielsen et al. (2014) includes parks managed for recreation, agriculture, and natural areas. Including multiple varieties of urban park incorporates multiple habitats as well, which influence species-area relationships.
While we used observations of urban park vegetation as evidence of management preferences, there are other management interactions outside of vegetation preference that can influence the diversity in a community. Fertilization, pesticide application, prioritizing play equipment, and access to local nurseries can all lead to variations in taxonomic and functional vegetation diversity (Kjelgren and Clark, 1993; Politi Bertoncini et al., 2012; Chan et al., 2014; Cavender-Bares et al., 2020). Urban soil profiles can be both heterogeneous within and among cities (Crum et al., 2016; Herrmann et al., 2018). Regional climate and human facilitation are major filters leading to taxonomic and functional diversity (Aronson et al., 2016; Pearse et al., 2018), however, future work would be inclined to explore other potential narrower urban filters on recreational park diversity.
Climate and management preferences both play key roles in determining recreational park structure and composition, through driving differences in taxonomic alpha and beta diversity while maintaining similarity in the value and distribution of service-based traits. We show that regional climate drivers affect the taxonomic diversity and composition of each city’s parks. Furthermore, the number of functional strategies also reflects a stabilization of FDis among cities. By showing how Shannon diversity of woody and turf species both responds to climatic shifts, FDis of woody species and turf species diverge in this regard, we can infer woody species FDis is influenced by park management preference while turf has a strong limitation to extreme climates. Integrating woody, turf, and spontaneous vegetation with multiple metrics of diversity allows for these results and answers a call for work to incorporate multiple functional types within a singular study (Nielsen et al., 2014). To develop a comprehensive diversity framework for entire cities, future work should incorporate more cities to represent more regional climates and differences in local horticultural preference.
Following the paradigms of urban ecology “in”, “of” and “for” the city, our hypothesis required a synthesis of both climate drivers and the influence of park managers’ preference to understand the patterns of recreational park diversity (Pickett et al., 2016). Our study synthesizes a biotic (ecology “in” the city) and a social-ecological (ecology “of” the city) influencer of diversity and our results provide a rationale for why specific vegetation types and functions are more influenced by either climate or preference. Our results show the influence of management preference guiding diversity in recreational parks, where service-based traits and FDis (excepting turf) does not change while taxonomic alpha diversity decreases into arid regions. Using our approach, we can identify functional co-benefits that could enhance the selection of park vegetation to provide climate resiliency along with traditional park management. The baseline days of extreme heat in Palm Springs are projected to increase from 135 to 179 days >35°C by the end of the century (Sun et al., 2015), creating opportunities for the current stewardship for service-based function to shift in these extreme cities as the availability of viable vegetation decreases. By incorporating the results of this study with local urban park planning are cities at risk of extreme heat, we can move to practicing ecology “for” the city as well.
The datasets generated for this study can be found in the 10.6086/D1FT1R.
PI, DB, CS, and GJ designed the study. PI, DB, and MR collected all the data for the study. PI and DB completed the statistical analysis of the data. PI wrote the manuscript. All authors contributed to the editing of the manuscript and approved the submitted version.
This work was supported by NSF CBE – 1444758 and CNH – 1924288.
The authors declare that the research was conducted in the absence of any commercial or financial relationships that could be construed as a potential conflict of interest.
We would like to acknowledge the various park management organizations that allowed us to sample within the study sites. We also like to acknowledged Holly Andrews, Dion Kuchera, and Stuart Schwab who provided critique on the final manuscript.
Abu-Dieyeh, M., and Watson, A. (2005). Impact of mowing and weed control on broadleaf weed population dynamics in turf. J. Plant Interact. 1, 239–252. doi: 10.1080/17429140601015226
Ackerly, D. D. (2003). Community Assembly, Niche Conservatism, and Adaptive Evolution in Changing Environments. Evolution 164, 164–184.
Anderson, E. C., and Minor, E. S. (2019). Assessing social and biophysical drivers of spontaneous plant diversity and structure in urban vacant lots. Sci. Total Environ. 653, 1272–1281. doi: 10.1016/j.scitotenv.2018.11.006
Anderson, M. J., Crist, T. O., Chase, J. M., Vellend, M., Inouye, B. D., Freestone, A. L., et al. (2011). Navigating the multiple meanings of β diversity: a roadmap for the practicing ecologist. Ecol. Lett. 14, 19–28. doi: 10.1111/j.1461-0248.2010.01552.x
Aronson, M. F., Nilon, C. H., Lepczyk, C. A., Parker, T. S., Warren, P. S., Cilliers, S. S., et al. (2016). Hierarchical filters determine community assembly of urban species pools. Ecology 97, 2952–2963. doi: 10.1890/07-1861.1
Aronson, M. F. J., Lepczyk, C. A., Evans, K. L., Goddard, M. A., Lerman, S. B., MacIvor, J. S., et al. (2017). Biodiversity in the city: key challenges for urban green space management. Front. Ecol. Environ. 15:189–196. doi: 10.1002/fee.1480
Avolio, M. L., Pataki, D. E., Gillespie, T. W., Jenerette, G. D., McCarthy, H. R., Pincetl, S., et al. (2015). Tree diversity in southern California’s urban forest: the interacting roles of social and environmental variables. Front. Ecol. Evol. 3:73. doi: 10.3389/fevo.2015.00073
Avolio, M. L., Pataki, D. E., Trammell, T. L. E., and Endter-Wada, J. (2018). Biodiverse cities: the nursery industry, homeowners, and neighborhood differences drive urban tree composition. Ecol. Monogr. 88, 259–276. doi: 10.1002/ecm.1290
Ayala-Azcárraga, C., Diaz, D., and Zambrano, L. (2019). Characteristics of urban parks and their relation to user well-being. Landsc. Urban Plan. 189, 27–35. doi: 10.1016/j.landurbplan.2019.04.005
Baur, J. W. R., Tynon, J. F., and Gómez, E. (2013). Attitudes about urban nature parks: a case study of users and nonusers in Portland. Oregon. Landsc. Urban Plan. 117, 100–111. doi: 10.1016/j.landurbplan.2013.04.015
Beard, J. B. (2013). Origins of North American Turfgrasses in Turfgrass: Biology, Use, and Management Agronomy Monograph SV - 56. Madison, WI: American Society of Agronomy, 1–35. doi: 10.2134/agronmonogr56.c1
Bolund, P., and Hunhammar, S. (1999). Ecosystem services in urban areas. Ecol. Econ. 29, 293–301. doi: 10.1016/S0921-8009(99)00013-0
Braun-Blanquet, J. (1932). Plant Sociology. The Study of Plant Communities. New York, NY: McGraw-Hill Book Co., Inc.
Cavender-Bares, J., Padullés Cubino, J., Pearse, W. D., Hobbie, S. E., Lange, A. J., Knapp, S., et al. (2020). Horticultural availability and homeowner preferences drive plant diversity and composition in urban yards. Ecol. Appl. 0, 1–16. doi: 10.1002/eap.2082
Chan, C.-S., Marafa, L. M., and Van Den Bosch, C. C. K. (2014). Changing perspectives in urban park management: a longitudinal study of Hong Kong. Manag. Leis. 20, 1–21. doi: 10.1080/13606719.2014.944411
Chave, J., Coomes, D., Jansen, S., Lewis, S. L., Swenson, N. G., and Zanne, A. E. (2009). Towards a worldwide wood economics spectrum. Ecol. Lett. 12, 351–366. doi: 10.1111/j.1461-0248.2009.01285.x
Christians, N. E., Patton, A. J., and Law, Q. D. (2016). Fundamentals of Turfgrass Management: Fifth Edn. Hoboken, New Jersey: Wiley. doi: 10.1002/9781119308867
Conway, T. M., and Vander Vecht, J. (2015). Growing a diverse urban forest: species selection decisions by practitioners planting and supplying trees. Landsc. Urban Plan. 138, 1–10. doi: 10.1016/j.landurbplan.2015.01.007
Crum, S. M., Liang, L. L., and Jenerette, G. D. (2016). Landscape position influences soil respiration variability and sensitivity to physiological drivers in mixed-use lands of Southern California. USA. J. Geophys. Res. Biogeosci. 121, 2530–2543. doi: 10.1002/2016JG003469
De Micco, V., and Aronne, G. (2012). “Morpho-Anatomical Traits for Plant Adaptation to Drought,” in Plant Responses to Drought Stress: From Morphological to Molecular Features, ed. R. Aroca (Berlin: Springer), 37–61. doi: 10.1007/978-3-642-32653-0_2
De Wet, J. M. J., and Harlan, J. R. (1975). Weeds and Domesticates: Evolution in the man-made habitat. Econ. Bot. 29, 99–108. doi: 10.1007/BF02863309
Díaz, S., Kattge, J., Cornelissen, J. H. C., Wright, I. J., Lavorel, S., Dray, S., et al. (2015). The global spectrum of plant form and function. Nature 529, 1–17. doi: 10.1038/nature16489
Fukami, T., Bezemer, T. M., Mortimer, S. R., and Van Der Putten, W. H. (2005). Species divergence and trait convergence in experimental plant community assembly. Ecol. Lett. 8, 1283–1290. doi: 10.1111/j.1461-0248.2005.00829.x
Gillespie, T. W., de Goede, J., Aguilar, L., Jenerette, G. D., Fricker, G. A., Avolio, M. L., et al. (2017). Predicting tree species richness in urban forests. Urban Ecosyst. 20, 839–849. doi: 10.1007/s11252-016-0633-2
Goodness, J. (2018). Urban landscaping choices and people’s selection of plant traits in Cape Town, South Africa. Environ. Sci. Pol. 85, 182–192. doi: 10.1016/j.envsci.2018.02.010
Herrmann, D. L., Schifman, L. A., and Shuster, W. D. (2018). Widespread loss of intermediate soil horizons in urban landscapes. Proc. Natl. Acad. Sci. U.S.A. 115, 6751–6755. doi: 10.1073/pnas.1800305115
Hobbs, R. J., Higgs, E., Hall, C. M., Bridgewater, P., Chapin, F. S., Ellis, E. C., et al. (2014). Managing the whole landscape: historical, hybrid, and novel ecosystems. Front. Ecol. Environ. 12:557–564. doi: 10.1890/130300
Homer, C. G., Fry, J. A., and Barnes, C. A. (2012). The National Land Cover Database. Reston, VA: NLCD, doi: 10.3133/fs20123020
Jenerette, G. D., Clarke, L. W., Avolio, M. L., Pataki, D. E., Gillespie, T. W., Pincetl, S., et al. (2016). Climate tolerances and trait choices shape continental patterns of urban tree biodiversity. Glob. Ecol. Biogeogr. 25, 1367–1376. doi: 10.1111/geb.12499
Jim, C. Y., and Chen, W. Y. (2008). Pattern and divergence of tree communities in Taipei’s main urban green spaces. Landsc. Urban Plan. 84, 312–323. doi: 10.1016/j.landurbplan.2007.09.001
Johnson, A. D., and Gerhold, H. D. (2001). Carbon storage by utility-compatible trees. J. Arboric. 27, 57–68.
Johnson, A. L., Tauzer, E. C., and Swan, C. M. (2015). Human legacies differentially organize functional and phylogenetic diversity of urban herbaceous plant communities at multiple spatial scales. Appl. Veg. Sci. 18, 513–527. doi: 10.1111/avsc.12155
Kendal, D., Dobbs, C., Gallagher, R. V., Beaumont, L. J., Baumann, J., Williams, N. S. G., et al. (2018). A global comparison of the climatic niches of urban and native tree populations. Glob. Ecol. Biogeogr. 27, 629–637. doi: 10.1111/geb.12728
Kendal, D., Williams, N. S. G., and Williams, K. J. H. (2012). Drivers of diversity and tree cover in gardens, parks and streetscapes in an Australian city. Urban For. Urban Green. 11, 257–265. doi: 10.1016/j.ufug.2012.03.005
Kjelgren, R. K., and Clark, J. R. (1993). Growth and water relations of liquidambar-styraciflua L in an urban park and Plaza. Trees Struct. Funct. 7, 195–201. doi: 10.1007/BF00202073
Knapp, S., Dinsmore, L., Fissore, C., Hobbie, S. E., Jakobsdottir, I., Kattge, J., et al. (2012). Phylogenetic and functional characteristics of household yard floras and their changes along an urbanization gradient. Ecology 93, 83–98. doi: 10.1890/11-0392.1
Konijnendijk, C. C., Nilsson, K., Randrup, T. B., and Schipperijn, J. (2005). Urban Forest and Trees. Berlin: Springer.
Kraft, N. J. B., Adler, P. B., Godoy, O., James, E. C., Fuller, S., and Levine, J. M. (2015). Community assembly, coexistence and the environmental filtering metaphor. Funct. Ecol. 29, 592–599. doi: 10.1111/1365-2435.12345
Kröber, W., Böhnke, M., Welk, E., Wirth, C., and Bruelheide, H. (2012). Leaf trait-environment relationships in a subtropical broadleaved forest in South-East China. PLoS One 7:e35742. doi: 10.1371/journal.pone.0035742
Laliberte, E., and Legendre, P. (2010). A distance-based framework for measuring functional diversity from multiple traits A distance-based framework for measuring from multiple traits functional diversity. Ecology 91, 299–305. doi: 10.1890/08-2244.1
Laliberté, E., Legendre, P., and Shipley, B. (2015). FD: Measuring Functional Diversity from Multiple Traits, and Other Tools for Functional Ecology. R Packag., Version 1.0-12. Available online at: http://cran.r-project.org/web/packages/FD/FD.pdf.
Larson, K. L., Nelson, K. C., Samples, S. R., Hall, S. J., Bettez, N., Cavender-Bares, J., et al. (2016). Ecosystem services in managing residential landscapes: priorities, value dimensions, and cross-regional patterns. Urban Ecosyst. 19, 95–113. doi: 10.1007/s11252-015-0477-1
Larson, L. R., Jennings, V., and Cloutier, S. A. (2016). Public parks and wellbeing in urban areas of the United States. PLoS One 11:e153211. doi: 10.1371/journal.pone.0153211
Maitner, B. S., Boyle, B., Casler, N., Condit, R., Donoghue, J., Durán, S. M., et al. (2018). The bien r package: a tool to access the botanical information and ecology network (BIEN) database. Methods Ecol. Evol. 9, 373–379. doi: 10.1111/2041-210X.12861
McCarthy, H. R., Pataki, D. E., and Jenerette, G. D. (2011). Plant water-use efficiency as a metric of urban ecosystem services. Ecol. Appl. 21, 3115–3127. doi: 10.1890/11-0048.1
Nielsen, A. B., van den Bosch, M., Maruthaveeran, S., and van den Bosch, C. K. (2014). Species richness in urban parks and its drivers: a review of empirical evidence. Urban Ecosyst. 17, 305–327. doi: 10.1007/s11252-013-0316-1
Niinemets, Ü, and Peñuelas, J. (2008). Gardening and urban landscaping: significant players in global change. Trends Plant Sci. 13, 60–65. doi: 10.1016/j.tplants.2007.11.009
Oksanen, J., Kindt, R., Legendre, P., O’Hara, B., Simpson, G. L., Solymos, P. M., et al. (2008). The vegan package. Community Ecol. Packag. 190.
Padullés Cubino, J., Cavender-Bares, J., Hobbie, S. E., Pataki, D. E., Avolio, M. L., Darling, L. E., et al. (2018). Drivers of plant species richness and phylogenetic composition in urban yards at the continental scale. Landsc. Ecol. 34, 63–77. doi: 10.1007/s10980-018-0744-7
Pataki, D. E., Alig, R. J., Fung, A. S., Golubiewski, N. E., Kennedy, C. A., Mcpherson, E. G., et al. (2006). Urban ecosystems and the North American carbon cycle. Glob. Chang. Biol. 12, 2092–2102. doi: 10.1111/j.1365-2486.2006.01242.x
Pataki, D. E., McCarthy, H. R., Gillespie, T., Jenerette, G. D., and Pincetl, S. (2013). A trait-based ecology of the Los Angeles urban forest. Ecosphere 4, 1–20. doi: 10.1890/ES13-00017.1
Pearse, W. D., Bares, J. C., Hobbie, S. E., Avolio, M. L., Bettez, N. D., Chowdhury, R. R., et al. (2018). Homogenization of plant diversity, composition, and structure in North American urban yards. Ecosphere 9:e02105. doi: 10.1002/ecs2.2105
Pickett, S. T. A., Cadenasso, M. L., Childers, D. L., McDonnell, M. J., and Zhou, W. (2016). Evolution and future of urban ecological science: ecology in, of, and for the city. Ecosyst. Heal. Sustain. 2:e01229. doi: 10.1002/ehs2.1229
Pincetl, S., Gillespie, T. W., Pataki, D. E., Porse, E., Jia, S., Kidera, E., et al. (2019). Evaluating the effects of turf-replacement programs in Los Angeles. Landsc. Urban Plan. 185, 210–221. doi: 10.1016/j.landurbplan.2019.01.011
Pincetl, S., Prabhu, S. S., Gillespie, T. W., Jenerette, G. D., and Pataki, D. E. (2013). The evolution of tree nursery offerings in Los Angeles County over the last 110 years. Landsc. Urban Plan. 118, 10–17. doi: 10.1016/j.landurbplan.2013.05.002
Politi Bertoncini, A., Machon, N., Pavoine, S., and Muratet, A. (2012). Local gardening practices shape urban lawn floristic communities. Landsc. Urban Plan. 105, 53–61. doi: 10.1016/j.landurbplan.2011.11.017
Poorter, H., Niinemets, Ü, Poorter, L., Wright, I. J., and Villar, R. (2009). Causes and consequences of variation in leaf mass per area (LMA): a meta-analysis. New Phytol 182, 565–588. doi: 10.1111/j.1469-8137.2009.02830.x
Robinson, S. L., and Lundholm, J. T. (2012). Ecosystem services provided by urban spontaneous vegetation. Urban Ecosyst. 15, 545–557. doi: 10.1007/s11252-012-0225-8
Roman, L. A., Pearsall, H., Eisenman, T. S., Conway, T. M., Fahey, R. T., Landry, S., et al. (2018). Human and biophysical legacies shape contemporary urban forests: a literature synthesis. Urban For. Urban Green 31, 157–168. doi: 10.1016/j.ufug.2018.03.004
Savi, T., Bertuzzi, S., Branca, S., Tretiach, M., and Nardini, A. (2015). Drought-induced xylem cavitation and hydraulic deterioration: risk factors for urban trees under climate change? New Phytol. 205, 1106–1116. doi: 10.1111/nph.13112
Schmid, R., and Brenzel, K. N. (2001). Sunset Western Garden Book. Oakland, CA: Sunset Publishing Corporation. doi: 10.2307/1223916
Shiflett, S. A., Liang, L. L., Crum, S. M., Feyisa, G. L., Wang, J., and Jenerette, G. D. (2017). Variation in the urban vegetation, surface temperature, air temperature nexus. Sci. Total Environ. 579, 495–505. doi: 10.1016/j.scitotenv.2016.11.069
Spasojevic, M. J., Grace, J. B., Harrison, S., and Damschen, E. I. (2014). Functional diversity supports the physiological tolerance hypothesis for plant species richness along climatic gradients. J. Ecol. 102, 447–455. doi: 10.1111/1365-2745.12204
Spasojevic, M. J., and Suding, K. N. (2012). Inferring community assembly mechanisms from functional diversity patterns: the importance of multiple assembly processes. J. Ecol. 100, 652–661. doi: 10.1111/j.1365-2745.2011.01945.x
Sun, F., Walton, D. B., and Hall, A. (2015). A hybrid dynamical–statistical downscaling technique, Part II: end-of-century warming projections predict a new climate state in the los angeles region. J. Clim. 28, 4618–4636. doi: 10.1175/JCLI-D-14-00197.1
Swan, C. M., Johnson, A., and Nowak, D. J. (2016). Differential organization of taxonomic and functional diversity in an urban woody plant metacommunity. Appl. Veg. Sci. Online Ver. 20, 7–17. doi: 10.1111/avsc.12266
Swenson, N. G., and Enquist, B. J. (2007). Ecological and evolutionary determinants of a key plant functional trait: wood density and its community-wide variation across latitude and elevation. Am. J. Bot. 94, 451–459. doi: 10.3732/ajb.94.3.451
Talal, M. L., and Santelmann, M. V. (2019). Plant community composition and biodiversity patterns in urban parks of portland. Oregon. Front. Ecol. Evol. 7:201. doi: 10.3389/fevo.2019.00201
Talal, M. L., and Santelmann, M. V. (2020). Vegetation management for urban park visitors: a mixed methods approach in Portland. Oregon. Ecol. Appl. 30:e02079. doi: 10.1002/eap.2079
Threlfall, C. G., Ossola, A., Hahs, A. K., Williams, N. S. G., Wilson, L., and Livesley, S. J. (2016). Variation in vegetation structure and composition across urban green space types. Front. Ecol. Evol 4:66. doi: 10.3389/fevo.2016.00066
Tinsley, H. E. A., Tinsley, D. J., and Croskeys, C. E. (2002). Park usage, social milieu, and psychosocial benefits of park use reported by older urban park users from four ethnic groups. Leis. Sci. 24, 199–218. doi: 10.1080/01490400252900158
Trammell, T. L. E., Pataki, D. E., Still, C. J., Ehleringer, J. R., Avolio, M. L., Bettez, N., et al. (2019). Climate and lawn management interact to control C 4 plant distribution in residential lawns across seven U.S. cities. Ecol. Appl. 29, e01884. doi: 10.1002/eap.1884
van der Maarel, E. (2007). Transformation of cover-abundance values for appropriate numerical treatment - Alternatives to the proposals by Podani. J. Veg. Sci. 18, 767–770. doi: 10.1111/j.1654-1103.2007.tb02592.x
van der Maarel, E., and Franklin, J. (2015). Vegetation Ecology, 2nd Edn. Hoboken, NJ: John Wiley & Sons, doi: 10.1017/CBO9781107415324.004
Vieira, J., Matos, P., Mexia, T., Silva, P., Lopes, N., Freitas, C., et al. (2018). Green spaces are not all the same for the provision of air purification and climate regulation services: The case of urban parks. Environ. Res. 160, 306–313. doi: 10.1016/j.envres.2017.10.006
Wang, H., Maher, B. A., Ahmed, I. A., and Davison, B. (2019). Efficient removal of ultrafine particles from diesel exhaust by selected tree species: implications for roadside planting for improving the quality of urban air. Environ. Sci. Technol. 53, 6906–6916. doi: 10.1021/acs.est.8b06629
Weems, C. M. (2016). Examining the Spatial Distribution of Park Access and Trajectories of Gentrification in Seattle, Washington 1990 - 2010. PhD Diss. Oregon State. Corvallis, OR: Oregon State University, 302.
Westoby, M. (1998). A leaf-height-seed (LHS) plant ecology strategy scheme. Plant Soil 199, 213–227. doi: 10.1023/A:1004327224729
Wheeler, M. M., Neill, C., Groffman, P. M., Avolio, M., Bettez, N., Cavender-Bares, J., et al. (2017). Continental-scale homogenization of residential lawn plant communities. Landsc. Urban Plan. 165, 54–63. doi: 10.1016/j.landurbplan.2017.05.004
Wherley, B. G., and Sinclair, T. R. (2009). Differential sensitivity of C3 and C4 turfgrass species to increasing atmospheric vapor pressure deficit. Environ. Exp. Bot. 67, 372–376. doi: 10.1016/j.envexpbot.2009.07.003
Wright, I. J., Reich, P. B., Westoby, M., Ackerly, D. D., Baruch, Z., Bongers, F., et al. (2004). The worldwide leaf economics spectrum. Nature 428, 821–827. doi: 10.1038/nature02403
Zanne, A. E., Lopez-Gonzalez, G., Coomes, D. A., Ilic, J., Jansen, S., Lewis, S. L., et al. (2009). Data from: towards a worldwide wood economics spectrum. Ecol. Lett. doi: 10.5061/dryad.234
Keywords: ecosystem services, park, biodiversity, functional diversity, urban, park management
Citation: Ibsen PC, Borowy D, Rochford M, Swan CM and Jenerette GD (2020) Influence of Climate and Management on Patterns of Taxonomic and Functional Diversity of Recreational Park Vegetation. Front. Ecol. Evol. 8:501502. doi: 10.3389/fevo.2020.501502
Received: 30 September 2019; Accepted: 22 September 2020;
Published: 22 October 2020.
Edited by:
Serge Morand, Centre National de la Recherche Scientifique (CNRS), FranceReviewed by:
Francisco J. Escobedo, Del Rosario University, ColombiaCopyright © 2020 Ibsen, Borowy, Rochford, Swan and Jenerette. This is an open-access article distributed under the terms of the Creative Commons Attribution License (CC BY). The use, distribution or reproduction in other forums is permitted, provided the original author(s) and the copyright owner(s) are credited and that the original publication in this journal is cited, in accordance with accepted academic practice. No use, distribution or reproduction is permitted which does not comply with these terms.
*Correspondence: Peter C. Ibsen, cGlic2UwMDFAdWNyLmVkdQ==
Disclaimer: All claims expressed in this article are solely those of the authors and do not necessarily represent those of their affiliated organizations, or those of the publisher, the editors and the reviewers. Any product that may be evaluated in this article or claim that may be made by its manufacturer is not guaranteed or endorsed by the publisher.
Research integrity at Frontiers
Learn more about the work of our research integrity team to safeguard the quality of each article we publish.