- 1Department of Botany and Biodiversity Research, University of Vienna, Vienna, Austria
- 2Bird Collection, 1st Zoological Department, Museum of Natural History Vienna, Vienna, Austria
- 3Konrad Lorenz Research Center, Core Facility for Behaviour and Cognition, University of Vienna, Grünau im Almtal, Austria
- 4Department of Behavioural & Cognitive Biology, University of Vienna, Vienna, Austria
Human-induced climate change and the destruction of natural habitats are two of the main threats to biodiversity worldwide. Animals can use local weather conditions as environmental cues for optimal breeding conditions, but climate change can cause severe phenological mismatches. Migratory species that have a shorter time period for their settlement decision, or species that breed in heavily transformed habitats, might be specifically sensitive to such change. Here, we analyzed the arrival and egg-laying dates of Eurasian kestrels (Falco tinnunculus) in Vienna (415 km2), Austria, gathered by academic and citizen scientists between 2010 and 2018. To identify critical time windows in which weather variables affect phenology, we used a sliding window approach and considered the degree of urbanization as an additional predictor to unravel habitat-dependent relationships. Furthermore, we assessed the relationship between arrival and egg-laying (i.e., the length of the time gap in-between). We found that arrival dates were not influenced by urbanization, and that egg-laying started earlier in drier weather conditions prior to arrival, and earlier in more natural areas than in the urban center. The time gap between arrival and egg-laying was slightly shorter in breeding pairs that arrived later at their nest sites. Our results might indicate a strategy to mitigate later arrival by relatively earlier egg-laying through reducing the length of the courtship period. Such a behavioral adaptation could avoid negative effects of a later onset of breeding on their reproductive success, which is known from previous studies in our urban population.
Introduction
Human-induced rapid environmental change (Sih, 2013), such as climate change and destruction of natural habitats, threatens biodiversity globally, posing new challenges throughout all habitats and from the species to community level (Pimm and Raven, 2000; Walther et al., 2002; Root et al., 2003; Parmesan, 2006; Hendry et al., 2017). Due to the continuous growth of human populations and associated resource use (Vitousek, 1994), both climate and land-use change are expected to intensify further within this century (Sala et al., 2000). In fact, the United Nations Secretariat considers climate change and land-use change in form of urbanization to be the most significant sources of global environmental change (United-Nations, 2019).
One key mechanism induced by global change is phenological shift, i.e., an altered timing of seasonal life cycle activities or events (Walther et al., 2002), which influences animal reproduction and population viability (Walther et al., 2002; Parmesan and Yohe, 2003; Root et al., 2003; Parmesan, 2006; Jetz et al., 2007; Møller et al., 2008; Visser et al., 2012). These shifts might be caused by changing abiotic factors, for instance, snowmelt affecting the flowering of plants (Inouye, 2008), but also entail biotic interactions (Miller-Rushing et al., 2010). However, phenological shifts can be positive or negative. For example, earlier springs and longer frost-free seasons with reduced snowfall advance flowering of plants and egg-laying in birds (Zohner, 2019), which might promote the exploitation of newly available resources (Miller-Rushing et al., 2010) with associated fitness advantages. On the other hand, such shifts can cause temporal mismatches between trophic levels, e.g., plants and pollinators or predators and prey (Stenseth and Mysterud, 2002; Edwards and Richardson, 2004; Visser and Both, 2005; Visser et al., 2006; Both et al., 2009; Renner and Zohner, 2018). In urban surroundings, phenological shifts could be exacerbated by the urban heat island effect (Oke, 1982; Streutker, 2003) as urban core areas across the globe have consistently higher ambient temperatures and less pronounced fluctuations in diel temperatures than their adjacent non-urban areas (reviewed by Arnfield, 2003). Many bird species breeding in urban areas respond with an advanced reproductive phenology, e.g., laying earlier than their non-urban conspecifics (Deviche and Davies, 2014).
The seasonality of the environment restricts time windows suitable for reproduction or growth of organisms (Visser and Both, 2005). For breeding birds, the question of when and where to breed determines their own and their offspring’s fitness (Daan and Tinbergen, 1997). Although birds are highly mobile, they are inherently dependent on their imminent surroundings as soon as they start breeding. To optimize reproduction, birds need to time the hatching of their young as close to the environmental optimum as possible, but they need to initiate the breeding process well before this environmental optimum occurs (Perrins, 1970; Daan et al., 1989). Consequently, predictions of ideal conditions – especially the sufficient availability of suitable food to raise offspring – are based on environmental (Baker, 1938; Murton and Westwood, 1977; Wingfield, 1983) but also social cues at the time before breeding [‘conspecific attraction’ (Stamps, 1994) or ‘public information hypothesis’ (Danchin et al., 1998; Doligez et al., 2003)].
Long-term environmental predictors for breeding birds of the temperate zone are photoperiodical cues (reviewed by Sharp, 1996). But the longer the time gap between the perception of the cue(s) and the fitness consequences, the higher the probability of mismatches (Padilla and Adolph, 1996). This becomes even more challenging in a severely altered environment, such as urban core areas, because here species face different environmental conditions than they experienced in the fitness landscape they evolved in (Tilman and Lehman, 2001).
To adjust the fine-tuning to local conditions, additional factors closer to the actual onset of breeding might be important predictors to find the environmental optimum (Visser et al., 2010), especially spring temperatures [i.e., less than 1 month before egg-laying; see review (Williams et al., 2015)]. Getting the timing right matters, because earlier clutches have more eggs, higher hatching and fledging rates and earlier broods are more likely to recruit into the breeding population (Perrins, 1970; Verboven and Visser, 1998; Grüebler and Naef-Daenzer, 2010; Sumasgutner et al., 2016; Harriman et al., 2017). The fitness advantages of earlier broods can be attributed to the quality of the environment, which naturally deteriorates as the season advances [‘date hypothesis’ (Perrins, 1970); see also reviews of Verhulst and Nilsson (2008) and Harriman et al. (2017)], and to the individual quality of the parents, enabling an earlier increase of the female’s fitness toward breeding condition [‘quality hypothesis’ within the Ideal Dominance Distribution or Ideal Despotic Distribution (Fretwell, 1972), e.g., through superior hunting skills of the male, body condition of the female, quality of the occupied territory, and previous breeding experience (Perrins, 1970; Drent and Daan, 1980; Newton, 1980; Chastel et al., 1995; Forslund and Pärt, 1995; Daunt et al., 1999; Kokko, 1999; Sergio et al., 2007)]. Parents may have to face a trade-off considering breeding benefits (which might be related to the date hypothesis) as well as fitness costs (which might be related to the quality hypothesis) associated with the timing of breeding (Verhulst and Nilsson, 2008; Grüebler and Naef-Daenzer, 2010). Similarly, earlier-arriving individuals in migratory species are usually fitter than later-arriving ones, which settle on progressively lower-quality territories (‘sequential settlement’; Sergio et al., 2007).
One species that appears intrinsically resilient to urbanization and even proliferates in human-modified environments is the Eurasian kestrel Falco tinnunculus (hereafter ‘kestrel’). In this study, we analyze long-term data (2010–2018) on arrival from wintering grounds and the timing of breeding from the kestrel population in Vienna, Austria. The kestrel is the most common raptor species of the Palearctic region (Ferguson-Lees and Christie, 2005) and Vienna holds the highest density of non-colonial breeding individuals (Wichmann et al., 2009; Sumasgutner et al., 2014a). In natural areas, kestrels mainly breed in deserted or usurped corvid nests or in cliff cavities (Village, 1990), but they opportunistically use anthropogenic structures available in agricultural areas (Costantini et al., 2014; Sumasgutner et al., 2019b) and urban settings (Kübler et al., 2005; Sumasgutner et al., 2014a, b). If voles, which are the kestrel’s main prey, decline in availability due to natural cycles (Korpimäki, 1986; Valkama et al., 1995) or become less available due to urbanization (Sumasgutner et al., 2014a), kestrels adjust by broadening their diet width and hunting habitat (Korpimäki, 1986; Valkama et al., 1995; Kübler et al., 2005; Mikula et al., 2013; Sumasgutner et al., 2013; Kreiderits et al., 2016) and by increasing hunting effort and enlarging hunting areas (Riegert et al., 2007a,b). Consequentially, there is a strong implication that kestrels are urban adaptors.
Previous studies showed that weather parameters during winter, arrival and courtship affect breeding productivity of kestrels in Vienna; higher precipitation in winter and also spring rainfall reduces productivity, probably due to delayed egg-laying (Kreiderits et al., 2016). In Mediterranean kestrels, dry and mild winters reduce breeding productivity, but at the same time higher spring rainfall delays egg-laying dates (Costantini et al., 2010b), indicating that different breeding stages are sensitive to different time-windows. Thus, it remains a worthy endeavor to identify which weather predictors are the most relevant during which period throughout the breeding cycle. Besides photoperiodism, these critical time windows might be additional cues for settlement decisions, hence, having a significant effect on the reproduction of kestrels in general and explaining large-scale variation of egg-laying dates within the Western Palearctic (Meijer et al., 1992; Carrillo and González-Dávila, 2009). However, if photoperiodism is the sole predictor, an adaptive response to global change would be heavily limited.
In this study we first (i) determine the critical time window(s) (start and duration) and key weather variables which predict egg-laying dates of kestrels in Vienna. We expected the critical time window(s) to coincide with the winter before breeding, arrival and the courtship period. This could be evident in either (a) several shorter critical time windows, or (b) one long critical time window starting before arrival and ending during courtship. We furthermore expected precipitation to be the strongest predictor with a larger effect in more natural compared to urban nest sites as cavities in buildings might provide better shelter than open nests. A similar assessment of the arrival time at the nest site after migration was not possible due to the lack of information where kestrels of the study population overwinter.
Second (ii), we investigate if the onset of breeding is determined by the arrival time itself. This would be the case if courtship duration (time gap from arrival until egg-laying) stays constant from early to late arrivals. Alternatively, egg-laying could be relatively earlier (shortening time gap) or relatively later (elongating time gap). Shortening of time gaps could indicate a strategy of mediating later arrival (and thus avoiding potential related fitness costs of living in urban environments for the parents) by a relatively earlier onset of breeding. Contrary, late arrival could result in an increased time gap between arrival and egg-laying due to worsening environmental conditions for reproduction over the course of the breeding season. Furthermore, these time gaps could vary depending on the degree of urbanization. For example, a shorter time gap at the most urbanized nest sites would indicate that the females reach breeding condition earlier in an urban setting than in suburban areas at the city’s periphery.
Our final objective was to build a global model to predict arrival time and egg-laying dates to gain a deeper understanding of the ecological processes shaping the population dynamics of kestrels along an urbanization gradient.
Materials and Methods
Study Species
The population density of kestrels in Vienna ranges between 89–122 breeding pairs/100 km2 (Sumasgutner et al., 2014b) and is relatively high compared to previously published estimates [60–96 breeding pairs (bp)/100 km2; Wichmann et al., 2009], densities reported in other European cities [e.g., 22.9–33.3 bp/100 km2 in Berlin, Germany (Kupko et al., 2000) or 40–55 bp/100 km2 in Paris, France (Malher et al., 2010)], and rural areas in Austria with 8–30 bp/100 km2 (Gamauf, 1991). Kestrels are considered partial migrants with a post-breeding migration that varies immensely with latitude (Village, 1990) and starts in September/October (Holte et al., 2016). It is yet unclear where the kestrel population of Vienna is migrating to. Unlike populations studies in other European cities (Romanowski, 1996; Riegert and Fuchs, 2011), kestrels in Vienna disperse in mid-summer and temporarily leave the city during winter (Sumasgutner et al., 2014a). Only a few, primarily male individuals are known to spend the winter within urban areas of Vienna (Sumasgutner et al., 2014b). Within Europe, females and juveniles travel larger distances than males and adults (Terraube et al., 2015). In Vienna, inner-city territories were occupied slightly before territories in more natural areas in 2010 and 2011, indicating a preference for inner-city nest sites (Sumasgutner et al., 2014a).
Study Area and Urbanization Gradient
Vienna (48°12′N, 16°22′E, 150–500 m a.s.l., 415 km2, 1.91 million inhabitants), the capital of Austria, is considered a ‘green’ city with approximately 50% of the total area being urban green space. We quantified a soil seal factor (SSF) as percentage of impervious/sealed surfaces within a buffer circle of r = 500 m (78.5 ha) around each nest site, corresponding to the lower end of kestrel hunting area sizes reported from Kiel, Germany (range of 90–310 ha; Beichle, 1980) and České Budějovice, Czech Republic (range of 80–2,500 ha; Riegert et al., 2007b). We chose the smaller scale for our home-range level urbanization score, as high densities (such as in kestrels in Vienna) usually result in smaller home range sizes in urban raptors (e.g., Dykstra et al., 2001; Rutz, 2006; Dykstra et al., 2018). The SSF was our proxy for an urbanization gradient. We excluded rural areas, defined as areas with SSF < 1%, which mainly consist of larger agricultural, forest and conservation areas located within the cities’ boundaries, thus limiting the study area to 243 km2.
The SSF was calculated using ArcMap (ESRI Inc., 2017) from land allocation maps provided by the Environmental Protection Bureau of Vienna. These maps indicated 51 different land cover categories, which were summarized into impervious/sealed and unsealed surfaces (Figure 1). 35 land cover categories, such as buildings, roadways, pavements or parking lots, were assumed to be sealed surfances. The remaining 16 land cover categories like unsealed yards, grasslands, forests, agricultural areas, wine yards, cemeteries, gravel pits and various sorts of water bodies were classified as unsealed soil (Supplementary Table S1). Since the land allocation maps are renewed within periods of 4 years, the SSF was calculated based on one map that was digitized between 2010 and 2013 for all nest sites occupied before 2014 and on a map that was digitized between 2014 and 2017 for all other nest sites. Thus, some nest sites which were occupied by kestrels in both time periods were attributed with two different SSFs respective to the year observed. The SSF of all observed nest sites ranged from 4.77 to 98.52%.
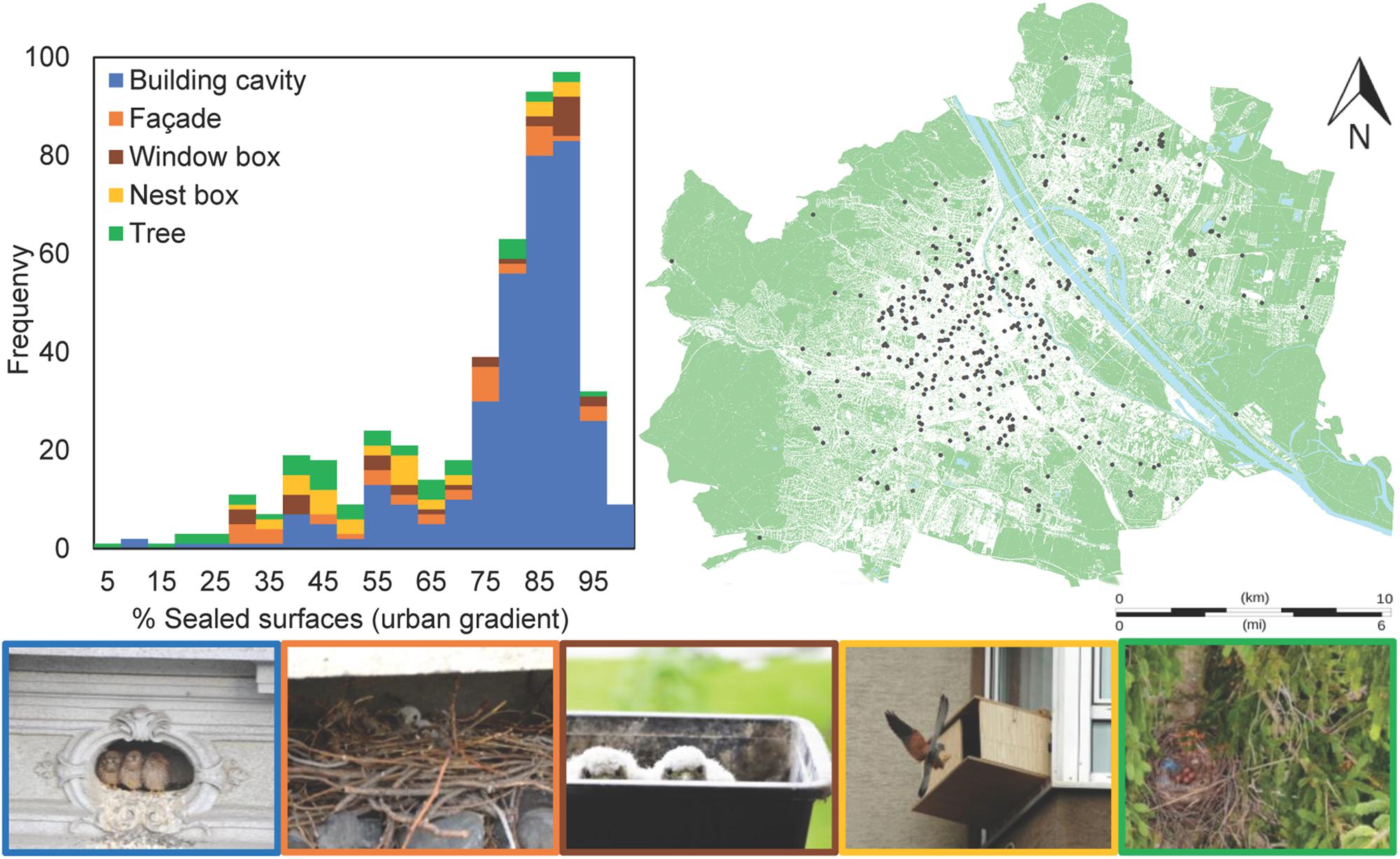
Figure 1. (Left) Distribution of nest types along the urban gradient (n = 484, 2010–2018): roof openings or other building cavities (66%), corvid nests on the façade (7%) or in trees (8%), window boxes (6%) and specifically provided nest boxes (6%). (Right) Location of nest sites monitored by citizen science community (occupied in at least 1 year; n = 366; gray points). Water bodies in blue, sealed surfaces in white and greenspace. (Below) Building cavity ©C Hofmann, corvid nest in façade ©M Graf, window box ©P Sumasgutner, nest box ©GF Witting and corvid nest in tree ©SC McPherson.
The Monitoring Program
The population has been monitored systematically since 2010 and incorporated systematic observations by academic scientists, data contributed by ornithologists involved in breeding-bird surveys and citizen science data reported by phone, e-mail, social media, or via BirdLife Austria and the online platform bird.at. The contribution of each citizen varied strongly in extent and quality. While plenty of citizens did not report observations repeatedly, various contributors became a distinctive part of the project, reliably reporting on the arrival and reproduction at “their” nest site season after season. Often comprehensive pictures of the brood were provided. All reports were documented and notifications of (yet) unknown contributors without any supporting footage were verified in situ by academic scientists.
The following analyses include all data reported from 2010 to 2018 by academic and citizen scientists. Arrival and egg-laying dates were extracted from the notes taken by academic scientists and, if necessary, backed up by revising written communication with contributing citizens. Several citizen scientists stated when they first observed the kestrels at their nest upon return from their wintering grounds. As kestrels show very prominent behavior at the nest site, we assumed the time gap between arrival at the nest site and first notice by the citizens to be quite short. In case no specific arrival date was reported, the first date of correspondence with the citizen scientists was used as a substitute.
Several nest sites in Vienna offer a direct view from buildings located vis-à-vis, facilitating surveys without nest disturbance. Nesting in flower boxes on windowsills easily allows for daily nest checks. However, several nest sites cannot be seen from a vantage point and can only be accessed via attics or façades, by climbing trees or with the help of the Vienna Fire Fighters, who supported the monitoring program since its very beginning. Observations from the ground can give relevant cues on phenology but cannot be as precise as direct nest checks. In 7 of 9 years of the monitoring program, nestlings of accessible nests were at least examined once, measured and ringed which allowed an age estimation based on morphometric measurements and a backdating of the hatching and egg-laying dates (Kostrzewa and Kostrzewa, 1987, 1993). Therefore, the egg-laying date was either observed directly, or deduced by calculating 30 days back from the observed or estimated hatching date (Village, 1990). We further specified if observations on the breeding stage were based on a direct assessment or citizen scientists.
Weather Data
Data on temperature and precipitation were obtained from the Central Institute for Meteorology and Geodynamics (ZAMG) as well as the Tutiempo Network, S.L. Both provide data from the weather station ‘Vienna inner-city,’ which is located in the 4th district of Vienna, 1.3 km from the center. Four different weather variables were analyzed separately: daily (24 h) average (Tave), maximum (Tmax) and minimum temperature (Tmin) (all given in°C), and daily total precipitation (PP, given in mm).
Statistical Analysis
All statistical analyses were performed in R 3.6.1. (R Development Core Team, 2020). Confidence intervals were set to 95% and significance is referred to as P ≤ 0.05. Arrival and egg-laying dates were processed as Julian days and followed a normal distribution. Of all recorded arrival dates, dates before the 1st of February and after the 21st of June were excluded (n = 11). Dates before 1st of February were likely to refer to males over-wintering in Vienna; dates after 21st of June were rare statistical outliers, which are likely to result from relocations after nesting failure.
In 2013 and 2018, we did not have a team member dedicated to correspond with our citizen scientists from the beginning of the breeding season as in other years, thus, data from these 2 years were excluded from the model on arrival dates (n = 3 each), leaving 515 observations to be analyzed (Supplementary Figure S1, left). For egg-laying dates, years 2016 and 2018 were excluded (n2016 = 0, n2018 = 6) as no banding (with age-assessment of offspring) took place, leaving n = 114 observations to be analyzed (Supplementary Figure S1, right). Additionally, arrival and egg-laying dates were documented at the same nest and within the same year in 68 cases, thus allowing for an exact assessment of the time gap between arrival and egg-laying (Δ Days). These observations (hereafter ‘corresponding observations’) were obtained irregularly in the years 2010–2012, 2014–2015, and 2017–2018.
To test hypothesis (i) and determine the time window(s) during which weather variables affect egg-laying dates (and hence, kestrels are sensitive to weather conditions), we followed a sliding window approach (Brommer et al., 2008; Williams et al., 2015). For this purpose, multiple Pearson’s correlations were calculated for the annual mean egg-laying dates and the average of weather variables for various alternative time windows (Figure 2). We chose the length to vary between 1–90 days and tested every possible time window within a period starting 90 days before the mean annual laying date (n = 29295 Pearson’s correlations). We performed the sliding window approach for each weather variable separately and calculated R2 values from the Pearson’s correlations.
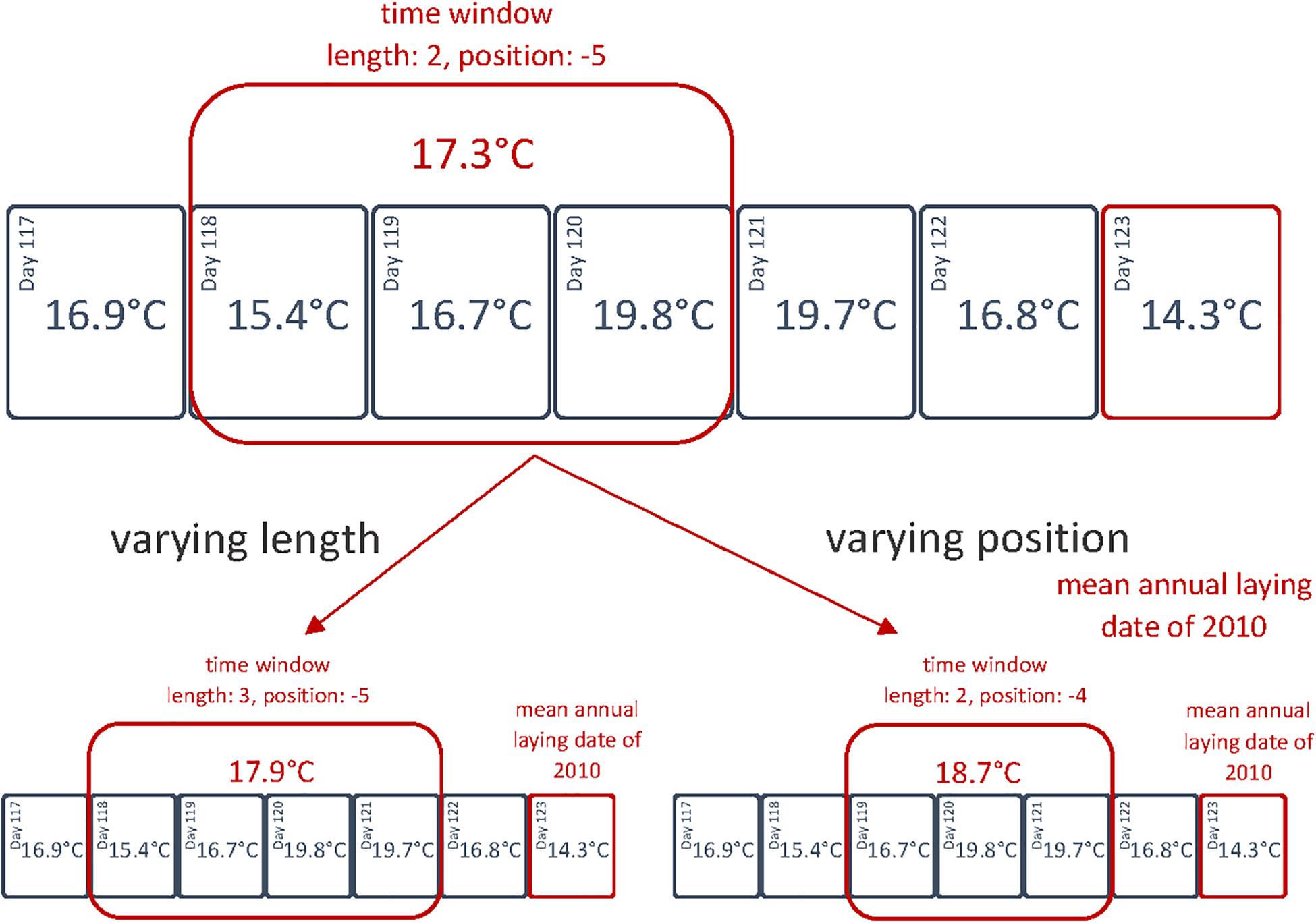
Figure 2. Illustration of the sliding window approach in 2010 for the daily average temperature. Each time window is defined by its length (in nights, therefore 0 includes 24 h) and position (starting point in days, with the mean annual laying date being day 0).
To test if (ii) the onset of breeding was already determined by the arrival time itself, we used the data set of corresponding observations mentioned above and performed a linear regression with Δ Days as dependent variable and arrival date as an independent variable, using the lm() command in the base package (R Development Core Team, 2020).
We calculated linear mixed-effect models (LMMs) via maximum likelihood with a Gaussian error distribution. We used the Julian date of arrival or egg-laying as a response variable, and fitted nest ID (as a random term), observer category (as fixed factor in two levels: ‘academic scientist’ versus ‘citizen scientist’), year of observation (as fixed factor), and SSF (as continuous fixed effect). For the model on egg-laying dates, we integrated the two most informative weather variables within the optimal time window assessed in (i). The arrival date was considered as an additional continuous fixed effect when working with the data set of corresponding observations.
All LMMs were built with lmer() in the package lme4 (Bates et al., 2015). Additionally, the packages LMERConvenienceFunctions (Tremblay and Ransijn, 2015) and car (Fox and Weisberg, 2019) were used for model validation by visual inspection of residuals. The conditional R2-values [hereafter ‘R2LMMc’; (Nakagawa et al., 2017) of the models were calculated with r.squaredGLMM() of the MuMIn package (Bartoń, 2016); significance of explanatory terms was assessed using their partial (Type III) significance values (χ2-tests)]. For data visualization, the additional packages ggplot2 (Wickham, 2009) and ggpubr (Kassambara, 2018) were used. Effect plots were made using the package lattice (Sarkar, 2008).
Results
Time Windows and Weather Variables Affecting Egg-Laying Date
Precipitation (PP) and maximum daily temperature (Tmax) proved to be the most informative weather variables with the highest explanatory capacity (Figure 3), while average daily temperature (Tave) was ranked second and daily minimum temperature (Tmin) appeared to be least informative (Supplementary Figure S2). For PP, we found several, partly overlapping critical time windows. Two patterns could be derived from the critical time windows (Figure 3). First, time windows incorporating data from the last 3 weeks before mean egg-laying showed little explanatory capacity, whilst windows ending about 20 days before the mean annual laying date had high R2-values (>0.5) but varied in position and length. Second, time windows of more than 3 weeks length which started from around 80 days before egg-laying were more informative, having R2-values > 0.5. Highest R2-values were recorded where the time windows of the two patterns were identical, with the maximum R2 = 0.82 associated with a time window of 58 days duration, starting 79 days (approx. 11 weeks) and ending 21 days (3 weeks) before egg-laying. Within this time window, PP was correlated with egg-laying date in a way that higher precipitation corresponded with later egg-laying [R(5) = 0.90, P = 0.005].
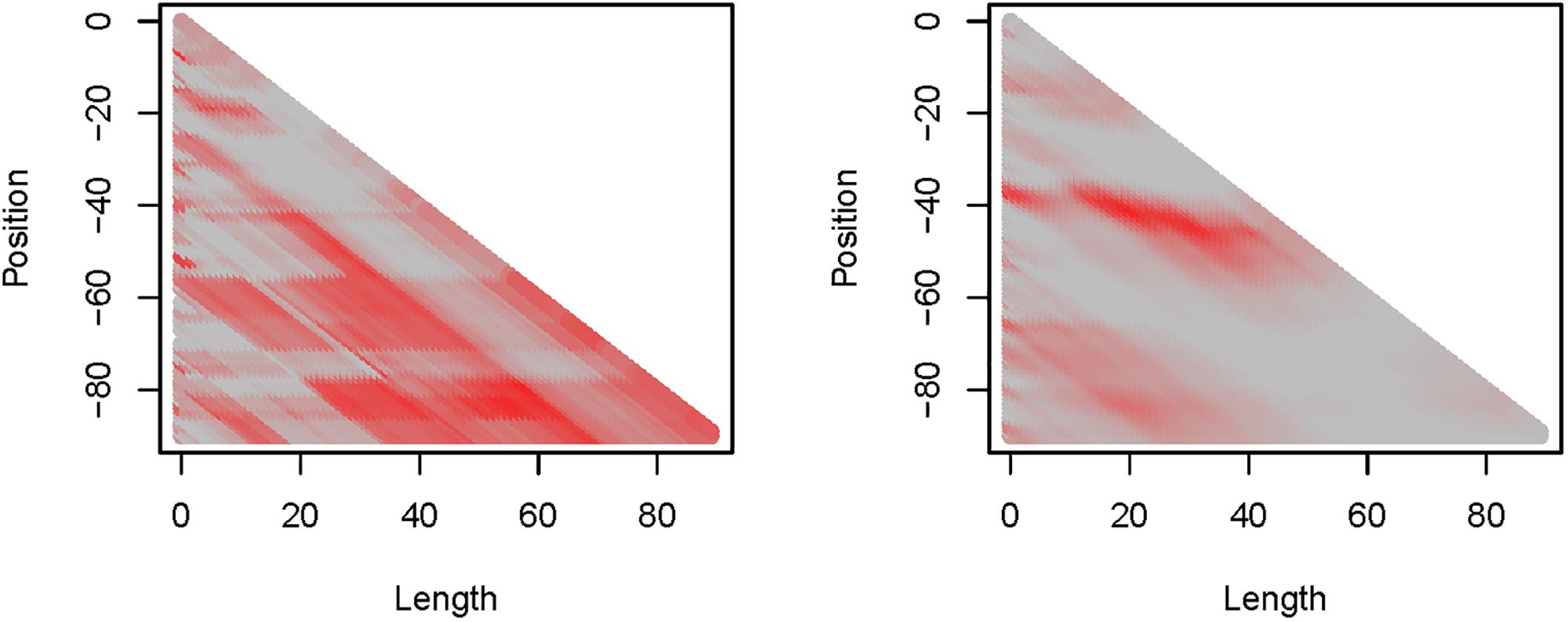
Figure 3. Depiction of R2-values derived by the sliding window approach on (left) precipitation (PP) and (right) daily maximum temperature (Tmax). Both show a maximum R2 = 0.82: PP at length = 58 and position = –79 (approx. 11–3 weeks before egg-laying), Tmax at length = 20 and position = –40 (approx. 6–3 weeks before egg-laying).
For Tmax, fewer critical time windows and fewer patterns were found compared to PP (Figure 3). Time windows of about 20 days that started approximately 1 month before egg-laying were most informative. The maximum R2 = 0.82 was assigned to a time window of 20 days duration, which started 40 days and ended 20 days before egg-laying. During this time window, Tmax was negatively correlated with later egg-laying dates [R(5) = −0.90, P = 0.005]. Among the other weather variables Tmin had the lowest R2-values [max. R2 = 0.63; R(5) = 0.79, P = 0.034] and Tave reached a maximum informative value of R2 = 0.71 [R(5) = −0.84, P = 0.017]. All temperature parameters (Tmin, Tmax, and Tave) were highly positively correlated with each other [Tmin vs. Tmax: r(1258) = 0.93, P < 0.001; Tmin vs. Tave: R(1258) = 0.97, P < 0.001; Tmax vs. Tave: R(1258) = 0.98; P < 0.001].
Arrival Date and Environmental Conditions
For arrival dates, we fitted SSF, observer category and year as fixed effects and nest ID as a random term (Table 1A; R2LMMc = 0.21). Year [χ2(6,515) = 27.94; P < 0.001] and observer category [χ2(1,513) = 3.95; P = 0.047] were significant (Figure 4). The estimates on arrival dates varied from 6.24 to −4.50 days between years, with the earliest reports in 2017 and the latest in 2011. The mean arrival date determined by citizen scientists was day 97.1 ± 15.1 (7th of April; n = 319), whereas the mean arrival date ascertained by academic scientists was day 103.2 ± 12.9 (13th of April; n = 194). Thus, citizen scientists reported arrivals on average 1 week earlier. SSF did not significantly affect arrival dates [χ2(1,509) = 1.36; P = 0.243].
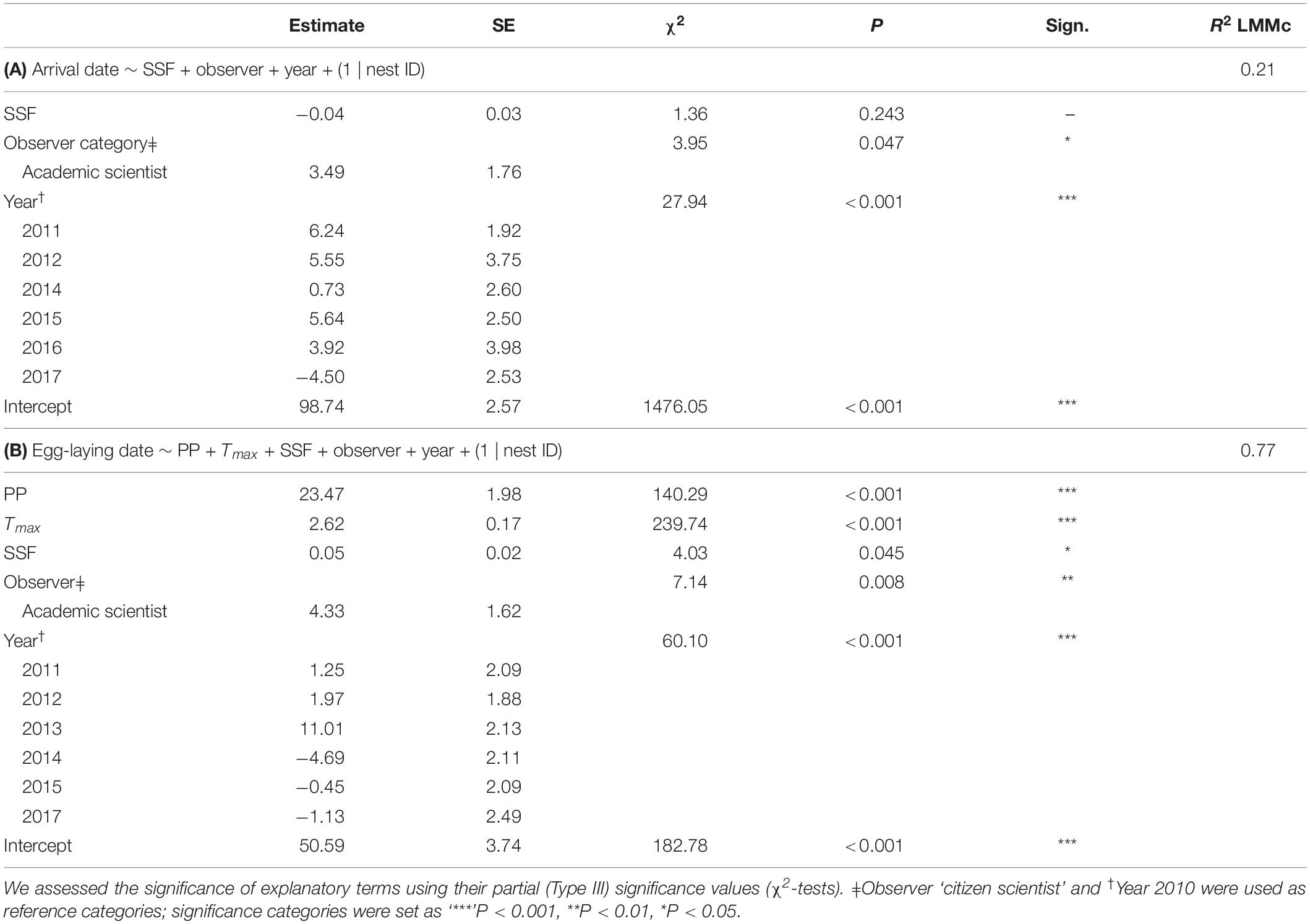
Table 1. LMMs on arrival dates (years 2010–2012 and 2014–2017) and egg-laying dates (years 2010–2015 and 2017), exploring the effects of Soil Seal Factor (SSF), observer category (academic vs. citizen scientist), year and, in the final model on egg-laying dates, precipitation (PP, daily precipitation mean from 21 to 79 days before the egg-laying) and maximum temperature (Tmax, daily maximum temperature mean from 20 to 40 days before the egg-laying date).
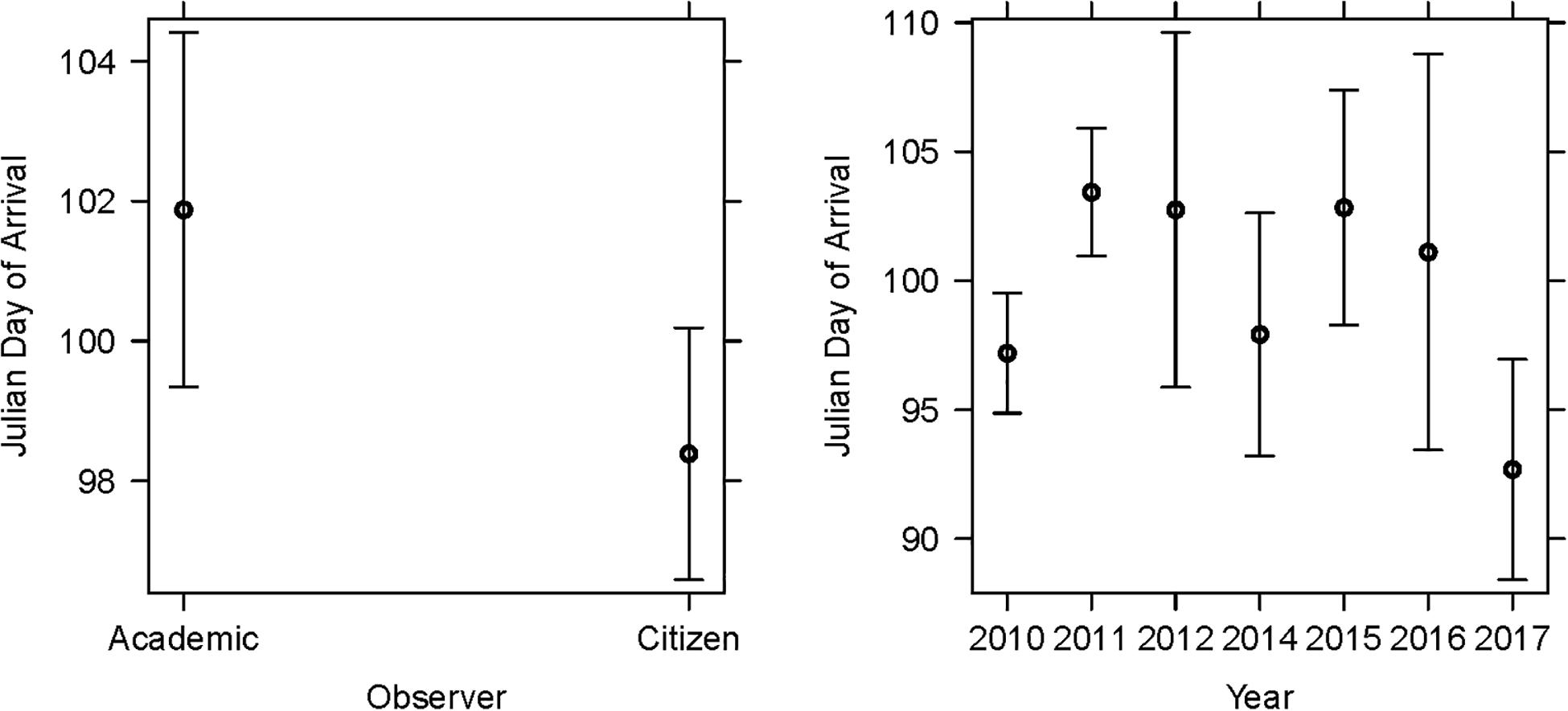
Figure 4. The LMM on arrival dates featured observer category and study year as significant explanatory terms. The overall R2c of the LMM was 0.21. Model details in Table 1A.
Egg-Laying Date and Environmental Conditions
There was no co-linearity between PP and Tmax within the critical time windows [r(n = 209) = 0.12; P = 0.062], hence, both were fitted into the same model. SSF and observer category were additional co-variates and nest ID was fitted as a random term (Table 1B, R2LMMc = 0.77). Significant explanatory terms were PP [χ2(1,211) = 140.29; P < 0.001], Tmax [χ2(1,211) = 239.74; P < 0.001], SSF [χ2(1,206) = 4.03; P = 0.045], observer category [χ2(1,209) = 7.14; P = 0.008], and year [χ2(6,211) = 60.10; P < 0.001]. While year had an ambivalent effect, observations by academic scientists and higher PP, Tmax, and SSF were positively correlated with later egg-laying dates (Figure 5). The mean egg-laying date reported was day 122.4 ± 19.2 (2nd of May; n = 30) for citizen scientists and day 123.8 ± 14.2 (4th of May; n = 179) for academic scientists, but note the unbalanced sample size.
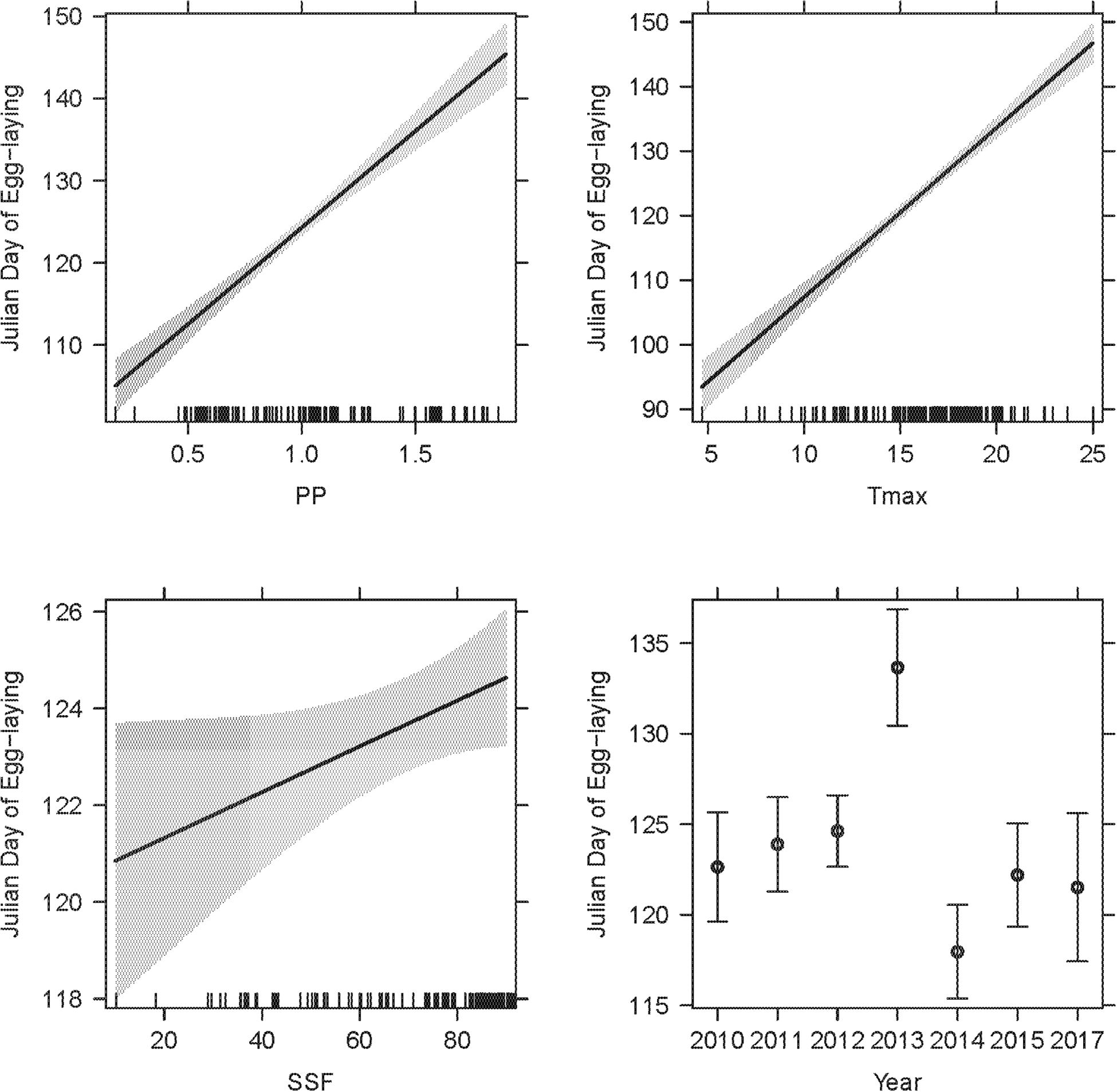
Figure 5. The LMM on egg-laying dates featured two weather variables (PP, Tmax), year, SSF and observer category (not depicted) as significant explanatory terms. The overall R2LMMc was 0.77. Model details in Table 1B.
Dependency of Egg-Laying on Arrival Date
A final LMM was performed using the smaller data set of corresponding observations (n = 68) which provided arrival and egg-laying dates from the same nests and breeding seasons. Weather variables, arrival dates, SSF and year were fitted as fixed effects and nest ID as a random term (R2LMMc = 0.85; Table 2). Observer category could not be included as the respective events were not necessarily reported by the same observer. While the overall significant year effect [χ2(6,68) = 19.06, P = 0.004] had no clear direction (i.e., there was no increasing advance or delay over the course of the years, but egg-laying started earlier in some years and later in others), PP [χ2(1,68) = 71.65, P < 0.001] and Tmax [χ2(1,68) = 49.38, P < 0.001] were both positively correlated with later egg-laying dates (Figure 6). Additionally, later arrival resulted overall in slightly later egg-laying [χ2(1,68) = 3.83, P = 0.050], while there was no variation along the urban gradient [SSF χ2(1,68) = 0.04, P = 0.850]. However, the time gap between arrival and egg-laying date (Δ Days) decreased for later arrival dates [Figure 7; F(1,66) = 33.29, P < 0.001, R2 = 0.33, estimate = −0.59 ± 0.10 SE], which means egg-laying started relatively earlier with corresponding later arrival date. Δ Days decreased by approximately half a day per later day of arrival.
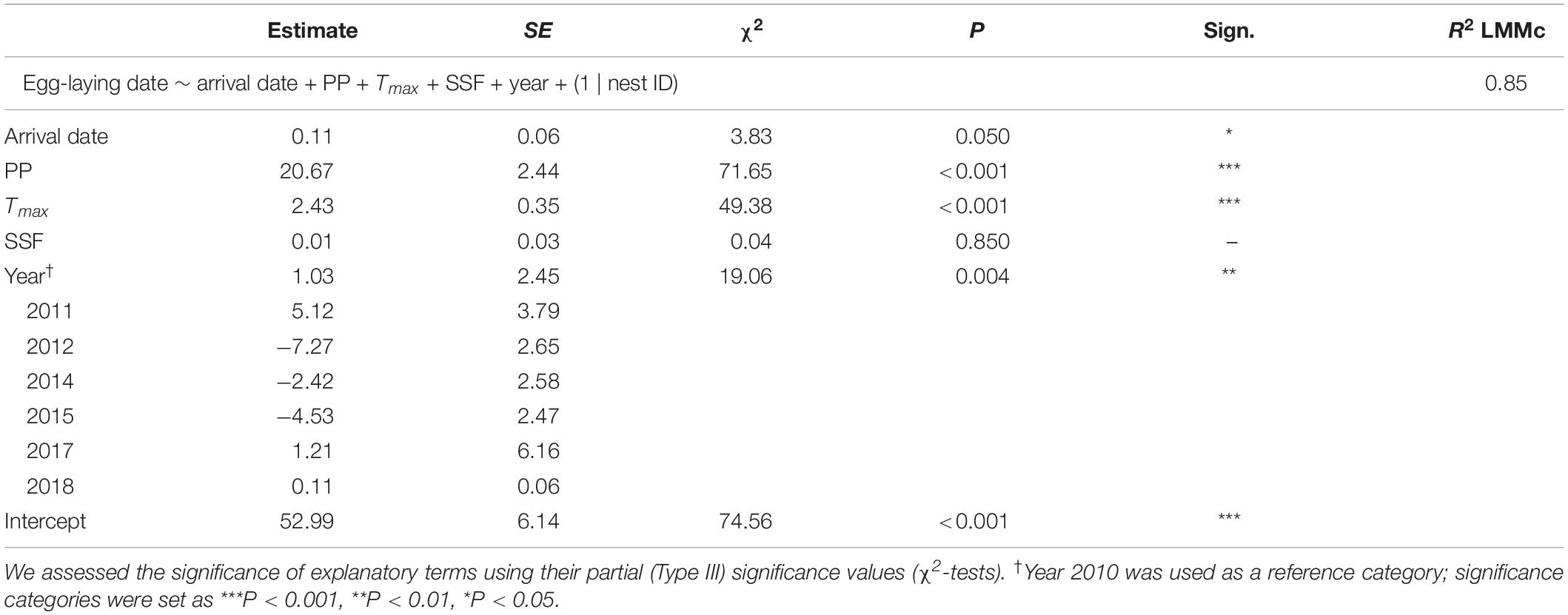
Table 2. LMM on egg-laying dates from corresponding observations (2010–2012, 2014–2015, and 2017–2018) exploring the effects of arrival date, precipitation (PP, mean from 21 to 79 days before egg-laying), maximum temperature (Tmax, mean from 20 to 40 days before egg-laying), Soil Seal Factor (SSF) and year.
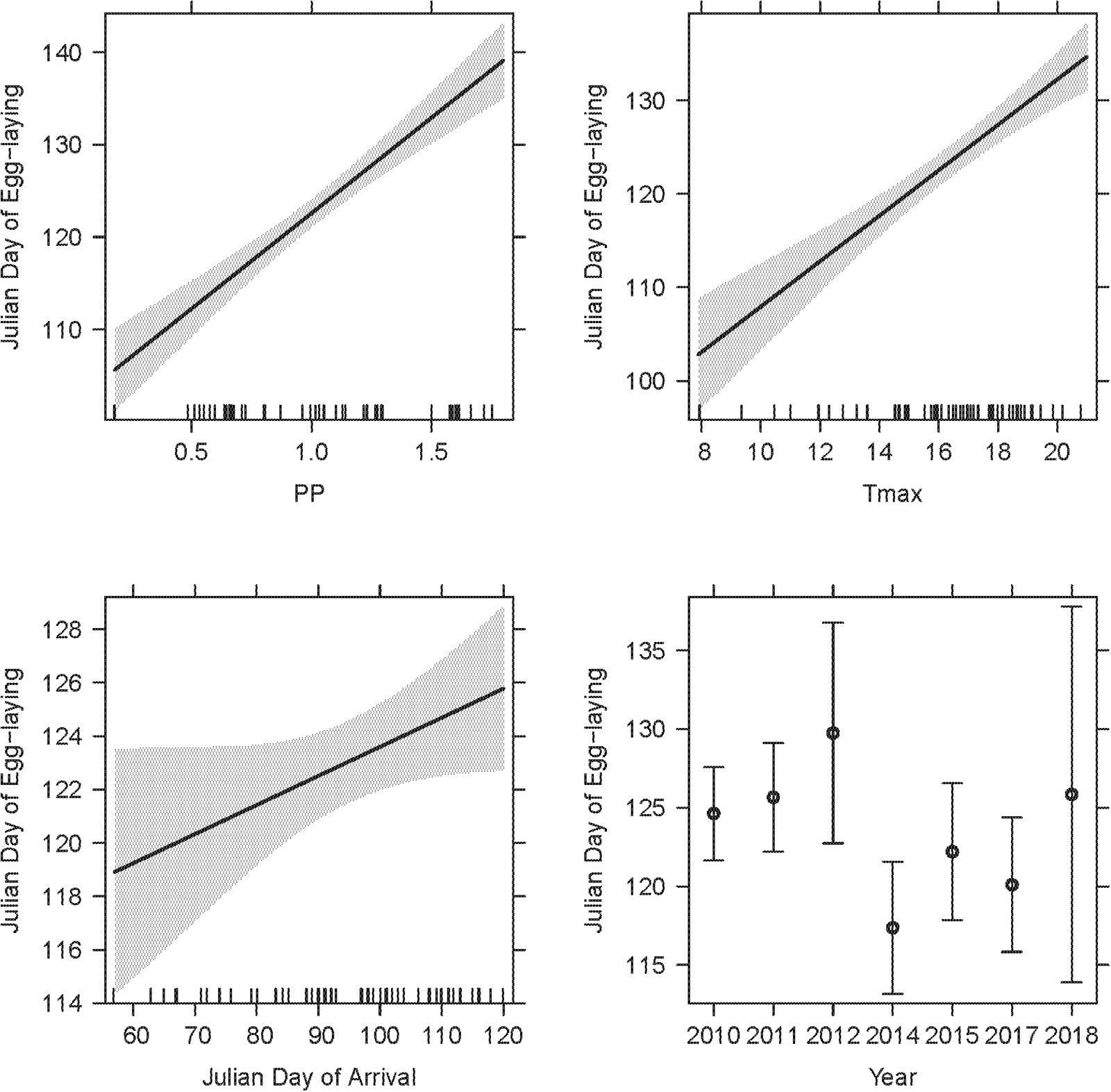
Figure 6. The LMM on egg-laying dates from corresponding observations featured two weather variables (PP, Tmax), year and arrival date as significant explanatory terms. SSF (not depicted) was not significant. The overall R2c of the LMM was 0.85. Model details in Table 2.
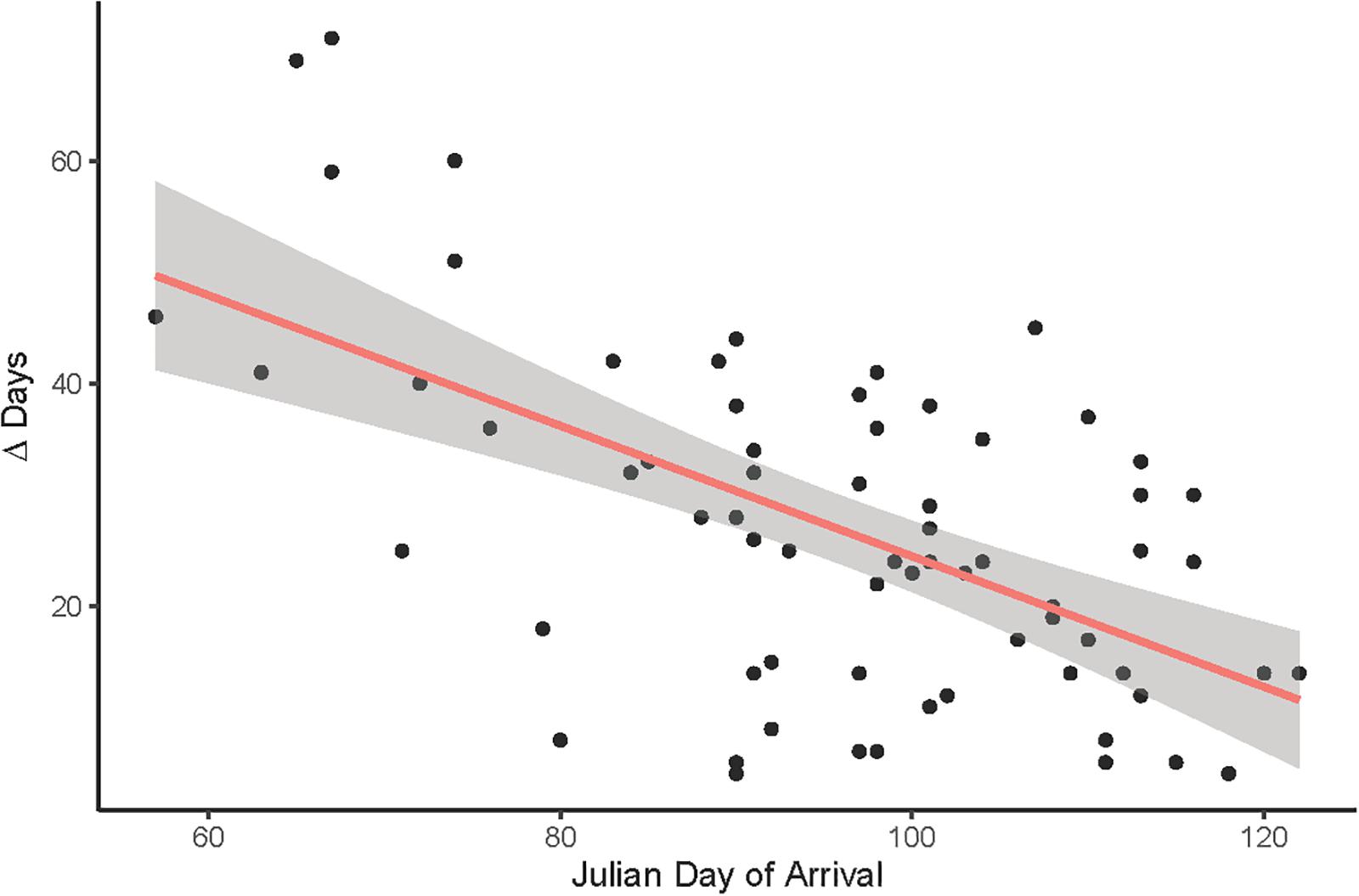
Figure 7. The time gap between arrival and egg-laying date (Δ Days) shortens for later arrival dates when pooling corresponding arrival and egg-laying observations from all years (n = 68). R2 = 0.28, P < 0.001.
Discussion
Our results suggest that the breeding phenology of urban kestrels in Vienna is highly affected by weather variables. Precipitation was the most informative variable for predicting egg-laying dates, within a time window starting before the arrival at the nest site and ending 3 weeks before egg-laying. Additionally, the model on egg-laying dates identified observer category as a significant predictor and indicated earlier egg-laying in more natural areas, while also revealing strong inter-annual variation. Finally, the time gap between arrival and egg-laying was shorter in breeding pairs that arrived later at their nest sites, perhaps to take advantage of potentially favorable conditions at the wintering grounds while avoiding egg-laying delay and the associated declines in breeding productivity with later onset of breeding known from the urban breeding habitat.
Weather Shapes Breeding Phenology
In general, the reproduction of birds is influenced by temperature and precipitation (Crick and Sparks, 1999), but only a more detailed identification of critical time windows allows us to predict potential impacts of climate change (van de Pol and Cockburn, 2011). Our associative approach on the critical time windows (Brommer et al., 2008) revealed that the most informative time windows spanned from roughly 11–3 weeks before egg-laying for precipitation and 5 to 3.5 weeks for temperature. The mechanistic pathways behind the influence of precipitation and temperature on egg-laying include direct effects on energetic demands of females and gonadal growth of both sexes, and indirect effects on food sources (Dunn, 2004) that in turn control prey availability and ultimately hunting success. However, a previous study on the kestrel population of Vienna verified a stronger connection between breeding performance and weather parameters than between breeding performance and diet composition (Kreiderits et al., 2016). Nevertheless, prey availability could not be assessed for the entire long-term study period used in this current investigation.
Our findings emphasize the role of precipitation as cue for clutch initiation, but surprisingly, the critical time windows started way before the peak of arrivals at the nest site (after migration) 4–3 weeks before egg-laying. We consider two potential reasons. Firstly, kestrels are short-distance migrants (Village, 1990), although the detailed wintering grounds and migration routes of the Viennese kestrel population remain unknown. It is therefore possible, but at this stage speculative, that they experience similar macroclimates at their wintering grounds and breeding sites. In Europe, precipitation and temperature are closely related to the North Atlantic Oscillation in terms of long-term trends and in the frequency of extreme weather events (Hurrell, 1995; Scaife et al., 2008). Both local weather and winter North Atlantic Oscillation can explain variation in egg-laying dates of Mediterranean kestrels (Costantini et al., 2010a). However, in contrast to our population the one in Rome only shows facultative winter dispersion (Costantini et al., 2010b), a phenomenon that also seems to have increased in frequency in Vienna (pers. obs.) but comprehensive data on this issue are currently missing. Secondly, previous findings have shown that dry and mild winters have positive effects on the proportion of mammals in the kestrel’s diet (Kreiderits et al., 2016) – which is their main prey (Village, 1990). In consequence, the weather before breeding might have direct and indirect effects on phenology.
Interestingly, the weather conditions closer to egg-laying appeared to be less influential, as the critical time windows ended 3 weeks before egg-laying, indicating a time gap between cue and response. The time window did not overlap with the actual development of the eggs within the body, which supposedly starts 9 days before laying of the first egg (Meijer et al., 1989). Lag times are generally considered disadvantageous, as they enhance the probability of mismatches between environmental optimum and breeding effort, but time scales are dependent on the environmental variability (Padilla and Adolph, 1996). So far, negative consequences of such mismatches on the reproductive performance have been mainly reported for birds depending on a close match in timing of egg laying and the biomass peak of caterpillars used as food for the nestlings [e.g., great tits Parus major (Charmantier et al., 2008); European pied flycatcher Ficedula hypoleuca (Visser et al., 2006, 2012)]. However, as vertebrate prey does not show such a pronounced seasonal biomass peak as found in caterpillars, it may be easier for kestrels to compensate for late territory acquisition, particularly when they are capable of reducing the time gap until egg-laying.
Although both climatic variables featured significantly into our models, we assume precipitation to be more informative than maximum daily temperature. Despite the inter-annual variation of weather variables and egg-laying dates, it is inevitable that daily maximum temperatures will increase with advancing time of the year. Hence, we argue that earlier breeders will naturally face colder temperatures within the given time window rather than higher temperatures postponing egg-laying as implied by our findings. Precipitation on the other hand showed less seasonal trends. Higher amounts of rainfall during the critical time window delayed egg-laying, which supports previous studies on kestrels (Carrillo and González-Dávila, 2009; Costantini et al., 2010a; Kreiderits et al., 2016). This delay might be caused by prey availability, as higher rainfall reduces both prey activity (Brown, 1956) and the hunting activity of kestrels (Rijnsdorp et al., 1981). Higher food availability is generally known to advance the egg-laying date, as supplementary fed kestrels lay their eggs earlier than their conspecifics without supplementary food (Aparicio, 1994; Aparicio and Bonal, 2002). Furthermore, in kestrels the males provide supplementary food to the females long before egg laying during the courtship period (Village, 1990). When this supplementary food may be important for the females to achieve a minimum body condition required for initiating egg production, then negative consequences of rainfall may already effect the egg laying date weeks in advance.
Compensation of Later Arrival by Shortening of Courtship Period
Another significant predictor for egg-laying date was the arrival date itself, but kestrels which arrive later at the nest site do not necessarily delay their egg-laying by the same amount of time. The courtship period, defined as the time from arrival until egg-laying, decreased dramatically with later arrival (half a day decrease per later day of arrival). This might be a strategy to mitigate expected fitness costs of the late arrival for the offspring (Daan et al., 1989), while reducing fitness costs for the parents. Meaning, urban core areas might be attractive as breeding territories due to a high nest site availability but also are characterized by a low food availability (Sumasgutner et al., 2014a, b) known to lead to impaired health (Sumasgutner et al., 2018) and lower fledging success (Sumasgutner et al., 2014a).
Because of this challenge the parents might benefit from extending their time in probably more rural wintering habitat to gain a better body condition, which in turn positively influences breeding productivity (Drent and Daan, 1980). Indeed, experimentally supplemented food (Dijkstra et al., 1982; Korpimäki and Wiehn, 1998) and superior hunting skills of the male (Masman et al., 1986) are known to lead to considerably earlier laying dates in kestrels. However, in several raptor species including kestrels, egg-laying date is also influenced by individual age and breeding experience (Forslund and Pärt, 1995; Daunt et al., 1999; Sumasgutner et al., 2014c, 2019a), intraspecific competition for territories (e.g., Sergio et al., 2007) or social cues provided by conspecifics (Danchin et al., 2004; Sumasgutner et al., 2014c). Ultimately, it remains difficult to assess the effect of shortened courtship periods after later arrival in an overall lower quality breeding habitat without including information on the body condition of breeding adults, individual age and breeding experience, migration distances and quality of the wintering habitats of the observed individuals. Such data are not yet available for the study population.
Observer Category Matters
The underlying monitoring program is designed as a citizen science project, so the data is provided by academic and citizen scientists contributing their observations. We found a significant relationship between arrival and egg-laying dates and observer category. Citizen scientists observed the arrivals of kestrels on average 1 week earlier than academic scientists. This is very logical for methodological reasons. Firstly, most citizen scientists monitor a nest site close to their work place or home, which allow daily nest checks. Secondly, offices and homes often offer a direct view into the nest sites, a clear advantage over working from street level. Thirdly, the systematic monitoring by academic scientists was initiated only once the first reports of citizen scientists accumulated.
In contrast, mean egg-laying dates only differed by less than 1.5 days between citizen scientists and academic scientists. However, only a few citizen scientists reported egg-laying dates, usually those with a direct view into the nest content such as a planter box on the windowsill. The academic scientists on the other hand back-dated most egg-laying dates when assessing nestlings’ development during banding (Kostrzewa and Kostrzewa, 1987, 1993; Village, 1990). Hence, we see a strong methodological constraint to this result as citizen scientists simply have limited options to assess egg-laying dates, but we included the variable in all analyses to control for such possible effects.
We conclude that citizen scientists add valuable data to this project. Citizen science is known to decidedly increase the scale of ecological field surveys by broadening the sample size and geographical extent and is highly valued as complementary approach in synergy with research by academic scientists (Dickinson et al., 2010; Miller-Rushing et al., 2012; Chandler et al., 2017). However, due to the above-mentioned factors, observer category should be considered when analyzing data on arrival or egg-laying dates to control for variation linked to the methodology.
No Effect of Urbanization on Arrival but Indicative for Egg-Laying Dates
Interestingly, with this larger data set, we could not confirm a previous finding of earlier arrival dates in more urbanized areas (effect of the Soil Seal Factor), even though the direction remained the same. The original study was limited to the years 2010–2012, with a marginally non-significant result (Sumasgutner et al., 2014a). With this longer data-set, urbanization had a marginally significant effect on egg-laying dates, in a way that later egg-laying was observed in more urbanized areas. This is remarkable, as urbanization usually correlates positively with earlier egg-laying dates in several passerine bird species (Chamberlain et al., 2009; Møller et al., 2015; de Jong et al., 2018) and also some raptor species [Cooper’s hawks Accipiter cooperi (Boal and Mannan, 1999); Crested goshawks Accipiter trivirgatus (Lin et al., 2015); Peregrine falcons Falco peregrinus (Sumasgutner et al., 2020); and Tawny owls Strix aluco (Solonen, 2014)]. This phenological shift is often attributed to the urban heat-island effect (Oke, 1982; Arnfield, 2003; Streutker, 2003), which is likely to exist in Vienna. Higher ambient temperatures in urban areas are caused by the heat-absorbing properties of urban structures (i.e., sealed surfaces) together with the scattering effects of air pollution, which traps radiated heat within the atmosphere of the city. The average temperature difference between cities and the surrounding countryside is usually around 2.9°C (Imhoff et al., 2010). In our study we accessed detailed weather data for the entire length of the study period from one weather station and were thus not able to obtain finer scale data per territory which would be needed to shed light on possible local differences.
The expected shift in breeding phenology appears less consistently in urban raptors, as two studies even reported a weak (non-significant) evidence for a later onset of breeding at urban sites [in Kettel et al., 2018: Bald eagles Haliaeetus leucocephalus (Millsap et al., 2004); and Burrowing owls Athene cunicularia (Conway et al., 2006)]. Furthermore, a comparative study on Eurasian kestrels in Israel also found pairs breeding on average 2 and 8 days earlier in more rural environments, compared to towns and cities (Charter et al., 2007). Overall, raptors respond less consistently to urbanization (Marzluff, 2001), and all reported differences in an urban raptor review (Kettel et al., 2018) were based on a one by one urban-rural comparison, while reviewed studies using an urban gradient approach did not find any effect of urban land cover on the onset of egg-laying [in Barn owls Tyto alba (Frey et al., 2011), and American kestrels Falco sparverius (Strasser and Heath, 2013); but see Sumasgutner et al., 2020].
Conclusion
We need to acquire knowledge on the wintering grounds of the studied kestrel population to gain a deeper understanding of arrival dates and factors which might ultimately shape breeding phenology. For example, the time of departure and migration speed could vary, depending on year, but also between locations where kestrels might overwinter (Lehikoinen et al., 2004) which could result in carry-over effects which would then, in turn, be visible in high inter-annual variation of arrival times which were also well pronounced in our data. Additional to unknown migration distances, we can also only speculate about local weather conditions or prey availability on the wintering grounds.
However, we gained a much better understanding on variation in egg-laying dates. Most evidence thus far focuses on weather conditions experienced close to the egg-laying date itself or uses monthly means (Costantini et al., 2010a), which might limit the explained variation compared to the sliding window approach applied here (Brommer et al., 2008; Williams et al., 2015). We found that, beside precipitation and temperature, the degree of urbanization was another potentially relevant factor, with kestrels breeding in more natural areas laying their clutches earlier than their conspecifics in urban core areas. However, the effect size of this result was rather small, and so we suggest a future investigation on nesting microclimate (see for instance Catry et al., 2011 on temperature nest type correlations which could also play an important role in our system).
When testing the effect of arrival date on egg-laying dates we also found a shortened courtship period in later arriving breeding pairs, resulting in relatively earlier egg-laying. Later clutches generally face higher fitness costs and are less likely to produce offspring which recruits into the breeding population (Perrins, 1970). The shortening of the courtship period might be a strategy to mitigate the negative effects of later broods while taking advantage of potentially favorable condition at the wintering ground. Ultimately, this would add to the body of evidence that kestrels are able to adapt physiologically and behaviorally to urban environments, despite our concern about cities creating an ecological trap for the species (Sumasgutner et al., 2014a). Considering their potential ability to use environmental cues for the fine-tuning of breeding phenology to the environmental optimum, European kestrels might be able to adapt to the human-induced climate change in the long-run.
Data Availability Statement
All datasets generated for this study are included in the article/Supplementary Material.
Ethics Statement
The animal study was reviewed and approved by Environmental Protection Bureau of Vienna (MA22/1263/2010/3) and the Ministry for Science and Research (BM.WF ± 66.006/0021-II/3b/2013). All data collection was conducted in strict accordance with current Austrian law and followed the Weatherall Report and the guidelines for the treatment of animals in behavioral research and teaching (ASAB, 2018).
Author Contributions
The idea for this study originally came from PS, CS, and KH. The Viennese kestrel project and all citizen science involvement were coordinated by PS and AG. Environmental data was collected by PS and KH. Help by others is accordingly acknowledged. The statistical analyses were performed by KH and CS. The manuscript was prepared by KH and PS with edits provided by CS. All authors contributed to the article and the submitted version was approved by KH, CS, and PS.
Conflict of Interest
The authors declare that the research was conducted in the absence of any commercial or financial relationships that could be construed as a potential conflict of interest.
Dedication
This article is dedicated to Anita Gamauf (∗1962–2018†).
Funding
The Viennese kestrel project was financially supported by the Vienna Science and Technology Fund H-2483/2012 (https://www.wien.gv.at/amtshelfer/kultur/archiv/forschung/hoc hschuljubilaeumsstiftung.html), the Vienna Environmental Protection Bureau MA 22-2220/2010 (https://www.wien.gv.at/umweltschutz/), and the Forschungsförderung der Deutschen Ornithologen-Gesellschaft (http://www.do-g.de/forschungsfoerd erung/). PS was funded by the Doctoral Research Fellowship of the University of Vienna, DOC-fFORTE Grant of the Austrian Academy of Science, and the Marietta Blau Grant of the OEAD. The funders had no role in study design, data collection and analysis, decision to publish, or preparation of the manuscript.
Acknowledgments
We wish to thank all people in Vienna who reported kestrel breeding sites during this citizen science project, the Vienna Fire Fighters as well as the Vienna Chimney Sweepers for facilitating access to several nesting sites and Ferdinand Schmeller from the Environmental Protection Bureau (MA22) for the continuous support. Furthermore, we would like to thank Tomislav Gaspar, Anna Kreiderits, Marius Adrion, Christian Schano, and Laura Wemer for their great help during field work and data collection between 2013 and 2018 and Johannes Wessely for assistance with the sliding window approach.
Supplementary Material
The Supplementary Material for this article can be found online at: https://www.frontiersin.org/articles/10.3389/fevo.2020.00247/full#supplementary-material
References
Aparicio, J. M. (1994). The seasonal decline in clutch size: an experiment with supplementary food in the kestrel, Falco tinnunculus. Oikos 71, 451–458.
Aparicio, J. M., and Bonal, R. (2002). Effects of sood supplementation and habitat selection on timing of Lesser Kestrel breeding. Ecology 83, 873–877. doi: 10.1890/0012-9658(2002)083[0873:eofsah]2.0.co;2
Arnfield, A. J. (2003). Two decades of urban climate research: a review of turbulence, exchanges of energy and water, and the urban heat island. Int. J. Climatol. 23, 1–26. doi: 10.1002/joc.859
ASAB (2018). Guidelines for the treatment of animals in behavioural research and teaching. Anim. Behav. 135, I–X.
Baker, J. R. (1938). “The evolution of breeding seasons,” in Evolution. Essays on Aspects of Evolutionary Biology, ed. G. R. De Beer (Oxford: Oxford University Press), 161–177.
Bates, D., Maechler, M., Bolker, B., and Walker, S. (2015). Fitting linear mixed-effects models using lme4. J. Statist. Softw. 67, 1–48.
Beichle, U. (1980). Siedlungsdichte, Jagdreviere und Jagdweise des Turmfalken (Falco tinnunculus) im Stadtgebiet von Kiel. Corax 8, 3–12.
Boal, C. W., and Mannan, R. W. (1999). Comparative breeding ecology of Cooper’s Hawks in urban and exurban areas of southeastern Arizona. J. Wildlife Manage. 63, 77–84.
Both, C., Van Asch, M., Bijlsma, R. G., Van Den Burg, A. B., and Visser, M. E. (2009). Climate change and unequal phenological changes across four trophic levels: constraints or adaptations? J. Anim. Ecol. 78, 73–83. doi: 10.1111/j.1365-2656.2008.01458.x
Brommer, J. E., Rattiste, K., and Wilson, A. J. (2008). Exploring plasticity in the wild: laying date-temperature reaction norms in the common gull Larus canus. Proc. Biol. Sci. 275, 687–693. doi: 10.1098/rspb.2007.0951
Brown, L. E. (1956). Field experiments on the activity of the small mammals, Apodemus, Clethrionomys and Microtus. Proc. Zool. Soc. Lond. 126, 549–564. doi: 10.1111/j.1096-3642.1956.tb00452.x
Carrillo, J., and González-Dávila, E. (2009). Latitudinal variation in breeding parameters of the common kestrel Falco tinnunculus. Ardeola 56, 215–228.
Catry, I., Franco, A. M. A., and Sutherland, W. J. (2011). Adapting conservation efforts to face climate change: modifying nest-site provisioning for lesser kestrels. Biol. Conserv. 144, 1111–1119. doi: 10.1016/j.biocon.2010.12.030
Chamberlain, D. E., Cannon, A. R., Toms, M. P., Leech, D. I., Hatchwell, B. J., and Gaston, K. J. (2009). Avian productivity in urban landscapes: a review and meta-analysis. Ibis 151, 1–18. doi: 10.1111/j.1474-919x.2008.00899.x
Chandler, M., See, L., Copas, K., Bonde, A. M. Z., López, B. C., Danielsen, F., et al. (2017). Contribution of citizen science towards international biodiversity monitoring. Biol. Conserv. 213, 280–294. doi: 10.1016/j.biocon.2016.09.004
Charmantier, A., Mccleery, R. H., Cole, L. R., Perrins, C., Kruuk, L. E., and Sheldon, B. C. (2008). Adaptive phenotypic plasticity in response to climate change in a wild bird population. Science 320, 800–803. doi: 10.1126/science.1157174
Charter, M., Izhaki, I., Bouskila, A., Leshem, Y., and Penteriani, V. (2007). Breeding success of the Eurasian Kestrel (Falco tinnunculus) nesting on buildings in Israel. J. Raptor Res. 41, 139–143. doi: 10.3356/0892-1016(2007)41[139:bsotek]2.0.co;2
Chastel, O., Weimerskirch, H., and Jouventin, P. (1995). Body condition and seabird reproductive performance: a study of three petrel species. Ecology 76, 2240–2246. doi: 10.2307/1941698
Conway, C. J., Garcia, V., Smith, M. D., Ellis, L. A., and Whitney, J. L. (2006). Comparative demography of burrowing Owls in agricultural and urban landscapes in southeastern Washington. J. Field Ornithol. 77, 280–290. doi: 10.1111/j.1557-9263.2006.00054.x
Costantini, D., Carello, L., and Dell’omo, G. (2010a). Patterns of covariation among weather conditions, winter North Atlantic Oscillation index and reproductive traits in Mediterranean kestrels. J. Zool. 280, 177–184. doi: 10.1111/j.1469-7998.2009.00649.x
Costantini, D., Carello, L., and Dell’omo, G. (2010b). Temporal covariation of egg volume and breeding conditions in the Common Kestrel (Falco tinnunculus) in the Mediterranean region. Ornis Fennica 87, 144–152.
Costantini, D., Dell’omo, G., La Fata, I., and Casagrande, S. (2014). Reproductive performance of Eurasian Kestrel Falco tinnunculus in an agricultural landscape with a mosaic of land uses. Ibis 156, 768–776. doi: 10.1111/ibi.12181
Crick, H. Q., and Sparks, T. H. (1999). Climate change related to egg-laying trends. Nature 399, 423–423. doi: 10.1038/20839
Daan, S., Dijkstra, C., Drent, R., and Meijer, T. (1989). “Food supply and the annual timing of avian reproduction,” in Proceedings of the XIX International Ornithological Congress, (Ottawa, ON: University of Ottawa Press), 392–407.
Daan, S., and Tinbergen, J. M. (1997). “Adaptation of life histories,” in Behavioural Ecology: An Evolutionary Approach, 4th Edn, eds J. R. Krebs and N. B. Davies (Oxford: Oxford University Press), 311–333.
Danchin, E., Boulinier, T., and Massot, M. (1998). Conspecific reproductive success and breeding habitat selection: implications for the study of coloniality. Ecology 79, 2415–2428. doi: 10.1890/0012-9658(1998)079[2415:crsabh]2.0.co;2
Danchin, É, Giraldeau, L.-A., Valone, T. J., and Wagner, R. H. (2004). public information: from nosy neighbors to cultural evolution. Science 305, 487–491. doi: 10.1126/science.1098254
Daunt, F., Wanless, S., Harris, M., and Monaghan, P. (1999). Experimental evidence that age-specific reproductive success is independent of environmental effects. Proc. R. Soc. Lond. Ser. B Biol. Sci. 266, 1489–1493. doi: 10.1098/rspb.1999.0805
de Jong, M., Van Den Eertwegh, L., Beskers, R. E., De Vries, P. P., Spoelstra, K., and Visser, M. E. (2018). Timing of avian breeding in an urbanised world. Ardea 106, 31–38.
Deviche, P., and Davies, S. (2014). “Reproductive phenology of urban birds: environmental cues and mechanisms,” in Avian Urban Ecology: Behavioural and Physiological Adaptations, eds D. Gil and H. Brumm (Oxford: Oxford University Press), 98–115. doi: 10.1093/acprof:osobl/9780199661572.003.0008
Dickinson, J. L., Zuckerberg, B., and Bonter, D. N. (2010). Citizen science as an ecological research tool: challenges and benefits. Annu. Rev. Ecol. Evol. Syst. 41, 149–172. doi: 10.1146/annurev-ecolsys-102209-144636
Dijkstra, C., Vuursteen, L., Daan, S., and Masman, D. (1982). Clutch size and laying date in the kestrel Falco tinnunculus: effect of supplementary food. Ibis 124, 210–213. doi: 10.1111/j.1474-919x.1982.tb03766.x
Doligez, B., Cadet, C., Danchin, E., and Boulinier, T. (2003). When to use public information for breeding habitat selection? The role of environmental predictability and density dependence. Anim. Behav. 66, 973–988. doi: 10.1006/anbe.2002.2270
Drent, R., and Daan, S. (1980). The prudent parent: energetic adjustments in avian breeding. Ardea 68, 225–252. doi: 10.5253/arde.v68.p225
Dunn, P. (2004). Breeding dates and reproductive performance. Adv. Ecol. Res. 35, 69–87. doi: 10.1016/s0065-2504(04)35004-x
Dykstra, C. R., Bloom, P. H., and Mccrary, M. D. (2018). “Red-shouldered hawks: adaptable denizens of the suburbs,” in Urban Raptors: Ecology and Conservation of Birds of Prey in Cities, eds C. W. Boal and C. R. Dykstra (Washington, DC: Island Press), 110–125. doi: 10.5822/978-1-61091-841-1_8
Dykstra, C. R., Hays, J. L., Daniel, F. B., and Simon, M. M. (2001). Home range and habitat use of suburban red-shouldered hawks in southwestern Ohio. Wilson Bull. 113, 308–316. doi: 10.1676/0043-5643(2001)113[0308:hrahuo]2.0.co;2
Edwards, M., and Richardson, A. J. (2004). Impact of climate change on marine pelagic phenology and trophic mismatch. Nature 430, 881–884. doi: 10.1038/nature02808
Ferguson-Lees, J., and Christie, D. A. (2005). Raptors of the World. Princeton, NJ: Princeton Field Guides.
Forslund, P., and Pärt, T. (1995). Age and reproduction in birds - hypotheses and tests. Trends Ecol. Evol. 10, 374–378. doi: 10.1016/s0169-5347(00)89141-7
Fretwell, S. D. (1972). Populations in a Seasonal Environment. Princeton, NJ: Princeton University Press.
Frey, C., Sonnay, C., Dreiss, A., and Roulin, A. (2011). Habitat, breeding performance, diet and individual age in Swiss Barn Owls (Tyto alba). J. Ornithol. 152, 279–290. doi: 10.1007/s10336-010-0579-8
Gamauf, A. (1991). Greifvögel in Österreich. Bestand – Bedrohung – Gesetz. Wien: Umweltbundesamt Wien.
Grüebler, M. U., and Naef-Daenzer, B. (2010). Fitness consequences of timing of breeding in birds: date effects in the course of a reproductive episode. J. Avian Biol. 41, 282–291. doi: 10.1111/j.1600-048x.2009.04865.x
Harriman, V. B., Dawson, R. D., Bortolotti, L. E., and Clark, R. G. (2017). Seasonal patterns in reproductive success of temperate−breeding birds: experimental tests of the date and quality hypotheses. Ecol. Evol. 7, 2122–2132. doi: 10.1002/ece3.2815
Hendry, A. P., Gotanda, K. M., and Svensson, E. I. (2017). Human influences on evolution, and the ecological and societal consequences. Philos. Trans. R. Soc. B Biol. Sci. 372:20160028. doi: 10.1098/rstb.2016.0028
Holte, D., Köppen, U., and Schmitz-Ornés, A. (2016). Partial migration in a Central European raptor species: an analysis of ring re-encounter data of Common Kestrels Falco tinnunculus. Acta Ornithol. 51, 39–54. doi: 10.3161/00016454ao2016.51.1.004
Hurrell, J. W. (1995). Decadal trends in the North Atlantic Oscillation: regional temperatures and precipitation. Science 269, 676–679. doi: 10.1126/science.269.5224.676
Imhoff, M. L., Zhang, P., Wolfe, R. E., and Bounoua, L. (2010). Remote sensing of the urban heat island effect across biomes in the continental USA. Remote Sens. Environ. 114, 504–513. doi: 10.1016/j.rse.2009.10.008
Inouye, D. W. (2008). Effects of climate change on phenology, frost damage, and floral abundance of montane wildflowers. Ecology 89, 353–362. doi: 10.1890/06-2128.1
Jetz, W., Wilcove, D. S., and Dobson, A. P. (2007). Projected impacts of climate and land-use change on the global diversity of birds. PLoS Biol. 5:e157. doi: 10.1371/journal.pbio.0050157
Kettel, E. F., Gentle, L. K., Quinn, J. L., and Yarnell, R. W. (2018). The breeding performance of raptors in urban landscapes: a review and meta-analysis. J. Ornithol. 159, 1–18. doi: 10.1007/s10336-017-1497-9
Kokko, H. (1999). Competition for early arrival in migratory birds. J. Anim. Ecol. 68, 940–950. doi: 10.1046/j.1365-2656.1999.00343.x
Korpimäki, E. (1986). Diet variation, hunting habitat and reproductive output of the kestrel Falco tinnunculus in the light of the optimal diet theory. Ornis Fennica 63, 84–90.
Korpimäki, E., and Wiehn, J. (1998). Clutch size of kestrels: Seasonal decline and experimental evidence for food limitation under fluctuating food conditions. Oikos 83, 259–272.
Kostrzewa, R., and Kostrzewa, A. (1987). Zur Jugendentwicklung des Turmfalken (Falco tinnunculus) ein Altersbestimmungsschlüssel. Ökologie der Vögel 9, 119–125.
Kostrzewa, R., and Kostrzewa, A. (1993). Der Turmfalke. Überlebensstrategien eines Greifvogels. Wiesbaden: Aula Verlag.
Kreiderits, A., Gamauf, A., Krenn, H. W., and Sumasgutner, P. (2016). Investigating the influence of local weather conditions and alternative prey composition on the breeding performance of urban Eurasian kestrels (Falco tinnunculus). Bird Study 63, 369–379. doi: 10.1080/00063657.2016.1213791
Kübler, S., Kupko, S., and Zeller, U. (2005). The Kestrel (Falco tinnunculus) in Berlin: investigation of breeding biology and feeding ecology. J. Ornithol. 146, 271–278. doi: 10.1007/s10336-005-0089-2
Kupko, S., Schlottke, L., and Rinder, J. (2000). Der Turmfalke (Falco tinnunculus L.) im berliner stadtgebiet - eine auswertung unter besonderer berücksichtigung der monitoringfläche Nr. 376 (Berlin-West). Populationsökologie Greifvogel- und Eulenarten 4, 359–372.
Lehikoinen, E. S. A., Sparks, T. H., and Zalakevicius, M. (2004). Arrival and departure dates. Adv. Ecol. Res. 35, 1–31. doi: 10.1016/s0065-2504(04)35001-4
Lin, W., Lin, A., Lin, J., Wang, Y., and Tseng, H. (2015). Breeding performance of Crested Goshawk Accipiter trivirgatus in urban and rural environments of Taiwan. Bird Study 62, 177–184. doi: 10.1080/00063657.2015.1005570
Malher, F., Lesaffre, G., Zucca, M., and Coatmeur, J. (2010). Oiseaux nicheurs de Paris. Un atlas urbain. Paris: Corif. Delachaux et Niestlé.
Marzluff, J. M. (2001). “Worldwide urbanization and its effects on birds,” in Avian Ecology and Conservation in an Urbanizing World, eds J. M. Marzluff, R. Bowman, and R. Donnelly (Boston, MA: Kluwer Academic Publishers), 19–48.
Masman, D., Gordijn, M., Daan, S., and Dijkstra, C. (1986). Ecological energetics of the Kestrel: field estimates of energy intake throughout the year. Ardea 74, 24–39.
Meijer, T., Deerenberg, C., Daan, S., and Dijkstra, C. (1992). Egg-laying and photorefractoriness in the European Kestrel Falco tinnunculus. Ornis Scandinavica 23, 405–410.
Meijer, T., Masman, D., and Daan, S. (1989). Energetics of reproduction in female kestrels. Auk 106, 549–559.
Mikula, P., Hromada, M., and Tryjanowski, P. (2013). Bats and Swifts as food of the European Kestrel (Falco tinnunculus) in a small town in Slovakia. Ornis Fennica 90, 178–185.
Miller-Rushing, A., Primack, R., and Bonney, R. (2012). The history of public participation in ecological research. Front. Ecol. Environ. 10:285–290. doi: 10.1890/110278
Miller-Rushing, A. J., Høye, T. T., Inouye, D. W., and Post, E. (2010). The effects of phenological mismatches on demography. Philos. Trans. R. Soc. B Biol. Sci. 365, 3177–3186. doi: 10.1098/rstb.2010.0148
Millsap, B., Breen, T., McConnell, E., Steffer, T., Phillips, L., Douglass, N., et al. (2004). Comparative fecundity and survival of Bald Eagle fledged from suburban and rural natal areas in Florida. J. Wildlife Manage. 68, 1018–1031. doi: 10.2193/0022-541x(2004)068[1018:cfasob]2.0.co;2
Møller, A. P., Díaz, M., Grim, T., Dvorská, A., Flensted-Jensen, E., Ibáñez-Álamo, J. D., et al. (2015). Effects of urbanization on bird phenology: a continental study of paired urban and rural populations. Clim. Res. 66, 185–199. doi: 10.3354/cr01344
Møller, A. P., Rubolini, D., and Lehikoinen, E. (2008). Populations of migratory bird species that did not show a phenological response to climate change are declining. Proc. Natl. Acad. Sci. 105, 16195–16200. doi: 10.1073/pnas.0803825105
Murton, R. K., and Westwood, N. (1977). Avian Breeding Cycles. New York, NY: Oxford University Press.
Nakagawa, S., Johnson, P. C., and Schielzeth, H. (2017). The coefficient of determination R 2 and intra-class correlation coefficient from generalized linear mixed-effects models revisited and expanded. J. R. Soc. Interface 14:20170213. doi: 10.1098/rsif.2017.0213
Newton, I. (1980). The role of food in limiting bird numbers. Ardea 68, 11–30. doi: 10.5253/arde.v68.p11
Oke, T. R. (1982). The energetic basis of the urban heat island. Q. J. R. Meteorol. Soc. 108, 1–24. doi: 10.1002/qj.49710845502
Padilla, D. K., and Adolph, S. C. (1996). Plastic inducible morphologies are not always adaptive: the importance of time delays in a stochastic environment. Evol. Ecol. 10, 105–117. doi: 10.1007/bf01239351
Parmesan, C. (2006). Ecological and evolutionary responses to recent climate change. Annu. Rev. Ecol. Evol. Syst. 37, 637–669. doi: 10.1146/annurev.ecolsys.37.091305.110100
Parmesan, C., and Yohe, G. (2003). A globally coherent fingerprint of climate change impacts across natural systems. Nature 421, 37–42. doi: 10.1038/nature01286
Perrins, C. M. (1970). The timing of birds’ breeding seasons. Ibis 112, 242–255. doi: 10.1111/j.1474-919x.1970.tb00096.x
Pimm, S. L., and Raven, P. (2000). Extinction by numbers. Nature 403, 843–845. doi: 10.1038/35002708
R Development Core Team (2020). A Language and Environment for Statistical Computing. R Version 4.0.0. Vienna: R Foundation for Statistical Computing.
Renner, S. S., and Zohner, C. M. (2018). Climate change and phenological mismatch in trophic interactions among plants, insects, and vertebrates. Annu. Rev. Ecol. Evol. Syst. 49, 165–182. doi: 10.1146/annurev-ecolsys-110617-062535
Riegert, J., Dufek, A., Fainová, D., Mikeš, V., and Fuchs, R. (2007a). Increased hunting effort buffers against vole scarcity in an urban Kestrel Falco tinnunculus population. Bird Study 54, 353–361. doi: 10.1080/00063650709461495
Riegert, J., Fainová, D., Mikeš, V., and Fuchs, R. (2007b). How urban Kestrels Falco tinnunculus divide their hunting grounds: Partitioning or cohabitation? Acta Ornithol. 42, 69–76. doi: 10.3161/068.042.0101
Riegert, J., and Fuchs, R. (2011). Fidelity to roost sites and diet composition of wintering male urban Common Kestrels Falco tinnunculus. Acta Ornithol. 46, 183–189. doi: 10.3161/000164511x625955
Rijnsdorp, A., Daan, S., and Dijkstra, C. (1981). Hunting in the kestrel, Falco tinnunculus, and the adaptive significance of daily habits. Oecologia 50, 391–406. doi: 10.1007/bf00344982
Romanowski, J. (1996). On the diet of urban Kestrels (Falco tinnunculus) in Warsaw. Buteo 8, 123–130.
Root, T. L., Price, J. T., Hall, K. R., Schneider, S. H., Rosenzweig, C., and Pounds, J. A. (2003). Fingerprints of global warming on wild animals and plants. Nature 421, 57–60. doi: 10.1038/nature01333
Rutz, C. (2006). Home range size, habitat use, activity patterns and hunting behaviour of urban-breeding Northern Goshawks Accipiter gentilis. Ardea 94, 185–202.
Sala, O. E., Stuart Chapin, F. III, Armesto, J. J., Berlow, E., Bloomfield, J., Dirzo, R., et al. (2000). Global biodiversity scenarios for the Year 2100. Science 287, 1770–1774. doi: 10.1126/science.287.5459.1770
Scaife, A. A., Folland, C. K., Alexander, L. V., Moberg, A., and Knight, J. R. (2008). European climate extremes and the North Atlantic Oscillation. J. Clim. 21, 72–83. doi: 10.1175/2007jcli1631.1
Sergio, F., Blas, J., Forero, M. G., Donzar, J. A., and Hiraldo, F. (2007). Sequential settlement and site dependence in a migratory raptor. Behav. Ecol. 18, 811–821. doi: 10.1093/beheco/arm052
Sharp, P. J. (1996). Strategies in avian breeding cycles. Anim. Reprod. Sci. 42, 505–513. doi: 10.1016/0378-4320(96)01556-4
Sih, A. (2013). Understanding variation in behavioural responses to human-induced rapid environmental change: a conceptual overview. Anim. Behav. 85, 1077–1088. doi: 10.1016/j.anbehav.2013.02.017
Solonen, T. (2014). Timing of breeding in rural and urban Tawny Owls Strix aluco in southern Finland: effects of vole abundance and winter weather. J. Ornithol. 155, 27–36. doi: 10.1007/s10336-013-0983-y
Stamps, J. (1994). Territorial behavior: testing the assumptions. Adv. Study Behav. 23, 173–232. doi: 10.1016/s0065-3454(08)60354-x
Stenseth, N. C., and Mysterud, A. (2002). Climate, changing phenology, and other life history traits: nonlinearity and match–mismatch to the environment. Proc. Natl. Acad. Sci. U.S.A. 99, 13379–13381. doi: 10.1073/pnas.212519399
Strasser, E. H., and Heath, J. A. (2013). Reproductive failure of a humantolerant species, the American Kestrel, is associated with stress and human disturbance. J. Appl. Ecol. 50, 912–919. doi: 10.1111/1365-2664.12103
Streutker, D. R. (2003). Satellite-measured growth of the urban heat island of Houston, Texas. Remote Sens. Environ. 85, 282–289. doi: 10.1016/s0034-4257(03)00007-5
Sumasgutner, P., Adrion, M., and Gamauf, A. (2018). Carotenoid coloration and health status of urban Eurasian kestrels (Falco tinnunculus). PLoS One 13:e0191956. doi: 10.1371/journal.pone.0191956
Sumasgutner, P., Jenkins, A., Amar, A., and Altwegg, R. (2020). Nest boxes buffer the effects of climate on breeding performance in an African urban raptor. PLoS One 15:e0234503. doi: 10.1371/journal.pone.0234503
Sumasgutner, P., Koeslag, A., and Amar, A. (2019a). Senescence in the city: exploring ageing patterns of a long-lived raptor across an urban gradient. J. Avian Biol. 50:e02247.
Sumasgutner, P., Krenn, H. W., Düesberg, J., Gaspar, T., and Gamauf, A. (2013). Diet specialisation and breeding success along an urban gradient: the Kestrel (Falco tinnunculus) in Vienna, Austria. Beiträge zur Jagd- und Wildforschung 38, 385–397.
Sumasgutner, P., Nemeth, E., Tebb, G., Krenn, H. W., and Gamauf, A. (2014a). Hard times in the city –attractive nest sites but insufficient food supply lead to low reproduction rates in a bird of prey. Front. Zool. 11:48. doi: 10.1186/1742-9994-11-48
Sumasgutner, P., Schulze, C. H., Krenn, H. W., and Gamauf, A. (2014b). Conservation related conflicts in the nest-site selection of the Eurasian Kestrel (Falco tinnunculus) and the distribution of its avian prey. Landsc. Urban Plan. 127, 94–103. doi: 10.1016/j.landurbplan.2014.03.009
Sumasgutner, P., Tate, G. J., Koeslag, A., and Amar, A. (2016). Family morph matters: factors determining survival and recruitment in a long-lived polymorphic raptor. J. Anim. Ecol. 85, 1043–1055. doi: 10.1111/1365-2656.12518
Sumasgutner, P., Terraube, J., Coulon, A., Villers, A., Chakarov, N., Kruckenhauser, L., et al. (2019b). Landscape homogenization due to agricultural intensification disrupts the relationship between reproductive success and main prey abundance in an avian predator. Front. Zool. 16:31. doi: 10.1186/s12983-019-0331-z
Sumasgutner, P., Vasko, V., Varjonen, R., and Korpimäki, E. (2014c). Public information revealed by pellets in nest sites is more important than ecto-parasite avoidance in the settlement decisions of Eurasian kestrels. Behav. Ecol. Sociobiol. 68, 2023–2034. doi: 10.1007/s00265-014-1808-6
Terraube, J., Vasko, V., and Korpimäki, E. (2015). Mechanisms and reproductive consequences of breeding dispersal in a specialist predator under temporally varying food conditions. Oikos 124, 762–771. doi: 10.1111/oik.01974
Tilman, D., and Lehman, C. (2001). Human-caused environmental change: impacts on plant diversity and evolution. Proc. Natl. Acad. Sci. 98, 5433–5440. doi: 10.1073/pnas.091093198
Tremblay, A., and Ransijn, J. (2015). LMERConvenienceFunctions: Model Selection and Post-Hoc Analysis for (G) LMER Models. R Package Version 2.
United-Nations (2019). Department of Economic and Social Affairs, Population Division. World Urbanization Prospects: The 2018 Revision (ST/ESA/SER.A/420). New York, NY: United Nations Department of Economic and Social Affairs.
Valkama, J., Korpimäki, E., and Tolonen, P. (1995). Habitat utilization, diet and reproductive success in the Kestrel in a temporally and spatially heterogeneous environment. Ornis Fennica 72, 49–61.
van de Pol, M., and Cockburn, A. (2011). Identifying the critical climatic time window that affects trait expression. Am. Nat. 177, 698–707. doi: 10.1086/659101
Verboven, N., and Visser, M. E. (1998). Seasonal variation in local recruitment of great tits: the importance of being early. Oikos 81, 511–524.
Verhulst, S., and Nilsson, J. -Å (2008). The timing of birds’ breeding seasons: a review of experiments that manipulated timing of breeding. Philos. Trans. R. Soc. B Biol. Sci. 363, 399–410. doi: 10.1098/rstb.2007.2146
Visser, M. E., and Both, C. (2005). Shifts in phenology due to global climate change: the need for a yardstick. Proc. R. Soc. B Biol. Sci. 272, 2561–2569. doi: 10.1098/rspb.2005.3356
Visser, M. E., Caro, S. P., Van Oers, K., Schaper, S. V., and Helm, B. (2010). Phenology, seasonal timing and circannual rhythms: towards a unified framework. Philos. Trans. R. Soc. B Biol. Sci. 365, 3113–3127. doi: 10.1098/rstb.2010.0111
Visser, M. E., Holleman, L. J., and Gienapp, P. (2006). Shifts in caterpillar biomass phenology due to climate change and its impact on the breeding biology of an insectivorous bird. Oecologia 147, 164–172. doi: 10.1007/s00442-005-0299-6
Visser, M. E., Te Marvelde, L., and Lof, M. E. (2012). Adaptive phenological mismatches of birds and their food in a warming world. J. Ornithol. 153, 75–84. doi: 10.1007/s10336-011-0770-6
Vitousek, P. M. (1994). Beyond global warming: ecology and global change. Ecology 75, 1861–1876. doi: 10.2307/1941591
Walther, G.-R., Post, E., Convey, P., Menzel, A., Parmesan, C., Beebee, T. J. C., et al. (2002). Ecological responses to recent climate change. Nature 416, 389–395.
Wichmann, G., Dvorak, M., Teufelbauer, N., and Berg, H.-M. (2009). Die Vogelwelt Wiens – Atlas der Brutvögel. Wien: Verlag Naturhistorisches Museum Wien.
Williams, T. D., Bourgeon, S., Cornell, A., Ferguson, L., Fowler, M., Fronstin, R. B., et al. (2015). Mid-winter temperatures, not spring temperatures, predict breeding phenology in the European starling Sturnus vulgaris. R. Soc. Open Sci. 2:140301. doi: 10.1098/rsos.140301
Wingfield, J. (1983). “Environmental and endocrine control of avian reproduction: An ecological approach,” in Avian endocrinology: Environment and Ecological Perspectives, eds S. Mikami, K. Hiomma, and M. Wada (Tokyo: Japan Scientific Societies Press), 265–288.
Keywords: urban ecology, timing of breeding, citizen science, urban gradient, urban raptor, bird migration, direct assessment hypothesis, sequential settlement
Citation: Huchler K, Schulze CH, Gamauf A and Sumasgutner P (2020) Shifting Breeding Phenology in Eurasian Kestrels Falco tinnunculus: Effects of Weather and Urbanization. Front. Ecol. Evol. 8:247. doi: 10.3389/fevo.2020.00247
Received: 25 March 2020; Accepted: 07 July 2020;
Published: 24 July 2020.
Edited by:
Mark C. Mainwaring, University of Montana, United StatesReviewed by:
Daniela Campobello, University of Palermo, ItalyPierre J. Deviche, Arizona State University, United States
Copyright © 2020 Huchler, Schulze, Gamauf and Sumasgutner. This is an open-access article distributed under the terms of the Creative Commons Attribution License (CC BY). The use, distribution or reproduction in other forums is permitted, provided the original author(s) and the copyright owner(s) are credited and that the original publication in this journal is cited, in accordance with accepted academic practice. No use, distribution or reproduction is permitted which does not comply with these terms.
*Correspondence: Petra Sumasgutner, cGV0cmEuc3VtYXNndXRuZXJAdW5pdmllLmFjLmF0
†ORCID: Katharina Huchler, orcid.org/0000-0001-9013-2928; Christian H. Schulze, orcid.org/0000-0002-2097-422X; Petra Sumasgutner, orcid.org/0000-0001-7042-3461