- 1Caribou Ungava, Département de Biologie, Université Laval, Québec, QC, Canada
- 2Département de Biologie, Centre d’Études Nordiques, Université Laval, Québec, QC, Canada
- 3Ministère des Forêts, de la Faune et des Parcs, Direction de l’Expertise sur la Faune Terrestre, l’Herpétofaune et l’Avifaune, Québec, QC, Canada
- 4ONCFS – Unité Ongulés Sauvages, Gières, France
Human disturbances are rapidly increasing in northern and Arctic regions, raising concerns about the recovery and persistence of declining caribou (Rangifer tarandus) populations. Yet, the consequences of behavioral responses toward human disturbances on vital rates rarely have been investigated. Herein, we assessed the cumulative and instantaneous effects of human disturbances (roads, human settlements, mines and mining exploration) at different temporal scales on the mortality risk of 254 GPS- collared migratory caribou monitored in two herds, the Rivière-aux-Feuilles (RFH) and Rivière-George (RGH) herds, in northern Québec and Labrador, Canada. We also assessed the relative importance of human disturbances on caribou mortality risk compared with non-anthropogenic factors, including habitat use by caribou, predation risk by gray wolves (Canis lupus), and local weather conditions. Human disturbances alone, exclusive of hunting, had a limited impact on mortality risk of caribou. Repeated exposure to disturbances did not have detectable effects on mortality risk during the early life period (1−7 years old), but more abundant precipitation (RFH) or the use of areas with a higher predation risk (RGH) did so. At the seasonal scale, non-anthropogenic factors, particularly the use of highly selected habitat by caribou and air temperature, had a greater effect than anthropogenic factors on the mortality risk in the RFH. Caribou of the RFH using more frequently higlhy selected habitats decreased their chance of mortality during winter, whereas individuals using warmer areas during summer faced a higher risk of mortality. At the daily scale, we observed that anthropogenic and non-anthropogenic factors generally had either no effect on the daily risk of mortality, or their effects were undistinguishable from the effect of latitude, with which they were highly correlated. The only exception was for the RFH in winter, for which the daily risk of mortality increased 10 folds for each 10-km increment closer to industrial disturbances. Although the impacts of human disturbances on caribou survival were limited to specific regions and areas, we nevertheless detected a negative effect on survival on the RFH, even at the currently low level of human development. Our study highlights the importance of assessing effects of human disturbances at various spatiotemporal scales, and of considering the relative influence of other non-anthropogenic factors to fully understand drives of wildlife populations.
Introduction
In the last decades, the human footprint has increased rapidly, including >70% of ecoregions around the world (Venter et al., 2016). The increased human pressure on ecosystems has been identified as the main cause of species extinction and biodiversity loss (Brooks et al., 2002; Davies et al., 2006; Fahrig and Rytwinski, 2009). Effects of human disturbances on wildlife vary from short-term modifications in behavior, activity budgets and nutrition (Dussault et al., 2007; Benhaiem et al., 2008; Marchand et al., 2014), to processes occurring at larger spatiotemporal scales, such as resources and range use (Moreau et al., 2012), distribution (Shackelford et al., 2018), population dynamics (Sorensen et al., 2008), and species interactions (Courbin et al., 2009).
Quantifying impacts of human disturbances on vital rates of wildlife is of particular interest in ecology because it can help determine if and how individual responses to disturbances translate into consequences at the population level. Human disturbances can directly effect survival, including mortality caused by collision with vehicles (Forman and Alexander, 1998; Lodé, 2000) or when species vulnerability to hunting increases because of human development (Lebel et al., 2012; Plante et al., 2017). Most effects of disturbance, however, are not directly lethal for wildlife, but include energetic and lost-opportunity costs (Frid and Dill, 2002). These costs can accumulate over time and space, and ultimately translate into long-term consequences on individual performance and vital rates (Bradshaw et al., 1998; Johnson and St-Laurent, 2011). Over time, the consequences of these indirect effects on population dynamics and trophic interactions can exceed those from direct sources of mortality (Creel and Christianson, 2008). Nevertheless, many studies still focus on single development projects and short-term behavioral responses toward disturbances, without further investigating the potential cumulative effects over broad spatiotemporal scales, and perhaps more importantly, without considering the consequences on vital rates (Weladji and Forbes, 2002; Tablado and Jenni, 2017).
Multiple natural factors may also act simultaneously with human disturbances to further effect vital rates in wildlife populations (Yamasaki et al., 2008; Tablado and Jenni, 2017; Ramey et al., 2018). Consequently, the study of relationships between habitat use and animal performance is an effective tool to assess the relative importance of multiple limiting factors acting simultaneously (Gaillard et al., 2010; DeCesare et al., 2014; Uboni et al., 2017). Patterns of habitat use often are assumed to represent an aggregated response to multiple factors acting at different spatiotemporal scales, and should reflect priorities or trade-offs among competing needs (Senft et al., 1987; Rettie and Messier, 2000). Linking habitat use to vital rates may thus help to reveal the relative importance of multiple natural and anthropogenic factors for wildlife populations (McLoughlin et al., 2010).
Animals may not be able to minimize the effect of one or multiple limiting factors without compromising their performance to face other factors. In risky environments for example, individuals can fail to reduce predation risk sufficiently through habitat use (DeCesare et al., 2014). Alteration of the environment also can create discrepancies between perceived and true habitat suitability (Schlaepfer et al., 2002). If poor or risky habitats are used or become attractive to individuals despite reduced quality, those habitats become ecological traps where individuals are attracted despite their reduced chance of survival (Battin, 2004). Such non-ideal or maladaptive behaviors have mostly been reported in rapidly changing environments (e.g., human-altered landscapes) where modifications of the habitat occur at a much faster rate than the potential adaptation rate of animals (Battin, 2004; Robertson and Hutto, 2006).
In past decades, northern and Arctic regions have witnessed a drastic increase in human disturbances, and serious concerns have been raised regarding the ability of individuals to adapt, or populations to persist in these newly modified landscapes (United Nation Environmental Programme [UNEP], 2001). Caribou and reindeer (Rangifer tarandus; hereafter caribou) are at the very heart of these considerations because they represent a key species both ecologically and culturally (Bergerud et al., 2008). Although caribou populations have fluctuated in the past, the current decline of most caribou populations worldwide indicates that large-scale modifications induced by the development of human activities may contribute to this situation (Vors and Boyce, 2009; Festa-Bianchet et al., 2011). Caribou could be particularly sensitive to human disturbances because they occupy broad ranges and have a limited intrinsic capacity of population growth because of their low productivity (Cardillo et al., 2005; Fahrig and Rytwinski, 2009). Numerous studies have also reported strong behavioral responses of caribou toward human disturbances (Vistnes and Nellemann, 2008), such as the avoidance of infrastructures over several kilometers (e.g., Boulanger et al., 2012; Johnson and Russell, 2014). Until recently, human development has been relatively limited in the ranges of the migratory tundra caribou compared with what has been experienced by boreal caribou populations. Yet, very few studies have assessed effects of human disturbance on vital rates of migratory caribou (i.e., reproductive rate only; Nellemann et al., 2003; Cameron et al., 2005). As human development continues in the north, it is crucial to assess the impacts of human disturbances on the vital rates and demography of migratory tundra caribou before levels of development occur that would impede population persistence and recovery (Festa-Bianchet et al., 2011).
To assess effects of human disturbances on the survival of wide-ranging animals such as migratory caribou, we need to consider natural limiting factors acting independently or simultaneously with human disturbances. Climate, predation, parasites, insect harassment, and diseases are natural factors known to reduce survival that may also ultimately contribute to the decline of caribou populations (Festa-Bianchet et al., 2011; Mallory and Boyce, 2018). Although warmer temperatures during summer have been positively associated with the growth of some caribou herds (Mallory and Boyce, 2018), those temperatures also can increase insect harassment, parasite load, and heat stress (Soppela et al., 1986; Weladji et al., 2003), all entailing physiological costs and energy expenditures that deteriorate body condition (Toupin et al., 1996; Pachkowski et al., 2013). Warmer temperatures can advance melt-down and delay freeze-up of water bodies, which could dramatically increase movement costs for caribou (Leblond et al., 2016), or lead to death when caribou try to cross over thin ice (Poole et al., 2010). Warmer temperatures during winter can also increase the frequency and intensity of rain-on-snow events, which limit access to food resources and may lead to major mortality events (Forbes et al., 2016; Berger et al., 2018). Climate warming is also increasing the frequency and intensity of fires in the tundra and boreal forest, thus shrinking the available habitat for caribou (Rupp et al., 2006; Joly et al., 2011; Skatter et al., 2017).
Although caribou have cohabited with natural predators for centuries and have evolved efficient antipredator tactics (e.g., long-distance migrations; Seip, 1991; Bergerud et al., 2008), human disturbances could modify predator-prey relationships and further increase the impact of predation (Latham et al., 2011; Dussault et al., 2012; Newton et al., 2017; DeMars and Boutin, 2018). For example, linear infrastructures can facilitate gray wolf (Canis lupus) movements and access to certain areas of caribou ranges, which further increases effects of predation on population dynamics (James and Stuart-Smith, 2000; DeMars and Boutin, 2018).
Our overarching hypothesis was that effects of human disturbances on the mortality risk of eastern migratory caribou as well as the relative importance of such disturbances compared with natural factors known to affect survival, including habitat use by caribou, predation risk by wolves and local weather conditions (i.e air temperature and precipitation) were driving caribou abundance. We focused on two caribou herds located in northern Québec and Labrador, Canada, the Rivière-aux-Feuilles (RFH) and the Rivière-George herds (RGH), between 2009 and 2016. Over the last decades, these two herds, which are part of the eastern migratory caribou designatable unit, have declined to a point where they have been recommended to be listed as Endangered in COSEWIC (2017). Simultaneously with these declines, the region has experienced a moderate increase in human activity, owing mainly to an expansion of the mining (MRNF, 2012). Previous research showed that caribou of these herds avoided human disturbances over distances up to 23 km, which can cause substantial habitat loss within their range (Plante et al., 2018). Despite this avoidance, caribou remain more vulnerable to harvest near human infrastructures (Plante et al., 2017). Thus, there is a need to better understand and quantify the interactions between human disturbances and the mortality risk of caribou.
We assessed the cumulative and instantaneous effects of human disturbances on mortality risk in the two caribou herds by considering three temporal scales: early life, seasonal, and daily scales. We first evaluated whether repeated exposure to disturbances increased mortality risk during the early period of caribou life (1−7 years old) and at the seasonal scale (winter and summer). We also evaluated whether daily exposure to disturbances increased daily mortality risk of individuals. Secondly, we used a conceptual framework inspired by DeCesare et al. (2014) to compare the relative importance of human disturbances and non-anthropogenic factors on mortality risk of caribou. We first considered a habitat use hypothesis, where mortality risk of caribou is mostly affected by the proportion of time spent in highly selected habitats (H1; Figure 1A). We expected that caribou spending less time in habitats that are strongly selected at the population level would face a higher risk of mortality because of the potentially lower quality of these habitats or to the marginality of their behavior (e.g., bolder personalities), which could increase predation risk (Lesmerises et al., 2019). Alternatively, the use of highly selected habitats could be a “non-ideal” behavior, being inefficient at reducing the mortality risk associated with one or more factors. We thus considered the two alternative hypotheses where, regardless of the use of highly selected habitats, most variation in the mortality risk would be explained by exposure to anthropogenic or other non-anthropogenic factors. Accordingly, we considered the disturbance risk and predation risk hypotheses, where individuals more exposed to disturbances (H2; Figure 1B), or predation risk (H3; Figure 1C), respectively, have a higher mortality risk. We also considered a weather hypothesis, where adverse temperature and precipitation conditions encountered by individuals during winter (e.g., heavy snow) and summer (e.g., warm temperature) increase mortality risk (H4; Figure 1D). We also tested the hypothesis of additive effects of habitat use and other anthropogenic or natural factors on survival (H5; Figure 1E). Finally, we considered the hypothesis of maladaptive habitat use (Battin, 2004; Robertson and Hutto, 2006), where spending more time in highly selected habitats could increase exposure to disturbances or predation risk and consequently reduce survival (H6; Figure 1F).
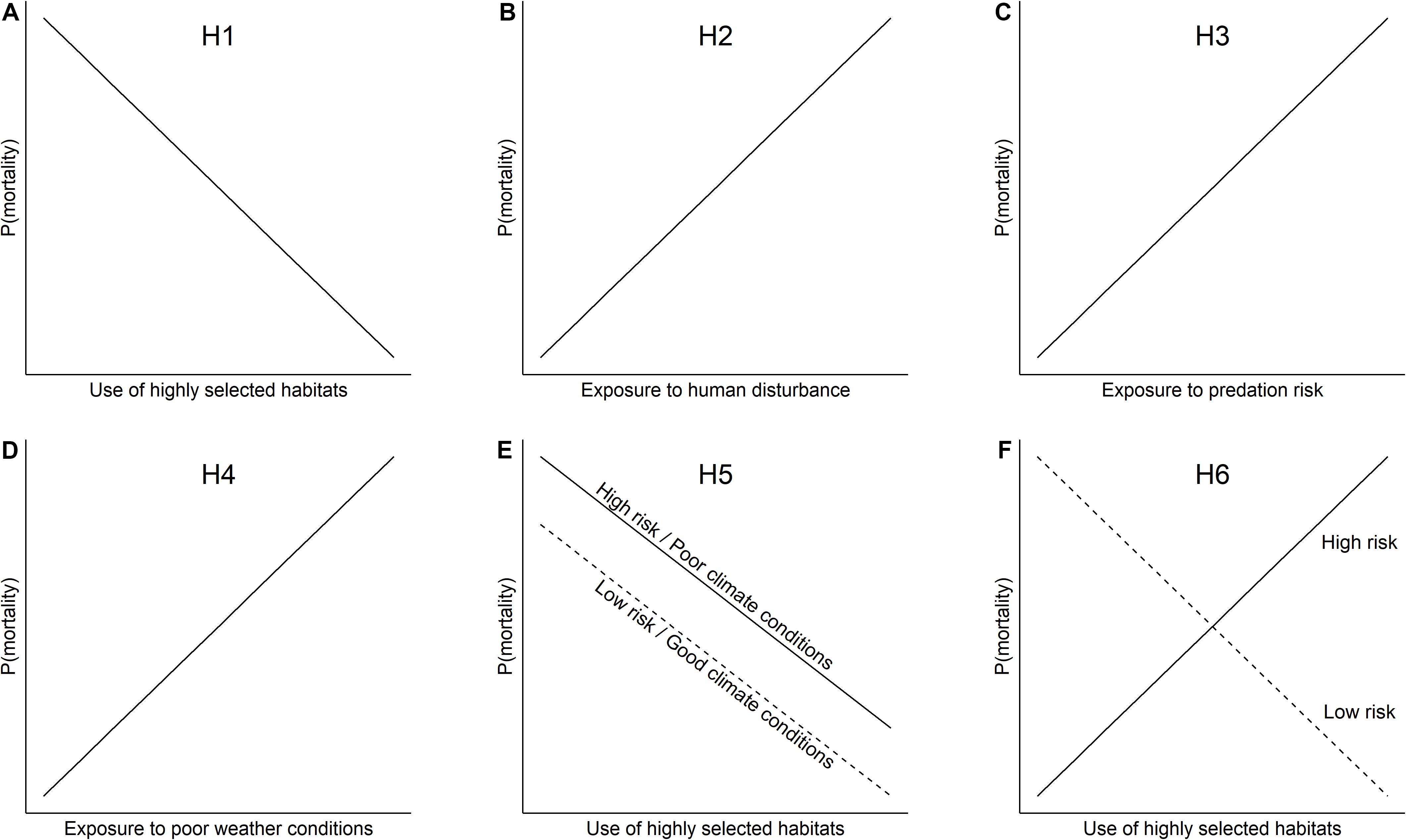
Figure 1. Hypotheses and predictions tested to explain variations in the risk of mortality of eastern migratory caribou of the Rivière-aux-Feuilles (RFH) and Rivière-George herds (RGH), northern Québec and Labrador, Canada (2009–2016). Competing hypotheses H1 through H6 (as given in the text) included (A) the use of highly selected habitats (% of individual locations in highly selected habitats), (B) exposure to disturbance risk, (C) exposure to predation risk, (D) exposure to poor weather conditions, (E) additive effects, and (F) maladaptive habitat use.
Materials and Methods
Study Area
The RFH and RGH have both experienced steep declines in the last decades. The RFH has peaked at >500,000 individuals around 2001 (Couturier et al., 2004), and decreased to an estimated population size of 199,000 (CI 90% = 183,080−214,920) in Taillon et al. (2016). The RGH has experienced a more drastic decline, with an estimated population peak at 823,375 (CI 90% = 721,375−925,375) individuals around 1993 (Couturier et al., 2004), 74,000 (CI 90%=60,680-87,320) in 2010, and an estimated population of <8,900 individuals (CI 90% = 8,232−9,568) in MFFP and NLFLR (2016, unpubl. data). Numerous factors are suspected to have contributed to these declines, including resource limitation and density-dependent effects, climate, hunting and predation (Côté et al., 2010).
The RFH and RGH range over more than 1,000,000 km2 north of the 51st parallel in northern Québec and Labrador, mostly within the Nunavik and Nunatsiavut regions of Canada (Figure 2). The area is characterized by subarctic and arctic climates, with short and cool summers (1981−2010; 9.7°C on average for the warmest trimesters) followed by long and cold winters (−19.5°C on average for the coldest trimesters; Berteaux et al., 2018). Precipitation averages 898 mm⋅year–1, mostly falling as snow between October and March. The RFH and RGH ranges are characterized by numerous lakes and rolling hills with more rugged terrain in the northeast portion of the RGH range.
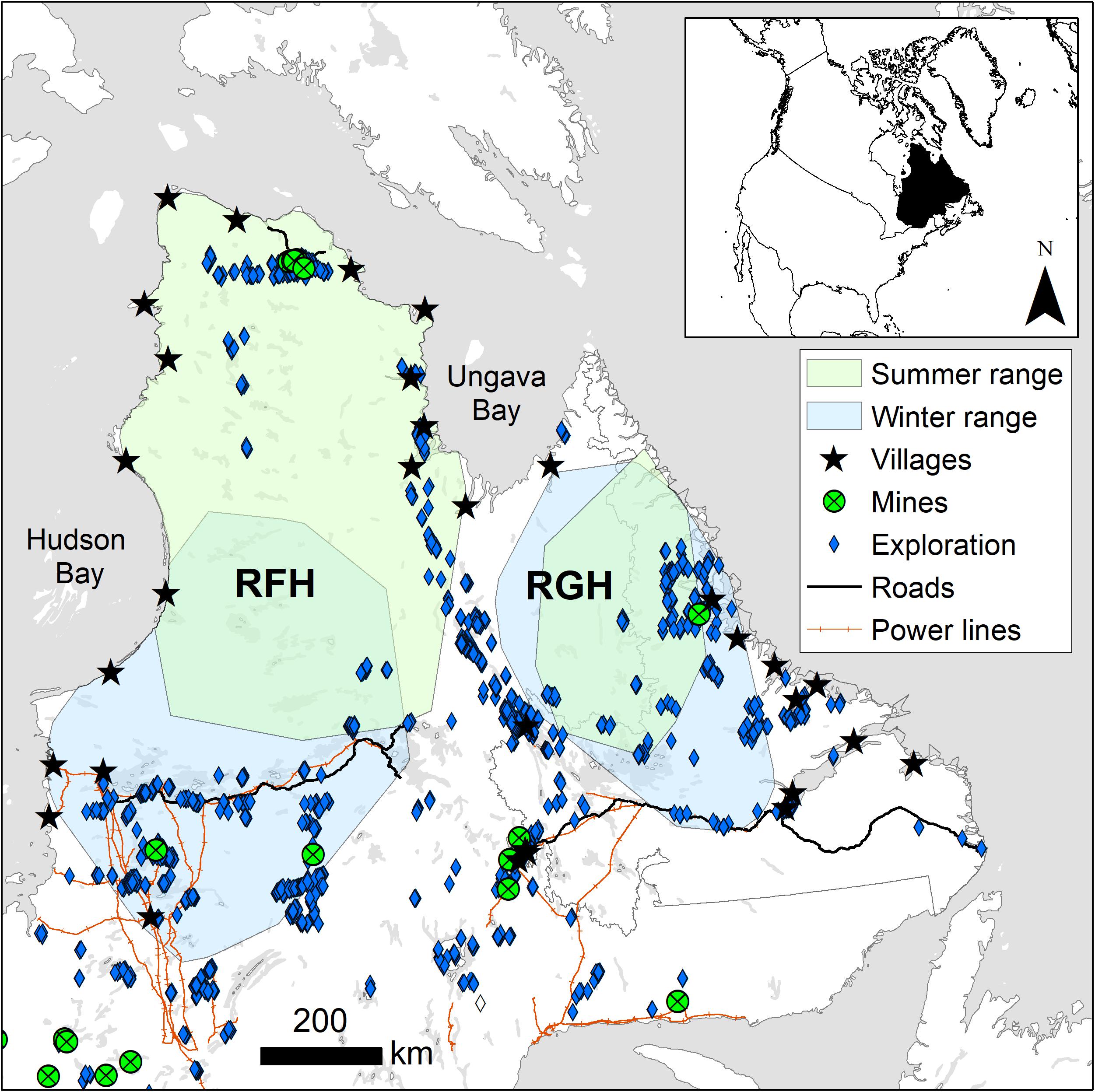
Figure 2. Winter and summer ranges of eastern migratory caribou of the Rivière-aux-Feuilles (RFH) and Rivière-George herds (RGH) and distribution of human disturbances in northern Québec and Labrador, Canada (2009–2016).
In April, caribou of the RFH undertake a northward migration from their winter range, located in the boreal-taiga forests, to their calving ground and summer range, in the Arctic tundra (mean distance [2000−2011] = 615 km; Le Corre et al., 2017). Vegetation on the RFH summer range is mainly composed of shrubs (Betula sp. and Salix sp.), grasses, herbaceous plants and lichens (Latifovic et al., 2017). In autumn, the RFH migrates south and returns to its winter range in the taiga forest, which is dominated by black spruce (Picea mariana) and tamarack (Larix larcina) interspersed with lakes and open areas covered by shrubs and lichens. Caribou of the RGH used to undertake similar but shorter migrations between summer and winter ranges (mean distance from 2000 to2011 = 350 km; Le Corre et al., 2017). Migration paths have continued to shorten in recent years (Caribou Ungava, unpublished results), resulting in great overlap between summer and winter ranges for the 2009−2016 period. Summer range was composed mainly of grasses and shrubs, with conifer forest in the southern portion and in valleys. Winter range had a similar composition, but with a higher proportion of conifer forest because the range extended further south of the tree line.
Caribou of the RFH and RGH cohabit with two large predators: the gray wolf and the black bear (Ursus americanus). Wolves are known to predate both adults and calves (Bergerud et al., 2008), whereas black bears mainly predate calves opportunistically (Zager and Beecham, 2006; Dussault et al., 2012). Other large ungulates such as moose (Alces alces) and muskox (Ovibos moschatus) occur at low densities in the area. Caribou of the RFH and RGH are the main large prey available year-round for wolves on these ranges (M. Bonin, unpublished results).
Humans represent both a direct and an indirect risk of mortality for caribou of the RFH and RGH. Caribou are an important subsistence food for northern communities (Bergerud et al., 2008). On the RGH, sport hunting was discontinued in 2012, and ban on all hunting in Labrador was enacted in 2013. Despite this, subsistence harvesting continued through the remainder of the study period. Hunting is known to have been a predominant source of mortality for the RGH through the study period and to the present (Department of Fisheries and Land Resources, Newfoundland, unpubl. data). On the RFH, autumn and winter sport hunting and subsistence harvest occurred throughout the study period. Indirectly, human disturbances could affect caribou survival through habitat loss and cumulative costs associated with the behavioral and physiological responses of avoiding infrastructures. Human disturbances include human settlements, mostly located along the coast, mining operations and exploration (mostly drilling sites), three major roads stretching outside settlements, and hydroelectric infrastructures (power lines, reservoirs and dams; Figure 2). Winter ranges are more disturbed than summer ranges, but human disturbances remain at relatively low density across the area (Table 1).
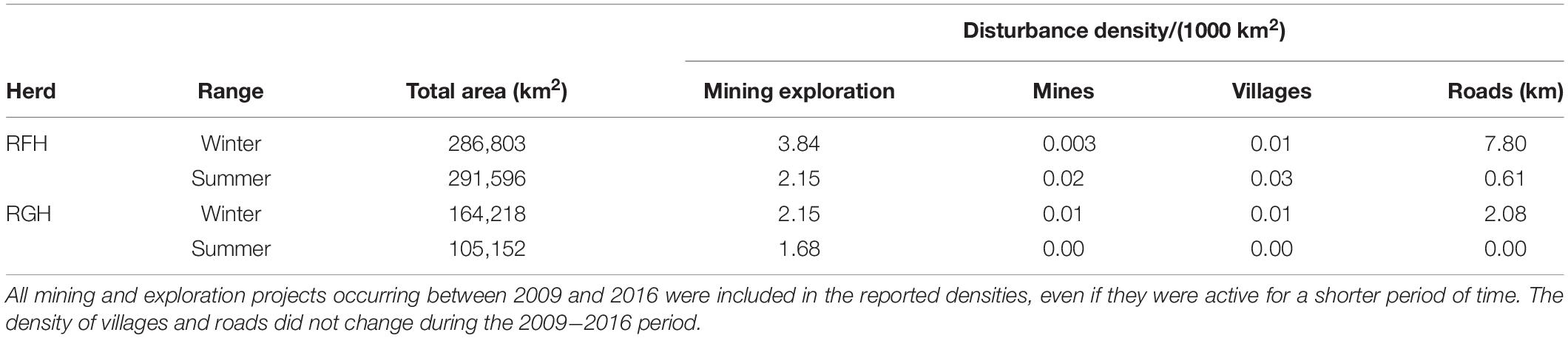
Table 1. Total area (km2) of caribou seasonal ranges and density of human disturbances (per 1000 km2) in the ranges of the Rivière-aux-Feuilles (RFH) and Rivière-George (RGH) herds in northern Québec and Labrador, Canada, 2009−2016.
Capture and Monitoring of Caribou
We focused on the mortality risk of adult female caribou because population dynamics are highly sensitive to small variation in the survival of adult females (Gaillard et al., 2000). Between 2009 and 2016, we captured 254 yearling and adult females (RFH = 119; RGH = 135) using a net gun fired from a helicopter, and we equipped them with GPS collars (GPS Iridium and Globalstar, Vectronic Aerospace GmbH) programmed to record locations every 1 to 13 h (MFFP, NLFLR and Caribou Ungava, unpubl. data). We determined environmental characteristics used by caribou and the survival status of individuals (alive or dead) using the information provided by GPS collars. We considered individuals as dead when we received a mortality signal or, in cases where mortality sensors failed, when caribou stopped moving permanently. In many instances (one-half of the individuals; Table 2), the cause of death was difficult to determine because we could visit carcasses only several months after death. We thus pooled all mortality causes in analyses.
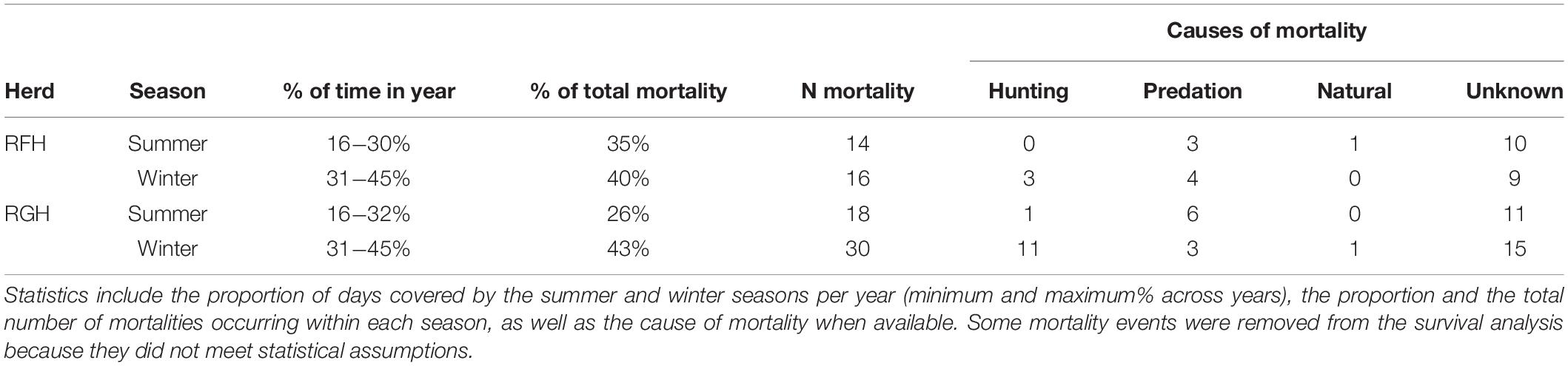
Table 2. Descriptive statistics of migratory caribou mortalities during summer and winter of 2009−2016 for the Rivière-aux-Feuilles (RFH) and Rivière-George (RGH) herds in northern Québec and Labrador, Canada.
Human Disturbance Effects on Mortality Risk
We first assessed the effects of human disturbances on caribou mortality risk at three temporal scales: early life (1−7 years old), seasonal, and daily scales. This allowed us to distinguish cumulative effects of disturbances (effects detectable over a season or a critical part of the lifetime) from instantaneous effects (effects observed within a day). We defined human disturbances as infrastructures and their potential associated human presence or activity. We had no information on the nature or level of activity associated with each infrastructure, but assumed that the effects of infrastructures on caribou behavior include the human activity occurring at, or around, infrastructures. For all scales of analysis, we quantified caribou exposure to a combination of all types of disturbances, which included mines, mining exploration, villages and roads. We excluded hydroelectric infrastructures because they were located at the southern limit of winter ranges of both herds. Caribou exposure to this type of disturbance was thus limited and previous research indicated a limited impact of this disturbance on caribou behavior (Plante et al., 2018). We also quantified exposure to industrial (mines and mining exploration) and non-industrial disturbances (villages and roads) separately. We expected non-industrial disturbances to have both instantaneous (e.g., higher risk of harvest near villages and roads) and cumulative effects on survival (e.g., stress and avoidance costs), whereas we mainly expected cumulative effects for industrial disturbances, because there is no harvest near exploration and mining sites. We restricted our assessment to disturbances located within 100 km of caribou locations. We considered caribou as exposed to disturbance within this radius because it included the largest zones of influence of disturbances reported for these herds (max = 23 km; Plante et al., 2018), and it encompassed the area where the risk of harvest by sport and subsistence hunters was the highest (90% of harvest occurred within <100 km of an infrastructure).
Early Life Risk of Mortality
We first quantified the effect of repeated exposure to human disturbances on the mortality risk during the early life of caribou (1−7 years old). We wanted to evaluate the possibility that the costs of repeated exposure to disturbance over the first 1−7 years-of- life could accumulate over time to a point where it would impact survival. We suspected that, if disturbance effects on survival were mostly observed through cumulative effects over time, smaller scales (one season or day) would not be enough to detect such effects. We modeled early life mortality risk of 112 female caribou (RFH = 62; RGH = 50) captured as yearling and monitored continuously until their death or the end of the study. We limited our analysis to caribou captured as yearling to avoid uncertainty in age estimation (aging for individuals captured at >1 year was based on tooth wear). Among this sub-sample, 40 caribou died during the study (RFH = 17; RGH = 23). The age at death was calculated as the difference in days between estimated birth date (set at June 15th the year before capture for all individuals; Taillon et al., 2016) and date of death. Because our study extended from 2009 to 2016, individuals that survived throughout the study period reached a maximum of 7 years old. This is near the upper limit of the prime-age age class for most large herbivores and explains why we considered our study to target the early life of migratory caribou (Gaillard et al., 2000). We quantified early life exposure to disturbances by computing the proportion of locations within 100 km of a disturbance during the complete monitoring period of each individual. We tested effects of human exposure on early life rate of mortality using Cox proportional hazard models (function coxph in Survival package, R 3.5.1 Software, R Core Team, 2018). We verified the assumption of proportional hazard over time (function cox.zph), and excluded models for which the assumption was violated. We assessed the mortality risk using the hazard ratio (HR; exponential of β), that is the ratio between hazard rates of one unit of the factors tested. As such, mortality risk increased when HR > 1, decreased when HR < 1 and was not significantly influenced by the tested variable when the 95% CI included 1. We quantified the proportion of variance in mortality risk explained by human disturbances using the Nagelkerke’s adjusted R2 (function r.squaredLR in package MuMIn), and we verified model performance compared with the null model using a likelihood ratio test (in coxph output; Hosmer and Lemeshow, 2000).
Seasonal Mortality Risk
At the seasonal scale, we compared exposure to human disturbances for individuals that survived throughout our study, and individuals that died within a given season. We focused on summer and winter seasons because most mortalities occurred during these seasons and mortality events were too rare in other biological seasons to perform the survival analysis (Table 2). Summer and winter seasons were temporally delineated using First Passage Times that identify variations in caribou movements between seasons (Le Corre et al., 2014). We used the mean date of initiation and completion of migrations for all individuals to define each season on a yearly basis. Depending on the year, summer season started between mid-June and mid-July (RFH: 30 June – 10 July; RGH: 19 June – 8 July) and ended between the end of August and mid-October (RFH: 31 August– 20 October). Winter season started between mid-November and the end of December (RFH: 20 November – 11 December; RGH: 9 November – 31 December) and ended between the end of March and the beginning of May (RFH: 27 March – 27 April; RGH: 9 April – 5 May).
We used 465 individual-seasons to model summer mortality risk (RFH = 233 with 14 mortalities; RGH = 232 with 18 mortalities), and 329 individual-seasons to model winter mortality risk (RFH = 147 with 14 mortalities; RGH = 1 82 with 25 mortalities). We quantified repeated exposure of caribou to disturbances by calculating the proportion of seasonal locations within a 100-km radius of any disturbances, and types of industrial and non-industrial disturbances separately. We statistically tested the relationship between exposure to disturbances and mortality risk using mixed-effects Cox proportional hazard models (coxme, package Survival in R). We set the year as a random effect to account for the annual variations in exposure to disturbances, in the number of active industrial activities, and in the length of summer or winter seasons across years (Table 2). We determined the proportion of variance explained by disturbances and the performance of the model compared with a null model using the same approach as for the early life scale.
Daily Mortality Risk
As for the seasonal analysis, we focused the daily survival analysis on mortalities occurring during summer and winter. We used 858 individual-days during summer (RFH = 5 57 with 14 mortalities; RGH = 301 with 18 mortalities), and 636 individual-days during winter (RFH = 394 with 14 mortalities; RGH = 2 42 with 25 mortalities). We assessed the effect of human disturbances on daily mortality risk by comparing distance to disturbances of individuals that would eventually die and of individuals that survived during the 24 h before the death occurred. We created statistical clusters, which included the locations of an individual during the 24 h before it died and the locations of all caribou that were alive during the same period. For each caribou location, we calculated the Euclidian distance to the nearest disturbance of any type, and to the nearest industrial and non-industrial disturbances. We truncated distance values at 100 km, and averaged them over the 24-h period. To facilitate the interpretation of the coefficients associated with daily risk of mortality and make that value comparable to the analyses performed at other scales, we multiplied distance values by −1. By doing so, higher coefficients represented greater exposure to disturbances, as for the other scales. We used conditional logistic regressions (clogit, package Survival in R). We could not assess model performance compared to the null model using a likelihood ratio test because the calculation of R2 was not possible for this type of model.
Relative Importance of Human Disturbances and Natural Factors
Use of Highly Selected Habitats by Caribou
We compared the relative impact of human disturbances on caribou mortality to that of natural factors at the three temporal scales of analysis. Following our hypotheses, we evaluated the role of habitat use by caribou on mortality risk (H1, Figure 1A). The observed patterns of habitat use were considered as aggregate responses of caribou toward multiple factors. These responses were described using Resource Selection Functions (RSFs; Manly et al., 2002) on 334 female caribou (RFH = 168; RGH = 166). The RSF was performed at the herd level, for each season separately (see Supplementary Appendix 1 for details). The RSF included 5 to 6 vegetation cover types, the Normalized Difference Vegetation Index (NDVI; 250 m 16-day composite VI from the MODIS MOD13Q1 product; Didan, 2015) as an index of vegetation productivity, the elevation and the proximity to water. We assessed the predictive performance of RSF models with a k-fold cross-validation (Boyce et al., 2002). When predictive performance was good (rspearman > 0.70), we used the selection coefficients to predict the relative probability of occurrence of caribou on seasonal ranges.
We then described the individual patterns of use of highly selected habitats relative to the population RSF to assess how individual variations affected mortality. We first extracted the RSF score of the population model under each caribou location. We then defined highly selected habitats as the highest 25% of RSF scores among those for caribou locations. For the early life and seasonal scales, we quantified the use of highly selected habitats by calculating the proportion of caribou locations in these habitats. For the daily scale, we directly compared the RSF score at caribou locations for individuals that died and individuals that survived during the 24 h preceding death.
Predation Risk by Wolves
We determined whether caribou more exposed to predation risk by wolves faced an increased risk of mortality (H3; Figure 1C). The predation risk was defined based on RSF models of 42 wolves equipped with GPS collars in the caribou seasonal ranges (RFH = 28; RGH = 14; see Supplementary Appendix 1 for details). We included the same variables we used for caribou RSFs, i.e., vegetation cover type, NDVI, elevation and proximity to water in the wolf RSF models. We also used the same seasonal areas and periods for describing habitat selection by wolves that we used for caribou. We avoided including indices of caribou use in habitat selection-models for wolves because we aimed at identifying habitat characteristics associated with higher wolf occurrence, independently of caribou use. We extracted the relative probability of wolf occurrence at each caribou location and we defined risky habitats as those having a RSF score ≥ 75th percentiles among RSF scores occurring at caribou locations. We calculated the proportion of locations of each caribou in risky habitats when assessing the effect on early life and seasonal mortality risk, and used RSF scores of wolves to make predictions at caribou locations when assessing daily mortality risk.
Weather Conditions
For weather conditions (H4; Figure 1D), we extracted the mean daily temperature (°C) and total daily amount of precipitation (kg/m2) at each caribou location (National Oceanic and Atmospheric Administration [NOAA], 2017 climatic data; 35 km × 35 km-resolution). To ensure that weather conditions were comparable among individuals. even if they had different monitoring periods, we calculated the daily differences between temperatures and precipitations at locations of an individual and the mean values of these variables for all other individuals monitored during the same day. Therefore, we used weather conditions indices that contrasted the conditions faced by an individual compared to average conditions faced by all monitored individuals during a given day. We chose to model differences in the meteorological conditions encountered by individuals versus the mean value instead of actual local values because caribou in both herds spread over a large area within a given season, resulting in large differences in conditions encountered by individuals. This approach allowed us to test the effect of encountered conditions on survival, while keeping a certain amount of variability among individuals. It also limited the effect of extreme values on survival output, i.e., when individuals were far from the main groups.
Finally, we tested the hypotheses of additive effects of habitat use and human disturbances, predation or weather (H5; Figure 1E) by including different combinations of these factors in the models. We also tested the hypothesis of maladaptive habitat use in regards to human disturbances or predation risk (H6; Figure 1F) by including the interaction between the use of highly selected habitats and caribou exposure to human disturbances or predation risk by wolves in other candidate models.
Model Selection
We used the Akaike Information Criterion (AIC; Burnham and Anderson, 2002) to compare the relative fit of the models derived from our hypotheses. For nested models with ΔAIC ≤ 2, we retained the model with fewer variables because additional covariates were considered uninformative (Arnold, 2010). Otherwise, we interpreted all non-nested models with ΔAIC ≤ 2. We also compared our candidate models to a null model to verify that covariates included in models improved model fit (Mac Nally et al., 2018). We relied on the likelihood ratio test to assess the performance of best models compared with null models. At the daily scale, we could not test models including only the intercept with conditional logistic functions. We thus compared model performance to a model including only the latitude as a covariate (see next section for details). We verified multicollinearity among variables included in the same model with the variance inflation factor (VIF). We assumed no multicollinearity when VIF scores were <5 (Zuur et al., 2010).
Correlations and Confounding Effects
We also examined the correlations between all variables considered in the candidate models using Pearson’s paired tests of correlations (cor.test in R, Supplementary Appendix 2). This was done prior to the modeling step for two reasons. First, some factors could not be tested in the same model because of over-parametrization issues due to the low number of mortalities (King and Zeng, 2001). Thus, the collinearity of these variables could not be assessed with multicollinearity tests. Yet, their correlation would indicate some redundancy among factors used to explain variation in mortality risk of caribou, potentially affecting the interpretation of results. Variables that were weakly correlated (<0.50) were tested in the same set of candidate models. When correlation was >0.50, we retained the variable providing the best fit according to the model selection process. Secondly, we suspected that exposure of caribou to human disturbances and predation risk, as well as the effect of weather conditions on survival, could vary substantially with latitude. Caribou are highly mobile, even outside migration periods, which could make exposure to anthropogenic and non-anthropogenic factors highly variable across individuals, or over time, even within a single season. For example, during summer caribou of the RFH were more exposed to disturbances when they reached the northern tip of the Ungava peninsula because of the aggregation of infrastructures in this confined area. Exposure to disturbances, and their effects on the mortality risk of caribou could thus be correlated with latitude. We could not directly test the effect of latitude on early life and seasonal survival because latitudes used by individuals varied markedly during these periods. To ensure that effects could be attributed to the tested variable and not the confounding effect of latitude, we explored the potential correlation between the variables and latitude. When the correlation with latitude was >0.50, we tested the effect of the variable on mortality risk despite potential confounding effects of latitude, but we interpreted the results accordingly. At the daily scale, we could better test the effect of latitude on mortality risk by including a latitude model in the candidate set to assess whether other variables explained more variability in mortality risk than latitude alone. Model performance at this scale of analysis could not be assessed with a likelihood ratio test based on a null model (see above). Instead, we used latitude as the null model for comparison.
Results
Human Disturbance Effects
Early Life Mortality Risk
For the early life period (1−7 years old), all models except the one including all disturbance types for the RGH satisfied the assumption of proportional hazards. In all cases, models indicated no effect of human disturbances on the mortality risk of caribou over the early life period, for both the RFH and RGH (Figure 3A and Table 3).
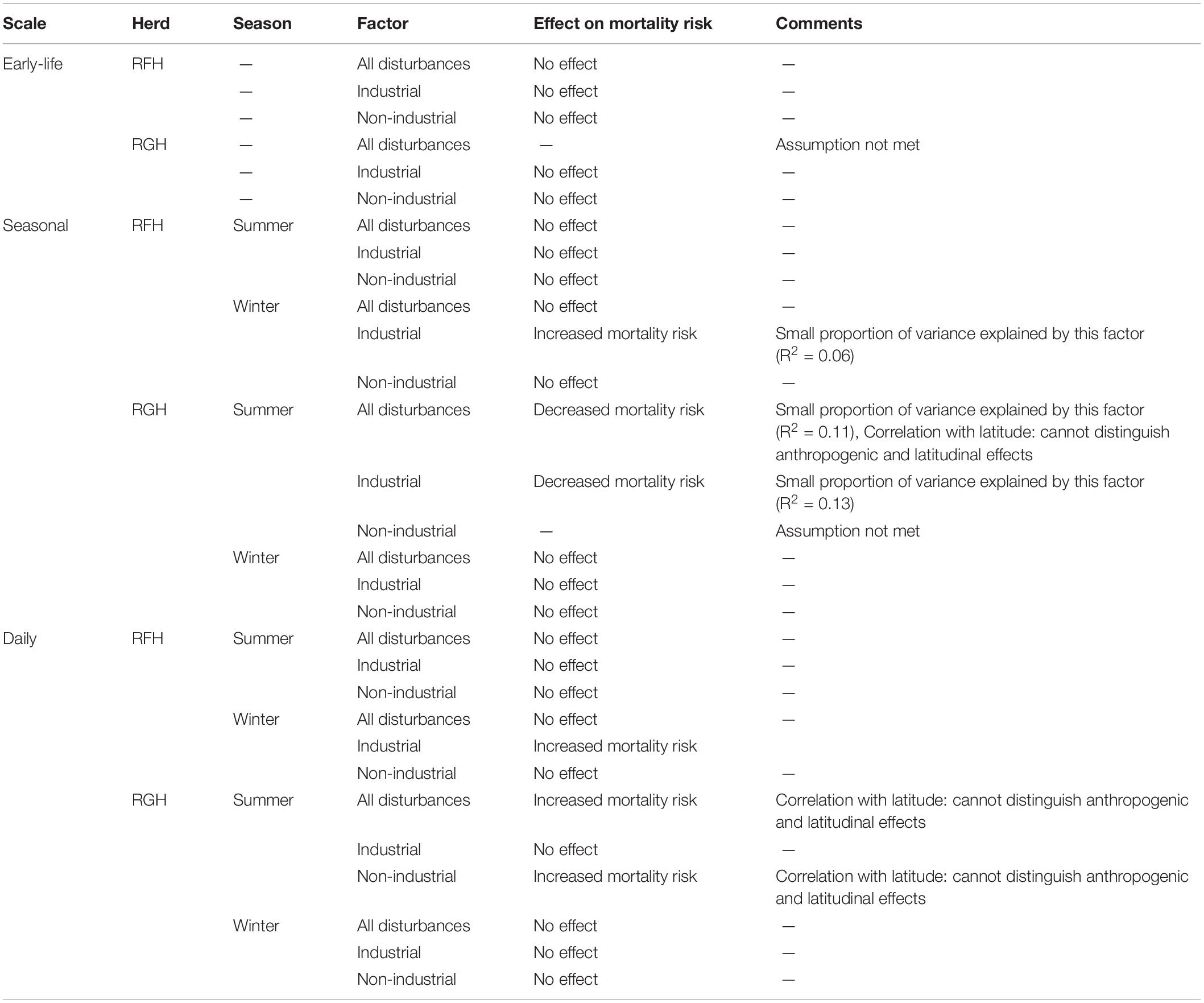
Table 3. Summary of the effects of human disturbances (based on Cox-proportional hazard analyses) on early-life, seasonal and daily mortality risk of migratory caribou of the Rivière-aux-Feuilles (RFH) and Rivière-George herds (RGH), northern Québec and Labrador, Canada, 2009−2016.
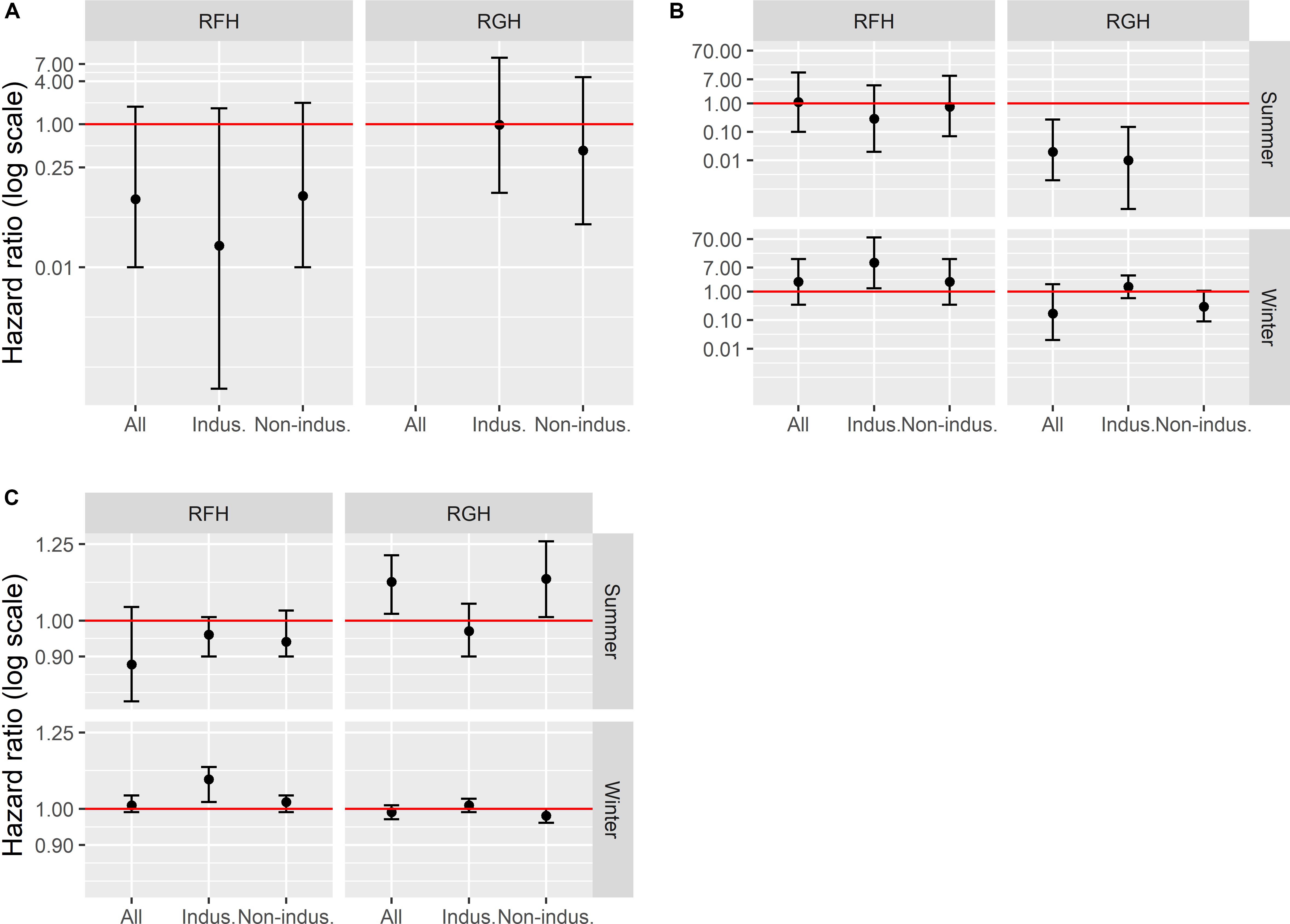
Figure 3. Human disturbance effects [hazard ratio (HR), represented on a log scale] on caribou mortality risk at three temporal scales, early-life 1–7 years old; (A), seasonal (B) and daily (C) mortality risk, and 95% confidence intervals, on the Rivière-aux-Feuilles (RFH) and Rivière-George herd (RGH), northern Québec, Canada, 2009–2016. A hazard ratio < 1 indicates that exposure to disturbances decreased mortality risk, whereas a hazard ratio >1 indicates that exposure to disturbances increased mortality risk (red line = hazard ratio of 1). Disturbances tested included all disturbance types (mines, mining exploration, roads, and human settlements), industrial disturbances only (indus.; mine and mining exploration) and non-industrial disturbances only (non-indus.; roads and human settlements).
Seasonal Mortality Risk
At the seasonal scale, we documented significant effects of human disturbance on caribou mortality risk, which differed across herds and seasons. For the RFH, repeated exposure to human disturbances had no effect on the mortality risk of caribou during summer (Figure 3B and Table 3). During winter, we observed that a greater exposure to industrial disturbances increased the risk of mortality. The risk of dying was 10 times higher for individuals exposed to industrial disturbances throughout winter than for individuals never exposed (Hazard Ratio, HR = 10.3, 95% CI = 1.3−79.8). Caribou exposure to industrial disturbances explained a small proportion of the variation observed in caribou mortality risk (R2 = 0.06) and the uncertainty around the estimate was large. During winter, caribou exposure to disturbances was correlated with latitude (r = 0.58), with caribou more exposed to disturbances at lower latitudes.
For the RGH, the model testing the effect of non-industrial disturbances on summer mortality risk was discarded because it did not converge and the condition of proportional hazard was not met. The other models for the RGH indicated that caribou more exposed to human disturbances in general, or to industrial disturbances in particular, faced a lower risk of mortality during summer (Figure 3B). Caribou exposed to disturbance throughout summer were 1.4 to 1.7 time more likely to die for each 10% decrease in exposure for all disturbances or industrial disturbances only, respectively (all disturbances: HR for a 10% increment in exposure = 0.69, 95% CI = 0.54−0.88; industrial disturbances: HR for a 10% increment in exposure = 0.60, 95% CI = 0.44−0.82). Exposure to these disturbances explained a limited proportion of the variation in caribou mortality risk (R2all = 0.11, R2industrial = 0.13). The correlation between the distance of caribou to human disturbances and latitude was significant (r = 0.52), but weak for industrial disturbances only (r = 0.19). During winter, our results indicated that the human disturbance factors we assessed did not affect caribou mortality risk in this herd.
Daily Mortality Risk
On the RFH, daily mortality risk of caribou during summer was not affected by human disturbance (Figure 3C and Table 3). During winter, we noted that caribou closer to industrial disturbances faced an increased risk of mortality. The mortality risk of caribou was 11 times higher for each 10-km increment toward industrial disturbances (HR for a 10-km increment = 10.7, 95% CI = 10.2−11.3).
On the RGH, we determined that proximity to disturbances in general, and of non-industrial disturbances in particular, increased the daily mortality risk during summer (Figure 3C). Mortality risk was, on average, 11 times higher for each 10-km increment toward disturbances (HR for a 10-km increment: all disturbances = 11.2; 95% CI = 10.2−12.1; non-industrial = 11.3; 95% CI = 10.1−12.6). The correlation between proximity to disturbances and latitude was significant (Rall disturbances = 0.52, Rnon–industrial = 0.62), indicating that caribou were more exposed to disturbance in the southern portion of their summer range. Yet, caribou died on average 200 km south of where caribou that survived were during the same day. Thus, we cannot distinguish effects of latitude and human disturbances on daily survival during summer for this herd and season. During winter, we observed no effect of human disturbances on daily survival for the RGH (Figure 3C).
Relative Importance of Human Disturbances and Natural Factors
Early Life Mortality Risk
Human disturbance was not the most influential factor on early life mortality risk of caribou of the RFH and RGH (Table 1 of Supplementary Appendix 3 and Table 4). On the RFH, the predation risk and precipitation models received equivalent support. Caribou using areas which received more precipitations faced a higher risk of mortality during the early life period. The precipitation model predicted that the mortality risk of caribou was 68 times higher for each increase of 100 g/m2 in precipitation (R2 = 0.46). Surprisingly, caribou using riskier areas in terms of predation by wolves more frequently had a better chance of surviving throughout the first 7 years-of-life (R2 = 0.44). The predation model predicted that mortality risk of caribou was 1.76 time higher for each 10% decrease in predation risk exposure (HR for 10% increment in predation risk exposure = 0.57; 95% CI = 0.40−0.80). We note that for this herd, predation risk and habitat highly selected by caribou were strongly correlated during winter (r = 0.82), but not during summer (r = 0.13). For the RGH, we also found support for the predation risk hypothesis, but contrary to the RFH, caribou frequently using riskier areas in terms of predation risk faced an increased risk of mortality during the early-life period (HR for 10% increment in predation risk exposure = 1.48, 95% CI = 1.12−1.96; R2 = 0.25).
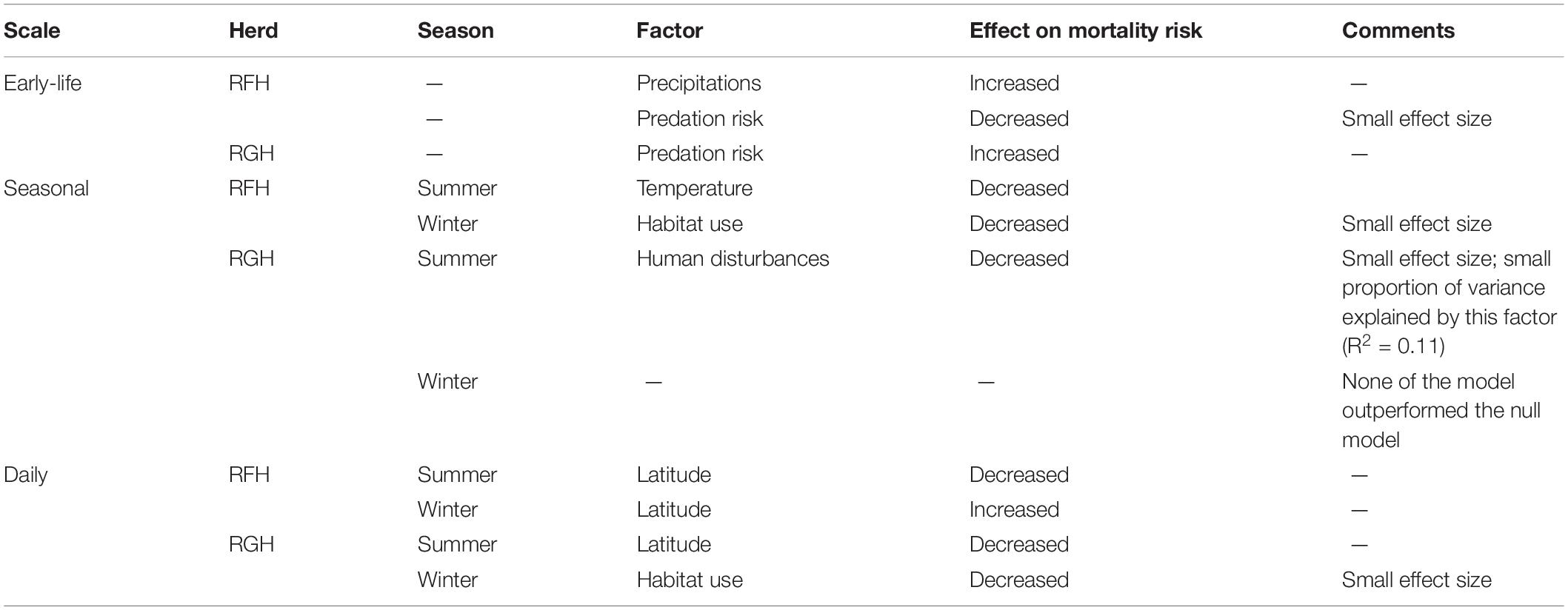
Table 4. Summary of the effects of the most important factors affecting early-life, seasonal and daily mortality risk of migratory caribou of the Rivière-aux-Feuilles (RFH) and Rivière-George herds (RGH), northern Québec and Labrador, Canada, 2009−2016.
Seasonal Mortality Risk
At the seasonal scale, the effect of human disturbances on the mortality risk were limited compared with those of non-anthropogenic factors. For the RFH, model selection for summer mortality risk indicated support for the temperature model (Table 2 of Supplementary Appendix 3 and Table 4). The risk of mortality was higher for caribou using areas relatively warmer than those used by other caribou during the same day. The risk of mortality was 6.5 times higher for each increase of 1°C in the difference of temperature between the area used by a caribou and the population mean (HR = 6.49, 95% CI = 3.0−14.4; R2 = 0.25).
During winter, model selection for the RFH supported the habitat use hypothesis. Individuals spending more time in highly selected habitats faced a lower risk of mortality during winter. Caribou were 2 times more likely to die for each 10% decrease in the use of highly selected habitat (HR for 10% increment in use = 0.47, 95% CI = 0.27−0.84; R2 = 0.17).
On the RGH, summer survival was mainly explained by exposure to disturbances (Supplementary Appendix 3 and Table 4). The best model indicated that caribou more exposed to disturbances had a lower chance of dying during summer, as mentioned previously (Figure 3). None of the models including only non-anthropic factors outperformed the null model (H0 with ΔAIC ≤ 2). During winter, none of our hypotheses outperformed the null model (H0 with ΔAIC ≤ 2).
Daily Mortality Risk
At the daily scale, human disturbances had negligible effects compared to non-anthropogenic factors. On the RFH, the latitude model outperformed all other models for both summer and winter seasons (H0 with ΔAIC ≤ 2; Table 2 of Supplementary Appendix 3 and Table 4). During summer, caribou mainly died at lower latitudes (HR for increment of 1 km = 0.98, 95% C I = 0.97−0.99), whereas during winter, caribou mainly died at higher latitudes (HR for increment of 1 km = 1.01, 95% CI = 1.00−1.02).
On the RGH, model selection for summer indicated strong support for the latitude model. This model predicted that individuals died on average 200 km south of where individuals that survived were on the same day (HR for increment of 1 km = 0.98, 95% CI = 0.97−0.99). During winter, we found support for the habitat use model, where individuals using highly selected habitat at the population level experienced lower mortality risk. The mortality risk was 1.7 times higher for each 10% decrease in the use of highly selected habitat (HR for 10% increment in use = 0.57, 95% CI = 0.37, 0.84).
Discussion
Caribou and reindeer populations are declining across their circumarctic distribution, and long-term widespread changes in the landscape resulting from climate change and human development are likely contributing to these declines (Vors and Boyce, 2009; Festa-Bianchet et al., 2011; Environment Canada, 2012). Although industrial development is relatively recent in migratory caribou ranges of northern Québec and Labrador, we previously reported strong behavioral responses toward human disturbances, which translated into significant functional habitat loss (Plante et al., 2018). Yet, the consequences of these modifications in behavior and habitat use on animal performance and population trends had yet to be investigated.
We demonstrated that human disturbances can impact caribou survival, but the direction and magnitude of those effects varied greatly across the herds and the three considered temporal scales. Our results indicate both positive and negative impacts of human disturbances on caribou survival. Yet, the interpretation of these effects requires caution because they either explained little variation in mortality risk or their effect were indistinguishable from that of latitude. In addition, we suggest that, in most instances, disturbances were not the dominant or unique factor explaining survival of caribou from the RFH and RGH. Indeed, effects of natural factors on caribou survival prevailed over those of anthropogenic ones. None of the six hypotheses presented in our conceptual framework received consistent support across temporal scales.
Early Life Mortality Risk
Our results first indicate that human disturbances did not have detectable cumulative impacts on caribou survival in the long-term (early life period; 1−7 years old) at the current level of development in the RFH and RGH ranges. This result does not support the conclusions of a growing number of empirical results revealing the existence of long-term cumulative impacts of human disturbances on Rangifer vital rates (Sorensen et al., 2008; Environment Canada, 2011; Johnson et al., 2015). We believe that this discrepancy is attributable to the relatively low level of human development on the RFH and RGH ranges compared with those evaluated in other studies on boreal caribou. For the boreal caribou ecotype, reductions in population size have been tightly linked to the cumulative habitat loss caused by habitat alteration through forestry operations and avoidance of infrastructures (Sorensen et al., 2008; Environment Canada, 2011; Johnson et al., 2015). Moreover, disturbance densities have reached critical levels in annual ranges of many boreal caribou populations (Environment Canada, 2011), making the avoidance of disturbances almost impossible because of the lack of suitable alternative habitat (Gill et al., 2001). This habitat alteration is also causing an increase in predation risk for caribou through numerical responses of alternative prey and predators, and increased access and efficiency of predators in modified landscapes (Environment Canada, 2011). This outcome is unlikely for migratory caribou of the RFH and RGH because the mechanisms through which human disturbances may impact these caribou are likely different from other herds. Undisturbed habitat is still readily available for migratory caribou of the RFH and RGH (Figure 2), and individuals may avoid disturbances over large distances (Plante et al., 2018).
Early life survival of migratory caribou was mainly affected by patterns of habitat use in relation to predation risk (RFH and RGH) and climatic conditions (precipitation; RFH). We documented contrasting effects of the use of habitats with high predation risk for the two herds. In the RFH, using risky areas more often appeared to increase survival. For this herd, caribou and wolves mainly occurred in forested habitats during winter (selected by both species, Supplementary Appendix 1). The winter season covers 31−45% of the year, and patterns observed during this season could have a great influence on the results at the early life scale. Because caribou and wolves used the same habitats during a large portion of the year, we suspect that the positive effect of using risky habitats on caribou survival may be partly related to the positive outcomes of the tactic of habitat use by caribou. In the RGH, increased exposure to risky habitats decreased caribou survival. It is known that, in addition to harvesting, predation is a significant cause of mortality for caribou in northern Québec and Labrador, wolves being responsible for at least 10−33% of mortalities (see also Table 2). This discrepancy in the effect of predation risk between the two herds may arise from our predation risk index not considering the population size of predators, variable spatio-temporal access of predators to caribou, rates of encounters, and success of predation events. It is also possible that limiting factors during the study period could have differed between the two herds, with a stronger effect of predation risk for the RGH compared to the RFH. In addition, we could not test the hypothesis that wolves avoided or selected proximity to infrastructures because of the low sample size of marked wolves and their distribution on caribou ranges.
Our long-term assessment of anthropogenic and natural factors may not have adequately portray the conditions encountered by caribou throughout the early life period, or that survival was mainly impacted by the accumulation of immediate factors acting at shorter temporal scales than the one we investigated. For example, caribou may be highly sensitive to a specific factor during a given season, but could compensate for its negative effects in the following season (Darling and Côté, 2008), which would limit our ability to detect its influence over the first 7 years of their adult life. Many of the factors we tested may have also acted simultaneously in the population, but affected early life survival of each individual differently. In addition, we could not consider intrinsic factors, such as body condition, that are often identified as major determinants of survival in long-lived mammals (Gaillard et al., 2003).
Seasonal Mortality Risk
At the seasonal scale, repeated exposure to industrial disturbances reduced survival of the RFH during winter. The RFH winter range bears the highest density of disturbances across our study areas. This seasonal effect on survival may be a consequence of cumulative costs of short-term behavioral responses toward disturbances, such as avoidance (Plante et al., 2018), increased stress level (Wasser et al., 2011), movement rate (Dussault et al., 2007), or vigilance (Benhaiem et al., 2008). To test this hypothesis, future work should concentrate on quantifying physiological responses to human disturbances at different temporal scales, to determine whether chronic stress responses can influence survival (Wasser et al., 2011). The seasonal effect we observed on survival also could be the result of cumulative risk exposure to direct mortality sources such as hunting. Whether caribou faced chronic stress or increased risk of harvest near infrastructures, the consequence was the same for the RFH, and resulted from the accumulation of exposure to human disturbance over time and space. We note that exposure of caribou from the RFH to industrial disturbances was correlated with latitude, with individuals more exposed in the southern portion of their winter range. Hence, we cannot confirm that the negative effects on caribou survival is entirely attributable to industrial disturbances. In addition, industrial disturbances explained only a small portion of the variability in winter mortality risk (6%), indicating that other factors are driving caribou survival during this period.
Our model revealed that repeated exposure to human disturbance was beneficial for survival of the RGH caribou during summer. These effects are likely the consequences of the correlation between caribou exposure to human disturbance and latitude. Indeed, human disturbances were not randomly distributed in caribou seasonal ranges, especially in summer range. This, combined with the highly mobile nature of caribou during summer, created strong temporal and latitudinal variations in exposure to human disturbances. This result implies that summer mortality risk in the RGH was probably not influenced by human disturbances, but rather by other factors correlated with latitude for example, vegetation composition, vegetation productivity, or abundance of predators.
Overall, seasonal survival of migratory caribou was mainly driven by the use of highly selected habitats (RFH) and climatic conditions (RFH; temperature). Caribou of the RFH using habitats similarly to the rest of the population, i.e., spending more time in strongly selected habitat at the population level, faced a lower risk of mortality during winter. Conversely, caribou exhibiting marginal behaviors compared with the population had a higher risk of mortality. Marginal individuals can face a higher risk of mortality because they are not as good at avoiding or defending themselves against predators when isolated from most of the population. These caribou also might have bolder personalities, which may place them in riskier situations (Lesmerises et al., 2019). Alternatively, habitats strongly selected by the population are presumably high-quality habitats that may contribute to maintain or improve body condition, and ultimately survival. Previous research indicates a strong influence of habitat productivity on the body condition of calves and females in the RFH and RGH (Couturier et al., 2009a, b), which could in turn affect survival. Density-dependent effects of food availability on nutrition, body condition, and survival, may have decreased in the last decades, especially for the RGH, which strongly declined during our study period. This may explain why we did not identify habitat quality as an influent factor for the survival of caribou of the RGH.
We documented that using sites with warmer temperatures during summer increased the seasonal mortality risk of caribou of the RFH herd. Although caribou are believed to be tolerant to warm temperatures (Hagemoen and Reimers, 2002), they could suffer from high thermoregulatory costs and heat stress during warm summer days (Soppela et al., 1986). More importantly, warm temperatures in arctic summers are associated with increased insect activity and harassment (Weladji et al., 2003). In periods of intense insect harassment, caribou reduce their food intake, shorten their resting periods and increase their movements (Toupin et al., 1996; Mörschel and Klein, 1997; Hagemoen and Reimers, 2002). These alterations of activity entail energetic costs that could compromise body condition and eventually survival (Helle and Tarvainen, 1984; Weladji et al., 2003), especially if caribou do not subsequently compensate for lost feeding opportunities (Colman et al., 2003).
Daily Mortality Risk
At a shorter time scale, we observed contrasting results regarding effects of human disturbances on daily survival, but those effects were undistinguishable from latitudinal effects. On the RFH, the models indicated that caribou benefited from being closer to human disturbances during summer, but again, this relationship likely originated from differential range-use patterns by individuals, not from disturbance. Caribou that died during summer generally remained further south, whereas exposure to human disturbances mainly occurred in the north. Perhaps individuals in poorer body condition were unable to reach the northern part of their summer range and died at southern locations. In the RGH range, however, proximity to villages and roads reduced daily survival during summer, but again, latitudinal effects prevailed over anthropogenic ones. These results underline the complexity of testing the effect of punctual or permanent infrastructures on highly mobile species such as migratory caribou.
Behavioral vs. Fitness Consequences of Human Disturbances
Our study underpins the importance of not relying exclusively on behavioral responses to draw conclusions on the impacts of human disturbance on wildlife populations. Although strong behavioral responses toward disturbances were reported for the RFH and RGH (Plante et al., 2018), effects on survival, although detectable, were limited. Behavioral responses toward disturbances could be the mechanism through which caribou could minimize the negative impacts of disturbance on their condition and survival. Our study thus exemplifies a non-linear relationship between behavioral responses and consequences on fitness (Gill et al., 2001). We also highlight the importance of considering cumulative effects of human development over an array of spatiotemporal scales. We acknowledge, however, that cumulative effects on migratory caribou survival appear to be limited by the currently low levels of human disturbances. Nonetheless, reporting an effect over a season is significant considering the potential antagonist or compensatory processes occurring over time, which could have weakened the anthropogenic effects we observed.
Management Implications
Empirical evidences of the negative impacts of habitat loss and fragmentation resulting from human development are abundant for boreal caribou populations, indicating that cumulative effects are likely the ultimate factor responsible for their decline in Canada (Sorensen et al., 2008; Environment Canada, 2011). In the RFH and RGH ranges, human development is relatively recent and the human footprint is still limited by remoteness and harsh conditions. Yet, the accelerated and projected development in recent years (MRNF, 2012) raises multiple questions regarding the conservation and management of these declining herds. Our results document that effects of human disturbance (exclusive of hunting) on survival are not predominant and widespread at the current level of development in northern Québec and Labrador. Nevertheless, we observed meaningful impacts even considering the low level of development compared to that experienced by most boreal caribou populations. Predicting the level at which development and human disturbance would induce more significant impacts on caribou survival is difficult, but our results imply that the impact would increase with the density of disturbances within caribou ranges. Yet, this context offers a great opportunity to anticipate the consequences of various development scenarios instead of juggling with the consequences retrospectively.
More importantly, effects of human disturbances on population dynamics need to be further investigated for these herds to clarify their role in the current population declines. Although human development per se was not the primary driver of survival in the RFH and RGH, development may contribute as one of many factors generating population declines. Management and conservation guidelines established for boreal caribou may not apply to migratory ecotypes for many reasons, including the marked difference in range use and movements. Wide-ranging caribou could be particularly vulnerable to human development, because of the large area they need to fulfill vital activities such as foraging and predator avoidance. Further development could increase the risk of disrupting migration behavior, with unknown consequences on population persistence. Yet, the current plans of development in the north still relies on the impact assessment of individual projects. Migratory caribou are also facing the increasing threat of climate change. Warmer climate and more frequent extreme weather events are and will likely negatively affect migratory caribou populations through multiple complex processes. Yet, the impacts of climate change on wildlife populations are arduously manageable. Restricting human development could improve caribou survival in certain areas and periods, and this could constitute the only possible action to help compensate for the negative impacts expected from unmanageable threats such as climate change.
Data Availability Statement
The data analyzed in this study is subject to the following licenses/restrictions: Migratory caribou data in Quebec are considered sensitive, and are protected by a sharing agreement with the Ministry of Forests, Wildlife and Parks (Ministère des Forêts, de la Faune et des Parcs). Requests to access these datasets should be directed to Joëlle Taillon, Ph.D., researcher, Ministère des Forêts, de la Faune et des Parcs, Québec, Québec, G1S 4X4,am9lbGxlLnRhaWxsb25AbWZmcC5nb3V2LnFjLmNh.
Ethics Statement
The animal study was reviewed and approved by the Animal Care Committee of Laval University.
Author Contributions
SP designed the study with the help of CD, JR, and SC. SP realized the analyses and wrote the first draft of the manuscript. CD, JR, MG, and SC helped with the analyses and commented the manuscript before submission.
Funding
Caribou Ungava was supported by the Natural Sciences and Engineering Research Council of Canada (NSERC RDCPJ 46512-14). SP was supported by the Fonds de Recherche Québécois pour la Nature et les Technologies (FRQNT 183343) and Caribou Ungava.
Conflict of Interest
The authors declare that the research was conducted in the absence of any commercial or financial relationships that could be construed as a potential conflict of interest.
Acknowledgments
We are grateful to Caribou Ungava partners (in alphabetical order): Air Inuit, ArcticNet, Azimut Exploration, Centre d’Études Nordiques, Grand Council of Crees, Exploration Osisko, Canada Foundation for Innovation, GlenCore-Mine Raglan, Hydro-Québec, Makivik Corporation, Government of Newfoundland-Labrador – Wildlife Division, Government of Québec – Ministère des Forêts de la Faune et des Parcs, TataSteel Minerals Canada Limited, and the Torngat Wildlife Plants and Fisheries Secretariat. We are especially grateful to V. Brodeur, D. Grenier, C. Jutras, J. Pisapio, S. Rivard, J. Taillon, and N. Trudel for their contribution to fieldwork and data collection. We also thank S. Biondo, F. Huot, and M. Le Corre for sharing data, and S. Hamel for statistical advices.
Supplementary Material
The Supplementary Material for this article can be found online at: https://www.frontiersin.org/articles/10.3389/fevo.2020.00154/full#supplementary-material
References
Arnold, T. W. (2010). Uninformative parameters and model selection using Akaike’s information criterion. J. Wildl. Manage. 74, 1175–1178. doi: 10.1371/journal.pone.0206711
Battin, J. (2004). When good animals love bad habitats: ecological traps and the conservation of animal populations. Conserv. Biol. 18, 1482–1491. doi: 10.1111/j.1523-1739.2004.00417.x
Benhaiem, S., Delon, M., Lourtet, B., Cargnelutti, B., Aulagnier, S., Hewison, A. J. M., et al. (2008). Hunting increases vigilance levels in roe deer and modifies feeding site selection. Anim. Behav. 76, 611–618. doi: 10.1016/j.anbehav.2008.03.012
Berger, J., Hartway, C., Gruzdev, A., and Johnson, M. (2018). Climate degradation and extreme icing events constrain life in cold-adapted mammals. Sci. Rep. 8:1156. doi: 10.1038/s41598-018-19416-9
Bergerud, A. T., Luttich, S. N., and Camps, L. (2008). The Return of Caribou to Ungava. Montreal: McGill-Queen’s University Press.
Berteaux, D., Casajus, N., and Ropars, P. (2018). Portrait du Climat du Nord du Québec et du Labrador Pour la Période 1981-2010. Rimouski, QC: Université du Québec à Rimouski.
Boulanger, J., Poole, K. G., Gunn, A., and Wierzchowski, J. (2012). Estimating the zone of influence of industrial developments on wildlife: a migratory caribou Rangifer tarandus groenlandicus and diamond mine case study. Wildl. Biol. 18, 164–179. doi: 10.2981/11-045
Boyce, M. S., Vernier, P. R., Nielsen, S. E., and Schmiegelow, F. K. A. (2002). Evaluating resource selection functions. Ecol. Model. 157, 281–300. doi: 10.1016/s0304-3800(02)00200-4
Bradshaw, C. J. A., Boutin, S., and Hebert, D. M. (1998). Energetic implications of disturbance caused by petroleum exploration to woodland caribou. Can. J. Zool. 76, 1319–1324. doi: 10.1139/z98-076
Brooks, T. M., Mittermeier, R. A., Mittermeier, C. G., Fonseca, G. A. B. D., Rylands, A. B., Konstant, W. R., et al. (2002). Habitat loss and extinction in the hotspots of biodiversity. Conserv. Biol. 16, 909–923. doi: 10.1046/j.1523-1739.2002.00530.x
Burnham, K. P., and Anderson, D. R. (2002). Model Selection and Multimodel Inference: A Practical Information-Theoretic Approach, 2nd Edn. New York, NY: Springer.
Cameron, R. D., Smith, W. T., White, R. G., and Griffith, B. (2005). Central Arctic caribou and petroleum development: distributional, nutritional, and reproductive implications. Arctic 58, 1–9.
Cardillo, M., Mace, G., Jones, K., Bielby, J., Bininda-Emonds, O., Sechrest, W., et al. (2005). Multiple causes of high extinction risk in large mammal species. Science 309, 1239–1241. doi: 10.1126/science.1116030
Colman, J. E., Pedersen, C., Hjermann, D. Ø., Holand, Ø., Moe, S. R., and Reimers, E. (2003). Do wild reindeer exhibit grazing compensation during insect harassment? J. Wildl. Manage. 67, 11–19.
Côté, S., Festa-Bianchet, M., Dussault, C., Tremblay, J.-P., Brodeur, V., Simard, M., et al. (2010). “Caribou herd dynamics: Impacts of climate change on traditional and sport harvesting,” in Nunavik and Nunatsiavit: From Science to Policy – An Integrated Regional Impact Study (IRIS) of Climate Change and Modernization, eds M. Allard and M. Lemay (Québec: ArcticNet), 249–269.
Courbin, N., Fortin, D., Dussault, C., and Courtois, R. (2009). Landscape management for woodland caribou: the protection of forest blocks influences wolf-caribou co-occurrence. Landsc. Ecol. 24, 1375–1388. doi: 10.1007/s10980-009-9389-x
Couturier, S., Côté, S. D., Huot, J., and Otto, R. (2009a). Body-condition dynamics in a northern ungulate gaining fat in winter. Can. J. Zool. 87, 367–378. doi: 10.1139/z09-020
Couturier, S., Côté, S. D., Otto, R. D., Weladji, R. B., and Huot, J. (2009b). Variation in calf body mass in migratory caribou: the role of habitat, climate and movements. J. Mammal. 90, 442–452. doi: 10.1644/07-mamm-a-279.1
Couturier, S., Otto, J. R., and Rivard, S. (2004). Demography of the Migratory Tundra Caribou (Rangifer tarandus) of the Nord-du-Québec Region and Labrador. Québec: Ministère des Ressources naturelles.
Creel, S., and Christianson, D. (2008). Relationships between direct predation and risk effects. Trends Ecol. Evol. 23, 194–201. doi: 10.1016/j.tree.2007.12.004
Darling, E. S., and Côté, I. M. (2008). Quantifying the evidence for ecological synergies. Ecol. Lett. 11, 1278–1286. doi: 10.1111/j.1461-0248.2008.01243.x
Davies, R. G., Orme, C. D. L., Olson, V., Thomas, G. H., Ross, S. G., Ding, T.-S., et al. (2006). Human impacts and the global distribution of extinction risk. Proc. Biol. Sci. 273, 2127–2133.
DeCesare, N. J., Hebblewhite, M., Bradley, M., Hervieux, D., Neufeld, L., and Musiani, M. (2014). Linking habitat selection and predation risk to spatial variation in survival. J. Anim. Ecol. 83, 343–352. doi: 10.1111/1365-2656.12144
DeMars, C. A., and Boutin, S. (2018). Nowhere to hide: effects of linear features on predator–prey dynamics in a large mammal system. J. Anim. Ecol. 87, 274–284. doi: 10.1111/1365-2656.12760
Didan, K. (2015). Data From MOD13Q1 MODIS/Terra Vegetation Indices 16-Day L3 Global 250m SIN Grid V006. NASA EOSDIS Land Processes DAAC. Available online at: https://doi.org/10.5067/MODIS/MOD13Q1.006 (accessed September 28, 2017).
Dussault, C., Ouellet, J.-P., Laurian, C., Courtois, R., Poulin, M., and Breton, L. (2007). Moose movement rates along highways and crossing probability models. J. Wildl. Manage. 71, 2338–2345.
Dussault, C., Pinard, V., Ouellet, J.-P., Courtois, R., and Fortin, D. (2012). Avoidance of roads and selection for recent cutovers by threatened caribou: fitness-rewarding or maladaptive behaviour? Proc. Natl. Acad. Sci. Biol. U.S.A. 279, 4481–4488. doi: 10.1098/rspb.2012.1700
Environment Canada (2011). Scientific Assessment to Inform the Identification of Critical Habitat for Woodland Caribou (Rangifer tarandus caribou), Boreal Population, in Canada: 2011 Update. Ottawa: Environment Canada.
Environment Canada (2012). Recovery Stategy for the Woodland Caribou (Rangifer tarandus caribou), Boreal Population, in Canada. Species at Risk Act Recovery Strategy Series. Ottawa: Environment Canada.
Fahrig, L., and Rytwinski, T. (2009). Effects of roads on animal abundance: an empirical review and synthesis. Ecol. Soc. 14:20.
Festa-Bianchet, M., Ray, J. C., Boutin, S., Côté, S., and Gunn, A. (2011). Conservation of caribou (Rangifer tarandus) in Canada: an uncertain future. Can. J. Zool. 89, 419–434. doi: 10.1371/journal.pone.0136933
Forbes, B. C., Kumpula, T., Meschtyb, N., Laptander, R., Macias-Fauria, M., Zetterberg, P., et al. (2016). Sea ice, rain-on-snow and tundra reindeer nomadism in arctic Russia. Biol. Lett. 12:20160466. doi: 10.1098/rsbl.2016.0466
Forman, R. T. T., and Alexander, L. E. (1998). “Roads and their major ecological effects,” in Annual Review of Ecology and Systematics, ed. D. G. Fautin (Palo Alto, CA: Annual Review Inc), 207–232.
Frid, A., and Dill, L. (2002). Human-caused disturbance stimuli as a form of predation risk. Conserv. Ecol. 6:11.
Gaillard, J. M., Festa-Bianchet, M., Yoccoz, N. G., Loison, A., and Toigo, C. (2000). Temporal variation in fitness components and population dynamics of large herbivores. Annu. Rev. Ecol. Syst. 31, 367–393. doi: 10.1146/annurev.ecolsys.31.1.367
Gaillard, J.-M., Hebblewhite, M., Loison, A., Fuller, M., Powell, R., Basille, M., et al. (2010). Habitat–performance relationships: finding the right metric at a given spatial scale. Philos. Trans. R. Soc. B. 365, 2255–2265. doi: 10.1098/rstb.2010.0085
Gaillard, J.-M., Loison, A., Festa-Bianchet, M., Yoccoz, N., and Solberg, E. J. (2003). “Ecological correlates of life span in populations of large herbivorous mammals,” in Evolutionary, Ecological, and Demographic Perspectives, eds J. R. Carey and S. Tuljapurkar (New York, NY: Population Council), 39–56.
Gill, J. A., Norris, K., and Sutherland, W. J. (2001). Why behavioural responses may not reflect the population consequences of human disturbance. Biol. Conserv. 97, 265–268. doi: 10.1016/s0006-3207(00)00002-1
Gillies, C. S., Hebblewhite, M., Nielsen, S. E., Krawchuk, M. A., Aldridge, C. L., Frair, J. L., et al. (2006). Application of random effects to the study of resource selection by animals. J. Anim. Ecol. 75, 887–898. doi: 10.1111/j.1365-2656.2006.01106.x
Hagemoen, R. I. M., and Reimers, E. (2002). Reindeer summer activity pattern in relation to weather and insect harassment. J. Anim. Ecol. 71, 883–892. doi: 10.1046/j.1365-2656.2002.00654.x
Helle, T., and Tarvainen, L. (1984). Effects of insect harassment on weight gain and survival in reindeer calves. Rangifer 4, 24–27.
James, A. R. C., and Stuart-Smith, A. K. (2000). Distribution of caribou and wolves in relation to linear corridors. J. Wildl. Manage. 64, 154–159.
Johnson, C. J., Ehlers, L. P. W., and Seip, D. R. (2015). Witnessing extinction: cumulative impacts across landscapes and the future loss of an evolutionarily significant unit of woodland caribou in Canada. Biol. Conserv. 186, 176–186. doi: 10.1016/j.biocon.2015.03.012
Johnson, C. J., and Russell, D. E. (2014). Long-term distribution responses of a migratory caribou herd to human disturbance. Biol. Conserv. 177, 52–63. doi: 10.1016/j.biocon.2014.06.007
Johnson, C. J., and St-Laurent, M.-H. (2011). “Unifying framework for understanding impacts of human developments on wildlife,” in Energy Development and Wildlife Conservation in Western North America, ed. D. Naugle (Washington, DC: Island Press), 27–54. doi: 10.5822/978-1-61091-022-4_3
Joly, K., Klein, D. R., Verbyla, D. L., Rupp, T., and Chapin, F. S. (2011). Linkages between large-scale climate patterns and the dynamics of Arctic caribou populations. Ecography 34, 345–352. doi: 10.1111/j.1600-0587.2010.06377.x
King, G., and Zeng, L. (2001). Logistic regression in rare events data. Polit. Anal. 9, 137–163. doi: 10.1093/oxfordjournals.pan.a004868
Klaczek, M. R., Johnson, C. J., and Cluff, H. D. (2015). Den site selection of wolves (Canis lupus) in response to declining caribou (Rangifer tarandus groenlandicus) density in the central Canadian Arctic. Polar Biol. 38, 2007–2019. doi: 10.1007/s00300-015-1759-z
Latham, A. D. M., Latham, M. C., Boyce, M. S., and Boutin, S. (2011). Movement responses by wolves to industrial linear features and their effect on woodland caribou in northeastern Alberta. Ecol. Appl. 21, 2854–2865. doi: 10.1890/11-0666.1
Latifovic, R., and Pouliot, D. (2005). Multitemporal land cover mapping for Canada: methodology and products. Can. Aeronaut. Space. J. 31, 347–363.
Latifovic, R., Pouliot, D., and Olthof, I. (2017). Circa 2010 land cover of Canada: local optimization methodology and product development. Remote Sens. 9:1098. doi: 10.3390/rs9111098
Le Corre, M., Dussault, C., and Côté, S. D. (2014). Detecting changes in the annual movements of terrestrial migratory species: using the first-passage time to document the spring migration of caribou. Mov. Ecol. 2:19. doi: 10.1186/s40462-014-0019-0
Le Corre, M., Dussault, C., and Côté, S. D. (2017). Weather conditions and variation in timing of spring and fall migrations of migratory caribou. J. Mammal. 98, 260–271.
Lebel, F., Dussault, C., Massé, A., and Côté, S. D. (2012). Influence of habitat features and hunter behavior on white-tailed deer harvest. J. Wildl. Manage. 76, 1431–1440. doi: 10.1002/jwmg.377
Leblond, M., St-Laurent, M.-H., and Côté, S. D. (2016). Caribou, water and ice - Fine-scale movements of a migratory arctic ungulate in the context of climate change. Mov. Ecol. 4:14. doi: 10.1186/s40462-016-0079-4
Lesmerises, F., Dussault, C., and St-Laurent, M.-H. (2019). Effect of behavioral marginality on survival of an alpine ungulate. Ecosphere 10:e02806.
Lodé, T. (2000). Effect of a motorway on mortality and isolation of wildlife populations. AMBIO 29, 163–166. doi: 10.1579/0044-7447-29.3.163
Mac Nally, R., Duncan, R. P., Thomson, J. R., and Yen, J. D. L. (2018). Model selection using information criteria, but is the “best” model any good? J. Appl. Ecol. 55, 1441–1444. doi: 10.1111/1365-2664.13060
Mallory, C. D., and Boyce, M. S. (2018). Observed and predicted effects of climate change on Arctic caribou and reindeer. Environ. Rev. 26, 13–25. doi: 10.1139/er-2017-0032
Manly, B. F., Thomas, D. L., McDonald, T. L., and Erickson, W. P. (2002). Resource Selection by Animals: Statistical Design and Analysis for Field Studies. Berlin: Springer.
Marchand, P., Garel, M., Bourgoin, G., Dubray, D., Maillard, D., and Loison, A. (2014). Impacts of tourism and hunting on a large herbivore’s spatio-temporal behavior in and around a French protected area. Biol. Conserv. 177, 1–11. doi: 10.1016/j.biocon.2014.05.022
McLoughlin, P. D., Boyce, M. S., Coulson, T., and Clutton-Brock, T. (2006). Lifetime reproductive success and density-dependent, multi-variable resource selection. Proc. R. Soc. B. 273, 1449–1454. doi: 10.1098/rspb.2006.3486
McLoughlin, P. D., Morris, D. W., Fortin, D., Vander Wal, E., and Contasti, A. L. (2010). Considering ecological dynamics in resource selection functions. J. Anim. Ecol. 79, 4–12. doi: 10.1111/j.1365-2656.2009.01613.x
Moreau, G., Fortin, D., Couturier, S., and Duchesne, T. (2012). Multi-level functional responses for wildlife conservation: the case of threatened caribou in managed boreal forests. J. Appl. Ecol. 49, 611–620. doi: 10.1111/j.1365-2664.2012.02134.x
Mörschel, F. M., and Klein, D. R. (1997). Effects of weather and parasitic insects on behavior and group dynamics of caribou of the Delta herd, Alaska. Can. J. Zool. 75, 1659–1670. doi: 10.1139/z97-793
NASA (2016). Land Processes Ditributed Active Archive Center (LPDAAC), USGS Earth Resources Observation and Science (EROS) Center. Sioux Falls: Vegetation Indices 16-Day L3 Global 250 m - MOD13Q1.
National Oceanic and Atmospheric Administration [NOAA] (2017). Climate Dataset: North American Regional Reanalysis (NARR). Boulder, CO: Physical Sciences Laboratory.
Nellemann, C., Vistnes, I., Jordhøy, P., Strand, O., and Newton, A. (2003). Progressive impact of piecemeal infrastructure development on wild reindeer. Biol. Conserv. 113, 307–317. doi: 10.1016/s0006-3207(03)00048-x
Newton, E. J., Patterson, B. R., Anderson, M. L., Rodgers, A. R., Vander, Vennen, L. M., and Fryxell, J. M. (2017). Compensatory selection for roads over natural linear features by wolves in northern Ontario: implications for caribou conservation. PLoS One 12:e0186525. doi: 10.1371/journal.pone.0186525
Pachkowski, M., Côté, S. D., and Festa-Bianchet, M. (2013). Spring-loaded reproduction: effects of body condition and population size on fertility in migratory caribou (Rangifer tarandus). Can. J. Zool. 91, 473–479. doi: 10.1139/cjz-2012-0334
Pettorelli, N. (2013). The Normalized Difference Vegetation Index. Oxford, UK: Oxford University Press.
Plante, S., Dussault, C., and Côté, S. D. (2017). Landscape attributes explain migratory caribou vulnerability to sport hunting. J. Wildl. Manage. 81, 238–247. doi: 10.1002/jwmg.21203
Plante, S., Dussault, C., Richard, J. H., and Côté, S. D. (2018). Human disturbance effects and cumulative habitat loss in endangered migratory caribou. Biol. Conserv. 224, 129–143. doi: 10.1016/j.biocon.2018.05.022
Poole, K. G., Gunn, A., Patterson, B. R., and Dumond, M. (2010). Sea ice and migration of the Dolphin and Union caribou herd in the Canadian Arctic: an uncertain future. Arctic 63, 414–428.
R Core Team (2018). R: A language and Environment for Statistical Computing. Vienna: R Foundation for Statistical Computing. Available online at: https://www.R-project.org/
Ramey, R. R., Thorley, J. L., and Ivey, A. S. (2018). Local and population-level responses of Greater sage-grouse to oil and gas development and climatic variation in Wyoming. PeerJ 6:e5417. doi: 10.7717/peerj.5417
Rettie, W. J., and Messier, F. (2000). Hierarchical habitat selection by woodland caribou: its relationship to limiting factors. Ecography 23, 466–478. doi: 10.1034/j.1600-0587.2000.230409.x
Robertson, B. A., and Hutto, R. L. (2006). A framework for understanding ecological traps and an evaluation of existing evidence. Ecology 87, 1075–1085. doi: 10.1890/0012-9658(2006)87[1075:affuet]2.0.co;2
Rupp, T. S., Olson, M., Adams, L. G., Dale, B. W., Joly, K., Henkelman, J., et al. (2006). Simulating the influences of various fire regimes on caribou winter habitat. Ecol. Appl. 16, 1730–1743. doi: 10.1890/1051-0761(2006)016[1730:stiovf]2.0.co;2
Schlaepfer, M. A., Runge, M. C., and Sherman, P. W. (2002). Ecological and evolutionary traps. Trends Ecol. Evol. 17, 474–480. doi: 10.1016/s0169-5347(02)02580-6
Senft, R. L., Coughenour, M. B., Bailey, D. W., Rittenhouse, L. R., Sala, O. E., and Swift, D. M. (1987). Large herbivore foraging and ecological hierarchies. Bioscience 37, 789–799. doi: 10.1111/mec.14842
Shackelford, N., Standish, R. J., Ripple, W., and Starzomski, B. M. (2018). Threats to biodiversity from cumulative human impacts in one of North America’s last wildlife frontiers. Conserv. Biol. 32, 672–684. doi: 10.1111/cobi.13036
Skatter, H. G., Charlebois, M. L., Eftestøl, S., Tsegaye, D., Colman, J. E., Kansas, J. L., et al. (2017). Living in a burned landscape: Woodland caribou (Rangifer tarandus caribou) use of postfire residual patches for calving in a high fire – low anthropogenic Boreal Shield ecozone. Can. J. Zool. 95, 975–984. doi: 10.1139/cjz-2016-0307
Soppela, P., Nieminen, M., and Timisjärvi, J. (1986). Thermoregulation in reindeer. Rangifer 1, 273–278.
Sorensen, T., McLoughlin, P. D., Hervieux, D., Dzus, E., Nolan, J., Wynes, B., et al. (2008). Determining sustainable levels of cumulative effects for boreal caribou. J. Wildl. Manage. 72, 900–905. doi: 10.2193/2007-079
Tablado, Z., and Jenni, L. (2017). Determinants of uncertainty in wildlife responses to human disturbance. Biol. Rev. 92, 216–233. doi: 10.1111/brv.12224
Taillon, J., Brodeur, V., and Rivard, S. (2016). ). Biological Status of Migratory Caribou, Leaf River Herd. Québec: Ministère des Forêts.
Toupin, B., Huot, J., and Manseau, M. (1996). Effect of insect harassment on the behaviour of the Rivière George caribou. Arctic 49, 375–382.
Uboni, A., Smith, D. W., Stahler, D. R., and Vucetich, J. A. (2017). Selecting habitat to what purpose? The advantage of exploring the habitat–fitness relationship. Ecosphere 8:e01705. doi: 10.1002/ecs2.1705
United Nation Environmental Programme [UNEP] (2001). GLOBIO-Global Methodology for Mapping Human Impacts on the Biosphere, eds C. Nellemann, L. Kullerud, I. Vistnes, B. C. Forbes, G. P. Kofinas, B. P. Kaltenborn, et al. (Nairobi: UNEP).
Venter, O., Sanderson, E. W., Magrach, A., Allan, J. R., Beher, J., Jones, K. R., et al. (2016). Sixteen years of change in the global terrestrial human footprint and implications for biodiversity conservation. Nat. Commun. 7:12558. doi: 10.1038/ncomms12558
Vistnes, I., and Nellemann, C. (2008). The matter of spatial and temporal scales: a review of reindeer and caribou response to human activity. Polar Biol. 31, 399–407. doi: 10.1007/s00300-007-0377-9
Vors, L. S., and Boyce, M. S. (2009). Global declines of caribou and reindeer. Glob. Change Biol. 15, 2626–2633. doi: 10.1111/j.1365-2486.2009.01974.x
Wasser, S. K., Keim, J. L., Taper, M. L., and Lele, S. R. (2011). The influences of wolf predation, habitat loss, and human activity on caribou and moose in the Alberta oil sands. Front. Ecol. Environ. 9, 546–551. doi: 10.2307/41479958
Weladji, R. B., and Forbes, B. C. (2002). Disturbance effects of human activities on Rangifer tarandus habitat: implications for life history and population dynamics. Polar Geogr. 26, 171–186. doi: 10.1080/789610191
Weladji, R. B., Holand, Ø., and Almøy, T. (2003). Use of climatic data to assess the effect of insect harassment on the autumn weight of reindeer (Rangifer tarandus) calves. J. Zool. 260, 79–85. doi: 10.1017/s0952836903003510
Yamasaki, S. H., Duchesneau, R., Doyon, F., Russell, J. S., and Gooding, T. (2008). Making the case for cumulative impacts assessment: modelling the potential impacts of climate change, harvesting, oil and gas, and fire. For. Chron. 84, 349–368. doi: 10.5558/tfc84349-3
Zager, P., and Beecham, J. (2006). The role of American black bears and brown bears as predators on ungulates in North America. Ursus 17, 95–108. doi: 10.2192/1537-6176(2006)17[95:troabb]2.0.co;2
Keywords: habitat use, human disturbance, migratory caribou, mortality risk, predation risk, weather conditions
Citation: Plante S, Dussault C, Richard JH, Garel M and Côté SD (2020) Untangling Effects of Human Disturbance and Natural Factors on Mortality Risk of Migratory Caribou. Front. Ecol. Evol. 8:154. doi: 10.3389/fevo.2020.00154
Received: 19 December 2019; Accepted: 05 May 2020;
Published: 24 June 2020.
Edited by:
R. Terry Bowyer, University of Alaska Fairbanks, United StatesReviewed by:
Katherine Parker, University of Northern British Columbia, CanadaRobert Weladji, Concordia University, Canada
Copyright © 2020 Plante, Dussault, Richard, Garel and Côté. This is an open-access article distributed under the terms of the Creative Commons Attribution License (CC BY). The use, distribution or reproduction in other forums is permitted, provided the original author(s) and the copyright owner(s) are credited and that the original publication in this journal is cited, in accordance with accepted academic practice. No use, distribution or reproduction is permitted which does not comply with these terms.
*Correspondence: Sabrina Plante, U2FicmluYS5wbGFudGUuNkB1bGF2YWwuY2E=