- 1Laboratório de Mamíferos, Departamento de Ciências Biológicas, Escola Superior de Agricultura Luiz de Queiroz, Universidade de São Paulo, Piracicaba, Brazil
- 2Center for Conservation Genomics, Smithsonian Conservation Biology Institute, National Zoological Park, Washington, DC, United States
- 3Coordenação de Zoologia, Museu Paraense Emílio Goeldi, Belém, Brazil
- 4Data Science Lab, Office of the Chief Information Officer, Smithsonian Institution, Washington, DC, United States
- 5Division of Mammals, National Museum of Natural History, Smithsonian Institution, Washington, DC, United States
- 6Department of Biology and Department of Environmental Science and Policy, George Mason University, Fairfax, VA, United States
Tree squirrels (Sciurinae, Sciurini) represent a diverse radiation that successfully colonized Europe, Asia and the Americas during the Miocene-Pliocene, but information on their evolutionary history remains unclear. In the Neotropics, they have been shown to exhibit the highest rate of diversification amongst all arboreal squirrels, with strikingly high species accumulation rates in the past 3 Mya. In this study, we investigated the tempo and mode of diversification of tree squirrels using a mitogenome dataset that includes 43 Sciurini species. Our results corroborate the date of origin of the tribe Sciurini around 14 Mya (13.4–15.5) but suggest that their ancestral area was most likely in North America. This is in contrast to previous findings that suggested that the ancestors of this tribe occupied Eurasia. We estimated that cladogenetic events leading to the Eurasian lineages occurred twice at 10.5 and 9.7 Mya. Current North American genera originated in a temporal window from 6.2–2.3 Mya, and the origin of the Neotropical radiation was estimated to have occurred around 6 Mya in northwestern South America, in the Pacific dominion. Remarkably, our results indicate that tree squirrels entered South America at an earlier date than previously estimated. This could have happened either through a land corridor connecting the Caribbean islands or through the Panamanian land bridge. Most cladogenetic events in Eurasia and North America appear to have occurred either late in the Miocene or in the Pleistocene, while the majority of Neotropical cladogenetic events occurred along the Pliocene—right after the South American invasion. We found a fairly constant speciation rate for tree squirrels (averaging 0.29), which contrasts with the peak of lineage accumulation observed in the Pliocene. The absence of fluctuations in the diversification rate may be the result of several extinction events that were responsible for equalizing the number of lineages maintained over time. Finally, we conclude that the South American invasion was not as recent as previously inferred, but the diversification there was indeed very fast.
Introduction
Tree squirrels (Sciurinae, Sciurini) represent a diverse radiation that successfully colonized forested landscapes of Europe, Asia and the Americas during the Miocene and Pliocene (Mercer and Roth, 2003; Pečnerová et al., 2015). The timing of the origin of the tribe is imprecise and has been estimated to have occurred from 19 to 13 million years ago (Mya; Mercer and Roth, 2003; Fabre et al., 2012; Pečnerová et al., 2015; Zelditch et al., 2015). In addition, the uncertainties regarding the location of their ancestral geographic range have also contributed to the blurred inferences of the evolutionary history of tree squirrels. Pečnerová and Martínková (2012) suggested that Sciurini originated in the northern hemisphere, not distinguishing between Eurasia and North America. A similar result was found by Rocha et al. (2016), who proposed a Holarctic distribution for the ancestor of tree squirrels. Subsequently, Pečnerová et al. (2015) estimated a more restricted ancestral range of Sciurini, confined to the Palearctic region.
Discrepancies on inferences about the spatiotemporal diversification of Sciurini are likely a result of the limited datasets, in terms of geographic and taxonomic coverage, employed by previous analyses. None of the aforementioned studies included more than a third of the South American tree squirrel species and also missed some Central and North American taxa (sensu Thorington et al., 2012; Vivo and Carmignotto, 2015); therefore, the lack of so many taxa and presumed lineages might mislead phylogenetic and, consequently, biogeographic inferences. A recent comprehensive phylogenomic study conducted by Abreu-Jr et al. (2020), that included samples from 40 of the 43 recognized species of Sciurini (sensu Thorington et al., 2012; Vivo and Carmignotto, 2015; Hope et al., 2016), revealed an underestimated diversity on the genus and species group levels within the tribe and clarified several important points with regards to the phylogenetic relationships and systematics of tree squirrels. This study did not find support for the recognition of two species previously considered as valid—Sciurus richmondi sensu Thorington et al. (2012) and Microsciurus venustus sensu Vivo and Carmignotto (2015)— and found evidence for the recognition of six additional lineages that might represent species to be named or revalidated. This new taxonomic proposal suggested a diversity of 46 species within Sciurini which were tentatively organized in 14 genera coined by previous authors (see Abreu-Jr et al., 2020). The mitogenome dataset produced in that study is highly suitable to investigate the tempo and mode of evolution of tree squirrels, especially across the Neotropical region, where the taxonomic and geographic sampling were strengthened.
In the Neotropics, tree squirrels have been shown to exhibit the highest rate of diversification amongst all arboreal squirrels (a non-phylogenetic ecological category that includes members of four subfamilies; Roth and Mercer, 2008), with strikingly high species accumulation rates in the past three million years, presumably after the final closure of the Panamanian land bridge and the establishment of the Great American Biotic Interchange (GABI). The GABI is one of the most significant events of transcontinental fauna dispersal that occurred during the temporal window from the late Pliocene to the late Pleistocene, wherein several mammal lineages migrated from North America to South America and the other way around (Simpson, 1983; Stehli and Webb, 1985; Woodburne, 2010). Among rodents, tree squirrels and sigmodontine rodents arguably shared a very successful history of diversification and colonization of South America accompanying the GABI (Patterson and Pascual, 1972; Simpson, 1983).
However, alternative scenarios proposed either a diversification at the generic level in tropical portions of North and Central America, followed by an invasion of South America, after the Panamanian land bridge arose; or an early entrance in South America, sometime in the Miocene, prior to the establishment of the Panamanian land bridge and the GABI (see detailed explanation in D’Elía, 2000). For sigmodontine rodents, current hypotheses suggest an early arrival in South America (Smith and Patton, 1999; Steppan et al., 2004; Parada et al., 2013), in the Miocene, supporting the scenario proposed by Hershkovitz (1966, 1969) and Reig (1984). For squirrels, current hypotheses sustain the entrance of this group in South America during the GABI, after the Panamanian land bridge arose (Mercer and Roth, 2003), but results so far are still inconclusive, as most of the South American squirrel diversity remained unstudied.
These rodents are an ideal group of mammals that can help to address a wide range of biogeographic questions, such as the timing of the dispersal events across the Americas and colonization within South America, and the potential relevance of specific areas as sources of diversity within the Neotropics. The South American continent has a history of major geological (such as the Andes uplift and the Amazon basin formation) and climatic (such as the Pleistocene climate fluctuations) changes that occurred in the past 10 million years. These events gave rise to a great variety of environments and new habitats that leveraged the rapid diversification of several lineages of the tree of life (Hoorn et al., 2010).
In this study, we test current hypotheses on the tempo and mode of diversification of tree squirrels (Pečnerová and Martínková, 2012; Pečnerová et al., 2015) employing a comprehensive dataset (mitogenome data) and a diverse taxonomic coverage (including 43 Sciurini putative species; Abreu-Jr et al., 2020). We provided a time-scaled phylogeny to infer the timing of the origin and diversification of the main lineages, and performed biogeographic analyses to estimate ancestral ranges and evaluate the drivers of diversification at a global scale. In addition to the broad-scale analysis that includes all Sciurini species—and since the Neotropics is home of the most diverse assemblage of species—we also performed a finer-scale analysis focused exclusively on the diversification processes of Neotropical taxa, to unravel their colonization history across Central and South Americas. Finally, we tested the hypothesis that tree squirrels represent a rapid-diversified radiation (Roth and Mercer, 2008) by estimating speciation rates and investigating diversification rate fluctuations through time.
Materials and Methods
Dataset Taxonomic Composition
Our dataset is composed of 14 genera and 43 species of Sciurini delimited in a recent contribution (Abreu-Jr et al., 2020). Taxonomic identifications at the genus and species levels follow Abreu-Jr et al. (2020). At the species level, those authors kept the museum identifications for several specimens, especially those housed at the American Museum of Natural History (AMNH) and at the Smithsonian National Museum of Natural History (USNM), which had been made by some of the main authorities on tree squirrel taxonomy (e.g., R. W. Thorington and M. de Vivo). For material not previously identified, they performed identifications by examining the morphology of the vouchers, consulting original descriptions and other relevant literature. In cases of unviability to examine vouchers, original museum identifications were accepted if (i) those identifications correspond to the known geographic distribution of the taxon in question, and (ii) phylogenetic analyses of their DNA sequences were consistent with the museum identification. The currently valid species of tree squirrels not included in this study are: Tamiasciurus fremonti sensu Hope et al. (2016), Microsciurus santanderensis and M. simonsi sensu Vivo and Carmignotto (2015). We used as outgroup two representatives of the tribe Pteromyini (the sister-tribe of Sciurini): Glaucomys volans (USNM 569823) and Hylopetes phayrei (USNM 584420). A complete list of specimens analyzed in this study accompanied by geographic information and GenBank accession numbers for complete mitogenomes is provided as (Supplementary Table S1).
DNA Matrix, Alignment and Saturation Test
We selected the 13 mitochondrial protein-coding genes (CDS) from one sample of each of the 43 putative species of Sciurini to perform the analyses. The CDS matrix was aligned using MUSCLE (Edgar, 2004), with up to eight interactions. We investigated the presence of saturation in our dataset by plotting transitions and transversions against nucleotide divergence in DAMBE7 (Xia, 2018).
Divergence Times Estimate
Divergence times were estimated using a Bayesian framework in BEAST 2.6.1 (Bouckaert et al., 2019). We applied a single nucleotide substitution model (GTR) with a gamma category of 4 to our dataset and implemented a lognormal relaxed clock with a Yule tree prior. The analysis was conducted by running 600 million generations of Markov chain Monte Carlo (MCMC), sampling every 60,000 generations. The results of MCMC runs were visualized in Tracer 1.7 (Rambaut et al., 2018) to confirm a minimum of 200 effective sample size for all parameters. A time-calibrated tree was generated with TreeAnnotator v2.6.0 (Bouckaert et al., 2019), considering 10% of burnin and selecting the maximum clade credibility as the target tree. We do not specify posterior limit for the nodes and we selected mean heights for the common ancestor heights. BEAST analysis was performed in the CIPRES Science Gateway (Miller et al., 2010).
We employed four calibrations points representing three distinct types of priors: (i) fossil record – the first known fossil record of Tamiasciurus hudsonicus, dated from the Irvingtonian (from 1.9 to 0.25 Mya; Steele, 1998), was implemented as uniform prior for the root of Tamiasciurus; (ii) fossil record – Sciurus alleni was first reported from the Late Pleistocene (from 0.126 to 0.0117 Mya; Jakway, 1958) and it was constrained as uniform prior for the split between S. alleni and S. oculatus; (iii) geological events plus fossil record – the crown of Nearctic Sciurus was constrained with uniform prior between 13.6 and 7.4 Mya, considering that Sciurus was present in North America since the late Miocene (Clarendonian; Sciurus olsoni; Emry et al., 2005) and this radiation supposedly arrived from Eurasia (Pečnerová et al., 2015), thus the colonization occurred before the initial opening of the Bering Strait about 7.4 Mya (Marincovich and Gladenkov, 1999); (iv) secondary estimation – we constrained the root of Sciurini with log-normal prior with offset of 13.4 Mya (M = 0.5 and S = 1.0), according to results of Upham et al. (2019), which is currently the most comprehensive phylogeny of mammals.
Ancestral Range and Diversification Events Estimate
We estimated the ancestral range of the phylogenetic nodes and investigated the historical events (e.g., vicariance, dispersal) that might be evoked to explain the diversification pattern of the tribe Sciurini. These analyses were performed employing a maximum likelihood framework using the R package BioGeoBEARS (Matzke, 2013). The time-scaled topology provided by BEAST with only one individual per species was used as the input tree. Six models of evolution—which distinctively incorporate vicariance, dispersal and extinction throughout the cladogenesis—were tested, as follows: (1) Dispersal-Extinction-Cladogenesis (DEC; Ree, 2005), (2) DEC + founder-event speciation (“jump”; DEC + J), (3) Dispersal-Vicariance Analyses (DIVALIKE; Ronquist, 1997), (4) DIVALIKE + J, (5) Bayesian inference of historical biogeography for discrete areas (BAYAREALIKE; Landis et al., 2013), and (6) BAYAREALIKE + J. The best-fitting model was selected based on the Akaike Information Criterion weights (AICw; Wagenmakers and Farrell, 2004).
These biogeographic analyses were performed in two geographic scales: global and Neotropical. The first included all Sciurini species and we investigated their colonization history across large continental areas; species were coded as occupying: (1) Eurasia, (2) Borneo, (3) North America, (4) Central America, and (5) South America. The second focused exclusively on the diversification of Neotropical taxa. For this finer-scale analysis, we followed the Neotropical biogeographical dominions proposed by Morrone (2014) to categorize the current geographical range of species, which were coded as occurring at: (1) the Mexican transition zone, (2) the Mesoamerican dominion, (3) the Pacific dominion, (4) the Boreal Brazilian dominion, (5) the South Brazilian dominion, (6) the South-eastern Amazonian dominion, and (7) the Parana dominion.
Lineage Through Time and Speciation Rates
We investigated the diversification pattern of Sciurini by constructing a lineage through time (LTT) plot using the R package Ape (Paradis and Schliep, 2019). Speciation rates and the presence and temporal location of putative diversification rate shifts were explored by employing the Bayesian Analysis of Macroevolutionary Mixtures (BAMM; Rabosky, 2014; Rabosky et al., 2017). We implemented a speciation-extinction model, run for 10 million generations and sampled every 2,000 generations in BAMM v2.5.0. We incorporated a sampling frequency of 0.93, considering that we have sampled about 93% of the extant species of Sciurini (43 out of the 46 putative species; Abreu-Jr et al., 2020). Results of BAMM analysis were processed and visualized using the R package BAMMtools (Rabosky et al., 2014).
Results
Phylogeny of the Tribe Sciurini
Our final mitogenome alignment was 11,278 bp long and included 45 species (43 ingroup and two outgroup). The mean sequence length per sample was 10,360 bp (ranging from 1,393 to 11,278 bp) and the overall matrix missing data (including gaps and undetermined characters) was 7.4%. We found no evidence for nucleotide substitution saturation in our sequences that could influence the phylogenetic signal (Supplementary Figure 1). The maximum clade credibility tree obtained from our BEAST analysis recovered similar relationships to those previously reported by Abreu-Jr et al. (2020), with all nodes strongly supported (PP ≥ 0.98), excepted by the node of the genus Echinosciurus, which did not receive a significant support (PP < 0.95).
Model Selection in Ancestral Range Estimates
The analyses performed with BioGeoBEARS at the global scale recovered DEC + J as the most suitable model to explain the diversification of Sciurini (LnL = −47.61, AICwt = 0.82; Table 1), considering distinct evolutionary events, such as dispersal, extinction and vicariance. The ancestral range estimation focused on the Neotropical taxa recovered that the model BAYAREALIKE + J better fit our data (LnL = −68.79, AICwt = 0.85; Table 2), favoring dispersal-extinction scenarios rather than vicariance events.
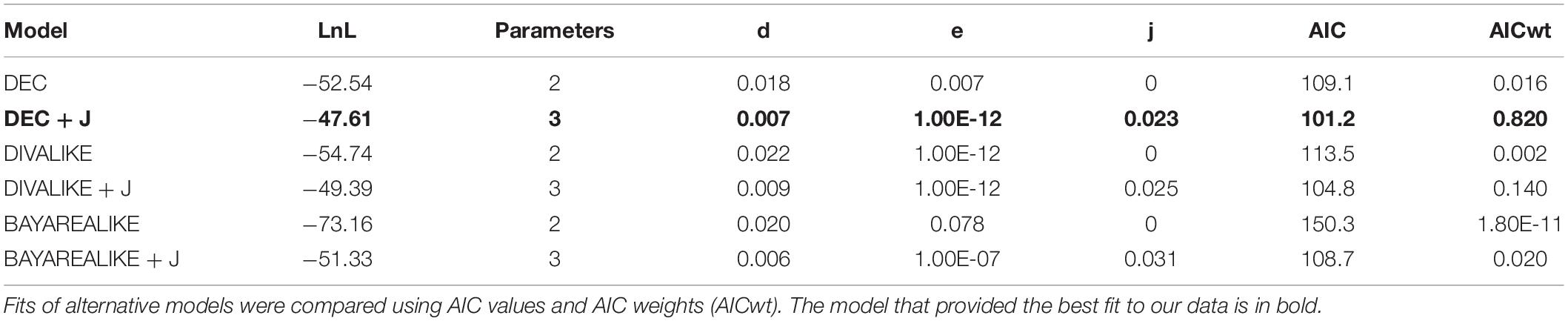
Table 1. Results of the six biogeographic models implemented by BioGeoBEARS on the ancestral range estimation of Sciurini in a global scale.
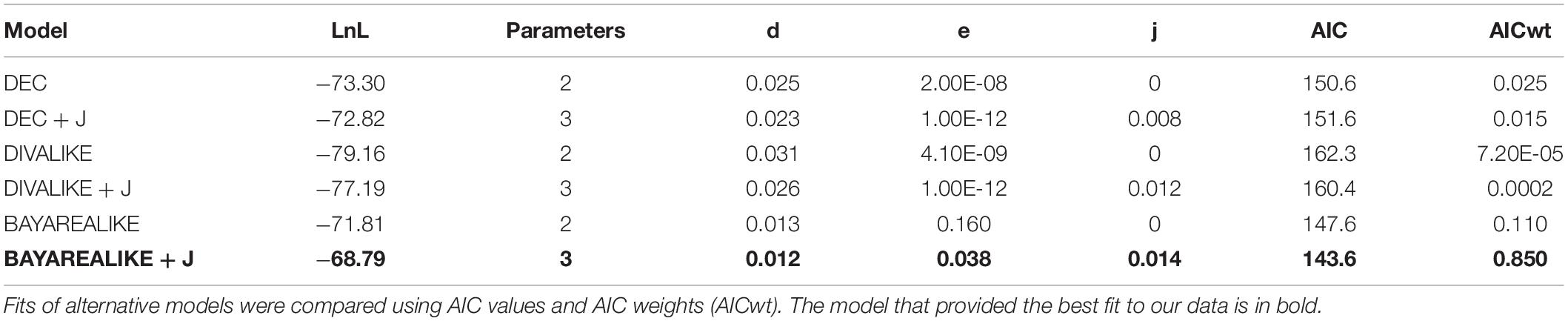
Table 2. Results of the six biogeographic models implemented by BioGeoBEARS on the ancestral range estimation of Sciurini in a Neotropical scale.
Spatiotemporal Diversification of Sciurini at a Global Scale
The origin of the tribe Sciurini was estimated at 14.1 Mya (13.4–15.5; Figure 1; see also Supplementary Figure 2) and its ancestral geographic range was inferred with highest probability to North America (58.6%; Figure 1). An area including both North America and Eurasia was estimated with the second highest probability of occurrence for the ancestor of Sciurini (30.6%; Supplementary Table S2). The first lineage to diverge within the tribe was the genus Tamiasciurus. All of the known species of this genus are from North America and its ancestor was also estimated to have occurred in North America. The subsequent diversification event, leading to the Borneo endemic genus Rheithrosciurus, occurred at 10.5 Mya (9.5–11.7) and the ancestral range of this node was also in North America. Likewise, the ancestral ranges of the nodes leading to the Eurasian genus Sciurus (at 9.7 Mya), the North American genus Hesperosciurus (at 7.6 Mya), and the clade including both North American genera Neosciurus and Parasciurus (at 6.2 Mya), were most likely to be in North America. In summary, diversification events generating the major lineages from North America and Eurasia took place in the mid-late Miocene, whereas most speciation events involving extant species in those lineages occurred in the Pleistocene (Figure 1).
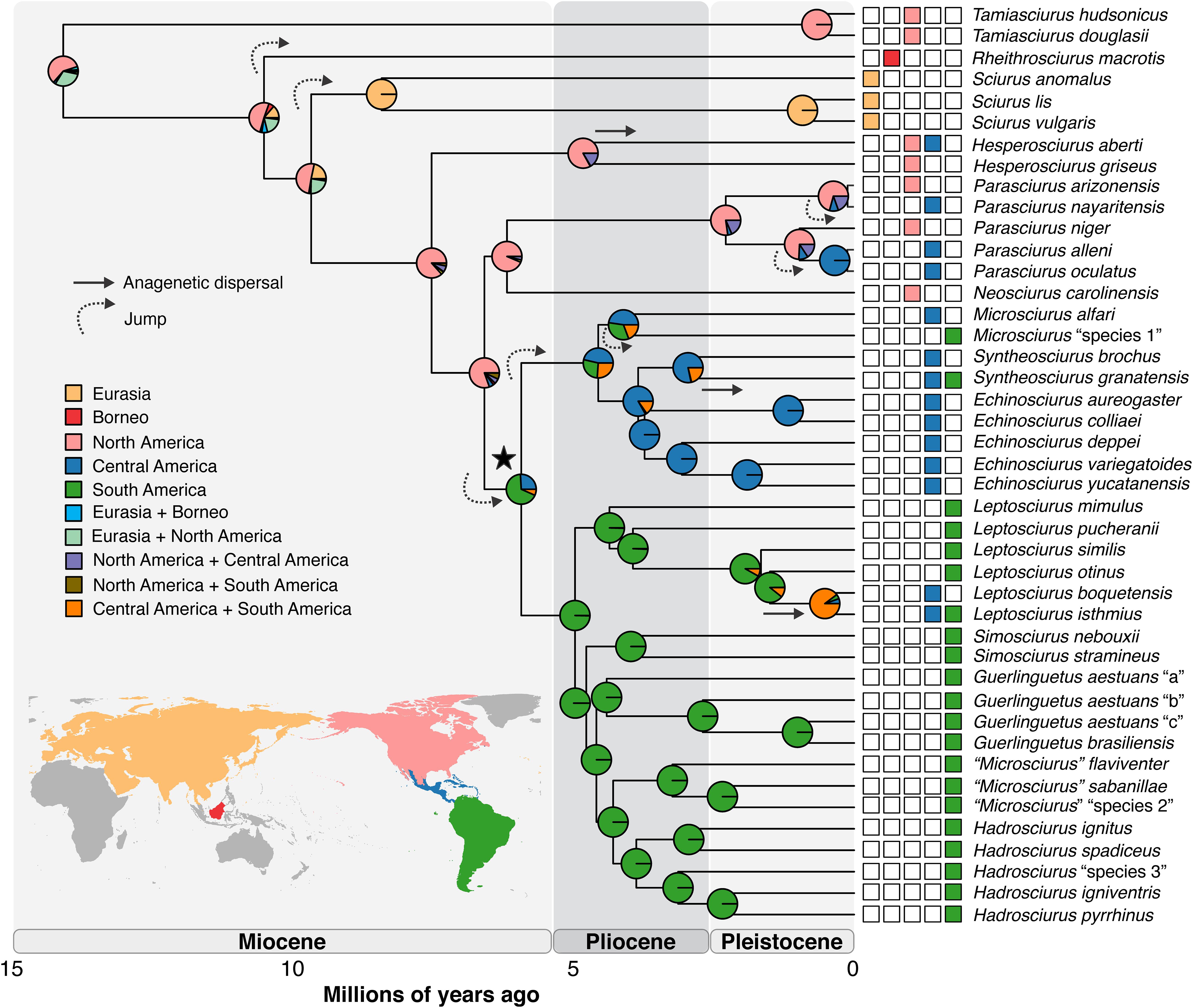
Figure 1. Bayesian maximum clade credibility chronogram based on 13 mitochondrial protein-coding genes (11,278 bp) of 43 species of Sciurini and two species of Pteromyini used as outgroup (not shown). Ancestral ranges for Sciurini estimated by DEC + J model in the global scale analysis are illustrated. Colors in key boxes and map correspond to coloring of internal nodes (estimated probabilities of ancestral ranges) and terminal boxes (current distribution of species). Strait arrows denote anagenetic dispersal events and curved arrows designate founder-event speciation (jump). Black star identifies the node of Neotropical clade.
The large clade including exclusively Neotropical taxa (marked with a black star in Figure 1) originated with highest probability in South America at 5.9 Mya (5.2–6.5). Within this clade, the Central American radiation (including genera Microsciurus, Syntheosciurus, and Echinosciurus) originated at 4.6 Mya (3.8–5.3) and the South American lineage (including genera Leptosciurus, Simosciurus, Guerlinguetus, “Microsciurus,” and Hadrosciurus) at 5.0 Mya (4.3–5.6). Therefore, these Central and South American radiations, as well as most Neotropical genera, originated in the early-mid Pliocene and experienced a rapid diversification, with speciation events occurring mostly in the Pliocene and in the Plio-Pleistocene transition, and only a few during the Pleistocene (Figure 1).
The ancestral range estimation of the tribe Sciurini on a global scale (Figure 1) allows the inference of seven founder-event speciation and three anagenetic dispersals, while it does not indicate the occurrence of vicariance events during the evolutionary history of tree squirrels (see Supplementary Figures 3, 4). The founder events (i.e., a jump of a lineage to a new area of occurrence outside of the ancestral range of the node; Matzke, 2013) were inferred for the ancestors of (1) the genus Rheithrosciurus from North America to Borneo, (2) the Eurasian genus Sciurus from North America to the Palearctic region, (3) the large Neotropical radiation from North America to South America, (4) the Central American clade from South America to Central America, (5) the clade Parasciurus alleni + P. oculatus from North America to Central America, (6) Parasciurus nayaritensis from North America to Central America, and (7) Microsciurus “species 1” from Central America to South America. Anagenetic dispersals included (1) the colonization of Central America by Hesperosciurus aberti—although this species occurs in Central America mainly in the Sierra Madre Occidental and there is a discussion whether this region is part of the Nearctic region (Holt et al., 2013) or it belongs to the Neotropical region (Morrone, 2014)—, (2) colonization of South America by Syntheosciurus granatensis, and (3) colonization of Central America by the ancestor of Leptosciurus boquetensis and L. isthmius.
Spatiotemporal Diversification of Sciurini in the Neotropics
Our biogeographic analyses focused on Neotropical Sciurini suggested with highest probability (92.2%) that this large clade originated in the northwestern portion of South America in the Pacific dominion (Figure 2; Supplementary Table S3). The Pacific dominion was also recovered with highest probability as the ancestral range of the Central American (93.6%) and South American (99.0%) major radiations. Within the Central American radiation, two genera originated in this same region: Microsciurus at 4.1 Mya (3.3–5) and Syntheosciurus at 3 Mya (2.1–3.7). The genus Echinosciurus originated at 3.7 Mya (3–4.4) in an area including the Mexican transition zone and the Mesoamerican dominion.
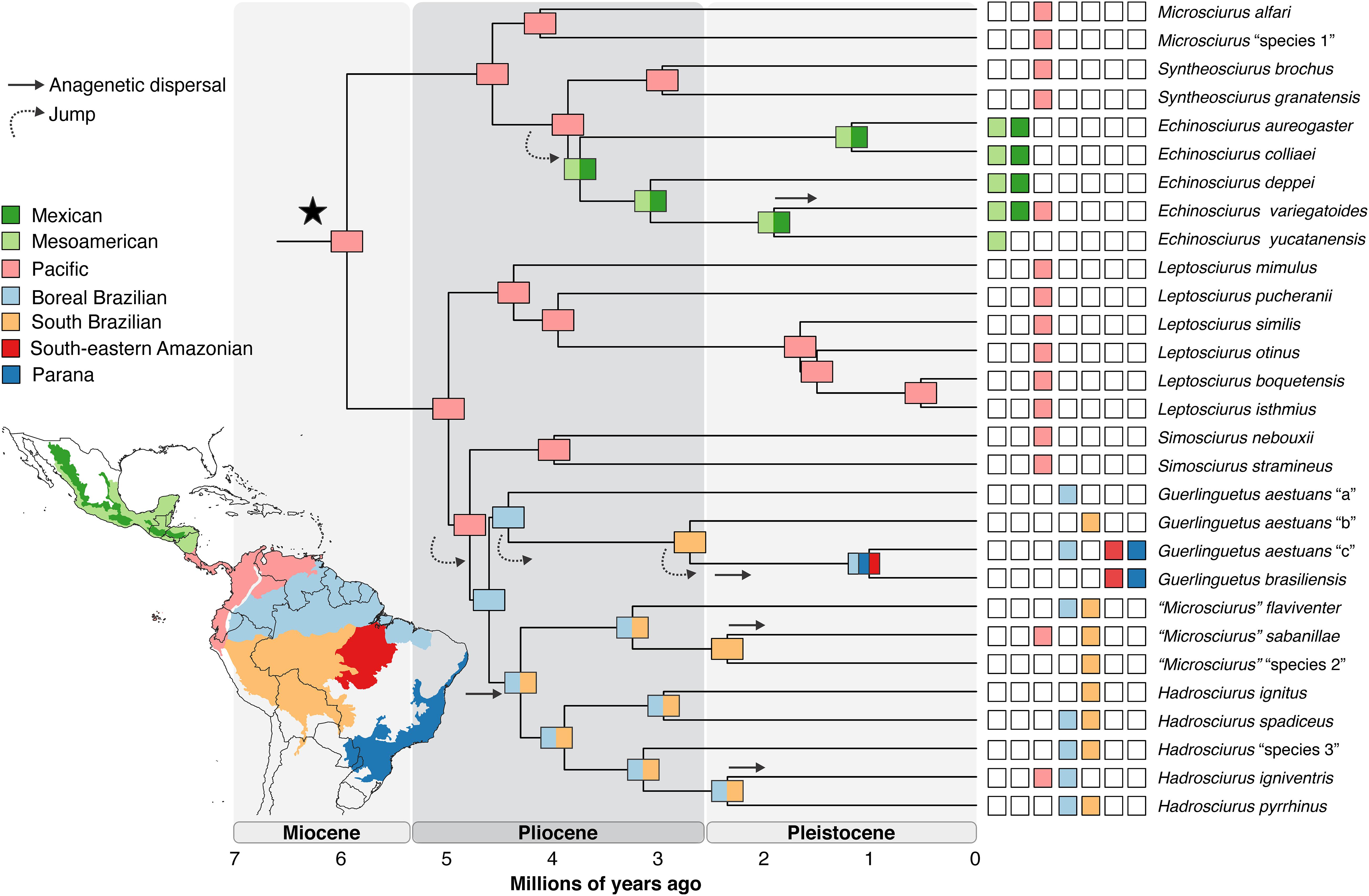
Figure 2. The most probable biogeographical scenario for Neotropical Sciurini based on the set of ancestral ranges that received the highest likelihood in the Neotropical scale analysis, as inferred by BAYAREALIKE + J model. Colors in key boxes and map correspond to coloring of internal tree nodes (most probable ancestral ranges) and terminal taxa (current distribution of species). Strait arrows denote anagenetic dispersal events and curved arrows designate founder-event speciation (jump).
With regards to the South American radiation, the ancestors of the first two genera occupied the northwestern portion of the continent, west of the Andes, in the Pacific dominion: Leptosciurus that originated at 4.4 Mya (3.6–5.1) and Simosciurus that originated at 4 Mya (3.1–4.7). The following genera had their origins on the east side of the Andean cordillera, along the Amazon basin. The genus Guerlinguetus originated at 4.4 Mya (3.9–5.1) in the Boreal Brazilian dominion. The ancestor of the genera “Microsciurus” and Hadrosciurus occupied a large area including the Boreal Brazilian and the South Brazilian dominions. The origin of “Microsciurus” dated to 3.2 Mya (2.5–3.7) and the origin of Hadrosciurus was estimated to 3.9 Mya (3.6–4.9). In summary, the majority of the cladogenetic events observed for Neotropical tree squirrels took place in the northern portion of South America and southern Central America during the Pliocene. Two major dispersal events apparently led to the colonization of Mesoamerica by the genus Echinoscirus and the colonization of the east of the Andes by the most recent common ancestor of the genera Guerlinguetus, “Microsciurus” and Hadrosciurus (Figure 2).
The scenario recovered by this analysis allows the inference of four founder-event speciation and five anagenetic dispersal events during the diversification of the tribe Sciurini across the Neotropics, and, once again, no vicariance event was inferred (see Supplementary Figures 5, 6). The founder-event speciation corresponds to the jump of the ancestors of (1) the genus Echinosciurus from the Pacific dominion to the Mexican transition zone and Mesoamerican dominion, (2) the genera Guerlinguetus, “Microsciurus” and Hadrosciurus from the Pacific dominion to the Boreal Brazilian dominion, (3) (Guerlinguetus aestuans “b”, (G. aestuans “c”, G. brasiliensis) from the Boreal Brazilian dominion to the South Brazilian dominion, and (4) (Guerlinguetus aestuans “c”, G. brasiliensis) from the South Brazilian dominion to Boreal Brazilian, South-eastern Amazon, and Parana dominions. Anagenetic dispersal occurred with the colonization of (1) the Pacific dominion by Echinosciurus variegatoides, (2) the South Brazilian dominion by the ancestor of “Microsciurus” and Hadrosciurus, (3) the Boreal Brazilian and Parana dominions by the ancestor of Guerlinguetus aestuans “c” and G. brasiliensis, (4) the Pacific dominion by “Microsciurus” sabanillae, and (5) the Pacific dominion by Hadrosciurus igniventris.
Diversification Rates of the Tribe Sciurini
The LTT plot indicated an initial low and constant net diversification rate from about 14 to 10.5 Mya, followed by a moderate increase from 10.5 to 5 Mya, and an acceleration on the diversification rate from 5 Mya to the present (Figure 3A). This period of rapid inflation on the number of lineages, along the Pliocene and Pleistocene, coincides with the South American invasion and colonization by tree squirrels (Figure 3A; red star). The Bayesian approach, however, showed a pattern of constant speciation rate through time, with no inflection point and with a slight decrease on the magnitude of speciation over time (Figure 3B). The BAMM analysis also recovered the null model “no shifts” on speciation rate as the most probable (0.66) to represent the diversification of Sciurini. The mean speciation rate of Sciurini was 0.29.
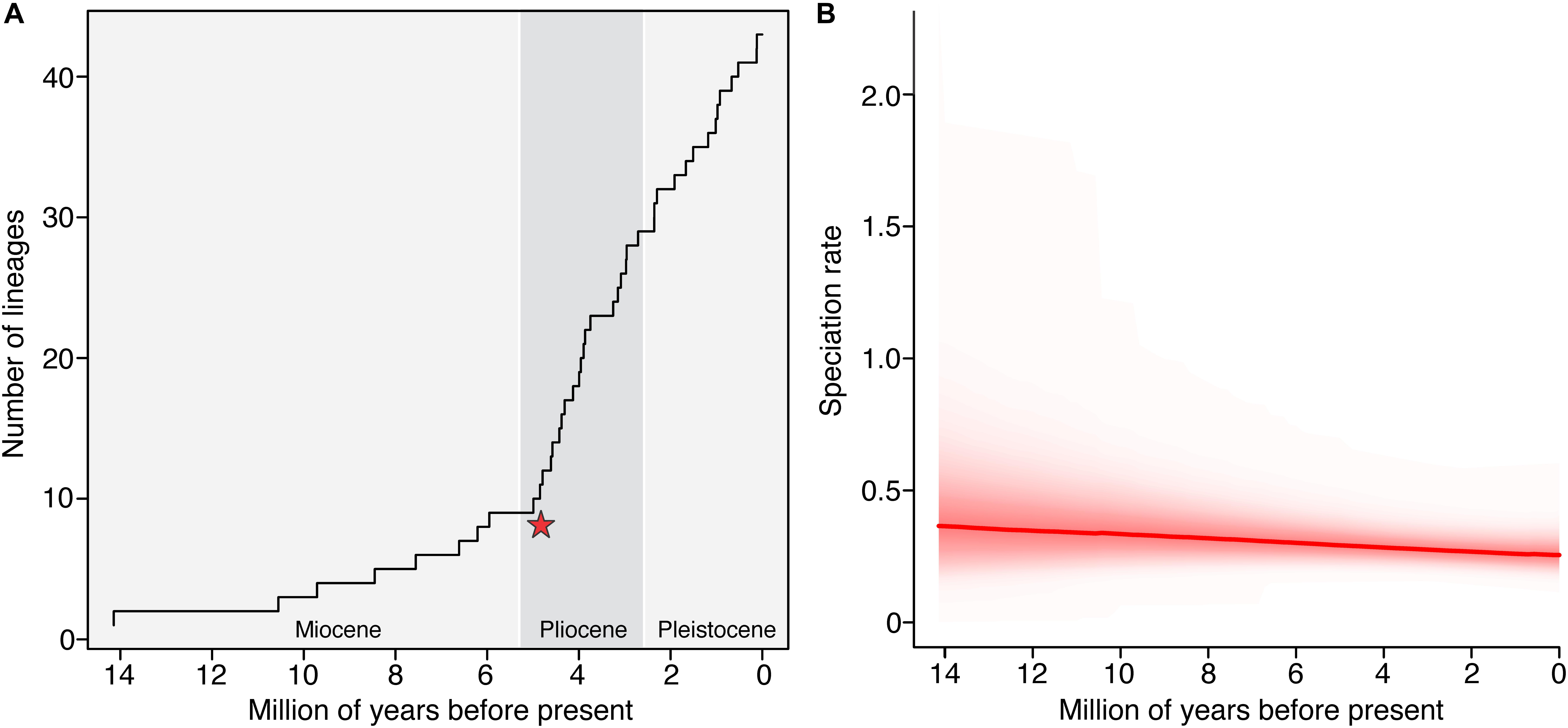
Figure 3. Speciation plots of Sciurini. (A) Lineage-through-time (LTT) plot showing a rapid increase in lineage accumulation after the South American invasion (red star). (B) BAMM analysis demonstrating the mostly constant speciation rate of Sciurini through time; solid red line shows median estimate and thin red lines (cloud) indicate probability distribution.
Discussion
The phylogenetic hypothesis presented here is concordant with the inferences depicted in Abreu-Jr et al. (2020), as we employed the same taxa and data source. We included in our analyses 43 of the 46 currently recognized species of tree squirrels (see Abreu-Jr et al., 2020). Previous biogeographic analyses have included up to 28 Sciurini species, and less than a third of South American tree squirrel species (Pečnerová and Martínková, 2012; Pečnerová et al., 2015). Therefore, this is the most comprehensive study investigating the biogeographic history of the tribe Sciurini, both in number of species and geographic coverage.
The models with best fitting scores on each biogeographic analysis are distinct in their criteria, as one detects vicariant events (DEC) and the other (BAYAREALIKE) does not (Matzke, 2013). Both approaches employed to investigate the range of evolution of tree squirrels (analysis of the whole tribe and analysis focused on Neotropical taxa) did not recover vicariant events during the evolutionary history of Sciurini. These results suggest that vicariance was not a main driver of the diversification processes of tree squirrels at either global or local (Neotropical) scales (but extinctions might mask instances of vicariance; see discussion below). Dispersal events, on the other hand, were commonly recovered and founder-events were estimated to have happened more times than anagenetic dispersals in both approaches.
Our time estimation for the origin of Sciurini was similar to the result of Mercer and Roth (2003) and Upham et al. (2019), supporting a more recent date around 14 Mya compared to the estimation of Zelditch et al. (2015) and Pečnerová et al. (2015) around 18 and 19 Mya, respectively. This is also relatively more recent compared to the origin of the other tribes of the family Sciuridae that have been hypothesized to have happened before 18 Mya (Roth and Mercer, 2008). Our estimates suggested that the most recent common ancestor of Sciurini probably inhabited exclusively North America (near 60% of probability). This is in contrast to previous findings that suggested that the ancestors of this tribe originated in Eurasia or in a large area that included Eurasia and North America (Pečnerová and Martínková, 2012; Pečnerová et al., 2015; Rocha et al., 2016). Despite the higher probability for an exclusive North American origin, our results do not rule out the possibility of an origin that included both the Nearctic and Palearctic regions (supported as the ancestral range of Sciurini with about 30% of probability).
Pečnerová and Martínková (2012) suggested two alternative working hypotheses for the initial diversification and dispersal of tree squirrels. The first one postulates that the ancestor of the genus Sciurus dispersed from North America to Eurasia after diversification from Tamiasciurus. This ancestor diverged again in Eurasia and returned to North America via the Bering land bridge. The second hypothesis claims that ancestors of both Tamiasciurus and Sciurus originally occupied Eurasia and colonized the Americas in two distinct occasions, also crossing over Beringia. However, the results obtained by Pečnerová and Martínková (2012) do not allow them to choose conclusively between these two hypotheses and our results indicate a higher likelihood for a third distinct scenario. We found that the ancestors of Tamiasciurus, Rheithrosciurus, and the ancestor of the clade from which Sciurus diverged, occupied the Nearctic region with highest probability. Our results suggest that the ancestors of Rheithrosciurus and Sciurus dispersed from North America to Eurasia in two independent events, through subsequent jump dispersal events some thousand years apart (Figure 4). The cladogenetic events leading to Rheithrosciurus dated at 10.5 Mya and to the Eurasian Sciurus at 9.7 Mya, which supports the colonization of the Palearctic region by these two lineages before the opening of the Bering Strait around 7.4 Mya (Marincovich and Gladenkov, 1999).
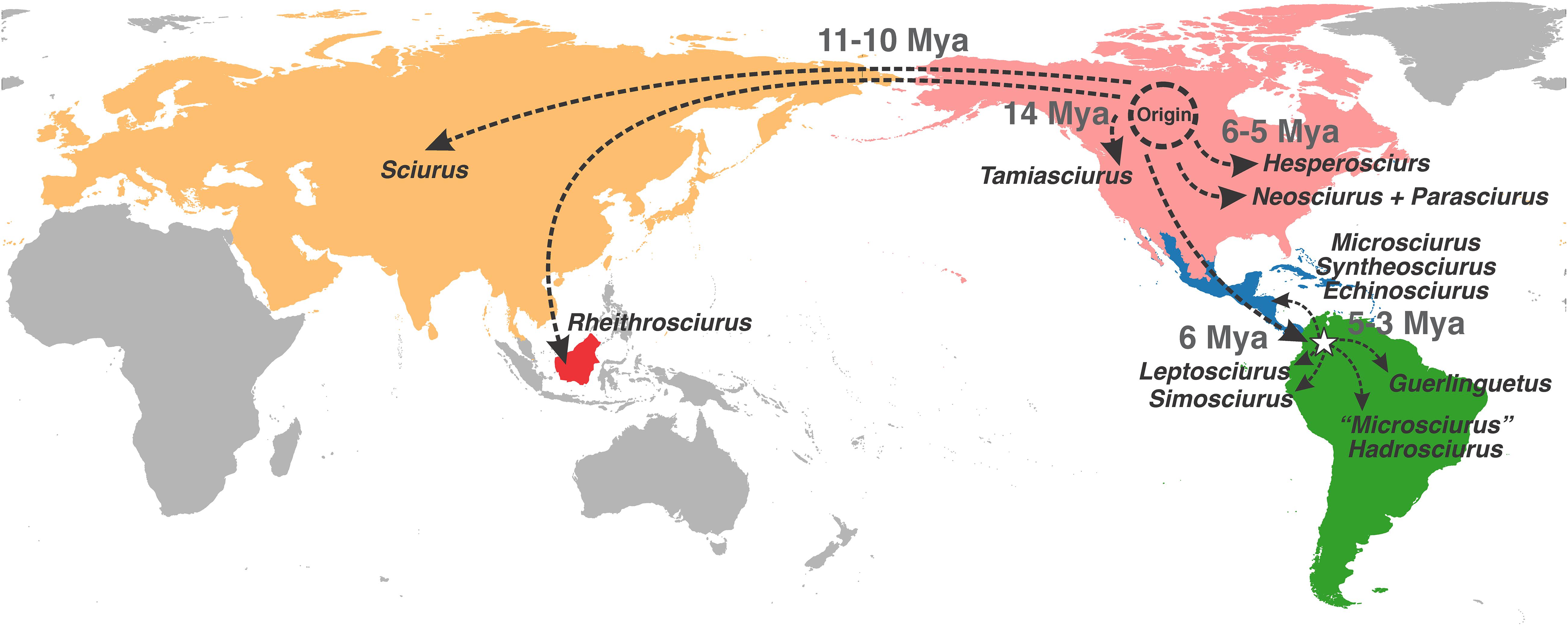
Figure 4. Map summarizing the inferred colonization scenario for the tribe Sciurini showing the timing and the place of its origin and diversification in North America, the two independent dispersions into Eurasia, the invasion of South America, the re-invasion of Central America, and the diversification in South America.
Rheithrosciurus macrotis, endemic to Borneo (Thorington et al., 2012), possibly colonized the island overland from southeast Asia and has been isolated there since its arrival. Borneo is connected to mainland areas through the Sunda Shelf, and the distribution of shallow marine carbonates and the depths of sea water on the Sunda Shelf indicate the presence of past dispersal routes from the continent (perhaps via Java) to Borneo (Hall, 2001). Unlike Rheithrosciurus, the genus Sciurus successfully spread throughout Asia and Europe, and its species are currently widespread in the Palearctic region (Thorington et al., 2012).
Besides Tamiasciurus, the remaining Nearctic genera, Hesperosciurus, Parasciurus, and Neosciurus, also had their ancestors occupying North America. The estimated origin of Hesperosciurus dated to the late Pliocene (around 4.8 Mya), Parasciurus to the late Pleistocene (around 2.3 Mya), and Neosciurus diverged in the late Miocene (around 6.2 Mya). Thus, despite exhibiting the same ancestral range, the North American groups originated in distinct periods, during a relatively large temporal window (about 3.9 Mya) compared to the South American genera (see below).
The ancestral range of the large Neotropical clade was in South America, which indicates that the colonization of Central America by Neotropical taxa was via movement north back from South America (Figures 1, 4). Remarkably, our estimates indicate that this clade originated around 6 Mya, an earlier date than ever suggested. Previous studies had estimated that tree squirrels colonized South America around 3 Mya (Mercer and Roth, 2003; Roth and Mercer, 2008; Pečnerová et al., 2015) and they argued that the South American invasion was conditioned by the closure of the Isthmus of Panama.
The date of the Panamanian land bridge formation—allegedly the moment that triggered the GABI—has gained a lot of attention and discussion in the scientific literature in the past decade. Current biological data suggest an early emergence of the Isthmus, around 6–8 Mya, and indicate that the biotic turnover associated with GABI was much longer and more complex than traditionally recognized, beginning perhaps as early as the Oligocene–Miocene transition (Bacon et al., 2015; Montes et al., 2015; Jaramillo et al., 2017; Molnar, 2017). However, comprehensive geological, paleontological, and also biological reviews pointed to scant evidence and analytical bias in some of the aforementioned studies, and sustained the conventional hypothesis of the formation of the Panamanian Isthmus sensu stricto around 3–2.8 Mya (Lessios, 2008, 2015; Leigh et al., 2014; Marko et al., 2015; O’Dea et al., 2016).
In addition to this ongoing discussion, Agnolin et al. (2019) proposed an alternative hypothesis to explain biotic interchanges between North and South America based on Pre-GABI land connections. The hypothesis from Agnolin et al. (2019) relies on paleontological and geological evidences for a large corridor of shallow waters and emergent spans of land connecting North and South America through the Caribbean islands during the Oligocene-Miocene, known as GAARlandia (Iturralde-Vinent and MacPhee, 1999). The presence of GAARlandia could help to explain the occurrence of early migrants (from the Miocene) in North and South America (see Pinto-Sánchez et al., 2012), considering the lack of strong geological evidence to sustain the early closure of the Panamanian Isthmus. Hershkovitz (1972); Savage (1974) and Reig (1984) also defended a much earlier entrance of land mammals in South America, in the early Miocene, prior to the GABI and the emergence of the land bridge, based on the patterns of diversity and endemism of sigmodontine rodents.
Our results for the first entrance of tree squirrels in South America could either fit this alternative route of colonization through GAARlandia or the traditional route recognized via the Panamanian Isthmus. Even if the complete establishment of the Isthmus occurred in the Pliocene, it might have been permeable to biota migration since the Oligocene (Molnar, 2008; Eizirik, 2012; Carrillo et al., 2014; Bloch et al., 2016). Marshall (1979) already defended that events of marine regression on Upper Miocene would have favored dispersion between South and Central/North America, via the Isthmus, suggesting its permeability. The timeframe of this invasion is also similar to what has been shown for sigmodontine rodents (Smith and Patton, 1999; Steppan et al., 2004; Parada et al., 2013). To the best of our knowledge, no previous hypothesis ever suggested that these two distinct lineages of rodents may have shared a parallel and similar history of invasion and dispersal throughout South America and re-invasion of Central America. During a similar lapse of time, sigmodontine rodents appear to have been more successful in adapting to a greater variety of ecosystems (including grasslands, shrublands, wetlands) and spreading as far as the temperate zone at the southern border of the continent, reaching a diversity of hundreds of species (Burgin et al., 2018). Squirrels, on the other hand, remained restricted to the mid-northern portion of the continent, inhabiting only dense and continuous forests (Vivo and Carmignotto, 2015), exhibiting much less species diversity.
The predominantly Central American radiation (including the genera Microsciurus, Syntheosciurus and Echinosciurus) is more likely to have originated in Central America around 4.6 Mya (probably southern Central America as suggested by the analyses focused on Neotropical taxa, see below), from a jump dispersal from South America. Also, most ancestors of the extant species within this radiation occurred in Central America. Two species, one from the genus Microsciurus (M. “species 1”) and one from the genus Syntheosciurus (S. granatensis), colonized back to South America (Figure 1).
The major South American radiation is slightly older than the Central American one, with its date of origin estimated to around 5 Mya. Within this lineage all the ancestors occupied South America, except the most recent common ancestor of Leptosciurus boquetensis and L. isthmius, estimated to have inhabited Central America (Figure 1). Previous studies (Pečnerová and Martínková, 2012; Pečnerová et al., 2015) suggested a directional diversification process following the latitudinal gradient from North America to South America giving rise to all Neotropical taxa. Our results, supported by ancestral range estimation of Sciurini in both global and Neotropical scales, do not corroborate this hypothesis, and indicate a much more complex pattern, with distinct colonization events from South to Central America and vice-versa and perhaps through distinct dispersal routes.
Several Central American vertebrate lineages that originated along the Pliocene-Pleistocene boundary have been shown to exhibit their ancestral ranges in northern South America (Barrantes, 2009; Saldarriaga-Córdoba et al., 2017; Maestri et al., 2019). Savage (2002) proposed a two-step dispersal pulse hypothesis to explain amphibians and reptiles of South America inhabiting Middle America. The first colonization episode, according to him, occurred about 3.5 Mya when the sea level lowered and many taxa were able to invade Mesoamerica as far as Mexico. The second episode took place in the Pleistocene and the majority of taxa could not disperse further than southern Nicaragua or Costa Rica. The first episode described by Savage (2002) coincides with the diversification and dispersion of the genus Echinosciurus to the Mesoamerican dominion and Mexican transition zone (see Figure 2), and the second episode coincides with the dispersal into Central America by Leptosciurus boquetensis and L. isthmius (see Figure 1). Both episodes of Central American colonization can be plausibly explained via the traditional dispersal route across the Isthmus of Panama.
The biogeographic analysis focused on Neotropical taxa recovered the origin of this clade in the Pacific dominion, which ranges from southern Central America to northwestern South America. This was estimated to have occurred in the late Miocene (around 6 Mya), when the Panamanian land bridge was still emerging and during the final uplift stages of the Andean Cordillera (Hoorn et al., 2010; Stange et al., 2018). This region was also the ancestral range of four genera: Microsciurus and Syntheosciurus (composed of Central and South American species), Leptosciurus (composed of species occupying mainly the northwestern portion of South America), and Simosciurus (composed of two species occurring in the coast of Ecuador and Peru). Within the mainly Central American radiation, a founder-event speciation is inferred, leading to the colonization of the Mexican transition zone and Mesoamerican dominion by the ancestor of the genus Echinosciurus around 3.7 Mya. Moreover, among these species, E. variegatoides colonized back to the Pacific dominion through anagenetic dispersal. Some groups of sigmodontine rodents also had their origin estimated to be in the late Miocene or Pliocene in northern South America, suggesting that this region has played an important role on the origin and diversification of forest rodents (Maestri et al., 2019). In the mid-Pliocene, when several of the cladogenetic events of the Central American radiation have been estimated, important geological events such as the development of the Sierra Madre oriental and occidental and the volcanism of the Trans-Mexican Volcanic Belt were taking place (Sedlock et al., 1993). This intense geologic activity culminating with the formation and expansion of highland forests might have played a crucial role on the diversification of several biotic components (see Morrone, 2010), perhaps including the tree squirrels.
The ancestors of the remaining South American genera (Guerlinguetus, “Microsciurus”, Hadrosciurus) occupied areas east of the Andes with highest probability. This was the point (approximately 4.8 Mya) in the evolutionary history of Sciurini that tree squirrels crossed over the Andes and through a founder-event colonized large forested areas in the eastern portion of South America. Guerlinguetus showed the highest probability of ancestral occupancy in the Boreal Brazilian dominion, which includes the northern bank of the Amazon basin and the Guiana Shield. The ancestors of the genera “Microsciurus” and Hadrosciurus occupied an area including the Boreal Brazilian and South Brazilian dominions. Within Guerlinguetus, the ancestor of three species (G. aestunas “b”, G. aestunas “c” and G. brasiliensis) colonized the South-eastern Amazonian dominion through a founder-event speciation. Through another founder event speciation, the ancestor of G. aestuans “c” + G. brasiliensis colonized the South-eastern Amazonian dominion. Subsequently, it expanded its range, reaching the Boreal Brazilian dominion and, for the first and only time in the tree squirrel diversification, the Atlantic Forest on the coast of Brazil (see Supplementary Figure 6 for details). South-eastern Amazon and Atlantic Forest were the last two areas occupied by tree squirrels in South America.
The timing of Atlantic Forest colonization by tree squirrels (around 1 Mya) is comparable to the timing of the entrance of some species of sigmodontine rodents (such as species in the genera Euryoryzomys, Hylaeamys and Oligoryzomys; Steppan and Schenk, 2017; Maestri et al., 2019). At least two main distinct spatiotemporal routes have been proposed between the Amazon and the Atlantic Forest (Costa, 2003; Batalha-Filho et al., 2013; Ledo and Colli, 2017): older (middle to late Miocene) connections between southeastern Atlantic Forest and western Amazonia (the SE-NW bridge), and younger (Pliocene and Pleistocene) connections across northeastern Brazil (the NE bridge). As our results indicate a young invasion, squirrels might have used NE routes, possibly favored by interglacial periods of the Pleistocene, as the NE bridge hypothesis assumes.
Within the genera “Microsciurus” and Hadrosciurus, most species remained restricted to the Amazon basin and adjacent areas (along Boreal Brazilian and South Brazilian dominions). Only one species in each genus (“Microsciurus” sabanillae and Hadrosciurus igniventris) dispersed to the eastern slopes of the Andean cordillera and invaded the border of the Pacific dominion (Figure 2).
All South American genera originated from 4.4 to 3.2 Mya. This very short temporal window (about 1.2 My) overlaps with the South American Land Mammal Ages (SALMAs) of the Montehermosan (from about 6.8 to 4 Mya) and the Chapadmalalan (from about 4 to 3.4 Mya) (Flynn and Swisher, 1995). At this time, the Andean uplift was complete and the Amazon basin was in its final stages of formation with a large supply of Andean-derived sediments (Hoorn et al., 2010). These massive geological changes produced a great variety of new ecosystems in South America that favored the diversification of many groups of organisms.
South American genera usually do not overlap their distribution, except in a small area on the northern bank of the Amazon river, where one species of Guerlinguetus (G. aestuans “c”) is sympatric with two species of Hadrosciurus (H. igniventris and H. spadiceus), and in the western Amazon basin, where species from “Microsciurus” and Hadrosciurus are mostly sympatric. It is important to mention that these last two genera are the most morphologically dissimilar among all South American forms. Species of “Microsciurus” are small squirrels with head and body length ranging from 125 to 180 mm, and species from Hadrosciurus are large, with head and body length ranging from 220 to 325 mm (Vivo and Carmignotto, 2015). It is also noteworthy that along lowland areas in the western Amazon basin, species of “Microsciurus” are more commonly found in non-flooded upland forests, while species of Hadrosciurus inhabit mainly seasonally inundated floodplain forests (Emmons and Feer, 1997). Besides tree squirrels, South America is the home of another remarkable radiation of Sciuridae, the Neotropical pygmy squirrel, Sciurillus pusillus. This singular radiation diverged early in the history of the family (about 35 Mya) and is included in its own subfamily, Sciurillinae (Mercer and Roth, 2003; Steppan et al., 2004; Roth and Mercer, 2008). The diversification and colonization of South America by the Neotropical pygmy squirrels are enigmatic pieces on the evolutionary history of this group.
Regarding speciation events generating the 43 extant species of Sciurini analyzed here, three species originated in the Miocene, 17 in the Pliocene, and 23 in the Pleistocene. The events in the Miocene were: the diversification of the Borneo endemic species Rheithrosciurus macrotis, the Eurasian Sciurus anomalus, and the North American Neosciurus carolinensis. During the Pliocene, the great majority of speciation events took place in South America, where ten species (from all genera) originated; in Central America five species originated (also from all genera) and in North America only one speciation event occurred (within the genus Hesperosciurus). In the Pleistocene, eight speciation events occurred in South America (within the genera Leptosciurus, Guerlinguetus, “Microsciurus”, and Hadrosciurus), six in North America (within the genera Tamiasciurus and Parasciurus), and six in Central America (within the genera Echinosciurus and Lepstosciurus). In summary, most speciation events in North America occurred late in the Pleistocene, and in South America the majority occurred during the Pliocene or in the Pliocene-Pleistocene transition. Moreover, most cladogenetic events in South and Central America took place in the Pliocene. In North America and Eurasia, cladogenetic events occurred mainly early in the Miocene or late in the Pleistocene (Figure 1).
Pleistocene climatic fluctuations were probably a determining factor in the diversification process within North American lineages, for instance across the genera Tamiasciurus (see Arbogast et al., 2001; Chavez et al., 2011) and Parasciurus. In the Neotropics, Pleistocene fluctuations apparently played a secondary role, as dozens of speciation events occurred before the intensification of climatic cycles. It seems that the Central and South American lineages rapidly adapted to the new (and perhaps vacant) environments and experienced explosive diversification. This is quite different from the history of other rodent radiations in South America, rats and spiny rats, where most of the speciation events took place in the Pleistocene (Fabre et al., 2012; Steppan and Schenk, 2017).
Our analyses revealed a mostly constant (slightly decreasing) speciation rate through time for the tribe Sciurini. However, we observed a peak of lineage accumulation since the beginning of the Pliocene, which coincides with the Neotropical invasion, followed by a rapid diversification in this area. The constancy of the speciation rate (with no shifts during the Pliocene) might indicate that several extinctions may have occurred during the evolutionary history of tree squirrels. In this way, the number of species that went extinct possibly equalized the evident increase in species accumulation since the Pliocene and, therefore, the number of species maintained over time is similar to the number of extinct species. This could also strengthen the prevalence of founder-event speciation and overlook the presence of vicariant events in the ancestral range estimates of Sciurini, as extinctions might be expected to eliminate instances of vicariance and inflate instances of “jump” dispersal. Moreover, our analysis yielded a mean diversification rate around 0.29 for tree squirrels, which is slightly higher than the estimate of Zelditch et al. (2015) and similar to the diversification rate of muroid rodents in the same time frame (Steppan and Schenk, 2017). Finally, we conclude that the South American invasion was not as recent as previously inferred, but the speciation was indeed very fast, mostly restricted to a short period of time in the Pliocene, much earlier than for other groups of rodents.
Data Availability Statement
The dataset generated for this study can be found in the Figshare Repository (https://doi.org/10.6084/m9.figshare.12288185.v1). GenBank accession numbers for all complete mitogenomes are provided in the Supplementary Table S1.
Author Contributions
EA-J, and SP conceived the project. EA-J analyzed the data. EA-J, SP, AP, and JM interpreted and discussed the results. EA-J wrote the manuscript with contributions from SP, MT, DW, AP, JM. SP, AP, DW, and JM secured funding for this project. All authors contributed to the article and approved the submitted version.
Funding
This work was supported by the Conselho Nacional de Desenvolvimento Científico e Tecnológico through a doctoral fellowships to EA-J (147145/2016-3, 203692/2017-9, and 165553/2017-0) and through a productivity scholarship to AP (304156/2019-1); by the American Society of Mammalogists through the Latin American Student Field Research Award to EA-J, providing support for a field expedition to the Rio Purus, BR; by the American Museum of Natural History through a Collection Study Grant, allowing EA-J to collect morphological data from tree squirrels housed at the AMNH; by the Smithsonian Institution through postdoctoral fellowships to SP and MT (Smithsonian Women’s Committee), and through research funds provided to SP, MT, DW, and JM, covering costs associated to laboratory procedures and DNA sequencing; by the National Geographic Society through an Explorers Grant to SP (NGS-381R-18), providing support to visit and collect samples housed at the MUSM and for a field expedition to Parque Nacional del Rio Abiseo, PE, and through Support for Women + Dependent Care to SP (NGS 3758), allowing her to visit and collect additional data in North American museums; and by the Fundação de Amparo à Pesquisa do Estado de São Paulo to AP (09/16009-1), providing support for several field expeditions to the Amazon, BR.
Conflict of Interest
The authors declare that the research was conducted in the absence of any commercial or financial relationships that could be construed as a potential conflict of interest.
Acknowledgments
We are thankful to the following curators and collection support staff, who loaned us preserved or destructive historical tissue samples of valuable specimens included in this study: R. S. Voss, E. Hoeger, and N. Duncan (AMNH); B. D. Patterson and A. W. Ferguson (FMNH); R. M. Timm and M. Eifler (KU); J. A. Oliveira and M. Weksler (MN); J. Silva Junior (MPEG); M. Campbell, J. Cook, and J. Dunnum (MSB); V. Pacheco (MUSM); C. Conroy, J. L. Patton, and F. J. M. Pascal (MVZ); D. P. Lunde, and N. R. Edmison (USNM). We also thank L. Parker, M. Venkatraman, S. Castañeda, and N. McInerney who provided crucial help during lab work.
Supplementary Material
The Supplementary Material for this article can be found online at: https://www.frontiersin.org/articles/10.3389/fevo.2020.00230/full#supplementary-material
FIGURE S1 | Nucleotide substitution saturation plot. The number of transitions (s) and transversions (v) in the mitochondrial genome dataset is plotted against the F84 distances.
FIGURE S2 | Bayesian maximum clade credibility chronogram based on 13 mitochondrial protein-coding genes (11,278 bp) of 43 species of Sciurini and two species of Pteromyini used as outgroup. The mean heights along with the 95% highest posterior density intervals of dates are shown at each node.
FIGURE S3 | Chronogram employed on the range evolution estimation of the tribe Sciurini on a global scale, showing the numbers of the nodes.
FIGURE S4 | The most probable biogeographical scenario for Sciurini based on the set of ancestral ranges that received the highest likelihood in the global scale analysis, as inferred by DEC + J model. Colors in key boxes and map correspond to coloring of boxes within the tree (most probable ancestral ranges) and at terminals (current distribution of species).
FIGURE S5 | Chronogram employed on the range evolution estimation of Sciurini on a Neotropical scale, showing the numbers of the nodes.
FIGURE S6 | The most probable biogeographical scenario for Sciurini based on the set of ancestral ranges that received the highest likelihood in the Neotropical scale analysis, as inferred by BAYAREALIKE + J model. Colors in key boxes and map correspond to coloring of boxes within the tree (most probable ancestral ranges) and at terminals (current distribution of species).
TABLE S1 | List of specimens analyzed in this study, with geographic information and GenBank accession numbers for complete mitochondrial genomes. Taxonomic identifications follow Abreu-Jr et al. (2020). Voucher acronyms refer to the following scientific collections: American Museum of Natural History, United States (AMNH); Field Museum of Natural History, United States (FMNH); University of Kansas Natural History Museum, United States (KU); Museu Nacional da Universidade Federal do Rio de Janeiro, Brazil (MN); Museu Paraense Emilio Goeldi, Brazil (MPEG); Museum of Southwestern Biology, University of New Mexico, United States (MSB); Museo de Historia Natural, Universidad Nacional Mayor de San Marcos, Peru (MUSM); Museum of Vertebrate Zoology, University of California, United States (MVZ); Smithsonian National Museum of Natural History, United States (USNM).
TABLE S2 | Alternative states of ancestral range estimated by DEC + J model in the global scale analyses. Node numbers are the same depicted in the phylogeny of Supplementary Figure S3.
TABLE S3 | Alternative states of ancestral range estimated by BAYAREALIKE + J model in the Neotropical scale analyses. Node numbers are the same depicted in the phylogeny of Supplementary Figure 5. Areas acronyms are: Mexican transition zone (MEX), Mesoamerican dominion (MES), Pacific dominion (PAC), Boreal Brazilian dominion (BBR), South Brazilian dominion (SBR), South-eastern Amazonian dominion (SEA), and Parana dominion (PAR).
References
Abreu-Jr, E. F., Pavan, S. E., Tsuchiya, M. T. N., Wilson, D. E., Percequillo, A. R., and Maldonado, J. E. (2020). Museomics of tree squirrels: a dense taxon sampling of mitogenomes reveals hidden diversity, phenotypic convergence, and the need of a taxonomic overhaul. BMC Evol. Biol. 20, 1–25. doi: 10.1186/s12862-020-01639-y
Agnolin, F. L., Chimento, N. R., and Lucero, S. O. (2019). Pre-gabi biotic connections between the Americas: an alternative model to explain the “less-splendid isolation” of South America. Rev. Geol. Am. Cent. 61, 91–106. doi: 10.15517/RGAC.V61I0.40089
Arbogast, B. S., Browne, R. A., and Weigl, P. D. (2001). Evolutionary genetics and pleistocene biogeography of North American tree squirrels (Tamiasciurus). J. Mammal. 82, 302–319. doi: 10.1093/jmammal/82.2.302
Bacon, C. D., Silvestro, D., Jaramillo, C., Smith, B. T., Chakrabarty, P., and Antonelli, A. (2015). Biological evidence supports an early and complex emergence of the Isthmus of Panama. Proc. Natl. Acad. Sci. U.S.A. 112, 6110–6115. doi: 10.1073/pnas.1423853112
Barrantes, G. (2009). The role of historical and local factors in determining species composition of the highland avifauna of Costa Rica and Western Panamá. Rev. Biol. Trop. 57, 333–349. doi: 10.15517/rbt.v57i0.21360
Batalha-Filho, H., Fjeldså, J., Fabre, P.-H., and Miyaki, C. Y. (2013). Connections between the Atlantic and the Amazonian forest avifaunas represent distinct historical events. J. Ornithol. 154, 41–50. doi: 10.1007/s10336-012-0866-7
Bloch, J. I., Woodruff, E. D., Wood, A. R., Rincon, A. F., Harrington, A. R., Morgan, G. S., et al. (2016). First North American fossil monkey and early Miocene tropical biotic interchange. Nature 533, 243–246. doi: 10.1038/nature17415
Bouckaert, R., Vaughan, T. G., Barido-Sottani, J., Duchêne, S., Fourment, M., Gavryushkina, A., et al. (2019). BEAST 2.5: an advanced software platform for Bayesian evolutionary analysis. PLoS Comput. Biol. 15:e1006650. doi: 10.1371/journal.pcbi.1006650
Burgin, C. J., Colella, J. P., Kahn, P. L., and Upham, N. S. (2018). How many species of mammals are there? J. Mammal. 99, 1–14. doi: 10.1093/jmammal/gyx147
Carrillo, J. D., Forasiepi, A., Jaramillo, C., and Sánchez-Villagra, M. R. (2014). Neotropical mammal diversity and the great American biotic interchange: spatial and temporal variation in South America’s fossil record. Front. Genet. 5:451. doi: 10.3389/fgene.2014.00451
Chavez, A. S., Saltzberg, C. J., and Kenagy, G. J. (2011). Genetic and phenotypic variation across a hybrid zone between ecologically divergent tree squirrels (Tamiasciurus). Mol. Ecol. 20, 3350–3366. doi: 10.1111/j.1365-294X.2011.05184.x
Costa, L. P. (2003). The historical bridge between the Amazon and the forest of brazil a study of molecular phylogeography with small mammals. J. Biogeogr. 30, 71–86. doi: 10.1046/j.1365-2699.2003.00792.x
D’Elía, G. (2000). Comments on recent advances in understanding sigmodontine phylogeny and evolution. Mastozool. Neotrop. 7, 47–54.
Edgar, R. C. (2004). MUSCLE: multiple sequence alignment with high accuracy and high throughput. Nucleic Acids Res. 32, 1792–1797. doi: 10.1093/nar/gkh340
Eizirik, E. (2012). “A molecular view on the evolutionary history and biogeography of Neotropical carnivores (Mammalia, Carnivora),” in Bones, Clones, and Biomes: The History and Geography of Recent Neotropical Mammals, eds B. D. Patterson and L. P. Costa (Chicago, IL: University of Chicago Press), 123–142. doi: 10.7208/chicago/9780226649214.003.0007
Emmons, L. H., and Feer, F. (1997). Neotropical Rainforest Mammals: a Field Guide, Second Edn. Chicago, IL: The University of Chicago Press.
Emry, R. J., Korth, W. W., and Bell, M. A. (2005). A Tree Squirrel (Rodentia, Sciuridae, Sciurini) from the Late Miocene (Clarendonian) of Nevada. J. Vertebr. Paleontol. 25, 228–235. doi: 10.1671/0272-4634(2005)025[0228:atsrss]2.0.co;2
Fabre, P.-H., Hautier, L., Dimitrov, D., and Douzery, E. J. (2012). A glimpse on the pattern of rodent diversication: a phylogenetic approach. BMC Evol. Biol. 12:88. doi: 10.1186/1471-2148-12-88
Flynn, J. J., and Swisher, C. C. III (1995). “Cenozoic South American land mammal ages: correlation to global geochronologies,” in Geochronology, Time Scales and Global Stratigraphic Correlation, eds W. Berggren, D. V. Kent, M.-P. Aubry, and J. Hardenbol (Tulsa, OK: Society of Economic Paleontologists and Mineralogists), 317–334. doi: 10.2110/pec.95.04.0317
Hall, R. (2001). “Cenozoic reconstructions of SE Asia and the SW Pacific: changing patterns of land and sea,” in Faunal and Floral Migrations and Evolution in SE Asia-Australasia, eds I. Metcalfe, J. M. B. Smith, M. Morwood, and I. D. Davidson (Lisse: Swets & Zeitlinger Publishers), 35–56.
Hershkovitz, P. (1966). “Mice, land bridges and Latin American faunal interchange,” in Ectoparasites of Panama, eds R. L. Wenzel, and V. J. Tipton (Chicago, IL: Field Museum of Natural History), 725–751.
Hershkovitz, P. (1969). The recent mammals of the neotropical region: a zoogeographic and ecological review. Quaterly Rev. Biol. 44, 1–70. doi: 10.1086/405975
Hershkovitz, P. (1972). “The recent mammals of the Neotropical region: a zoogeographic and ecological review,” in Evolution, Mammals and Southern Continents, eds A. Keast, F. C. Erk, and B. P. Glass (Albany, NY: State University of New York Press), 311–431.
Holt, B. G., Lessard, J.-P., Borregaard, M. K., Fritz, S. A., Araújo, M. B., Dimitrov, D., et al. (2013). An update of Wallace’s zoogeographic regions of the world. Science 339, 74–78. doi: 10.1126/science.1228282
Hoorn, C., Wesselingh, F. P., ter Steege, H., Bermudez, M. A., Mora, A., Sevink, J., et al. (2010). Amazonia through time: andean uplift, climate change, landscape evolution, and biodiversity. Science 330, 927–931. doi: 10.1126/science.1194585
Hope, A. G., Malaney, J. L., Bell, K. C., Salazar-Miralles, F., Chavez, A. S., Barber, B. R., et al. (2016). Revision of widespread red squirrels (genus: Tamiasciurus) highlights the complexity of speciation within North American forests. Mol. Phylogenet. Evol. 100, 170–182. doi: 10.1016/j.ympev.2016.04.014
Iturralde-Vinent, M. A., and MacPhee, R. D. E. (1999). Paleogeography of the Caribbean region: implications for cenozoic biogeography. Bull. Am. Museum Nat. Hist. 238, 1–95.
Jakway, G. E. (1958). Pleistocene lagomorpha and rodentia from the San Josecito Cave, Nuevo Leon, Mexico. Trans. Kansas Acad. Sci. 61, 313–327.
Jaramillo, C., Montes, C., Cardona, A., Silvestro, D., Antonelli, A., and Bacon, C. D. (2017). Comment (1) on “Formation of the Isthmus of Panama” by O’Dea et al. Sci. Adv. 3:e1602321. doi: 10.1126/sciadv.1602321
Landis, M. J., Matzke, N. J., Moore, B. R., and Huelsenbeck, J. P. (2013). Bayesian analysis of biogeography when the number of areas is large. Syst. Biol. 62, 789–804. doi: 10.1093/sysbio/syt040
Ledo, R. M. D., and Colli, G. R. (2017). The historical connections between the Amazon and the Atlantic forest revisited. J. Biogeogr. 44, 2551–2563. doi: 10.1111/jbi.13049
Leigh, E. G., O’Dea, A., and Vermeij, G. J. (2014). Historical biogeography of the isthmus of Panama. Biol. Rev. 89, 148–172. doi: 10.1111/brv.12048
Lessios, H. A. (2008). The great American schism: divergence of marine organisms after the rise of the central American isthmus. Annu. Rev. Ecol. Evol. Syst. 39, 63–91. doi: 10.1146/annurev.ecolsys.38.091206.095815
Lessios, H. A. (2015). Appearance of an early closure of the isthmus of Panama is the product of biased inclusion of data in the metaanalysis. Proc. Natl. Acad. Sci. U.S.A. 112:E5765. doi: 10.1073/pnas.1514719112
Maestri, R., Upham, N. S., and Patterson, B. D. (2019). Tracing the diversification history of a Neogene rodent invasion into South America. Ecography 42, 683–695. doi: 10.1111/ecog.04102
Marincovich, L., and Gladenkov, A. Y. (1999). Evidence for an early opening of the Bering Strait. Nature 397, 149–151. doi: 10.1038/16446
Marko, P. B., Eytan, R. I., and Knowlton, N. (2015). Do large molecular sequence divergences imply an early closure of the Isthmus of Panama? Proc. Natl. Acad. Sci. U.S.A. 112:E5766. doi: 10.1073/pnas.1515048112
Marshall, L. G. (1979). A model for paleobiogeography of South American Cricetine rodents. Paleobiology 5, 126–132. doi: 10.1017/s0094837300006412
Matzke, N. J. (2013). Probabilistic historical biogeography: new models for founder-event speciation, imperfect detection, and fossils allow improved accuracy and model-testing. Front. Biogeogr. 5, 242–248. doi: 10.21425/f5fbg19694
Mercer, J. M., and Roth, V. L. (2003). The Effects of cenozoic global change on squirrel phylogeny. Science 299, 1568–1572. doi: 10.1126/science.1079705
Miller, M. A., Pfeiffer, W., and Schwartz, T. (2010). “Creating the CIPRES science gateway for inference of large phylogenetic trees,” in Proceedings of the Gateway Computing Environments Workshop, 14 Nov. 2010, New Orleans, LA.
Molnar, P. (2008). Closing of the central American seaway and the ice age: a critical review. Paleoceanography 23, 1–15. doi: 10.1029/2007PA001574
Molnar, P. (2017). Comment (2) on “Formation of the Isthmus of Panama” by O’Dea et al. Sci. Adv. 3:e1602320. doi: 10.1126/sciadv.1602320
Montes, C., Cardona, A., Jaramillo, C., Pardo, A., Silva, J. C., Valencia, V., et al. (2015). Middle miocene closure of the central American seaway. Science 348, 226–229. doi: 10.1126/science.aaa2815
Morrone, J. J. (2010). Fundamental biogeographic patterns across the Mexican transition zone: an evolutionary approach. Ecography 33, 355–361. doi: 10.1111/j.1600-0587.2010.06266.x
Morrone, J. J. (2014). Biogeographical regionalisation of the neotropical region. Zootaxa 3782, 1–110. doi: 10.11646/zootaxa.3782.1.1
O’Dea, A., Lessios, H. A., Coates, A. G., Eytan, R. I., Restrepo-Moreno, S. A., Cione, A. L., et al. (2016). Formation of the Isthmus of Panama. Sci. Adv. 2:e1600883. doi: 10.1126/sciadv.1600883
Parada, A., Pardiñas, U. F. J., Salazar-Bravo, J., D’Elía, G., and Palma, R. E. (2013). Dating an impressive Neotropical radiation: molecular time estimates for the Sigmodontinae (Rodentia) provide insights into its historical biogeography. Mol. Phylogenet. Evol. 66, 960–968. doi: 10.1016/j.ympev.2012.12.001
Paradis, E., and Schliep, K. (2019). ape 5.0: an environment for modern phylogenetics and evolutionary analyses in R. Bioinformatics 35, 526–528. doi: 10.1093/bioinformatics/bty633
Patterson, B., and Pascual, R. (1972). “The fossil mammal fauna of South America,” in Evolution, Mammals and Southern Continents, eds A. Keast, F. C. Erk, and B. P. Glass (Albany, NY: State University of New York Press), 247–309.
Pečnerová, P., and Martínková, N. (2012). Evolutionary history of tree squirrels (Rodentia, Sciurini) based on multilocus phylogeny reconstruction. Zool. Scr. 41, 211–219. doi: 10.1111/j.1463-6409.2011.00528.x
Pečnerová, P., Moravec, J. C., and Martínková, N. (2015). A skull might lie: modeling ancestral ranges and diet from genes and shape of tree squirrels. Syst. Biol. 64, 1074–1088. doi: 10.1093/sysbio/syv054
Pinto-Sánchez, N. R., Ibáñez, R., Madriñán, S., Sanjur, O. I., Bermingham, E., and Crawford, A. J. (2012). The great american biotic interchange in frogs: multiple and early colonization of Central America by the South American genus Pristimantis (Anura: Craugastoridae). Mol. Phylogenet. Evol. 62, 954–972. doi: 10.1016/j.ympev.2011.11.022
Rabosky, D. L. (2014). Automatic detection of key innovations, rate shifts, and diversity-dependence on phylogenetic trees. PLoS One 9:e89543. doi: 10.1371/journal.pone.0089543
Rabosky, D. L., Grundler, M., Anderson, C., Title, P., Shi, J. J., Brown, J. W., et al. (2014). BAMMtools: an R package for the analysis of evolutionary dynamics on phylogenetic trees. Methods Ecol. Evol. 5, 701–707. doi: 10.1111/2041-210X.12199
Rabosky, D. L., Mitchell, J. S., and Chang, J. (2017). Is BAMM flawed? Theoretical and practical concerns in the analysis of multi-rate diversification models. Syst. Biol. 66, 477–498. doi: 10.1093/sysbio/syx037
Rambaut, A., Drummond, A. J., Xie, D., Baele, G., and Suchard, M. A. (2018). Posterior summarization in Bayesian phylogenetics using Tracer 1.7. Syst. Biol. 67, 901–904. doi: 10.1093/sysbio/syy032
Ree, R. H. (2005). Detecting the historical signature of key innovations using stochastic models of character evolution and cladogenesis. Evolution 59, 257–265. doi: 10.1111/j.0014-3820.2005.tb00986.x
Reig, O. A. (1984). Distribuição geográfica e história evolutiva dos roedores muroideos sulamericanos (Cricetidae: Sigmodontinae). Rev. Bras. Genét. II 2, 333–365.
Rocha, R. G., Leite, Y. L. R., Costa, L. P., and Rojas, D. (2016). Independent reversals to terrestriality in squirrels (Rodentia: Sciuridae) support ecologically mediated modes of adaptation. J. Evol. Biol. 29, 2471–2479. doi: 10.1111/jeb.12975
Ronquist, F. (1997). Dispersal-vicariance analysis: a new approach to the quantification of historical biogeography. Syst. Biol. 46, 195–203. doi: 10.1093/sysbio/46.1.195
Roth, V. L., and Mercer, J. M. (2008). Differing rates of macroevolutionary diversification in arboreal squirrels. Curr. Sci. 95, 857–861.
Saldarriaga-Córdoba, M., Parkinson, C. L., Daza, J. M., Wüster, W., and Sasa, M. (2017). Phylogeography of the Central American lancehead Bothrops asper (Serpentes: Viperidae). PLoS One 12:e0187969. doi: 10.1371/journal.pone.0187969
Savage, J. M. (1974). The Isthmian Link and the evolution of neotropical mammals. Los Angeles County Mus. Contr. Sci. 260, 1–51.
Savage, J. M. (2002). The Amphibians and Reptiles of Costa Rica: A Herpetofauna between Two Continents, between Two Seas. Chicago, IL: University of Chicago Press.
Sedlock, R. L., Ortega-Gutierrez, F., Speed, R. C., and Ortega-gutierrez, F. (1993). Tectonostratigraphic Terranes and Tectonic Evolution of Mexico. Boulder, CO: The Geological Society of America, doi: 10.1130/SPE278-p1
Simpson, G. G. (1983). Splendid Isolation: the Curious History of South American Mammals. New Haven, CT: Yale University Press.
Smith, M. F., and Patton, J. L. (1999). Phylogenetic relationships and the radiation of sigmodontine rodents in south america: evidence from cytochrome b. J. Mamm. Evol. 6, 89–128.
Stange, M., Sánchez-Villagra, M. R., Salzburger, W., and Matschiner, M. (2018). Bayesian divergence-time estimation with genome-wide single-nucleotide polymorphism data of sea catfishes (Ariidae) supports miocene closure of the Panamanian Isthmus. Syst. Biol. 67, 681–699. doi: 10.1093/sysbio/syy006
Steele, M. A. (1998). Tamiasciurus hudsonicus. Mamm. Species 586, 1–9. doi: 10.1007/978-1-4020-6754-9_9813
Stehli, F. G., and Webb, S. D. (eds). (1985). The Great American Biotic Interchange. Boston, MA: Springer US. doi: 10.1007/978-1-4684-9181-4
Steppan, S. J., Adkins, R. M., and Anderson, J. (2004). Phylogeny and divergence-date estimates of rapid radiations in muroid rodents based on multiple nuclear genes. Syst. Biol. 53, 533–553. doi: 10.1080/10635150490468701
Steppan, S. J., and Schenk, J. J. (2017). Muroid rodent phylogenetics: 900-Species tree reveals increasing diversification rates. PLoS One 12:e0183070. doi: 10.1371/journal.pone.0183070
Thorington, R. W., Koprowski, J. L., Steele, M. A., and Whatton, J. (2012). Squirrels of the World. Baltimore, MD: The Johns Hopkins University Press.
Upham, N. S., Esselstyn, J. A., and Jetz, W. (2019). Inferring the mammal tree: species-level sets of phylogenies for questions in ecology, evolution, and conservation. PLoS Biol. 17:e3000494. doi: 10.1371/journal.pbio.3000494
Vivo, M., and Carmignotto, A. P. (2015). “Family Sciuridae G. Fischer, 1817,” in Mammals of South America, Volume 2, Rodents, eds J. L. Patton, U. F. J. Pardiñas, and G. D’Elía (Chicago, IL: The University of Chicago Press), 1–48.
Wagenmakers, E. J., and Farrell, S. (2004). AIC model selection using Akaike weights. Psychon. Bull. Rev. 11, 192–196. doi: 10.3758/BF03206482
Woodburne, M. O. (2010). The great American biotic interchange: dispersals, tectonics, climate, sea level and holding pens. J. Mamm. Evol. 17, 245–264. doi: 10.1007/s10914-010-9144-8
Xia, X. (2018). DAMBE7: new and improved tools for data analysis in molecular biology and evolution. Mol. Biol. Evol. 35, 1550–1552. doi: 10.1093/molbev/msy073
Keywords: ancestral range, founder-event speciation, Isthmus of Panama, mitogenome, Neotropics, Sciurini
Citation: Abreu-Jr EF, Pavan SE, Tsuchiya MTN, Wilson DE, Percequillo AR and Maldonado JE (2020) Spatiotemporal Diversification of Tree Squirrels: Is the South American Invasion and Speciation Really That Recent and Fast? Front. Ecol. Evol. 8:230. doi: 10.3389/fevo.2020.00230
Received: 31 January 2020; Accepted: 23 June 2020;
Published: 23 July 2020.
Edited by:
John Lad Koprowski, University of Arizona, United StatesReviewed by:
Bruce D. Patterson, Field Museum of Natural History, United StatesBrian Scott Arbogast, University of North Carolina at Wilmington, United States
Copyright © 2020 Abreu-Jr, Pavan, Tsuchiya, Wilson, Percequillo and Maldonado. At least a portion of this work is authored by Don Wilson and Jesús Maldonado on behalf of the U.S. Government and, as regards Drs. Wilson and Maldonado and the U.S. Government are not subject to copyright protection in the United States. Foreign and other copyrights may apply. This is an open-access article distributed under the terms of the Creative Commons Attribution License (CC BY). The use, distribution or reproduction in other forums is permitted, provided the original author(s) and the copyright owner(s) are credited and that the original publication in this journal is cited, in accordance with accepted academic practice. No use, distribution or reproduction is permitted which does not comply with these terms.
*Correspondence: Edson Fiedler de Abreu-Jr, ZWRzb24uYWJyZXVAdXNwLmJy