- 1FitzPatrick Institute of African Ornithology, DST-NRF Centre of Excellence, Department of Biological Sciences, University of Cape Town, Cape Town, South Africa
- 2Department of Zoology, University of Cambridge, Cambridge, United Kingdom
- 3Centre for Evolutionary Biology, School of Biological Sciences, University of Western Australia, Perth, WA, Australia
Variation in weather patterns can influence reproductive effort and success not only within but also between breeding seasons. Where environmental conditions can be highly variable between years, the weather, and particularly extreme weather events such as heat waves and droughts, may exert a strong influence on reproductive effort (number of breeding attempts) and success (number of surviving young) from one breeding season to the next. We used a 15-year dataset for a cooperatively breeding bird, the southern pied babbler Turdoides bicolor, to determine the impact of high temperatures and drought on reproductive effort and success. We tested the influence on reproductive effort and success of mean daily maximum air temperature and drought both within a breeding season, to determine the relative importance of current conditions, and during the previous breeding season, to determine the relative importance of compensatory effects in response to prior conditions. Reproductive effort and success were lower during breeding seasons characterized by drought, and higher in the breeding seasons that followed droughts, but were not predicted by mean daily maximum temperatures measured over the full length of the breeding season. We provide evidence of compensatory breeding following drought in a bird species endemic to a semi-arid ecosystem and suggest that compensatory mechanisms may be an important part of both long-term population persistence and post-drought population recovery.
Introduction
The sequential, and often cyclical (Korpimäki, 1986; Marra et al., 2015; Nater et al., 2018), nature of biological systems means that each event or process experienced by an individual may have measurable downstream effects (Harrison et al., 2011). Non-fatal effects, such as poor physical condition or delayed breeding, can influence reproduction or survival over time (Marra et al., 2015). Variations in seasonal weather patterns and the occurrence of extreme weather events such as droughts, floods, and heatwaves are among the primary drivers of variation in individual success in vertebrates, influencing reproduction and survival both directly and indirectly, often via effects on resource availability (Harrison et al., 2011). To date, most research on the within and between season effects of weather patterns and extreme events has focused on the influence within an annual cycle (Saino et al., 2011; Laplante et al., 2019) of (a) conditions during early development on individual success as a nestling (Pérez et al., 2008; Auer and Martin, 2017; Ospina et al., 2018), fledgling (Blomberg et al., 2014; de Zwaan et al., 2019), or adult (Porcelli et al., 2016; Hsu et al., 2017; Marshall et al., 2017) and (b) seasonal weather conditions on body mass (Loison and Langvatn, 1998; van de Pol et al., 2016), behavior (Akresh et al., 2019; Rauber et al., 2019), survival (Berryman and Lima, 2006; Gardner et al., 2017, 2018; Woodworth et al., 2017; Chiffard et al., 2019), and reproductive investment (Clutton-Brock et al., 1991; Monteuil-Spencer, 2017).
Effects of extreme weather events can be cumulative, and can have devastating consequences for population persistence (Cruz-McDonnell and Wolf, 2016; Wingfield et al., 2017; Bourne et al., 2020b), particularly if such events re-occur more quickly than populations are able to recover from them (Enright et al., 2015). Although weather conditions in highly seasonal temperate environments can vary considerably between years (Griesser et al., 2017), distinct seasonal weather patterns lead to the relatively predictable onset and duration of annual breeding seasons (Greño et al., 2008; Simmonds et al., 2017; Laplante et al., 2019). This differs somewhat from more arid sub-tropical environments, where breeding seasons typically span many months and animals respond flexibly and opportunistically to highly variable weather conditions and less defined seasonality (Griffith et al., 2016; Hidalgo Aranzamendi et al., 2019). In hotter, drier, and less predictable environments, the effects of variation in weather patterns and of extreme weather events may manifest as foregone or failed breeding in poor years (McCreedy and van Riper, 2015; Conrey et al., 2016; van de Ven, 2017; Cooper et al., 2019; Moore and Martin, 2019; Sharpe et al., 2019). This may subsequently lead to greater investment in breeding in relatively more favorable years (Jetz and Rubenstein, 2011; Lerch et al., 2018), but this effect has received much less research attention.
Few studies have explicitly tested the different explanatory power of processes occurring within vs. between annual cycles to predict survival and reproductive success [although see Gardner et al. (2017) and Griesser et al. (2017)]. Additionally, few studies have considered the effects of weather conditions in one breeding season on individual success in the subsequent breeding season [although see Moyes et al. (2006)]. Species living in more arid sub-tropical environments are potentially useful models for studying the effects of weather patterns and extreme events between years, because these ecosystems are characterized by high interannual variability and frequent extremes in temperature and rainfall (McKechnie et al., 2012). High temperatures and droughts have been linked to delayed or failed reproduction in a number of arid and semiarid-zone species (Cunningham et al., 2013; Cruz-McDonnell and Wolf, 2016; Nater et al., 2018; van de Ven et al., 2020a). Compensatory responses to severe weather events, like heat waves and droughts, may act over relatively long timescales to reduce or offset the negative effects of exposure to high temperatures or drought on reproductive success. These could be extremely important for population persistence over time (Wiley, 2017; Paniw et al., 2019), yet have been relatively little studied in subtropical environments.
Here, we consider the relative influence of weather conditions within vs. between annual cycles (consecutive austral summer breeding seasons) on reproductive effort (number of clutches laid and incubated) and success (number of young surviving to nutritional independence) in a cooperatively breeding bird, the southern pied babbler Turdoides bicolor (hereafter “pied babblers”), endemic to a semi-arid subtropical region in southern Africa. We explore the potential for compensatory mechanisms in response to severe weather conditions characteristic of semi-arid environments, specifically high temperatures and drought. We additionally consider the influence of group size on reproductive effort and success, and whether effects of high temperatures or drought were moderated by group size. Cooperative species may respond differently to external stressors than non-cooperative species, because reproductive outcomes can be affected by both the presence and behavior of other group members (Crick, 1992; Cockburn et al., 2008; Meade et al., 2010; Langmore et al., 2016; Wiley and Ridley, 2016). We predict that high temperatures and drought experienced within a breeding season will suppress both reproductive effort and success, and that pied babblers will compensate for this suppression by increasing investment in reproductive effort and success in breeding seasons that follow hot and dry conditions. We expect positive effects of group size on both reproductive effort and success, hypothesizing that, if cooperation helps to buffer against environmental effects (Rubenstein and Lovette, 2007; Cornwallis et al., 2017; Lukas and Clutton-Brock, 2017; van de Ven et al., 2020a), reproduction should be less affected by high temperatures and drought in larger groups.
Method
Study Site and System
Fieldwork was undertaken at the 33 km2 Kuruman River Reserve (KRR; 26°58’S, 21°49’E) and neighboring farms in the southern African Kalahari. Droughts are a regular feature of the local climate (Jury, 2013; Tokura et al., 2018) and rainfall has been declining, and high temperature extremes increasing in both frequency and severity, over the last 20 years (Kruger and Sekele, 2013; van Wilgen et al., 2016; van de Ven, 2017).
Pied babblers are medium-sized (60–90 g), cooperatively breeding passerines (Ridley, 2016). Groups range in size from 3 to 15 individuals, consisting of a single breeding pair with subordinate adult helpers (Nelson-Flower et al., 2011). Pied babblers are sexually mature one year after hatching and are defined as adults at this age (Ridley, 2016). Pied babblers may attempt to breed several times within a single breeding season, re-laying if breeding attempts fail and/or if conditions allow (Ridley and Raihani, 2008; Raihani et al., 2010). They can also produce overlapping broods, whereby the breeding pair initiates and incubates a new clutch while the helpers are still feeding dependent fledglings from a previous breeding attempt (Ridley and Raihani, 2008). Some breeding occurs over winter when there has been late-season rain, but the breeding season typically extends from September to March (austral summer) (Ridley, 2016; Bourne et al., 2020a) and thus annual breeding cycles cross calendar years.
Groups in the study population were visited weekly during each of the 15 breeding seasons between September 2005 and February 2020 to check group composition and record life history events such as breeding, immigration, and dispersal. Pied babblers are territorial and groups can be reliably located by visits to each territory (Golabek et al., 2012). Detailed life-history data have been collected from a study population of pied babblers at the KRR since 2003 (Ridley and Raihani, 2007; Ridley, 2016). Birds in the study population are habituated to observation at distances of 1–5 m and uniquely identifiable by a combination of metal and color leg-rings (Ridley and Raihani, 2007).
Data Collection
Breeding Effort and Success
Breeding effort was defined as the number of breeding attempts initiated, as determined by the number of discrete clutches laid and incubated per group per breeding season. Breeding success was defined as the total number of nutritionally independent young raised per group per season. Fledglings are considered nutritionally independent by 90 days of age (calculated from day of hatching), by which age they typically receive <1 feed per hour from adult group members (Ridley and Raihani, 2007).
Collection of nest life history data followed Ridley and van den Heuvel (2012): nests were located by observing nest-building during weekly monitoring visits; incubation start, hatch, and fledge dates were determined by checking nests every two to three days; and breeding attempts were considered to have failed when nests were no longer attended or dependent fledglings were not seen on two consecutive visits. In most cases, it was not possible to determine the proximate cause of nest failure or fledgling death. Average group size (the average number of adults present in the group over a breeding season; mean = 4.1 ± 1.4, range: 2–9) was recorded for each group per breeding season.
Temperature and Rainfall
Daily maximum air temperature (Tmax, °C) and rainfall (mm) data were collected from an on-site weather station (Vantage Pro2, Davis Instruments, Hayward, United States). Missing data from 2009, 2010, and 2011 were sourced from a nearby South African Weather Services weather station (Van Zylsrus, 28 km from the KRR) which produces significantly repeatable temperature measurements (Lin’s concordance correlation coefficient rc = 0.957, 95 % CI: 0.951–0.962), and moderately repeatable rainfall measurements (rc = 0.517, 95 % CI: 0.465–0.566) in comparison with the on-site weather station. Differences in rainfall were small (mean difference = 0.045 ± 3.075 mm; 95 % CI = -5.981 to 6.072 mm), suggesting that both weather stations adequately detected wet vs. dry periods. Long term rainfall data for the region, used to determine the presence or absence of a meteorological drought within a breeding season, was obtained from a South African Weather Services weather station at Twee Rivieren (∼120 km from the KRR; available until 2013).
Daily Tmax values were averaged for each breeding season in which nest monitoring occurred (September–March, MeanTMaxSeason(1,2,..,t)), and for the preceding breeding season (previous September–March, Mean TMaxSeason(t–1)). Rainfall was summed for each breeding season in which nest monitoring occurred (RainSeason(t)), and for the preceding breeding season (RainSeason(t–1)). Following Mayaud et al. (2017), meteorological drought within a breeding season (DroughtSeason(t)) or preceding breeding season (DroughtSeason(t–1)) was defined as ≤75% of average precipitation between September and March (≤135.75 mm), using the 30-year period 1984–2013 to determine average precipitation.
Statistical Analyses
Statistical analyses were conducted in R v 3.4.1 R Core Team (2017). To determine which variables predicted (a) number of breeding attempts and (b) breeding success per group per year, we used generalized linear mixed effects models with a Poisson distribution (log link) in the package lme4 (Bates et al., 2015). Model selection with Akaike’s information criterion corrected for small sample size (AICc) and maximum likelihood estimation was used to test a series of models to determine which best explained patterns of variation in the data (Harrison et al., 2018). Where multiple models were within 5 AICc of the top model, top model sets were averaged using the package MuMIn (Barton, 2015). All continuous explanatory variables were scaled by centering and standardising by the mean (Harrison et al., 2018). Rainfall and drought within the same annual cycle were highly correlated (F1,154 ≥ 359.940, p < 0.001), since these variables represent the same pattern in different ways. We used the categorical variable, drought vs no drought, as the rainfall parameter in all analyses because models including this variable had a consistently lower AICc. Drought is also of direct interest for our study because it represents the presence or absence of extreme weather in our study system. Measures of temperature and drought were also correlated (F1,154 ≥ 9.706, p < 0.002), with droughts typically occurring in hotter years. Group sizes were slightly smaller in years following droughts, but the difference is not statistically significant (mean group size in breeding seasons following drought = 4.1 ± 1.3; mean group size in breeding seasons following no drought = 4.6 ± 1.5; F1,188 = 2.515, p = 0.116). Correlated variables were not included in the same additive models, but interactions between correlated variables were tested (Harrison et al., 2018). Model terms with confidence intervals not intersecting zero were considered to explain significant patterns within our data (Grueber et al., 2011). Model fits were assessed by checking dispersion parameters using the package RVAideMemoire (Herve, 2019).
Groups in which the breeding pair split during the breeding season (n = 18 of 177 group-seasons) were excluded from analysis, since the continuity of the breeding pair is an important determinant of reproductive success in pied babblers (Wiley and Ridley, 2018). Exploratory analyses showed that reproductive allocation in the previous season (e.g., number of days invested in breeding in Season(t–1)) were not associated with variation in breeding effort or success in our study system, and we therefore did not include prior reproductive allocation in the model sets tested here (see Supplementary Tables S1–S4 and Supplementary Figure S1). Average group size, DroughtSeason(t), DroughtSeason(t–1), MeanTMaxSeason(t) and MeanTMaxSeason(t–1), and the two-way interactions between all climate and group size variables were included as fixed effects, with group identity included as a random effect in both analyses. Since interactions between group size and environmental parameters on reproduction could provide evidence for a moderating effect of group size, we conducted sensitivity power analyses (Cohen, 1988; Leon and Heo, 2009; Greenland et al., 2016), using the package pwr (Champely et al., 2018), to confirm that we had sufficient sample size to detect small main effects in both analyses (Cohen’s f2 < 0.06) and moderate-large effects of two-way interactions in analyses of reproductive effort (f2 = 0.17) and reproductive success (f2 = 0.27).
Results
The average summer maximum daily temperature at the study site from 2005 to 2020 was 34.5 ± 1.4°C (range in annual average summer maximum temperatures, September–March, 32.4–37.8°C). Summer rainfall averaged 174.0 ± 70.1 mm (range 64.4–291.2 mm). Droughts occurred in 6 of 15 summer breeding seasons studied (2006/07, 2012/13, 2014/15, 2015/16, 2018/19, and 2019/2020; Figure 1). DroughtSeason(t) and DroughtSeason(t–1) were independent (X21 = 0.228, p = 0.633). Group size averaged 4.1 ± 1.4 adults per group per season (range 2–9 adults), and we analyzed data from an average of 13 ± 4 groups per breeding season (range 6–19 groups).
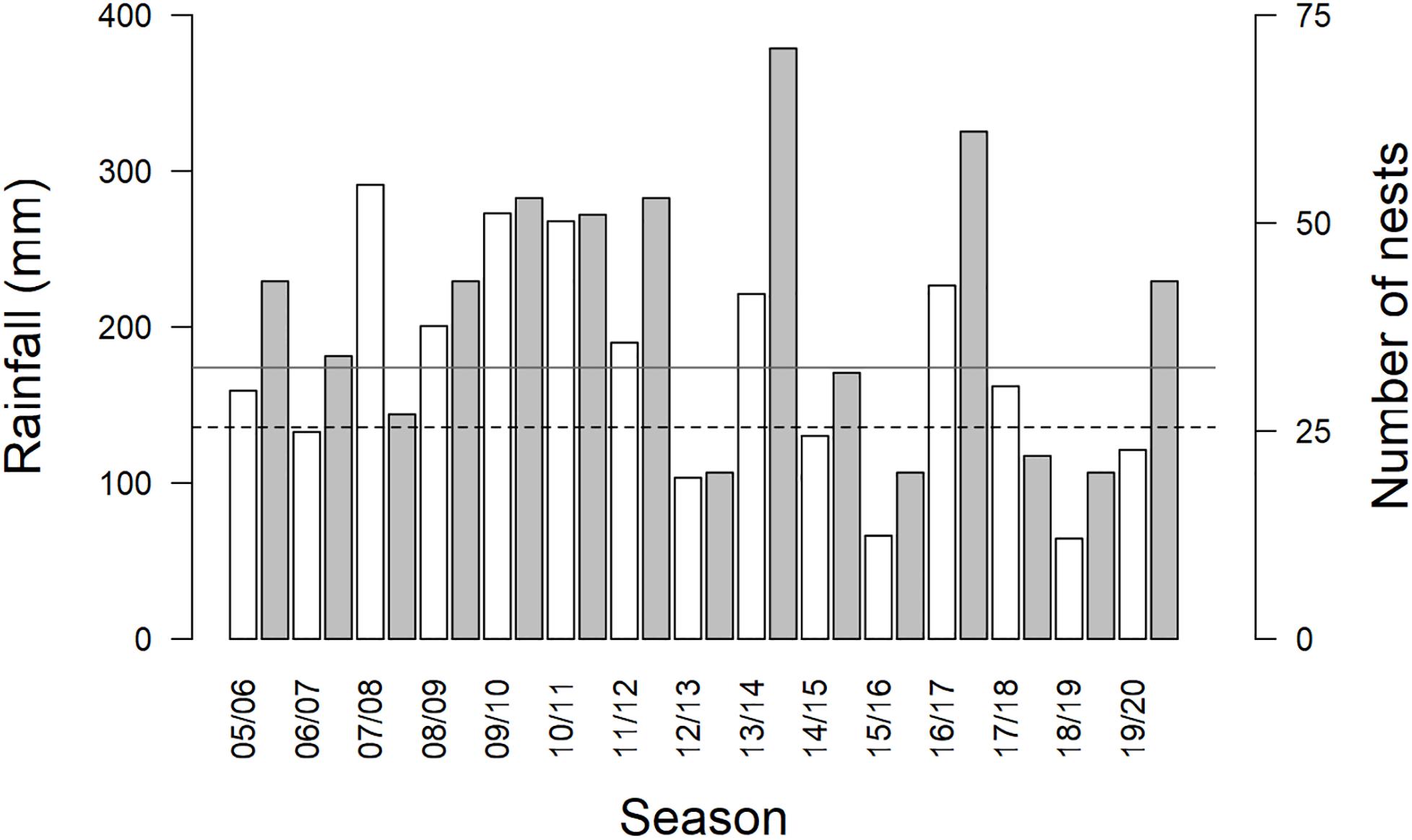
Figure 1. Total rainfall (mm, white bars) and number of nests (gray bars) per austral summer breeding season (September–March). Drought occurred in six of fifteen breeding seasons. Rainfall bars below the dashed line at 135.75 mm rainfall indicate drought. Mean rainfall for the study site (174 mm) is shown on the figure by the gray solid horizontal line. Increases in the number of nests disproportionate to increases in rainfall occurred in several years following drought (e.g. 13/14, 16/17).
We observed particularly large increases in the total number of breeding attempts in several breeding seasons that followed a drought (DroughtSeason(t–1); for example, see 2013/2014 and 2016/2017 in Figure 1). These increases were disproportionate to the amount of precipitation recorded within the breeding season (RainSeason(t)). The number of breeding attempts per group varied between breeding seasons (range 1–9, mean = 3.2 ± 1.8; n = 190 group-seasons). Breeding effort was lower in breeding seasons characterized by drought (DroughtSeason(t)), averaging 2.1 ± 0.9 attempts per group compared to 4.0 ± 1.9 attempts in non-drought breeding seasons (Figure 2 and Table 1). Breeding effort increased when there had been a drought in the previous breeding season (DroughtSeason(t–1)), averaging 4.1 ± 2.2 attempts per group in breeding seasons preceded by drought compared to 2.8 ± 1.5 attempts when not preceded by drought (Figure 2 and Table 1). We found no evidence for an effect of temperature, group size, or any interaction between group size and weather on the number of breeding attempts per group per season (see Supplementary Table S5 for full model output).
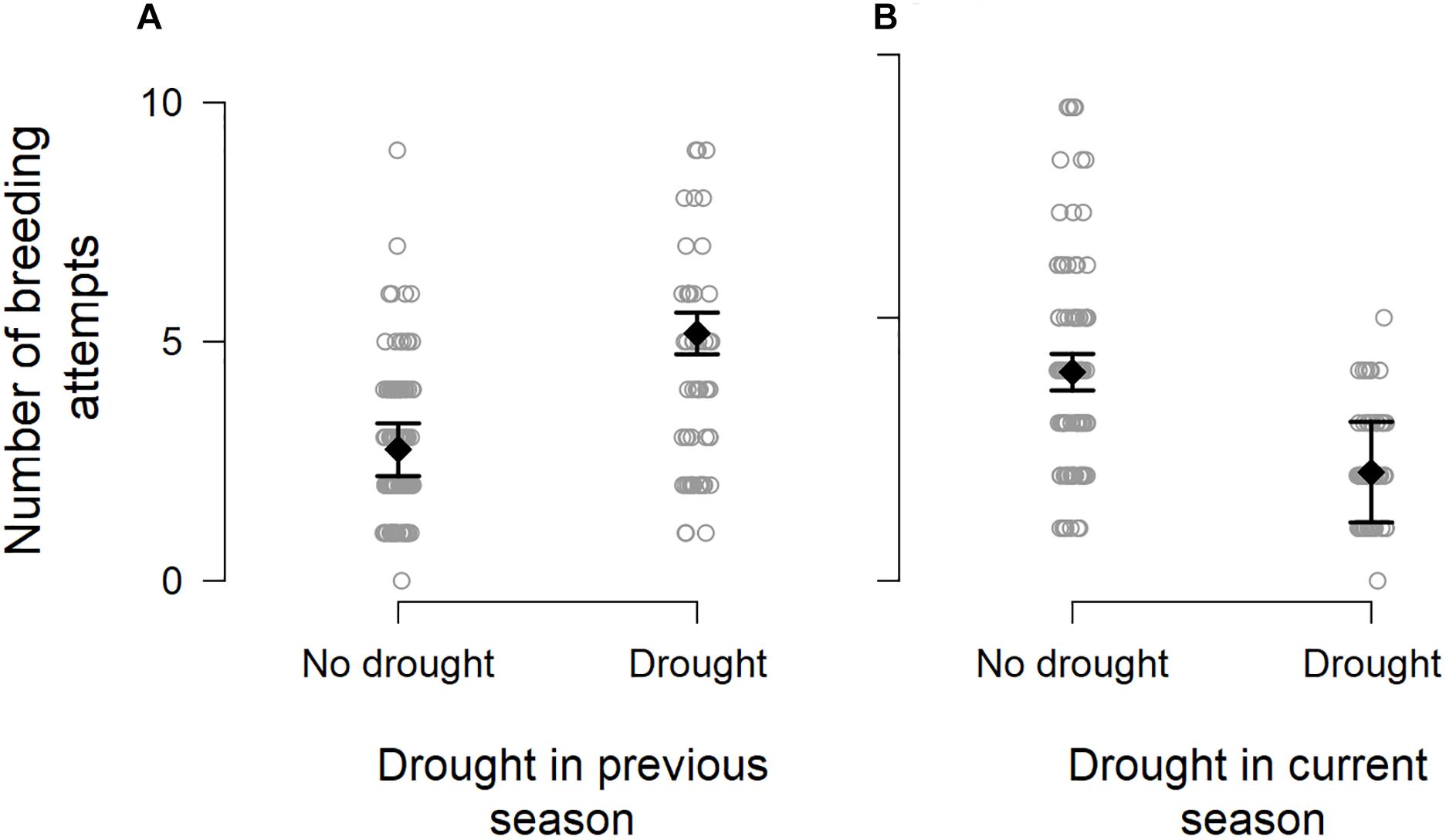
Figure 2. Number of breeding attempts initiated per group per breeding season in relation to (A) the occurrence of drought in a previous breeding season, showing the model-predicted mean (black filled diamond) ± 1.96 × standard error (black whiskers), and (B) the occurrence of drought during the current breeding season. Data points are jittered for improved visibility.
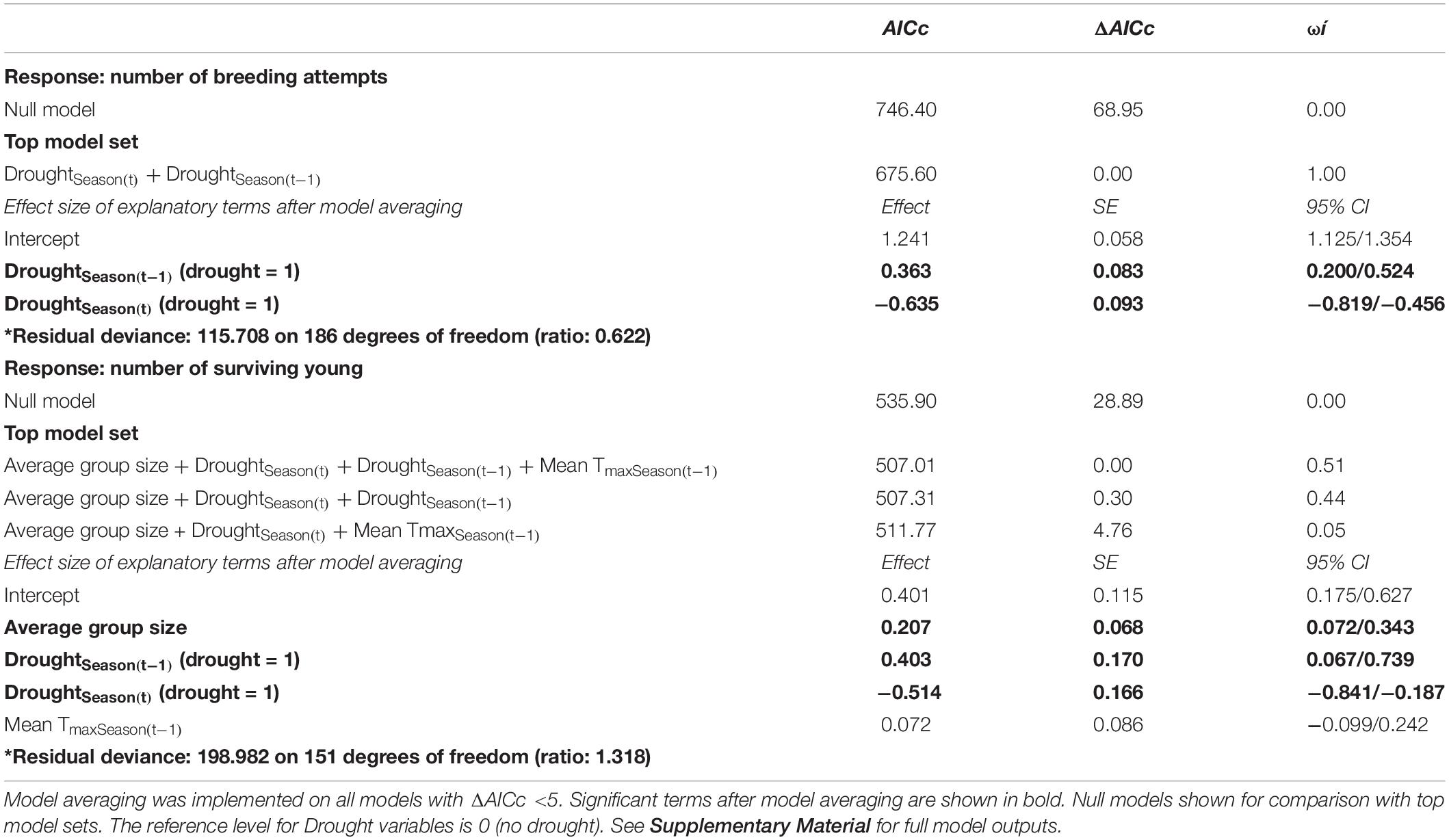
Table 1. Top GLMM model sets for analyses on the number of breeding attempts per group per season (n = 190 group-seasons from 39 groups over 15 breeding seasons) and the number of surviving young per group (n = 156 group-seasons from 32 groups over 15 breeding seasons).
Breeding success per group varied between breeding seasons (range 0–7 independent young produced, mean = 1.6 ± 1.5; n = 156 group-seasons) and, like the number of breeding attempts, was suppressed in breeding seasons characterized by drought within the current breeding season (DroughtSeason(t)), averaging 1.0 ± 1.1 surviving young per group compared to 1.9 ± 1.7 surviving young in non-drought breeding seasons (Figure 3 and Table 1). Breeding success increased when there had been a drought in the previous breeding season (DroughtSeason(t–1)), averaging 2.1 ± 1.7 surviving young per group in breeding seasons preceded by drought compared to 1.4 ± 1.4 attempts when not preceded by drought. Larger groups produced more surviving young than smaller groups (Figure 3C and Table 1), but there was no evidence for an effect of temperature or any interaction between group size and weather on breeding success (see Supplementary Table S6 for full model output).
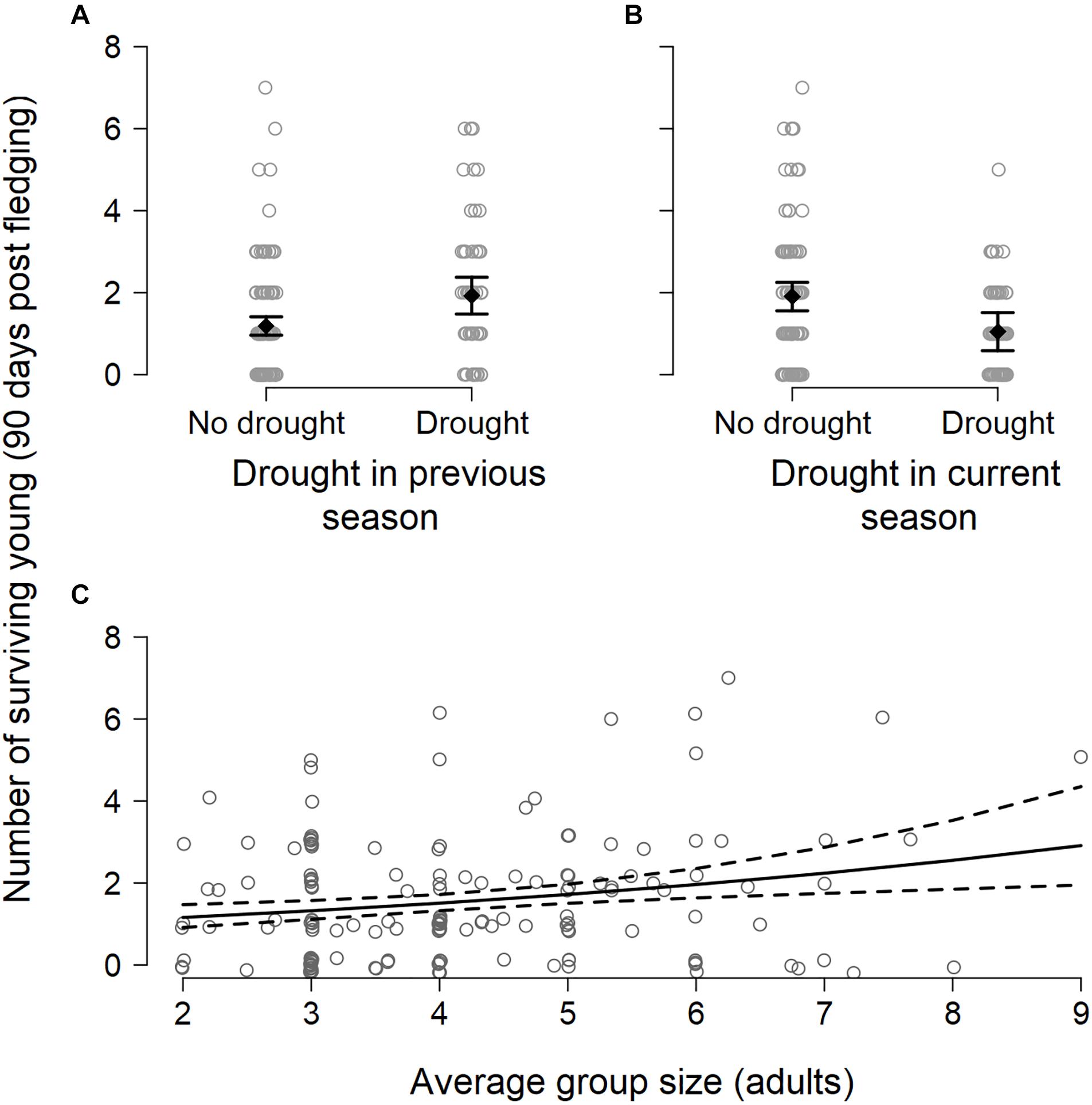
Figure 3. Number of surviving young (90 days post fledging) produced per group per breeding season in relation to (A) the occurrence of drought in a previous breeding, showing the model-predicted mean (black filled diamond) ± 1.96 × standard error (black whiskers), (B) the occurrence of drought during the current breeding season, and (C) average group size during the current breeding season. Data are jittered for improved visibility.
Discussion
We investigated the relative importance of impacts of within and between year variation in temperature, rainfall, and group size on reproduction in a cooperatively breeding bird, and the potential role of compensatory mechanisms in response to drought. Both breeding effort and success were lower in pied babblers when they experienced droughts during the breeding season. Pied babbler groups initiated more breeding attempts and were more successful in breeding seasons following drought, indicating the presence of compensatory behavior in response to harsh conditions. Drought was the most important climate-related predictor of reproductive effort and success in pied babblers, a finding which is consistent with other studies of birds breeding in subtropical environments (Morrison and Bolger, 2002; Skagen and Yackel Adams, 2012; Zuckerberg et al., 2018; Hidalgo Aranzamendi et al., 2019). While high temperatures during breeding attempts are commonly associated with reduced reproductive success in birds (Cunningham et al., 2013; van de Ven et al., 2020b), including in this population of pied babblers (Wiley and Ridley, 2016; Bourne et al., 2020a), we did not find strong effects of mean daily maximum temperatures calculated over the full length of the breeding season. Our broad-scale analysis considers all breeding attempts within a season, rather than individual nests or fledglings, and, in semi-arid systems, temperature effects are often immediate and direct [via effects on physiology of individuals (McKechnie, 2019)], while rainfall effects are often lagged and generally indirect [via effects on available food resources (Cumming and Bernard, 1997; Hidalgo Aranzamendi et al., 2019)]. Our decision to analyze the data at the scale of the full breeding season may, therefore, explain why we identify stronger drought than temperature effects. We found no evidence that group size interacted with climatic factors to moderate the effects of climate extremes: while larger groups were able to produce more surviving young than smaller groups on average (also see Ridley, 2016; Ridley and van den Heuvel, 2012), this pattern did not vary according to climatic conditions.
Pied babblers undertake most breeding during September to December each year, largely independent of rainfall (Bourne et al., 2020a), but will breed until later in the season in response to rainfall within the breeding season (Ridley, 2016). Pied babblers are able to re-clutch while raising dependent fledglings when conditions allow, due to the presence of task partitioning among group members (Ridley and Raihani, 2008; Ridley and van den Heuvel, 2012). These reproductive behaviors provide a mechanism through which pied babblers can respond flexibly to interannual variation in rainfall, laying and incubating more clutches and producing more surviving young in breeding seasons that follow a drought. An alternative explanation for the pattern that we observed could be that higher numbers of clutches initiated in non-drought years indicate higher rates of nest predation, an important cause of reproductive failure in birds (Mayer et al., 2009; DeGregorio et al., 2015; Mortensen and Reed, 2018). Previous research in sociable weavers (Philetairus socius) has shown that reproductive effort (defined as number of clutches laid and incubated) increases when predation is high (Mares et al., 2017). In cases of high reproductive effort in response to high predation risk, the number of fledglings produced per breeding attempt is typically low (Mares et al., 2017). Additionally, in arid environments, higher predation rates are often associated with warm, dry weather (McCreedy and van Riper, 2015; Kozma et al., 2017). Our results show that pied babblers produced fewer surviving young during droughts and more surviving young per breeding attempt in breeding seasons following a drought. This represents an effect of greater investment in breeding during breeding seasons following a drought, rather than simply more clutches being laid due to higher rates of predation or nest failure. The pattern of producing more surviving young per breeding attempt in breeding seasons following a drought also cannot be explained by years following droughts being significantly wetter (Iknayan and Beissinger, 2018; Sharpe et al., 2019), since droughts were not consistently followed by wetter conditions (Figure 1).
We found that larger groups of pied babblers produced more surviving young, a benefit of cooperation that may be important for post-drought recovery and overall population persistence in this species (Wiley, 2017). The observed group size effect is likely driven by the presence of helpers reducing predation risk at nests (Raihani and Ridley, 2007; Valencia et al., 2016), and enabling the production of multiple, overlapping broods per breeding season (Ridley and Raihani, 2008; Valencia et al., 2016). Cooperative species also tend to raise more broods to independence per breeding season than non-cooperative species (Ridley and van den Heuvel, 2012). Group size did not interact with temperature or rainfall to predict either reproductive effort or reproductive success, suggesting that breeding pied babblers respond similarly to variation in the weather regardless of helper number (van de Ven et al., 2020a). Flexible responses to drought, including compensatory breeding in breeding seasons following a drought, were observed across all group sizes, confirming that pied babblers do not vary their response to climatic conditions in relation to group size.
In summary, we demonstrate that weather conditions in both previous and current annual cycles exert a powerful influence on reproductive effort and success in a cooperatively breeding, desert-adapted passerine. Cooper et al. (2019) and Griesser et al. (2017) provide evidence that birds across a range of environments can distinguish relative weather conditions over shorter timescales and may be able to adjust their reproductive allocation depending on variable within-season weather conditions. Our data suggest that pied babblers can respond to relative conditions over fairly long timescales and, importantly, they may engage in compensatory breeding when environmental conditions allow (see Hatchwell, 1999 for compensatory adjustments to parental care in cooperatively breeding birds). Compensatory breeding in years following drought is therefore likely to be an extremely important part of post-drought recovery and overall population persistence in this (Wiley, 2017) and other species (van de Ven, 2017; Paniw et al., 2019). However, given that rapid increases in the frequency and severity of droughts are predicted under climate change (MacKellar et al., 2014; Wise and Lensing, 2019), compensatory mechanisms which have been successful at maintaining populations in the past may prove insufficient to allow population recovery between extreme events (Enright et al., 2015; Cruz-McDonnell and Wolf, 2016). An improved understanding of the ways in which animals employ flexible breeding strategies and compensatory mechanisms is thus critical for informing population viability models and conservation management actions when accounting for the impact of climate variability and change on animal populations.
Data Availability Statement
The datasets underlying all analyses presented in this study have been archived at the University of Cape Town’s open access institutional data repository, ZivaHub (a figshare platform), where they are publicly available at doi: 10.25375/uct.12471569.v1.
Ethics Statement
Permission to conduct this research was given by the University of Cape Town Animal Ethics Committee under animal ethics permit numbers R2012/2006/V15/AR and 2016/V6/SC and by the South African Department of Environment and Nature Conservation under fauna research permit number 1216/2016.
Author Contributions
AR started habituation of the study animals and collection of life history data in 2003 and has maintained it ever since; this was central to making the study possible. AB undertook all fieldwork from 2016 onwards and drafted the manuscript. AB and AR analyzed the data. All authors conceived the study, contributed substantially to revisions, and gave final approval for publication.
Funding
This work was funded by a South African Department of Science and Technology Centre of Excellence grant (grant number UID: 40470) awarded to the University of Cape Town and facilitated by the National Research Foundation, the University of Cape Town Postgraduate Funding Office, the Oppenheimer Memorial Trust (Grant No. 20747/01 to AB), the British Ornithologists’ Union, the Australian Research Council (Grant No. FT110100188 to AR), the National Research Foundation of South Africa (Grant No. 110506 to SC), and a BBSRC David Phillips Fellowship (BB/J014109/1 to CS). The opinions, findings and conclusions are those of the authors alone, and the National Research Foundation accepts no liability whatsoever in this regard. The KRR was financed by the Universities of Cambridge and Zurich, the MAVA Foundation, and the European Research Council (Grant No. 294494 to Tim Clutton-Brock), and received logistical support from the Mammal Research Institute of the University of Pretoria.
Conflict of Interest
The authors declare that the research was conducted in the absence of any commercial or financial relationships that could be construed as a potential conflict of interest.
Acknowledgments
We thank the management teams at the Kuruman River Reserve (KRR) and surrounding farms, Van Zylsrus, South Africa, for making the work possible. We thank Sello Matjee, Paige Ezzey, and Lesedi Moagi for fieldwork assistance during 2016–2019, and all past and present staff and students of the Pied Babbler Research Project for data collected since 2003. We thank Dr. Todd Erickson and the reviewers MG and DR for their valuable comments on previous drafts, which improved the manuscript.
Supplementary Material
The Supplementary Material for this article can be found online at: https://www.frontiersin.org/articles/10.3389/fevo.2020.00190/full#supplementary-material
References
Akresh, M. E., King, D. I., and Marra, P. P. (2019). Examining carry-over effects of winter habitat on breeding phenology and reproductive success in prairie warblers (Setophaga discolor). J. Avian Biol. 50:jav.02025.
Auer, S. K., and Martin, T. E. (2017). Parental care mitigates carry-over effects of poor early conditions on offspring growth. Behav. Ecol. 28, 1176–1182. doi: 10.1093/beheco/arx082
Barton, K. (2015). MuMIn: Multi-Model Inference. Available online at: https://cran.r-project.org/web/packages/MuMIn/MuMIn.pdf (accessed December 17, 2019).
Bates, D., Maechler, M., Bolker, B., and Walker, S. (2015). Fitting linear mixed effects models using lme4. J. Stat. Softw. 67, 1–48.
Berryman, A., and Lima, M. (2006). Deciphering the effects of climate on animal populations: diagnostic analysis provides new interpretation of Soay sheep dynamics. Am. Nat. 168, 784–795. doi: 10.1086/508670
Blomberg, E. J., Sedinger, J. S., Gibson, D., Coates, P. S., and Casazza, M. L. (2014). Carryover effects and climatic conditions influence the postfledging survival of greater sage-grouse. Ecol. Evol. 4, 4488–4499. doi: 10.1002/ece3.1139
Bourne, A. R., Cunningham, S. J., Spottiswoode, C. N., and Ridley, A. R. (2020a). High temperatures drive offspring mortality in a cooperatively breeding bird. Proc. R. Soc. Lond. Biol. Sci. [bioRxiv].
Bourne, A. R., Cunningham, S. J., Spottiswoode, C. N., and Ridley, A. R. (2020b). Hot droughts compromise interannual survival across all group sizes in a cooperatively breeding bird. Ecol. Lett. [bioRxiv].
Champely, S., Ekstrom, C., Dalgaard, P., Gill, J., Weibelzahl, S., Anandkumar, A., et al. (2018). pwr: Basic Functions for Power Analysis. Available online at: https://github.com/heliosdrm/pwr (accessed December 17, 2019).
Chiffard, J., Delestrade, A., Yoccoz, N. G., Loison, A., and Besnard, A. (2019). Warm temperatures during cold season can negatively affect adult survival in an alpine bird. Ecol. Evol. 9, 12531–12543. doi: 10.1002/ece3.5715
Clutton-Brock, T. H., Price, O. F., Albon, S. D., and Jewell, P. A. (1991). Persistent instability and population regulation in Soay sheep. J. Anim. Ecol. 60, 593–608.
Cockburn, A., Sims, R. A., Osmond, H. L., Green, D. J., Double, M. C., and Mulder, R. A. (2008). Can we measure the benefits of help in cooperatively breeding birds: The case of superb fairy-wrens Malurus cyaneus? J. Anim. Ecol. 77, 430–438. doi: 10.1111/j.1365-2656.2007.01351.x
Cohen, J. (1988). Statistical Power Analysis for the Behavioral Sciences, 2nd Editio Edn. New York, NY: Lawrence Erlbam Associates.
Conrey, R. Y., Skagen, S. K., Yackel Adams, A. A., and Panjabi, A. O. (2016). Extremes of heat, drought and precipitation depress reproductive performance in shortgrass prairie passerines. Ibis 158, 614–629. doi: 10.1111/ibi.12373
Cooper, C. E., Withers, P. C., Hurley, L. L., and Griffith, S. C. (2019). The field metabolic rate, water turnover, and feeding and drinking behavior of a small avian desert granivore during a summer heatwave. Front. Physiol. 10:1405. doi: 10.3389/fphys.2019.01405
Cornwallis, C. K., Botero, C. A., Rubenstein, D. R., Downing, P. A., West, S. A., and Griffin, A. S. (2017). Cooperation facilitates the colonization of harsh environments. Nat. Ecol. Evol. 1:0057.
Crick, H. P. (1992). Load-lightening in cooperatively breeding birds and the cost of reproduction. Ibis 1, 56–61. doi: 10.1111/j.1474-919x.1992.tb07230.x
Cruz-McDonnell, K. K., and Wolf, B. O. (2016). Rapid warming and drought negatively impact population size and reproductive dynamics of an avian predator in the arid southwest. Glob. Chang. Biol. 22, 237–253. doi: 10.1111/gcb.13092
Cumming, G. S., and Bernard, R. T. F. (1997). Rainfall, food abundance and timing of parturition in African bats. Oecologia 111, 309–317. doi: 10.1007/s004420050240
Cunningham, S. J., Martin, R. O., Hojem, C. L., and Hockey, P. A. R. (2013). Temperatures in excess of critical thresholds threaten nestling growth and survival in a rapidly-warming arid savanna: a study of common fiscals. PLoS One 8:e74613. doi: 10.1371/journal.pone.0074613
de Zwaan, D. R., Camfield, A. F., MacDonald, E. C., and Martin, K. (2019). Variation in offspring development is driven more by weather and maternal condition than predation risk. Funct. Ecol. 33, 447–456. doi: 10.1111/1365-2435.13273
DeGregorio, B. A., Westervelt, J. D., Weatherhead, P. J., and Sperry, J. H. (2015). Indirect effect of climate change: shifts in ratsnake behaviour alter intensity and timing of avian nest predation. Ecol. Model. 312, 239–246. doi: 10.1016/j.ecolmodel.2015.05.031
Enright, N. J., Fontaine, J. B., Bowman, D. M. J. S., Bradstock, R. A., and Williams, R. J. (2015). Interval squeeze: altered fire regimes and demographic responses interact to threaten woody species persistence as climate changes. Front. Ecol. Evol. 13, 265–272. doi: 10.1890/140231
Gardner, J. L., Rowley, E., De Rebeira, P., Rebeira, A., De, and Brouwer, L. (2018). Associations between changing climate and body condition over decades in two southern hemisphere passerine birds. Clim. Chang. Responses 5, 1–14.
Gardner, J. L., Rowley, E., De Rebeira, P., Rebeira, A., De, Brouwer, L., et al. (2017). Effects of extreme weather on two sympatric Australian passerine bird species. Philos. Trans. R. Soc. B 372:20160148. doi: 10.1098/rstb.2016.0148
Golabek, K., Ridley, A. R., and Radford, A. (2012). Food availability affects strength of seasonal territorial behaviour in a cooperatively breeding bird. Anim. Behav. 83, 613–619. doi: 10.1016/j.anbehav.2011.11.034
Greenland, S., Senn, S. J., Rothman, K. J., Carlin, J. B., Poole, C., Goodman, S. N., et al. (2016). Statistical tests, P values, confidence intervals, and power: a guide to misinterpretations. Eur. J. Epidemiol. 31, 337–350.
Greño, J. L., Belda, E. J., and Barba, E. (2008). Influence of temperatures during the nestling period on post-fledging survival of great tit Parus major in a Mediterranean habitat. J. Avian Biol. 39, 41–49. doi: 10.1111/j.0908-8857.2008.04120.x
Griesser, M., Wagner, G. F., Drobniak, S. M., and Ekman, J. (2017). Reproductive trade-offs in a long-lived bird species: condition-dependent reproductive allocation maintains female survival and offspring quality. J. Evol. Biol. 30, 782–795. doi: 10.1111/jeb.13046
Griffith, S. C., Mainwaring, M. C., Sorato, E., and Beckmann, C. (2016). High atmospheric temperatures and ‘ambient incubation’ drive embryonic development and lead to earlier hatching in a passerine bird. R. Soc. Open Sci. 3:150371. doi: 10.1098/rsos.150371
Grueber, C. E., Nakagawa, S., Laws, R. S., and Jamieson, I. G. (2011). Multimodal inference in ecology and evolution: challenges and solution. J. Evol. Biol. 24, 699–711. doi: 10.1111/j.1420-9101.2010.02210.x
Harrison, X. A., Blount, J. D., Inger, R., Norris, D. R., and Bearhop, S. (2011). Carry-over effects as drivers of fitness differences in animals. J. Anim. Ecol. 80, 4–18. doi: 10.1111/j.1365-2656.2010.01740.x
Harrison, X. A., Donaldson, L., Correa-cano, M. E., Evans, J., Fisher, D. N., Goodwin, C. E. D., et al. (2018). A brief introduction to mixed effects modelling and multi-model inference in ecology. PeerJ 6, 1–32.
Hatchwell, B. J. (1999). Investment strategies of breeders in avian cooperative breeding systems. Am. Nat. 154, 205–219. doi: 10.1086/303227
Herve, M. (2019). RVAideMemoire: Testing and Plotting Procedures for Biostatistics. Available online at: https://cran.r-project.org/package=RVAideMemoire (accessed March 4, 2020).
Hidalgo Aranzamendi, N., Hall, M. L., Kingma, S. A., van de Pol, M., and Peters, A. (2019). Rapid plastic breeding response to rain matches peak prey abundance in a tropical savannah bird. J. Anim. Ecol. 88, 1799–1811. doi: 10.1111/1365-2656.13068
Hsu, B.-Y., Dijkstra, C., and Groothuis, T. G. G. (2017). Organizing effects of adverse early-life condition on body mass, compensatory growth and reproduction: experimental studies in rock pigeons. J. Avian Biol. 48, 1166–1176. doi: 10.1111/jav.01172
Iknayan, K. J., and Beissinger, S. R. (2018). Collapse of a desert bird community over the past century driven by climate change. Proc. Natl. Acad. Sci. U.S.A. 115, 8597–8602. doi: 10.1073/pnas.1805123115
Jetz, W., and Rubenstein, D. R. (2011). Environmental uncertainty and the biogeography of cooperative breeding in birds. Curr. Biol. 21, 72–78. doi: 10.1016/j.cub.2010.11.075
Jury, M. R. (2013). Climate trends in southern Africa. S. Afr. J. Sci. 109, 1–11. doi: 10.1590/sajs.2013/980
Korpimäki, E. (1986). Gradients in population fluctuations of Tengmalm’s owl Aegolius funereus in Europe. Oecologia 69, 195–201. doi: 10.1007/bf00377621
Kozma, J. M., Burkett, L. M., Kroll, A. J., Thornton, J., and Mathews, N. E. (2017). Factors associated with nest survival of Black-throated Sparrows, desert-breeding nest-site generalists. J. Field Ornithol. 88, 274–287. doi: 10.1111/jofo.12209
Kruger, A. C., and Sekele, S. S. (2013). Trends in extreme temperature indices in South Africa: 1962-2009. Int. J. Climatol. 33, 661–676. doi: 10.1002/joc.3455
Langmore, N. E., Bailey, L. D., Heinsohn, R. G., Russell, A. F., and Kilner, R. M. (2016). Egg size investment in superb fairy-wrens: helper effects are modulated by climate. Proc. R. Soc. B 283:20161875. doi: 10.1098/rspb.2016.1875
Laplante, M. P., McKinnon, E. A., Love, O. P., and Vézina, F. (2019). Flexible response to short-term weather in a cold-adapted songbird. J. Avian Biol. 50:jav.01766.
Leon, A. C., and Heo, M. (2009). Sample sizes required to detect interactions between two binary fixed-effects in a mixed-effects linear regression model. Comput. Stat. Data Anal. 53, 603–608. doi: 10.1016/j.csda.2008.06.010
Lerch, B. A., Nolting, B. C., and Abbott, K. C. (2018). Why are demographic Allee effects so rarely seen in social animals? J. Anim. Ecol. 87, 1547–1559. doi: 10.1111/1365-2656.12889
Loison, A., and Langvatn, R. (1998). Short- and long-term effects of winter and spring weather on growth and survival of red deer in Norway. Oecologia 116, 489–500. doi: 10.1007/s004420050614
Lukas, D., and Clutton-Brock, T. (2017). Climate and the distribution of cooperative breeding in mammals. R. Soc. Open Sci. 4:160897. doi: 10.1098/rsos.160897
MacKellar, N., New, M., and Jack, C. (2014). Observed and modelled trends in rainfall and temperature for South Africa: 1960-2010. S. Afr. J. Sci. 110, 1–13. doi: 10.1590/sajs.2014/20130353
Mares, R., Doutrelant, C., Paquet, M., Spottiswoode, C. N., and Covas, R. (2017). Breeding decisions and output are correlated with both temperature and rainfall in an arid-region passerine, the sociable weaver. R. Soc. Open Sci. 4:170835. doi: 10.1098/rsos.170835
Marra, P. P., Cohen, E. B., Loss, S. R., Rutter, J. E., and Tonra, C. M. (2015). A call for full annual cycle research in animal ecology. Biol. Lett. 11:20150552. doi: 10.1098/rsbl.2015.0552
Marshall, H. H., Vitikainen, E. I. K., Mwanguhya, F., Businge, R., Kyabulima, S., Hares, M. C., et al. (2017). Lifetime fitness consequences of early-life ecological hardship in a wild mammal population. Ecol. Evol. 7, 1712–1724. doi: 10.1002/ece3.2747
Mayaud, J. R., Bailey, R. M., and Wiggs, G. F. S. (2017). Modelled responses of the Kalahari Desert to 21st century climate and land use change. Sci. Rep. 7, 3887–3898.
Mayer, P. M., Smith, L. M., Ford, R. G., Watterson, D. C., Mccutchen, M. D., and Ryan, M. R. (2009). Nest construction by a ground-nesting bird represents a potential trade-off between egg crypticity and thermoregulation. Oecologia 159, 893–901. doi: 10.1007/s00442-008-1266-9
McCreedy, C., and van Riper, C. (2015). Drought-caused delay in nesting of Sonoran Desert birds and its facilitation of parasite- and predator-mediated variation in reproductive success. Auk 132, 235–247. doi: 10.1642/auk-13-253.1
McKechnie, A. E. (2019). “Physiological and morphological effects of climate change,” in Effects of Climate Change on Birds, eds P. O. Dunn and A. P. Moller (Oxford: Oxford University Press), 120–133. doi: 10.1093/oso/9780198824268.003.0010
McKechnie, A. E., Hockey, P. A. R., and Wolf, B. O. (2012). Feeling the heat: Australian landbirds and climate change. Emu 112, i–vii. doi: 10.1071/muv112n2_ed
Meade, J., Nam, K. B., Beckerman, A. P., and Hatchwell, B. J. (2010). Consequences of “load-lightening” for future indirect fitness gains by helpers in a cooperatively breeding bird. J. Anim. Ecol. 79, 529–537. doi: 10.1111/j.1365-2656.2009.01656.x
Monteuil-Spencer, C. (2017). Relationships between Winter Energetic Condition and Reproductive Investment in a Wild Bird. Ph.D. thesis, Queen’s University, Ontario, Canada.
Moore, M. P., and Martin, R. A. (2019). On the evolution of carry-over effects. J. Anim. Ecol. 88, 1832–1844. doi: 10.1111/1365-2656.13081
Morrison, S. A., and Bolger, D. T. (2002). Variation in a sparrow’s reproductive success with rainfall: food and predator-mediated processes. Oecologia 133, 315–324. doi: 10.1007/s00442-002-1040-3
Mortensen, J. L., and Reed, J. M. (2018). Parental incubation patterns and the effect of group size in a Neotropical cooperative breeder. Auk Ornithol. Adv. 135, 669–692. doi: 10.1642/auk-17-236.1
Moyes, K., Coulson, T., Morgan, B. J. T., Donald, A., Morris, S. J., and Clutton-Brock, T. H. (2006). Cumulative reproduction and survival costs in female red deer. Oikos 115, 241–252. doi: 10.1111/j.2006.0030-1299.15200.x
Nater, C. R., van Benthem, K. J., Canale, C. I., Schradin, C., and Ozgul, A. (2018). Density feedbacks mediate effects of environmental change on population dynamics of a semi-desert rodent. J. Anim. Ecol. 87, 1534–1546. doi: 10.1111/1365-2656.12888
Nelson-Flower, M. J., Hockey, P. A. R., O’Ryan, C., Raihani, N. J., Du Plessis, M. A., and Ridley, A. R. (2011). Monogamous dominant pairs monopolize reproduction in the cooperatively breeding pied babbler. Behav. Ecol. 22, 559–565. doi: 10.1093/beheco/arr018
Ospina, E. A., Merrill, L., and Benson, T. J. (2018). Incubation temperature impacts nestling growth and survival in an open-cup nesting passerine. Ecol. Evol. 8, 3270–3279. doi: 10.1002/ece3.3911
Paniw, M., Maag, N., Cozzi, G., Clutton-Brock, T., and Ozgul, A. (2019). Life history responses of meerkats to seasonal changes in extreme environments. Science 363, 631–635. doi: 10.1126/science.aau5905
Pérez, J. H., Ardia, D. R., Chad, E. K., and Clotfelter, E. D. (2008). Experimental heating reveals nest temperature affects nestling condition in tree swallows (Tachycineta bicolor). Biol. Lett. 4, 468–471. doi: 10.1098/rsbl.2008.0266
Porcelli, D., Gaston, K. J., Butlin, R. K., and Snook, R. R. (2016). Local adaptation of reproductive performance during thermal stress. J. Evol. Biol. 30, 422–429. doi: 10.1111/jeb.13018
R Core Team (2017). R: A Language and Environment for Statistical Computing. Vienna: R Foundation for Statistical Computing.
Raihani, N. J., Nelson-Flower, M. J., Golabek, K. A., and Ridley, A. R. (2010). Routes to breeding in cooperatively breeding pied babblers Turdoides bicolor. J. Avian Biol. 41, 681–686. doi: 10.1111/j.1600-048x.2010.05211.x
Raihani, N. J., and Ridley, A. R. (2007). Variable fledging age according to group size: trade-offs in a cooperatively breeding bird. Biol. Lett. 3, 624–627. doi: 10.1098/rsbl.2007.0435
Rauber, R., Clutton-Brock, T. H., and Manser, M. B. (2019). Drought decreases cooperative sentinel behavior and affects vocal coordination in meerkats. Behav. Ecol. 30, 1158–1166.
Ridley, A. R. (2016). “Southern pied babblers: the dynamics of conflict and cooperation in a group-living society,” in Cooperative Breeding in Vertebrates: Studies of Ecology, Evolution, and Behavior, eds J. L. Dickinson and W. Koenig (Cambridge: Cambridge University Press), 115–132. doi: 10.1017/cbo9781107338357.008
Ridley, A. R., and Raihani, N. J. (2007). Variable postfledging care in a cooperative bird: causes and consequences. Behav. Ecol. 18, 994–1000. doi: 10.1093/beheco/arm074
Ridley, A. R., and Raihani, N. J. (2008). Task partitioning increases reproductive output in a cooperative bird. Behav. Ecol. 19, 1136–1142. doi: 10.1093/beheco/arn097
Ridley, A. R., and van den Heuvel, I. (2012). Is there a difference in reproductive performance between cooperative and non-cooperative species? A southern African comparison. Behaviour 8, 821–848. doi: 10.1163/1568539x-00003005
Rubenstein, D. R., and Lovette, I. J. (2007). Temporal environmental variability drives the evolution of cooperative breeding in birds. Curr. Biol. 17, 1414–1419. doi: 10.1016/j.cub.2007.07.032
Saino, N., Ambrosini, R., Rubolini, D., Von Hardenberg, J., Provenzale, A., Hüppop, K., et al. (2011). Climate warming, ecological mismatch at arrival and population decline in migratory birds. Proc. R. Soc. B Biol. Sci. 278, 835–842. doi: 10.1098/rspb.2010.1778
Sharpe, L., Cale, B., and Gardner, J. L. (2019). Weighing the cost: the impact of serial heatwaves on body mass in a small Australian passerine. J. Avian Biol. 50:jav.02355.
Simmonds, E. G., Sheldon, B. C., Coulson, T., and Cole, E. F. (2017). Incubation behavior adjustments, driven by ambient temperature variation, improve synchrony between hatch dates and caterpillar peak in a wild bird population. Ecol. Evol. 7, 9415–9425. doi: 10.1002/ece3.3446
Skagen, S. K., and Yackel Adams, A. A. (2012). Weather effects on avian breeding performance and implications of climate change. Ecol. Appl. 22, 1131–1145. doi: 10.1890/11-0291.1
Tokura, W., Jack, S. L., Anderson, T., and Hoffman, M. T. (2018). Long-term variability in vegetation productivity in relation to rainfall, herbivory, and fire in Tswalu Kalahari Reserve. Koedoe 60, 1–18.
Valencia, J., Mateos, C., de la Cruz, C., and Carranza, J. (2016). Maternal allocation in eggs when counting on helpers in a cooperatively breeding bird. J. Avian Biol. 48, 536–543. doi: 10.1111/jav.01020
van de Pol, M., Bailey, L. D., McLean, N., Rijsdijk, L., Lawson, C. R., and Brouwer, L. (2016). Identifying the best climatic predictors in ecology and evolution. Methods Ecol. Evol. 7, 1246–1257. doi: 10.1111/2041-210x.12590
van de Ven, T. M. F. N. (2017). Implications of Climate Change on the Reproductive Success of the Southern Yellow-Billed Hornbill Tockus leucomelas. Cape Town: University of Cape Town.
van de Ven, T. M. F. N., Fuller, A., and Clutton-Brock, T. (2020a). Effects of climate change on pup growth and survival in a cooperative mammal, the meerkat. Funct. Ecol. 34, 194–202. doi: 10.1111/1365-2435.13468
van de Ven, T. M. F. N., McKechnie, A. E., Er, S., and Cunningham, S. J. (2020b). High temperatures are associated with substantial reductions in breeding success and offspring quality in an arid-zone bird. Oecologia 193, 225–235. doi: 10.1007/s00442-020-04644-6
van Wilgen, N. J., Goodall, V., Holness, S., Chown, S. L., and McGeoch, M. A. (2016). Rising temperatures and changing rainfall patterns in South Africa’s national parks. Int. J. Climatol. 36, 706–721. doi: 10.1002/joc.4377
Wiley, E. M. (2017). Examining How Multilevel Population Dynamics and Climate Influence Breeding Behaviour, Within-Group Stability and Demography in a Cooperatively Breeding Bird. Ph.D. thesis, The University of Western Australia, Crawley.
Wiley, E. M., and Ridley, A. R. (2016). The effects of temperature on offspring provisioning in a cooperative breeder. Anim. Behav. 117, 187–195. doi: 10.1016/j.anbehav.2016.05.009
Wiley, E. M., and Ridley, A. R. (2018). The benefits of pair bond tenure in the cooperatively breeding pied babbler (Turdoides bicolor). Ecol. Evol. 8, 7178–7185. doi: 10.1002/ece3.4243
Wingfield, J. C., Pérez, J. H., Krause, J. S., Word, K. R., González-Gómez, P. L., Lisovski, S., et al. (2017). How birds cope physiologically and behaviourally with extreme climatic events. Philos. Trans. R. Soc. B Biol. Sci. 372:20160140. doi: 10.1098/rstb.2016.0140
Wise, D. H., and Lensing, J. R. (2019). Impacts of rainfall extremes predicted by climate-change models on major trophic groups in the leaf litter arthropod community. J. Anim. Ecol. 88, 1486–1497. doi: 10.1111/1365-2656.13046
Woodworth, B. K., Wheelwright, N. T., Newman, A. E., Schaub, M., and Norris, D. R. (2017). Winter temperatures limit population growth rate of a migratory songbird. Nat. Commun. 8:14812.
Keywords: climate change, compensatory breeding, cooperative breeding, drought, environmental change, southern pied babbler, weather
Citation: Bourne AR, Cunningham SJ, Spottiswoode CN and Ridley AR (2020) Compensatory Breeding in Years Following Drought in a Desert-Dwelling Cooperative Breeder. Front. Ecol. Evol. 8:190. doi: 10.3389/fevo.2020.00190
Received: 09 March 2020; Accepted: 27 May 2020;
Published: 30 June 2020.
Edited by:
Stuart Peter Sharp, Lancaster University, United KingdomReviewed by:
Michael Griesser, University of Zurich, SwitzerlandDustin R. Rubenstein, Columbia University, United States
Copyright © 2020 Bourne, Cunningham, Spottiswoode and Ridley. This is an open-access article distributed under the terms of the Creative Commons Attribution License (CC BY). The use, distribution or reproduction in other forums is permitted, provided the original author(s) and the copyright owner(s) are credited and that the original publication in this journal is cited, in accordance with accepted academic practice. No use, distribution or reproduction is permitted which does not comply with these terms.
*Correspondence: Amanda R. Bourne, YWJvdXJuZS51Y3RAZ21haWwuY29t